- 1Bio-Protection Research Centre, Lincoln University, Lincoln, New Zealand
- 2Weeds, Pests and Biosecurity Group, AgResearch Ltd., Lincoln, New Zealand
- 3Knowledge and Analytics Science Group, AgResearch Ltd., Lincoln, New Zealand
New Zealand pastures largely comprising Lolium ryegrass species (Poales: Poaceae) are worth $19.6B and are subject to major pest impacts. A very severe pest is the Argentine stem weevil Listronotus bonariensis (Kuschel) (Coleoptera: Curculionidae). This has been previously suppressed by the importation biological control agent, Microctonus hyperodae Loan (Hymenoptera: Braconidae). However, this suppression has recently declined and is subject to investigation. It has been hypothesised that grass type influences the parasitism avoidance behaviour by the weevil and thus parasitism rates. This study explored the hypothesis using three common pasture grasses: a diploid Lolium perenne x Lolium multiflorum hybrid ryegrass (cv. Manawa), a tetraploid Italian ryegrass L. multiflorum Lam. (cv. Tama), and a diploid perennial ryegrass L. perenne L. (cv. Samson). The described laboratory-based microcosm methodology determined the extent of weevil avoidance behaviour on each of these three grasses when subjected to the parasitoid. Such reaction was gauged by the extent of reduced weevil on-plant presence and feeding compared to the control populations. In the absence of the parasitoid, the hybrid cv. Manawa ryegrass is as highly favoured by the weevil as the tetraploid cv. Tama. On diploid cv. Samson, feeding is considerably less. In the presence of the parasitoid, weevils on the tetraploid cv. Tama plants showed little avoidance activity in response to the parasitoid and it can be argued that the benefits of staying on this plant outweighed the possibility of parasitism. Conversely and surprisingly, in the parasitoid’s presence, weevils on diploid cv. Manawa showed very strong avoidance behaviour leading to levels of exposure similar to those found on the less-preferred diploid cv. Samson. These findings reflect how weevil parasitism rates have declined in most Lolium grasses, particularly diploids, since the 1990s, but not in the tetraploid L. multiflorum. This contribution supports the hypothesis that the decline in weevil parasitism rates has been the result of rapid evolution arising from parasitoid-induced selection pressure and the countervailing effect of the nutritional quality of the host plants.
Introduction
This contribution describes an atypical tri-trophic relationship, whereby congeneric host plants variably influence a pest’s parasitoid avoidance behaviour when in the presence of its natural enemy. The interaction comprises Loilium ryegrass (Poales: Poaceae), the Argentine stem weevil Listronotus bonariensis (Kuschel) (referred to hereafter as L. bonariensis or ‘the weevil’), and its thelytokous parasitoid Microctonus hyperodae Loan (Hymenoptera: Braconidae) (referred to hereafter as M. hyperodae or ‘the parasitoid’).
Listronotus bonariensis is the most serious pest of Gramineae in New Zealand’s improved pastures, causing NZD $200 million worth of damage per annum (Ferguson et al., 2019). The species was first described in New Zealand in 1927 (Marshall, 1937) but it probably arrived far earlier (Stewart et al., 2022). Its biology has been extensively described (e.g., Barker et al., 1989; Goldson et al., 2011). The weevil overwinters in diapause with up to three generations in spring to autumn (Goldson et al., 2011). Significantly, the pasture damage is caused by the stem-mining habit of the four larval instars. The koinobiontic solitary parthenogenetic parasitoid M. hyperodae also undergoes a similar number of generations. This parasitoid is host specific (Goldson et al., 1992), is considered to have a high searching efficiency (Tomasetto et al., 2018), and probably uses olfactory cues to search for unparasitised hosts. The Gramineae most damaged by the weevil typically comprises three grass types. The least susceptible is the widely distributed and ubiquitous diploid perennial ryegrass Lolium perenne L., e.g., Samson, then diploid hybrid ryegrass (L. perenne x L. multiflorum), e.g., Manawa, and the most susceptible but relatively uncommon is tetraploid Italian ryegrass, Lolium multiflorum Lam, e.g., Tama (Goldson, 1982; Barker, 1989).
The parasitoid M. hyperodae was imported from South America in the early 1990s for biological control of L. bonariensis and was released at various sites throughout New Zealand (Goldson et al., 1990, 1993; McNeill et al., 2002). Ensuing high parasitism rates of up to 90% in the adults in the common L. perenne pastures (Barker and Addison, 2006) were subsequently shown to have had an appreciable impact on the weevil populations (Barker, 2013). However, it was against this background of significant success that mean parasitism rates declined from ca. 70 to 30% after ca. 14 generations (Tomasetto et al., 2017c) with the concurrent reappearance of the pest’s outbreaks (Popay et al., 2011). Goldson et al. (2015) suggested that this was possibly the result of selection favouring enhanced parasitoid avoidance behaviour by the weevil, and it was posited this selection was facilitated by a lack of complexity in New Zealand’s pastures (Goldson et al., 2014) combined with an unequal evolutionary arms race with the weevil being sexual and the parasitoid parthenogenetic (e.g., Goldson et al., 2014; Casanovas et al., 2018). While resistance in importation biological control is very rare (e.g., Pennisi, 2017), a similar evolutionary response was found by Pascoal et al. (2014) in Hawaii, who observed that male crickets (Teleogryllus oceanicus Le Guillou) had evolved to become non-stridulating twice via different mutation events to avoid detection by a parasitoid fly (Ormia ochracea Bigot).
Recent field and laboratory work in New Zealand has shown that weevil parasitism levels across the diploid Lolium pasture grasses have undergone a marked reduction between the 1990s and 2015 onwards independent of weevil population density (Goldson and Tomasetto, 2016; Tomasetto et al., 2017c; Goldson et al., 2020, 2022). However, the nationwide decline was not found in the less-common and short-lived tetraploid L. multiflorum pastures, perhaps because the weevils had undergone less selection pressure on this less-common grass. That parasitism levels remained unchanged from the 1990s suggests that whatever mechanism leads to reduced parasitism in diploid grasses does not operate in tetraploid L. multiflorum grasses (Goldson et al., 2015; Goldson and Tomasetto, 2016; Tomasetto et al., 2017a). Notably, hybrid cultivars showed the same extent of parasitism decline as L. perenne pastures (Goldson and Tomasetto, 2016) despite being favoured as hosts by the weevil (Goldson, 1982; Barker, 1989).
Given the increasing understanding of the observed laboratory and field patterns of parasitism decline, this contribution examines in more detail how grass cultivars affect weevil parasitoid-avoidance behaviour. It has been hypothesised that the variation in parasitism decline on the different Lolium grasses was due to either enhanced parasitoid-avoidance behaviour by reducing the weevil’s exposure or effects of plant architecture (Goldson et al., 2015). It has since been shown that plant orientation (vertical vs. horizontal placement of tillers) has little effect on parasitism rates (Goldson and Tomasetto, 2016). Shields (2019) conducted a microcosm-based investigation which suggested that parasitoid-avoidance behaviour in the form of reduced feeding and on-plant presence could have contributed to the widespread decline in parasitism rates. This contribution seeks to provide insight into how this happens through plant-mediated effects.
Materials and Methods
Grass Selection
The Lolium cultivars used in the experiments are New Zealand’s main pasture grasses and have long been used in investigating pasture interactions with L. bonariensis and M. hyperodae (e.g., Kelsey, 1958; Pottinger, 1961; Goldson, 1982; Barker, 1989; Goldson et al., 2015; Goldson and Tomasetto, 2016). These grasses were diploid hybrid ryegrass cv. Grasslands Manawa (L. perenne x L. multiflorum) (hereafter referred to as Manawa), tetraploid Italian ryegrass, Lolium multiflorum cv. Grasslands Tama (hereafter referred to as Tama), and diploid perennial ryegrass Lolium perenne cv. Grasslands Samson (hereafter referred to as Samson). For simplicity, the cultivars used were endophyte free.
Insect Sampling and Laboratory Maintenance
Adult L. bonariensis were collected on 8 January 2018 at Lincoln (43.64397oS, 172.44292oE) during the peak emergence of the first summer generation adults (late December to early February) when parasitism rates were low (Goldson et al., 1998; Phillips and Kean, 2017). This timing reduced the probability of weevil-parasitoid interactions prior to the experiment. As determined by availability, the weevils were maintained in cages at an artificially reversed 16:8 light:dark photoperiod, 60% humidity, and ca. 23°C prior to the experiment. Bouquets of Tama were replaced twice a week as food. Prior to the experiment, the weevils were starved for three days but were provided with water-soaked dental wicks.
Weevils were collected again at Lincoln in late February and early March 2018 from which parasitoids were reared. At this time high field parasitism rates occur (Goldson et al., 1998; Phillips and Kean, 2017). The samples were maintained in the conditions described above pending prepupal M. hyperodae emergence. Pupae were kept in Petri dishes with water-soaked dental wicks to maintain high humidity. Parasitoid adults were kept in Petri dishes in the same ambient conditions and provided with dental wicks soaked with a 10% honey solution (Phillips et al., 1996). All adult parthenogenetic parasitoids used in the experiments were ≤ 5 days old.
Plant-Mediated Behavioural Experiment Protocol
An arena configuration was used to measure L. bonariensis’ behavioural responses to M. hyperodae on the three different host grasses (Manawa, Tama, and Samson). Each grass had a non-parasitoid control. This six-treatment experiment had a randomised block design with 15 replicates.
Each arena comprised an upper section of a transparent polyvinyl chloride jar (230 mm × 12 mm × 8 mm) inserted 20 mm into a 165 mm diameter plant pot containing pasteurised and moistened immature pallic soil (Landcare Research Ltd. 2016). To prevent the weevils from climbing the arena’s sides, polytetrafluoroethylene was added to the lower internal surface, and each arena was capped with.1-mm mesh for ventilation. Twenty-four hours before the experiment, four-week-old plants cropped to 150 mm and comprising 5-10 tillers were planted into each arena. On the day of the experiment, 2 h prior to the onset of darkness, 10 adult weevils were added to each arena, and 90 min later a single parasitoid was added to the non-controls.
For logistical reasons, the behavioural data were collected through direct visual observation on 30 March 2018 (5 blocks), 7 April 2018 (5 blocks), and 12 April 2018 (5 blocks) based on availability of parasitoids ≤5 days old. Each block consisted of one replicate of each treatment. The data from each treatment were analysed together regardless of which block they were recorded from. Data collection commenced at the onset of darkness and was conducted during four consecutive 40- to 60-min observation periods under red light. Weevil behaviours measured were the following:
Presence on plant: This comprised the number of weevils on the plant. These were taken to be at risk of parasitism, whereas individuals absent from the plant were presumed less susceptible because they were often stationary or semi-buried in the soil.
Feeding: These were weevils on above-ground portions of plants with their heads down and rostrums penetrating the grass epidermis. When a weevil is feeding, the abdomen is slanted upwards, thereby exposing some inter-sternite membranes and anus, making them vulnerable to parasitoid oviposition (Phillips, 2002). Feeding coincided with presence on plant.
After the behavioural experiments were completed, all the leaves were removed from all the plants in all treatments and stored between microscope slides at −20°C for further feeding-damage analysis. The area (mm2) of the weevil feeding scars on each leaf segment was measured using the Olympus CellSens imaging software in conjunction with an Olympus SZX12 stereo microscope at 7.0-12.5 X magnification with an Olympus SC180 camera attached.
Statistical Analysis
The behavioural variables analysed were the percentages of the 10 weevils in each replicate that were ‘present on plant’ and ‘feeding’. R version 3.6.3 (R Core Team, 2018) was used to analyse these data using a generalised linear mixed effects model with penalised quasi-likelihood estimation (GLMMPQL) to indicate the overall behavioural trends. The GLMMPQL was applied to all data collected in all observation periods and modelled correlation among repeated observations as first-order autoregressive random effects. The fixed effects of the GLMMPQL were grass cultivar (Manawa, Tama, and Samson), parasitoid presence/absence, and their interaction using binomial distributions through a logit link function. The GLMMPQL analysis was conducted using the glmmPQL function in the R package MASS (Venables and Ripley, 2002).
The behavioural responses within each of the four observation periods were investigated by fitting a generalised linear model (GLM) with a binomial error term using GenStat Release 20.1 (VSN International, 2019). The model terms for these analyses were replicate (a blocking factor), grass cultivar, parasitoid presence/absence trait responses, and their interaction. A logit (log-odds) link function was specified, and the dispersion parameter was estimated rather than fixed. A GLM was independently fitted for each behaviour in each of the four observation periods. Each GLM analysis estimated the means and least significant difference (LSD) at P < 0.05 for comparing each of the 6C2 = 15 pairs of means. These LSDs were relatively large for comparing two large means, small for comparing two small means, and intermediate for comparing small and large means.
Weevil feeding-scar analyses were conducted using GenStat Release 20.1 (VSN International, 2019) and assumed that 10 weevils were unable to consume all the plant material available to them during a 6-h observation. Untransformed feeding-scar areas were analysed by the ANOVA with all assumptions met.
Results
With reference to the results, the GLMMPQL analyses provided overall patterns using all the data available irrespective of observation period, whereas GLM focused on behaviour within each of the four observational periods.
On-Plant Presence of Listronotus bonariensis in Response to Microctonus hyperodae
Cultivar Effect on Weevil On-Plant Response in the Presence of the Parasitoid vs. the Paired Absence Controls
The GLMMPQL analysis showed that overall the observation intervals combined the percentage of weevils that were on the plants with M. hyperodae varied depending on the host grasses. Weevils on Manawa with the parasitoid had a significantly lower on-plant presence than those in the Manawa control (t = 2.4, df = 341, P < 0.02) (Figure 1). There were no significant differences in the on-plant presence of weevils on Tama (t = 0.5, df = 341, P > 0.05) or Samson (t = 0.7, df = 341, P > 0.05) irrespective of parasitoid presence or absence (Figure 1).
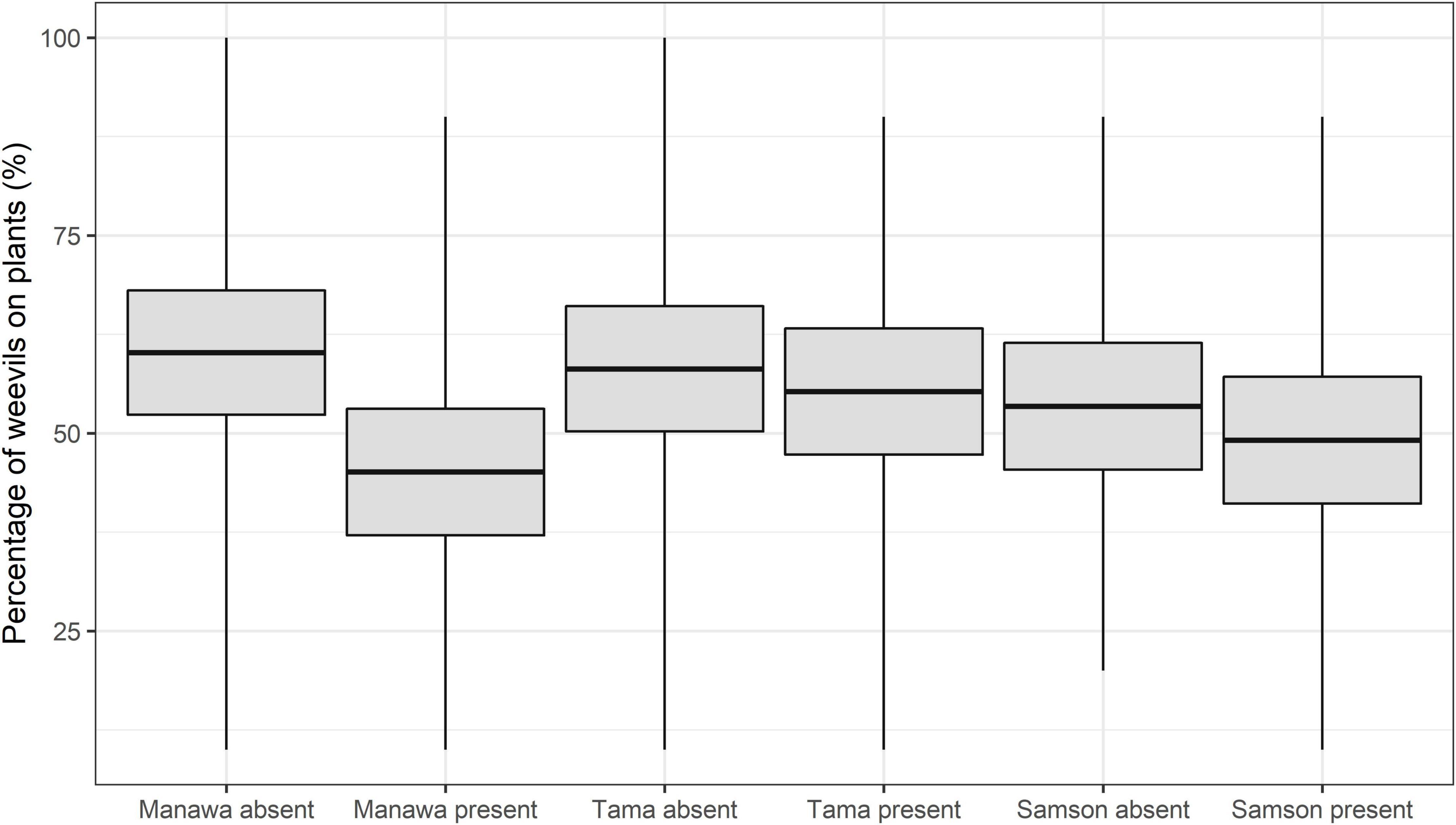
Figure 1. Mean percent of Listronotus bonariensis on Lolium grasses cv. Manawa, cv. Tama, and cv. Samson in the absence or presence of the parasitoid Microctonus hyperodae. The rectangles show the mean (central line) and 95% CL, and the tails show the range.
Similarly, the GLM analysis showed that on Manawa in the presence of the parasitoid, there were significantly lower percentages of weevils on plant in all four observation periods compared to the controls (df = 65 for observation periods 1-2, P < 0.01, P < 0.05, respectively, df = 66 for observation periods 3-4, P < 0.05, P < 0.01, respectively) (Table 1). Weevils on the Tama showed no significant on-plant response to the parasitoid. On Samson, there were significantly lower percentages of weevils on plant in the presence of the parasitoid (mean ± SEM: 42.2% ± 5.5%) compared to the control (58.7% ± 5.2%) only in observation period 4 (df = 66, P < 0.05) (Table 1).
Inter-Cultivar Comparisons of Weevil On-Plant Presence as Affected by the Parasitoid
The GLM analysis showed that in the presence of M. hyperodae, there were significantly fewer weevils on Manawa (39.6 ± 5.3%) than on Tama (54.7 ± 5.2%) in observation period 4 (df = 66, P < 0.05) (Table 1). Similarly, in the presence of the parasitoid, significantly more weevils were present on Tama (58.0 ± 4.3%) than on Samson (43.7 ± 4.4%) in observation period 3 (df = 66, P < 0.05) (Table 1).
Feeding Responses of Listronotus bonariensis in the Presence of Microctonus hyperodae on the Three Grass Cultivars
The feeding responses by L. bonariensis to M. hyperodae on the three grass cultivars were found to be far more pronounced than their on-plant presence responses (Table 1).
Cultivar Effect on Weevil Feeding in the Presence of the Parasitoid vs. the Paired Absence Controls
The GLMMPQL analysis showed that, in the presence of M. hyperodae, weevil feeding was significantly reduced on all grass cultivars (t < 3.3, df = 341, P < 0.04) (Figure 2). However, this response was most apparent on Manawa (t = 3.2, df = 341, P < 0.002) (Figure 2).
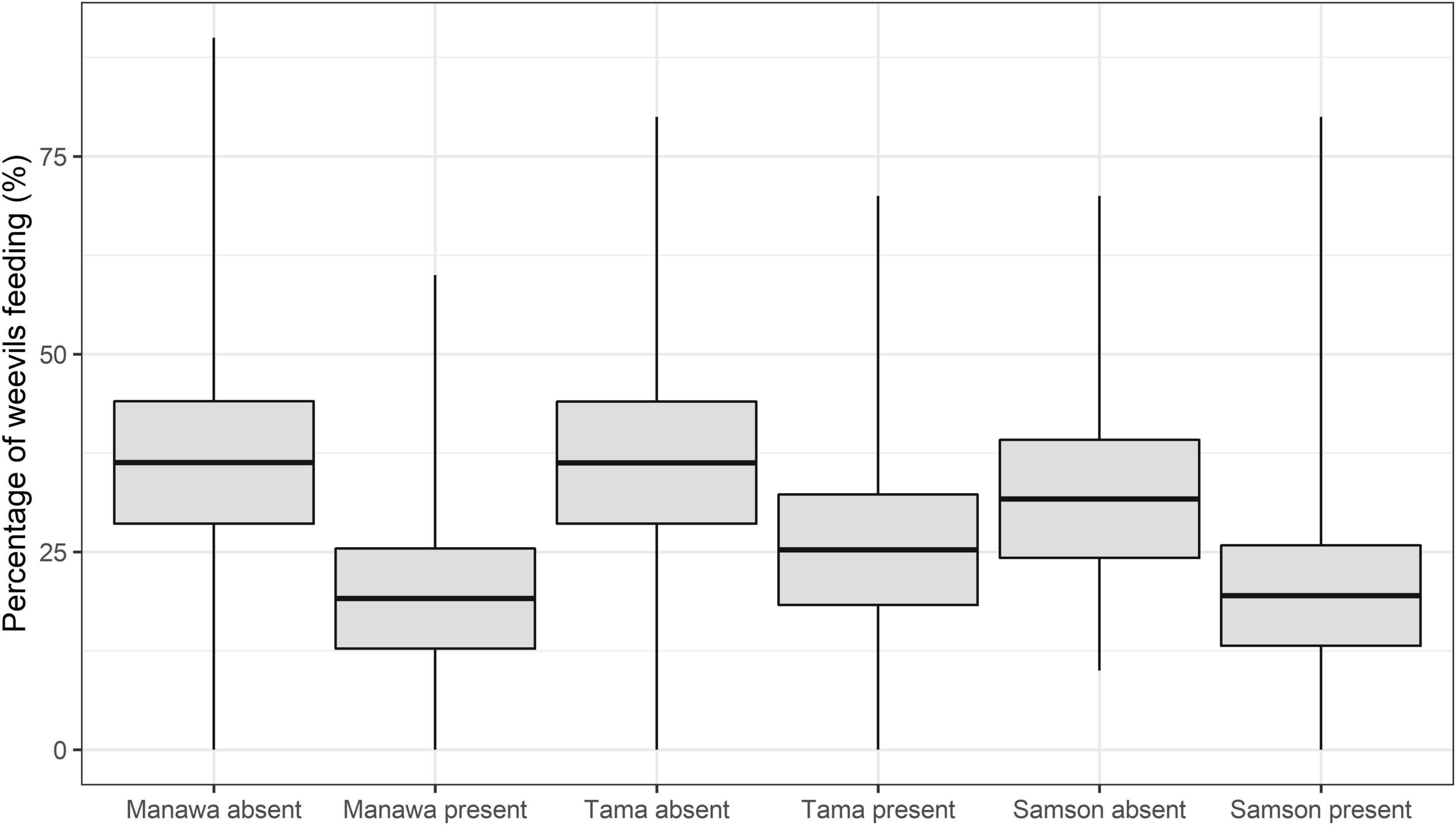
Figure 2. The mean percent feeding of the weevil L. bonariensis in the absence or presence of the parasitoid M. hyperodae on Lolium grasses cv. Manawa, cv. Tama, and cv. Samson. The rectangles show the mean (central line) and 95% CL, and the tails show the range.
Consistent with the GLMMPQL analysis, the GLM showed that weevils on Manawa fed less in the presence of the parasitoid compared to its control in all observation periods (df = 65 for observation periods 1-2, P < 0.05, P < 0.001, respectively (df = 66 for observation periods 3-4, P < 0.01, P < 0.01 respectively) (Table 2). Weevils on Tama fed significantly less in the presence of the parasitoid (mean ± SEM: 19.3% ± 4.4%) compared to the control (38.2% ± 5.7%) only in observation period 4 (df = 66, P < 0.05) (Table 2). Weevils on Samson fed less in the presence of the parasitoid compared to the control only in observation periods 3 and 4 (df = 66, P < 0.01) (Table 2).
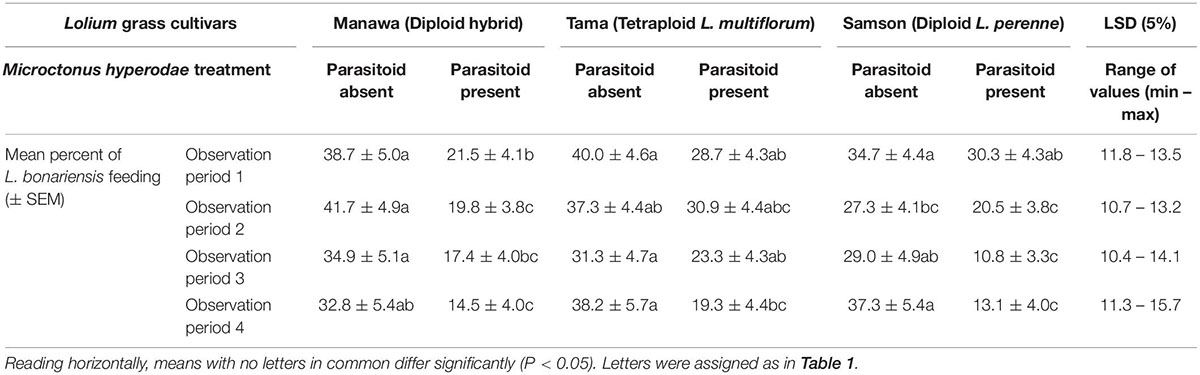
Table 2. Mean percentages (± SEM) of L. bonariensis feeding on three Lolium grass cultivars in the absence (control) or presence of M. hyperodae.
Inter-Cultivar Comparisons of Weevil Feeding Responses as Affected by the Parasitoid
In the presence of the parasitoid, the GLM revealed only one significant difference between cultivars in the percentage of weevil feeding. In observation period 3, more weevils fed on Tama (23.3 ± 4.3%) than Samson (10.8 ± 3.3%) (df = 66, P < 0.05). However, fewer weevils also tended to feed on Manawa and Samson than Tama when the parasitoid was present (Table 2).
Grass Consumption by Listronotus bonariensis in Response to Microctonus hyperodae
Cultivar Effect on Weevil Grass Consumption in the Presence of the Parasitoid vs. the Paired Absence Controls
The ANOVA indicated that, on Manawa, the feeding area was significantly less in the presence of the parasitoid (mean ± SEM: 292.7 mm2 ± 46.5 mm2) than in its absence (424.6 mm2 ± 46.5 mm2) (df = 69, P < 0.05) (Figure 3). However, on Tama and Samson, there were no significant differences between parasitoid treatments (Figure 3).
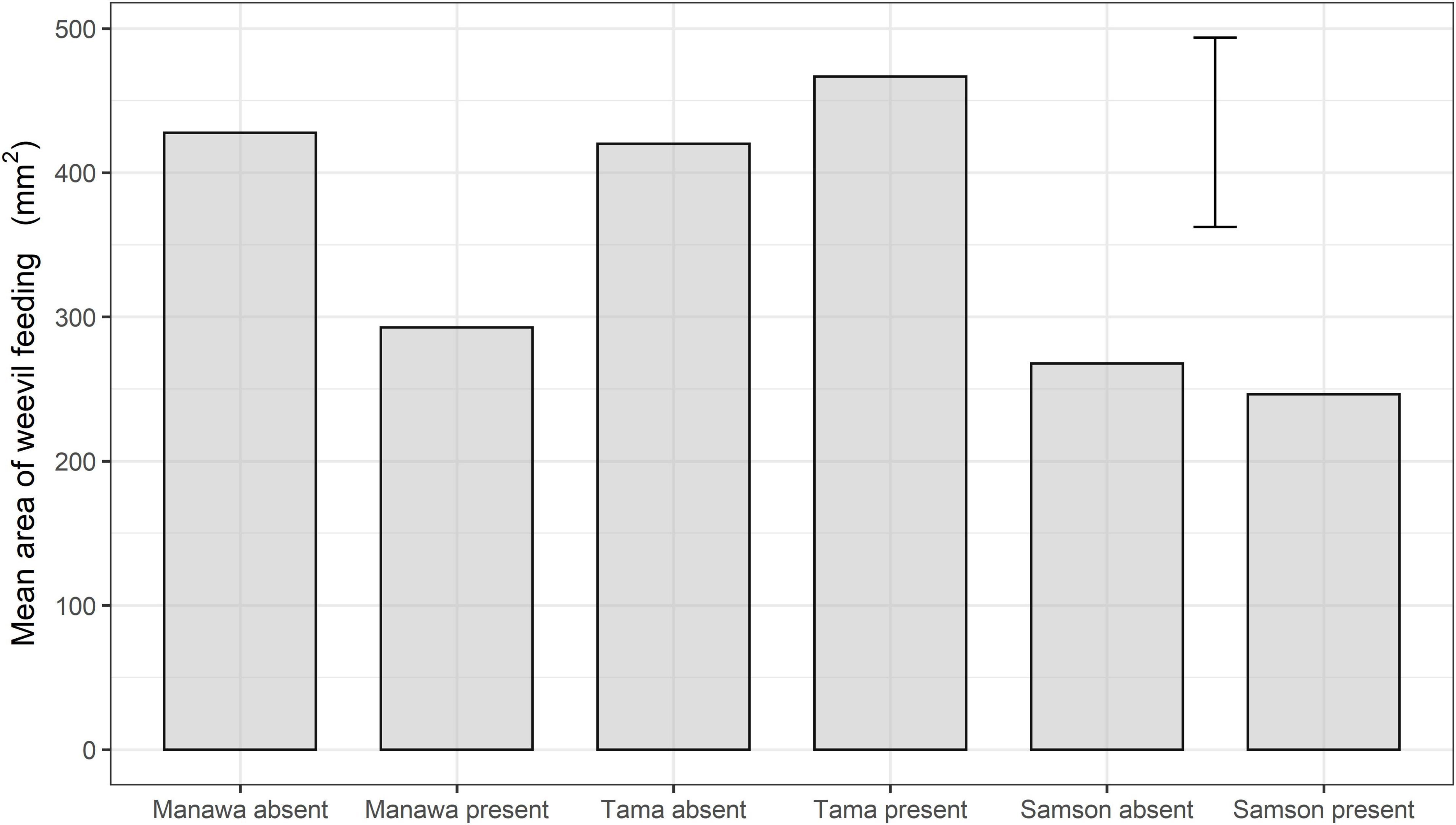
Figure 3. Mean feeding area (mm2) by the weevil L. bonariensis on Manawa, Tama, and Samson Lolium grasses in the absence or presence of the parasitoid M. hyperodae. The histogram shows the mean and an error term based on Fisher’s unprotected least significant differences (5%).
Weevil Feeding Area and the Effects of Parasitoid Presence
In the presence of the parasitoid, the feeding scar area in Manawa was reduced significantly by 31% compared to the control. There we no significant differences in either Tama or Samson (Figure 3).
The feeding area was significantly higher on Tama than on Samson both in the absence (df = 69, P < 0.05) and the presence of the parasitoid (df = 69, P < 0.001) (Figure 3). In the absence of the parasitoid, there was significantly more weevil feeding on Manawa (424.6 mm2 ± 46.5 mm2) than on Samson (267.7 mm2 ± 46.5 mm2) (df = 69, P < 0.05).
Discussion
Host Plants Affecting Parasitoid-Avoidance Behaviours
This contribution has elucidated how host-plant species and/or their ploidy influences the extent of the selected-for L. bonariensis-avoidance behaviour in the presence of M. hyperodae (Shields, 2019). The diploid Manawa induced the greatest extent of avoidance behaviour by the weevils, which was manifested by lower on-plant presence and reduced feeding. Such behaviours were delayed on both the diploid Samson and the tetraploid Tama, with the latter being minor (Figures 1–3 and Tables 1, 2). These results therefore provide an explanation for the observed range of field and laboratory observations of weevil parasitism rates in pastures comprising different species and cultivars (Goldson et al., 2015; Goldson and Tomasetto, 2016; Tomasetto et al., 2017a,b).
Diploid Manawa
In the absence of the parasitoid, Manawa is a preferred host plant for the weevil, comparable with Tama (Goldson, 1982; Barker, 1989), thereby being characterised by similar weevil on-plant presence, feeding intensity (Figures 1–3 and Tables 1, 2), and ovipositional effort (Goldson, 1982; Barker, 1989). However, in the presence of the parasitoid, the weevil’s avoidance response to the parasitoid was significantly stronger on Manawa than on the other cultivars with greater reductions in on-plant presence, percentage of weevil feeding, and leaf area consumed (Figures 1–3 and Tables 1, 2). Significantly, in the 1990s, parasitism rates in Manawa were typically ca. 75%, in keeping with the other diploid Lolium grasses at that time (Goldson and Tomasetto, 2016). However, through parasitoid selection pressure causing enhanced weevil avoidance behaviour, parasitism levels have now declined to < ca. 46% in both the Manawa and several L. perenne cultivars (Goldson and Tomasetto, 2016; Tomasetto et al., 2017b).
These recently observed contrasting weevil behaviours on Manawa that now occur in the presence and absence of the parasitoid may well reflect the cultivar’s genetics in that it has characteristics of both L. perenne and L. multiflorum. In the breeding of this hybrid cultivar for livestock production, Corkill (1945) sought a medium tillered ryegrass that maximised the desirable features of palatability (e.g., less lignin and cellulose (Barker, 1989)) and the rapid establishment of L. multiflorum, while at the same time maintaining some degree of the density and persistency of L. perenne. Generally, it has been found that characteristics of high-performance pasture grasses for livestock production similarly support high weevil populations (e.g., Goldson, 1982; Barker, 1989; Ferguson et al., 2019).
Tetraploid Tama
Tama has maintained the same high parasitism levels that were originally found in the other grasses in the 1990s (Goldson and Tomasetto, 2016; Tomasetto et al., 2017c). This is reflected by the absence of any decline in weevil on-plant presence and limited feeding reduction when the parasitoid was present (Figure 1 and Table 2). Such a result would indicate that the weevils continue to be vulnerable to attack.
Unlike the other grasses discussed in this contribution, short-lived Tama plantings (and similar tetraploid L. multiflorum varieties) are not widespread (B.R. Belgrave, Grasslanz Technology Ltd., pers. comm.). This therefore may limit the potential for L. bonariensis to respond to selection pressure exerted by the M. hyperodae on Tama due to the cultivar’s relative scarcity. Further, the preference of weevils for Tama and other short-lived tetraploid L. multiflorum cultivars compared to diploid and other tetraploid cultivars (Goldson, 1982; Barker, 1989) could also work against any parasitoid-avoidance behaviour due to Tama’s superior nutritional quality (Charlton and Stewart, 1999; Sattler et al., 2016). These attractive short-lived tetraploid qualities include higher cell content of water (Sugiyama, 2006), nitrogen (Gaynor and Hunt, 1982), and water-soluble carbohydrates (Vartha and Bailey, 1974; Aderibigbe et al., 1982).
Diploid Samson
Lolium perenne pastures such as Samson are distributed across New Zealand and are favoured by farmers due to their persistence arising from their continuous production of secondary tillers (O’Connor et al., 2020). As mentioned above, in the 1990s, parasitism levels were often around 75% (Barker, 2013; Goldson and Tomasetto, 2016); however, these have now reduced to as little as ca. 14% in the central North Island and ca. 32% in central South Island regions of New Zealand (Shields et al., 2022). In keeping with these results, it was found that when exposed to the parasitoid, weevils on Samson showed similar responses to weevils on those on Manawa, though to a lesser extent (Figure 2 and Tables 1, 2). Possibly, the behavioural responses on Samson were less obvious than on Manawa because, in general, less feeding was observed (Figure 3), probably due to L. perenne having higher cellulose and sclerenchyma cell content (Evans, 1964, 1967).
Varying Effects of Ploidy on Parasitism Rates
This study has highlighted an effect on L. bonariensis parasitoid-avoidance behaviour and parasitism rates based on the influence of tetraploidy in L. multiflorum such as Tama. Unlike the other grasses, there was minimal weevil parasitoid-avoidance behaviour and no parasitism decline (e.g., Goldson et al., 2015; Goldson and Tomasetto, 2016; Tomasetto et al., 2017a). Conversely, reduced parasitism rates have indeed been found in diploid L. multiflorum to a similar extent to that found in both diploid and tetraploid L. perenne (Goldson and Tomasetto, 2016; Tomasetto et al., 2017b). As well as lack of evolved behaviour on Tama (see above), a possible explanation for the observed weevil’s ongoing presence and feeding is that tetraploidy enhances characteristics (Charlton and Stewart, 1999; Sattler et al., 2016) favourable to the weevil (Barker et al., 1989), as such higher nitrogen content (Gaynor and Hunt, 1982). There may also be additional plant characteristics that contribute to the weevil’s behaviour on tetraploid L. multiflorum such as plant volatiles, but this was beyond the scope of this contribution.
The weevil’s parasitoid-avoidance pattern was found to be very different in the case of the hybrid Manawa grass. Here, in the parasitoid’s absence, the weevil exhibits behaviour similar to that of Tama. Conversely, in the parasitoid’s presence, the weevil shows all of the characteristics of those found on diploid Lolium plants. It is interesting to surmise that such departure from the pattern above could be the result of the hybrid having genetic components of both L. perenne and L. multiflorum. Notably though, it is unlikely that this ‘perennial and Italian ryegrass’ cross would have comprised any polyploidy as these typically have low seed germination rates (Griffiths et al., 1971).
Conclusion
This study is one of the few that has demonstrated how evolved parasitoid-avoidance behaviours of a weevil pest species have varied according to the effect of plant species and cultivars. These findings also accommodate the original observations in 1990s that all pasture grasses had similar levels of parasitism and how varying selection pressures according to the grass type has now led to uneven levels of parasitism (Goldson and Tomasetto, 2016). Such information will provide insight into the management of successful importation biological control agents, particulary in broad-acre applications including pastures.
Data Availability Statement
The raw data supporting the conclusions of this article will be made available by the authors, without undue reservation upon request.
Author Contributions
MS, SG, SW, and CP conceived the ideas and designed the study. MS conducted the experiments. MS, SW, and SG collected the data. MS, CV, and CP analysed the data. MS and SG led the manuscript writing. All authors contributed to the drafts and gave final approval for publication.
Funding
This project was supported by the New Zealand Tertiary Education Commission Centre of Research Excellence grant to the Bio-Protection Research Centre (BPRC) and AgResearch. Publishing was partially funded by the Lincoln University Open Access Fund.
Conflict of Interest
SG, CP, and CV were employed by AgResearch Ltd.
The remaining authors declare that the research was conducted in the absence of any commercial or financial relationships that could be construed as a potential conflict of interest.
Publisher’s Note
All claims expressed in this article are solely those of the authors and do not necessarily represent those of their affiliated organizations, or those of the publisher, the editors and the reviewers. Any product that may be evaluated in this article, or claim that may be made by its manufacturer, is not guaranteed or endorsed by the publisher.
Acknowledgments
The authors are grateful for the collaboration from colleagues at AgResearch Ltd., and the BPRC including Jacquelyn Bennett, Kate Scanlan, Malvika Bana, Dave Saville, Federico Tomasetto, Hazel Chapman, and John Caradus.
References
Aderibigbe, A. O., Church, D. C., Frakes, R. V., and Petersen, R. G. (1982). Factors determining palatability of ryegrass to cattle. J. Anim. Sci. 54, 164–172.
Barker, G. M. (1989). Grass host preferences of Listronotus bonariensis (Coleoptera: Curculionidae). J. Econ. Entomol. 82, 1807–1816. doi: 10.1093/jee/82.6.1807
Barker, G. M. (2013). Biology of the introduced biocontrol agent Microctonus hyperodae (Hymenoptera: Braconidae) and its host Listronotus bonariensis (Coleoptera: Curculionidae) in Northern New Zealand. Environ. Entomol. 42, 902–914. doi: 10.1603/EN11248
Barker, G. M., and Addison, P. J. (2006). Early impact of endoparasitoid Microctonus hyperodae (Hymenoptera: Braconidae) after its establishment in Listronotus bonariensis (Coleoptera: Curculionidae) populations of Northern New Zealand Pastures. J. Econ. Entomol. 99, 273–287. doi: 10.1603/0022-0493-99.2.273
Barker, G. M., Pottinger, R. P., and Addison, P. J. (1989). Population dynamics of the Argentine stem weevil (Listronotus bonariensis) in pastures of Waikato. New Zealand. Agric. Ecosyst. Environ. 26, 79–115. doi: 10.1016/0167-8809(89)90021-2
Casanovas, P., Goldson, S. L., and Tylianakis, J. M. (2018). Asymmetry in reproduction strategies drives evolution of resistance in biological control systems. PLoS One 13:e0207610. doi: 10.1371/journal.pone.0207610
Charlton, J. F. L., and Stewart, A. V. (1999). Pasture species and cultivars used in New Zealand-a list. Proc. New Zeal. Grassl. Assoc. 61, 147–166.
Corkill, L. (1945). Short rotation ryegrass, its breeding and characteristics. New Zeal. J. Agric. 71, 465–468.
Evans, P. S. (1964). A Study of leaf strength in four ryegrass varieties. New Zeal. J. Agric. Res. 7, 508–513. doi: 10.1080/00288233.1964.10416378
Evans, P. S. (1967). Leaf strength studies of pasture grasses II. Strength, cellulose content and sclerenchyma tissue proportions of eight grasses grown as single plants. J. Agric. Sci. 69, 175–181. doi: 10.1017/S0021859600018566
Ferguson, C. M., Barratt, B. I. P., Bell, N., Goldson, S. L., Hardwick, S., Jackson, M., et al. (2019). Quantifying the economic cost of invertebrate pests to New Zealand’s pastoral industry. New Zeal. J. Agric. Res. 62, 255–315. doi: 10.1080/00288233.2018.1478860
Gaynor, D. L., and Hunt, W. F. (1982). “Nitrogen and water effects on Hyperoides bonariensis susceptibility in perennial ryegrass in New Zealand (Coleoptera: Curculionidae),” in Proceedings of the 3rd Australasian Conference on Grassland Invertebrate Ecology, ed. K. E. Lee (Washington, DC: Government Printing Division).
Goldson, S. L. (1982). An examination of the relationship between Argentine stem weevil Listronotus bonariensis (Kuschel) and several of its host grasses. New Zeal. J. Agric. Res. 25, 395–403. doi: 10.1080/00288233.1982.10417903
Goldson, S. L., Barker, G. M., Chapman, H. M., Popay, A. J., Stewart, A. V., Caradus, J. R., et al. (2020). Severe insect pest impacts on New Zealand pasture: the plight of an ecological outlier. J. Insect Sci. 20, 1–17. doi: 10.1093/jisesa/ieaa018
Goldson, S. L., Barron, M. C., Kean, J. M., and Van Koten, C. (2011). Argentine stem weevil (Listronotus bonariensis, Coleoptera: Curculionidae) population dynamics in Canterbury, New Zealand dryland pasture. Bull. Entomol. Res. 101, 295–303. doi: 10.1017/S0007485310000507
Goldson, S. L., McNeill, M. R., Bana, M., Olaniyan, O., Popay, A. J., Barratt, B. I. P., et al. (2022). Parasitoid-based biocontrol ecology of Listronotus bonariensis (Kuschel) in New Zealand’s exotic pasture ecosystems; implications of sampling bias, relict behaviour and pest dispersal. New Zeal. J. Agric. Res. [Epub ahead of print].
Goldson, S. L., McNeill, M. R., Phillips, C. B., and Proffitt, J. R. (1992). Host specificity testing and suitability of the parasitoid Microctonus hyperodae (Hym.: Braconidae, Euphorinae) as a biological control agent of Listronotus bonariensis (Col.: Curculionidae) in New Zealand. Entomophaga 37, 483–498. doi: 10.1007/BF02373121
Goldson, S. L., McNeill, M. R., Proffitt, J. R., Barker, G. M., Addison, P. J., Barratt, B. I. P., et al. (1993). Systematic mass rearing and release of Microctonus hyperodae (Hym.: Braconidae, Euphorinae), a parasitoid of the Argentine stem weevil Listronotus bonariensis (Col.: Curculionidae) and records of its establishment in New Zealand. Entomophaga 38, 527–536.
Goldson, S. L., McNeill, M. R., Stufkens, M. W., Proffitt, J. R., Pottinger, R. P., and Farrell, J. A. (1990). “Importation and quarantine of Microctonus hyperodae, a South American parasitoid of Argentine stem weevil,” in Proceedings of the 43rd New Zealand Weed and Pest Control Conference, (Washington, DC: Government Printing Division), 334–338.
Goldson, S. L., Proffitt, J. R., and Baird, D. B. (1998). Establishment and phenology of the parasitoid Microctonus hyperodae (Hymenoptera: Braconidae) in New Zealand. Environ. Entomol. 27, 1386–1392. doi: 10.1093/ee/27.6.1386
Goldson, S. L., and Tomasetto, F. (2016). Apparent acquired resistance by a weevil to its parasitoid is influenced by host plant. Front. Plant Sci. 7:1259. doi: 10.3389/fpls.2016.01259
Goldson, S. L., Tomasetto, F., and Popay, A. J. (2014). Biological control against invasive species in simplified ecosystems: its triumphs and emerging threats. Curr. Opin. Insect Sci. 5, 50–56. doi: 10.1016/j.cois.2014.09.003
Goldson, S. L., Tomasetto, F., and Popay, A. J. (2015). Effect of Epichloë endophyte strains in Lolium spp. cultivars on Argentine stem weevil parasitism by Microctonus hyperodae. New Zeal. Plant Prot. 68, 204–211.
Griffiths, D. J., Pegler, R. A. D., and Tonguthaisri, T. (1971). Cross compatibility between diploid and tetraploid perennial ryegrass (Lolium perenne L.). Euphytica 20, 102–112. doi: 10.1007/BF00146780
Kelsey, J. M. (1958). Damage in ryegrasses by Hyperodes griseus Hust. New Zeal. J. Agric. Res. 1, 790–795. doi: 10.1080/00288233.1958.10431585
Marshall, G. A. K. (1937). New Curculionidae (Col.) from New Zealand. Trans. New Zeal. Inst. 67, 316–340.
McNeill, M. R., Goldson, S. L., Proffitt, J. R., Phillips, C. B., and Addison, P. J. (2002). A description of the commercial rearing and distribution of Microctonus hyperodae (Hymenoptera: Braconidae) for biological control of Listronotus bonariensis (Kuschel) (Coleoptera: Curculionidae). Biol. Control 24, 167–175. doi: 10.1016/S1049-9644(02)00018-X
O’Connor, J. R., Crush, J. R., and Jahufer, Z. (2020). Identifying morphological traits associated with vegetative persistence in the perennial ryegrass (Lolium perenne L.) cultivar “Grasslands Samson.”. J. New Zeal. Grasslands 82, 139–147. doi: 10.33584/JNZG.2020.82.434
Pascoal, S., Cezard, T., Eik-Nes, A., Gharbi, K., Majewska, J., Payne, E., et al. (2014). Rapid convergent evolution in wild crickets. Curr. Biol. 24, 1369–1374. doi: 10.1016/j.cub.2014.04.053
Pennisi, E. (2017). In a first, natural selection defeats a biocontrol insect. Science 356:570. doi: 10.1126/science.356.6338.570
Phillips, C. B. (2002). Observations of oviposition behavior of Microctonus hyperodae Loan and M. aethiopoides Loan (Hymenoptera: Braconidae: Euphorinae). J. Hymenopt. Res. 11, 326–337.
Phillips, C. B., Barker, G. M., Roberts, R. L., McNeill, M. R., and Goldson, S. L. (1996). “Fecundity of wild and laboratory reared ecotypes of Microctonus hyperodae Loan (Hymenoptera: Braconidae),” in Proceedings of the Forty Ninth New Zealand Plant Protection Conference, Nelson, 285–290.
Phillips, C. B., and Kean, J. M. (2017). Response of parasitoid egg load to host dynamics and implications for egg load evolution. J. Evol. Biol. 30, 1313–1324. doi: 10.1111/jeb.13095
Popay, A. J., McNeill, M. R., Goldson, S. L., and Ferguson, C. M. (2011). The current status of Argentine stem weevil (Listronotus bonariensis) as a pest in the North Island of New Zealand. New Zeal. Plant Prot. 64, 55–62.
Pottinger, R. P. (1961). Argentine stem weevil Hyperodes bonariensis, an insect pest of pastures. Canterbury Chamb. Commer. Agric. Bull. 380:5.
R Core Team (2018). R: A Language and Environment for Statistical Computing. Vienna: R Foundation for Statistical Computing.
Sattler, M. C., Carvalho, C. R., and Clarindo, W. R. (2016). The polyploidy and its key role in plant breeding. Planta 243, 281–296.
Shields, M. W. (2019). Host-Parasitoid Avoidance Behaviour in the Context of Contemporary Evolution in Insect Classical Biological Control. [PhD thesis]. Lincoln: Lincoln University.
Shields, M. W., Wratten, S. D., van Koten, C., Phillips, C. B., Gerard, P. J., and Goldson, S. L. (2022). Behavioural mechanisms drive contemporary evolution in a failing insect-parasitoid classical biological control programme. Ecol. Evol. [Epub ahead of print].
Stewart, A. V., Goldson, S. L., Marris, J. W. M., Rubenstein, J. M., and Rolston, P. M. (2022). Purity of century old New Zealand forage seed samples based on detected weed seed, fungal and insect contaminants, in particular Argentine stem weevil and ryegrass. New Zeal. J. Agric. Res. [Epub ahead of print].
Sugiyama, S. -i. (2006). Responses of shoot growth and survival to water stress gradient in diploid and tetraploid populations of Lolium multiflorum and L. perenne. Grassland Sci. 52, 155–160. doi: 10.1111/j.1744-697X.2006.00062.x
Tomasetto, F., Casanovas, P., Brandt, S. N., and Goldson, S. L. (2018). Biological control success of a pasture pest: has its parasitoid lost its functional mojo? Front. Ecol. Evol. 6:215.
Tomasetto, F., Goldson, S., and Olaniyan, S. (2017a). Italian Ryegrass Does It Better: Effect of Lolium Multiflorum on Argentine Stem Weevil Parasitism by Microctonus Hyperodae. Available online at: http://researcharchive.lincoln.ac.nz/handle/10182/9239 (accessed November 24, 2021).
Tomasetto, F., Olaniyan, O., and Goldson, S. L. (2017b). Ploidy in Lolium spp. cultivars affects Argentine stem weevil parasitism by Microctonus hyperodae. New Zeal. Plant Protoc. 70:326. doi: 10.30843/nzpp.2017.70.98
Tomasetto, F., Tylianakis, J. M., Reale, M., Wratten, S., and Goldson, S. L. (2017c). Intensified agriculture favors evolved resistance to biological control. Proc. Natl. Acad. Sci. U.S.A. 114, 3885–3890. doi: 10.1073/pnas.1618416114
Vartha, E. W., and Bailey, R. W. (1974). Soluble carbohydrate composition of a winter-growing tetraploid annual ryegrass, ‘Grasslands Tama’ Westerwolds. New Zeal. J. Exp. Agric. 2, 17–22.
Venables, W. N., and Ripley, B. D. (2002). Modern Applied Statistics with S. New York, NY: Springer.
Keywords: biological control of insect, host plant effect, Lolium multiflorum, Lolium perenne, parasitoid, pasture, ploidy, rapid evolution
Citation: Shields MW, Wratten SD, Phillips CB, Van Koten C and Goldson SL (2022) Plant-Mediated Behavioural Avoidance of a Weevil Towards Its Biological Control Agent. Front. Plant Sci. 13:923237. doi: 10.3389/fpls.2022.923237
Received: 19 April 2022; Accepted: 30 May 2022;
Published: 23 June 2022.
Edited by:
Manuel Martinez, Polytechnic University of Madrid, SpainReviewed by:
Jana Collatz, Agroscope (Switzerland), SwitzerlandMónica Asunción Hurtado Ruiz, University of Jaume I, Spain
Copyright © 2022 Shields, Wratten, Phillips, Van Koten and Goldson. This is an open-access article distributed under the terms of the Creative Commons Attribution License (CC BY). The use, distribution or reproduction in other forums is permitted, provided the original author(s) and the copyright owner(s) are credited and that the original publication in this journal is cited, in accordance with accepted academic practice. No use, distribution or reproduction is permitted which does not comply with these terms.
*Correspondence: Morgan W. Shields, morgan.shields@ecan.govt.nz
†These authors have contributed equally to this work
‡deceased:Deceased