- 1School of Life, Qufu Normal University, Qufu, China
- 2College of Forestry, Inner Mongolia Agricultural University, Hohhot, China
- 3Center for Ecological Research and Key Laboratory of Sustainable Forest Ecosystem Management–Ministry of Education, College of Forestry, Northeast Forestry University, Harbin, China
- 4College of Forestry, Northwest A&F University, Yangling, China
Of all forest biomes, boreal forests are experiencing the most significant warming. Drought caused by warming has a dramatic impact on species in boreal forests. However, little is known about whether the growth of trees and shrubs in boreal forests responds consistently to warming and drought. We obtained the tree-ring width data of 308 trees (Larix gmelinii and Pinus sylvestris var. mongolica) and 133 shrubs (Pinus pumila) from 26 sites in northeastern China. According to the climate data from 1950 to 2014, we determined three extreme drought years (1954, 1967, and 2008). The response difference of radial growth of trees and shrubs in boreal forests to drought was compared using resilience index, moving correlation and response analysis. The results showed that high temperature (mean and maximum temperature) in previous and current growing seasons promoted the growth of P. pumila, but inhibited the growth of trees. On the contrary, wetter conditions (higher PDSI) promoted tree growth but were not conducive to P. pumila growth in high latitudes. Moving correlation analysis showed similar results. In addition, water deficit was more likely to inhibit P. pumila growth in low latitudes. The drought resistance of P. pumila was stronger than that of L. gmelinii and P. sylvestris var. mongolica. Therefore, the growth loss and recovery time of P. pumila during drought was less than those of trees. We concluded that L. gmelinii and P. sylvestris var. mongolica are more prone to growth decline than P. pumila after the drought caused by climate warming. In the future climate warming, shrub growth may benefit more than trees. Our findings are of great significance in predicting the future changes in ecosystem composition and species distribution dynamics in extreme climate susceptible areas.
Introduction
According to the latest assessment report of IPCC, due to the increase in atmospheric greenhouse gas concentration caused by human activities, the global average surface temperature has increased by 1.09°C by the early 21st century (IPCC, 2021). Climate warming has led to an increasing frequency, amplitude and duration of extreme climate events, which is expected to continue in the future (Li et al, 2020). This poses a key challenge to the stability of forest ecosystems and the multiple ecosystem services they provide (Seidl et al., 2017; Bottero et al., 2021). Extreme climate (such as extreme drought) can affect plant function, primary and secondary growth, tree recruitment and mortality, carbon and water balance of forest ecosystems (Senf et al., 2020; Bottero et al., 2021). Over the past few decades, a decline in global tree growth and an increase in forest mortality has been observed in various forest biomes through severe drought events related to global warming (Choat et al., 2018; Arellano et al., 2019; Senf et al., 2020).
Boreal forests are widespread, accounting for approximately one-third of the global forest area (Sullivan et al., 2021) and play a key role in the global carbon cycle, water cycle and climate change (Gauthier et al., 2015). Especially they are experiencing the most significant warming of any forest biome (IPCC, 2021). Due to significant climate change, the growth of trees in boreal forests has been seriously affected, especially coniferous trees (Mayor et al., 2017; Lopez et al., 2021; Sturm et al., 2022). Many studies have been conducted on the impacts of drought on forests in North America and Europe, but few studies have assessed the impact of drought on boreal forests in Asia (Bottero et al., 2021; Sturm et al., 2022). In addition, there is a significant drought legacy effect on tree growth. In 1–4 years after severe drought events, the recovery time of tree growth can be used to understand the impact of drought on boreal forest ecosystem, including the cycle of carbon feedback on climate change (Anderegg et al., 2013; Camarero et al., 2021a; Iqbal et al., 2021). In some cases, the lag response of tree radial growth to precipitation and temperature anomalies may last for 36–57 months, and high antecedent precipitation improves tree radial growth (Ogle et al., 2015; Jiang et al., 2019). The legacy effect of drought on growth is highly variable, either slowly decreasing or rapidly increasing (Kannenberg et al., 2020). The sensitivity of radial growth to climate change is often manifested in changes in ring width and is closely related to the resilience of drought events (Lloret et al., 2011; Mérian and Lebourgeois, 2011; Duan et al., 2022). The growth resilience of different species varies greatly, depending on the specific physiological response of species to drought events (Anderegg et al., 2013; Gazol et al., 2017, 2018; Duan et al., 2022). However, the resistance and resilience of forest species depend not only on species-specific patterns but also on life forms. Wu et al. (2018) found that the legacy effect of drought on vegetation growth is different for trees, shrubs and grass. Deep-rooted trees showed drought legacy reaction and reduced growth within 4 years after extreme drought. In contrast, the drought legacy effects of shrubs and grasses are about 2 and 1 year, respectively. Species mortality caused by drought is related to species-specific water deficit sensitivity and may lead to changes in species composition, making more drought-tolerant species dominant (Férriz et al., 2021). Therefore, as extreme climate events increase, exploring the response of different species to extreme climate events is crucial to understanding forest dynamics (Babst et al., 2019; Bastos et al., 2020; McDowell et al., 2020; Zweifel et al., 2021).
Trees and shrubs respond differently to global climate change in many natural environments due to their different life forms (Morales et al., 2012; Götmark et al., 2016; Shetti, 2018). Previous studies have shown that shrubs have been more sensitive to climate warming than trees over the past few decades (Morales et al., 2012; Pellizzari et al., 2017). Drought will increase the risk of xylem cavitation of larch and reduce tree vitality (Oberhuber et al., 2014). Therefore, the occurrence of drought is beneficial to pine forests and exacerbates the decline of larch forests in the same area (Dulamsuren et al., 2009). Under the continuous influence of climate warming, pine will gradually replace the natural population of larch in Siberian forests (Urban et al., 2019). Boreal forests are particularly vulnerable to climate change because they are usually located on permafrost (Helbig et al., 2016; Zhang et al., 2019a,b). Meanwhile, tall shrubs are encroaching into alpine and Arctic tundra landscapes, possibly responding to rising air temperatures (Tape et al., 2006; Forbes et al., 2010; Hallinger et al., 2010; IPCC, 2021). Pellizzari et al. (2017) found that the decline in tree growth in the Mediterranean region after the 1980s may be caused by drought stress exacerbated by climate warming, but the drought will not affect juniper trees. We also found that larch and pine trees are more vulnerable to global warming than shrubs (Yang et al., 2020). The negative effect of temperature in growing season on the growth of larch and pine is greater than that on Pinus pumila, and the promotion effect of precipitation in winter and spring on P. pumila is the greatest (Yang et al., 2020). However, it is not entirely clear whether the trees and shrubs in boreal forests of China have such different responses to extreme climate change.
Pinus sylvestris var. mongolica and Larix gmelinii are important tree species in boreal forests of Asia (Zhang et al., 2019a), and P. pumila is an important shrub species in boreal forests. In recent decades, drought has occurred frequently in northeast China, which strongly impacts on the radial growth of major tree species in boreal forests, especially in semi-arid sites (Zhang et al., 2021). After a serious drought, water-sensitive trees usually reduce their radial growth and recover previous growth when subsequent rainfall increases (Fang et al., 2017). To clarity, the impact of drought events on the growth of different species and life-form species in boreal forests. We investigated the growth pattern of three main species in boreal forest, northeast China: Dahurian larch (L. gmelinii), Mongolian pine (P. sylvestris var. mongolica), Siberian dwarf pine (P. pumila) and their response to climate change. The study aims to compare the response of tree and shrub growth to drought to help predict the future dynamics of boreal forests and feedback to the global climate system. We hypothesize that: (1) the main climate limiting factors of radial growth of L. gmelinii, P. sylvestris var. mongolica and P. pumila are different; (2) Compared with shrubs, tree growth has strong resistance to drought and weak recovery and resilience; (3) After a drought, the recovery time and total growth reduction in radial growth of tree species are less than those of shrubs.
Materials and Methods
Filed Sampling
The study area is located in the boreal forests of northeastern China, with a latitude range from 44°06′ to 52°59′ N and a longitude from 120°44′ to 128°28′ E (Table 1, Figure 1). It belongs to the cold temperate continental monsoon climate, and it is cool and rainy in summer and cold and dry in winter. The mean annual precipitation (1950–2014) is between 415.6 and 636.2 mm, and more than 68% occurs from June to August. The mean annual temperature ranges from −6.3°C to 0.5°C. January is the coldest month (mean minimum temperature −38.2°C at ZL site), and July is the warmest month (mean maximum temperature 25.3°C at TS site). The annual frost-free period is 80–120 days, with early and late frost in September and May.
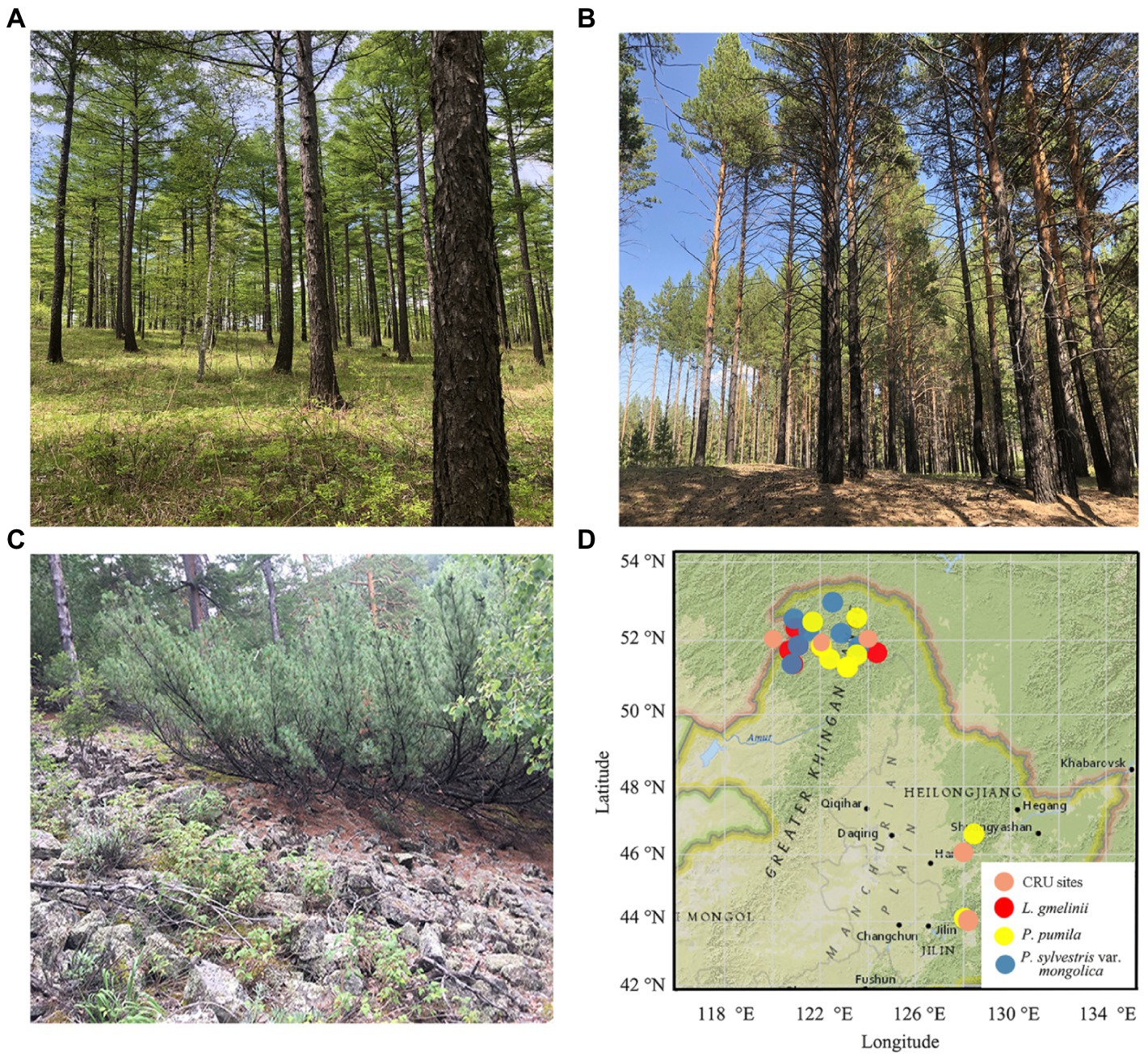
Figure 1. Field photos (A: Larix gmelinii; B: Pinus sylvestris var. Mongolica; C: Pinus pumila) and location map of tree–ring sampling sites (D) (red circle, Larix gmelinii; yellow circle, Pinus pumila; blue circle, Pinus sylvestris var. mongolica) in the northeast of China. Fieldwork was conducted from 2015 to 2018 at 26 sites.
The study area is mainly the boreal forest dominated by L. gmelinii and accompanied by P. sylvestris var. mongolica, Betula platyphylla, and Populus davidiana. Shrubs mainly include P. pumila, Rhododendron dauricum, Vaccinium vitis-idaea, Rosa dahurica, et al. The main herbs include Maianthemum bifolium, Sanguisorba officinalis, and Trientalis europaea, et al. The soil is brown coniferous forest soil. Pinus sylvestris var. mongolica and L. gmelinii are coniferous trees, and P. pumila is a creeping shrub with many trunks. Pinus pumila mainly grows in two vegetation types: they are high-altitude subalpine plants and low-altitude canopy trees. The former grows in the subalpine zone with fruticulose and herbaceous plants under its canopy. The latter grows under the canopy of arboreal trees (e.g., L. gmelinii, P. sylvestris var. mongolica, and Betula ermanii; Okuda et al., 2008). The subalpine krummholz forest dominated by P. pumila is mainly distributed in the altitude range of 900–1,500 m in the Daxing’an Mountains.
Tree-Ring Sampling and Chronology Development
At each site, 32–54 tree-ring cores were collected at breast height from healthy trees using a 5.15-mm-diameter increment borer. Two cores were collected from each tree. One disc including the entire stem cross section was collected from the base of P. pumila trunks with a handsaw. Discs were obtained only from the largest trunk of isolated, mature, healthy P. pumila individuals. A total of 576 cores from 126 L. gmelinii and 182 P. sylvestris var. mongolica and 133 discs from P. pumila were sampled at the 26 sites.
To remove the non-climate signals related to age or the effects of stand dynamics, each ring-width series were detrended and standardized by fitting a negative exponential curve or linear line using the ARSTAN program (Cook and Holmes, 1986). The tree-ring index was obtained by dividing the ring width by the fitted value for each ring. All detrended series were averaged to chronologies using the bi-weight robust mean (Cook and Holmes, 1986). The standard chronologies (STD) were used in the subsequent analyses.
Statistical analyses were used to compare chronologies among localities and species for the period 1950–2014. The expressed population signal (EPS), defined as the proportion of each series signal of the total series variance, was used to quantify the reliability of the chronology (Wigley et al., 1984). The mean sensitivity (MS) and the first-order autocorrelation (AC1) were calculated on the detrended individual index series and averaged to measure the year-to-year variability and how current-year growth was influenced by previous-year climatic factors. The mean series correlation between trees (Rbar) allowed us to evaluate the strength of the common growth signal over time. The standard deviation (SD) of inter-annual ring width variability was calculated as a proportion of mean ring-width. Variance in the first eigenvector (VF1) of all series identifies the common growth variability among all trees at each site. The signal-to-noise ratio (SNR) is a measure of the strength of the common high-frequency signal in the ring-width indexes of trees from the same site.
Climate Data
We used CRU TS 3.23 0.5° × 0.5° gridded monthly and seasonal temperature and precipitation data to analyze growth–climate relationships for the period 1950–2014 because no nearby weather stations exist. The data were extracted from the sample area using the KNMI Climate Explorer web page.1 The CRU database is formed by interpolated values from regional meteorological stations. In areas with a low density of weather stations CRU data contained inhomogeneities (McAfee et al., 2014), especially precipitation in alpine regions. Therefore, we verified the CRU precipitation data with the correlation between the observation data of the meteorological station and the CRU precipitation data. The monthly total precipitation (P), mean (Tmean), minimum (Tmin), and maximum temperature (Tmax) were used to analyze growth-climate relationships. Seasons were defined as: winter is from December of the previous year to February of the current year, spring as March–May, summer as June–August, and autumn as September–November.
Statistical Analyses
The Pearson correlation was used to determine the relationship between the tree-ring index and monthly and seasonal climate variables to determine the main climate factors that limit the radial growth of each species. Radial growth is affected by the current and previous year’s climate (Fritts, 1976). Therefore, climate variables over 12 months, from November of the previous year to October of the current year, were used for the correlation analysis. To investigate the temporal stability of growth–climate relationship, we carried out a moving 21-year window correlation analysis using DENDROCLIM2002 to analyze the temporal stability of dendroclimatic relations (Biondi and Waikul, 2004).
To analyze the radial growth of L. gmelinii, P. sylvestris var. mongolica, and P. pumila responses during and after drought events, resistance, resilience, and the relative resilience were calculated (Lloret et al., 2011). These drought events were selected based on the PDSI (Palmer Drought Severity Index). We used the resistance, recovery and resilience indices defined by Lloret et al. (2011) to quantify individual tree responses to the drought events.
Rt = Dr/PreDrRc = PostDr/DrRs = PostDr/PreDrRRS = ((PostDr – Dr)/PreDr)
where PreDr and PostDr indicate the mean ring width before and after three drought years, respectively; Dr indicates the ring width in the drought year.
A retrospective study of tree-ring widths allowed us to calculate resistance, resilience and recovery indices for three drought events: 1954, 1967, and 2008. We used the length of the growth recovery time (GRT) and total growth reduction that were put forward by Thurm et al. (2016) and Móricz et al. (2021). GRT represents the time (unit is the year) required to recover the predrought growth level again, including the drought years. TGR means the total growth reduction caused by the drought, and we calculated the TGR index, including drought year and the accumulated loss of growth during GRT.
Results
Comparison of the Chronological Characteristics in Different Species
Sampled L. gmelinii and P. sylvestris var. mongolica were older than P. pumila, XLLG had the highest SNR, and SLPS had the highest VF1 and Rbar (Supplementary Table S1). The AC1 of P. pumila and P. sylvestris var. mongolica was higher than that of L. gmelinii, while the MS of L. gmelinii was the highest among the three species (Figure 2). Statistics related to the common growth signal and the mean correlations among individuals within each site (Rbar, EPS, VF1, SNR, and SD) were usually higher for the trees than the P. pumila. All analyses indicated that the 26 chronologies were rich in climatic signals and suitable for analyzing growth–climate relationships.
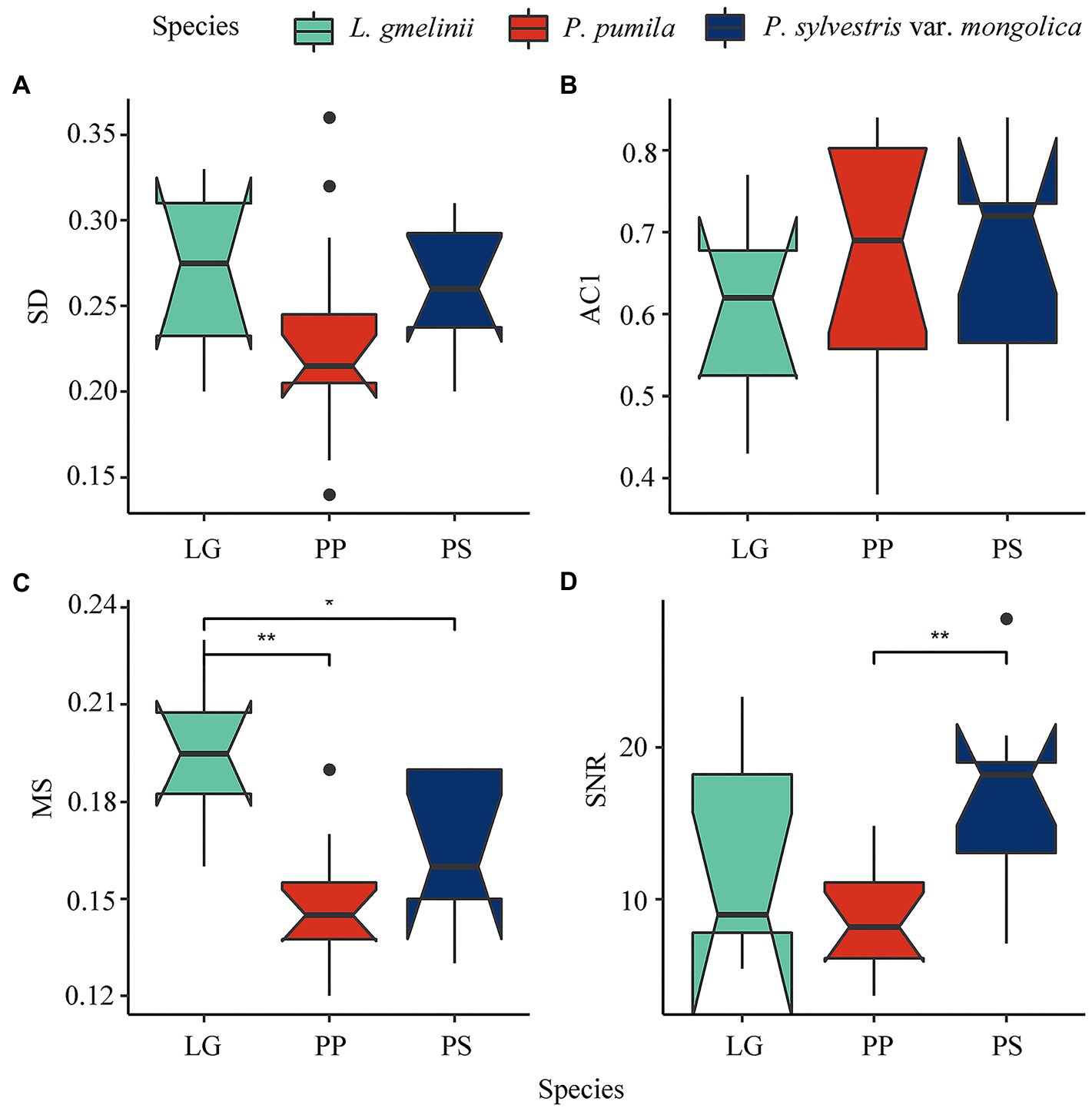
Figure 2. Boxplot of three species chronology main statistics (A: SD; B: ACI; C: MS; D: SNR) in this study, ** represent p < 0.01; * represent p < 0.01, those without * markings are not significant. SD, standard deviation; AC1, first order autocorrelation; MS, mean sensitivity; SNR, signal-to-noise ratio.
The ring-width chronology of P. pumila showed a lower Rbar for growth between individuals than tree species indicating that its radial growth is less consistent than that in P. sylvestris var. mongolica and L. gmelinii (Supplementary Table S1). There is a high degree of consistency between the chronologies of the same species (Supplementary Figure S1). In 1954, 1967, and 2008, the three species formed narrower rings (Figure 3 and Supplementary Figure S2). The chronological consistency of L. gmelinii was higher than that of P. sylvestris var. mongolica and P. pumila. The year 1986 was not considered a drought year for all three species because the ring-width index of L. gmelinii was greater than 1 (Figure 3).
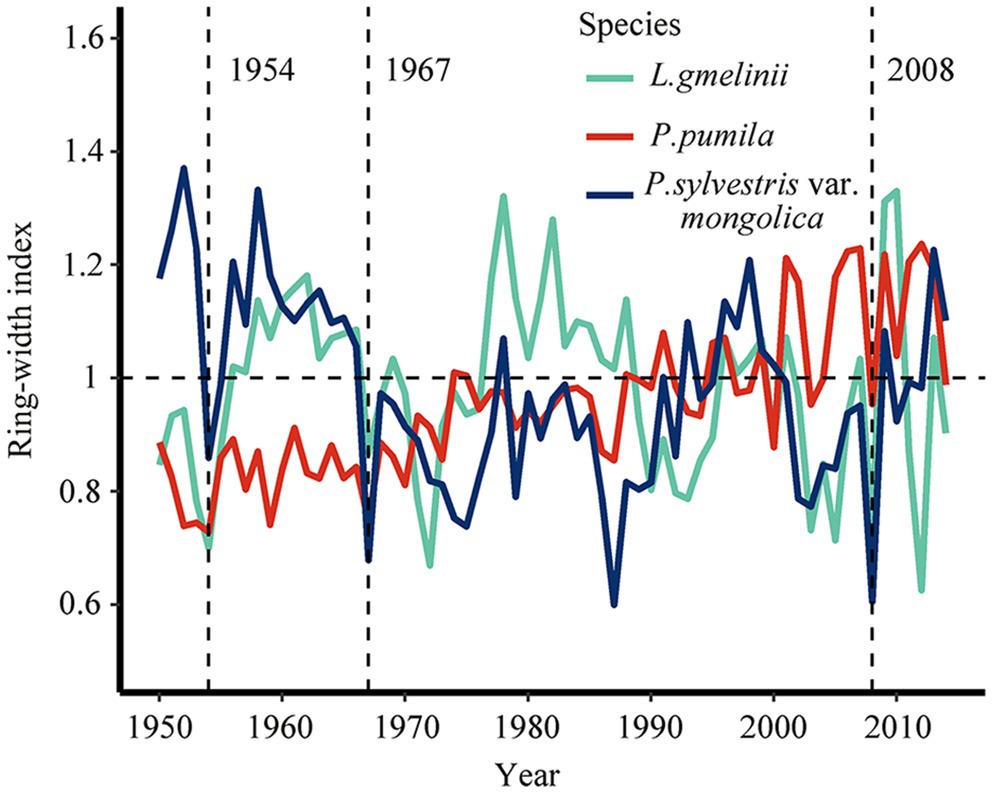
Figure 3. Changes in the ring-width index of Larix gmelinii (green line), Pinus pumila (red line), and Pinus sylvestris var. mongolica (blue line) in northeast China, the vertical dash lines represent drought years (1954, 1967, and 2008).
Growth–Climate Relationships of Different Species
The growth of L. gmelinii and P. sylvestris var. mongolica was negatively correlated with maximum temperature in the previous winter (Figure 4A and Supplementary Figures S3, S4). In contrast, high temperatures in the previous winter were related to increasing P. pumila ring widths (Supplementary Figures S5, S6). Warm spring and summer conditions were not conducive to the radial growth of L. gmelinii and P. sylvestris var. mongolica. However, P. pumila was positively correlated with minimum temperature in the current growing season (Figure 4B and Supplementary Figures S4, S5). Wet conditions in the previous winter enhanced the radial growth of L. gmelinii and P. sylvestris var. mongolica but had an inhibiting effect on the P. pumila (Figures 4C,D). The impact of winter precipitation on P. pumila radial growth was greater than for larch and pine. In addition, wet conditions (high PDSI) was more beneficial to the radial growth of larch and pine than P. pumila, which indicated that trees are more sensitive to moisture (Figure 4D and Supplementary Figures S3–S5).
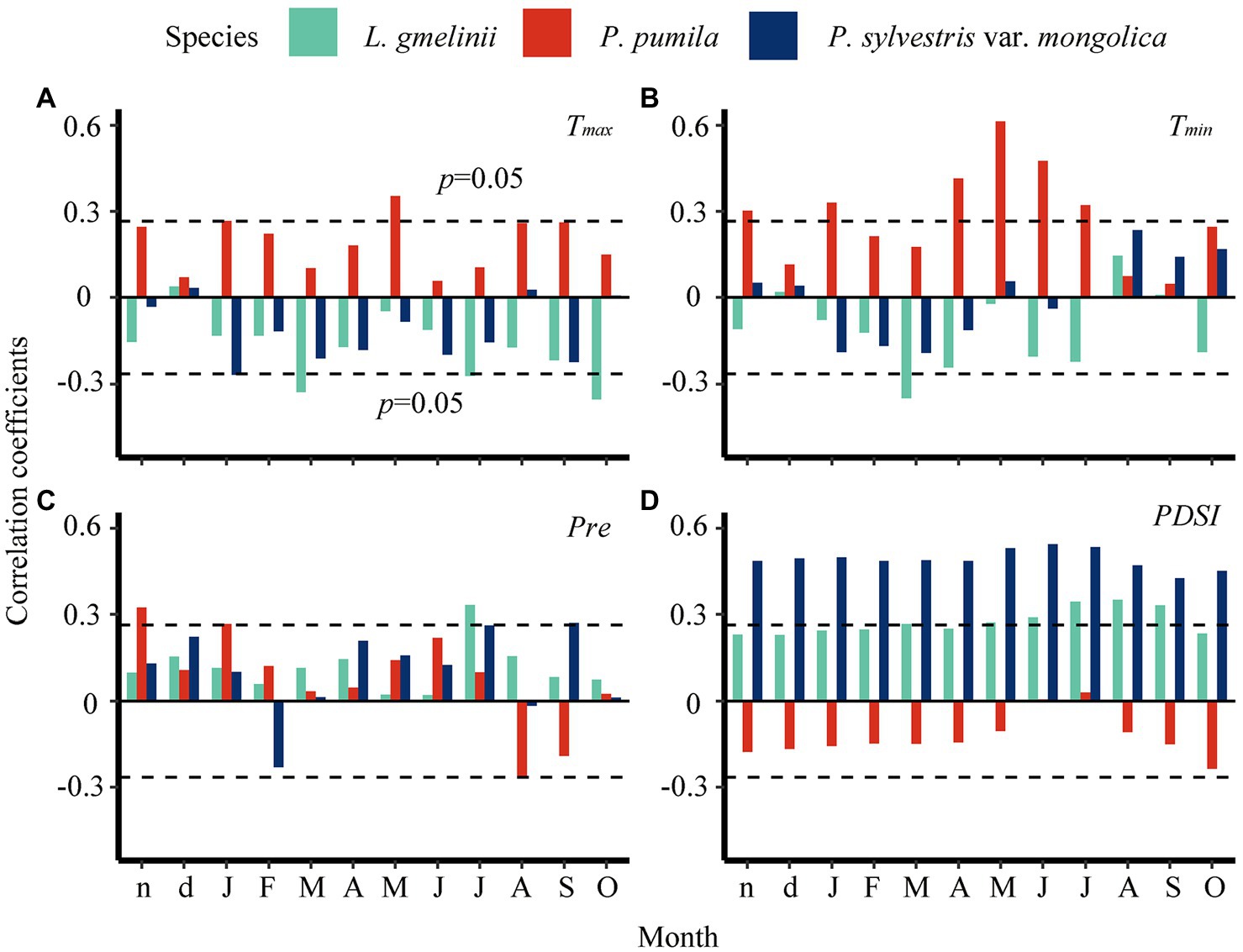
Figure 4. Pearson correlation of ring-width index with monthly climate data from previous November (lower case n) to current October (upper case O) during 1950–2014. Horizontal dashed lines indicate the 95% significance levels. Tmax–monthly maximum temperature (A), Tmin–monthly minimum temperature (B), Pre–monthly precipitation (C), PDSI–Palmer Drought Severity Index (D).
Moving 21-year window correlation analysis results indicated that P. sylvestris var. mongolica was positively correlated with PDSI, with a decreasing correlation between 1995 and 2005 and increasing in the last decade (Figure 5A and Supplementary Figure S7). However, the positive correlation between L. gmelinii and PDSI turned negative after 1990 and a positive correlation in the recent decade. Unlike the two arboreal conifers, the correlation between P. pumila and PDSI changed from negative to positive around 1980 and shifted to significant negative around 2000. Larch at low latitudes was negatively correlated with PDSI, and L. gmelinii and P. sylvestris var. mongolica at high latitudes were positively correlated with PDSI (Figure 5B). There was a negative correlation between PDSI and P. pumila at low latitudes and a positive correlation between PDSI and P. sylvestris var. mongolica and L. gmelinii at high latitudes.
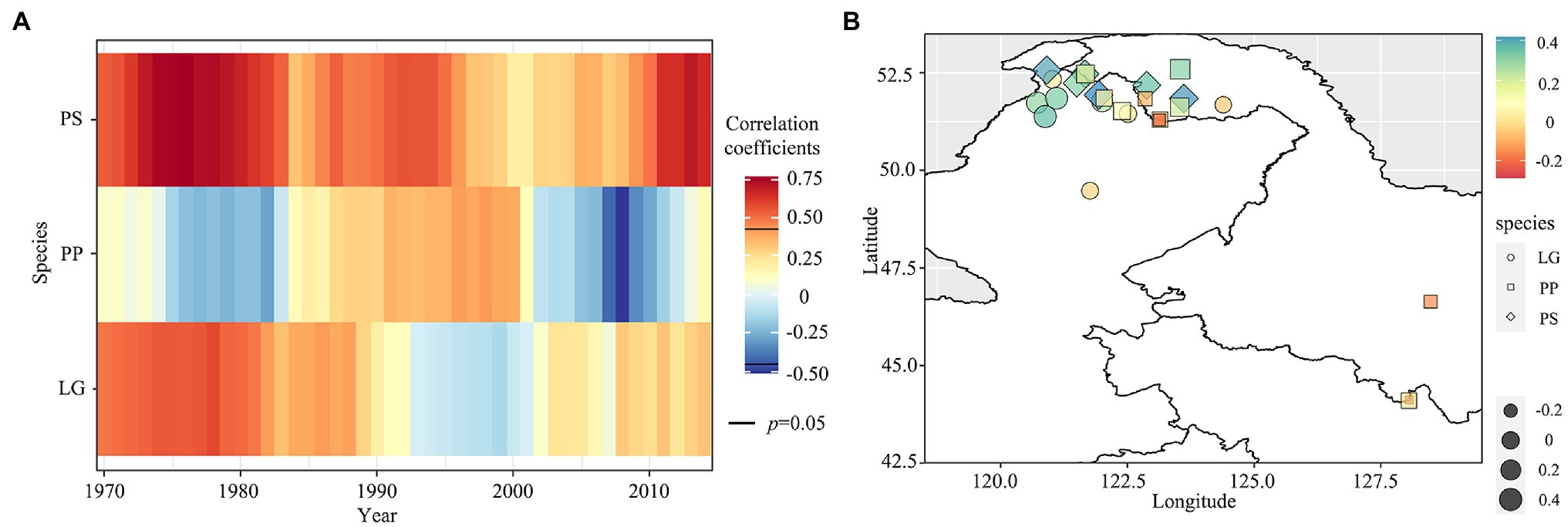
Figure 5. The 21-year moving correlation analysis between the ring-width index of three species and minimum PDSI from June to August during the period 1970–2014 (A); the person correlation between the ring-width index and minimum PDSI from June to August in different sites (B).
Resistance, Recovery Time, and Growth Changes of Different Species to Drought Events
The resistance of L. gmelinii and P. pumila was stronger than P. sylvestris var. mongolica (Figure 5), indicating that L. gmelinii and P. pumila have less growth loss than P. sylvestris var. mongolica during drought events (Figure 6). The strong recovery of P. sylvestris var. mongolica suggests that it could recover faster after drought in 1967 and 2008. In 2008, L. gmelinii showed the highest resilience, and in 1954, P. sylvestris var. mongolica showed the lowest resilience. In addition, L. gmelinii showed high relative resilience during the three drought events. Along the latitudinal gradient, P. pumila resistance increased and resilience decreased with decreasing latitude, while L. gmelinii and P. sylvestris var. mongolica showed the opposite trend (Supplementary Figure S9).
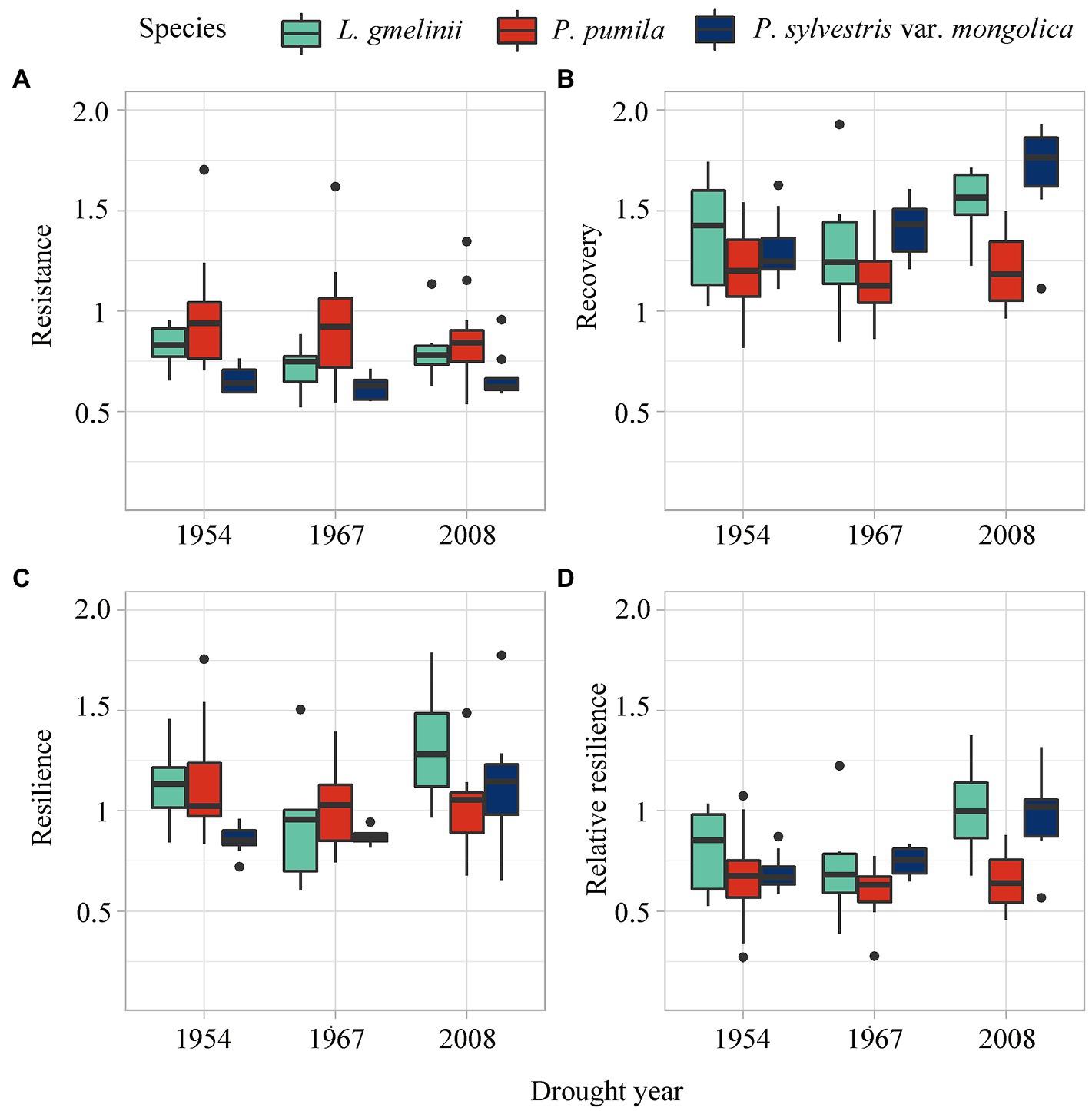
Figure 6. Resistance (A), recovery (B), resilience (C), and the relative resilience (D) of L. gmelinii, P. pumila and P. sylvestris var. mongolica response to three drought events.
In 1954 and 1967, P. sylvestris var. mongolica needed the longest recovery time; as a result, the P. sylvestris var. mongolica had the maximum total growth reduction during drought (Figure 7). In 2008, the growing loss of all three species was similar, while the recovery time of L. gmelinii was longer. In 1954 and 1967, P. pumila had the minimum growth reduction, and the recovery time of P. pumila was in the middle of the three drought events.
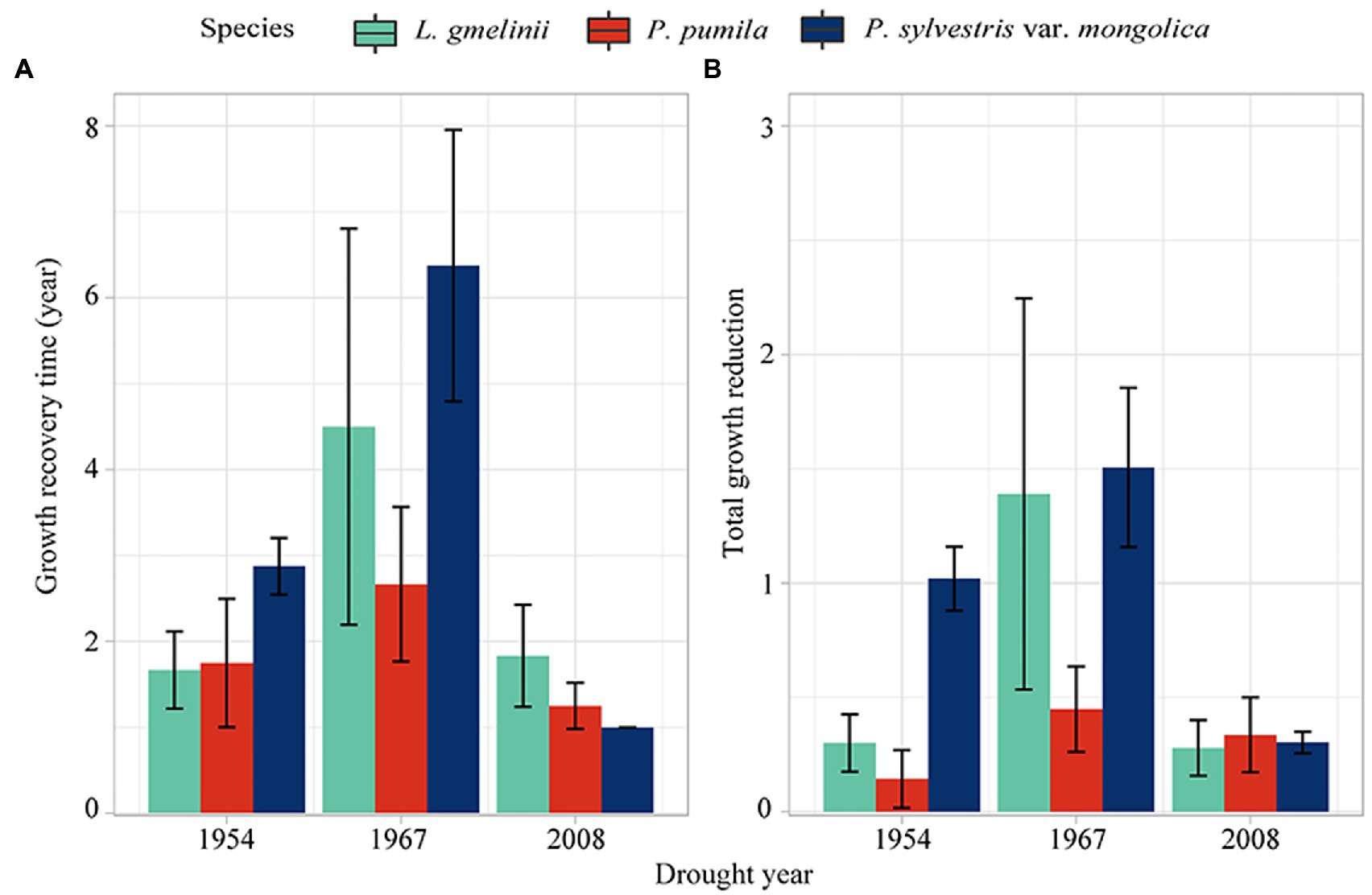
Figure 7. The growth recovery time (GRT) (A) and total growth reduction (TGR) (B) of L. gmelinii, P. pumila and P. sylvestris var. mongolica radial growth to drought events.
Discussion
Differences in the Responses of Three Species to Climate Change
Among the three species in boreal forests widely distributed in northeast China, the growth of P. pumila showed an upward trend, the growth of P. sylvestris var. mongolica showed a downward trend, and the growth of L. gmelinii was relatively stable. Pinus pumila is mainly distributed in treeline, while L. gmelinii and P. sylvestris var. mongolica are distributed in relatively lower altitudes. Thus, tree growth could be restricted by water deficits due to climate warming, while shrub growth is promoted by rising temperature (Miller et al., 2017; Harvey et al., 2020). Radial growth is a sensitive and closely tracked clue to climate change (Camarero et al., 2021a). Shrub and tree growth trends reveal differences in the response of different life form species to climate change, which has been reported in polar, alpine, and Mediterranean biological communities (Pellizzari et al., 2017; Treml et al., 2019; Lopez et al., 2021; Šenfeldr et al., 2021). Some studies have found that warming can promote shrub growth and expansion (Hallinger et al., 2010; Frost and Epstein, 2014). Physiological differences between trees and shrubs often lead to different strategies for growth response to climate change (Lyu et al., 2017; Zhirnova et al., 2020; Li et al., 2021). Trees respond more strongly to climate change in the macro-environment, and shrubs are more sensitive to microclimate near the ground because of their dwarfism (Pellizzari et al., 2017; Šenfeldr et al., 2021). During the growing season, trees are exposed to higher air and stem temperatures than shrubs, and the growing season of shrubs is shorter than that of trees (Gazol and Camarero, 2012). Although only one shrub of P. pumila was used in this study, it is representative in the timberline of northeast China and can represent the response of other timberline shrubs to some extent. Therefore, the growth of P. pumila was positively correlated with temperature, while that of L. gmelinii and P. sylvestris var. mongolica are the opposite. In addition to different phenological periods, the biomass allocation patterns of shrubs and trees are also different (Treml et al., 2019). The proportion of photosynthetic tissue in the stems of shrubs is larger than that of trees (Šenfeldr et al., 2021).
Larix gmelinii and P. sylvestris var. mongolica negatively correlate with spring and summer temperature (Figure 4), and this signal is more obvious in L. gmelinii. Precipitation has little effect on the radial growth of P. pumila. This is consistent with Šenfeldr et al. (2021)’s results. They found that precipitation is much less important for trees and shrubs growth than temperature. The two tree species have different defoliation patterns and different ways of dealing with climate change, among which P. sylvestris var. mongolica is more sensitive to water deficits. Defoliation results from plants adapting to seasonal stresses such as drought or low temperature (Sousa-Silva et al., 2018). As a comprehensive effect of temperature and precipitation, both L. gmelinii and P. sylvestris var. mongolica had a significant positive correlation with PDSI. Previous studies have found that L. gmelinii and P. sylvestris var. mongolica have similar growth–climate responses in Siberia (De Grandpré et al., 2011; Belokopytova et al., 2021).
The response of P. sylvestris var. mongolica growth to temperature rise is closely related to the increased early spring water availability. Drought in winter is a severe threat to the growth of cold-tolerant trees, with boreal trees in winter for up to 5 months annually. Therefore, if there is enough water in the early growing season, trees may be more able to withstand the stress caused by warming and even increase their growth (Zhang et al., 2019a). Because of fallen leaves, the growth of L. gmelinii seems to start later than that of P. sylvestris var. mongolica. Due to the rapid melting of snow in the early growing season, P. sylvestris var. mongolica benefits more from snowmelt than larch in various environments (Belokopytova et al., 2021). Deciduous traits enable L. gmelinii to maintain water in early spring by reducing transpiration loss, while P. sylvestris var. mongolica with evergreen needles needs sufficient water supply in the early growing season (Rossi et al., 2009; Li et al., 2021). The negative correlation between P. pumila growth and PDSI may be due to more precipitations and higher air humidity at higher altitudes.
Responses of Tree and Shrub Growth to Drought Events
Pinus pumila growth is less affected by drought, while trees are more vulnerable to extreme drought (Figure 6). Water stress caused by climate change has triggered a pervasive increase in large-scale tree diebacks and mortality events worldwide (Lewis et al., 2011; Anderegg et al., 2019; Camarero et al., 2021b). Tall trees need an effective long-distance transport channel to resist gravity and friction to transport water from soil to leaves (Choat et al., 2018). In contrast, shrubs require less water because of their low height, shorter paths, and lower soil water potential. In addition, larger tree crowns are more exposed to the canopy positions resulting in higher evaporation and reduced drought tolerance (Kunert et al., 2017; McGregor et al., 2021).
Among the three species, P. pumila has the highest resistance and the lowest recovery to drought. Compared with the growth of the two tree species, L. gmelinii had higher drought resistance, while P. sylvestris var. mongolica had higher drought recovery in 1967 and 2008. Zhang et al. (2021) reported that L. gmelinii experienced frequent extreme drought had lower drought resistance and higher resilience and was better adapted to extreme droughts. P. pumila is distributed at high altitudes and usually grows on steep slopes or shallow rocky soils. Dwarfism makes P. pumila drought resistant, but its habitat characteristics make it difficult to recover after drought. The differences in foliage habits (evergreen and deciduous) and strategies to deal with water deficit between P. sylvestris var. mongolica and L. gmelinii resulted in lower drought resistance and greater growth loss P. sylvestris var. mongolica (Belokopytova et al., 2021). Isohydric P. sylvestris immediately regulates transpiration through stomata closure to prevent massive xylem embolism, resulting in reduced photosynthetic rate and growth (Irvine et al., 1998). Conifers are usually more sensitive to stomatal regulation, but larch is less sensitive than pine (Dulamsuren et al., 2009; Khansaritoreh et al., 2018). Anisohydric larch maintains active transpiration to maintain a high photosynthetic rate rather than sacrificing needles and fine roots during severe droughts (Piper and Fajardo, 2014). Therefore, the growth loss of P. sylvestris var. mongolica was greater than that of L. gmelinii in drought events. In addition, besides the drought adaptation of root and stem xylem (Chenlemuge et al., 2013), reducing transpiration through needle abscission and stomatal closure also is an effective drought resistance strategy (Khansaritoreh et al., 2018). Li et al. (2021) found that L. gmelinii had a similar drought resistance mechanism. However, most plants die due to high hydraulic damage caused by drought rather than reduced carbohydrate storage (Anderegg et al., 2013; Sperry and Love, 2015; Gessler et al., 2017).
Droughts in the 21st century are likely to become more widespread, intense and persistent (Lévesque et al., 2013; Swenson et al., 2017; Stovall et al., 2019; Ault, 2020). Drought can lead to reduced tree growth and significantly increased mortality (Fang and Zhang, 2019). The recent increase in drought stress seems to have resulted in the transfer of the natural distribution of larch to high latitudes (Mamet et al., 2019). However, for some drought-tolerant shrubs, drought may put them in a favorable position in interspecific competition to replace trees at high altitudes and latitudes (Myers-Smith et al., 2015). Therefore, compared with trees, future warming may be conducive to the growth and distribution of shrubs. In addition, L. gmelinii and P. sylvestris var. mongolica may be replaced by Larix sibirica or fast-growing deciduous broadleaf trees in some areas (Kharuk et al., 2009; Mack et al., 2021). Due to drought, the shifts in forest structure may affect the carbon balance and provide positive/negative feedback for warming at the regional level.
Conclusion
Different species developed different growth strategies to cope with climate change. Water deficit was the dominant limiting factor for the radial growth of P. sylvestris var. mongolica; however, the effect on P. pumila was less. Future warming and drought will likely inhibit the growth of pine and larch. There was a negative correlation between PDSI and P. pumila at the low latitude and a positive correlation between PDSI and P. sylvestris var. mongolica and L. gmelinii at high latitude. The resistance of L. gmelinii and P. pumila was stronger than that of P. sylvestris var. mongolica and had less growth loss and shorter recovery times during the drought. Comparing differences in the resistance of shrubs and trees to drought events can help us better predict and understand the response of changing ecosystem dynamics to warming.
Data Availability Statement
The original contributions presented in the study are included in the article/Supplementary Material, further inquiries can be directed to the corresponding author.
Author Contributions
JY, QZ, and XW conceived the idea and contributed to the study design, discussed the results, and wrote the manuscript. JY, WS, and XZ performed data collection in the field and contributed to chronology data analysis. WS and XZ performed meteorological data collection and analysis. XW funded the study. All authors contributed to interpreting the results, discussion, and approved the final manuscript.
Funding
This work was supported by the Key Project of the China National Key Research and Development Program (2021YFD2200401), the National Natural Science Foundation of China (41877426), and the Fund of Eco-meteorological Innovation Open Laboratory in Northeast China, China Meteorological Bureau (stqx2018zd02).
Conflict of Interest
The authors declare that the research was conducted in the absence of any commercial or financial relationships that could be construed as a potential conflict of interest.
Publisher’s Note
All claims expressed in this article are solely those of the authors and do not necessarily represent those of their affiliated organizations, or those of the publisher, the editors and the reviewers. Any product that may be evaluated in this article, or claim that may be made by its manufacturer, is not guaranteed or endorsed by the publisher.
Acknowledgments
We thank all the Forest Department staff who helped in the field sampling.
Supplementary Material
The Supplementary Material for this article can be found online at: https://www.frontiersin.org/articles/10.3389/fpls.2022.912916/full#supplementary-material
Footnotes
References
Anderegg, W. R. L., Kane, J. M., and Anderegg, L. D. L. (2013). Consequences of widespread tree mortality triggered by drought and temperature stress. Nat. Clim. Chang. 3, 30–36. doi: 10.1038/nclimate1635
Anderegg, L. D. L., and HilleRisLambers, J. (2019). Local range boundaries vs. large‐scale trade‐offs: Climatic and competitive constraints on tree growth. Ecol. Lett. 22, 787–796. doi: 10.1111/ele.13236
Arellano, G., Medina, N. G., Tan, S., Mohamad, M., and Davies, S. J. (2019). Crown damage and the mortality of tropical trees. New Phytol. 221, 169–179. doi: 10.1111/nph.15381
Ault, T. R. (2020). On the essentials of drought in a changing climate. Science 368, 256–260. doi: 10.1126/science.aaz5492
Babst, F., Bouriaud, O., Poulter, B., Trouet, V., Girardin, M. P., and Frank, D. C. (2019). Twentieth century redistribution in climatic drivers of global tree growth. Sci. Adv. 5:eaat4313. doi: 10.1126/sciadv.aat4313
Bastos, A., Ciais, P., Friedlingstein, P., Sitch, S., Pongratz, J., Fan, L., et al. (2020). Direct and seasonal legacy effects of the 2018 heat wave and drought on European ecosystem productivity. Sci. Adv. 6:eaba2724. doi: 10.1126/sciadv.aba2724
Belokopytova, L. V., Meko, D. M., Zhirnova, D. F., Babushkina, E. A., and Vaganov, E. A. (2021). Spatial classification of moisture-sensitive pine and larch tree-ring chronologies within Khakass–Minusinsk depression, South Siberia. Trees 35, 2133–2139. doi: 10.1007/s00468-021-02196-7
Biondi, F., and Waikul, K. (2004). DENDROCLIM2002: a C++ program for statistical calibration of climate signals in tree–ring chronologies. Comput. Geosci. 30, 303–311. doi: 10.1016/j.cageo.2003.11.004
Bottero, A., Forrester, D. I., Cailleret, M., Kohnle, U., Gessler, A., Michel, D., et al. (2021). Growth resistance and resilience of mixed silver fir and Norway spruce forests in Central Europe: contrasting responses to mild and severe droughts. Glob. Chang. Biol. 27, 4403–4419. doi: 10.1111/gcb.15737
Camarero, J. J., Gazol, A., Sánchez-Salguero, R., Fajardo, A., McIntire, E. J. B., Gutiérrez, E., et al. (2021a). Global fading of the temperature–growth coupling at alpine and polar treelines. Glob. Chang. Biol. 27, 1879–1889. doi: 10.1111/gcb.15530
Camarero, J. J., Gazol, A., Sangüesa-Barreda, G., Vergarechea, M., Alfaro-Sánchez, R., Cattaneo, N., et al. (2021b). Tree growth is more limited by drought in rear–edge forests most of the times. For. Ecosyst. 8, 1–15. doi: 10.1186/s40663-021-00303-1
Chenlemuge, T., Hertel, D., Dulamsuren, C., Khishigjargal, M., Leuschner, C., and Hauck, M. (2013). Extremely low fine root biomass in Larix sibirica forests at the southern drought limit of the boreal forest. Flora 208, 488–496. doi: 10.1016/j.flora.2013.08.002
Choat, B., Brodribb, T. J., Brodersen, C. R., Duursma, R. K., López, R., and Medlyn, B. E. (2018). Triggers of tree mortality under drought. Nature 558, 531–539. doi: 10.1038/s41586-018-0240-x
Cook, E. R., and Holmes, R. L. (1986). User’s Manual for program ARSTAN. Tucson: Laboratory of Tree–Ring Research University of Arizona.
De Grandpré, L., Tardif, J. C., Hessl, A., Pederson, N., Conciatori, F. R., Green, T., et al. (2011). Seasonal shift in the climate responses of Pinus sibirica, Pinus sylvestris, and Larix sibirica trees from semi–arid, north–Central Mongolia. Can. J. For. Res. 41, 1242–1255. doi: 10.1139/x11-051
Duan, C. Y., Li, M. Y., Fang, L. D., Cao, Y., Wu, D. D., Liu, H., et al. (2022). Greater hydraulic safety contributes to higher growth resilience to drought across seven pine species in a semi–arid environment. Tree Physiol. 42, 727–739. doi: 10.1093/treephys/tpab137
Dulamsuren, C., Hauck, M., Bader, M., Osokhjargal, D., Oyungerel, S., Nyambayar, S., et al. (2009). Water relations and photosynthetic performance in Larix sibirica growing in the forest–steppe ecotone of northern Mongolia. Tree Physiol. 29, 99–110. doi: 10.1093/treephys/tpn008
Fang, O., and Zhang, Q. B. (2019). Tree resilience to drought increases in the Tibetan plateau. Glob. Chang. Biol. 25, 245–253. doi: 10.1111/gcb.14470
Fang, J., Zhu, J., and Shi, Y. (2017). The responses of ecosystems to global warming. Chin. Sci. Bull. 63, 136–140. doi: 10.1360/N972017-00916
Férriz, M., Martin-Benito, D., Cañellas, I., and Gea-Izquierdo, G. (2021). Sensitivity to water stress drives differential decline and mortality dynamics of three co–occurring conifers with different drought tolerance. For. Ecol. Manag. 486:118964. doi: 10.1016/j.foreco.2021.118964
Forbes, B. C., Fauria, M. M., and Zetterberg, P. (2010). Russian Arctic warming and ‘greening’ are closely tracked by tundra shrub willows. Glob. Chang. Biol. 16, 1542–1554. doi: 10.1111/j.1365-2486.2009.02047.x
Frost, G. V., and Epstein, H. E. (2014). Tall shrub and tree expansion in Siberian tundra ecotones since the 1960s. Glob. Chang. Biol. 20, 1264–1277. doi: 10.1111/gcb.12406
Gauthier, S., Bernier, P., Kuuluvainen, T., Shvidenko, A. Z., and Schepaschenko, D. G. (2015). Boreal forest health and global change. Science 349, 819–822. doi: 10.1126/science.aaa9092
Gazol, A., and Camarero, J. J. (2012). Mediterranean dwarf shrubs and coexisting trees present different radial–growth synchronies and responses to climate. Plant Ecol. 213, 1687–1698. doi: 10.1007/s11258-012-0124-3
Gazol, A., Camarero, J. J., Anderegg, W. R. L., and Vicente-Serrano, S. M. (2017). Impacts of droughts on the growth resilience of northern hemisphere forests. Glob. Ecol. Biogeogr. 26, 166–176. doi: 10.1111/geb.12526
Gazol, A., Camarero, J. J., Vicente-Serrano, S. M., Sánche-Salguero, R., Gutiérrez, E., de Luis, M., et al. (2018). Forest resilience to drought varies across biomes. Glob. Chang. Biol. 24, 2143–2158. doi: 10.1111/gcb.14082
Gessler, A., Schaub, M., and McDowell, N. G. (2017). The role of nutrients in drought–induced tree mortality and recovery. New Phytol. 214, 513–520. doi: 10.1111/nph.14340
Götmark, F., Götmark, E., and Jensen, A. M. (2016). Why be a shrub? A basic model and hypotheses for the adaptive values of a common growth form. Front. Plant Sci. 7:1095. doi: 10.3389/fpls.2016.01095
Hallinger, M., Manthey, M., and Wilmking, M. (2010). Establishing a missing link: warm summers and winter snow cover promote shrub expansion into alpine tundra in Scandinavia. New Phytol. 186, 890–899. doi: 10.1111/j.1469-8137.2010.03223.x
Harvey, J. E., Smiljanić, M., Scharnweber, T., Buras, A., Cedro, A., Cruz-García, R., et al. (2020). Tree growth influenced by warming winter climate and summer moisture availability in northern temperate forests. Glob. Chang. Biol. 26, 2505–2518. doi: 10.1111/gcb.14966
Helbig, M., Pappas, C., and Sonnentag, O. (2016). Permafrost thaw and wildfire: equally important drivers of boreal tree cover changes in the Taiga Plains, Canada. Geophys. Res. Lett. 43, 1598–1606. doi: 10.1002/2015GL067193
IPCC (2021) “Climate Change 2021: the Physical Science Basis.” Contribution of Working Group I to the Sixth Assessment Report of the Intergovernmental Panel on Climate Change. Cambridge Press, Cambridge, UK.
Iqbal, S., Zha, T., Jia, X., Hayat, M., Qian, D., Bourque, C. P. A., et al. (2021). Interannual variation in sap flow response in three xeric shrub species to periodic drought. Agric. For. Meteorol. 297:108276. doi: 10.1016/j.agrformet.2020.108276
Irvine, J., Perks, M. P., Magnani, F., and Grace, J. (1998). The response of Pinus sylvestris to drought: stomatal control of transpiration and hydraulic conductance. Tree Physiol. 18, 393–402. doi: 10.1093/treephys/18.6.393
Jiang, P., Liu, H., Piao, S., Ciais, P., Wu, X., Yin, Y., et al. (2019). Enhanced growth after extreme wetness compensates for post–drought carbon loss in dry forests. Nat. Commun. 10:195. doi: 10.1038/s41467-018-08229-z
Kannenberg, S. A., Schwalm, C. R., and Anderegg, W. R. L. (2020). Ghosts of the past: how drought legacy effects shape forest functioning and carbon cycling. Ecol. Lett. 23, 891–901. doi: 10.1111/ele.13485
Khansaritoreh, E., Schuldt, B., and Dulamsuren, C. (2018). Hydraulic traits and tree–ring width in Larix sibirica Ledeb. as affected by summer drought and forest fragmentation in the Mongolian forest steppe. Ann. For. Sci. 75, 1–12. doi: 10.1007/s13595-018-0701-2
Kharuk, V. I., Ranson, K. J., Im, S. T., and Dvinskaya, M. L. (2009). Response of Pinus sibirica and Larix sibirica to climate change in southern Siberian alpine forest–tundra ecotone. Scand. J. For. Res. 24, 130–139. doi: 10.1080/02827580902845823
Kunert, N., Aparecido, L. M. T., Wolff, S., Higuchi, N., dos Santos, J., de Araujo, A. C., et al. (2017). A revised hydrological model for the Central Amazon: the importance of emergent canopy trees in the forest water budget. Agric. For. Meteorol. 239, 47–57. doi: 10.1016/j.agrformet.2017.03.002
Lévesque, M., Saurer, M., Siegwolf, R., Eilmann, B., Brang, P., Bugmann, H., et al. (2013). Drought response of five conifer species under contrasting water availability suggests high vulnerability of Norway spruce and European larch. Glob. Chang. Biol. 19, 3184–3199. doi: 10.1111/gcb.12268
Lewis, S. L., Brando, P. M., Phillips, O. L., Heijden, G. M. F., and Nepstad, D. (2011). The 2010 amazon drought. Science 331:554. doi: 10.1126/science.1200807
Li, W., Jiang, Y., Dong, M., Du, E., Wu, F., and Zhao, S. (2021). Species–specific growth–climate responses of Dahurian larch (Larix gmelinii) and Mongolian pine (Pinus sylvestris var. mongolica) in the greater Khingan range, Northeast China. Dendrochronologia 65:125803. doi: 10.1016/j.dendro.2020.125803
Li, X., Piao, S., Wang, K., Wang, X., Wang, T., Ciais, P., et al. (2020). Temporal trade–off between gymnosperm resistance and resilience increases forest sensitivity to extreme drought. Nat. Ecol. Evol. 4, 1075–1083. doi: 10.1038/s41559-020-1217-3
Lloret, F., Keeling, E. G., and Sala, A. (2011). Components of tree resilience: effects of successive low-growth episodes in old ponderosa pine forests. Oikos 120, 1909–1920. doi: 10.1111/j.1600-0706.2011.19372.x
Lopez, J. G., Tor-Ngern, P., Oren, R., Kozii, N., Laudon, H., and Hasselquist, N. J. (2021). How tree species, tree size, and topographical location influenced tree transpiration in northern boreal forests during the historic 2018 drought. Glob. Chang. Biol. 27, 3066–3078. doi: 10.1111/gcb.15601
Lyu, S., Wang, X., Zhang, Y., and Li, Z. (2017). Different responses of Korean pine (Pinus koraiensis) and Mongolia oak (Quercus mongolica) growth to recent climate warming in Northeast China. Dendrochronologia 45, 113–122. doi: 10.1016/j.dendro.2017.08.002
Mack, M. C., Walker, X. J., Johnstone, J. F., Alexander, H. D., Melvin, A. M., Jean, M., et al. (2021). Carbon loss from boreal forest wildfires offset by increased dominance of deciduous trees. Science 372, 280–283. doi: 10.1126/science.abf3903
Mamet, S. D., Brown, C. D., Trant, A. J., and Laroque, C. P. (2019). Shifting global Larix distributions: northern expansion and southern retraction as species respond to changing climate. J. Biogeogr. 46, 30–44. doi: 10.1111/jbi.13465
Mayor, J. R., Sanders, N. J., Classen, A. T., Bardgett, R. D., Clément, J. C., Fajardo, A., et al. (2017). Elevation alters ecosystem properties across temperate treelines globally. Nature 542, 91–95. doi: 10.1038/nature21027
McAfee, S. A., Guentchev, G., and Eischeid, J. (2014). Reconciling precipitation trends in Alaska: 2. Gridded data analyses. J. Geophys. Res. Atmos. 119, 13820–13837. doi: 10.1002/2014JD022461
McDowell, N. G., Allen, C. D., Anderson-Teixeira, K., Aukema, B. H., BondLamberty, B., Chini, L., et al. (2020). Pervasive shifts in forest dynamics in a changing world. Science 368, 1–10. doi: 10.1126/science.aaz9463
McGregor, I. R., Helcoski, R., Kunert, N., Tepley, A. J., Gonzalez-Akre, E. B., Herrmann, V., et al. (2021). Tree height and leaf drought tolerance traits shape growth responses across droughts in a temperate broadleaf forest. New Phytol. 231, 601–616. doi: 10.1111/nph.16996
Mérian, P., and Lebourgeois, F. (2011). Size–mediated climate–growth relationships in temperate forests: a multi–species analysis. For. Ecol. Manag. 261, 1382–1391. doi: 10.1016/j.foreco.2011.01.019
Miller, A. E., Wilson, T. L., Sherriff, R. L., and Walton, J. (2017). Warming drives a front of white spruce establishment near western treeline, Alaska. Glob. Chang. Biol. 23, 5509–5522. doi: 10.1111/gcb.13814
Morales, A. I. G. C., Mendoza, J. M. O., Gozalbo, M. E., and Martínez, J. J. C. (2012). Arboreal and prostrate conifers coexisting in Mediterranean high mountains differ in their climatic responses. Dendrochronologia 30, 279–286. doi: 10.1016/j.dendro.2012.02.004
Móricz, N., Illés, G., Mészáros, I., Garamszegi, B., Berki, I., Bakacsi, Z., et al. (2021). Different drought sensitivity traits of young sessile oak (Quercus petraea (Matt.) Liebl.) and Turkey oak (Quercus cerris L.) stands along a precipitation gradient in Hungary. For. Ecol. Manag. 492:119165. doi: 10.1016/j.foreco.2021.119165
Myers-Smith, I. H., Elmendorf, S. C., Beck, P. S., Wilmking, M., Hallinger, M., Blok, D., et al. (2015). Climate sensitivity of shrub growth across the tundra biome. Nat. Clim. Chang. 5, 887–891. doi: 10.1038/nclimate2697
Oberhuber, W., Kofler, W., Schuster, R., and Wieser, G. (2014). Environmental effects on stem water deficit in co–occurring conifers exposed to soil dryness. Int. J. Biometeorol. 59, 417–426. doi: 10.1007/s00484-014-0853-1
Ogle, K., Barron-Gaffordet, G. A., Bentlet, L. P., Young, J. M., Huxman, T. E., Loik, M. E., et al. (2015). Quantifying ecological memory in plant and ecosystem processes. Ecol. Lett. 18, 221–235. doi: 10.1111/ele.12399
Okuda, M., Sumida, A., Ishii, H., Vetrova, V. P., and Hara, T. (2008). Establishment and growth pattern of Pinus pumila under a forest canopy in Central Kamchatka. Ecol. Res. 23, 831–840. doi: 10.1007/s11284-007-0445-1
Pellizzari, E., Camarero, J. J., Gazol, A., Granda, E., Shetti, R., Wilmking, M., et al. (2017). Diverging shrub and tree growth from the polar to the Mediterranean biomes across the European continent. Glob. Chang. Biol. 23, 3169–3180. doi: 10.1111/gcb.13577
Piper, F. I., and Fajardo, A. (2014). Foliar habit, tolerance to defoliation and their link to carbon and nitrogen storage. J. Ecol. 102, 1101–1111. doi: 10.1111/1365-2745.12284
Rossi, S., Rathgeber, C. B. K., and Deslauriers, A. (2009). Comparing needle and shoot phenology with xylem development on three conifer species in Italy. Ann. For. Sci. 66, 206–208. doi: 10.1051/forest/2008088
Seidl, R., Thom, D., Kautz, M., Martin-Benito, D., Peltoniemi, M., Vacchiano, G., et al. (2017). Forest disturbances under climate change. Nat. Clim. Chang. 7, 395–402. doi: 10.1038/nclimate3303
Senf, C., Buras, A., Zang, C. S., Rammig, A., and Seidl, R. (2020). Excess forest mortality is consistently linked to drought across Europe. Nat. Commun. 11:6200. doi: 10.1038/s41467-020-19924-1
Šenfeldr, M., Kaczka, R., Buras, A., Samusevich, A., Herrmann, C., Spyt, B., et al. (2021). Diverging growth performance of co–occurring trees (Picea abies) and shrubs (Pinus mugo) at the treeline ecotone of central European mountain ranges. Agric. For. Meteorol. 308–309:108608. doi: 10.1016/j.agrformet.2021.108608
Shetti, R. (2018). Potential of shrubs in the evolving field of arctic and alpine dendroecology: methods in shrub dendro-ecology, understanding the processes influencing shrub growth in the arctic and alpine ecosystems. PhD Thesis. University of Greifswald, Greifswald, Germany.
Sousa-Silva, R., Verheyen, K., Ponette, Q., Bay, E., Sioen, G., Titeux, H., et al. (2018). Tree diversity mitigates defoliation after a drought–induced tipping point. Glob. Chang. Biol. 24, 4304–4315. doi: 10.1111/gcb.14326
Sperry, J. S., and Love, D. M. (2015). What plant hydraulics can tell us about responses to climate–change droughts. New Phytol. 207, 14–27. doi: 10.1111/nph.13354
Stovall, A. E. L., Shugart, H., and Yang, X. (2019). Tree height explains mortality risk during an intense drought. Nat. Commun. 10:4385. doi: 10.1038/s41467-019-12380-6
Sturm, J., Santos, M. J., Schmid, B., and Damm, A. (2022). Satellite data reveal differential responses of Swiss forests to unprecedented 2018 drought. Glob. Chang. Biol. 28, 2956–2978. doi: 10.1111/gcb.16136
Sullivan, P. F., Brownlee, A. H., Ellison, S. B. Z., and Cahoon, S. M. P. (2021). Comparative drought sensitivity of co-occurring white spruce and paper birch in interior Alaska. J. Ecol. 109, 2448–2460. doi: 10.1111/1365-2745.13654
Swenson, N. G., Iida, Y., Howe, R., Wolf, A., Umaña, M. N., Petprakob, K., et al. (2017). Tree co–occurrence and transcriptomic response to drought. Nat. Commun. 8:1996. doi: 10.1038/s41467-017-02034-w
Tape, K. D., Sturm, M., and Racine, C. H. (2006). The evidence for shrub expansion in northern Alaska and the Pan-Arctic. Glob. Chang. Biol. 12, 686–702. doi: 10.1111/j.1365-2486.2006.01128.x
Thurm, E. A., Uhl, E., and Pretzsch, H. (2016). Mixture reduces climate sensitivity of Douglas–fir stem growth. For. Ecol. Manag. 376, 205–220. doi: 10.1016/j.foreco.2016.06.020
Treml, V., Hejda, T., and Kašpa, R. J. (2019). Differences in growth between shrubs and trees: how does the stature of woody plants influence their ability to thrive in cold regions? Agric. For. Meteorol. 271, 54–63. doi: 10.1016/j.agrformet.2019.02.036
Urban, J., Rubtsov, A. V., Urban, A. V., Shashkin, A. V., and Benkova, V. E. (2019). Canopy transpiration of a Larix sibirica and Pinus sylvestris forest in Central Siberia. Agric. For. Meteorol. 271, 64–72. doi: 10.1016/j.agrformet.2019.02.038
Wigley, T. M., Briffa, K. R., and Jones, P. D. (1984). On the average value of correlated time series, with applications in dendroclimatology and hydrometeorology. J. Clim. Appl. Meteorol. 23, 201–213. doi: 10.1175/1520-0450(1984)023<0201:OTAVOC>2.0.CO;2
Wu, X., Liu, H., Li, X., Ciais, P., Flurin, B., Guo, W., et al. (2018). Differentiating drought legacy effects on vegetation growth over the temperate northern hemisphere. Glob. Chang. Biol. 24, 504–516. doi: 10.1111/gcb.13920
Yang, J., Cooper, D. J., Li, Z., Song, W., Zhang, Y., Zhao, B., et al. (2020). Differences in tree and shrub growth responses to climate change in a boreal forest in China. Dendrochronologia 63, 125744–125710. doi: 10.1016/j.dendro.2020.125744
Zhang, X., Bai, X., Hou, M., Chen, Z., and Manzanedo, R. D. (2019a). Warmer winter ground temperatures trigger rapid growth of Dahurian larch in the permafrost forests of Northeast China. J. Geophys. Res. Biogeosci. 124, 1088–1097. doi: 10.1029/2018JG004882
Zhang, X., Li, X., Manzanedo, R. D., D’Orangeville, L., Lv, P., Wang, C., et al. (2021). High risk of growth cessation of planted larch under extreme drought. Environ. Res. Lett. 16:014040. doi: 10.1088/1748-9326/abd214
Zhang, X., Manzanedo, R. D., D’Orangeville, L., Rademacher, T. T., Li, J., Bai, X., et al. (2019b). Snowmelt and early to mid-growing season water availability augment tree growth during rapid warming in southern Asian boreal forests. Glob. Chang. Biol. 25, 3462–3471. doi: 10.1111/gcb.14749
Zhirnova, D. F., Babushkina, E. A., Belokopytova, L. V., and Vaganov, E. A. (2020). To which side are the scales swinging? Growth stability of Siberian larch under permanent moisture deficit with periodic droughts. For. Ecol. Manag. 459, 117841–117810. doi: 10.1016/j.foreco.2019.117841
Keywords: climate change, drought, resistance, tree rings, shrub
Citation: Yang J, Zhang Q, Song W, Zhang X and Wang X (2022) Radial Growth of Trees Rather Than Shrubs in Boreal Forests Is Inhibited by Drought. Front. Plant Sci. 13:912916. doi: 10.3389/fpls.2022.912916
Edited by:
Ze-Xin Fan, Xishuangbanna Tropical Botanical Garden (CAS), ChinaReviewed by:
Feng Chen, Yunnan University, ChinaHonghui Wu, Institute of Agricultural Resources and Regional Planning (CAAS), China
Copyright © 2022 Yang, Zhang, Song, Zhang and Wang. This is an open-access article distributed under the terms of the Creative Commons Attribution License (CC BY). The use, distribution or reproduction in other forums is permitted, provided the original author(s) and the copyright owner(s) are credited and that the original publication in this journal is cited, in accordance with accepted academic practice. No use, distribution or reproduction is permitted which does not comply with these terms.
*Correspondence: Xiaochun Wang, wangx@nefu.edu.cn