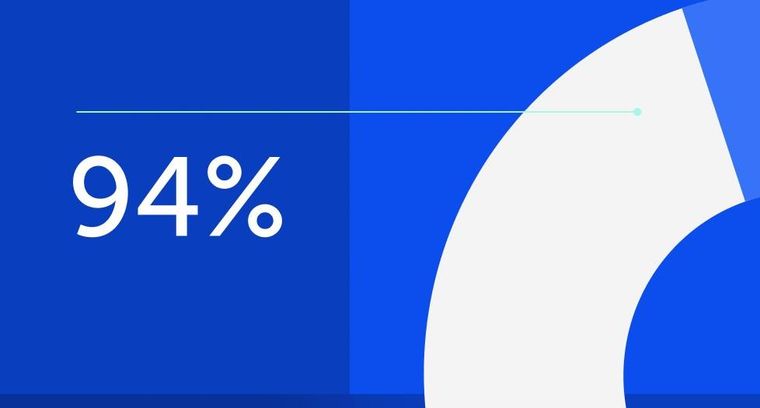
94% of researchers rate our articles as excellent or good
Learn more about the work of our research integrity team to safeguard the quality of each article we publish.
Find out more
ORIGINAL RESEARCH article
Front. Plant Sci., 30 June 2022
Sec. Plant Biotechnology
Volume 13 - 2022 | https://doi.org/10.3389/fpls.2022.911203
Actinidia arguta Sieb.Zucc. is a fruit that is rich in flavonoids. Nevertheless, details of flavonoid formation and the potential mechanism behind flavonoid biosynthesis have not previously been reported. In order to explore the biosynthetic regulation mechanism of flavonoids in A. arguta Sieb.Zucc., we conducted a combination of extensive targeted metabolite analysis and analyzed transcriptomes to determine the flavonoids present and the genes bound up with flavonoid biosynthesis in the two main cultivated varieties of A. arguta Sieb.Zucc. in Northern China. The maturity period is from August to September. A total of 118 flavonoids were found in fruits. Among them, 39 flavonoids were accumulated at significant levels after fruit ripening. Transcriptome analysis indicated that most flavonoid biosynthesis structural genes and certain regulatory genes exhibited differential expression between the two varieties. Correlation analysis of transcriptome and metabolite profiles showed that the ways of expression of 21 differentially expressed genes related to structure and regulation between the 2 varieties were more highly correlated with 7 flavonoids after fruit ripening. These results contribute to the development of A. arguta Sieb.Zucc. as a food and drug homologous functional food.
Transcriptome and widely targeted metabolomics were used to analyze the biosynthesis mechanism of flavonoids in Actinidia arguta Sieb.Zucc., so as to provide valuable information for its development as a food and drug homologous functional food.
Actinidia arguta Sieb.Zucc. commonly known as soft jujube kiwifruit, macaque pear, or rattan melon, is a perennial plant in the Actinidiaceae family. It is distributed in Jilin, Heilongjiang, Liaoning, and Shandong Provinces in North and Northwest China, and matures from June to August each year. It’s one of the famous and economically important wild fruits in the Changbai Mountain Area of China. Due to its green color, juiciness, and delicately sweet and sour flavor, it is known as “the precious fruit of the world” (Zuo et al., 2012; Hãdãrugã et al., 2016). A. arguta Sieb.Zucc. is nutritionally rich and contains a variety of functional components, such as amino acids, vitamins, and flavonoids. It is an excellent raw material for the growth of functional health foods (Latocha et al., 2014; Pal et al., 2015; Almeida et al., 2018). Flavonoids are one of the main active components of A. arguta Sieb.Zucc. grown in Changbai Mountain. They have anti-oxidation and anti-viral functions. In addition to preventing cardiovascular disease, cerebrovascular disease, and hyperuricemia, they contribute to liver protection and immunity (Latocha et al., 2013; Hu et al., 2016; Jiang et al., 2020). A. arguta Sieb.Zucc. has broad application prospects for the development of flavonoid products in the field of medicine and food homology. However, the key compounds present in the fruit have not been systematically analyzed.
Thus far, 10 flavonoids have been detected in A. arguta Sieb.Zucc. leaves, including quercetin, isorhamnetin, and kaempferol. Furthermore, a total of 70,631 unigenes have been identified in this plant from transcriptome data, which, in fruits and leaves, includes 29,617 up-regulated and 2976 down-regulated genes. Key genes, including AaF3′5′H-1H and AaF3H-1, have higher levels of expression in the leaves than in other tissues (Tan et al., 2021). The fruits of two A. arguta Sieb.Zucc. varieties were RNA sequenced (RNA-Seq), and indicated that leucoanthocyanidin dioxygenase (LDOX) may be the key gene regulating anthocyanin synthesis in kiwifruit pulp of the red-fruit variety “tianyuanhong” (Li et al., 2018). In addition, comparing homologous genes in Arabidopsis thaliana indicated that the majority of structural genes bound up with flavonoid biosynthesis in bitter mustard have been appraised, and the functions of some have been verified (Gupta et al., 2011; Park et al., 2011; Li C. et al., 2012; Li C. L. et al., 2012; Li X. et al., 2012; Yao et al., 2016; Zhang et al., 2017). FtMYB1 and FtMYB2 belong to the R2R3 MYB family and share high homology with AtMYB123/TT2. They actively mediate proanthocyanidin accumulation in transgenic tobacco by affecting the expression of genes bound up with flavonoid structure, including PAL, CHI, FLS, F3H, DFR, and ANS (Bai et al., 2014). Similarly, FtMYB15 and FtWD10 also have a positive effect on anthocyanin and procyanidin biosynthesis by enhancing the expression of flavonoid biosynthesis genes in early and late tobacco and tartary buckwheat (Yao et al., 2017; Luo et al., 2018). In addition, the transcription factor FtMYB116, which is induced by blue and red light, promotes rutin accumulation in light-induced bitter mustard via the direct regulation of F3′H expression and the indirect regulation of PAL, CHS, FLS, and F3H expression (Zhang et al., 2019).
More than a dozen flavonoids and structural genes have been identified in the leaves of A. arguta Sieb.Zucc. However, there have been no reports of systematically studying the composition of flavonoids in A. arguta Sieb.Zucc. and analyzing the molecular mechanisms of flavonoid biosynthesis. Here, we performed extensive transcriptomic and targeted metabolite analysis of flavonoids in the mature fruits of two important A. arguta Sieb.Zucc. cultivars grown in Northern China. A total of 118 flavonoids were identified, 39 of which showed different accumulation patterns between the 2 varieties. In addition, dozens of structural and transcriptional genes related to the regulation of flavonoid biosynthesis were found DEGs. Among them, the expression patterns of 21 were more strongly correlated with 7 flavonoids between the 2 varieties after fruit ripening. Certain DEG expression levels were validated using qRT-PCR. This data contributes to a better understanding of flavonoid biosynthesis in A. arguta Sieb.Zucc. at both the molecular and metabolic levels and provides precious information for future fruit quality improvement, cultivation of novel strains, and development of functional foods.
Mature fruit which have a fruit maturity from August to September was collected from 8-year-old A. arguta Sieb.Zucc. varieties (Qssg and Lc) grown in Northeast, North, and Northwest China and Shandong Province. There were three independent biological replicates for each sample. Collect the fresh fruit samples and put them in liquid nitrogen.
Total flavonoids were measured in the fruit samples. Metware Biotechnology Co., Ltd. (Wuhan, China) conducted 785 metabolite analyses. In short, cryopreserved samples at ultra-low temperatures were freeze-dried and ground with a grinder until it was a powder (Mixer Mill MM 400, Retsch, Haan, Germany) at 30 Hz for 1.5 min. Next, 100 mg of powder was mixed with 1.2 ml of 70% methanol. Samples were vortexed once every 30 min for 30 s a total of six times, then incubated overnight at 4°C. Retain the filtrate with a microporous membrane. A UPLC-MS/MS system was used to analyze the samples. Flavonoids were identified using a local database. A multiple reaction monitoring (MRM) model was used to quantitatively analyze the flavonoids (Chen et al., 2013; Dong et al., 2014, 2019). Flavonoids were further annotated online with PlantCyc1 and the KEGG.2 Principal component analysis (PCA) and orthogonal partial least squares discriminant analysis (OPLS-DA) were used to determine differentially accumulated flavonoids, where |log2 (fold change)| ≥ 1 was used as the threshold.
An EasySpin Plus Plant RNA Kit (Aidlab) was used to obtain total RNA from frozen fruits, which was then treated with DNase I (Takara) to clear it of contaminating DNA. mRNA was cleaned from the total RNA with a Dynabeads mRNA Purification Kit (Invitrogen, Waltham, MA, United States). The resulting purified mRNA was fragmented, after which first-strand cDNA was synthesized using random hexamer primers, and second-strand cDNA was synthesized with a NEBNext Ultra RNA Library Preparation Kit (New England Biolabs, Ipswich, MA, United States). cDNA was purified, ends were repaired, and the fragments were ligated to adapters. cDNA was then fragmented again after which it was enriched via PCR and the last cDNA library was built for Illumina paired-end sequencing. Biomarker Technology Co., Ltd. (Beijing, China) sequenced the cDNA library using the HiSeq Xten PE150 platform (Illumina, San Diego, CA, United States).
Based on MVDB V2.0 built by Maiwei (Wuhan) Biotechnology Co., Ltd. database and metabolite information public database. Qualitative analysis is carried out according to the secondary spectrum information. The quantitative analysis of metabolites is completed by triple quadrupole mass spectrometry multi reaction monitoring mode (MRM). The metabolic material spectra of different samples are obtained, and the mass spectrum peaks of substances are integrated by peak area. The mass spectrum peaks of the same metabolite in different samples are integrated and corrected, and the mass spectrum data are processed by Software Analyst 1.63. Multivariate statistical analysis was used to conduct PCA and cluster analysis on the two groups of samples. The stability and reliability of the model were predicted according to partial least squares discriminant analysis (PLS-DA) and OPLS-DA. Multi-dimensional statistical variable importance in the project (VIP) value, one-dimensional statistical p-value and differential multiple were used to screen differential metabolites. Through the pheatmap program in R (v3.2), the differential metabolic components in the fruit of A. arguta Sieb.Zucc. were clustered and analyzed, and the heat map was drawn. The differential metabolites in each group were screened by hierarchical clustering, and the corresponding differential metabolites were submitted to KEGG website for related pathway analysis.
Fastp software is used to strictly control the quality of RNA data and the adapter sequences and low-quality reads were removed to obtain clean reads, which we then mapped to the ACTIN reference gene sequence.3 Unigenes were determined using hisat2 and stringtie. Gene functional annotation was performed using BLAST to search several databases, namely the NCBI NR database, Swiss-Prot, GO, COG, KOG, Pfam, and KEGG, using an e-value cutoff of 10–5. The expression level for each gene was determined and then normalized according to the number of FPKM. The deseq software package was used to identify DEGs between samples. FDR < 0.05 and |log2 (fold change)| ≥ 1 were used as thresholds for defining DEGs. Functional enrichment analysis was performed on the DEGs using GO annotations and KEGG pathways.
qRT-PCR was used to validate the RNA-Seq data and measure the expression of structural genes involved in flavonoid biosynthesis. qRT-PCR analysis was conducted according to previously outlined methods (Park et al., 2011; Tan et al., 2021). The amplification cycle procedure is as follows: the reverse transcription operation is carried out with the reverse transcription kit (TUREscript 1st Stand cDNA SYNTHESIS Kit) of Aidlab Company. A total 20 μl reaction system is adopted and the reverse transcription reaction conditions were 42°C for 40 min and 65°C for 10 min. Fluorescence quantitative PCR procedure and system: 95°C 3 min, 95°C 10 s, 60°C 30 s. Taking ACTIN as the internal reference, the gene expression data are presented as relative expression, the relative expression of genes in each sample and each group was calculated by 2–ΔΔCt. Three biological replicates were performed on each sample. Supplementary Table 1 lists the primers used in qRT-PCR.
The correlation analysis was promoted by identifying the correlation coefficient of the content of flavonoids and the transcriptional changes in both differentially expressed flavonoids and differentially expressed genes. Both of them are rich in flavonoids and flavonol biosynthesis pathway (ko00941), flavonoid biosynthesis pathway (ko00942), and secondary metabolite biosynthesis pathway (ko00943). The interaction network between DEGs and differentially accumulated flavonoids was visualized with Cytoscape2.8.
To study the composition of flavonoids during the fruit ripening stage of A. arguta Sieb.Zucc. varieties Qssg and Lc (Figure 1), widely targeted metabonomics was used for the first time. LC-ESI-QTrap-MS/MS and multiple reaction monitoring were used to generate multi-peak maps. Specific ions for each substance were identified with the triple four-stage rod, while the signal strength (CPS) of the characteristic ions was generated using a detector. We opened the sample off-line mass spectrometry file using the multiquant software to conduct qualitative and quantitative analyses of the metabolite spectrum in Qssg and Lc A. arguta Sieb.Zucc.
A total of 118 flavonoids were identified, including 18 flavone, 6 dihydroflavonols, 12 Dihydroflavones, 12 flavonols, 64 flavonols, 2 chalcone compounds, 3 flavonoid carboglycosides, and 1 isoflavone (Supplementary Table 2). PCA highlighted that these two varieties were significantly different; 67.94% of differences between the samples could be explained by PC1 (40.97%) and PC2 (26.97%), indicating a change in the pattern of flavonoid accumulation between the two varieties (Figure 2). Hierarchical cluster analysis (HCA) based on the relative content of flavonoids in different cultivated varieties of A. arguta Sieb.Zucc. during fruit ripening showed that the concentration of most detected flavonoids changed greatly during fruit ripening. Among the 39 different flavonoids, comparing Qssg with Lc, 12 flavonoids in the fruit were up-regulated, that is, the relative content increased, and the increased metabolic components accounted for 30.8% of the 39 different metabolic components; 27 components were down regulated, that is, the relative content decreased, and the reduced metabolic components accounted for 69.2% of the 39 differential metabolic components. This further confirmed the difference in PCA results between the two main samples (Figure 3).
Figure 2. Principal component analysis score map of metabolites in Qssg and Lc fruits. Each point represents an independent biological repeat.
Figure 3. Cluster heat map of metabolites in Qssg and Lc fruits. There are obvious differences between the two samples.
Orthogonal partial least squares discriminant analysis can maximize the distinction among categories and identify differentially accumulated metabolites. It uses OSC and PLS-DA techniques to identify variable differences. The abundance of the 118 flavonoids was compared between samples using the OPLS-DA model (Figure 4). Lc samples were found to the left of the confidence interval, while the Qssg samples were found to the right of the confidence interval, clearly demonstrating the ability of the model to discriminate between the two varieties.
Figure 4. Orthogonal partial least squares discriminant analysis score map of metabolites in Qssg and Lc fruits. Each point represents an independent biological repeat.
We generated two principal components from OPLS-DA; the contribution rate was 62.1% for principal component 1 and 9.74% for principal component 2 [r2x = 0.807, r2y = 0.998 (p = 0.29), Q2 = 0.869 (p < 0.005)]. The OPLS-DA results were verified by performing 200 replicates of the analysis. Model verification (Figure 5) proved that the OPLS-DA model was biologically meaningful and superior to the PCA model.
Figure 5. Orthogonal partial least squares discriminant analysis model validation diagram of metabolites in Qssg and Lc fruits (p < 0.005; n = 200, that is, 200 alignment experiments were carried out).
The OPLS-DA results (Figure 5), the multivariable analysis, and the VIP value of VIP of the OPLS-DA model represent the influence intensity of the intergroup difference of the related metabolites when classifying and discriminating samples in models from each group. It is generally considered that metabolites with VIP ≥1 have a significant difference. Three classes and 39 species of differentially accumulated flavonoids were identified (Supplementary Table 3), accounting for 33.1% of all identified flavonoids (118). Specifically, 56.4% of the differentially accumulated flavonoids were flavonols, 10.3% were flavones, 10.3% were dihydroflavonoids, 10.3% were flavanols, 5.1% were chalcones, 5.1% were flavone carboglycosides, and 2.5% were dihydroflavonols. This suggests the presence of significant differences between flavonoids between different varieties of A. arguta Sieb.Zucc.
To facilitate observation of the change law of flavonoids, significantly different flavonoids were normalized, and a clustering heat map was generated (Figure 6).
Compare the difference multiple changes of quantitative information of flavonoids in two A. arguta Sieb.Zucc. samples of Qssg and Lc, and process the difference multiple with log2. The 20 most common differentially expressed flavonoids are shown (Figure 7). The relative flavonoid content of Lc variety fruits was significantly higher (1.34 times) than the Qssg fruits.
Figure 7. Difference multiples of flavonoids in Qssg and Lc fruits variation diagram. (The abscissa is the difference multiple of differential metabolites, with 2 as the base pair, and the ordinate is the differential metabolite. Red and green represent up regulation and down regulation of differential metabolites, respectively).
Pathway enrichment analysis of differentially accumulated flavonoids was conducted with KEGG (Figure 8). The 39 significantly different flavonoids identified were mainly distributed among 3 metabolic pathways: (1) flavonoid and flavonol biosynthesis pathways, primarily for kaempferol-3-o-neohesperidin Luteolin-7-o-neohesperidin, quercetin-3-o- sangbu diglycoside, quercetin-3-o-(2″-o-xylosyl)-rutoside, and kaempferol-3-o-rutoside; (2) flavonoid biosynthesis pathways, mainly including naringin-7-o-glucoside and isolyceride; and (3) secondary metabolite biosynthesis pathways, primarily kaempferol-3-o-rutoside.
To further study potential molecular mechanisms of flavonoid biosynthesis in A. arguta Sieb.Zucc., six cDNA libraries were constructed for high-throughput RNA-Seq analysis. Each library obtained 281,758,296 reads from 439,76,908 to 43,580,384, and each library obtained 269,732,354 clean reads from 41,844,326 to 41,708,692 (Supplementary Table 4). The q30 percentage (which includes sequences having an error rate < 0.1%) for each library exceeded 91%, and the mean GC content was 47.47%. A total of 78.54–80.76% of the clean reads were mapped to the transcripts of each library assembled and de redundant by Trinity, which were used as the reference sequence, and the clean reads of each sample were compared to the reference sequence. A total of 43,686 unique genes were identified. The analyses suggest high-quality RNA-Seq data suitable for use in further analyses.
For samples with biological duplication, DESeq2 was used for differential expression analysis between sample groups to obtain the differential expression gene set between the two biological conditions. The volcanic map can visually show the overall distribution of differential genes in the two groups of samples (Figure 9). DEGs in the mature fruits of two A. arguta Sieb.Zucc. varieties were identified by first analyzing the correlation coefficient between the profile clustering of gene expression and biological replicates. This analysis indicated that many genes exhibited differential expression in various samples. Correlation coefficients for the levels of gene expression of the biological replicates were >0.8 (Figure 10), indicating that the biological replicates were consistent with one another and the data were therefore valid for the identification of DEGs. Using threshold values of FDR < 0.05 and |log2 (fold change)| ≥ 1, DEGs between the 2 varieties were identified; there were 6497 up-regulated and 5153 down-regulated genes compared between Qssg and Lc.
To understand the biological functions of DEGs, we used Blast GO to determine enriched GO terms. Among the 55 enriched functional groups, there were 27 biological processes, 16 cell components, and 12 molecular functions (Figure 11 and Supplementary Table 5). Among the biological processes, the most frequent annotations were metabolic processes, cellular processes, and single biological processes. For cell component annotations, the most common terms were organelle, cell part, and cell. For molecular function annotations, the most represented terms were binding, catalytic activity, and transporter activity.
KEGG analysis was conducted to identify enriched metabolic pathways in the DEGs. There were 4024 DEGs distributed among 142 KEGG pathways (Supplementary Table 6). Among them, there were 42 significantly enriched pathways (p-value < 0.05) (Supplementary Table 7). Flavonoid biosynthesis (ko00941), flavonoid and flavonol biosynthesis (ko00944), and secondary metabolite biosynthesis (ko01110) pathways were all enriched, and the flavonoid biosynthesis (ko00941) and secondary metabolite biosynthesis (ko01110) pathways were significantly enriched. Flavonoid biosynthesis (ko00941) and secondary metabolite biosynthesis (ko01110) exist in the pathway of significant enrichment (Figure 12A). All of the enriched pathways could be further divided into five categories: cellular processes, genetic information managing, environmental information, metabolism, and tissue systems. Among these categories, the metabolism category contained the most pathways, and the highest numbers of DEGs were involved in amino acid biosynthesis (ko01230; 185 genes), carbon metabolism (ko01200; 190 genes), and sucrose and starch metabolism (ko00500; 207 genes) (Figures 12B–D).
Figure 12. Enrichment pathways of different genes in Qssg and Lc fruits. (A) Scatter diagram of enrichment of differential gene KEGG in Qssg and Lc fruits. (B–D) Column diagram of enrichment of differential gene GO in Qssg and Lc.
The enriched KEGG pathways and gene functional annotations were used to determine which DEGs encode flavonoid biosynthesis-related enzymes, flavonoid, and flavonol biosynthesis-related enzymes, and secondary metabolite biosynthesis-related enzymes. In total, 41 were found, including 6 CsUGT134 genes, 17 LOC, 2 AT2, 1 CHS, 1 CHI, 3 C4H, 1HCT, 1 CCoAOMT, 1 F3H, 2 CFOL, 1 DFR, 3 LAR2, 1 LSAT, and 1 GSCOC (Supplementary Table 8). Among those, 6 UGT9491, 16 LOC, 2 AT2, 1 CHS, 2 C4H, 1 HCT, 1 CCoAOMT, 1 F3H, 2 LAR2, and 1 GSCOC were significantly up-regulated in the 2 varieties. A total of 2 CFOL, 1 CHI, 1 C4H, 1 DFR, 1 LAR2, 1 LOC, and 1 LSAT were significantly down-regulated between the two varieties.
To validate the expression of the DEGs related to flavonoid biosynthesis, qRT-PCR was performed for 11 structural genes (1 CsUGT134, 1 LAR2, 1 C4H, 1 CFOL, 1 CHI, 2 LOC, 1 GSCOC, 1 CCoAOMT, 1 DFR, and 1 AT2) in the fruits of the 2 varieties at maturity. The selected genes were highly correlated between RNA-Seq and qPCR data, effectively validating the transcriptome data (Figures 13C,D).
Figure 13. The differential flavonoid-related regulatory gene network in Qssg and Lc Actinidia arguta Sieb.Zucc. fruits and qRT-PCR confirmation map. (A,B) Twenty-one structural genes and 7 flavonoids showed higher correlation coefficient values (r > 0.8). (C) The relative expression of seven structural genes in Lc was higher than that in Qssg. (D) The relative expression of four structural genes in Lc was lower than that in Qssg. Most of the selected genes showed high correlation between the qPCR and RNA-Seq datasets, thus validating the transcriptome data (2–ΔΔCt value of the internal reference gene is ACTIN).
To better outline, the regulatory network controlling flavonoid biosynthesis, quantitative changes in flavonoids, and gene expression were compared. Based on the results of DEGs and DEMs rich in flavone biosynthesis pathway, 21 structural genes and 7 flavonoids (Supplementary Table 9) exhibited high correlation coefficients (r > 0.8). The interaction network is shown (Figures 13A,B). The 21 structural genes were as follows: 2 CsUGT134, 1 LAR2, 1 C4H, 2 CFOL, 1 CHI, 9 LOC, 1 GSCOC, 1 CCoAOMT, 1 DFR, and 2 AT2 genes. These are known to be the key structural genes related to the flavonoid biosynthesis pathway. The seven flavonoids were apigenin-7-o-glucoside, luteolin-7-o-neohesperidin (honeysuckle glycoside), kaempferol-3-o-rutoside (fireworks glycoside), quercetin-3-o-sangbu diglycoside, quercetin-3-o-(2″-o-xylosyl)-rutoside, naringin-7-o-glucoside (cherry glycoside), and isolyceride (phloridin chalcone). Eleven DEGs were further selected to verify the transcriptome results. qRT-PCR showed that the expression levels of C4H (Cluster-10307.4519), CsUGT134 (Cluster-10307.5267), AT2 (Cluster-10307.55417), LOC (Cluster-10307.81571, Cluster-10307.4971), DFR (Cluster-10307.30160), and CFOL (Cluster-10307.10288) in Lc were higher than those in Qssg. The expression levels of CHI (Cluster-10307.69071), LAR2 (Cluster-10307.9753), GSCOC (Cluster-6365.0), and CCOAOMT (Cluster-10307.82368) were inhibited in Lc. This means that these structural enzymes are required for flavonoid biosynthesis in A. arguta Sieb.Zucc. and the expression patterns among members may be opposite. It indicates that there are polygenic families, which may have different control mechanisms on the biosynthesis of flavonoids in A. arguta Sieb.Zucc. (Figure 13 and Supplementary Table 10).
Actinidia arguta Sieb.Zucc. is rich in bioactive flavonoids, which have been used to develop various health foods such as beverages, dried fruits, and wine. However, the flavonoid composition and potential mechanism regulating flavonoid biosynthesis in A. arguta Sieb.Zucc. remains largely unclear. Now, we conducted a comprehensive transcriptomic and metabolite analysis to determine the flavonoid composition in A. arguta Sieb.Zucc. and identify genes related to flavonoid biosynthesis.
We analyzed the metabolites to determine 118 flavonoids, greatly broadening our knowledge of flavonoids in the mature fruits of the 2 main varieties of A. arguta Sieb.Zucc. grown in Northern China. Among these flavonoids, 18 flavone, and 64 flavonol compounds were the main flavonoids. More than half of the 118 flavonoids were glycosides, which are the most abundant form of flavonoids in plants (Ren et al., 2014; Kim et al., 2018; Richardson et al., 2018; Wang et al., 2021). It is worth noting that one isoflavone (daidzein) and one flavone compound (procyanidin) were identified in these fruits for the first time. It has been reported that isoflavones can supple estrogen and have the same human health care functions as other flavonoids, and also function in cancer prevention (Pei et al., 2018; Nakajima and Ohizumi, 2019). Thus far, isoflavones have only been found in some legumes, which are therefore the main source of isoflavones for functional food production (Park et al., 2018). Our identification of isoflavones in the fruit of A. arguta Sieb.Zucc. not only provides a new source of isoflavones, but also provides new opportunities for developing food and drug homologous functional health foods using this fruit. We also found that the composition and abundance of flavonoids were significantly different between the two main cultivated varieties at the fruit ripening stage. This not only provides a solid theoretical and practical basis for the comprehensive development of A. arguta Sieb.Zucc., but also provides key information for artificial cultivation of A. arguta Sieb.Zucc. in Northern China.
Two types of genes are bound up with plant flavonoid biosynthesis (Chen et al., 2017; Nabavi et al., 2020). The first is structural genes encoding enzymes that can catalyze the biosynthesis of different flavonoids; the second is regulatory genes that regulate structural gene transcription (Liu et al., 2016; Nabavi et al., 2020). In this experiment, we used gene functional annotation and KEGG enrichment analysis to determine 41 DEGs encoding enzymes known to be involved in flavonoid biosynthesis: CSUGT134, LOC, AT2, CHS, CHI, C4H, HCT, CCoAOMT, F3H, CFOL, DFR, LAR2, LSAT, and GSCOC. In many plants, naringin, saonol, kaempferol, myricetin, dihydromyricetin, and dihydroquercetin are catalyzed by positively regulating the expression of CHS, CHI, F3H, F3′H, and FLS (Liu et al., 2002; Deavours and Dixon, 2005; Chen et al., 2015; Wang et al., 2017, 2019; Matsui et al., 2018; Zhai et al., 2019). AaF3H, AaLDOX, AaUFGT, AaMYB, AabHLH, and AaHB2 showed the best possibility as candidate genes. The regulatory network of flavonoid biosynthesis was established, indicating that the differentially expressed candidate genes were involved in the accumulation of metabolites (Li et al., 2018). In the KEGG pathway, it is also noted that CHS, CHI, F3H, CFOL, LOC, LSAT, FNSI, DFR, F3′H, FLS, and HIDH may play the role of structure and regulatory genes in the biosynthesis path of flavonoids such as aspen (flavone1), rutin (flavone2), and daidzein (flavone3). In particular, DFR, F3′H, and FLS compete with the substrate dihydrokaempferol DHK. The expression of FLS is relatively high, and its coding enzyme activity is high, which finally promotes the relative content of rutin; the expression of LOC was relatively low, and the relative contents of populin and daidzein were relatively low.
Among these DEGs are key genes involved in flavonoid biosynthesis, namely 3 CSUGT134, 1 LAR2, 1 C4H, 2 CFOL, 1 CHI, 9 LOC, 1 GSCOC, 1 CCoAOMT, 1 DFR, and 2 AT2.
By assessing the transcriptomes, we determined 43,686 genes in A. arguta Sieb.Zucc., including 11,650 DEGs. These genes regulate the metabolism, cellular processes, processing of genetic material, environmental inputs, and organizational system of A. arguta Sieb.Zucc.
For some structural genes, mRNA expression levels were closely correlated with the accumulation of specific flavonoids, suggesting that this flavonoid biosynthesis gene expression promoted flavonoid accumulation during fruit ripening in A. arguta Sieb.Zucc. Here, we found that 1 CHS, 1 F3H, 2 LAR2, 6 CSUGT134, 16 LOC, 2 AT2, 2 C4H, 1 HCT, 1 CCoAOMT, and 1 GSCOC genes were significantly up-regulated, and 1 CHI, 1 LAR2, 2 CFOL, 1 C4H, 1 DFR, 1 LOC, and 1 LSAT genes were significantly down-regulated. We studied the relation between the metabolite and transcriptome data, which demonstrated that the expression of structural genes regulating total flavonoids in Lc varieties was higher than that in Qssg varieties and combining these data was an effective strategy to identify genes bound up with flavonoid biosynthesis.
In conclusion, we here identified flavonoid components present in A. arguta Sieb.Zucc. fruits and measured differential gene expression and flavonoid accumulation between the two main cultivars grown in Northern China. Differentially expressed structural genes bound up with flavonoid biosynthesis were identified and validated with qRT-PCR. We also analyzed flavonoid biosynthesis gene expression levels that affect flavonoid accumulation. Through the correlation analysis between transcriptome and metabolite analysis, some flavonoids catalyzed by the expression of CsUGT134, LAR2, C4H, CFOL, CHI, LOC, GSCOC, CCoAOMT, DFR, and AT2 genes were known. These results expand the current understanding of flavonoid composition, accumulation patterns, and biosynthesis mechanisms in A. arguta Sieb.Zucc. The data provide valuable new information for future development of homologous functional foods making use of A. arguta Sieb.Zucc.
The datasets presented in this study can be found in online repositories. The names of the repository/repositories and accession number(s) can be found in the article/Supplementary Material.
LC designed the project and took part in the project administration and funding acquisition. YbW carried out the experiment and wrote the draft for the first time. JS, HJ, and MI helped to review and edit the manuscript. FY and YD have provided the experimental materials and valuable opinions. LC and YW had overall responsibility for this project, including project ideas, guidance on experimental design, data analysis, manuscript writing, and revision. All authors have read and approved the final manuscript.
This work was financially supported by the National Key R&D Program of China (Grant No. 2019YFD1001002) and the National Natural Science Foundation of China (Grant No. 31572150). The role of the funding body in the design of the study, the collection, analysis, and interpretation of data and in writing the manuscript is management and supervision.
YD was employed by Shandong Xianda Agrochemical Co., Ltd.
The remaining authors declare that the research was conducted in the absence of any commercial or financial relationships that could be construed as a potential conflict of interest.
All claims expressed in this article are solely those of the authors and do not necessarily represent those of their affiliated organizations, or those of the publisher, the editors and the reviewers. Any product that may be evaluated in this article, or claim that may be made by its manufacturer, is not guaranteed or endorsed by the publisher.
The Supplementary Material for this article can be found online at: https://www.frontiersin.org/articles/10.3389/fpls.2022.911203/full#supplementary-material
Almeida, D., Pinto, D., Santos, J., Vinha, A. F., Palmeira, J., Ferreira, H. N., et al. (2018). Hardy kiwifruit leaves (Actinidia arguta): an extraordinary source of value-added compounds for food industry. Food Chem. 259, 113–121. doi: 10.1016/j.foodchem.2018.03.113
Bai, Y.-C., Li, C.-L., Zhang, J.-W., Li, S.-J., Luo, X.-P., Yao, H.-P., et al. (2014). Characterization of two tartary buckwheat R2R3-MYB transcription factors and their regulation of proanthocyanidin biosynthesis. Physiol. Plant. 152, 431–440. doi: 10.1111/ppl.12199
Chen, J., Yuan, H., Zhang, L., Pan, H., Xu, R., Zhong, Y., et al. (2015). Cloning, expression and purification of isoflavone-2’-hydroxylase from Astragalus membranaceus Bge. Var. mongolicus (Bge.) Hsiao. Protein Exp. Purif. 107, 83–89. doi: 10.1016/j.pep.2014.11.010
Chen, W., Gong, L., Guo, Z., Wang, W., Zhang, H., Liu, X., et al. (2013). A novel integrated method for large-scale detection, identification, and quantification of widely targeted metabolites: application in the study of rice metabolomics. Mol. Plant 6, 1769–1780. doi: 10.1093/mp/sst080
Chen, W.-K., Bai, X.-J., Cao, M.-M., Cheng, G., Cao, X.-J., Guo, R.-R., et al. (2017). Dissecting the variations of ripening progression and flavonoid metabolism in grape berries grown under double cropping system. Front. Plant Sci. 8:1912. doi: 10.3389/fpls.2017.01912
Deavours, B. E., and Dixon, R. A. (2005). Metabolic engineering of isoflavonoid biosynthesis in alfalfa. Plant Physiol. 138, 2245–2259. doi: 10.1104/pp.105.062539
Dong, T., Han, R., Yu, J., Zhu, M., Zhang, Y., Gong, Y., et al. (2019). Anthocyanins accumulation and molecular analysis of correlated genes by metabolome and transcriptome in green and purple asparaguses (Asparagus officinalis, L.). Food Chem. 271, 18–28. doi: 10.1016/j.foodchem.2018.07.120
Dong, X., Chen, W., Wang, W., Zhang, H., Liu, X., and Luo, J. (2014). Comprehensive profiling and natural variation of flavonoids in rice. J. Integr. Plant Biol. 56, 876–886. doi: 10.1111/jipb.12204
Gupta, N., Sharma, S. K., Rana, J. C., and Chauhan, R. S. (2011). Expression of flavonoid biosynthesis genes vis-à-vis rutin content variation in different growth stages of Fagopyrum species. J. Plant Physiol. 168, 2117–2123. doi: 10.1016/j.jplph.2011.06.018
Hãdãrugã, D. I., Pantea, C., and Hãdãrugã, N. G. (2016). Antioxidant activity and kinetics on kiwi fruit (Actinidia deliciosa) ethanolic extracts by 2,2-diphenyl-1-picrylhydrazyl (DPPH⋅) method. J. Agroaliment. Process. Techol. 22, 207–211.
Hu, Y., Hou, Z., Liu, D., and Yang, X. (2016). Tartary buckwheat flavonoids protect hepatic cells against high glucose-induced oxidative stress and insulin resistance via MAPK signaling pathways. Food Funct. 7, 1523–1536. doi: 10.1039/c5fo01467k
Jiang, L. L., Gong, X., Ji, M. Y., Wang, C. C., Wang, J. H., and Li, M. H. (2020). Bioactive compounds from plant-based functional foods: a promising choice for the prevention and management of hyperuricemia. Foods 9:973. doi: 10.3390/foods9080973
Kim, B., Woo, S., Kim, M.-J., Kwon, S.-W., Lee, J., Sung, S. H., et al. (2018). Identification and quantification of flavonoids in yellow grain mutant of rice (Oryza sativa L.). Food Chem. 241, 154–162. doi: 10.1016/j.foodchem.2017.08.089
Latocha, P., Krupa, T., Jankowski, P., and Radzanowska, J. (2014). Changes in postharvest physicochemical and sensory characteristics of hardy kiwifruit (Actinidia arguta and its hybrid) after cold storage under normal versus controlled atmosphere. Postharvest Biol. Technol. 88, 21–33. doi: 10.1016/j.postharvbio.2013.09.005
Latocha, P., Wolosiak, R., Worobiej, E., and Krupa, T. (2013). Clonal differences in antioxidant activity and bioactive constituents of hardy kiwifruit (Actinidia arguta) and its year-to-year variability. J. Sci. Food Agric. 93, 1412–1419. doi: 10.1002/jsfa.5909
Li, C., Bai, Y., Li, S., Chen, H., Han, X., Zhao, H., et al. (2012). Cloning, characterization, and activity analysis of a flavonol synthase gene FtFLS1 and its association with flavonoid content in tartary buckwheat. J. Agric. Food Chem. 60, 5161–5168. doi: 10.1021/jf205192q
Li, C. L., Bai, Y. C., Chen, H., Zhao, H. X., Shao, J. R., and Wu, Q. (2012). Cloning, characterization and functional analysis of a phenylalanine ammonia-lyase gene (FtPAL) from Fagopyrum tataricum gaertn. Plant Mol. Biol. Rep. 30, 1172–1182. doi: 10.1007/s11105-012-0431-9
Li, X., Thwe, A. A., Park, N. I., Suzuki, T., Kim, S. J., and Park, S. U. (2012). Accumulation of phenylpropanoids and correlated gene expression during the development of tartary buckwheat sprouts. J. Agric. Food Chem. 60, 5629–5635. doi: 10.1021/jf301449a
Li, Y., Fang, J., Qi, X., Lin, M., Zhong, Y., and Sun, L. (2018). A key structural gene, AaLDOX, is involved in anthocyanin biosynthesis in all red-fleshed kiwifruit (Actinidia arguta) based on transcriptome analysis. Gene 648, 31–41. doi: 10.1016/j.gene.2018.01.022
Liu, C.-J., Blount, J. W., Steele, C. L., and Dixon, R. A. (2002). Bottlenecks for metabolic engineering of isoflavone glycoconjugates in Arabidopsis. Proc. Natl. Acad. Sci. U. S. A. 99, 14578–14583. doi: 10.1073/pnas.212522099
Liu, X., Luo, Y., Wu, H., Xi, W., Yu, J., Zhang, Q., et al. (2016). Systematic analysis of O-methyltransferase gene family and identification of potential members involved in the formation of O-methylated flavonoids in Citrus. Gene 575, 458–472. doi: 10.1016/j.gene.2015.09.048
Luo, X., Zhao, H., Yao, P., Li, Q., Huang, Y., Li, C., et al. (2018). An R2R3-MYB transcription factor FtMYB15 involved in the synthesis of anthocyanin and proanthocyanidins from tartary buckwheat. J. Plant Growth Regul. 37, 76–84. doi: 10.1007/s00344-017-9709-3
Matsui, K., Oshima, Y., Mitsuda, N., Sakamoto, S., Nishiba, Y., Walker, A. R., et al. (2018). Buckwheat R2R3 MYB transcription factor FeMYBF1 regulates flavonol biosynthesis. Plant Sci. 274, 466–475. doi: 10.1016/j.plantsci.2018.06.025
Nabavi, S. M., Šamec, D., Tomczyk, M., Milella, L., Russo, D., Habtemariam, S., et al. (2020). Flavonoid biosynthetic pathways in plants: versatile targets for metabolic engineering. Biotechnol. Adv. 38:107316. doi: 10.1016/j.biotechadv.2018.11.005
Nakajima, A., and Ohizumi, Y. (2019). Potential benefits of nobiletin, a citrus flavonoid, against Alzheimer’s disease and Parkinson’s disease. Int. J. Mol. Sci. 20:3380. doi: 10.3390/ijms20143380
Pal, R. S., Kumar, V. A., Arora, S., Sharma, A. K., Kumar, V., and Agrawal, S. (2015). Physicochemical and antioxidant properties of kiwifruit as a function of cultivar and fruit harvested month. Braz. Arch. Biol. Technol. 58, 262–271. doi: 10.1590/s1516-8913201500371
Park, N. I., Li, X., Suzuki, T., Kim, S.-J., Woo, S.-H., Park, C. H., et al. (2011). Differential expression of anthocyanin biosynthetic genes and anthocyanin accumulation in tartary buckwheat cultivars ‘Hokkai T8’ and ‘Hokkai T10’. J. Agric. Food Chem. 59, 2356–2361. doi: 10.1021/jf200020b
Park, S.-Y., Kim, J. K., Kim, E.-H., Kim, S.-H., Prabakaran, M., and Chung, I.-M. (2018). Comparison of 12 isoflavone Profiles of soybean(Glycine max(L.)Merrill) seed sprouts from three different countries. Korean J. Crop Sci. 63, 360–377. doi: 10.7740/kjcs.2018.63.4.360
Pei, R., Zhang, J., Tian, L., Zhang, S., Han, F., Yan, S., et al. (2018). Identification of novel QTL associated with soybean isoflavone content. Crop J. 6, 244–252. doi: 10.1016/j.cj.2017.10.004
Ren, Q., Li, Y., Wu, C., Wang, C., Jin, Y., and Zhang, J. (2014). Metabolism of secondary metabolites isolated from Tartary buckwheat and its extract. Food Chem. 154, 134–144. doi: 10.1016/j.foodchem.2013.12.107
Richardson, D. P., Ansell, J., and Drummond, L. N. (2018). The nutritional and health attributes of kiwifruit: a review. Eur. J. Nutr. 57, 2659–2676. doi: 10.1007/s00394-018-1627-z
Tan, C., Wang, Z., Feng, X., Pan, B., Irfan, M., and Liu, C. (2021). Transcriptomic and metabolomics of flavonoid compounds in Actinidia arguta var. arguta. J. King Saud Univ. Sci. 33:101605. doi: 10.1016/j.jksus.2021.101605
Wang, F., Ren, X., Zhang, F., Qi, M., Zhao, H., Chen, X., et al. (2019). A R2R3-type MYB transcription factor gene from soybean, GmMYB12, is involved in flavonoids accumulation and abiotic stress tolerance in transgenic Arabidopsis. Plant Biotechnol. Rep. 13, 219–233. doi: 10.1007/s11816-019-00530-7
Wang, N., Xu, H., Jiang, S., Zhang, Z., Lu, N., Qiu, H., et al. (2017). MYB12 and MYB22 play essential roles in proanthocyanidin and flavonol synthesis in red-fleshed apple (Malus sieversii f. niedzwetzkyana). Plant J. 90, 276–292. doi: 10.1111/tpj.13487
Wang, R., Ren, C., Dong, S., Chen, C., Xian, B., Wu, Q., et al. (2021). Integrated metabolomics and transcriptome analysis of flavonoid biosynthesis in safflower (Carthamus tinctorius L.) with different colors. Front. Plant Sci. 12:712038. doi: 10.3389/fpls.2021.712038
Yao, P., Zhao, H., Luo, X., Gao, F., Li, C., Yao, H., et al. (2017). Fagopyrum tataricum FtWD40 functions as a positive regulator of anthocyanin biosynthesis in transgenic tobacco. J. Plant Growth Regul. 36, 755–765. doi: 10.1007/s00344-017-9678-6
Yao, P. F., Zhao, H. X., Luo, X. P., Gao, F., Yao, H. P., Li, C. L., et al. (2016). Chalcone synthase homologous genes cloning and expression pattern in flowering Fagopyrum tataricum Gaertn. Russ. J. Plant Physiol. 63, 790–799. doi: 10.1134/S1021443716060169
Zhai, R., Zhao, Y., Wu, M., Yang, J., Li, X., Liu, H., et al. (2019). The MYB transcription factor PbMYB12b positively regulates flavonol biosynthesis in pear fruit. BMC Plant Biol. 19:85. doi: 10.1186/s12870-019-1687-0
Zhang, D., Jiang, C., Huang, C., Wen, D., Lu, J., Chen, S., et al. (2019). The light-induced transcription factor FtMYB116 promotes accumulation of rutin in Fagopyrum tataricum. Plant Cell Environ. 42, 1340–1351. doi: 10.1111/pce.13470
Zhang, L., Li, X., Ma, B., Gao, Q., Du, H., Han, Y., et al. (2017). The tartary buckwheat genome provides insights into rutin biosynthesis and abiotic stress tolerance. Mol. Plant 10, 1224–1237. doi: 10.1016/j.molp.2017.08.013
Keywords: Actinidia arguta Sieb.Zucc., flavonoids, biosynthesis, widely targeted metabolome, transcriptome, joint analysis
Citation: Wang Y, Wang Y, Sun J, Dai Y, Yang F, Jiang H, Irfan M and Chen L (2022) Metabolomic and Transcriptomic Analysis of Flavonoid Biosynthesis in Two Main Cultivars of Actinidia arguta Sieb.Zucc. Grown in Northern China. Front. Plant Sci. 13:911203. doi: 10.3389/fpls.2022.911203
Received: 02 April 2022; Accepted: 02 June 2022;
Published: 30 June 2022.
Edited by:
Ahmad Arzani, Isfahan University of Technology, IranReviewed by:
Gholamreza Khaksar, Chulalongkorn University, ThailandCopyright © 2022 Wang, Wang, Sun, Dai, Yang, Jiang, Irfan and Chen. This is an open-access article distributed under the terms of the Creative Commons Attribution License (CC BY). The use, distribution or reproduction in other forums is permitted, provided the original author(s) and the copyright owner(s) are credited and that the original publication in this journal is cited, in accordance with accepted academic practice. No use, distribution or reproduction is permitted which does not comply with these terms.
*Correspondence: Lijing Chen, Y2hlbmxpamluZzE5OTdAMTI2LmNvbQ==; Y2hlbmxpamluZ0BzeWF1LmVkdS5jbg==
†These authors share first authorship
Disclaimer: All claims expressed in this article are solely those of the authors and do not necessarily represent those of their affiliated organizations, or those of the publisher, the editors and the reviewers. Any product that may be evaluated in this article or claim that may be made by its manufacturer is not guaranteed or endorsed by the publisher.
Research integrity at Frontiers
Learn more about the work of our research integrity team to safeguard the quality of each article we publish.