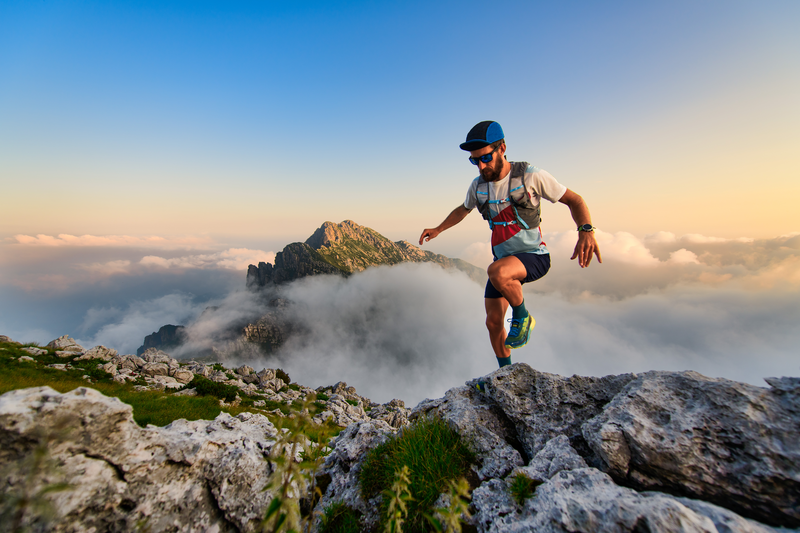
95% of researchers rate our articles as excellent or good
Learn more about the work of our research integrity team to safeguard the quality of each article we publish.
Find out more
ORIGINAL RESEARCH article
Front. Plant Sci. , 29 July 2022
Sec. Functional Plant Ecology
Volume 13 - 2022 | https://doi.org/10.3389/fpls.2022.909603
This article is part of the Research Topic Leaf Functional Traits: Ecological and Evolutionary Implications View all 16 articles
Intrinsic water use efficiency (iWUE) is a critical eco-physiological function allowing plants to adapt to water- and nutrient-limited habitats in arid and semi-arid regions. However, the distribution of iWUE in coexisting species along aridity gradients and its controlling factors are unknown. We established two transects along an aridity gradient in the grasslands of Losses Plateau (LP) and Inner Mongolia Plateau (MP) to elucidate the patterns and underlying mechanisms of iWUE distribution in coexisting species along aridity gradient. We determined leaf carbon (δ13C) and oxygen (δ18O) stable isotopes, functional traits related to carbon fixation, and limiting resources. Bulk leaf δ13C and δ18O were used as proxies for time-integrated iWUE and stomatal conductance (gs) during the growing season. Our results showed that variability in iWUE within transect was primarily controlled by species, sampling sites and an interactive effect between species and sampling sites. Mean values of iWUE (iWUEMean) increased and coefficient of variation (CV) in iWUE (iWUECV) decreased with an increase in aridity, demonstrating that increases in aridity lead to conservative and convergent water use strategies. Patterns of iWUEMean and iWUECV were controlled primarily by the ratio of soil organic carbon to total nitrogen in LP and soil moisture in MP. This revealed that the most limited resource drove the distribution patterns of iWUE along aridity gradients. Interspecific variation in iWUE within transect was positively correlated with Δ18O, indicating that interspecific variation in iWUE was primarily regulated by gs. Furthermore, relationship between iWUE and multi-dimensional functional trait spectrum indicated that species evolved species-specific strategies to adapt to a harsh habitat by partitioning limiting resources. Overall, these findings highlighted the interactive effects of limiting resources and leaf functional traits on plant adaptation strategies for iWUE, and emphasized the importance of considering biological processes in dissecting the underlying mechanisms of plant adaptation strategies at large regional scales.
Arid and semi-arid regions constitute ~41% of the global land area (Reynolds et al., 2007), and are predicted to expand in the future (Yao et al., 2020). Limited water and nutrient resources in these regions severely restrict plant growth, survival, and reproduction (Martin-StPaul et al., 2017). To avoid hydraulic failure and carbon starvation (Choat et al., 2018), plants open or close the stomata to balance carbon uptake and water losses (Galmes et al., 2007). Consequently, intrinsic water use efficiency (iWUE), the ratio of carbon gain in photosynthetic rate (A) to stomatal conductance (gs), is a critical eco-physiological strategy for plant water–carbon regulation in the process of adaptation to water- and nutrient-limited habitats (Moreno-Gutierrez et al., 2012; Querejeta et al., 2018; Wang et al., 2018).
Diverse trade-offs between carbon gain and water loss (iWUE) facilitate species coexistence in habitats with harsh and unpredictable environmental conditions (Moreno-Gutierrez et al., 2012; Bermúdez and Retuerto, 2014; Wang et al., 2018). In generally, plants with a conservative water use strategy (high iWUE) are better able to adapt to water-limited habitats than those with low iWUE (Flexas et al., 2016; Aparecido et al., 2020). However, opportunistic water-use strategy (low iWUE) is advantageous for nutrient acquisition (Querejeta et al., 2018). Along environmental gradients, limiting resources act as environmental filters, shape the expression of plant eco-physiological functions (Bahar et al., 2016), determine trait distribution of each species in the community, and theoretically drive species toward an “optimum” for a set of environmental conditions (Mitchell et al., 2018). However, understanding of iWUE distribution in coexisting species in response to aridity gradients, and its controlling factors remain incomplete.
Given that iWUE is regulated by a rapid response of A and gs to environmental variables (Prentice et al., 2014; Basu et al., 2021), time-integrated iWUE can reflect adaptability of plants to their environment, especially in areas with large environmental fluctuations (Maxwell et al., 2018; Guerrieri et al., 2019). Leaf carbon isotope discrimination during photosynthesis (Δ13C) is linearly related to the ratio of the partial pressures of intercellular (Ci) to ambient (Ca) CO2 (Ci/Ca), and is influenced by both A and gs over a period when the leaf is produced (Farquhar et al., 1989; Maxwell et al., 2018; Prieto et al., 2018). Time integrated iWUE can be estimated independently from the carbon isotope ratios (δ13C) of leaf tissue, allowing for more extensive studies of plant water use strategies at broader spatial scales than traditional leaf gas exchange measurements (Prentice et al., 2014).
According to the least-cost theory (Wright et al., 2003), water and nitorgen (N) are expected to be the most important environmental filters shaping the distribution of iWUE in each species in communities along aridity gradients. Previous studies conducted in arid and semi-arid areas focused primarily on spatial patterns of iWUE in response to water variables. As expected, leaf iWUE in drylands increases with decreasing precipitation (Zheng and Shangguan, 2007; Liu et al., 2013; Wang et al., 2016) or soil moisture (SM) (Ale et al., 2018) to reduce water cost. Alternatively, iWUE may decrease to reduce N cost when low soil N increases the cost of soil N uptake (Wright et al., 2003). In general, soil N supply (i.e., mineralization and nitrification rates) exhibits a decreasing trend with increasing water stress (Feyissa et al., 2021). However, it is not clear whether water or N-limitation dominates the distribution of iWUE in co-occuring species in communities along aridity gradients. In addition, evidence is accumulating in support of diverse water use strategies in resource-limited ecosystems (Moreno-Gutierrez et al., 2012; Bermúdez and Retuerto, 2014; Wang et al., 2018). However, it has not been established how this diversity varies with the strength of environmental filtering.
Large interspecific differences in iWUE along environmental gradients may be driven by variability in leaf functional traits within species (Maxwell et al., 2018; Rumman et al., 2018; Tang et al., 2021), because leaf functional traits can determine species persistence against strong environmental filters along a resource-use strategies gradient (Maracahipes et al., 2018; Guo et al., 2021; Li et al., 2022). Theoretically, many leaf functional traits correlate with variability in iWUE, including leaf N per unit area (Prentice et al., 2014) and specific leaf area (SLA) (Maxwell et al., 2018; Prieto et al., 2018). However, these are likely secondary to the primary effects of A and gs (Roden and Farquhar, 2012). In general, the first response of plants to dryness stress is to reduce gs, and photosynthetic capacity begins to decrease with the increase in dryness stress (Utkhao and Yingjajaval, 2015). However, it is challenging to determine whether variability in time-integrated iWUE derived from Δ13C is the result of changes in A, or gs, or both (Scheidegger et al., 2000; Farquhar et al., 2007). Given that the leaf oxygen isotope (δ18O) is unaffected by variability in A, oxygen isotope enrichment in leaf tissue above source water (Δ18O) may help in disentangling the independent effects of A and gs on iWUE (Guerrieri et al., 2019).
In this study, we aimed to gain insight into the response of iWUE distribution in coexisting species to aridity gradient, and elucidated the underlying mechanisms. The Loess Plateau (LP) and the Inner Mongolia Plateau (MP) are located mainly in arid and semi-arid regions in China. The preliminary limiting resource for plants is soil N in LP, and water in MP (Ren et al., 2021). Thus, LP and MP provide ideal conditions for an exploration of water use strategies in coexisting species in response to resource-limiting habitats. We hypothesized that (1) increased aridity may lead to a divergence in iWUE distribution, (2) distribution patterns of iWUE along an aridity gradient may be controlled by the most limited resource such as N in LP, and water in MP, and (3) interspecific variation in iWUE is influenced primarily by gs, rather than A, because high VPD and dry soil conditions can lead to severely reduced gs before manifesting in photosynthetic capacity (Utkhao and Yingjajaval, 2015). To test these hypotheses, we established two grassland transects along aridity gradients from east to west in LP and TP. We used bulk leaf δ13C and δ18O to calculate iWUE and Δ18O, respectively. The latter was used to reflect variability in gs among species.
This study was conducted along two grassland transects, one in the Loess Plateau (LP) and one in the Inner Mongolia Plateau (MP) (Figure 1). LP has a typical arid and semi-arid temperate continental monsoon climate, and an extremely fragmented hilly loess landscape due to severe soil and water erosion (Yue et al., 2019). MP experiences arid and semi-arid continental climate.
Figure 1. Geographic characteristics of the grassland transects in Loess Plateau (LP) and Inner Mongolia Plateau (MP).
The LP transect was 600 km long from east (Lucheng District, 113.36°E, 36.29°N) to west (Shapotou District, 104.44°E, 37.46°N), with a wide range in annual total precipitation (189–599 mm) and aridity (0.51–0.63) (Figure 1). Aridity was calculated as 1- mean annual precipitation/ potential evapotranspiration. The MP transect was 1,200 km long from east (Changling County, 123.51°E, 44.59°N) to west (Erenhot, 112.15°E, 43.63°N), with a smaller range in precipitation (183–425 mm) and greater aridity (0.42–0.83). Each transect includes 10 sampling sites at an interval of about 60–150 km from east to west (Lyu et al., 2021). Grassland types include meadow grassland (n = 3), typical grassland (n = 4), and desert grassland (n = 3).
Field sampling was carried out during the peak growing season (July–August) in 2018. We delineated a 1 km × 1 km sampling area within each sampling site, and tried to collected as many different species as possible (Zhang et al., 2019). Plant species were identified by experienced plant taxonomists. Overall, 574 and 433 plant species were found at LP and MP, respectively, including trees, shrubs, herbs and ferns (Supplementary Table S1).
At least 3 individuals per species per sampling area were selected as replicates. For trees and shrubs, we collected branches from the sunny-side of crowns. For herbs, we collected mature whole plants. Ten, fully expanded and mature leaves (n = 50) collected from each species were mixed uniformly into one sample for subsequent analyses. Soil samples were collected from the 0 to 10 cm layer using a soil auger, with eight replications at each site.
Leaf apparent morphology was determined using six to 10 fresh leaves per species. Leaf area (LA) was measured with a portable scanner (Cano Scan LIDE 110, Japan). LA values were obtained using an Image J software (Schneider et al., 2012). Leaf dry mass was determined after drying at 60°C. Specific leaf area (SLA) was obtained by dividing LA by leaf dry mass. Then, bulked dried leaf materials were finely ground for subsequent analyses.
Elemental analyzer (Vario Max CN Element Analyser, Elementar, Hanau, Germany) was used to determine bulk-leaf nitrogen (N) content. Leaf N per unit area (Narea) was calculated from N content and SLA. Elemental analyzer (Model Flash 2000HT, Thermo Fisher Scientific, Bremen, Germany) coupled to an isotope ratio mass spectrometer in continuous-flow mode (Model 253 plus, Thermo Fisher Scientific, Bremen, Germany) was used to analyze carbon (δ13C) and oxygen (δ18O) isotopes of bulk leaf samples. Isotope ratios are expressed as per mil deviations relative to Vienna Pee Dee Belemnite standard, VPDB for δ13C and VSMOW for δ18O. Long-term precision for the instrument were <0.1‰ for δ13C and <0.2‰ for δ18O.
There were 519 and 402 species with bulk leaf δ13C < −20‰ (C3 species) (Supplementary Table S1), and 55 and 31 species with bulk leaf δ13C > −20‰ (C4 species), respectively, in LP and MP. In generally, the iWUE of C4 species is usually several times higher than that of the C3 species (Pinto et al., 2014), and the iWUE of the C3 and C4 species is not distributed continuously when put together. Consequently, previous studies often investigated C3 and C4 species separately when analyzing plant water use strategies (Cornwell et al., 2018; Rumman et al., 2018). Given that the number of C4 species in the two transects is relatively small, we only selected C3 species to elucidated the patterns and underlying mechanisms of iWUE distribution in coexisting species along aridity gradient.
Time-integrated leaf intrinsic water use efficiency (iWUE, μmol mol−1) during the growing season was derived from bulk leaf δ13C based on the well-established theory linking CO2 partial pressure of leaf intercellular space (Ci) to ambient (Ca) (Ci/Ca) with carbon isotopic carbon discrimination (Δ13C) during photosynthesis (Farquhar et al., 1989; Guerrieri et al., 2019). According to Fick's first law of diffusion, iWUE is linearly related to Ci/Ca, and can be expressed as:
where A is the photosynthetic rate; gs is the stomatal conductance of CO2. The Δ13C can be calculated as Farquhar et al. (1989):
where, δ13Ca is δ13C values of atmospheric CO2; δ13CL is δ13C values of bulk-leaf tissue. According to the “simple” form of isotopic discrimination that does not include effects due to mesophyll conductance and photorespiration (Farquhar et al., 1989; Guerrieri et al., 2019), Ci/Ca can be expressed as:
where a is the isotope fractionation constant during CO2 diffusion through stomata (4.4‰); b is the isotope fractionation constant during fixation by Rubisco (27‰). Combining Equation (1) and (3), iWUE can be calculated as follows:
Oxygen isotope enrichment of leaf tissue above source water (Δ18O) was calculated using
Equation (5) (Barbour, 2007):
where δ18OL and δ18Os are oxygen isotope values of bulk leaf and source water, respectively. We assumed that δ18O in precipitation reflects that of soil water (i.e., source water), modified by evaporation (Guerrieri et al., 2019).
Climate variables for 1970–2000 were extracted from the meteorological database of the WorldClim at 0.1° spatial resolution (https://www.worldclim.org/). Mean annual precipitation and temperature, growing season (April to October) precipitation, temperature and actual water vapor pressure were calculated from monthly values. Aridity was obtained from CGIAR-CSI (https://cgiarcsi.community). Data for soil moisture (SM) (at ~10 cm depth) were obtained from a remote-sensing-based surface soil moisture dataset at 0.1° spatial resolution, and ~10-day temporal resolution (Chen et al., 2021). Vapor pressure deficit (VPD) was derived from actual water vapor pressure and temperature (Grossiord et al., 2020). Soil total N content (TN), and the ratio of soil organic carbon to TN (SOC/TN) were obtained from the “Functional Trait database of terrestrial ecosystems in China (China_Trait).”
Blomberg's K values were used to evaluate the phylogenetic signal of iWUE (Blomberg et al., 2003) using “phytools” and “Picante” package in R software (version 3.5.1, R Development Core version Team, Vienna, Austria), because phylogenetic relationships are an important source of inter-specific differences in leaf functional traits. A significant phylogenetic signal (P < 0.05) and large K value indicated that iWUE was constrained by phylogeny (Blomberg et al., 2003). Our results showed that no significant phylogenetic signals of iWUE were observed, and the Blomberg's K values were small (Supplementary Table S2).
Normality of iWUE was tested using the Shapiro–Wilk test (SPSS, Chicago, IL, USA). One-way ANOVA with Duncan's post hoc multiple comparisons was used to compare the differences in iWUE among transects and communities, and to partition variances in iWUE within-site from among site (SPSS, Chicago, IL, USA). Multivariate analysis of covariance was used to partition the contribution of sampling site, species and their interaction to variances in iWUE (SPSS, Chicago, IL, USA).
All iWUE values of C3 species in each sampling site were used to calculate frequency distribution characteristics, such as mean, variance, standard deviation, coefficients of variation, range, skewness and kurtosis (SPSS, Chicago, IL, USA). Simple linear regressions between frequency distribution characteristics of iWUE and aridity were conducted to test for the first hypothesis.
Pearson correlation analyses were used to test bivariate relationships between SOC/TN, SM, VPD and iWUE (SPSS, Chicago, IL). To test for the hypothesis (2), we conducted variation partitioning (R software, version 3.5.1, R Development Core version Team, Vienna, Austria) to quantify the relative contributions of water (SM and VPD) and SOC/TN to the spatial patterns of iWUE along aridity gradients, combining with the results of Pearson correlation analyses.
Simple linear regressions between iWUE and leaf functional traits (Δ18O, SLA and Narea) were conducted (R2018b, MathWorks, Inc). The result of simple linear regressions between iWUE and Δ18O was used to test for hypothesis (3).
A multivariate associations among iWUE, Δ18O, SLA, and Narea were analyzed with principal component analysis (PCA) (R2018b, MathWorks, Inc) to understand the role of iWUE and Δ18O in multidimensional leaf functional traits. Another PCA was performed excluding iWUE and using the remaining three traits (Δ18O, SLA, and Narea). Simple linear regressions between iWUE and axes scores of each species of second PCA were conducted to further explain the drivers of interspecific variation in iWUE along aridity gradient.
Intrinsic water use efficiency (iWUE) was distributed normally across all plant species in Loess (LP) and Inner Mongola (MP) Plateaus (Figures 2A,B). iWUE ranged from 28.17 to 141.97 μmol mol−1 in LP and from 47.30 to 152.00 μmol mol−1 in MP (Supplementary Table S3). iWUE was significantly lower in LP than in MP (P < 0.001).
Figure 2. Frequency distribution of intrinsic water use efficiency (iWUE) in Loess Plateau (LP) (A) and Inner Mongola Plateau (MP) (B).
Within transects, iWUE of co-existing species in each sampling site was distributed normally (P > 0.05), except for one sampling site in MP (P = 0.03) (Supplementary Table S3). iWUE varied widely within and across sampling sites, and over 60% of variability in iWUE originated from within-site (Supplementary Table S4). When treated as categorical variables, variability in iWUE was primarily controlled by species (46 and 63% in LP and MP, respectively), followed by sampling site (30 and 19% in LP and MP, respectively), and the interaction between species and sites (22 and 16% in LP and MP, respectively) (Figure 3). It indicated that interaction of leaf functional traits and environmental factors drove the patterns of iWUE.
Figure 3. Contributions of species (red), site (green), and their interaction (blue) to variation in intrinsic water use efficiency. LP, Loess Pleateau; MP, Inner Mongolia Plateau.
Mean values of iWUE (iWUEMean) significantly increased with aridity in LP and MP (Figures 4A,B). Coefficient of variation (CV) of iWUE (iWUECV) decreased with increasing aridity in LP and MP (Figures 4C,D). However, the variance, standard deviation, range, kurtosis, and skewness of iWUE did not show clear patterns along aridity gradients (Supplementary Table S3).
Figure 4. Patterns of mean value (iWUEmean) (A,B) and coefficient of variation (iWUECV) (C,D) of intrinsic water use efficiency (iWUE) along aridity gradients in Loess Plateau (LP) and Inner Mongola Plateau (MP).
iWUEMean in LP was negatively related to soil moisture (SM) and to the ratio of soil organic carbon content to total nitrogen content (SOC/TN), and positively related to vapor pressure deficit (VPD) (Table 1). SOC/TN, SM, and VPD explained 94% of variation in iWUEMean, and the strongest predictor was SOC/TN (Figure 5A). iWUECV was negatively related to SM and SOC/TN (Table 1). SOC/TN and SM explained 76% of variation in iWUECV, and the strongest predictor was SOC/TN (Figure 5B).
Table 1. Pearson's correlation coefficients between intrinsic water use efficiency and environmental variables in Loess Plateau (LP) and Inner Mongola Plateau (MP).
Figure 5. Variation partitioning (R2) of environmental factors in mean (A,C) and coefficient of variation (CV) (B,D) of iWUE of co-occuring species in LP (A,B) and MP (C,D). LP, Loess Pleateau; MP, Inner Mongolia Plateau; SM, soil moisture; VPD, vapor pressure deficit, VPD; SOC/TN, the ratio of soil organic carbon content to soil total nitrogen content.
In MP, iWUEMean was negatively related to SM and SOC/TN (Table 1). SOC/TN and SM jointly explained 91% of variation in iWUEMean, and the strongest predictor was SM (Figure 5C). iWUECV was positively related to SM (Table 1). SM explained 57% of variation in iWUECV (Figure 5D).
iWUE decreased with specific leaf area (SLA) (P < 0.001) (Figure 6A), and increased with leaf nitrogen per unit area (Narea) (Figure 6B) and with 18O enrichment in leaf water above source water (Δ18O) (Figure 6C).
Figure 6. Relationships between intrinsic water use efficiency and specific leaf area (SLA) (A), leaf nitrogen per unit area (Narea) (B), and the 18O enrichment in leaf water above source water (Δ18O) (C). LP, Loess Pleateau; MP, Inner Mongolia Plateau.
Principal component analysis (PCA) was conducted to quantify the effect of plant functional trait combinations on inter-specific variability in iWUE. The dominant leaf functional traits of Axis1 were SLA, Narea, Δ18O, and iWUE in LP (Figure 7A and Supplementary Table S5) and MP (Figure 7B and Supplementary Table S5). Axis2 was mainly loaded by Narea and iWUE in LP (Figure 7A), and SLA, Δ18O, and iWUE in MP (Figure 7B). Variability in iWUE was mainly controlled by Axis1 (r2 = 0.29) in LP (Figure 7C), and by Axis1 (r2 = 0.30) and Axis2 (r2 = 0.18) in MP (Figure 7D).
Figure 7. Principal component analysis (PCA) of intrinsic water use efficiency (iWUE) and leaf functional traits (A,B) and relationship between the principal component axes of PCA of leaf functional traits and iWUE (C,D). SLA, specific leaf area; Narea, leaf nitrogen per unit area, and Δ18O, the 18O enrichment in leaf water above source water. LP, Loess Pleateau; MP, Inner Mongolia Plateau.
Species in LP and MP adopt opportunistic water use strategies to cope with water- and nutrient-limiting habitats (Figure 2). Time-integrated iWUE in LP and MP were within the range but distributed at a relatively lower end of global values. For example, two multi-species datasets show that the instantaneous iWUE ranged from 5 to 324.00 μmol mol−1 (Flexas et al., 2016; Gago et al., 2016). Furthermore, leaf δ13C of species in this study were also distributed at the relatively depleted end of the global δ13C dataset (Supplementary Figure S1). The reason may be that an opportunistic water use strategy is advantageous for maximizing nutrient capture (Querejeta et al., 2018; Salazar-Tortosa et al., 2018). Water and nutrient supply in arid and semi-arid habitats are highly heterogeneous in space and time due to the limited and variable rainfall (Chesson et al., 2004). Nutrient uptake from soil is tightly linked to the transpiration-driven mass flow of water (Salazar-Tortosa et al., 2018). Species with low iWUE always exhibit high stomatal conductance (gs) and transpiration rate (Moreno-Gutierrez et al., 2012), allowing rapid nutrient absorption when soil water and nutrients are available.
Within transect, we found that iWUE of co-occuring species in each sampling site exhibited high variability, however, functional convergence was observed along the aridity gradient (Figures 4C,D). This result is contrary to our first hypothesis. Species with diverse and contrasting water use stratergies are known to coexist in dry and nutrient-poor habitats, such as Karst (Wang et al., 2021), Mediterranean (Moreno-Gutierrez et al., 2012), coastal sand dunes (Bermúdez and Retuerto, 2014) and epiphytic (Querejeta et al., 2018) ecosystems. This demonstrates that species differences in eco-physiological function related to the use of resources facilitate species niche segregation (Moreno-Gutierrez et al., 2012; Bermúdez and Retuerto, 2014). There are two mechanisms that might explain the convergent water use strategies along aridity gradient: (1) Inter-specific competition decreased with increasing aridity (Wu et al., 2017). As the number of species (plant species richness) occuring within each community significantly decreases (Supplementary Table S1), available resources for each species may increase (Zhu et al., 2015). (2) The filtering effect of water stress on iWUE of species increased with increasing aridity. The reason is that species with similar physiological parameters can enter a given harsh habitats (Reich et al., 2003; Maracahipes et al., 2018). These results demonsteated that environmental filtering existed within transect, although species exhibited diverse water use strategies in water and nutrient-limited LP and MP.
Futhermore, we also found that co-occuring species within a sampling site shifted their water use strategies from opportunistic (low iWUE and high gs) to conservative (low iWUE and high gs) with an increase in aridity in LP and MP (Figures 4A,B), consistent with previous studies (Zheng and Shangguan, 2007; Liu et al., 2013; Wang et al., 2016; Ale et al., 2018). This reveals that plants lowered their water loss to adopt to the increasing water stress (i.e., low soil moisture and high water vapor pressure deficit) (Supplementary Table S3). However, the slope of the relationship between aridity and mean values of iWUE (iWUEmean) in MP (Figure 4B, slope = 102.304) was steeper than that in LP (Figure 4A, slope = 188.901). This indicates that the negative effect of water stress on iWUE is higher in MP than in LP. We attribute this difference to dissimilarity in limited resources between the two transects.
The variability in iWUEmean along an aridity gradient was mainly determined by SOC/TN in LP, and by soil moisture in MP (Figure 5), which supports our second hypothesis. A previous study conducted in this study area demonstrated that plant N availability in LP was lower than that in MP, and increased with aridity in MP, but showed no clear trend in LP (Unpublished data). These results demonstrate that high plant N availability could meet N demand to reduce water cost across the transect in MP (high iWUE and low gs); however, plants in LP promoted N uptake to meet plant N demand at the expense of high water loss (low iWUE and high gs) (Wright et al., 2003). Our findings highlight the importance of balance between acquisitive and conservative strategies along the water and nitrogen gradients: opportunistic water use strategies allows species to established in habits with low soil N habitats due to their high nitorgen acquisition capacity, while conservative water use strategies promote resistance of species to water stress in relatively high soil N habitats (Wright et al., 2003; Maracahipes et al., 2018).
Previous studies demonstrated that Δ18O in co-existing species can be used as a reliable indicator of interspecific differences in gs in strongly water-limited ecosystems, and that leaf δ13C and δ18O can be used to separate the independent effects of photosynthetic rates and gs on iWUE (Moreno-Gutierrez et al., 2012; Querejeta et al., 2018). However, the effects of climatic (vapor pressure deficit [VPD] and temperature) variability and differences in δ18O of plant source water on leaf δ18O (Δ18O) should be considered across large spatial scales (Prieto et al., 2018). In this study, mean values of Δ18O in C3 species within a sampling site was not affected by VPD or temperature (P > 0.05) (Supplementary Table S6). The effect of source water across sites was corrected using δ18O of precipitation at each site (Maxwell et al., 2018). However, the water source partitioning in C3 species in each sampling site may attenuate the positive correlation between iWUE and Δ18O. The slopes of the linear regression between Δ18O and iWUE across sites within transect did not differ statistically (Supplementary Figure S2 and Supplementary Table S7). Furthermore, water-source partitioning is not common in grassland communities (Bachmann et al., 2015). Consequently, differences in Δ18O in co-occurring species within communities should be determined mainly by the interspecific variation in gs (Wang and Wen, 2022).
A positive relationship was found between Δ18O and iWUE both within (Supplementary Figure S2 and Supplementary Table S7) and among sampling sites (Figure 6B), indicating that the variability in iWUE was determined mainly by stomatal regulation (Moreno-Gutierrez et al., 2012; Prieto et al., 2018; Querejeta et al., 2018). This conclusion supported our third hypothesis. Moreno-Gutierrez et al. (2012) found that the large interspecific difference in δ13C in a Mediterranean ecosystem was controlled by Δ18O rather than photosynthetic rate. However, in this study, a positive relationship between leaf nitrogen per unit area (Narea) and iWUE indicated that variability in iWUE was also affected by the interspecific variation in photosynthetic rate (Figure 6B), and that species invested more N to compensate for the negative effect of low gs on photosynthetic rates (Flexas et al., 2016). A previous study demonstrated that species with low leaf-specific leaf areas (SLA) had small and thick leaves, which benefit reductions in water loss (Wright et al., 2017). The negative relationship between iWUE and SLA demonstrated that species increased construction costs to prevent water loss (Maxwell et al., 2018).
In this study, the covariation of iWUE, Δ18O, SLA and Narea in LP and MP (Figure 7) demonstrates that water loss and carbon gain processes were tightly coupled in the study area. It also shows that iWUE and Δ18O can be included in the traditional leaf economic spectrum in drylands (Reich, 2014; Prieto et al., 2018). This leaf economic spectrum defined a water use strategy gradient from conservative (high iWUE and low gs) with high Narea to profligate (low iWUE and high gs) with high SLA (Prieto et al., 2018). These results revealed that water losses and carbon gain processes in arid and semi-arid regions are tightly coupled (Prieto et al., 2018; Yin et al., 2018; Shi et al., 2021). A second dimension of trait space was observed in LP (Narea) (Figure 7A) and MP (SLA and Δ18O) (Figure 7B), demonstrating that the most limiting resource was N in LP and water in MP (Wang et al., 2021). The limiting-resource spectrum differentiated species along a uniform gradient from resource acquisition to high resource conservative traits (Baltzer and Thomas, 2010; Bermúdez and Retuerto, 2014). Variability in iWUE was mainly controlled by the leaf economic spectrum in LP, and by both—leaf economic spectrum and limiting resource spectrum in MP. This supports the idea that species evolved species-specific strategies to adapt to harsh habitats by partitioning limiting resources (Bermúdez and Retuerto, 2014).
In this study, we found that species adopted opportunistic water use strategies to adapt to water- and N-limited habitats in arid and semi-arid regions. Variability in iWUE was primarily controlled by species, followed by sampling site, and the interaction between species and sampling site. Within transect, co-occurring species within a sampling site shifted their water use strategies from opportunistic (low iWUE and high gs) to conservative (low iWUE and high gs) with an increase in aridity in LP and MP, and water use strategies of co-occurring species gradually converged. This distribution pattern was driven mainly by the most limited resource, i.e., SOC/TN in LP, and soil moisture in MP. High variability in iWUE mainly determined by stomatal regulation. Furthermore, variability in iWUE was mainly controlled by leaf economic spectrum in LP, and by both—leaf economic spectrum and limiting resource spectrum in MP, indicating that species evolved species-specific strategies to adapt to a limiting habitat by partitioning limiting resources. This study demonstrated that the limiting resource and leaf functional traits jointly determined the distribution patterns of water use strategies of species in LP and MP along an aridity gradient, and emphasized the importance of considering biological processes in dissecting the underlying mechanisms of plant adaptation strategies at large regional scales.
The original contributions presented in the study are included in the article/Supplementary material, further inquiries can be directed to the corresponding author/s.
JW and XW conceived and designed the research. JW conducted stable isotope measurements, data analysis, and wrote the manuscript. XW revised the manuscript. All authors contributed to the article and approved the submitted version.
This work was funded by the Strategic Priority Research Program of the Chinese Academy of Sciences (XDA23070202) and National Natural Science Foundation of China (Grant No. 41991234).
We thank the members in grassland transect investigation for field sampling, and Functional Trait database of terrestrial ecosystems in China (China_Traits) for sharing all auxiliary plant and soil data.
The authors declare that the research was conducted in the absence of any commercial or financial relationships that could be construed as a potential conflict of interest.
All claims expressed in this article are solely those of the authors and do not necessarily represent those of their affiliated organizations, or those of the publisher, the editors and the reviewers. Any product that may be evaluated in this article, or claim that may be made by its manufacturer, is not guaranteed or endorsed by the publisher.
The Supplementary Material for this article can be found online at: https://www.frontiersin.org/articles/10.3389/fpls.2022.909603/full#supplementary-material
Ale, R., Zhang, L., Li, X., Raskoti, B. B., Pugnaire, F. I., and Luo, T. X. (2018). Leaf delta C-13 as an indicator of water availability along elevation gradients in the dry Himalayas. Ecol. Indic. 94, 266–273. doi: 10.1016/j.ecolind.2018.07.002
Aparecido, L. M. T., Woo, S., Suazo, C., Hultine, K. R., and Blonder, B. (2020). High water use in desert plants exposed to extreme heat. Ecol. Lett. 23, 1189–1200. doi: 10.1111/ele.13516
Bachmann, D., Gockele, A., Ravenek, J. M., Roscher, C., Strecker, T., Weigelt, A., et al. (2015). No evidence of complementary water use along a plant species richness gradient in temperate experimental grasslands. PLoS ONE 10,e0116367. doi: 10.1371/journal.pone.0116367
Bahar, N. H., Ishida, F. Y., Weerasinghe, L. K., Guerrieri, R., O'Sullivan, O. S., Bloomfield, K. J., et al. (2016). Leaf-level photosynthetic capacity in lowland Amazonian and high-elevation Andean tropical moist forests of Peru. New Phytol. 214, 903–904. doi: 10.1111/nph.14079
Baltzer, J. L., and Thomas, S. C. (2010). A second dimension to the leaf economics spectrum predicts edaphic habitat association in a tropical forest. PLoS ONE 5:7. doi: 10.1371/journal.pone.0013163
Barbour, M. (2007). Stable oxygen isotope composition of plant tissue: a review. Funct. Plant Biol. 34, 83–94. doi: 10.1071/FP06228
Basu, S., Ghosh, S., and Chattopadhyay, D. (2021). Disentangling the abiotic versus biotic controls on C3 plant leaf carbon isotopes: inferences from a global review. Earth Sci. Rev. 222,103839. doi: 10.1016/j.earscirev.2021.103839
Bermúdez, R., and Retuerto, R. (2014). Together but different: co-occurring dune plant species differ in their water- and nitrogen-use strategies. Oecologia 174, 651–663. doi: 10.1007/s00442-013-2820-7
Blomberg, S. P., Garland, T., and Ives, A. R. (2003). Testing for phylogenetic signal in comparative data: behavioral traits are more labile. Evolution 57, 717–745. doi: 10.1111/j.0014-3820.2003.tb00285.x
Chen, Y., Feng, X., and Fu, B. (2021). An improved global remote-sensing-based surface soil moisture (RSSSM) dataset covering 2003–2018. Earth Syst. Sci. Data 13, 1–31. doi: 10.5194/essd-13-1-2021
Chesson, P., Gebauer, R. L. E., Schwinning, S., Huntly, N., Wiegand, K., Ernest, M. S. K., et al. (2004). Resource pulses, species interactions, and diversity maintenance in arid and semi-arid environments. Oecologia 141, 236–253. doi: 10.1007/s00442-004-1551-1
Choat, B., Brodribb, T. J., Brodersen, C. R., Duursma, R. A., Lopez, R., and Medlyn, B. E. (2018). Triggers of tree mortality under drought. Nature 558, 531–539. doi: 10.1038/s41586-018-0240-x
Cornwell, W. K., Wright, I. J., Turner, J., Maire, V., Barbour, M. M., Cernusak, L. A., et al. (2018). Climate and soils together regulate photosynthetic carbon isotope discrimination within C3 plants worldwide. Glob. Ecol. Biogeogr. 27, 1056–1067. doi: 10.1111/geb.12764
Farquhar, G., Ehleringer, J., and Hubick, K. (1989). Carbon isotope discrimination and photosynthesis. Annu. Rev. Plant Physiol. Plant Mol. Biol. 40, 503–537. doi: 10.1146/annurev.pp.40.060189.002443
Farquhar, G. D., Cernusak, L. A., and Barnes, B. (2007). Heavy water fractionation during transpiration. Plant Physiol. 143, 11–18. doi: 10.1104/pp.106.093278
Feyissa, A., Yang, F., Wu, J. J., Chen, Q., Zhang, D. D., and Cheng, X. L. (2021). Soil nitrogen dynamics at a regional scale along a precipitation gradient in secondary grassland of China. Sci. Total Environ. 781,146736. doi: 10.1016/j.scitotenv.2021.146736
Flexas, J., Díaz-Espejo, A., Conesa, M. A., Coopman, R. E., Douthe, C., Gago, J., et al. (2016). Mesophyll conductance to CO2 and Rubisco as targets for improving intrinsic water use efficiency in C-3 plants. Plant Cell Environ. 39, 965–982. doi: 10.1111/pce.12622
Gago, J., Daloso, D. D., Figueroa, C. M., Flexas, J., Fernie, A. R., and Nikoloski, Z. (2016). Relationships of leaf net photosynthesis, stomatal conductance, and mesophyll conductance to primary metabolism: a multispecies meta-analysis approach. Plant Physiol. 171, 265–279. doi: 10.1104/pp.15.01660
Galmes, J., Medrano, H., and Flexas, J. (2007). Photosynthetic limitations in response to water stress and recovery in Mediterranean plants with different growth forms. New Phytol. 175, 81–93. doi: 10.1111/j.1469-8137.2007.02087.x
Grossiord, C., Buckley, T. N., Cernusak, L. A., Novick, K. A., Poulter, B., Siegwolf, R. T. W., et al. (2020). Plant responses to rising vapor pressure deficit. New Phytol. 226, 1550–1566. doi: 10.1111/nph.16485
Guerrieri, R., Belmecheri, S., Ollinger, S. V., Asbjornsen, H., Jennings, K., Xiao, J. F., et al. (2019). Disentangling the role of photosynthesis and stomatal conductance on rising forest water-use efficiency. Proc. Natl. Acad. Sci. U. S. A. 116, 16909–16914. doi: 10.1073/pnas.1905912116
Guo, X. C., Shi, P. J., Niinemets, U., Holscher, D., Wang, R., Liu, M. D., et al. (2021). “Diminishing returns” for leaves of five age-groups of Phyllostachys edulis culms. Am. J. Bot. 108, 1662–1672. doi: 10.1002/ajb2.1738
Li, Y., Shi, P., Niinemets, Ü., Song, Y., Yu, K., Schrader, J., et al. (2022). Diminishing returns among lamina fresh and dry mass, surface area, and petiole fresh mass among nine Lauraceae species. Am. J. Bot. 109, 377–392. doi: 10.1002/ajb2.1812
Liu, Y. J., Niu, H. S., and Xu, X. L. (2013). Foliar delta C-13 response patterns along a moisture gradient arising from genetic variation and phenotypic plasticity in grassland species of Inner Mongolia. Ecol. Evol. 3, 262–267. doi: 10.1002/ece3.453
Lyu, S. D., Wang, J., Song, X. W., and Wen, X. F. (2021). The relationship of δD and δ18O in surface soil water and its implications for soil evaporation along grass transects of Tibet, Loess, and Inner Mongolia Plateau. J. Hydrol. 600. doi: 10.1016/j.jhydrol.2021.126533
Maracahipes, L., Carlucci, M. B., Lenza, E., Marimon, B. S., Marimon, B. H., Guimarães, F. A. G., et al. (2018). How to live in contrasting habitats? Acquisitive and conservative strategies emerge at inter- and intraspecific levels in savanna and forest woody plants. Perspect. Plant Ecol. Evol. Syst. 34, 17–25. doi: 10.1016/j.ppees.2018.07.006
Martin-StPaul, N., Delzon, S., and Cochard, H. (2017). Plant resistance to drought depends on timely stomatal closure. Ecol. Lett. 20, 1437–1447. doi: 10.1111/ele.12851
Maxwell, T., Silva, L., and Horwath, W. (2018). Integrating effects of species composition and soil properties to predict shifts in montane forest carbon-water relations. Proc. Natl. Acad. Sci. U. S. A. 115, E4219–E4226. doi: 10.1073/pnas.1718864115
Mitchell, R. M., Wright, J. P., and Ames, G. M. (2018). Species' traits do not converge on optimum values in preferred habitats. Oecologia 186, 719–729. doi: 10.1007/s00442-017-4041-y
Moreno-Gutierrez, C., Dawson, T. E., Nicolas, E., and Querejeta, J. I. (2012). Isotopes reveal contrasting water use strategies among coexisting plant species in a Mediterranean ecosystem. New Phytol. 196, 489–496. doi: 10.1111/j.1469-8137.2012.04276.x
Pinto, H., Sharwood, R. E., Tissue, D. T., and Ghannoum, O. (2014). Photosynthesis of C3, C3-C4, and C4 grasses at glacial CO2. J. Exp. Bot. 65, 3669–3681. doi: 10.1093/jxb/eru155
Prentice, I. C., Dong, N., Gleason, S. M., Maire, V., and Wright, I. J. (2014). Balancing the costs of carbon gain and water transport: testing a new theoretical framework for plant functional ecology. Ecol. Lett. 17, 82–91. doi: 10.1111/ele.12211
Prieto, I., Querejeta, J., Segrestin, J., Volaire, F., and Roumet, C. (2018). Leaf carbon and oxygen isotopes are coordinated with the leaf economics spectrum in Mediterranean rangeland species. Funct. Ecol. 32, 612–625. doi: 10.1111/1365-2435.13025
Querejeta, J. I., Iván, P., Torres, P., Campoya, M., Alguacila, M. M., and Roldán, A. (2018). Water-spender strategy is linked to higher leaf nutrient concentrations across plant species colonizing a dry and nutrient-poor epiphytic habitat. Environ. Exp. Bot. 153, 302–310. doi: 10.1016/j.envexpbot.2018.06.007
Reich, P. (2014). The world-wide ‘fast-slow' plant economics spectrum: a traits manifesto. J. Ecol. 102, 275–301. doi: 10.1111/1365-2745.12211
Reich, P. B., Buschena, C., Tjoelker, M. G., Wrage, K., Knops, J., Tilman, D., et al. (2003). Variation in growth rate and ecophysiology among 34 grassland and savanna species under contrasting N supply: a test of functional group differences. New Phytol. 157, 617–631. doi: 10.1046/j.1469-8137.2003.00703.x
Ren, T., He, N., Liu, Z., Li, M., Zhang, J., Li, A., et al. (2021). Environmental filtering rather than phylogeny determines plant leaf size in three floristically distinctive plateaus. Ecol. Indic. 130,108049. doi: 10.1016/j.ecolind.2021.108049
Reynolds, J. F., Stafford Smith, D. M., Lambin, E. F., Turner, B. L., Mortimore, M., Batterbury, S. P. J., et al. (2007). Global desertification: building a science for dryland development. Science 316, 847–851. doi: 10.1126/science.1131634
Roden, J. S., and Farquhar, G. D. (2012). A controlled test of the dual-isotope approach for the interpretation of stable carbon and oxygen isotope ratio variation in tree rings. Tree Physiol. 32, 490–503. doi: 10.1093/treephys/tps019
Rumman, R., Atkin, O. K., Bloomfield, K. J., and Eamus, D. (2018). Variation in bulk-leaf 13C discrimination, leaf traits and water-use efficiency-trait relationships along a continental-scale climate gradient in Australia. Glob. Change Biol. 24, 1186–1200. doi: 10.1111/gcb.13911
Salazar-Tortosa, D., Castro, J., Villar-Salvador, P., Vinegla, B., Matias, L., Michelsen, A., et al. (2018). The “isohydric trap”: a proposed feedback between water shortage, stomatal regulation, and nutrient acquisition drives differential growth and survival of European pines under climatic dryness. Glob. Change Biol. 24, 4069–4083. doi: 10.1111/gcb.14311
Scheidegger, Y., Saurer, M., Bahn, M., and Siegwolf, R. (2000). Linking stable oxygen and carbon isotopes with stomatal conductance and photosynthetic capacity: a conceptual model. Oecologia 125, 350–357. doi: 10.1007/s004420000466
Schneider, C. A., Rasband, W. S., and Eliceiri, K. W. (2012). NIH Image to ImageJ: 25 years of image analysis. Nat. Methods 9, 671–675. doi: 10.1038/nmeth.2089
Shi, P. J., Jiao, Y. B., Diggle, P. J., Turner, R., Wang, R., and Niinemets, U. (2021). Spatial distribution characteristics of stomata at the areole level in Michelia cavaleriei var. platypetala (Magnoliaceae). Ann. Bot. 128, 875–885. doi: 10.1093/aob/mcab106
Tang, S. B., Lai, Y., Tang, X. L., Phillips, O. L., Liu, J. F., Chen, D. X., et al. (2021). Multiple environmental factors regulate the large-scale patterns of plant water use efficiency and nitrogen availability across China's forests. Environ. Res. Lett. 16,10. doi: 10.1088/1748-9326/abe3bb
Utkhao, W., and Yingjajaval, S. (2015). Changes in leaf gas exchange and biomass of Eucalyptus camaldulensis in response to increasing drought stress induced by polyethylene glycol. Trees Struct. Funct. 29, 1581–1592. doi: 10.1007/s00468-015-1240-1
Wang, C., Liu, D. W., Luo, W. T., Fang, Y. T., Wang, X. B., Lu, X. T., et al. (2016). Variations in leaf carbon isotope composition along an arid and semi-arid grassland transect in northern China. J. Plant Ecol. 9, 576–585. doi: 10.1093/jpe/rtw006
Wang, J., and Wen, X. (2022). Divergence and conservative of stomatal conductance in coexisting species in response to climatic stress in Tibetan Plateau. Ecol. Indic. 138,108843. doi: 10.1016/j.ecolind.2022.108843
Wang, J., Wen, X., Zhang, X., and Li, S. (2018). The strategies of water–carbon regulation of plants in a subtropical primary forest on karst soils in China. Biogeosciences 15, 4193–4203. doi: 10.5194/bg-15-4193-2018
Wang, J., Wen, X. F., Lyu, S. D., and Guo, Q. J. (2021). Transition in multi-dimensional leaf traits and their controls on water use strategies of co-occurring species along a soil limiting-resource gradient. Ecol. Indic. 128,107838. doi: 10.1016/j.ecolind.2021.107838
Wright, I. J., Dong, N., Maire, V., Prentice, I. C., Westoby, M., Diaz, S., et al. (2017). Global climatic drivers of leaf size. Science 357,917–21. doi: 10.1126/science.aal4760
Wright, I. J., Reich, P. B., and Westoby, M. (2003). Least-cost input mixtures of water and nitrogen for photosynthesis. Am. Nat. 161, 98–111. doi: 10.1086/344920
Wu, J., Chavana-Bryant, C., Prohaska, N., Serbin, S. P., Guan, K. Y., Albert, L. P., et al. (2017). Convergence in relationships between leaf traits, spectra and age across diverse canopy environments and two contrasting tropical forests. New Phytol. 214, 1033–1048. doi: 10.1111/nph.14051
Yao, J. Y., Liu, H. P., Huang, J. P., Gao, Z. M., Wang, G. Y., Li, D., et al. (2020). Accelerated dryland expansion regulates future variability in dryland gross primary production. Nat. Commun. 11,10. doi: 10.1038/s41467-020-15515-2
Yin, Q., Wang, L., Lei, M., Dang, H., Quan, J., Tian, T., et al. (2018). The relationships between leaf economics and hydraulic traits of woody plants depend on water availability. Sci. Total Environ. 621, 245–252. doi: 10.1016/j.scitotenv.2017.11.171
Yue, P., Zhang, Q., Zhang, L., Li, H. Y., Yang, Y., Zeng, J., et al. (2019). Long-term variations in energy partitioning and evapotranspiration in a semiarid grassland in the Loess Plateau of China. Agric. For. Meteorol. 278,107671. doi: 10.1016/j.agrformet.2019.107671
Zhang, J., He, N., Liu, C., Xu, L., Chen, Z., Li, Y., et al. (2019). Variation and evolution of C:N ratio among different organs enable plants to adapt to N-limited environments. Glob. Change Biol. 26, 2534–2543. doi: 10.1111/gcb.14973
Zheng, S. X., and Shangguan, Z. P. (2007). Spatial patterns of foliar stable carbon isotope compositions of C-3 plant species in the Loess Plateau of China. Ecol. Res. 22, 342–353. doi: 10.1007/s11284-006-0024-x
Keywords: intrinsic water use efficiency, carbon stable isotope, oxygen stable isotope, environment filter, leaf economic spectrum
Citation: Wang J and Wen X (2022) Limiting resource and leaf functional traits jointly determine distribution patterns of leaf intrinsic water use efficiency along aridity gradients. Front. Plant Sci. 13:909603. doi: 10.3389/fpls.2022.909603
Received: 31 March 2022; Accepted: 29 June 2022;
Published: 29 July 2022.
Edited by:
Peijian Shi, Nanjing Forestry University, ChinaReviewed by:
Pedro Manuel Villa, Universidade Federal de Viçosa, BrazilCopyright © 2022 Wang and Wen. This is an open-access article distributed under the terms of the Creative Commons Attribution License (CC BY). The use, distribution or reproduction in other forums is permitted, provided the original author(s) and the copyright owner(s) are credited and that the original publication in this journal is cited, in accordance with accepted academic practice. No use, distribution or reproduction is permitted which does not comply with these terms.
*Correspondence: Jing Wang, d2FuZ2ppbmcuMTViQGlnc25yci5hYy5jbg==; Xuefa Wen, d2VueGZAaWdzbnJyLmFjLmNu
Disclaimer: All claims expressed in this article are solely those of the authors and do not necessarily represent those of their affiliated organizations, or those of the publisher, the editors and the reviewers. Any product that may be evaluated in this article or claim that may be made by its manufacturer is not guaranteed or endorsed by the publisher.
Research integrity at Frontiers
Learn more about the work of our research integrity team to safeguard the quality of each article we publish.