- 1Department of Soils and Water, Faculty of Agriculture, Fayoum University, Fayoum, Egypt
- 2Department of Botany and Microbiology, Faculty of Science, Zagazig University, Zagazig, Egypt
- 3Department of Agricultural Microbiology, Faculty of Agriculture, Zagazig University, Zagazig, Egypt
- 4Department of Biology, College of Science, United Arab Emirates University, Al-Ain, United Arab Emirates
- 5Khalifa Center for Genetic Engineering and Biotechnology, United Arab Emirates University, Al-Ain, United Arab Emirates
- 6Harry Butler Institute, Murdoch University, Murdoch, WA, Australia
The exogenous application of osmoprotectants [e.g., proline (Pro)] is an important approach for alleviating the adverse effects of abiotic stresses on plants. Field trials were conducted during the summers of 2017 and 2018 to determine the effects of deficit irrigation and exogenous application of Pro on the productivity, morph-physiological responses, and yield of maize grown under two irrigation systems [surface irrigation (SI) and drip irrigation (DI)]. Three deficit irrigation levels (I100, I85, and I70, representing 100, 85, and 70% of crop evapotranspiration, respectively) and two concentrations of Pro (Pro1 = 2 mM and Pro2 = 4 mM) were used in this study. The plants exposed to drought stress showed a significant reduction in plant height, dry matter, leaf area, chlorophyll content [soil plant analysis development (SPAD)], quantum efficiency of photosystem II [Fv/Fm, Fv/F0, and performance index (PI)], water status [membrane stability index (MSI) and relative water content (RWC)], and grain yield. The DI system increased crop growth and yield and reduced the irrigation water input by 30% compared with the SI system. The growth, water status, and yield of plants significantly decreased with an increase in the water stress levels under the SI system. Under the irrigation systems tested in this study, Pro1 and Pro2 increased plant height by 16 and 18%, RWC by 7 and 10%, MSI by 6 and 12%, PI by 6 and 19%, chlorophyll fluorescence by 7 and 11%, relative chlorophyll content by 9 and 14%, and grain yield by 10 and 14%, respectively, compared with Pro0 control treatment (no Pro). The interaction of Pro2 at I100 irrigation level in DI resulted in the highest grain yield (8.42 t ha–1). However, under the DI or SI system, exogenously applied Pro2 at I85 irrigation level may be effective in achieving higher water productivity and yield without exerting any harmful effects on the growth or yield of maize under limited water conditions. Our results demonstrated the importance of the application of Pro as a tolerance inducer of drought stress in maize.
Introduction
Maize is one of the main cereal crops worldwide, followed by wheat, which is a staple food in Egypt (OECD/FAO, 2020). It is either consumed freshly or indirectly as corn oil, starch, fructose, glucose, and livestock feed (Ranum et al., 2014). Owing to its low inherent production and high demand of maize, Egypt has become the fourth largest importer of maize in the world (OECD/FAO, 2020). The total maize production in Egypt is approximately 6.4 million tons, and 85,000 hectares of cultivated land is estimated for its production. Its consumption is approximately 16.1 million tons with a self-sufficiency ratio of 42% (Ibrahim et al., 2007). Thus, to minimize the gap between maize production and consumption, it is important to manage irrigation water more efficiently and enhance domestic maize production by following non-traditional procedures, such as growing in cultivated areas or planting high-yield varieties (Ouda et al., 2017).
In arid and semi-arid regions, water shortage particularly affects food security (Misra, 2014; Mancosu et al., 2015). Approximately 70–80% of the available freshwater is required for agriculture (Food and Agriculture Organization [FAO], 2017). Egypt depends on limited water resources from the Nile River (55 billion m3 of water/year) (MWRI, 2014). Over 95% of irrigated lands in Egypt are managed using surface irrigation (SI) systems, with a low irrigation efficiency of 45–50% (Osman et al., 2016; Abdou et al., 2021; Ali et al., 2021). Therefore, rational water governance seeks to reduce water losses and enhance crop productivity to withstand high evaporative demand. Assuming severe water supply shortages, a deficit and highly efficient irrigation strategy of drip irrigation (DI) system is highly recommended over the SI system to increase the benefits per unit of water.
The application of irrigation water below the evapotranspiration (ET) demand is known as deficit irrigation, which optimizes economic output when there is limited water supply (Pereira et al., 2012; Chai et al., 2016). Plants under deficit irrigation systems receive less irrigation water than the actual amount of water required at plant growth stages and/or during the total crop cultivation period (Badal et al., 2013). Hence, plants are exposed to water stress to some extent under deficit irrigation systems (Wakchaure et al., 2018; Parkash and Singh, 2020). A decrease in the ET rate of plants exposed to water stress results in severe water stress symptoms, such as leaf rolling, diminishing leaf area, and stunted growth and yield of plants (Wang et al., 2014). Drought stress induces several physiological, biochemical, and photosynthetic changes by regulating stomatal closure or controlling CO2 flow into the mesophyll tissues (Yuan et al., 2016).
Approximately 20–25% of the maize cultivation areas are affected by drought globally (Shahrokhi et al., 2020). Sensitivity to drought stress can lead to dramatic fluctuations in maize yield, which is a common condition in Egypt (Gomaa et al., 2017). Maize exhibits distinct responses to water deficit depending on the developmental stage, crop tolerance level, and severity of the applied water stress treatments (Song et al., 2019; Cheng et al., 2021). The yield reduction due to water stress is primarily attributed to the disruption of physiobiochemical processes, inhibition of photosynthesis, and stunted plant growth and development (Song et al., 2019; Sharma et al., 2020; Cheng et al., 2021).
However, several studies have revealed that plants tend to accumulate various compatible solutes, such as soluble sugars and amino acids [e.g., proline (Pro)], as an adaptive tolerance strategy to increase salinity and/or drought tolerance (Giri, 2011; Semida et al., 2015; Arteaga et al., 2020). Pro is an essential amino acid that accumulates in various plant tissues, particularly in the leaves (Hayat et al., 2012). Proline accumulation plays an indispensable role in the regulation of cell osmosis (El Moukhtari et al., 2020). Additionally, under unfavorable conditions of water stress, Pro stabilizes membranes (Xia et al., 2020) and protects enzymes (Qamar et al., 2019; El-Nashaar et al., 2020), proteins, and macromolecules from denaturation (Agami et al., 2019). The effectiveness of exogenously applied Pro depends on the plant developmental stage and variety, Pro application rate, and the time of application (Semida et al., 2020). Excessive concentrations of free Pro can disrupt cell growth and protein functions. Hayat et al. (2007) observed that the foliar application of Pro (30 mM) negatively affects maize plants.
Using Pro as an antioxidant is an effective, unconventional, and inexpensive approach for mitigating the harmful effects of drought on maize production under different irrigation systems. Further studies are highly recommended to reduce the gap between water consumption and production of maize in arid and semi-arid regions. Therefore, this study aimed to elucidate the ameliorative effects of Pro at different nontoxic application rates on the morph-physiological and yield attributes of maize plants exposed to water stress. Furthermore, irrigation water requirements for maize plants grown under different irrigation systems and water scarcity conditions were determined.
Materials and Methods
Experimental Site
Field experiments were performed in the summers of 2017 and 2018 at the Agricultural Research Station in the Fayoum University, Fayoum Governorate, Egypt (latitude, 29°02′ and 29°35′ N and longitude, 30°23′ and 31°05′ E). The experimental treatments were arranged in a spilt-split plot in a completely randomized block design with three replications. The two irrigation systems, SI and DI, were set in the main plots. Three deficit irrigation levels (I100, I85, and I70 of ET) were employed in the subplots, and three concentrations of Pro (Pro0 = 0/control; Pro1 = 2 mM; and Pro2 = 4 mM) were used in the sub-sub plots.
Initial Soil Characteristics of the Experimental Site
The soil in the field experiment was sandy loam; the water content at −0.33 and −15 bar pressure was retained by 20.52 and 8.87%, respectively. At a soil depth of 0.0–60 cm, the dry bulk density of the soil was 1.47 mg m–3, saturated hydraulic conductivity was 2.34 cm h–1, mean soil pest extract (ECe) was 3.75 dS m–1, and pH (soil:water suspension, 1:2.5) was 7.77. At the same soil depth, the mean organic matter and CaCO3 concentration were 13.8 and 87.8 g kg–1, respectively (Table 1).
The initial physical and chemical characteristics of the soil at the experimental site were determined according to the methods proposed by Page et al. (1982) and Klute and Dirksen (1986).
Experimental Treatments
Irrigation Systems
The SI and DI systems were implemented and installed at the experimental site as described in the following section.
SI System
Under the SI system, the quantity of irrigation water applied (IWA) for each plot was mainly controlled using a plastic pipe (spiles) with a diameter of 2 inches. For each experimental plot, one spile was constructed to direct the irrigation water. The quantity of IWA was estimated using the following equation (Israelsen and Hansen, 1962):
where Q is the irrigation water discharge (L s–1), C is the discharge coefficient, A is the cross-sectional area of the spile (cm2), g is gravity acceleration (cm s–2), and h is the mean of the influential head of water (cm) above the pipe.
DI System
Under the DI system, irrigation water was provided through polyvinyl chloride pipes. A distance of 70 cm was maintained between the lateral lines, and emitters were spaced at 30 cm. An emitter discharge rate of 2 L h–1 was achieved with pumping at a pressure of 2 bar.
Water Stress Treatments
Three different water stress levels [I100, I85, and I70 (%) of ETc] were implemented. Maize plants were irrigated with the corresponding amounts of irrigation water, which were determined according to the daily reference crop ET as follows (Allen et al., 1998):
where Epan is the evaporation rate from Class A pan (mm day–1) and Kpan is the pan evaporation coefficient (0.85). ETc is the sum of the water evaporation from the soil surface and transpiration (water loss) primarily from the plant leaves. ETo is the reference ET. Kc is the crop coefficient; the Kc values at the initial, mid, and end stages were 0.70, 1.20, and 0.35, respectively. The irrigation water requirements (IWR) for each plot were determined using the following equation (Abd El-Wahed and Ali, 2013):
where IWR is the water requirement for irrigation (m3), A is the irrigated plot area (m2), ETc is the crop ET (mm day–1), Ii is the interval between irrigations (days), Kr is the coverage coefficient, and Ea is the irrigation efficiency (%).
Antioxidant Applications
The antioxidant Pro was applied at three different concentrations : Pro0 (control) = 0, Pro1 = 2 mM, and Pro2 = 4 mM. It was applied three times as a foliar spray [30, 45, and 60 days after sowing (DAS)].
Meteorological Parameters
The monthly weather data for the summers of 2017 and 2018 were collected from the meteorological station of the Fayoum Governorate, Egypt (Figure 1). The climate in the experimental field was arid and characterized by low or no precipitation. During the period from May to September of each year, the maximum and minimum air temperatures were 40.4°C and 20.15°C, respectively, and the relative humidity fluctuated from 35 to 42%. The mean Epan values during the cultivation period (May–September) were 6.48 and 6.50 mm day–1 for the first and second growing seasons, respectively.
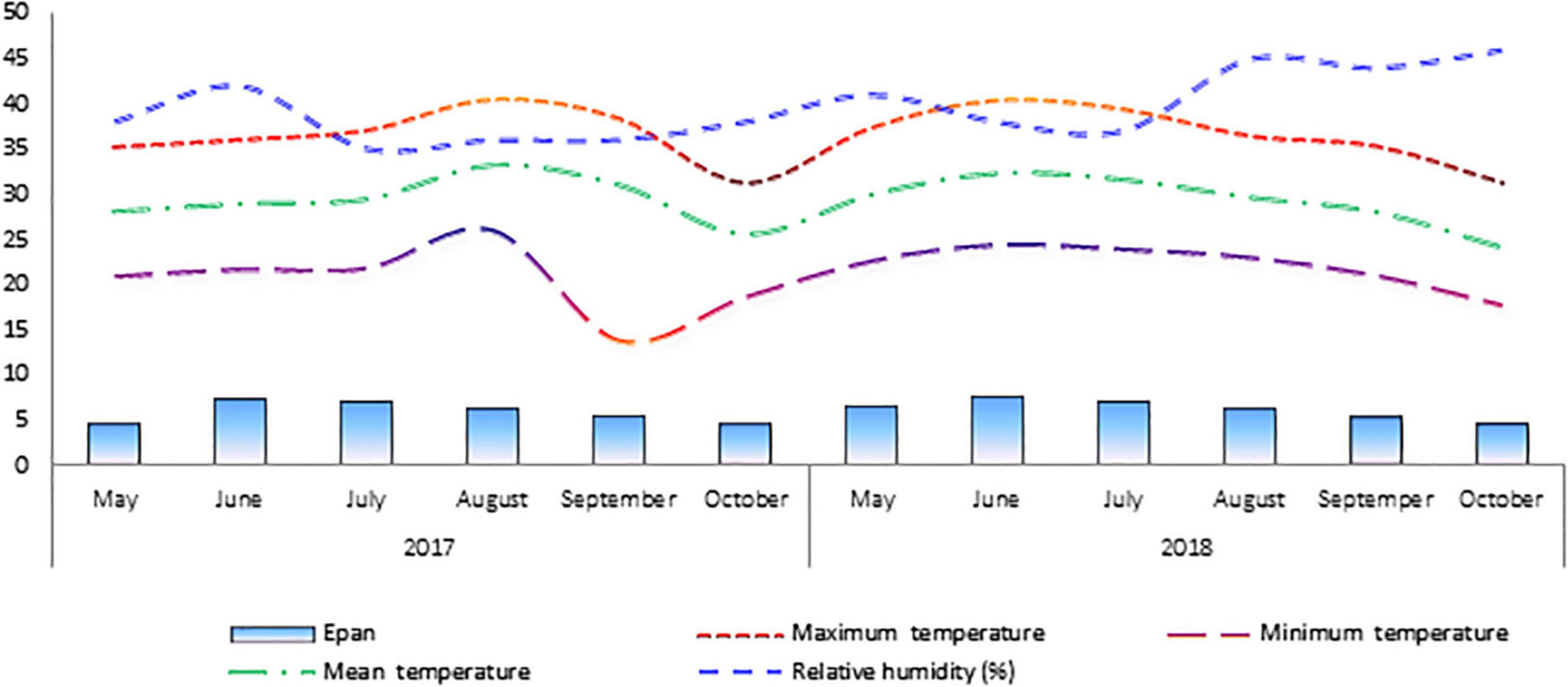
Figure 1. Monthly meteorological parameters in Fayoum Governorate in both seasons (2017 and 2018). The evaporation rate Epan (mm day−1).
Agricultural Management Practices
Maize (Zea mays, hybrid 321) was cultivated on June 5 during the summers of both years (2017 and 2018) in hills that were 30 cm apart, with a distance of 70 cm between the lateral lines. The experimental plots were fertilized using N:P:K (200:100:75; kg ha–1). Total superphosphate (15.5% P2O5) along with potassium sulfate (48% K2O) was applied before cultivation. Nitrogen fertilizer was applied using two equivalent doses, with the first and second doses applied at the first and second irrigation events, respectively.
All other agricultural operations required for the growth and development of maize plants were performed similarly in all plots according to the recommendations of the Egyptian Ministry of Agriculture. Maize plants were harvested 120 DAS.
Physiological Maize Parameters
Ten plant samples (70 DAS) were randomly selected from each plot to measure their physiological responses to the treatments applied.
Relative Water Content
The leaf samples for relative water content (RWC) measurement were randomly collected in the morning (8:00 a.m.–9:00 a.m.). RWC (%) was determined according to the following equation (Hayat et al., 2007):
where FW is the fresh weight measured within 2 h after the excision of leaves; TW is the turgid weight computed by soaking the leaves in distilled water and leaving them at room temperature for 16–18 h, followed by rapid and careful dry-blotting on tissue paper. The small leaf pieces were later oven-dried at 70°C for 48 h to assess the dry weight (DW).
Leaf Membrane Stability Index
MSI (%) was measured using the method described by Premachandra et al. (1990). Small leaf strips (0.2 g) of equal size were prepared and placed in two sets of test tubes, each containing 10 mL of distilled water. The test tubes of the first set were incubated in a water bath at 40°C for 30 min, and ECe was subsequently estimated (C1), whereas those of the second set were incubated in a boiling water bath at 100°C for 15 min, followed by ECe measurement (C2). MSI =
Relative Chlorophyll Content Values
The relative chlorophyll content SPAD was determined using SPAD 502 (Konicaminolta. Inc., Tokyo), as described by Maxwell and Johnson (2000).
Chlorophyll Fluorescence
Chlorophyll fluorescence (Fv/Fm) was determined using a portable fluorometer (Spoustová et al., 2013).
Performance Index
The performance index (PI) of photosynthesis was determined according to the method proposed by Clark et al. (2000).
During harvesting (120 DAS), ten plants from each plot were collected to determine their height (cm), stem diameter (cm), leaf number plant−1, root weight, cob weight (g), 100-grain weight (g), grain yield (t ha–1), and biomass yield (t ha–1).
Water Productivity
Water productivity (WP) is expressed as the grain yield (kg) per IWA (m3). The values were used to evaluate the variation in different treatments for producing the maximum yield from the water unit consumed by the maize plants. The WP values were calculated (Jensen, 1983) as per the following equation:
Statistical Analysis
The study was designed in a completely randomized block design (spilt-split plot) with three replications, and the obtained data were statistically analyzed according to the procedures outlined by Gomez and Gomez (1984) using the GenStat statistical package, 12th edition (VSN International Ltd., Oxford, United Kingdom).
Results
Morphological Characteristics of Maize Plants
Table 2 indicated that all maize growth parameters, i.e., plant height (cm), stem diameter (cm), leaf number plant−1, and root weight (g), were significantly affected by the applied water stress levels, exogenous Pro treatments, and their interactions under different irrigation systems. Plants under the DI system showed a considerable increase in the plant height (17.82%), stem diameter (17.86%), and leaf number plant−1 (18.77%) compared with those under the SI system. An exception was observed for the root weight in which the highest value (54.85 g plant−1; mean value of both seasons) was observed under the SI system. Additionally, fully irrigated maize plants (I100) exhibited higher growth rates than drought-stressed maize plants. Compared with the control (I100), the mean values (during the summers of both years) of plant height, stem diameter, leaf number plant−1, and root weight decreased by 10.51, 9.61, 3.01, and 11.14%, respectively, at a moderate stress level (I85). These values decreased by 17.38, 22.55, 10.94, and 20.60%, respectively, under a severe stress level (I70).
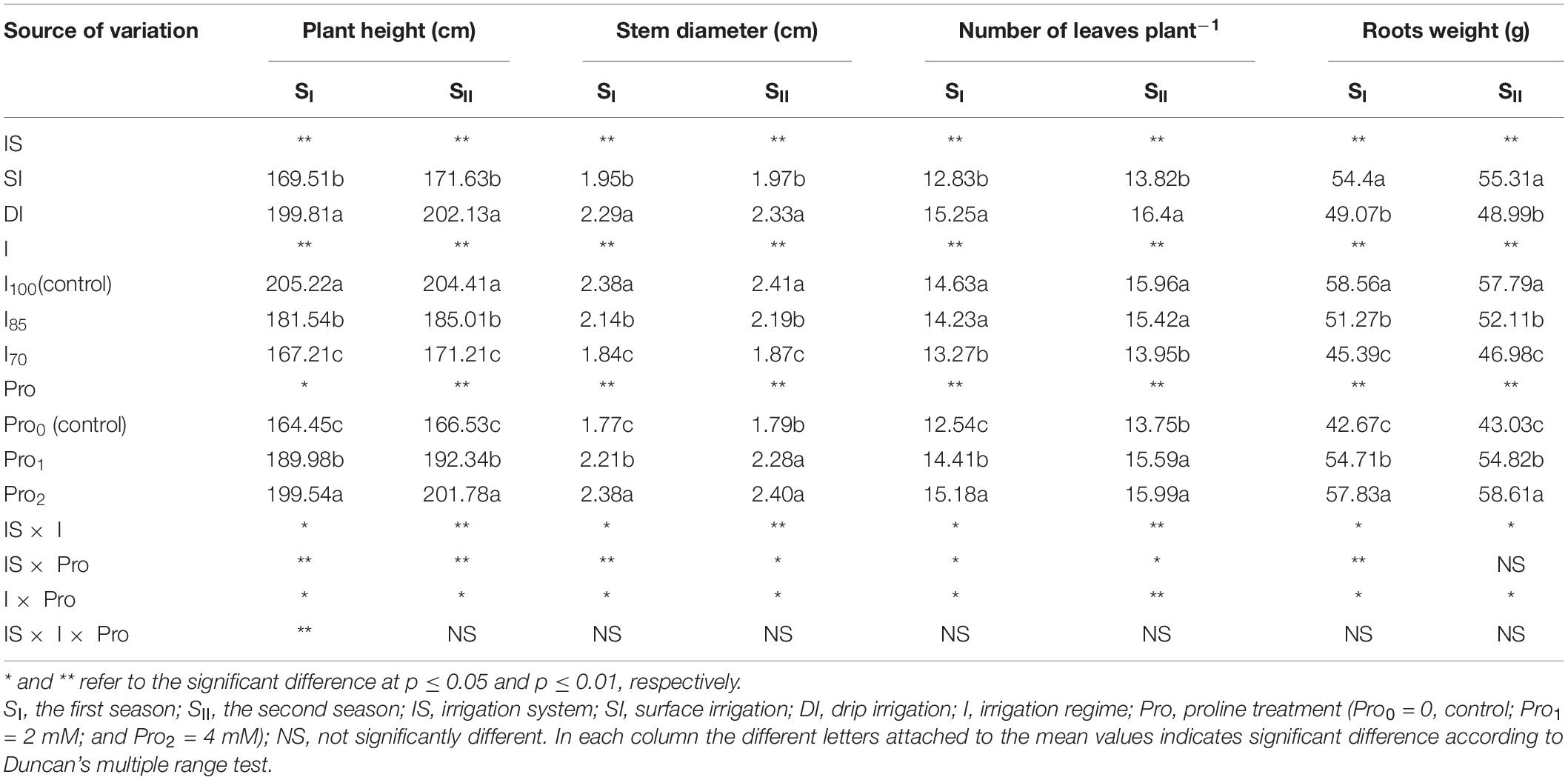
Table 2. Effect of water stress treatments and proline application rates on maize growth traits under different irrigation systems.
The exogenously applied Pro ameliorated the adverse effects of drought stress on maize plants. The maximum plant height, stem diameter, leaf number plant−1, and root weight were 199.54 cm, 2.39 cm, 15.58, and 58.22 g, respectively, for plants treated with Pro2 during the summers of both years. After increasing the level of the exogenously applied Pro, the plant height, stem diameter, leaf number plant−1, and root weight under Pro1 treatment increased by 15.51, 26.12, 14.15, and 27.81%, whereas those under Pro2 treatment increased by 21.25, 34.27, 18.67, and 35.87%, respectively, compared with the control (Pro0).
Plant Water Status (RWC and MSI)
As shown in Table 3, the two indicators of plant water status, RWC (%) and MSI, showed a significant response to water stress and Pro treatments under the two irrigation systems during the summers of both years, whereas the combined effect of these treatments (IS × I × P) on the aforementioned indicators was not significant. The results showed that maize plants under the DI system exhibited better physiological responses than those under the SI system. Additionally, water stress considerably reduced RWC by 10.51 and 17.38% in maize plants under moderate (I85) and severe (I70) stress levels, respectively, compared with those under no stress (controls; I100). The exogenous application of Pro mitigated the drought-induced inhibitory effects on RWC. Compared with the control (Pro0), the mean values of RWC increased by 15.51 and 21.25% with increased concentrations of Pro1 and Pro2, respectively. Furthermore, the highest mean MSI (67.98%) was observed in adequately irrigated plants (100% ETc), and the lowest mean MSI (59.69%) was observed in plants exposed to high water deficit levels (I70). Cell membrane integrity is highly susceptible to drought stress. Water stress leads to the disruption of cell membrane stability, thus increasing electrolyte leakage and decreasing membrane integrity. The mean MSI increased by 6.88 and 13.25% for Pro1- and Pro2-treated plants, respectively, compared with that for non-Pro-treated plants during the summers of both years.
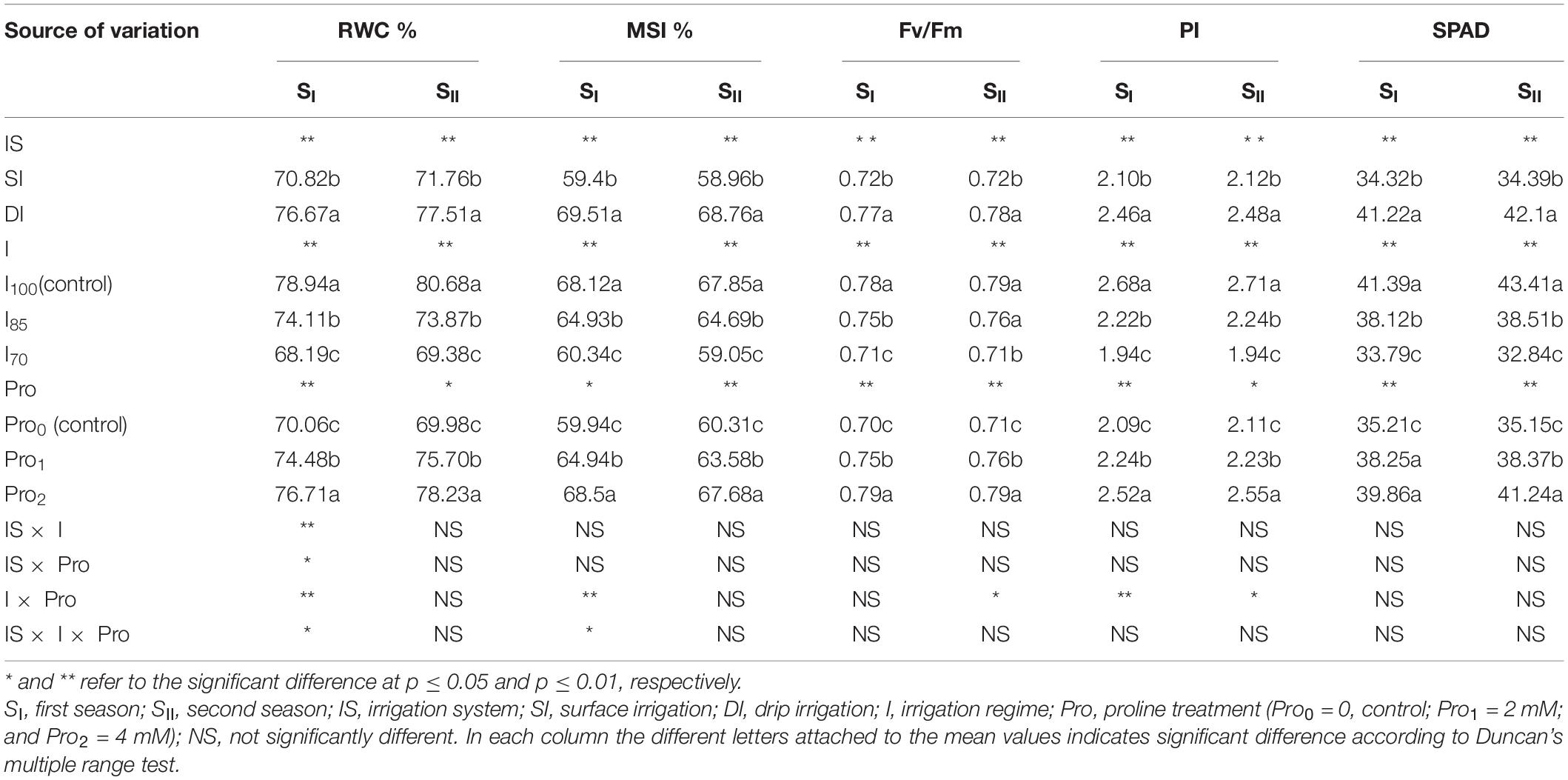
Table 3. Effect of water stress and proline application rates on relative water content (RWC), membrane stability index (MSI), chlorophyll fluorescence (Fv/Fm), performance index (PI), and relative chlorophyll content (SPAD) values under different irrigation systems.
Fv/Fm, PI, and Soil Plant Analysis Development
The data presented in Table 3 revealed that maize plants showed significant differences in Fv/Fm, PI, and SPAD in response to water stress and Pro foliar treatments under the SI and DI systems during the summers of both years; however, the effect of their interaction was not significant. The values for these traits increased by 7.64, 17.06, and 21.26% for Fv/Fm, PI, and SPAD, respectively, under the DI system compared with the SI system. Water stress adversely affects photosynthesis. The Fv/Fm, PI, and SPAD values showed a consistent decrease with increasing water deficit. Compared with sufficiently irrigated plants (I100), the Fv/Fm, PI, and SPAD values decreased by 3.82, 17.25, and 9.59%, respectively, in plants grown under the moderate-deficit irrigation level (I85) and by 9.55, 28.01, and 21.36%, respectively, in those under the high-deficit irrigation level (I70). Furthermore, this study indicated that the harmful effects of drought stress on photosynthesis can be alleviated in maize through foliar Pro application. The exogenous application of Pro (Pro2 concentration) on maize plants exposed to water stress increased the Fv/Fm, PI, and SPAD values by 12.06, 20.71, and 15.27%, respectively, compared with the control (Pro0).
Yield and Yield Components
Table 4 showed that the yield and yield components of maize, such as cob weight (g), 100-grain weight (g), grain yield (t ha–1), and biomass yield (t ha–1), differed significantly in response to water stress and Pro applications under the SI and DI systems during the summers of both years. The effects of interaction were not significant between (IS × I) and (IS × P) for the 100-grain weight parameter. Except of grain yield, the interaction effect (IS × IR × P) on all abovementioned traits was not significant (P > 0.05). Maize plants exposed to water stress had a lower yield than fully irrigated plants. Compared with the full irrigation level (I100), the cob weight (g), 100-grain weight (g), grain yield (t ha–1), and biomass yield (t ha–1) of maize plants decreased by 4.33, 4.45, 6.36, and 9.24%, respectively, at the moderate-deficit irrigation level (I85) and by 7.49, 14.90, 25.44, and 17.97% at the high-deficit irrigation level (I70). The exogenously applied Pro increased the yield of maize plants under deficit irrigation; the cob weight, 100-grain weight, grain yield, and biomass yield increased (as mean values of both seasons) by 11.70, 21.07, 10.93, and 15.44%, respectively, at moderate Pro (Pro1) application rates and by 15.61, 27.89, 15.99, and 23.05%, respectively, at high Pro (Pro2) application rates, compared with the control (Pro0).
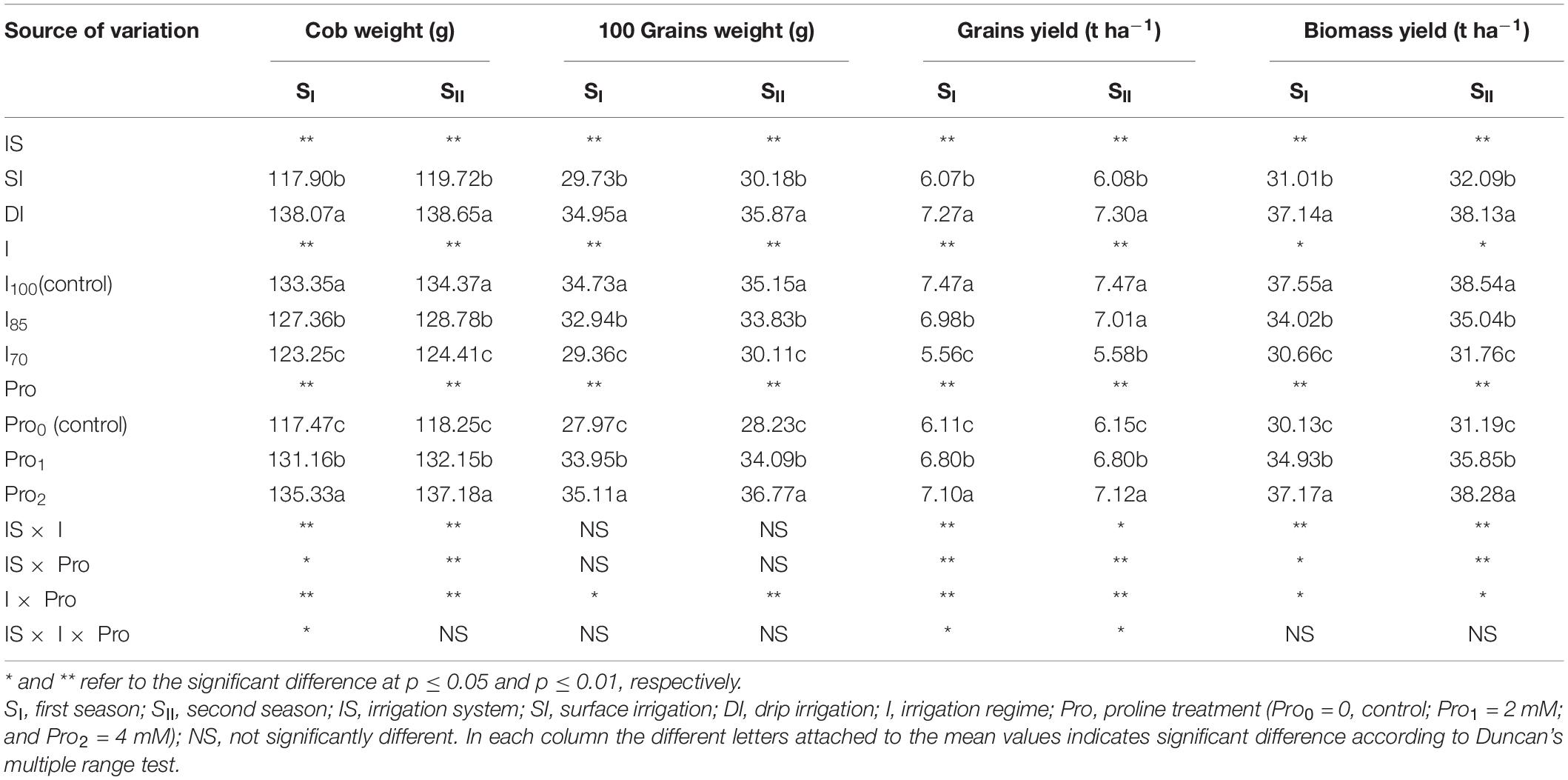
Table 4. Effect of water stress and proline application rates on yield and yield components of maize crop under different irrigation systems.
Irrigation Water Applied
Table 5 showed that IWA varied according to the designed irrigation regime and system. The highest values of irrigation water inputs were observed under the SI system at the full irrigation level. The need for irrigation gradually decreased as the water deficit level increased in the DI system because its high-efficiency design saved 29.41% of IWA compared with the SI system.
Water Productivity
As shown in Figure 2, the highest WP (1.80 kg m–3) was observed at I85 with a high Pro application rate (Pro2) under the DI system. In contrast, the lowest (0.79 kg m–3) WP was observed at I100 with a low Pro application rate (Pro1) under the SI system. For each irrigation level and Pro application treatment, DI considerably enhanced WP compared with SI. DI delivers water near each plant, leading to high water-use efficiency. Among the water stress treatments, the irrigation regime of I85, in which the reduction in grain yield was lower than the amount of irrigation water saved, showed the highest WP-value compared with other irrigation regimes.
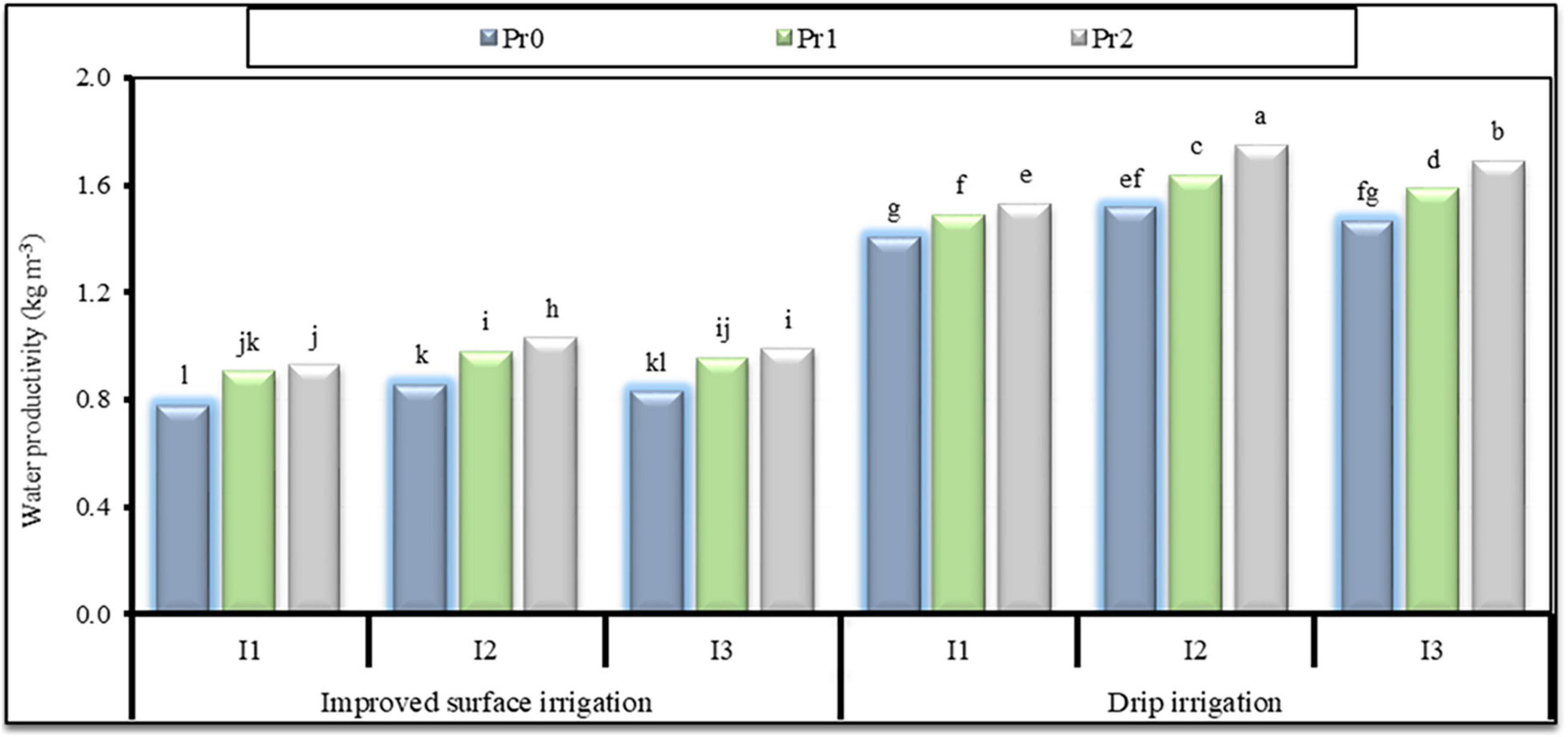
Figure 2. Effect of water stress and proline (Pr) application rates (Pr0 = 0, control; Pr1 = 2 mM; and Pr2 = 4 mM), on water productivity (as mean values of the two seasons) under different irrigation systems. Different letters on the bars refer to significant differences among means based on Fisher’s least significant difference test at the p < 0.05 level.
Effect of Irrigation Systems and Irrigation Regimes on Salt Distribution Pattern
Figure 3 showed that SI, particularly when coupled with high levels of irrigation water (I100), enhanced the downward movement or migration of soluble salts, thus reducing the concentrations of salt accumulated in the lower soil layers by 10.14% compared with those accumulated on the soil surface (0–20 cm). In contrast, DI with low irrigation input (I70) promoted the upward movement of salts, thus increasing soil salinity in the same layer (0–20 cm) by 14.38% compared with the initial soil salinity.
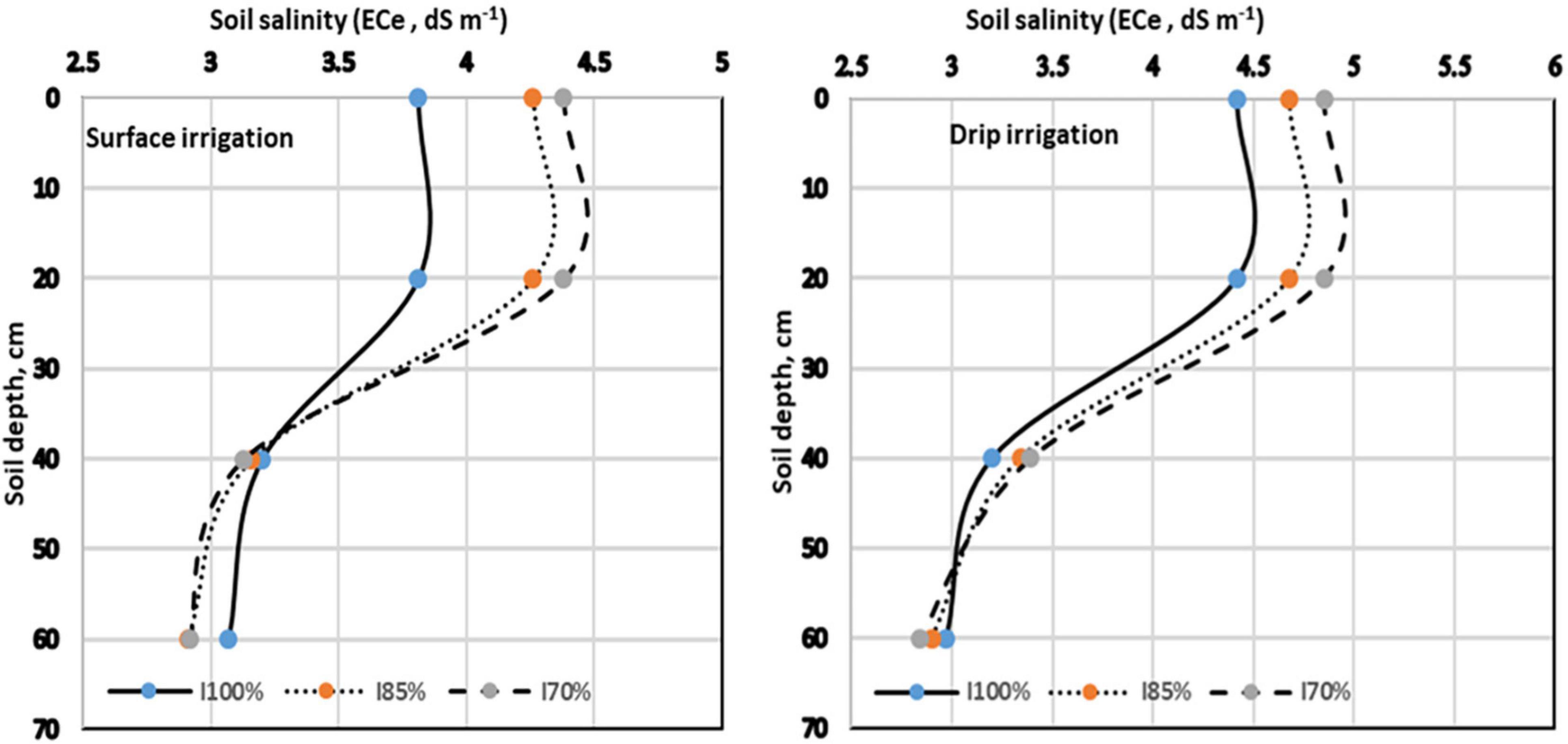
Figure 3. Effect of irrigation systems and irrigation regimes on salt distribution pattern. ECe, soil electrical conductivity.
Discussion
Water stress adversely affects the growth and development of plants, particularly when the stress conditions remain constant. When plants are exposed to stress, they accumulate an array of metabolites, such as amino acids. Pro plays a highly beneficial role in plants exposed to various stress conditions. Besides acting as an excellent osmolyte, Pro has three fundamental biological roles in stress response, i.e., acting as a metal chelator, antioxidative defense molecule, and signaling molecule. Some reports; however, have indicated the toxic effects of Pro when applied exogenously at high concentrations. In this study, we aimed to (i) investigate the causes underlying the reduction in the growth and yield of maize plants induced by water stress and (ii) determine the crucial role of Pro in mitigating these negative effects at different nontoxic application rates of Pro to improve WP under water scarce conditions.
Our results demonstrated significant differences in the vegetative growth of maize plants in response to the two irrigation systems (Table 2). The DI system was relatively superior in the maize growth parameters, except for root weight, compared with the SI system. Similar to previous reports (Ghamarnia et al., 2013; Mahgoub et al., 2017), the observed increase in the root weight of plants under the SI system could be a result of the downward movement of irrigation water due to gravity, which promotes the penetration of roots through the soil profile to greater depths to extract water from the deeper layers. Maize plants exposed to water stress showed growth retardation. The inhibition of maize growth due to water stress was evident because of the decrease in the length, volume, and water potential of the root system, which disrupted the water extraction by the roots. This results in decreased cell division and elongation, leading to stunted plant growth. These results were consistent with those of previous studies (El-Samnoudi et al., 2019; Song et al., 2019; Sah et al., 2020; Cheng et al., 2021). However, the exogenous application of Pro not only provided osmoprotection but also enhanced the growth of the plants. The beneficial effect of Pro in enhancing maize growth characteristics under low irrigation levels might be attributed to its role in osmoregulation, and maintenance of the tertiary structure of proteins and enzymes, to help growing plants tolerate drought stress. These results were similar to those of the previous reports (Abdelaal et al., 2018, 2020; Qamar et al., 2019; El-Nashaar et al., 2020).
Additionally, drought adversely affected the plant water retention (RWC and MSI) and photosynthesis (Table 3). The decrease in water uptake under deficit irrigation regimes was associated with a decrease in leaf water potential. Drought alters the plant water status and stomatal functioning and inhibits chlorophyll biosynthesis, thus reducing the photosynthetic rates. Dehydration in the plant cell protoplasm could be considered as an effect of drought on the RWC of maize leaves. Similar observations were reported in a previous study (Xia et al., 2020). The foliar application of Pro enhanced the plant water status. This was evident by the reduction in water efflux under drought stress, which helped the cells maintain their cell turgor or osmotic balance. These results are consistent with those of another study conducted by Al-Khazrji et al. (2020). It is suggested that the exogenous application of Pro enhances membrane stability, thus preventing electrolyte leakage, as previously documented by Hayat et al. (2012).
Water stress further decreased the values of the physiological parameters in maize seedlings. This was evident by the disruption of plant photosynthetic potential, possibly due to the stomatal closure and/or metabolic destruction, such as the impairment of photosystem 1 (PSI) and photosystem II (PSII), which are chlorophyll-binding protein complexes (Ibrahim et al., 2019; Zheng et al., 2022). Furthermore, water stress increases the generation of reactive oxygen species (ROS), resulting in oxidative damage to plants and degradation of chlorophyll pigments (Hasanuzzaman et al., 2020; Mansoor et al., 2022). Alternatively, Pro may be responsible for scavenging ROS and other free radicals (Kaul et al., 2008). It may also protect plants from stress injuries by stabilizing membranes and proteins, allowing the transport of mitochondrial electrons, enhancing antioxidant enzyme activity, thus increasing stomatal conductance and facilitating higher CO2 diffusion through leaves. This promotes higher photosynthetic capacity (Tùmová et al., 2018; Agami et al., 2019; Altuntaş et al., 2020; Semida et al., 2020; Tariq et al., 2021; Abdou et al., 2022). Hence, the foliar application of Pro can enhance the photosynthetic rate of maize under drought stress.
The decreased water uptake from the soil might be responsible for the detrimental effects of water stress on grain and biomass yields of maize (Table 4), resulting in the abovementioned inhibition in plant growth and development, disruption of photosynthetic pigments, and deficits in plant water content. Accordingly, a significant decrease in maize yield under water stress conditions was observed. The results are consistent with those of previous studies (Vazirimehr et al., 2014; Sah et al., 2020; Shah et al., 2020).
The present study demonstrated that the increase in the yield and yield components achieved by the application of Pro was higher than the reduction in the yield caused by drought stress, confirming that Pro treatment successfully compensated for the adverse effects of drought stress on the growth and yield of maize. Furthermore, the yield and yield components of maize under the DI system were higher than those under the SI system (Qamar et al., 2019; Abdelaal et al., 2020; El-Nashaar et al., 2020; Li et al., 2021), and WP increased consistently with increasing concentrations of exogenously applied Pro (Table 5). These results are consistent with those of the previous studies by Semida et al. (2015) and Semida et al. (2020) who found that pressurized irrigation systems via subsurface drip and/or surface drip increase the water-use efficiency and grain yield of maize compared with the SI system.
Notably, the monitoring of the salt distribution pattern in the soil profile (depth, 0–60 cm) based on different applied irrigation systems and treatments showed that the salt distribution pattern was different after plant harvest in the second season compared with the determined initial ECe values before maize planting. The results were consistent with those of the studies by Semida et al. (2015) and Ali et al. (2016) who reported that the highest salinity was observed in the deeper soil layers depending on the wetting front under the SI system. The lowest salinity was observed on the surface; however, under DI, salt accumulation increased on the soil surface.
Conclusion
With increasing water scarcity, well-designed deficit DI regimes and the exogenously applied antioxidant Pro can optimize the maize production and WP when the available water is insufficient to provide full irrigation. The DI system efficiently saved irrigation water input by 30% compared with the SI system. Among the tested irrigation levels, the full level (I100) resulted in the highest grain yield. The moderate-deficit irrigation level (I85) showed the maximum WP. The growth, physiological aspects, and grain yield of maize increased significantly after the foliar application of Pro to maize plants exposed to water stress. Therefore, using exogenous Pro at 4 mM for maize plants under the DI system and irrigating with 85% of their ET demand may be a promising agro-management strategy for improving the yield and WP of maize crops grown in arid and semi-aired regions, especially when water scarcity constraints the sustainability of maize production.
Data Availability Statement
The original contributions presented in this study are included in the article, further inquiries can be directed to the corresponding author/s.
Author Contributions
AE-AI, TA, YA, SA, KE-T, and NA: conceptualization. AE-AI, TA, YA, and NA: methodology. AE-AI, TA, SA, KE-T, and NA: formal analysis. TA, SA, KE-T, and NA: investigation and writing—original draft preparation. AE-AI, TA, YA, ME-S, HA, and NA: data curation. TA, ME-S, SA, KE-T, and NA: writing—review and editing. TA and HA: visualization. SA and KE-T: funding acquisition. All authors have read and agreed to the published version of the manuscript.
Funding
This project was funded by the Khalifa Center for Biotechnology and Genetic Engineering-UAEU (grant no. 31R286) to SA and the Abu Dhabi Research Award (AARE2019) for Research Excellence-Department of Education and Knowledge (ADEK) (grant no. 21S105) to KE-T.
Conflict of Interest
The authors declare that the research was conducted in the absence of any commercial or financial relationships that could be construed as a potential conflict of interest.
Publisher’s Note
All claims expressed in this article are solely those of the authors and do not necessarily represent those of their affiliated organizations, or those of the publisher, the editors and the reviewers. Any product that may be evaluated in this article, or claim that may be made by its manufacturer, is not guaranteed or endorsed by the publisher.
Acknowledgments
KE-T would like to thank the library at Murdoch University, Australia, for providing valuable online resources and comprehensive databases.
References
Abd El-Wahed, M. H., and Ali, E. A. (2013). Effect of irrigation systems, amounts of irrigation water and mulching on corn yield, water use efficiency and net profit. Agric. Water Manag. 120, 64–71. doi: 10.1016/j.agwat.2012.06.017
Abdelaal, K. A. A., Attia, K. A., Alamery, S. F., El-Afry, M. M., Ghazy, A. I., Tantawy, D. S., et al. (2020). Exogenous application of proline and salicylic acid can mitigate the injurious impacts of drought stress on barley plants associated with physiological and histological characters. Sustainability 12:1736. doi: 10.3390/su12051736
Abdelaal, K. A. A., Hafez, Y. M., El-Afry, M. M., Tantawy, D. S., and Alshaal, T. (2018). Effect of some osmoregulators on photosynthesis, lipid peroxidation, antioxidative capacity, and productivity of barley (Hordeum vulgare L.) under water deficit stress. Environ. Sci. Pollut. Res. 25, 30199–30211. doi: 10.1007/s11356-018-3023-x
Abdou, N. M., Abdel-razek, M. A., El-Mageed, S. A. A., and Semida, W. M. (2021). High nitrogen fertilization modulates morpho-physiological responses, yield, and water productivity of lowland rice under deficit irrigation. Agronomy 11:1291. doi: 10.3390/agronomy11071291
Abdou, N. M., El-Saadony, F. M. A., Roby, M. H. H., Mahdy, H. A. A., El-Shehawi, A. M., Elseehy, M. M., et al. (2022). Foliar spray of potassium silicate, aloe extract composite and their effect on growth and yielding capacity of roselle (Hibiscus sabdariffa L.) under water deficit stress conditions. Saudi J. Biol. Sci. (in press). doi: 10.1016/j.sjbs.2022.02.033
Agami, R. A., Alamri, S. A. M., Abd El-Mageed, T. A., Abousekken, M. S. M., and Hashem, M. (2019). Salicylic acid and proline enhance water use efficiency, antioxidant defense system and tissues’ anatomy of wheat plants under field deficit irrigation stress. J. Appl. Bot. Food Qual. 92, 360–370. doi: 10.5073/JABFQ.2019.092.048
Ali, A., Hussain, M., Habib, H. S., Kiani, T. T., Anees, M. A., and Rahman, M. A. (2016). Foliar spray surpasses soil application of potassium for maize production under rainfed conditions. Turkish J. F. Crop. 21, 36–43. doi: 10.17557/tjfc.66054
Ali, M., Abbasi, G. H., Jamil, M., Raza, M. A. S., and Ahmad, S. (2021). Characterization of maize hybrids (Zea mays L.) for salt tolerance at seedling stage. Pak. J. Sci. Ind. Res. 64, 160–166. doi: 10.52763/PJSIR.BIOL.SCI.64.2.2021.160.166
Al-Khazrji, O. A. R., Al-Saedi, S. A., and Alkhateb, B. A. A. H. (2020). Leaf area, proline and relative water content in the leaves of maize plant under the effect of soil bacteria, organic matter and water stress. Plant Arch. 20, 405–412.
Allen, R. G., Pereira, L. S., Raes, D., and Smith, M. (1998). Guidelines for Computing Crop Water Requirements-FAO Irrigation and Drainage Paper 56. ARPAV (2000). La Caratterizzazione Climatica della Regione Veneto, Quaderni per. Geophysics, Vol. 156., Rome: Food and Agriculture Organisation of the United Nations, 178.
Altuntaş, C., Demiralay, M., Sezgin Muslu, A., and Terzi, R. (2020). Proline-stimulated signaling primarily targets the chlorophyll degradation pathway and photosynthesis associated processes to cope with short-term water deficit in maize. Photosynth. Res. 144, 35–48. doi: 10.1007/s11120-020-00727-w
Arteaga, S., Yabor, L., Díez, M. J., Prohens, J., Boscaiu, M., and Vicente, O. (2020). The use of proline in screening for tolerance to drought and salinity in common bean (Phaseolus vulgaris L.) genotypes. Agronomy 10:817. doi: 10.3390/agronomy10060817
Badal, E., El-Mageed, T. A. A., Buesa, I., Guerra, D., Bonet, L., and Intrigliolo, D. S. (2013). Moderate plant water stress reduces fruit drop of “Rojo Brillante” persimmon (Diospyros kaki) in a Mediterranean climate. Agric. Water Manag. 119, 154–160. doi: 10.1016/j.agwat.2012.12.020
Chai, Q., Gan, Y., Zhao, C., Xu, H., Waskom, R. M., Niu, Y., et al. (2016). Regulated deficit irrigation for crop production under drought stress. A review. Agron. Sustain. Dev. 36:3. doi: 10.1007/s13593-015-0338-6
Cheng, M., Wang, H., Fan, J., Zhang, F., and Wang, X. (2021). Effects of soil water deficit at different growth stages on maize growth, yield, and water use efficiency under alternate partial root-zone irrigation. Water 13:148. doi: 10.3390/w13020148
Clark, A. J., Landolt, W., Bucher, J. B., and Strasser, R. J. (2000). Beech (Fagus sylvatica) response to ozone exposure assessed with a chlorophyll a fluorescence performance index. Environ. Pollut. 109, 501–507. doi: 10.1016/S0269-7491(00)00053-1
El Moukhtari, A., Cabassa-Hourton, C., Farissi, M., and Savouré, A. (2020). How does proline treatment promote salt stress tolerance during crop plant development? Front. Plant Sci. 11:1127. doi: 10.3389/fpls.2020.01127
El-Nashaar, F., Hafez, Y. M., Abdelaal, K. A. A., Abdelfatah, A., Badr, M., El-Kady, S., et al. (2020). Assessment of host reaction and yield losses of commercial barley cultivars to Drechslera teres the causal agent of net blotch disease in Egypt. Fresenius Environ. Bull. 29, 2371–2377.
El-Samnoudi, I. M., Ibrahim, A. E. M., Tawwab, A. R. A. E., and El-Mageed, T. A. A. (2019). Combined effect of poultry manure and soil mulching on soil properties, physiological responses, yields and water-use efficiencies of sorghum plants under water stress. Commun. Soil Sci. Plant Anal. 50, 2626–2639. doi: 10.1080/00103624.2019.1671445
Food and Agriculture Organization [FAO] (2017). Water for Sustainable Food and Agriculture-A Report Produced for the G20 Presidency of Germany. Rome: Food and Agriculture Organization.
Ghamarnia, H., Parandyn, M. A., Arji, I., and Rezvani, V. (2013). An evaluation and comparison of drip and conventional furrow irrigation methods on maize. Arch. Agron. Soil Sci. 59, 733–751. doi: 10.1080/03650340.2012.671934
Giri, J. (2011). Glycinebetaine and abiotic stress tolerance in plants. Plant Signal. Behav. 6, 1746–1751. doi: 10.4161/psb.6.11.17801
Gomaa, M. A., Rehab, I. F., Salama, F. A., and Al-Deeb, A. S. M. (2017). Water-stress in relation to maize (Zea mays L.) grain yield, plant height and proline content. Alex. J. Agric. Sci. 2017, 311–317. doi: 10.21608/alexja.2017.5789
Gomez, K. A., and Gomez, A. A. (1984). Statistical Procedures for Agricultural Research. New York, NY: John Wiley & Sons.
Hasanuzzaman, M., Bhuyan, M. H. M. B., Zulfiqar, F., Raza, A., Mohsin, S. M., Mahmud, J. A., et al. (2020). Reactive oxygen species and antioxidant defense in plants under abiotic stress: Revisiting the crucial role of a universal defense regulator. Antioxidants 9:681. doi: 10.3390/antiox9080681
Hayat, S., Ali, B., Hasan, S. A., and Ahmad, A. (2007). Brassinosteroid enhanced the level of antioxidants under cadmium stress in Brassica juncea. Environ. Exp. Bot. 60, 33–41. doi: 10.1016/j.envexpbot.2006.06.002
Hayat, S., Hayat, Q., Alyemeni, M. N., Wani, A. S., Pichtel, J., and Ahmad, A. (2012). Role of proline under changing environments: a review. Plant Signal. Behav. 7, 1456–1466. doi: 10.4161/psb.21949
Ibrahim, A. M., Abd El-Mageed, T. A., Abdou, N. M., and Abohamid, Y. M. (2019). Effect of salicylic acid on growth, physiological responses and yield of maize grown under drought stress. Fayoum J. Agric. Res. Dev. 33, 18–30.
Ibrahim, M. E., Bekheta, M. A., El-Moursi, A., and Gaafar, N. A. (2007). Improvement of growth and seed yield quality of Vicia faba L. plants as affected by application of some bioregulators. Aust. J. Basic Appl. Sci. 1, 657–666.
Israelsen, O. W., and Hansen, V. C. (1962). Irrigation Principles and Practices. New York, NY: John Wiley & Sons Inco, 448.
Jensen, M. E. (1983). Design and Operation of Farm Irrigation Systems. St. Joseph, MICH: American Society of Agricultural Engineers, 829.
Kaul, S., Sharma, S. S., and Mehta, I. K. (2008). Free radical scavenging potential of L-proline: evidence from in vitro assays. Amino Acids 34, 315–320. doi: 10.1007/s00726-006-0407-x
Klute, A., and Dirksen, C. (1986). “Hydraulic conductivity and diffusivity: laboratory methods,” in Methods of Soil Analysis: Part 1—Physical and Mineralogical Methods, ed. A. Klute (Madison, WI: Soil Science Society of America, American Society of Agronomy), 687–734. doi: 10.2136/sssabookser5.1.2ed.c28
Li, G., Shah, A. A., Khan, W. U., Yasin, N. A., Ahmad, A., Abbas, M., et al. (2021). Hydrogen sulfide mitigates cadmium induced toxicity in Brassica rapa by modulating physiochemical attributes, osmolyte metabolism and antioxidative machinery. Chemosphere 263:127999. doi: 10.1016/j.chemosphere.2020.127999
Mahgoub, N. A., Ibrahim, A. M., and Ali, O. M. (2017). Effect of different irrigation systems on root growth of maize and cowpea plants in sandy soil. Eurasian J. Soil Sci. 6, 374–379. doi: 10.18393/ejss.319952
Mancosu, N., Snyder, R. L., Kyriakakis, G., and Spano, D. (2015). Water scarcity and future challenges for food production. Water 7, 975–992. doi: 10.3390/w7030975
Mansoor, S., Ali, W. O., Lone, J. K., Manhas, S., Kour, N., Alam, P., et al. (2022). Reactive oxygen species in plants: from source to sink. Antioxidants 11:225. doi: 10.3390/antiox11020225
Maxwell, K., and Johnson, G. N. (2000). Chlorophyll fluorescence – A practical guide. J. Exp. Bot. 51, 659–668. doi: 10.1093/jxb/51.345.659
Misra, A. K. (2014). Climate change and challenges of water and food security. Int. J. Sustain. Built Environ. 3, 153–165. doi: 10.1016/j.ijsbe.2014.04.006
MWRI (2014). Water Scarcity in Egypt: The Urgent Need for Regional Cooperation among the Nile Basin Countries. Cairo: Ministry of Water Resources and Irrigation (MWRI).
Osman, R., Ferrari, E., and McDonald, S. (2016). Water scarcity and irrigation efficiency in Egypt. Water Econ. Policy 2:1650009. doi: 10.1142/S2382624X16500090
Ouda, S. A. H., Zohry, A. E. H., Alkitkat, H., Morsy, M., Sayad, T., and Kamel, A. (2017). Future of Food Gaps in Egypt : Obstacles and Opportunities. Cham: Springer Nature. doi: 10.1007/978-3-319-46942-3
Page, A. L., Miller, R. H., and Keeny, D. R. (1982). “Methods of soil analysis,” in Part II: Chemical and Microbiological Methods, 2nd Edn, eds A. L. Page, R. H. Miller, D. R. Keeney, D. E. Baker, R. H. Miller, R. Ellis, et al. (Madison, WI: American Society of Agronomy), 225–246.
Parkash, V., and Singh, S. (2020). A review on potential plant-based water stress indicators for vegetable crops. Sustainability 12:3945. doi: 10.3390/SU12103945
Pereira, L. S., Cordery, I., and Iacovides, I. (2012). Improved indicators of water use performance and productivity for sustainable water conservation and saving. Agric. Water Manag. 108, 39–51. doi: 10.1016/j.agwat.2011.08.022
Premachandra, G. S., Saneoka, H., and Ogata, S. (1990). Cell membrane stability, an indicator of drought tolerance, as affected by applied nitrogen in soyabean. J. Agric. Sci. 115, 63–66. doi: 10.1017/S0021859600073925
Qamar, R., Noreen, S., Safdar, M., and Babar, M. E. (2019). Influence of exogenous application of proline on some physio-biochemical parameters of maize (Zea mays L.) under drought stress. Int. J. Sci. Res. Publ. 9, 858–869. doi: 10.29322/ijsrp.9.08.2019.p92117
Ranum, P., Peña-Rosas, J. P., and Garcia-Casal, M. N. (2014). Global maize production, utilization, and consumption. Ann. N. Y. Acad. Sci. 1312, 105–112. doi: 10.1111/nyas.12396
Sah, R. P., Chakraborty, M., Prasad, K., Pandit, M., Tudu, V. K., Chakravarty, M. K., et al. (2020). Impact of water deficit stress in maize: phenology and yield components. Sci. Rep. 10:2944. doi: 10.1038/s41598-020-59689-7
Semida, W. M., Abd El-Mageed, T. A., Howladar, S. M., Mohamed, G. F., and Rady, M. M. (2015). Response of Solanum melongena L. seedlings grown under saline calcareous soil conditions to a new organo-mineral fertilizer. J. Anim. Plant Sci. 25, 485–493.
Semida, W. M., Abdelkhalik, A., Rady, M. O. A., Marey, R. A., and Abd El-Mageed, T. A. (2020). Exogenously applied proline enhances growth and productivity of drought stressed onion by improving photosynthetic efficiency, water use efficiency and up-regulating osmoprotectants. Sci. Hortic. 272:109580. doi: 10.1016/j.scienta.2020.109580
Shah, A. A., Khan, W. U., Yasin, N. A., Akram, W., Ahmad, A., Abbas, M., et al. (2020). Butanolide alleviated cadmium stress by improving plant growth, photosynthetic parameters and antioxidant defense system of Brassica oleracea. Chemosphere 261:127728. doi: 10.1016/j.chemosphere.2020.127728
Shahrokhi, M., Khorasani, S. K., and Ebrahimi, A. (2020). Evaluation of drought tolerance indices for screening some of super sweet maize (Zea mays L. var. Saccharata) inbred lines. AGRIVITA J. Agric. Sci. 42, 435–448. doi: 10.17503/agrivita.v42i3.2574
Sharma, A., Wang, J., Xu, D., Tao, S., Chong, S., Yan, D., et al. (2020). Melatonin regulates the functional components of photosynthesis, antioxidant system, gene expression, and metabolic pathways to induce drought resistance in grafted Carya cathayensis plants. Sci. Total Environ. 713:136675. doi: 10.1016/j.scitotenv.2020.136675
Song, L., Jin, J., and He, J. (2019). Effects of severe water stress on maize growth processes in the field. Sustainability 11:5086. doi: 10.3390/su11185086
Spoustová, P., Synková, H., Valcke, R., and Èeøovská, N. (2013). Chlorophyll a fluorescence as a tool for a study of the potato virus Y effects on photosynthesis of non-transgenic and transgenic Pssu-ipt tobacco. Photosynthetica 51, 191–201. doi: 10.1007/s11099-013-0023-4
Tariq, M., Shah, A. A., Yasin, N. A., Ahmad, A., and Rizwan, M. (2021). Enhanced performance of Bacillus megaterium OSR-3 in combination with putrescine ammeliorated hydrocarbon stress in Nicotiana tabacum. Int. J. Phytoremediation 23, 119–129. doi: 10.1080/15226514.2020.1801572
Tùmová, L., Tarkowská, D., Øøová, K., Marková, H., Koèová, M., Rothová, O., et al. (2018). Drought-tolerant and drought-sensitive genotypes of maize (Zea mays L.) differ in contents of endogenous brassinosteroids and their drought-induced changes. PLoS One 13:e0197870. doi: 10.1371/journal.pone.0197870
Vazirimehr, M. R., Ganjali, H. R., Keshtehgar, A., and Rigi, K. (2014). Seed priming effect on the number of rows per ear, grain weight and economic yield corn in Sistan region. Int. J. Biosci. 6655, 87–91. doi: 10.12692/ijb/4.4.87-91
Wakchaure, G. C., Minhas, P. S., Meena, K. K., Singh, N. P., Hegade, P. M., and Sorty, A. M. (2018). Growth, bulb yield, water productivity and quality of onion (Allium cepa L.) as affected by deficit irrigation regimes and exogenous application of plant bio–regulators. Agric. Water Manag. 199, 1–10. doi: 10.1016/j.agwat.2017.11.026
Wang, Y., Tang, J., Zhang, H., Schroder, J. L., and He, Y. (2014). Phosphorus availability and sorption as affected by long-term fertilization. Agron. J. 106, 1583–1592. doi: 10.2134/agronj14.0059
Xia, H., Xu, T., Zhang, J., Shen, K., Li, Z., and Liu, J. (2020). Drought-induced responses of nitrogen metabolism in Ipomoea batatas. Plants 9:1341. doi: 10.3390/plants9101341
Yuan, X. K., Yang, Z. Q., Li, Y. X., Liu, Q., and Han, W. (2016). Effects of different levels of water stress on leaf photosynthetic characteristics and antioxidant enzyme activities of greenhouse tomato. Photosynthetica 54, 28–39. doi: 10.1007/s11099-015-0122-5
Keywords: deficit irrigation, irrigation system, maize, plant water status, proline, water productivity
Citation: Ibrahim AE-A, Abd El Mageed T, Abohamid Y, Abdallah H, El-Saadony M, AbuQamar S, El-Tarabily K and Abdou N (2022) Exogenously Applied Proline Enhances Morph-Physiological Responses and Yield of Drought-Stressed Maize Plants Grown Under Different Irrigation Systems. Front. Plant Sci. 13:897027. doi: 10.3389/fpls.2022.897027
Received: 15 March 2022; Accepted: 19 April 2022;
Published: 14 July 2022.
Edited by:
Nasim Ahmad Yasin, University of the Punjab, PakistanReviewed by:
Waheed Ullah Khan, University of the Punjab, PakistanAqeel Ahmad, Chinese Academy of Sciences (CAS), China
Copyright © 2022 Ibrahim, Abd El Mageed, Abohamid, Abdallah, El-Saadony, AbuQamar, El-Tarabily and Abdou. This is an open-access article distributed under the terms of the Creative Commons Attribution License (CC BY). The use, distribution or reproduction in other forums is permitted, provided the original author(s) and the copyright owner(s) are credited and that the original publication in this journal is cited, in accordance with accepted academic practice. No use, distribution or reproduction is permitted which does not comply with these terms.
*Correspondence: Synan AbuQamar, c2FidXFhbWFyQHVhZXUuYWMuYWU=; Khaled El-Tarabily, a3RhcmFiaWx5QHVhZXUuYWMuYWU=