- 1Plant Physiology and Biochemistry Section, Department of Botany, Aligarh Muslim University, Aligarh, India
- 2Department of Botany, Government Degree College Doda, Doda, India
- 3The UWA Institute of Agriculture, The University of Western Australia, Perth, WA, Australia
The application of phytohormones through seed priming could enhance quality of important medicinal and aromatic plants (MAPs) under heavy metal stress. We evaluated the potential of salicylic acid (SA) priming for overcoming the adverse effects of cadmium stress in Mentha arvensis L. plants. Suckers of plants were primed with SA before transplanting them into soil. At 30 days after transplanting, two doses (50 and 100 μm) of CdCl2 were applied to the soil. Both Cd treatments altered plant growth, photosynthetic pigments, leaf gas exchange attributes, and mineral nutrient contents. The 50 and 100 μm Cd treatments increased endogenous Cd content by 97.95 and 98.03%, electrolyte leakage (EL) by 34.21 and 44.38%, hydrogen peroxide (H2O2) by 34.71 and 55.80%, malondialdehyde (MDA) by 53.08 and 63.15%, and superoxide content (O2–•) by 24.07 and 38.43%, respectively. Cd triggered the up-regulation of antioxidant enzyme activities (superoxide dismutase, SOD; catalase, CAT; ascorbate peroxidase, APX; and glutathione reductase GR) and increased osmolyte biosynthesis and, interestingly, secondary metabolite (SM) accumulation. The presence of SA and Cd had an additive effect on these parameters. Nevertheless, plants primed with SA regulated stomatal conductance under Cd stress. SA priming to menthol mint plants under Cd stress overcome the effects of Cd stress while increasing SMs.
Introduction
Heavy metal (HM) contamination is ubiquitous in all the spheres of the environment, viz. biosphere, lithosphere, atmosphere, hydrosphere, and anthroposphere. The plant–soil interface acts as a channel for different HMs to circulate into different environmental compartments and ultimately reach humans and plants through the food chain. In particular, cadmium (Cd) is a potent and noteworthy environmental contaminant. In most soils, Cd is present as a trace element. However, due to high industrialization and sophisticated anthropogenic activities, such as the discharge of sewage sludge, mining, phosphorous fertilizers, and industrial effluents, excessive amounts of Cd are bio-available in vast tracts of lands (Zhang et al., 2009; He et al., 2015; Gao et al., 2018; Zaid et al., 2019a; Shah et al., 2020a,b). Cd pollution is the representative HM contamination in soil, as Cd2+ ions are readily absorbed by crop plants (DalCorso et al., 2008; Rizwan et al., 2018). In plants, Cd can close stomata, generate reactive oxygen species (ROS), oxidize membranes, lipids and proteins, and decrease photosynthetic potential, subsequently disturbing plant growth and development (Zaid and Mohammad, 2018; Bari et al., 2019; Pourghasemian et al., 2019).
Menthol mint (Mentha avensis L.) is a wild mint variety widely cultivated for its phytopharmaceutical importance (Nigam et al., 2019). Soil Cd enrichment is posing a major threat to its productivity by inducing oxidative stress. M. arvensis L. owes its aromatic properties to the presence of an essential oil (EO) rich in SMs and volatile constituents, including menthol, menthone, methyl acetate, and carvacrol, that impart its characteristic mint flavor (Tiwari, 2016). Advances in M. arvensis trichome research, stomatal conductance, and SMs biosynthesis under Cd stress have received little attention. Till date, no study has reported plant growth regulator (PGR)-mediated mechanisms of action for enhancing tolerance to Cd stress and SM production in M. arvensis.
Plant stress physiologists have attempted to reduce Cd contamination in soils and crop plants using various organic and inorganic molecules (Rizwan et al., 2016, 2017, 2019b; Zaid and Mohammad, 2018). The application of PGRs to reduce Cd uptake and alleviate Cd toxicity and to boost yield and quality attributes might be a cost-effective, practical, sustainable strategy for MAPs. External supplementation of PGRs is considered an effective practice for reducing the toxic effects of Cd contamination in different crop plants (Rizwan et al., 2016; Zaid and Mohammad, 2018). We recently showed that the external application of PGRs and mineral nutrients had a synergistic effect on alleviating the negative effect of Cd stress on menthol mint plants (Zaid and Mohammad, 2018; Zaid et al., 2020). The incorporation of M. arvensis biochar in the soil increased HM tolerance in M. arvensis in terms of improving yield and nutrient uptake (Nigam et al., 2019). The concomitant application of depolymerized polysaccharides and PGRs modulated physiological activities, active constituents, and EO production in menthol mint plants (Naeem et al., 2017). Bose et al. (2013) studied the synergistic effect of PGRs and diterpenoid on growth attributes, trichome size, density, and number, EO biosynthesis and the expression of some oil biosynthetic pathway genes. The effect of exogenous treatment of SA on growth, photosynthetic traits and EO production of peppermint plants exposed to soil-applied Cd was studied and the results advocated the ameliorative role of SA (Ahmad et al., 2018).
Seed priming before sowing is an important strategy to ensure optimum hydration for seeds without enabling radical emergence (Hussain et al., 2019b). Priming tends to enhance plant tolerance to abiotic stress by improving plant growth and cellular homeostasis under various stress conditions (Jisha et al., 2013; Savvides et al., 2016). SA is a potent PGR known to impart stress resistance in diverse crop plants (Khan et al., 2015; Ahmad et al., 2019; Santisree et al., 2020). Studies have shown that priming with PGRs is a promising field in plant stress physiology and crop stress management, which can overcome the toxic impacts of abiotic stresses in plants (Lal et al., 2018; Moulick et al., 2018; Panuccio et al., 2018; Sheteiwy et al., 2018). Priming with PGRs is regarded as an eco-friendly, cost-effective, and sustainable practice for reducing the effects of HM stress in plants (Sytar et al., 2018). Hassan and Mansoor (2017) showed that seed priming with PGRs alleviated Cd toxicity in mungbean. Priming with SA could boost growth, photosynthetic capacity and ameliorate the ill effects of HM stress in plants. For example in maize, Zanganeh et al. (2018) studied the coordinated role of seed priming with SA and sodium hydrosulfide under Pb stress. Shahnawaz et al. (2017) studied the impact of Al toxicity on barley cultivars and its alleviation through seed priming with ascorbic acid and SA. In a recent study, we identified the potential of exogenous SA for overcoming nickel stress in Indian mustard plants (Zaid et al., 2019b). However, the role of SA via priming under Cd stress in M. arvensis in relation to SM modulation and physio-biochemical traits has not been evaluated till date. Therefore, the present study aimed to determine (a) the effects of Cd stress on growth traits, photosynthetic potential, stomatal conductance, and yield and quality traits, (b) the potential of priming suckers with SA to mitigate the adverse impacts of Cd stress, and (c) whether SA priming enhances SM production in menthol mint plants in Cd-contaminated soils.
Materials and Methods
Sucker Priming and Stress Treatment
The experiment was performed in a net house at the Botany Department, Aligarh Muslim University Aligarh, India (27°52′N, 78°51′E, 187.45 m altitude), with average day/night temperatures of 26 ± 3/14 ± 2°C, 65 ± 5% relative humidity, 815 ± 20 μmol m−2 s−1 photosynthetically active radiation (PAR), and 10–12 h critical photoperiod. Uniform suckers of M. arvensis Linn. “Kushal” were hand-picked for surface sterilization with 5% NaOCl solution for 6 min, followed by repeated washing with deionized and double distilled water (DDW). The suckers were primed with a solution of SA (10−6 M) in the dark at room T for 30 min and then transplanted to earthen pots. Each pot (25 × 25 cm) contained a 5 kg mixture of soil and farmyard manure (4:1). The physicochemical characteristics of the soil were sandy loam texture, pH (1:2) 7.9, EC (1:2) 0.58 mmhos cm−1, and 96.8, 9.6, and 158.91 mg kg−1 soil available N, P, and K, respectively. A basal dose of N, P, and K (N100P50K50; Khanuja et al., 2006) was applied, being 44.6 mg N, 22.3 mg P2O5, and 27.3 mg K2O kg−1 soil as urea, single superphosphate and muriate of potash, respectively. After germination, plants were thinned, and three healthy seedlings were maintained in each pot. Thirty days after transplanting (DAT), two Cd treatments (50 and 100 μm) were applied to pots. A dilute stock aqueous solution of cadmium chloride (CdCl2) was prepared by dissolving 1.63 g of CdCl2 in 100 ml of DDW and then the required quantity prepared by further diluting the stock solution with DDW. Control plants were provided with DDW only. The experimental pots were positioned in a randomized complete block design. In all, there were six treatments with five replications (n = 5): Control (CN; 0 μm Cd + 0 M SA); Salicylic acid (SA 10−6 M + Cd 0 μm); Cadmium (50 μm + 0 M SA); Cadmium + Salicylic acid (50 μm + 10−6 M SA); Cadmium (100 μm + 0 M SA); Cadmium + Salicylic acid (100 μm + 10−6 M SA). The plants were harvested at 100 DAT. Biochemical parameters and antioxidant enzyme activities of the menthol mint plants were determined in the topmost fully expanded young leaves.
Determination of Growth Parameters
Plant height (PH) was measured with a scale. Plant fresh and dry weights were weighed by using an electronic balance (Verbal 100 Super, Varanasi, Balance Works, Varanasi, India). After recording fresh weight, samples were oven-dried for dehydration at 70°C for 24 h and then weighed. The number of leaves per pot was counted manually. Leaf area was determined using a leaf area meter (AM 350, ADC Bioscientific, Hoddesdon, Herts, United Kingdom).
Determination of Photosynthetic Pigments
Photosynthetic pigment determination followed the protocol of Lichtenthaler and Buschmann (2001). Fresh leaf tissue (100 mg) was harvested and ground with 100% acetone (v/v) using a deep freezer cooled mortar and pestle. The absorbance of the pigment solution was recorded at 663, 645, and 470 nm to determine the contents of chlorophyll a, chlorophyll b, and carotenoids, respectively, using a spectrophotometer (ELICO SL 171 MINI SPEC) and dimethyl sulfoxide (DMSO) as a blank. Total chlorophyll (Chl) content was estimated by adding the contents of chlorophyll a and b. All pigments were expressed as mg g−1 leaf FW.
Determination of Leaf Gas Exchange Attributes
Leaf gas exchange measurements [net photosynthetic rate (PN), stomatal conductance (gs), carbon dioxide assimilation rate (Ci), and water use efficiency (WUE)] were measured on the uppermost fully expanded leaves in full and bright sunlight between 10:00 h and 12:00 h using IRGA (Li-COR 6400, Li-COR, Lincoln, NE, United States).
Determination of Oxidative Stress Biomarkers
The superoxide (O2–•) content was determined by estimating nitrite production from the reaction of hydroxylamine with superoxide anions by adopting the standard method of Wang and Luo (1990). A 1 g leaf sample was homogenized in phosphate buffer (50 mm, pH 7.8) and polyvinylpyrrolidone solution and centrifuged for 20 min at 4°C. The supernatant was mixed with potassium phosphate buffer and hydroxylamine hydrochloride (50 mm, pH 7.8), then dissolved in a 3-aminobenzenesulphonic acid (17 mm) and 1-naphthylamine (7 mm) and incubated for 20 min at room T. The absorbance was measured at 530 nm and the O2–• generation rate calculated from the standard curve of sodium nitrite and expressed as μmol g−1 leaf FW.
Thiobarbituric acid reactive substances (TBARS) content was determined by estimating the MDA equivalents following the method of Hodges et al. (1999). Liquid N2 frozen leaf tissue (0.5 g) was homogenized in 80% ethanol. The homogenate was centrifuged at 3000 g for 10 min. The supernatant (1 ml) was removed and added to an equal volume of 20% trichloroacetic acid (TCA), 0.65% thiobarbituric acid (TBA), and 0.01% butylated hydroxytoluene. The samples were heated at 90°C for 30 min, followed by gradual cooling to room T. Absorbance of the samples was read at 440, 532, and 600 nm, relative to a blank sample with no TBA present, to record MDA concentration.
The method of Sullivan and Ross (1979) was used to determine electrolyte leakage (EL); the detailed protocols described in Zaid and Mohammad (2018). Electrolyte leakage was calculated as follows:
Electrolyte leakage (%) = [(ECb–ECa)/(ECc) × 100].
The standard method of Jana and Choudhari (1981) was used for H2O2 determination. A fresh leaf sample (500 mg) was homogenized in 3.0 ml of phosphate buffer (50 mm and pH 6.8) and centrifuged at 6000 g for 30 min. After that, 3.0 ml of extract was removed and 1 ml of 0.1% titanium chloride in 20% (v/v) H2SO4 added to it. Centrifugation was carried out at 6000 g for 15 min. The absorbance of the reaction mixture was read at 410 nm on a spectrophotometer (ELICO SL 171 MINI SPEC, India).
Estimation of Antioxidant Activities
Freshly collected leaf material (500 mg) was frozen and ground to a powder using liquid N2 in a pre-chilled mortar and pestle. After then, the samples were centrifuged at 12,000 g for 30 min at 4°C. The pellet was discarded and the supernatant used to determine different enzyme activities.
Superoxide dismutase activity (SOD, EC1.15.1.1) was measured using the nitroblue tetrazolium (NBT) reduction method (Kono, 1978). Catalase (CAT, 1.11.1.6) activity was determined by monitoring the disappearance of H2O2 for 2 min at 240 nm (Aebi, 1984). The complete methodology for SOD and CAT determination is described elsewhere (Khanam and Mohammad, 2018). Ascorbate peroxidase (APX, 1.11.1.11) activity was determined using the protocol of Nakano and Asada (1981). Glutathione reductase (GR, 1.6.4.2) activity was measured according to the method of Smith et al. (1988). Enzyme activities were expressed in enzyme units g−1 FM. All spectrophotometric analyses were conducted with a spectrophotometer (ELICO SL 171 MINISPEC).
Determination of Proline, Glycine Betaine, and Leaf Mineral Nutrients
Proline (Pro) and glycine betaine (GB) contents were determined according to the methods of Bates et al. (1973) and Grieve and Grattan (1983), respectively.
For mineral nutrient determinations, oven-dried leaf material (100 mg) was added to a 50 ml Kjeldahl flask containing an acid-peroxide solution and heated on a dry block heater. Aliquots of this solution were used to determine leaf N, P, and K contents. Leaf N content was determined using the protocol of Lindner (1944). The absorbance of the reaction mixture was read at 525 nm. Leaf P content was determined following the methodology of Fiske and Subbarow (1925). The intensity of the blue color formed was recorded at 620 nm. Leaf K content was determined by flame emission spectroscopy using a flame photometer (Model, ELICO, CL22D, India), running at 10 psi pressure (Hald, 1947).
Scanning Electron Microscopy and Energy Dispersive X-ray Analyzer
The method of Zaid and Mohammad (2018) was used for scanning electron microscopy (SEM) and energy dispersive X-ray analyzer (EDX) analysis. Fresh leaves from axillary positions were collected and fixed with 2.5% glutaraldehyde plus 2% paraformaldehyde in 0.1 M phosphate buffer (pH 7.0) for 4 h. This was followed by a second fixing with 1% osmium tetroxide in P buffer (pH 7.0) for 1 hand washing with P buffer for 20 min. Fixation was followed by alcohol dehydration in a graded series of ethanol (50, 70, 80, 90, 95, and 100%). Fixed and dehydrated leaves were dehydrated in a Carl Zeiss EVO 40 (Germany) scanning electron microscope critical point dryer with liquid CO2. The leaf samples were coated with gold–palladium and observed under a Carl Zeiss EVO 40 (Germany) scanning electron microscope at high voltage (15 kV) and magnifications of 4,000 × for stomata and 100 × for peltate glandular trichomes (PGT).
Estimation of Essential Oil Content and Secondary Metabolites by Gas Chromatography
Essential oil (EO) content was determined following the hydro-distillation technique of Guenther (1972), whereby 50 g of freshly collected leaves were allowed for 3 h distillation. The oil was collected in vials of Clevenger apparatus and weighed. The oil percentage was calculated as follows: EO percentage = oil content/leaf weight × 100. Secondary metabolite (menthol, menthone, and methyl acetate) contents of the EO were determined by gas chromatography (GC) using the known peak area in the chromatogram (Adams, 2007). The estimation of SMs used a gas chromatograph (Nucon 5,700, New Delhi, India) equipped with an AT-1000 stainless steel column, flame ionization detector, and integrator. N gas was used as the carrier. N, H, and O flow rates were adjusted at 0.5, 0.5, and 5mLs−1, respectively. The sample size was 2 μl. The T recorded was 160°C oven, 250°C detector, and 250°C injector.
Statistical Analysis
Data were analyzed following one-way analysis of variance (ANOVA) using SPSS software version 17. The values presented are means ± SE (n = 5). Different letters indicate significant differences between treatments at p ≤ 0.05. Principal component analysis was performed using Minitab Mtb EXE (2) software (Minitab Inc., State College PA, United States).
Results
Effect of Salicylic Acid on Plant Growth Parameters Under Cadmium Stress
The Cd treatments significantly decreased (p ≤ 0.05) plant fresh and dry weights, by 18.16 and 40.84% at 50 μm and 8.52 and 26.13% at 100 μm, respectively, relative to the control (Table 1). The SA + Cd treatments increased plant fresh weights by 7.57 and 26.66% and dry weights by 20.94 and 12.08% at 50 and 100 μM, respectively, relative to the respective Cd-only treatments. PH declined significantly (p ≤ 0.05) by 10.25 and 17.70%, relative to the control plants. Priming with SA increased PH in Cd-stressed plants by 6.56 and 7.37% at 50 and 100 μm, respectively, relative to the respective Cd-only treatments (Table 1).
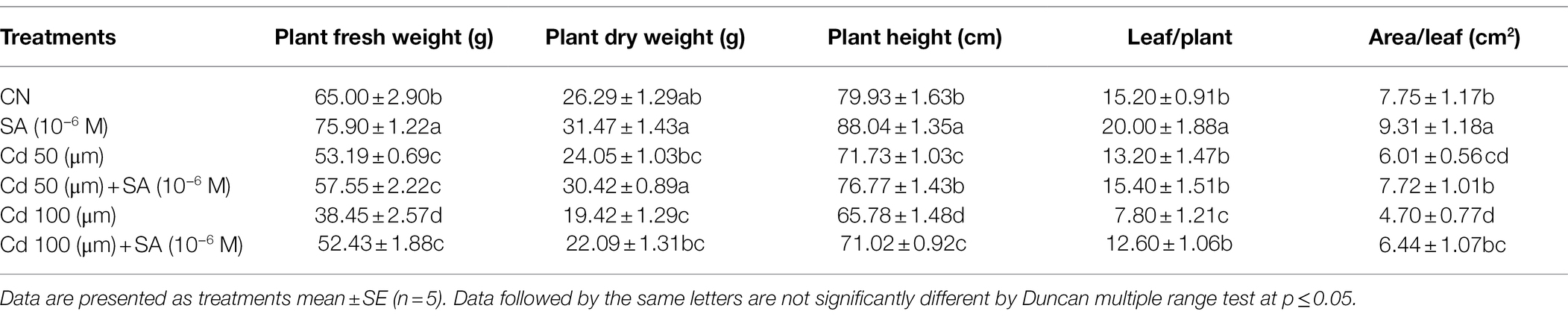
Table 1. Plant fresh and dry weights (g), plant height (cm), and leaf number and area per plant, of Mentha arvensis cultivar “Kushal” grown under 50 and 100 μm Cd levels and primed with or without salicylic acid.
Leaf number declined by 13.15 and 48.68% at 50 and 100 μm, respectively, relative to the control plants. Priming with SA increased leaf number by 14.28 and 38.09% at 50 and 100 μm, respectively, relative to the respective Cd-only treatments. Leaf area also declined significantly (p ≤ 0.05) by 22.45 and 39.35% at 50 and 100 μm, respectively, relative to the control plants. Priming with SA increased leaf area by 22.15 and 27.01% at 50 and 100 μm, respectively, relative to the respective Cd-only treatments (Table 1).
Salicylic Acid Restored Photosynthetic Pigments and Gas Exchange Attributes
The Cd treatments had a negative effect on photosynthetic pigments (Chl a, b, total Chl, and carotenoids). Chl a and b contents declined by 7.94 and 5.71% at 50 μm and 15.23 and 20% at 100 μm, respectively, relative to the control plants. Total Chl and carotenoid content also declined by 7.52 and 16.12% at 50 μm and 3.22 and 16.12% at 100 μm, respectively, relative to the control plants (Table 2). Priming with SA restored the photosynthetic pigment inhibition, increasing Chl a by 2.79 and 3.03%, Chl b by 13.15 and 12.50%, total Chl by 4.79 and 4.87%, and Caro content by 6.25 and 7.14% at 50 and 100 μm, respectively, relative to the respective Cd-only treatments (Table 2).
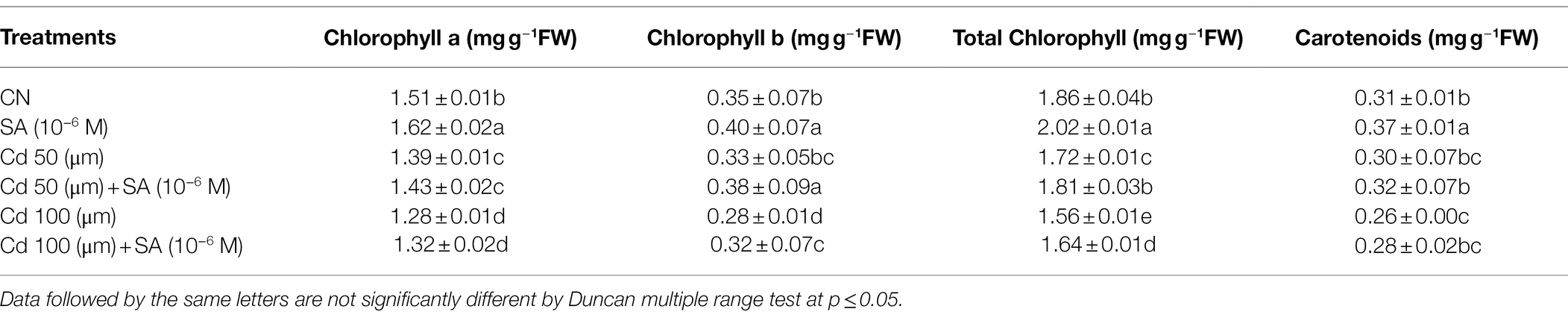
Table 2. Contents of chlorophyll a, b, total chlorophyll, and carotenoids (mg g−1FW) of Mentha arvensis cultivar “Kushal” grown under 50 and 100 μm Cd levels and primed with or without salicylic acid.Data are presented as treatments mean ± SE (n = 5).
The Cd treatments reduced PN by 29.47 and 55.88% at 50 and 100 μm, respectively, relative to the control plants. Priming with SA significantly improved PN by 16.83 and 13.23% at 50 and 100 μm, respectively, relative to the respective Cd-only treatments (Figure 1A). Priming with SA reversed the decline in gs and Ci caused by Cd stress to values 3.35 and 1.89% at 50 μm and 3.85 and 1.83% at 100 μm higher than the respective Cd-only treatments (Figures 1B,C). The 50 and 100 μm Cd treatments decreased WUE by 24.44 and 46.66%, respectively, relative to the control plants. Priming with SA increased WUE by 24.44 and 25% at 50 and 100 μm, respectively, relative to the respective Cd-only treatments (Figure 1D).
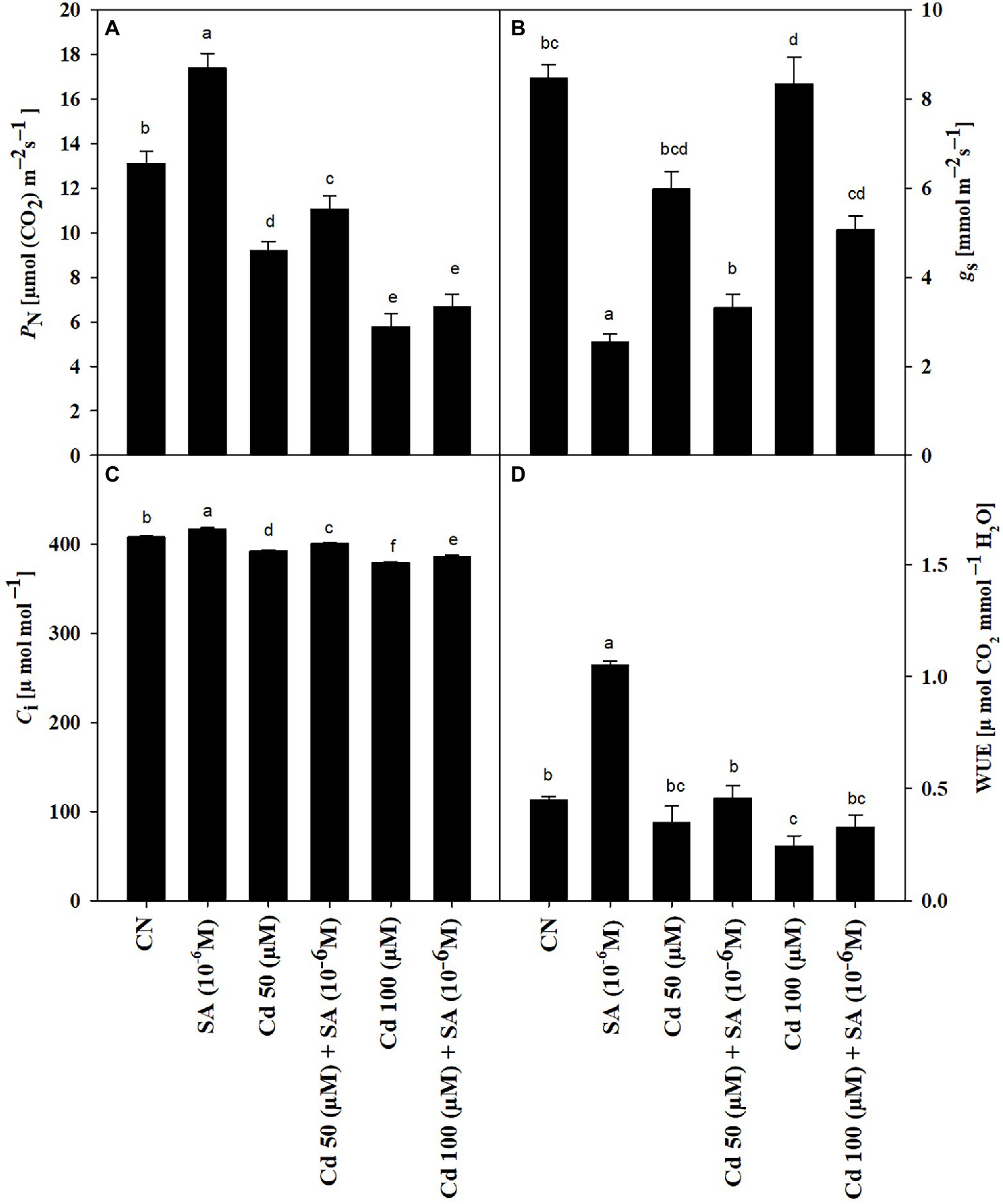
Figure 1. (A) Net photosynthetic rate, PN (μmol CO2 m−2 s−1), (B) stomatal conductance, gs (mmol m−2 s−1), (C) intercellular CO2 concentration, Ci (μmol mol−1), and (D) water use efficiency, WUE (μmol CO2 mmol−1 H2O) of Mentha arvensis cultivar “Kushal” grown under 50 and 100 μm Cd levels and primed with or without salicylic acid. Data are presented as treatments mean ± SE (n = 5). Data followed by the same letters are not significantly different by Duncan multiple range test at p ≤ 0.05.
Salicylic Acid Reduced Thiobarbituric Acid Reactive Substance, Electrolyte Leakage, Hydrogen Peroxide Content, and Superoxide Ion Production Under Cadmium Stress
The Cd treatments increased TBARS content, EL, H2O2 content, and O2–• production by 53.08, 34.21, 34.71 and 24.07% at 50 μM, respectively, relative to the control (Figures 2A–D), which was intensified further at 100 μM. Priming with SA significantly reduced TBARS content by 32.98%, EL by 32.83%, H2O2 content by 19.06%, and O2–• production by 25.58% in the 50 μm Cd treatment, relative to respective Cd-only treatment. At 100 μm Cd, SA priming decreased TBARS content by 30.33%, EL by 10.47%, in H2O2 content by 18.60% and O2–• production by 26.77%, relative to respective Cd-only treatment (Figures 2A–D).
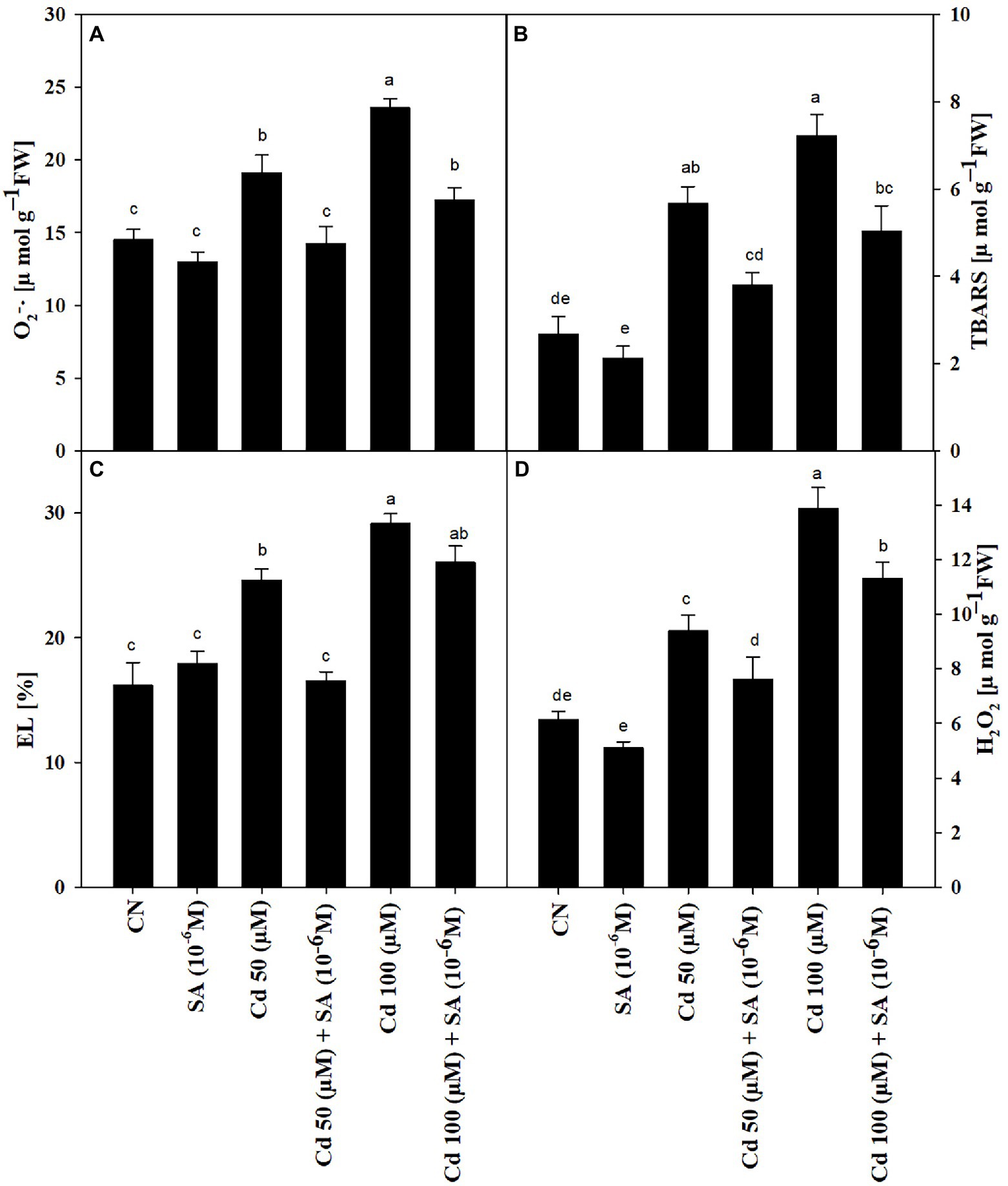
Figure 2. (A) Superoxide, O2–· (μmol g−1 FW), (B) thiobarbituric acid reactive substances, TBARS (μmol g−1 FW), (C) electrolyte leakage, EL (%), and (D) hydrogen peroxide, H2O2 (μmol g−1 FW) of Mentha arvensis cultivar “Kushal” grown under 50 and 100 μm Cd levels and primed with or without salicylic acid. Data are presented as treatments mean ± SE (n = 5). Data followed by the same letters are not significantly different by Duncan multiple range test at p ≤ 0.05.
Salicylic Acid Enhanced Antioxidant Enzyme Activities
The Cd treatments increased the activities of SOD, CAT, APX, and GR by 3.27 and 7.90%, 7.27 and 11.79%, 40.73 and 67.47%, 30.32 and 56.57% at 50 and 100 μm, respectively, relative to the control plants. Priming with SA had an additive impact on SOD, CAT, APX, and GR activities, which increased by 2.18 and 2.88%, 2.13 and 3.09%, 23.20 and 25.16%, and 9.23 and 12.88% at 50 and 100 μm, respectively, relative to the respective Cd-only treatments (Figures 3A–D).
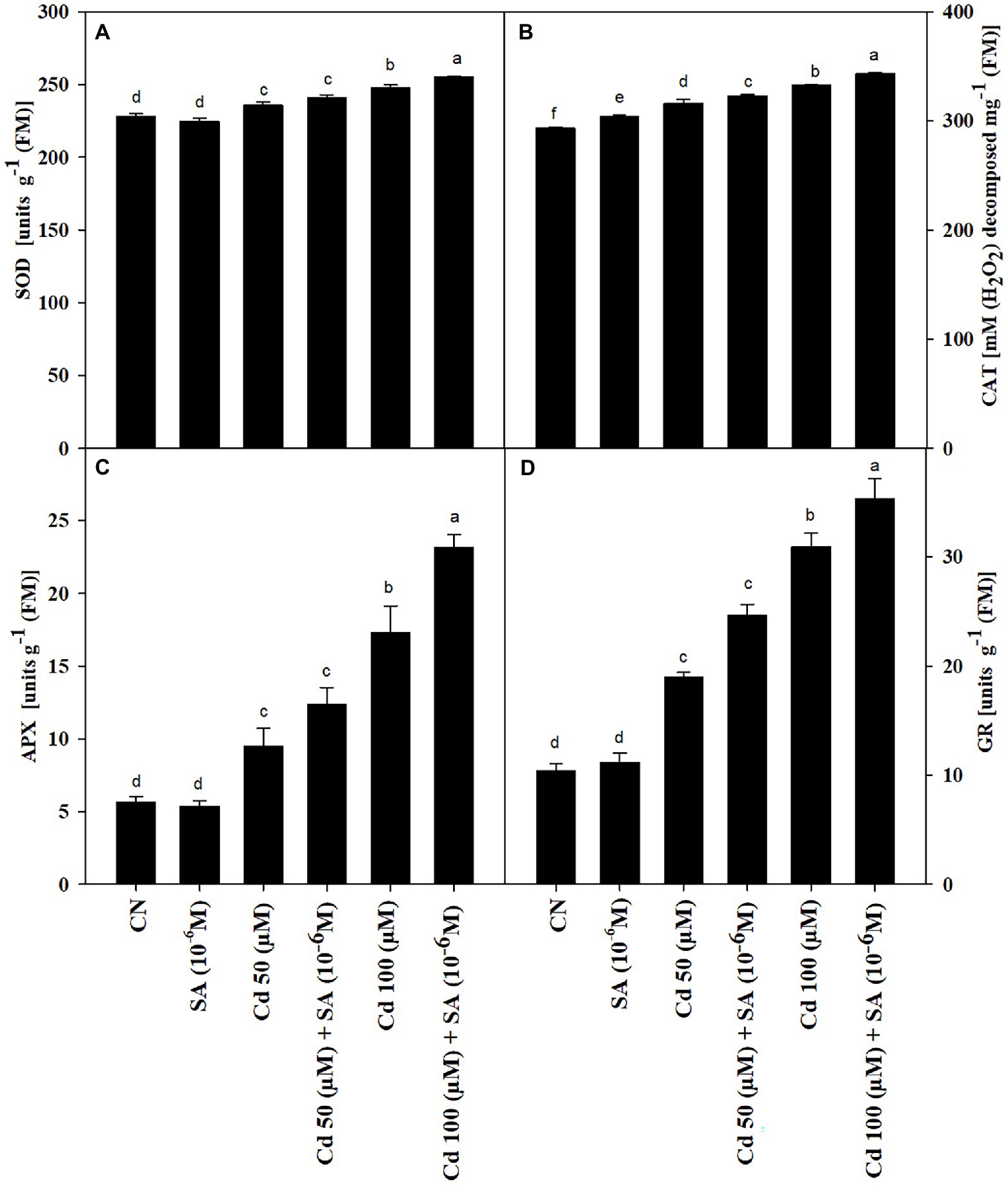
Figure 3. Activities of (A) superoxide dismutase, SOD (units g−1 FM), (B) catalase, CAT (units g−1 FM), (C) ascorbate peroxidase, APX (units g−1 FM), and (D) glutathione reductase, GR (units g−1 FM) of Mentha arvensis cultivar “Kushal” grown under 50 and 100 μm Cd levels and primed with/without salicylic acid. Data are presented as treatments mean ± SE (n = 5). Data followed by the same letters are not significantly different by Duncan multiple range test at (p ≤ 0.05).
Effect of Salicylic Acid and Cadmium on Osmoprotectants and Mineral Nutrient Contents
The Cd treatments triggered the accumulation of pro and GB in the presence or absence of SA. The pro and GB contents increased significantly (p ≤ 0.05) by 1.39-fold and 1.83-fold, and 2.69-fold and 2.98-fold, at 50 μm and 100, respectively, relative to the control plants. Priming with SA increased pro and GB contents by 1.41-fold and 1.29-fold at 50 μm and 1.39-fold and 1.41-fold at 100 μm, relative to the respective Cd-only treatments (Figures 4A,B).
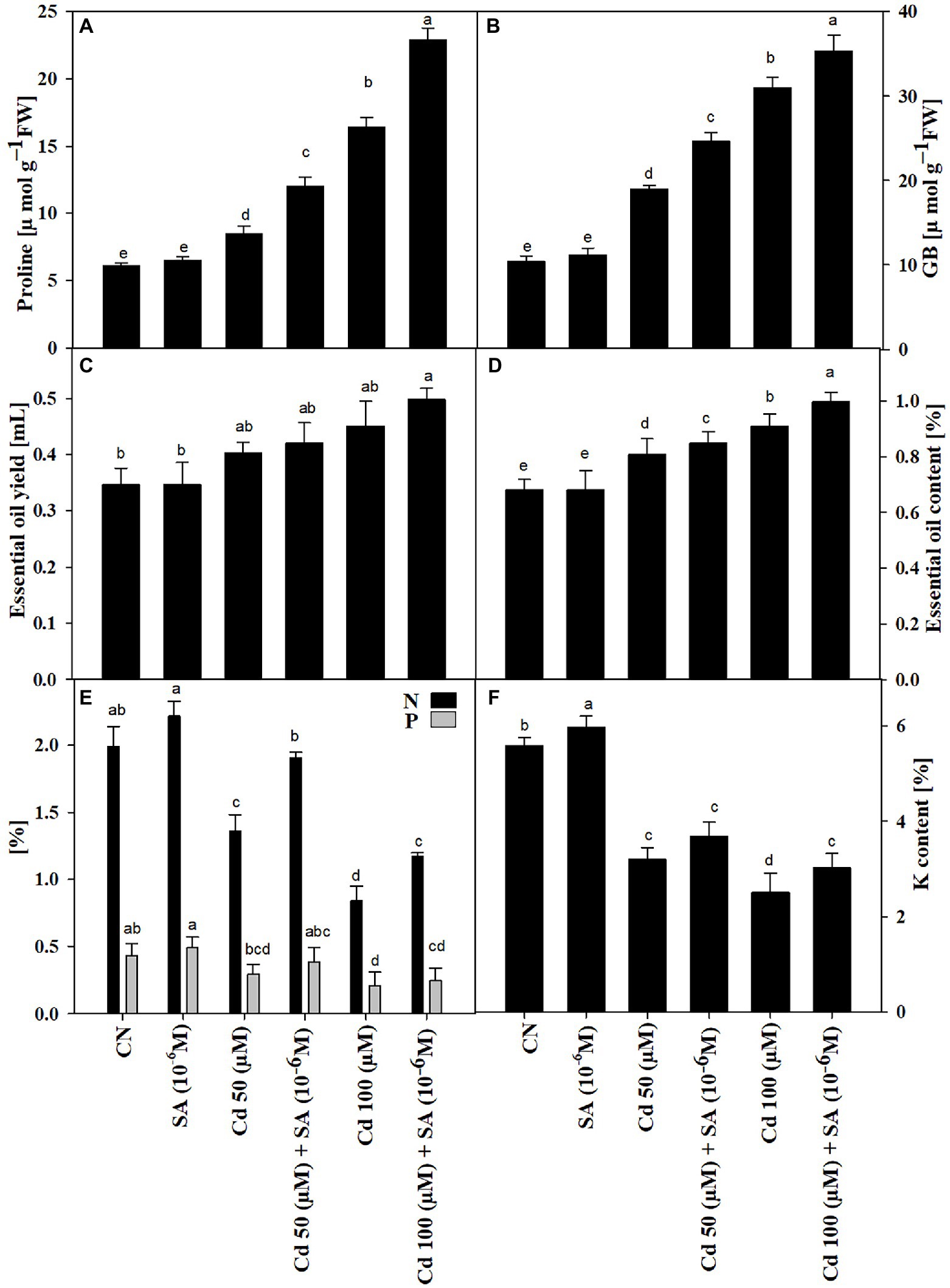
Figure 4. Contents of (A) proline (μmolg−1 FW), (B) glycine betaine, GB (μmolg−1 FW), (C) essential oil yield, EO (mL), (D) essential oil content, EO (%), (E) nitrogen and phosphorous (%), and (F) potassium content (%) of Mentha arvensis cultivar “Kushal” grown under 50 and 100 μm Cd levels and primed with or without salicylic acid. Data are presented as treatments mean ± SE (n = 5). Data followed by the same letters are not significantly different by Duncan multiple range test at p ≤ 0.05.
The Cd treatments decreased the mineral nutrient (N, P, and, K) contents, relative to the control plants. Priming with SA increased N content by 28.42%, P by 23.68%, and K by 13.55% at 50 μm and N by 28.20%, P by 16.94% and K (not significant) by 16.66% at 100 μm, relative to the respective Cd-only treatments (Figures 4E,F).
Salicylic Acid Reduced Cadmium Content as Evidenced by EDX
The plants in the 50 and 100 μm Cd treatments accumulated 97.95 and 98.03% more Cd, respectively, than the control plants. Priming with SA reduced Cd content by 79.59 and 29.41% at 50 and 100 μm, respectively, relative to the respective Cd-only treatments (Figure 5D).
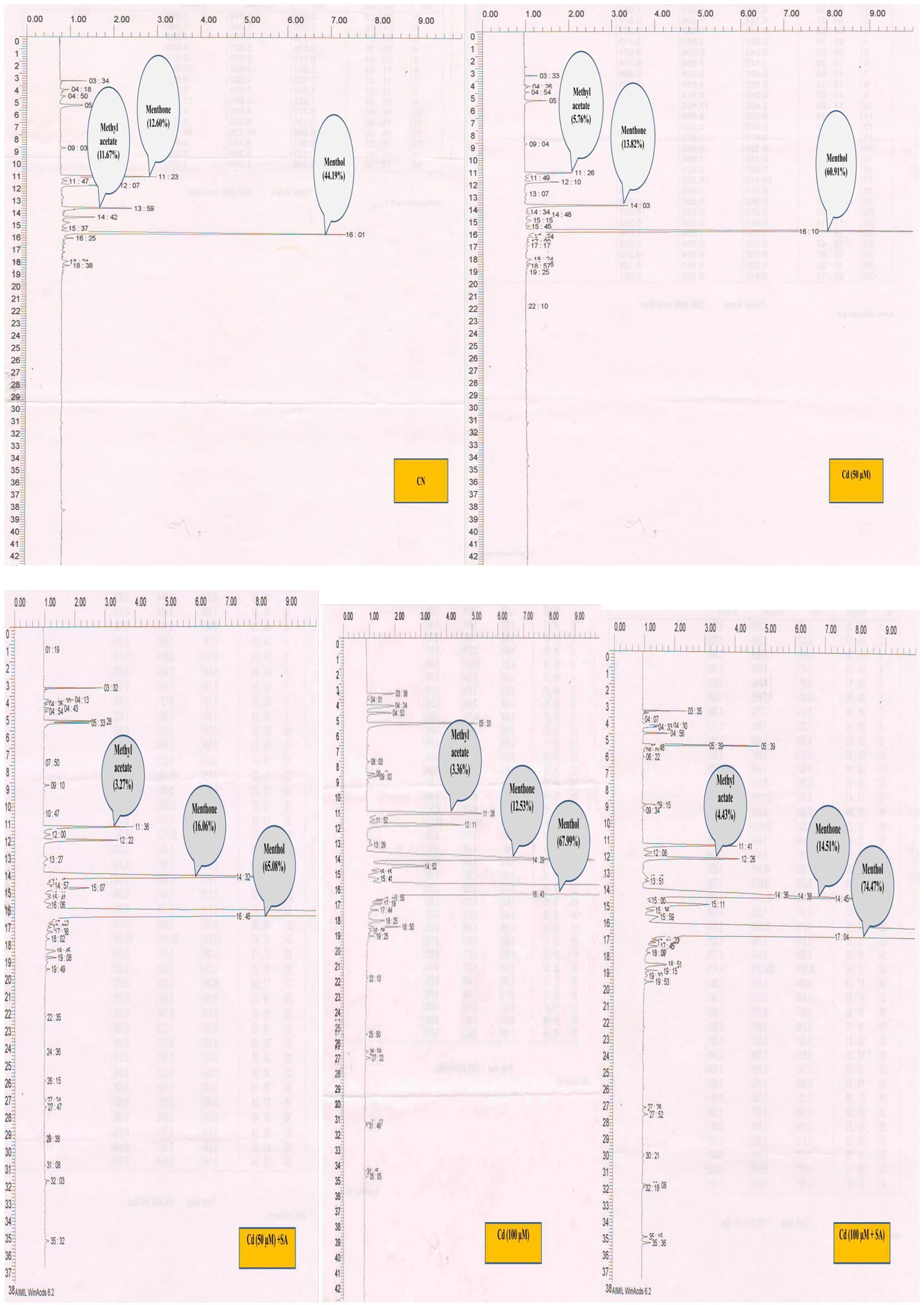
Figure 5. Cadmium-mediated effect of salicylic acid (SA) sucker priming on menthol, menthone and methyl acetate content (GC graph) of Mentha arvensis cultivar “Kushal” in different treatments. GC chromatograms showing the peaks of different components of the essential oil. Abbreviations: CN, control; Cd, cadmium.
Salicylic Acid and Cadmium Enhanced Essential Oil Content and Secondary Metabolite Production
The Cd treatments increased EO content and EO yield. Priming with SA had an additive effect, increasing EO content by 4.76% and EO yield by 8.16% at 50 and 100 μm, respectively, relative to the respective Cd-only treatments (Figures 4C,D).
Priming with SA triggered the production of SM in menthol mint plants in both Cd treatments; 50 μm Cd increased menthol content by 6.40% and menthone by 13.94% but decreased methyl acetate, and 100 μm Cd increased menthol content by 8.70%, menthone by 13.64%, and methyl acetate by 24.15%, relative to the respective Cd-only treatments (Figure 6).
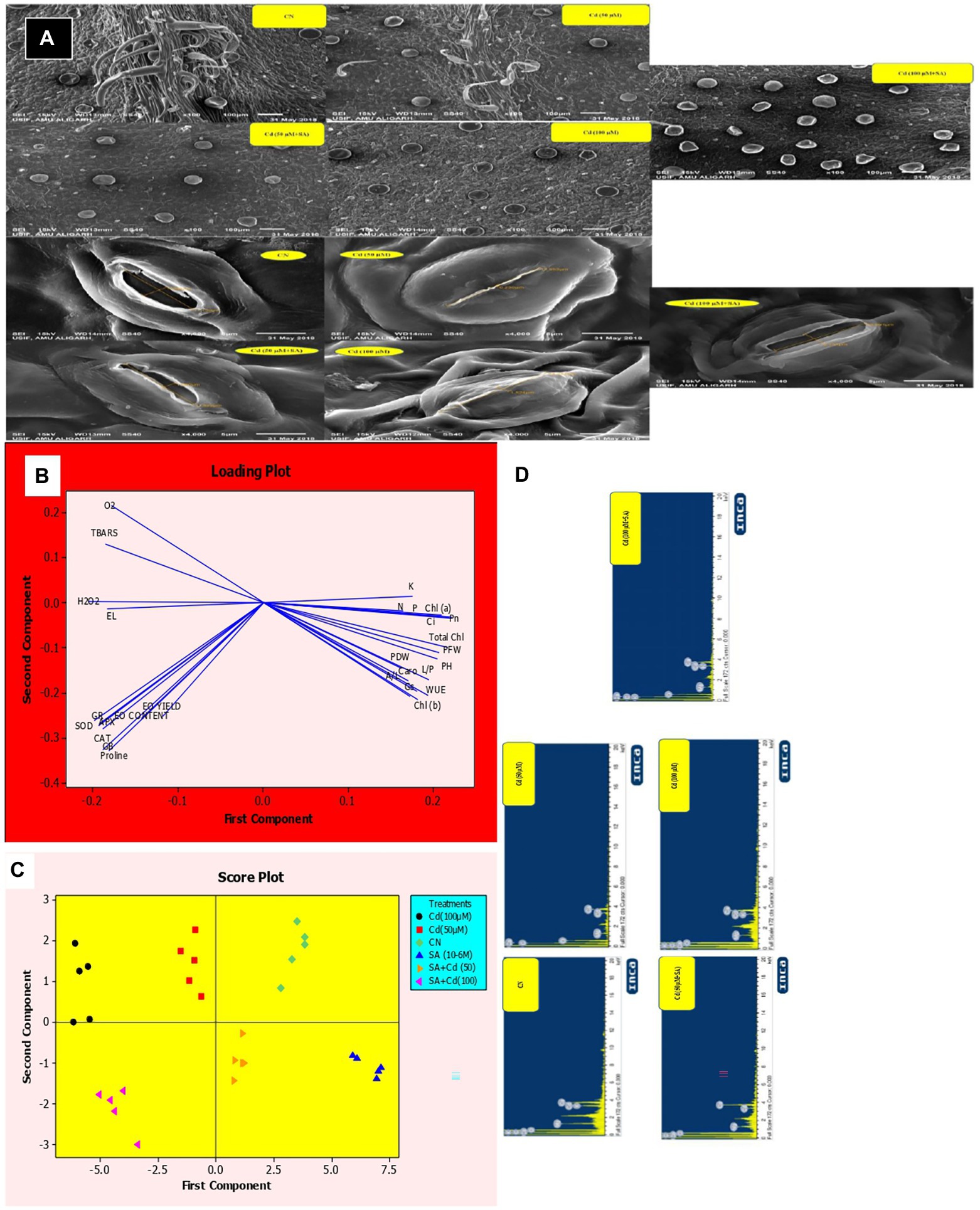
Figure 6. A: Cadmium-mediated effect of salicylic acid (SA) sucker priming on peltate glandular trichome (PGT) density of Mentha arvensis cultivar “Kushal” at different treatments. Abbreviations: CN, control; Cd, cadmium. Stomatal behavior of M. arvensis leaves in different treatments. The opening and closing of stomatal behavior were observed under a scanning electron microscope at 4000 × magnification in M. arvensis leaves treated with 50 and 100 μm CdCl2 at 30 days after transplantation (DAT). A single representative of each treatment is shown. Principal component analysis (PCA) of various parameters of menthol mint plants under cadmium (Cd) and salicylic priming (SA), (B) Loading plot of different parameters showing positive and negative correlations, (C) Score plot differentiating the treatments. PFW, plant fresh weight; PDW, plant dry weight; PH, plant height; L/P, number of leaves per plant; A/L, area per leaf; Chl (a), chlorophyll a; Chl (b), chlorophyll b; Total Chl, total chlorophyll; Caro, carotenoids; PN, net photosynthetic rate; gs, stomatal conductance; Ci, intercellular CO2 concentration; WUE, water use efficiency; O2, superoxide ion; TBARS, thiobarbituric acid reactive substances; EL, electrolyte leakage; H2O2, hydrogen peroxide; SOD, superoxide dismutase activity; CAT, catalase; APX, ascorbate peroxidase; GR, glutathione reductase; GB, glycine betaine; EO, essential oil; N, nitrogen; P, phosphorous; K, potassium, and (D) EDX spectrum showing the content of Cd of M. arvensis cultivar “Kushal” at different treatments. Abbreviations: CN, control; Cd, cadmium.
Effect of Salicylic Acid and Cadmium on Stomatal Response and Peltate Glandular Trichome Density Under Electron Microscopy
SA enriched the number of PGT in menthol mint plants, progressively from the control to the 100 μm Cd + SA treatment (Figure 5A).
Analysis of SEM showed that the Cd treatments induced stomatal closure. The control plants had a normal and open stomatal aperture with 3.08 μm pore size and characteristic guard cells, while the stomata in the 50 and 100 μm Cd treatments had partially closed with 0.25 μm and 1.52 μm pore size, respectively. Priming with SA decreased the Cd effects to open stomata with 1.68 μm and 2.73 μm pore size in the 50 and 100 μm Cd treatments, respectively (Figure 5A).
Discussion
Cadmium contamination of agricultural land has become a principal constraint to crop production (Zaid and Mohammad, 2018; Hussain et al., 2019a; Rizwan et al., 2019a) as it substantially increases oxidative stress, thereby reducing nutritional homeostasis, growth and metabolism, photosynthetic yield, and quality and quantity of crops (Rizwan et al., 2016, 2019b; Zaid and Mohammad, 2018; Greco et al., 2019). This study was conducted on menthol mint plants to unravel various mechanisms underlying priming with SA that improve Cd-inhibited growth, photosynthesis and yield, and quality attributes.
Significant alterations in growth and photosynthesis parameters were observed in menthol mint plants under Cd stress. Similarly, reduced growth and photosynthesis under Cd stress have been observed in peppermint (Ahmad et al., 2018), wheat (Hussain et al., 2019b), tomato (Alyemeni et al., 2018), and Lolium perenne (Zhang et al., 2018). Plant height, fresh and dry weights, and leaf number and area declined in the Cd treatments. The reduced growth and biomass in Cd-treated plants might be because Cd obstructs the progression of the cell cycle and irreversibly alters the proton pumps thereby disrupting membrane permeability (Gomes-Junior et al., 2006; Liu et al., 2015). Thus, the Cd-induced disruptions in membrane permeability may have hindered ion absorption, as reflected in the reduced N, P, and K contents, and thereby hampered plant growth. Priming with SA overcame the Cd-induced growth inhibition. Under Cd stress, SA-induced expression of genes and maintains optimum endogenous SA accumulation that potentiated the Cd-induced growth inhibition (Wang et al., 2019). SA can also reduce the endogenous Cd content and various ROS (Zhang et al., 2015). Thus, the optimum redox balance maintained by SA in the present study under Cd stress increased growth and biomass yield in menthol mint plants.
The values of photosynthetic pigments (Chl a, b, total Chl, and Caro contents) and leaf gas exchange attributes (PN, gs, Ci, and WUE) progressively declined with increasing levels of soil-applied Cd, relative to the control plants (Table 2; Figure 1). The Cd-induced inhibition of chlorophyll biosynthesis is due to the retention of Cd2+ ions in the thylakoids and stroma of the chloroplasts (Lysenko et al., 2018), and the substitution of Mg2+ bound into the chlorophyll molecule (Rydzyński et al., 2018). The reduction in leaf gas exchange attributes at both levels of Cd stress was due to stomatal inhibition (Figure 5A) that restricted gas exchange between the environment and menthol mint plants, and these results are in line with those of Zaid and Mohammad (2018). Priming with SA increased the leaf gas exchange values and nullified the reduction in photosynthesis. Under Cd stress, continuous gas exchange (Figure 5A) due to SA priming induced stomatal recovery to maintain proper gas flow.
The increased Cd content (Figure 5D) under Cd stress declined substantially with SA priming, which was reflected in the increased activity of antioxidant enzymes and biosynthesis of osmolytes (proline and GB; Figures 4A,B). The application of SA decreased the accumulation of Cd in two melon cultivars (Zhang et al., 2015). Karimi and Ghasempour (2019) reported that SA restrained Ni content in Alyssum inflatum. Cd levels in the present study causes a state of oxidative stress by orchestrating the production of ROS (H2O2 and O2–• Figures 2A,D), ion leakage (EL, Figure 2C) and lipid oxidation (TBARS, Figure 2B). However, there is an increment in the activities of antioxidants (SOD, CAT, APX, and GR) (Figures 3A–D), which scavenge Cd-induced excess ROS and maintain an optimum redox homeostatic state (Zaid and Mohammad, 2018). Under Cd stress, SA triggered the expression of selected genes related to ROS and SA to regain the redox state (Li et al., 2019), which in turn enhanced the tolerance of menthol mint plants to Cd stress. Exogenous supplements of SA under Cd stress can increase endogenous SA (Wang et al., 2019); the enhanced biosynthesis of SA in the present study might have scavenged excess ROS and thus lowered TBARS and EL levels in the Cd treatments (Figures 2B,C). Similarly, pre-soaking mungbean seeds in SA solution alleviated Cd-induced toxicity (Roychoudhury et al., 2016). Interestingly, low Cd content in SA-treated plants (Figure 5D) further reduced Cd-induced stress, indicating that the induced up-regulation of redox-related enzymes by SA priming under Cd stress plays a crucial role in scavenging intracellular ROS, thus, highlighting the importance PGRs priming in maintaining redox equilibrium under elevated levels of Cd in menthol mint plants.
Osmolytes, such as proline and GB, are compatible cytosolutes with modulatory roles in plants exposed to metal stress, including detoxifying ROS and chelating HM ions (Ashraf and Foolad, 2007; Bharwana et al., 2014; Zouari et al., 2016). Priming with SA increased the biosynthesis of osmolytes under Cd stress, which was maintained at 100 μM Cd (Figures 4A,B). Increased accumulation of osmolytes with SA priming resulted in the detoxification of ROS and chelation of Cd2+ ions as evidenced by EDX (Figure 5D). Similar SA-mediated increases in osmolytes under metal stress have been reported in crop plants including Alyssum inflatum (Karimi and Ghasempour, 2019), Melissa officinalis (Soltani Maivan et al., 2017), Triticum aestivum (Alamri et al., 2018), and Zea mays (Zanganeh et al., 2018).
Mineral nutrient contents (N, P, and, K) decreased as Cd levels increased from 50 to 100 μm (Figures 4E,F). Cd restricts the uptake of these important mineral elements (Zaid and Mohammad, 2018). In the present study, Cd contamination increased the endogenous Cd content that restricts mineral uptake because excess Cd2+ ions impose antagonistic effects on ion uptake (Nazar et al., 2012). Priming with SA restored mineral contents by either directly increasing their content or indirectly decreasing Cd2+ ions. Our study corroborates the findings of Ahmad et al. (2011). In bean plants exposed to short-term Cd stress, nutrient concentrations also increased in the SA treatment (Saidi et al., 2013).
Plants produce myriad of SMs, which protect against various environmental pressures (Berini et al., 2018). PGRs, especially SA, mediate a tolerance trade-off by eliciting SM production in plants (Singh and Dwivedi, 2018). HMs alter the production of SMs in plants; we observed significant modulation in menthol, menthone, and methyl acetate under Cd stress (Figure 6). Similarly, Paeizi et al. (2018) observed marked changes in medicinal alkaloid production in response to metal stress under PGR in Catharanthus roseus. We also observed an overall increase in EO and SM production with SA priming, which agrees with the findings of Darvizheh et al. (2018) in Echinacea purpurea. The increase in SMs by SA was due to an increase in PGT density. As observed in Figure 5A, SA increased the density of PGT under Cd, with the maximum density observed at 100 μM Cd; hence, increased PGT density is directly responsible for increasing EO and SM biosynthesis. SA also triggered SM biosynthesis in a Taxus baccata callus culture under glucose-mediated alterations (Sarmadi et al., 2018). In a recent study, Mohammadi et al. (2019) reported SA-induced modulations in EO constituents in different ecotypes of Thymus species under water stress. Exogenous PGR-mediated increases in SM production under Cd stress are also due to the up-regulation of transcriptional expressions of genes responsible for their biosynthesis (Chen et al., 2017). Moreover, the sustained increments in growth, photosynthesis, and mineral content of SA-treated plants under Cd stress are likely to culminate in maximized EO and SM contents in menthol mint plants.
Principal component analysis (PCA) identified the relationships between the studied traits, their variation, and whether SA priming can improve Cd-stress resistance and differentiate between the various treatments according to their toxicity and amelioration. Both of the Cd treatments affected plant growth, photosynthetic traits and mineral nutrient contents and they were positively correlated; however, oxidative stress biomarkers, antioxidant enzymes, and EO yield and content, which increased with Cd levels, were also positively correlated and clustered opposite the growth, photosynthetic and nutrient content parameters (Figure 5B). Our hypothesis was further confirmed that Cd-mediated oxidative stress induced repressions in menthol mint plants by affecting growth, photosynthetic traits, and mineral nutrient contents. The most toxic treatment was 100 μm Cd, followed by 50 μm Cd, and both were clustered next to each other. The score plot in PCA distinguishes various treatments according to their toxicities (Figure 6C). Starting from left side, SA under CN was the best treatment. It was followed by CN, SA + Cd (50), Cd (50), SA + Cd (100), and Cd (100). Priming plants with SA ameliorated the Cd-induced overall repressions in menthol mint plants.
Conclusion
In summary priming of menthol mint with SA plants can act as a low-cost, sustainable option in not only overcoming the Cd-induced adverse effects but also increasing its yield and quality attributes. A representation of the present work that how SA priming can overcome Cd stress and increase yield and quality attributes in menthol mint is presented in graphical abstract.
Data Availability Statement
The original contributions presented in the study are included in the article/supplementary material; further inquiries can be directed to the corresponding authors.
Author Contributions
AZ and FM designed the experiment. AZ carried out the experimental analysis, wrote the draft, and revised the manuscript. FM corrected the draft and overall supervised the work. KS critically analyzed the manuscript and edited it for language. All authors contributed to the article and approved the submitted version.
Conflict of Interest
The authors declare that the research was conducted in the absence of any commercial or financial relationships that could be construed as a potential conflict of interest.
Publisher’s Note
All claims expressed in this article are solely those of the authors and do not necessarily represent those of their affiliated organizations, or those of the publisher, the editors and the reviewers. Any product that may be evaluated in this article, or claim that may be made by its manufacturer, is not guaranteed or endorsed by the publisher.
Acknowledgments
AZ is grateful to UGC (New Delhi) India and Aligarh Muslim University, Aligarh, India, for providing the research fellowship. We thank the University Sophisticated Instrumentation Facility (USIF) A.M.U., Aligarh for SEM and EDX analysis. The results reported are part of Ph.D Thesis of Dr. Abbu Zaid.
References
Adams, R. P. (2007). Identification of Essential oil Components by gas Chromatography/mass Spectrometry. 4th Edn. Carol Stream, USA: Allured Publishing Corporation.
Aebi, H. (1984). Catalase in vitro. Methods Enzymol. 105, 121–126. doi: 10.1016/S0076-6879(84)05016-3
Ahmad, B., Jaleel, H., Sadiq, Y., Khan, M. M. A., and Shabbir, A. (2018). Response of exogenous salicylic acid on cadmium induced photosynthetic damage, antioxidant metabolism and essential oil production in peppermint. Plant Growth Regul. 86, 273–286. doi: 10.1007/s10725-018-0427-z
Ahmad, P., Nabi, G., and Ashraf, M. (2011). Cadmium-induced oxidative damage in mustard [(Brassica juncea L.) Czern. & Coss.] plants can be alleviated by salicylic acid. South Afr. J. Bot. 77, 36–44. doi: 10.1016/j.sajb.2010.05.003
Ahmad, B., Zaid, A., Sadiq, Y., Bashir, S., and Wani, S. H. (2019). “Role of selective exogenous elicitors in plant responses to abiotic stress tolerance,” in Plant abiotic Stress Tolerance. eds. M. Hasanuzzaman, K. Hakeem, K. Nahar, and H. Alharby (Cham: Springer), 273–290.
Alamri, S. A. D., Siddiqui, M. H., Al-Khaishany, M. Y., Ali, H. M., Al-Amri, A., and AlRabiah, H. K. (2018). Exogenous application of salicylic acid improves tolerance of wheat plants to lead stress. Adv. Agric. Sci. 6, 25–35.
Alyemeni, M. N., Ahanger, M. A., Wijaya, L., Alam, P., Bhardwaj, R., and Ahmad, P. (2018). Selenium mitigates cadmium-induced oxidative stress in tomato (Solanum lycopersicum L.) plants by modulating chlorophyll fluorescence, osmolyte accumulation, and antioxidant system. Protoplasma 255, 459–469. doi: 10.1007/s00709-017-1162-4
Ashraf, M. F. M. R., and Foolad, M. (2007). Roles of glycine betaine and proline in improving plant abiotic stress resistance. Environ. Exp. Bot. 59, 206–216. doi: 10.1016/j.envexpbot.2005.12.006
Bari, M. A., Akther, M. S., Reza, M. A., and Kabir, A. H. (2019). Cadmium tolerance is associated with the root-driven coordination of cadmium sequestration, iron regulation, and ROS scavenging in rice. Plant Physiol. Biochem. 136, 22–33. doi: 10.1016/j.plaphy.2019.01.007
Bates, L. S., Waldren, R. P., and Teare, I. D. (1973). Rapid determination of free proline for water-stress studies. Plant Soil 39, 205–207. doi: 10.1007/BF00018060
Berini, J. L., Brockman, S. A., Hegeman, A. D., Reich, P. B., Muthukrishnan, R., Montgomery, R. A., et al. (2018). Combinations of Abiotic Factors Differentially Alter Production of Plant Secondary Metabolites in Five Woody Plant Species in the Boreal-Temperate Transition Zone. Front. Plant Sci. 9:1257. doi: 10.3389/fpls.2018.01257
Bharwana, S. A., Ali, S., Farooq, M. A., Iqbal, N., Hameed, A., Abbas, F., et al. (2014). Glycine betaine-induced lead toxicity tolerance related to elevated photosynthesis, antioxidant enzymes suppressed lead uptake and oxidative stress in cotton. Turk. J. Bot. 38, 281–292. doi: 10.3906/bot-1304-65
Bose, S. K., Yadav, R. K., Mishra, S., Sangwan, R. S., Singh, A. K., Mishra, B., et al. (2013). Effect of gibberellic acid and calliterpenone on plant growth attributes, trichomes, essential oil biosynthesis and pathway gene expression in differential manner in Mentha arvensis L. Plant Physiol. Biochem. 66, 150–158. doi: 10.1016/j.plaphy.2013.02.011
Chen, Q., Wu, K., Tang, Z., Guo, Q., Guo, X., and Wang, H. (2017). Exogenous ethylene enhanced the cadmium resistance and changed the alkaloid biosynthesis in Catharanthus roseus seedlings. Acta Physiol. Plant. 39:267. doi: 10.1007/s11738-017-2567-6
DalCorso, G., Farinati, S., Maistri, S., and Furini, A. (2008). How plants cope with cadmium: staking all on metabolism and gene expression. J. Integr. Plant Biol. 50, 1268–1280. doi: 10.1111/j.1744-7909.2008.00737.x
Darvizheh, H., Zahedi, M., Abaszadeh, B., and Razmjoo, J. (2018). Effects of irrigation regime and foliar application of salicylic acid and Spermine on the contents of essential oil and Caffeic acid derivatives in Echinacea purpurea L. J. Plant Growth Regul. 37, 1267–1285. doi: 10.1007/s00344-018-9874-z
Fiske, C. H., and Subbarow, Y. (1925). The colorimetric determination of phosphorus. J. Biol. Chem. 66, 375–400. doi: 10.1016/S0021-9258(18)84756-1
Gao, M., Zhou, J., Liu, H., Zhang, W., Hu, Y., Liang, J., et al. (2018). Foliar spraying with silicon and selenium reduces cadmium uptake and mitigates cadmium toxicity in rice. Sci. Total Environ. 631, 1100–1108. doi: 10.1016/j.scitotenv.2018.03.047
Gomes-Junior, R. A., Moldes, C. A., Delite, F. S., Pompeu, G. B., Gratão, P. L., Mazzafera, P., et al. (2006). Antioxidant metabolism of coffee cell suspension cultures in response to cadmium. Chemosphere 65, 1330–1337. doi: 10.1016/j.chemosphere.2006.04.056
Greco, M., Sáez, C. A., Contreras, R. A., Rodríguez-Rojas, F., Bitonti, M. B., and Brown, M. T. (2019). Cadmium and/or copper excess induce interdependent metal accumulation, DNA methylation, induction of metal chelators and antioxidant defences in the seagrass Zostera marina. Chemosphere 224, 111–119. doi: 10.1016/j.chemosphere.2019.02.123
Grieve, C. M., and Grattan, S. R. (1983). Rapid assay for determination of water soluble quaternary ammonium compounds. Plant Soil 70, 303–307. doi: 10.1007/BF02374789
Guenther, E. (1972). The Essential Oils. History-origin in Plants-Production-Analysis. Vol. 1. Malabar, Florida: Kreiger Publishing Company.
Hald, P. M. (1947). The flame photometer for the measurement of sodium and potassium in biological materials. J. Biol. Chem. 167, 499–510. doi: 10.1016/S0021-9258(17)31003-7
Hassan, M., and Mansoor, S. (2017). Priming seeds with phytohormones alleviates cadmium toxicity in mungbean (Vigna radiate L.Wilczek) seedlings. Pak. J. Bot. 49, 2071–2078.
He, S., He, Z., and Yang, X. (2015). Soil biogeochemistry, plant physiology and phytoremediation of cadmium-contaminated soils. Adv. Agron. 134, 135–225. doi: 10.1016/bs.agron.2015.06.005
Hodges, D. M., DeLong, J. M., Forney, C. F., and Prange, R. K. (1999). Improving the thiobarbituric acid-reactive-substances assay for estimating lipid peroxidation in plant tissues containing anthocyanin and other interfering compounds. Planta 207, 604–611. doi: 10.1007/s004250050524
Hussain, A., Ali, S., Rizwan, M., ur Rehman, M. Z., Qayyum, M. F., Wang, H., et al. (2019a). Responses of wheat (Triticum aestivum) plants grown in a cd contaminated soil to the application of iron oxide nanoparticles. Ecotoxicol. Environ. Saf. 173, 156–164. doi: 10.1016/j.ecoenv.2019.01.118
Hussain, A., Rizwan, M., Ali, Q., and Ali, S. (2019b). Seed priming with silicon nanoparticles improved the biomass and yield while reduced the oxidative stress and cadmium concentration in wheat grains. Environ. Sci. Pollut. Res. 26, 7579–7588. doi: 10.1007/s11356-019-04210-5
Jana, S., and Choudhari, M. A. (1981). Glycolate metabolism of three submerged aquatic angiosperms during ageing. Aquat. Bot. 12, 345–354. doi: 10.1016/0304-3770(82)90026-2
Jisha, K. C., Vijayakumari, K., and Puthur, J. T. (2013). Seed priming for abiotic stress tolerance: an overview. Acta Physiol. Plant. 35, 1381–1396. doi: 10.1007/s11738-012-1186-5
Karimi, N., and Ghasempour, H. R. (2019). Salicylic acid and jasmonic acid restrains nickel toxicity by ameliorating antioxidant defense system in shoots of metallicolous and non-metallicolous Alyssum inflatum Náyr. Populations. Plant Physiol. Biochem. 135, 450–459. doi: 10.1016/j.plaphy.2018.11.015
Khan, M. I. R., Fatma, M., Per, T. S., Anjum, N. A., and Khan, N. A. (2015). Salicylic acid-induced abiotic stress tolerance and underlying mechanisms in plants. Front. Plant Sci. 6:462. doi: 10.3389/fpls.2015.00462
Khanam, D., and Mohammad, F. (2018). Plant growth regulators ameliorate the ill effect of salt stress through improved growth, photosynthesis, antioxidant system, yield and quality attributes in Mentha piperita L. Acta Physiol. Plant. 40:188. doi: 10.1007/s11738-018-2769-6
Khanuja, S. P. S., Kalra, A., Singh, A., Patra, D. D., Patra, N. K., Tondon, S., et al. (2021). Form Bulletin: Menthol Mint Cultivation. Central Institute of Medicinal and Aromatic Plants, Lucknow, India.
Kono, Y. (1978). Generation of superoxide radical during autoxidation of hydroxylamine and an assay for superoxide dismutase. Arch. Biochem. Biophys. 186, 189–195. doi: 10.1016/0003-9861(78)90479-4
Lal, S. K., Kumar, S., Sheri, V., Mehta, S., Varakumar, P., Ram, B., et al. (2018). “Seed Priming: An Emerging Technology to Impart Abiotic Stress Tolerance in Crop Plants,” in Advances in Seed Priming. eds. A. Rakshit and H. Singh (Singapore: Springer), 41–50.
Li, Q., Wang, G., Wang, Y., Yang, D., Guan, C., and Ji, J. (2019). Foliar application of salicylic acid alleviate the cadmium toxicity by modulation the reactive oxygen species in potato. Ecotoxicol. Environ. Saf. 172, 317–325. doi: 10.1016/j.ecoenv.2019.01.078
Lichtenthaler, H. K., and Buschmann, C. (2001). “Chlorophylls and carotenoids: measurement and characterization by UV Vis spectroscopy,” in Current Protocols in Food Analytical Chemistry. eds. R. E. Wrolstad, T. E. Acree, H. An, E. A. Decker, M. H. Penner, and D. S. Reid et al. (New York, NY, USA: John Wiley and Sons), F4.3.1–F4.3.8.
Lindner, R. (1944). Rapid analytical methods for some of the more common inorganic constituents of plant tissues. Plant Physiol. 19, 76–89. doi: 10.1104/pp.19.1.76
Liu, S., Yang, R., Pan, Y., Ma, M., Pan, J., Zhao, Y., et al. (2015). Nitric oxide contributes to minerals absorption, proton pumps and hormone equilibrium under cadmium excess in Trifolium repens L. plants. Ecotoxicol. Environ. Saf. 119, 35–46. doi: 10.1016/j.ecoenv.2015.04.053
Lysenko, E. A., Klaus, A. A., Kartashov, A. V., and Kusnetsov, V. V. (2018). Distribution of cd and other cations between the stroma and thylakoids: a quantitative approach to the search for cd targets in chloroplasts. Photosynth. Res. 139, 1–22. doi: 10.1007/s11120-018-0528-6
Mohammadi, H., Amirikia, F., Ghorbanpour, M., Fatehi, F., and Hashempour, H. (2019). Salicylic acid induced changes in physiological traits and essential oil constituents in different ecotypes of Thymus kotschyanus and Thymus vulgaris under well-watered and water stress conditions. Ind. Crop. Prod. 129, 561–574. doi: 10.1016/j.indcrop.2018.12.046
Moulick, D., Santra, S. C., and Ghosh, D. (2018). Seed priming with se mitigates as-induced phytotoxicity in rice seedlings by enhancing essential micronutrient uptake and translocation and reducing As translocation. Environ. Sci. Pollut. Res. 25, 26978–26991. doi: 10.1007/s11356-018-2711-x
Naeem, M., Aftab, T., Idrees, M., Singh, M., Ali, A., Khan, M. M. A., et al. (2017). Modulation of physiological activities, active constituents and essential oil production of Mentha arvensis L. by concomitant application of depolymerised carrageenan, triacontanol and 28-homobrassinolide. J. Essent. Oil Res. 29, 179–188. doi: 10.1080/10412905.2016.1213188
Nakano, Y., and Asada, K. (1981). Hydrogen peroxide is scavenged by ascorbate-specific peroxidase in spinach chloroplasts. Plant Cell Physiol. 22, 867–880.
Nazar, R., Iqbal, N., Masood, A., Khan, M. I. R., Syeed, S., and Khan, N. A. (2012). Cadmium toxicity in plants and role of mineral nutrients in its alleviation. Amer. J. Plant Sci. 3, 1476–1489. doi: 10.4236/ajps.2012.310178
Nigam, N., Khare, P., Yadav, V., Mishra, D., Jain, S., Karak, T., et al. (2019). Biochar-mediated sequestration of Pb and cd leads to enhanced productivity in Mentha arvensis. Ecotoxicol. Environ. Saf. 172, 411–422. doi: 10.1016/j.ecoenv.2019.02.006
Paeizi, M., Karimi, F., and Razavi, K. (2018). Changes in medicinal alkaloids production and expression of related regulatory and biosynthetic genes in response to silver nitrate combined with methyl jasmonate in Catharanthus roseus in vitro propagated shoots. Plant Physiol. Biochem. 132, 623–632. doi: 10.1016/j.plaphy.2018.10.015
Panuccio, M. R., Chaabani, S., Roula, R., and Muscolo, A. (2018). Bio-priming mitigates detrimental effects of salinity on maize improving antioxidant defense and preserving photosynthetic efficiency. Plant Physiol. Biochem. 132, 465–474. doi: 10.1016/j.plaphy.2018.09.033
Pourghasemian, N., Landberg, T., Ehsanzadeh, P., and Greger, M. (2019). Different response to cd stress in domesticated and wild safflower (Carthamus spp.). Ecotoxicol. Environ. Saf. 171, 321–328. doi: 10.1016/j.ecoenv.2018.12.052
Rizwan, M., Ali, S., Abbas, T., Zia-ur-Rehman, M., Hannan, F., Keller, C., et al. (2016). Cadmium minimization in wheat: a critical review. Ecotoxicol. Environ. Saf. 130, 43–53. doi: 10.1016/j.ecoenv.2016.04.001
Rizwan, M., Ali, S., Akbar, M. Z., Shakoor, M. B., Mahmood, A., Ishaque, W., et al. (2017). Foliar application of aspartic acid lowers cadmium uptake and cd-induced oxidative stress in rice under cd stress. Environ. Sci. Pollut. Res. 24, 21938–21947. doi: 10.1007/s11356-017-9860-1
Rizwan, M., Ali, S., Ali, B., Adrees, M., Arshad, M., Hussain, A., et al. (2019b). Zinc and iron oxide nanoparticles improved the plant growth and reduced the oxidative stress and cadmium concentration in wheat. Chemosphere 214, 269–277. doi: 10.1016/j.chemosphere.2018.09.120
Rizwan, M., Ali, S., ur Rehman, M. Z., Rinklebe, J., Tsang, D. C., Bashir, A., et al. (2018). Cadmium phytoremediation potential of Brassica crop species: a review. Sci. Total Environ. 631, 1175–1191. doi: 10.1016/j.scitotenv.2018.03.104
Rizwan, M., Ali, S., Zia urRehman, M., Adrees, M., Arshad, M., Qayyum, M. F., et al. (2019a). Alleviation of cadmium accumulation in maize (Zea mays L.) by foliar spray of zinc oxide nanoparticles and biochar to contaminated soil. Environ. Pollut. 248, 358–367. doi: 10.1016/j.envpol.2019.02.031
Roychoudhury, A., Ghosh, S., Paul, S., Mazumdar, S., Das, G., and Das, S. (2016). Pre-treatment of seeds with salicylic acid attenuates cadmium chloride-induced oxidative damages in the seedlings of mung bean (Vigna radiate L.Wilczek). Acta Physiol. Plant. 38:11. doi: 10.1007/s11738-015-2027-0
Rydzyński, D., Piotrowicz-Cieślak, A. I., Grajek, H., and Michalczyk, D. J. (2018). Chlorophyll degradation by tetracycline and cadmium in spinach (Spinacia oleracea L.) leaves. Int. J. Environ. Sci. Technol. 16, 6301–6314. doi: 10.1007/s13762-018-2142-8
Saidi, I., Ayouni, M., Dhieb, A., Chtourou, Y., Chaïbi, W., and Djebali, W. (2013). Oxidative damages induced by short-term exposure to cadmium in bean plants: protective role of salicylic acid. South Afr. J. Bot. 85, 32–38. doi: 10.1016/j.sajb.2012.12.002
Santisree, P., Jalli, L. C. L., Bhatnagar-Mathur, P., and Sharma, K. K. (2020). Emerging roles of salicylic acid and jasmonates in plant abiotic stress responses. Protective chemical agents in the amelioration of plant abiotic stress. Biochem. molecular perspect. 1, 342–373. doi: 10.1002/9781119552154.ch17
Sarmadi, M., Karimi, N., Palazón, J., Ghassempour, A., and Mirjalili, M. H. (2018). The effects of salicylic acid and glucose on biochemical traits and taxane production in a Taxus baccata callus culture. Plant Physiol. Biochem. 132, 271–280. doi: 10.1016/j.plaphy.2018.09.013
Savvides, A., Ali, S., Tester, M., and Fotopoulos, V. (2016). Chemical priming of plants against multiple abiotic stresses: mission possible? Trends Plant Sci. 21, 329–340. doi: 10.1016/j.tplants.2015.11.003
Shah, A. A., Ahmed, S., Ali, A., and Yasin, N. A. (2020a). 2-Hydroxymelatonin mitigates cadmium stress in cucumis sativus seedlings: modulation of antioxidant enzymes and polyamines. Chemosphere 243:125308. doi: 10.1016/j.chemosphere.2019.125308
Shah, A. A., Bibi, F., Hussain, I., Yasin, N. A., Akram, W., Tahir, M. S., et al. (2020b). Synergistic effect of bacillus thuringiensis iags 199 and putrescine on alleviating cadmium-induced phytotoxicity in capsicum annum. Plan. Theory 9:1512. doi: 10.3390/plants9111512
Shahnawaz, M. D., Chouhan, R., and Sanadhya, D. (2017). Impact of aluminium toxicity on physiological aspects of barley (Hordeum vulgare L.) cultivars and its alleviation through ascorbic acid and salicylic acid seed priming. Int. J. Curr. Microbiol. App. Sci. 6, 875–891. doi: 10.20546/ijcmas.2017.605.098
Sheteiwy, M. S., Gong, D., Gao, Y., Pan, R., Hu, J., and Guan, Y. (2018). Priming with methyl jasmonate alleviates polyethylene glycol-induced osmotic stress in rice seeds by regulating the seed metabolic profile. Environ. Exp. Bot. 153, 236–248. doi: 10.1016/j.envexpbot.2018.06.001
Singh, A., and Dwivedi, P. (2018). Methyl-jasmonate and salicylic acid as potent elicitors for secondary metabolite production in medicinal plants: A review. J. Pharma. Phytochem. 7, 750–757.
Smith, L. K., Vierheller, T. L., and Thorne, C. A. (1988). Assay of glutathione reductase in crude tissue homogenates using 5,5-thiobis (2-nitro benzoic acid). Ann. Biochem. 175, 408–413. doi: 10.1016/0003-2697(88)90564-7
Soltani Maivan, E., Radjabian, T., Abrishamchi, P., and Talei, D. (2017). Physiological and biochemical responses of Melissa officinalis L. to nickel stress and the protective role of salicylic acid. Arch. Agron. Soil Sci. 63, 330–343. doi: 10.1080/03650340.2016.1207241
Sullivan, C. Y., and Ross, W. M. (1979). “Selecting the drought and heat resistance in grain sorghum,” in Physiology in crop Plants. eds. H. Mussel and R. C. Staples (New York: Wiley), 263–328.
Sytar, O., Kumari, P., Yadav, S., Brestic, M., and Rastogi, A. (2018). Phytohormone priming: regulator for heavy metal stress in plants. J. Plant Growth Regul. 38, 739–752. doi: 10.1007/s00344-018-9886-8
Tiwari, P. (2016). Recent advances and challenges in trichome research and essential oil biosynthesis in Mentha arvensis L. Ind. Crop. Prod. 82, 141–148. doi: 10.1016/j.indcrop.2015.11.069
Wang, A. G., and Luo, G. H. (1990). Quantitative relation between the reaction of hydroxylamine and superoxide anion radicals in plants. Plant Physiol. Commun. 6, 55–57.
Wang, Y. Y., Wang, Y., Li, G. Z., and Hao, L. (2019). Salicylic acid-altering Arabidopsis plant response to cadmium exposure: underlying mechanisms affecting antioxidation and photosynthesis-related processes. Ecotoxicol. Environ. Saf. 169, 645–653. doi: 10.1016/j.ecoenv.2018.11.062
Zaid, A., Bhat, J. A., Wani, S. H., and Masoodi, K. Z. (2019a). Role of nitrogen and sulfur in mitigating cadmium induced metabolism alterations in plants. The J. Plant Sci. Res. 35, 121–141. doi: 10.32381/JPSR.2019.35.01.11
Zaid, A., and Mohammad, F. (2018). Methyl jasmonate and nitrogen interact to alleviate cadmium stress in Mentha arvensis by regulating physio-biochemical damages and ros detoxification. J. Plant Growth Regul. 37, 1331–1348. doi: 10.1007/s00344-018-9854-3
Zaid, A., Mohammad, F., and Fariduddin, Q. (2020). Plant growth regulators improve growth, photosynthesis, mineral nutrient and antioxidant system under cadmium stress in menthol mint (Mentha arvensis L.). Physiol. Mol. Biol. Plants 26, 25–39. doi: 10.1007/s12298-019-00715-y
Zaid, A., Mohammad, F., Wani, S. H., and Siddique, K. M. (2019b). Salicylic acid enhances nickel stress tolerance by up-regulating antioxidant defense and glyoxalase systems in mustard plants. Ecotoxicol. Environ. Saf. 180, 575–587. doi: 10.1016/j.ecoenv.2019.05.042
Zanganeh, R., Jamei, R., and Rahmani, F. (2018). Impacts of seed priming with salicylic acid and sodium hydrosulfide on possible metabolic pathway of two amino acids in maize plant under lead stress. Mol. Biol. Res. Comm. 7:83. doi: 10.22099/mbrc.2018.29089.1317
Zhang, J., Sun, W., Li, Z., Liang, Y., and Song, A. (2009). Cadmium fate and tolerance in rice cultivars. Agr. Sus. Devel. 29, 483–490. doi: 10.1051/agro/2009008
Zhang, H., Xu, N., Li, X., Long, J., Sui, X., Wu, Y., et al. (2018). Arbuscular Mycorrhizal Fungi (Glomus mosseae) improves growth, photosynthesis and protects photosystem II in leaves of Lolium perenne L. in cadmium contaminated soil. Front. Plant Sci. 9:01156. doi: 10.3389/fpls.2018.01156
Zhang, Y., Xu, S., Yang, S., and Chen, Y. (2015). Salicylic acid alleviates cadmium-induced inhibition of growth and photosynthesis through upregulating antioxidant defense system in two melon cultivars (Cucumis melo L.). Protoplasma 252, 911–924. doi: 10.1007/s00709-014-0732-y
Zouari, M., Elloumi, N., Ahmed, C. B., Delmail, D., Rouina, B. B., Abdallah, F. B., et al. (2016). Exogenous proline enhances growth, mineral uptake, antioxidant defense, and reduces cadmium-induced oxidative damage in young date palm (Phoenix dactylifera L.). Ecol. Eng. 86, 202–209. doi: 10.1016/j.ecoleng.2015.11.016
Keywords: cadmium stress, Mentha arvensis, photosynthesis, salicylic acid, secondary metabolites
Citation: Zaid A, Mohammad F and Siddique KHM (2022) Salicylic Acid Priming Regulates Stomatal Conductance, Trichome Density and Improves Cadmium Stress Tolerance in Mentha arvensis L. Front. Plant Sci. 13:895427. doi: 10.3389/fpls.2022.895427
Edited by:
Mohammad Anwar Hossain, Bangladesh Agricultural University, BangladeshReviewed by:
Tetiana Kalachova, Academy of Sciences of the Czech Republic, CzechiaAnis Ali Shah, University of Education Lahore, Pakistan
Copyright © 2022 Zaid, Mohammad and Siddique. This is an open-access article distributed under the terms of the Creative Commons Attribution License (CC BY). The use, distribution or reproduction in other forums is permitted, provided the original author(s) and the copyright owner(s) are credited and that the original publication in this journal is cited, in accordance with accepted academic practice. No use, distribution or reproduction is permitted which does not comply with these terms.
*Correspondence: Abbu Zaid, emFpZGFiYnUxOWdtYWlsLmNvbQ==; Kadambot H. M. Siddique, a2FkYW1ib3Quc2lkZGlxdWVAdXdhLmVkdS5hdQ==
†ORCID: Abbu Zaid, orcid.org/0000-0002-2090-0212