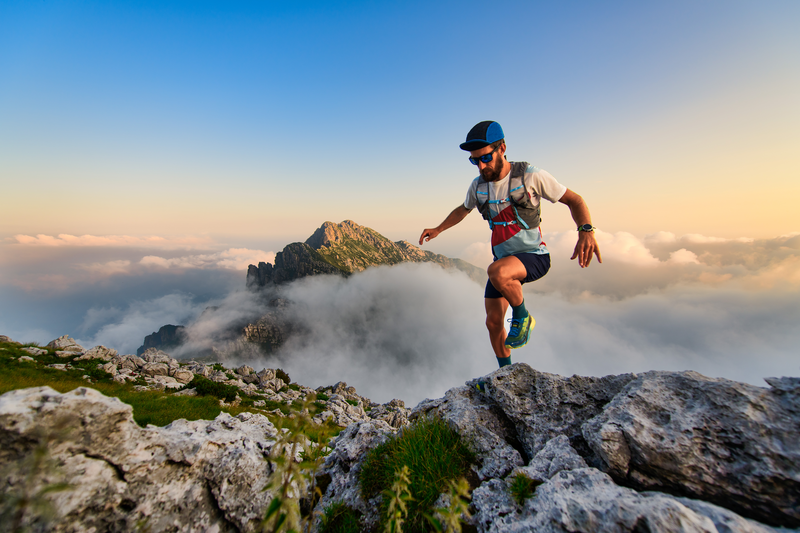
94% of researchers rate our articles as excellent or good
Learn more about the work of our research integrity team to safeguard the quality of each article we publish.
Find out more
REVIEW article
Front. Plant Sci. , 07 April 2022
Sec. Plant Breeding
Volume 13 - 2022 | https://doi.org/10.3389/fpls.2022.892381
This article is part of the Research Topic Marker-Assisted Selection (MAS) in Crop Plants View all 45 articles
Plant trichomes are specialized epidermal cells that are widely distributed on plant aerial tissues. The initiation and progression of trichomes are controlled in a coordinated sequence of multiple molecular events. During the past decade, major breakthroughs in the molecular understanding of trichome development were achieved through the characterization of various trichomes defective mutants and trichome-associated genes, which revealed a highly complex molecular regulatory network underlying plant trichome development. This review focuses on the recent millstone in plant trichomes research obtained using genetic and molecular studies, as well as ‘omics’ analyses in model plant Arabidopsis and fiber crop cotton. In particular, we discuss the latest understanding and insights into the underlying molecular mechanisms of trichomes formation at multiple dimensions, including at the chromatin, transcriptional, post-transcriptional, and post-translational levels. We summarize that the integration of multi-dimensional trichome-associated genes will enable us to systematically understand the molecular regulation network that landscapes the development of the plant trichomes. These advances will enable us to address the unresolved questions regarding the molecular crosstalk that coordinate concurrent and ordered the changes in cotton fiber initiation and progression, together with their possible implications for genetic improvement of cotton fiber.
Plant trichomes are the unicellular or multicellular appendages originating from epidermal cells and are widely distributed on the surface of different organs of plants, i.e., stems, leaves, petioles, flowers, and seed coats (Hülskamp et al., 1994; Pattanaik et al., 2014). Trichomes on vegetative organs are physical barriers that protect against ultraviolet (UV) radiation, excessive transpiration, and insect herbivory (Serna and Martin, 2006; Wang et al., 2019b). Seed coat trichomes primarily increase seed dispersal, but are also significant resources in the textile industry (e.g., cotton fibers; Wang et al., 2019b). Trichome development is initiated by a variety of external environmental factors and endogenous developmental signals (Serna and Martin, 2006; Qi et al., 2011). Environmental factors include wounding and insect attack, which are associated with trichome phenotypes. Variations in trichome density are likely a result of adaptation to different environments (Arteaga et al., 2021). Endogenous developmental signals include phytohormone signals. Gibberellic acid (GA) and jasmonic acid (JA) alter trichome development by crosstalk with transcription factors (Yan et al., 2017). In addition, cytokinin (CK), salicylic acid (SA), and ethylene also affect trichome development (Maes et al., 2008; Matías-Hernández et al., 2015; Yu et al., 2022).
Arabidopsis thaliana, a model plant, has trichomes that are typically unicellular, non-glandular, and have two to three branches (Szymanski et al., 2000; Grebe, 2012). Similarly, cotton fibers are also composed of non-glandular (but non-branched) single cells (Wang et al., 2019b). In Arabidopsis, trichome development generally involves initiation followed by four rounds of endoreplication and branching (Hülskamp et al., 1994; Yang and Ye, 2013; Figure 1A). Many studies have uncovered that trichome initiation begins when epidermal cells collect signals from neighboring cells and subsequently undergo cell differentiation regulated by evolutionarily conserved transcription factors that are involved in patterning processes and trichome morphogenesis. Cotton fiber development is also divided into four major sequential and overlapping developmental stages: fiber initiation, fiber elongation (primary cell wall synthesis), cell wall thickening (secondary cell wall deposition), and fiber maturation (Figure 1B). Currently, molecular mechanisms of fiber development (especially initiation and elongation) are widely studied, and some key genes have been characterized. Due to their unique cell structures, trichomes serve as an excellent model system to study all aspects of plant differentiation at the single cell level including cell fate differentiation and morphogenesis (Balkunde et al., 2010; Yang and Ye, 2013).
Figure 1. Core regulators controlling Arabidopsis trichome development and cotton fiber development. (A) Key regulators are involved in Arabidopsis trichome development. GL1-GL3/EGL3-TTG1 is the core complex to regulate Arabidopsis trichome initiation, which is needed for the activation of GL2/TTG2. C2H2 zinc finger proteins GIS3, ZFP5, and ZFP6 that regulate trichome initiation through GA and CK signalling (Sun et al., 2015). GL2 function partially redundant with TTG2, downstream of the GL1-GL3/EGL3-TTG1 in trichome initiation. Single-repeat MYBs TRY, CPC, ETC1, and TCL1 prevent the formation of GL1-GL3/EGL3-TTG1 complex. TEM2 significantly represses the expression GL1 and EGL3 to inhibit trichome development. Some regulators affect trichome development by endoreduplication pathway. In addition, KAK and RPT2a inhibit the expression of GL3/EGL3 causing reduced trichome branching. In addition to regulating trichome initiation, GL3/EGL3 is also involved in trichome branching. TCP4 suppresses trichome branching by direct transcriptional activation of GIS (Wang et al., 2019b). (B) Key regulators are involved in cotton fiber development. MYB, bHLH, and HD-ZIP TFs are important regulators in cotton fiber development. GhMYB25 and GhMYB25-like have been identified as regulators of fiber initiation, and GhJAZ2 negatively regulates fiber initiation by interacting with GhMYB25-like. GhMML4 is known to regulate the fiber development by binding with GhWDR. GhHD1 regulates fiber initiation, while GhHOX3 regulates fiber elongation. GhHOX3 interacts with GhHD1, enhancing transcriptional activity of GhHOX3. In fiber elongation stage, GhMYB212 directly controls expression of sucrose transporter gene GhSWEET12 (Wang et al., 2019b). GhFP1 directly binds to GhDWF4 and GhCPD promoters to activate BR biosynthesis and signaling to regulate fiber elongation. GhMYB7 directly regulates fiber cellulose synthesis by binding to three different cis-elements in the GhCesA4, GhCesA7, and GhCesA8 promoters. GhTCP4 maintains the balance between cotton fiber cell elongation and cell wall synthesis by interacting with GhHOX3.
Cotton fiber and Arabidopsis trichome initiation share some similarities but have a different mode of molecular regulation (Tian and Zhang, 2021; Wang et al., 2021a). Several key genes involved in Arabidopsis trichome initiation have been identified that form a transcriptional network involving three major groups of transcription factors: R2R3-type MYB-basic helix–loop–helix (bHLH)-WD40 repeat (WDR) protein (Payne et al., 2000; Serna and Martin, 2006; Grebe, 2012; Pattanaik et al., 2014; Tian and Zhang, 2021). These regulatory proteins form a trimeric activator complex (MBW complex) that positively regulates trichome initiation by activating expression of GLABRA2 (GL2; Arteaga et al., 2021). Genetic and molecular evidences have demonstrated that the MBW complex interacts with DELLA and JAZ proteins and mediates GA and JA signaling to control trichome initiation (Qi et al., 2014). In addition, mutations in MBW complex genes lead to smaller and less branched trichomes (Payne et al., 2000). Single repeat R3-MYB proteins have been reported to repress trichome initiation by interfering the function of the MBW complex (Schellmann et al., 2002; Wang et al., 2007; Wester et al., 2009; Wang and Chen, 2014), which results in the repression of GL2 (Serna and Martin, 2006; Ishida et al., 2008; Wang and Chen, 2014; Doroshkov et al., 2019). In cotton, some genes that control fiber development have been identified as homologues of Arabidopsis trichome regulators (Walford et al., 2012; Guan et al., 2014a; Table 1). Ectopic expression of some of these cotton homologues alters trichome development in Arabidopsis (Wang et al., 2004; Zhang et al., 2010; Guan et al., 2014a). However, a cotton (Gossypium hirsutum) MIXTA-like transcription factor, GhMML4_D12, is known to regulate the fiber development by binding with GhWDR but not with a bHLH protein (Tian and Zhang, 2021). Moreover, several spontaneous “lintless” or “naked” cotton mutants have normal leaf and stem trichomes (Ruan, 2005; Tian and Zhang, 2021; Wang et al., 2021a). This evidence suggests that cotton fiber initiation differs from trichome initiation on vegetative organs.
As described above, the regulatory network of trichome development is rigorous and complex. Thus, it is necessary to dissect molecular mechanisms of trichome development in multiple dimensions in order to understand the genetic mechanisms involved. In this review, we focus on multi-dimensional regulatory modes in Arabidopsis and cotton, describing the latest results in research related to chromatin-mediated, transcriptional, post-transcriptional, and post-translational regulation of trichome development (Figure 2). These data provide valuable insight into the regulatory network of trichome development, ultimately accelerating understanding of the molecular mechanisms of cotton fiber development.
Figure 2. Multi-dimensional regulation of Arabidopsis trichome and cotton fiber development. The control of trichome and fiber development involves multi-dimensional regulation. Here shows chromatin-mediated, transcriptional, post-transcriptional, and post-translational regulation modes. Chromatin-mediated regulation of trichome and fiber development, including histone modification and maintenance of chromatin states. Multiple transcription factors are involved in trichome and fiber development. The post-transcriptional regulation of trichome and fiber development is mainly caused by miRNA. Post-translational modifications, such as ubiquitination, is important in regulating trichome and fiber development. White words represent the genes are involved in trichome-related genes in Arabidopsis; Red words represents the genes are involved in cotton fiber development. Pre-miRNA: premature microRNA; m6A: N6-methyladenosine; RISC: RNA-induced silencing complex; Ub: ubiquitin.
Transcription factors (TFs) are the main drivers of evolution and domestication because they have the potential to fine-tune traits and improve crops (Schilling et al., 2019). Previous studies have clarified the identity of some TFs involved in trichome improvement (Oppenheimer et al., 1991; Rerie et al., 1994; Morohashi et al., 2007; Bouyer et al., 2008). For example, R2R3-MYB, bHLH, WD40, and HD-ZIP IV TFs appear to be essential for trichome development. Analysis of these important transcriptional regulators involved in the development of epidermal hair of Arabidopsis and fiber of cotton could contribute to systematically understand the molecular mechanisms of trichome development.
MYB transcription factor constitutes a large and functionally diverse plant transcription factor family with a major role in plant-specific processes including biotic and abiotic stress responses (Mengiste et al., 2003), cell fate and identity determination (Kang et al., 2009), growth and developmental processes (Millar and Gubler, 2005), general and specialized metabolic responses (Zhou et al., 2009). [DE]Lx2[RK]x3Lx6Lx3R is a conserved motif in MYB proteins that is critical for interaction with GLABRA3 (GL3; Zimmermann et al., 2004). In Arabidopsis, GL1 encodes an R2R3-MYB TF, a regulator that functions at the earliest known stage of trichome initiation. Mutations in the GL1 gene cause glabrous leaves (Oppenheimer et al., 1991). MYB23 is functionally equivalent to GL1, and they redundantly control trichome initiation at leaf edges (Kirik et al., 2005). MYB82 driven by the promoter of GL1 was able to rescue the gl1 mutant glabrous phenotypes, revealing that the MYB82 protein is also functionally similar to the GL1 protein (Liang et al., 2014). MYB5 regulates trichome elongation and branching, and minimal changes in trichome morphology have been noted in the myb5 mutant; however, the myb5/myb23 double mutant produces a higher number of small and two-branched trichomes than the single mutant. Thus, MYB5 and MYB23 regulate trichome branching and extension in a partially redundant manner (Li et al., 2009). MYB106/NOK is a MIXTA-like TF that negatively regulates trichome branching in Arabidopsis (Jakoby et al., 2008). A group of single repeat R3-MYB TFs, including TRIPTYCHON (TRY; Schellmann et al., 2002), CAPRICE (CPC; Schellmann et al., 2002), TRICHOMELESS (TCL) 1 and 2 (Wang et al., 2007; Gan et al., 2011), and ENHANCER OF TRY AND CPC (ETC) 1–3 (Kirik et al., 2004; Wester et al., 2009) are negative regulators of trichome formation. For example, TCL1 negatively regulates trichome initiation, and over-expression of TCL1 directly suppresses GL1 transcription (Wang et al., 2007).
In cotton, some MYB TFs have evolved special roles in fiber development. GhMYB2 is a key regulator of fiber development in cotton and is homologous to AtGL1, which functions in trichome initiation in Arabidopsis (Guan et al., 2014a). Ectopic expression of Gossypium arboreum MYB2 and GhMYB2A can complement the gl1 mutant phenotype in Arabidopsis (Wang et al., 2004; Guan et al., 2014a). GhMYB5 exhibits a high similarity to AtMYB5 and is related to fiber initiation and elongation (Wang et al., 2021c). Sequence variations in the cis-elements of Gossypium barbadense MYB5 and GhMYB5 lead to differences in gene expression that are associated with natural variation in fiber development (Wang et al., 2021c). GhMYB109 plays key role in cotton fiber development; GhMYB109 knockdowns show a significant reduction in fiber length, unveiling a largely conserved role of R2R3-MYB genes in the cell fate determination (Pu et al., 2008). Fibers are elongated using sucrose as a direct carbon source. GhMYB212 directly controls expression of GhSWEET12, a sucrose transporter gene, in expanding fibers and is therefore required for cotton fiber elongation (Sun et al., 2019). MYBMIXTA-like TFs (MMLs) belong to the special subgroup 9 of R2R3-MYB proteins (Brockington et al., 2012; Hu et al., 2016). GhMML7 (GhMYB25) and GhMML3 (GhMYB25-like) proteins have been identified as regulators of fiber initiation and elongation. GhMML7-silenced cotton plants show a significant reduction in fiber length and leaf trichome numbers (Machado et al., 2009), whereas silencing GhMML3 has no significant influence on trichome development (Walford et al., 2011). GhJAZ2, a repressor of JA signaling, negatively regulates fiber initiation by interacting with GhMML3 (Hu et al., 2016). GhMML4 is a sister MYB TF to GhMML3 that controls lint fiber development. Additionally, both of these two genes are tandemly arranged on the D12 chromosome (Wu et al., 2018). Cellulose contents during secondary cell wall (SCW) deposition phase of mature cotton fiber reach up to 90% (Han et al., 2013; Huang et al., 2021), and GhMYB7 directly regulates fiber cellulose synthesis by binding to three different cis-elements in the GhCesA4, GhCesA7, and GhCesA8 promoters (Huang et al., 2021). Over-expression of GhMYB7 significantly accelerates cellulose biosynthesis in the SCW, resulting in shorter fibers with thicker walls.
In Arabidopsis, GL3 and ENHANCER OF GLABRA3 (EGL3) are functionally redundant bHLH TFs (Payne et al., 2000). Although mutation of gl3 modestly affects trichome number and branching, egl3 mutants have no significant trichome defects (Morohashi et al., 2007). Notably, gl3/egl3 mutants have a completely glabrous phenotype (Zhang et al., 2003). Trichome initiation is co-regulated by GL1 together with GL3/EGL3 (Schiefelbein, 2003). GL3 and EGL3 are upregulated during trichome initialization and in young trichomes, then expression decreases in mature trichomes.
In cotton, GhDEL65 (a functional homologue of Arabidopsis GL3 and EGL3) regulates early fiber development (Shangguan et al., 2016). Ectopic expression of GhDEL65 in the Arabidopsis gl3/egl3 double mutant partly rescues the trichome-less phenotype, and over-expressing GhDEL65 in wild-type Arabidopsis plants results in increased trichome density (Shangguan et al., 2016). GhFP1 encodes a bHLH protein that positively regulates fiber elongation (Liu et al., 2020). GhFP1 directly binds to the GhDWF4 and GhCPD promoters to activate brassinosteroid (BR) biosynthesis and signaling, as well as appropriate concentration of BR promotes cotton fiber elongation (Sun et al., 2005). Over-expression of GhFP1 promotes trichome development in Arabidopsis (Liu et al., 2020). PACLOBUTRAZOL RESISTANCE 1 (PRE1) is specifically expressed in fiber cells, and core cis-element variation in GhPRE1 contributes to fiber formation (Zhao et al., 2018).
The Arabidopsis protein TRANSPARENT TESTA GLABRA1 (TTG1) has four WD40 repeats and regulates trichome differentiation; loss of TTG1 function results in a glabrous phenotype (Walker et al., 1999). TTG1 physically binds with GL3 and forms a complex to control trichome initiation (Payne et al., 2000).
In cotton, GhTTG1 and GhTTG3 are functional homologues of AtTTG1 (Humphries et al., 2005). Expression of either gene in Arabidopsis ttg1 mutants rescues trichome development. GhWDR is a novel WD40-repeat protein. The number of WD40-repeat domains in GhWDR differs from those of AtTTG1 and GhTTG1-GhTTG4, implying functional differentiation (Tian et al., 2020). GhWDR is expressed in the entire process of fiber development, suggesting key contributions (Tian et al., 2020).
A highly conserved DNA-binding homeodomain (HD) and leucine zipper (ZIP) motif characterize the HD-ZIP proteins, which constitute one of the largest plant-specific TF families (Tang et al., 2019). The ZIP motif mediates homodimerization and heterodimerization (Henriksson et al., 2005). In Arabidopsis, HD-ZIPs have been grouped into four major classes (I-IV) based on the exon-intron structures, similarity of nucleotide sequence, and the presence of additional sequences. (Henriksson et al., 2005; Perotti et al., 2019; Tang et al., 2019). HD-ZIP IV proteins have StAR-related lipid-transfer (START) and StAR-associated conserved (SAD) domains (Schrick et al., 2004; Figure 3B). HD-ZIP IV genes often exhibit predominant expression in a single tissue layer, typically limited to the epidermis and occasionally the subepidermal cell layer and they are closely related to trichome development (Abe et al., 2003; Gao et al., 2015). GL2 was identified as the first identified HD-ZIP IV gene and is necessary for initiation and maintenance of trichomes (Marks et al., 2009). In gl2 plants, trichome morphology is variable and expansion is aberrant (Szymanski et al., 1998). GL2 has been shown to have functional redundancy with HDG11 (Khosla et al., 2014). hdg11 mutants have an excessively branched trichome phenotype (Nakamura et al., 2006). The HD-ZIP IV subfamily also contains ARABIDOPSIS THALIANA MERISTEM LAYER1 (ATML1) and its paralogue PROTODERMAL FACTOR2 (PDF2). ATML1 and PDF2 regulate the expression of meristem layer 1 (L1)-specific genes in epidermal cells, and atml1/pdf2 double mutants have serious defects in shoot epidermal cell differentiation (Takada et al., 2013; Ogawa et al., 2015). GA is known to induce degradation of the REPRESSOR OF ga1-3 (RGA) protein and activate the MBW complex to promote GL2 expression, positively regulating trichome development (Qi et al., 2014). DELLA proteins also directly interact with ATML1 and PDF2, leading to the inhibition of L1-box gene expression (Rombolá-Caldentey et al., 2014).
In cotton, the GL2 homologs MERISTEM LAYER 1 (GbML1), GhHD1, GaHOX1, and GhHOX3 are highly expressed in trichomes (Guan et al., 2008; Zhang et al., 2010; Walford et al., 2012; Shan et al., 2014). These cotton genes modify trichome development when ectopically expressed in Arabidopsis. GbML1 controls cotton fiber development and interacts with a key regulator, GbMYB25 (Zhang et al., 2010). GbML1 over-expression in Arabidopsis increases leaf and stem trichome density (Zhang et al., 2010). GhHD1 is an L1-specific gene that regulates cotton epidermal cell differentiation (Walford et al., 2012). Knockout and over-expression experiments suggest that GhHD1 has positive roles in trichome initiation (Walford et al., 2012). Recently, GaHD1 was identified in the glabrous and fibreless cotton mutant line SMA-4; GaHD1 is a candidate gene for trichome and fiber initiation (Ding et al., 2020). GaHOX1 expression under the control of the GL2 promoter could rescue the abnormal trichome phenotype of the Arabidopsis glabrous mutant gl2-2 (Guan et al., 2008). Phylogenetic analysis shows that GhHOX1 is most closely related to AtGL2, whereas GhHD1 is most closely related to AtML1 and AtPDF2 (Figure 3A). Furthermore, over-expressing GhHOX3 significantly increases fiber length, but GhHOX3 knockdowns show decreased stem trichome density and fiber length, suggesting a vital role of GhHOX3 in fiber elongation (Shan et al., 2014). Interestingly, GhHOX3 interacts with GhHD1, enhancing transcriptional activity of GhHOX3 (Shan et al., 2014). These results indicate that HD-ZIP IV proteins play crucial roles in the molecular regulation of cotton trichome and fiber development.
Figure 3. Relationships of HD-ZIP TF members in Arabidopsis and cotton. (A) Phylogenetic tree of HD-ZIP TFs in Arabidopsis and cotton by the neighbor-end joining method using MEGA 10.0. (B) Gene structure of the HD-ZIP IV TFs in Arabidopsis and cotton. ATHB1/HAT5 (At3g01470), ATHB3/HAT7 (At5g15150), ATHB5 (At5g65310), ATHB6 (At2g22430), ATHB7 (At2g46680), ATHB12 (At3g61890), ATHB13 (At1g69780), ATHB16 (At4g40060), ATHB20 (At3g01220), ATHB21 (At2g18550), ATHB22 (At2g36610), ATHB23 (At1g26960), ATHB40 (At4g36740), ATHB51 (At5g03790), ATHB52 (At5g53980), ATHB53 (At5g66700), ATHB54 (At1g27050), ATHB2/HAT4 (At4g16780), ATHB4 (At2g44910), ATHB17 (At2g01430), ATHB18 (At1g70920), HAT1 (At4g17460), HAT2 (At5g47370), HAT3 (At3g60390), HAT9 (At2g22800), HAT14 (At5g06710), HAT22 (At4g37790), ATHB8 (At4g32880), PHAVOLUTA/ATHB9 (At1g30490), PHABULOSA/ATHB14 (At2g34710), CORONA/ATHB15 (At1g52150), REVOLUTA/IFL1 (At5g60690), AtGL2 (At1g79840), AtML1 (At4g21750), AtPDF2 (At4g04890), AtANL2 (At4g00730), AtHDG1 (At3g61150), AtHDG2 (At1g05230), AtHDG3 (At2g32370), AtHDG4 (At4g17710), AtHDG5 (At5g46880), AtHDG6 (At4g25530), AtHDG7 (At5g52170), AtHDG8 (At3g03260), AtHDG9 (At5g17320), AtHDG10 (At1g34650), AtHDG11 (At1g73360), AtHDG12 (At1g17920), GhHD1 (Gh_A06G1283), GhHOX1 (AF530913), GhHOX3 (Gh_A12G2462).
WD40-repeat proteins provide a scaffold in protein–protein interactions between R2R3-MYB and bHLH proteins. In Arabidopsis, GL1, GL3/EGL3, and TTG1 form a complex that induces trichome initiation by activating GL2 expression (Arteaga et al., 2021). Single-repeat R3 MYB proteins (single-repeat MYBs) play key roles in controlling the trichome patterning in Arabidopsis. It was suggested that single-repeat MYBs compete with GL1 in binding to GL3/EGL3, thereby preventing the formation of activator complex GL1-GL3/EGL3-TTG1. Significantly, GL1-GL3/EGL3-TTG1 is needed for the activation of GL2, which is a positive regulator of trichome development (Ishida et al., 2008). In addition, GA induces RGA protein degradation, activating the GL1-GL3/EGL3-TTG1 complex and ultimately promoting GL2 expression (Qi et al., 2014).
Protein–protein interaction analysis revealed that conserved amino acid signature ([DE]Lx2[RK]x3Lx6Lx3R) of MYB protein family is the structural basis of interaction between MYB and R/B-like BHLH proteins (Zimmermann et al., 2004). In cotton, MMLs comprise a specific family that regulates fiber development. Although GhMML4_D12 lacks the ([DE]Lx2[RK]x3Lx6Lx3R) motif, preventing interaction with bHLH proteins (Tian et al., 2020), it regulates lint fiber development by interacting with GhWDR, which is similar to the GL1-GL3/EGL3-TTG1 complex involved in Arabidopsis trichome development (Tian et al., 2020). Thus, cotton has likely evolved a specific regulatory network for fiber development.
In Arabidopsis, other TFs have also been identified that control trichome development. C2H2 zinc finger proteins, including GLABROUS INFLORESCENCE STEMS (GIS), GIS2, GIS3, ZINC FINGER PROTEIN5 (ZFP5), ZFP6, and ZFP8 are key factors that regulate trichome initiation through GA and CK signaling (Gan et al., 2006, 2007; Zhou et al., 2011, 2013). GIS3 is located upstream of GIS, GIS2, ZFP8, GL1, and GL3; GIS and GIS2 are direct targets of GIS3 (Sun et al., 2015). The TEOSINTE BRANCHED/CYCLOIDEA/PCF (TCP) class II protein TCP4 suppresses trichome branching by direct transcriptional activation of GIS (Vadde et al., 2018). A membrane-associated NAC (NAM, ATAF1/2, and CUC) TF, NTM1-LIKE8 (NTL8), is a regulator that acts upstream of TRY and TCL1 in trichome initiation (Tian et al., 2017). TTG2 encodes a WRKY protein that is a direct target of GL1 and has functional redundancy with GL2 in regulating trichome development (Johnson et al., 2002; Ishida et al., 2007).
In cotton, in addition to the core regulatory factors described above, WRKY, TCP, and NAC TFs also play important roles in regulating fiber development. GhWRKY16 plays a crucial role in fiber initiation and elongation and is phosphorylated by GhMPK3-1 to directly upregulate downstream genes involved in early fiber development (Wang et al., 2021b). The functions of several TCP genes have been characterized with respect to fiber development. GhTCP14 is a class I TCP gene that participates in fiber initiation and elongation and responds to exogenous auxin (Wang et al., 2013). Arabidopsis GhTCP14 over-expressors have enhanced trichome and root hair development. A class II TCP protein, GhTCP4, maintains the balance between cotton fiber cell elongation and cell wall synthesis by interacting with GhHOX3 (Cao et al., 2020). Over-expression of GhTCP4 accelerates biosynthesis of the SCW in fiber cells, resulting in shorter fibers and thicker walls. The NAC TF GhFSN1 is a positive regulator of fiber SCW biosynthesis, and over-expression increases wall thickness and slightly decreases fiber length (Zhang et al., 2018).
Chromatin stability and dynamics are important in regulation of gene expression (Hung et al., 2020). Changes in chromatin structure are closely associated with DNA methylation, histone modifications, and DNA binding to histones (Peterson and Laniel, 2004). DNA methylation status affects the binding ability of proteins to chromatin. Histone modifications include acetylation (ac), methylation (me), ubiquitination (ub), and phosphorylation. Modifications such as H3K9ac and H3K4 trimethylation (H3K4me3) are generally associated with active transcription, whereas H3K9me2 and H3K27me3 are involved in transcriptional inhibition (Zheng et al., 2016). The chromatin assembly factor CAF-1 is a histone chaperone that promotes chromatin formation and maintains specific chromatin states (Exner et al., 2008). CAF-1 is required for Arabidopsis trichome branching in an endoreduplication-independent pathway (Exner et al., 2008).
Histone acetylation is dynamically maintained by histone acetyltransferases and histone deacetylases (HDACs). In Arabidopsis, the histone acetyltransferase GCN5 is a member of the Spt-Ada-Gcn5 acetyltransferase (SAGA) complex (Wu et al., 2021). GCN5 regulates expression of genes involved in trichome development by acetylating histone H3 (Wu et al., 2021) and regulates histone acetylation of promoters in GL1, GL2, GL3, and CPC, which are involved in trichome initiation (Wang et al., 2019a). Moreover, GCN5 is required for trichome branching, as demonstrated by the significantly less branched phenotype of the gcn5-1 mutant (Kotak et al., 2018). Histone methylation, regulated by methyltransferases and demethylases, results in gene activation or repression by affecting the chromatin state (Hung et al., 2020). JMJ29 is a histone demethylase that contains a JmjC domain and is responsible for demethylation at H3K9me1/2 in Arabidopsis (Hung et al., 2020). JMJ29 directly demethylates H3K9 on the GL3 locus, thereby regulating GL3 expression in trichome initiation (Figure 4).
Figure 4. GL3/EGL3 and GL1 are involved in multi-dimensional regulation of Arabidopsis trichrome development. GL3/EGL3 and GL1 are important regulators of Arabidopsis trichome development. They control trichome development by different regulation modes. JMJ29 directly demethylates H3K9 on the GL3 locus, thereby regulating GL3 expression in trichome initiation (chromatin-mediated regulation). As TFs, GL3/EGL3 and GL1 regulate the expression of trichome development-related genes (transcriptional regulation). In Arabidopsis, miR828 promotes the degradation of GL1 and inhibits trichome formation (post-transcriptional regulation). In addition, ubiquitination modification controls the level of GL3. UPL3 mediates the proteasome degradation of GL3/EGL3 and represses the formation of GL1-GL3/EGL3-TTG1 complex (post-translational regulation).
In cotton, HDAC activity is essential for fiber initiation, and GhHDA5 primarily deacetylates H3K9ac marks (Kumar et al., 2018). GhHDA5 knockdowns show significantly suppressed fiber initiation and yield. GhH2A12 is a histone H2A gene that controls fiber initiation and early elongation by regulating cell cycle-related genes (Hao et al., 2014). GhH2A12 over-expression delays fiber initiation and results in shorter fibers. These results provide deeper insights into the molecular mechanisms of chromatin-mediated regulation of trichome development.
In plants, DNA methylation occurs at CG, CHG, and CHH sites through distinct pathways (Song et al., 2015). In cotton, CHH methylation is distinctly changed during ovule and fiber development, and CHG and CHH methylation contribute to homoeologous gene expression bias in ovules and fibers (Song et al., 2015). Moreover, heterochromatic DNA hypermethylation affects G. barbadense fiber differentiation through an H3K9me2-dependent pathway (Wang et al., 2016).
Gene expression regulation at the post-transcriptional level is crucial for plant growth and development. Non-coding RNA (ncRNA) regulation and mRNA modification have emerged as important components of post-transcriptional gene expression. ncRNAs include long noncoding RNAs (lncRNAs), small interfering RNAs (siRNAs), and microRNAs (miRNAs). lncRNAs are defined as ncRNAs with transcripts longer than 200 nt; some lncRNAs are known to manipulate fiber initiation (Hu et al., 2018). miRNAs are small single-stranded ncRNAs that are 20–22 nt in length and have important roles in modulating post-transcriptional gene expression in plants (Voinnet, 2009; Pattanaik et al., 2014). N6-methyladenosine (m6A) controls mRNA fate and expression and is the most abundant and widespread internal mRNA modification. m6A can be dynamically read, written, and erased (Wei et al., 2018). RNA-binding proteins with a YTH domain act as anchors for m6A readers.
Recent studies have identified miRNAs that are involved in regulating trichome development in Arabidopsis (Figure 2), such as miR156 (Yu et al., 2010), miR171 (Xue et al., 2014), miR172 (Lian et al., 2021), and miR319 (Vadde et al., 2018). For example, miR156 targets SQUAMOSA PROMOTER BINDING PROTEIN LIKE9 (SPL9), which represses trichome formation on the inflorescence, to regulate trichome development in Arabidopsis (Yu et al., 2010). Overexpressing miR156 can cause ectopic trichome on the stem and floral, while plants with improved transcripts level of SPL9 significantly decrease of trichome density. Furthermore, TCL1 and TRY, which are negative regulators of trichome development, are target genes of SPL9. LOST MERISTEMS (LOM) 1, LOM2, and LOM3 are targeted by miR171; miR171 over-expression decreases trichome density on Arabidopsis stem and floral organs (Xue et al., 2014). Interestingly, LOM1-3 are involved in modulating SPL9 activity. miR156 and miR171 form a regulatory network through direct interaction of their target proteins. miR172 family members have different expression patterns and functional specificity, and elevated levels of miR172 promote trichome formation on the abaxial leaf surfaces (Lian et al., 2021). A recent study provided insight into the coordinated regulation of trichome initiation in Paulownia tomentosa by miR319/TCP19 and GA signaling (Fan et al., 2020), showing that miR319 over-expression significantly elevated trichome density. In Arabidopsis, TCP4 is targeted by miR319 and suppresses trichome branching (Vadde et al., 2018).
Several studies have identified ncRNAs that are expressed during cotton fiber development (Guan et al., 2014b; Wang et al., 2015; Wan et al., 2016). Through genome and RNA sequencing, lncRNAs in G. barbadense have been shown to exhibit homoeologous expression bias (Wang et al., 2015). GhMML3-derived endogenous siRNA involved in regulation of fiber cell development by mediating the self-cleavage of GhMML3 transcript and subsequently result in the development of naked seeds (Wan et al., 2016). In cotton, miR828 and miR858 have been shown to control fiber development by targeting GhMYB2 homologs. Another report demonstrated that miRNA156/157 is essential for fiber elongation in G. barbadense (Liu et al., 2014).
In Arabidopsis, 11 YTH proteins have been identified that have a highly conserved C-terminal region, EVOLUTIONARILY CONSERVED C-TERMINAL REGION (ECT) 1–11 (Ok et al., 2005). ECT2 is an m6A reader that controls normal trichome morphology in Arabidopsis (Wei et al., 2018; Figure 2); its deletion leads to defects in trichome branching (Scutenaire et al., 2018). ECT2 can bind to the promoters of TTG1, DISTORTED TRICHOME 2 (DIS2), and IRREGULAR TRICHOME BRANCH 1 (ITB1), which are m6A-modified genes that regulate trichome development (Liang et al., 2020). In ect2 mutants, TTG1, ITB1, and DIS2 transcripts are degraded, which affects trichome branching (Wei et al., 2018). These results demonstrate molecular mechanisms by which m6A mediates trichome development through recruiting reader proteins.
Great progress has been made in understanding the regulatory mechanism of the MBW complex in trichome development, but it remains important to clarify the factors that regulate the transcriptional activator complex at the post-translational dimension. Post-translational modifications include (but not limited to), acetylation, glycosylation, ubiquitination, phosphorylation, glycation, SUMOylation, methylation, nitration, and oxidation. The ubiquitin/26S proteasome system (UPS) is an important regulator and mediates the proteasomal degradation of TFs (Patra et al., 2013). The UPS consists of the enzymes E1, E2, and E3; E3 is the key factor that determines substrate specificity (Vierstra, 2009). Moreover, histone H2B is ubiquitinated by E2 enzymes (UBC1, UBC2, and UBC3) and E3 ligases (HUB1 and HUB2), and H2B mono-ubiquitination (H2Bub1) triggers plant growth (Fleury et al., 2007; Feng et al., 2018).
In Arabidopsis, KAKTUS (KAK) encodes a HECT-type E3 ubiquitin ligase that negatively regulates endoreduplication cycles in trichome branching by accelerating GL3 and EGL3 degradation (El Refy et al., 2004). Genetic studies have revealed a specific role of ubiquitin protein ligase 3 (UPL3) in trichome development (Downes et al., 2003; Patra et al., 2013), and proteasomal degradation of GL3 and EGL3 is mediated by UPL3 (Figure 4). In addition, UPL3 is downregulated in gl3 mutants. As a subunit of the 26S proteasome, REGULATORY PARTICLE AAA-ATPASE 2a (RPT2a) also controls trichome branching by negatively regulating endoreduplication (Sako et al., 2010). Mutation of rpt2a suppresses the gl3 phenotype, but rpt2a/gl3 double mutants have normal trichome branching. These findings highlight the importance of post-translational regulation in trichome development.
GhHUB2 is a cotton ubiquitin ligase involved in fiber elongation and SCW deposition (Feng et al., 2018). GhHUB2 interacts with a fiber transcriptional repressor, GhKNL1, and degrades GhKNL1 through the ubiquitin-26S proteasome pathway. Furthermore, protein phosphorylation and acetylation have been reported to be involved in fiber development (Ma et al., 2014; Singh et al., 2020). These reports provide new insights for further study into the mechanisms of fiber development in cotton.
Trichome development is a highly complex process, involving the coordinated function of many genes and signaling pathways to integrate a variety of exogenous and endogenous factors. Previous studies have supported a model of trichome development as controlled by a multi-dimensional regulatory network, including chromatin-mediated, transcriptional, post-transcriptional, and post-translational regulation. Elucidation of molecular and genetic regulatory mechanisms of trichome development is ongoing, and further insight is necessary to understand trichome development from the perspective of multi-dimensional network modules and their interactions. For example, some genes have been shown to be involved in Arabidopsis trichome development at multiple regulatory levels. The TF GL3 is involved in trichome initiation and branching and is regulated by JMJ29 demethylase through demethylation of H3K9me2 (Hung et al., 2020). GL3 also forms a core complex of trichome initiation with GL1 and TTG1 to regulate the expression of GL2/TTG2 (Wang et al., 2021a); furthermore, it can be ubiquitinated by UPL3, resulting in degradation of the GL1-GL3/EGL3-TTG1 complex (Patra et al., 2013).
Comparative studies into the molecular mechanisms in Arabidopsis trichomes and cotton fibers will accelerate the understanding of trichome development in different plant species. Cotton fiber development is a longer process than that of Arabidopsis trichomes and involves a more complex regulatory network. However, the mechanism of Arabidopsis trichome development provides a molecular basis for understanding cotton fiber development; ectopic expression of genes regulating cotton fiber initiation and elongation significantly affect trichome phenotypes in Arabidopsis, reflecting functional homology between the species (Table 1). However, the regulatory networks of cotton fiber development are distinct from those of Arabidopsis trichomes. For example, the MML4-WDR complex is present in cotton in place of the Arabidopsis GL1-GL3/EGL3-TTG1 complex (Tian and Zhang, 2021). In addition to the mechanisms reviewed here, fiber development also includes metabolic regulatory pathways, such as sucrose metabolism (Ding et al., 2021). Fiber elongation phase utilizes sucrose as a direct carbon source during cellulose biosynthesis and also provides turgor pressure to accelerate fiber elongation. However, molecular mechanism of transcriptional regulation of sucrose transportation from ovules into elongating fibers remains elusive.
After the release of recent updated cotton reference genome (Ma et al., 2021), the identification of the molecular regulatory mechanisms of fiber development will be accelerated. Previous reports have shown that some homologous genes are differentially expressed in allotetraploid cotton, and homoeolog bias is responsible for ovule and fiber development (Zhao et al., 2018; Ando et al., 2021). Moreover, epigenetic modifications, such as histone modification and DNA methylation, are important factors influencing homoeology bias (Zheng et al., 2016; Kumar et al., 2018). Large number of homoeologous genes with expression bias designates that they have been profoundly sub-functionalized for cotton fiber development. These findings will promote the study of functional differences in homoeologs.
In the future, additional studies should be conducted to study trichome optimization. The rapid development and effective application of CRISPR gene editing techniques in plants will allow for easier generation of gene knockins and knockouts (Miki et al., 2018; Lu et al., 2020) compared to older techniques such as cross-breeding to produce target phenotypes. In higher plants, knockdown of negative regulators by gene editing is expected to confer positive, beneficial phenotypes. For example, glume trichomes affect rice quality. Conversely, for positive regulatory factors, economic benefits could be greatly improved by knocking in genes, such as those associated with increased cotton fiber yield.
RZ and CL conceived the concept of the review. YaW, QZ, ZM, YWe, MA, and YuW compiled the literature. YaW and CL designed the figures. YaW, RZ, and CL wrote the paper. All authors contributed to the article and approved the submitted version.
We regret that many original articles on plant epidermal hairs development and cotton fiber development. CL and RZ were supported by the National Natural Science Foundation of China (32072115 and 31771850), the National Special Program for GMO Development of China (No. 2016ZX08009003-003-004 and 2016ZX08005-004), and the Agricultural Science and Technology Innovation Program of Chinese Academy of Agricultural Sciences.
The authors declare that the research was conducted in the absence of any commercial or financial relationships that could be construed as a potential conflict of interest.
All claims expressed in this article are solely those of the authors and do not necessarily represent those of their affiliated organizations, or those of the publisher, the editors and the reviewers. Any product that may be evaluated in this article, or claim that may be made by its manufacturer, is not guaranteed or endorsed by the publisher.
Abe, M., Katsumata, H., Komeda, Y., and Takahashi, T. (2003). Regulation of shoot epidermal cell differentiation by a pair of homeodomain proteins in Arabidopsis. Development 130, 635–643. doi: 10.1242/dev.00292
Ando, A., Kirkbride, R. C., Jones, D. C., Grimwood, J., and Chen, Z. J. (2021). LCM and RNA-seq analyses revealed roles of cell cycle and translational regulation and homoeolog expression bias in cotton fiber cell initiation. BMC Genomics 22:309. doi: 10.1186/s12864-021-07579-1
Arteaga, N., Savic, M., Méndez-Vigo, B., Fuster-Pons, A., Torres-Pérez, R., Oliveros, J. C., et al. (2021). MYB transcription factors drive evolutionary innovations in Arabidopsis fruit trichome patterning. Plant Cell 33, 548–565. doi: 10.1093/plcell/koaa041
Balkunde, R., Pesch, M., and Hülskamp, M. (2010). Trichome patterning in Arabidopsis thaliana: From genetic to molecular models. Curr. Top. Dev. Biol. 91, 299–321. doi: 10.1016/S0070-2153(10)91010-7
Bouyer, D., Geier, F., Kragler, F., Schnittger, A., Pesch, M., Wester, K., et al. (2008). Two-dimensional patterning by a trapping/depletion mechanism: The role of TTG1 and GL3 in Arabidopsis Trichome formation. PLoS Biol. 6:e141. doi: 10.1371/journal.pbio.0060141
Brockington, S. F., Alvarez-Fernandez, R., Landis, J. B., Alcorn, K., Walker, R. H., Thomas, M. M., et al. (2012). Evolutionary analysis of the MIXTA gene family highlights potential targets for the study of cellular differentiation. Mol. Biol. Evol. 30, 526–540. doi: 10.1093/molbev/mss260
Cao, J. F., Zhao, B., Huang, C. C., Chen, Z. W., Zhao, T., Liu, H. R., et al. (2020). The miR319-targeted GhTCP4 promotes the transition from cell elongation to wall thickening in cotton fiber. Mol. Plant 13, 1063–1077. doi: 10.1016/j.molp.2020.05.006
Ding, M., Cao, Y., He, S., Sun, J., Dai, H., Zhang, H., et al. (2020). GaHD1, a candidate gene for the Gossypium arboreum SMA-4 mutant, promotes trichome and fiber initiation by cellular H2O2 and Ca2+ signals. Plant Mol. Biol. 103, 409–423. doi: 10.1007/s11103-020-01000-3
Ding, X., Li, X., Wang, L., Zeng, J., Huang, L., Xiong, L., et al. (2021). Sucrose enhanced reactive oxygen species generation promotes cotton fiber initiation and secondary cell wall deposition. Plant Biotechnol. J. 19, 1092–1094. doi: 10.1111/pbi.13594
Doroshkov, A. V., Konstantinov, D. K., Afonnikov, D. A., and Gunbin, K. V. (2019). The evolution of gene regulatory networks controlling Arabidopsis thaliana L. trichome development. BMC Plant Biol. 19:53. doi: 10.1186/s12870-019-1640-2
Downes, B. P., Stupar, R. M., Gingerich, D. J., and Vierstra, R. D. (2003). The HECT ubiquitin-protein ligase (UPL) family in Arabidopsis: UPL3 has a specific role in trichome development. Plant J. 35, 729–742. doi: 10.1046/j.1365-313X.2003.01844.x
El Refy, A., Perazza, D., Zekraoui, L., Valay, J. G., Bechtold, N., Brown, S., et al. (2004). The Arabidopsis KAKTUS gene encodes a HECT protein and controls the number of endoreduplication cycles. Mol. Gen. Genomics. 270, 403–414. doi: 10.1007/s00438-003-0932-1
Exner, V., Gruissem, W., and Hennig, L. (2008). Control of trichome branching by chromatin assembly factor-1. BMC Plant Biol. 8:54. doi: 10.1186/1471-2229-8-54
Fan, D., Ran, L., Hu, J., Ye, X., Xu, D., Li, J., et al. (2020). miR319a/TCP module and DELLA protein regulate trichome initiation synergistically and improve insect defenses in Populus tomentosa. New Phytol. 227, 867–883. doi: 10.1111/nph.16585
Feng, H., Li, X., Chen, H., Deng, J., Zhang, C., Liu, J., et al. (2018). GhHUB2, a ubiquitin ligase, is involved in cotton fiber development via the ubiquitin-26S proteasome pathway. J. Exp. Bot. 69, 5059–5075. doi: 10.1093/jxb/ery269
Fleury, D., Himanen, K., Cnops, G., Nelissen, H., Boccardi, T. M., Maere, S., et al. (2007). The Arabidopsis thaliana homolog of yeast BRE1 has a function in cell cycle regulation during early leaf and root growth. Plant Cell 19, 417–432. doi: 10.1105/tpc.106.041319
Gan, Y., Kumimoto, R., Liu, C., Ratcliffe, O., Yu, H., and Broun, P. (2006). GLABROUS INFLORESCENCE STEMS modulates the regulation by gibberellins of epidermal differentiation and shoot maturation in Arabidopsis. Plant Cell 18, 1383–1395. doi: 10.1105/tpc.106.041533
Gan, Y., Liu, C., Yu, H., and Broun, P. (2007). Integration of cytokinin and gibberellin signalling by Arabidopsis transcription factors GIS, ZFP8 and GIS2 in the regulation of epidermal cell fate. Development 134, 2073–2081. doi: 10.1242/dev.005017
Gan, L., Xia, K., Chen, J. G., and Wang, S. (2011). Functional characterization of TRICHOMELESS2, a new single-repeat R3 MYB transcription factor in the regulation of trichome patterning in Arabidopsis. BMC Plant Biol. 11:176. doi: 10.1186/1471-2229-11-176
Gao, Y., Gao, S., Xiong, C., Yu, G., Chang, J., Ye, Z., et al. (2015). Comprehensive analysis and expression profile of the homeodomain leucine zipper IV transcription factor family in tomato. Plant Physiol. Biochem. 96, 141–153. doi: 10.1016/j.plaphy.2015.07.025
Grebe, M. (2012). The patterning of epidermal hairs in Arabidopsis-updated. Curr. Opin. Plant Biol. 15, 31–37. doi: 10.1016/j.pbi.2011.10.010
Guan, X. Y., Li, Q. J., Shan, C. M., Wang, S., Mao, Y. B., Wang, L. J., et al. (2008). The HD-zip IV gene GaHOX1 from cotton is a functional homologue of the Arabidopsis GLABRA2. Physiol. Plant. 134, 174–182. doi: 10.1111/j.1399-3054.2008.01115.x
Guan, X., Pang, M., Nah, G., Shi, X., Ye, W., Stelly, D. M., et al. (2014a). miR828 and miR858 regulate homoeologous MYB2 gene functions in Arabidopsis trichome and cotton fiber development. Nat. Commun. 5:3050. doi: 10.1038/ncomms4050
Guan, X., Song, Q., and Chen, Z. J. (2014b). Polyploidy and small RNA regulation of cotton fiber development. Trends Plant Sci. 19, 516–528. doi: 10.1016/j.tplants.2014.04.007
Han, L. B., Li, Y. B., Wang, H. Y., Wu, X. M., Li, C. L., Luo, M., et al. (2013). The dual functions of WLIM1a in cell elongation and secondary wall formation in developing cotton fibers. Plant Cell 25, 4421–4438. doi: 10.1105/tpc.113.116970
Hao, J., Chen, S., Tu, L., Hu, H., and Zhang, X. (2014). GhH2A12, a replication-dependent histone H2A gene from Gossypium hirsutum, is negatively involved in the development of cotton fiber cells. Plant Cell Rep. 33, 1711–1721. doi: 10.1007/s00299-014-1649-9
Henriksson, E., Olsson, A. S. B., Johannesson, H., Johansson, H., Hanson, J., Engström, P., et al. (2005). Homeodomain leucine zipper class I genes in Arabidopsis. Expression patterns and phylogenetic relationships. Plant Physiol. 139, 509–518. doi: 10.1104/pp.105.063461
Hu, H., He, X., Tu, L., Zhu, L., Zhu, S., Ge, Z., et al. (2016). GhJAZ2 negatively regulates cotton fiber initiation by interacting with the R2R3-MYB transcription factor GhMYB25-like. Plant J. 88, 921–935. doi: 10.1111/tpj.13273
Hu, H., Wang, M., Ding, Y., Zhu, S., Zhao, G., Tu, L., et al. (2018). Transcriptomic repertoires depict the initiation of lint and fuzz fibers in cotton (Gossypium hirsutum L.). Plant Biotechnol. J. 16, 1002–1012. doi: 10.1111/pbi.12844
Huang, J., Chen, F., Guo, Y., Gan, X., Yang, M., Zeng, W., et al. (2021). GhMYB7 promotes secondary wall cellulose deposition in cotton fibers by regulating GhCesA gene expression through three distinct cis-elements. New Phytol. 232, 1718–1737. doi: 10.1111/nph.17612
Hülskamp, M., Misŕa, S., and Jürgens, G. (1994). Genetic dissection of trichome cell development in Arabidopsis. Cell 76, 555–566. doi: 10.1016/0092-8674(94)90118-x
Humphries, J. A., Walker, A. R., Timmis, J. N., and Orford, S. J. (2005). Two WD-repeat genes from cotton are functional homologues of the Arabidopsis thaliana TRANSPARENT TESTA GLABRA1 (TTG1) gene. Plant Mol. Biol. 57, 67–81. doi: 10.1007/s11103-004-6768-1
Hung, F. Y., Chen, J. H., Feng, Y. R., Lai, Y. C., Yang, S., and Wu, K. (2020). Arabidopsis JMJ29 is involved in trichome development by regulating the core trichome initiation gene GLABRA3. Plant J. 103, 1735–1743. doi: 10.1111/tpj.14858
Ishida, T., Hattori, S., Sano, R., Inoue, K., Shirano, Y., Hayashi, H., et al. (2007). Arabidopsis TRANSPARENT TESTA GLABRA2 is directly regulated by R2R3 MYB transcription factors and is involved in regulation of GLABRA2 transcription in epidermal differentiation. Plant Cell 19, 2531–2543. doi: 10.1105/tpc.107.052274
Ishida, T., Kurata, T., Okada, K., and Wada, T. (2008). A genetic regulatory network in the development of trichomes and root hairs. Annu. Rev. Plant Biol. 59, 365–386. doi: 10.1146/annurev.arplant.59.032607.092949
Jakoby, M. J., Falkenhan, D., Mader, M. T., Brininstool, G., Wischnitzki, E., Platz, N., et al. (2008). Transcriptional profiling of mature arabidopsis trichomes reveals that NOECK encodes the MIXTA-like transcriptional regulator MYB106. Plant Physiol. 148, 1583–1602. doi: 10.1104/pp.108.126979
Johnson, C. S., Kolevski, B., and Smyth, D. R. (2002). TRANSPARENT TESTA GLABRA2, a trichome and seed coat development gene of Arabidopsis, encodes a WRKY transcription factor. Plant Cell 14, 1359–1375. doi: 10.1105/tpc.001404
Kang, Y. H., Kirik, V., Hulskamp, M., Nam, K. H., Hagely, K., Lee, M. M., et al. (2009). The MYB23 gene provides a positive feedback loop for cell fate specification in the Arabidopsis root epidermis. Plant Cell 21, 1080–1094. doi: 10.1105/tpc.108.063180
Khosla, A., Paper, J. M., Boehler, A. P., Bradley, A. M., Neumann, T. R., and Schrick, K. (2014). HD-zip proteins GL2 and HDG11 have redundant functions in Arabidopsis trichomes, and GL2 activates a positive feedback loop via MYB23. Plant Cell 26, 2184–2200. doi: 10.1105/tpc.113.120360
Kirik, V., Lee, M. M., Wester, K., Herrmann, U., Zheng, Z., Oppenheimer, D., et al. (2005). Functional diversification of MYB23 and GL1 genes in trichome morphogenesis and initiation. Development 132, 1477–1485. doi: 10.1242/dev.01708
Kirik, V., Simon, M., Huelskamp, M., and Schiefelbein, J. (2004). The ENHANCER OF TRY AND CPC1 gene acts redundantly with TRIPTYCHON and CAPRICE in trichome and root hair cell patterning in Arabidopsis. Dev. Biol. 268, 506–513. doi: 10.1016/j.ydbio.2003.12.037
Kotak, J., Saisana, M., Gegas, V., Pechlivani, N., Kaldis, A., Papoutsoglou, P., et al. (2018). The histone acetyltransferase GCN5 and the transcriptional coactivator ADA2b affect leaf development and trichome morphogenesis in Arabidopsis. Planta 248, 613–628. doi: 10.1007/s00425-018-2923-9
Kumar, V., Singh, B., Singh, S. K., Rai, K. M., Singh, S. P., Sable, A., et al. (2018). Role of GhHDA5 in H3K9 deacetylation and fiber initiation in Gossypium hirsutum. Plant J. 95, 1069–1083. doi: 10.1111/tpj.14011
Li, S. F., Milliken, O. N., Pham, H., Seyit, R., Napoli, R., Preston, J., et al. (2009). The Arabidopsis MYB5 transcription factor regulates mucilage synthesis, seed coat development, and trichome morphogenesis. Plant Cell 21, 72–89. doi: 10.1105/tpc.108.063503
Lian, H., Wang, L., Ma, N., Zhou, C. M., Han, L., Zhang, T. Q., et al. (2021). Redundant and specific roles of individual MIR172 genes in plant development. PLoS Biol. 19:e3001044. doi: 10.1371/journal.pbio.3001044
Liang, G., He, H., Li, Y., Ai, Q., and Yu, D. (2014). MYB82 functions in regulation of trichome development in Arabidopsis. J. Exp. Bot. 65, 3215–3223. doi: 10.1093/jxb/eru179
Liang, Z., Riaz, A., Chachar, S., Ding, Y., Du, H., and Gu, X. (2020). Epigenetic modifications of mRNA and DNA in plants. Mol. Plant 13, 14–30. doi: 10.1016/j.molp.2019.12.007
Liu, Z. H., Chen, Y., Wang, N. N., Chen, Y. H., Wei, N., Lu, R., et al. (2020). A basic helix–loop–helix protein (GhFP1) promotes fiber elongation of cotton (Gossypium hirsutum) by modulating brassinosteroid biosynthesis and signalling. New Phytol. 225, 2439–2452. doi: 10.1111/nph.16301
Liu, N., Tu, L., Tang, W., Gao, W., Lindsey, K., and Zhang, X. (2014). Small RNA and degradome profiling reveals a role for miRNAs and their targets in the developing fibers of Gossypium barbadense. Plant J. 80, 331–344. doi: 10.1111/tpj.12636
Lu, Y., Tian, Y., Shen, R., Yao, Q., Wang, M., Chen, M., et al. (2020). Targeted, efficient sequence insertion and replacement in rice. Nat. Biotechnol. 38, 1402–1407. doi: 10.1038/s41587-020-0581-5
Ma, Q., Wu, M., Pei, W., Li, H., Li, X., Zhang, J., et al. (2014). Quantitative phosphoproteomic profiling of fiber differentiation and initiation in a fiberless mutant of cotton. BMC Genomics 15:466. doi: 10.1186/1471-2164-15-466
Ma, Z., Zhang, Y., Wu, L., Zhang, G., Sun, Z., Li, Z., et al. (2021). High-quality genome assembly and resequencing of modern cotton cultivars provide resources for crop improvement. Nat. Genet. 53, 1385–1391. doi: 10.1038/s41588-021-00910-2
Machado, A., Wu, Y., Yang, Y., Llewellyn, D. J., and Dennis, E. S. (2009). The MYB transcription factor GhMYB25 regulates early fiber and trichome development. Plant J. 59, 52–62. doi: 10.1111/j.1365-313X.2009.03847.x
Maes, L., Inzé, D., and Goossens, A. (2008). Functional specialization of the TRANSPARENT TESTA GLABRA1 network allows differential hormonal control of Laminal and marginal Trichome initiation in Arabidopsis rosette leaves. Plant Physiol. 148, 1453–1464. doi: 10.1104/pp.108.125385
Marks, M. D., Wenger, J. P., Gilding, E., Jilk, R., and Dixon, R. A. (2009). Transcriptome analysis of Arabidopsis wild-type and gl3-sst Sim trichomes identifies four additional genes required for trichome development. Mol. Plant 2, 803–822. doi: 10.1093/mp/ssp037
Matías-Hernández, L., Aguilar-Jaramillo, A. E., Cigliano, R. A., Sanseverino, W., and Pelaz, S. (2015). Flowering and trichome development share hormonal and transcription factor regulation. J. Exp. Bot. 67, 1209–1219. doi: 10.1093/jxb/erv534
Mengiste, T., Chen, X., Salmeron, J., and Dietrich, R. (2003). The BOTRYTIS SUSCEPTIBLE1 gene encodes an R2R3MYB transcription factor protein that is required for biotic and abiotic stress responses in Arabidopsis. Plant Cell 15, 2551–2565. doi: 10.1105/tpc.014167
Miki, D., Zhang, W., Zeng, W., Feng, Z., and Zhu, J. K. (2018). CRISPR/Cas9-mediated gene targeting in Arabidopsis using sequential transformation. Nat. Commun. 9:1967. doi: 10.1038/s41467-018-04416-0
Millar, A. A., and Gubler, F. (2005). The Arabidopsis GAMYB-like genes, MYB33 and MYB65, are microRNA-regulated genes that redundantly facilitate anther development. Plant Cell 17, 705–721. doi: 10.1105/tpc.104.027920
Morohashi, K., Zhao, M., Yang, M., Read, B., Lloyd, A., Lamb, R., et al. (2007). Participation of the Arabidopsis bHLH factor GL3 in trichome initiation regulatory events. Plant Physiol. 145, 736–746. doi: 10.1104/pp.107.104521
Nakamura, M., Katsumata, H., Abe, M., Yabe, N., Komeda, Y., Yamamoto, K. T., et al. (2006). Characterization of the class IV homeodomain-leucine zipper gene family in Arabidopsis. Plant Physiol. 141, 1363–1375. doi: 10.1104/pp.106.077388
Ogawa, E., Yamada, Y., Sezaki, N., Kosaka, S., Kondo, H., Kamata, N., et al. (2015). ATML1 and PDF2 play a redundant and essential role in Arabidopsis embryo development. Plant Cell Physiol. 56, 1183–1192. doi: 10.1093/pcp/pcv045
Ok, S. H., Jeong, H. J., Bae, J. M., Shin, J. S., Luan, S., and Kim, K. N. (2005). Novel CIPK1-associated proteins in Arabidopsis contain an evolutionarily conserved c-terminal region that mediates nuclear localization. Plant Physiol. 139, 138–150. doi: 10.1104/pp.105.065649
Oppenheimer, D. G., Herman, P. L., Sivakumaran, S., Esch, J., and Marks, M. D. (1991). A myb gene required for leaf trichome differentiation in Arabidopsis is expressed in stipules. Cell 67, 483–493. doi: 10.1016/0092-8674(91)90523-2
Patra, B., Pattanaik, S., and Yuan, L. (2013). Ubiquitin protein ligase 3 mediates the proteasomal degradation of GLABROUS 3 and ENHANCER OF GLABROUS 3, regulators of trichome development and flavonoid biosynthesis in Arabidopsis. Plant J. 74, 435–447. doi: 10.1111/tpj.12132
Pattanaik, S., Patra, B., Singh, S. K., and Yuan, L. (2014). An overview of the gene regulatory network controlling trichome development in the model plant, Arabidopsis. Front. Plant Sci. 5:259. doi: 10.3389/fpls.2014.00259
Payne, C. T., Zhang, F., and Lloyd, A. M. (2000). GL3 encodes a bHLH protein that regulates trichome development in Arabidopsis through interaction with GL1 and TTG1. Genetics 156, 1349–1362. doi: 10.1093/genetics/156.3.1349
Perotti, M. F., Ribone, P. A., Cabello, J. V., Ariel, F. D., and Chan, R. L. (2019). AtHB23 participates in the gene regulatory network controlling root branching, and reveals differences between secondary and tertiary roots. Plant J. 100, 1224–1236. doi: 10.1111/tpj.14511
Peterson, C. L., and Laniel, M. A. (2004). Histones and histone modifications. Curr. Biol. 14, R546–R551. doi: 10.1016/j.cub.2004.07.007
Pu, L., Li, Q., Fan, X., Yang, W., and Xue, Y. (2008). The R2R3 MYB transcription factor GhMYB109 is required for cotton fiber development. Genetics 180, 811–820. doi: 10.1534/genetics.108.093070
Qi, T., Huang, H., Wu, D., Yan, J., Qi, Y., Song, S., et al. (2014). Arabidopsis DELLA and JAZ proteins bind the WD-repeat/bHLH/MYB complex to modulate gibberellin and jasmonate signaling synergy. Plant Cell 26, 1118–1133. doi: 10.1105/tpc.113.121731
Qi, T., Song, S., Ren, Q., Wu, D., Huang, H., Chen, Y., et al. (2011). The jasmonate-ZIM-domain proteins interact with the WD-repeat/bHLH/MYB complexes to regulate jasmonate-mediated anthocyanin accumulation and trichome initiation in Arabidopsis thaliana. Plant Cell 23, 1795–1814. doi: 10.1105/tpc.111.083261
Rerie, W. G., Feldmann, K. A., and Marks, M. D. (1994). The GLABRA2 gene encodes a homeo domain protein required for normal trichome development in Arabidopsis. Genes Dev. 8, 1388–1399. doi: 10.1101/gad.8.12.1388
Rombolá-Caldentey, B., Rueda-Romero, P., Iglesias-Fernández, R., Carbonero, P., and Oñate-Sánchez, L. (2014). Arabidopsis DELLA and two HD-ZIP transcription factors regulate GA signaling in the epidermis through the L1 box cis-element. Plant Cell 26, 2905–2919. doi: 10.1105/tpc.114.127647
Ruan, Y. L. (2005). Recent advances in understanding cotton fiber and seed development. Seed Sci. Res. 15, 269–280. doi: 10.1079/SSR2005217
Sako, K., Maki, Y., Imai, K. K., Aoyama, T., Goto, D. B., and Yamaguchi, J. (2010). Control of endoreduplication of trichome by RPT2a, a subunit of the 19S proteasome in Arabidopsis. J. Plant Res. 123, 701–706. doi: 10.1007/s10265-010-0321-x
Schellmann, S., Schnittger, A., Kirik, V., Wada, T., Okada, K., Beermann, A., et al. (2002). TRIPTYCHON and CAPRICE mediate lateral inhibition during trichome and root hair patterning in Arabidopsis. EMBO J. 21, 5036–5046. doi: 10.1093/emboj/cdf524
Schiefelbein, J. (2003). Cell-fate specification in the epidermis: a common patterning mechanism in the root and shoot. Curr. Opin. Plant Biol. 6, 74–78. doi: 10.1016/s136952660200002x
Schilling, S., Kennedy, A., Pan, S., Jermiin, L. S., and Melzer, R. (2019). Genome-wide analysis of MIKC-type MADS-box genes in wheat: pervasive duplications, functional conservation and putative neofunctionalization. New Phytol. 225, 511–529. doi: 10.1111/nph.16122
Schrick, K., Nguyen, D., Karlowski, W. M., and Mayer, K. F. X. (2004). START lipid/sterol-binding domains are amplified in plants and are predominantly associated with homeodomain transcription factors. Genome Biol. 5:R41. doi: 10.1186/gb-2004-5-6-r41
Scutenaire, J., Deragon, J. M., Jean, V., Benhamed, M., Raynaud, C., Favory, J. J., et al. (2018). The YTH domain protein ECT2 is an m6a reader required for normal trichome branching in Arabidopsis. Plant Cell 30, 986–1005. doi: 10.1105/tpc.17.00854
Serna, L., and Martin, C. (2006). Trichomes: different regulatory networks lead to convergent structures. Trends Plant Sci. 11, 274–280. doi: 10.1016/j.tplants.2006.04.008
Shan, C. M., Shangguan, X. X., Zhao, B., Zhang, X. F., Chao, L. M., Yang, C. Q., et al. (2014). Control of cotton fiber elongation by a homeodomain transcription factor GhHOX3. Nat. Commun. 5:5519. doi: 10.1038/ncomms6519
Shangguan, X. X., Yang, C. Q., Zhang, X. F., and Wang, L. J. (2016). Functional characterization of a basic helix-loop-helix (bHLH) transcription factor GhDEL65 from cotton (Gossypium hirsutum). Physiol. Plant. 158, 200–212. doi: 10.1111/ppl.12450
Singh, P. K., Gao, W., Liao, P., Li, Y., Xu, F. C., Ma, X. N., et al. (2020). Comparative acetylome analysis of wild-type and fuzzless-lintless mutant ovules of upland cotton (Gossypium hirsutum cv. Xu142) unveils differential protein acetylation may regulate fiber development. Plant Physiol. Biochem. 150, 56–70. doi: 10.1016/j.plaphy.2020.02.031
Song, Q., Guan, X., and Chen, Z. J. (2015). Dynamic roles for small RNAs and DNA methylation during ovule and fiber development in allotetraploid cotton. PLoS Genet. 11:e1005724. doi: 10.1371/journal.pgen.1005724
Sun, W., Gao, Z., Wang, J., Huang, Y., Chen, Y., Li, J., et al. (2019). Cotton fiber elongation requires the transcription factor GhMYB212 to regulate sucrose transportation into expanding fibers. New Phytol. 222, 864–881. doi: 10.1111/nph.15620
Sun, Y., Veerabomma, S., Abdel-Mageed, H. A., Fokar, M., Asami, T., Yoshida, S., et al. (2005). Brassinosteroid regulates fiber development on cultured cotton ovules. Plant Cell Physiol. 46, 1384–1391. doi: 10.1093/pcp/pci150
Sun, L., Zhang, A., Zhou, Z., Zhao, Y., Yan, A., Bao, S., et al. (2015). GLABROUS INFLORESCENCE STEMS3 (GIS3) regulates trichome initiation and development in Arabidopsis. New Phytol. 206, 220–230. doi: 10.1111/nph.13218
Szymanski, D. B., Jilk, R. A., Pollock, S. M., and Marks, M. D. (1998). Control of GL2 expression in Arabidopsis leaves and trichomes. Development 125, 1161–1171. doi: 10.1242/dev.125.7.1161
Szymanski, D. B., Lloyd, A. M., and Marks, M. D. (2000). Progress in the molecular genetic analysis of trichome initiation and morphogenesis in Arabidopsis. Trends Plant Sci. 5, 214–219. doi: 10.1016/s1360-1385(00)01597-1
Takada, S., Takada, N., and Yoshida, A. (2013). ATML1 promotes epidermal cell differentiation in Arabidopsis shoots. Development 140, 1919–1923. doi: 10.1242/dev.094417
Tang, Y., Bao, X., Wang, S., Liu, Y., Tan, J., Yang, M., et al. (2019). A physic nut stress-responsive HD-zip transcription factor, JcHDZ07, confers enhanced sensitivity to salinity stress in transgenic Arabidopsis. Front. Plant Sci. 10:942. doi: 10.3389/fpls.2019.00942
Tian, Y., Du, J., Wu, H., Guan, X., Chen, W., Hu, Y., et al. (2020). The transcription factor MML4_D12 regulates fiber development through interplay with the WD40-repeat protein WDR in cotton. J. Exp. Bot. 71, 3499–3511. doi: 10.1093/jxb/eraa104
Tian, H., Wang, X., Guo, H., Cheng, Y., Hou, C., Chen, J. G., et al. (2017). NTL8 regulates trichome formation in Arabidopsis by directly activating R3 MYB genes TRY and TCL1. Plant Physiol. 174, 2363–2375. doi: 10.1104/pp.17.00510
Tian, Y., and Zhang, T. (2021). MIXTAs and phytohormones orchestrate cotton fiber development. Curr. Opin. Plant Biol. 59:101975. doi: 10.1016/j.pbi.2020.10.007
Vadde, B. V. L., Challa, K. R., and Nath, U. (2018). The TCP4 transcription factor regulates trichome cell differentiation by directly activating GLABROUS INFLORESCENCE STEMS in Arabidopsis thaliana. Plant J. 93, 259–269. doi: 10.1111/tpj.13772
Vierstra, R. D. (2009). The ubiquitin-26S proteasome system at the nexus of plant biology. Nat. Rev. Mol. Cell Biol. 10, 385–397. doi: 10.1038/nrm2688
Voinnet, O. (2009). Origin, biogenesis, and activity of plant MicroRNAs. Cell 136, 669–687. doi: 10.1016/j.cell.2009.01.046
Walford, S. A., Wu, Y., Llewellyn, D. J., and Dennis, E. S. (2011). GhMYB25-like: a key factor in early cotton fiber development. Plant J. 65, 785–797. doi: 10.1111/j.1365-313X.2010.04464.x
Walford, S. A., Wu, Y., Llewellyn, D. J., and Dennis, E. S. (2012). Epidermal cell differentiation in cotton mediated by the homeodomain leucine zipper gene, GhHD-1. Plant J. 71, 464–478. doi: 10.1111/j.1365-313X.2012.05003.x
Walker, A. R., Davison, P. A., Bolognesi-Winfield, A. C., James, C. M., Srinivasan, N., Blundell, T. L., et al. (1999). The TRANSPARENT TESTA GLABRA1 locus, which regulates trichome differentiation and anthocyanin biosynthesis in Arabidopsis, encodes a WD40 repeat protein. Plant Cell 11, 1337–1349. doi: 10.1105/tpc.11.7.1337
Wan, Q., Guan, X., Yang, N., Wu, H., Pan, M., Liu, B., et al. (2016). Small interfering RNAs from bidirectional transcripts of GhMML3_A12 regulate cotton fiber development. New Phytol. 210, 1298–1310. doi: 10.1111/nph.13860
Wang, S., and Chen, J. G. (2014). Regulation of cell fate determination by single-repeat R3 MYB transcription factors in Arabidopsis. Front. Plant Sci. 5:133. doi: 10.3389/fpls.2014.00133
Wang, T., Jia, Q., Wang, W., Hussain, S., Ahmed, S., Zhou, D. X., et al. (2019a). GCN5 modulates trichome initiation in Arabidopsis by manipulating histone acetylation of core trichome initiation regulator genes. Plant Cell Rep. 38, 755–765. doi: 10.1007/s00299-019-02404-2
Wang, L., Kartika, D., and Ruan, Y. L. (2021a). Looking into ‘hair tonics’ for cotton fiber initiation. New Phytol. 229, 1844–1851. doi: 10.1111/nph.16898
Wang, S., Kwak, S. H., Zeng, Q., Ellis, B. E., Chen, X. Y., Schiefelbein, J., et al. (2007). TRICHOMELESS1 regulates trichome patterning by suppressing GLABRA1 in Arabidopsis. Development 134, 3873–3882. doi: 10.1242/dev.009597
Wang, N. N., Li, Y., Chen, Y. H., Lu, R., Zhou, L., Wang, Y., et al. (2021b). Phosphorylation of WRKY16 by MPK3-1 is essential for its transcriptional activity during fiber initiation and elongation in cotton (Gossypium hirsutum). Plant Cell 33, 2736–2752. doi: 10.1093/plcell/koab153
Wang, N., Ma, Q., Wu, M., Pei, W., Song, J., Jia, B., et al. (2021c). Genetic variation in MYB5_A12 is associated with fiber initiation and elongation in tetraploid cotton. Plant Biotechnol. J. 19, 1892–1894. doi: 10.1111/pbi.13662
Wang, M., Wang, P., Tu, L., Zhu, S., Zhang, L., Li, Z., et al. (2016). Multi-omics maps of cotton fiber reveal epigenetic basis for staged single-cell differentiation. Nucleic Acids Res. 44, 4067–4079. doi: 10.1093/nar/gkw238
Wang, S., Wang, J. W., Yu, N., Li, C. H., Luo, B., Gou, J. Y., et al. (2004). Control of plant trichome development by a cotton fiber MYB gene. Plant Cell 16, 2323–2334. doi: 10.1105/tpc.104.024844
Wang, Z., Yang, Z., and Li, F. (2019b). Updates on molecular mechanisms in the development of branched trichome in Arabidopsis and nonbranched in cotton. Plant Biotechnol. J. 17, 1706–1722. doi: 10.1111/pbi.13167
Wang, M., Yuan, D., Tu, L., Gao, W., He, Y., Hu, H., et al. (2015). Long noncoding RNAs and their proposed functions in fiber development of cotton (Gossypium spp.). New Phytol. 207, 1181–1197. doi: 10.1111/nph.13429
Wang, M. Y., Zhao, P. M., Cheng, H. Q., Han, L. B., Wu, X. M., Gao, P., et al. (2013). The cotton transcription factor tcp14 functions in auxin-mediated epidermal cell differentiation and elongation. Plant Physiol. 162, 1669–1680. doi: 10.1104/pp.113.215673
Wei, L. H., Song, P., Wang, Y., Lu, Z., Tang, Q., Yu, Q., et al. (2018). The m6A reader ECT2 controls trichome morphology by affecting mRNA stability in Arabidopsis. Plant Cell 30, 968–985. doi: 10.1105/tpc.17.00934
Wester, K., Digiuni, S., Geier, F., Timmer, J., Fleck, C., and Hülskamp, M. (2009). Functional diversity of R3 single-repeat genes in trichome development. Development 136, 1487–1496. doi: 10.1242/dev.021733
Wu, C. J., Liu, Z. Z., Wei, L., Zhou, J. X., Cai, X. W., Su, Y. N., et al. (2021). Three functionally redundant plant-specific paralogs are core subunits of the SAGA histone acetyltransferase complex in Arabidopsis. Mol. Plant 14, 1071–1087. doi: 10.1016/j.molp.2021.03.014
Wu, H., Tian, Y., Wan, Q., Fang, L., Guan, X., Chen, J., et al. (2018). Genetics and evolution of MIXTA genes regulating cotton lint fiber development. New Phytol. 217, 883–895. doi: 10.1111/nph.14844
Xue, X. Y., Zhao, B., Chao, L. M., Chen, D. Y., Cui, W. R., Mao, Y. B., et al. (2014). Interaction between two timing MicroRNAs controls trichome distribution in Arabidopsis. PLoS Genet. 10:e1004266. doi: 10.1371/journal.pgen.1004266
Yan, T., Chen, M., Shen, Q., Li, L., Fu, X., Pan, Q., et al. (2017). HOMEODOMAIN PROTEIN1 is required for jasmonate-mediated glandular trichome initiation inArtemisia annua. New Phytol. 213, 1145–1155. doi: 10.1111/nph.14205
Yang, C., and Ye, Z. (2013). Trichomes as models for studying plant cell differentiation. Cell. Mol. Life Sci. 70, 1937–1948. doi: 10.1007/s00018-012-1147-6
Yu, N., Cai, W. J., Wang, S., Shan, C. M., Wang, L. J., and Chen, X. Y. (2010). Temporal control of trichome distribution by MicroRNA156-targeted SPL genes in Arabidopsis thaliana. Plant Cell 22, 2322–2335. doi: 10.1105/tpc.109.072579
Yu, D., Li, X., Li, Y., Ali, F., Li, F., and Wang, Z. (2022). Dynamic roles and intricate mechanisms of ethylene in epidermal hair development in Arabidopsis and cotton. New Phytol. 234, 375–391. doi: 10.1111/nph.17901
Zhang, F., Gonzalez, A., Zhao, M., Payne, C. T., and Lloyd, A. (2003). A network of redundant bHLH proteins functions in all TTG1-dependent pathways of Arabidopsis. Development 130, 4859–4869. doi: 10.1242/dev.00681
Zhang, J., Huang, G. Q., Zou, D., Yan, J. Q., Li, Y., Hu, S., et al. (2018). The cotton (Gossypium hirsutum) NAC transcription factor (FSN1) as a positive regulator participates in controlling secondary cell wall biosynthesis and modification of fibers. New Phytol. 217, 625–640. doi: 10.1111/nph.14864
Zhang, F., Zuo, K., Zhang, J., Liu, X., Zhang, L., Sun, X., et al. (2010). An L1 box binding protein, GbML1, interacts with GbMYB25 to control cotton fiber development. J. Exp. Bot. 61, 3599–3613. doi: 10.1093/jxb/erq173
Zhao, B., Cao, J. F., Hu, G. J., Chen, Z. W., Wang, L. Y., Shangguan, X. X., et al. (2018). Core cis-element variation confers subgenome-biased expression of a transcription factor that functions in cotton fiber elongation. New Phytol. 218, 1061–1075. doi: 10.1111/nph.15063
Zheng, D., Ye, W., Song, Q., Han, F., Zhang, T., and Chen, Z. J. (2016). Histone modifications define expression bias of homoeologous genomes in allotetraploid cotton. Plant Physiol. 172, 1760–1771. doi: 10.1104/pp.16.01210
Zhou, Z., An, L., Sun, L., Zhu, S., Xi, W., Broun, P., et al. (2011). Zinc finger protein5 is required for the control of trichome initiation by acting upstream of zinc finger protein8 in Arabidopsis. Plant Physiol. 157, 673–682. doi: 10.1104/pp.111.180281
Zhou, J., Lee, C., Zhong, R., and Ye, Z. H. (2009). MYB58 and MYB63 are transcriptional activators of the lignin biosynthetic pathway during secondary cell wall formation in Arabidopsis. Plant Cell 21, 248–266. doi: 10.1105/tpc.108.063321
Zhou, Z., Sun, L., Zhao, Y., An, L., Yan, A., Meng, X., et al. (2013). Zinc finger protein 6 (ZFP6) regulates trichome initiation by integrating gibberellin and cytokinin signaling in Arabidopsis thaliana. New Phytol. 198, 699–708. doi: 10.1111/nph.12211
Keywords: trichome, Arabidopsis, cotton, fiber, multi-dimensional regulation
Citation: Wang Y, Zhou Q, Meng Z, Abid MA, Wang Y, Wei Y, Guo S, Zhang R and Liang C (2022) Multi-Dimensional Molecular Regulation of Trichome Development in Arabidopsis and Cotton. Front. Plant Sci. 13:892381. doi: 10.3389/fpls.2022.892381
Received: 09 March 2022; Accepted: 21 March 2022;
Published: 07 April 2022.
Edited by:
Baohua Wang, Nantong University, ChinaReviewed by:
Ling Xu, Zhejiang Sci-Tech University, ChinaCopyright © 2022 Wang, Zhou, Meng, Abid, Wang, Wei, Guo, Zhang and Liang. This is an open-access article distributed under the terms of the Creative Commons Attribution License (CC BY). The use, distribution or reproduction in other forums is permitted, provided the original author(s) and the copyright owner(s) are credited and that the original publication in this journal is cited, in accordance with accepted academic practice. No use, distribution or reproduction is permitted which does not comply with these terms.
*Correspondence: Chengzhen Liang, bGlhbmdjaGVuZ3poZW5AY2Fhcy5jbg==; Rui Zhang, emhhbmdydWlAY2Fhcy5jbg==
†These authors have contributed equally to this work
Disclaimer: All claims expressed in this article are solely those of the authors and do not necessarily represent those of their affiliated organizations, or those of the publisher, the editors and the reviewers. Any product that may be evaluated in this article or claim that may be made by its manufacturer is not guaranteed or endorsed by the publisher.
Research integrity at Frontiers
Learn more about the work of our research integrity team to safeguard the quality of each article we publish.