- National Key Laboratory of Crop Genetic Improvement, Hubei Hongshan Laboratory, MARA Key Laboratory of Crop Ecophysiology and Farming System in the Middle Reaches of the Yangtze River, College of Plant Science and Technology, Huazhong Agricultural University, Wuhan, China
Ratoon rice is proposed as a promising way to improve rice productivity via increasing harvest frequency. Nitrogen (N) is the most effective in promoting the development and growth of ratoon plants. However, limited information is available on how different N management practices affect the biomass production of the ratoon crop (RC) through influencing canopy light interception, radiation use efficiency (RUE), and N utilization. Field experiments were conducted in central China in 2016 and 2017 to examine the effects of N management practices on the biomass accumulation of RC and the underlying physiological mechanisms. The N rates (100 vs. 200 kg N ha−1) in the main crop (MC) had a small and inconsistent effect on the biomass accumulation of RC. N application at 15 days after heading of MC for promoting bud development (Nbud, 100 kg N ha–1) increased total biomass production of RC by 17.2–19.1%, due to the improvements in both pre- and post-heading biomass production during the ratoon season (BPratoon). N application at 1–2 days after harvesting of MC for promoting the growth of regenerated tillers (Ntiller, 100 kg N ha–1) increased total biomass production of RC by 7.8–15.9% due to the improvements in post-heading BPratoon alone or both pre- and post-heading BPratoon. The differences in BPratoon caused by Nbud and Ntiller were associated with crop growth rate, leaf area index, RUE, and N uptake of RC. Total N uptake of RC was improved by Nbud through increasing stubble N content at harvest of MC and by Ntiller through increasing plant N uptake during the ratoon season. N use efficiency for BPratoon was reduced by Ntiller but not by Nbud. These results suggest that both Nbud and Ntiller play important roles in improving biomass production in RC, although Nbud was more efficient than Ntiller.
Introduction
Rice (Oryza sativa L.) is the dominant staple food, sustaining more than 65% of the population in China (Huang and Zou, 2018). Maintaining a continuous increase in rice production is vital to meet the demand of a growing population, thus ensuring national food security (Peng et al., 2009). It has been suggested that most of this increase must come from increasing harvest frequency on the existing farmland (Ray and Foley, 2013) because it is difficult to expand farmland area and improve yield potential (Deng et al., 2019; FAOSTAT, 2020). Ratoon rice, the production of a second crop (ratoon crop, RC) developed by regenerated tillers from nodal buds on the stubble left after the first crop (main crop, MC) harvest (Harrell et al., 2009), is a promising way to boost total productivity via increasing harvest frequency (Wang et al., 2020). Without the activities of land preparation, sowing, and transplanting, the grain yield of RC can be as high as 60% of that of MC (Firouzi et al., 2018; Zheng et al., 2021). Compared with China’s other rice cropping systems, ratoon rice exhibits higher annual productivity than single-season rice and higher net economic return than double-season rice (Yuan et al., 2019). As a consequence, ratoon rice has developed rapidly in China and has been a hot research topic worldwide in recent years (Wang et al., 2021; Yu et al., 2022; Zheng et al., 2022; Zhou et al., 2022).
Many studies have focused on improving the grain yield of RC through optimizing cultural practices, especially nitrogen (N) fertilizer management (Ichii, 1988; Wang et al., 2019; Yang et al., 2021). N is the most essential nutrient element that affects the development and growth of regenerated tillers (Santos et al., 2003). It is reported that the grain yield of RC could be improved by increased N rates for both the main (Nmain) and ratoon seasons (Bahar and De Datta, 1977; Zheng et al., 2004; Xu et al., 2015). For the ratoon season, N applications for promoting bud development (Nbud) and promoting the growth of regenerated tillers (Ntiller) are two common N management practices, which are applied 15–20 days after the heading of MC and immediately after the harvest of MC, respectively (Xiong et al., 2000). In previous studies, the importance of Nbud vs. Ntiller for increasing the grain yield of RC has been a controversy. Some of them showed that Nbud had a more evident and positive influence on the grain yield of RC than Ntiller, but the reverse was true for others (Turner and McIlrath, 1988; Yuan et al., 1996; Wang et al., 2019). Despite this discrepancy, it was proved that the yield improvement of RC by both Nbud and Ntiller was mainly attributed to aboveground total biomass production rather than the harvest index (Wang et al., 2019; Yang et al., 2021). Increasing biomass production has also been considered an important approach to further improve rice yield after the Green Revolution (Laza et al., 2003). However, limited information is available on how different N management practices affect the biomass production of RC.
Plant biomass is the product of solar radiation intercepted by the canopy and the efficiency with which the radiation is converted into biomass (radiation use efficiency, RUE) (De Costa et al., 2006). Intercepted solar radiation is determined by total incident radiation during the rice growth duration and the percentage of radiation intercepted by the canopy (Huang et al., 2016). Physiologically, plant N status influences canopy light interception through effects on canopy morphological characteristics such as leaf area, angle, and orientation (Wei et al., 2018). In addition, RUE is dependent on photosynthesis and respiration, both of which are sensitive to N uptake by rice plants (Sinclair and Horie, 1989; Reich et al., 2006). In general, high N uptake can achieve high RUE and consequently high biomass production (Li et al., 2012). In contrast, N use efficiency (NUE) has tended to decrease as the N rate has increased (Peng et al., 2006). Recent studies by Wang et al. (2019) and Yang et al. (2021) compared the effects of Nbud and Ntiller on NUE for grain production (NUEg) of RC and indicated that NUEg of RC was reduced by Ntiller but not by Nbud. However, whether the NUE for biomass production (NUEb) of RC could be reduced with Nmain, Nbud, or Ntiller application remains unclear.
In this study, biomass accumulation, canopy light interception, RUE, N uptake, and NUEb of ratoon rice were determined in a 2-year field experiment with different N treatments (Nmain, Nbud, and Ntiller). The objective was to examine the effects of N management on biomass accumulation of RC and its relationships with RUE and N utilization. This is the follow-up study of our previous report (Wang et al., 2019).
Materials and Methods
Site Description
Field experiments were conducted at Jiupu Village (30°23′ N, 115°43′ E), Chidong Town, Qichun County, Hubei Province, central China, during four crop growing seasons of the rice ratooning system in 2016 and 2017. Hubei Province is located in a subtropical monsoon climate zone, where ratoon rice is typically planted with MC from March to August and with RC from August to October or November. The daily mean temperature, rainfall, and solar radiation during the rice-growing seasons are shown in Figure 1. The topsoil (0–20 cm layer) of the experimental field had a clay loam texture with the following characteristics: pH 4.7, 34.5 g kg–1 organic matter, 2.1 g kg–1 total N, 11.9 mg kg–1 Olsen P, and 217.2 mg kg–1 available K.
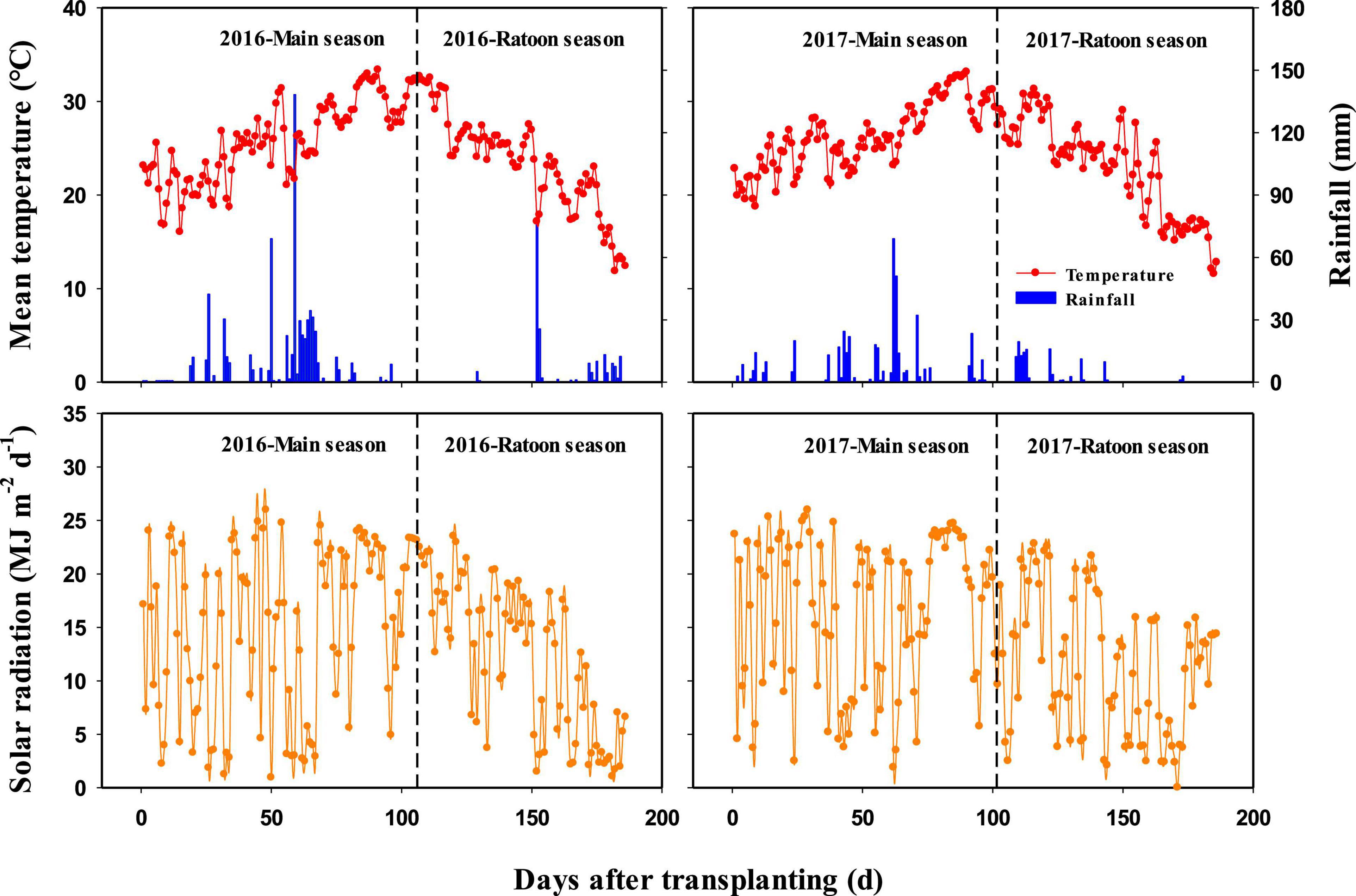
Figure 1. Daily mean temperature, rainfall, and solar radiation during rice growing seasons in 2016 and 2017.
Experimental Design and Crop Management
The treatments were laid out in a split-split-split-plot design with four replications. The rice variety was designated as the main plot, Nmain as the subplot, Nbud as the sub-subplot, and Ntiller as the sub-sub-subplot. Each sub-sub-subplot measured 5.0 m × 5.0 m. The varieties consisted of a hybrid Liangyou6326 (LY6326) and an inbred Huanghuazhan (HHZ), which have been widely grown for ratoon rice in the study region. For Nmain, two N levels were a moderate application rate (100 kg N ha–1) and a high application rate (200 kg N ha–1). At both N levels, N was split-applied with 40% as basal (1 day before transplanting), 30% at early tillering (7 days after transplanting), and 30% at panicle initiation in MC. For both Nbud and Ntiller, two N levels were a zero-N control (0 kg N ha–1) and a high application rate (100 kg N ha–1). At a high N level, Nbud was applied 15 days after the heading of MC, and Ntiller was applied 1–2 days after the harvest of MC. All N fertilizers were applied in the form of urea. To minimize seepage between plots, all bunds were covered with plastic film inserted into the soil to form a barrier.
In both years, 38-day-old seedlings from wet bed nurseries were manually transplanted on 29 April. Transplanting was done at a hill spacing of 13.3 cm × 30.0 cm with two seedlings per hill. Before transplanting, 40 kg P ha–1, 60 kg K ha–1, and 5 kg Zn ha–1 were incorporated into the soil together with basal N. In addition, 60 kg K ha–1 was applied in all plots at the time of Nbud application. The sources of P, K, and Zn were calcium superphosphate, potassium chloride, and zinc sulfate heptahydrate, respectively. The MC was harvested manually on August 12, 2016 and August 09, 2017, with a stubble height of 45 cm. Details of other crop management, such as irrigation, disease, pest, and weed, were described by Wang et al. (2019). The growth durations for two varieties grown in MC and RC are provided in Supplementary Table 1.
Sampling and Measurements
In each plot, 12 hills were sampled at the heading and maturity of both MC and RC. At the heading, the green leaf area was measured using a leaf area meter (LI-3100, LI-COR Inc., Lincoln, NE, United States) to determine the leaf area index. The dry weight of whole plants was measured after oven-drying at 80°C to constant weight to determine pre-heading biomass production. At the maturity of MC, plants were cut into lower and upper parts, which included the tissues below and above the stubble height (45 cm), respectively. For the lower part, the regenerated buds that developed during the main season were stripped out from each node of stubble. The upper part was further divided into straw and panicle. Eventually, plant samples were separated into stubble, regenerated bud, straw, and panicle. At the maturity of RC, plant samples were separated into stubble (left in MC), straw (regenerated in RC), and panicle. The dry weight of each component was measured after oven-drying at 80°C to a constant weight. Total biomass production was the total dry weight of stubble, regenerated bud (only for MC), straw, and panicle. For MC, post-heading biomass production was the difference between total biomass production and pre-heading biomass production. Pre- and post-heading crop growth rates were calculated as the ratio of biomass production to growth duration from transplanting to heading and from heading to harvest, respectively. For RC, biomass production during the ratoon season (BPratoon), pre- and post-heading BPratoon, and pre- and post-heading crop growth rates were calculated according to the following formulas:
The samples of each component at maturity of MC and RC were ground to measure N concentration using an elemental analyzer (Elementar Vario MAX CNS/CN, Elementar Trading Co., Ltd., Germany). The N content of each component was calculated as the product of N concentration and dry weight. Total N uptake (TNU) at maturity was the sum of the N content of each component. The N uptake during the ratoon season (NUratoon) was the difference between TNU of RC and N contents of stubble and regenerated bud at harvest of MC. The NUEb was calculated as the ratio of total biomass production to TNU for MC and the ratio of BPratoon to NUratoon for RC.
Canopy light interception was measured during the main (from transplanting to harvest of MC) and ratoon seasons (from harvest of MC to harvest of RC). The measurements were performed between 1,100 and 1,300 h at an interval of 7–15 days using a linear photosynthetically active radiation ceptometer (AccuPAR LP-80, Decagon Devices Inc., Pullman, WA, United States). In each plot, light intensity inside the canopy was measured by placing the light bar in the middle of two rows, slightly above the water surface. Three readings were taken between rows and another three within rows. Light intensity above the canopy was immediately recorded after the light measurement inside the canopy. Canopy light interception was calculated as the percentage of light intercepted by the canopy [100 × (light intensity above the canopy − light intensity below the canopy)/light intensity above the canopy]. Intercepted radiation during a growth period was calculated using the average canopy light interception and accumulated incident solar radiation during this growth period [1/2 × (canopy light interception at the beginning of the growth period + canopy light interception at the end of the growth period) × accumulated incident solar radiation during the growth period]. The intercepted radiation during the entire growing season was the summation of IR during each growth period. The RUE during the entire growing season was calculated as the ratio of total biomass production to intercepted radiation for MC and the ratio of BPratoon to intercepted radiation for RC.
Data Analysis
Statistical data analysis was performed using Statistix 9.0 (Analytical Software, Tallahassee, FL, United States). All data were subjected to ANOVA using a linear model (general AOV/AOCV). ANOVA was conducted separately for each year, and individual effects of variety and Nmain and their interactions were detected for MC, and individual effects of variety, Nmain, Nbud, and Ntiller and all possible interactions were detected for RC. Mean separation was run with Tukey’s honestly significant difference (HSD) test at the 0.05 probability level. Regression analysis was conducted in a linear regression procedure to determine the coefficient of determination (R2). All graphical representations of data were performed using SigmaPlot 12.5 (Systat Software Inc., Point Richmond, CA, United States).
Results
Biomass Accumulation and Crop Growth Rate of Main Crop
Total biomass production of MC was significantly affected by Nmain in both years (Table 1 and Supplementary Table 2). Compared with a moderate N rate, a high N rate increased total biomass production by 8.7% on average across varieties and years. This increase was attributed to both higher pre- and post-heading biomass production, although the differences in these two traits between N rates were insignificant in most cases. Similarly, a high N rate increased pre- and post-heading crop growth rates, thus increasing the total crop growth rate over the entire growing season.
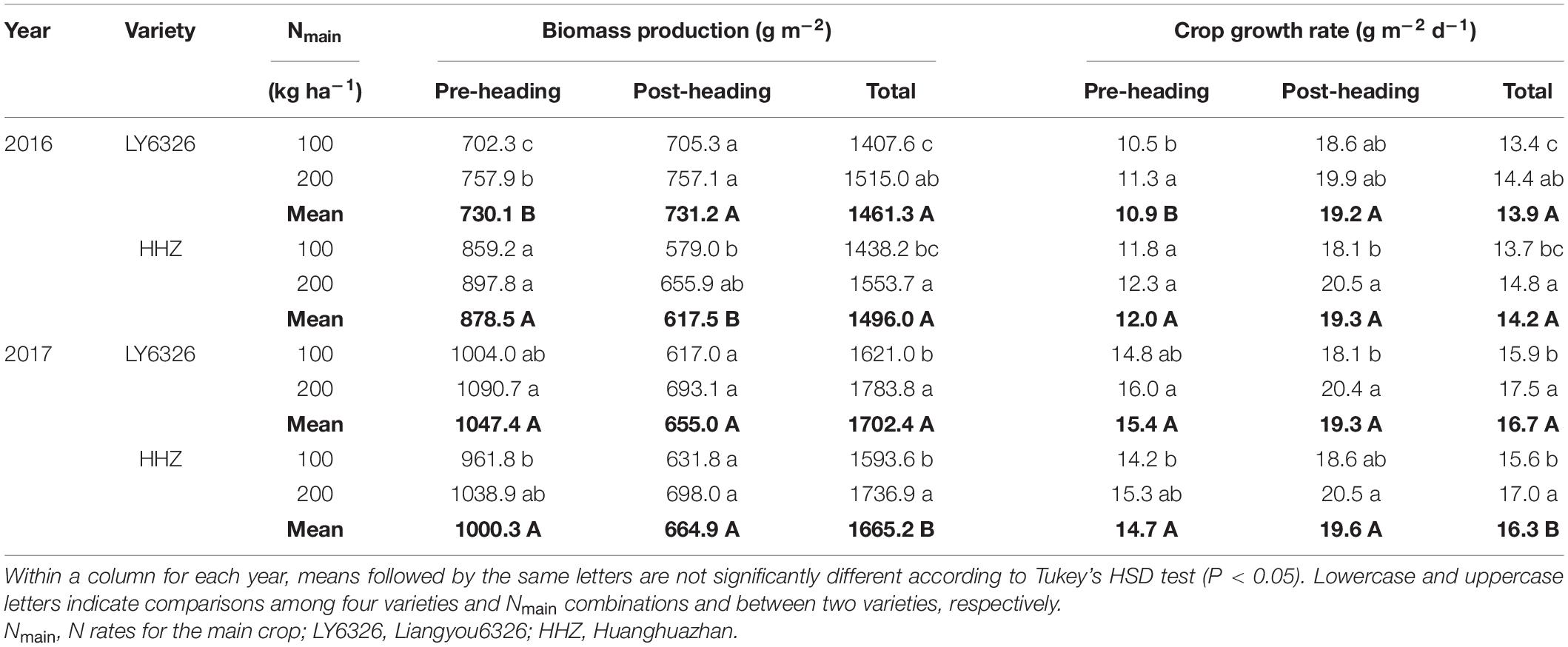
Table 1. Biomass production and crop growth rate for two varieties grown in the main crop of 2016 and 2017.
Leaf Area Index, Intercepted Radiation, and Radiation Use Efficiency of Main Crop
The leaf area index at the heading was significantly increased by a high N rate of Nmain except for HHZ in 2016 (Table 2). The high N rate also increased total intercepted radiation, and the difference between N rates was significant in 2016 but not in 2017. This increase was attributed to both higher pre- and post-heading intercepted radiation. No significant differences between N rates were observed in RUE in each growth period.
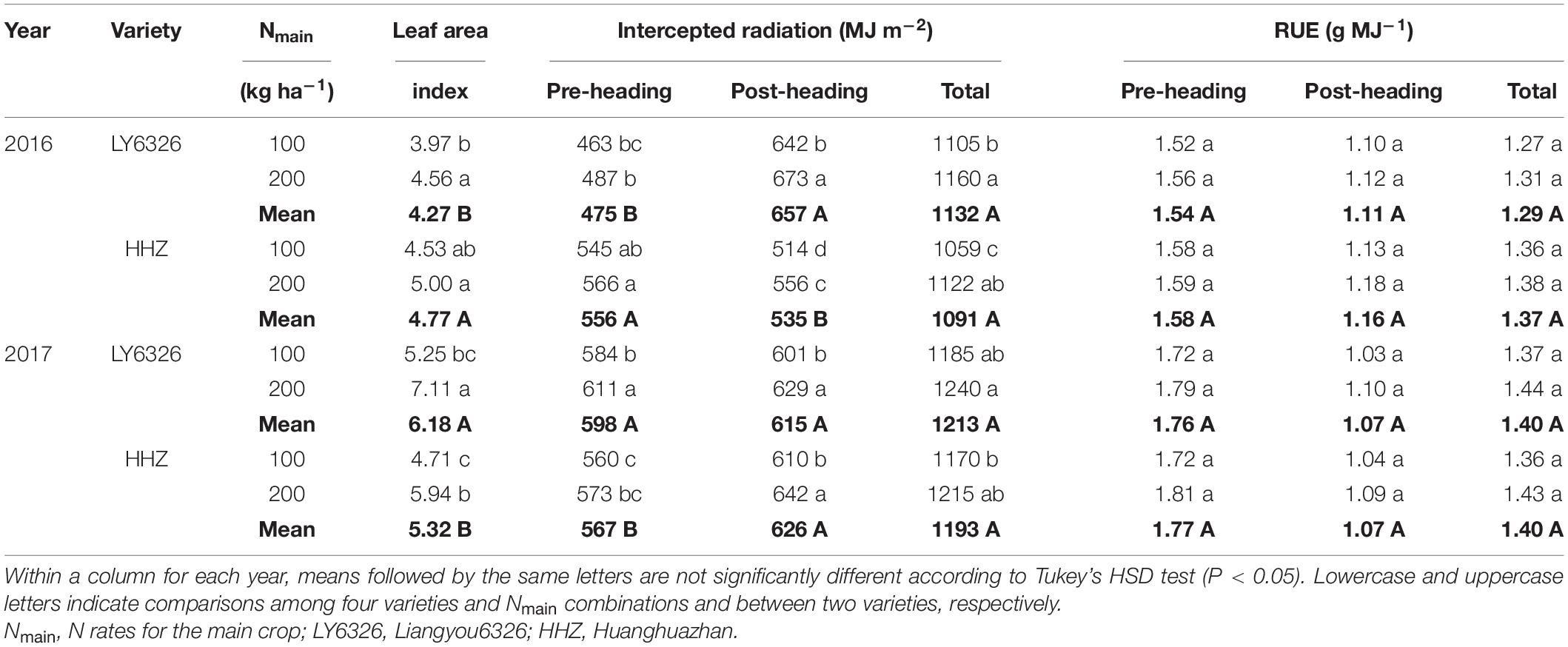
Table 2. Leaf area index at heading, intercepted radiation, and radiation use efficiency (RUE) for two varieties grown in the main crop of 2016 and 2017.
N Uptake and N Use Efficiency of Main Crop
Total N uptake was significantly increased by the high N rate of Nmain, and the increase was 12.8% on average across varieties and years (Table 3). In contrast, NUEb was consistently decreased by a high N rate, although the difference between N rates was significant only for LY6326 in 2016.
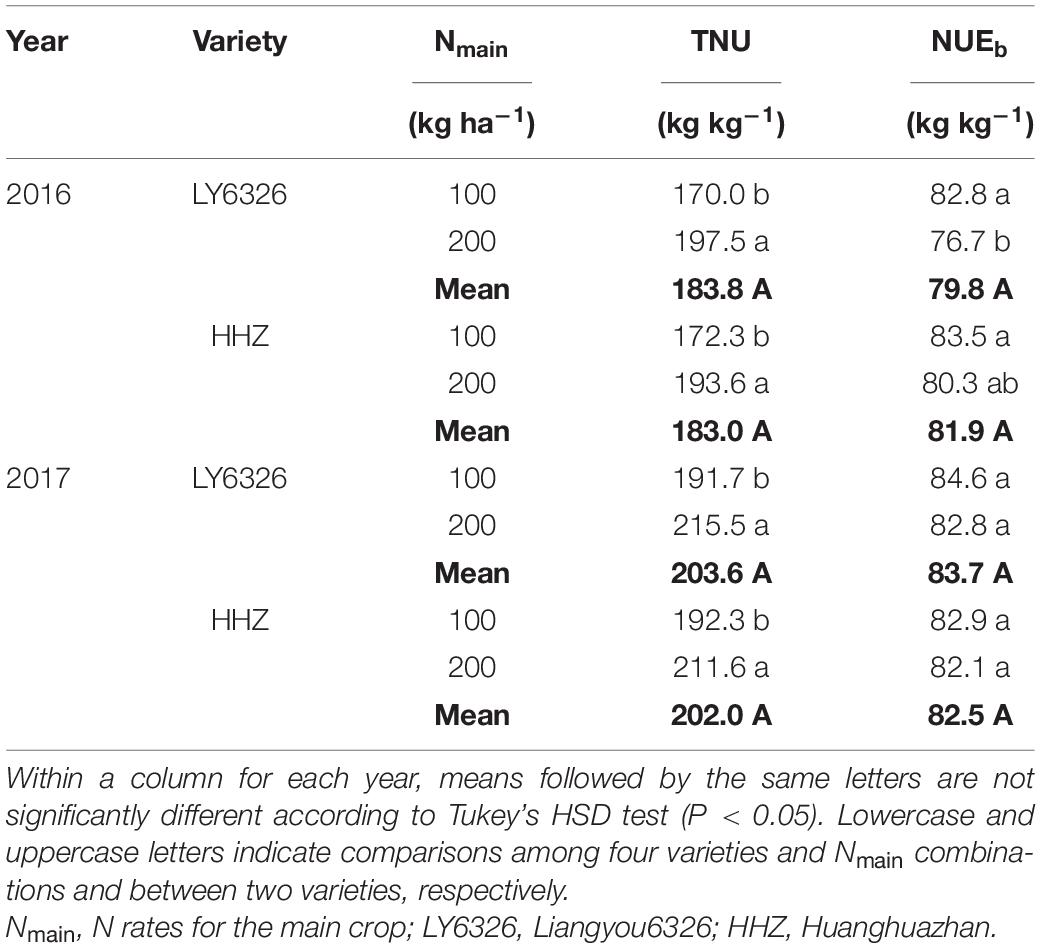
Table 3. Total N uptake (TNU) and N use efficiency for biomass production (NUEb) for two varieties grown in the main crop of 2016 and 2017.
Related Traits of Left Stubble and Regenerated Bud at Harvest of Main Crop
Nmain had small and inconsistent effects on dry weight, N concentration, and N content of left stubble at harvest of MC (Figure 2). Significant differences between Nbud treatments were observed in stubble N concentration and content but not in stubble dry weight. With Nbud application, stubble N concentration and content were increased by 49.0 and 47.9%, respectively, in 2016, and by 23.4 and 21.9%, respectively, in 2017.
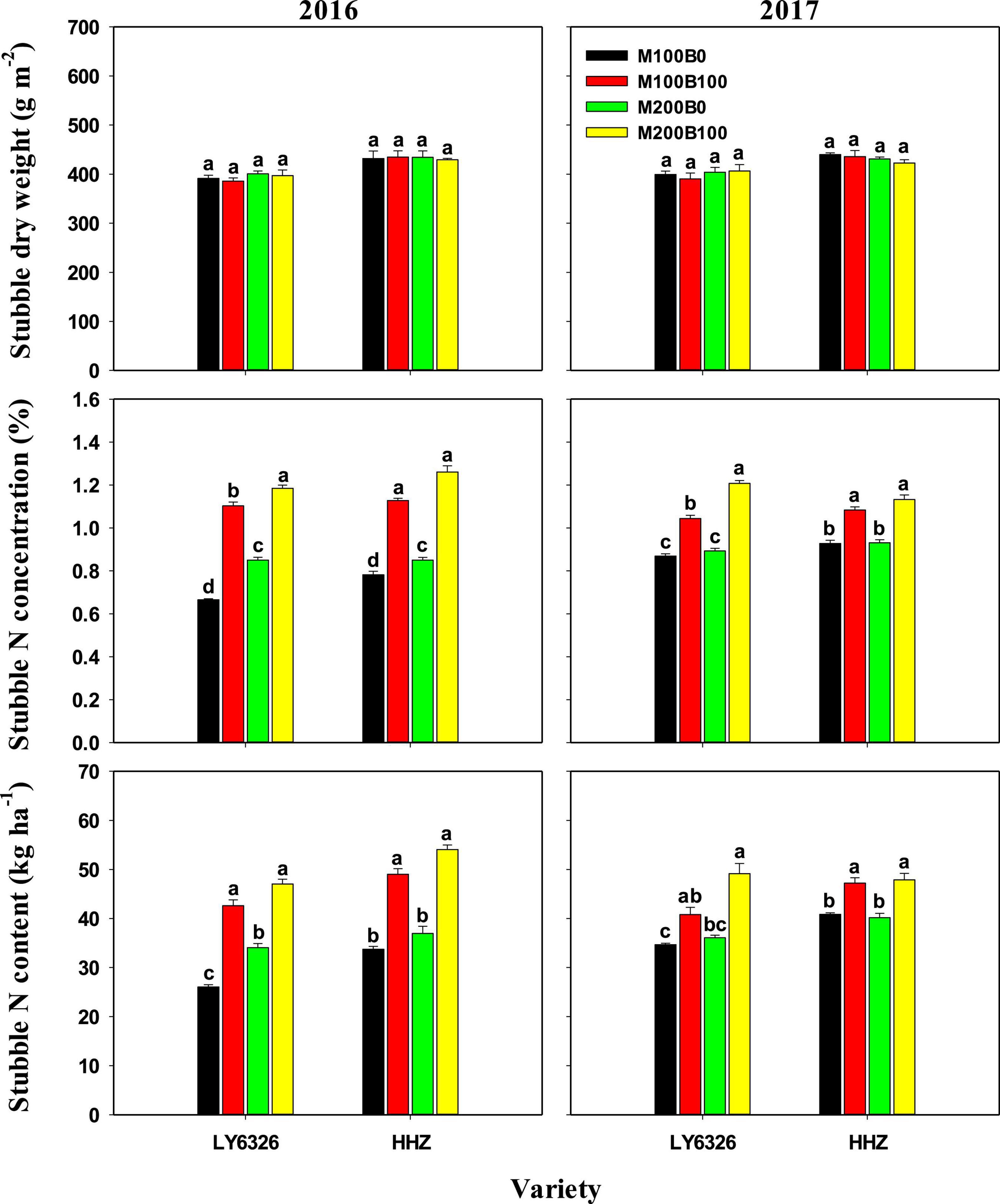
Figure 2. Stubble dry weight and N concentration and content at harvest of the main crop in 2016 and 2017. For each variety, means (±SE) followed by the same letters are not significantly different according to Tukey’s HSD test (P < 0.05). M100 and M200, Nmain of 100 and 200 kg N ha–1, respectively; B0 and B100, Nbud of 0 and 100 kg N ha–1, respectively. LY6326, Liangyou6326; HHZ, Huanghuazhan.
Nmain also had small and inconsistent effects on dry weight, N concentration, and N content of regenerated bud at harvest of MC (Figure 3). Significant differences between Nbud treatments were observed in all three traits in 2016. In 2017, a consistent difference between Nbud treatments was observed only for bud N concentration. With Nbud application, bud dry weight, N concentration, and N content were increased by 23.5, 25.6, and 52.3%, respectively, in 2016. In 2017, Nbud application increased bud N concentration by 37.0%. Due to the decreased bud dry weight, bud N content was much lower in 2017 compared with 2016, whereas there was no difference in bud N concentration between 2 years.
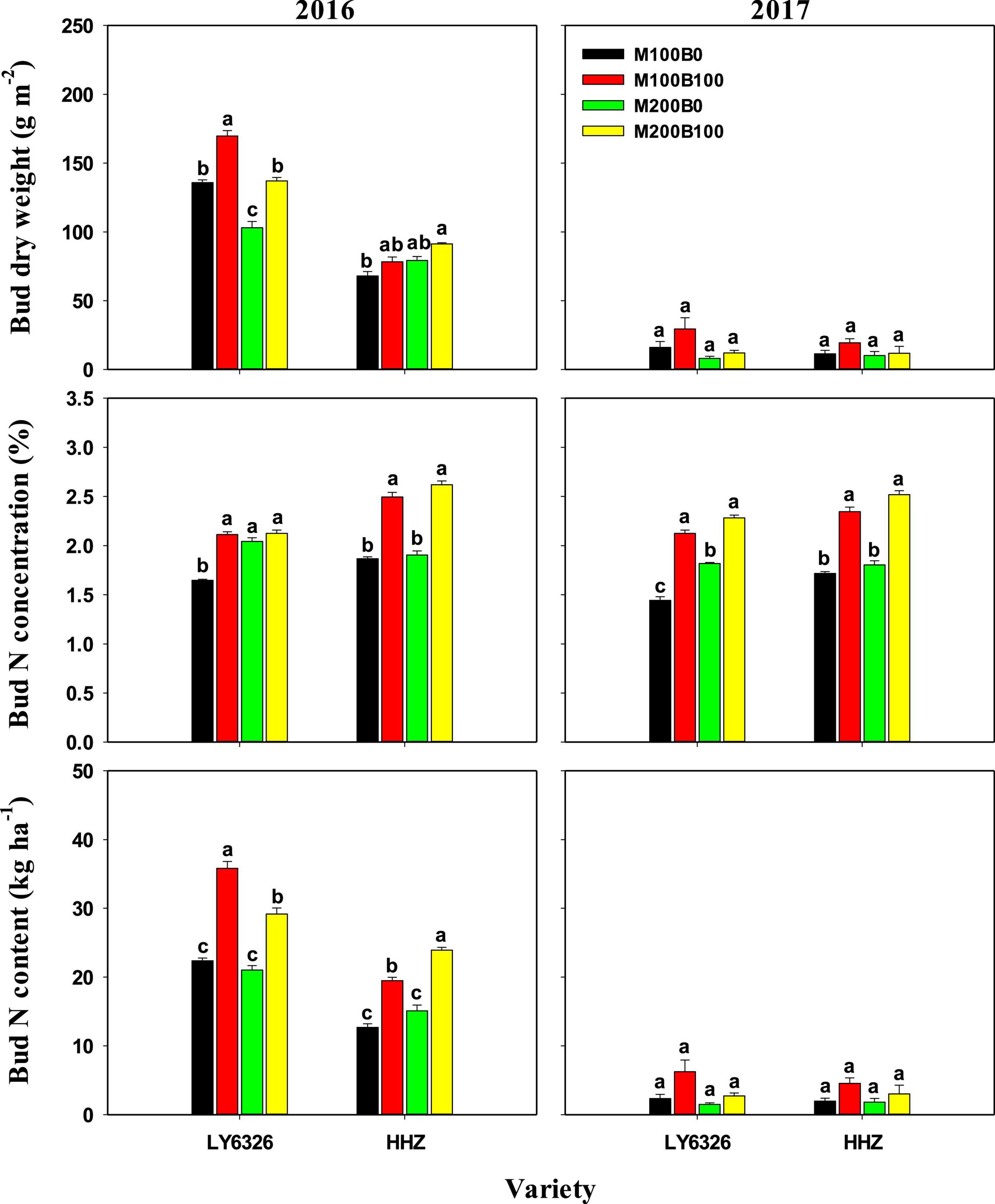
Figure 3. Bud dry weight and N concentration and content at harvest of the main crop in 2016 and 2017. For each variety, means (±SE) followed by the same letters are not significantly different according to Tukey’s HSD test (P < 0.05). M100 and M200, Nmain of 100 and 200 kg N ha–1, respectively; B0 and B100, Nbud of 0 and 100 kg N ha–1, respectively. LY6326, Liangyou6326; HHZ, Huanghuazhan.
Biomass Accumulation and Crop Growth Rate of Ratoon Crop
Total biomass production of RC was significantly affected by all experimental factors (i.e., Nmain, Nbud, Ntiller, and variety) in both years (Supplementary Tables 3, 4). The high N rate of Nmain increased total biomass production by 13.5% and 8.7% for LY6326 in 2016 and 2017, respectively, but Nmain had a small effect on total biomass production for HHZ in both years (Tables 4, 5). Both Nbud and Ntiller applications increased total biomass production, although the effect of Nbud on total biomass production was more consistent than that of Ntiller across years. On average, Nbud increased total biomass production by 17.2 and 19.1% in 2016 and 2017, respectively, and Ntiller increased total biomass production by 7.8 and 15.9% in 2016 and 2017, respectively. Overall, Nbud had the largest effect on total biomass production of RC, followed by Ntiller and Nmain.
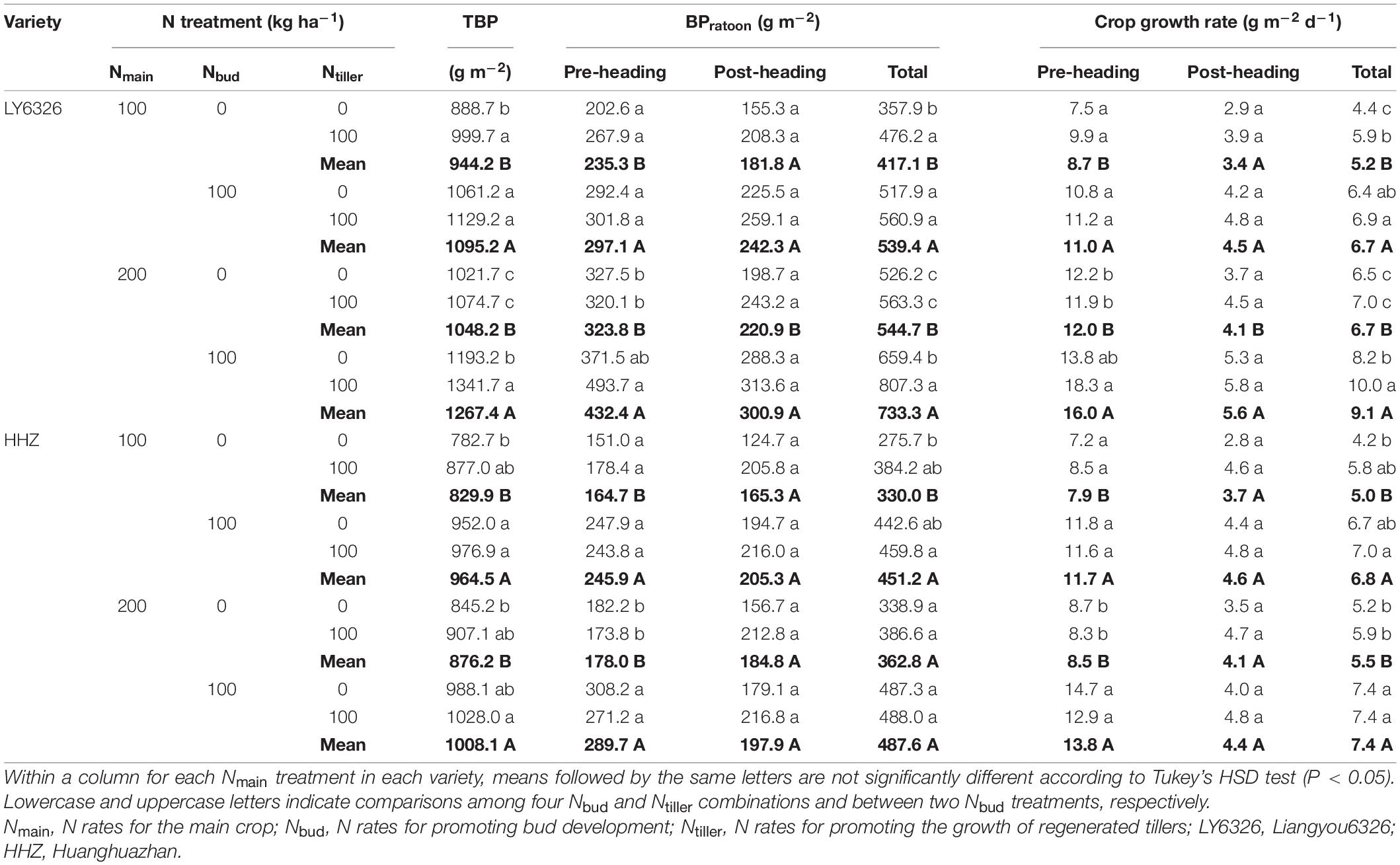
Table 4. Total biomass production (TBP), biomass production during ratoon season (BPratoon), and crop growth rate for two varieties grown in ratoon crop of 2016.
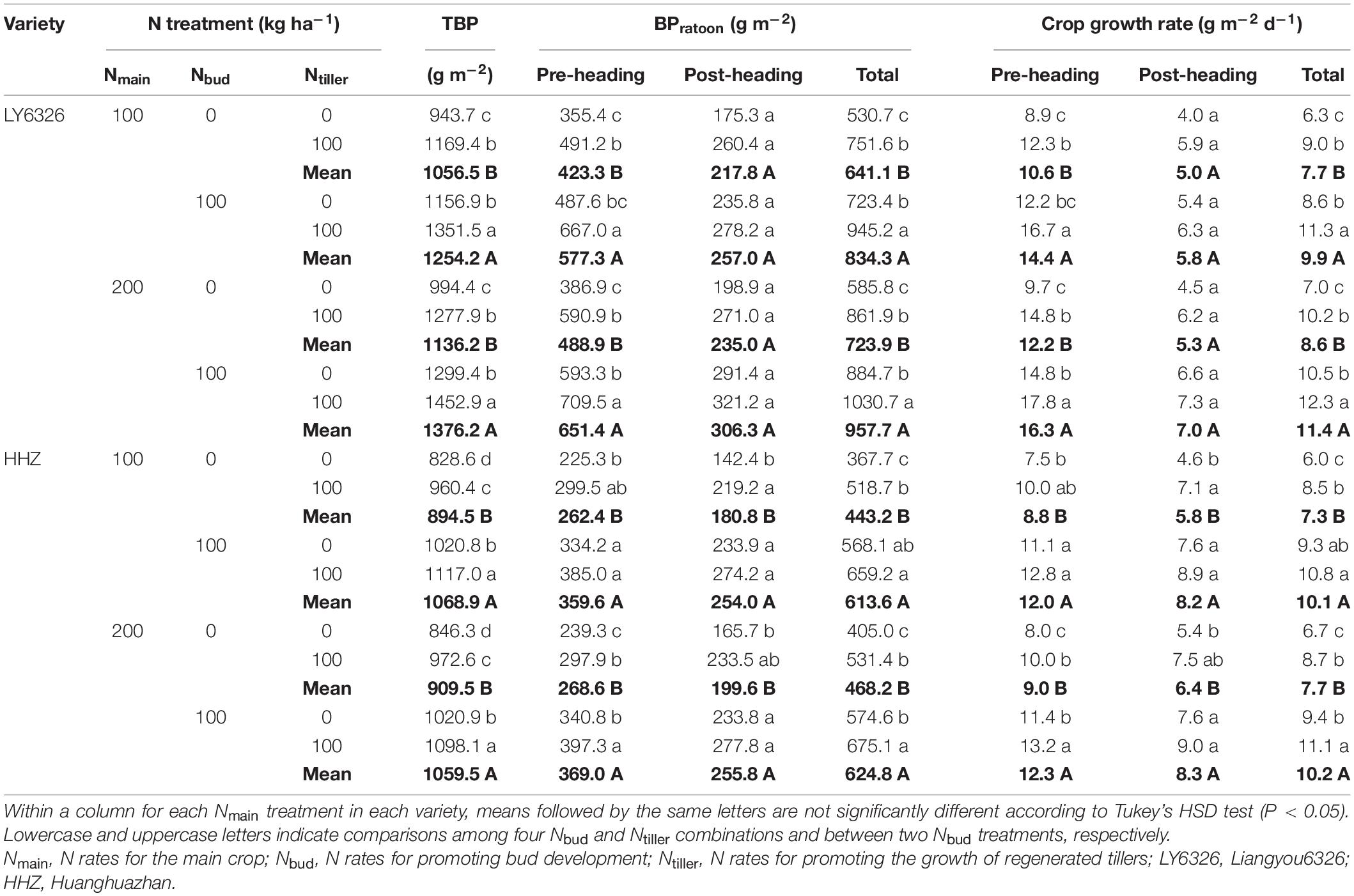
Table 5. Total biomass production (TBP), biomass production during ratoon season (BPratoon), and crop growth rate for two varieties grown in ratoon crop of 2017.
The effect of Nmain on total BPratoon was small and inconsistent, and total BPratoon was mainly affected by Nbud and Ntiller (Tables 4, 5). With Nbud application, total BPratoon was increased by 33.6 and 33.1% in 2016 and 2017, respectively. The application of Ntiller also increased total BPratoon, but the improvement in 2016 (14.4%) was smaller than that in 2017 (28.7%). Both pre- and post-heading BPratoon contributed to the increase in total BPratoon by Nbud, although the contribution from pre-heading BPratoon was greater than that from post-heading BPratoon. The increase in total BPratoon by Ntiller was explained by post-heading BPratoon alone in 2016 and by both pre- and post-heading BPratoon in 2017. The effects of Nbud and Ntiller on crop growth rate were similar to those on BPratoon.
Leaf Area Index, Intercepted Radiation, and Radiation Use Efficiency of Ratoon Crop
Nmain had small and inconsistent effects on leaf area index at heading, intercepted radiation, and RUE. The application of Nbud significantly and consistently increased leaf area index across the years, whereas the effect of Ntiller on leaf area index was significant in 2017 but not in 2016 (Tables 6, 7). Even though both Nbud and Ntiller applications increased intercepted radiation in each growth period, all the improvements were relatively small. Compared with Ntiller, Nbud had a more consistent effect on total RUE across years. With Nbud application, total RUE was increased by 28.1 and 29.5% in 2016 and 2017, respectively, resulting from the increases in both pre- and post-heading RUE. With Ntiller application, total RUE was increased by 7.9 and 23.3% in 2016 and 2017, resulting from the increases in post-heading RUE alone and both pre- and post-heading RUE, respectively.
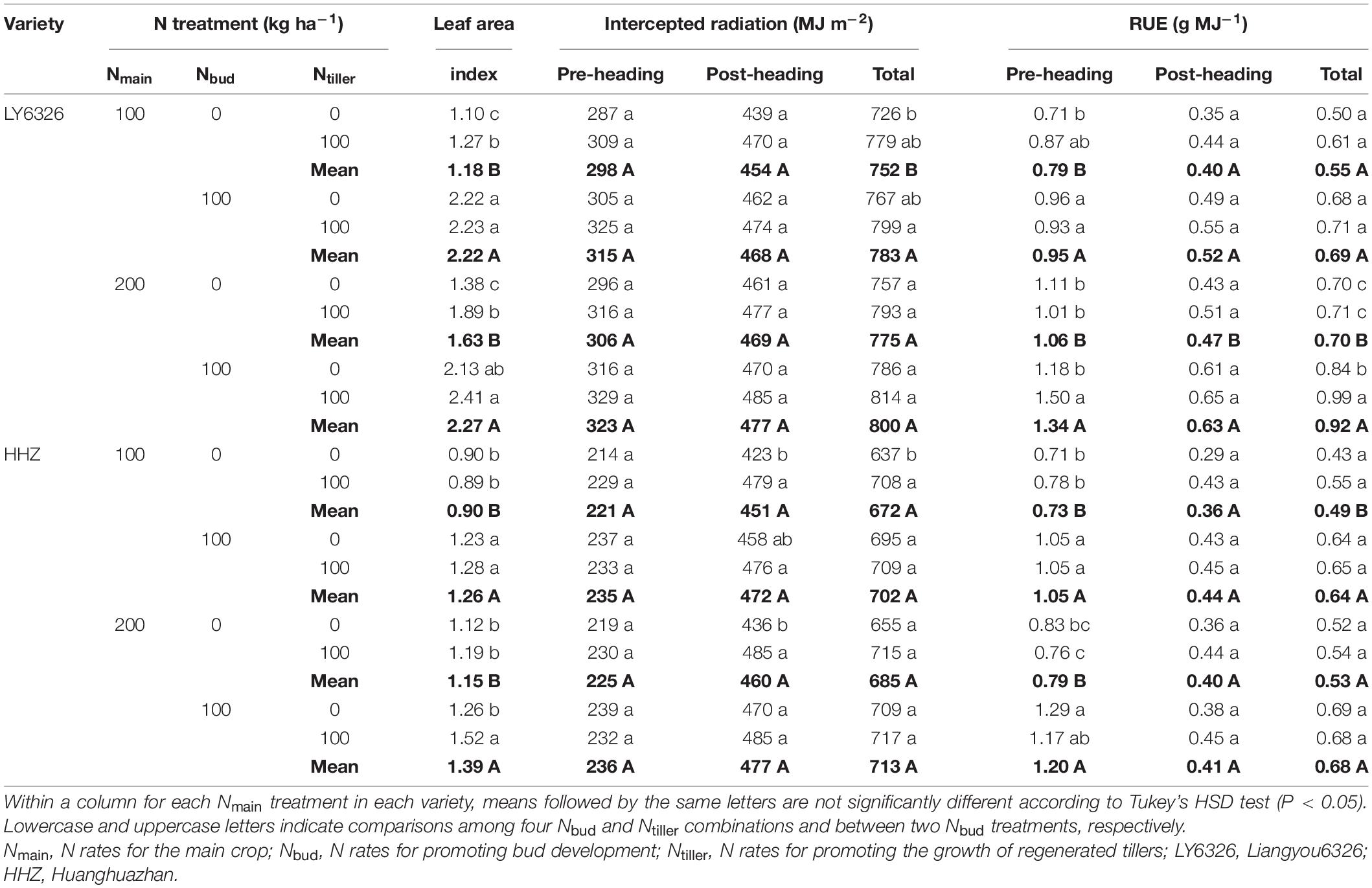
Table 6. Leaf area index at heading, intercepted radiation, and radiation use efficiency (RUE) for two varieties grown in ratoon crop of 2016.
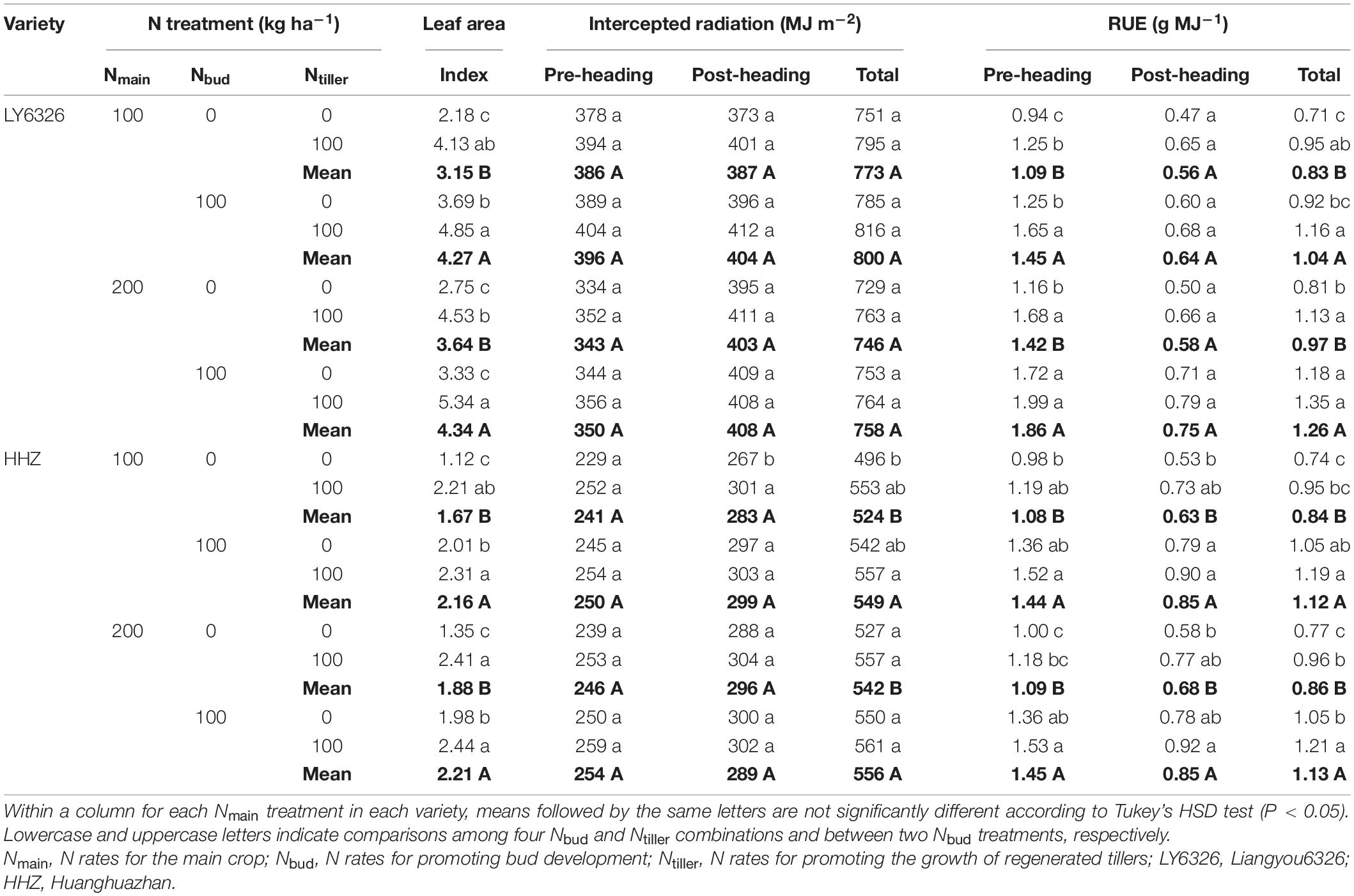
Table 7. Leaf area index at heading, intercepted radiation, and radiation use efficiency (RUE) for two varieties grown in ratoon crop of 2017.
N Uptake and N Use Efficiency of Ratoon Crop
Nmain had small and inconsistent effects on N uptake and NUE of RC. Both Nbud and Ntiller had significant effects on TNU in both years (Supplementary Tables 5, 6). With Nbud and Ntiller applications, TNU was increased by 27.8 and 31.8%, respectively (Tables 8, 9). The applications of Nbud and Ntiller also increased UNratoon, but the effect of Ntiller on UNratoon was larger and more consistent than that of Nbud across the years. With Ntiller application, UNratoon was increased by 101.9 and 61.1% in 2016 and 2017, respectively. The effect of Nbud on the UNratoon was significant in 2017 but not in 2016. As a consequence, NUratoon/TNU was mainly affected by Ntiller rather than Nbud. For NUEb, Nbud and Ntiller had opposite effects in both years. NUEb was increased with the Nbud application, and the effect was significant in 2016 but not in 2017. In contrast, Ntiller application decreased NUEb by 43.9 and 20.8% in 2016 and 2017, respectively.
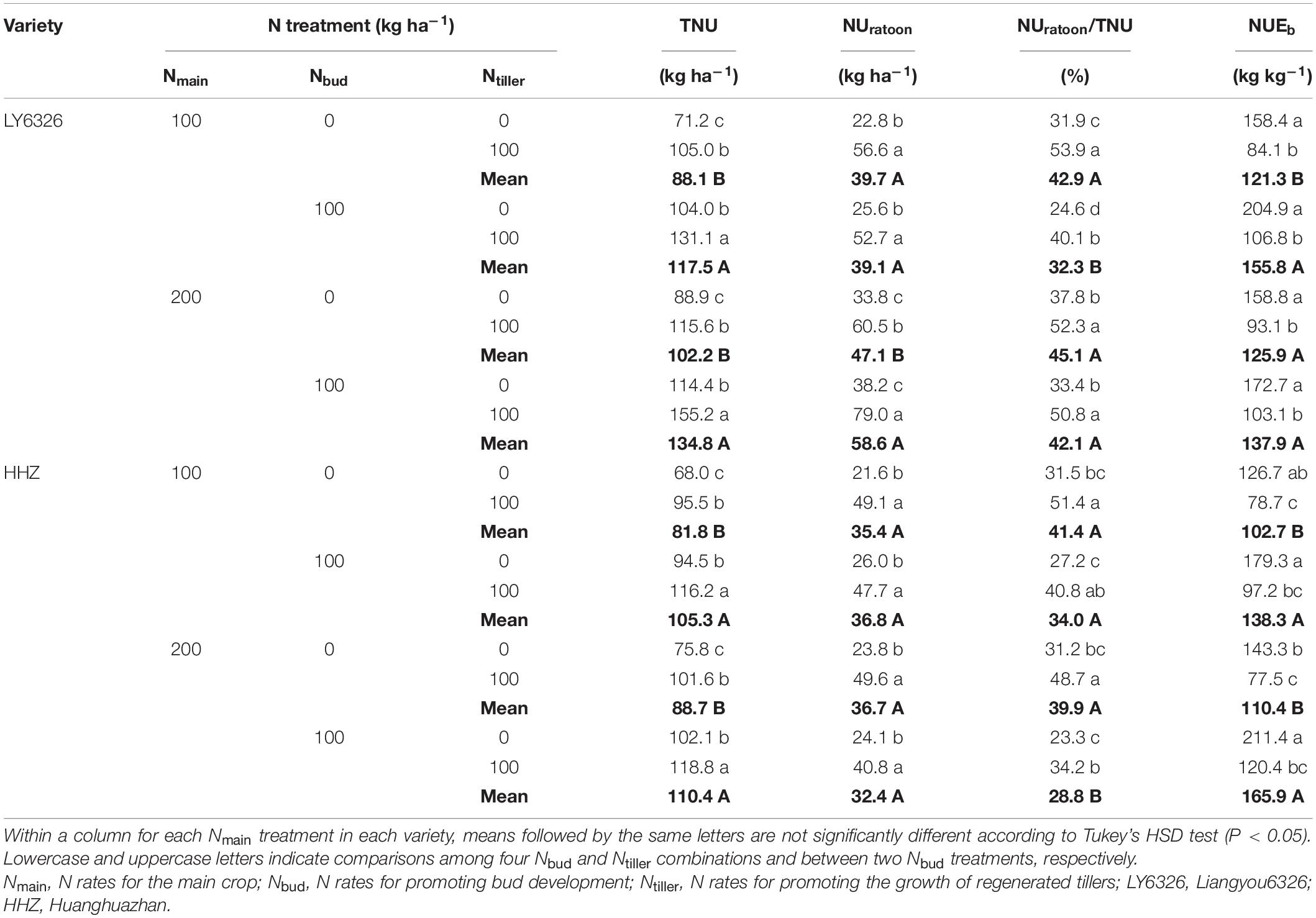
Table 8. Total N uptake (TNU), N uptake during ratoon season (NUratoon) and its ratio to total N uptake (NUratoon/TNU), and nitrogen use efficiency for biomass production during ratoon season (NUEb) for two varieties grown in ratoon crop of 2016.
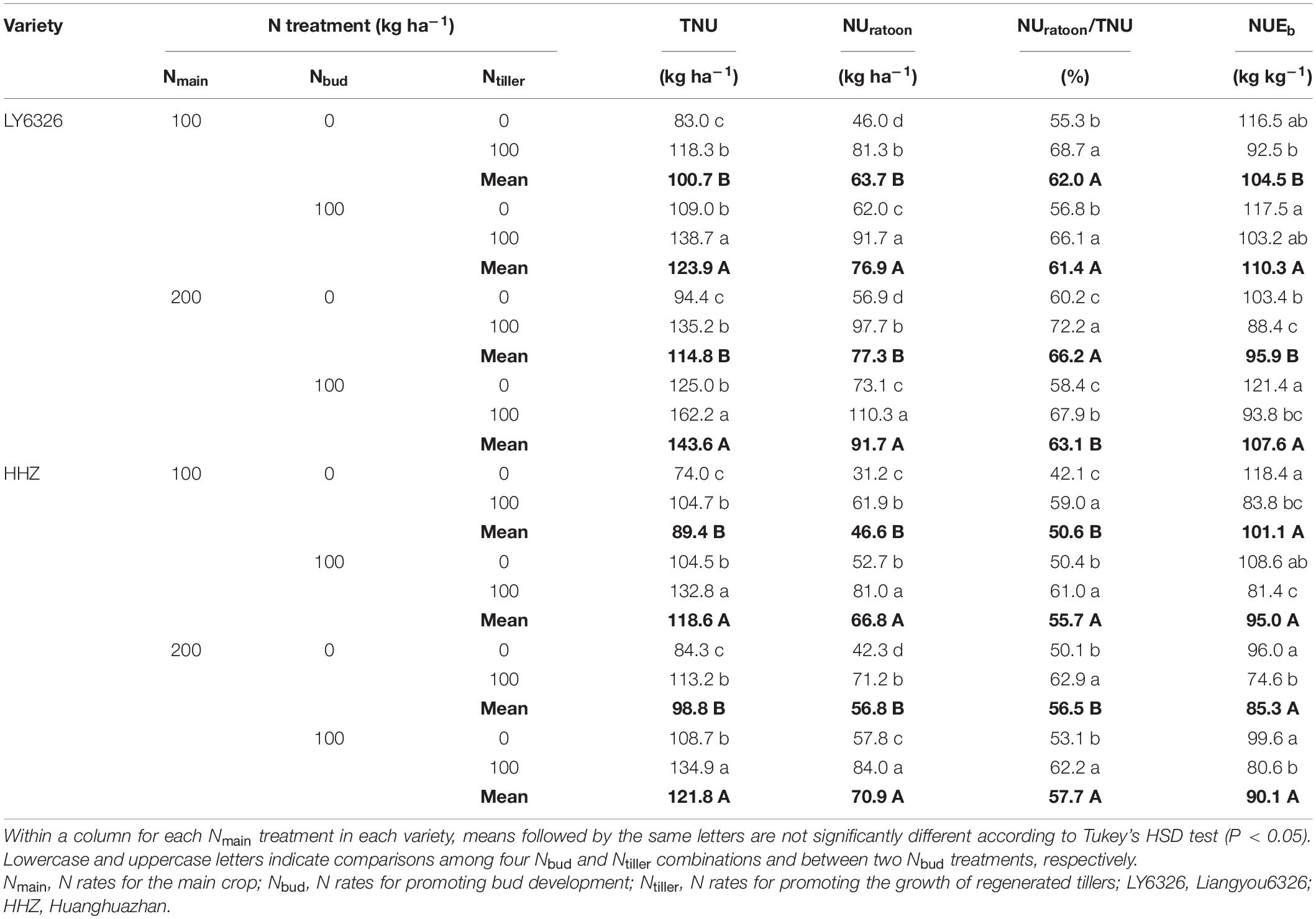
Table 9. Total N uptake (TNU), N uptake during ratoon season (NUratoon) and its ratio to total N uptake (NUratoon/TNU), and nitrogen use efficiency for biomass production during ratoon season (NUEb) for two varieties grown in ratoon crop of 2017.
Regression Analysis
In RC, total BPratoon was significantly and positively related to total intercepted radiation and total RUE in both years, and the coefficients of determination were higher for total RUE (R2 = 0.977 in 2016 and R2 = 0.615 in 2017) than for total intercepted radiation (R2 = 0.721 in 2016 and R2 = 0.565 in 2017) (Figure 4). Total BPratoon was also significantly and positively related to NUratoon, and the coefficients of determination were 0.411 and 0.782 in 2016 and 2017, respectively. However, there was an insignificant relationship between total BPratoon and NUEb in both years.
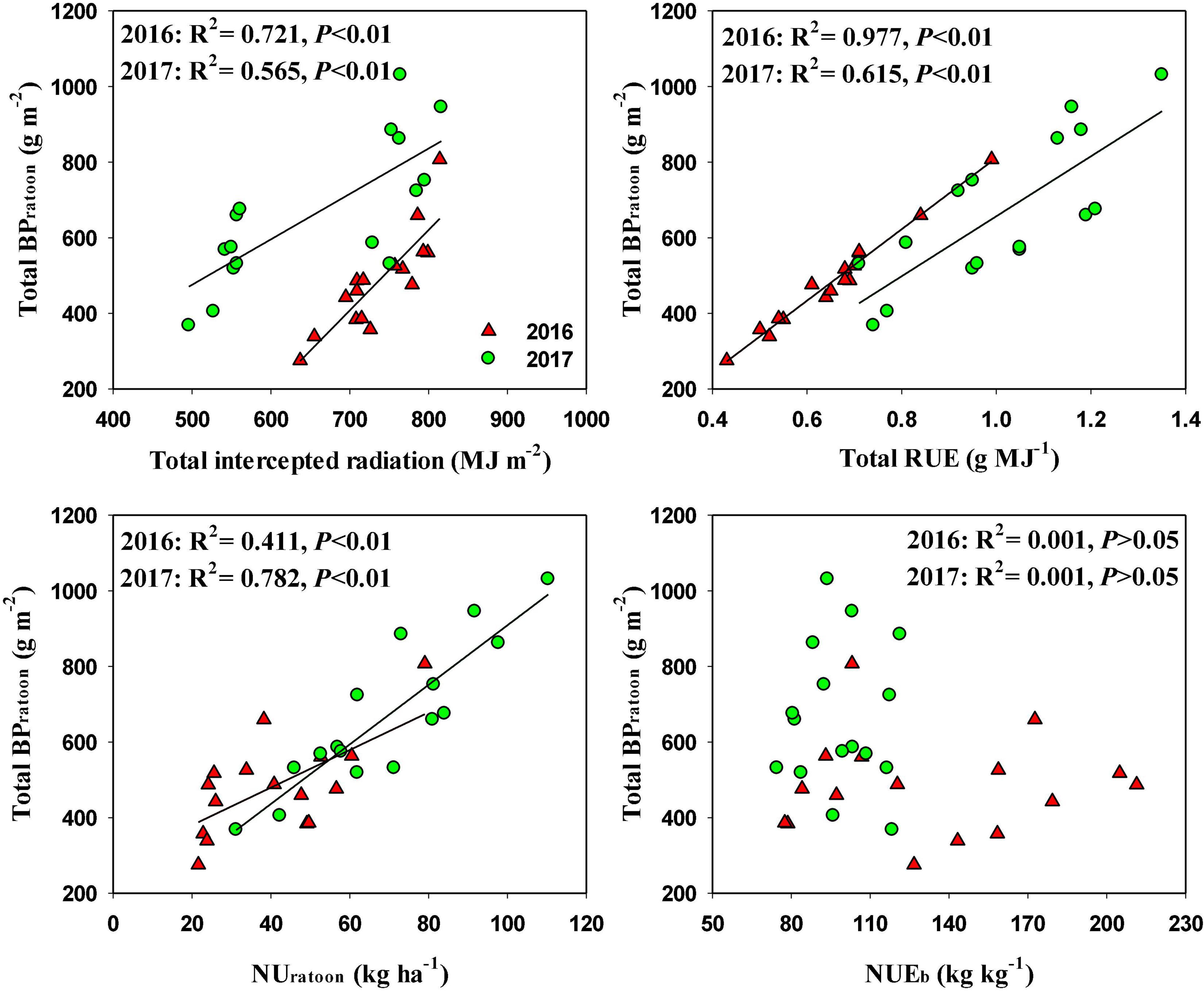
Figure 4. Relationships total biomass production during ratoon season (BPratoon) and total intercepted radiation, total radiation use efficiency (RUE), N uptake during ratoon season (UNratoon), and N use efficiency for biomass production (NUEb) in ratoon crop of 2016 and 2017.
Discussion
Rice yield is determined by biomass production and harvest index (Yoshida, 1981). However, it is widely accepted that there is less scope to further increase the harvest index after the Green Revolution (Laza et al., 2003), and achieving greater rice yield primarily depends on increasing biomass production (Peng et al., 1999). Consistently, our previous study showed that total biomass production instead of harvest index explained the differences in grain yield of RC caused by Nbud and Ntiller (Wang et al., 2019). In this study, we further proved that the positive effects of Nbud and Ntiller on total biomass production were mainly due to increased BPratoon, because there were small differences in dry weights of stubble and regenerated bud at harvest of MC under different N treatments. Both pre- and post-heading BPratoon contributed to the increase in total BPratoon by Nbud, although the contribution from pre-heading BPratoon was greater than that from post-heading BPratoon. However, the increase in total BPratoon by Ntiller was attributed to increased post-heading BPratoon alone or both increased pre- and post-heading BPratoon. Overall, the effect of Nbud on BPratoon was more evident and consistent than that of Ntiller. In addition, Nmain had an even smaller effect on BPratoon than Ntiller.
Biomass production is a function of growth duration and crop growth rate (Yoshida, 1981). In our study, there was a similar growth duration under different N treatments for both varieties (Supplementary Table 1). The differences in BPratoon caused by Nbud and Ntiller were solely attributed to the crop growth rate. The application of Nbud and Ntiller could facilitate the development and growth of regenerated buds, thus increasing crop growth rate in the ratoon season. Besides, the high crop growth rate is largely driven by an increased leaf area index (Loomis and Connor, 1992; Ying et al., 1998). Our result showed that the leaf area index at the heading stage of RC was improved with Nbud and Ntiller applications, although the improvement by Ntiller was not as great as that by Nbud.
In another approach, biomass production can be improved through increasing intercepted radiation or RUE or both (Huang et al., 2016). It is also reported that optimal N application is important to intercept more photosynthetically active radiation and obtain high RUE (Li et al., 2012). In our study, Nbud and Ntiller significantly increased RUE in the ratoon season, whereas the effects of these two N treatments on intercepted radiation were relatively small. With Nbud and Ntiller applications, total RUE was increased by 28.8 and 15.6%, respectively, and total intercepted radiation was increased by only 3.5 and 5.0%, respectively. The increase in RUE could be a result of improved canopy photosynthesis in the ratoon season with Nbud and Ntiller applications. Moreover, the regression analysis indicated that BPratoon was highly related to RUE. These results demonstrated that RUE was the key factor that determines the variation in BPratoon under different N management practices. This finding was consistent with a previous study, which stated that further improvement in biomass production in rice depended on the increased RUE rather than intercepted radiation (Mitchell and Sheehy, 2006).
Nbud and Ntiller increased TNU at the maturity of RC in different ways. For RC, plant N accumulation consisted of the N contents in the stubble and regenerated bud at harvest of MC and the N uptake by the plant during the ratoon season (NUratoon). Nbud increased TNU mainly by increasing the N contents in the stubble and regenerated bud at harvest of MC, and partially by increasing NUratoon. In contrast, Ntiller increased TNU by increasing NUratoon. The underpinning factor for this disparity in N uptake between these two N treatments resulted from the different times of application for Nbud and Ntiller. In general, Nbud is applied around the middle point between heading and harvest of MC, and it is not easy to be directly absorbed by RC plants. However, applied Nbud could accumulate in left stubble after the harvest of MC, which provides N nutrients for the development of regenerated buds (Zhang et al., 1980). Ma et al. (1992) explored the N uptake and distribution of Nbud in ratoon rice using 15N isotope identification technology and reported that the remobilization of Nbud remaining in left stubble played an important role in supporting the growth of RC plants. In contrast, Ntiller is applied after the harvest of MC, and it can be rapidly absorbed and utilized by the regenerated tillers in RC. Despite this, NUEb of RC was significantly reduced by Ntiller, which might be due to excessive N fertilizer input (Peng et al., 2010). Under the conditions of our study, Nbud significantly increased plant N accumulation and biomass production of RC but not reduced NUEb.
There was a similar stubble dry weight at harvest of MC and total biomass production in RC between 2016 and 2017. However, the BPratoon of 2016 was lower than that of 2017, resulting in a higher dry weight of regenerated buds developed during the main season. In 2016, the field was flooded with a water depth of 25–40 cm continuously for 8 days when MC was in the late stage of panicle development (Figure 1). The partial submergence limited the grain filling of MC (Lin et al., 1997), which led to more assimilates accumulated in stem and sheath that accelerated the development and growth of regenerated buds. In this case, more Nbud was absorbed by the regenerated buds before MC was harvested in 2016, as compared with 2017. At harvest of MC, the N content in regenerated buds ranged from 12.7 to 35.8 kg ha–1 in 2016, whereas that ranged from 1.5 to 6.2 kg ha–1 in 2017. These results also partially explained why the effects of Nbud on the crop growth rate, RUE, leaf area index, and BPratoon of RC were larger than those of Ntiller and why the effects of Nbud on UNratoon and NUratoon/TNU of RC were smaller than those of Ntiller in 2016. Therefore, the importance of Nbud and Ntiller in improving the biomass accumulation and yield formation of RC not only depends on the stubble N status of MC but also is associated with the effect of the environment on the development of regenerated buds. Our findings would provide more evidence for contradictions about the effects of Nbud vs. Ntiller on plant growth of RC in different field trials.
Conclusion
Biomass production of RC was mainly affected by Nbud and Ntiller rather than Nmain. The improvement in biomass production during the ratoon season by Nbud was larger than that by Ntiller, due to more evident and consistent effects of Nbud on the crop growth rate, leaf area index, radiation use efficiency, and plant N uptake. NUE for biomass production during the ratoon season was reduced by Ntiller but not by Nbud.
Data Availability Statement
The original contributions presented in the study are included in the article/Supplementary Material, further inquiries can be directed to the corresponding author.
Author Contributions
CZ collected and analyzed the data and wrote the manuscript. YW conducted the field experiments and collected and analyzed the data. DY, SX, and YS collected the data. JH provided advice on experimental implementation. SP conceived and supervised the field experiments. FW supervised the field experiments and revised the manuscript. All authors contributed to the article and approved the submitted version.
Funding
This study was supported by the National Key Research and Development Program of China (2021YFD1901205), the Fundamental Research Funds for the Central Universities (2662020ZKPY015), the Major International (Regional) Joint Research Project of the National Natural Science Foundation of China (32061143038), and the Earmarked Fund for China Agriculture Research System (CARS-01-20).
Conflict of Interest
The authors declare that the research was conducted in the absence of any commercial or financial relationships that could be construed as a potential conflict of interest.
Publisher’s Note
All claims expressed in this article are solely those of the authors and do not necessarily represent those of their affiliated organizations, or those of the publisher, the editors and the reviewers. Any product that may be evaluated in this article, or claim that may be made by its manufacturer, is not guaranteed or endorsed by the publisher.
Supplementary Material
The Supplementary Material for this article can be found online at: https://www.frontiersin.org/articles/10.3389/fpls.2022.889542/full#supplementary-material
References
Bahar, F. A., and De Datta, S. K. (1977). Prospects of increasing tropical rice production through ratooning. Agron. J. 36, 536–554. doi: 10.2134/agronj1977.0002196200690
De Costa, W. A. J. M., Weerakoon, W. M. W., Herath, H. M. L. K., Amaratunga, K. S. P., and Abeywardena, R. M. I. (2006). Physiology of yield determination of rice under elevated carbon dioxide at high temperature in a subhumid tropical climate. Field Crops Res. 96, 336–347. doi: 10.1016/j.fcr.2005.08.002
Deng, N., Grassini, P., Yang, H., Huang, J., Cassman, K. G., and Peng, S. (2019). Closing yield gaps for rice self-sufficiency in China. Nat. Commun. 10:1725. doi: 10.1038/s41467-019-09447-9
FAOSTAT (2020). FAO Statistic Yearbook 2020. Available online at: http://www.fao.org/faostat/en/#data (accessed March 3, 2022).
Firouzi, S., Nikkhah, A., and Aminpanah, H. (2018). Resource use efficiency of rice production upon single cropping and ratooning agro-systems in terms of bioethanol feedstock production. Energy 150, 694–701. doi: 10.1016/j.energy.2018.02.155
Harrell, D. L., Bond, J. A., and Blanche, S. (2009). Evaluation of main-crop stubble height on ratoon rice growth and development. Field Crops Res. 114, 396–403. doi: 10.1016/j.fcr.2009.09.011
Huang, M., and Zou, Y. (2018). Integrating mechanization with agronomy and breeding for food security in China. Field Crops Res. 224, 22–27. doi: 10.1016/j.fcr.2018.05.001
Huang, M., Shan, S., Zhou, X., Chen, J., Cao, F., Jiang, L., et al. (2016). Leaf photosynthetic performance related to higher radiation use efficiency and grain yield in hybrid rice. Field Crops Res. 193, 87–93. doi: 10.1016/j.fcr.2016.03.009
Ichii, M. (1988). “Some factors influencing the growth of rice Ratoon,” in Rice Ratooning, eds W. H. Smith and V. Kumble (Manila: International Rice Research Institute Press), 41–46. doi: 10.1104/pp.106.082891
Laza, R. C., Peng, S., Akita, S., and Saka, H. (2003). Contribution of biomass partitioning and translocation of grain yield under sub-optimum growing conditions in irrigated rice. Plant Prod. Sci. 6, 28–35. doi: 10.1626/pps.6.28
Li, D., Tang, Q., Zhang, Y., Qin, J., Li, H., Chen, L., et al. (2012). Effect of nitrogen regimes on grain yield, nitrogen utilization, radiation use efficiency, and sheath blight disease intensity in super hybrid rice. J. Integr. Agric. 11, 134–143. doi: 10.1016/S1671-2927(12)60791-3
Lin, W., Sun, F., Peng, K., Li, L., Wang, H., and Xia, W. (1997). Effects of flooding on the yield and the yield components of rice. J. Hunan Agric. Univ. 23, 50–54. (In Chinese with English abstract)
Loomis, R. S., and Connor, D. J. (1992). Crop Ecology: Productivity and Management in Agricultural Systems. Cambridge: Cambridge University Press.
Ma, J., Wang, H., Sun, X., and Ren, G. (1992). Distribution of budding 15N fertilizer and it’s effect in ratooning rice. Southwest China J. Agric. Sci. 5, 41–46. (In Chinese with English abstract),
Mitchell, P. L., and Sheehy, J. E. (2006). Supercharging rice photosynthesis to increase yield. New Phytol. 171, 688–693. doi: 10.1111/j.1469-8137.2006.01855.x
Peng, S., Buresh, R. J., Huang, J., Yang, J., Zou, Y., Zhong, X., et al. (2006). Strategies for overcoming low agronomic nitrogen use efficiency in irrigated rice systems in China. Field Crops Res. 96, 37–47. doi: 10.1016/j.fcr.2005.05.004
Peng, S., Buresh, R. J., Huang, J., Zhong, X., Zou, Y., Yang, J., et al. (2010). Improving nitrogen fertilization in rice by site-specific N management. A review. Agron. Sustain. Dev. 30, 649–656. doi: 10.1051/agro/2010002
Peng, S., Cassman, K. G., Virmani, S. S., Sheey, J., and Khush, G. S. (1999). Yield potential trends of tropical rice since the release of IR8 and the challenge of increasing rice yield potential. Crop Sci. 39, 1552–1559. doi: 10.2135/cropsci1999.3961552x
Peng, S., Tang, Q., and Zou, Y. (2009). Current status and challenges of rice production in China. Plant Prod. Sci. 12, 3–8. doi: 10.1626/pps.12.3
Ray, D. K., and Foley, J. A. (2013). Increasing global crop harvest frequency: recent trends and future directions. Environ. Res. Lett. 8:044041. doi: 10.1088/1748-9326/8/4/044041
Reich, P., Tjoelker, M., Machado, J., and Oleksyn, J. (2006). Universal scaling of repiratory metabolism, size and nitrogen in plants. Nature 439, 457–461. doi: 10.1038/nature04282
Santos, A. B., Fageria, N. K., and Prabhu, A. S. (2003). Rice ratooning management practices for higher yields. Commun. Soil Sci. Plant Anal. 34, 881–918. doi: 10.1081/css-120018981
Sinclair, T., and Horie, T. (1989). Leaf nitrogen, photosynthesis, and crop radiation use efficiency: a review. Crop Sci. 29, 90–98. doi: 10.2135/cropsci1989.0011183X002900010023x
Turner, F. T., and McIlrath, W. O. (1988). “N fertilizer management for maximum Ratoon crop yields,” in Rice Ratooning, eds W. H. Smith and V. Kumble (Manila: International Rice Research Institute Press), 187–194. doi: 10.3389/fpls.2016.01017
Wang, F., Huang, J., and Peng, S. (2021). Research and development of mechanized rice ratooning technology in China. China Rice 27, 1–6. (In Chinese with English abstract), doi: 10.3969/j.issn.1006-8082.2021.01.001
Wang, W., He, A., Jiang, G., Sun, H., Jiang, M., Man, J., et al. (2020). Ratoon rice technology: a green and resource-efficient way for rice production. Adv. Agron. 159, 135–167. doi: 10.1016/bs.agron.2019.07.006
Wang, Y., Zheng, C., Xiao, S., Sun, Y., Huang, J., and Peng, S. (2019). Agronomic responses of ratoon rice to nitrogen management in central China. Field Crops Res. 241:107569. doi: 10.1016/j.fcr.2019.107569
Wei, H., Meng, T., Li, X., Dai, Q., Zhang, H., and Yin, X. (2018). Sink-source relationship during rice grain filling is associated with grain nitrogen concentration. Field Crops Res. 215, 23–28. doi: 10.1016/j.fcr.2017.09.029
Xiong, H., Ran, M., Xu, F., and Hong, S. (2000). Achievements and developments of ratooning rice in south of China. Acta Agron. Sin. 26, 297–304. (In Chinese with English abstract), doi: 10.3321/j.issn:0496-3490.2000.03.008
Xu, F., Xiong, H., Zhang, L., Zhu, Y., Jiang, P., Guo, X., et al. (2015). Progress in research of yield formation of ratooning rice and its high-yielding key regulation technologies. Sci. Agric. Sin. 48, 1702–1717. (In Chinese with English abstract), doi: 10.3864/j.issn.0578-1752.2015.09.04
Yang, D., Peng, S., Zheng, C., Xiang, H., Huang, J., Cui, K., et al. (2021). Effects of nitrogen fertilization for bud initiation and tiller growth on yield and quality of rice ratoon crop in central China. Field Crops Res. 272:108286. doi: 10.1016/j.fcr.2021.108286
Ying, J., Peng, S., He, Q., Yang, H., Yang, C., Visperas, R. M., et al. (1998). Comparison of high-yield rice in tropical and subtropical environments. I. Determinants of grain and dry matter yields. Field Crops Res. 57, 71–84. doi: 10.1016/S0378-4290(98)00077-X
Yoshida, S. (1981). Fundamentals of Rice Crop Science. Manila: International Rice Research Institute Press.
Yu, X., Tao, X., Liao, J., Liu, S. C., Xu, L., Yuan, S., et al. (2022). Predicting potential cultivation region and paddy area for ratoon rice production in China using Maxent model. Field Crops Res. 275:108372. doi: 10.1016/j.fcr.2021.108372
Yuan, J., Sun, X., Tian, Y., and Ma, J. (1996). Study on nitrogen requirement and split application of nitrogen fertilizer in ratooning rice. Acta Agron. Sin. 22, 345–352. (In Chinese with English abstract),
Yuan, S., Cassman, K. G., Huang, J., Peng, S., and Grassini, P. (2019). Can ratoon cropping improve resource use efficiencies and profitability of rice in central China? Field Crops Res. 234, 66–72. doi: 10.1016/j.fcr.2019.02.004
Zhang, M., Liu, Z., Chen, A., Qiu, Q., and Lin, L. (1980). Studies on the distribution of photosynthetic products and the absorption of nitrogen nutrients in regenerative rice by using isotopes 14C and 15N. Sci. Agric. Sin. 3, 1–5. (In Chinese with English abstract),
Zheng, C., Wang, Y., Xu, W., Yang, D., Yang, G., Yang, C., et al. (2021). Border effects of the main and ratoon crops in rice ratooning system. J. Integr. Agric. Available online at: https://www.chinaagrisci.com/Jwk_zgnykxen/EN/abstract/abstract373646.shtml (accessed March 3, 2022).
Zheng, C., Wang, Y., Yuan, S., Xiao, S., Sun, Y., Huang, J., et al. (2022). Heavy soil drying during mid-to-late grain filling stage of the main crop to reduce yield loss of the ratoon crop in a mechanized rice ratooning system. Crop J. 10, 280–285. doi: 10.1016/j.cj.2021.06.003
Zheng, J., Lin, W., Li, Y., Jiang, Z., and Zhuo, C. (2004). Nitrogen uptake and grain yield effects of double-cropping rice at different nitrogen application rates in the first crop of ratoon rice. Chin. J. Eco Agric. 12, 123–127. (In Chinese with English abstract),
Keywords: biomass, nitrogen management, nitrogen utilization, radiation use efficiency, ratoon rice
Citation: Zheng C, Wang Y, Yang D, Xiao S, Sun Y, Huang J, Peng S and Wang F (2022) Biomass, Radiation Use Efficiency, and Nitrogen Utilization of Ratoon Rice Respond to Nitrogen Management in Central China. Front. Plant Sci. 13:889542. doi: 10.3389/fpls.2022.889542
Received: 04 March 2022; Accepted: 24 March 2022;
Published: 27 April 2022.
Edited by:
Adnan Noor Shah, Khwaja Fareed University of Engineering and Information Technology (KFUEIT), PakistanReviewed by:
Shakeel Ahmad, Bahauddin Zakariya University, PakistanVeysel Turan, Bingöl University, Turkey
Copyright © 2022 Zheng, Wang, Yang, Xiao, Sun, Huang, Peng and Wang. This is an open-access article distributed under the terms of the Creative Commons Attribution License (CC BY). The use, distribution or reproduction in other forums is permitted, provided the original author(s) and the copyright owner(s) are credited and that the original publication in this journal is cited, in accordance with accepted academic practice. No use, distribution or reproduction is permitted which does not comply with these terms.
*Correspondence: Fei Wang, ZndhbmdAbWFpbC5oemF1LmVkdS5jbg==
†These authors have contributed equally to this work and share first authorship