- College of Environmental Science and Security Engineering, Tianjin University of Technology, Tianjin, China
In arid and semiarid regions with water shortage, forestry development is limited by water availability. Understanding how tree sap flow responds to water stress and microclimatic variables is essential for the management of trees and the understanding of the eco-physiological properties of trees in arid areas. In the city of Tianjin in northern China, we measured the sap flow of Fraxinus pennsylvanica, a widely distributed urban greening tree species in semiarid regions of China. We measured the sap flow in four F. pennsylvanica trees over 6 months (April–September 2021), using a thermal diffusion probe method, and simultaneously monitored microclimatic variables and soil moisture. Results indicated that high nighttime sap flow velocity might be produced under the water stress condition. In addition, the nighttime sap flow velocity under the water stress condition was more susceptible to the combined effects of meteorological factors at night. The daytime sap flow velocity exerted a highly significant positive effect on the nighttime sap flow velocity during the whole research period, and the model fit was higher in the early growing season than that in the late growing season (early growing season: R2 = 0.51, P < 0.01; late growing season: R2 = 0.36, P < 0.01). Vapor pressure deficit had a positive effect on daytime sap flow. However, net vapor pressure deficit restrained daytime sap flow velocity when the intercorrelation between the microclimatic variables was removed. Our study highlights that drought areas perhaps have higher nighttime sap flow and that more emphasis should be placed on nighttime sap flow and the response of nighttime sap flow to microclimatic variables. In addition, the influence of other microclimatic variables on vapor pressure deficit needs to be considered when analyzing the relationship between daytime sap flow and vapor pressure deficit. An increase in net VPD can suppress the daytime sap flow.
Introduction
Liquid water entering the root system from the soil is transported from the conducting tissue to leaves under transpiration pull. The sap flow along the conducting tissue of plants is indispensable for maintaining the hydraulic relationship between soil and atmosphere, supplying oxygen to xylem parenchymal cells, and facilitating nutrient uptake (Hubbart et al., 2007). Sap flow is an essential indicator of tree growth conditions, and water loss estimation, including dynamic changes and influencing factors, has been extensively investigated from the perspective of a single tree and stand (Paudel et al., 2015; Kupper et al., 2018). The traditional theory assumes that sap flow only occurs during the day because leaf stomata of trees and other C3 plants close at night. However, emerging research shows that nighttime sap flow is observed for many species and in a range of habitats (Yu et al., 2019; Fricke, 2020). Therefore, neglecting nighttime transpiration will lead to the underestimation of vegetation water consumption in the study of plant transpiration (O’Keefe et al., 2020). In addition, global climate observations demonstrated that land warms faster at night than during the day, and this trend can lead to an increase in plant water requirement at night (Zeppel et al., 2015). The nighttime sap flow movement in plants can facilitate the transport of nutrients and oxygen for plant growth, which in turn affects plant growth status and productivity (Chen et al., 2020b; Liu et al., 2021).
Furthermore, nighttime sap flow plays an important role in removing embolisms and enhancing the adaptation of plants to drought conditions (Fricke, 2019). Therefore, the nighttime sap flow is as significant as daytime sap flow in the study of tree transpiration. Previous studies on impact factors reported that vapor pressure deficit (VPD) and solar radiation (Rs) are dominant factors affecting the sap flow during the day and VPD is the critical driving factor related to nighttime sap flow (Di et al., 2019). Although critical driving factors vary during the day and night, the nighttime sap flow is partly a supplement to the water deficit caused by transpiration during the day. Stomatal activity and physiological regulation of plants demonstrate a certain relationship despite their difference during the day and night (Kupper et al., 2018). Analyzing the relationship between daytime and nighttime sap flow as well as their similarities and differences are necessary. Therefore, we hypothesize that daytime and nighttime sap flow velocities are slightly correlated despite the difference among the dominant influencing factors.
The sap flow in the soil–plant–atmosphere continuum is mainly influenced by environmental factors and its own physiological control. Soil moisture is the main source of plant sap flow because it affects the overall level of sap flow (Zhao et al., 2017; Montoro et al., 2020). Previous studies indicated that Rs and VPD are the main factors that influence transpiration in a single tree in terms of meteorological factors affecting plant sap flow (Deng et al., 2021). Another study indicated that solar radiation is the maximum impact factor and potential evapotranspiration and solar radiation are positively correlated with relative changes in the sap flow volume of plants with an R2 of 0.75 (Sun et al., 2021). However, the degree of influence of meteorological factors on sap flow varies for different moisture conditions. A previous report indicated that drought weakens the extent to which meteorological factors can explain changes in tree water use (Di et al., 2019). Discussing the relationship between sap flow and meteorological factors by dividing soil moisture into periods of sufficiency and stress is necessary. We hypothesize that the response of Fraxinus pennsylvanica to meteorological factors varies under different moisture conditions.
The sap flow of urban greening tree species is affected by both global warming temperatures and intensification of the urban heat island effect under the current trend of climate change (Roetzer et al., 2021). The increased water requirements of urban trees and limitations of water resources are both issues that must be investigated and addressed. However, studies on daytime sap flow in trees have mainly been carried out in plantations. The lack of information and studies on the diurnal sap flow of urban landscape trees has led to the underestimation of plant water consumption and subsequent decline in their growth and longevity (Wu et al., 2020). Therefore, the common green tree species of F. pennsylvanica found in North China is used as the research object of this study to investigate diurnal sap flow change patterns. This work aims to provide a theoretical basis for water requirements of F. pennsylvanica and irrigation strategies for urban green spaces essential in the sustainable development and management of cities. We hypothesize that (1) the relationship between nighttime and daytime sap flow velocity of F. pennsylvanica is significantly correlated but responds differently to driving factors and (2) the response of sap flow velocity to meteorological factors also varies under diverse soil moisture conditions (with and without water stress). Sap flux velocity of F. pennsylvanica was monitored from April to September 2021 using a thermal diffusive probe system to test the hypotheses. The analysis mainly aimed to (1) identify the dynamic characteristics of daytime and nighttime sap flows, (2) ascertain the relationship between sap flows under different moisture conditions and meteorological factors, and (3) determine the relationships between daytime and nighttime sap flows and variations in their responses to driving factors.
Materials and Methods
Study Site
The study was conducted at Tianjin University of Technology (38°51e st°51e s 116°51e s 1°20e s 11Xiqing District, Tianjin. The low and flat area is located in the northeastern part of the North China Plain at an altitude of about 5 m above sea level. The region is characterized by a warm temperate semihumid continental monsoon climate with four distinct seasons (Chao, 2019). The mean annual air temperature is 11.6°C and the mean annual precipitation is 586.1 mm, including 443.2 mm in the summer. The average annual sunshine is 2810.4 h, the average annual relative humidity is 63%, and the average annual evaporation is 1709.7 mm (Tianjin Meteorological Bureau).
The tree species used in the experiment was F. pennsylvanica, which is a major greening species in northern China. F. pennsylvanica can reach a maximum height of 10 m, and its growing season is from April to November. This tree is excellent for urban greening because of its characteristics of cold tolerance, low humidity requirement, salinity tolerance, and drought tolerance (Aiyun et al., 2019). Four trees were selected and used as samples according to the conditions of the study site (Table 1). The straight and well-grown trees demonstrated no discernible effects of disease or insect damage. Tree diameter at breast height was measured using a breast diameter ruler, which was placed around the tree at the height of 1.3 m above the ground before directly reading the value. Tree height was measured with an ultrasonic altimeter (Vertex IV, Haglof Inc., Sweden) by maintaining the instrument in a vertical orientation, aiming the crosshairs at the measurement target, and directly reading the height of the tree. Crown width was measured using a measuring tape and compass. The average of all measurements was calculated three times in the east-west and north–south directions using tape.
Sap Flow Measurements
We used the thermal diffusion probe (TDP) to monitor the F. pennsylvanica sap flow continuously between April and September 2021. A set of Granier-type thermal dissipation probes (TDP, Dynamax Inc., United States) was installed in each tree. Each set of thermal diffusion probes contains two probes: a reference probe containing a thermocouple inside it and a heating probe housing the thermocouple and heating element. The probes are 10 mm long and 2 mm in diameter. The heating probe is placed above the measuring position, and the reference probe is placed below the measuring position, with both probes placed parallel to the ground. The probes are inserted vertically at a height of approximately 1.3 m, with a spacing of 10 cm. The probes are installed on the north side of each sample tree at breast height (Jonard et al., 2011) because using the sap flow velocity on the north side of the tree generates a minimal error in estimating stand sap flow (Moon et al., 2017). Spherical foam was placed around the probes and then covered with aluminum foil to reduce the effects of solar radiation and prevent infiltration of rainwater. The sap flow velocity near the heating probes affects the measured temperature. On the basis of thermal diffusion theory of liquid rates, high sap flow velocity indicates that a large amount of heat is removed from the heating probe. A low heating probe temperature corresponds to a slight temperature difference between heating and reference probes. Therefore, the sap flow velocity can be calculated using the temperature difference between the upper and lower probes in the sample tree. A data collector (DT80, Data Taker Inc., China) was applied to determine the temperature difference between heating and reference probes every 10 s, and the average value was recorded every hour. The sap flow velocity (cm⋅h–1) was calculated from the differential temperature using the following empirical equation (Granier, 1987):
Where Ki is the parameter, ΔTM is the maximum temperature difference between the upper and lower probes in 24 h (°C), ΔTi is the instantaneous temperature difference value at a certain moment (°C), and Vi is the sap flow velocity (cm • h–1).
Environmental Factor Measurements
Meteorological data, including atmospheric temperature (Ta,°C), relative humidity (RH, %), solar radiation (Rs, W⋅m–2), precipitation (P, mm), and other meteorological variables, were simultaneously measured with the sap flow through a small weather station (WatchDog 2000, SPECTRUM Inc., United States) at the study site. Vapor pressure deficit (VPD, kPa) was calculated from the measured Ta and RH using the following the empirical equation (Zhao et al., 2017):
A soil moisture monitoring system (HOBO, LI-COR Inc., United States) consisting of soil moisture sensors and a data collector was also installed in the experimental area to monitor the volumetric water content. The sensors are inserted vertically in the soil layer at depths of 5, 20, 30, and 40 cm around the root system of each sample tree (approximately 20 cm near the main roots and as close as possible to the roots without harming the root system). The soil volumetric water content is automatically recorded and the average value is obtained every hour and then stored in the data collector of the soil moisture monitoring system. A PC can be connected to read the soil moisture data directly. The soil relative extractable water (REW) was used to classify the soil moisture status and calculated as follows (Granier et al., 2000b; Chen et al., 2014b):
Where VWC is the volumetric soil water content (cm3 cm–3), VWCFC is the soil water content at field capacity (VWCFC was used max VWC during the study period due to unavailability of VWC at field capacity), and VWCmin is the minimum soil water content during the study period. The dimensionless REW is the relative effective water content of the soil that demonstrates values from 0 to 1. Plants are usually considered under soil water stress when REW < 0.4 (Chen et al., 2020b).
Data Processing and Analysis
Although continuous monitoring was performed from April 2021 to September 2021, about 15% of data during the observation period were missing due to power outages and equipment failures. Microsoft Excel was used to convert the temperature difference data into sap flow data, and night was defined in hours when solar radiation was 0 W⋅m–2. The months of April, May, and June were designated as the early part of the F. pennsylvanica growing season and July, August, and September as the late part of the F. pennsylvanica growing season (Aiyun et al., 2019). Correlation data were processed with SPSS 22.0 using partial correlation and principal component analysis (PCA), and images were plotted using Origin 10.0.
Results
Variation in Daytime and Nighttime Sap Flow Velocity
The trend of sap flow velocity from April to September is shown in Figure 1. The daily variation of sap flow velocity in different months showed a single-peaked curve. The daytime sap flow velocity was significantly higher than the nighttime sap flow velocity. The sap flow velocity rises rapidly after starting early in the morning, reaches a peak, remains stable for a period of time, and finally decreases quickly until the evening. However, the magnitude of the sap flow velocity varies every month. The sap flow velocity in April and May is significantly lower and starts later than those in other months. The average daily sap flow velocity in April was the weakest during the study period at 1.609 cm⋅h–1 and the highest in July at 4.824 cm⋅h–1. Characteristics of nighttime sap flow velocity in different months (Figure 1) presented that the sap flow velocity is maintained at a flat and low level at night. The nighttime sap flow velocity is lower in April and May than that in other months. In addition, the sap flow is more active in the first half of the night than that in the second half of the night.
Influencing Factors of Sap Flow Velocity
Characteristics of Soil Water Content
The average daily soil water content of the soil layer with a depth of 40 cm was higher than that of 5, 20, and 30 cm. The moisture content of soil layers with a depth of 20 and 30 cm was similar (Figure 2). The dynamics of soil moisture all responded to rainfall events, especially continuous rainfall. For example, the average daily water content of soil layers with a depth of 5, 20, 30, and 40 cm increased from 0.154, 0.247, 0.248, and 0.314 cm3 cm–3 to 0.393, 0.456, 0.455, and 0.497 cm3 ⋅cm–3, respectively, for the largest continuous rainfall event in July (July 28–30) throughout the study period. The soil water content increased to some extent in all layers when continuous rainfall events occurred. Notably, the increase was high in the soil water content close to the surface layer. Soil moisture increased rapidly with each successive rainfall and decreased slowly during the subsequent consecutive rain-free days. However, differences were also observed in the magnitude of the decrease, with the surface soil moisture content decreasing rapidly. Large differences were also recorded in soil water content between months due to rainfall. Figure 2 and Supplementary Table 1 illustrated the satisfactory soil moisture conditions in July due to its many days of precipitation.
Characteristics of Microclimatic Factors and Response of Sap Flow Velocity to Meteorological Factors
The box plot in Figure 3 clearly presented that the distribution of meteorological factor values differs significantly. The overall RH was higher at night than that during the day, while the temperature and water vapor pressure deficits were lower at night than those during the day. Ta and RH were higher, but Rs was not significantly higher in the summer months of June, July, and August compared with those in other months. Such finding is due to the intense rainy weather in summer that results in low average solar radiation. The sap flow velocity was highly significantly and positively correlated with both Rs and Ta for all months during the daytime, and the highest correlation was found between Ta and sap flow velocity in July with a correlation of 0.591. The sap flow velocity was significantly and positively correlated with Ta and VPD for all months at night. At night, the strongest correlation between sap flow velocity and microclimatic factor occurred in August, with a correlation of 0.489 between sap flow velocity and VPD (Table 2).
Response of Sap Flow Velocity to Microclimatic Factors Under Different Soil Water Content
The mode of action of the combination of factors on sap flow velocity under different circumstances must be considered, given that environmental factors exist interdependently when influencing sap flow. Soil moisture conditions can determine the overall level of tree transpiration (Deng et al., 2021). Hence, the sap flow velocity under different soil water conditions was analyzed according to meteorological factors of the same period. Sap flow and meteorological factor data of several days before and after typical rainfall during the study period were selected separately for analysis while considering the high degree of influence of rainfall on soil moisture. Available data before and after typical continuous rainfall events with and without water stress conditions in June and August were selected for the corresponding analysis. The comparison of sap flow velocity data corresponding to consecutive rainfall events demonstrated that the mean nighttime sap flow velocity is higher during all periods of water stress than those without water stress (Table 3). Under the water stress condition, the nighttime sap flow velocities in the two events were 1.79 ± 0.69 cm⋅h–1 and 1.91 ± 0.28 cm⋅h–1. Under the no-water stress condition, the nighttime sap flow velocities in the two events were 1.49 ± 0.21 cm⋅h–1 and 1.62 ± 0.26 cm⋅h–1. The correlation between sap flow velocity and meteorological factors also varied under different moisture conditions (Table 4). The results of the partial correlation analysis, excluding the correlation between environmental factors, indicated that sap flow velocity under water stress is only correlated with Ta during nighttime and the correlation coefficient was 0.357 (Table 4). However, Pearson’s correlation analysis, which takes into account interactions of environmental factors (Supplementary Table 2), shows that the correlation between sap flow and environmental factors was excellent. For example, nighttime sap flow velocity was highly significantly negatively correlated with RH under water stress with a correlation coefficient of 0.738, and highly significantly positively correlated with Ta and VPD with correlation coefficients of 0.458 and 0.696. In addition, the correlation between sap flow and environmental factors was generally higher at night with water stress than that without water stress. The combined effect of environmental factors was generally higher without water stress than that with water stress during the day (Supplementary Table 2). Ta was highly significantly and positively correlated with sap flow velocity regardless of day and night or soil water conditions (Table 4).

Table 3. Relationship between daytime and nighttime sap flow velocity under different water conditions.

Table 4. Partial correlation between sap flow velocity and meteorological factors under different water conditions.
Relationship Between Daytime and Nighttime Sap Flow Velocity
The nighttime sap flow velocity was significantly and positively correlated with the daytime sap flow velocity (Table 5), and the fit was higher in the early growing season than that in the late growing season (early growing season: R2 = 0.51, P < 0.01; late growing season: R2 = 0.36, P < 0.01)(Figure 4). The fit between daytime and nighttime sap flow velocities was R2 = 0.52 for the entire study period. We can conclude that daytime and nighttime sap flow velocities of F. pennsylvanica are significantly and positively correlated. Table 5 indicates that a highly significant positive correlation exists between daytime and nighttime sap flow velocities with or without water stress, but the correlation is significantly higher without water stress. Therefore, an increase in daytime sap flow velocity will likely to produce a higher nighttime sap flow velocity under ideal moisture conditions. A highly significant positive correlation exists between daytime and nighttime sap flows for both sunny and cloudy conditions, but no correlation was observed for rainy day condition. Daytime and nighttime sap flow velocities on sunny days demonstrated the maximum correlation, with a fit of R2 = 0.797 (Table 5).
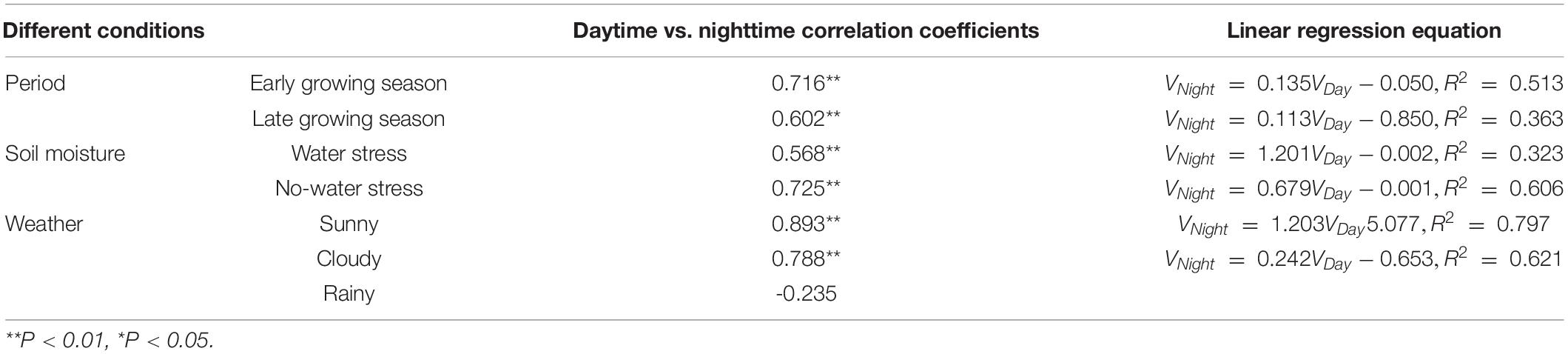
Table 5. Relationship between daytime and nighttime sap flow velocity under different soil moisture and weather conditions.
Comparing the overall environmental factors in the early and late growing seasons (Supplementary Table 3), it can be seen that rainfall is significantly higher in the late growing season than in the early growing season. RH and Ta are also higher in the later than in the early growing season. However, Rs and VPD are higher in the early growing season than in the late growing season. The sap flow velocity at each of the two periods was correlated with the corresponding simultaneous driving factors to investigate the relationship between daytime and nighttime sap flow velocities at different periods and driving factors further (Table 6). Ta was the factor with the maximum effect on sap flow velocity during both daytime and nighttime in the early growing season. Factors Rs and VPD presented the maximum influence on the respective daytime and nighttime sap flow velocities in the late growing season.

Table 6. Partial correlation coefficients between sap flow velocity and driving factors at different periods.
In addition, influencing factors interacted with one another with complex effects, and partial influencing factors are usually highly correlated with one another (Supplementary Tables 4–7). PCA is commonly used to detect and explain the underlying structure of various covariates. PCA reduces not only the dimensionality of the data set but also condenses the relevant data set into a few composite variables that retain the majority of information of original variables and thus appropriately reflect the combined information of influencing factors. Therefore, PCA was performed on the driving factors of sap flow, and the results are shown in Supplementary Table 8. Two principal components were extracted under each period of day and night in the principal component analysis. Among them, principal components of daytime and nighttime in the early growing season can explain 74.21 and 85.53% of the total data information, respectively. Meanwhile, principal components of daytime and nighttime in the late growing season can explain 78.75 and 90.80% of the total data information, respectively.
Expressions for the first (F1) and second (F2) principal components of Rs (X1), Ta (X2), RH (X3), VPD (X4), and VWC (X5) are listed in Supplementary Table 8. Regression equations were established with F1 and F2 as independent variables and standardized sap flow velocity data (F) as dependent variables for the corresponding periods. Expressions and equations for each period are presented in Supplementary Table 9. Regression models for driving factors and sap flow velocity were obtained by reverting the standardized variables to the original variables.
The early growing season model is expressed as follows:
The late growing season model is expressed as follows:
Discussion
Daytime and Nighttime Characteristics of Sap Flow Velocity and Influencing Factors
The sap flow velocity of F. pennsylvanica demonstrated an evident diurnal rhythm. The diurnal alternation of sap flow velocity exhibited a single peak with slight fluctuations. This finding is consistent with the results of Hua et al. (2021). The low and stable level of F. pennsylvanica sap flow velocity indicated that the plant root system still maintains a certain degree of water uptake capacity at night to meet the water balance and replenish the water loss caused by transpiration during the day (Han et al., 2019). Daytime sap flow velocity is significantly greater than the nighttime counterpart because of strong transpiration during the daytime. The xylem water column generates tension and a large amount of water enters the roots; the water enters the tree in a passive way. The sap flow at night was caused by root water potential, and water was actively absorbed into the tree to replace the water lost by daytime transpiration (Wu et al., 2020). In addition, the sap flow is more active in the first half of the night than that in the second half of the night. This phenomenon is mainly because the transpiration during the daytime causes a water potential difference between the root system and the soil of the tree after sunset. Thus, a high sap flow velocity occurs immediately after nightfall to replenish the water. The sap flow velocity decreases as the tree water deficit continues to decrease (Jie et al., 2019). Note that the sap flow velocity of F. pennsylvanica varied every month. The sap flow velocity was significantly higher in July, August, and September than that in April, May, and June during the study period. The sap flow velocity was highest in July but the lowest in April. Previous studies on the interannual variation of sap flow velocity also indicated that the maximum sap flow velocity occurs in July likely due the increased summer precipitation that provided satisfactory soil moisture conditions for plants (Iqbal et al., 2021). The nighttime sap flow velocity from June to September is likely due to high daytime water depletion that results in water deficit in trees. Hence, the nighttime sap flow velocity is kept high to maintain the water balance of trees taking up water at night to replace the water loss during the day (Han et al., 2019). VPD exerts a strong influence on the sap flow velocity in the Pearson’s correlation analysis (Supplementary Tables 2, 10, 11); however, the results of the partial correlation analysis after removing the correlation between environmental factor variables indicated that the degree of influence of VPD on sap flow decreases significantly (Tables 2, 4, 6). The positive effect of VPD on sap flow became negative after removing the correlation between environmental factors even during the daytime. This particular phenomenon was also seen in the study by Antezana-Vera and Marenco (2021). The effect of net VPD on the sap flow is negative during the daytime and positive during nighttime when the influence of other factors on VPD is removed. However, the effect of VPD on daytime sap flow became positive when effects of other environmental factors on VPD were considered. Thus, assuming that net VPD restrained daytime sap flow velocity and the influence of Ta, Rs, RH on VPD results in the positive effect of VPD on the sap flow while considering the influence of Ta and Rs and RH on VPD is reasonable because VPD is a function of Ta and RH and Ta is also influenced by Rs (Antezana-Vera and Marenco, 2020).
Response of Sap Flow Velocity to Microclimatic Factors Under Different Water Conditions
The main factors influencing the variation of sap flow velocity include physiological structure, soil water supply conditions, and meteorological factors. Among them, soil water affects the overall level of sap flow, and meteorological factors influence transient changes in sap flow (Granier et al., 2000a). Soil moisture content is a key factor affecting the overall level of sap flow velocity. Rainfall can provide temporal recharge of soil water that can lead to increased sap flow velocity through hydraulic conduction in the xylem (Shen et al., 2015). The degree of response of sap flow velocity to its associated factors varies under different water conditions. Previous studies on the relationship between sap flow and environmental factors under two different soil conditions indicated that diverse water supply conditions are dominant factors leading to various responses of sap flow to meteorological factors (Wu et al., 2018). In addition, the sap flow is susceptible to the influence of meteorological factors when the soil water content is sufficient (Chen et al., 2014a). Meteorological factors interact with one another, and changes in the sap flow are the result of a combination of meteorological factors. Therefore, the sensitivity of sap flow to the overall meteorological factors should be considered a composite result without excluding the correlation between factors. The results of Pearson as correlation analysis are listed in Supplementary Table 2. The sensitivity of sap flow velocity to meteorological factors was greater without water stress during the daytime but higher under water stress at night. Another study has also pointed out that moso bamboo produces considerable nighttime sap flow under drought conditions (Tong et al., 2021). The increasing nighttime sap flow can reduce the formation of xylem embolism and air pockets in plants and avoid hydraulic failure due to drought while alleviating their water deficit (Klein et al., 2018).
Relationship Between Daytime and Nighttime Sap Flow Velocity
Daytime sap flow velocity exerted a highly significant positive effect (P < 0.01) on nighttime sap flow velocity throughout the study period, and the correlation was strong in the early part of the growing season. Zhao et al. (2017) indicated that this phenomenon might be due to many rainfall events in the late growing season. The results of our study indicated that no significant correlation exists between daytime and nighttime sap flows on rainy days. The number of rainy days in the late growing season accounted for one-half of the total number of days (23 days in July, 10 days in August, and 13 days in September). Therefore, we assumed that the lower correlation between daytime and nighttime in the late growing season compared to the early growing season is caused by the high number of rainy days in the late growing season. In addition, a highly significant positive correlation is observed between daytime and nighttime sap flow velocities both with and without water stress periods, although the correlation was significantly higher in the absence of water stress. Therefore, an increase in daytime sap flow velocity will likely produce high nighttime sap flow velocity under ideal moisture conditions. Oogathoo et al. (2020) indicated that saturated VPD and Rs are the main drivers of transpiration. The results of our study indicated that daytime sap flow is correlated with Ta and Rs, and nighttime sap flow is correlated with Ta and VPD, except in the case of water stress. Therefore, the sap flow of F. pennsylvanica is mainly controlled by hydrothermal factors. The correlation between plant nighttime sap flow and VPD can be used to determine whether nighttime sap flow is used for transpiration (Dawson et al., 2007). The presence of transpiration by nighttime sap flow of F. pennsylvanica can be assumed because a highly significant correlation between nighttime sap flow velocity and VPD was revealed in this study. Hence, we can speculate that the highly significant positive correlation between nighttime and daytime sap flow velocities will likely lead to rehydration of F. pennsylvanica at night. The trees suffered from severe water deficit in the evening due to the strong transpiration and high sap flow during the day. High nighttime sap flow was generated to replenish tree water. These findings clearly demonstrated that the nighttime sap flow of F. pennsylvanica during the study period is a combination of nocturnal rehydration and transpiration.
Conclusion
During the study period, the sap flow velocity was highest in July and lowest in April. The daytime sap flow velocity was correlated with Ta and Rs in all months, and the nighttime sap flow velocity was correlated with Ta and VPD in all months in terms of influencing factors. Therefore, the sap flow of F. pennsylvanica is mainly controlled by hydrothermal factors. A particular finding of this paper is that the effect of net VPD on daytime sap flow is negative, but the effect of VPD on sap flow when combined with other environmental factors is positive. Therefore, when considering the role of VPD, it is important to analyze it in different situations. This result furthered our knowledge of the relationship between plants and environmental factors.
Higher nighttime sap flow velocity is produced under water stress conditions. Therefore, more emphasis should be placed on the nocturnal water consumption of plants in arid areas than in wet areas. Daytime and nighttime sap flow velocities of F. pennsylvanica were highly significantly and positively correlated. A highly significant positive correlation exists between daytime and nighttime sap flows for both sunny and cloudy conditions. Therefore, the nighttime sap flow can be well predicted by the daytime flow in the absence of rainy weather conditions. The principal components of driving factors were extracted using principal component regression analysis, and a regression model of driving factors and the sap flow velocity was constructed. It provides a theoretical basis for the prediction of sap flow velocity and the management of F. pennsylvanica.
Issues related to trunk sap flow still require further exploration. Monitoring may be ineffective at extremely low sap flow and reverse sap flow cannot be observed due to limitations of the TDP method. Therefore, the heat ratio method (HRM) is considered in our follow-up investigation because it can measure zero, low, and reverse flows of plants (Sun et al., 2022). Various forms of stomatal regulation at different times due to the circadian rhythm of plants affect transpiration (Cirelli et al., 2015). The regulation of stomatal conductance through the starch metabolism of the plant (Lascève et al., 2010) presents a corresponding effect on transpiration. The combination of environmental factors and physiological effects requires further investigation to determine whether environmental factors can affect plant physiological processes and their corresponding responses.
Data Availability Statement
The raw data supporting the conclusions of this article will be made available by the authors, without undue reservation.
Author Contributions
YS drafted, revised the manuscript, and contributed to data analysis. XW and YqS participated in the experiments and performed the maintenance of the instruments. HW designed, coordinated the study, and revised the manuscript. All authors contributed to the article and approved the submission.
Funding
This study was supported by the National Natural Science Foundation of China under the project “Reconstructing the response mechanism of runoff erosion on plant community structure in loose mounds” (41907047).
Conflict of Interest
The authors declare that the research was conducted in the absence of any commercial or financial relationships that could be construed as a potential conflict of interest.
Publisher’s Note
All claims expressed in this article are solely those of the authors and do not necessarily represent those of their affiliated organizations, or those of the publisher, the editors and the reviewers. Any product that may be evaluated in this article, or claim that may be made by its manufacturer, is not guaranteed or endorsed by the publisher.
Supplementary Material
The Supplementary Material for this article can be found online at: https://www.frontiersin.org/articles/10.3389/fpls.2022.884526/full#supplementary-material
References
Aiyun, S., Linshui, D., Jixiang, C., Ling, P., Jingtao, L., Jiangbao, X., et al. (2019). Comparation of seasonal dynamics of mineral elements contents in different organs of male and female plants of Fraxinus pennsylvanica. Sci. Silvae Sin. 55:9.
Antezana-Vera, S. A., and Marenco, R. A. (2020). Sap flow rates of Minquartia guianensis in central Amazonia during the prolonged dry season of 2015–2016. J. For. Res. 32, 2067–2076. doi: 10.1007/s11676-020-01193-9
Antezana-Vera, S. A., and Marenco, R. A. (2021). Transpiration of Swartzia tomentifera in response to microclimatic variability in the central Amazon: the net effect of vapor pressure déficit. Cerne 27:e–102999. doi: 10.1590/01047760202127012999
Chao, H. (2019). Characteristics of agrometeorological conditions in xiqing district of tianjin in recent 30 years. J. Agric. Catastrophol. 9, 23–24, 79. doi: 10.19383/j.cnki.nyzhyj.2019.06.010
Chen, D., Wang, Y., Liu, S., Wei, X., and Wang, X. (2014a). Response of relative sap flow to meteorological factors under different soil moisture conditions in rainfed jujube (Ziziphus jujuba Mill.) plantations in semiarid Northwest China. Agric. Water Manag. 136, 23–33. doi: 10.1016/j.agwat.2014.01.001
Chen, L., Zhang, Z., Zha, T., Mo, K., Zhang, Y., and Fang, X. (2014b). Soil water affects transpiration response to rainfall and vapor pressure deficit in poplar plantation. New For. 45, 235–250. doi: 10.1007/s11056-014-9405-0
Chen, Z., Zhang, Z., Chen, L., Cai, Y., Zhang, H., Lou, J., et al. (2020a). Sparse pinus tabuliformis stands have higher canopy transpiration than dense stands three decades after thinning. Forests 11:70. doi: 10.3390/f11010070
Chen, Z., Zhang, Z., Sun, G., Chen, L., Xu, H., and Chen, S. (2020b). Biophysical controls on nocturnal sap flow in plantation forests in a semiarid region of northern China. Agric. For. Meteorol. 284:107904. doi: 10.1016/j.agrformet.2020.107904
Cirelli, D., María, A., Equiza, V., and Tyree, M. T. (2015). Populusspecies from diverse habitats maintain high nighttime conductance under drought. Tree Physiol. 36, 229–242. doi: 10.1093/treephys/tpv092
Dawson, T. E., Burgess, S. S. O., Tu, K. P., Oliveira, R. S., Santiago, L. S., Fisher, J. B., et al. (2007). Nighttime transpiration in woody plants from contrasting ecosystems. Tree Physiol. 27, 561–575. doi: 10.1093/treephys/27.4.561
Deng, Y., Wu, S., Ke, J., and Zhu, A. (2021). Effects of meteorological factors and groundwater depths on plant sap flow velocities in karst critical zone. Sci. Total Environ. 781:146764. doi: 10.1016/j.scitotenv.2021.146764
Di, N., Xi, B., Clothier, B., Wang, Y., Li, G., and Jia, L. (2019). Diurnal and nocturnal transpiration behaviors and their responses to groundwater-table fluctuations and meteorological factors of Populus tomentosa in the North China Plain. For. Ecol. Manage. 448, 445–456. doi: 10.1016/j.foreco.2019.06.009
Fricke, W. (2019). Night-time transpiration – favouring growth? Trends Plant Sci. 24, 311–317. doi: 10.1016/j.tplants.2019.01.007
Fricke, W. (2020). Energy costs of salinity tolerance in crop plants: nighttime transpiration and growth. New Phytol. 225, 1152–1165. doi: 10.1111/nph.15773
Granier, A. (1987). Evaluation of transpiration in a Douglas-fir stand by means of sap flow measurements. Tree Physiol. 3, 309–320. doi: 10.1093/treephys/3.4.309
Granier, A., Biron, P., and Lemoine, D. (2000a). Water balance, transpiration and canopy conductance in two beech stands. Agric. For. Meteorol. 100, 291–308. doi: 10.1016/s0168-1923(99)00151-3
Granier, A., Loustau, D., and Breda, N. (2000b). A generic model of forest canopy conductance dependent on climate, soil water availability and leaf area index. Ann. For. Sci. 57, 755–765. doi: 10.1051/forest:2000158
Han, C., Chen, N., Zhang, C., Liu, Y., Khan, S., Lu, K., et al. (2019). Sap flow and responses to meteorological about the Larix principis-rupprechtii plantation in Gansu Xinlong mountain, northwestern China. For. Ecol. Manage. 451:117519. doi: 10.1016/j.foreco.2019.117519
Hua, L., Yu, F., Qiu, Q., He, Q., Su, Y., Liu, X., et al. (2021). Relationships between diurnal and seasonal variation of photosynthetic characteristics of Eucalyptus plantation and environmental factors under dry-season irrigation with fertilization. Agric. Water Manage. 248:106737. doi: 10.1016/j.agwat.2021.106737
Hubbart, J. A., Kavanagh, K. L., Robert, P., Tim, L., and Alisa, S. (2007). Cold air drainage and modeled nocturnal leaf water potential in complex forested terrain. Tree Physiol. 27, 631–639. doi: 10.1093/treephys/27.4.631
Iqbal, S., Zha, T. S., Jia, X., Hayat, M., Qian, D., Bourque, C. P. A., et al. (2021). Interannual variation in sap flow response in three xeric shrub species to periodic drought. Agric. For. Meteorol. 297:108276. doi: 10.1016/j.agrformet.2020.108276
Jie, Z., Yongmao, C., Zuosinan, C. L., and Zhiqiang, Z. (2019). Influencing factors and characteristics of nighttime sap flow of Acer truncatum in Beijing mountainous area. Acta Ecol. Sin. 39, 195–208.
Jonard, F., André, F., Ponette, Q., Vincke, C., and Jonard, M. (2011). Sap flux density and stomatal conductance of European beech and common oak trees in pure and mixed stands during the summer drought of 2003. J. Hydrol. 409, 371–381. doi: 10.1016/j.jhydrol.2011.08.032
Klein, T., Zeppel, M. J. B., Anderegg, W. R. L., Bloemen, J., De Kauwe, M. G., Hudson, P., et al. (2018). Xylem embolism refilling and resilience against drought-induced mortality in woody plants: processes and trade-offs. Ecol. Res. 33, 839–855. doi: 10.1007/s11284-018-1588-y
Kupper, P., Ivanova, H., Sõber, A., Rohula-Okunev, G., and Sellin, A. (2018). Night and daytime water relations in five fast-growing tree species: effects of environmental and endogenous variables. Ecohydrology 11:e1927. doi: 10.1002/eco.1927
Lascève, G. J., Leymarie, J., and Vavasseur, A. (2010). Alterations in light-induced stomatal opening in a starch-deficient mutant of Arabidopsis thaliana L. deficient in chloroplast phosphoglucomutase activity. Plant Cell Environ. 20, 350–358. doi: 10.1046/j.1365-3040.1997.d01-71.x
Liu, Z. Q., Liu, Q. Q., Wei, Z. J., Yu, X. X., Jia, G. D., and Jiang, J. (2021). Partitioning tree water usage into storage and transpiration in a mixed forest. For. Ecosyst. 8:72. doi: 10.1186/s40663-021-00353-5
Montoro, A., Torija, I., Maas, F., and López-Urrea, R. (2020). Lysimeter measurements of nocturnal and diurnal grapevine transpiration: effect of soil water content, and phenology. Agric. Water Manage. 229:105882. doi: 10.1016/j.agwat.2019.105882
Moon, M., Kim, T., Park, J., Cho, S., Ryu, D., and Kim, H. S. (2017). Variation in sap flux density and its effect on stand transpiration estimates of Korean pine stands. J. For. Res. 20, 85–93. doi: 10.1007/s10310-014-0463-0
O’Keefe, K., Bell, D. M., Mcculloh, K. A., and Nippert, J. B. (2020). Bridging the flux gap: sap flow measurements reveal species-specific patterns of water use in a tallgrass prairie. J. Geophys. Res. 125:e2019JG005446. doi: 10.1029/2019JG005446
Oogathoo, S., Houle, D., Duchesne, L., and Kneeshaw, D. (2020). Vapour pressure deficit and solar radiation are the major drivers of transpiration of balsam fir and black spruce tree species in humid boreal regions, even during a short-term drought. Agric. For. Meteorol. 291:108063. doi: 10.1016/j.agrformet.2020.108063
Paudel, I., Naor, A., Gal, Y., and Cohen, S. (2015). Simulating nectarine tree transpiration and dynamic water storage from responses of leaf conductance to light and sap flow to stem water potential and vapor pressure deficit. Tree Physiol. 35, 425–438. doi: 10.1093/treephys/tpu113
Roetzer, T., Moser-Reischl, A., Rahman, M. A., Hartmann, C., Paeth, H., Pauleit, S., et al. (2021). Urban tree growth and ecosystem services under extreme drought. Agric. For. Meteorol. 308:108532. doi: 10.1016/j.agrformet.2021.108532
Shen, Q., Gao, G., Fu, B., and Lü, Y. (2015). Sap flow and water use sources of shelter-belt trees in an arid inland river basin of Northwest China. Ecohydrology 8, 1446–1458. doi: 10.1002/eco.1593
Sun, X., Li, J., Cameron, D., and Moore, G. (2021). On the use of sap flow measurements to assess the water requirements of three Australian native tree species. Agronomy 12:52. doi: 10.3390/agronomy12010052
Sun, X., Li, J., Cameron, D., and Moore, G. (2022). Sap flow monitoring of two Australian native tree species in a suburban setting: implications for tree selection and management. Plant Sci. 317:111194. doi: 10.1016/j.plantsci.2022.111194
Tong, C. Z., Zhang, X., Xie, J. B., Mei, T. T., Fang, D. M., and Li, Y. (2021). Water use strategies of different aged moso bamboo culms under summer drought. For. Ecol. Manage. 498:119567. doi: 10.1016/j.foreco.2021.119567
Wu, J., Liu, H., Zhu, J., Gong, L., Xu, L., Jin, G., et al. (2020). Nocturnal sap flow is mainly caused by stem refilling rather than nocturnal transpiration for Acer truncatum in urban environment. Urban For. Urban Green. 56:126800. doi: 10.1016/j.ufug.2020.126800
Wu, Y., Zhang, Y., An, J., Liu, Q., and Lang, Y. (2018). Sap flow of black locust in response to environmental factors in two soils developed from different parent materials in the lithoid mountainous area of North China. Trees 32, 675–688. doi: 10.1007/s00468-018-1663-6
Yu, K., Goldsmith, G. R., Wang, Y., and Anderegg, W. R. L. (2019). Phylogenetic and biogeographic controls of plant nighttime stomatal conductance. New Phytol. 222, 1778–1788. doi: 10.1111/nph.15755
Zeppel, M. J., Lewis, J. D., Phillips, N. G., and Tissue, D. (2015). Consequences of nocturnal water loss : a synthesis of regulating factors and implications for capacitance, embolism and use in models. Tree Physiol. 34:1047. doi: 10.1093/treephys/tpu089
Keywords: sap flow, semiarid regions, Fraxinus pennsylvanica, driving factors, partial correlation
Citation: Su Y, Wang X, Sun Y and Wu H (2022) Sap Flow Velocity in Fraxinus pennsylvanica in Response to Water Stress and Microclimatic Variables. Front. Plant Sci. 13:884526. doi: 10.3389/fpls.2022.884526
Received: 26 February 2022; Accepted: 14 April 2022;
Published: 10 May 2022.
Edited by:
Sergio Rossi, Université du Québec à Chicoutimi, CanadaReviewed by:
Shalini Oogathoo, Université du Québec à Montréal, CanadaRicardo A. Marenco, National Institute of Amazonian Research (INPA), Brazil
Cheng Cheng Wang, Hainan Normal University, China
Jie Li, RMIT University, Australia
Copyright © 2022 Su, Wang, Sun and Wu. This is an open-access article distributed under the terms of the Creative Commons Attribution License (CC BY). The use, distribution or reproduction in other forums is permitted, provided the original author(s) and the copyright owner(s) are credited and that the original publication in this journal is cited, in accordance with accepted academic practice. No use, distribution or reproduction is permitted which does not comply with these terms.
*Correspondence: Hailong Wu, d3VoYWlsb25nQGVtYWlsLnRqdXQuZWR1LmNu