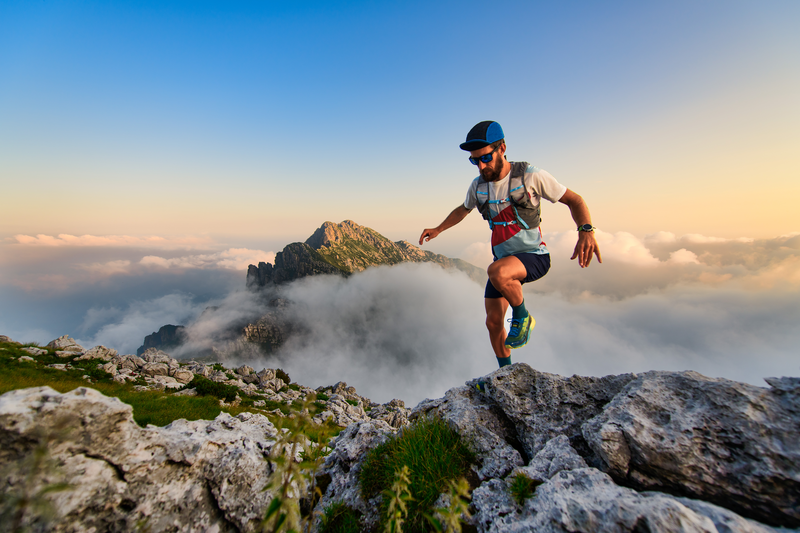
94% of researchers rate our articles as excellent or good
Learn more about the work of our research integrity team to safeguard the quality of each article we publish.
Find out more
ORIGINAL RESEARCH article
Front. Plant Sci. , 02 June 2022
Sec. Plant Pathogen Interactions
Volume 13 - 2022 | https://doi.org/10.3389/fpls.2022.882440
This article is part of the Research Topic Women in Plant Science - Focus On Plant-Microbe Interaction View all 8 articles
The objective of this study was to investigate the re-emergence of a previously important crop pathogen in Europe, Puccinia graminis f.sp. tritici, causing wheat stem rust. The pathogen has been insignificant in Europe for more than 60 years, but since 2016 it has caused epidemics on both durum wheat and bread wheat in local areas in southern Europe, and additional outbreaks in Central- and West Europe. The prevalence of three distinct genotypes/races in many areas, Clade III-B (TTRTF), Clade IV-B (TKTTF) and Clade IV-F (TKKTF), suggested clonal reproduction and evolution by mutation within these. None of these genetic groups and races, which likely originated from exotic incursions, were detected in Europe prior to 2016. A fourth genetic group, Clade VIII, detected in Germany (2013), was observed in several years in Central- and East Europe. Tests of representative European wheat varieties with prevalent races revealed high level of susceptibility. In contrast, high diversity with respect to virulence and Simple Sequence Repeat (SSR) markers were detected in local populations on cereals and grasses in proximity to Berberis species in Spain and Sweden, indicating that the alternate host may return as functional component of the epidemiology of wheat stem rust in Europe. A geographically distant population from Omsk and Novosibirsk in western Siberia (Russia) also revealed high genetic diversity, but clearly different from current European populations. The presence of Sr31-virulence in multiple and highly diverse races in local populations in Spain and Siberia stress that virulence may emerge independently when large geographical areas and time spans are considered and that Sr31-virulence is not unique to Ug99. All isolates of the Spanish populations, collected from wheat, rye and grass species, were succesfully recovered on wheat, which underline the plasticity of host barriers within P. graminis. The study demonstrated successful alignment of two genotyping approaches and race phenotyping methodologies employed by different laboratories, which also allowed us to line up with previous European and international studies of wheat stem rust. Our results suggest new initiatives within disease surveillance, epidemiological research and resistance breeding to meet current and future challenges by wheat stem rust in Europe and beyond.
Stem rust on wheat, caused by the basidiomycete Puccinia graminis f.sp. tritici (Pgt) Eriks. & E. Henn., has historically been considered a main threat for wheat production globally due to the risks of high losses in grain yield and quality (Singh et al., 2015). The disease, which is also known as black rust, became less prevalent in Europe during the second half of the twentieth century, due to a combination of efforts to eradicate common barberry (Berberis vulgaris), the alternate host for multiple formae speciales of Pg (Lind, 1915; Stakman, 1923; Hermansen, 1968), as well as advances in plant breeding and plant disease management. Nevertheless, stem rust persisted as a potential threat mainly in warmer wheat growing areas in Central and Eastern Europe (Bartoš et al., 1996). In Africa, the situation has changed since 1999 by the emergence of a new race group, termed ‘Ug99,’ which carries virulence to stem rust resistance gene Sr31, thereby leaving many wheat cultivars susceptible to stem rust (Pretorius et al., 2000; Singh et al., 2011). Since then, stem rust of different genetic groups and races have emerged in East Africa, for example race TKTTF, which resulted in epidemics in Ethiopia in 2013 with yield losses close to 100% in the most grown wheat cultivar., ‘Digalu’ (Olivera et al., 2015).
In 2013, stem rust reappeared on several wheat cultivars at several locations in Germany (Olivera Firpo et al., 2017) along with sporadic incidences in southern Denmark, eastern Sweden and the United Kingdom (Lewis et al., 2018). A more widespread outbreak was reported from Sicily in Italy in 2016, where thousands of hectares of both bread and durum wheat were affected (Bhattacharya, 2017). Preliminary analyses of infected plant samples from Sicily suggested the presence of a new race in Europe, TTRTF (termed TTTTF in Bhattacharya (2017)), which carried virulence to Sr13b of particular relevance for durum wheat (Patpour et al., 2020). These events were followed by the detection of stem rust on late maturing wheat and barley in local areas in Sweden in 2017, characterized by cool temperatures and an unusual wet growing season. Since then, stem rust has been observed every year on wheat, barley and rye in these areas (Kjellström, 2021). Earlier studies in Sweden have shown that stem rust on oat and rye reproduce asexually on the cereal host and sexually on the alternate host, B. vulgaris (Berlin et al., 2012).
A new initiative to establish a European early-warning system for wheat rust diseases, RustWatch, was launched in May 2018, including the coordination of national wheat rust surveillance and rust resistance breeding efforts.1 This initiative builds on the achievements of the Global Rust Reference Center (GRRC) at Aarhus University, Denmark, and the Borlaug Global Rust Initiative, which was launched in 2008 in response to the emergence of the stem rust race Ug99 and multiple variants (McIntosh and Pretorius, 2011). The role of the alternate host in the epidemiology of Pgt in Europe at present time was an important part of the RustWatch initiative, following the increase in prevalence in at least Sweden and the United Kingdom, after the lapse in legislation about planting and eradication of common barberry in the 1990s (Berlin et al., 2012; Saunders et al., 2019).
In this study, we investigated whether stem rust outbreaks in Europe since 2016 could be connected to a local outbreak in Germany in 2013, and to which extent the populations were influenced by exotic incursions from outside Europe as opposed to evolution by mutation and/or sexual recombination in local populations in proximity to Berberis spp. These hypotheses were explored by extensive sampling of stem rust from new outbreaks in agricultural areas in Europe as well as in local areas in Sweden and Spain, where the alternate host has been documented, and from a distant wheat growing area in western Siberia, Russia, where more than 1 million hectares of wheat were affected by stem rust epidemics in 2015 with yield losses of up to 20–30% (Shamanin et al., 2016). All samples were genotyped to investigate genetic variability and relatedness, and subsets were race typed to investigate phenotypic diversity for virulence and epidemic potential on European wheat varieties.
A total of 479 samples of stem rust from mainly wheat, occasionally other cereals, and grasses, were collected from 17 European countries and western Siberia, Russia (Table 1). The number of samples varied across years and locations, largely reflecting sporadic and variable occurrence and sampling efforts of wheat stem rust.
Twenty-five reference isolates of diverse geographical origin, including isolates representing the German outbreak in 2013, and DNA samples hosted by the USDA ARS Cereal Disease Lab, Minnesota, United States, were included to ensure alignment with previous studies and genotyping methodologies (Olivera Firpo et al., 2017; Lewis et al., 2018; Szabo et al., 2022). Live isolates were exchanged under strict quarantine conditions, and DNA samples representing all previously defined clades/genetic groups in Pgt were tested in duplicate using SNP and SSR genotyping approaches (Table 2). Mailing and shipment of samples from the field followed the protocol by (Olivera et al., 2015). Most of the samples from western Siberia were collected during a surveillance trip in 2016 and additional samples from the same areas were submitted to GRRC in 2017. Samples from Sweden were part of a comprehensive stem rust survey in 2018–2019, following the first detection of a sexual Pgt population on barley and wheat in proximity to common barberry in the autumn of 2017. Samples from Spain were collected from cereal and grass hosts in areas in close proximity to indigenous subspecies of the alternate host, B. vulgaris, and in contrasting areas where the alternate host was not present. Samples from France, 2021, were part of a national stem rust survey initiated as an early reaction to the widespread appearance of the disease in June–July 2021.
Table 2. Reference isolates of diverse origin, including isolates from the outbreak in Germany in 2013 to facilitate alignment of race, SSR genotype and SNP genotype in Puccinia graminis f.sp. tritici.
Recovery was facilitated by placing stem and/or leaves on moist filter papers in petri dishes under humid conditions at 18°C for 1–2 days to promote the formation of urediniospores. Thereafter, the infected tissue of each sample was gently rubbed on the adaxial side of seedling leaves of the universally stem rust susceptible wheat lines Morocco, Line E and McNair 701. The seedlings were grown in pots, 12–15 plants per pot and treated with 5 ml of 0.5% Antergon MH180 (Nordisk Alkali, Randers, Denmark) to regulate plant growth and enhance spore production. The inoculated seedlings were misted with water and incubated in a dew chamber at 18°C in darkness for 24 h under high relative humidity (RH), after which they were transferred to spore-proof greenhouse cabins set to 20°C during day/18°C during night with a 16 h photoperiod of natural light and supplemental sodium light (200 μmol s−1 m−2) and 8 h darkness. Plants were watered automatically with added micronutrients for 10 min every 12 h. The pots were covered with cellophane bags (Helmut Schmidt Verpackungsfolien GmbH, Königswinter, Germany) before onset of sporulation to avoid cross contamination between samples. Urediniospores from each sample were harvested, dried, and stored in a liquid nitrogen cryo-container (−196°C), or freezer (−80°C), until further use.
Genomic DNA was extracted from leaf segments representing 459 samples, typically 1–2 cm, containing single pustules of Pgt taken from multiplication pots or directly from infected stem segments of incoming samples. The specimens were dried and stored in a desiccator for 24–48 h. Prior to DNA extraction, each specimen was ground with one steel ball (ø 4 mm) and equal amounts of acid washed sand in a 2 ml Eppendorf tube at 1500× strikes for 180 s using a Geno/Grinder 2010 (SPEX SamplePrep, United States). The powdered material was suspended in 300 μl lysis buffer PN and 1 μl RNaseA (100 mg/ml) from the Sbeadex® Mini Plant Kit (LGC Genomics, Germany). The lysate was incubated at 65°C for 30 min followed by centrifugation at 10,000 rpm for 10 min. 50 μl of the supernatant was used for DNA extraction, automated with a KingFisher™ Magnetic Particle Processor (Thermo Fisher Scientific, United States) according to manufacturer instructions for the Sbeadex® Mini Plant Kit (LGC Genomics, Germany). DNA was eluted in 50 μl of elution buffer provided by the manufacturer.
Seventeen Simple Sequence Repeat (SSR) primer pairs previously applied by Stoxen (2012), Jin et al. (2009), and Zhong et al. (2009), were used for molecular genotyping (Supplementary Table S1). Forward primers were fluorescently 5′-labeled and synthesized by Thermo Fisher Scientific. Reverse primers were synthesized by Eurofins Genomics. SSR loci were amplified in two multiplex PCR reactions using the Type-it microsatellite PCR kit (QIAGEN) in a total volume of 11 μl containing 1.10 μl primer mix (2.0 μm of each primer), 1.10 μl Q-solution, 5.50 μl Type-it Multiplex PCR Master Mix, 2.30 μl Nuclease-Free H2O and 1 μl of undiluted DNA. The PCR was run at 95°C for 5 min followed by 12 cycles at 95°C for 30 s, 63°C (−0.5°C per cycle) for 90 s and 72°C for 30 s, followed by 23 cycles at 95°C for 30 s, 57°C for 90 s and 72°C for 30 s and a final extension step at 72°C for 10 min. The PCR program was run on Applied Biosystems™2,720 Thermal Cycler (Thermo Fisher Scientific). PCR reactions were diluted 1:200 in Nuclease-Free H2O before size fractionation on Applied Biosystems™ 3,730 DNA analyzer (Thermo Fisher Scientific) using the service at KIGene, Karolinska University Hospital, Stockholm, Sweden. The amplicons were visualized in GeneMarker® (Softgenetics), and allele sizes (Supplementary Tables S1, S2) were manually scored using the GeneScan™ 600 Liz ® Size Standard (Thermo Fisher Scientific).
A subset of live bulk samples were selected according to year, country, location, host origin and SSR genotype, which resulted in 256 representative isolates that were successfully race phenotyped using a core set of 20 North American stem rust differential lines according to Jin et al. (2008). Four seedlings of each differential line were sown in pots with a 1:1 Pindstrup Substrate peat mix containing slow-release plant nutrients (Pindstrup Mosebrug A/S, Ryomgaard Denmark). The plants were grown in spore-proof cabins in the greenhouse at 17°C during day/12°C during night until full development of first leaf and second leaf half unfolded (approx. 11 days after sowing). Spore samples from cold storage were heat-shocked at 43°C for 5 min. Inoculation of the seedlings was done by spraying 4–5 mg of suspended urediniospores in 5 ml engineered fluid 3 M™ Novec™ 7,100, (3 M, St. Paul, MN, United States) using an airbrush spray gun (standard class, Revell GmbH, Bünde, Germany) in a biosafety cabinet, followed by mist water to ensure dew formation. Seedlings were incubated in a dew chamber at 18°C in darkness for 24 h, then transferred to spore-proof cabins in the quarantine greenhouse with a 16 h photoperiod of natural light and supplemental sodium light (200 μmol s−1 m−2), temperature of 20–22°C day/18–20°C night and RH of 80–90%.
Seedling infection types (IT) were scored on the first and second leaf 17 days post-inoculation using a 0–4 scale (Stakman et al., 1962; McIntosh et al., 1995). Isolates conferring ‘low’ ITs, i.e., 0, 0; 1, 1+, 2, and 2+, or combinations thereof, were considered ‘avirulent’ (incompatible), whereas ITs of 3-, 3, 3+, and 4 were considered ‘high’ (i.e., compatible, ‘virulent’; Supplementary Table S3). In case of distinct different ITs on individual differential lines, which could indicate the presence of more than a single race, one to three single pustules were re-isolated. This procedure was repeated until no further signs of mixed ITs were observed in subsequent race assays. Subsets of isolates representing prevalent clades and races, and four unique races from Spain were tested on 35 additional lines representing diverse sources of stem rust resistance (Supplementary Table S4). The seeds were supplied by Cereal Disease Laboratory, USDA, and Agriculture and Agri-Food Canada (AAFC) and multiplied at GRRC; sporadic morphological off-type plants were discarded prior to subsequent seed multiplication. The race typing was generally repeated two to three times, and race nomenclature was based on a modified letter code (Roelfs and Martens, 1984) proposed by Jin et al. (2008). Morocco and McNair were used as susceptible controls in all experiments.
A set of 53–55 European wheat varieties from 18 breeding companies in 12 countries were evaluated for reaction to seven Pgt races representing different genetic groups: Clade III-B (TTRTF, IT76c-18), Clade IV-B (TKTTF, ES34-20), Clade IV-F (TKKTF, DK221a-19), Clade I (TTKSK, KE305c-17 and TTKTT, KE181a-18), Clade VIII (RFCNC, CZ59-20), and an unnamed clade (SKGBR, ES178b2–19). Six seedlings per accession were inoculated under the experimental conditions and procedures described above for race identification. Infection types were scored on the first and second leaf 17 days post-inoculation using the 0–4 scale, where host lines with IT responses below 2+ were considered ‘resistant,’ ITs 2+ and 3- were considered ‘intermediate’ and lines with ITs of 3 and above were considered ‘susceptible.’ Wheat cultivars Morocco and McNair were used as susceptible control (Supplementary Table S5).
Duplicate single pustule isolates derived from a bulk sample were removed from the dataset, except when the results revealed multiple genotypes or races within the original sample. Samples that failed recovery were genotyped in case of none or very few samples were recovered from a certain location and year.
Simple Sequence Repeat marker results were investigated through a series of population genetic analyses. The reliability of SSR markers describing the population structure was assessed through the detection of multilocus genotypes (MLGs) plotted against the number of loci using the “poppr” package version 2.9.3 implemented in the R environment (Kamvar et al., 2014, 2015). Expected and observed heterozygosity, standard error (SE) of these and inbreeding coefficients (FIS = (Mean He - Mean Ho) /Mean He) were calculated in GenAlEx 6.5 (Peakall and Smouse, 2012). The genetic diversity within genetic groups as well as within populations was assessed by the number of MLGs detected within each group using the “poppr” package version 2.9.3 implemented in the R environment (Kamvar et al., 2014, 2015). The Shannon–Wiener index (H) was used to measure genotypic diversity (Shannon, 2001). The standardized index of association (rbarD) among SSR loci with 1,000 permutations was calculated to test for association among SSR loci and infer the mode of reproduction of the different populations (Agapow and Burt, 2001). To avoid the effect of epidemic clonality, calculations were repeated using clone-corrected datasets, in which only one representative of each repeated MLG was considered.
The population structure of isolates of different geographical origin was investigated by Discriminant Analysis of Principal Components (DAPC), a multivariate method implemented in the “adegenet” R package version 2.1.5 (Jombart, 2008; Jombart et al., 2010). The find.clusters function was used to determine the de novo optimal number of clusters (K), which is normally associated with the lowest value in the ‘elbow’ of the Bayesian Information Criterion (BIC) curve. A cross-validation function (xvalDapc) with 1,000 replicates, associated with the lowest root mean square error, was used to define the optimal number of principal components (PCs) to be retained in the DAPC analysis. Membership probabilities of individual isolates associated with a specific MLG and genetic cluster were calculated using the “adegenet” R package. The relationship of isolates of different MLGs, including reference isolates representing previously defined genetic groups (Table 2), was calculated using Bruvo distance and visualized in an unrooted Neighbor Joining tree using 2000 bootstraps in the “poppr” R package using a cut-off value of 75%.
The spatial and temporal distribution of samples of wheat stem rust in this study reflected the large differences in disease prevalence across countries and years, but with a clear trend of increasing incidence of stem rust on wheat in Europe since 2016 (Figure 1; Table 1). Relatively high sampling intensity was made during epidemic situations in southern Italy and western Siberia (Russia), and from non-epidemic situations in most other areas with few and sporadic local outbreaks. Samples from Spain represented two contrasting ecological zones, i.e., areas with nearby plants of alternate hosts of Berberis spp., and other areas distant from such plants. In this study, stem rust was not detected in France until 2019 (one sample from a single location), which increased to 40 samples from 28 locations in 2021. Eleven isolates from the outbreak in Germany (2013), Denmark (2013) and Sweden (2014) and six isolates from a local study in Sweden by Berlin et al. (unpublished data) were included as references to earlier and ongoing national studies.
Figure 1. Sampling sites in Europe 2016–2021. Each dot represents a sampling site where one or more samples were collected for genotype/race analyses. Additional sampling sites near Omsk and Novosibirsk in Western Siberia not shown.
More than 80% of the samples considered were successfully recovered (cf. Table 1), which provided a unique opportunity to connect information about genetic variability in SSR genotype and virulence phenotype. A total of 540 isolates (2016–2021) were successfully genotyped, of which 81 represented duplicate isolates of identical genotypes/races derived from individual bulk samples. Additionally, 46 samples showed signs of more than a single genotype per sample, mainly from areas with high pathogen diversity. None of the 81 duplicate isolates were considered further, leaving a total of 459 isolates for onwards analyses. Three genotypes detected in the outbreaks of stem rust in Europe 2013–2014 were resampled, i.e., Clade IV-E.1, Clade IV-E.2 and Clade VIII.
The majority of the isolates were assigned to four distinct and previously defined clades, which were often present in multiple countries (Figure 2). Isolates of Clade III-B were the most common, containing one prevalent and four additional MLGs, but only a single race known as the ‘Sicily race,’ TTRTF (Table 3). In this study, TTRTF was first detected in Sicily (2016), but in 2017 and later years, the race was also detected in mainland Italy and Sardinia, Austria, Croatia, Czech Republic, Slovak Republic, Slovenia, and Spain. Race TTRTF has virulence to Sr13b, of particular relevance for durum wheat, Sr35, Sr37 and Sr50 (Supplementary Table S4). Clade IV-F, consisting of a single MLG and race (TKKTF), was first detected in 2018 in this study (Italy, Poland and Sweden). Clade IV-F has spread to a total of 13 European countries by 2021, but so far not associated with epidemics outside Italy. Clade IV-B containing race TKTTF was first detected in 2017 in Europe (Croatia), and in subsequent years in seven additional countries, including Ireland (2020), where stem rust is rarely observed. Clade I (Ug99 race group) was not detected in this study. The results confirm the spreading of relatively few clones across multiple countries in northern and western Europe in recent years, giving rise to sporadic incidences of stem rust on wheat in some countries, and more systematic and widespread occurrence in countries like Italy and France (2021).
Figure 2. Frequency distribution of genetic groups among 459 isolates of Puccinia graminis f. sp. tritici in Europe between 2016 and 2021. The designation of distinct groups follows the nomenclature suggested by Szabo et al. (2022). ‘Others’ refer to genetic groups, which have not yet been named internationally. Downloaded 3 February 2022.
Table 3. Races detected in Europe within previously defined genetic groups (clades) and number of MLGs in these.
Multiple genetic groups, which have not been named previously, were termed ‘Others’ (Figure 2; Table 3). These groups were prevalent in local areas in Sweden, Spain, and Russia, often sampled from cereals in proximity to the alternate host, Berberis spp. Many of the races were characterized by avirulence on multiple wheat differential lines (Supplementary Tables S3, S4) and some of the isolates revealed unexpected, intermediate infection types. In summary, the results suggested the presence of local highly diverse Pgt populations in Russia, Spain and Sweden consisting of multiple races, and in most other areas, clonal populations consisting of only few genetic groups and races.
The genetic structure of Pgt was investigated for seven geographically and ecologically different sampling areas (populations), i.e., Italy, Spain (Barberry area), Spain (non-Barberry area), Sweden, Russia (Siberia), France and Europe (other). The latter represents 13 countries (cf. Table 1), where wheat stem rust was observed only sporadically, resulting in relatively few samples compared to a large geographical sampling area (Table 4).
Table 4. Genetic diversity of geographical spaced populations of Puccinia graminis f.sp. tritici in Europe and Russia.
The 17 SSR primers revealed a total of 104 multilocus genotypes (MLGs) based on a total of 179 different alleles (Table 4; Supplementary Figure S1). The seven populations revealed very different degree of genetic diversity. The populations in Italy, Spain (non-barberry area) and France had low number of genotypes compared to sampling size, low Shannon–Weiner diversity index, high level of linkage disequilibrium (Table 4) and excess of observed heterozygosity as compared to the expected under Hardy–Weinberg (HW) equilibrium (Figure 3). These features are strong signatures of clonal reproduction. In contrast, the populations from Spain (barberry area), Sweden and Russia showed strong signatures of sexual reproduction, revealed by high numbers of genotypes compared to sampling size, high diversity indexes and linkage disequilibria close to zero in Russia and slightly higher in Spain (barberry area) and Sweden. Observed/expected heterozygosity were in HW proportions for Russia or lower than expected for Spain (barberry) and Sweden, which may indicate some degree of inbreeding in the last two mentioned. This is in line with FIS values of 0.14 and 0.25, respectively, for these two populations as opposed to 0.019 for Russia and negative values for the clonal populations in Italy, France and Spain (non-barberry; Figure 3). Samples from other European countries, representing relatively few samples collected across several years, had parameter values between these extremes, which suggests that regions in Europe, where stem rust is not yet established, may be influenced by incursions of individuals of diverse evolutionary origin.
Figure 3. Observed heterozygosity (Ho), expected heterozygosity (He) and inbreeding coefficients (Fis) of geographical spaced populations of Puccinia graminis f.sp. tritici in Europe and Siberia. Samples from outside these areas were not considered due to low sample sizes in individual countries. Level of significance: p < 0.01**.
The DAPC analysis revealed five genetic clusters based on 20 retained PCs and six discriminant (DA) eigenvalues, which were sufficient to capture the genetic structure of the whole dataset (Figure 4; Supplementary Figure S2). This corresponded with the optimal value of BIC indicated by the lowest value of the elbow observed in the BIC curve (Supplementary Figure S3). The 104 MLGs detected among the 459 isolates included in the study were clustered in the DAPC analyses based on membership probabilities larger than 0.9999 (Supplementary Table S6). Cluster 1 consisted of previously defined clades, such as Clade IV-A.1, Clade IV-B, Clade IV-F, Clade IV-E.1, and Clade IV-E.2. Cluster 4 consisted of five closely related MLGs in Clade III-B. Both clusters (CL1 and CL4), consisting of previously defined groups, were clearly separated from the three others. Cluster 5 consisted of a diverse population from Russia (western Siberia) and Clade VIII consisting of only two MLGs mainly detected in eastern Europe. Cluster 2 consisted of MLGs representing isolates from Sweden, a single MLG detected in Norway, a different MLG detected in Czech Republic and a re-sampled MLG detected in Denmark. Finally, Cluster 3 consisted of 28 MLGs from Spain and 8 MLGs from Sweden.
Figure 4. Discriminant analysis of principal components (DAPC) for the 104 multilocus genotypes (MLGs) detected (clone corrected data). The axes represent the first two linear discriminants. Each ellipsis represents distinct genetic clusters, and dots represent individuals associated with unique MLGs. Eigenvalues indicate the amount of genetic information retained by the PCA (left inset) and the discriminant function (DA, right inset).
The overall conclusions from the DAPC analyses were confirmed by phylogenetic analyses (Figure 5), which also comprised reference isolates representing genetic groups reported in previous European and international studies (cf. Table 2). One branch consisted of already defined clades, most of which have been previously detected in Europe, i.e., clade IV-A.1, IV-A.2, IV-E.1, IV-E.2, and IV-F (equivalent to CL1, Figure 4). Clade III-B formed a unique group of five closely related MLGs, equivalent to CL4 in Figure 4. A second branch consisted of 38 diverse MLGs from two local areas near Omsk and Novosibirsk in western Siberia, Russia, which corresponded with CL5 in Figure 4. A Clade III-A reference isolate and an isolate from the German outbreak in 2013 (unpublished) were part of CL5. Whereas the two MLGs in the newly defined Clade VIII grouped with the Russian isolates in the DAPC analysis, this was not the case in the NJ tree, where they were located within a third branch consisting of genetically diverse isolates from Scandinavia and Czech Republic (CL2 in Figure 4). A fourth branch consisted of 36 MLGs representing isolates from Spain and Sweden, corresponding to CL3 (Figure 4).
Figure 5. Unrooted Neighbor Joining tree (clone-corrected data) using Bruvo’s distance showing the genetic relationship of European stem rust isolates (2016–2021) and additional reference isolates representing previously defined genetic groups (Clades) detected in Europe and elsewhere. National letter codes are indicative: ES (Spain), SE (Sweden), RU (Russia). Red dots represent isolates used for assessment of host susceptibility.
In conclusion, the local sexual populations in Spain (barberry area), Sweden and Russia, respectively, were highly diverse and formed distinct genetic groups. These were clearly separated from previously named and prevalent clades, each consisting of one or few races and/or MLGs. One MLG from Clade VIII, which was already detected in the German outbreak in 2013, was resampled in Czech Republic, Slovakia and Hungary in the present study, and in another case, an MLG first detected in a sexual population in Sweden (2019) was detected in Denmark (2020). Other reference isolates such as Clade I (Ug99), Clade II, Clade III-A, Clade IV-C and Clade IV-D, were not detected in this study.
In addition to typical races found in Europe, samples from barberry areas in Spain, Sweden, and Russia revealed a high number of unique races in low frequencies and unusual virulence combinations, and occasional unexpected infection types (IT) on specific combinations of differential lines (Supplementary Tables S3, S4).
In Spain, 54 of 58 genotyped isolates were successfully virulence phenotyped based on the IT responses on 20 wheat lines collectively assembled to differentiate races in Pgt (Table 5). The results were highly dependent on sampling area, i.e., high race diversity in areas in proximity of alternate hosts of Berberis spp. (28 races among 30 isolates), and low diversity in areas where the alternate host was not detected near sampling sites (4 races among 24 isolates). In general, different races were represented by different genotypes (MLGs). Only in four cases, different races shared the same genotype, i.e., races within MLG ID number 6, 11, 23 and 89, respectively (Table 5).
Table 5. Virulence diversity of 31 races of Puccinia graminis f.sp. tritici in areas in Spain in proximity of Berberis species and in areas where the alternate host was absent.
The presence of Sr31-virulence in 22 races was striking, irrespective of host origin, representing wheat, rye, and a wild grass (Elymus repens). A subset of four of these isolates were tested on 35 additional wheat lines representing diverse and occasionally newly identified sources of resistance (Supplementary Table S4). These results confirmed highly unusual and different virulence patterns, including confirmation of virulence to Sr31 using three wheat lines, Federation*4/Kavkaz, Sr31/6*LMPG and Siouxland, as well as virulence to Sr22, Sr24, Sr44, Sr45, Sr49, and Sr50. In addition, we observed higher than expected ITs for three incompatible interactions involving Sr31 (2, 2+ and 22+), Sr24 (22+), and Sr50 (2-). The fact that Sr31-virulent races were observed for samples collected from three host genera (Triticum, Secale and Elymus), and the lack of differentiation according to host origin with respect to virulence/race pattern in general, demonstrated that wheat adapted stem rust from local areas in Spain were able to infect and reproduce on rye and wild grasses.
In contrast, the samples from agricultural areas separated from alternate hosts, Berberis spp., revealed only four races; TKTTF (from bread wheat, durum wheat and spelt), TKRTF (bread wheat), and TKKTF and TTRTF, the latter two collected from both bread wheat and durum wheat. The four races were assigned to three clades that were also detected at multiple locations outside Spain (Figure 2).
Twenty-nine live isolates from Sweden revealed 13 race phenotypes, representing five reference samples from a local study in Sweden (2017), two collections from barley in 2018 and one collection from wheat in 2019 and 2020 (Table 6). A single race/genotype (QCHNC; MLG40) was resampled twice from a single wheat plot in Denmark (2020).
TKKTF was detected in two different clades, IV-E.1, and IV-F, but also represented in a reference isolate collected in Sweden in 2014 (Clade IV-E.2). Except for TKKTF and TTQKF, the races in Sweden were characterized by a relatively high number of avirulences. None of the races, except TKKTF and QCHNC mentioned above, were detected outside Sweden. In contrast to Spain, Sr24-, Sr31-, and Sr50-virulences were not detected. The unusual virulence patterns and complexity of races confirmed the high degree of genotypic diversity and separation from other populations in the study.
Fifty-three live isolates from western Siberia were race phenotyped, representing the majority of samples from two neighboring regions in 2016 and 2017 that were successfully genotyped (Table 7). A total of 35 races were detected, of which only five were found more than once. All races were different from races detected elsewhere in the study, which was confirmed by the fact that none of previously defined clades in Europe, Africa, Asia and North America were detected (Table 7). In general, different races were represented by different genotypes, except MLG 100, which was represented by four races (LCCSF, LCRSF, QCHSF, and QCRSF). Interestingly, a single race has Sr31-virulence (race profile no. 8), but is indeed very different from known races of Ug99 and Sr31-virulent races from Spain (Table 5, this study). The Sr31-virulence was confirmed by repeated tests using Sr31 resistance in three genetic backgrounds. Overall, the races had different virulence/avirulence pattern ranging from individuals with relatively few virulences, for example race profile 1 (virulences: 5, 9 g, 17, 9a, 9d, 10, 38) to individuals with high number of virulences such as race profile 35 (virulences: 5, 21, 9e, 7b, 6, 8a, 9 g, 36, 9b, 17, 9a, 10, 38, Tmp).
Seedling tests using seven isolates representing diverging genetic groups (Figure 5) demonstrated that most of the investigated wheat varieties were susceptible to most of the races (Table 8; Supplementary Table S5). The two Ug99 races revealed susceptibility to the highest number of varieties, whereas the race SKGBR from a recombining population in Spain was less adapted to the varieties investigated. Two durum wheat varieties (Svevo and Marco Aurelio) showed resistance to all races except the Sicily race (TTRTF). Information about the detailed responses of individual varieties to individual races are provided in Supplementary Table S5.
Table 8. Seedling response of European wheat varieties to three clades representing prevalent races of P. graminis f.sp. tritici in Europe, a race from a recombining population in Spain, a race from the German outbreak 2013, and two races representing Ug99 from East Africa.
The present study represents a comprehensive dataset based on SSR genotyping and race phenotyping of more than 400 samples of Puccinia graminis f. sp. tritici, which were collected in 17 European countries and Siberia between 2016 and 2021. By comparing to results of isolates of non-European origin, hosted by the GRRC,2 and reference isolates from the USDA ARS Cereal Disease Lab, we were able to address hypotheses about possible origin and spatial patterns of the re-emergence of wheat stem rust in Europe since the outbreak in Sicily in 2016 (Bhattacharya, 2017). Sampling in local areas in proximity to the alternate host, Berberis spp., and in corresponding non-Berberis areas, allowed us to investigate the current role of Berberis spp. in wheat stem rust epidemiology in Europe. This is a question, which has occupied scientists and plant breeders historically Lind (1915); Stakman (1923); Hermansen (1968) and in recent years the discussion about the potential role of common barberry has been brought up again due to the repeal of Berberis eradication laws in several European countries in the 1990s (Berlin et al., 2012) and the replanting of rust susceptible Berberis spp. for environmental purposes in the United Kingdom (Saunders et al., 2019). The conclusions in this study were supported by different population genetic and plant pathology approaches, which generally resulted in similar outcomes.
Since the local outbreaks in Germany in 2013 (Olivera Firpo et al., 2017), Sicily in 2016 (Patpour et al., 2020), Sweden in 2017 (Berlin et al., 2012), and Siberia in 2016–2017 (Shamanin et al., 2020), stem rust has been increasingly frequent in northern and western Europe, for example in France (2021), where it has been reported only sporadically since the 1970s (Massenot, 1978). This is consistent with aerial spread of urediniospores across large distances, giving rise to observations of stem rust on wheat in new areas in Europe. The detection of relatively few clonal lineages (genotypes) of particular races, some of which have been detected outside Europe in previous years Olivera et al. (2019),3 underline the capacity of well-adapted Pgt races to reproduce clonally and spread across large areas within relatively few years (Hermansen, 1968; Brown and Hovmøller, 2002; Hovmøller et al., 2016; Walter et al., 2016).
It may be difficult to establish the exact geographical or evolutionary origin of newly emerged genotypes/races of airborne plant pathogens due to lack of regular and extensive population surveys. In this study, we used a combination of molecular genotype and race phenotype assays, which were designed for resolving long-term genetic relationships using selective neutral markers, and short-term responses to host induced selection governed by R-genes in host plants, respectively. We observed evidence of resampling of some of the genotypes/races, which have been detected previously in Europe, for example in the local outbreaks in Germany (2013): Clade VIII (RFCNC), Clade IV-E.1 (TKKTF), and Clade IV-E.2 (TKKTF). Clade VIII was resampled several times in Czech Republic and Slovakia (race RFCNC) and in Hungary (race RFCPC). Otherwise, Clade VIII has been reported in a single isolate (race RSBNC) from Italy in 1985 (Szabo et al., 2022).
Clade III-B consisted of a single race (TTRTF) and five closely related MLGs and may designate the return of stem rust in Europe at epidemic levels (Bhattacharya, 2017). The most prevalent MLG and race within Clade III-B was prevalent in Eritrea in East Africa in the same year (Patpour et al., 2020), but the first observation dates back to 2014 in Georgia, where it was sampled at low frequency in a genetically diverse population (Olivera et al., 2019). Clade IV-F, which in this study contained a single race (TKKTF), was first detected in Europe in 2018, but earlier on detected in Georgia in 2014 in the southern Caucasus region (Olivera et al., 2019), and in 2015 and 2016 in Azerbaijan, Iraq and Eritrea (GRRC).4 Clade IV-B, which in this study was first detected in Croatia in 2017, became widespread in Western Europe between 2019 and 2021 (this study), and recently race TKTTF was confirmed in the United Kingdom and Ireland (Tsushima et al., 2022). Alignment efforts at GRRC using isolates from the latter study (Ireland: 2020; UK: 2019, 2021) confirmed the presence of Clade IV-B and race TKTTF in these countries. The same genetic group has been widespread in multiple countries in East Africa and the Middle East in previous years (GRRC), but in East Africa harboring two races, TKTTF and TTTTF (GRRC), suggesting a close relationship between these.
In contrast, race TKTTF was detected in three distinct but related genetic groups. In East Africa, TKTTF, also known as the ‘Digalu’ race, was assigned Clade IV-A.1 and Clade IV-B (Olivera et al., 2015), and in the German outbreak in 2013, TKTTF was represented in both Clade-A.1 and Clade-A.2 (Olivera Firpo et al., 2017). This may suggest independent evolution of virulence in these genetic groups, stressing that a combination of molecular genotype and race phenotype assays are crucial to resolve the population biology of biotrophic plant pathogens such as Pgt, where disease prevention is often governed by R-genes in the host plant.
In contrast to the clonal population structure in most areas investigated in this study, high levels of diversity with respect to SSR and virulence were detected in local areas in Spain, Sweden and western Siberia where alternate hosts for yellow- and stem rust, Berberis vulgaris and/or indigenous subspecies are present (Rodriguez-Algaba et al., 2021). The indicators of sexual reproduction in these local areas were among others, (i) high numbers of MLGs and races relative to sample sizes, (ii) high diversity indexes, (iii) low index of association (almost random association in several cases), and (iv) deficit or proportional levels of heterozygosity compared to expected under HW equilibrium. For the Spanish (barberry) and Swedish populations, the observed heterozygosity was lower than the expected indicating some degree of inbreeding in these local, diverse populations in proximity to the alternate host.
Several of the same features have been observed in sexually reproduced populations of P. striiformis on wheat in the Himalayan region of Asia (Ali et al., 2014; Hovmøller et al., 2016; Thach et al., 2016; Chen et al., 2021), oat and rye stem rust populations in Sweden (Berlin et al., 2012), wheat stem rust in Georgia (Olivera et al., 2019), and with respect to race distribution in local populations of wheat stem rust in the United States (Groth and Roelfs, 1982). The features above are strong indicators of Berberis spp. as an important component in the epidemiology of wheat stem rust in Europe, although unique genotypes/races from local populations in Sweden/Spain were rarely observed in distant areas. We observed a unique MLG and race in Denmark in 2020 that was first detected in a local sexual population in Sweden in 2019, and a unique genotype sampled in Norway in 2021 was also part of the sexual population in Sweden. Overall, our observations suggest that stem rust is persistent in Europe from one year to the next, and more intensive sampling efforts would most likely reveal additional cases of resampling of genotypes derived from sexual reproduction on Berberis spp. in Europe.
The study revealed highly interesting features with respect to race and virulence in the European population. It was striking that in most cases, only a single race was detected within an individual MLG, occasionally two races only differentiated by a single virulence character. The SSR genotyping therefore to a large extent reflects the presence of individual races, which represents a cheap and rapid complementary tool for time consuming and costly race assays. However, in a longer time and area perspective, additional virulences may emerge within existing genotypes, in stem rust most evident by the evolution of 15 races within the Ug99 genetic lineage [Singh et al. (2011); Patpour et al. (2015); RustTracker website], and in wheat yellow rust there are multiple examples Wellings and McIntosh (1990); Hovmøller et al. (2011); and Thach et al. (2016). The latter feature stresses the importance of a continuous search for additional genotyping markers and procedures in pathogen surveillance programs to keep pace with the ongoing evolution of new races.
Sr31-virulence was observed in multiple and highly diverse races in local populations in Spain, and a single case in a population from Siberia. None of these were related to Ug99, demonstrating that Sr31-virulence is not unique to Ug99 (Pretorius et al., 2000), which has been the general conception since the emergence of this historical race in East Africa in 1999 (Olivera et al., 2022). The latter study reported a single isolate carrying virulence to Sr31 collected in 2018 in one of the local barberry areas in Spain, in proximity to the ones represented in our study. Twenty-two of the 28 recovered races from these areas carried Sr31-virulence in our study, irrespective of cereal/grass host origin, i.e., bread wheat, rye and a grass weed, Elymus spp. All isolates of these diverse races were successfully recovered and tested on wheat differential lines, which demonstrate that wheat adapted stem rust may also grow on rye, barley and wild grasses. These observations call for additional studies about existing and potential break-down of the host barriers that have been used to define formae speciales within P. graminis (Anikster, 1985). The presence of a Sr31-virulent race in an independent Siberian population stress that virulence may emerge independently and multiple times when large geographical areas and time spans are considered.
The present study was facilitated by long-term commitments of collaborators from leading rust diagnostic laboratories in Europe, United States and Russia. We demonstrated a successful alignment of two genotyping approaches and race phenotyping methodologies employed by different laboratories, which represents a major step forward in our efforts to strengthen coordinated rust surveillance for international research projects and global early warning systems for not only stem rust, but also yellow rust and leaf rust infecting wheat. Alignment and common standards for experimentation and exchange of data and results has made it possible to present new results from multiple laboratories in a global context, as demonstrated by the Wheat Rust Toolbox hosted by GRRC and associated websites for everyone interested. The burning question in years ahead is to which extent it will be possible to sustain the attention of both public and private donors to further develop even more timely and accurate early warning for disease outbreaks in major agricultural crops in Europe and beyond.
The present study has several implications for plant health with respect to wheat production in Europe and beyond. The lack of stem rust resistance in widely grown European wheat varieties stress an urgent need to initiate new breeding efforts with a focus on stem rust resistance in current and future breeding programs, which could involve both conventional breeding methodologies (Singh et al., 2015) and use of new approaches (Luo et al., 2021; Wulff and Krattinger, 2022). The rapid recolonization of stem rust across large wheat growing areas, where the disease has been absent for decades, the documentation of recent exotic incursions of highly virulent races from areas outside Europe, and the existence of genetically diverse, local stem rust populations in proximity to the alternate host, Berberis spp., capable of infecting multiple host species such as barley, bread wheat, durum wheat, spelt, rye and grass weeds, stress the importance of regular and coordinated pathogen and disease surveillance efforts at both European and global scales. Epidemiological studies about similarities/differences in aggressiveness between isolates of prevalent clades and potential temperature adaptation of stem rust to cooler climates would also be highly relevant.
The original contributions presented in the study are included in the article/supplementary material. The SSR genotypic data of the 459 isolates are available at https://doi.org/10.5281/zenodo.6598616.
MH, MP, JR-A, and AJ designed the study and wrote the manuscript. MP recovered and multiplied live isolates and performed the race phenotyping. JR-A, TT, and AJ performed the genotyping and data analyses. LS performed the SNP genotyping of reference isolates at CDL. AB, YJ, and DV designed sampling strategy and facilitated sampling in barberry areas in Sweden and Spain, respectively. VS and ES designed sampling strategy and facilitated sampling in Western Siberia. BR, KF, PC, AH, SS, KM, and RV facilitated sampling in Italy, Germany, Poland, Czechia, Slovakia and France, respectively, and ensured alignment with race phenotyping results in the national rust surveillance programs in these countries. JH uploaded genotyping and phenotyping data to the Wheat Rust Toolbox and developed the graphical presentation of results on maps. All authors contributed to the article and approved the submitted version.
Major part of this work was supported by the European Union’s Horizon 2020 research and innovation programme under grant agreement no. 773311 (RustWatch), long-term alignment efforts across genotyping platforms (SNP and SSR) conducted by the Cereal Disease Lab and Aarhus University (GRRC), and rust sampling in proximity to Berberis spp. in Spain were supported by Delivering Genetic Gain in Wheat project supported by the Bill and Melinda Gates Foundation, the UK Department for International Development, and Innovation Fund Denmark, Ministry of Higher Education and Science (grant no. 19052, MULTIRES). Sampling and characterization of samples from Sweden 2017–2018 was supported by grants from FORMAS (2017-02311) and Jordbruksverket (2018-02-27).
The authors declare that the research was conducted in the absence of any commercial or financial relationships that could be construed as a potential conflict of interest.
All claims expressed in this article are solely those of the authors and do not necessarily represent those of their affiliated organizations, or those of the publisher, the editors and the reviewers. Any product that may be evaluated in this article, or claim that may be made by its manufacturer, is not guaranteed or endorsed by the publisher.
The authors are grateful to Janne Holm Hansen and Jakob Sørensen, Aarhus University, for technical assistance during stem rust genotyping and phenotyping experiments at GRRC, the contribution of the CERCA program/Generalitat de Catalunya (http://cerca.cat), Prof. Carlos Cantero, University of Lleida, for valuable contribution to sampling in Spain, and surveillance and sampling in Western Siberia facilitated Alexey Morgunov, International Maize and Wheat Improvement Center (CIMMYT). Sampling in France 2019–2021 was possible thanks to the help of several contributors to the national multi-partner network on wheat rust (GIE Recherches Génétiques Céréales, CETAC, GEVES, ARVALIS-Institut du Végétal, INRAE). The authors are thankful to Diane Saunders for submission of isolates for alignment with recent studies in the United Kingdom and Ireland, and to more than 90 people (Supplementary Table S7), who submitted stem rust field samples to the Global Rust Reference Center, Aarhus University, Denmark, during the study.
The Supplementary Material for this article can be found online at: https://www.frontiersin.org/articles/10.3389/fpls.2022.882440/full#supplementary-material
Agapow, P. M., and Burt, A. (2001). Indices of multilocus linkage disequilibrium. Mol. Ecol. Notes 1, 101–102. doi: 10.1046/j.1471-8278.2000.00014.x
Ali, S., Gladieux, P., Leconte, M., Gautier, A., Justesen, A. F., Hovmoller, M. S., et al. (2014). Origin, migration routes and worldwide population genetic structure of the wheat yellow rust pathogen Puccinia striiformis f.sp. tritici. PLoS Pathog. 10:e1003903. doi: 10.1371/journal.ppat.1003903
Anikster, Y. (1985). “The Formae Speciales” in The Cereal Rusts. eds. A. P. Roelfs and W. R. Bushnell (New York: Academic Press, Inc.), 115–130.
Bartoš, P., Stuchlíková, E., and Hanušová, R. (1996). Adaptation of wheat rusts to the wheat cultivars in former Czechoslovakia. Euphytica 92, 95–103. doi: 10.1007/bf00022834
Berlin, A., Djurle, A., Samils, B., and Yuen, J. (2012). Genetic variation in Puccinia graminis collected from oats, Rye, and barberry. Phytopathology 102, 1006–1012. doi: 10.1094/phyto-03-12-0041-r
Bhattacharya, S. (2017). Deadly new wheat disease threatens Europe’s crops. Nature 542, 145–146. doi: 10.1038/nature.2017.21424
Brown, J. K. M., and Hovmøller, M. S. (2002). Aerial dispersal of pathogens on the global and continental scales and its impact on plant disease. Science 297, 537–541. doi: 10.1126/science.1072678
Chen, W., Zhang, Z., Ma, X., Zhang, G., Yao, Q., Kang, Z., et al. (2021). Phenotyping and genotyping analyses reveal the spread of Puccinia striiformis f. sp. tritici aeciospores from susceptible barberry to wheat in Qinghai of China. Front. Plant Sci. 12, 12:304. doi: 10.3389/fpls.2021.764304
Groth, J. V., and Roelfs, A. P. (1982). Effect of sexual and asexual reproduction on race abundance in cereal rust fungus populations. Phytopathology 72, 1503–1507. doi: 10.1094/Phyto-72-1503
Hermansen, J. E. (1968). Studies on the spread and survival of cereal rust and mildew diseases in Denmark. Friesia 8, 1–206.
Hovmøller, M. S., Sørensen, C. K., Walter, S., and Justesen, A. F. (2011). Diversity of Puccinia striiformis on cereals and grasses. Annu. Rev. Phytopathol. 49, 197–217. doi: 10.1146/annurev-phyto-072910-095230
Hovmøller, M. S., Walter, S., Bayles, R. A., Hubbard, A., Flath, K., Sommerfeldt, N., et al. (2016). Replacement of the European wheat yellow rust population by new races from the Centre of diversity in the near-Himalayan region. Plant Pathol. 65, 402–411. doi: 10.1111/ppa.12433
Jin, Y., Szabo, L. J., Pretorius, Z. A., Singh, R. P., Ward, R., and Fetch, T. (2008). Detection of virulence to resistance gene Sr24 Within race TTKS of Puccinia graminis f. sp. tritici. Plant Dis. 92, 923–926. doi: 10.1094/pdis-92-6-0923
Jin, Y., Szabo, L. J., Rouse, M. N., Fetch, T., Pretorius, Z. A., Wanyera, R., et al. (2009). Detection of virulence to resistance gene Sr36 Within the TTKS race lineage of Puccinia graminis f. sp. tritici. Plant Dis. 93, 367–370. doi: 10.1094/pdis-93-4-0367
Jombart, T. (2008). Adegenet: a R package for the multivariate analysis of genetic markers. Bioinformatics 24, 1403–1405. doi: 10.1093/bioinformatics/btn129
Jombart, T., Devillard, S., and Balloux, F. (2010). Discriminant analysis of principal components: a new method for the analysis of genetically structured populations. BMC Genet. 11:94. doi: 10.1186/1471-2156-11-94
Kamvar, Z. N., Brooks, J. C., and Grünwald, N. J. (2015). Novel R tools for analysis of genome-wide population genetic data with emphasis on clonality front. Genet. 6:208. doi: 10.3389/fgene.2015.00208
Kamvar, Z. N., Tabima, J. F., and Grunwald, N. J. (2014). Poppr: an R package for genetic analysis of populations with clonal, partially clonal, and/or sexual reproduction. PeerJ 2:e281. doi: 10.7717/peerj.281
Kjellström, C. (2021). Population structure of Puccinia Graminis, the cause of stem rust on wheat, barley, and rye in Sweden. MSc Master, Swedish University of Agricultural Sciences, SLU.
Lewis, C. M., Persoons, A., Bebber, D. P., Kigathi, R. N., Maintz, J., Findlay, K., et al. (2018). Potential for re-emergence of wheat stem rust in the United Kingdom. Commun. Biol. 1:13. doi: 10.1038/s42003-018-0013-y
Luo, M., Xie, L., Chakraborty, S., Wang, A., Matny, O., Jugovich, M., et al. (2021). A five-transgene cassette confers broad-spectrum resistance to a fungal rust pathogen in wheat. Nat. Biotechnol. 39, 561–566. doi: 10.1038/s41587-020-00770-x
Massenot, M. (1978). Changes in the race composition of Puccinia graminis f.sp. tritici in France in 1977. Cereal Rust. Bull. 6:14.
McIntosh, R. A., Hart, G. E., and Gale, M. D. (1995). "Catalogue of wheat symbols for wheat," in Proceedings of the Eighth International Wheat Genetics Symposium; July 20-25, 1993; (Beijing, China), 1333–1451.
McIntosh, R. A., and Pretorius, Z. A. (2011). Borlaug global rust initiative provides momentum for wheat rust research. Euphytica 179, 1–2. doi: 10.1007/s10681-011-0389-y
Olivera Firpo, P. D., Newcomb, M., Flath, K., Sommerfeldt-Impe, N., Szabo, L. J., Carter, M., et al. (2017). Characterization of Puccinia graminis f. sp. tritici isolates derived from an unusual wheat stem rust outbreak in Germany in 2013. Plant Pathol. 66, 1258–1266. doi: 10.1111/ppa.12674
Olivera, P., Newcomb, M., Szabo, L. J., Rouse, M., Johnson, J., Gale, S., et al. (2015). Phenotypic and genotypic characterization of race TKTTF of Puccinia graminis f. sp tritici that caused a wheat stem rust epidemic in southern Ethiopia in 2013-14. Phytopathology 105, 917–928. doi: 10.1094/phyto-11-14-0302-fi
Olivera, P. D., Sikharulidze, Z., Dumbadze, R., Szabo, L. J., Newcomb, M., Natsarishvili, K., et al. (2019). Presence of a Sexual Population of Puccinia graminisf. sp.triticiin Georgia Provides a Hotspot for Genotypic and Phenotypic Diversity. Phytopathology 109, 2152–2160. doi: 10.1094/phyto-06-19-0186-r
Olivera, P. D., Villegas, D., Cantero-Martínez, C., Szabo, L. J., Rouse, M. N., Luster, D. G., et al. (2022). A unique race of the wheat stem rust pathogen with virulence on Sr31 identified in Spain and reaction of wheat and durum cultivars to this race. Plant pathol. 71, 873–889. doi: 10.1111/ppa.13530
Patpour, M., Hovmoller, M., Justesen, A. F., Newcomb, M., Olivera Firpo, P. D., Jin, Y., et al. (2015). Emergence of virulence to SrTmp in the Ug99 race group of wheat stem rust, Puccinia graminis f. sp. tritici, in Africa. Plant Dis. 100:522. doi: 10.1094/PDIS-06-15-0668-PDN
Patpour, M., Justesen, A. F., Tecle, A. W., Yazdani, M., Yasaie, M., and Hovmøller, M. S. (2020). First report of race TTRTF of wheat stem rust (Puccinia graminis f. sp. tritici) in Eritrea. Plant Dis. 104:973. doi: 10.1094/pdis-10-19-2133-pdn
Peakall, R., and Smouse, P. E. (2012). GenAlEx 6.5: genetic analysis in excel. Population genetic software for teaching and research--an update. Bioinformatics 28, 2537–2539. doi: 10.1093/bioinformatics/bts460
Pretorius, Z. A., Singh, R. P., Wagoire, W. W., and Payne, T. S. (2000). Detection of virulence to wheat stem rust resistance gene Sr31 in Puccinia graminis. f. sp. tritici in Uganda. Plant Dis. 84:203. doi: 10.1094/pdis.2000.84.2.203b
Rodriguez-Algaba, J., Hovmøller, M. S., Villegas, D., Cantero-Martínez, C., Jin, Y., and Justesen, A. F. (2021). Two indigenous Berberis species From Spain were confirmed as alternate hosts of the yellow rust fungus Puccinia striiformis f. sp. tritici. Plant Dis. 105, 2281–2285. doi: 10.1094/pdis-02-21-0269-sc
Roelfs, A. P., and Martens, J. W. (1984). "Proposal for an International System of Race Nomenclature for Puccinia Graminis f.Sp. tritici.", in: 6th European and Mediterranean Cereal Rusts Conference; September 4-7, 1984; (Grignon, France: Cereal Rusts Foundation).
Saunders, D. G. O., Pretorius, Z. A., and Hovmøller, M. S. (2019). Tackling the re-emergence of wheat stem rust in Western Europe. Commun. Biol. 2:51. doi: 10.1038/s42003-019-0294-9
Shamanin, V., Pototskaya, I., Shepelev, S., Pozherukova, V., Salina, E., Skolotneva, E., et al. (2020). Stem rust in Western Siberia – race composition and effective resistance genes. Vavilov J. Gene. Breed. 24, 131–138. doi: 10.18699/VJ20.608
Shamanin, V., Salina, E., Wanyera, R., Zelenskiy, Y., Olivera, P., and Morgounov, A. (2016). Genetic diversity of spring wheat from Kazakhstan and Russia for resistance to stem rust Ug99. Euphytica 212, 287–296. doi: 10.1007/s10681-016-1769-0
Shannon, C. E. (2001). A mathematical theory of communication. Mob. Comput. Commun. 5, 3–55. doi: 10.1145/584091.584093
Singh, R. P., Hodson, D. P., Huerta-Espino, J., Jin, Y., Bhavani, S., Njau, P., et al. (2011). The emergence of Ug99 races of the stem rust fungus is a threat to world wheat production. Annu. Rev. Phytopathol. 49, 465–481. doi: 10.1146/annurev-phyto-072910-095423
Singh, R. P., Hodson, D. P., Jin, Y., Lagudah, E. S., Ayliffe, M. A., Bhavani, S., et al. (2015). Emergence and spread of new races of wheat stem rust fungus: continued threat to food security and prospects of genetic control. Phytopathology 105, 872–884. doi: 10.1094/phyto-01-15-0030-fi
Stakman, E. C. (1923). "Barberry eradication prevents black rust in Western Europe ", in Washington: United States Department of Agriculture, Department, Circular.
Stakman, E. C., Stewart, D. M., and Loegering, W. Q. (1962). "Identification of physiologic races of Puccinia graminis var. tritici ". (Washington D.C.: United States Department of Agriculture Research Service).
Stoxen, S. (2012). Population Structure of Puccinia Graminis f. sp. tritici in the United States. MSc Master, University of Minnesota
Szabo, L., Olivera Firpo, P. D., Wanyera, R., Visser, B., and Jin, Y. (2022). Development of a diagnostic assay for differentiation between genetic groups in clades I, II, III and IV of Puccinia graminis f. sp. tritici. Plant Dis.. [Epub ahead of print]. doi: 10.1094/pdis-10-21-2161-re
Thach, T., Ali, S., de Vallavieille-Pope, C., Justesen, A. F., and Hovmøller, M. S. (2016). Worldwide population structure of the wheat rust fungus Puccinia striiformis in the past. Fungal Genet. Biol. 87, 1–8. doi: 10.1016/j.fgb.2015.12.014
Tsushima, A., Lewis, C. M., Flath, K., Kildea, S., and Saunders, D. G. O. (2022). Wheat stem rust recorded for the first time in decades in Ireland. Plant Pathol. 71, 890–900. doi: 10.1111/ppa.13532
Walter, S., Ali, S., Kemen, E., Nazari, K., Bahri, B., Enjalbert, J., et al. (2016). Molecular markers for tracking the origin and worldwide distribution of invasive strains of Puccinia striiformis. Ecol. Evol. 6, 2790–2804. doi: 10.1002/ece3.2069
Wellings, C. R., and McIntosh, R. A. (1990). Puccinia striiformis f.sp. tritici in Australasia: pathogenic changes during the first 10 years. Plant Pathol. 39, 316–325. doi: 10.1111/j.1365-3059.1990.tb02509.x
Wulff, B. B. H., and Krattinger, S. G. (2022). The long road to engineering durable disease resistance in wheat. Curr. Opin. Biotechnol. 73, 270–275. doi: 10.1016/j.copbio.2021.09.002
Keywords: Puccinia graminis, black rust, re-emergence, exotic incursion, common barberry, virulence, Sr31
Citation: Patpour M, Hovmøller MS, Rodriguez-Algaba J, Randazzo B, Villegas D, Shamanin VP, Berlin A, Flath K, Czembor P, Hanzalova A, Sliková S, Skolotneva ES, Jin Y, Szabo L, Meyer KJG, Valade R, Thach T, Hansen JG and Justesen AF (2022) Wheat Stem Rust Back in Europe: Diversity, Prevalence and Impact on Host Resistance. Front. Plant Sci. 13:882440. doi: 10.3389/fpls.2022.882440
Received: 23 February 2022; Accepted: 03 May 2022;
Published: 02 June 2022.
Edited by:
Xiangming Xu, National Institute of Agricultural Botany (NIAB), United KingdomReviewed by:
Firdissa Eticha Bokore, Agriculture and Agri-Food Canada (AAFC), CanadaCopyright © 2022 Patpour, Hovmøller, Rodriguez-Algaba, Randazzo, Villegas, Shamanin, Berlin, Flath, Czembor, Hanzalova, Sliková, Skolotneva, Jin, Szabo, Meyer, Valade, Thach, Hansen and Justesen. This is an open-access article distributed under the terms of the Creative Commons Attribution License (CC BY). The use, distribution or reproduction in other forums is permitted, provided the original author(s) and the copyright owner(s) are credited and that the original publication in this journal is cited, in accordance with accepted academic practice. No use, distribution or reproduction is permitted which does not comply with these terms.
*Correspondence: Mogens S. Hovmøller, bW9nZW5zLmhvdm1vbGxlckBhZ3JvLmF1LmRr; Annemarie F. Justesen, YW5uZW1hcmllZmVqZXIuanVzdGVzZW5AYWdyby5hdS5kaw==
†These authors have contributed equally to this work and share first authorship
Disclaimer: All claims expressed in this article are solely those of the authors and do not necessarily represent those of their affiliated organizations, or those of the publisher, the editors and the reviewers. Any product that may be evaluated in this article or claim that may be made by its manufacturer is not guaranteed or endorsed by the publisher.
Research integrity at Frontiers
Learn more about the work of our research integrity team to safeguard the quality of each article we publish.