- 1Institute for Sustainable Plant Protection, National Research Council of Italy, Sesto Fiorentino, Italy
- 2Department of Agriculture, Food and Environment, University of Pisa, Pisa, Italy
- 3Department of Land, Environment, Agriculture, and Forestry (TESAF), University of Padova, Legnaro, Italy
- 4Department of Chemistry, University of Padova, Padova, Italy
Peptaibols are non-ribosomal linear peptides naturally produced by a wide variety of fungi and represent the largest group of peptaibiotic molecules produced by Trichoderma species. Trichogin GA IV is an 11-residue lipopeptaibol naturally produced by Trichoderma longibrachiatum. Peptaibols possess the ability to form pores in lipid membranes or perturb their surface, and have been studied as antibiotics or anticancer drugs in human medicine, or as antimicrobial molecules against plant pathogens. When applied to plants, peptaibols may also elicit defense responses. A major drawback to the exploitation and application of peptaibols in agriculture is their poor water solubility. In a previous study, we designed water-soluble Lys-containing Trichogin GA IV analogs, which were able to inhibit the growth of several fungal plant pathogens in vitro. In the present study, we shed light on the mechanism underpinning their efficacy on plants, focusing on six Trichogin GA IV analogs. Our results highlighted peptide hydrophilicity, rather than helix stability, as the major determinant of their activity against B. cinerea infection in tomato leaves. The peptides showed preventive but not curative efficacy against infection, and lack of translaminar activity, with results reproducible on two tomato cultivars, Marmande and Micro-Tom. Reactive oxygen species (ROS) detection analysis in tomato and Arabidopsis, and expression of defense genes in tomato, highlighted a transient and limited impact of the peptides on the plant defense system. The treatment did not result in significant modulation of defense genes or defense priming. The antimicrobial effect thus emerges as the only mechanism behind the plant protection ability exerted by water-soluble Trichogin GA IV analogs, and limited effects on the plant metabolism are expected to occur.
Introduction
Peptaibols are a class of non-ribosomal linear peptides naturally produced by a variety of fungi, mostly belonging to the order Hypocreales, such as Clonostachys spp., Mycogone spp., and Sepedonium spp. among others, although Trichoderma species seem actually to be the main producers (Szekeres et al., 2005; Leitgeb et al., 2007; Montesinos, 2007). The first peptaibols were isolated in the late 1960s from Trichoderma viride and named suzukacillin and alamethicin (Leitgeb et al., 2007). Peptaibols are 5–20 residue-long peptides characterized by the presence of an acylated N terminus, non-proteinogenic amino acids, such as isovaline (Iva) or α-aminoisobutyric acid (Aib), and a C-terminal amino alcohol. Accordingly, their name derives from peptide + Aib + amino alcohol (pept-aib-ol; Szekeres et al., 2005; Leitgeb et al., 2007). Peptaibols represent the largest group of peptaibiotics produced by Trichoderma species (Zeilinger et al., 2016).
Peptaibols possess stable and amphipathic helical structures and can self-assemble to form pores in lipid membranes or perturb their surface (Szekeres et al., 2005; Zeilinger et al., 2016). The membrane activity of these metabolites has led several researchers to investigate their possible exploitation as antibiotics or anticancer drugs in human medicine (Leitgeb et al., 2007; Shi et al., 2010; Dalzini et al., 2016). Peptaibols possess interesting features also for plant protection purposes. Indeed, they have been found to act as antimicrobial molecules against plant pathogens (Shi et al., 2012; Zeilinger et al., 2016; De Zotti et al., 2020; Sella et al., 2021) and to elicit defense reactions in various plants (Engelberth et al., 2000; Viterbo et al., 2007; Luo et al., 2010; Rippa et al., 2010). It is not clear whether peptaibols may act as defense priming stimuli (Mauch-Mani et al., 2017; Baccelli et al., 2020).
As natural peptide molecules, peptaibols are expected to degrade to nontoxic amino acids when introduced into the environment, thus meeting current legislation requirements and public acceptance (De Zotti et al., 2020). Various limitations however hamper their exploitation in agriculture, in particular, difficulties regarding the purification procedures from naturally-producing fungi and, above all, their poor solubility in water (De Zotti et al., 2020). For these reasons, our group recently designed water-soluble analogs of the lipopeptaibol Trichogin GA IV for plant protection purposes (De Zotti et al., 2020; Sella et al., 2021).
Trichogin GA IV is a short-length (11 residues) helical and hydrophobic lipopeptaibol naturally produced by the fungus T. longibrachiatum (Rebuffat et al., 1999; Toniolo et al., 2001a). Native Trichogin GA IV possesses three Aib residues within its sequence, it is blocked at its N-terminus by an n-octanoyl (n-Oct) moiety and has a 1,2-aminoalcohol (Leucinol, Lol) at its C-terminus (De Zotti et al., 2012a). Trichogin GA IV shows remarkable resistance to proteolysis mostly due to its well-developed helical conformation and possesses antibacterial activity against methicillin-resistant human pathogens (De Zotti et al., 2009). We recently reported rationally-designed, water-soluble, Lys-containing Trichogin GA IV analogs with antimicrobial activity against fungal plant pathogens (De Zotti et al., 2020; Sella et al., 2021). In particular, Pep 4 and Pep 4r, both sharing Gly to Lys substitutions at positions 5 and 6 (K5K6), with Pep 4r also bearing a Lol to Leu-NH2 substitution at its C-terminus, inhibited in vitro growth of the fungal plant pathogens Botrytis cinerea, Bipolaris sorokiniana, Fusarium graminearum, Penicillium expansum, and Pyricularia oryzae. In addition, they were effective in preventing B. cinerea infections on grape and bean tissues, and P. oryzae infections on barley and rice leaves, without apparent phytotoxic effects when sprayed at a concentration of 50 μM (De Zotti et al., 2020; Sella et al., 2021).
In the present study, we investigate the mechanism behind their efficacy in planta, by focusing on the protection against B. cinerea infection in tomato. To this purpose, we used six Trichogin GA IV-derived peptides bearing Gly to Lys, Lol to Leu-NH2, and/or Gly to Aib substitutions, two of which designed and produced in the present study (Table 1). Reactive oxygen species (ROS) induction on tomato and Arabidopsis leaves, and expression of defense genes in tomato were studied to elucidate the ability of the peptides to induce, or prime, plant defenses.
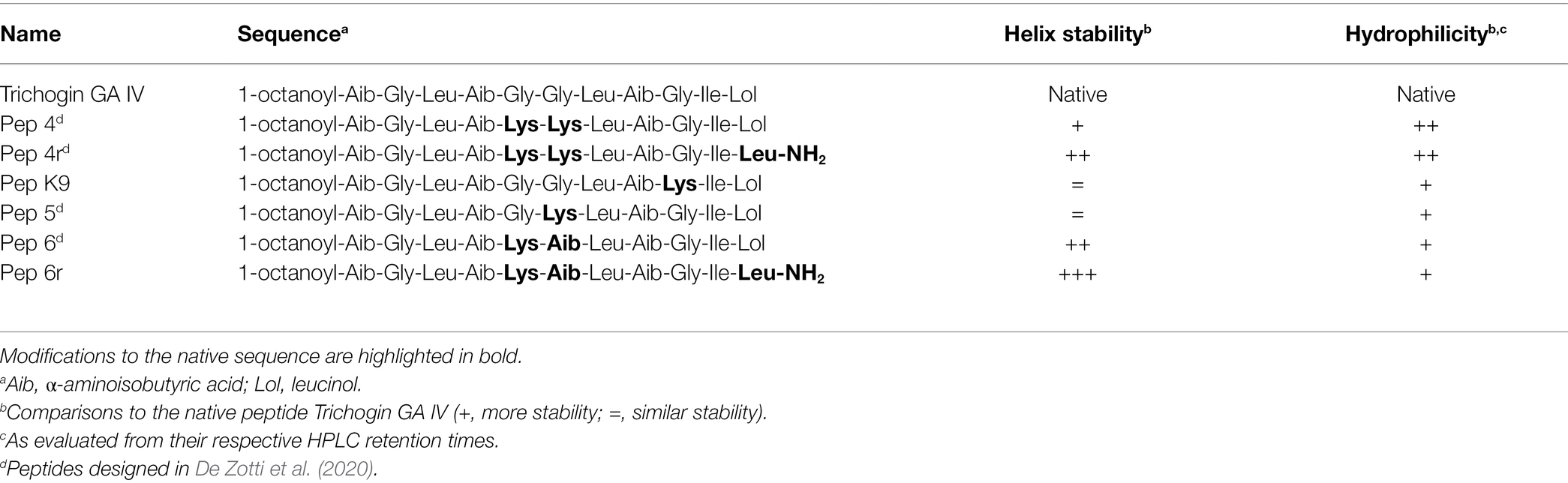
Table 1. Sequence and chemical features of Trichogin GA IV-derived peptides tested in the present study.
Materials and Methods
Peptide Design and Synthesis
Trichogin GA IV-derived peptides (Table 1) were produced by solid-phase peptide synthesis as described in De Zotti et al. (2020). The C-terminal Leucinol was obtained by using a 2-chlorotrytil resin preloaded with the 1,2-amino alcohol. A yield of about 70% on the purified peptides (purity > 95%) was achieved for all analogs. Pep K9 and Pep 6r were designed and synthesized in the present study. Details on the design and synthesis of Pep 4, Pep 4r, Pep 5, and Pep 6 are provided in De Zotti et al. (2020). C-terminal amide peptides were produced on a Rink-amide resin. Purity was checked by analytical HPLC on a C18 column (Phenomenex Jupiter 5 μm, 300 Å, 250 × 4.5 mm). The gradient used was 60%–100% B in 30 min; eluent A H2O/CH3CN 9:1 with 0.05% trifluoroacetic acid (TFA); eluent B CH3CN/H2O 9:1 with 0.05% TFA. HPLC retention times were used to evaluate hydrophilicity. Helix stability was evaluated by combining literature computational considerations (Chou and Fasman, 1978; Lyu et al., 1991), and circular dichroism analysis performed on Trichogin analogs in solvents of different polarity (De Zotti et al., 2012a).
Plant Material and Growth Conditions
Tomato (Solanum lycopersicum) seeds belonging to the cultivars Marmande and Micro-Tom were purchased from two specialty stores: Cooperativa Agricola Legnaia (Florence, Italy) and Gargini Sementi (Lucca, Italy), respectively. Plants were sown in Jiffy-7 peat pellets and transferred into commercial soil 2 weeks after germination. Plants were grown on a bench in a growth room under LED lights “Toplight Greenhouse Plus—Natural” (35% Blue, 17% Green; and 48% Red; C-LED srl, Imola, Italy) with 12 h of light (650 μmol m2 s−1), at 28°C (day)/21°C (night), until treatment.
For ROS detection, Arabidopsis thaliana ecotype Columbia-0 (Col-0) plants were grown in Jiffy-7 peat pellets in a dedicated growth chamber at 21°C (day)/18°C (night), 12 h of light (100 μmol m2 s−1), for 5 weeks.
Pathogen Strain and Cultivation
Botrytis cinerea strain B05.10 (Staats and van Kan, 2012) was grown on potato dextrose agar (PDA for microbiology, VWR International srl, Italy) at 21°C (day)/18°C (night), with 12 h of light (100 μmol m2 s−1) to stimulate and standardize conidia production. These conditions were obtained in the growth chamber used for A. thaliana growth, where detached tomato leaves and whole plantlets were also incubated after peptide treatment and during infections. Botrytis cinerea cultures were maintained under these conditions until conidia collection generally obtained after 10–15 days.
Plant Treatments
Treatments with Trichogin GA IV-derived peptides were performed on either detached leaves or whole plantlets, as described below. Peptide treatments for ROS detection were performed as described in the appropriate paragraph.
To test the efficacy of the peptides in preventing B. cinerea infection, 10–12 mature and healthy leaves were cut from different Micro-Tom or Marmande plants and placed in 90 mm-Petri dishes containing a filter paper disc wet with 1 ml sterile water, to ensure high internal relative humidity (RH) conditions during incubation. Leaves were sprayed with a 10-ml pump atomizer on the lower (abaxial) leaf surface with 50 μM peptide solution (approximately 300 μl per leaf), or sterile distilled water as a control. Peptides at the concentration of 100 μM were tested only on cv. Marmande leaves, according to the same procedure. In most cases, peptides were sprayed as peptide solution in water, without any addition of adjuvant. However, the use of the commercial surfactant Silwet L-77 AG (Momentive Performance Materials Inc., NY, United States) was assessed in some tests, as specified below and in the result section, with the aim of enhancing the biological activity of the peptides. The plates were sealed with parafilm and incubated in the growth chamber at 21°C (day)/18°C (night), with 12 h of light (100 μmol m2 s−1) until pathogen inoculation. Inoculations were performed 48 or 120 h after treatment, as described in the next paragraph.
Tests for curative efficacy, i.e., the ability of the peptides to stop ongoing infections, were performed by spraying detached leaves with 50 μM peptides 24 h after B. cinerea inoculation (see next paragraph). In this case, Micro-Tom or Marmande infected leaves were sprayed with a peptide solution containing 0.01% v/v Silwet L-77 AG.
To demonstrate the efficacy of Pep 4 to prevent B. cinerea infection on whole tomato plants, six 2-week-old Micro-Tom plants were sprayed on the adaxial leaf surfaces with 50 μM Pep 4 (approximately 700 μl per plant) containing 0.01% v/v Silwet L-77 AG. Six control plants were sprayed with sterile distilled water containing 0.01% v/v Silwet L-77 AG. After treatment, plants were incubated for 48 h at 21°C (day)/18°C (night), 12 h of light (100 μmol m2 s−1) until infection.
For gene expression studies, 30 2-week-old Micro-Tom plants were used to investigate the ability of the peptide to induce or prime defense gene expression against B. cinerea. In this case, 15 plants were sprayed with 50 μM Pep 4 containing 0.01% v/v Silwet L-77 AG, and 15 plants were sprayed with water containing 0.01% v/v Silwet L-77 AG as a control. Plants were incubated for 48 h as described above, then three control and three Pep 4-treated plants were sampled for RNA extraction (see appropriate paragraph). The remaining plants were inoculated (see next paragraph) or mock treated with potato dextrose broth (PDB, Laboratorios Conda S.A., Spain) yielding the following samples for gene expression studies: control + mock at 6 and 24 h post infection (hpi; i.e., treated with water 48 h before inoculation and then inoculated with PDB and collected at 6 or 24 hpi); control + B. cinerea at 6 and 24 hpi (i.e., treated with water 48 h before inoculation and then inoculated with B. cinerea and collected at 6 or 24 hpi); Pep 4 + mock at 6 and 24 hpi (i.e., treated with Pep 48 h before inoculation and then inoculated with PDB and collected at 6 or 24 hpi); Pep 4 + B. cinerea at 6 and 24 hpi (i.e., treated with Pep 48 h before inoculation and then inoculated with B. cinerea and collected at 6 or 24 hpi). At the end of each sampling time, all true leaves from each plant were frozen in liquid nitrogen and stored at −80°C until RNA extraction.
Pathogen Inoculation and Disease Quantification
All treatments and inoculations in this study were performed 2–3 h after lights in the growth chamber were on. Inoculations on detached leaves were performed by applying a single 10-μl droplet of B. cinerea B05.10 conidial suspension (1 × 106 conidia/ml in PDB) on a side of the midrib. In a few cases, bigger leaves were inoculated with two droplets applied on each side of the midrib. Inoculations were performed 48 h after peptide treatment either on the previously treated leaf surface or on the opposite untreated surface, in order to highlight translaminar or resistance-inducing activity (see Results). Inoculations 120 h after treatment were also performed on Pep 4- and Pep 4r-treated leaves in order to assess the duration of the protection. For curative efficacy, untreated healthy leaves were inoculated on the abaxial leaf surface 24 h before peptide treatment. Infected leaves were sealed in Petri dishes containing 1 ml water as described above to maintain high RH conditions needed for infection development, and incubated at 21°C (day)/18°C (night), 12 h of light (100 μmol m2 s−1). Disease severity was determined 3 days after infection by measuring the necrotic lesion diameter.
Two-week-old Micro-Tom plants were inoculated by spraying adaxial leaf surfaces with a conidial suspension containing 1 × 106 conidia/ml in PDB. Approximately, 800 μl of conidial suspension were sprayed on each plant. For gene expression studies, that amount was decreased to approximately 600 μl per plant, while mock plants were sprayed with the same amount of PDB. Plants were incubated at 21°C (day)/18°C (night), with 12 h of light (100 μmol m2 s−1), in closed transparent boxes containing free water on the bottom to ensure high RH conditions needed for infection development. Disease severity was determined after 3 days by classifying each plant on the basis of the visible leaf necrosis, as follows: Class I: no or point-limited necrosis; Class II: spreading lesions affecting <50% of the leaf surface; Class III: spreading lesions affecting >50% of the leaf surface; and Class IV: extensive necrosis and plant collapse.
Concerning the plants used for gene expression studies, disease severity was determined at the end of the experiment (24 hpi) by counting the number of necrotic spots visible on the leaf surface.
RNA Extraction and Gene Expression Analyses
Total RNA was extracted from 80 to 100 mg of ground leaf material by using RNeasy Plant Mini Kit (Qiagen, Italy), with guanidine thiocyanate extraction buffer RLT. RNA was quantified in a Qubit fluorometer (Thermo Fisher Scientific, MA, United States) by using Qubit RNA BR Assay Kit (Life Technologies, Italy). RNA integrity was evaluated by agarose gel electrophoresis. RNA samples (400 ng each) were treated with Amplification Grade DNase I (Sigma-Aldrich) and reverse-transcribed into cDNA by using Maxima First Strand cDNA Synthesis Kit for RT-qPCR (Thermo Fisher Scientific, MA, United States). qPCRs were performed in a StepOne Real-Time PCR System (Applied Biosystems). Reactions (20 μl) were carried out with 20 ng of cDNA, 250 nM of each primer, and Fast SYBR Green Master Mix (Applied Biosystems) by setting the recommended thermal-cycling conditions for the fast mode (Fast SYBR® Green Master Mix Protocol, Applied Biosystems). The following genes were analyzed: Proteinase inhibitor II (PIN2), Beta-1,3-glucanase A (GluA), Pathogenesis-related protein 1 (PR1), 1-aminocyclopropane-1-carboxylate oxidase 1 (ACO1), Pathogenesis-related genes transcriptional activator (PTI5), Lipoxygenase A (Lox1.1), and Polygalacturonase inhibitor protein (PGIP). Relative gene expression values were calculated by 2-ΔΔCt method as described in Livak and Schmittgen (2001). Actin-7 was selected as the endogenous reference gene after comparing its transcriptional stability against three candidate reference genes: Ubiquitin, Tubulin, and Glyceraldehyde-3-phosphate dehydrogenase (GPDH; Supplementary Figure S1). Transcriptional stability was tested in 10 cDNA samples representative of all the experimental conditions analyzed. Before performing relative expression calculations, the performance of each amplification run was checked by melting curve analysis, and the amplification plots were compared to ensure that amplification efficiencies between target and reference genes were approximately equal (data not shown; Schmittgen and Livak, 2008). The list of genes analyzed in this study and their primer sequences are provided in Supplementary Table S1. The primer sequences were designed in the present study, with the exception of ACO1, Actin-7, Ubiquitin, and GPDH (Mannucci et al., 2022).
Reactive Oxygen Species Detection on Leaves
Trichogin GA IV-derived peptides at the concentration of 50 μM in water were applied as 10-μl droplets on the abaxial surface of detached Micro-Tom and A. thaliana leaves. Five droplets were applied on each leaf, and three leaves per treatment were analyzed. Incubations were performed in Petri dishes for 24 and 48 h as previously described. ROS production by leaves was visualized by staining with the cell-permeable probe 2′,7′-dichlorodihydrofluorescein diacetate (H2DCFDA; Sigma-Aldrich, United States), as reported in Luti et al. (2020). For staining, leaves were soaked for 1 h in a 10 μM H2DCFDA solution in 20 mM phosphate buffer, pH 6.8. After H2DCFDA incubation, leaves were washed twice with phosphate buffer and mounted on glass slides for microscopy analysis, which was performed under a confocal Leica TCS SP5 scanning microscope (Leica, Germany; λex 460 and λem 512 nm).
Statistical Analyses
The efficacy of different peptide treatments against B. cinerea infection on detached leaves was analyzed by one-way ANOVA and Tukey–Kramer multiple comparison post-test when p < 0.05. Efficacies between two peptide concentrations, or between control and treated leaves, were analyzed by unpaired t-test (p < 0.05). Gene expression data were analyzed by unpaired t-test by comparing to the appropriate calibrator sample (control 48 hpt or control + mock 6 and 24 hpi) as detailed in the figure legends (p < 0.05). Analyses were performed in GraphPad software (GraphPad Software Inc., CA, United States). Disease severity was evaluated by Fisher’s exact test (p < 0.05).
Results
Peptide Design and Characterization
Following a published protocol (De Zotti et al., 2020), we synthesized six Trichogin GA IV-derived peptides with improved water solubility and/or conformational stability (Table 1). The analogs were characterized by one to three amino acid substitutions in the native Trichogin GA IV sequence. Gly to Lys substitutions were performed, with positional differences, in all peptide variants to confer water solubility (Table 1). The Gly-Gly dipeptide in the middle of the native sequence of Trichogin GA IV forms a kink in the 3D-structure, which lessens its helical content (Venanzi et al., 2009). Accordingly, being a better helix promoter than Gly (Chou and Fasman, 1978), Lys was also able to stabilize the peptide structure when placed in that kink. Similarly, the Gly-to-Aib substitution in that kink (Pep 6 and Pep 6r) further rigidified the helix, since Aib is a strong helix-inducer (Toniolo et al., 2001b). Finally, by replacing Gly with Lys at position 9 (Pep K9), i.e., far from that kink, we aimed to selectively evaluate the enhancement of hydrophilicity against helix stabilization effects shown by the other variants (position 9 is already involved in a strong helical segment, see Toniolo et al., 1994). The C-terminal leucine amide in the two most helical peptide analogs (Pep 4r and Pep 6r) introduced an additional hydrogen bond, further strengthening the helix (Table 1).
Protection by Trichogin GA IV-Derived Peptides Against Botrytis cinerea Infection on Tomato Leaves
The efficacy of the six Trichogin-derived peptides (Pep 4, Pep 4r, Pep K9, Pep 5, Pep 6, and Pep 6r, Table 1) against B. cinerea infections on tomato was firstly tested on cv. Marmande leaves. Leaves were sprayed with a 50 μM peptide solution 48 h before point inoculating B. cinerea conidia. In a first test, both treatments and infections were performed on the abaxial surface of detached leaves. The peptides Pep 4, Pep 4r, and Pep K9 turned out to reduce significantly disease severity compared to control, as determined by necrotic lesion measurements (Figure 1A) and no efficacy increase occurred when leaves were treated with 100 μM peptide solutions (Supplementary Figure S2). Neither 50 nor 100 μM spray treatments caused visible phytotoxic effects on leaves (data not shown), and 50 μM was selected as a suitable concentration for the following assays.
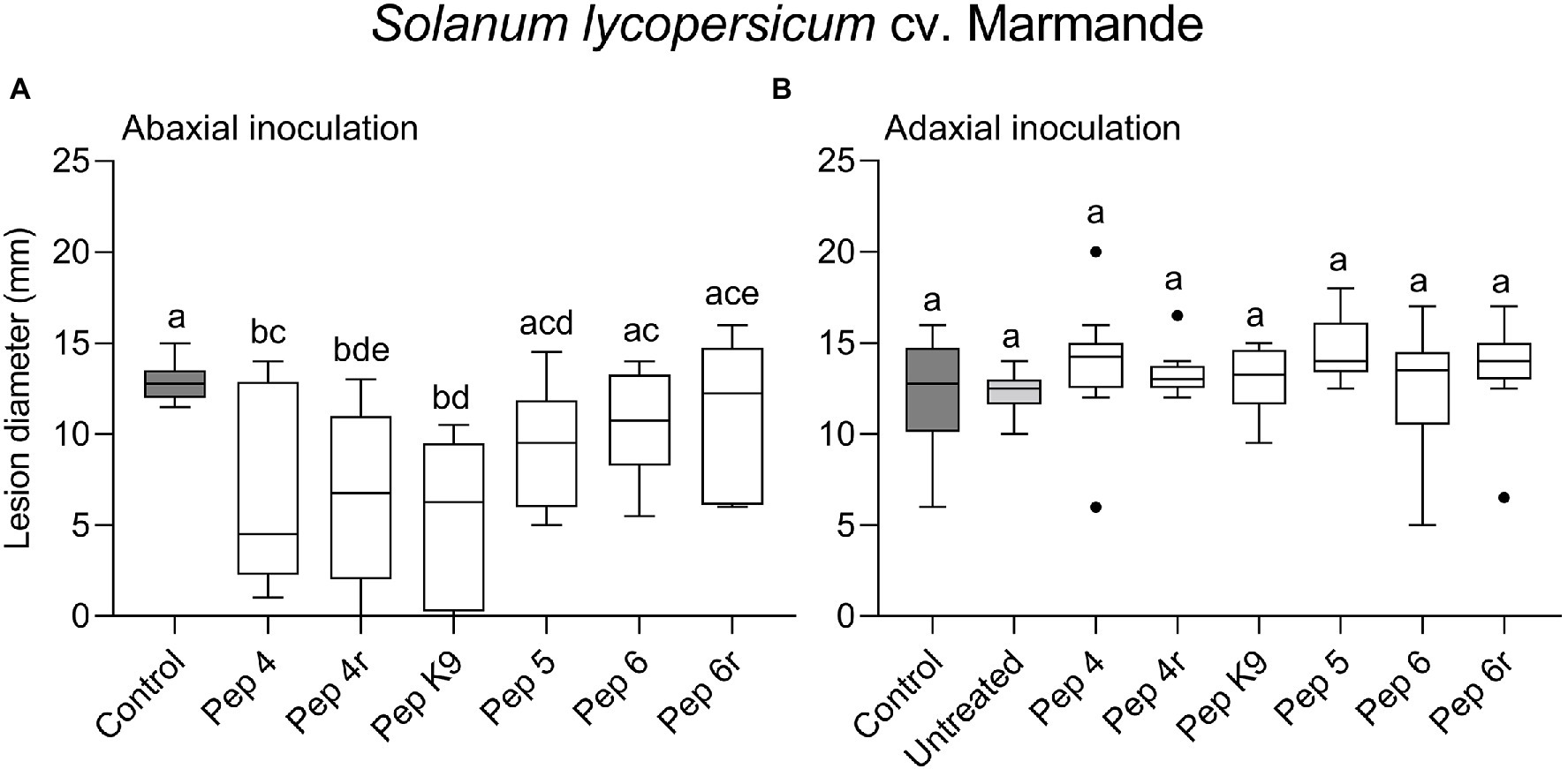
Figure 1. Preventive efficacy of Trichogin GA IV-derived peptides against Botrytis cinerea infection on Solanum lycopersicum cv. Marmande leaves. Treatments were performed on the lower (abaxial) surface by spraying 50 μM peptide solution in water (A), or in 0.01% v/v of surfactant Silwet L-77 AG (B). The pathogen was inoculated 48 h later by drop inoculation with a conidial suspension of B. cinerea. Conidia were applied on the abaxial (peptide-treated; A) or adaxial (untreated; B) leaf surface. Lesion diameters were measured 3 days after infection. Control leaves were treated with water (A) or water containing 0.01% Silwet L-77 (B). Values from 12 biological replicates (A) or 9–14 biological replicates (B) are reported as Tukey boxplots. Data were analyzed by one-way ANOVA with Tukey–Kramer post-test (p < 0.05). Statistically significant differences are shown by different letters.
To get clues on the ability of the peptides to act as penetrating fungicides or induce localized resistance, the peptides were sprayed on the abaxial leaf surface and the pathogen was inoculated 48 h later on the opposite (adaxial) untreated leaf surface. In this case, the treatments were not effective in restricting lesion development compared to control (Figure 1B). The surfactant Silwet L-77 AG, which was used as an adjuvant in this experiment, did not interfere with fungal infection compared to the untreated control (Figure 1B). The protective effect of peptide treatment was also tested on a different tomato cultivar (Micro-Tom; Figure 2). Again, while Pep 4, Pep 4r, and Pep K9 turned out to be effective as protectants against B. cinerea (Figure 2A), the peptides did not reduce significantly disease progression when the pathogen was being inoculated on the opposite, untreated leaf surface (Figure 2B). The duration of the protection was investigated by treating leaves with Pep 4 and Pep 4r and inoculating the fungus 5 days later. As shown in Figure 3, the peptides were still effective in reducing lesion diameters 5 days after the peptide treatments.
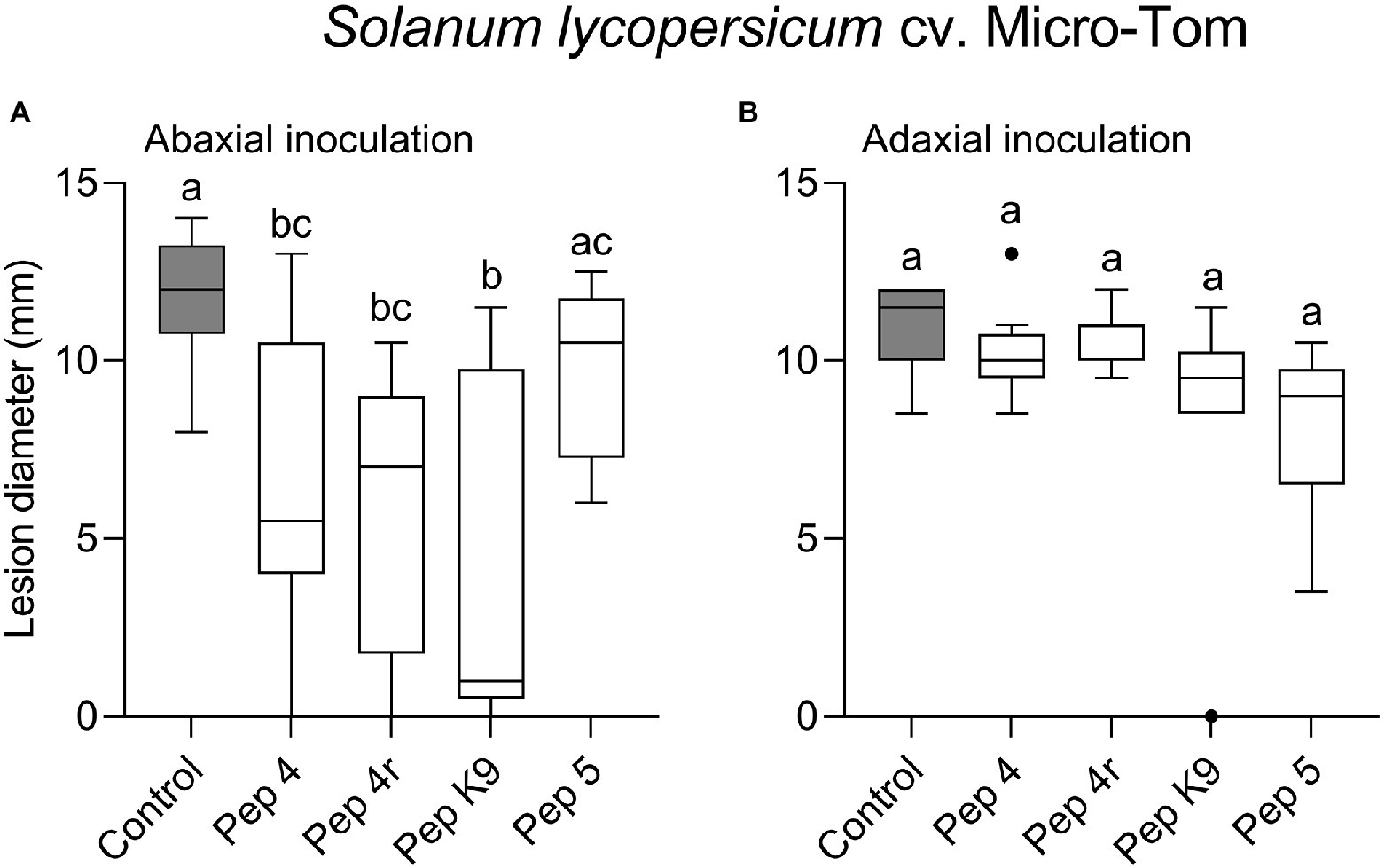
Figure 2. Preventive efficacy of selected Trichogin GA IV-derived peptides against Botrytis cinerea infection on Solanum lycopersicum cv. Micro-Tom leaves. Treatments were performed by spraying the lower (abaxial) surface with 50 μM peptide solution in water. The pathogen was inoculated 48 h later by drop inoculation with a conidial suspension of B. cinerea. Conidia were applied on the abaxial (peptide-treated; A) or adaxial (untreated; B) leaf surface. Lesion diameters were measured 3 days after infection. Control leaves were treated with water. Values from nine biological replicates are reported as Tukey boxplots. Data were analyzed by one-way ANOVA with Tukey–Kramer post-test (p < 0.05). Statistically significant differences are shown by different letters. The experiment in (A) was repeated with similar results.
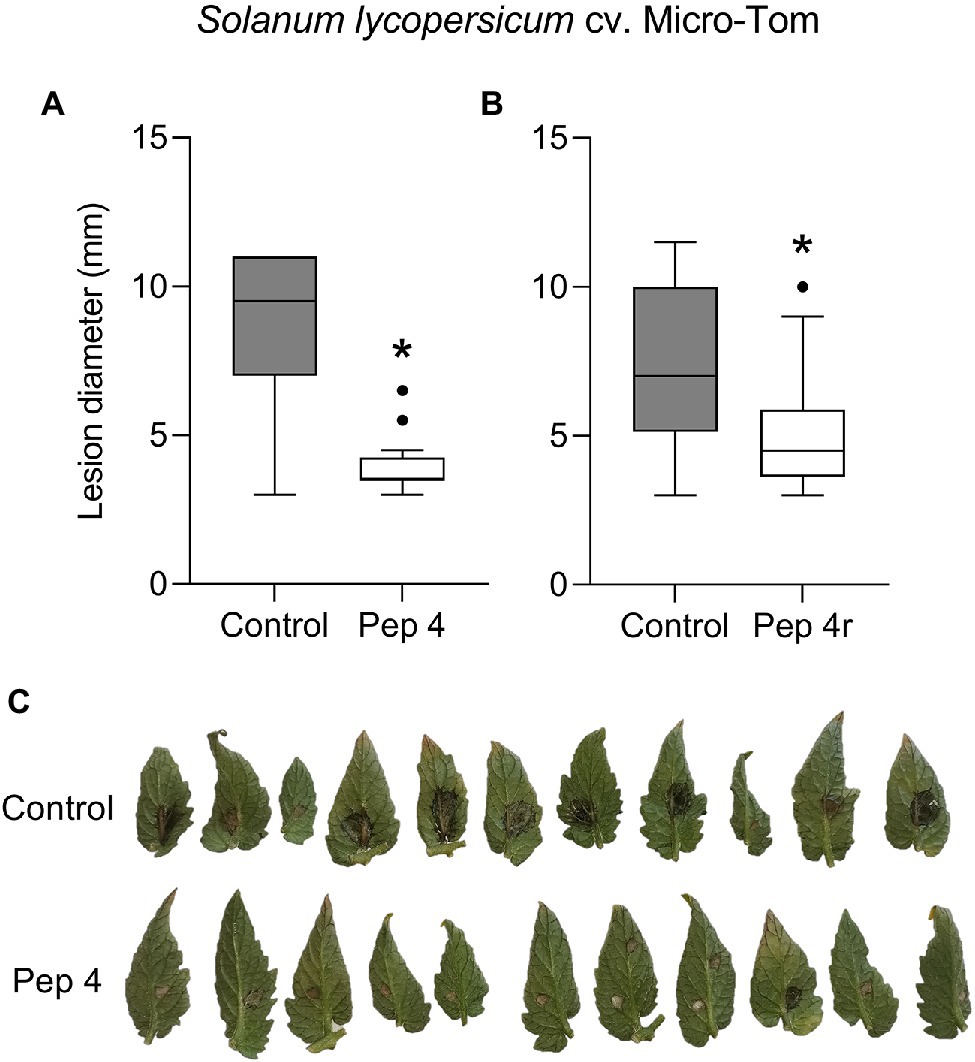
Figure 3. Trichogin GA IV-derived peptides Pep 4 (A,C) and Pep 4r (B) protect tomato from Botrytis cinerea infection up to 5 days after treatment. Solanum lycopersicum cv. Micro-Tom leaves were treated on the lower (abaxial) surface by spraying 50 μM peptide solution in water, and drop inoculated 120 h later with a conidial suspension of B. cinerea. Conidia were applied on the abaxial (peptide-treated) leaf surface. Lesion diameters were measured 3 days after infection. Control, leaves treated with water. Values from 11 to 13 biological replicates (A) or 12–16 biological replicates (B) are reported as Tukey boxplots. Data were analyzed by t-test (p < 0.05). Statistically significant differences are shown by asterisk. Representative pictures concerning Pep 4 protection from B. cinerea infection are shown (C).
Finally, to ascertain whether the protective effect detected on detached leaves occurred on whole tomato plants, 2-week-old Micro-Tom plants were sprayed with 50 μM Pep 4 containing 0.01% Silwet L-77 AG to promote leaf adhesion, and inoculated 48 h later by spraying B. cinerea conidia. As visible in Figure 4, Pep 4-treated plants displayed reduced leaf necrotic symptoms in comparison to control plants.
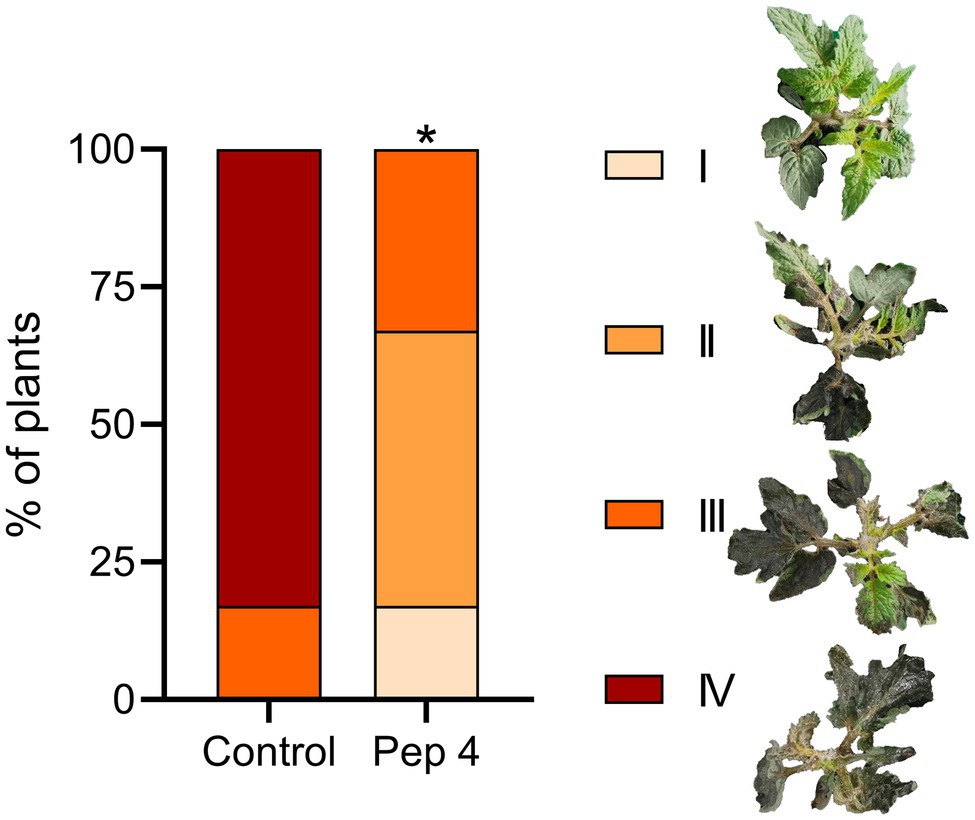
Figure 4. Pep 4 protects tomato plants from Botrytis cinerea infection. Two-week-old Solanum lycopersicum cv. Micro-Tom plants were sprayed on the upper (adaxial) leaf surfaces with 50 μM peptide solution in 0.01% v/v of Silwet L-77 AG, and spray inoculated on the same surface 48 h later with a conidial suspension of B. cinerea. Disease severity was evaluated by assigning plants to different classes, ranging from I (no or point limited necrosis) to IV (extensive necrosis and plant collapse) according to the representative examples. Statistically significant differences in class distribution were analyzed by Fisher’s exact test compared to control (water containing 0.01% v/v of Silwet L-77 AG). *p < 0.05 (n = 6).
Trichogin GA IV-Derived Peptides Do Not Show Curative Efficacy
Curative efficacy against B. cinerea infection was investigated on both tomato cultivars, Marmande and Micro-Tom. Leaves were treated with the peptides 24 h after point inoculation of B. cinerea conidia, i.e., when infection symptoms beneath the inoculation droplet were already visible (not shown). Pep 4, Pep 4r, and Pep K9 at 50 μM with 0.01% Silwet L-77 AG were ineffective in reducing disease severity compared to control on either cultivar (Figures 5A,B), indicating that Trichogin GA IV-derived peptides do not possess any curative efficacy against B. cinerea infections.
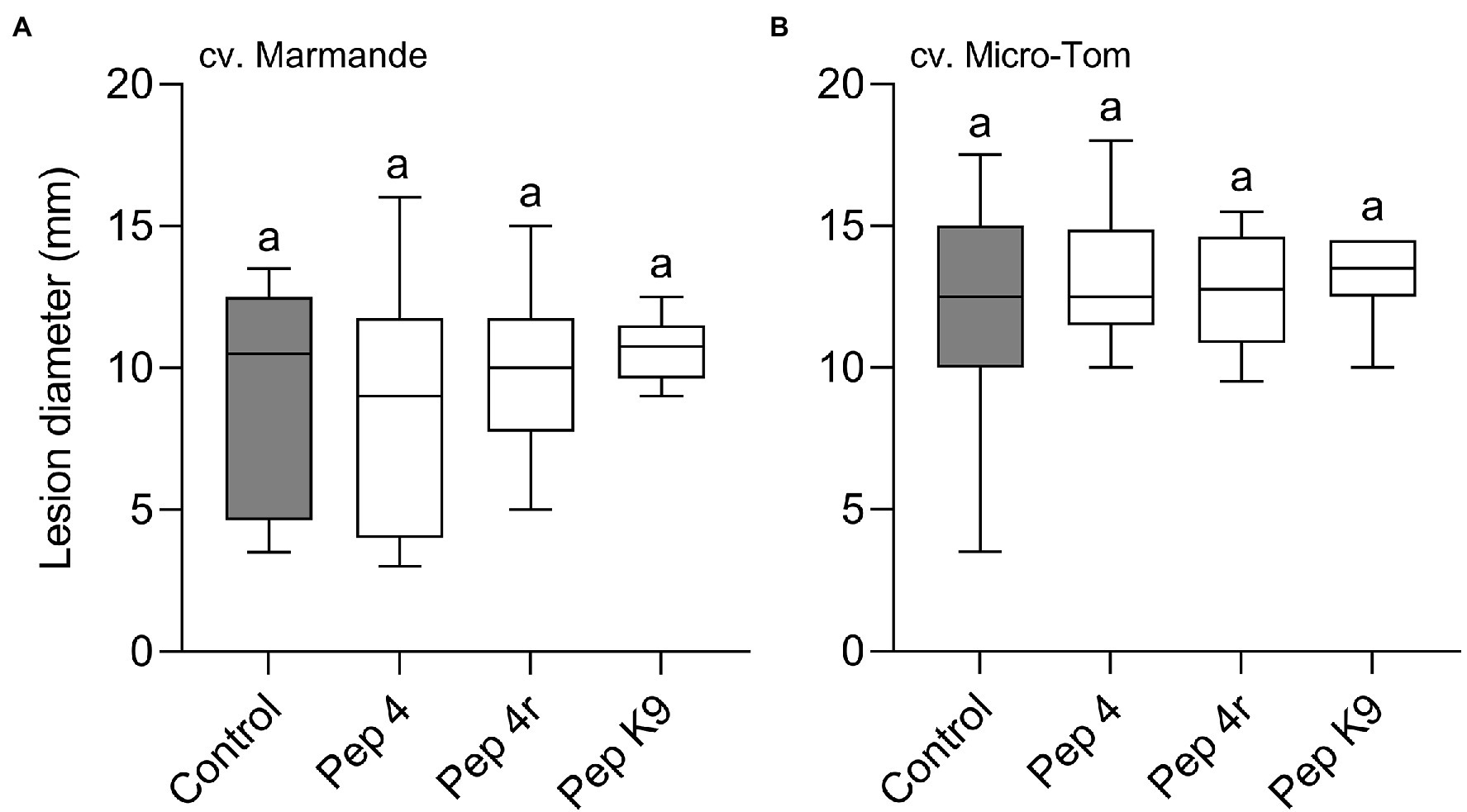
Figure 5. Trichogin GA IV-derived peptides do not show curative efficacy against Botrytis cinerea infection. The leaf abaxial surface of Solanum lycopersicum cv. Marmande (A) or Micro-Tom (B) was first drop inoculated with a conidial suspension of B. cinerea, and, 24 h later, was sprayed with 50 μM peptide solution in water containing 0.01% v/v of Silwet L-77 AG. Lesion diameters were measured 3 days after pathogen inoculation. Control leaves were treated with water containing 0.01% v/v of Silwet L-77. Values from 12 to 13 (A) or 13 to 15 (B) biological replicates are reported as Tukey boxplots. Data were analyzed by one-way ANOVA with Tukey–Kramer post-test (p < 0.05). Statistically significant differences are shown by different letters.
Induction of ROS Production
To understand more about their mode of action, peptides Pep 4, Pep 4r, and Pep K9 (all showing efficacy against B. cinerea), and Pep 6 and Pep 6r for comparison, were applied to healthy Micro-Tom and A. thaliana leaves. ROS were determined by fluorescence microscopy with the H2DCFDA probe. As visible in Figure 6A, only Pep 4-treated leaves showed ROS production on tomato leaves after 24 and 48 h. Fluorescent-stained ROS were mostly confined beneath the point of droplet application with no apparent spread to the surrounding zones. A similar result was obtained on A. thaliana leaves, although in that case both Pep 4 and Pep K9 induced ROS production on leaves (Figure 6B).
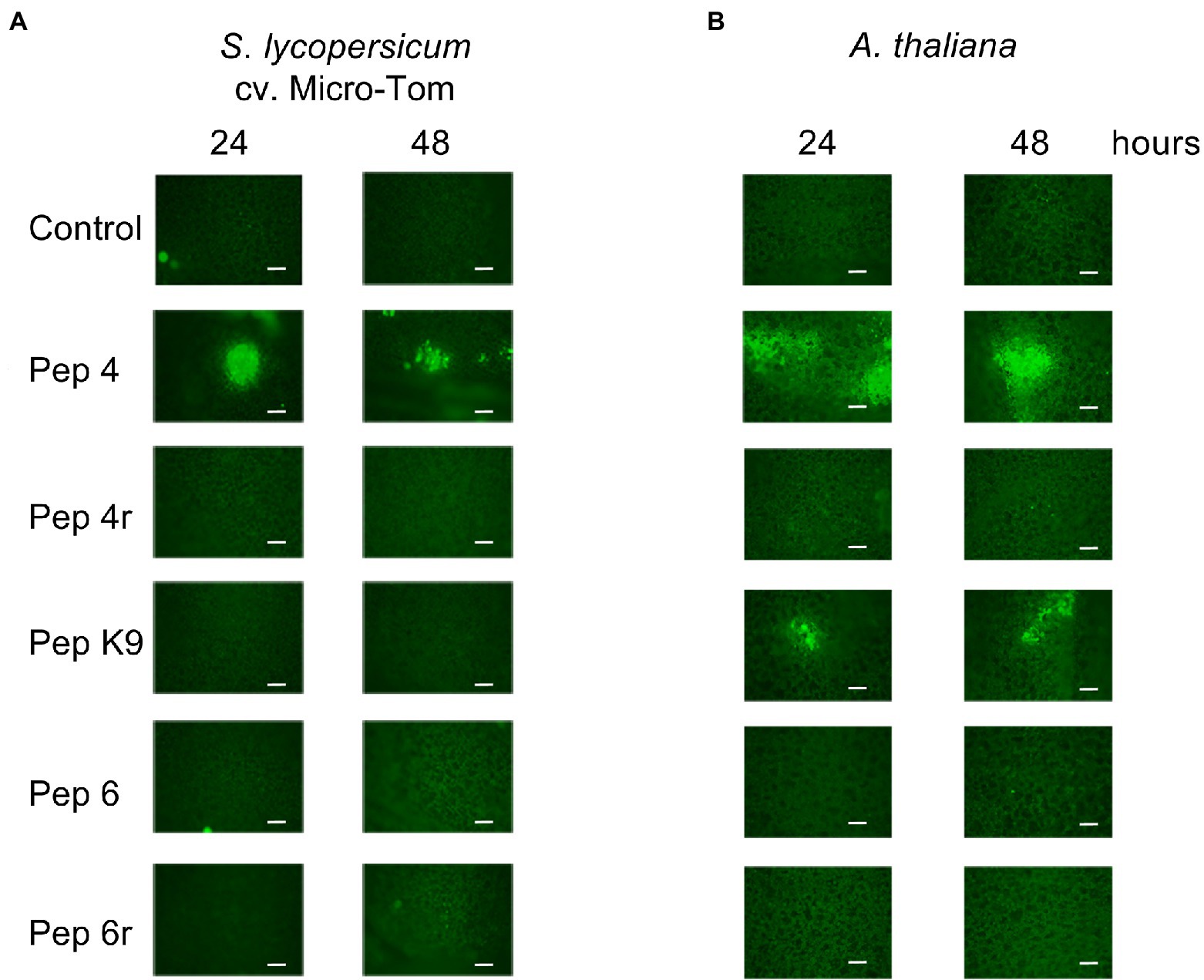
Figure 6. Reactive oxygen species (ROS) detection on Solanum lycopersicum cv. Micro-Tom (A) or Arabidopsis thaliana Col-0 (B) leaves. Leaves were treated with droplets containing 50 μM Trichogin GA IV-derived peptides for 24 and 48 h, and subsequently stained with the fluorescent probe 2′,7′-dichlorodihydrofluorescein diacetate (H2DCFDA) to visualize ROS production in situ. A representative picture per treatment is shown. Scale bars: 200 μm.
Impact of Peptide Treatment on Plant Defense Gene Expression
The induction of ROS production prompted us to investigate the ability of Pep 4 to prime defenses. To do so, a gene expression analysis was designed. The following defense-related genes were selected: the ethylene (ET) biosynthesis gene 1-aminocyclopropane-1-carboxylate oxidase 1 (ACO1), the jasmonic acid (JA)-related gene Lipoxygenase A (Lox1.1), the salicylic acid (SA)-marker gene Pathogenesis-related protein 1 (PR1), and some defense genes known to be affected during B. cinerea infection in tomato, such as Pathogenesis-related genes transcriptional activator 5 (PTI5), Polygalacturonase-inhibiting protein (PGIP), Proteinase inhibitor II (PIN2), and Beta-1,3-glucanase A (GluA; Harel et al., 2014; Sun et al., 2017; Gupta et al., 2021). Gene expression was analyzed at three different time points in order to investigate whether Pep 4 could directly induce defenses ahead of infection (48 hpt), or rather prime them for earlier/stronger induction during infection (6 and 24 hpi; Mauch-Mani et al., 2017). At each time point, control and mock-inoculated plants were collected for RNA extraction. Before collecting 24 hpi samples, the effectiveness of the treatment was verified and confirmed (Supplementary Figure S3).
Pep 4 did not significantly upregulate defense genes at 48 h post treatment (Figure 7A). The same held true at 6 and 24 hpi in mock-treated plants (i.e., 54 and 72 h after initial Pep 4 treatment; Figures 7B,C). The only exception was represented by a moderate down-regulation detectable for GluA in Pep 4 + mock plants after 24 hpi (i.e., 72 h after initial Pep 4 treatment; Figure 7C). In contrast, B. cinerea infection markedly altered gene expression: at 6 hpi, mRNA levels for Pti5, ACO1, and PR1 genes were significantly higher (≥3-fold) in infected plants not treated with Pep 4 (Figure 7B). At 24 hpi, the same three genes turned out to be strongly up-regulated (>20-fold; Figure 7C), and all the genes analyzed were modulated: PIN2 was significantly upregulated only in control + infection plants; GluA, Pti5, PGIP, ACO1, and PR1 were upregulated in both control + infection and Pep 4 + infection plants, and their relative expression reached a similar fold-change value in control + infection and Pep 4 + infection; Lox1.1 was instead strongly down-regulated in both control + infection and Pep 4 + infection plants (>50-fold; Figure 7C). Overall, these results suggested that Pep 4 treatments did not significantly induce defense gene expression nor did they enhance the defense response of the plant during infection.
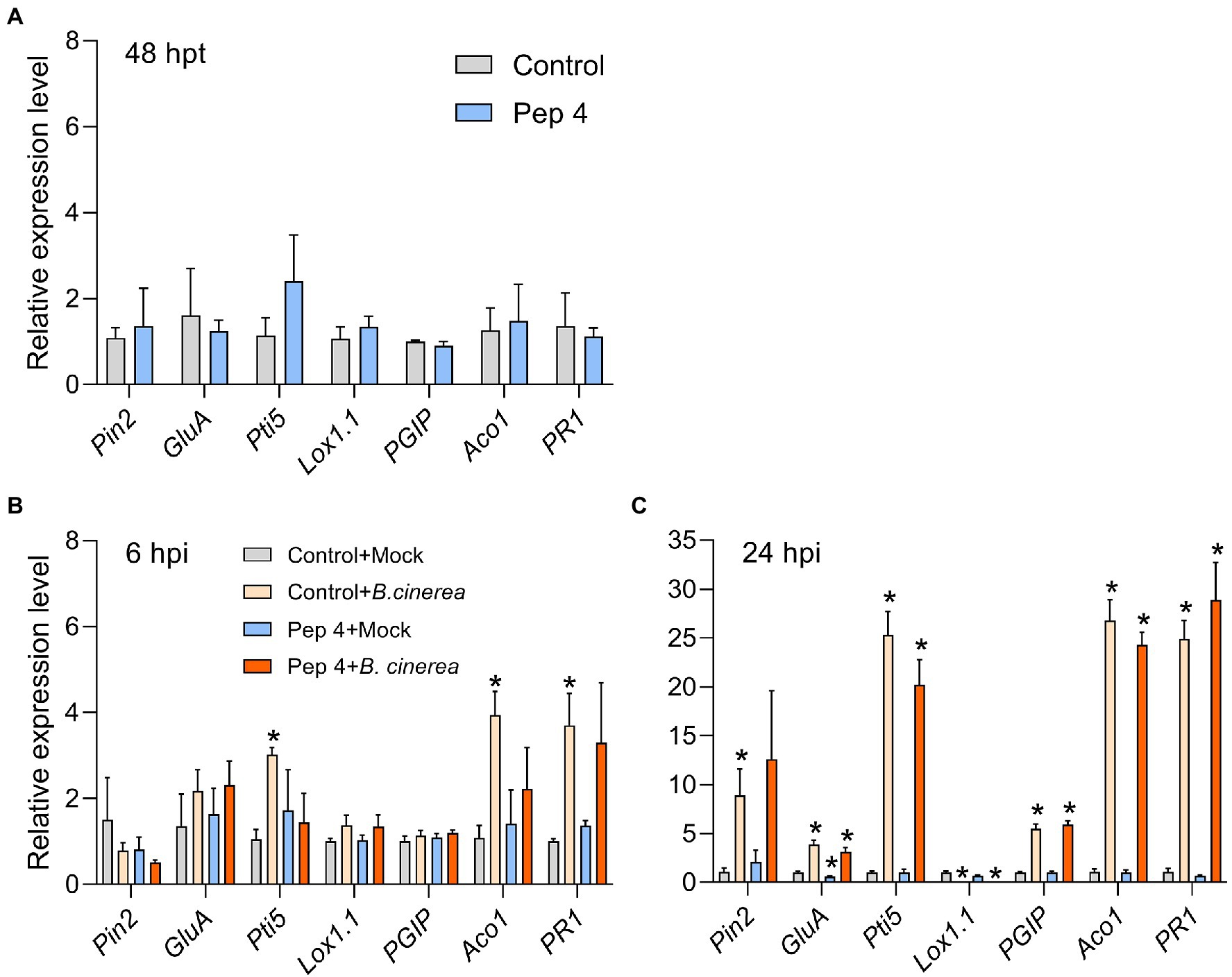
Figure 7. Expression of defense genes in Solanum lycopersicum cv. Micro-Tom plants treated with Pep 4 (A) and subsequently infected with Botrytis cinerea (B,C). Peptide treatments were performed by spraying 50 μM Pep 4 in water containing 0.01% v/v Silwet L-77 AG. Pathogen inoculations were performed by spraying a conidial suspension of B. cinerea. Hpt, hours post treatment; hpi, hours post infection. Control, water containing 0.01% v/v Silwet L-77 AG. Mock inoculations were performed by spraying the fungal culture medium potato dextrose broth. Genes analyzed: 1-aminocyclopropane-1-carboxylate oxidase 1(ACO1), Lipoxygenase A (Lox1.1), Pathogenesis-related protein 1 (PR1), Pathogenesis-related genes transcriptional activator 5 (PTI5), Polygalacturonase inhibitor protein (PGIP), Proteinase inhibitor II (PIN2), and Beta-1,3-glucanase A (GluA). Actin-7 was used as the endogenous reference gene for normalization. Control (A) or control + mock (B,C) were used as the calibrator sample (grey bars) for calculations. Average fold change values from three biological replicates (different plants) and two technical replicates ±SEM are shown. Statistical analysis was performed by t-test by comparing to the calibrator sample. Significant differences are marked by asterisk (p < 0.05).
Discussion
Building on our previous work (De Zotti et al., 2020), we studied here the plant protection ability of six Trichogin GA IV-derived peptides characterized by one to three substitutions in the native Trichogin GA IV sequence (Table 1): Gly to Lys substitutions occurred in all the peptide analogs (Lys is a cationic residue that gives hydrophilicity to the peptide sequence); Gly to α-aminoisobutyric acid (Aib) substitutions were present in Pep 6 and Pep 6r (Aib is a strong helix-inducer; De Zotti et al., 2012b); leucinol (Lol) to leucine amide substitutions were present in Pep 4r and Pep 6r (the introduction of an additional hydrogen bond strengthens the helix). By replacing the Gly residue with Lys at position 9 (Pep K9), we aimed to evaluate the enhancement of the peptide hydrophilicity against the helix stabilization effect.
In our previous study, a few Trichogin GA IV-derived peptides rationally modified to acquire water-solubility showed toxic activity against plant pathogens (De Zotti et al., 2020). In particular, we reported Pep 4 as one of the most effective molecules in inhibiting germination and growth of B. cinerea in vitro, and restricting B. cinerea infections on bean and grape tissues (De Zotti et al., 2020).
In the present study, by using tomato and Arabidopsis plants, we investigated the mode of action in planta of six Trichogin GA IV-derived peptides, with special attention to Pep 4.
We found that Pep 4 and Pep 4r (De Zotti et al., 2020), as well as Pep K9 (designed in the present study), were effective in restricting B. cinerea infections on tomato leaves. These peptide analogs were effective on both the tomato cultivars tested, namely Marmande and Micro-Tom (Figures 1, 2), whereas Pep 5, Pep 6, and Pep 6r did not show protection capacity. This first result suggests peptide hydrophilicity, rather than helix stability, as the major determinant of their efficacy in planta (Table 1).
The treatments were effective only when the peptides were sprayed before the application of B. cinerea conidia on leaves, whereas no protection was detectable when the peptides were sprayed on ongoing infections (Figure 5). Therefore, we may conclude that Trichogin GA IV-derived peptaibols possess preventive but not curative efficacy against B. cinerea infections.
Although very limited investigations have been carried out up to date, some peptaibols have been found to act as defense elicitors and resistance inducers in plants. Alamethicin was reported, for instance, to induce the synthesis of defense hormones (Engelberth et al., 2000), the emission of volatile organic compounds (Bruinsma et al., 2009), and lead to programmed cell death and callose deposition (Rippa et al., 2010). Two synthetic peptaibols, TvBI and TvBII from T. virens Gv29-8, could instead induce systemic resistance against Pseudomonas syringae pv. lachrymans in Cucumis sativus plants (Viterbo et al., 2007). Trichokonin from T. pseudokoningii SMF2 was able to induce ROS production and resistance against tobacco mosaic virus in tobacco (Luo et al., 2010) and Pectobacterium carotovorum subsp. carotovorum (Pcc) in Chinese cabbage (Li et al., 2014), respectively. Importantly, N-1-octanoylated, C-terminal methyl ester analogs of Trichogin GA IV were reported to induce PDF1.2 and PR2 defense gene expression in Arabidopsis (Rippa et al., 2010). For these reasons, we hypothesized that the preventive efficacy of our synthetic analogs could be due to a dual activity when sprayed on plants: antimicrobial (toxic) against the fungus, and resistance-inducing on the plant defense system.
The ROS results (Figure 6), especially concerning Pep 4, prompted us to investigate, by gene expression analysis, the resistance-inducing hypothesis. The ET biosynthetic gene ACO1, the JA-related gene Lox1.1, and the SA-marker gene PR1 were selected for their involvement in hormone-signaling pathways and their reported modulation during B. cinerea-tomato interaction: upregulation of PR1 and ACO1 genes, and downregulation of the Lox1.1 gene have been reported (Harel et al., 2014; Sun et al., 2017; Gupta et al., 2021). Importantly, SA-dependent defenses are known to positively regulate basal resistance against B. cinerea in tomato (Achuo et al., 2004). Other defense genes known to be upregulated during B. cinerea-tomato interaction were PTI5, PGIP, PIN2, and GluA (Harel et al., 2014; Sun et al., 2017; Gupta et al., 2021). Some of these, such as PGIP, PIN2, and GluA, encode for enzymes that may play a direct antifungal role: polygalacturonase-inhibiting proteins (PGIPs) are capable of inhibiting fungal endopolygalacturonases, thereby limiting plant tissue colonization (De Lorenzo et al., 2001); proteinase inhibitors may inhibit extracellular proteases secreted by B. cinerea and contribute to plant resistance (Turrà et al., 2020); beta-1,3-glucanases can degrade fungal cell wall glucans (van Loon et al., 2006).
However, the gene expression analysis did not show any considerable induction of defense genes by Pep 4 in a period of 48–72 h post treatment (Figure 7). The presence of defense priming could be similarly excluded since Pep 4 did not appear to prime defense genes for a quicker/stronger activation during infection with B. cinerea (Figures 7B,C). On the contrary, the gene expression level indicated that the plant response was delayed in Pep 4-treated plants, as visible at 6 hpi (Figure 7B), as the probable effect of the antimicrobial activity exerted by Pep 4 which delayed/reduced the infection level. A lack of resistance-inducing/priming ability was consistent with the lack of translaminar activity observed on leaves (Figures 1B, 2B). The ability to prime defenses and induce resistance, if present, would have indeed probably allowed highlighting an efficacy on both treated and untreated leaf surfaces, evidence that we were able to exclude.
In conclusion, with this study, it seems possible to indicate the antimicrobial effect as the only mechanism behind the plant protection ability exerted by Trichogin GA IV-derived peptides. The antimicrobial effect shown on leaves likely affected conidial germination, in a way similar to what we reported in vitro (De Zotti et al., 2020; Sella et al., 2021). Since B. cinerea hyphae are similarly susceptible to Pep 4 treatment (unpublished results), we hypothesize that the peptides cannot overcome the leaf cuticle to target the infectious hyphae in the leaf mesophyll.
Synthetic analogs of Trichogin GA IV have been previously demonstrated to retain the native ability to interact with membranes (De Zotti et al., 2012a,b), therefore their antimicrobial effect is very probably due to their membrane activity (Sella et al., 2021). This activity may also be responsible for the induction of plant ROS production observed after leaf treatment with Pep 4 and Pep K9. However, the gene expression analysis did not highlight considerable modulation of defense genes by Pep 4. PR1 gene modulation, for instance, which is known to occur at late time points after several biotic or abiotic stresses (Akbudak et al., 2020; Sewelam et al., 2021), was not altered by Pep 4 treatment in a 48–72 h period, indicating a limited stress occurrence. Therefore, the ROS result combined with the gene expression analysis suggest a transient and limited impact of Pep 4 on the plant defense system, not resulting in the activation of defense priming.
Further studies will elucidate whether, in the long term, repeated applications of Trichogin GA IV-derived peptides, despite their limited plant activity, may impact plant fitness and growth. The analog Pep 4r, showing high efficacy against infection and lack of ROS elicitation, could represent the best choice for future applications.
Data Availability Statement
The original contributions presented in the study are included in the article/Supplementary Material; further inquiries can be directed to the corresponding author.
Author Contributions
IB, LS, and FF conceived the present study. MDZ designed and synthesized Trichogin GA IV-derived peptides. IB and LS designed the experiments on plants. IB, SL, and RB carried out the experiments on plants. IB analyzed data and wrote the manuscript. All the authors provided their feedback during writing. All authors contributed to the article and approved the submitted version.
Funding
This research was funded by the Italian Ministry for University and Research, PRIN 20173LBZM2. MDZ is grateful to the University of Padova (Italy) for funding (grant number: P-DiSC#04BIRD2019-UNIPD) and to the Italian Ministry for the Economic Development, MISE (grant number: PoC@Unipd–CUP 96I20000120004; Project: ECOPEP).
Conflict of Interest
The authors declare that the research was conducted in the absence of any commercial or financial relationships that could be construed as a potential conflict of interest.
Publisher’s Note
All claims expressed in this article are solely those of the authors and do not necessarily represent those of their affiliated organizations, or those of the publisher, the editors and the reviewers. Any product that may be evaluated in this article, or claim that may be made by its manufacturer, is not guaranteed or endorsed by the publisher.
Acknowledgments
The authors thank Matteo Parri (University of Florence) for his support during microscopy analysis.
Supplementary Material
The Supplementary Material for this article can be found online at: https://www.frontiersin.org/articles/10.3389/fpls.2022.881961/full#supplementary-material
References
Achuo, E. A., Audenaert, K., Meziane, H., and Höfte, M. (2004). The salicylic acid-dependent defence pathway is effective against different pathogens in tomato and tobacco. Plant Pathol. 53, 65–72. doi: 10.1046/j.1365-3059.2003.00947.x
Akbudak, M. A., Yildiz, S., and Filiz, E. (2020). Pathogenesis related protein-1 (PR-1) genes in tomato (Solanum lycopersicum L.): bioinformatics analyses and expression profiles in response to drought stress. Genomics 112, 4089–4099. doi: 10.1016/j.ygeno.2020.07.004
Baccelli, I., Benny, J., Caruso, T., and Martinelli, F. (2020). The priming fingerprint on the plant transcriptome investigated through meta-analysis of RNA-Seq data. Eur. J. Plant Pathol. 156, 779–797. doi: 10.1007/s10658-019-01928-3
Bruinsma, M., Pang, B., Mumm, R., van Loon, J. J., and Dicke, M. (2009). Comparing induction at an early and late step in signal transduction mediating indirect defence in Brassica oleracea. J. Exp. Bot. 60, 2589–2599. doi: 10.1093/jxb/erp125
Chou, P. Y., and Fasman, G. D. (1978). Empirical predictions of protein conformation. Annu. Rev. Biochem. 47, 251–276. doi: 10.1146/annurev.bi.47.070178.001343
Dalzini, A., Bergamini, C., Biondi, B., De Zotti, M., Panighel, G., Fato, R., et al. (2016). The rational search for selective anticancer derivatives of the peptide Trichogin GA IV: a multi-technique biophysical approach. Sci. Rep. 6:24000. doi: 10.1038/srep24000
De Lorenzo, G., D'Ovidio, R., and Cervone, F. (2001). The role of polygalacturonase-inhibiting proteins (PGIPs) in defense against pathogenic fungi. Annu. Rev. Phytopathol. 39, 313–335. doi: 10.1146/annurev.phyto.39.1.313
De Zotti, M., Biondi, B., Formaggio, F., Toniolo, C., Stella, L., Park, Y., et al. (2009). Trichogin GA IV: an antibacterial and protease-resistant peptide. J. Pept. Sci. 15, 615–619. doi: 10.1002/psc.1135
De Zotti, M., Biondi, B., Park, Y., Hahm, K.-S., Crisma, M., Toniolo, C., et al. (2012b). Antimicrobial lipopeptaibol trichogin GA IV: role of the three Aib residues on conformation and bioactivity. Amino Acids 43, 1761–1777. doi: 10.1007/s00726-012-1261-7
De Zotti, M., Biondi, B., Peggion, C., Formaggio, F., Park, Y., Hahm, K.-S., et al. (2012a). Trichogin GA IV: a versatile template for the synthesis of novel peptaibiotics. Org. Biomol. Chem. 10, 1285–1299. doi: 10.1039/C1OB06178J
De Zotti, M., Sella, L., Bolzonello, A., Gabbatore, L., Peggion, C., Bortolotto, A., et al. (2020). Targeted amino acid substitutions in a Trichoderma peptaibol confer activity against fungal plant pathogens and protect host tissues from Botrytis cinerea infection. Int. J. Mol. Sci. 21:7521. doi: 10.1094/PHYTO-02-13-0043-R
Engelberth, J., Koch, T., Kühnemann, F., and Boland, W. (2000). Channel-forming Peptaibols are potent elicitors of plant secondary metabolism and tendril coiling. Angew. Chem. Int. Ed. Eng. 39, 1860–1862. doi: 10.1002/(SICI)1521-3773(20000515)39:10<1860::AID-ANIE1860>3.0.CO;2-F
Gupta, R., Leibman-Markus, M., Marash, I., Kovetz, N., Rav-David, D., Elad, Y., et al. (2021). Root zone warming represses foliar diseases in tomato by inducing systemic immunity. Plant Cell Environ. 44, 2277–2289. doi: 10.1111/pce.14006
Harel, Y. M., Mehari, Z. H., Rav-David, D., and Elad, Y. (2014). Systemic resistance to gray mold induced in tomato by benzothiadiazole and Trichoderma harzianum T39. Phytopathology 104, 150–157. doi: 10.1094/PHYTO-02-13-0043-R
Leitgeb, B., Szekeres, A., Manczinger, L., Vágvölgyi, C., and Kredics, L. (2007). The history of alamethicin: a review of the most extensively studied peptaibol. Chem. Biodivers. 4, 1027–1051. doi: 10.1002/cbdv.200790095
Li, H. Y., Luo, Y., Zhang, X. S., Shi, W. L., Gong, Z. T., Shi, M., et al. (2014). Trichokonins from Trichoderma pseudokoningii SMF2 induce resistance against gram-negative Pectobacterium carotovorum subsp. carotovorum in Chinese cabbage. FEMS Microbiol. Lett. 354, 75–82. doi: 10.1111/1574-6968.12427
Livak, K. J., and Schmittgen, T. D. (2001). Analysis of relative gene expression data using real-time quantitative PCR and the 2(-Delta Delta C(T)) method. Methods 25, 402–408. doi: 10.1006/meth.2001.1262
Luo, Y., Zhang, D. D., Dong, X. W., Zhao, P. B., Chen, L. L., Song, X. Y., et al. (2010). Antimicrobial peptaibols induce defense responses and systemic resistance in tobacco against tobacco mosaic virus. FEMS Microbiol. Lett. 313, 120–126. doi: 10.1111/j.1574-6968.2010.02135.x
Luti, S., Bemporad, F., Vivoli Vega, M., Leri, M., Musiani, F., Baccelli, I., et al. (2020). Partitioning the structural features that underlie expansin-like and elicitor activities of cerato-platanin. Int. J. Biol. Macromol. 165, 2845–2854. doi: 10.1016/j.ijbiomac.2020.10.122
Lyu, P. C., Sherman, J. C., Chen, A., and Kallenbach, N. R. (1991). Alpha-helix stabilization by natural and unnatural amino acids with alkyl side chains. Proc. Natl. Acad. Sci. U. S. A. 88, 5317–5320. doi: 10.1073/pnas.88.12.5317
Mannucci, A., Bernardi, R., Huarancca Reyes, T., Santin, M., Castagna, A., Quartacci, M. F., et al. (2022). Differential expression of 1-Aminocyclopropane-1-carboxylate synthase and oxidase gene family in micro-tom tomato leaves and roots under short daily UV radiation. J. Plant Growth Regul. doi: 10.1007/s00344-022-10599-0 (Epub ahead of print).
Mauch-Mani, B., Baccelli, I., Luna, E., and Flors, V. (2017). Defense priming: an adaptive part of induced resistance. Annu. Rev. Plant Biol. 68, 485–512. doi: 10.1146/annurev-arplant-042916-041132
Montesinos, E. (2007). Antimicrobial peptides and plant disease control. FEMS Microbiol. Lett. 270, 1–11. doi: 10.1111/j.1574-6968.2007.00683.x
Rebuffat, S., Goulard, C., Bodo, B., and Roquebert, M.-F. (1999). The peptaibol antibiotics from Trichoderma soil fungi: structural diversity and membrane properties. Recent Res. Devel. Org. Bioorg. Chem. 3, 65–91.
Rippa, S., Eid, M., Formaggio, F., Toniolo, C., and Béven, L. (2010). Hypersensitive-like response to the pore-former peptaibol alamethicin in Arabidopsis thaliana. Chembiochem 11, 2042–2049. doi: 10.1002/cbic.201000262
Schmittgen, T. D., and Livak, K. J. (2008). Analyzing real-time PCR data by the comparative C(T) method. Nat. Protoc. 3, 1101–1108. doi: 10.1038/nprot.2008.73
Sella, L., Govind, R., Caracciolo, R., Quarantin, A., Vu, V. V., Tundo, S., et al. (2021). Transcriptomic and ultrastructural analyses of Pyricularia oryzae treated with fungicidal Peptaibol analogs of Trichoderma Trichogin. Front. Microbiol. 12:753202. doi: 10.3389/fmicb.2021.753202
Sewelam, N., El-Shetehy, M., Mauch, F., and Maurino, V. G. (2021). Combined abiotic stresses repress defense and cell wall metabolic genes and render plants more susceptible to pathogen infection. Plan. Theory 10:1946. doi: 10.3390/plants10091946
Shi, M., Chen, L., Wang, X. W., Zhang, T., Zhao, P. B., Song, X. Y., et al. (2012). Antimicrobial peptaibols from Trichoderma pseudokoningii induce programmed cell death in plant fungal pathogens. Microbiology 158, 166–175. doi: 10.1099/mic.0.052670-0
Shi, M., Wang, H. N., Xie, S. T., Luo, Y., Sun, C. Y., Chen, X. L., et al. (2010). Antimicrobial peptaibols, novel suppressors of tumor cells, targeted calcium-mediated apoptosis and autophagy in human hepatocellular carcinoma cells. Mol. Cancer 9:26. doi: 10.1186/1476-4598-9-26
Staats, M., and van Kan, J. A. (2012). Genome update of Botrytis cinerea strains B05.10 and T4. Eukaryot. Cell 11, 1413–1414. doi: 10.1128/EC.00164-12
Sun, G., Wang, H., Shi, B., Shangguan, N., Wang, Y., and Ma, Q. (2017). Control efficiency and expressions of resistance genes in tomato plants treated with ε-poly-l-lysine against Botrytis cinerea. Pestic. Biochem. Physiol. 143, 191–198. doi: 10.1016/j.pestbp.2017.07.007
Szekeres, A., Leitgeb, B., Kredics, L., Antal, Z., Hatvani, L., Manczinger, L., et al. (2005). Peptaibols and related peptaibiotics of Trichoderma. A review. Acta Microbiol. Immunol. Hung. 52, 137–168. doi: 10.1556/AMicr.52.2005.2.2
Toniolo, C., Crisma, M., Formaggio, F., and Peggion, C. (2001b). Control of peptide conformation by the Thorpe-Ingold effect (C alpha-tetrasubstitution). Biopolymers 60, 396–419. doi: 10.1002/1097-0282(2001)60:6<396::AID-BIP10184>3.0.CO;2-7
Toniolo, C., Crisma, M., Formaggio, F., Peggion, C., Epand, R. F., and Epand, R. M. (2001a). Lipopeptaibols, a novel family of membrane active, antimicrobial peptides. Cell. Mol. Life Sci. 58, 1179–1188. doi: 10.1007/PL00000932
Toniolo, C., Peggion, C., Crisma, M., Formaggio, F., Shui, X., and Eggleston, S. (1994). Structure determination of racemic trichogin A IV using centrosymmetric crystals. Nat. Struct. Mol. Biol. 1, 908–914. doi: 10.1038/nsb1294-908
Turrà, D., Vitale, S., Marra, R., Woo, S. L., and Lorito, M. (2020). Heterologous expression of PKPI and Pin1 proteinase inhibitors enhances plant fitness and broad-spectrum resistance to biotic threats. Front. Plant Sci. 11:461. doi: 10.3389/fpls.2020.00461
van Loon, L. C., Rep, M., and Pieterse, C. M. (2006). Significance of inducible defense-related proteins in infected plants. Annu. Rev. Phytopathol. 44, 135–162. doi: 10.1146/annurev.phyto.44.070505.143425
Venanzi, M., Bocchinfuso, G., Gatto, E., Palleschi, A., Stella, L., Formaggio, F., et al. (2009). Metal binding properties of fluorescent analogues of trichogin GA IV: a conformational study by time-resolved spectroscopy and molecular mechanics investigations. Chembiochem 10, 91–97. doi: 10.1002/cbic.200800617
Viterbo, A., Wiest, A., Brotman, Y., Chet, I., and Kenerley, C. (2007). The 18mer peptaibols from Trichoderma virens elicit plant defence responses. Mol. Plant Pathol. 8, 737–746. doi: 10.1111/j.1364-3703.2007.00430.x
Keywords: Trichoderma, antimicrobial peptides, peptaibiotics, Solanum lycopersicum, Micro-Tom, Marmande, ROS, plant pathogen
Citation: Baccelli I, Luti S, Bernardi R, Favaron F, De Zotti M and Sella L (2022) Water-Soluble Trichogin GA IV-Derived Peptaibols Protect Tomato Plants From Botrytis cinerea Infection With Limited Impact on Plant Defenses. Front. Plant Sci. 13:881961. doi: 10.3389/fpls.2022.881961
Edited by:
Prem Lal Kashyap, Indian Institute of Wheat and Barley Research (ICAR), IndiaReviewed by:
Soren (Hamed) Seifi, University of Guelph, CanadaParissa Taheri, Ferdowsi University of Mashhad, Iran
Copyright © 2022 Baccelli, Luti, Bernardi, Favaron, De Zotti and Sella. This is an open-access article distributed under the terms of the Creative Commons Attribution License (CC BY). The use, distribution or reproduction in other forums is permitted, provided the original author(s) and the copyright owner(s) are credited and that the original publication in this journal is cited, in accordance with accepted academic practice. No use, distribution or reproduction is permitted which does not comply with these terms.
*Correspondence: Ivan Baccelli, aXZhbi5iYWNjZWxsaUBpcHNwLmNuci5pdA==