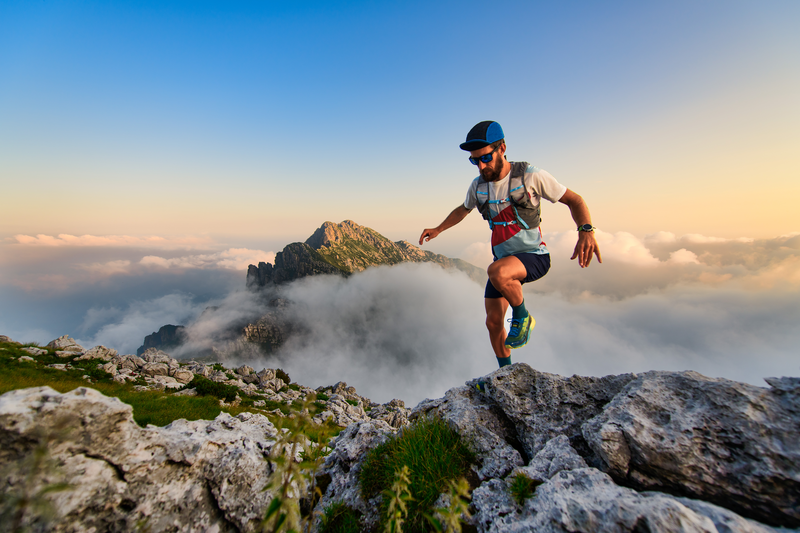
95% of researchers rate our articles as excellent or good
Learn more about the work of our research integrity team to safeguard the quality of each article we publish.
Find out more
ORIGINAL RESEARCH article
Front. Plant Sci. , 19 May 2022
Sec. Plant Physiology
Volume 13 - 2022 | https://doi.org/10.3389/fpls.2022.881282
This article is part of the Research Topic Plant Hydraulics Under Climate Change View all 10 articles
Water use efficiency (WUE) plays important role in understanding the interaction between carbon and water cycles in the plant-soil-atmosphere system. However, little is known regarding the impact of altered precipitation on plant WUE in arid and semi-arid regions. The study examined the effects of altered precipitation [i.e., ambient precipitation (100% of natural precipitation), decreased precipitation (DP, −50%) and increased precipitation (IP, +50%)] on the WUE of grass species (Stipa grandis and Stipa bungeana) and forb species (Artemisia gmelinii) in a temperate grassland. The results found that WUE was significantly affected by growth stages, precipitation and plant species. DP increased the WUE of S. grandis and S. bungeana generally, but IP decreased WUE especially in A. gmelinii. And the grasses had the higher WUE than forbs. For different growth stages, the WUE in the initial growth stage was lower than that in the middle and late growth stages. Soil temperature, available nutrients (i.e., NO3–, NH4+, and AP) and microorganisms under the altered precipitations were the main factors affecting plant WUE. These findings highlighted that the grasses have higher WUE than forbs, which can be given priority to vegetation restoration in arid and semi-arid areas.
Precipitation patterns are important environmental factors that affect the structure and process of terrestrial ecosystems and the key drivers of biological activity in semi-arid and arid ecosystems (Nielsen and Ball, 2015). With the intensification of climate change, the global precipitation pattern has undergone enormous changes, and the frequency of extreme manipulation events has been increasing (Sponseller, 2010). This affects the structure and function of plants and microbial communities, which in turn influences the water, energy and nutrient cycles of terrestrial ecosystems (Weltzin et al., 2003).
Water use efficiency (WUE) is the ratio of carbon (C) assimilation to water losses, which reflects the interaction between the C and water cycles for the plant-soil-atmosphere system (Bai et al., 2020). Moreover, it is also an important indicator to explore the adaptability of plants to environmental change and to predict the impact of global change (Nielsen and Ball, 2015). At the leaf scale, WUE is the ratio of photosynthetic rate to transpiration rate (Bai et al., 2020). C isotope fractionation is highly correlated with the ratio of photosynthetic C assimilation and transpiration rate, and C isotope fractionation is also highly correlated with plant WUE (Latif et al., 2014). Stable C isotope technology is used globally to determine WUE, and the main principle of this technology is that there is a strong correlation between the stable C isotope ratio (13C/12C, δ13C) or stability C isotope discrimination coefficient (Δ) and WUE of C3 plants, thus can be used it as an indicator of WUE (Latif et al., 2014). It is an effective method to study the long-term WUE of plants and reflects the use of water and adaptation of plants to water stress over time (Chen et al., 2011).
The factors affecting plant WUE mainly including plant species, environmental and regional factors, especially, soil moisture (SM) is one of the most direct factors (Law et al., 2010). Under limited soil water conditions, plants can control water losses and increase their WUE by reducing stomatal conductance, which varies among plant species and depends on water availability and carbon dioxide concentration in the atmosphere (Latif et al., 2014; Silva, 2015). Precipitation deficiency is frequently recorded as a result of global warming, and affects the terrestrial C and water cycles by reducing the C sequestration ability and aggravating the evaporation rate of plants (Battipaglia et al., 2014), which further influences the WUE of plants. However, one study reported that plant WUE also decrease with drought in semi-arid regions (Yang et al., 2016). Moreover, previous studies paid more attentions on the precipitation changes effect on WUE at the community scale, little information at the plant species scale. Furthermore, plant species is the key factor determined the WUE under the changes in precipitation patterns. Therefore, it is necessary to explore the variation tendency in plant WUE at the species scale, which are crucial for predicting the impact of future climatic change on plant C and water cycling processes.
The grassland area of the world is approximately 50 million km2, accounting for approximately 33.5% of global land area (Kang et al., 2007). As an important subsystem of terrestrial ecosystems, grasslands play a key role in global change and ecosystem function. Considering the background of global climate change, alterations in precipitation patterns will affect water utilization in plants directly and the functions and processes of grassland ecosystems indirectly. However, most of the previous studies had only focused on short-term (at the day scale) WUE of plants, a single plant species or single growth period responded to altered precipitation (Karatassiou and Noitsakis, 2010; Sensuła, 2015; Han et al., 2020). Therefore, this study first to explore the effect of altered precipitation on WUE among functional groups (two grasses and one forbs) following the whole growth period, and further to identify the key abiotic and biotic factors effecting on the dynamic of WUE in temperate grasslands. It would be useful to better understand the changes and the response mechanisms of WUE to altered precipitation patterns in grassland ecosystems.
This study was conducted at the national fixed grassland monitoring point (106°17′49.2′′E, 36°16′44.5′′N, 1,963 m a.s.l.) in the Yunwu Mountains on the Loess Plateau of China, where has a temperate continental climate and is located inland. The mean annual temperature is 7.12°C. The mean annual precipitation of 439.3 mm and where has a strong rainfall seasonality (mainly occurring between June and September). The soil types were gray cinnamonic soil and loessial soil. The study area is a typical temperate grassland, and the main vegetation types are Stipa. grandis, Stipa. bungeana, Artemisia. gmelinii, Agropyron cristatum, and Heteropappus altaicus et al.
The experiment platform was built in 2017 where as a randomized complete block design with five replicate blocks in the grassland. In each block, three 6 m × 6 m plots were established with a 1 m buffer zone between each plot. A transparent plastic rain sheltering rack (the uniformly laid rain shelter was constructed with PVC with a length of 5 m, a groove width of 30 cm, and a light transmittance of 95%) was adopted to support the collecting bucket and the artifact drip irrigation system to realize real-time collection and redistribution of the natural precipitation in the plots. Rain shelters intercepted half of the ambient precipitation (control) to form a reduced precipitation treatment (DP). The intercepted water was piped to an adjacent plot to form an increased precipitation manipulated (IP). To prevent the interference of soil moisture from the outside, 1-m deep PVC boards were embedded around the perimeter to prevent the surface soil moisture from seeping sideways (Figure 1).
The terminology of Allen et al. (2011) was used as the basis to divide all the plants into the two functional groups: grass (plant species of the Poaceae family) and forb (herbaceous, dicotyledonous broad-leaved plant). Based on a field survey, we found that there had three dominant species that are S. grandis, S. bungeana, and A. gmelinii, which were typical zonal vegetation and the main constructive species in the hilly and gully region on Loess Plateau. The three plants can be divided into two functional groups: grass species (S. grandis and S. bungeana) and forb species (A. gmelinii).
During the initial (May), middle (July), and final (September) stages of the growing season of 2019, we harvested the aboveground biomass of individuals in these plots, the biomass was mixed to form a composite sample, stored in ice boxes, and brought back to the laboratory to measure relevant indexes. The plant δ13C were analyzed by isotope ratio mass spectrometer (IRMS, Thermo-Fisher Scientific, Bremen, Germany) after the samples were dried at 60°C for 72 h. After removing the litter layer, soil samples were collected from each quadrat. In each plot, three soil cores (5 cm diameter) were randomly collected with an “S” shape using a soil drilling sampler (9 cm inner diameter), and the soils were combined to provide one composite soil sample per plot. The soil samples were passed through a 2 mm sieve to remove roots and stones. Then, all soil samples were separated into two parts; one part was used for the determination of soil properties (air-dried and stored at 25°C) and the other part was utilized for soil microbial biomass (stored at 4°C).
Soil moisture (%) and soil temperature (ST, °C) were measured using a soil moisture meter (Takeme-10, Mianyang, China). The soil organic carbon content (SOC) was analyzed using exothermic heating and oxidation using the potassium dichromate method (Nelson and Sommers, 1982). The soil total nitrogen content (TN) was determined using the Kjeldahl method (Bremner, 1996). The soil available nitrogen content (AN) was determined using the alkali-hydrolyzed diffusion method (Stanford, 1982). Soil total phosphorus (TP) and available phosphorus (AP) were determined using melted molybdenum, antimony, and scandium colorimetry (Olsen and Sommers, 1982). Soil nitrate nitrogen (NO3––N) and ammoniacal nitrogen content (NH4+–N) were measured using a continuous flow analytical system (Autoanalyzer 3, Bran and Luebbe, Germany).
Microbial biomass for carbon (MBC), nitrogen (MBN), and phosphorus (MBP) concentrations were analyzed using the chloroform fumigation extraction method (Vance et al., 1987). The specific experimental methods referred to Li J. W. et al. (2019).
The isotopic discrimination in photosynthesis can be described by the model proposed by Farquhar et al. (1989):
Where Ci is the intercellular CO2 concentration, Ca is the ambient CO2 concentration, a (4.4‰) represents the fractionation effect that occurs during CO2 diffusion through the stomata, and b (27‰) represents the discrimination associated with carboxylation. The leaf conductivity used for water vapor loss (gH2O) and CO2 absorption (gCO2) has the following relationship:
In addition, the net photosynthetic rate (A) of the plant has the following relationship with gCO2 (Hietz et al., 2005):
Combined with the above formula, WUE (A/gH2O) can be determined by Δ13C:
So,
where A is the net photosynthesis, g is the stomatal conductance, and 1.6 is the ratio of diffusivities of water and CO2 in air.
Two-way ANOVA were performed to test the main effects of precipitation manipulated, growth stages, and their interactive effects on WUE, soil properties and ecological stoichiometry characteristics of the microbial biomass. Significance was evaluated at the 0.05 level (P < 0.05). We have carried out normality analysis and the test of homogeneity of variance before ANOVA analysis. When significance was observed at the P < 0.05 level, Post Hoc Duncan’s multiple range test was used to carry out the multiple comparisons. Pearson correlation analysis was used to determine the relationship between WUE and abiotic and biotic properties in the soil. Furthermore, a stepwise regression model was applied to detect the main factors affecting soil and microbial properties that affected plant WUE. The structural equation modeling framework was performed using Amos 22.0 to estimate the direct and indirect effects of environmental variables on plant WUE.
Plant WUE was significantly affected by growth stage, precipitation treatment, and their interaction (P < 0.001; Table 1). For S. grandis and S. bungeana, the WUE of the DP was higher than ambient precipitation and IP generally, among them, significant differences were found in the middle growth stage of S. grandis and in the final growth stage of S. bungeana. However, IP decreased WUE, especially in S. bungeana and A. gmelinii (Figure 2). Among the different growth stages, the WUE of the S. grandis, S. bungeana, and A. gmelinii in the initial growth stage was lower than that in the other growth stages.
Table 1. ANOVA (P-value) analysis of the effects of growth stages and precipitation manipulation on plant WUE, soil properties, and microbial biomass ecological stoichiometry characteristics.
Figure 2. Response of plant WUE to precipitation manipulation at different growth stages. CK, ambient precipitation; DP, decrease precipitation (–50%); IP, increase precipitation (+50%); WUE, water use efficiency. Different small letters mean significant difference among different treatments at the 0.05 level; different capital letters mean significant difference among different growing stages at the 0.05 level.
For S. grandis, the factors that were significantly related to WUE were ST, NH4+−N, NO3––N, MBC, and MBC:MBP. For S. bungeana, ST, NH4+–N, MBC, and MBC:MBP were significantly correlated with WUE. The WUE of A. gmelinii was significantly correlated with ST, TN, SOC:TN, NO3––N, MBC, and MBC:MBP (Figure 3). Moreover, the stepwise regression models detected that the WUE of S. grandis was mainly influenced by NO3––N and NH4+–N, ST, NO3––N, and NH4+–N had strong effects on WUE of S. bungeana, and the influencing factors for A. gmelinii were ST, MBC:MBP, NO3––N, AP, and TN (Table 2).
Figure 3. Correlation analysis of factors affecting WUE with precipitation manipulation. CK, ambient precipitation; DP, decrease precipitation (–50%); IP, increase precipitation (+50%). WUE, water use efficiency; SM, soil moisture; ST, soil temperature; SOC, soil organic carbon; TN, soil total nitrogen; TP, soil total phosphorus; SOC:TN, SOC:TN ratio; SOC:TP, SOC:TP ratio; TN:TP, TN:TP ratio; NH4+–N, soil NH4+–N content; NO3––N, soil NO3––N content; AN, soil available nitrogen content; AP, soil available phosphorus content; MBC, microbial biomass carbon; MBN, microbial biomass nitrogen; MBP, microbial biomass phosphorus; MBC:MBN, MBC:MBN ratio; MBC:MBP, MBC:MBP ratio; MBN:MBP, MBN:MBP ratio. Red indicates positive correlation and blue indicates negative correlation. The larger the circle, the greater the absolute value of the correlation coefficient. *P ≤ 0.05.
Table 2. Multiple regression equation between WUE and soil properties and microbial biomass ecological stoichiometry characteristics.
The final structural equation model accurately fitted the data describing the interaction pathways among WUE, soil properties, and microbial biomass ecological stoichiometry in response to altered precipitation (Figure 4).
Figure 4. Structural equation model of the effect of soil properties and microbial biomass ecological stoichiometry on WUE with precipitation manipulation. SM, soil moisture; ST, soil temperature; TN, soil total nitrogen; SOC:TN, soil organic carbon: soil total nitrogen; NH4+–N, soil NH4+–N content; NO3––N, soil NO3––N content; AP, soil available phosphorus content; MBC, microbial biomass carbon; MBN, microbial biomass nitrogen; MBP, microbial biomass phosphorus; MBC:MBN, MBC:MBN ratio; MBC:MBP, microbial biomass carbon: microbial biomass phosphorus ratio. The final model fit the data well: S. grandis (χ2 = 4.96, df = 6, P = 0.55); S. bungeana (χ2 = 5.95, df = 6, P = 0.43); A. gmelinii (χ2 = 12.57, df = 10, P = 0.25). Numbers at arrows are standardized path coefficients. Width of the arrows indicates the strength of the relationships. The red lines indicate positive and significant, the black lines indicate negative significant, and the dashed lines indicate insignificant, respectively; R2 values indicate the proportion of the variance explained for each endogenous variable; result for goodness-of-fit tests are also reported underneath each plot (P > 0.05 indicates a good fit); *P < 0.05; **P < 0.01; ***P < 0.001.
The main factors associated with the WUE of S. grandis and S. bungeana were NO3––N, NH4+–N, ST, MBC, and MBC:MBP, and the final model explained 63 and 56% of the variation in WUE of S. grandis and S. bungeana, respectively. In addition, ST, TN, SOC:TN, AP, NO3––N, MBC, and MBC:MBP played a critical role in the WUE of A. gmelinii, and the final model explained 62% of the variation in WUE. As a driving force, SM indirectly affects WUE by having positive or negative effects on other factors.
Higher WUE indicates generally more dry matter production or C uptake per water loss (Gao et al., 2009). Generally, DP increased WUE and IP decreased WUE (Figure 2). Plants have higher WUE under drought, mainly because the stomatal conductance and transpiration rate of leaves decrease, but the change in net photosynthetic rate is relatively small (Latif et al., 2014). However, it is different in increasing photosynthesis and stomatal conductance of plants when higher SM under the IP conditions. The same increase in precipitation will greatly increase the amount of water available for evaporation, but which has little effect on promoting the photosynthetic rate of plants (Tian et al., 2010), thus reducing the WUE of plants.
Certain studies have reported that plants of different functional group are characterized by diversities in ecological strategies (Davison et al., 2020). Such variable strategies make plants to regulate the ability of resource absorption, utilization and transformation under changing environments, indicating mutable phenotypic plasticity of different function groups (Davison et al., 2020). The unique biological characteristics of grasses and forbs have adopted an underground strategy for storing in infertile soils potentially (Chapin, 1980). In our study, the WUE of A. gmelinii under different precipitation treatments showed no obvious change trend (Figure 2), and generally the WUE was higher in grasses than forbs given its larger leaf area. Although leaves are beneficial for intercepting the maximum light resources, it also means more water loss. Therefore, plant controls water loss through stomatal regulation resulting in a reduction in photosynthetic rate per unit area and thus leading to lower WUE, which showed a trade-off between different functional groups to maximize access to light and control water loss (Reich et al., 1997) and indicated that future vegetation restoration can be dominated by the functional groups with high resource competitiveness and WUE such as grasses in arid and semi-arid regions.
Plants WUE is synchronized with the total primary productivity, which is lower at the beginning and the end of the growing period, but higher at the growth peak (Tang et al., 2015), suggesting WUE varied with plant growth. However, our study showed that the WUE of the plants in the middle and final growth stages was significantly higher than that at the initial stage of growth (Figure 2). The reasons may be that in the middle and final growth stages when the temperature, amount of water evapotranspiration and the water demand of plants is high, the imbalance between water loss caused by vegetation evapotranspiration and the amount of SM replenishment will make the available water more and more short (Reynolds et al., 2004) and increase the plant WUE.
The SM is the main determinant of plant WUE (Law et al., 2010). However, our study found that SM could not adequately explain the change in WUE (Figures 3, 4), indicating that small precipitation events do not effectively replenish the water available to plants in the arid area (Wright et al., 2001). At the same time, the weak correlation between WUE and soil water content may also be caused by the fact that the ecohydrological process mainly depends on the amount of water available in the phenological period, and the supplementation of SM through precipitation does not necessarily coincide with plant demand (Dong et al., 2011). In addition, precipitation has a delayed effect, which means that the effect of supplementation of SM on plants growth and community structure is a cumulative process (Sala et al., 2012; Bunting et al., 2017). Therefore, the effects of precipitation change on plant community may not be reflected if only did one growing season’s study. Therefore, long-term observation data are urgently needed to explore the effects of precipitation changes on the structure and function for plants.
Temperature is also an important factor affecting plant C isotope fractionation, which affect C isotope fractionation by regulating Ci/Ca (Liu and Li, 2007), and affect plant WUE by causing a photosynthetic carboxylase reaction directly. Previous studies have found that WUE decreases with an increase in temperature due to high temperatures limit the stomatal conduction of plants (Ponton et al., 2006). With increasing temperature, the water vapor pressure difference between the inside and outside of the leaves increases and the transpiration of plants becomes intense, leading to a drop of leaf water potential that intensifies the change in transpiration and decreases WUE accordingly. However, our study found that ST had a significant positive effect on WUE (Figure 4), which may be supported by reduced stomatal conductance and high internal resistance of the thick epidermis (Latif et al., 2014). Our results also showed that ST affects plant WUE by affecting soil microbes [i.e., MBC (P < 0.05) and MBC:MBP] indirectly and positively. Soil microbes can form parasitic, symbiotic, or saprophytic relationships with plants directly or by promoting the decomposition of SOM, nutrient transformation, oxidation, nitration and the N biogeochemical processes of soil (van der Heijden et al., 2008). The rise of ST increased the activity of soil microbes, which affected the extracellular enzymes involved in SOM decomposition (Li et al., 2022), the regulation of nutrient cycling and thus affecting plant WUE.
Soil moisture is one of the crucial factors affecting soil nutrient cycling both directly and indirectly. Plants consume excessive amounts of soil water content and nutrients during periods of rapid growth and reproduction, leading to plant growth being gradually limited by N and P (Deng et al., 2021). Our results showed that altered precipitation had a significant effect on TN and TN:TP positively at the initial growth stage (Table 3), because SM accelerates the decomposition of SOM with an increase in precipitation, and leading to the accumulation of soil TN (Cui et al., 2019; Deng et al., 2021). In our study, there was a significant negative correlation between WUE and soil TN of A. gmelinii (Figure 4). More C is often fixed by plants in low-N soil and more biomass per unit of N is produced (high N use efficiency, NUE) (Aerts and Chapin, 2000). Some studies have shown that there is a trade-off between plant WUE and NUE, where plants often decrease their WUE with the increase in NUE (Gong et al., 2011). Physiological constraints in the leaf have been used to explain the trade-off when plants increase intercellular CO2 concentration of leaves by opening their stomata, which increases photosynthesis per unit of N in leaves. However, it will increase transpiration and reducing WUE (Gong et al., 2011). Therefore, reduced WUE by greater water availability frequently increases NUE. Similarly, N in leaf tissue increases when N availability increases (NUE decreases), which promotes the photosynthetic capacity of leaves, thereby increasing WUE (Ripullone et al., 2004).
Table 3. Response of soil physical-chemical properties to precipitation manipulation at different growth stages.
Soil inorganic N mainly resulted from the ammonification and nitrification activities of soil microorganisms, which transform soil organic N into NH4+–N and NO3––N (Chapin et al., 2011). In our study, the NH4+–N and NO3––N showed a decreasing trend under DP conditions (Table 3), which may be related to the reduction in ammonification and nitrification rate. In addition, NH4+–N played a negatively critical role in WUE, but NO3––N had a significant positive effect on WUE (Figure 4). Giese et al. (2011) observed that the pools of NO3––N and NH4+–N were controlled by precipitation. When the precipitation is low, NH4+–N is the main form of inorganic N available, and the content of NO3––N increases with an increase in precipitation. However, NH4+–N is an effective N that is converted from soil organic N by microbial mineralization, which can be absorbed by soil but not easily leached and can be directly absorbed and utilized by plants. Moreover, NO3––N is negatively charged and is not easily absorbed by soil colloids, and as it is easily lost because of leaching with an increase in precipitation, it results in a large loss of N (Deluca et al., 2002). Soil NH4+–N and NO3––N can be absorbed and utilized by plants and affecting the WUE directly.
Soil AP is an important indicator for evaluating the ability of soil to provide P. In our study, AP content increased with increasing precipitation (Table 3). This is because that increased precipitation can improve the utilization rate of P by plants, promote the growth of plant roots, enhances the osmotic regulation ability of plants, and maintain the balance between water absorption and water loss (Gutiérrez-Boem and Thomas, 1998). Moreover, increased precipitation will enhance soil microbial activity and thus accelerate the P cycle rate, which may lead to an increase in AP content. Our results also showed that soil AP had a significant positive effect on the WUE of A. gmelinii, and the reason is probably that AP can improve the water relationship of plants to a certain extent and significantly affect the growth status of plants. In addition, P plays a very important role in the improvement of photosynthesis as it is an important player in the photosynthetic process, and can affect the catalytic rate of Rubisco carboxylase either directly or indirectly, and promote photosynthesis in plants (Pieters et al., 2001).
Changes in precipitation patterns may affect soil microbial biomass and its community structure by changing SM, ST, nutrient, and SOM input from aboveground and underground plant residues either directly or indirectly (Hawkes et al., 2011). Soil microbes are the driving force of SOM decomposition, which are closely related to C, N, and P cycling in soil (Xu et al., 2013). The ratios of microbe C:N:P can be used to determine the nutritional status and limitations of microbial growth (Zhou et al., 2019). In our study, soil microbial biomass was negatively affected by DP treatment generally (Figure 5), suggesting that the decrease in precipitation inhibited the proliferation and turnover of soil microorganisms (Wang et al., 2015). The consumer-driven nutrient cycling theory highlights that the biogeochemical cycle of nutrients can be promoted by microorganisms when these nutrients are required to meet their own needs (Elser and Urabe, 1999). Therefore, a high MBC represents high microbial activities, which reflects the strong ability of material circulation and promote plant growth and development of the ecosystem.
Figure 5. Response of soil microbial biomass C, N, P ecological stoichiometry characteristics to precipitation manipulation at different growth stages. CK, ambient precipitation; DP, decrease precipitation (–50%); IP, increase precipitation (+50%); MBC, microbial biomass carbon; MBN, microbial biomass nitrogen; MBP, microbial biomass phosphorus; MBC:MBN, MBC:MBN ratio; MBC:MBP, MBC:MBP ratio; MBN:MBP, MBN:MBP ratio. Different small letters mean significant difference among different treatments at the 0.05 level; different capital letters mean significant difference among different growing stages at the 0.05 level.
The MBC:MBP reflects the potential of soil microorganisms to regulate soil P availability (Li P. et al., 2019). A low MBC:MBP indicates that P is relatively abundant compared with soil SOC. At the time, microorganisms mineralize SOM to supplement the soil P pool, which further increases the restriction of soil C on microorganisms. A high MBC:MBP indicates that the soil is relatively rich in SOC and limited in P. At this time, microorganisms need to assimilate more P and maintain their normal growth and development, which shows that the ability of P fixation is improved. In our study, MBC and MBC:MBP affected WUE indirectly by affecting soil available nutrients (NH4+–N, NO3––N, and AP). The higher MBC and the greater population of microorganisms influence the content of AN in soil through their N fixation and nitrification activities positively (Leff et al., 2015). Moreover, the release of extracellular enzymes and the decomposition of substrates by microorganisms also affect the supply of nutrients such as AP in the soil (Makino et al., 2010). However, a low MBC:MBP indicates that soil microorganisms have a greater potential to release P through circulation and thus play a significant role in replenishing the soil effective P pool.
Changes in precipitation patterns had significant effects on the WUE of two functional groups in the grassland ecosystem. DP increased the WUE of grass species (S. grandis and S. bungeana) generally, but IP decreased WUE especially in forb species (A. gmelinii). The WUE was higher in grasses than forbs. Moreover, soil hydrothermal conditions, soil nutrients (i.e., NO3––N, NH4+–N, and AP) and soil microbial activities were the main factors affecting the WUE of plants. Among them, ST played a key role. SM affected WUE through changes in ST, soil nutrients, and microbes indirectly. These results suggested that SM is not the main factor determining the change in WUE, but soil available nutrients play a critical role in affecting plant WUE in arid grassland ecosystems. In addition, the functional groups with high resource competitiveness and WUE such as grass species can be selected for vegetation restoration in arid and semi-arid areas. These findings provide a better understanding of the change and response process of plants WUE in grassland ecosystems to altered precipitation pattern, which will reveal the drivers and underlying mechanisms of WUE are crucial to predicting the impact of future climatic change on plant C and water cycling processes.
The raw data supporting the conclusions of this article will be made available by the authors, without undue reservation.
LDe, JPL, ZS, and ZH conceived the ideas and designed the study. XH, JWL, YL, LDo, XW, and WL measured soil nutrients and soil microbial biomass. XH wrote the first draft of the manuscript. All authors contributed critically to the drafts and gave final approval for publication.
This study was supported by the National Natural Science Foundation of China (41730638), the Science and Technology Innovation Program of Shaanxi Academy of Forestry Sciences (SXLK2021-0206), the Major Basic Research Development Program of Shaanxi Province, China (2021ZDLSF05-02), the Funding of Top Young talents of Ten Thousand talents Plan in China (2021), the Funding of Special Support Plan of Young Talents Project of Shaanxi Province (2018) and National Forestry and Grassland Administration in China (20201326015).
The authors declare that the research was conducted in the absence of any commercial or financial relationships that could be construed as a potential conflict of interest.
All claims expressed in this article are solely those of the authors and do not necessarily represent those of their affiliated organizations, or those of the publisher, the editors and the reviewers. Any product that may be evaluated in this article, or claim that may be made by its manufacturer, is not guaranteed or endorsed by the publisher.
Aerts, R., and Chapin, F. (2000). The mineral nutrition of wild plants revisited: a re-evaluation of processes and patterns. Adv. Ecol. Res. 30, 1–67. doi: 10.1016/S0065-2504(08)60016-1
Allen, V. D., Batello, C., Berretta, E. J., Hodgson, J., Kothmann, M., Li, X., et al. (2011). An international terminology for grazing lands and grazing animals. Grass Forage Sci. 66, 2–28. doi: 10.1111/j.1365-2494.2010.00780.x
Bai, Y. J., Zha, T. S., Bourque, C. P. A., Jia, X., Ma, J. Y., Liu, P., et al. (2020). Variation in ecosystem water use efficiency along a southwest-to-northeast aridity gradient in China. Ecol. Indic. 110:105932. doi: 10.1016/j.ecolind.2019.105932
Battipaglia, G., Micco, V. D. E., Brand, W. A., Saurer, M., Aronne, G., Linke, P., et al. (2014). Drought impact on water use efficiency and intra-annual density fluctuations in Erica arborea on Elba (Italy). Plant Cell Environ. 37, 382–391. doi: 10.1111/pce.12160
Bremner, J. (1996). Chloroform fumigation and the release of soil nitrogen: a rapid direct extraction method to measure microbial biomass nitrogen in soil - ScienceDirect. Soil Biol. Biochem. 17, 837–842. doi: 10.1016/0038-0717(85)90144-0
Bunting, E. L., Munson, S. M., and Villarreal, M. L. (2017). Climate legacy and lag effects on dryland plant communities in the south western U.S. Ecol. Indic. 74, 216–229. doi: 10.1016/j.ecolind.2016.10.024
Chapin, F. S. (1980). The mineral nutrition of wild plants. Annu. Rev. Ecol. Syst. 11, 233–260. doi: 10.1146/annurev.es.11.110180.001313
Chapin, F. S., Matson, P. A., and Mooney, H. A. (2011). Principles of Terrestrial Ecosystem Ecology. Berlin: Springer.
Chen, J., Chang, S. X., and Anyia, A. O. (2011). The physiology and stability of leaf carbon isotope discrimination as a measure of water-use efficiency in Barley on the Canadian Prairies. J. Agron. Crop Sci. 197, 1–11. doi: 10.1111/j.1439-037X.2010.00440.x
Cui, Y. X., Fang, L. C., Deng, L., Guo, X. B., Han, F., Ju, W. L., et al. (2019). Patterns of soil microbial nutrient limitations and their roles in the variation of soil organic carbon across a precipitation gradient in an arid and semi-arid region. Sci. Total Environ. 658, 1440–1451. doi: 10.1016/j.scitotenv.2018.12.289
Davison, J., Garcia de Leon, D., Zobel, M., Moora, M., Bueno, C. G., Barcelo, M., et al. (2020). Plant functional groups associate with distinct arbuscular mycorrhizal fungal communities. N. Phytol. 226, 1117–1128. doi: 10.1111/nph.16423
Deluca, T., Nilsson, M. C., and Zackrisson, O. (2002). Nitrogen mineralization and phenol accumulation along a fire chronosequence in northern Sweden. Oecologia 133, 206–214. doi: 10.1007/s00442-002-1025-2
Deng, L., Peng, C. H., Li, J. W., Liu, Y. L., Hai, X. Y., Liu, Q. Y., et al. (2021). Effects of droughts on soil carbon and nitrogen dynamics in natural ecosystems. Earth Sci Rev. 214:103501. doi: 10.1016/j.earscirev.2020.103501
Dong, G., Guo, J. X., Chen, J. Q., Sun, G., Gao, S., Hu, L. J., et al. (2011). Effects of spring drought on carbon sequestration, evapotranspiration and water use efficiency in the Songnen meadow steppe in northeast China. Ecohydrology 4, 211–224. doi: 10.1002/eco.200
Elser, J. J., and Urabe, J. (1999). The stoichiometry of consumer-driven nutrient recycling: theory, observations, and consequences. Ecology 80, 735–751. doi: 10.1890/0012-9658(1999)080[0735:tsocdn]2.0.co;2
Farquhar, G. D., Ehleringer, J. R., and Hubick, K. T. (1989). Carbon isotope discrimination and photosynthesis. Annu. Rev. Plant Phys. 40, 503–537. doi: 10.1146/annurev.pp.40.060189.002443
Gao, L., Yang, J., and Liu, R. X. (2009). Effects of soil moisture levels on photosynthesis, transpiration, and moisture use efficiency of female and male plants of Hippophae rhamnoides ssp.sinensis. Acta Ecol. Sin. 29, 6025–6034.
Giese, M., Gao, Y. Z., Lin, S., and Brueck, H. (2011). Nitrogen availability in a grazed semi-arid grassland is dominated by seasonal rainfall. Plant Soil 340, 157–167. doi: 10.1007/s11104-010-0509-9
Gong, X. Y., Chen, Q., Lin, S., Brueck, H., Dittert, K., Taube, F., et al. (2011). Tradeoffs between nitrogen- and water-use efficiency in dominant species of the semiarid steppe of inner Mongolia. Plant Soil 340, 227–238. doi: 10.1007/s11104-010-0525-9
Gutiérrez-Boem, F. H., and Thomas, G. W. (1998). Phosphorus nutrition affects wheat response to water deficit. Agron J. 90, 166–171. doi: 10.2134/agronj1998.00021962009000020008x
Han, T., Ren, H., Wang, J., Lu, H., Song, G., and Chazdon, R. L. (2020). Variations of leaf eco-physiological traits in relation to environmental factors during forest succession. Ecol Indic. 117:106511. doi: 10.1016/j.ecolind.2020.106511
Hawkes, C. V., Kivlin, S. N., Rocca, J. D., Huguet, V., Thomsen, M. A., and Suttle, K. B. (2011). Fungal community responses to precipitation. Glob. Change Biol. 17, 1637–1645. doi: 10.1111/j.1365-2486.2010.02327.x
Hietz, P., Wanek, W., and Dunisch, O. (2005). Long-term trends in cellulose delta 13C and water-use efficiency of tropical Cedrela and Swietenia from Brazil. Tree Physiol. 25, 745–752. doi: 10.1093/treephys/25.6.745
Kang, L., Han, X., Zhang, Z., and Sun, O. J. (2007). Grassland ecosystems in China: review of current knowledge and research advancement. Philos Trans R Soc Lond B Biol. Sci. 362, 997–1008. doi: 10.1098/rstb.2007.2029
Karatassiou, M., and Noitsakis, B. (2010). Changes of the photosynthetic behaviour in annual C-3 species at late successional stage under environmental drought conditions. Photosynthetica 48, 377–382. doi: 10.1007/s11099-010-0049-9
Latif, A. A., Olivier, N., Stephane, M. O., Langlade, N. B., Thierry, L., Philippe, G., et al. (2014). Genetic control of water use efficiency and leaf carbon isotope discrimination in Sunflower (Helianthus annuus L.) subjected to two drought scenarios. PLoS One 9:e101218. doi: 10.1371/journal.pone.0101218
Law, B. E., Anthoni, P. M., and Aber, J. D. (2010). Measurements of gross and net ecosystem productivity and water vapour exchange of a Pinus ponderosa ecosystem, and an evaluation of two generalized models. Glob. Change Biol. 6, 155–168. doi: 10.1046/j.1365-2486.2000.00291.x
Leff, J. W., Jones, S. E., Prober, S. M., Barberán, A., Borer, E. T., Firn, J. L., et al. (2015). Consistent responses of soil microbial communities to elevated nutrient inputs in grasslands across the globe. Proc. Natl. Acad. Sci. U.S.A. 112, 10967–10972. doi: 10.1073/pnas.1508382112
Li, J. W., Dong, L. B., Liu, Y. L., Wu, J. Z., Wang, J., Shangguan, Z. P., et al. (2022). Soil organic carbon variation determined by biogeographic patterns of microbial carbon and nutrient limitations across a 3, 000-km humidity gradient in China. Catena 209:105849. doi: 10.1016/j.catena.2021.105849
Li, J. W., Liu, Y. L., Hai, X. Y., Shangguan, Z. P., and Deng, L. (2019). Dynamics of soil microbial C:N:P stoichiometry and its driving mechanisms following natural vegetation restoration after farmland abandonment. Sci. Total Environ. 693:133613. doi: 10.1016/j.scitotenv.2019.133613
Li, P., Muledeer, T., Tian, D., and Feng, Z. Z. (2019). Seasonal dynamics of soil microbial biomass carbon, nitrogen and phosphorus stoichiometry across global forest ecosystems. Chin. J. Plant Ecol. 43, 532–542. doi: 10.17521/cjpe.2019.0075
Liu, H. Y., and Li, J. Y. (2007). Application of carbon isotope in revealing environment changes. Chin. Agricult. Sci. Bull. 23, 217–221. doi: 10.3969/j.issn.1000-6850.2007.06.049
Makino, W., Cotner, J. B., Sterner, R. W., and Elser, J. J. (2010). Are bacteria more like plants or animals? Growth rate and resource dependence of bacterial C:N:P stoichiometry. Funct. Ecol. 17, 121–130. doi: 10.1046/j.1365-2435.2003.00712.x
Nelson, D. W., and Sommers, L. E. (1982). “Total carbon, organic carbon and organic matter,” in Methods of Soil Analysis, Part 2, eds A. L. Page, R. H. Miller, and D. R. Keeney (Madison, WI: American Society of Agronomy), 539–579. doi: 10.2134/agronmonogr9.2.2ed.c29
Nielsen, U. N., and Ball, B. A. (2015). Impacts of altered precipitation regimes on soil communities and biogeochemistry in arid and semi-arid ecosystems. Glob. Change Biol. 21, 1407–1421. doi: 10.1111/gcb.12789
Olsen, S. R., and Sommers, L. E. (1982). “Phosphorus: phosphorus soluble in sodium bicarbonate,” in Methods of Soil Analysis, Part 2, Chemical and Microbiological Properties, 2nd Edn, eds A. L. Page, R. H. Miller, and D. R. Keeney (Madison, WI: American Society of Agronomy), 421–422.
Pieters, A. J., Paul, M. J., and Lawlor, D. W. (2001). Low sink demand limits photosynthesis under Pi deficiency. J. Exp. Bot. 52, 1083–1091. doi: 10.1093/jexbot/52.358.1083
Ponton, S., Flanagan, L. B., Alstad, K. P., Johnson, B. G., Morgenstern, K., Kljun, N., et al. (2006). Comparison of ecosystem water use efficiency among Douglasfir forest, aspen forest, and grassland using eddy covariance and carbon isotope techniques. Glob. Change Biol. 12, 294–310. doi: 10.1111/j.1365-2486.2005.01103.x
Reich, P. B., Walters, M. B., and Ellsworth, D. S. (1997). From tropics to tundra: global convergence in plant functioning. Proc. Natl. Acad. Sci. U.S.A. 94, 13730–13734. doi: 10.1073/pnas.94.25.13730
Reynolds, J. F., Kemp, P. R., Ogle, K., and Fernández, R. J. (2004). Modifying the ‘Pulse-Reserve’ paradigm for deserts of North America: precipitation pulses, soil water, and plant responses. Oecologia 141, 194–210. doi: 10.1007/s00442-004-1524-4
Ripullone, F., Lauteri, M., Grassi, G., Amato, M., and Borghetti, M. (2004). Variation in nitrogen supply changes water use efficiency of Pseudotsuga menziesii and Populus x euroamericana; a comparison of three approaches to determine water-use efficiency. Tree Physiol. 24, 671–679. doi: 10.1093/treephys/24.6.671
Sala, O. E., Gherardi, L. A., Reichmann, L., Jobbágy, E., and Peters, D. (2012). Legacies of precipitation fluctuations on primary production: theory and data synthesis. Philos Trans. R. Soc. B. 367, 3135–3144. doi: 10.1098/rstb.2011.0347
Sensuła, B. M. (2015). Spatial and short-temporal variability of δ13C and δ15N and water-use efficiency in pine needles of the three forests along the most industrialized part of Poland. Water Air Soil Poll. 226:362. doi: 10.1007/s11270-015-2623-z
Silva, L. C. R. (2015). From air to land: understanding water resources through plant-based multidisciplinary research. Trends Plant Sci. 20, 399–401. doi: 10.1016/j.tplants.2015.05.007
Sponseller, R. A. (2010). Precipitation pulses and soil CO2 flux in a Sonoran Desert ecosystem. Glob. Change Biol. 13, 426–436. doi: 10.1111/j.1365-2486.2006.01307.x
Stanford, G. (1982). “Assessment of soil nitrogen availability,” in Nitrogen in Agricultural Soils, ed. F. J. Stevenson. doi: 10.2134/agronmonogr22.c17
Tang, X. G., Li, H. P., Griffis, T. J., Xu, X. B., Ding, Z., and Liu, G. H. (2015). Tracking ecosystem water use efficiency of cropland by exclusive use of MODIS EVI data. Remote Sens. 7, 11016–11035. doi: 10.3390/rs70911016
Tian, H. Q., Chen, G. S., Liu, M. L., Zhang, C., Sun, G., Lu, C. Q., et al. (2010). Model estimates of net primary productivity, evapotranspiration, and water use efficiency in the terrestrial ecosystems of the southern United States during 1895–2007. For. Ecol. Manag. 259, 1311–1327. doi: 10.1016/j.foreco.2009.10.009
van der Heijden, M. G. A., Bardgett, R. D., and van Straalen, N. M. (2008). The unseen majority: soil microbes as drivers of plant diversity and productivity in terrestrial ecosystems. Ecol. Lett. 11, 296–310. doi: 10.1111/j.1461-0248.2007.01139.x
Vance, E. D., Brooke, P. C., and Jenkinson, D. S. (1987). An extraction method for measuring soil microbial biomass C. Soil Biol. Biochem. 19, 703–707. doi: 10.1016/0038-0717(87)90052-6
Wang, N., Wang, M. J., Li, S. L., Wang, N. N., Feng, F. J., and Han, S. J. (2015). Effects of precipitation variation on growing seasonal dynamics of soil microbial biomass in broadleaved Korean pine mixed forest. Chin. J. Appl. Ecol. 26, 1297–1305. doi: 10.13287/j.1001-9332.20150302.015
Weltzin, J. F., Loik, M. E., Schwinning, S., Williams, D. G., Fay, P. A., Haddad, B. M., et al. (2003). Assessing the response of terrestrial ecosystems to potential changes in precipitation. Bioscience 53, 941–952. doi: 10.1641/0006-3568(2003)053[0941:atrote]2.0.co;2
Wright, I. J., Reich, P. B., and Westoby, M. (2001). Strategy shifts in leaf physiology, structure and nutrient content between species of high- and low-rainfall and high- and low-nutrient habitats. Funct. Ecol. 15, 423–434. doi: 10.1046/j.0269-8463.2001.00542.x
Xu, X., Thornton, P. E., and Post, W. M. (2013). A global analysis of soil microbial biomass carbon, nitrogen and phosphorus in terrestrial ecosystems. Glob. Ecol. Biogeogr. 22, 737–749. doi: 10.1111/geb.12029
Yang, Y., Guan, H., Batelaan, O., McVicar, T. R., Long, D., Piao, S., et al. (2016). Contrasting responses of water use efficiency to drought across global terrestrial ecosystems. Sci. Rep. 6:23284. doi: 10.1038/srep23284
Keywords: climate change, grassland, precipitation, soil microbe, soil nutrients, soil temperature, water use efficiency
Citation: Hai X, Li J, Li J, Liu Y, Dong L, Wang X, Lv W, Hu Z, Shangguan Z and Deng L (2022) Variations in Plant Water Use Efficiency Response to Manipulated Precipitation in a Temperate Grassland. Front. Plant Sci. 13:881282. doi: 10.3389/fpls.2022.881282
Received: 22 February 2022; Accepted: 28 March 2022;
Published: 19 May 2022.
Edited by:
Daniel Johnson, University of Georgia, United StatesReviewed by:
Romà Ogaya, Ecological and Forestry Applications Research Center (CREAF), SpainCopyright © 2022 Hai, Li, Li, Liu, Dong, Wang, Lv, Hu, Shangguan and Deng. This is an open-access article distributed under the terms of the Creative Commons Attribution License (CC BY). The use, distribution or reproduction in other forums is permitted, provided the original author(s) and the copyright owner(s) are credited and that the original publication in this journal is cited, in accordance with accepted academic practice. No use, distribution or reproduction is permitted which does not comply with these terms.
*Correspondence: Lei Deng, bGVpZGVuZ0Btcy5pc3djLmFjLmNu
Disclaimer: All claims expressed in this article are solely those of the authors and do not necessarily represent those of their affiliated organizations, or those of the publisher, the editors and the reviewers. Any product that may be evaluated in this article or claim that may be made by its manufacturer is not guaranteed or endorsed by the publisher.
Research integrity at Frontiers
Learn more about the work of our research integrity team to safeguard the quality of each article we publish.