- 1Division of Genetics, ICAR-Indian Agricultural Research Institute, New Delhi, India
- 2Division of Plant Pathology, ICAR-Indian Agricultural Research Institute, New Delhi, India
Sheath blight caused by necrotrophic fungus Rhizoctonia solani Kühn is one of the most serious diseases of rice. Use of high yielding semi dwarf cultivars with dense planting and high dose of nitrogenous fertilizers accentuates the incidence of sheath blight in rice. Its diverse host range and ability to remain dormant under unfavorable conditions make the pathogen more difficult to manage. As there are no sources of complete resistance, management through chemical control has been the most adopted method for sheath blight management. In this review, we provide an up-to-date comprehensive description of host-pathogen interactions, various control measures such as cultural, chemical, and biological as well as utilizing host plant resistance. The section on utilizing host plant resistance includes identification of resistant sources, mapping QTLs and their validation, identification of candidate gene(s) and their introgression through marker-assisted selection. Advances and prospects of sheath blight management through biotechnological approaches such as overexpression of genes and gene silencing for transgenic development against R. solani are also discussed.
Introduction
Rice (Oryza sativa L.) serves as the primary diet for approximately 67% of the world population. In the Asian region, the demand for rice production is the highest in the world, due to the increased preference for rice among the population (Mohanty, 2013). Throughout the world, productivity of rice is affected by several biotic and abiotic factors. There are about 50 different biotic factors that can cause potential yield loss in rice including fungi, bacteria, viruses, nematodes and insects. Of the disease-causing organisms, fungal pathogens impose a greater challenge in sustaining rice production (Webster and Gunnell, 1992).
Among the fungal diseases causing significant yield loss in rice, sheath blight is ranked the second most important after rice blast (Pan et al., 1999). The sheath blight pathogen has two stages, Rhizoctonia solani Kühn, the anamorph stage and a teleomorph stage, Thanatephorus cucumeris (Frank) Donk. Belonging to the division Basidiomycota, R. solani is a necrotrophic fungus that produces sclerotia of varying sizes but with uniform texture, which can remain dormant for many years (Mukherjee, 1978). The disease causes a yield reduction ranging from 20 to 50% depending on the severity of infection (Groth and Bond, 2007; Margani and Widadi, 2018). In the recent past, sheath blight has become a major threat, especially under intensive rice cultivation. Monoculture of high-yielding semi-dwarf rice varieties, heavy doses of nitrogenous fertilizers and the favorable micro-environment facilitated by the crop density are implicated as the major factors favouring the sharp increase in the disease incidence (Savary et al., 1995; Cu et al., 1996). Reported for the first time in Japan in 1910 (Miyake, 1910), sheath blight disease had spread all across the world. R. solani is a very destructive pathogen. Taking advantage of the large host range (Kozaka, 1965), the pathogen often survives on the alternate hosts during hostile conditions, making the disease very difficult to manage. Besides, it can also survive in soil and dead plant debris by producing resting structures such as sclerotia.
To incite the disease in rice plants, the fungal inoculum should come in contact with the live host tissues in the field. The inoculum can be a runner hypha or a sclerotium and in rare cases basidiospores, often floating in the irrigation water. By this mode, inoculum can travel and spread to different locations in the field or from the irrigation canals where alternate hosts can supply sufficient inoculum. In rice, R. solani can infect the plant at any growth stage (Dath, 1990). The incidence of sheath blight is more severe in early maturing, semi-dwarf, highly tillering and compact cultivars (Bhunkal et al., 2015b). The disease severity and incidence increase with plant age (Singh et al., 2004). The resistance and susceptibility in the rice genotypes are distinct in mature plants as compared to seedlings (Dath, 1990). The sheath blight progression is slow in initial growth stages, while it is fast at tillering and later stages of growth (Thind et al., 2008).
Although several cultural, chemical and biological control strategies have been suggested to manage sheath blight disease of rice (Yellareddygari et al., 2014; Datta and Vurukonda, 2017), chemical control has been the most widely used method so far. However, this method is relatively less sustainable in crop production because of the increased cost of production, development of fungicide tolerance and apprehensions of residual toxicity. Biological strategies targeting host plant resistance have been advocated as the most viable solution, which includes mapping of gene(s) or quantitative trait loci (QTLs) governing disease resistance and introgression to elite cultivars through molecular breeding. Additionally, novel biotechnological approaches like RNAi, transgenics and genome-editing approaches can also be used to generate a new resistance spectrum against R. solani. There are several reviews made previously on the sheath blight tolerance in rice, but most of which provide relatively less focus on breeding for resistance. In the present review, we have made a comprehensive update on the understanding of the pathophysiology of R. solani keeping in view crop varietal improvement and biological management of the sheath blight disease in rice. The review also summarizes a critical analysis of the pathogen diversity, host range, pathogenicity and genetics of rice plant resistance. Various approaches adopted in managing the disease through development of resistant varieties have also been described including the novel biotechnological approaches.
Diversity of R. Solani
Morphological Diversity Based on Anastomosis of Vegetative Hyphae
Anastomosis is a key process for a large number of filamentous fungi that facilitates the fusion of cell walls, cytoplasm and nucleus between genetically similar groups. An anastomosis group (AG) is a collection of closely related isolates grouped based on the ability of vegetative hyphae to anastomose/fuse with one another (Parmeter et al., 1969). R. solani is classified into different AGs based on their hyphal capability to fuse with tester hyphal mycelium (Carling, 1996; Craven et al., 2008). The fungus is assigned with fourteen different AGs starting from AG1 to AG13 and AGB1 as a bridging group. The 14 AGs exhibit wide variation in morphology of mycelial colony, nutritional requirement, host range and pathogenic virulence (Carling et al., 2002a,b; Ajayi-Oyetunde and Bradley, 2018). The anastomosis grouping of R. solani causing sheath blight of rice indicated that it belonged to AG1 group. Further grouping of AGs into different intraspecific subgroups (ISGs) have been carried out based on their DNA sequence and its homology, colony morphology, pathogenicity, isozyme pattern, rDNA-internal transcribed sequences and fatty acid composition. Classification of AG1 resulted in three subgroups, AG1-IA, AG1-IB, and AG1-IC, all causing blight (Ogoshi, 1987; Sneh et al., 1991; Carling, 1996). Among these, majority of the rice sheath blight pathogen belongs to the AG1-IA subgroup.
Genetic Variability in R. Solani
Considerable morphological, pathogenic and genetic diversity has been established within R. solani isolates obtained from different parts of the world (Shu et al., 2014; Yugander et al., 2015). Taheri et al. (2007) could group a set of 150 isolates of R. solani collected from different parts of India into 33 groups at an 80% genetic similarity level using amplified fragment length polymorphism markers. Twenty-nine isolates from Bangladesh were grouped into two clusters by Ali et al. (2004) while Moni et al. (2016) grouped 18 isolates into four clusters. However, there was no significant correlation between virulence variation and genetic groups identified based on random amplified polymorphic DNA (RAPD) markers (Yi et al., 2002). In China, 175 isolates of R. solani belonging to AG1-IA showed considerable variability in virulence (Wang et al., 2015c). They could classify the isolates into weakly virulent, moderately virulent and highly virulent classes based on disease severity, which represented 28.0, 63.4 and 8.6% of isolates, respectively. Further establishing the genetic variability, as many as 80 alleles were detected using RAPD markers from 25 R. solani isolates collected from different geographic regions of India (Singh et al., 2015). The number of alleles per locus varied from 1 to 7.
Initially, the genome size of R. solani was estimated to be between 36.9 and 42.5 Mb with 11 chromosomes ranging in size from 0.6 to 6 Mb (Keijer, 1996). Later, a draft genome sequence of R. solani AG1-IA strain with a size of 36.94 Mb was released using next-generation sequencing technology (Zheng et al., 2013). Subsequently, another draft genome sequence of R. solani AG1-IA strain, 1802/KB (GenBank accession number KF312465) isolated from a popular rice variety from Malaysia, was generated with a size of 28.92 Mb (Nadarajah et al., 2017). Besides, a web-based database, RSIADB was constructed using the genome sequence (10489 genes) and annotation information for R. solani AG1-1A to analyze its draft genome and transcriptome (Chen et al., 2016).
Host Range
Rhizoctonia solani is pathogenic against a diverse range of about 250 host plant species belonging to members of Poaceae, Fabaceae, Solanaceae, Amaranthaceae, Brassicaceae, Rubiaceae, Malvaceae, Asteraceae, Araceae, Moraceae, and Linaceae (Chahal et al., 2003). As many as 188 plant species belonging to 32 families were found to be infected by this fungus in Japan (Kozaka, 1961). Tsai (1974) reported R. solani infection in 20 species of 11 families in Taiwan, while it was found to infect 10 types of grasses and a Cyperus spp. in Thailand (Dath, 1990). In India, it has been reported on 62 economically important plants and 20 families of weeds (Roy, 1993). Several weed plant species have been identified to act as collateral hosts for the pathogen in absence of rice plants (Acharya and Sengupta, 1998), and serve as inoculum and aid in further spread of the disease (Kannaiyan and Prasad, 1980; Srinivas et al., 2014).
Disease Symptoms
On infection, the fungus causes a range of symptoms including sheath blight, foliar blight, leaf blight, web-blight, head rot, bottom rot and brown patch in different crops. In rice, R. solani mainly attacks the leaf sheath and leaf blades and in severe cases, the whole plant including the emerging panicles may be affected (Rangaswami and Mahadevan, 1998). The disease symptoms on the infected plant can be visualized within 24–72 h after infection depending on the environmental conditions. Although the disease can occur at any growth phase, rice crop is most vulnerable at the tillering phase (Singh et al., 1988). Fungal mycelium determines the size and shape of lesions which are produced in patches of varying sizes (Ou et al., 1973). The typical symptom (Figure 1) is the appearance of greenish-gray water-soaked lesions on the leaf sheath near the water level that are circular, oblong or ellipsoid and about 1 cm long. These lesions enlarge and attain irregular shape, the center of which becomes gray white with brown margins. Lesions may appear on any part of the sheath and several lesions may coalesce to encircle the whole stem. Under favorable conditions, the infection may spread to upper leaf sheaths and leaf blades, which ultimately results in the rotting of leaf sheath and drying up of the whole leaf. In severe cases, the infection spreads to the panicle affecting grain filling and leading to the discoloration of seeds with brownish-black spots or black to ashy gray patches (Singh et al., 2016). In acute cases, the disease causes the death of the whole leaf, tiller and even the whole plant. At the field level, the infection usually affects the plants in a circular pattern referred to as ‘bird’s nest’ (Hollier et al., 2009).
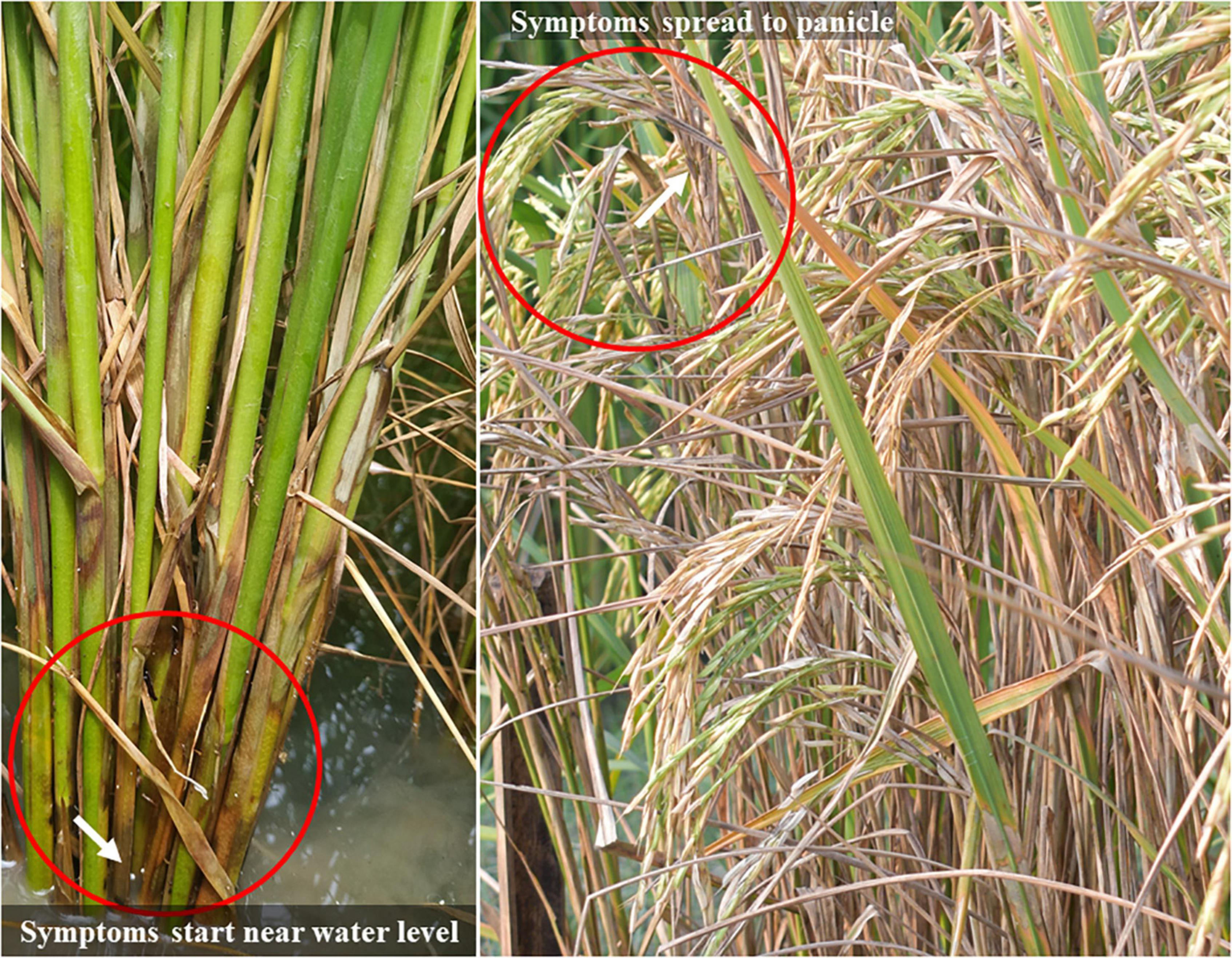
Figure 1. Symptom of sheath blight disease in rice; left side shows the initial symptoms appear on leaf sheath starting from water level, and the right side shows the disease spread up to panicle.
The Disease Cycle
Rhizoctonia solani is a seed- and soil-borne pathogen, which survives through sclerotia and mycelia in infected seeds or soil in tropical environments. In soil, infected plant debris is the major carrier that may arise from rice or weed hosts (Figure 2). In temperate regions, soil and crop residue borne sclerotia act as the primary source of inoculum, which can spread through irrigation water from one field to another (Kozaka, 1970). Under favorable conditions, the sclerotia germinate to form mycelia, which on establishing contact with the rice plant surface grows and produces infection structures such as infection cushions and lobate appressoria. These infection structures aid mycelial penetration into the plant tissues. However, in some cases, infection occurs through stomata, where no infection structures are observed (Marshall and Rush, 1980). The pathogen spreads both vertically and horizontally with a horizontal spread of up to 20 cm/day under field conditions is reported (Savary et al., 1995). Plant to plant and field to field spread of the disease takes place through floating sclerotia and mycelia dispersed through rainfall and irrigation water runoff. Infected seeds are the primary source of inoculum for the spread of this disease to new areas. The seed infection and transmission of the pathogen from seed to seedlings in the form of lesions varies from 4.6–14.0% under field conditions (Sivalingam et al., 2006). Wind also helps in the secondary spread of the disease by dispersing the basidiospores to new fields. The basidia hymenium acts as a continuous source of secondary inoculum.
GEOGRAPHICAL DISTRIBUTION OF R. SOLANI
Since its first report in Japan in 1910, the pathogen has spread to most of all the rice growing areas in the world (Figure 3). This disease is recognized as a serious problem in the top ten rice growing countries viz. China, India, Indonesia, Bangladesh, Vietnam, Thailand, Burma, Philippines, Pakistan and Brazil (Singh et al., 2016). Incidence of sheath blight disease of rice in India was reported for the first time from Gurdaspur in Punjab (Paracer and Chahal, 1963). Later on, the disease has become a major problem in rice producing areas of eastern Uttar Pradesh, Uttarakhand, Bihar, West Bengal, Haryana, Odisha, Chhattisgarh, Tamil Nadu, Kerala, Karnataka, Andhra Pradesh, Jammu and Kashmir, Madhya Pradesh, Assam, Tripura and Manipur. The disease incidence was particularly severe among the high yielding semi-dwarf rice varieties, owing to their narrow genetic base, high dependency on chemical fertilizers and favorable weather. Due to the widespread incidence, economic losses to the tune of up to 58% in rice yield have been reported (Chahal et al., 2003).
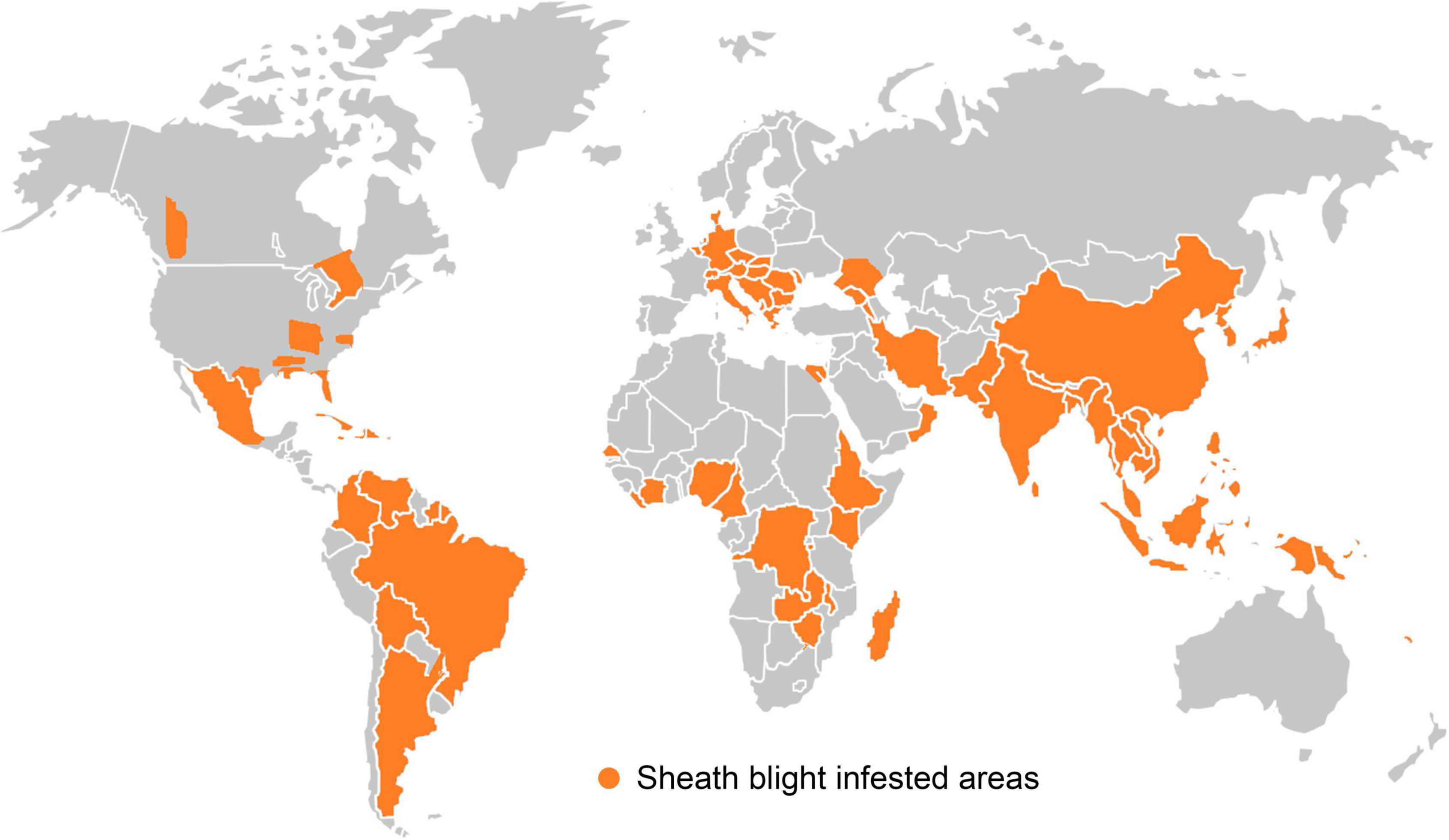
Figure 3. Geographical distribution of sheath blight disease occurrence in different countries of the world.
Pre-disposing Factors Affecting the Epidemiology
High ambient air temperature in combination with high relative humidity in the forenoon and wet leaves are major predisposing factors for sheath blight development in rice (Castilla et al., 1996; Biswas et al., 2011). Favorable temperature and evaporation rate results in 23.0 and 61.1% of disease incidence under field conditions, respectively (Lenka et al., 2008). The maximum progression of the disease is observed at the temperature range of 25°–30°C and relative humidity of 80–100% (Thind et al., 2008; Bhunkal et al., 2015a). The disease severity and yield loss increase with excess nitrogen application (Tang et al., 2007), and are accentuated in the presence of brown plant hopper and rice root-knot nematode, Hirschmaniella oryzae (Dath, 1990) and rice tungro virus (Sarkar and Chowdhury, 2007). Another factor under which severe incidence is seen is when the crop canopy is dense with high contact frequency between tissues (Huang et al., 2007). There is also a difference seen between the disease incidence among two sub-species of rice, indica and japonica, with the former having relatively higher tolerance than japonica. However, Lee and Rush (1983) reported that japonica cultivars with short and medium grains have higher resistance than long grain indica rice cultivar from the southern United States. Indicating the importance of nitrogen, Dath (1990) found a reduction in disease severity with the use of slow-release nitrogenous fertilizer such as Crotonylidene diurea (CDU) and Guanyl urea phosphate with the solo application of silica, phosphorus and potash. Increased dose of nitrogen and phosphorus reduces the incubation period as well as phenolic contents, leading to high disease severity, while application of K, Zn, S, and Fe reduce disease severity (Prasad et al., 2010). Application of soil amendments including neem cake, farm yard manure (FYM), vermicompost and rice husk (Senapoty, 2010) and spraying Ganoderma diethyl ester formulation (Sajeena et al., 2008) can reduce the disease incidence. Long-term field experiments revealed that R. solani sclerotia population and sheath blight disease severity remained low in conventional seeded plots as compared to stale seedbeds and no-till seedbeds (Cartwright et al., 1997). Minimal tillage also promotes sheath blight development (Rodriguez et al., 1999). Besides, the rate of infection was less in direct-seeded rice than in transplanted rice irrespective of spacing. Certain crop cycles can also influence the disease incidence pattern as seen with soybean in rotation with rice which leads to a heavy incidence of sheath blight (Rodriguez et al., 2003; Groth and Bond, 2007).
Host-Pathogen Interaction Between Rice and R. solani
To colonize and establish the disease in rice plants, R. solani employs a variety of tactics. Effector proteins are used by pathogens to infect the host plant and cause disease. R. solani is known to produce several effector molecules (Table 1) with varying functions enabling successful colonization. The primary requirement for R. solani infection is the degradation of the plant cell wall. R. solani AG1-IA is predicted to produce as many as 223 carbohydrate-active enzymes (CAZymes) such as glycoside hydrolases, glucosyltransferases, and polysaccharide lyases (Zheng et al., 2013). Polygalacturonase hydrolyses the pectin in the plant cell wall, which results in cell death (Chen et al., 2017). During the infection process, the pathogen secretes oxalate and transgenic rice plants overexpressing oxalate oxidase break oxalate and enhance resistance against sheath blight (Molla et al., 2013). R. solani has also been reported to use α-1,3-glucans to mask the chitin on its surface and evade the host defense mechanism (Fujikawa et al., 2012). When an extracellular signal is received, the fungi activate different signal transduction pathways for pathogenicity. One of them is the membrane-bound heterotrimeric guanine nucleotide-binding (G) protein-mediated signaling (Li et al., 2007). The Gα subunit of G protein upon activation regulates downstream effectors, such as adenylate cyclase, phospholipase, ion transporters, and mitogen-activated protein kinase (MAPK) involved in various biological processes including pathogenicity (Neves et al., 2002). Li et al. (2007) reported that two G proteins (Gβ and Gγ) regulate pathogenesis by monitoring the adenylate cyclase and MAP kinase pathway. Rga1, a Gα subunit gene, affects pathogenicity and its disruption decreased vegetative growth and pathogenicity of the rice sheath blight pathogen (Charoensopharat et al., 2008). The genome sequence of R. solani AG1-IA revealed that a group of secondary molecules including G protein-coupled receptors (GPCR), G protein subunits, MAPK pathway, cAMP pathway and calcium–calcineurin pathway genes may play a major role in pathogenesis (Zheng et al., 2013).
When a pathogen attacks a plant, the plant uses various pathways and defense mechanisms to prevent it from colonizing. On infection by R. solani, rice plants respond by activating various signaling pathways and producing antimicrobial compounds. The plant immune system is of two types, PTI (PAM- pathogen associated molecular triggered immunity) and ETI (effector-triggered immunity). PTI is the first line of defense in plants, which is initiated when pattern recognition receptors (PRRs) recognize non-self molecular patterns from pathogens. PTI induces a relatively weak immune response that restricts colonization by invading organisms. ETI, the second line of defense, is initiated when a cognate resistance (R) protein directly or indirectly recognizes highly variable pathogen molecules called avirulence (Avr) effectors and induces a hypersensitive reaction (Liu W. et al., 2014). Pathogenesis related proteins (PR proteins) are produced by the host plant only in pathological or related stress situations. PR3 and PR4 families of chitinases that hydrolyze the β-1,4 linkages between N-acetylglucosamine residues of chitin, a structural polysaccharide of the cell wall of R. solani are differentially induced in rice plants. Chitin fragments are recognized by LysM receptor-like proteins (Gust et al., 2012). POC1, a cationic pathogen-induced peroxidase is upregulated in rice on R. solani infection (Taheri and Tarighi, 2010). Most PRs are induced by the action of salicylic acid (SA), Jasmonic acid (JA), or ethylene (ET), and possess antimicrobial activities. A JA-deficient rice mutant, Hebiba, exhibited enhanced susceptibility to the sheath blight disease (Taheri and Tarighi, 2010). It was found that transgenic plants overexpressing WRKY30 could improve disease resistance by accumulating more JA and conferred resistance to sheath blight by activating the JA/ET signaling cascade. Transcriptome analysis of sheath blight resistant and susceptible rice cultivars infected with R. solani led to the identification of 7624 differentially expressed genes (DEGs), mainly associated with cell wall, β-glucanase, respiratory burst, phenylpropanoids and lignin (Yuan et al., 2018; Molla et al., 2020).
Management of Sheath Blight Disease
Currently, sheath blight disease of rice is largely managed through the use of fungicides, utilization of genetic resistance/tolerance, cultural practices and biological control are also strategically adopted in the integrated management. Although rice germplasm shows diverse responses to R. solani infection, yet, none of the rice varieties, landraces, weedy types or wild relatives have been identified as immune or completely resistant to this disease. However, some of the genotypes have been found to be partially resistant.
Chemical Control
In the absence of effective host plant resistance against sheath blight pathogen in rice, the management of sheath blight disease is mainly carried out through the use of chemicals (Naik et al., 2017). Foliar spray and seed treatment are the most popular method of fungicidal application against R. solani. Even though both systemic and non-systemic fungicides are used for chemical management, systemic fungicides offer better management of this disease (Naik et al., 2017). Timely application of selective fungicides between panicle differentiation and heading stage offers effective protection against this disease. Periodical monitoring of the rice field and application of fungicides at the initial stages of infection especially at booting stage is recommended for managing sheath blight in susceptible varieties (Singh et al., 2016; Uppala and Zhou, 2018).
Several chemical formulations are in use for the control of sheath blight in rice (Table 2). The major focus in the development has been on the identification of fungicides with novel target sites and diverse modes of action. Presently, the Strobilurin group of systemic fungicides are the most preferred chemical group to manage sheath blight disease in rice (Yellareddygari et al., 2014). Strobilurin group of fungicides are derivatives of β-methoxy acrylates and are obtained from forest-grown wild mushrooms (Strobilurus tenacellus). Azoxystrobin from this group is very effective for not only controlling the disease but also found to enhance yield as well (Groth and Bond, 2007). Triazole fungicides are also commonly used in sheath blight management. Application of other chemicals such as Flutolanil, Carbendazim, Iprobenfos, Mancozeb, Thifluzamide and Validamycin also offers effective control of this disease.
The use of a single chemical with the same mode of application for a prolonged time leads to the evolution of resistance in the fungus (Uppala and Zhou, 2018). Hence, a combinatory chemical formulation such as Azoxystrobin 18.2% + Difenoconazole 11.4% (Bhuvaneswari and Raju, 2012; Kumar et al., 2018); Propiconazole + Difenoconazole (Kandhari, 2007); Prothioconazole + Tebuconazole 240 g/kg SC (Chen et al., 2021). Captan 70% + Hexaconazole 5% (Pramesh et al., 2017); Trifloxystrobin 25% + Tebuconazole 50% (Shahid et al., 2014; Rashid et al., 2020); Carbendazim + Mancozeb (Prasad et al., 2006; Kumar et al., 2013); Carbendazim 25% + Flusilazole 12.5% SE (Sanjay et al., 2012) etc., are recommended to manage the disease. The chemical method of control is applicable for all areas, irrespective of varieties and has an advantage in a reduction in disease occurrence, spread and enhance yield. However, it has several disadvantages such as environmental hazards that could deteriorate soil health, and cause groundwater pollution. The toxic residue may enter the food chain affecting the health of both humans and animals. It is difficult for a new chemical to have a balancing role in disease management and environmental safety. Therefore, the use of non-chemical control options like cultural, biological, and development and use of resistant varieties offers a viable solution to sheath blight management.
Cultural Practices
Historical records on varietal susceptibility, prior disease incidence, prevailing weather conditions and disease spread help in devising appropriate cultural practices for managing sheath blight disease of rice (Singh et al., 2019). Agro-morphological traits of rice including plant height, stem thickness and tiller angle, length and width of flag leaf, days to heading and planting density affect the susceptibility of rice to R. solani.
Plant height has been found to show a strong negative association between relative lesion length (Willocquet et al., 2012). Wider spacing reduces the sheath blight severity by improving the canopy thickness. Split application and use of slow-releasing nitrogenous fertilizers have been found to reduce sheath blight infection (Roy, 1986). The effect of dose of nitrogen fertilizer on disease spread has been higher than the effect of plant density (Zhang et al., 1995). Similar to nitrogen, higher doses of phosphorous fertilizers increase the disease incidence, while potassic fertilizers have been found to reduce it (Sarkar et al., 1991). Silicon application to rice fields through carbonized rice husk helps delay the disease spread without any negative effect on yield (Sabes et al., 2020). A waste product from charcoal production (Bamboo tar) was reported to inhibit multiple diseases including rice sheath blight (Maliang et al., 2021). Timely removal of weeds which are alternate host for R. solani, removal of plant debris, crop rotation with non-host crops reduces the sheath blight incidence by minimizing the primary inoculum sclerotia (Singh et al., 2019).
Biological Control
In addition to chemical and cultural control, biological control has been suggested as a very promising strategy to manage necrotrophic fungus. Plant extracts or botanicals are very effective in managing the disease. Extracts from garlic, ginger, neem leaf and clove inhibit more than 80% mycelial growth in R. solani (Chakrapani et al., 2020; Rajeswari et al., 2020). Microbial antagonism is a common property found between microorganisms and it is most predominant among soil microbes. This effect of antagonism between the pathogen and beneficial microbes in the soil will lead to a reduction in disease development to a greater extent. There are several biocontrol agents (BCAs) belonging to actinomycetes, fungi and bacteria. Actinomycetes colonize the plant roots and represent a greater portion of the rhizosphere microflora. Actinomycetes against R. solani in tomatoes could reduce the disease incidence by up to 63% (Singh et al., 2017). One of the most common actinomycetes, Streptomyces spp. is reported to reduce the growth of R. solani up to 50% and disease suppression up to 53.3% (Patil et al., 2010). Ethyl acetate extracted from Streptomyces diastatochromogenes, KX852460 have been found to inhibit mycelial growth, reduce sclerotia formation and suppress lesion length on R. solani AG3 (Ahsan et al., 2019). Another group of potential BCAs mostly used against Rhizoctonia is fungal antagonists. Many species of Trichoderma, Corticium, Aspergillus and Gliocladium have been used for managing sheath blight disease (Chinnaswami et al., 2021). For effective management, these BCAs are applied as a soil treatment, foliar spray and root dipping of seedlings. Different strains of Trichoderma have been reported to inhibit Rhizoctonia growth by up to 71% and reduce the sheath blight infestation by up to 59% (Mishra et al., 2020). Trichoderma can be applied alone or in combination with other BCAs like Vesicular arbuscular mycorrhiza, Pseudomonas and yeasts for both controlling the pathogen and supplementing growth factors (Mathivanan et al., 2005; Mohammed et al., 2020). Plant growth-promoting rhizobacteria (PGPR) are the most common group of bacterial BCAs used against a wide range of plant pathogens for disease reduction. PGPR also helps in increasing root growth, phosphate solubilization, nitrogen uptake, iron-chelating siderophores and phytohormone synthesis. Among the different PGPR, Pseudomonas and Bacillus provide an effective way of systemic resistance against sheath blight. Rice seedlings treated with different strains of Pseudomonas fluorescence helped to increase the chitinase activity responsible for the suppression of sheath blight disease (Radjacommare et al., 2004). Bacillus sp. having a broad range of antibiotic properties was also very useful in reducing the growth of Rhizoctonia (Abbas et al., 2019; Raj et al., 2019). The combination of Bacillus subtilis strain MBI600 with Azoxystrobin helps not only disease suppression but also increases the yield to 14% (Zhou et al., 2021). In a recent study, three strains of nitrogen-fixing cyanobacteria have been reported to significantly inhibit the growth of R. solani (Zhou et al., 2020). However, the effectiveness of BCAs in sheath blight is influenced by their ability to survive, multiply and control pathogens and also provide additional supplements promoting rice growth. Nanoparticles of Gold and Silver have antifungal activity against R. solani (Das and Dutta, 2021). Recently, silver nanoparticles from rice leaf extract have been reported to be very effective against R. solani infection in rice (Kora et al., 2020). Different biocontrol agents were screened against sheath blight for their timing of application in a greenhouse environment, treatment of these bio fungicides before pathogen inoculation has a great role against the disease (Tuyen and Hoa, 2022). Eugenol from clove (Syzygium aromaticum L.) has been found to control this pathogen by dehydrating the cell and increasing the cell membrane permeability (Zhao et al., 2021).
Crop Improvement Strategies Against R. Solani
Theoretically breeding for sheath blight resistance is mainly based on two approaches, disease escape and disease resistance. Disease escape mainly consists of plant architectural traits including plant height, heading date and stem thickness (Sattari et al., 2014; Susmita et al., 2019). The standard protocol for screening for disease resistance is based on relative lesion height (RLH) which is calculated in the percentage of ratio lesion height to plant height (Sharma et al., 1990; IRRI, 1996). Conventional breeding is more difficult in this case because of the direct influence of plant height on RLH during its screening protocol. Hence marker assisted breeding is highly preferred for the introgression of identified resistance QTLs. Marker assisted breeding has several advantages over conventional breeding as it helps in accurate selection of desired genotypes, saves time during selection, reduces linkage drag during introgression of genomic regions and helps in easier gene pyramiding.
Donors for Resistance
Development of resistant rice varieties through genetic improvement is a sustainable option for managing plant diseases. Since there are no genotypes with absolute resistance, identification of reliable resistance sources must be confined to the moderate to high levels of tolerance in the germplasm. There are several such genotypes reported (Table 3) that are being used in breeding sheath blight resistant cultivars. Among the cultivated species, the indica cultivars are reported to show better resistance than the japonica type (Liu et al., 2009; Willocquet et al., 2012). Additionally, some accessions of wild species such as O. rufipogon, O. nivara, O. meridionalis and O. barthii have been reported to be resistant to sheath blight disease (Prasad and Eizenga, 2008; Bashyal et al., 2017).
Genetics and Analysis of Quantitative Resistance
Several earlier studies indicate that the tolerance against sheath blight disease in rice is a quantitative trait governed by polygenes (Xu et al., 2011; Koshariya et al., 2018). Therefore, it is essential to map the genomic regions governing quantitative variation for tolerance among the source germplasm. Attempts on mapping quantitative trait loci (QTLs) have been taken up in rice for sheath blight tolerance. One of the earliest attempts by Li et al. (1995) used RFLP markers in an F4 population derived from Lemont/Teqing. Lemont was a highly susceptible japonica cultivar, while Teqing was a semidwarf high yielding Chinese indica variety with high tolerance to leaf blight. Since then a large number of QTLs governing resistance to sheath blight disease have been reported across all the 12 chromosomes of the rice genome (Table 4). A map showing the physical location of the reported QTLs and the linked markers is presented in Figure 4. Most of the earlier mapping populations were based on the partially resistant indica genotypes such as Teqing and Jasmine 85 and the susceptible japonica genotype, Lemont (Li et al., 1995; Pan et al., 1999; Wen et al., 2015). Using these mapping populations, a large number of QTLs governing sheath blight resistance have been mapped (Li et al., 1995; Zou et al., 2000; Liu et al., 2009; Eizenga et al., 2015). Eizenga et al. (2013, 2015) also have identified resistance sources from wild accessions of O. nivara and O. meridionalis. QTLs for resistance have been mapped from weedy rice also (Goad et al., 2020; Jia et al., 2022). Goad et al. (2020) reported four QTLs from RIL populations generated by crossing the rice cultivar, Dee-Geo-Woo-Gen (DGWG) with two weed species (straw hull and black hull awned). Yuan et al. (2019) utilized a RIL population from Lemont/Yangdao4 to map 128 minor effect QTLs, most of which clustered around 17 stable loci across the rice genome.
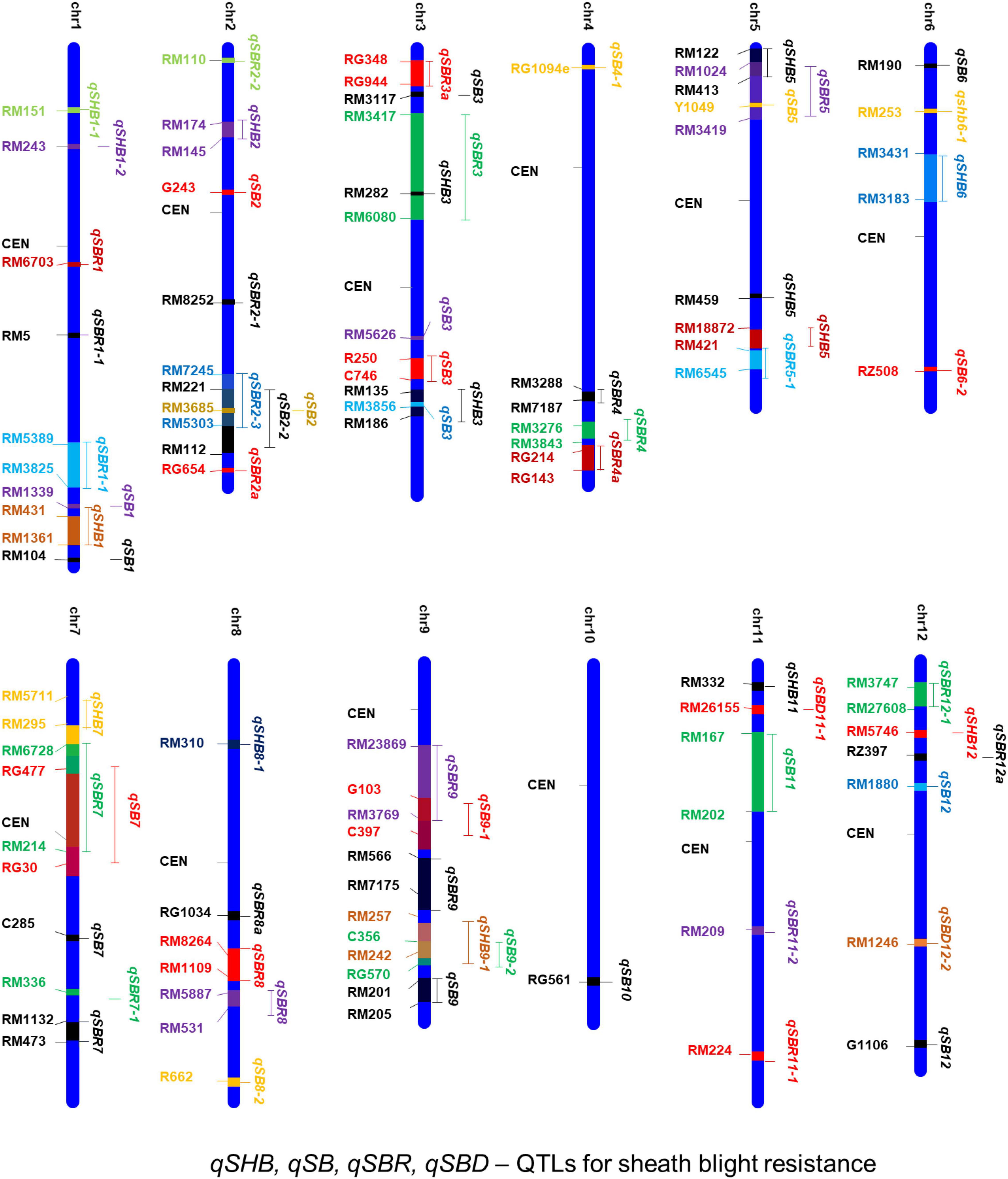
Figure 4. A consolidated chromosomal map showing the QTLs mapped and the markers linked to sheath blight resistance in rice. qSHB, qSB, qSBR, and qSBD indicate the quantitative trait loci for sheath blight disease resistance.
Genome Wide Association Studies
Identification of genomic regions associated with sheath blight resistance has also been carried out using genome wide association studies but on a limited scale. Jia L. et al. (2012) identified 10 marker-trait associations (MTAs) and three genotypes for resistance from a set of 217 core entries from USDA using 155 genome-wide simple sequence repeat (SSR) markers. Using a larger population of 456 rice accessions, Sun et al. (2014) identified 10 significant MTAs with 144 SSR markers. Chen Z. et al. (2019) reported 11 MTAs and two QTLs, qSB3 and qSB6 by screening 299 rice varieties with 44K SNPs. GWAS with 228 rice accessions genotyped with 700,000 SNPs identified two major MTAs associated with sheath blight resistance in rice (Oreiro et al., 2020). Zhang et al. (2019) identified 562 MTAs for lesion height, 134 for culm length and 75 MTAs for relative lesion height through GWAS on a set of 563 rice accessions genotyped with 220,335 SNPs. GWAS was conducted using 259 diverse verities and identified a regulation model against the disease (Wang et al., 2021).
Fine Mapping of QTLs
Although a large number of major and minor effect QTLs have been identified for sheath blight resistance in rice, efforts to fine map these QTLs have been limited. Chromosome segment substitution lines (CSSLs) are a group of homozygous lines, each having a different chromosome segment from the donor species. Individually one CSSL has a donor segment that overlaps the other donor segment in the next CSSL. Altogether, CSSLs contain the whole genomic DNA of donor species in different segment-wise. The CSSLs eliminate the genetic background effect, and enables, the fine mapping of QTLs (Eshed and Zamir, 1994). Channamallikarjuna et al. (2010) fine mapped a major QTL, qSBR11-1 for sheath blight resistance, which has been narrowed down to 0.85 Mb on chromosome 11. A set of 154 putative genes have been identified within this genomic region, out of which 11 chitinase genes in tandem repeats have been identified as candidate genes governing resistance to sheath blight disease. A major QTL qSB-11LE identified from the first QTL mapping effort (Li et al., 1995) and subsequent studies (Zou et al., 2000; Tan et al., 2005) has been fine mapped to a 78.8 kb genomic region, from which three candidate genes have been identified (Zuo et al., 2013). qSB-9TQ from Teqing has also been fine mapped to a region of 146 kb region using CSSLs (Zuo et al., 2014b).
Marker Assisted Breeding for Sheath Blight Resistance in Rice
Mapping and validation of QTLs are essential for their utilization in marker assisted breeding. Teqing is one of the most frequent donors for the QTLs, qSB7TQ, qSB9TQ and qSB12TQ. Marker assisted introgression of single or multiple of these QTLs were found to reduce the yield loss due to sheath blight disease (Wang et al., 2012; Chen Z. X. et al., 2014). Sheath blight resistance has been enhanced by the introgression of QTL, qSB9TQ along with QTL for tiller angle, TAC1TQ (Zuo et al., 2014a). Yin et al. (2008) introgressed three main effect QTLs namely, qSB7TQ, qSB9TQ and qSB11LE into Lemont to develop sheath blight resistant genotypes. In India, Tetep has been widely used as a donor source for both sheath blight as well as blast resistance. A major QTL, qSBR11-1 using ‘Tetep’ was introgressed along with another gene, Pi54 governing blast resistance into a bacterial blight resistant Basmati rice variety, ‘Improved Pusa Basmati 1’ leading to the development of improved near isogenic lines (NILs) with resistance to virulent strains of R. solani (Singh et al., 2012). qSBR11-1 and Pi54 have been pyramided into the high yielding variety, CO51 (Senthilvel et al., 2021). Gene(s) for multiple diseases resistance including bacterial leaf blight (xa5 + xa13 + Xa21), Blast (Pi54) and sheath blight (qSBR7-1 + qSBR11-1 + qSBR11-2) have been pyramided into the background of popular cultivar ASD 16 and ADT 43 using, Tetep and IRBB60 as donors (Ramalingam et al., 2020). Raveendra et al. (2020) introgressed sheath blight resistance from Tetep into the background of bacterial blight resistant genotypes, CB14004 and CB14002.
Biotechnological Approaches for Managing Sheath Blight Diseases of Rice
Comparison of transcripts between resistant and susceptible cultivars in response to Rhizoctonia led to the identification of Ethylene-insensitive protein 2, trans-cinnamate-4- monooxygenase and WRKY 33 transcriptome factor (Shi et al., 2020). Rice is endowed with resources and techniques enabling the study of the expression of these pathogen-related (PR) genes, anti-fungal genes and master genes for defense response affecting R. solani growth.
In the absence of stable sources of sheath blight resistance, genetic engineering offers promise in developing novel resistance in rice. Several potential genes from various species have been identified as candidates for engineering resistance against Rhizoctonia solani in rice (Table 5). Chitinase and glucanase are the most widely used genes for engineering resistance against R. solani. Lin et al. (1995) were the first to generate a transgenic line with constitutive expression of a chitinase gene (Chi11) leading to resistance to sheath blight disease of rice. Since then, many studies have demonstrated the effect of overexpression of the chitinase gene in rice. Chitinase cleaves at the β-1,4-glycosidic linkage of N-acetyl-D-glucosamine and glucanase cleaves at the β-1,3 linkage of glucan polymer, arresting the fungal invasion of the host tissues. Recent studies on overexpression of genes like WRKY13 (Lilly and Subramanian, 2019), OsBR2 (Maeda et al., 2019), RGB1 and RGG1 (Swain et al., 2019), LPA1 (Sun et al., 2019a,2020) and OsGSTU5 (Tiwari et al., 2020) have demonstrated the effectiveness of these genes in managing sheath blight of rice. Overexpression of the genes from the WRKY gene family namely, OsWRKY4 (Wang et al., 2015a), OsWRKY13 (Lilly and Subramanian, 2019), OsWRKY30 (Peng et al., 2012) and OsWRKY80 (Peng et al., 2016) have been reported to reduce R. solani infection in rice. A schematic representation of genes being utilized in the development of transgenics with resistance to sheath blight disease along with their mode of action is presented in Figure 5. Constitutive expression of Chi11 and β-1,3 glucanase genes in a transgenic line, Pusa Basmati-CG27, helped to validate their role in conditioning sheath blight resistance, based on which these genes were used in marker assisted improvement of White Ponni (Kannan et al., 2017). Over expression of a basic helix–loop–helix transcription factor (OsbHLH057) with cis-acting AATCA has been reported to be effective against both sheath blight and drought (Liu et al., 2022). Recently, Dauda et al. (2022) identified a set of Cytokinin glucosyltransferases (CGTs) in rice with the plant secondary product glycosyltransferases (PSPG) motif of 44-amino-acid consensus sequence characteristic of plant uridine diphosphate (UDP)-glycosyltransferases (UGTs), the validation of which showed upregulation of four genes namely LOC_Os07g30610.1, LOC_Os04g25440, LOC_Os04g25490, and LOC_Os04g25800 specifically under R. solani infection.
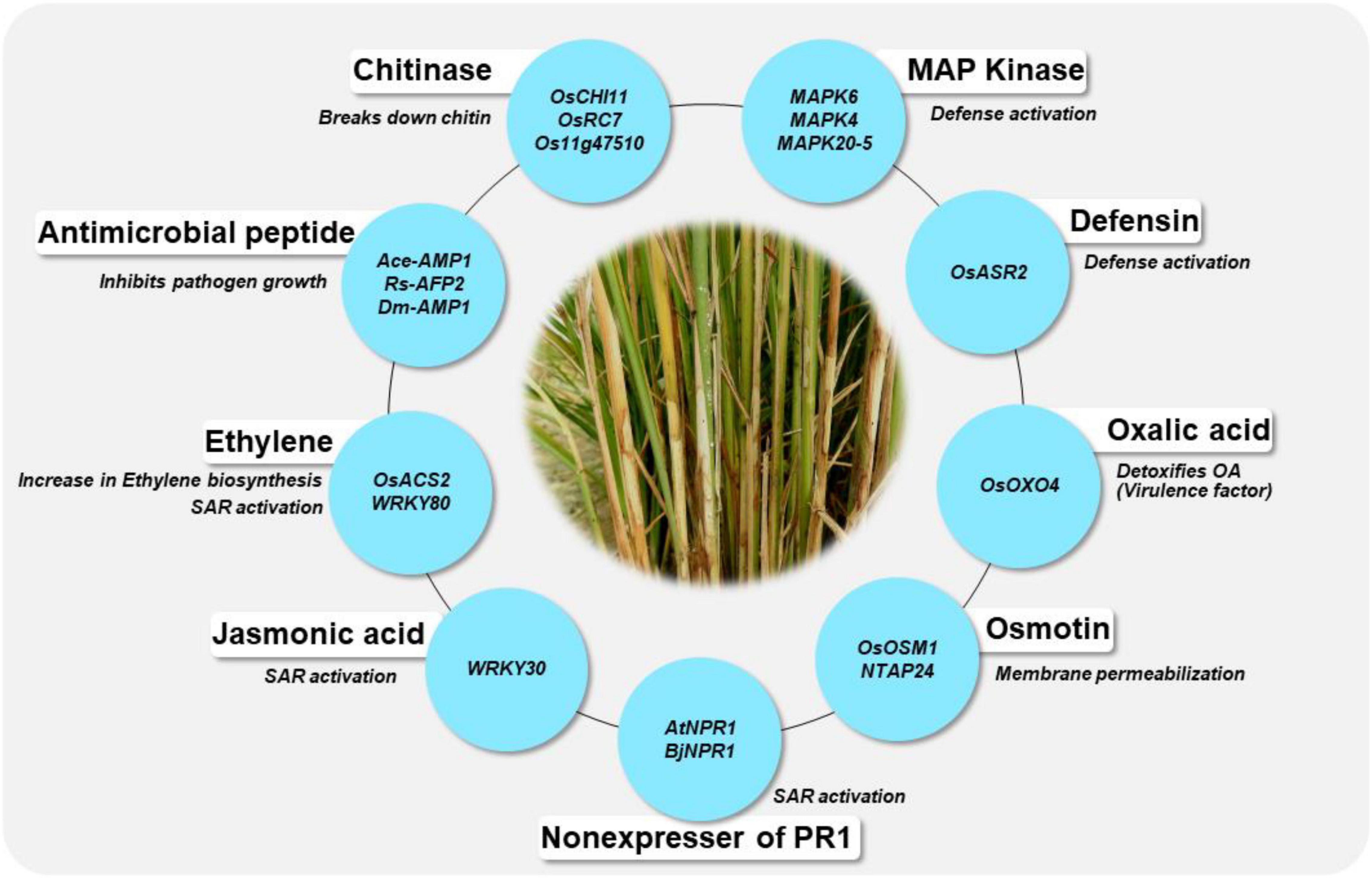
Figure 5. Genes are being utilized for the development of transgenics and their mode of action in conferring resistance to sheath blight disease of rice. The blue circle indicates the genes, the details of their mode of action are given in Table 5.
Small RNAs (siRNAs and miRNAs) play a major role in regulating several processes in plants by switching genes on and off leading to resistance to biotic/abiotic stresses. Host-induced gene silencing or RNA interference (RNAi) strategy has been utilized against Rhizoctonia by targeting pathogenicity linked MAP kinase genes (Tiwari et al., 2017) and polygalacturonase genes (Rao et al., 2019). Overexpression of a siRNA (SiR109944) targeting a gene, F-Box domain and LRR-containing protein 55, has been found to increase the susceptibility of rice to sheath blight disease (Qiao et al., 2020). An ethylene signaling gene, EIL1 has been found to positively regulate sheath blight resistance in rice (Sun et al., 2019b). Transcriptome analysis has revealed that the upregulation of genes controlling cytoskeleton, membrane integrity, and glycolytic pathway plays a major role in disease resistance (Samal et al., 2022). It is recently reported that lauric acid has a role against R. solani by modifying fatty acid metabolism leading to apoptosis (Wang et al., 2022).
Conclusion
Sheath blight is one of the diseases of major concern in rice with the potential to upset rice production and productivity. The causal agent, R. solani is a dynamic pathogen with a wide host range which enables it to overwinter and survive. R. solani has many anastomosis groups, among which AG1-IA is important as the rice sheath blight pathogen. Because of its versatility, the pathogen is very difficult to manage. Chemical control has been the most commonly used approach for management, which is not only environmentally unsafe but also leads to the evolution of novel virulent strains of the pathogen. Although there are other approaches such as cultural practices, and biological control to reduce the disease severity, utilizing host plant resistance is the most sustainable approach for managing this fungal disease. However, rice lacks absolute resistance to rice sheath blight, therefore moderate to high level of tolerance should be tapped as the source of resistance. There have been efforts to map QTLs among the tolerant lines, and many of them have been utilized in marker assisted breeding. However, the progress in molecular breeding has been slow as compared to other major diseases such as bacterial blight and blast diseases where effective genes have been widely available. Standard method of screening for sheath blight disease is based on relative lesion height (RLH) as given by IRRI. This RLH is directly influenced by plant height. Therefore, there is a need to develop a new method for screening against the disease with appropriate standardization. The breeding for sheath blight resistance also needs to focus on utilizing the QTLs through marker assisted introgression into popular cultivars. Several genes have been identified and some of them have been functionally characterized in rice and from other plant species, which provides an opportunity for the development of transgenics as well as genome-editing to create novel variations for managing the sheath blight of rice.
Data Availability Statement
The original contributions presented in the study are included in the article/supplementary material, further inquiries can be directed to the corresponding author/s.
Author Contributions
SK, AS, and KV proposed the idea. BB, MS, HB, and PB outlined the review. MS, AT, NS, and PC collected the materials and prepared the draft. RE, BB, SK, and KV edited the manuscript. All authors read and confirmed the final manuscript.
Funding
This work was supported by the Department of Biotechnology (DBT), Govt. of India, through the research entitled “Imparting sheath blight disease tolerance in rice” (NO. BT/NIPGR/flagship-Prog/2018-19).
Conflict of Interest
The authors declare that the research was conducted in the absence of any commercial or financial relationships that could be construed as a potential conflict of interest.
Publisher’s Note
All claims expressed in this article are solely those of the authors and do not necessarily represent those of their affiliated organizations, or those of the publisher, the editors and the reviewers. Any product that may be evaluated in this article, or claim that may be made by its manufacturer, is not guaranteed or endorsed by the publisher.
References
Abbas, A., Khan, S. U., Khan, W. U., Saleh, T. A., Khan, M. H. U., Ullah, S., et al. (2019). Antagonist effects of strains of Bacillus spp. against Rhizoctonia solani for their protection against several plant diseases: alternatives to chemical pesticides. C. R. Biol. 342, 124–135. doi: 10.1016/j.crvi.2019.05.002
Acharya, S., and Sengupta, P. K. (1998). Collateral hosts of rice sheath blight fungus Rhizoctonia solani. Oryza 35, 89–90.
Ahsan, T., Chen, J., Zhao, X., Irfan, M., Ishaq, H., and Wu, Y. (2019). Action mechanism of Streptomyces diastatochromogenes KX852460 against Rhizoctonia solani AG-3 involving basidiospores suppression and oxidative damage. Iran. J. Sci. Technol. Trans. A Sci. 43, 2141–2147. doi: 10.1007/s40995-019-00733-1
Ajayi-Oyetunde, O. O., and Bradley, C. A. (2018). Rhizoctonia solani: taxonomy, population biology and management of Rhizoctonia seedling disease of soybean. Plant Pathol. 67, 3–17. doi: 10.1111/ppa.12733
Ali, M. A., Kamal, M. M., Archer, S. A., Buddie, A., and Rutherford, M. (2004). Anastomosis and DNA fingerprinting of the rice isolates of Rhizoctonia solani Kühn using AFLP markers. Bangladesh J. Plant Pathol. 20, 1–8.
Ansari, M. M., Sharma, A., and Thangal, M. H. (1989). Evaluation of rice cultures against sheath blight. J. Andaman Sci. Assoc. 5, 89–90.
Bag, M. K., Yadav, M., and Mukherjee, A. K. (2016). Bio efficacy of strobilurin based fungicides against rice sheath blight disease. Transcriptomics 4, 1–2. doi: 10.4172/2329-8936.1000128
Bashyal, B. M., Rawat, K., Singh, D., Krishnan, S. G., Singh, A. K., Singh, N. K., et al. (2017). Screening and identification of new sources of resistance to sheath blight in wild rice accessions. Indian J. Genet. Plant Breed. 77, 341–347. doi: 10.5958/0975-6906.2017.00046.3
Bhaktvatsalam, G., Satyanarayana, K., Reddy, P. K., and John, V. T. (1978). Evaluation of sheath blight resistance in rice. Int. Rice Res. Newslett. 3, 9–10.
Bhunkal, N., Singh, R., and Mehta, N. (2015b). Assessment of losses and identification of slow blighting genotypes against sheath blight of rice. J. Mycol. Plant Pathol. 45, 285–292.
Bhunkal, N., Singh, R., and Mehta, N. (2015a). Progression and development of sheath blight of rice in relation to weather variables. J. Mycol. Plant Pathol. 45, 166–172.
Bhuvaneswari, V., and Raju, K. S. (2012). Efficacy of new combination fungicide against rice sheath blight caused by Rhizoctonia solani (Kühn). J. Rice Res. 5, 57–61.
Biswas, B., Dhaliwal, L. K., Chahal, S. K., and Pannu, P. P. S. (2011). Effect of meteorological factors on rice sheath blight and exploratory development of a predictive model. Indian J. Agric. Sci. 81, 256–260.
Borthakur, B. K., and Addy, S. K. (1988). Screening of rice (Oryza sativa) germplasm for resistance to sheath blight (Rhizoctonia solani). Indian J. Agric. Sci. 58, 537–538.
Cao, W., Zhang, H., Zhou, Y., Zhao, J., Lu, S., Wang, X., et al. (2022). Suppressing chlorophyll degradation by silencing OsNYC3 improves rice resistance to Rhizoctonia solani, the causal agent of sheath blight. Plant Biotechnol. J. 20, 335–349. doi: 10.1111/pbi.13715
Carling, D. E. (1996). “Grouping in Rhizoctonia solani by hyphal anastomosis reaction,” in Rhizoctonia Species: Taxonomy, Molecular Biology, Ecology, Pathology and Disease Control, eds B. Sneh, S. Jabaji-Hare, S. Neate, and G. Dijst (Dordrecht: Springer), 37–47. doi: 10.1007/978-94-017-2901-7_3
Carling, D. E., Baird, R. E., Gitaitis, R. D., Brainard, K. A., and Kuninaga, S. (2002a). Characterization of AG-13, a newly reported anastomosis group of Rhizoctonia solani. Phytopathology 92, 893–899. doi: 10.1094/PHYTO.2002.92.8.893
Carling, D. E., Kuninaga, S., and Brainard, K. A. (2002b). Hyphal anastomosis reactions, rDNA-internal transcribed spacer sequences, and virulence levels among subsets of Rhizoctonia solani anastomosis group-2 (AG-2) and AG-BI. Phytopathology 92, 43–50. doi: 10.1094/PHYTO.2002.92.1.43
Cartwright, R. D., Parsons, C. E., Ross, W. J., Eason, R., Lee, F. N., and Templeton, G. E. (1997). Effect of tillage system on sheath blight of rice. Res. Ser. Ark. Exp. Station 460, 245–250.
Castilla, N. P., Leano, R. M., Elazegui, F. A., Teng, P. S., and Savary, S. (1996). Effects of plant contact inoculation pattern, leaf wetness regime and nitrogen supply on the efficiency in rice sheath blight. J. Phytopathol. 144, 187–192. doi: 10.1111/j.1439-0434.1996.tb01512.x
Chahal, K. S., Sokhi, S. S., and Rattan, G. S. (2003). Investigations on sheath blight of rice in Punjab. Indian Phytopathol. 56, 22–26.
Chakrapani, K., Sinha, B., Chanu, W. T., Chakma, T., and Siram, T. (2020). Assessing in vitro antifungal activity of plant extracts against Rhizoctonia solani causing sheath blight of rice (Oryza sativa L). J. Pharmacogn. Phytochem. 9, 1497–1501.
Channamallikarjuna, V., Sonah, H., Prasad, M., Rao, G. J. N., Chand, S., Upreti, H. C., et al. (2010). Identification of major quantitative trait loci qSBR11-1 for sheath blight resistance in rice. Mol. Breed. 25, 155–166. doi: 10.1007/s11032-009-9316-5
Charoensopharat, K., Aukkanit, N., Thanonkeo, S., Saksirirat, W., Thanonkeo, P., and Akiyama, K. (2008). Targeted disruption of a G protein α subunit gene results in reduced growth and pathogenicity in Rhizoctonia solani. World J. Microbiol. Biotechnol. 24, 345–351. doi: 10.1007/s11274-007-9476-6
Che, K., Zhan, Q., Xing, Q., Wang, Z., Jin, D., He, D., et al. (2003). Tagging and mapping of rice sheath blight resistant gene. Theor. Appl. Genet. 106, 293–297. doi: 10.1007/s00122-002-1001-6
Chen, C., Kun, Z., Tao, D., Jianguo, F., Jin, Y., Zhen, H. E., et al. (2021). Development of prothioconazole + tebuconazole 240 g/kg SC and its control effect on rice sheath blight and wheat sharp eyespot in the field. Chin. J. Pest. Sci. 23, 578–586. doi: 10.16801/j.issn.1008-7303.2021.0050
Chen, L., Ai, P., Zhang, J., Deng, Q., Wang, S., Li, S., et al. (2016). RSIADB, a collective resource for genome and transcriptome analyses in Rhizoctonia solani AG 1 IA. Database 2016:baw031. doi: 10.1093/database/baw031
Chen, X., Lili, L., Zhang, Y., Zhang, J., Ouyang, S., Zhang, Q., et al. (2017). Functional analysis of polygalacturonase gene RsPG2 from Rhizoctonia solani, the pathogen of rice sheath blight. Eur. J. Plant Pathol. 149, 491–502. doi: 10.1007/s10658-017-1198-5
Chen, X., Wang, L., Zuo, S., Wang, Z., Chen, Z., Zhang, Y., et al. (2009). Screening of varieties and isolates for identifying interaction between host and pathogen of rice sheath blight. Acta Phytopathol. Sin. 39, 514–520.
Chen, X. J., Chen, Y. W., Zhang, L., He, Z., Huang, B. L., Chen, C., et al. (2019). Amino acid substitutions in a polygalacturonase inhibiting protein (OsPGIP2) increases sheath blight resistance in rice. Rice 12:56. doi: 10.1186/s12284-019-0318-6
Chen, Y., Yao, J., Yang, X., Zhang, A. F., and Gao, T. C. (2014). Sensitivity of Rhizoctonia solani causing rice sheath blight to fluxapyroxad in China. Eur. J. Plant Pathol. 140, 419–428. doi: 10.1007/s10658-014-0477-7
Chen, Z., Feng, Z., Kang, H., Zhao, J., Chen, T., Li, Q., et al. (2019). Identification of new resistance loci against sheath blight disease in rice through genome-wide association study. Rice Sci. 26, 21–31. doi: 10.1016/j.rsci.2018.12.002
Chen, Z. X., Zhang, Y. F., Feng, F., Feng, M. H., Jiang, W., Ma, Y. Y., et al. (2014). Improvement of Japonica rice resistance to sheath blight by pyramiding qSB-9TQ and qSB-7TQ. Field Crops Res. 161, 118–127. doi: 10.1016/j.fcr.2014.03.003
Chinnaswami, K., Mishra, D., Miriyala, A., Vellaichamy, P., Kurubar, B., Gompa, J., et al. (2021). Native isolates of Trichoderma as bio-suppressants against sheath blight and stem rot pathogens of rice. Egypt. J. Biol. Pest Control 31:12. doi: 10.1186/s41938-020-00356-4
Chu, J., Xu, H., Dong, H., and Xuan, Y. H. (2021). Loose plant architecture 1-interacting kinesin-like protein KLP promotes rice resistance to sheath blight disease. Rice 14:60. doi: 10.1186/s12284-021-00505-9
Craven, K. D., Vélëz, H., Cho, Y., Lawrence, C. B., and Mitchell, T. K. (2008). Anastomosis is required for virulence of the fungal necrotroph Alternaria brassicicola. Eukaryot. Cell 7, 675–683. doi: 10.1128/EC.00423-07
Crill, P., Nuque, F. L., Estrada, B. A., and Bandong, J. M. (1982). “The role of varietal resistance in disease management,” in Evolution of Gene Rotation Concept for Rice Blast Control, ed. IRRI (Los Banos: Int. Rice Res. Institute), 103–121.
Cu, R. M., Mew, T. W., Cassman, K. G., and Teng, P. S. (1996). Effect of sheath blight on yield in tropical, intensive rice production system. Plant Dis. 80, 1103–1108. doi: 10.1094/pd-80-1103
Das, A., and Dutta, P. (2021). Antifungal activity of biogenically synthesized silver and gold nanoparticles against sheath blight of rice. J. Nanosci. Nanotechnol. 21, 3547–3555. doi: 10.1166/jnn.2021.18996
Das, K., Datta, K., Sarkar, S. N., and Datta, S. K. (2021). Expression of antimicrobial peptide snakin-1 confers effective protection in rice against sheath blight pathogen, Rhizoctonia solani. Plant Biotechnol. Rep. 15, 39–54. doi: 10.1007/s11816-020-00652-3
Das, N. P. (1970). Resistance of some improved varieties of rice (Oryza sativa L.) to sheath blight caused by Rhizoctonia solani Kühn. Indian J. Agric. Sci. 40, 566–568.
Dath, A. P. (1990). Sheath Blight of Rice and its Management. Shidipura: Associated Publishing Co, 129.
Datta, A., and Vurukonda, S. S. K. P. (2017). Rice sheath blight: a review of the unsung fatal disease. Trends Biosci. 10, 9216–9219.
Datta, K., Tu, J., Oliva, N., Ona, I. I., Velazhahan, R., Mew, T. W., et al. (2001). Enhanced resistance to sheath blight by constitutive expression of infection-related rice chitinase in transgenic elite indica rice cultivars. Plant Sci. 160, 405–414. doi: 10.1016/S0168-9452(00)00413-1
Dauda, W. P., Shanmugam, V., Tyagi, A., Solanke, A. U., Kumar, V., Krishnan, S. G., et al. (2022). Genome-wide identification and characterisation of Cytokinin-O-Glucosyltransferase (CGT) genes of rice specific to potential pathogens. Plants 11:917. doi: 10.3390/plants11070917
De Yuan, P., Xu, X. F., Hong, W. J., Wang, S. T., Jia, X. T., Liu, Y., et al. (2020). Transcriptome analysis of rice leaves in response to Rhizoctonia solani infection and reveals a novel regulatory mechanism. Plant Biotechnol. Rep. 14, 559–573. doi: 10.1007/s11816-020-00630-9
Dey, S., Badri, J., Prakasam, V., Bhadana, V. P., Eswari, K. B., Laha, G. S., et al. (2016). Identification and agro-morphological characterization of rice genotypes resistant to sheath blight. Australas. Plant. Pathol. 45, 145–153. doi: 10.1007/s13313-016-0404-9
Dubey, A. K., Pandian, R. T. P., Rajashekara, H., Singh, V. K., Kumar, G., Sharma, P., et al. (2014). Phenotyping of improved rice lines and landraces for blast and sheath blight resistance. Indian J. Genet. Plant Breed. 74, 499–501. doi: 10.5958/0975-6906.2014.00876.1
Eizenga, G. C., Jia, M. H., Pinson, S. R., Gasore, E. R., and Prasad, B. (2015). Exploring sheath blight quantitative trait loci in a Lemont/O. meridionalis advanced backcross population. Mol. Breed. 35:140. doi: 10.1007/s11032-015-0332-3
Eizenga, G. C., Prasad, B., Jackson, A. K., and Jia, M. H. (2013). Identification of rice sheath blight and blast quantitative trait loci in two different O. sativa/O. nivara advanced backcross populations. Mol. Breed. 31, 889–907. doi: 10.1007/s11032-013-9843-y
Eshed, Y., and Zamir, D. (1994). A genomic library of Lycopersicon pennellii in L. esculentum: a tool for fine mapping of genes. Euphytica 79, 175–179. doi: 10.1007/bf00022516
FRAC (2021). Fungal control agents sorted by cross resistance pattern and mode of action. Fungicide Resistance Action Committee, Basel, Switzerland. Available online at: https://www.frac.info/docs/default-source/publications/frac-code-list/frac-code-list-2021–final.pdf (accessed April 15, 2022).
Fu, D., Chen, L., Yu, G., Liu, Y., Lou, Q., Mei, H., et al. (2011). QTL mapping of sheath blight resistance in a deep-water rice cultivar. Euphytica 180, 209–218. doi: 10.1007/s10681-011-0366-5
Fujikawa, T., Sakaguchi, A., Nishizawa, Y., Kouzai, Y., Minami, E., Yano, S., et al. (2012). Surface alpha-1,3-glucan facilitates fungal stealth infection by interfering with innate immunity in plants. PLoS Pathog. 8:e1002882. doi: 10.1371/journal.ppat.1002882
Gaihre, Y. R., Yamagata, Y., Yoshimura, A., and Nose, A. (2015). Identification of QTLs involved in resistance to sheath blight disease in rice line 32R derived from Tetep. Trop. Agric. Dev. 59, 154–160. doi: 10.11248/jsta.59.154
Gao, Y., Xue, C. Y., Liu, J. M., He, Y., Mei, Q., Wei, S., et al. (2021). Sheath blight resistance in rice is negatively regulated by WRKY53 via SWEET2a activation. Biochem. Biophys. Res. Commun. 585, 117–123. doi: 10.1016/j.bbrc.2021.11.042
Gao, Y., Zhang, C., Han, X., Wang, Z. Y., Ma, L., Yuan, D. P., et al. (2018). Inhibition of OsSWEET11 function in mesophyll cells improves resistance of rice to sheath blight disease. Mol. Plant Pathol. 19, 2149–2161. doi: 10.1111/mpp.12689
Ghosh, S., Gupta, S. K., and Jha, G. (2014). Identification and functional analysis of AG1-IA specific genes of Rhizoctonia solani. Curr. Genet. 60, 327–341. doi: 10.1007/s00294-014-0438-x
Goad, D. M., Jia, Y., Gibbons, A., Liu, Y., Gealy, D., Caicedo, A. L., et al. (2020). Identification of novel QTL conferring sheath blight resistance in two weedy rice mapping populations. Rice 13:21. doi: 10.1186/s12284-020-00381-9
Gokulapulan, C., and Nair, M. C. (1983). Field screening of sheath blight and rice root nematode. Int. Rice Res. Newslett. 8:4.
Groth, D. E., and Bond, J. A. (2007). Effects of cultivars and fungicides on rice sheath blight, yield, and quality. Plant Dis. 91, 1647–1650. doi: 10.1094/PDIS-91-12-1647
Gust, A. A., Willmann, R., Desaki, Y., Grabherr, H. M., and Nürnberger, T. (2012). Plant LysM proteins: modules mediating symbiosis and immunity. Trends Plant Sci. 17, 495–502. doi: 10.1016/j.tplants.2012.04.003
Han, P. Y., Xing, Z. Y., Chen, X. Z., Gu, L. S., Pan, B. X., Chen, L. X., et al. (2002). Mapping QTLs for horizontal resistance to sheath blight in an elite rice restorer line, Minghui 63. Acta Genet. Sin. 29, 622–626.
Helliwell, E. E., Wang, Q., and Yang, Y. (2013). Transgenic rice with inducible ethylene production exhibits broad spectrum disease resistance to the fungal pathogens Magnaporthe oryzae and Rhizoctonia solani. Plant Biotechnol. J. 11, 33–42. doi: 10.1111/pbi.12004
Hollier, C. A., Rush, M. C., and Groth, D. E. (2009). Sheath Blight of Rice. Louisiana Plant Pathology Disease Identification and Management Series. Online Publication 3123, LSU AgCentre Research & Extension, Baton Rouge, Lousiana. Available online at: https://www.lsuagcenter.com/NR/rdonlyres/C93A494B-8105-4804-9DFA-81190EC3F68B/58166/pub3123ShealthBlightofRiceHIGHRES.pdf. (accessed April 15, 2022).
Huang, S. W., Wang, L., Wang, Q. Y., Tang, S. Q., E-Zhi, G., and Wang, L. (2007). Disease and insect pest resistance and agronomic traits of rice variety ZH 5 with sheath blight resistance. Chin. J. Rice Sci. 21, 657–663.
IRRI (1996). Standard Evaluation System for the INGER Genetic Resource Center, 4th Edn. ed. J. S. Nanda (Endfield, NH: Science Publishers, Inc).
Jha, S., and Chattoo, B. B. (2009). Transgene stacking and coordinated expression of plant defensins confer fungal resistance in rice. Rice 2, 143–154. doi: 10.1007/s12284-009-9030-2
Jha, S., and Chattoo, B. B. (2010). Expression of a plant defensin in rice confers resistance to fungal phytopathogens. Transgenic Res. 19, 373–384. doi: 10.1007/s11248-009-9315-7
Jia, L., Yan, W., Zhu, C., Agrama, H. A., and Jackson, A. (2012). Allelic analysis of sheath blight resistance with association mapping in rice. PLoS One 7:e32703. doi: 10.1371/journal.pone.0032703
Jia, Y., Liu, G., Correa-Victoria, F. J., McClung, A. M., Oard, J. H., Bryant, R. J., et al. (2012). Registration of four rice germplasm lines with improved resistance to sheath blight and blast diseases. J. Plant Regist. 6, 95–100. doi: 10.3198/jpr2011.05.0281crg
Jia, Y., Singh, V., Gealy, D., Liu, Y., Ma, J., Thurber, C., et al. (2022). Registration of two rice mapping populations using weedy rice ecotypes as a novel germplasm resource. J. Plant Regist. 16, 162–175. doi: 10.1002/plr2.20174
Kandhari, J. (2007). Management of sheath blight of rice through fungicides and botanicals. Indian Phytopathol. 60, 214–217.
Kannaiyan, S., and Prasad, N. N. (1980). Dicot weed hosts of Rhizoctonia solani Kühn. Agric. Res. J. 18, 125–127.
Kannan, P., Parameswari, C., Prasanyaselvam, K., Sridevi, G., and Veluthambi, K. (2017). Introgression of sheath blight disease tolerance from the transgenic rice event Pusa Basmati1-CG27 to the variety white ponni through backcross breeding. Indian J. Genet. Plant Breed. 77, 501–507. doi: 10.5958/0975-6906.2017.00066.9
Karmakar, S., Molla, K. A., Chanda, P. K., Sarkar, S. N., Datta, S. K., and Datta, K. (2016). Green tissue-specific co-expression of chitinase and oxalate oxidase 4 genes in rice for enhanced resistance against sheath blight. Planta 243, 115–130. doi: 10.1007/s00425-015-2398-x
Keijer, J. (1996). “The initial steps of the infection process in Rhizoctonia solani,” in Rhizoctonia Species: Taxonomy, Molecular Biology, Ecology, Pathology and Disease Control, eds B. Sneh, S. Jabaji-Hare, S. Neate, and G. Dijst (Dordrecht: Springer), 149–162. doi: 10.1007/978-94-017-2901-7_13
Kim, J.-K., Jang, I. C., Wu, R., Zuo, W.-N., Boston, R. S., Lee, Y. H., et al. (2003). Co-expression of a modified maize ribosome-inactivating protein and a rice basic chitinase gene in transgenic rice plants confers enhanced resistance to sheath blight. Transgenic Res. 12, 475–484. doi: 10.1023/a:1024276127001
Kim, P., Xue, C. Y., Song, H. D., Gao, Y., Feng, L., Li, Y., et al. (2021). Tissue specific activation of DOF11 promotes rice resistance to sheath blight disease and increases grain weight via activation of SWEET14. Plant Biotechnol. J. 19, 409–411. doi: 10.1111/pbi.13489
Kora, A. J., Mounika, J., and Jagadeeshwar, R. (2020). Rice leaf extract synthesized silver nanoparticles: an in vitro fungicidal evaluation against Rhizoctonia solani, the causative agent of sheath blight disease in rice. Fungal Biol. 124, 671–681. doi: 10.1016/j.funbio.2020.03.012
Koshariya, A., Kumar, I., Pradhan, A., Shinde, U., Verulkar, S. B., Agrawal, T., et al. (2018). Identification of quantitative trait loci (QTL) associated with sheath blight tolerance in rice. Indian J. Genet. Plant Breed. 78, 196–201. doi: 10.5958/0975-6906.2018.00025.1
Kozaka, T. (1961). Ecological studies on sheath blight of rice plant caused by Pellicularia sasakii and its chemical control. Chugoku Agric. Res. 20, 1–13.
Kozaka, T. (1965). Ecology of Pellicularia sheath blight of rice plant and its chemical control. Jpn. J. Phytopathol. 31, 179–185. doi: 10.3186/jjphytopath.31.special1_179
Kozaka, T. (1970). Pellicularia sheath blight of rice plants and its control. Jpn. Agric. Res. Q. 5, 12–16.
Krishnamurthy, K., Balconi, C., Sherwood, J. E., and Giroux, M. J. (2001). Wheat puroindolines enhance fungal disease resistance in transgenic rice. Mol. Plant Microbe Interact. 14, 1255–1260. doi: 10.1094/MPMI.2001.14.10.1255
Kumar, M. P., Gowda, D. S., Moudgal, R., Kumar, N. K., Gowda, K. P., and Vishwanath, K. (2013). “Impact of fungicides on rice production in India,” in Showcases of Integrated Plant Disease Management from Around the World, ed. M. Nita (London: InTech), 77–98. doi: 10.5772/51009
Kumar, P., Ahlawat, S., Chauhan, R., Kumar, A., Singh, R., and Kumar, A. (2018). In vitro and field efficacy of fungicides against sheath blight of rice and post-harvest fungicide residue in soil, husk, and brown rice using gas chromatography-tandem mass spectrometry. Environ. Monit. Assess. 190:503. doi: 10.1007/s10661-018-6897-7
Kunihiro, Y., Qian, Q., Sato, H., Teng, S., Zeng, D. L., Fujimoto, K., et al. (2002). QTL analysis of sheath blight resistance in rice (Oryza sativa L.). Acta Genet. Sin. 29, 50–55.
Lee, F. N., and Rush, M. C. (1983). Rice sheath blight: a major rice disease. Plant Dis. 67, S829–S832.
Lenka, S., Mishra, S. K., Mohanty, S. K., and Saha, S. (2008). Role of weather parameters on sheath blight incidence in rice caused by Rhizoctonia solani Kühn. Oryza 45, 336–338.
Li, J., Xia, M., Wan, B., Zha, Z., and Yu, D. (2009). Genetic analysis and mapping of TWH gene in rice twisted hull mutant rice. Science 16, 79–82. doi: 10.1016/S1672-6308(08)60061-X
Li, L., Wright, S. J., Krystofova, S., Park, G., and Borkovich, K. A. (2007). Heterotrimeric G protein signalling in filamentous fungi. Annu. Rev. Microbiol. 61, 423–452. doi: 10.1146/annurev.micro.61.080706.093432
Li, S., Peng, X., Wang, Y., Hua, K., Xing, F., Zheng, Y., et al. (2019). The effector AGLIP1 in Rhizoctonia solani AG1 IA triggers cell death in plants and promotes disease development through inhibiting PAMP-triggered immunity in Arabidopsis thaliana. Front. Microbiol. 10:2228. doi: 10.3389/fmicb.2019.02228
Li, Z., Pinson, S. R. M., Marchetti, M. A., Stansel, J. W., and Park, W. D. (1995). Characterization of quantitative trait loci (QTLs) in cultivated rice contributing to field resistance to sheath blight (Rhizoctonia solani). Theor. Appl. Genet. 91, 382–388. doi: 10.1007/BF00220903
Lilly, J. J., and Subramanian, B. (2019). Gene network mediated by WRKY13 to regulate resistance against sheath infecting fungi in rice (Oryza sativa L.). Plant Sci. 280, 269–282. doi: 10.1016/j.plantsci.2018.12.017
Lin, Q. J., Chu, J., Kumar, V., Yuan, D. P., Li, Z. M., Mei, Q., et al. (2021). Protein phosphatase 2A catalytic subunit PP2A-1 enhances rice resistance to sheath blight disease. Front. Genome Ed. 3:632136. doi: 10.3389/fgeed.2021.632136
Lin, W., Anuratha, C. S., Datta, K., Potrykus, I., Muthukrishnan, S., and Datta, S. K. (1995). Genetic engineering of rice for resistance to sheath blight. Biotech 13, 686–691. doi: 10.1038/nbt0795-686
Liu, G., Jia, Y., Correa-Victoria, F. J., Prado, G. A., Yeater, K. M., Mcclung, A., et al. (2009). Mapping quantitative trait loci responsible for resistance to sheath blight in rice. Phytopathology 99, 1078–1084. doi: 10.1094/PHYTO-99-9-1078
Liu, G., Jia, Y., McClung, A., Oard, J. H., Lee, F. N., and Correll, J. C. (2013). Confirming QTLs and finding additional loci responsible for resistance to rice sheath blight disease. Plant Dis. 97, 113–117. doi: 10.1094/PDIS-05-12-0466-RE
Liu, J., Shen, Y., Cao, H., He, K., Chu, Z., and Li, N. (2022). OsbHLH057 targets the AATCA cis-element to regulate disease resistance and drought tolerance in rice. Plant Cell Rep. 1–15. doi: 10.1007/s00299-022-02859-w
Liu, J. M., Mei, Q., Xue, C. Y., Wang, Z. Y., Li, D. P., Zhang, Y. X., et al. (2021). Mutation of G-protein γ subunit DEP1 increases planting density and resistance to sheath blight disease in rice. Plant. Biotechnol. J. 19, 418–420. doi: 10.1111/pbi.13500
Liu, W., Liu, J., Triplett, L., Leach, J. E., and Wang, G. L. (2014). Novel insights into rice innate immunity against bacterial and fungal pathogens. Annu. Rev. Phytopathol. 52, 213–241. doi: 10.1146/annurev-phyto-102313-045926
Liu, X. L., Li, J. C., Noman, A., and Lou, Y. G. (2019). Silencing OsMAPK20-5 has different effects on rice pests in the field. Plant Signal. Behav. 14:e1640562. doi: 10.1080/15592324.2019.1640562
Liu, Y., Chen, L., Fu, D., Lou, Q., Mei, H., Xiong, L., et al. (2014). Dissection of additive, epistatic effect and QTL× environment interaction of quantitative trait loci for sheath blight resistance in rice. Hereditas 151, 28–37. doi: 10.1111/hrd2.00026
Maeda, S., Dubouzet, J. G., Kondou, Y., Jikumaru, Y., Seo, S., Oda, K., et al. (2019). The rice CYP78A gene BSR2 confers resistance to Rhizoctonia solani and affects seed size and growth in Arabidopsis and rice. Sci. Rep. 9:587. doi: 10.1038/s41598-018-37365-1
Maliang, H., Wang, P., Chen, A., Liu, H., Lin, H., and Ma, J. (2021). Bamboo tar as a novel fungicide: its chemical components, laboratory evaluation, and field efficacy against false smut and sheath blight of rice and powdery mildew and Fusarium wilt of cucumber. Plant Dis. 105, 331–338. doi: 10.1094/PDIS-06-20-1157-RE
Mandal, L., Verma, S. K., Kotasthane, A. S., Agrawal, T., Jalamkar, P., and Verulkar, S. B. (2018). Mapping of quantitative trait loci for sheath blight resistance in rice. Oryza 55, 260–270. doi: 10.5958/2249-5266.2018.00032.2
Marchetti, M. A., McClung, A. M., Webb, B. D., and Bollich, C. N. (1996). Registration of B82-761 long-grain rice germplasm resistant to blast and sheath blight. Crop Sci. 36:815. doi: 10.2135/cropsci1996.0011183x003600030066x
Margani, R., and Widadi, S. (2018). Utilizing Bacillus to inhibit the growth and infection by sheath blight pathogen, Rhizoctonia solani in rice. IOP Conf. Ser. Earth Environ. Sci. 142:012070. doi: 10.1088/1755-1315/142/1/012070
Marshall, D. S., and Rush, M. C. (1980). Infection cushion formation on rice sheaths by Rhizoctonia solani. Phytopathology 70, 947–950. doi: 10.1094/phyto-70-947
Mathivanan, N., Prabavathy, V. R., and Vijayanandraj, V. R. (2005). Application of talc formulations of Pseudomonas fluorescens Migula and Trichoderma viride Pers. ex S.F. Gray decrease the sheath blight disease and enhance the plant growth and yield in rice. J. Phytopathol. 154, 697–701. doi: 10.1111/j.1439-0434.2005.01042.x
Mishra, D., Rajput, R. S., Zaidi, N. W., and Singh, H. B. (2020). Sheath blight and drought stress management in rice (Oryza sativa) through Trichoderma spp. Indian Phytopathol. 73, 71–77. doi: 10.1007/s42360-019-00189-8
Miyagi, Y. (1990). “Fungicide use for the control of major rice diseases in Japan,” in Pest Management in Rice, eds B. T. Grayson, M. B. Green, and L. G. Copping (Dordrecht: Springer), 111–121. doi: 10.1007/978-94-009-0775-1_7
Mohammed, A. S., El Hassan, S. M., Elballa, M. M., and Elsheikh, E. A. (2020). The role of Trichoderma, VA mycorrhiza and dry yeast in the control of Rhizoctonia disease of potato (Solanum tuberosum L.). Univ. Khartoum J. Agric. Sci. 16, 285–301.
Molla, K. A., Karmakar, S., Chanda, P. K., Ghosh, S., Sarkar, S. N., Datta, S. K., et al. (2013). Rice oxalate oxidase gene driven by green tissue-specific promoter increases tolerance to sheath blight pathogen (Rhizoctonia solani) in transgenic rice. Mol. Plant Pathol. 14, 910–922. doi: 10.1111/mpp.12055
Molla, K. A., Karmakar, S., Chanda, P. K., Sarkar, S. N., Datta, S. K., and Datta, K. (2016). Tissue-specific expression of Arabidopsis NPR1 gene in rice for sheath blight resistance without compromising phenotypic cost. Plant Sci. 250, 105–114. doi: 10.1016/j.plantsci.2016.06.005
Molla, K. A., Karmakar, S., Molla, J., Bajaj, P., Varshney, R. K., Datta, S. K., et al. (2020). Understanding sheath blight resistance in rice: the road behind and the road ahead. Plant. Biotechnol. J. 18, 895–915. doi: 10.1111/pbi.13312
Moni, Z. R., Ali, M. A., and Alam, M. S. (2016). Morphological and genetical variability among Rhizoctonia solani isolates causing sheath blight disease of rice. Rice Sci. 23, 42–50. doi: 10.1016/j.rsci.2016.01.005
Mukherjee, N. (1978). Sheath blight of rice (Thanatephorus cucumeris) and its control possibilities. Pesticides 12, 39–40.
Nadarajah, K., Mat Razali, N., Cheah, B. H., Sahruna, N. S., Ismail, I., Tathode, M., et al. (2017). Draft genome sequence of Rhizoctonia solani anastomosis group 1 subgroup 1A strain 1802/KB isolated from rice. Genome Announc. 5:e01188-17. doi: 10.1128/genomeA.01188-17
Naik, R. G., Jayalakshmi, K., and Naik, T. B. (2017). Efficacy of fungicides on the management of sheath blight of rice. Int. J. Curr. Microbiol. Appl. Sci. 6, 611–614. doi: 10.20546/ijcmas.2017.609.075
Nelson, J. C., Oard, J. H., Groth, D., Utomo, H. S., Jia, Y., Liu, G., et al. (2012). Sheath-blight resistance QTLS in Japonica rice germplasm. Euphytica 184, 23–34. doi: 10.1007/s10681-011-0475-1
Neves, S. R., Ram, P. T., and Iyengar, R. (2002). G protein pathways. Science 296, 1636–1639. doi: 10.1126/science.1071550
Ogoshi, A. (1987). Ecology and pathogenicity of anastomosis and intraspecific groups of Rhizoctonia solani Kühn. Ann. Rev. Phytopathol. 25, 125–143. doi: 10.1146/annurev.py.25.090187.001013
Oreiro, E. G., Grimares, E. K., Atienza-Grande, G., Quibod, I. L., Roman-Reyna, V., and Oliva, R. (2020). Genome-wide associations and transcriptional profiling reveal ROS regulation as one underlying mechanism of sheath blight resistance in rice. Mol. Plant Microbe Interact. 33, 212–222. doi: 10.1094/MPMI-05-19-0141-R
Ou, S. H., Bandong, J. M., and Nuque, E. L. (1973). “Some studies on sheath blight of rice at IRRI,” in Proceedings of the International Rice. Research Conference April 23-27, Los Banos, 1–6.
Pan, X., Zou, J., Chen, Z., Lu, J., Yu, H., Li, H., et al. (1999). Tagging major quantitative trait loci for sheath blight resistance in a rice variety, Jasmine 85. Chin. Sci. Bull. 44, 1783–1789. doi: 10.1007/bf02886159
Panthapulakkal, N. S., Lung, S. C., Liao, P., Lo, C., and Chye, M. L. (2020). The overexpression of OsACBP5 protects transgenic rice against necrotrophic, hemibiotrophic and biotrophic pathogens. Sci. Rep. 10:14918. doi: 10.1038/s41598-020-71851-9
Paracer, C. S., and Chahal, D. S. (1963). Sheath blight of rice caused by Rhizoctonia solani Kühn – a new record in India. Curr. Sci. 32, 328–329.
Parmeter, J. R., Sherwood, R. T., and Platt, W. D. (1969). Anastomosis grouping among isolates of Thanatephorus cucumeris. Phytopathology 9, 1270–1278.
Parween, D., and Sahu, B. B. (2022). An Arabidopsis nonhost resistance gene, IMPORTIN ALPHA 2 provides immunity against rice sheath blight pathogen, Rhizoctonia solani. Curr. Res. Microb. Sci. 3:100109. doi: 10.1016/j.crmicr.2022.100109
Patil, H. J., Srivastava, A. K., Kumar, S., Chaudhari, B. L., and Arora, D. K. (2010). Selective isolation, evaluation and characterization of antagonistic actinomycetes against Rhizoctonia solani. World J. Microbiol. Biotechnol. 26, 2163–2170. doi: 10.1007/s11274-010-0400-0
Patkar, R. N., and Chattoo, B. B. (2006). Transgenic indica rice expressing ns-LTPlike protein shows enhanced resistance to both fungal and bacterial pathogens. Mol. Breed. 17, 159–171. doi: 10.1007/s11032-005-4736-3
Peng, X., Hu, Y., Tang, X., Zhou, P., Deng, X., Wang, H., et al. (2012). Constitutive expression of rice WRKY30 gene increases the endogenous jasmonic acid accumulation, PR gene expression and resistance to fungal pathogens in rice. Planta 236, 1485–1498. doi: 10.1007/s00425-012-1698-7
Peng, X., Wang, H., Jang, J. C., Xiao, T., He, H., Jiang, D., et al. (2016). OsWRKY80-OsWRKY4 module as a positive regulatory circuit in rice resistance against Rhizoctonia solani. Rice 9:63. doi: 10.1186/s12284-016-0137-y
Pinson, S. R., Capdevielle, F. M., and Oard, J. H. (2005). Confirming QTLs and finding additional loci conditioning sheath blight resistance in rice using recombinant inbred lines. Crop Sci. 45, 503–510. doi: 10.2135/cropsci2005.0503
Pramesh, D., Alase, S., Muniraju, K. M., and Kumara, M. K. (2017). A combination fungicide for the management of sheath blight, sheath rot and stem rot diseases of paddy. Int. J. Curr. Microbiol. 6, 3500–3509. doi: 10.20546/ijcmas.2017.609.430
Prasad, B., and Eizenga, G. C. (2008). Rice sheath blight disease resistance identified in Oryza spp. accessions. Plant Dis. 92, 1503–1509. doi: 10.1094/PDIS-92-11-1503
Prasad, D., Singh, R., and Singh, A. (2010). Management of sheath blight of rice with integrated nutrients. Indian Phytopathol. 63, 11–15.
Prasad, P. S., Naik, M. K., and Nagaraju, P. (2006). Screening of genotypes, fungicides, botanical and bio-agents against Rhizoctonia solani, the incitant of sheath blight of rice. Proceedings of the National Seminar on Frontiers in Plant Pathology 139.
Qi, Z. Q., Yu, J. J., Shen, L. R., Yu, Z. C., Yu, M. N., Du, Y., et al. (2017). Enhanced resistance to rice blast and sheath blight in rice (Oryza sativa L) by expressing the oxalate decarboxylase protein bacisubin from Bacillus subtilis. Plant Sci. 265, 51–60. doi: 10.1016/j.plantsci.2017.09.014
Qiao, L., Zheng, L., Sheng, C., Zhao, H., Jin, H., and Niu, D. (2020). Rice siR109944 suppresses plant immunity to sheath blight and impacts multiple agronomic traits by affecting auxin homeostasis. Plant J. 102, 948–964. doi: 10.1111/tpj.14677
Radjacommare, R., Kandan, A., Nandakumar, R., and Samiyappan, R. (2004). Association of the hydrolytic enzyme chitinase against Rhizoctonia solani in rhizobacteria treated rice plants. J. Phytopathol. 152, 365–370. doi: 10.1111/j.1439-0434.2004.00857.x
Raj, T. S., Muthukumar, A., Renganathan, P., Kumar, R. S. R., and Ann, H. (2019). Biological control of sheath blight of rice caused by Rhizoctonia solani Kühn using marine associated Bacillus subtilis. Int. Arch. Appl. Sci. Technol. 10, 148–153. doi: 10.15515/iaast.0976-4828.10.4.148153
Rajan, K. M., and Nair, P. V. (1979). Reaction of certain rice varieties to sheath blight and sheath rot diseases. Agric. Res. J. 17, 259–260.
Rajeswari, E., Padmodaya, B., Viswanath, K., and Sumathi, P. (2020). Evaluation of plant extracts on mycelial growth and viability of the sclerotia of Rhizoctonia solani Kühn in vitro and in soil. J. Pharmacogn. Phytochem. 9, 255–259.
Ram, T., Majumdar, N. D., Laha, G. S., Ansari, M. M., Kar, C. S., and Mishra, B. (2008). Identification of donors for sheath blight resistance in wild rice. Indian J. Genet. Plant Breed. 68, 317–319.
Ramalingam, J., Raveendra, C., Savitha, P., Vidya, V., Chaithra, T. L., Velprabakaran, S., et al. (2020). Gene pyramiding for achieving enhanced resistance to bacterial blight, blast, and sheath blight diseases in rice. Front. Plant Sci. 11:591457. doi: 10.3389/fpls.2020.591457
Rangaswami, G., and Mahadevan, A. (1998). Diseases of Crop Plants in India. New Delhi: PHI Learning Pvt, Ltd.
Rao, M. V. R., Parameswari, C., Sripriya, R., and Veluthambi, K. (2011). Transgene stacking and marker elimination in transgenic rice by sequential Agrobacterium-mediated co-transformation with the same selectable marker gene. Plant Cell Rep. 30, 1241–1252. doi: 10.1007/s00299-011-1033-y
Rao, T. B., Chopperla, R., Methre, R., Punniakotti, E., Venkatesh, V., Sailaja, B., et al. (2019). Pectin induced transcriptome of a Rhizoctonia solani strain causing sheath blight disease in rice reveals insights on key genes and RNAi machinery for development of pathogen derived resistance. Plant Mol. Biol. 100, 59–71. doi: 10.1007/s11103-019-00843-9
Rashid, M. M., Bhuiyan, M. R., Dilzahan, H. A., Hamid, M. A., Hasan, N., Khan, M. A. I., et al. (2020). Biological control of rice sheath blight disease (Rhizoctonia solani) using bio-pesticides and bio-control agents. Bangladesh Rice J. 24, 47–58. doi: 10.3329/brj.v24i1.53239
Raveendra, C., Vanniarajan, C., Ebenezar, E. G., and Ramalingam, J. (2020). Marker-assisted selection for sheath blight resistance in rice (Oryza sativa L.). Electron. J. Plant Breed. 11, 581–584. doi: 10.37992/2020.1102.096
Richa, K., Tiwari, I. M., Devanna, B. N., Botella, J. R., Sharma, V., and Sharma, T. R. (2017). Novel chitinase gene LOC_Os11g47510 from indica rice Tetep provides enhanced resistance against sheath blight pathogen Rhizoctonia solani in rice. Front. Plant Sci. 8:596. doi: 10.3389/fpls.2017.00596
Rodriguez, F. A., Vale, F. X. R., Korndorfer, G. H., Prabhu, A. S., Datnoff, L. E., Oliveira, A. M. A., et al. (2003). Influence of silicon on sheath blight of rice in Brazil. Crop Prot. 22, 23–29. doi: 10.1016/S0261-2194(02)00084-4
Rodriguez, H. A., Nass, H., Cardona, R., and Aleman, L. (1999). Alternatives to control of sheath blight caused by Rhizoctonia solani in rice. Fitopathol. Venez. 12, 18–21.
Roy, A. K. (1977). Screening of rice cultures against sheath blight. Indian J. Agric. Sci. 47, 259–260.
Roy, A. K. (1986). Effect of slow release nitrogenous fertilizers on incidence of sheath blight and yield of rice. Oryza 23, 198–199.
Sabes, P. L. P., Lon, M. M., Peter, M. A., Maruyama, J., Koyama, S., Watanbe, T., et al. (2020). Effect of increased silicon content of paddy rice on sheath blight development through carbonized rice husk application. Jpn. Agric. Res. Q. 54, 145–151. doi: 10.6090/jarq.54.145
Sadumpati, V., Kalambur, M., Vudem, D. R., Kirti, P. B., and Khareedu, V. R. (2013). Transgenic indica rice lines, expressing Brassica juncea nonexpressor of pathogenesis-related genes 1 (BjNPR1), exhibit enhanced resistance to major pathogens. J. Biotechnol. 166, 114–121. doi: 10.1016/j.jbiotec.2013.04.016
Sajeena, A., Babu, R. M., and Marimuthu, T. (2008). Ganosol the formulated extract of the mushroom Ganoderma sp. controls the sheath blight pathogen of rice, R. solani Kühn. Crop Res. 36, 318–321.
Samal, P., Molla, K. A., Bal, A., Ray, S., Swain, H., Khandual, A., et al. (2022). Comparative transcriptome profiling reveals the basis of differential sheath blight disease response in tolerant and susceptible rice genotypes. Protoplasma 259, 61–73. doi: 10.1007/s00709-021-01637-x
Sanjay, G., Thind, T. S., Kaur, R., and Kaur, M. (2012). Management of sheath blight of rice with novel action fungicides. Indian Phytopathol. 65, 92–93.
Sarkar, M. K., Sharma, B. D., and Gupta, P. K. S. (1991). The effect of plant spacing and fertilizer application on the sheath blight of rice caused by Rhizoctonia solani. Beitr. Trop. Landwirtsch. Veterinarmed. 29, 331–333.
Sarkar, S. C., and Chowdhury, A. K. (2007). Impact of challenge inoculation of tungro virus in rice plants infected by Helminthosporium oryzae and Rhizoctonia solani. J. Mycopathol. Res. 45, 69–72.
Sato, H., Ideta, O., Ando, I., Kunihiro, Y., Hirabayashi, H., Iwano, M., et al. (2004). Mapping QTLs for sheath blight resistance in the rice line WSS2. Breed. Sci. 54, 265–271. doi: 10.1270/jsbbs.54.265
Sattari, A., Fakheri, B., Noroozi, M., and Moazami Gudarzi, K. (2014). Breeding for resistance to sheath blight in rice. Int. J. Farm Alli Sci. 3, 970–979.
Savary, S., Castilla, N., Elazegui, F., McLaren, C., Ynalvez, M., and Teng, P. (1995). Direct and indirect effects of nitrogen supply and disease source structure on rice sheath blight spread. Phytopatholgy 85, 959–965. doi: 10.1094/phyto-85-959
Senapoty, D. (2010). Efficacy of soil amendments for the management of rice sheath blight. Indian Phytopathol. 63, 94–95.
Senthilvel, V., Chockalingam, V., Raman, R., Rangasamy, S., Sundararajan, T., and Jegadeesan, R. (2021). Performance of gene pyramided rice lines for blast and sheath blight resistance. Biol. Forum Int. J. 13, 913–917.
Sha, X. Y., and Zhu, L. H. (1989). Resistance of some rice varieties to sheath blight (ShB). Int. Rice Res. Newslett. 15, 7–8.
Shah, J. M., Singh, R., and Veluthambi, K. (2013). Transgenic rice lines constitutively co-expressing tlp-D34 and chi11 display enhancement of sheath blight resistance. Biol. Plant. 57, 351–358. doi: 10.1007/s10535-012-0291-z
Shahid, A. A., Shahbaz, M., and Ali, M. (2014). A comparative study of the commercially available fungicides to control sheath blight of rice in Lahore. J. Plant Pathol. Microb. 5, 2157–7471. doi: 10.4172/2157-7471.1000240
Sharma, A., McClung, A. M., Pinson, S. R., Kepiro, J. L., Shank, A. R., Tabien, R. E., et al. (2009). Genetic mapping of sheath blight resistance QTLs within tropical Japonica rice cultivars. Crop Sci. 49, 256–264. doi: 10.2135/cropsci2008.03.0124
Sharma, N. R., Teng, P. S., and Olivares, P. M. (1990). Comparison of assessment methods for rice sheath blight disease. Philipp. Phytopathol. 26, 20–24.
Shi, W., Zhao, S. L., Liu, K., Sun, Y. B., Ni, Z. B., Zhang, G. Y., et al. (2020). Comparison of leaf transcriptome in response to Rhizoctonia solani infection between resistant and susceptible rice cultivars. BMC Genomics 21:245. doi: 10.1186/s12864-020-6645-6
Shimono, M., Koga, H., Akagi, A. Y. A., Hayashi, N., Goto, S., Sawada, M., et al. (2012). Rice WRKY45 plays important roles in fungal and bacterial disease resistance. Mol. Plant Pathol. 13, 83–94. doi: 10.1111/j.1364-3703.2011.00732.x
Shu, C. W., Zou, C. J., Chen, J. L., Tang, F., Yi, R. H., and Zhou, E. X. (2014). Genetic diversity and population structure of Rhizoctonia solani AG-1 IA, the causal agent of rice sheath blight, in South China. Can. J. Plant Pathol. 36, 179–186. doi: 10.1080/07060661.2014.913685
Siddhartha, Verma, A., Bashyal, B. M., Gogoi, R., and Kumar, R. (2020). New nano-fungicides for the management of sheath blight disease (Rhizoctonia solani) in rice. Int. J. Pest Manage. 8, 1–10. doi: 10.1080/09670874.2020.1818870
Singh, A., Singh, V. K., Singh, S. P., Pandian, R. T. P., Ellur, R. K., Singh, D., et al. (2012). Molecular breeding for the development of multiple disease resistance in basmati rice. AoB Plants 2012:pls029. doi: 10.1093/aobpla/pls029
Singh, K. D., and Borah, P. (2000). Screening of local upland cultivars of Assam against sheath blight. Ann. Biol. 16, 161–162.
Singh, N. I., Devi, R. K., and Singh, K. U. (1988). Occurrence of rice sheath blight (ShB) Rhizoctonia solani Kühn. on rice panicles in India. Int. Rice Res. Newslett. 13:29.
Singh, P., Mazumdar, P., Harikrishna, J. A., and Babu, S. (2019). Sheath blight of rice: a review and identification of priorities for future research. Planta 250, 1387–1407. doi: 10.1007/s00425-019-03246-8
Singh, R., and Dodan, D. S. (1995). Reactions of rice genotypes to bacterial leaf blight, stem rot, and sheath blight in Haryana. Indian J. Mycol. Plant Pathol. 25, 224–227.
Singh, R., Murti, S., Mahilal, Tomer, A., and Prasad, D. (2015). Virulence diversity in Rhizoctonia solani causing sheath blight in rice. J. Plant Pathol. Microbiol. 6:296. doi: 10.4172/2157-7471.1000296
Singh, R., Sunder, S., and Kumar, P. (2016). Sheath blight of rice: current status and perspectives. Indian Phytopathol. 69, 340–351.
Singh, S. K., Shukla, V., Singh, H., and Sinha, A. P. (2004). Current status and impact of sheath blight in rice (Oryza sativa L.) – a review. Agric. Rev. 25, 289–297.
Singh, S. P., Gupta, R., Gaur, R., and Srivastava, A. K. (2017). Antagonistic actinomycetes mediated resistance in Solanum lycopersicon Mill. against Rhizoctonia solani Kühn. Biol. Sci. 87, 789–798. doi: 10.1007/s40011-015-0651-5
Sivalingam, P. N., Vishwakarma, S. N., and Singh, U. S. (2006). Role of seed-borne inoculum of Rhizoctonia solani in sheath blight of rice. Indian Phytopathol. 59, 445–452.
Sneh, B., Burpee, L., and Ogoshi, A. (1991). Identification of Rhizoctonia Species. St Paul, MN: American Phytopathol. Society Press.
Sridevi, G., Sabapathi, N., Meena, P., Nandakumar, R., Samiyappan, R., Muthukrishnan, S., et al. (2003). Transgenic indica rice variety Pusa Basmati constitutively expressing a rice chitinase gene exhibit enhanced resistance to Rhizoctonia solani. J. Plant Biochem. Biotechnol. 12, 93–101. doi: 10.1007/bf03263168
Srinivas, P., Ramesh Babu, S., and Ratan, V. (2014). Role of sclerotia, plant debris and different hosts on survival of rice sheath blight pathogen, Rhizoctonia solani. Int. J. Appl. Biol. Pharm. 5, 29–33.
Sun, Q., Li, D. D., Chu, J., Li, S., Zhong, L. J., Han, X., et al. (2020). Indeterminate domain proteins regulate rice defense to sheath blight disease. Rice 13:15. doi: 10.1186/s12284-020-0371-1
Sun, Q., Li, T. Y., Li, D. D., Wang, Z. Y., Li, S., Li, D. P., et al. (2019a). Overexpression of loose plant architecture 1 increases planting density and resistance to sheath blight disease via activation of PIN-FORMED 1a in rice. Plant Biotechnol. J. 17, 855–857. doi: 10.1111/pbi.13072
Sun, Q., Liu, Y., Wang, Z. Y., Li, S., Ye, L., Xie, J. X., et al. (2019b). Isolation and characterization of genes related to sheath blight resistance via the tagging of mutants in rice. Plant Gene 19:100200. doi: 10.1016/j.plgene.2019.100200
Sun, X., Lu, D., Ou-Yang, L., Hu, L., Bian, J., Peng, X., et al. (2014). Association mapping and resistant alleles analysis for sheath blight resistance in rice. Acta Agron. Sin. 40, 779–787. doi: 10.3724/sp.j.1006.2014.00779
Sunder, S., Singh, R., and Dodan, D. S. (2003). Standardization of inoculation methods and management of sheath blight of rice. Indian J. Plant Pathol. 21, 92–96.
Susmita, D., Jyothi, B., Ram, K., Chhabra, A. K., and Janghel, D. K. (2019). Current status of rice breeding for sheath blight resistance. Int. J. Curr. Microbiol. Appl. Sci. 8, 163–175. doi: 10.20546/ijcmas.2019.802.020
Swain, D. M., Sahoo, R. K., Chandan, R. K., Ghosh, S., Kumar, R., Jha, G., et al. (2019). Concurrent overexpression of rice G-protein β and γ subunits provide enhanced tolerance to sheath blight disease and abiotic stress in rice. Planta 250, 1505–1520. doi: 10.1007/s00425-019-03241-z
Taguchi-Shiobara, F., Ozaki, H., Sato, H., Maeda, H., Kojima, Y., Ebitani, T., et al. (2013). Mapping and validation of QTLs for rice sheath blight resistance. Breed. Sci. 63, 301–308. doi: 10.1270/jsbbs.63.301
Taheri, P., Gnanamanickam, S., and Höfte, M. (2007). Characterization, genetic structure, and pathogenicity of Rhizoctonia spp. associated with rice sheath diseases in India. Phytopathology 97, 373–383. doi: 10.1094/PHYTO-97-3-0373
Taheri, P., and Tarighi, S. (2010). Riboflavin induces resistance in rice against Rhizoctonia solani via jasmonate-mediated priming of phenylpropanoid pathway. J. Plant Physiol. 167, 201–208. doi: 10.1016/j.jplph.2009.08.003
Tan, C. X., Ji, X. M., Yang, Y., Pan, X. Y., Zuo, S. M., Zhang, Y. F., et al. (2005). Identification and marker-assisted selection of two major quantitative genes controlling rice sheath blight resistance in backcross generations. Acta Genet. Sin. 32, 399–405.
Tang, Q., Peng, S., Buresh, R. J., Zou, Y., Castilla, N. P., Mew, T. W., et al. (2007). Rice varietal difference in sheath blight development and its association with yield loss at different levels of N fertilization. Field Crops Res. 102, 219–227. doi: 10.1016/j.fcr.2007.04.005
Thind, T. S., Mohan, C., Sharma, V. K., Raj, P., Arora, J. K., and Singh, P. P. (2008). Functional relationship of sheath blight of rice with crop age and weather factors. Plant Dis. Res. 23, 34–40.
Tiwari, I. M., Jesuraj, A., Kamboj, R., Devanna, B. N., Botella, J. R., and Sharma, T. R. (2017). Host delivered RNAi, an efficient approach to increase rice resistance to sheath blight pathogen (Rhizoctonia solani). Sci. Rep. 7:7521. doi: 10.1038/s41598-017-07749-w
Tiwari, M., Srivastava, S., Singh, P. C., Mishra, A. K., and Chakrabarty, D. (2020). Functional characterization of tau class glutathione-S-transferase in rice to provide tolerance against sheath blight disease. 3 Biotech 10:84. doi: 10.1007/s13205-020-2071-3
Tsai, W. H. (1974). Assessment of yield losses due to rice sheath blight at different inoculation stages. J. Taiwan Agric. Res. 23, 188–194.
Tuyen, H. T. T., and Hoa, L. B. (2022). “Evaluation of the effectiveness of controlling sheath blight disease in rice caused by Rhizoctonia solani under greenhouse conditions by applying biofungicides,” in Global Changes and Sustainable Development in Asian Emerging Market Economies, Vol. 2, eds A. T. Nguyen and L. Hens (Cham: Springer), 505–516. doi: 10.1007/978-3-030-81443-4_31
Uppala, S., and Zhou, X. G. (2018). Field efficacy of fungicides for management of sheath blight and narrow brown leaf spot of rice. Crop Prot. 104, 72–77. doi: 10.1016/j.cropro.2017.10.017
Wang, A., Shu, X., Jing, X., Jiao, C., Chen, L., Zhang, J., et al. (2021). Identification of rice (Oryza sativa L.) genes involved in sheath blight resistance via a genome-wide association study. Plant Biotechnol. J. 19, 1553–1566. doi: 10.1111/pbi.13569
Wang, J., Yang, C., Hu, X., Yao, X., Han, L., Wu, X., et al. (2022). Lauric acid induces apoptosis of rice sheath blight disease caused by Rhizoctonia solani by affecting fungal fatty acid metabolism and destroying the dynamic equilibrium of reactive oxygen species. J. Fungi 8:153. doi: 10.3390/jof8020153
Wang, L., Liu, L. M., Hou, Y. X., Li, L., and Huang, S. W. (2015c). Pathotypic and genetic diversity in the population of Rhizoctonia solani AG 1-IA causing rice sheath blight in China. Plant Pathol. 64, 718–728. doi: 10.1111/ppa.12299
Wang, H., Meng, J., Peng, X., Tang, X., Zhou, P., Xiang, J., et al. (2015a). Rice WRKY4 acts as a transcriptional activator mediating defense responses toward Rhizoctonia solani, the causing agent of rice sheath blight. Plant Mol. Biol. 89, 157–171. doi: 10.1007/s11103-015-0360-8
Wang, R., Lu, L. X., Pan, X. B., Hu, Z. L., Ling, F., Yan, Y., et al. (2015b). Functional analysis of OsPGIP1 in rice sheath blight resistance. Plant Mol. Biol. 87, 181–191. doi: 10.1007/s11103-014-0269-7
Wang, Y., Pinson, S. R. M., Fjellstrom, R. G., and Tabien, R. E. (2012). Phenotypic gain from introgression of two QTL, qSB9-2 and qSB12-1, for rice sheath blight resistance. Mol. Breed. 30, 293–303. doi: 10.1007/s11032-011-9619-1
Webster, R. K., and Gunnell, P. S. (1992). Compendium of Rice Diseases. St Paul, MN: American Phytopathological Society, viii–62.
Wen, Z. H., Zeng, Y. X., Ji, Z. J., and Yang, C. D. (2015). Mapping quantitative trait loci for sheath blight disease resistance in Yangdao 4 rice. Genet. Mol. Res. 14, 1636–1649. doi: 10.4238/2015.March.6.10
Willocquet, L., Noel, M., Hamilton, R. S., and Savary, S. (2012). Susceptibility of rice to sheath blight: an assessment of the diversity of rice germplasm according to genetic groups and morphological traits. Euphytica 183, 227–241. doi: 10.1007/s10681-011-0451-9
Wu, Y. L. (1971). Varietal differences in sheath blight resistance of rice obtained in Southern Taiwan. Sabrao Newslett. 3, 1–5.
Xie, Q. J., Linscombe, S. D., Rush, M. D., and Jodari-Karimi, J. (1992). Registration of ‘LSBR-33’ and ‘LSBR-5’ sheath blight resistant germplasm lines of rice. Crop Sci. 32:507. doi: 10.2135/cropsci1992.0011183x003200020063x
Xu, Q., Yuan, X., Yu, H., Wang, Y., Tang, S., and Wei, X. (2011). Mapping quantitative trait loci for sheath blight resistance in rice using double haploid population. Plant Breed. 130, 404–406. doi: 10.1111/j.1439-0523.2010.01806.x
Xue, X., Cao, Z. X., Zhang, X. T., Wang, Y., Zhang, Y. F., Chen, Z. X., et al. (2016). Overexpression of OsOSM1 enhances resistance to rice sheath blight. Plant Dis. 100, 1634–1642. doi: 10.1094/PDIS-11-15-1372-RE
Yadav, S., Anuradha, G., Kumar, R. R., Vemireddy, L. R., Sudhakar, R., Donempudi, K., et al. (2015). Identification of QTLs and possible candidate genes conferring sheath blight resistance in rice (Oryza sativa L.). SpringerPlus 4:175. doi: 10.1186/s40064-015-0954-2
Yellareddygari, S. K. R., Reddy, M. S., Kloepper, J. W., Lawrence, K. S., and Fadamiro, H. (2014). Rice sheath blight: a review of disease and pathogen management approaches. J. Plant Pathol. Microbiol. 5:1000241. doi: 10.4172/2157-7471.1000241
Yi, R. H., Liang, C. Y., Zhu, X. R., and Zhou, E. X. (2002). Genetic diversity and virulence variation of rice sheath blight strains (Rhizoctonia solani AG-1-IA) from Guangdong Province. J. Trop. Subtrop. Bot. 10, 161–170.
Yin, Y. J., Zuo, S. M., Wang, H., Chen, Z. X., Ma, Y. Y., Zhang, Y. F., et al. (2008). Pyramiding effects of three quantitative trait loci for resistance to sheath blight using near-isogenic lines of rice. Chin. J. Rice Sci. 22, 340–346.
Yuan, C. H. E. N., Yuxiang, Z. E. N. G., Zhijuan, J. I., Yan, L. I. A. N. G., Zhihua, W. E. N., and Changdeng, Y. A. N. G. (2019). Identification of stable quantitative trait loci for sheath blight resistance using recombinant inbred line. Rice Sci. 26, 331–338. doi: 10.1016/j.rsci.2019.08.007
Yuan, Z., Zhang, Y., Xu, G., Bi, D., Qu, H., Zou, X., et al. (2018). Comparative transcriptome analysis of Rhizoctonia solani-resistant and -susceptible rice cultivars reveals the importance of pathogen recognition and active immune responses in host resistance. J. Plant Biol. 61, 143–158. doi: 10.1007/s12374-017-0209-6
Yugander, A., Ladhalakshmi, D., Prakasham, V., Mangrauthia, S. K., Prasad, M. S., Krishnaveni, D., et al. (2015). Pathogenic and genetic variation among the isolates of Rhizoctonia solani (AG 1-IA), the rice sheath blight pathogen. J. Phytopathol. 163, 465–474. doi: 10.1111/jph.12343
Zeng, Y. X., Xia, L. Z., Wen, Z. H., Ji, Z. J., Zeng, D. L., Qian, Q. I. A. N., et al. (2015). Mapping resistant QTLs for rice sheath blight disease with a doubled haploid population. J. Integr. Agric. 14, 801–810. doi: 10.1016/S2095-3119(14)60909-6
Zhang, F., Zeng, D., Zhang, C. S., Lu, J. L., Chen, T. J., Xie, J. P., et al. (2019). Genome-wide association analysis of the genetic basis for sheath blight resistance in rice. Rice 12:93. doi: 10.1186/s12284-019-0351-5
Zhang, G. F., Lu, C. T., Shen, X. C., and Wang, W. X. (1995). The synthesized ecological effect of rice density and nitrogen fertilizer on the occurrence of main rice pests. Acta Phytophylacica Sin. 22, 38–44.
Zhao, Y., Wang, Q., Wu, X., Jiang, M., Jin, H., Tao, K., et al. (2021). Unraveling the polypharmacology of a natural antifungal product, eugenol, against Rhizoctonia solani. Pest Manage. Sci. 77, 3469–3483. doi: 10.1002/ps.6400
Zheng, A., Lin, R., Zhang, D., Qin, P., Xu, L., Ai, P., et al. (2013). The evolution and pathogenic mechanisms of the rice sheath blight pathogen. Nat. Commun. 4:1424. doi: 10.1038/ncomms2427
Zhou, X. G., Kumar, K. V. K., Zhou, L. W., Reddy, M. S., and Kloepper, J. W. (2021). Combined use of PGPRs and reduced rates of azoxystrobin to improve management of sheath blight of rice. Plant Dis. 105, 1034–1041. doi: 10.1094/PDIS-07-20-1596-RE
Zhou, Y., Bao, J., Zhang, D., Li, Y., Li, H., and He, H. (2020). Effect of heterocystous nitrogen-fixing cyanobacteria against rice sheath blight and the underlying mechanism. Appl. Soil Ecol. 153:103580. doi: 10.1016/j.apsoil.2020.103580
Zhu, G., Liang, E. X., Lan, X., Li, Q., Qian, J. J., Tao, H. X., et al. (2019). ZmPGIP3 gene encodes a polygalacturonase-inhibiting protein that enhances resistance to sheath blight in rice. Phytopathology 109, 1732–1740. doi: 10.1094/PHYTO-01-19-0008-R
Zhu, Y., Zuo, S., Chen, Z., Chen, X., Li, G., Zhang, Y., et al. (2014). Identification of two major rice sheath blight resistance QTLs, qSB1-1HJX74 and qSB11HJX74, in field trials using chromosome segment substitution lines. Plant Dis. 98, 1112–1121. doi: 10.1094/PDIS-10-13-1095-RE
Zou, J. H., Pan, X. B., Chen, Z. X., Xu, J. Y., Lu, J. F., Zhai, W. X., et al. (2000). Mapping quantitative trait loci controlling sheath blight resistance in two rice cultivars (Oryza sativa L.). Theor. Appl. Genet. 101, 569–573. doi: 10.1007/s001220051517
Zuo, S., Wang, Z., Chen, X., Gu, F., Zhang, Y., Chen, Z., et al. (2009). Evaluation of resistance of a novel rice germplasm YSBR1 to sheath blight. Acta Agron. Sin. 35, 608–614. doi: 10.3724/SP.J.1006.2009.00608
Zuo, S., Yin, Y., Pan, C., Chen, Z., Zhang, Y., Gu, S., et al. (2013). Fine mapping of qSB-11LE, the QTL that confers partial resistance to rice sheath blight. Theor. Appl. Genet. 126, 1257–1272. doi: 10.1007/s00122-013-2051-7
Zuo, S., Zhang, Y., Yin, Y., Li, G., Zhang, G., Wang, H., et al. (2014b). Fine-mapping of qSB-9TQ, a gene conferring major quantitative resistance to rice sheath blight. Mol. Breed. 34, 2191–2203. doi: 10.1007/s11032-014-0173-5
Keywords: Rhizoctonia solani, rice sheath blight (ShB), biological control, disease resistance, transgenic rice, resistance QTLs
Citation: Senapati M, Tiwari A, Sharma N, Chandra P, Bashyal BM, Ellur RK, Bhowmick PK, Bollinedi H, Vinod KK, Singh AK and Krishnan SG (2022) Rhizoctonia solani Kühn Pathophysiology: Status and Prospects of Sheath Blight Disease Management in Rice. Front. Plant Sci. 13:881116. doi: 10.3389/fpls.2022.881116
Received: 22 February 2022; Accepted: 06 April 2022;
Published: 03 May 2022.
Edited by:
Nacer Bellaloui, Agricultural Research Service (USDA), United StatesReviewed by:
Binod Bihari Sahu, National Institute of Technology, Rourkela, IndiaRamalingam Jegadeesan, Tamil Nadu Agricultural University, India
Copyright © 2022 Senapati, Tiwari, Sharma, Chandra, Bashyal, Ellur, Bhowmick, Bollinedi, Vinod, Singh and Krishnan. This is an open-access article distributed under the terms of the Creative Commons Attribution License (CC BY). The use, distribution or reproduction in other forums is permitted, provided the original author(s) and the copyright owner(s) are credited and that the original publication in this journal is cited, in accordance with accepted academic practice. No use, distribution or reproduction is permitted which does not comply with these terms.
*Correspondence: S. Gopala Krishnan, Z29wYWxfaWNhckB5YWhvby5jby5pbg==