- 1College of Forestry and Landscape Architecture, South China Agricultural University, Guangzhou, China
- 2South China Botanical Garden, Chinese Academy of Sciences, Guangzhou, China
- 3Guangdong Provincial Key Laboratory of Silviculture, Protection and Utilization, Guangdong Academy of Forestry, Guangzhou, China
Vegetation succession can change the function and quality of the soil. Exploring the changes in soil properties during secondary forest restoration is of great significance to promote forest restoration and improve the ecological service function of subtropical ecosystems in South China. In this study, we chose three typical forests in subtropical China as restoration sequences, broadleaf–conifer mixed forest (EF), broad-leaved forest (MF), and old-growth forest (LF), to study the changes in soil physico-chemical and biological properties and the changes of soil comprehensive quality during the secondary succession of subtropical forest. The results showed that the soil physical structure was optimized with the progress of forest succession. The soil bulk density decreased gradually with the progress of forest restoration, which was significantly affected by soil organic carbon (p < 0.01). In LF, the soil moisture increased significantly (p < 0.05), and its value can reach 47.85 ± 1.93%, which is consistent with the change of soil porosity. With the recovery process, soil nutrients gradually accumulated. Except for total phosphorus (TP), there was obvious surface enrichment of soil nutrients. Soil organic carbon (15.43 ± 2.28 g/kg), total nitrogen (1.08 ± 0.12 g/kg), and total phosphorus (0.43 ± 0.03 g/kg) in LF were significantly higher than those in EF (p < 0.05). The soil available nutrients, that is, soil available phosphorus and available potassium decreased significantly in LF (p < 0.05). In LF, more canopy interception weakened the P limitation caused by atmospheric acid deposition, so that the soil C:P (37.68 ± 4.76) and N:P (2.49 ± 0.24) in LF were significantly lower than those in EF (p < 0.05). Affected by TP and moisture, microbial biomass C and microbial biomass N increased significantly in LF, and the mean values were 830.34 ± 30.34 mg/kg and 46.60 ± 2.27 mg/kg, respectively. Further analysis showed that total soil porosity (TSP) and TP (weighted value of 0.61) contributed the most to the final soil quality index (SQI). With the forest restoration, the SQI gradually increased, especially in LF the value of SQI was up to 0.84, which was significantly higher than that in EF and MF (p < 0.05). This result is of great significance to understanding the process of restoration of subtropical forests and improving the management scheme of subtropical secondary forests.
Introduction
Forests are universally known to be one of the most important terrestrial ecosystems in terms of the diverse ecological functions they serve, including carbon sequestration, biodiversity conservation, water, soil conservation and cleaning the air, etc. (Jim and Chen, 2009; Song et al., 2016; Kuehler et al., 2017). A serious environmental crisis has been caused by the reduction of forest area thus, forest restoration has gained a lot of attention from governments and ecologists (Moreno-Mateos et al., 2017). In recent years, ecosystem recovery which is proposed to solve the degradation issues has increasingly occurred worldwide (Moreno-Mateos et al., 2017).
Forest restoration has been documented to be accompanied by changes in the species’ structure and soil evolution (Prestes et al., 2020). Over the past few decades, a large number of studies have emerged on the succession process of subtropical secondary forests. It was reported that the diversity of species and canopy increased during the development of forest phytocenoses, together with the enhancement of the connection between populations (Bruelheide et al., 2011; Zhang et al., 2021). In addition, those changes in forest vegetation mentioned above also affect the amount of solar radiation reaching the forest floor (Dietz et al., 2020; Onoda and Bando, 2021). The inter-relationship between the vegetation and climate factors forms distinctive microhabitat conditions in different forests, which alters soil properties by affecting the process of litter decomposition and root development, and further impacts the coupling relationship of soil C-N, C-P, and N-P. According to interactive soil–vegetation feedback, the plant communities improve the soil structure and fertility, which ulteriorly allows the community to develop (Brooker et al., 2015; Chai et al., 2019). There is a natural coupling relationship between soil and vegetation systems, which is an important mechanism of forest development and succession, especially on a small spatial scale (van der Putten et al., 2013; Huang et al., 2018). In other words, forests in different succession stages influence soil status to various magnitudes (Pang et al., 2018).
Pedosphere is an active interface in the biogeochemical cycle of forest ecosystems, and its physical, chemical, and microbial characteristics can indicate the process of soil formation, soil development, and fertility along forest succession (Chen et al., 2019a). Soil quality index (SQI) can be used as a parameter that integrated various physical, chemical, and biological indexes to judge the development of soil, and find out the main controlling factors affecting the change of soil, so as to facilitate future operation and management. Shao et al. (2020) reported that water holding capacity, soil organic matter, total nitrogen, available potassium, and altitude appeared to be the main limiting factors of soil quality of Mount Tai, China. Muñoz-Rojas et al. (2016) found that in addition to organic carbon and C:N ratio, biological indicators are the most sensitive indicators to detect differences among soils in semiarid areas. Currently, few relevant studies have been conducted with respect to the impact of secondary forest restoration on soil properties in subtropical China, which limits the overall evaluation of soil quality in subtropical areas.
Xinfengjiang National Forest Park is located in Guangdong Province, China, and it includes forest types in different succession stages. Due to extensive deforestation in the 1950s, most of the stands in the study site are now secondary forests (Zhang et al., 2019b). In 1984, the Chinese government promulgated a new forest law, which implemented quota cutting for forests and encouraged afforestation. Since then, deforestation was prohibited in this study area and the forests here were allowed to gradually undergo self-succession in the three decades that followed. As those forests experienced intensive human disturbance and strict protection, they can be regarded as a suitable system to study the changes in soil properties along with the restoration in subtropical secondary forests. We selected three types of forests, named broadleaf–conifer mixed forest (EF), broad-leaved forest (MF), and old-growth forest (LF), which represent a typical forest restoration sequence in subtropical China. In this paper, we assume that the overall soil quality will be improved to a certain extent with forest succession. We intend to provide answers to the following problems. Firstly, how do soil physico-chemical properties and biological properties change along with forest succession? Secondly, what is the relationship between different soil properties? Thirdly, in the process of forest succession, what are the main controlling factors affecting the change of the soil? Our findings may provide references for sustainable forest management in South China.
Materials and Methods
Site Description
The study area is located in the Xinfengjiang National Forest Park (23°40′30″–24°46′39″ N, 114° 30′33″–114°36′30″ E), China, with a total area of 4479.47 hectares. The region has a subtropical monsoon climate, with a forest coverage rate of 78%. The vegetation types are mainly an evergreen broad-leaved forest, an evergreen coniferous forest, and a broadleaf–conifer mixed forest. The average temperature is 21.2°C and the annual average precipitation is about 1,420 mm, mainly from April to September, and the average relative humidity is about 76%. Bedrocks are classified as granite, basalt, and sandshale. Soils are classified as yellow and red earth, mostly medium loam and heavy loam (Huang et al., 2018).
In 2014, six plots were permanently set up in the study area, of which two duplicate plots were established for each stand type (Figure 1). Each plot with an area of 50 m × 50 m was close to the basic factors, such as altitude and slope. The selected plots covered three different types of forests in the area: a broadleaf–conifer mixed forest (EF), a broad-leaved forest (MF), and an old-growth forest (LF), which represented a sequence of forest restoration based on tree species composition and the degree of interference (Huang et al., 2018). The broadleaf–conifer mixed forest was developed from coniferous tree species, and the dominant tree species included Pinus massoniana and Cunninghamia lanceolata. The broad-leaved forest was partially logged before conservation, and the dominant tree species are Castanopsis chinensis, Schefflera octophylla, and Itea chinensis. The old-growth forest is relatively close to the central area and has not been disturbed much in the past, so it can be regarded as a well-developed forest in the later stage of succession. Its dominant tree species are Schima superba, Cinnamomum porrectum, and Machilus thunbergii.
Soil Sampling
In every plot, three randomly repeated small quadrats were set up with an area of 10 m × 10 m. We dug two holes about 1.3 m × 1 m in size for collecting soil samples in each quadrat. Soil samples were taken at 0–25 cm, 25–50 cm, 50–75 cm, and 75–100 cm layers for the determination of the soil’s physical and chemical properties. The soil microbial biomass was determined using the samples taken at 0–30 cm, 30–60 cm, and 60–90 cm layers. Soil sampling was conducted once during the rainy season and dry season, respectively, a total of 66 samples were collected (24, 24, and 18 for the determination of physical, chemical properties, and microbial biomass, respectively) each time for each stand. Three duplicate undisturbed soil cores were collected with 100 cm3 stainless steel cylinders from each soil layer. The disturbed soil samples were collected using a soil drill with a diameter of 3.5 cm from five points, at the four corners and the center of each quadrat, and then the samples from the five points in each layer were fully mixed making up the composite samples (the weight of each composite sample was about 1 kg). The fresh soil samples from every 30 cm were stored in a 4°C refrigerator for the determination of soil microbial biomass C (MBC) and microbial biomass N (MBN).
Measurements
The bulk density (BD), soil water content (SWC), and porosity were determined using the undisturbed soil sample cores using Kopecky’s cylinder method (Piaszczyk et al., 2020; Grzywna and Ciosmak, 2021). We first recorded the weight of the empty cylindrical soil auger with a volume of 100 cm3 (m0). After sampling, we weighed the cylindrical soil auger with the contained fresh soil sample (m1). We then soaked the cylindrical metal core with the undisturbed soil in water for 12–14 h and weighed it thereafter (m2). The specific calculation formula used is as follows:
where SWC is water content of soil (%); md is the dry soil weight (g); MWHC is the maximum water holding capacity (%).
Plant roots, debris, and gravels were removed before the analysis of the mixed soil, and the soil samples from every 25 cm were screened through a 2-mm mesh for available phosphorus (AP) and available potassium (AK); through a 1-mm mesh for soil alkaline hydrolysis nitrogen (AN); and through a 0.25-mm mesh for soil organic matter (SOM), total nitrogen (TN) and total phosphorus (TP; Chen et al., 2020). The SOM was quantified using the potassium dichromate oxidation method (Dong et al., 2018; Yang et al., 2020). The van Bemmelen factor (1.724) was used for conversion between SOM and SOC. The total nitrogen (TN) amount was measured using the Kjeldahl digestion method (Cao et al., 2020). The content of TP was determined using hydrochloric acid, hydrofluoric acid, and nitric acid digestion-inductively coupled plasma atomic emission spectrometry (ICP-AES; He et al., 2021). The content of AN was measured by the alkali-hydrolysis and diffusion method (Cui et al., 2018). The amount of AP was detected using a spectrophotometer (Wu et al., 2019). The content of AK was quantified using a flame photometer after the samples had been prepared using an ammonium acetate solution (Wu et al., 2019). The contents of MBC and MBN were determined by the chloroform fumigation extraction method (Dong et al., 2018).
Evaluation of Soil Quality Index
We selected a soil quality index (SQI) to comprehensively represent all the indicators we had measured to evaluate the overall soil quality. It has been widely used under the condition of minimum data sets (MDS), which enables researchers to select the most representative soil indicators and reduce data redundancy (Zhang et al., 2019a). The establishment of SQI mainly includes three steps: (1) selecting appropriate indicators to build MDS, (2) calculating the MDS index score and determining the index weight, (3) combining the index scores into SQI.
The principal component analysis method was used to screen MDS, select the principal components with values greater than 1, and rotate the maximum variance of the selected principal components to enhance the interpretability of irrelevant components (Li et al., 2013). In each selected principal component, the index with the absolute value of the selected factor load within 10% of the maximum factor load was the high factor load index (D’Hose et al., 2014). When only one high factor load index was left in the main component, the index enters MDS; when more than one high factor load index was left in the main component, Pearson’s correlation analysis was used to check whether other indexes should be deleted. If the correlation coefficient r < 0.7, all indexes were selected into MDS; if the correlation r > 0.7, the high factor load index with the largest sum of correlation coefficients was selected into MDS (Li et al., 2013; Zhang et al., 2019a). After determining the indicators of MDS, a non-linear scoring function was performed to transform the soil indicators into scores that ranged from 0 to 1. The sigmoidal function [Eq. (3)] was used as follows (Ciarkowska and Gambus, 2020):
Where S is the score of soil indicator, a is the maximum score (a = 1) reached by the function, x is the value of the indicator, x0 is the mean value of each soil indicator, and b is the value of the equation’s slope (Zhang et al., 2019a). Slope values (b) of −2.5 was set for “more is better” and 2.5 was set for “less is better” curve, respectively (Ciarkowska and Gambus, 2020). Weighting values for every indicator were assigned by commonality which was calculated using factor analysis (FA; Rahmanipour et al., 2014). Finally, with the scores and weighting values, SQI was calculated using the following equation [Eq. (4); Zhang et al., 2019a]:
Where Wi is the weighting value of the selected soil indicators determined by PCA, Si is the indicator score calculated by Eq. (3), and n is the number selected in MDS.
Statistical Analysis
Before statistical data analysis, the Kolmogorov–Smirnov test (p = 0.05) was performed on the experimental data and the normality of the data was judged. One-way ANOVA was used to compare the differences in soil properties between different vegetation types and soil depths. When the ANOVA results were significant according to the F value (p < 0.05), Duncan’s test was performed to compare the mean differences of soil variables. All sample mean values and standard errors in this study were processed and analyzed by Microsoft Excel 2010 (Microsoft Corporation, Redmond, WA, United States) and IBM SPSS Statistics 22.0 (International Business Machines Corporation, Armonk, NY, United States), and the data results were plotted using Origin 2021 (Originlab Corporation, Northampton, MA, United States).
Results
Differences in the Soil Physico-Chemical Properties of the Three Forests
As shown in Table 1, the BD increased with increasing soil depth in different forests, and the BD of the surface layer was significantly lower than that of other soil layers. The BD for the 0–100 cm depth gradually decreased along forest restoration, that is, EF (1.33 ± 0.03 g/cm3) > MF (1.28 ± 0.03 g/cm3) > LF (1.16 ± 0.03 g/cm3). The TSP decreased with increasing soil depth gradually in EF and MF, while the change in TSP was not significant between layers in LF. When averaged across the 0–100 cm depth, the TSP value was significantly decreased in the order LF (52.36 ± 0.85%) > MF (45.53 ± 1.17%) > EF (42.41 ± 0.85%). In this study, soil porosity in three forests consisted mainly of capillary porosity. As forest restoration continued, the CP increased significantly from 33.63 ± 0.93% (EF) to 48.77 ± 0.99% (LF). When averaged across the 0–100 cm depth, the NCP in EF (8.79 ± 0.72%) was significantly higher than that in MF (4.61 ± 0.48%) and LF (3.60 ± 0.54%). The MWHC was regarded as the amount of water held by the soil when all its pores are filled with water. In each forest, the MWHC gradually decreased with increasing soil depth. When averaged across the 0–100 cm depth, the MWHC (47.85 ± 1.93%) in LF was significantly higher than that in other forests (about 32.63 ± 1.39% in EF and 37.27 ± 1.59% in MF, respectively).
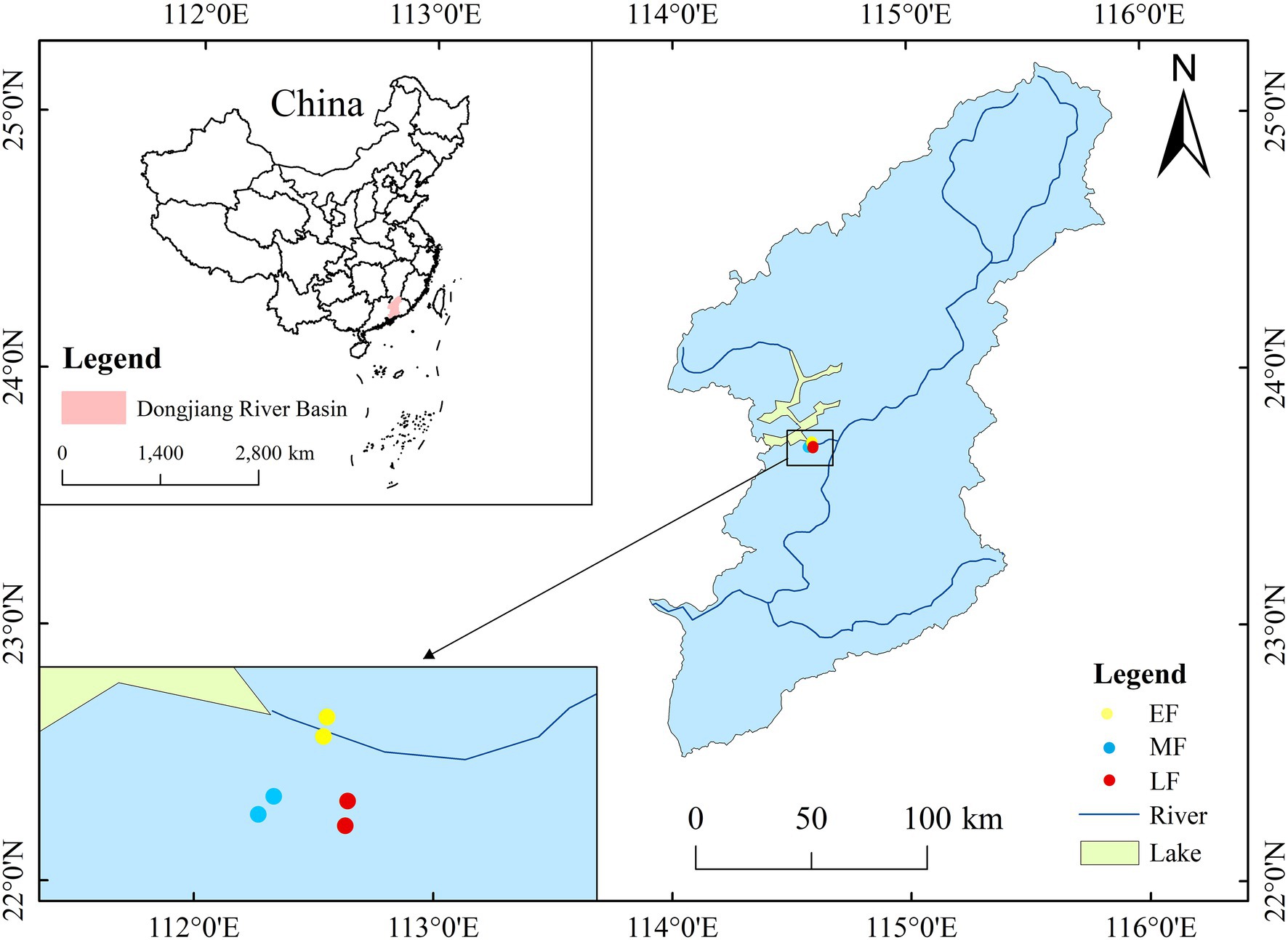
Figure 1. Location of the study area at the Xinfengjiang National Forest Park, Guangdong Province, China.
Differences in the Soil Nutrient Contents of the Three Forests
As shown in Figures 2A,B, the content of SOC and TN of each forest showed significant surface enrichment in the soil profile. The SOC content of the surface layer (0–25 cm) increased significantly alongside forest restoration with values ranging from 19.83 ± 1.69 g/kg to 32.03 ± 3.93 g/kg, and the TN content of LF was highest (1.92 ± 0.24 g/kg) among forests. When averaged across the 0–100 cm depth, the SOC content between forests was in the order LF (15.43 ± 2.28 g/kg) > MF (10.27 ± 1.40 g/kg) > EF (9.64 ± 1.41 g/kg), and the TN content increased from 0.67 ± 0.09 g/kg (EF) to 1.08 ± 0.12 g/kg (LF). As shown in Figure 2C, the variation of the TP content in the vertical section was relatively stable. The TP content for the 0–100 cm depth between forests increased in the order EF (0.13 ± 0.01 g/kg) < MF (0.28 ± 0.03 g/kg) < LF (0.43 ± 0.03 g/kg).
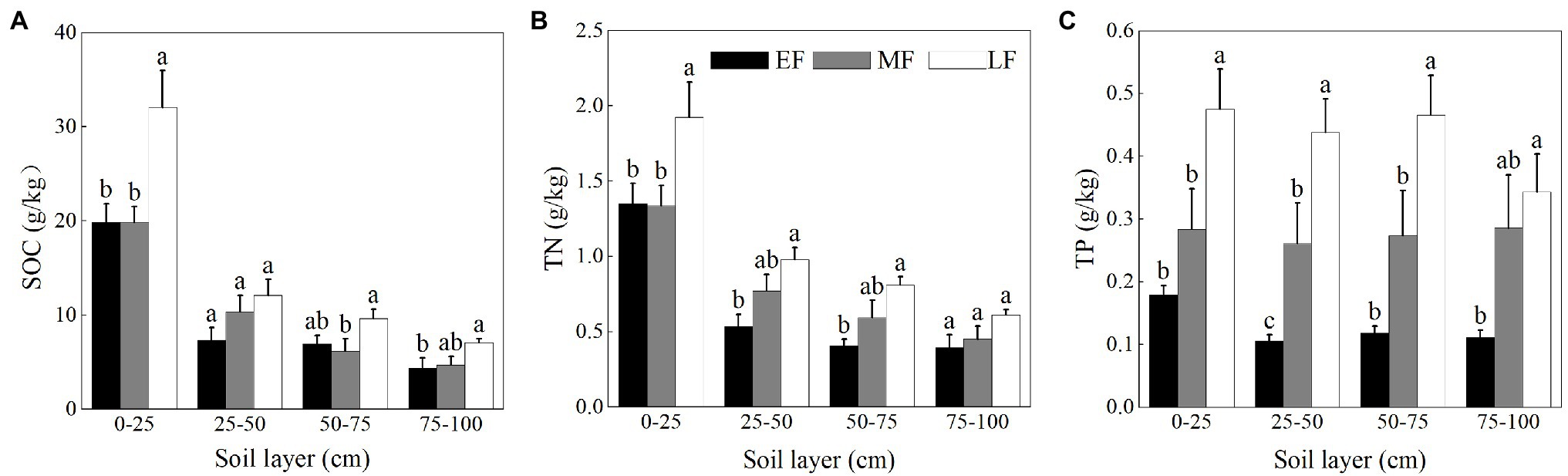
Figure 2. The contents of SOC (A), TN (B), and TP (C) in the 0–100 cm soil layer of different forests. Values are the means ± SE (n = 6). Lowercase letters above the columns represent statistically significant differences among stand types for the same soil layer (Duncan’s tests, p < 0.05). SOC, soil organic carbon; TN, soil total nitrogen; TP, soil total phosphorus; EF, broadleaf–conifer mixed forest; MF, broad-leaved forest; LF, old-growth forest.
As shown in Figure 3A, the AN content in the surface soil (0–25 cm) was significantly higher than that of the other soil layers in each forest. When averaged across the 0–100 cm depth, the AN content was ranked as LF (90.85 ± 9.98 mg/kg) > MF (76.80 ± 8.52 mg/kg) > EF (64.05 ± 8.97 mg/kg). The AP content of the surface soil (0–25 cm) in EF (1.20 ± 0.11 mg/kg) was significantly higher than that in other forests (0.58 ± 0.08 mg/kg in MF and 0.54 ± 0.10 mg/kg in LF, respectively; Figure 3B). The AP content for the 0–100 cm depth decreased from 0.64 ± 0.08 mg/kg (EF) to 0.39 ± 0.04 mg/kg (LF). As shown in Figure 3C, the AK content showed a decreasing trend with increasing soil depth in each forest, the average value of which was significantly decreased in the order EF (75.53 ± 6.81 mg/kg) > MF (49.13 ± 6.39 mg/kg) > LF (26.45 ± 3.73 mg/kg) for the 0–100 cm depth.
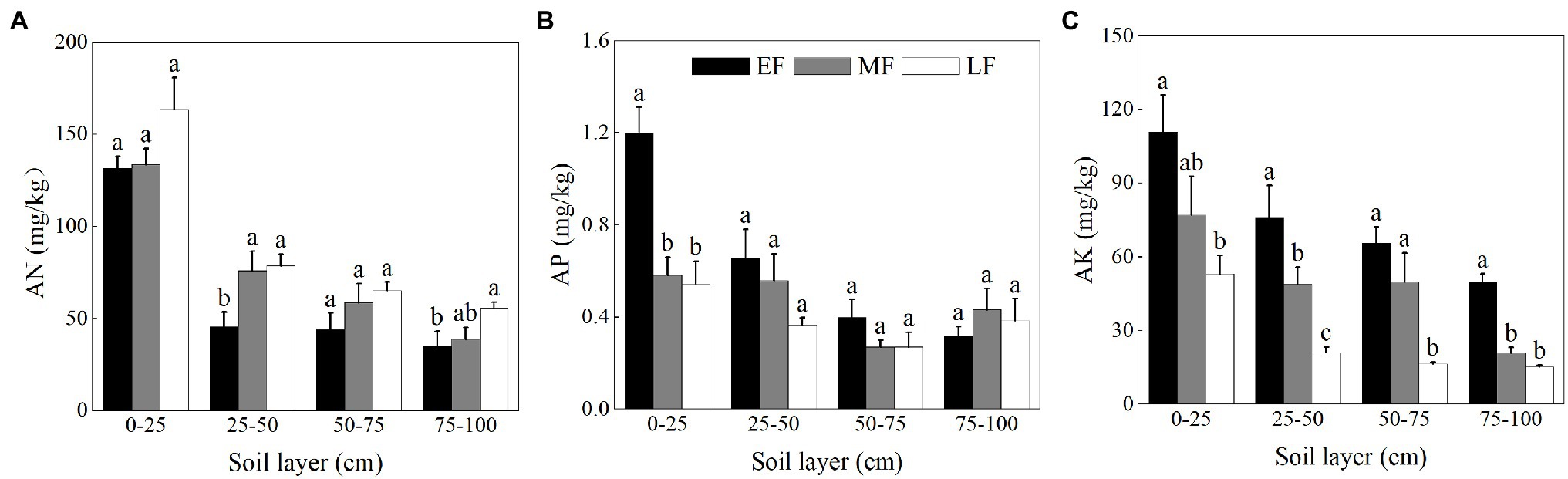
Figure 3. The contents of AN (A), AP (B), and AK (C) in the 0–100 cm soil layer of different forests. Values are the means ± SE (n = 6). Lowercase letters above the columns represent statistically significant differences among stand types for the same soil layer (Duncan’s tests, p < 0.05). AN, alkali-hydrolyzable nitrogen; AP, available phosphorous; AK, available potassium; EF, broadleaf–conifer mixed forest; MF, broad-leaved forest; LF, old-growth forest.
Differences in the Soil Stoichiometric Characteristics of the Three Forests
As shown in Figures 4A,B, the C:N and C:P showed an overall decreasing trend in the vertical soil profile in each forest. There was no significant difference in C:N at the depth of 0-100 cm between the three forests, which were 13.36 ± 0.88(EF), 12.23 ± 0.79(MF), 13.08 ± 0.69(LF). The C:P in the 0–25 cm soil layer was significantly higher than that in other soil layers (Figure 4B). When averaged across the 0–100 cm depth, the C:P decreased from 72.33 ± 6.90 (EF) to 37.68 ± 4.76 (LF). As shown in Figure 4C, the average N:P differed significantly between forests, that is, EF (4.64 ± 0.35) > MF (3.49 ± 0.42) > LF (2.49 ± 0.24).
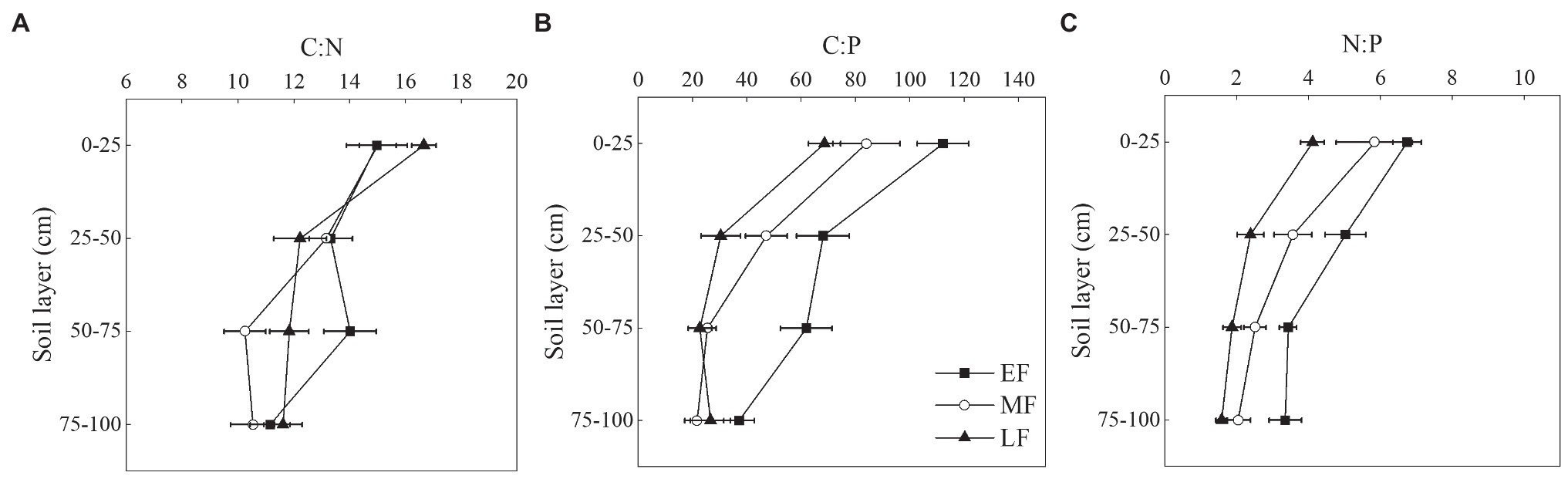
Figure 4. Vertical changes of C:N (A), C:P (B), and N:P (C) in the 0–100 cm soil layer of different forests. Values are the means ± SE (n = 6). C:N, SOC:TN; C:P, SOC:TP; N:P, TN:TP; EF, broadleaf–conifer mixed forest; MF, broad-leaved forest; LF, old-growth forest.
As shown in Figure 5A, the MBC did not differ significantly among soil layers in each forest. Within the 0–90 cm soil layer, there was a significant difference in the MBC between forests, that is, LF (830.34 ± 30.34 mg/kg) > MF (480.36 ± 19.77 mg/kg) > EF (319.03 ± 14.24 mg/kg). As shown in Figure 5B, the MBN for the 0–90 cm depth was significantly higher in LF (46.60 ± 2.27 mg/kg) than in other forests (15.78 ± 0.68 mg/kg in EF and 21.90 ± 1.41 mg/kg in MF, respectively). In each forest, the MBC:MBN was not significantly different between layers. When averaged across the 0–90 cm depth, there was no significant difference in the MBC:MBN between forests, that is, LF (19.75 ± 1.00) < EF (22.71 ± 1.55) < MF (23.66 ± 1.33) (Figure 5C).
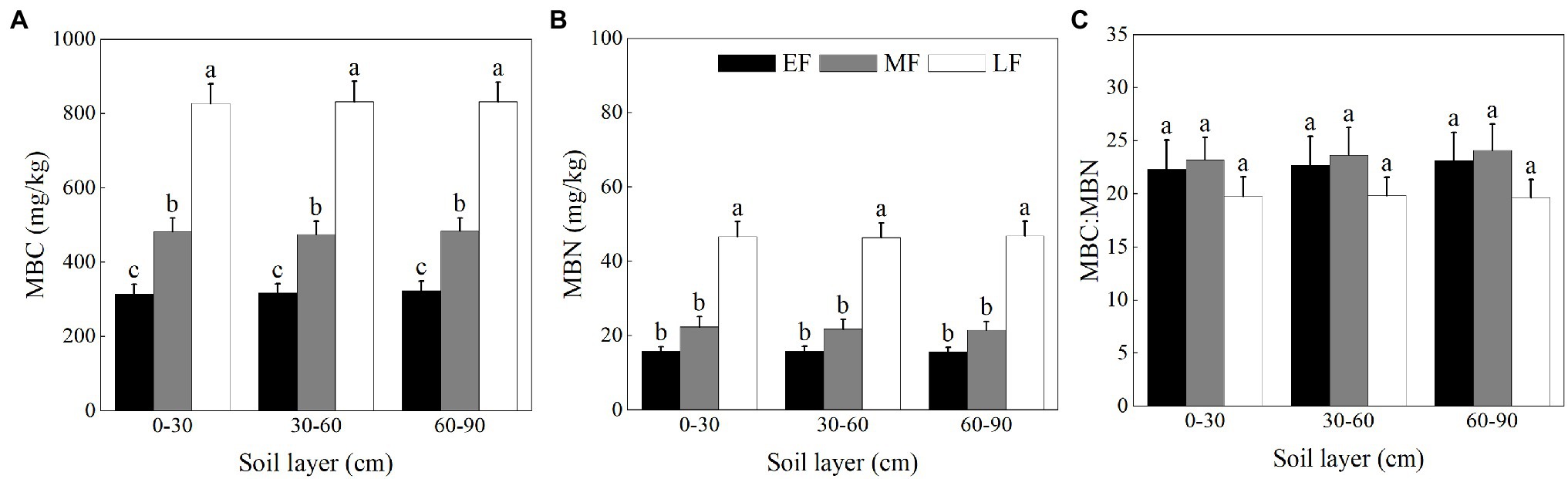
Figure 5. The contents of MBC (A), MBN (B), and the ratio of MBC:MBN (C) in the 0–100 cm soil layer in different forests. Values are the means ± SE (n = 6). Lowercase letters above the columns represent statistically significant differences among stand types for the same soil layer (Duncan’s tests, p < 0.05). MBC, soil microbial biomass C; MBN, soil microbial biomass N; EF, broadleaf–conifer mixed forest; MF, broad-leaved forest; LF, old-growth forest.
The Relationship Between Soil Properties and Comprehensive Evaluation
Figure 6 shows the relationship between different soil properties. The BD was negatively correlated with TSP (−0.79, p < 0.05) and SOC (−0.90, p < 0.01). MWHC was mainly affected by TSP (0.85, p < 0.01) and SOC (0.87, p < 0.01). The SOC was closely related to TN (0.99, p < 0.01) and AN (0.97, p < 0.01). The physical properties of soil also have a certain impact on the stoichiometric characteristics. The NCP was positively correlated with C:P (0.87, p < 0.01) and N:P (0.86, p < 0.01). As for soil microbial biomass C and N, the TP was positively correlated with MBC and MBN, and the correlation coefficients were 0.96 and 0.94, respectively (p < 0.01). While the content of AK was negatively correlated with MBC and MBN, and the correlation coefficients were −0.73 and −0.69, respectively (p < 0.05).
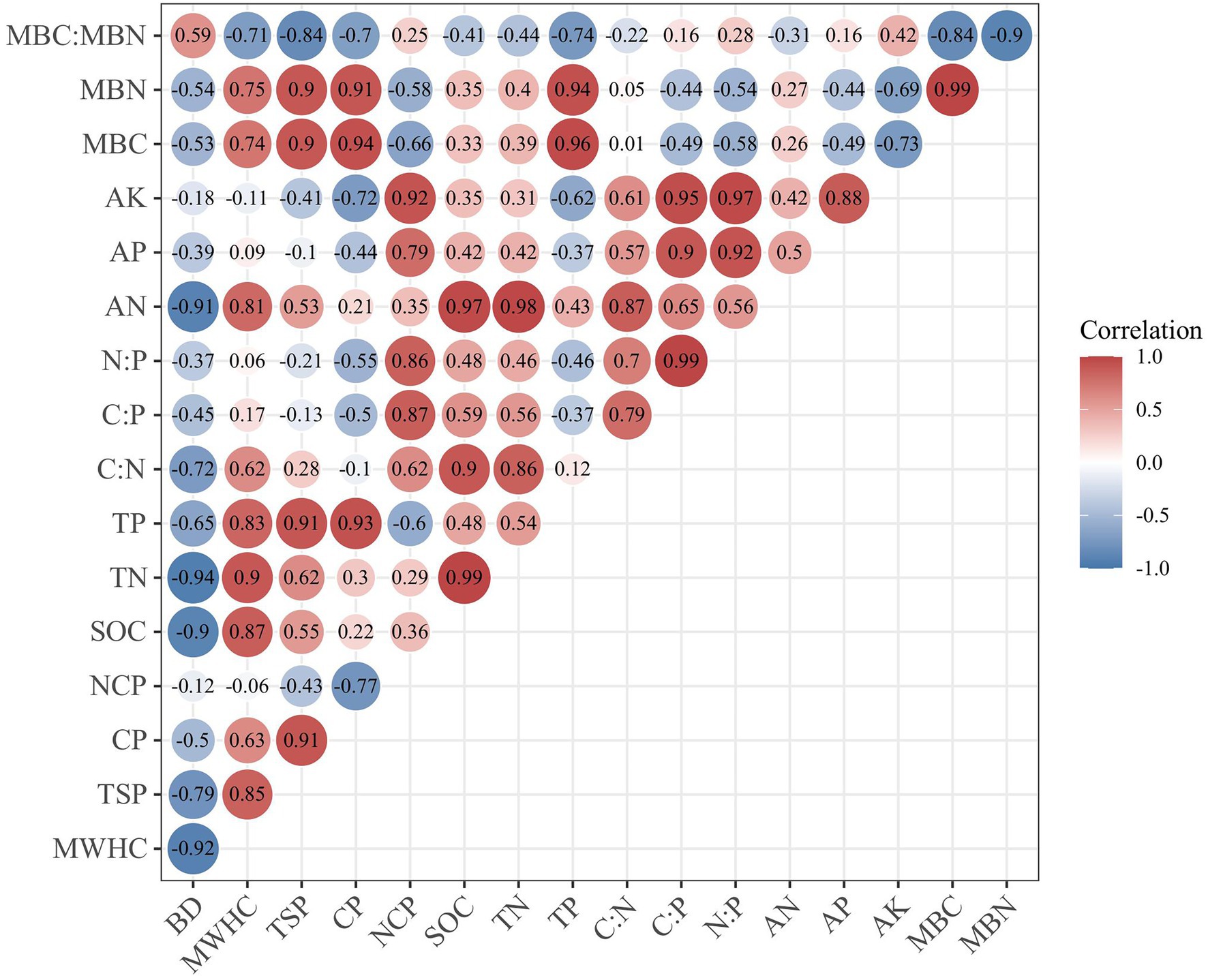
Figure 6. Pearson’s correlation coefficients among soil properties. BD, bulk density; MWHC, maximum soil water holding capacity; TSP, total soil porosity; CP, capillary porosity; NCP, non-capillary porosity; SOC, soil organic carbon; TN, soil total nitrogen; TP, soil total phosphorus; AN, alkali-hydrolyzable nitrogen; AP, available phosphorous; AK, available potassium; MBC, soil microbial biomass C; MBN, soil microbial biomass N.
A comprehensive index, that is, SQI was established to evaluate the change in soil properties. Based on the principal component analysis and the correlation between various properties, a soil quality index equation was constructed. The principal component analysis showed that values of the first two PCs were all ≥1 and explained 94.22% of the total variance. TSP, TP, and MWHC in the first principal component (PC1) are high-weight indicators (Appendix 1). MWHC had a high correlation with TSP and TP (Figure 6), and the load coefficients of TSP and TP were the same (Appendix 1), so TSP and TP were retained in PC1. In the second principal component (PC2), AP and AK were highly weighted factors and are significantly correlated with each other. AK was selected in PC2 because AK had a higher load factor (Appendix 1). Finally, we selected TSP, TP, and AK in MDS to calculate SQI. With the weighting values based on PCA (Appendix 2), the calculation of SQI was given by Eq. (5):
According to the calculation results of Eq. (5), the soil quality had been improved, respectively, during the forest restoration [(p < 0.05), that is, LF (0.84) > MF (0.72) > EF (0.60; Figure 7)].
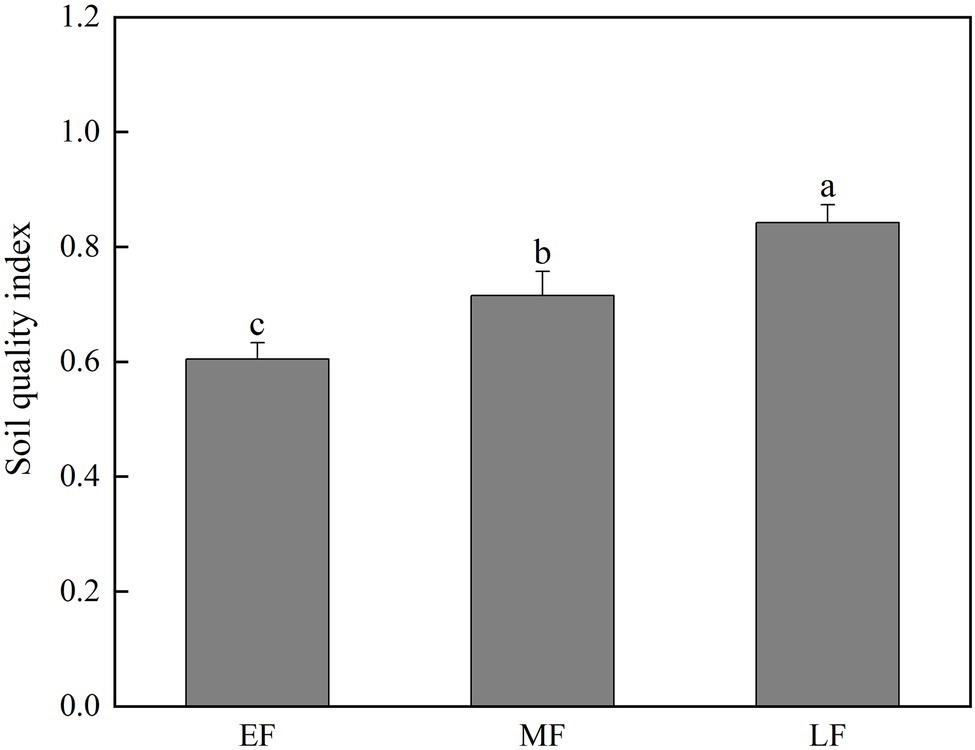
Figure 7. The 0–100 cm soil quality index under different forests types. Values are the means ± SE (n = 6). The lowercase letters above the column represent the statistically significant differences between different stand types (Duncan’s tests, p < 0.05). EF, broadleaf–conifer mixed forest; MF, broad-leaved forest; LF, old-growth forest.
Discussion
Changes in Soil Physico-Chemical Properties During Forest Restoration
In this study, the SOM increased along forest succession, consistent with the results of Huang et al. (2018) and Bu et al. (2018). The increase of SOM promotes the growth of roots and the activity of soil microorganisms, which is conducive to the development of soil pores and the formation of soil aggregates. In addition, the chemical conditions created by the secretion of organic acids by roots, microorganisms, and organic matter decomposition also promote the fracturing of soil particles, which eventually leads to the decrease of BD and the improvement of MWHC (Marschner et al., 2011; Liu et al., 2019; Pastore et al., 2020). In the vertical soil profile of different forests, the BD in the surface layer was significantly lower than that in other soil layers. This is because litter decomposition inputs a large amount of soil organic matter into the surface layer, which improves the surface soil structure (Porter et al., 2010; Freschet et al., 2013). Secondly, the increased density of deep soil was caused by the compaction of the soil by root growth (Shah et al., 2017). The combined action of these two processes led to a state of loose soil in the upper layer and compaction in the lower layer.
Vegetation types can significantly affect the soil’s physical and chemical properties (Srivastava et al., 2020; Wang et al., 2020). The research showed that there were significant differences in soil nutrient characteristics among the three forests. The SOC, TN, and TP were significantly higher in the late stage of forest restoration than that in other stages, indicating that vegetation restoration was conducive to the improvement of the soil carbon sink effect in south China (Hu et al., 2021). This phenomenon has been confirmed by a large number of studies (Jiang et al., 2017; Zhang et al., 2018). The vegetation provides the carbon and energy sources for the soil through root exudates and plant residues (Zhu et al., 2010) which may be related to the plant diversity and species turnover (Smith et al., 2002). As positive forest succession progresses, the increasing amounts of litter return result in higher content of SOM (Bu et al., 2018). Thereafter, root growth is promoted and fine root biomass increases (Feng et al., 2019), thus the SOC and TN increase significantly. It has been reported that the SOC played a key role in the availability of soil N and P, which could be explained in two aspects (Liu et al., 2021). On the one hand, the SOC could reduce the adsorption of N and P by competing with soil exchangeable base cations for adsorption sites (Schreeg et al., 2013; Liu et al., 2021). On the other hand, the SOC enhances the activity of soil microorganisms by providing energy, potentially promoting soil N and P mineralization (Lange et al., 2015; Chen et al., 2019b). The vertical distribution pattern of forest soil nutrients is related to its source (Bai et al., 2019). Our results showed that the SOC and TN were concentrated on the soil surface with dense roots, which were conducive to the absorption of nutrients by plants. This was consistent with the study by Liu et al. (2020). The content of the TP in each soil layer does not change by much in our study. A possible explanation for this was that the P was extracted from the soil parent material as a consequence of mainly weathering and biology. In addition, the growing forests satisfied the increasing nutrient demand by extending their roots deeper into the soil, which also reduced the spatial heterogeneity of P (Guo et al., 2021; Pastore et al., 2022).
The total amount of soil nutrients reflects the capacity of nutrient storage, while the available nutrient content mirrors the validity of nutrients. Analysis data indicated that the AN showed an increasing trend with the chronosequence, while the AP and AK showed an opposite trend. Researchers proposed that the concentrations of soil available nutrients (i.e., AN, AP, and AK) were species-dependent, which could be explained by the “complementary” or “mass” effects related to plant diversity in forest ecosystems (Zheng et al., 2017; Liu et al., 2021). Han et al. (2021) demonstrated that the content of AK showed a positive correlation with BD. In LF, BD decreased while nutrient leaching increased, which may also be the reason for the decline in soil AK content with forest succession. In this study, different kinds of soil available nutrients in each forest showed a downward trend in the profile. According to the results of correlation analysis, the AN showed a significantly positive relationship with MWHC (0.81) and SOC (0.97), consistent with the results of Gairola et al. (2012), which could be attributed to the patterns of litter return and water distribution. The AP (0.79) and the AK (0.92) showed a significantly positive relationship with NCP. The leaching properties of the soils might dominate the distribution of AP and AK among layers.
Changes in Soil Stoichiometric Ratio During Forest Restoration
Soil stoichiometry is the core of C, N, and P biogeochemistry (Sardans and Peñuelas, 2015), which regulates the aboveground and underground nutrient cycle of forest ecosystems (Güsewell and Gessner, 2009). In this study, soil C:N had no significant difference among forests, but the C:P and N:P significantly decreased alongside forest restoration. This situation might be due to the high level of atmospheric acid deposition in south China. In the early stage of forest restoration in a broadleaf–conifer mixed forest, the precipitation interception effect of the forest canopy was lower than that of the broad-leaved forest therefore, more acid rain fell on the soil surface (Li et al., 2015). Nitrogen (N) is one of the main elements that get deposited in the process of atmospheric acid deposition. The combination of exogenous N and acid deposition exacerbates the limitation of soil P (Tie et al., 2020). In the case of P limitation, microorganisms and plants usually secrete more phosphatase, which catalyzes the mineralization and decomposition of organic phosphorus to produce AP (Liu et al., 2014). Therefore, the content of AP in the early stage of forest restoration was higher than that in the late stage of forest restoration.
Along the soil vertical profile in this study, the stoichiometric ratio in the surface layer of different forests was higher than that in other soil layers. Subtropical China is one of the regions with the highest level of nitrogen deposition in the world (Liu et al., 2011). The increase of soil acidity under N deposition inhibits soil respiration by lowering bacterial and fungal biomass and proteolytic enzyme activity, which weakens the rates of litter decomposition and the ability of the microbial community to utilize a suite of C substrates, then the accumulation of soil carbon promotes (Mo et al., 2008). This phenomenon may further increase the C:N of surface soil and reduce the C:N of deep soil (Yu et al., 2017). Nitrogen deposition also promotes P absorption and plant growth (Deng et al., 2016; Li et al., 2016). In the soil surface layer with a dense root distribution, the decline of soil P concentration is more serious than that in the lower layer.
Changes in Soil Microbial Biomass C and N During Forest Restoration
Soil microbial biomass carbon (MBC) and microbial biomass nitrogen (MBN) are the main components of soil microbial biomass, and their subtle changes affect the turnover of the soil organic matter and nutrients (Yang et al., 2010). In this study, the contents of the MBC and the MBN increased significantly alongside forest restoration, and the MBC:MBN between forests was not significant. Vivanco and Austin (2008) reported that litter quality can affect microbial growth and reproduction by affecting microbial substrate decomposition and altering decomposition difficulty. Compared to the broad-leaved litter in the later stage of forest restoration, the contents of lignin, terpenoids, and phenols in the litter of the broadleaf–conifer mixed forest were more difficult to decompose, and the effectiveness of microbial substrate was lower. What’s more, the microbial groups and quantities that could be supported were relatively small in the broadleaf–conifer mixed forest (Chai et al., 2019; Yang et al., 2020). Therefore, the contents of the MBC and the MBN were relatively low in the early stage of forest restoration. In the vertical profile of soil, there was no significant difference in the MBC and the MBN contents among layers in each forest. Atmospheric acid deposition and litter in the subtropical forests of southern China provided a large amount of N and P elements to the soil. According to the results of the correlation analysis, the effect of N increment on the MBC of subtropical soils was not significant, and the soil microbial biomass was significantly affected by TP, which was consistent with Chu et al. (2007) P comes from the differentiation of the soil parent rock. In our study, the differences in P between different soil layers in each forest were not significant, which might be the reason for the insignificant difference in the contents of the MBC and the MBN between layers.
Soil microbial biomass is very sensitive to changes in soil environmental factors (Zhao et al., 2009). Hu et al. (2016) proposed that the improvement of soil porosity and moisture was the main reason for the increase in microbial biomass and activity. Larger soil porosity would make soil aeration better, which could provide optimal environmental conditions for soil microorganisms (Marinari et al., 2000). In this study, it was also reported that the contents of MBC (0.94, 0.90) and MBN (0.91, 0.90) were significantly positively correlated with soil capillary porosity and total porosity. Researchers proposed that larger soil porosity always indicated a stronger capacity of soil potential water conservation (Marinari et al., 2000). A synergistic relationship between soil microorganisms and soil moisture has been confirmed by a large number of studies (Kaneda and Kaneko, 2011; Borowik and Wyszkowska, 2016; Hawkes et al., 2017). In our study, the contents of the MBC (0.74) and the MBN (0.75) were positively correlated with the MWHC. As a critical carrier of material cycle and energy exchange, soil moisture plays an important role in the hydrological processes and nutrient circulation of forest ecosystems (Laclau et al., 2013). Large amounts of soil water would transport or diffuse soil nutrients to places where microorganisms need it (Illeris et al., 2003), thus microbial biomass became more abundant in pace with the soil water content improvement (Zhang et al., 2005; Baldrian et al., 2010). On the contrary, low soil water content would limit the mobility of nutrients and microbial activity.
Changes in Soil Quality Index During Forest Restoration
Based on the basic properties of soil, a comprehensive index (SQI) was constructed to reflect the changes in soil under forest restoration, which is of great significance to evaluate sustainable land use and effective management (Zhang et al., 2019a). In this study, 13 indexes based on soil microbial biomass and soil physico-chemical properties were selected, and MDS was selected by principal component analysis to evaluate soil quality. From the evaluation results, the soil quality in this area is relatively good, and with the forest restoration, the soil quality has improved to a certain extent, which is consistent with the previous research results (An et al., 2009; Yu and Jia, 2014; Guo et al., 2018). In our study, TSP and TP contributed the most to the comprehensive SQI, indicating that TSP and TP played an important role in determining soil quality. As an important index of soil nutrient content, TP can directly reflect soil fertility. P availability also further determines plant nutrient constraints and can affect processes important to ecosystem function (Guo et al., 2018). As an important physical index of soil, TSP can directly reflect the aeration, water storage, and hydraulic conductivity of soil (de Moraes et al., 2019). Therefore, it is very reasonable to select these two indicators in our MDS to reflect soil quality. In our study, due to the increase of litterfall production and turnover rate in the later stage of forest restoration, TSP and TP were improved, which further led to the increase in soil quality.
Conclusion
Our findings show that secondary forest restoration in southern China significantly affected soil physico-chemical and microbiological properties. As forest restoration proceeded, soil properties, that is, BD, porosity, and water holding capacity were significantly improved. The soil structure especially the topsoil was also optimized to a great extent. Total soil nutrients and soil microbial biomass gradually increased, while the amounts of the available nutrients, that is, AP and AK showed a downward trend. In addition to TP, there was a significant surface enrichment of forest soil nutrients. The SQI, which integrated physico-chemical and microbiological properties, demonstrated that higher soil quality was mainly affected by soil porosity and total phosphorus in this area. The SQI as a forest restoration evaluation method proved to be effective in revealing the local factors that require more attention for sustainable and scientific forestry management. The present study provides new evidence that soil properties improved during the restoration of a secondary subtropical forest, and is of great significance for evaluating the function and improving the management scheme of subtropical forests in South China.
Data Availability Statement
The datasets generated and analyzed during the current study are available from the corresponding authors on reasonable request.
Author Contributions
XZ, PL, and YF: data processing and writing. WZ: conceptualization and data collection. BN: language correction. FL, QZ, CQ, and XG: site maintenance and data collection. XL: experimental design and review. All authors contributed to the article and approved the submitted version.
Funding
This work was supported by the Forestry Science and Technology Innovation Project of Guangdong Province, China (2021KJCX003 and 2022KJCX015) and Operation Subsidy Program of Technology Innovation Platform of National Forestry and Grassland Administration (2021132084 and 2021132085).
Conflict of Interest
The authors declare that the research was conducted in the absence of any commercial or financial relationships that could be construed as a potential conflict of interest.
Publisher’s Note
All claims expressed in this article are solely those of the authors and do not necessarily represent those of their affiliated organizations, or those of the publisher, the editors and the reviewers. Any product that may be evaluated in this article, or claim that may be made by its manufacturer, is not guaranteed or endorsed by the publisher.
Acknowledgments
We gratefully acknowledge the support of the Dongjiangyuan Forest Ecosystem Research Station.
Supplementary Material
The Supplementary Material for this article can be found online at:
https://www.frontiersin.org/articles/10.3389/fpls.2022.878908/full#supplementary-material
References
An, S., Huang, Y., and Zheng, F. (2009). Evaluation of soil microbial indices along a revegetation chronosequence in grassland soils on the Loess Plateau, Northwest China. Appl. Soil Ecol. 41, 286–292. doi: 10.1016/j.apsoil.2008.12.001
Bai, X., Wang, B., An, S., Zeng, Q., and Zhang, H. (2019). Response of forest species to C:N:P in the plant-litter-soil system and stoichiometric homeostasis of plant tissues during afforestation on the Loess Plateau, China. Catena 183:104186. doi: 10.1016/j.catena.2019.104186
Baldrian, P., Merhautová, V., Petránková, M., Cajthaml, T., and Šnajdr, J. (2010). Distribution of microbial biomass and activity of extracellular enzymes in a hardwood forest soil reflect soil moisture content. Appl. Soil Ecol. 46, 177–182. doi: 10.1016/j.apsoil.2010.08.013
Borowik, A., and Wyszkowska, J. (2016). Soil moisture as a factor affecting the microbiological and biochemical activity of soil. Plant Soil Environ. 62, 250–255. doi: 10.17221/158/2016-PSE
Brooker, R. W., Bennett, A. E., Cong, W., Daniell, T. J., George, T. S., Hallett, P. D., et al. (2015). Improving intercropping: a synthesis of research in agronomy, plant physiology and ecology. New Phytol. 206, 107–117. doi: 10.1111/nph.13132
Bruelheide, H., Böhnke, M., Both, S., Fang, T., Assmann, T., Baruffol, M., et al. (2011). Community assembly during secondary forest succession in a Chinese subtropical forest. Ecol. Monogr. 81, 25–41. doi: 10.1890/09-2172.1
Bu, W., Huang, J., Xu, H., Zang, R., Ding, Y., Li, Y., et al. (2018). Plant functional traits are the mediators in regulating effects of abiotic site conditions on aboveground carbon stock-evidence from a 30 ha tropical forest plot. Front. Plant Sci. 9:1958. doi: 10.3389/fpls.2018.01958
Cao, L., Keener, H., Huang, Z., Liu, Y., Roger, R., and Xu, F. (2020). Effects of temperature and inoculation ratio on methane production and nutrient solubility of swine manure anaerobic digestion. Bioresour. Technol. 299:122552. doi: 10.1016/j.biortech.2019.122552
Chai, Y., Cao, Y., Yue, M., Tian, T., Yin, Q., Dang, H., et al. (2019). Soil abiotic properties and plant functional traits mediate associations between soil microbial and plant communities during a secondary forest succession on the loess plateau. Front. Microbiol. 10:895. doi: 10.3389/fmicb.2019.00895
Chen, X. D., Dunfield, K. E., Fraser, T. D., Wakelin, S. A., Richardson, A. E., and Condron, L. M. (2019a). Soil biodiversity and biogeochemical function in managed ecosystems. Soil Res. 58, 1–20. doi: 10.1071/SR19067
Chen, C., Fang, X., Xiang, W., Lei, P., Ouyang, S., and Kuzyakov, Y. (2020). Soil-plant co-stimulation during forest vegetation restoration in a subtropical area of southern China. For. Ecosyst. 7, 1–17. doi: 10.1186/s40663-020-00242-3
Chen, L., Xiang, W., Wu, H., Ouyang, S., Zhou, B., Zeng, Y., et al. (2019b). Tree species identity surpasses richness in affecting soil microbial richness and community composition in subtropical forests. Soil Biol. Biochem. 130, 113–121. doi: 10.1016/j.soilbio.2018.12.008
Chu, H., Lin, X., Fujii, T., Morimoto, S., Yagi, K., Hu, J., et al. (2007). Soil microbial biomass, dehydrogenase activity, bacterial community structure in response to long-term fertilizer management. Soil Biol. Biochem. 39, 2971–2976. doi: 10.1016/j.soilbio.2007.05.031
Ciarkowska, K., and Gambus, F. (2020). Building a quality index for soils impacted by proximity to an industrial complex using statistical and data-mining methods. Sci. Total Environ. 740:140161. doi: 10.1016/j.scitotenv.2020.140161
Cui, X., Zhang, Y., Gao, J., Peng, F., and Gao, P. (2018). Long-term combined application of manure and chemical fertilizer sustained higher nutrient status and rhizospheric bacterial diversity in reddish paddy soil of Central South China. Sci. Rep. 8, 16554–16511. doi: 10.1038/s41598-018-34685-0
de Moraes, M. T., Debiasi, H., Franchini, J. C., Bonetti, J. D. A., Levien, R., Schnepf, A., et al. (2019). Mechanical and hydric stress effects on maize root system development at different soil compaction levels. Front. Plant Sci. 10:1358. doi: 10.3389/fpls.2019.01358
Deng, M., Liu, L., Sun, Z., Piao, S., Ma, Y., Chen, Y., et al. (2016). Increased phosphate uptake but not resorption alleviates phosphorus deficiency induced by nitrogen deposition in temperate Larix principis-rupprechtii plantations. New Phytol. 212, 1019–1029. doi: 10.1111/nph.14083
D’Hose, T., Cougnon, M., De Vliegher, A., Vandecasteele, B., Viaene, N., Corneliset, W., et al. (2014). The positive relationship between soil quality and crop production: a case study on the effect of farm compost application. Appl. Soil Ecol. 75, 189–198. doi: 10.1016/j.apsoil.2013.11.013
Dietz, L., Collet, C., Dupouey, J. L., Lacombe, E., Laurent, L., and Gégout, J. C. (2020). Windstorm-induced canopy openings accelerate temperate forest adaptation to global warming. Glob. Ecol. Biogeogr. 29, 2067–2077. doi: 10.1111/geb.13177
Dong, L., Zhang, H., Wang, L., Yu, D., Yang, F., Shi, X., et al. (2018). Irrigation with sediment-laden river water affects the soil texture and composition of organic matter fractions in arid and semi-arid areas of Northwest China. Geoderma 328, 10–19. doi: 10.1016/j.geoderma.2018.05.002
Feng, C., Ma, Y., Jin, X., Wang, Z., Ma, Y., and Chen, H. Y. (2019). Soil enzyme activities increase following restoration of degraded subtropical forests. Geoderma 351, 180–187. doi: 10.1016/j.geoderma.2019.05.006
Freschet, G. T., Cornwell, W. K., Wardle, D. A., Elumeeva, T. G., Liu, W., Jackson, B. G., et al. (2013). Linking litter decomposition of above- and below-ground organs to plant-soil feedbacks worldwide. J. Ecol. 101, 943–952. doi: 10.1111/1365-2745.12092
Gairola, S., Sharma, C. M., Ghildiyal, S. K., and Suyal, S. (2012). Chemical properties of soils in relation to forest composition in moist temperate valley slopes of Garhwal Himalaya, India. Environmentalist 32, 512–523. doi: 10.1007/s10669-012-9420-7
Grzywna, A., and Ciosmak, M. (2021). The Assesment of physical variables of the soil quality index in the coal mine spoil. J. Ecol. Eng. 22, 143–150. doi: 10.12911/22998993/132431
Guo, Y., Abdalla, M., Espenberg, M., Hastings, A., Hallett, P., and Smith, P. (2021). A systematic analysis and review of the impacts of afforestation on soil quality indicators as modified by climate zone, forest type and age. Sci. Total Environ. 757:143824. doi: 10.1016/j.scitotenv.2020.143824
Guo, S., Han, X., Li, H., Wang, T., Tong, X., Ren, G., et al. (2018). Evaluation of soil quality along two revegetation chronosequences on the loess hilly region of China. Sci. Total Environ. 633, 808–815. doi: 10.1016/j.scitotenv.2018.03.210
Güsewell, S., and Gessner, M. O. (2009). N:P ratios influence litter decomposition and colonization by fungi and bacteria in microcosms. Funct. Ecol. 23, 211–219. doi: 10.1111/j.1365-2435.2008.01478.x
Han, C., Liu, Y., Zhang, C., Li, Y., Zhou, T., Khan, S., et al. (2021). Effects of three coniferous plantation species on plant-soil feedbacks and soil physical and chemical properties in semi-arid mountain ecosystems. For. Ecosyst. 8, 1–13. doi: 10.1186/s40663-021-00281-4
Hawkes, C. V., Waring, B. G., Rocca, J. D., and Kivlin, S. N. (2017). Historical climate controls soil respiration responses to current soil moisture. Proc. Natl. Acad. Sci. 114, 6322–6327. doi: 10.1073/pnas.1620811114
He, Y., Han, X., Wang, X., Wang, L., and Liang, T. (2021). Long-term ecological effects of two artificial forests on soil properties and quality in the eastern Qinghai-Tibet Plateau. Sci. Total Environ. 796:148986. doi: 10.1016/j.scitotenv.2021.148986
Hu, X., Li, Z., Chen, J., Nie, X., Liu, J., Wang, L., et al. (2021). Carbon sequestration benefits of the grain for Green Program in the hilly red soil region of southern China. Int. Soil Water Conserv. Res. 9, 271–278. doi: 10.1016/j.iswcr.2020.11.005
Hu, N., Li, H., Tang, Z., Li, Z., Li, G., Jiang, Y., et al. (2016). Community size, activity and C:N stoichiometry of soil microorganisms following reforestation in a Karst region. Eur. J. Soil Biol. 73, 77–83. doi: 10.1016/j.ejsobi.2016.01.007
Huang, F., Zhang, W., Gan, X., Huang, Y., Guo, Y., and Wen, X. (2018). Changes in vegetation and soil properties during recovery of a subtropical forest in South China. J. Mt. Sci. 15, 46–58. doi: 10.1007/s11629-017-4541-6
Illeris, L., Michelsen, A., and Jonasson, S. (2003). Soil plus root respiration and microbial biomass following water, nitrogen, and phosphorus application at a high arctic semi desert. Biogeochemistry 65, 15–29. doi: 10.1023/A:1026034523499
Jiang, F., Wu, X., Xiang, W., Fang, X., Zeng, Y., Ouyang, S., et al. (2017). Spatial variations in soil organic carbon, nitrogen and phosphorus concentrations related to stand characteristics in subtropical areas. Plant Soil 413, 289–301. doi: 10.1007/s11104-016-3101-0
Jim, C. Y., and Chen, W. Y. (2009). Ecosystem services and valuation of urban forests in China. Cities 26, 187–194. doi: 10.1016/j.cities.2009.03.003
Kaneda, S., and Kaneko, N. (2011). Influence of Collembola on nitrogen mineralization varies with soil moisture content. Soil Sci. Plant Nutr. 57, 40–49. doi: 10.1080/00380768.2010.551107
Kuehler, E., Hathaway, J., and Tirpak, A. (2017). Quantifying the benefits of urban forest systems as a component of the green infrastructure stormwater treatment network. Ecohydrology 10:e1813. doi: 10.1002/eco.1813
Laclau, J., Da Silva, E. A., Rodrigues Lambais, G., Bernoux, M., Le Maire, G., Stape, J. L., et al. (2013). Dynamics of soil exploration by fine roots down to a depth of 10 m throughout the entire rotation in Eucalyptus grandis plantations. Front. Plant Sci. 4:243. doi: 10.3389/fpls.2013.00243
Lange, M., Eisenhauer, N., Sierra, C. A., Bessler, H., Engels, C., Griffiths, R. I., et al. (2015). Plant diversity increases soil microbial activity and soil carbon storage. Nat. Commun. 6, 6707–6708. doi: 10.1038/ncomms7707
Li, Y., Li, B., Zhang, X., Chen, J., Zhan, F., Guo, X., et al. (2015). Differential water and soil conservation capacity and associated processes in four forest ecosystems in Dianchi Watershed, Yunnan Province, China. J. Soil Water Conserv. 70, 198–206. doi: 10.2489/jswc.70.3.198
Li, Y., Niu, S., and Yu, G. (2016). Aggravated phosphorus limitation on biomass production under increasing nitrogen loading: a meta-analysis. Glob. Change Biol. 22, 934–943. doi: 10.1111/gcb.13125
Li, P., Zhang, T., Wang, X., and Yu, D. (2013). Development of biological soil quality indicator system for subtropical China. Soil Tillage Res. 126, 112–118. doi: 10.1016/j.still.2012.07.011
Liu, X., Duan, L., Mo, J., Du, E., Shen, J., Lu, X., et al. (2011). Nitrogen deposition and its ecological impact in China: an overview. Environ. Pollut. 159, 2251–2264. doi: 10.1016/j.envpol.2010.08.002
Liu, X., Meng, W., Liang, G., Li, K., Xu, W., Huang, L., et al. (2014). Available phosphorus in forest soil increases with soil nitrogen but not total phosphorus: evidence from subtropical forests and a pot experiment. PLoS One 9:e88070. doi: 10.1371/journal.pone.0088070
Liu, Y., Miao, H., Chang, X., and Wu, G. (2019). Higher species diversity improves soil water infiltration capacity by increasing soil organic matter content in semiarid grasslands. Land Degrad. Dev. 30, 1599–1606. doi: 10.1002/ldr.3349
Liu, X., Tan, N., Zhou, G., Zhang, D., Zhang, Q., Liu, S., et al. (2021). Plant diversity and species turnover co-regulate soil nitrogen and phosphorus availability in Dinghushan forests, southern China. Plant Soil 464, 257–272. doi: 10.1007/s11104-021-04940-x
Liu, J., Wang, Z., Hu, F., Xu, C., Ma, R., and Zhao, S. (2020). Soil organic matter and silt contents determine soil particle surface electrochemical properties across a long-term natural restoration grassland. Catena 190:104526. doi: 10.1016/j.catena.2020.104526
Marinari, S., Masciandaro, G., Ceccanti, B., and Grego, S. (2000). Influence of organic and mineral fertilisers on soil biological and physical properties. Bioresour. Technol. 72, 9–17. doi: 10.1016/S0960-8524(99)00094-2
Marschner, P., Crowley, D., and Rengel, Z. (2011). Rhizosphere interactions between microorganisms and plants govern iron and phosphorus acquisition along the root axis – model and research methods. Soil Biol. Biochem. 43, 883–894. doi: 10.1016/j.soilbio.2011.01.005
Mo, J., Zhang, W., Zhu, W., Gundersen, P., Fang, Y., Li, D., et al. (2008). Nitrogen addition reduces soil respiration in a mature tropical forest in southern China. Glob. Change Biol. 14, 403–412. doi: 10.1111/j.1365-2486.2007.01503.x
Moreno-Mateos, D., Barbier, E. B., Jones, P. C., Jones, H. P., Aronson, J., López-López, J. A., et al. (2017). Anthropogenic ecosystem disturbance and the recovery debt. Nat. Commun. 8:14163. doi: 10.1038/ncomms14163
Muñoz-Rojas, M., Erickson, T. E., Dixon, K. W., and Merritt, D. J. (2016). Soil quality indicators to assess functionality of restored soils in degraded semiarid ecosystems. Restor. Ecol. 24, S43–S52. doi: 10.1111/rec.12368
Onoda, Y., and Bando, R. (2021). Wider crown shyness between broad-leaved tree species than between coniferous tree species in a mixed forest of Castanopsis cuspidata and Chamaecyparis obtusa. Ecol. Res. 36, 733–743. doi: 10.1111/1440-1703.12233
Pang, D., Cao, J., Dan, X., Guan, Y., Peng, X., Cui, M., et al. (2018). Recovery approach affects soil quality in fragile karst ecosystems of Southwest China: implications for vegetation restoration. Ecol. Eng. 123, 151–160. doi: 10.1016/j.ecoleng.2018.09.001
Pastore, G., Kaiser, K., Kernchen, S., and Spohn, M. (2020). Microbial release of apatite- and goethite-bound phosphate in acidic forest soils. Geoderma 370:114360. doi: 10.1016/j.geoderma.2020.114360
Pastore, G., Weig, A. R., Vazquez, E., and Spohn, M. (2022). Weathering of calcareous bedrocks is strongly affected by the activity of soil microorganisms. Geoderma 405:115408. doi: 10.1016/j.geoderma.2021.115408
Piaszczyk, W., Lasota, J., and Błońska, E. (2020). Effect of organic matter released from deadwood at different decomposition stages on physical properties of forest soil. Forests 11:24. doi: 10.3390/f11010024
Porter, C. H., Jones, J. W., Adiku, S., Gijsman, A. J., Gargiulo, O., and Naab, J. B. (2010). Modeling organic carbon and carbon-mediated soil processes in DSSAT v4.5. Oper. Res. 10, 247–278. doi: 10.1007/s12351-009-0059-1
Prestes, N. C. C. D., Massi, K. G., Silva, E. A., Nogueira, D. S., de Oliveira, E. A., Freitag, R., et al. (2020). Fire effects on understory forest regeneration in southern Amazonia. Front. For. Glob. Change 3:10. doi: 10.3389/ffgc.2020.00010
Rahmanipour, F., Marzaioli, R., Bahrami, H. A., Fereidouni, Z., and Bandarabadi, S. R. (2014). Assessment of soil quality indices in agricultural lands of Qazvin Province. Iran. Ecol. Indic. 40, 19–26. doi: 10.1016/j.ecolind.2013.12.003
Sardans, J., and Peñuelas, J. (2015). Trees increase their P:N ratio with size. Glob. Ecol. Biogeogr. 24, 147–156. doi: 10.1111/geb.12231
Schreeg, L. A., Mack, M. C., and Turner, B. L. (2013). Leaf litter inputs decrease phosphate sorption in a strongly weathered tropical soil over two time scales. Biogeochemistry 113, 507–524. doi: 10.1007/s10533-012-9781-5
Shah, A. N., Tanveer, M., Shahzad, B., Yang, G., Fahad, S., Ali, S., et al. (2017). Soil compaction effects on soil health and cropproductivity: an overview. Environ. Sci. Pollut. Res. 24, 10056–10067. doi: 10.1007/s11356-017-8421-y
Shao, G., Ai, J., Sun, Q., Hou, L., and Dong, Y. (2020). Soil quality assessment under different forest types in the Mount Tai, central eastern China. Ecol. Indic. 115:106439. doi: 10.1016/j.ecolind.2020.106439
Smith, C. K., de Assis Oliveira, F., Gholz, H. L., and Baima, A. (2002). Soil carbon stocks after forest conversion to tree plantations in lowland Amazonia. Brazil. For. Ecol. Manage. 164, 257–263. doi: 10.1016/S0378-1127(01)00599-0
Song, C., Lee, W. K., Choi, H. A., Kim, J., Jeon, S. W., and Kim, J. S. (2016). Spatial assessment of ecosystem functions and services for air purification of forests in South Korea. Environ. Sci. Pol. 63, 27–34. doi: 10.1016/j.envsci.2016.05.005
Srivastava, P., Singh, R., Bhadouria, R., Tripathi, S., and Raghubanshi, A. S. (2020). Temporal change in soil physicochemical, microbial, aggregate and available C characteristic in dry tropical ecosystem. Catena 190:104553. doi: 10.1016/j.catena.2020.104553
Tie, L., Zhang, S., Peñuelas, J., Sardans, J., Zhou, S., Hu, J., et al. (2020). Responses of soil C, N, and P stoichiometric ratios to N and S additions in a subtropical evergreen broad-leaved forest. Geoderma 379:114633. doi: 10.1016/j.geoderma.2020.114633
van der Putten, W. H., Bardgett, R. D., Bever, J. D., Bezemer, T. M., Casper, B. B., Fukami, T., et al. (2013). Plant-soil feedbacks: the past, the present and future challenges. J. Ecol. 101, 265–276. doi: 10.1111/1365-2745.12054
Vivanco, L., and Austin, A. T. (2008). Tree species identity alters forest litter decomposition through long-term plant and soil interactions in Patagonia, Argentina. J. Ecol. 96, 727–736. doi: 10.1111/j.1365-2745.2008.01393.x
Wang, H., Wu, J., Li, G., and Yan, L. (2020). Changes in soil carbon fractions and enzyme activities under different vegetation types of the northern Loess Plateau. Ecol. Evol. 10, 12211–12223. doi: 10.1002/ece3.6852
Wu, C., Liu, G., Huang, C., and Liu, Q. (2019). Soil quality assessment in Yellow River Delta: establishing a minimum data set and fuzzy logic model. Geoderma 334, 82–89. doi: 10.1016/j.geoderma.2018.07.045
Yang, Q., Zheng, F., Jia, X., Liu, P., Dong, S., Zhang, J., et al. (2020). The combined application of organic and inorganic fertilizers increases soil organic matter and improves soil microenvironment in wheat-maize field. J. Soils Sediments 20, 2395–2404. doi: 10.1007/s11368-020-02606-2
Yang, K., Zhu, J., Zhang, M., Yan, Q., and Sun, O. J. (2010). Soil microbial biomass carbon and nitrogen in forest ecosystems of Northeast China: a comparison between natural secondary forest and larch plantation. J. Plant Ecol. 3, 175–182. doi: 10.1093/jpe/rtq022
Yu, Y., and Jia, Z. Q. (2014). Changes in soil organic carbon and nitrogen capacities of Salix cheilophila Schneid along a revegetation chronosequence in semi-arid degraded sandy land of the Gonghe Basin, Tibet Plateau. Solid Earth 5, 1045–1054. doi: 10.5194/se-5-1045-2014
Yu, Z., Wang, M., Huang, Z., Lin, T. C., Vadeboncoeur, M. A., Searle, E. B., et al. (2017). Temporal changes in soil C-N-P stoichiometry over the past 60 years across subtropical China. Glob. Change Biol. 24, 1308–1320. doi: 10.1111/gcb.13939
Zhang, J., Guo, J., Chen, G., and Qian, W. (2005). Soil microbial biomass and its controls. J. For. Res. 16, 327–330. doi: 10.1007/BF02858201
Zhang, W., Qiao, W., Gao, D., Dai, Y., Deng, J., Yang, J., et al. (2018). Relationship between soil nutrient properties and biological activities along a restoration chronosequence of Pinus tabulaeformis plantation forests in the Ziwuling Mountains, China. Catena 161, 85–95. doi: 10.1016/j.catena.2017.10.021
Zhang, Y., Xu, X., Li, Z., Liu, M., Xu, C., Zhang, R., et al. (2019a). Effects of vegetation restoration on soil quality in degraded karst landscapes of southwest China. Sci. Total Environ. 650, 2657–2665. doi: 10.1016/j.scitotenv.2018.09.372
Zhang, H., Yang, Q., Zhou, D., Xu, W., Gao, J., and Wang, Z. (2021). How evergreen and deciduous trees coexist during secondary forest succession: insights into forest restoration mechanisms in Chinese subtropical forest. Glob. Ecol. Conserv. 25:e01418. doi: 10.1016/j.gecco.2020.e01418
Zhang, B., Zhang, G., Yang, H., and Zhu, P. (2019b). Temporal variation in soil erosion resistance of steep slopes restored with different vegetation communities on the Chinese Loess Plateau. Catena 182:104170. doi: 10.1016/j.catena.2019.104170
Zhao, X., Wang, Q., and Kakubari, Y. (2009). Stand-scale spatial patterns of soil microbial biomass in natural cold-temperate beech forests along an elevation gradient. Soil Biol. Biochem. 41, 1466–1474. doi: 10.1016/j.soilbio.2009.03.028
Zheng, X., Yuan, J., Zhang, T., Hao, F., Jose, S., and Zhang, S. (2017). Soil degradation and the decline of available nitrogen and phosphorus in soils of the main forest types in the Qinling Mountains of China. Forests 8:460. doi: 10.3390/f8110460
Keywords: forest restoration, physico-chemical properties, microbes, subtropical forests, soil quality index, soil stoichiometric ratio
Citation: Zhao X, Liu P, Feng Y, Zhang W, Njoroge B, Long F, Zhou Q, Qu C, Gan X and Liu X (2022) Changes in Soil Physico-Chemical and Microbiological Properties During Natural Succession: A Case Study in Lower Subtropical China. Front. Plant Sci. 13:878908. doi: 10.3389/fpls.2022.878908
Edited by:
Wenyan Han, Tea Research Institute (CAAS), ChinaCopyright © 2022 Zhao, Liu, Feng, Zhang, Njoroge, Long, Zhou, Qu, Gan and Liu. This is an open-access article distributed under the terms of the Creative Commons Attribution License (CC BY). The use, distribution or reproduction in other forums is permitted, provided the original author(s) and the copyright owner(s) are credited and that the original publication in this journal is cited, in accordance with accepted academic practice. No use, distribution or reproduction is permitted which does not comply with these terms.
*Correspondence: Weiqiang Zhang, happyzwq@sina.com; Xiaodong Liu, liuxd@scau.edu.cn
†These authors have contributed equally to this work and share first authorship