- 1The New Zealand Institute for Plant and Food Research Limited, Food Industry Science Centre, Palmerston North, New Zealand
- 2Plant Health and Environment Laboratory, Ministry for Primary Industries, Auckland, New Zealand
Certain viruses dramatically affect yield and quality of potatoes and have proved difficult to eradicate with current approaches. Here, we describe a reliable and efficient virus eradication method that is high throughput and more efficacious at producing virus-free potato plants than current reported methods. Thermotherapy, chemotherapy, and cryotherapy treatments were tested alone and in combination for ability to eradicate single and mixed Potato virus S (PVS), Potato virus A (PVA), and Potato virus M (PVM) infections from three potato cultivars. Chemotherapy treatments were undertaken on in vitro shoot segments for four weeks in culture medium supplemented with 100 mg L−1 ribavirin. Thermotherapy on in vitro shoot segments was applied for two weeks at 40°C (day) and 28°C (night) with a 16 h photoperiod. Plant vitrification solution 2 (PVS2) and cryotherapy treatments included a shoot tip preculture followed by exposure to PVS2 either without or with liquid nitrogen (LN, cryotherapy) treatment. The virus status of control and recovered plants following therapies was assessed in post-regeneration culture after 3 months and then retested in plants after they had been growing in a greenhouse for a further 3 months. Microtuber production was investigated using in vitro virus-free and virus-infected segments. We found that thermotherapy and cryotherapy (60 min PVS2 + LN) used alone were not effective in virus eradication, while chemotherapy was better but with variable efficacy (20–100%). The most effective result (70–100% virus eradication) was obtained by combining chemotherapy with cryotherapy, or by consecutive chemotherapy, combined chemotherapy and thermotherapy, then cryotherapy treatments irrespective of cultivar. Regrowth following the two best virus eradication treatments was similar ranging from 8.6 to 29% across the three cultivars. The importance of virus removal on yield was reflected in “Dunluce” free of PVS having higher numbers of microtubers and in “V500’ free of PVS and PVA having a greater proportion of microtubers > 5 mm. Our improved procedure has potential for producing virus-free planting material for the potato industry. It could also underpin the global exchange of virus-free germplasm for conservation and breeding programs.
Introduction
Potato (Solanum tuberosum) is the world’s third most important food crop after rice and wheat, and is the staple food of 1.3 billion people (Devaux et al., 2021). In 2019, the planted area of potatoes, globally, reached 17.3 million hectares, producing of over 370 million tons of which 45% was produced in China (25%), India (14%), and Russia (6%; FAOSTAT-Food and Agriculture Organization of the United Nations, 2021). In New Zealand, potato is a valuable commodity crop with 497,634 MT produced from 9,775 ha: in 2020, this production was valued at NZ$ 1.1 billion, including a significant export value close to NZ$ 106 million (Potatoes NZ, 2021). At the New Zealand Institute for Plant and Food Research Ltd. (PFR), we maintain field collections covering both old and new cultivars that have been used in breeding programs. A subset of these are held in tissue culture and a cryopreservation program to secure the collection is underway (Pathirana et al., 2019), with over 300 lines already secured.
High-quality seed potato is crucial for successful potato cultivation (Onofre et al., 2021). Viral diseases are one of the primary reason for degeneration of seed potatoes and thus constitute a major constraint for sustainable potato production (Awasthi and Verma, 2017; Priegnitz et al., 2019; Wasilewska-Nascimento et al., 2020). Vegetative propagation of potato results in virus transmission from generation to generation, with virus titers accumulating as a result of repeated propagation and infection events (Thomas-Sharma et al., 2016; Priegnitz et al., 2020). As well as inducing increased susceptibility to other pathogens, viral diseases cause economic losses due to their negative impacts on yield and tuber quality (Lin et al., 2014; Adolf et al., 2020).
Although around 57 viruses have been identified as infecting cultivated potatoes, only a few are reported to have a major economic impact. Potato virus M (PVM; Carlavirus), Potato virus A (PVA; Potyvirus), and Potato virus S (PVS; Carlavirus) are typically the most significant viral pathogens (either as single or as mixed infections) associated with substantial production losses (Wang et al., 2011; Fletcher, 2012; Kreuze et al., 2020). These virus species are disseminated through tubers and by aphids in a non-persistent manner. PVS and PVA are also transmitted by contact (Valkonen, 2007; Fuentes et al., 2021). Plants infected by PVM and PVS may not always show symptoms depending on the cultivar and virus isolates (Kreuze et al., 2020; Bradshaw, 2021). Furthermore, mixed infection can increase symptom severity and virus accumulation (Nyalugwe et al., 2012; Moreno and López-Moya, 2020).
Production of virus-free plants is necessary for successful management of viral diseases and for sustainable breeding activities including preservation of potato germplasm and global exchange of genetic resources (Naik and Khurana, 2003; Volmer et al., 2017; Ellis et al., 2020). Besides complete eradication, there are no effective measures for controlling viruses once the plants are infected (Nazarov et al., 2020; Rubio et al., 2020). Therefore, there is a need to develop efficient methods to eradicate virus species to ensure production and supply of high-healthy planting material for the potato industry. Various in vitro-based techniques have been used to eradicate viruses in potato plants. For example, meristem culture used either alone (Quazi and Martin, 1978; Wang et al., 2006; Zhang et al., 2019) or in combination with thermotherapy (Wang et al., 2006; AlMaarri et al., 2012; Waswa et al., 2017), chemotherapy (AlMaarri et al., 2012), and electrotherapy (AlMaarri et al., 2012). In meristem culture-based methods, the size of the explant affects the efficacy of virus eradication; it is usually necessary to excise shoot tips of 0.2 mm containing an apical dome with one or two leaf primordia (Wang et al., 2006; Zhang et al., 2019). Excision of such small shoot tips is laborious, time-consuming, and a highly skilled task: results can also be variable in terms of shoot regrowth and the frequency of virus eradication (Bettoni et al., 2016; Magyar-Tábori et al., 2021). The inability to guarantee complete removal of viral particles, especially in mixed infections, remains a limitation for these meristem culture-based methods (Faccioli and Marani, 1998; Zhang et al., 2019). Application of shoot tip cryotherapy as a novel method for plant virus eradication has also been tested in potato with mixed results (Wang et al., 2006; Jianming et al., 2012; Yi et al., 2014; Li et al., 2016, 2018a; Zhang et al., 2019). For example, Potato leafroll virus (PLRV) and Potato virus Y (PVY) have been successfully eliminated with high efficacy using cryogenic treatments (Wang et al., 2006; Yi et al., 2014; Zhang et al., 2019); however, this method completely failed in producing PVM- and PVS-free potato plants when they presented as a mixed infection (Li et al., 2018a; Zhang et al., 2019).
Improved potato virus eradication has been achieved by combining two or more in vitro-based techniques. Combining chemotherapy with thermotherapy (Fletcher et al., 1988; Fang et al., 2005; Dhital et al., 2007; Nasir et al., 2010; Antonova et al., 2017) and meristem culture (Zhang et al., 2019) or chemotherapy with cryotherapy (Kushnarenko et al., 2017) were shown to provide more effective virus eradication than just a single technique. However, the efficacy of these methods varied according to the virus species, their infection level (single or mixed, virus titer) and cultivars used, as well as the virus-host combination (Antonova et al., 2017; Waswa et al., 2017; Gong et al., 2019). Furthermore, duration and temperature of the thermotherapy treatment, types, and concentrations of antiviral agents used in chemotherapy, as well as the size of the excised shoot tip can affect the success of virus eradication (AlMaarri et al., 2012; Kushnarenko et al., 2017; Waswa et al., 2017; Magyar-Tábori et al., 2021). Therefore, standardization of the virus eradication methodology is important, especially when the plants have mixed infections.
Herein, we investigated the effect of chemotherapy, thermotherapy, and cryotherapy on eradication of PVM, PVA, and PVS from in vitro cultured shoots of three potato cultivars. More specifically, we combined several of these methods to develop a novel technology that is high throughput, easier, and more effective in eradicating virus. We believe that this technology can be easily adopted and transferred between laboratories, facilitating delivery of healthy propagating material to growers for commercial production and for germplasm conservation and plant exchange. Data demonstrating the positive effect that removal of these viruses has on microtuber yield are also presented.
Materials and Methods
Plant Material and Explant Preparation for in vitro Therapies
Tissue-cultured plants of three potato (Solanum tuberosum L.) cultivars “Dunluce,” “Tahi,” and “V500” were obtained from the Lincoln site of PFR and used in this study. Infected in vitro stock plants with PVM, PVA, and PVS were previously identified and confirmed using the reverse-transcription polymerase chain reaction (RT-qPCR) method (see below) prior to the experiments outlined here. Virus-infected cultures (“Dunluce” with PVS, “Tahi” with PVA and PVS, and “V500” with PVM and PVS) were multiplied and maintained in an actively growing state on basal medium (BM) composed of half-strength Murashige and Skoog (1962; MS) macronutrients, full-strength MS micronutrients, and Linsmaier and Skoog (1965; LS) vitamins supplemented with 30 g L−1 sucrose and 7.5 g L−1 agar. For all experiments, the pH of culture media was adjusted to 6 prior to autoclaving at 121°C for 20 min. Cultures were incubated in a growth room maintained at 24°C with a 16 h photoperiod at a photosynthetic flux density of 40 μmol s−1 m−2 provided by cool-white fluorescent tubes (hereafter called standard conditions). Subculture into fresh BM was performed every 4 weeks throughout the experiment.
The explants used for in vitro therapies were nodal sections (1 cm in length) obtained from 3-week-old in vitro stock plants and placed on 90 × 15 mm disposable polystyrene Petri plates containing 25 ml of BM at a density of 30 to 40 nodal sections per plate. The nodal sections were cultured under standard conditions to provide uniform explant material for the therapies. The apical shoot tips (1 mm) for cryotherapy were excised from the nodal sections after 1 week, and the shoots (1.5 cm) for chemotherapy/thermotherapy experiments were excised from the nodal sections after 2 weeks. Figure 1 shows the workflow for this study.
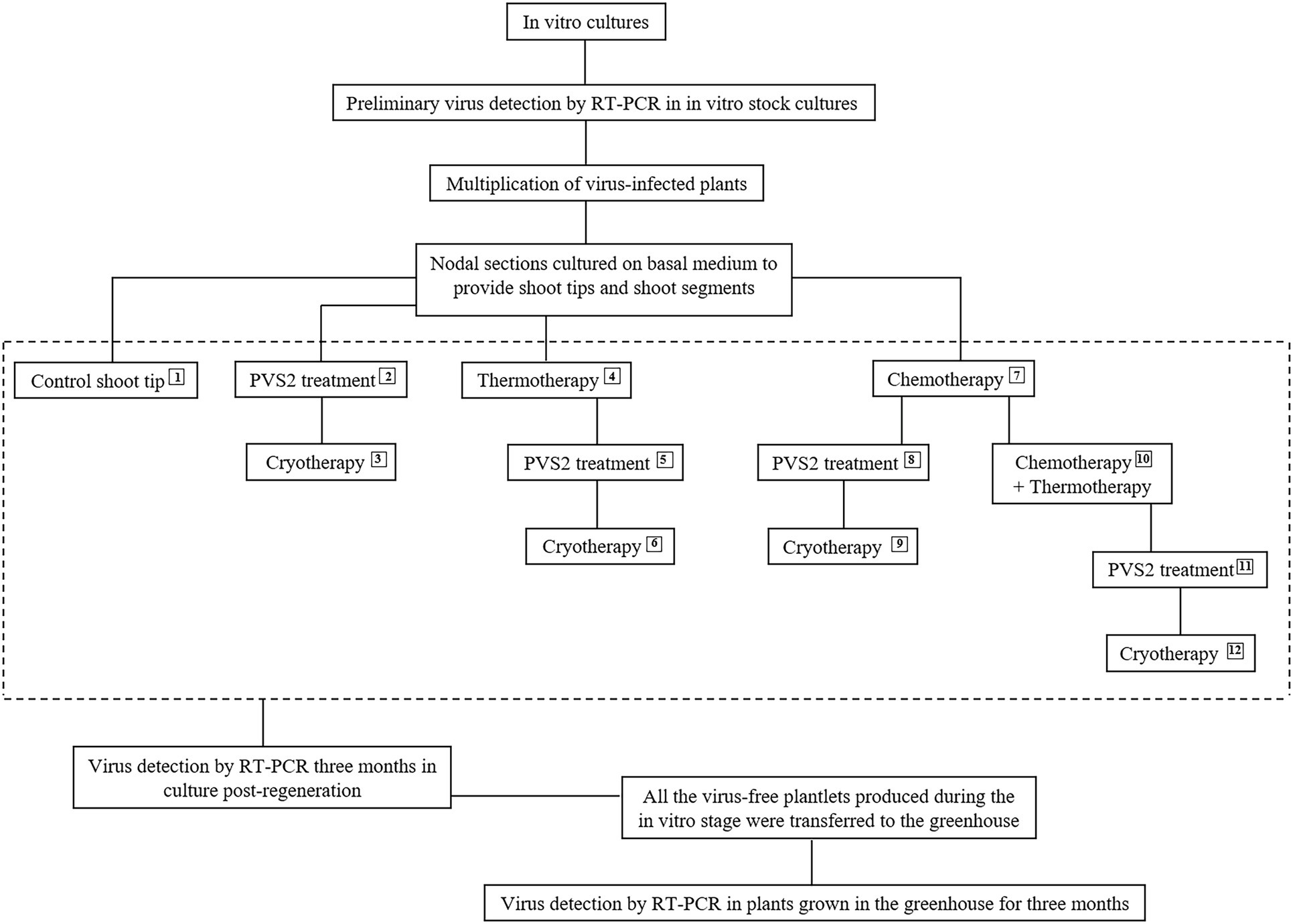
Figure 1. Flowchart depicting the 12 in vitro therapies used for eradicating Potato virus S, Potato virus A, and Potato virus M from infected in vitro-grown potato shoots. Plant vitrification solution 2 (PVS2) and cryotherapy treatments were performed on 1 mm apical shoot tips and thermotherapy and chemotherapy on 1–1.5 cm apical shoot segments.
In vitro Therapies for Virus Eradication
Control Shoot Tips (SP)
Uniform apical shoot tips (1 mm length) were excised from 1-week-old shoots and cultured on recovery medium (RM) consisting of MS macro and micro elements, LS vitamins, 25 g L−1 sucrose, 0.05 mg L−1 indole-3-acetic acid, 0.05 mg L−1 gibberellic acid, 0.3 mg L−1 zeatin, and 6 g L−1 agar at pH 5.7; (Kim et al., 2006 with modifications) and incubated in the dark at 24°C for 1 week before transfer to standard conditions.
Plant Vitrification Solution 2 Treatment (PVS2)
Uniform apical shoot tips (1 mm length) were excised from 1-week-old shoots and transferred to liquid preculture medium 1 (half-strength MS macronutrients, MS micronutrients, and LS vitamins containing 0.3 M sucrose) and maintained for 24 h on a shaker (50 rpm) in darkness at 24°C. They were then transferred to liquid preculture medium 2 (same salts as preculture medium 1 but with 0.7 M sucrose) and maintained under the same conditions for another 16 h. Precultured shoot tips were treated with plant vitrification solution 2 (PVS2) [filter-sterilized MS, 0.4 M sucrose, 30% (w/v) glycerol, 15% (w/v) ethylene glycol (EG), and 15% (w/v) dimethyl sulfoxide (DMSO), pH 5.8] (Sakai et al., 1990) at 22°C for 60 min (PVS2 treatment).
Following PVS2 treatment, the shoot tips were washed with unloading solution (filter-sterilized half-strength MS macronutrients, MS micronutrients + 1.2 M sucrose at pH 5.8) pre-warmed to 40°C and maintained in the solution for 20 min at room temperature (22°C). Thereafter, for recovery, shoot tips were transferred to an intermediate recovery medium (half-strength MS macronutrients, MS micronutrients supplemented with 0.6 M sucrose, and 7.5 g L−1 agar) overnight in the dark and then transferred to RM. Cultures were maintained 1 week in the dark at 24°C, before being transferred to standard conditions.
Additionally, the survival, shoot regrowth, and virus eradication efficiency from shoot tips after treatment with one of seven PVS2 exposure periods between 5 and 135 min in “Dunluce” and after short, medium, and long PVS2 exposure periods (5, 60, and 135 min, respectively) in “Tahi” and “V500” were assessed.
Cryotherapy
For cryotherapy (Cryo), similar steps to those described in “PVS2” were followed, and 2 minutes before the end of PVS2 incubations, PVS2-treated shoot tips were placed into a thin layer of PVS2 on sterile aluminum foil strips (~ 6 × 25 mm) and then plunged into LN. After 1 hour of LN exposure, the aluminum foil strips with shoot tips were thawed quickly by inverting the strips into unloading solution and recovered as described in “PVS2” above.
Thermotherapy (T)
Apical shoot segments (1–1.5 cm in length) were excised from 2-week-old shoots and placed in 98 × 60 mm disposable polystyrene clear tissue culture vessels with 50 ml BM at a density of 15 nodal sections per culture vessel. After 2 days of culture under standard conditions, the culture vessels containing shoot segments were moved into a growth chamber with 40% relative humidity at a photosynthetic photon flux density of 70 μmol s−1 m−2 provided by cool-white fluorescent tubes. The shoots were grown in an alternating temperature regime of 28°C for 8 h in darkness and 40°C for 16 h with light for 2 weeks. Apical shoot tips (1 mm length) were excised from heat-treated shoots and cultured on RM in the dark at 24°C for 1 week before transfer to standard conditions.
Thermotherapy + PVS2 (T + PVS2)
Apical shoot segments (1–1.5 cm in length) were subjected to thermotherapy, as described in “Thermotherapy”, after which apical shoot tips (1 mm length) were excised and subjected to PVS2 treatment (PVS2) as described in “PVS2”.
Thermotherapy + Cryotherapy (T + Cryo)
Apical shoot segments (1–1.5 cm in length) were subjected to thermotherapy, as described in “Thermotherapy”, after which apical shoot tips (1 mm length) were excised and subjected to cryotherapy (Cryo) as described in “Cryotherapy”.
Chemotherapy (C)
Apical shoot segments (1–1.5 cm in length) were excised from 2-week-old shoots and placed in 98 × 60 mm disposable polystyrene clear tissue culture vessels containing 50 ml BM supplemented with 100 mg L−1 ribavirin (Duchefa®, Haarlen, Netherlands) at a density of 15 nodal sections per culture vessel. Ribavirin was filter-sterilized and added to the medium after autoclaving. The cultures were maintained under standard conditions. After 4 weeks of ribavirin treatment, apical shoot tips (1 mm length) were excised and placed on RM and incubated in the dark at 24°C for 1 week before transfer to standard conditions.
Chemotherapy + PVS2 (C + PVS2)
Apical shoot segments (1–1.5 cm in length) were cultured on BM supplemented with 100 mg L−1 ribavirin for 4 weeks under standard conditions, as described in “Chemotherapy”, after which apical shoot tips (1 mm length) were excised and subjected to PVS2 treatment (PVS2) as described in “PVS2”.
Chemotherapy + Cryotherapy (C + Cryo)
Apical shoot segments (1–1.5 cm in length) were cultured on BM supplemented with 100 mg L−1 ribavirin for 4 weeks under standard conditions, as described in “Chemotherapy”, after which apical shoot tips (1 mm length) were excised and subjected to cryotherapy (Cryo) as described in “Cryotherapy”.
Chemotherapy Followed by Combined Chemotherapy and Thermotherapy [C + (C + T)]
Apical shoot segments (1–1.5 cm in length) were cultured on BM supplemented with 100 mg L−1 ribavirin for 2 weeks under standard conditions, as described in “Chemotherapy”, after which the tissue culture vessel containing the shoots in ribavirin medium was transferred to thermotherapy conditions as described in “Thermotherapy” for additional 2 weeks, followed by apical shoot tip isolation (1 mm length) and recovery process as described in “Thermotherapy”.
Chemotherapy Followed by Combined Chemotherapy and Thermotherapy, Then PVS2 [C + (C + T) + PVS2]
Apical shoot segments (1–1.5 cm in length) were treated as described in “Chemotherapy Followed by Combined Chemotherapy and Thermotherapy [C + (C + T)]” above, after which apical shoot tips (1 mm length) were excised and subjected to PVS2 treatment (PVS2), as described in “PVS2”.
Chemotherapy Followed by Combined Chemotherapy and Thermotherapy, Then Cryotherapy [C + (C + T) + Cryo]
Apical shoot segments (1–1.5 cm in length) were treated as described in “Chemotherapy Followed by Combined Chemotherapy and Thermotherapy [C + (C + T)]”, after which apical shoot tips (1 mm length) were excised and subjected to cryotherapy (Cryo), as described in “Cryotherapy”.
Shoot Tip Recovery
Shoot tip survival (shoot tips that exhibited growth of a green cell mass or leaf tissue; Figure 2A) and regrowth (shoot tips exhibiting organized shoots with a new leaf emerging; Figure 2B) were recorded 3–6 weeks post-culture on RM. Regenerated shoots with approx. 1 cm of growth were transferred to individual vials (30 ml capacity) with 10 ml of BM and grown under standard conditions (Figures 2B,C).
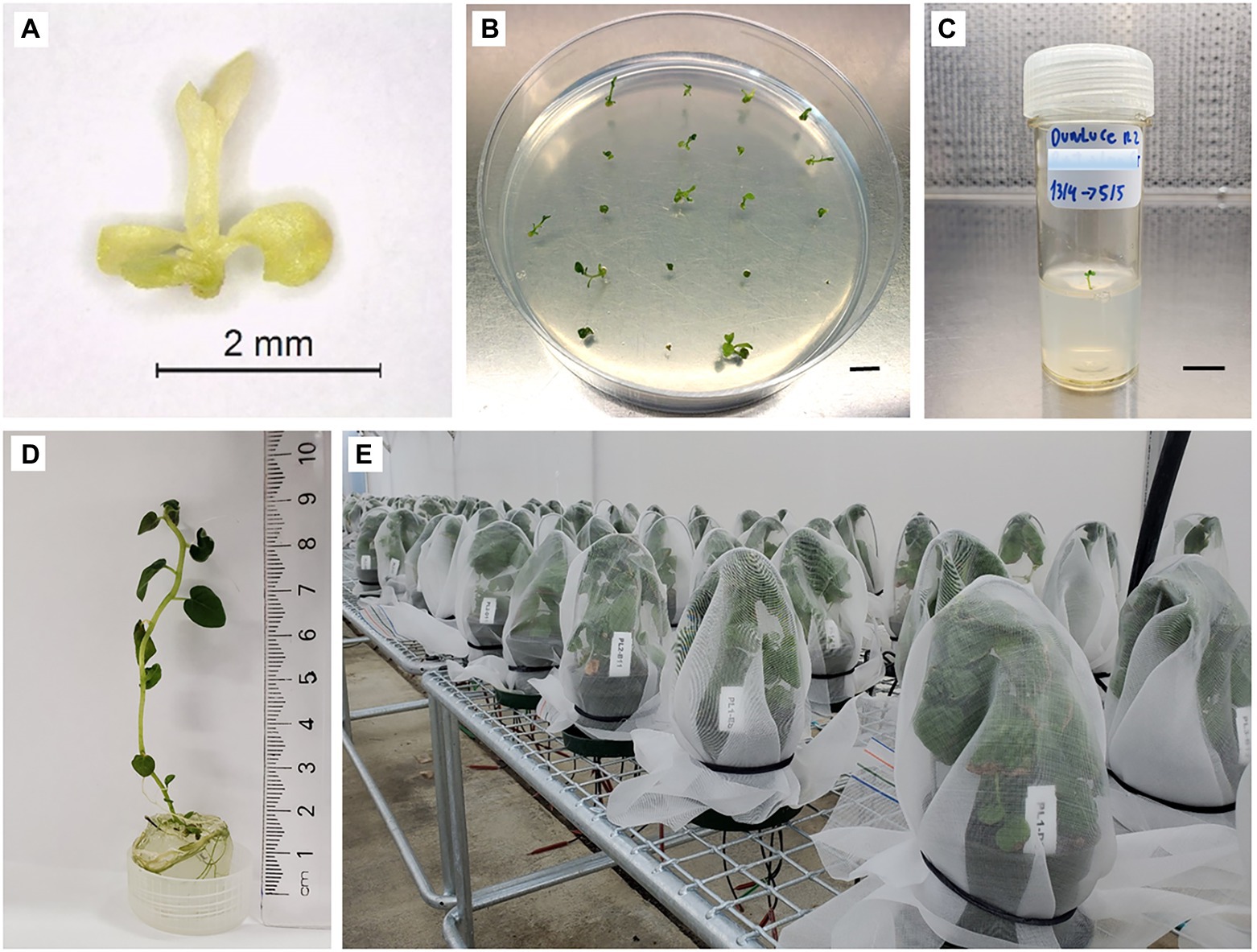
Figure 2. Shoot tip recovery process in potato “Dunluce” following a combined chemotherapy + cryotherapy treatment. (A) Shoot tip 1 week after combined chemotherapy + cryotherapy and (B) 3 weeks recovery from cryoexposure. (C) Shoot transferred to vial and (D) grown for 3 months. (E) Plants after 3 months of growth in the greenhouse. Bars = B 0.6 cm, C 0.7 cm.
Virus Detection
The virus status of plant materials was tested using RT-qPCR. Primers and probes (Table 1) were purchased from Integrated DNA Technologie.1 The potato mitochondrial NADH dehydrogenase subunit 5 (nad5) gene transcript (Khan et al., 2015) and the plant nuclear gene transcript exocyst complex component (sec3) were used as the plant positive controls for cDNA synthesis. RT-qPCR was performed on nucleic acids isolated from young leaves of tissue-cultured plantlets and greenhouse-grown plants by the commercial company SlipStream Automation2 using their standard in-house CTAB- and plate-based extraction and cDNA synthesis methodology. cDNA generated using SuperScriptIV3 from the RNA was diluted 5-fold with TE buffer (pH 8.0) for use in PCR. PCRs for PVS, PVA, PVM, and nad5 were performed in 5.5 μl volumes containing 0.9 x Roche Lightcycler 480 Master Mix,4 680 nM primers, 227 nM probe, and 2 μl diluted cDNA. Prior to amplification, cDNA was denatured at 95°C for 4 min and then cycled 40 times with 95°C/15 s and 60°C/45 s. Samples were scored with the LightCycler 480 software release 1.5.1.62 SP2 using the “Abs Quant/Fit Points” analysis with the Noise Band fixed at a fluorescence value of 3, 1.5, and 3 for PVA, PVM, and PVS, respectively. Samples with viral Ct values greater than 35 were interpreted as absent for virus. PCRs for sec3 were performed in 7 μl reactions using 1 x Roche Lightcycler 480 Master Mix, 3.12 mM Mg2+, 400 nM primers, and 2 μl cDNA. Prior to amplification, the cDNA was denatured at 95°C for 10 min and then cycled 45 times with 95°C/15 s, 60°C/15 s, and 72°C/30s. Following PCR, melt curve analysis was performed at 95°C/60s and 40°C/60s, followed by heating to 65°C and then to 95°C with continuous data acquisition at 25 acquisitions per degree Celsius. Results were scored with LightCycler 480 software release 1.5.1.62 SP2 using the “Abs Quant/Fit Points” analysis with the Noise Band fixed at a fluorescence value of 5.
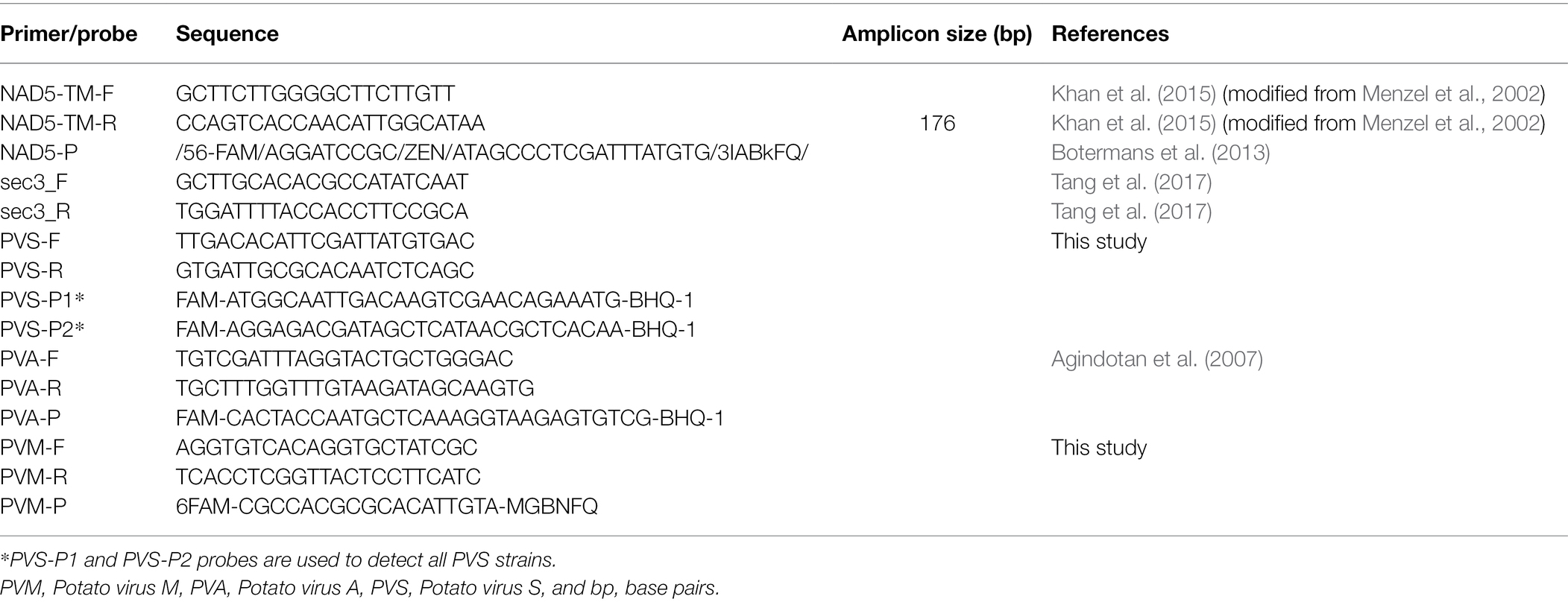
Table 1. Primers and probes used for detection of PVS, PVA, PVM, plant mitochondrial gene transcript NADH dehydrogenase subunit 5 (nad5), and plant nuclear gene transcript exocyst complex component (sec3).
Plantlet material was confirmed to have single or mixed virus infections prior to therapies by RT-qPCR. RT-qPCR-based diagnostic of stock plants revealed the presence of single virus infection of PVS for the cultivar “Dunluce,” PVS and PVA for “Tahi,” and PVS and PVM for “V500.” The presence of viruses in potato cultivars that underwent the in vitro therapies and controls were assessed at two different stages: firstly of cultures post-regeneration, 3 months after therapy treatments (Figure 2D), and secondly on all samples with initial negative test results after growing plants on the greenhouse for a further 3 months (Figure 2E).
In vitro Rooting, Acclimatization, and Plant Maintenance in the Greenhouse
One apical shoot segment (1.5 cm in length), containing two to three fully expanded in vitro leaves, from each virus-free plant was cultured in an individual vial (30 ml capacity) on BM under standard conditions for 2 weeks to initiate rooting. The rooted plantlets were then transferred to individual 12-cm diameter pots (800 ml capacity) containing a commercial substrate Daltons® potting mix (Daltons, Matamata, New Zealand) in an insect-proof greenhouse at 22 ± 2°C with a 12–14 h photoperiod under natural light. Plants were individually covered with insect-proof net to prevent possible virus-cross contamination (Figure 2E). After 3 months of growth (September to November), leaves were collected for further confirmation of the sanitary status by RT-qPCR.
Assessment of the Impact of Virus Infection on Microtuber Production
In vitro microtubers were induced using the method of Wakasa et al. (2020) with modifications as follows. Apical shoot segments (1 cm length) containing a single leaf and one to two axillary buds were obtained from 3-week-old in vitro virus-free and virus-infected stock plants. Five shoot segments were placed into 100 ml liquid BM in a 500-mL Erlenmeyer flask and were cultured on a shaker (75 rpm) under standard conditions for 2 weeks (Figures 3A–D). The BM was then discarded and replaced with liquid microtuber production medium (MPM; Li et al., 2013; Zhang et al., 2019) and grown in the dark at 24°C. The MPM is composed of BM supplemented with 60 g L−1 sucrose and 4 mg L−1 kinetin. Data on the number of microtubers produced per vessel and the size of microtubers (diameter) were recorded after 2 weeks of culture in liquid MPM (Figures 3E,F).
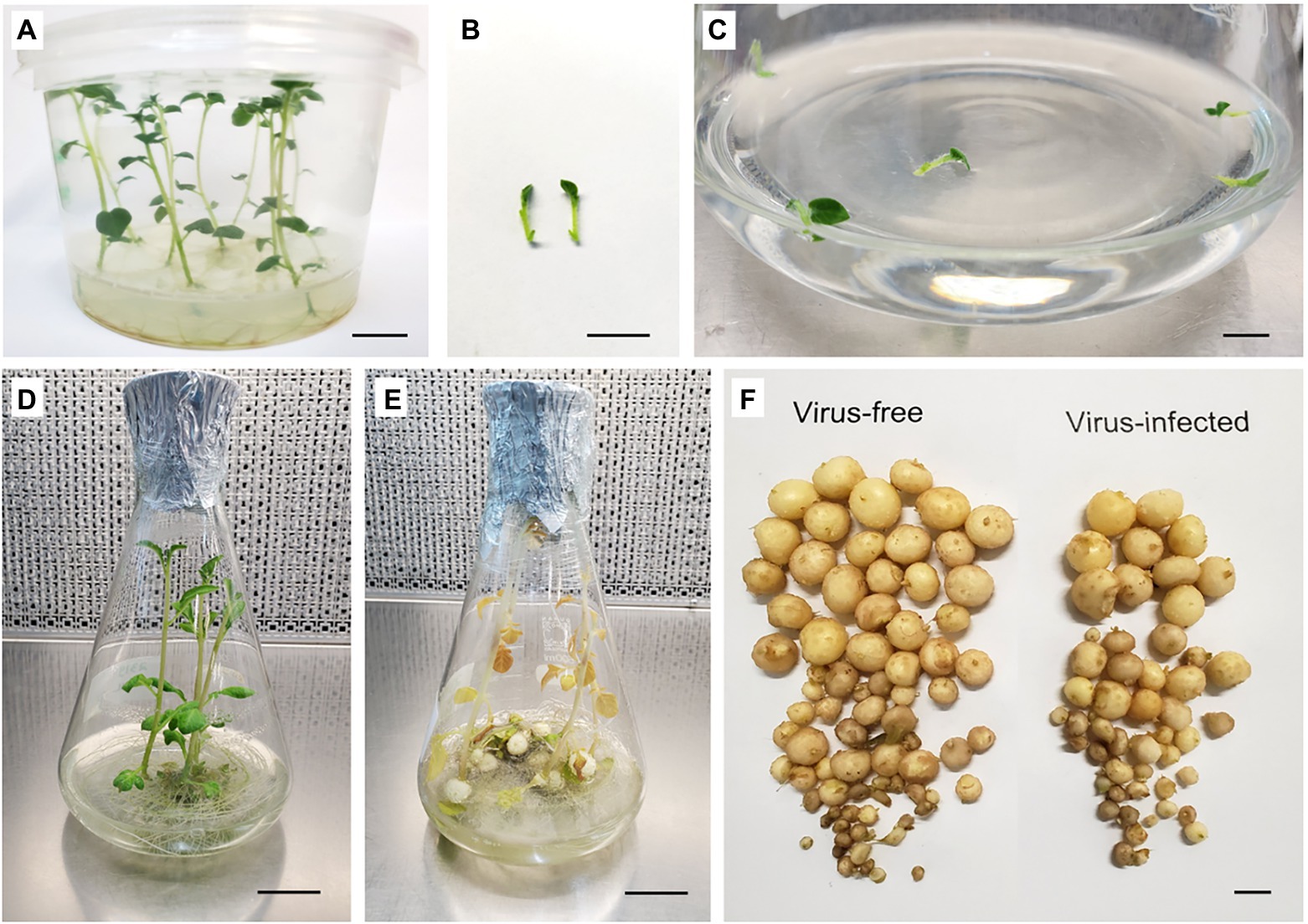
Figure 3. Microtuber production in in vitro cultures of potato cultivar “Dunluce.” (A) 3-week-old in vitro stock plants. (B) Shoot segments (1 cm) used as the explant source for microtuber production. (C) Shoot segments after initiation and (D) growth after 14 days of liquid culture at 24°C with a photoperiod of 16 h light day–1. (E) Cultures after 14 days in microtuber production medium at 24°C in the dark. (F) Microtubers produced on shoots derived from virus-free (left) and Potato Virus S-infected (right) in vitro plants of “Dunluce” potato. Bars = A, B, C, and F 1 cm; D and E 2.3 cm.
Data Analysis
A minimum of 20 shoot tips were used per replicate with three replicates per treatment in a randomized block design. Shoot tip survival and regrowth results were both analyzed using binomial generalized linear models (GLM) with logit link (logistic regression). For each treatment, the results were presented as the number of samples, the percentage success, and a 95% confidence interval for the percentage. Confidence limits for the predicted treatment means were calculated from the logistic regression results except for those means of 100% for which the confidence limits were replaced by limits calculated using the Wilson Score method (Agresti and Coull, 1998; Supplementary Tables 1–5). Comparisons between treatment means were made using pairwise contrasts.
The numbers of microtubers produced per vessel from virus-free and virus-infected sources were compared using one-way ANOVA separately for each cultivar., and the proportions of microtubers in each size class (<5 mm, 5–10 mm, >10 mm) were analyzed using a binomial GLM with a logit link separately for each cultivar and size class (Supplementary Tables 6, 7). All analyses were performed using Genstat 19th edition (VSN International, Hemel Hempstead, United Kingdom). Frequency of virus eradication was calculated as the percentage of virus-free plants over the total number of plants used for virus detection. Up to 10 plantlets recovered from in vitro therapies and controls were randomly selected from the population and virus tested using RT-qPCR. The total number of plantlets used for virus testing varied depending on the number of samples obtained in each treatment.
Results
Effects of the in vitro Therapies on Shoot Tip Viability and Virus Eradication
All the shoot tips in the controls survived and at least 96.8% regenerated shoots (Table 2). All the regenerated plants from the virus-infected positive controls remained virus-infected (Tables 3). After 2 weeks of thermotherapy alone or thermotherapy in combinations with chemotherapy, shoots developed a yellowish coloring at the tip. Despite this possible sign of stress, there was no significant impact on shoot tip regrowth of cultivar “Dunluce”; however, shoot tip regrowth of cultivars “Tahi” and ‘V500” was reduced compared to the control (Table 2). For “V500,” all the thermo- and chemo-treated shoot tips survived, but only a proportion produced viable plants (50.8 and 48.3%, respectively) with most shoot tips having yellowish growth that failed to develop (Table 2; Supplementary Tables 1, 2).
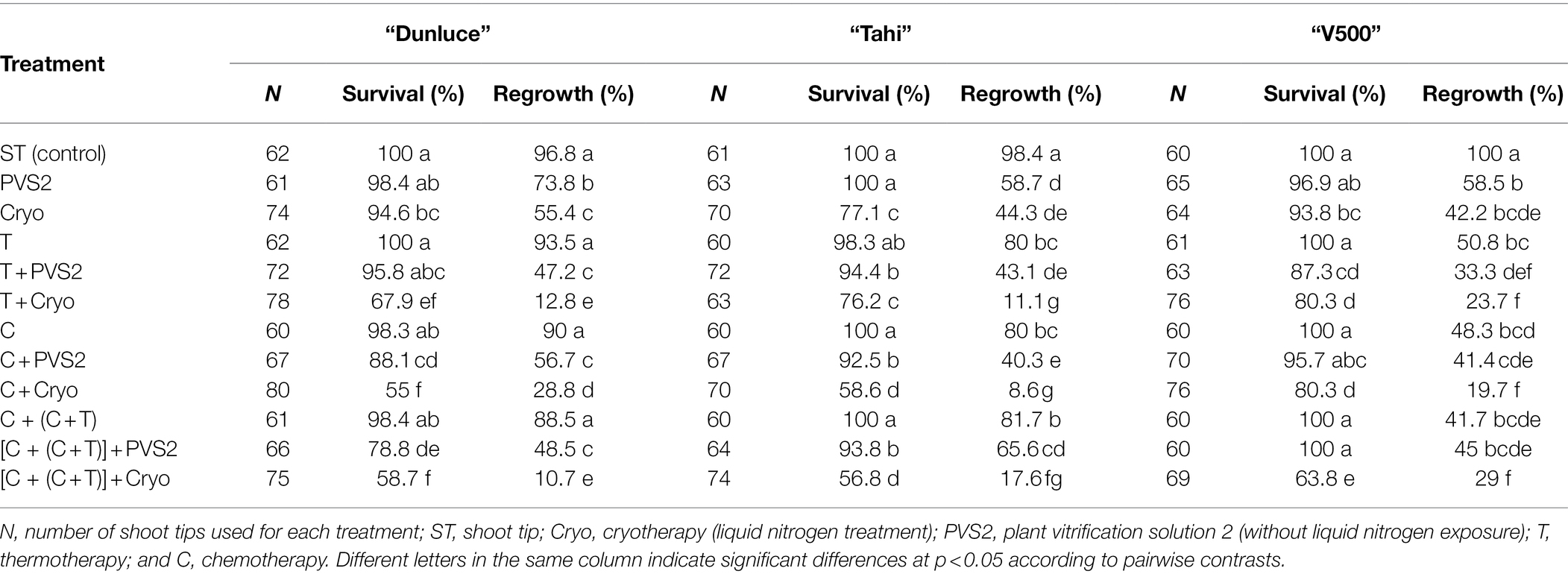
Table 2. Survival and regrowth levels (%) of shoot tips excised from in vitro-grown potato cultivars “Dunluce,” “Tahi,” and “V500” following each treatment of virus eradication.
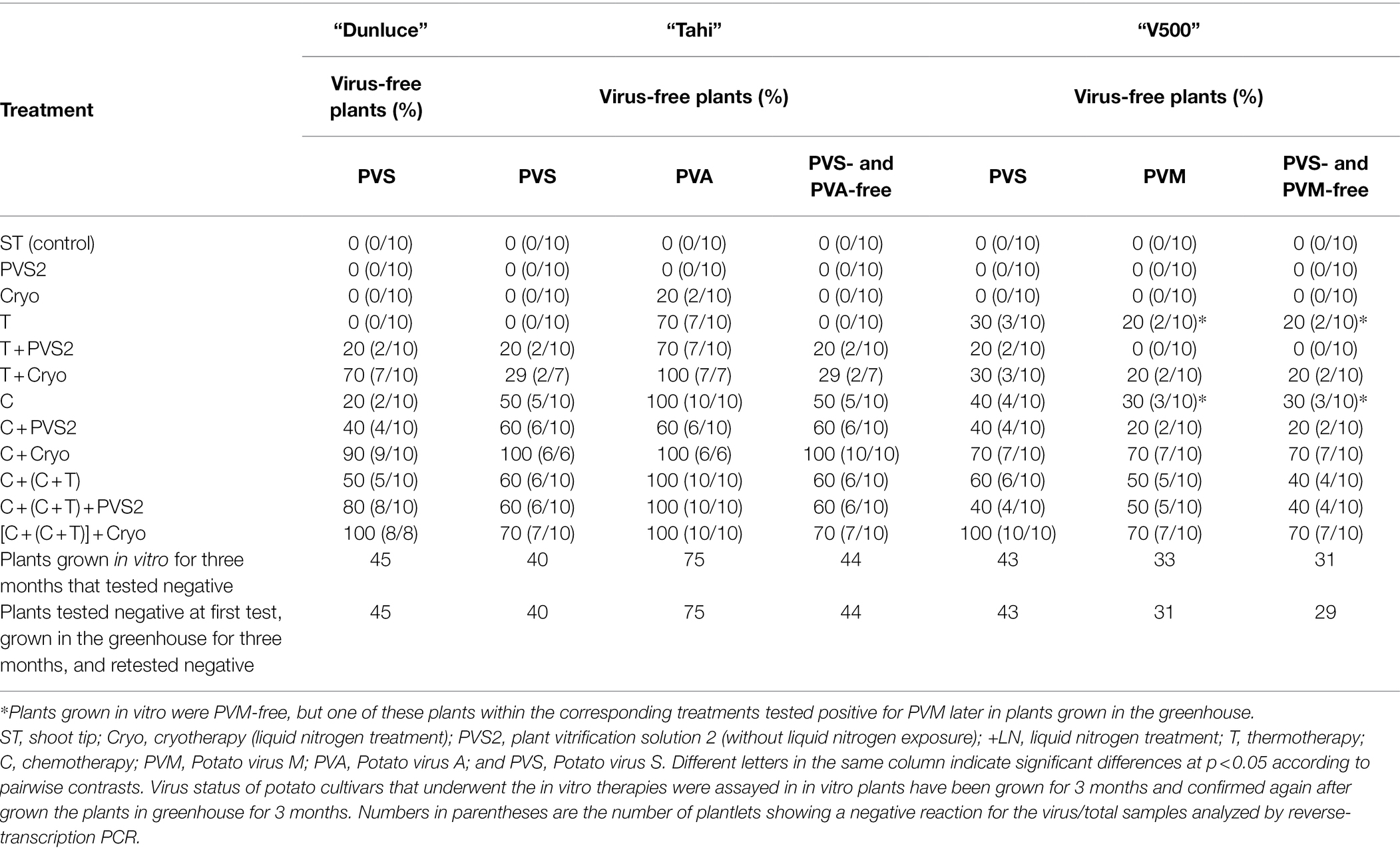
Table 3. Effect of virus eradication treatments on percentage of potato plants from cultivars “Dunluce,” “Tahi,” and “V500” that were virus-free in a single or mix-infection.
The effectiveness of virus eradication differed among the virus species: PVA was more readily eradicated than PVS and PVM, regardless of the method used (Table 3). Cryotherapy resulted in regrowth ranging from 42.2 to 55.4% across the three potato cultivars, however failed to eradicate PVS and PVM (Tables 2, 3). PVS2 treatment alone was not sufficient to eradicate any of the viruses. Shoot tips from “Tahi” recovered from cryotherapy were 20% PVA-free but still infected with PVS (Table 3).
Thermotherapy alone did not produce any virus-free plants in “Dunluce” and “Tahi”; meanwhile, in cultivar “V500,” one in 10 plants was free of PVS and PVM. Interestingly, two out of 10 thermotherapy-derived “V500” plants were free of PVM after 3 months in tissue culture but one subsequently tested positive for PVM after further plant growth in the greenhouse (Table 3).
Chemo-treated shoot tips produced similar rates of survival (about 100%) across the three potato cultivars with shoot regrowth varying from 48.3 to 90%, the lowest of which was for “V500” and the highest for “Dunluce” (Table 2). Chemotherapy alone was not always able to eradicate viruses; it was more efficient at eliminating PVA than PVS or PVM. After chemotherapy, “Tahi” initially infected with PVS and PVA had around 50% of plants free of virus. This compared to only 20% of plants free of virus for both “Dunluce” (which was only infected with PVS) and “V500” (which had a mixed infection of PVS and PVM). As noted for thermotherapy, three out of 10 “V500” plants submitted to chemotherapy were PVM-free after 3 months of in vitro culture post-regeneration. However, one of the progeny from this sample tested positive for PVM later in the greenhouse (Table 3).
Improvements on virus eradication were achieved through consecutive chemotherapy followed by combined chemotherapy and thermotherapy [C + (C + T)] treatments, with 50% of the evaluated “Dunluce” plants being free of PVS, 60% of the “Tahi” plants being free of PVS and PVA in mixed infection, and 40% of the “V500” plants being free of PVS and PVM in mixed infection (Table 3).
Thermotherapy or chemotherapy treatments followed by cryotherapy and the combination of all treatments affected shoot tip survival and regrowth in all three potato cultivars assessed (Table 2). When thermotherapy was combined with cryotherapy, the regrowth varied from 11.1% (“Tahi”) to 23.7% (“V500”). Chemotherapy in combination with cryotherapy resulted in a particularly low regrowth of 8.6% in “Tahi.” In general, shoot tips from treatments combined with either PVS2 (PVS2-treated shoot tips without LN exposure) or/and cryotherapy (PVS2 + LN exposure) treatments had the lowest regrowth (Table 2). Although regrowth was lower in shoot tips recovered after combining chemotherapy with cryotherapy, this method resulted in the highest frequencies of virus eradication in both single and mixed infections. All the “Tahi” plants recovered after chemotherapy combined with cryotherapy were free from both PVS and PVA, while nine out of 10 “Dunluce” plants evaluated were free from PVS and seven out of 10 “V500” plants were free from both PVS and PVM (Table 3).
The efficiency of virus eradication using thermotherapy followed by cryotherapy differed between virus species and cultivar. While 70% of the “Dunluce” plants evaluated were PVS-free, only 29% of the “Tahi” plants evaluated were free from both PVS and PVA, and 20% of the “V500” plants were free of PVS and PVM (Table 3).
Shoots exposed to chemotherapy followed by combined chemotherapy plus thermotherapy; then, cryotherapy {[C + (C + T)] + Cryo} had significantly decreased regrowth compared to the control or to shoots received individual treatments (Table 2). Although the shoot tip survival was relatively high (58.7–63.8%), most of the shoot tips produced just leaves with no evidence of a continued growth or turned into callus and failed to regenerate. The combination of all treatments resulted in shoot regrowth of 10.7–29% across the three potato cultivars with virus eradication rates of 70–100% (Table 3).
Virus-free plants, at low frequencies, could be found in shoots subjected to thermo- or/and chemo-therapy followed by PVS2 exposure for 60 min without freezing in LN (PVS2 treatment). However, the efficiency of virus eradication was much higher when shoot tips were thermo- or/and chemo-treated followed by LN exposure (Cryo; Table 3).
Effect of PVS2 Exposure Duration on Shoot Tip Viability and Virus Eradication
Survival and regrowth of shoot tips decreased as durations of PVS2 treatment increased, for all three cultivars. Extended PVS2 exposure duration of 135 min resulted in the least shoot tip survival and regrowth, even without LN exposure (Tables 4, 5; Supplementary Tables 3–5).
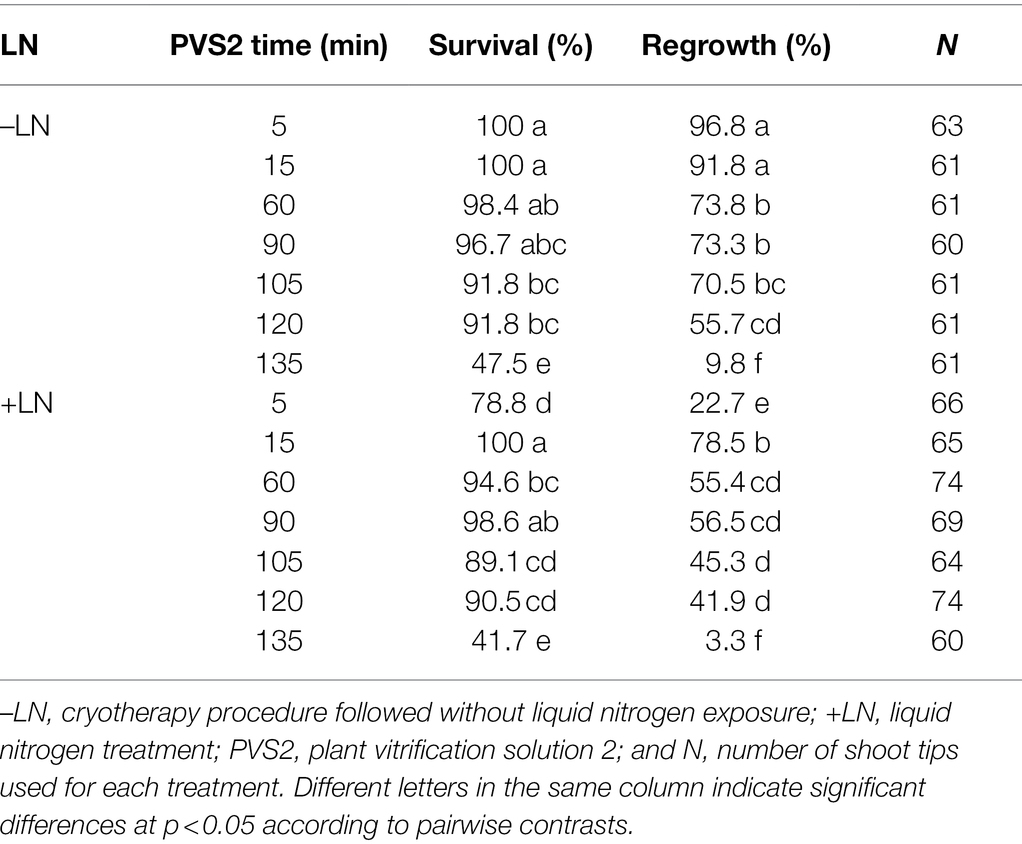
Table 4. Effect of seven plant vitrification solution 2 (PVS2) exposure durations without (–LN) and with freezing in liquid nitrogen (+LN) on the survival and regrowth of shoot tips excised from in vitro-grown potato “Dunluce” infected with Potato virus S.
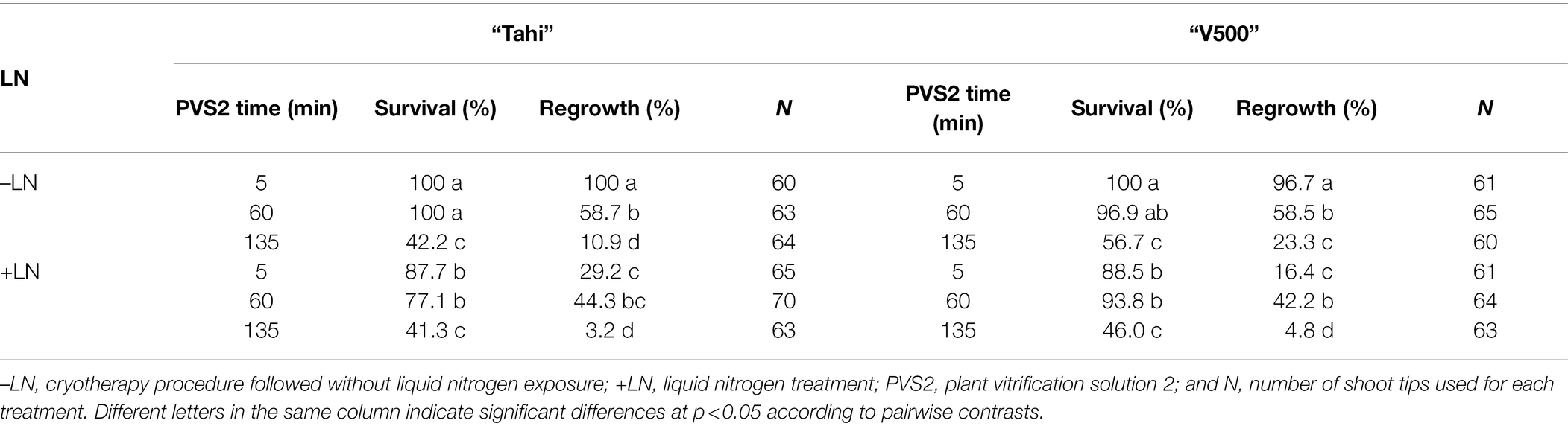
Table 5. Effect of three plant vitrification solution 2 (PVS2) exposure durations without (–LN) and with freezing in liquid nitrogen (+LN) on survival and regrowth of shoot tips excised from in vitro-grown potato cultivars “Tahi” (infected with Potato virus S and Potato virus A) and “V500” (infected with Potato virus S and Potato virus M).
Shoot tip survival of “Dunluce” was between 48 to 100% and 42 to 100% across the seven PVS2 exposure times for non-LN (without freezing in LN) treated and LN-treated (Cryo) explants, respectively (Table 4). Regrowth percentages in non-LN-treated shoot tips decreased from 97 to 3.3% as the PVS2 exposure time increased. In LN-treated shoot tips (Cryo), the maximum regrowth was 79% after 15 min of PVS2 exposure. Shoot tips exposed to PVS2 for a duration shorter or longer than 15 min showed reduced viability (Table 4). Although the regrowth of “Dunluce” shoot tips was high when incubated at the optimized PVS2 treatment duration (15 min) and exposed to LN, all recovered plants were infected with virus. Likewise, all shoot tips exposed to PVS2 without freezing in LN, were virus-infected regardless of the PVS2 exposure time. While the optimized vitrification solution exposure duration followed by LN treatment failed to eradicate PVS, one out of two plants tested free of this virus when a longer PVS2 exposure (135 min) was followed by LN treatment (Table 6). Longer PVS2 exposure followed by LN treatment (Cryo) resulted in regrowth of only two of out 60 treated shoot tips. The PVS was not able to be eradicated when shoot tips of cultivar “Dunluce” were exposed to PVS2 for a duration shorter than 135 min prior to LN treatment (Table 5).
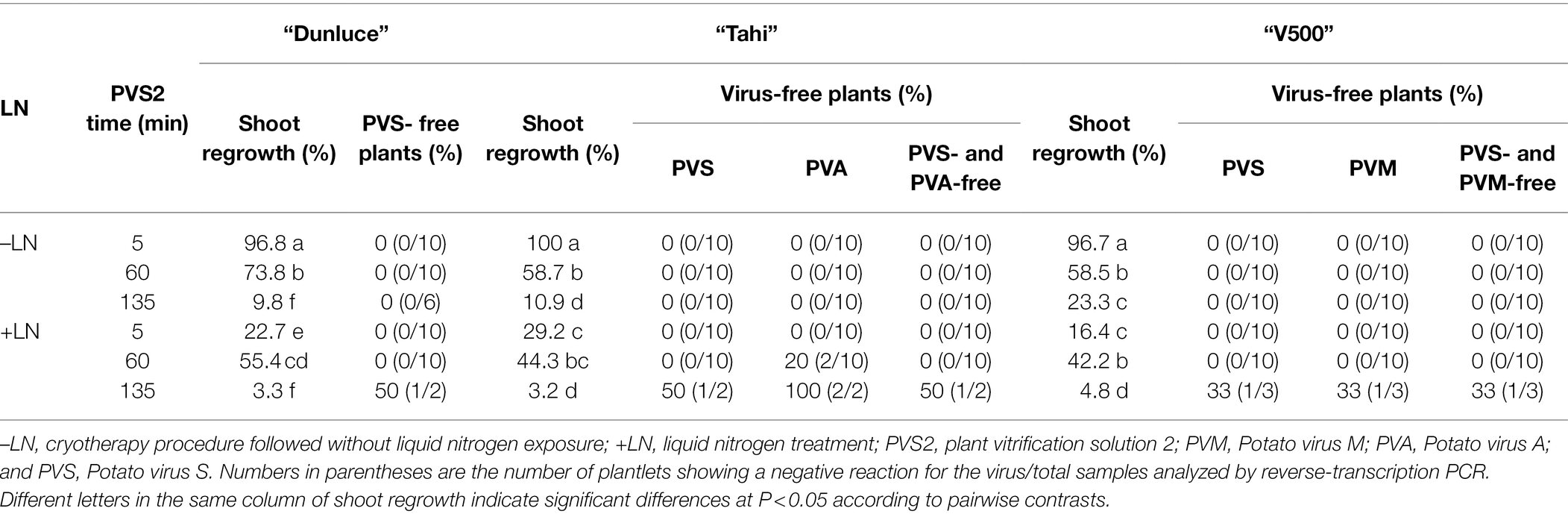
Table 6. Effect of plant vitrification solution 2 (PVS2) exposure duration of 5, 60, and 135 min without (–LN) and with freezing in liquid nitrogen (+LN) on shoot regrowth level and percentage of potato plants from cultivars “Dunluce,” “Tahi,” and “V500” that were virus-free in a single or mix-infection.
There were no significant differences in regrowth between shoot tips of “V500” and “Tahi” that were immersed in LN and those without LN immersion (PVS2 treatment) for the PVS2 exposure durations of 60 and 135 min (Table 5). Shoot regrowth was higher in explants that were exposed to PVS2 for 5 min without freezing in LN (97–100%) than those that were immersed in LN (16–29%; Supplementary Tables 4, 5). All the shoot tips that were exposed to PVS2 without LN treatment remained virus-infected. As observed in “Dunluce,” all the shoot tips of “Tahi” and “V500” that were exposed to PVS2 without LN treatment remained virus-infected. Only the shoot tips that were exposed to PVS2 for 135 min and immersed in LN produced virus-free plants (Table 6). Although one plant from each cultivar was free of mixed virus infection, only two and three out of 63 treated shoot tips of “Tahi” and “V500” were recovered after cryotherapy, respectively. Shoot tips exposed to PVS2 for a duration shorter than 135 min followed by LN treatment failed to eradicate mixed infections of PVS plus PVA and PVS plus PVM in “V500” and “Tahi” cultivars, respectively. For “Tahi,” although both plants recovered from shoot tips exposed to 135 min in PVS2 and immersed in LN were free of PVA, only one of those was also free of PVS (Table 6).
All regenerated potato plantlets were successfully rooted in vitro and survived the acclimatization process. No visible morphological difference was apparent in the plants following 3 months’ growth in the greenhouse. Except for two “V500” plants, all the plants that were virus-free at the in vitro stage were found to have a virus-free status after 3 months in the greenhouse. The two “V500” plants (from mixed-infected with PVS and PVM) tested positive for PVM; one was derived from thermotherapy alone and the other from chemotherapy alone (Table 3).
Comparative Assessment of Microtuber Production in the Virus-Free and Virus-Infected Potato Plants
Each of the three potato cultivars consistently produced 7–19 microtubers of varied size per vessel (Figure 4). Single infection of PVS in “Dunluce” had a negative impact on microtuber production in vitro: the number of microtubers per vessel was significantly lower in PVS-infected plantlets (12.6) than in PVS-free plantlets (19.4; Figures 3, 4 and Supplementary Table 6). Although there were no significant differences in the number of microtubers per vessel and proportions of microtubers in each size class between virus-free and virus-infected “V500” and “Tahi,” there was a tendency for higher proportions of large microtubers (>10 mm) to be produced in virus-free plantlets (Supplementary Table 7). A minimal increment of 5% in larger sized microtubers (>10 mm) was achieved when virus-free shoots were used as the source of material for microtuber production. Cultivar “V500” produced almost twice the percentage of small-sized microtubers from virus-infected (38.3%) material compared with virus-free (20.3%) material (Figure 4).
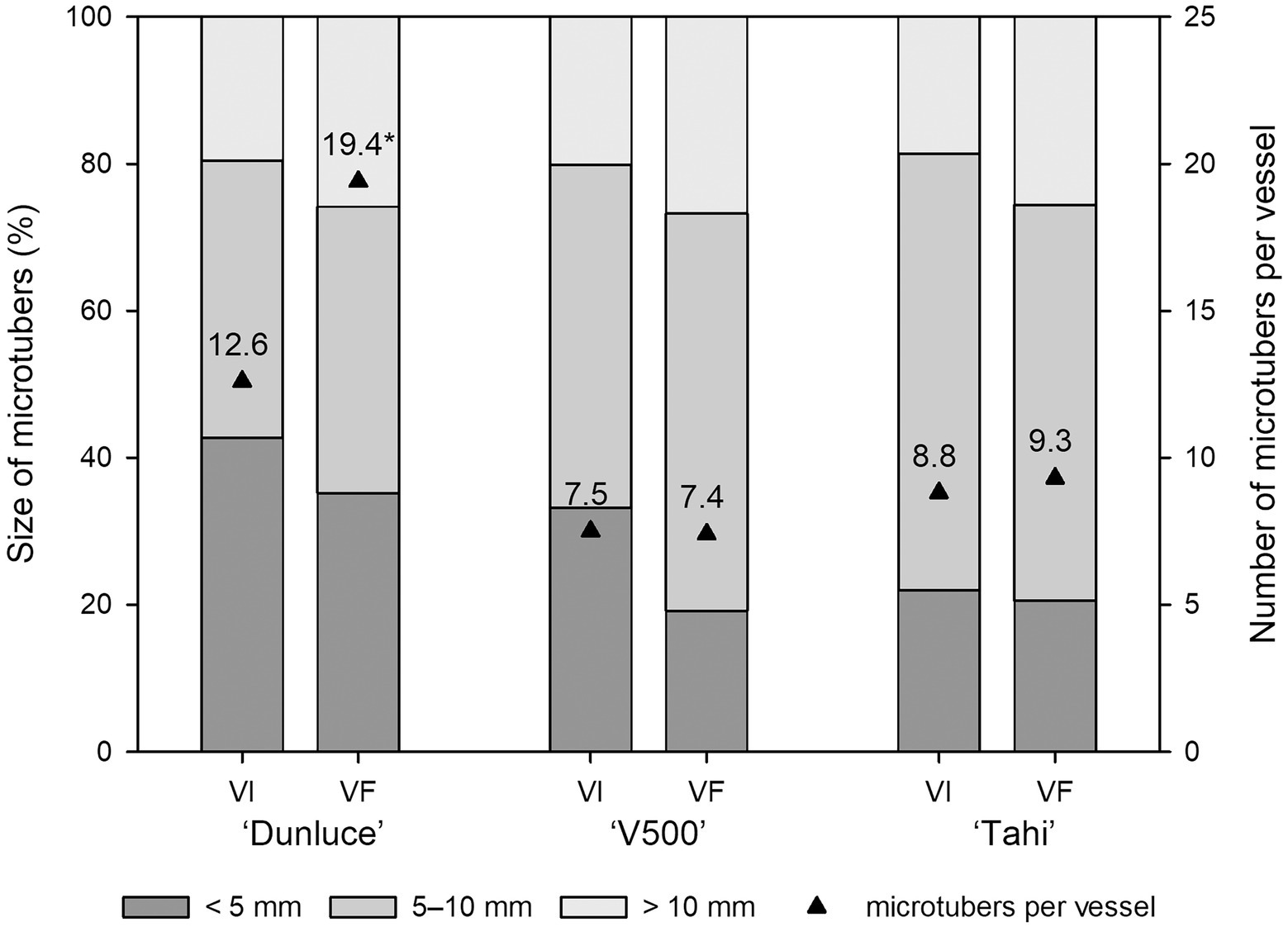
Figure 4. Effect of virus infection on proportion of microtuber size produced in potato cultivars “Dunluce” (infected with Potato virus S), “V500” (infected with Potato virus S and Potato virus M), and “Tahi” (infected with Potato virus S and Potato virus A) after 2 weeks in microtuber production media. VI virus-infected plants; VF virus-free plants. * indicates significant differences at p < 0.05 in microtuber numbers according to analysis of variance.
Discussion
Potato is highly susceptible to virus infections and viruses have long affected global potato production (Kreuze et al., 2020). Thus, considerable effort has been made to establish robust systems for obtaining and maintaining virus-free potato plants. The cultivation of virus-resistant plants might be a desirable and sustainable strategy to overcome this problem (Farnham and Baulcombe, 2006; Valkonen, 2008; Orbegozo et al., 2016). However, virus resistance is not easily achievable for most crops and viruses, and mutations in the viral genome or even mixed infection can overcome this resistance (García-Cano et al., 2006; Tian and Valkonen, 2013; Rubio et al., 2020). Therefore, the development of technologies to produce virus-free propagation material followed by the ongoing planting of high-healthy plants is still the most effective strategy for managing viruses in commercial production systems (Gong et al., 2019; Magyar-Tábori et al., 2021).
In this study, we combined several virus eradication methods (thermotherapy, chemotherapy, and cryotherapy) to efficiently eradicate single and mixed PVS, PVA, and PVM infection from virus-infected in vitro cultures of potato cultivars “Dunluce,” “Tahi,” and “V500”. We found that the combination of chemotherapy and cryotherapy (C + Cryo), or chemotherapy treatment prior to combining chemotherapy treatment with thermotherapy followed by cryotherapy {[C + (C + T)] + Cryo} resulted in high efficiency of PVS, PVA, and PVM eradication; 70–100% across the three potato cultivars tested. Though the regrowth percentage was low in shoots exposed to chemotherapy followed by cryotherapy (8.6–28.8%), high levels of virus-free plants were obtained. Indeed, plants of “Tahi” were 100% free from PVS and PVA, “Dunluce” were 90% PVS-free, and “V500” were 70% free from mixed infection of PVS and PVM. The combination of all treatments resulted in similar shoot regrowth (10.7–29%) across the three cultivars with virus eradication rates of between 70 and 100%: with rates for “Tahi” (mixed-infected with PVS and PVA) being lower than “V500” (mixed-infected with PVS and PVM) which was lower than “Dunluce” (PVS-infected). Although the efficacy of these two treatments in eradicating viruses was similar, incorporating thermotherapy was more time-consuming and required a temperature-controlled growth chamber; therefore, our study shows that the combination of chemotherapy and cryotherapy is a more appropriate strategy for eradication of PVS, PVM, and PVA from infected in vitro potato plants.
In this study, isolating and regrowing plants from 1 mm shoot tips (without any virus eradication treatment) completely failed to eradicate single infections of PVS, mixed infections of PVS and PVA, and PVS and PVM from infected in vitro potato shoots. In shoot tip culture, the size of excised shoot tip affects to the success of virus eradication (Bettoni et al., 2021a); our results confirm that the 1 mm shoot tips were not a suitable explant size for virus eradication. In addition, the virus location in the infected shoot tips may be a bottleneck to achieving efficient virus eradication by shoot tip culture (Wang et al., 2021). Previously, PLRV, PVY, PVS, and Potato virus X (PVX) have been eradicated by 0.2 mm shoot tip culture from in vitro diseased shoots (Wang et al., 2006; AlMaarri et al., 2012; Jianming et al., 2012; Zhang et al., 2019). Although 0.2 mm shoot tip culture could eradicate virus species, a proportion of recovered plants were still virus-infected, with eradication efficiency being related to the specific interaction between virus species and potato genotype. Improved virus eradication using shoot tip culture has been achieved when it is combined with thermotherapy (Waswa et al., 2017). High temperature treatments can prevent virus movement toward meristematic cells by inhibiting viral replication and degrading RNA and therefore, decreasing the viral particle load in infected shoot tips (Wang et al., 2008; Wang et al., 2018a). Wang et al. (2006) found that PLRV and PVY eradication frequencies increased from 56 to 90% and 62 to 93%, respectively, when 0.2 mm shoot tips were excised from shoots heat-treated at 36°C for 4 weeks, compared to shoot tips not subjected to heat treatment (thermotherapy). We found that thermotherapy involving alternating temperature of 28°C (8 h in dark) and 40°C (16 h in light) for 2 weeks followed by the excision of 1 mm shoot tips resulted in very low efficiency of virus eradication in “V500,” which was mixed-infected with PVS and PVM, and failed to eradicate either the single infection of PVS from “Dunluce” or the mixed infection of PVS and PVA from “Tahi.” Although 70% of “Tahi” shoots were free of PVA following this thermotherapy regime, none of the recovered plants were free of PVS. In general, prolonged heat treatment associated with high temperatures can increase the frequency of virus eradication, but at the same time reduces the viability of the treated explants, as host plants are often sensitive to these conditions (Zhao et al., 2018; Wang et al., 2018a; Farhadi-Tooli et al., 2022).
The combination of chemotherapy and cryotherapy reported here involved treatment of the shoots in medium containing 100 mg L−1 ribavirin for 4 weeks, followed by cryotherapy. Previously, Kushnarenko et al. (2017) have combined chemotherapy and cryotherapy procedures to eradicate virus species from in vitro shoots of potato. In their study, PVM and PVS were successfully eradicated from potato cultivars “Tamyr” and “Nartau” after three subcultures (45 days each; total of 135 days) on medium with 100 mg L−1 ribavirin followed by cryotherapy. In our study, in addition to PVM and PVS, we also successfully eradicated PVA by combining chemotherapy and cryotherapy. Since our procedure did not require such a long period of culture on ribavirin, it could provide a higher throughput and more cost effective technique, as well as reducing the potential for somaclonal variation through reducing the number of culture steps. In our study, 1-mm apical shoot tips were excised from plants treated with ribavirin for 4 weeks, then subjected to droplet-vitrification cryotherapy using 60 min PVS2 exposure at 22°C; in contrast, Kushnarenko et al. (2017) used slightly larger shoot tips (1.5–2.0 mm), a different cryotherapy technique (vitrification) and shorter PVS2 exposure (30 min). These differences might explain why a longer ribavirin treatment was necessary for the eradication of PVM and PVS in the study of Kushnarenko et al. (2017). We note that chemotherapy treatment alone could eliminate viruses from all three cultivars but with low efficacy; this could be significantly improved by combining chemotherapy with cryotherapy, specifically from 20 to 90% in “Dunluce,” 50 to 100% in “Tahi,” and 20 to 70% in “V500.”
In recent years, shoot tip cryotherapy has proven to be an effective method to eradicate viruses from in vitro infected plants in multiple species of economic importance (Cui et al., 2015; Pathirana et al., 2015; Bettoni et al., 2018, 2019a, 2021a; Bi et al., 2018; Souza et al., 2020; Farhadi-Tooli et al., 2022; Wang et al., 2022a). Cryotherapy makes use of LN exposure (−196°C) to selectively destroy vacuolated and differentiated cells that are known to harbor viruses within shoot tips (Wang et al., 2009; Wang and Valkonen, 2009). In this study, cryotherapy (60 min PVS2 exposure + LN) alone failed to eradicate virus in “Dunluce” singly infected with PVS or mixed infections of PVS and PVA in “Tahi,” and PVS and PVM in “V500.” Similarly, Kushnarenko et al. (2017) found that cryotherapy alone did not produce any potato plants free from mixed infection of PVS and PVM. Working on six PVS-infected potato cultivars, Zhang et al. (2019) found that successful PVS eradication using cryotherapy was cultivar dependent. About 17% of the “Iverpotet/Smaragd” cryo-recovered plants were PVS-free, while all of the other potato cultivars were still PVS infected after cryotherapy. Li et al. (2018a) also reported that cryotherapy alone completely failed to generate PVS and Potato spindle tuber viroid (PSTVd)-free plants from in vitro infected potato “Zihuabai” shoots. In our study, although cryotherapy resulted in low efficiency (20%) of PVA eradication in “Tahi,” the cryo-recovered plants were still PVS-infected. Recent research has shown that not all viruses can be eradicated using shoot tip cryotherapy, particularly in those that are able to infect regions within the meristem (Wang et al., 2008, 2018b, 2022a; Li et al., 2016, 2018a; Zhao et al., 2018; Mathew et al., 2021). The ongoing presence of PVS and PVM after cryotherapy might be because these viruses can be located very close to the meristem; hence, it is possible for a small portion of the infected cells to remain alive following cryo-treatment, meaning the regenerated plants will remain virus-infected.
Even though cryotherapy techniques are based on cryopreservation, unlike cryopreservation, cryotherapy protocols should seek to eliminate the maximum portion of differentiated and infected cells, particularly in plant species infected with viruses that are difficult to eradicate such as PVS and PVM. This is a balancing act: a strategy to improve virus eradication could be to use either shorter or longer PVS2 exposure durations than the optimal, but this may result in fewer cells from which to recover plants after LN exposure (Bi et al., 2018; Bettoni et al., 2019a, 2021b; Souza et al., 2020; Wang et al., 2022b). Working on Vitis vinifera Cabernet Sauvignon, Bi et al. (2018) found that Grapevine leafroll-associated virus-3 (GLRaV-3) was eradicated from diseased shoot tips independent of PVS2 exposure duration (50–100 min). Although, there was no influence of PVS2 exposure duration on GLRaV-3 eradication, it was evident that the long exposure to PVS2 (100 min) significantly reduced the percentage of cells surviving in the apical dome and leaf primordia. GLRaV-3 is a phloem-limited virus and, therefore, it might explain why Bi et al. (2018) did not observe any difference in virus eradication across the range of PVS2 times, even though longer PVS2 exposure duration of 100 min resulted in fewer cells that recover after LN exposure. In our study, the viability of shoot tips and the ability of cryotherapy to produce virus-free plants was directly associated with duration of exposure to PVS2. Extended PVS2 exposure durations of 135 min at room temperature followed by LN treatment resulted in very low shoot tip regrowth of 3.2–4.8% across the three potato cultivars; however, one out of 60 cryo-treated shoot tips from each cultivar did regenerate a virus-free plant irrespective of whether there was a single or mixed infection of PVS, PVM, and PVA. In vitrification-based methods, tolerance to freezing in LN is achieved through an osmotic process when shoot tips are placed in highly concentrated vitrification solutions (Volk et al., 2006). In addition to any LN effect, the long PVS2 exposure duration of 135 min used in this study might have selectively weakened and killed cells that harbored viruses through osmotic and chemical stress and therefore, influenced the production of virus-free plants after cryotherapy. It is worth noting that although cryotherapy alone (135 min PVS2 + LN) was able to produce at least one virus-free plant in each potato cultivar, when combined with chemotherapy it resulted in higher shoot regrowth and virus eradication frequencies.
Results obtained in the present study clearly demonstrate that PVS, PVA, and PVM in single or mixed infection negatively affect microtuber production of in vitro cultures of “Dunluce,” “Tahi,” and “V500.” This finding is consistent with results reported by Li et al. (2013, 2018b) and Zhang et al. (2019). Li et al. (2013) and Zhang et al. (2019) also noted that the co-infections of PLRV + PVY and PLRV + PVS and the triple infection of PVY and PVX + PVS could lead to negative effects on vegetative growth of potato in vitro. Negative effects on tuber yield of single or mixed infections of PVX + PVS have also been reported in greenhouse- and field-grown potato plants (Wright, 1977; Nyalugwe et al., 2012; Gong et al., 2019). Negative effects of virus infection on tuber yield of field-grown potato plants have been well documented and have long been a constraint for sustainable potato production (Brunt, 2011; Wang et al., 2011). This highlights the need to produce healthy and virus-free seed potatoes to support the potato industry.
To guard against possible false negatives, the virus status of plants that underwent in vitro therapies should be assessed for a second time after the plants have grown in the greenhouse: one should not rely just on the test result for in vitro plants. Virus titer may have been reduced and virus particles may occur in tissues that are not sampled for virus testing at the in vitro stage in some treatments, resulting in false negative, as we have shown in two “V500” plants (PVM-infected) derived from thermotherapy and chemotherapy treatments in this research and previously in raspberry and apple plants infected with Raspberry bushy dwarf virus and Apple hammerhead viroid, subjected to chemotherapy treatment (Mathew et al., 2021), and combined thermotherapy with cryotherapy (Bettoni et al., 2022), respectively. Therefore, repeat virus testing on progenies growing in the greenhouse and later in the field is recommended as standard best practice to confirm virus-free status of the plant materials.
In addition to presenting a protocol for virus eradication, we also identified an effective droplet-vitrification cryopreservation method to preserve potato shoot tips for long-term storage, using in vitro plants as source materials. Nodal sections from this material were placed on BM medium for 1 week to generate shoots from which uniform 1 mm apical shoots could be excised. Shoot tips were precultured on media enriched with sucrose, followed by PVS2 treatment for 15 min at 22°C prior to LN exposure. Shoot tips were warmed in unloading solution and placed on intermediate recovery medium overnight and, finally, transferred to recovery medium. This method resulted in high regrowth (79%) in potato cultivar “Dunluce.” Availability of a simple and efficient cryopreservation protocol that results in high levels of viability (≥ 40% after LN exposure), such as the one presented here, may facilitate the technology transfer between laboratories and the implementation of cryopreserved base collections (Volk et al., 2016; Bettoni et al., 2019b, 2021b).
Our study identified procedures that resulted in high frequencies of eradication of PVS, PVA, and PVM viruses from infected potato cultivars. The consistency of the results across three cultivars with single or mixed infections suggests that these procedures have great potential to assist the production and supply of virus-free planting materials for the potato industry. Furthermore, it might also be a valuable tool to support the global exchange of germplasm underpinning breeding activities.
Data Availability Statement
The datasets presented in the study are either included in the article or in the Supplementary Material: further inquiries can be directed to the corresponding author.
Author Contributions
JB, LM, RP, CW, DH, and JN conceived and designed the research and internal/external liaison with partners and service providers for contracts and IP rights protection. JB and LM planned and performed the experiments. JB, LM, and CW collected plant material for diagnosis. JB collected and interpreted the data, conducted the microtuber experiment, acclimatized and managed the plants in the greenhouse, prepared tables and figures, and wrote the original manuscript. DH wrote the diagnosis protocol. AM conducted the statistical analysis and helped prepare the figures. SK and JT developed the primers for virus diagnosis. All authors contributed to the article and approved the submitted version.
Funding
This research was funded by the New Zealand Institute for Plant and Food Research Limited SSIF funding (1972—“Breeding Technology Development”).
Conflict of Interest
JB LM, RP, CW, DH, AM, and JN were employed by The New Zealand Institute for Plant and Food Research Limited.
The remaining authors declare that the research was conducted in the absence of any commercial or financial relationships that could be construed as a potential conflict of interest.
Publisher’s Note
All claims expressed in this article are solely those of the authors and do not necessarily represent those of their affiliated organizations, or those of the publisher, the editors and the reviewers. Any product that may be evaluated in this article, or claim that may be made by its manufacturer, is not guaranteed or endorsed by the publisher.
Acknowledgments
The authors wish to thank Pam Fletcher and Samantha Baldwin for providing virus-infected potato tissue cultures, Andrew Mullan and Belinda Diepenheim for tissue culture media preparation, Julie Ryan and Ian King for technical assistance in the greenhouse, Juliana Souza for technical help with acclimatization of potato plants, Benjamin Franzmayr of Slipstream Automation for RT-qPCR analysis, and Keith Funnell and Mary Christey for providing internal review of the manuscript.
Supplementary Material
The Supplementary Material for this article can be found online at: https://www.frontiersin.org/articles/10.3389/fpls.2022.878733/full#supplementary-material
Footnotes
References
Adolf, B., Andrade-Piedra, J., Molina, F. R., Przetakiewicz, J., Hausladen, H., Kromann, P., et al. (2020). “Fungal, oomycete, plasmodiophorid diseases of potato,” in The Potato Crop. eds. H. Campos and O. Ortiz (Cham: Springer), 307–350.
Agindotan, B. O., Shiel, P. J., and Berger, P. H. (2007). Simultaneous detection of potato viruses, PLRV, PVA, PVX and PVY from dormant potato tubers by TaqMan® real-time RT-PCR. J. Virol. Methods 142, 1–9. doi: 10.1016/j.jviromet.2006.12.012
Agresti, A., and Coull, B. A. (1998). Approximate is better than “exact” for interval estimation of binomial proportions. Am. Stat. 52, 119–126. doi: 10.2307/2685469
AlMaarri, K., Massa, R., and AlBiski, F. (2012). Evaluation of some therapies and meristem culture to eliminate potato Y potyvirus from infected potato plants. Plant Biotechnol. 29, 237–243. doi: 10.5511/plantbiotechnology.12.0215a
Antonova, O. Y., Apalikova, O. V., Ukhatova, Y. V., Krylova, E. A., Shuvalov, O. Y., Shuvalova, A. R., et al. (2017). Eradication of viruses in microplants of three cultivated potato species (Solanun tuberosum L., S. phureja Juz. & bulk., S. stenotomum Juz. & Buk.) using combined thermos-chemotherapy method. Agric. Biol. 52, 95–104. doi: 10.15389/agrobiology.2017.1.95eng
Awasthi, L. P., and Verma, H. N. (2017). Current status of viral diseases of potato and their eco-friendly management – a critical review. Virol. Res. Rev. 1, 1–16. doi: 10.15761/VRR.1000122
Bettoni, J. C., Bonnart, R., and Volk, G. M. (2021b). Challenges in implementing plant shoot tip cryopreservation technologies. Plant Cell Tissue Organ. Cult. 144, 21–34. doi: 10.1007/s11240-020-01846-x
Bettoni, J. C., Costa, M. D., Gardin, J. P. P., Kretzschmar, A. A., and Pathirana, R. (2016). Cryotherapy: a new technique to obtain grapevine plants free of viruses. Rev. Bras. Frutic. 38:e-833. doi: 10.1590/0100-29452016833
Bettoni, J. C., Costa, M. D., Souza, J. A., Volk, G. M., Nickel, O., da Silva, F. N., et al. (2018). Cryotherapy by encapsulation-dehydration is effective for in vitro eradication of latent viruses from ‘Marubakaido’ apple rootstock. J. Biotechnol. 269, 1–7. doi: 10.1016/j.jbiotec.2018.01.014
Bettoni, J. C., Fazio, G., Costa, L. C., Hurtado-Gonzales, O. P., Rwahnih, M. A., Nedrow, A., et al. (2022). Thermotherapy followed by shoot tip cryotherapy eradicates latent viruses and apple hammerhead viroid from in vitro apple rootstocks. Plan. Theory 11:582. doi: 10.3390/plants11050582
Bettoni, J. C., Kretzschmar, A. A., Bonnart, R., Shepherd, A., and Volk, G. M. (2019b). Cryopreservation of 12 Vitis species using apical shoot tips derived from plants grown in vitro. HortSciece 54, 976–981. doi: 10.21273/HORTSCI13958-19
Bettoni, J. C., Marković, Z., Bi, W., Volk, G. M., Matsumoto, T., and Wang, Q.-C. (2021a). Grapevine shoot tip cryopreservation and cryotherapy: secure storage of disease-free plants. Plants 10:2190. doi: 10.3390/plants10102190
Bettoni, J. C., Souza, J. A., Volk, G. M., Costa, M. D., da Silva, F. N., and Kretzschmar, A. A. (2019a). Eradication of latent viruses from apple cultivar ‘Monalisa’ shoot tips using droplet-vitrification cryotherapy. Sci. Hortic. 250, 12–18. doi: 10.1016/j.scienta.2019.02.033
Bi, W. L., Hao, X.-Y., Cui, Z.-H., Pathirana, R., Volk, G. M., and Wang, Q.-C. (2018). Shoot tip cryotherapy for efficient eradication of grapevine leafroll-associated virus-3 from diseased grapevine in vitro plants. Ann. Appl. Biol. 173, 261–270. doi: 10.1111/aab.12459
Botermans, M., Van de Vossenberg, B., Verhoeven, J. T. J., Roenhorst, J., Hooftman, M., Dekter, R., et al. (2013). Development and validation of a real-time RT-PCR assay for generic detection of pospiviroids. J. Virol. Methods 187, 43–50. doi: 10.1016/j.jviromet.2012.09.004
Bradshaw, J. E. (2021). “Improving resistance to diseases and pests: a dynamic situation,” in Potato Breeding: Theory and Practice. ed. J. E. Bradshaw (Cham: Springer Nature), 247–337. doi: 10.1007/978-3-030-64414-7_5
Brunt, A. A. (2011). “The main viruses infecting potato crops,” in Virus and virus-like diseases of potatoes and production of seed-potatoes. eds. G. Loebenstein, P. H. Berger, A. Brunt, and R. H. Lawson (Dordrecht: Kluwer), 65–67. doi: 10.1007/978-94-007-0842-6_5
Cui, Z. H., Li, B. Q., Bi, W. L., and Wang, Q. C. (2015). Plant pathogen eradication by cryotherapy of shoot tips: development, achievements and prospective. Acta Hortic. 1083, 35–41. doi: 10.17660/ActaHortic.2015.1083.2
Devaux, A., Goffart, J. P., Kromann, P., Andrade-Piedra, J., Polar, V., and Hareau, G. (2021). The potato of the future: opportunities and challenges in sustainable Agri-food systems. Potato Res. 64, 681–720. doi: 10.1007/s11540-021-09501-4
Dhital, S. P., Sakha, B. M., and Lim, H. T. (2007). Utilization of shoot cuttings for elimination of PLRV and PVY by thermotherapy and chemotherapy from potato (Solanum tuberosum L.). Nepal J. Sci. Technol. 7, 1–6. doi: 10.3126/njst.v7i0.528
Ellis, D., Salas, A., Chavez, O., Gomez, R., and Anglin, N. (2020). “Ex situ conservation of potato [Solanum section Petota (Solanaceae)] genetic resources in genebanks,” in The Potato Crop. eds. H. Campos and O. Ortiz (Cham: Springer), 109–138.
Faccioli, G., and Marani, F. (1998). “Virus elimination by meristem tip culture and tip micrografting,” in Plant Virus Diseases Control. eds. A. Hadidi, A. R. K. Khetarpal, and H. Koganezawa (St. Paul, MN: APS Press), 346–380.
Fang, Y. L., Dhital, S. P., Li, K. H., Khu, D. M., Kim, H. Y., and Lim, H. T. (2005). Utilization of single nodal cuttings and therapies for eradication of double-infected potato viruses (PLRV and PVY) from in vitro plantlets of potato (Solanum tuberosum). J. Kor. Soc. Hortic. Sci. 46, 119–125.
FAOSTAT-Food and Agriculture Organization of the United Nations (2021). Crops and livestock products. Available at: http://www.fao.org/faostat/en/#data/QCL. (Accessed September 02, 2021).
Farhadi-Tooli, S., Ghanbari, A., Kermani, M. J., Zeinalabedini, M., Bettoni, J. C., Naji, A. M., et al. (2022). Droplet-vitrification cryotherapy and thermotherapy as efficient tools for the eradication of apple chlorotic leaf spot virus and apple stem grooving virus from virus-infected quince in vitro cultures. Eur. J. Plant Pathol. 162, 31–43. doi: 10.1007/s10658-021-02400-x
Farnham, G., and Baulcombe, D. C. (2006). Artificial evolution extends the spectrum of viruses that are targeted by a disease–resistance gene from potato. Proc. Natl. Acad. Sci. U. S. A. 103, 18828–18833. doi: 10.1073/pnas.0605777103
Fletcher, J. D. (2012). A virus survey of New Zealand fresh, process and seed potato crops during 2010-11. N. Z. Plant Prot. 65, 197–203. doi: 10.30843/nzpp.2012.65.5366
Fletcher, P. J., Fletcher, J. D., and Cross, R. J. (1988). Potato germplasm: in vitro storage and virus reduction. N. Z. J. Crop. Hortic. Sci. 26, 249–252. doi: 10.1080/01140671.1998.9514060
Fuentes, S., Gibbs, A. J., Adams, I. P., Wilson, C., Botermans, M., Fox, A., et al. (2021). Potato virus A isolates from three continents: their biological properties, phylogenetics, and prehistory. Phytopathology 111, 217–226. doi: 10.1094/PHYTO-08-20-0354-FI
García-Cano, E., Resende, R. O., Fernández-Muñoz, R., and Moriones, E. (2006). Synergistic interaction between tomato chlorosis virus and tomato spotted wilt virus results in breakdown of resistance in tomato. Phytopathology 96, 1263–1269. doi: 10.1094/PHYTO-96-1263
Gong, H., Igiraneza, C., and Dusengemungu, L. (2019). Major in vitro techniques for potato virus elimination and post eradication detection methods. A review. Am. J. Potato Res. 96, 379–389. doi: 10.1007/s12230-019-09720-z
Jianming, B., Xiaoling, C., Xinxiong, L., Huachun, G., Xia, X., and Zhie, Z. (2012). Can cryopreservation eliminate the potato virus X (PVX) and potato spindle tuber viroid (PSTVd)? Biosci. Meth. 3, 34–40. doi: 10.5376/bm.2012.03.0005
Khan, S., Mackay, J., Liefting, L., and Ward, L. (2015). Development of a duplex one-step RT-qPCR assay for the simultaneous detection of apple scar skin viroid and plant RNA internal control. J. Virol. Methods 221, 100–105. doi: 10.1016/j.jviromet.2015.04.032
Kim, H. H., Yoon, J. W., Park, Y. E., Cho, E. F., Sohn, J. K., Kim, T. S., et al. (2006). Cryopreservation of potato cultivated varieties and wild species: critical factors in droplet vitrification. Cryo Lett. 27, 223–234.
Kreuze, J. F., Souza-Dias, J. A. C., Jeevalatha, A., Figueira, A. R., Valkonen, J. P. T., and Jones, R. A. C. (2020). “Viral diseases in potato,” in The Potato Crop. eds. H. Campos and O. Ortiz (Cham: Springer), 389–430.
Kushnarenko, S., Romadanova, N., Aralbayeva, M., Zholamanova, S., Alexandrova, A., and Karpova, O. (2017). Combined ribavirin treatment and cryotherapy for efficient potato virus M and potato virus S eradication in potato (Solanum tuberosum L.) in vitro shoots. In vitro cell. Dev. Biol. Plant 53, 425–432. doi: 10.1007/s11627-017-9839-0
Li, J. W., Chen, H. Y., Li, J., Zhang, Z., Blystad, D. R., and Wang, Q. C. (2018b). Growth, microtuber production and physiological metabolism in virus-free and virus-infected potato in vitro plantlets grown under NaCl-induced salt stress. Eur. J. Plant Pathol. 152, 417–432. doi: 10.1007/s10658-018-1485-9
Li, B. Q., Feng, C. H., Hu, L. Y., Wang, M. R., and Wang, Q. C. (2016). Shoot tip culture and cryopreservation for eradication of apple stem pitting virus (ASPV) and apple stem grooving virus (ASGV) from apple rootstocks ‘M9’ and ‘M26’. Ann. Appl. Biol. 168, 142–150. doi: 10.1111/aab.12250
Li, J. W., Wang, M. R., Chen, H. Y., Zhao, L., Cui, Z. H., Zhang, Z., et al. (2018a). Long-term preservation of potato leafroll virus, potato virus S, and potato spindle tuber viroid in cryopreserved shoot tips. Appl. Microbiol. Biotechnol. 102, 10743–10754. doi: 10.1007/s00253-018-9405-7
Li, J. W., Wang, B., Song, X. M., Wang, R. R., Chen, L., Zhang, H., et al. (2013). Potato leafroll virus (PLRV) and potato virus Y (PVY) influence vegetative growth, physiological metabolism, and microtuber production of in vitro-grown shoots of potato (Solanum tuberosum L.). Plant. Cell. Tiss. Org. 114, 313–324. doi: 10.1007/s11240-013-0327-x
Lin, Y. H., Johnson, D. A., and Pappu, H. R. (2014). Effect of potato virus S infection on late blight resistance in potato. Am. J. Potato Res. 91, 642–648. doi: 10.1007/s12230-014-9394-8
Linsmaier, E. M., and Skoog, F. (1965). Organic growth factor requirements of tobacco tissue cultures. Physiol. Plant. 18, 100–127. doi: 10.1111/j.1399-3054.1965.tb06874.x
Magyar-Tábori, K., Mendler-Drienyovszki, N., Hanász, A., Zsombik, L., and Dobránszki, J. (2021). Phytotoxicity and other adverse effects on the in vitro shoot cultures caused by virus elimination treatments: reasons and solutions. Plan. Theory 10:670. doi: 10.3390/plants10040670
Mathew, L., Tiffin, H., Erridge, Z., McLachlan, A., Hunter, D., and Pathirana, R. (2021). Efficiency of eradication of raspberry bushy dwarf virus from infected raspberry (Rubus idaeus) by in vitro chemotherapy, thermotherapy and cryotherapy and their combinations. Plant Cell Tissue Organ Cult. 144, 133–141. doi: 10.1007/s11240-020-01829-y
Menzel, W., Jelkmann, W., and Maiss, E. (2002). Detection of four apple viruses by multiplex RT-PCR assays with coamplification of plant mRNA as internal control. J. Virol. Methods 99, 81–92. doi: 10.1016/s0166-0934(01)00381-0
Moreno, A. B., and López-Moya, J. J. (2020). When viruses play team sports: mixed infections in plants. Phytopathology 110, 29–48. doi: 10.1094/PHYTO-07-19-0250-FI
Murashige, T., and Skoog, F. (1962). A revised medium for rapid growth and bio assays with tobacco tissue cultures. Physiol. Planta. 15, 473–497. doi: 10.1111/j.1399-3054.1962.tb08052.x
Naik, P. S., and Khurana, S. P. (2003). Micropropagation in potato seed production: need to revise seed certification standards. J. Indian Potato Assoc. 30, 267–273.
Nasir, I. A., Tabassum, B., Latif, Z., Javed, M. A., Haider, M. S., Javed, M. A., et al. (2010). Strategies to control potato virus Y under in vitro conditions. Pak. J. Phytopathol. 22, 63–70.
Nazarov, P. A., Baleev, D. N., Ivanova, M. I., Sokolova, L. M., and Karakozova, M. V. (2020). Infectious plant diseases: etiology, current status, problems and prospects in plant protection. Acta Nat. 12, 46–59. doi: 10.32607/actanaturae.11026
Nyalugwe, E. P., Wilson, C. R., Coutts, B. A., and Jones, R. A. C. (2012). Biological properties of potato virus X in potato: effects of mixed infection with potato virus S and resistance phenotypes in cultivars from three continents. Plant Dis. 96, 43–54. doi: 10.1094/PDIS-04-11-0305
Onofre, K. F. A., Forbes, G. A., Andrade-Piedra, J. L., Buddenhagen, C. E., Fulton, J. C., Gatto, M., et al. (2021). An integrated seed health strategy and phytosanitary risk assessment: potato in the republic of Georgia. Agric. Syst. 191:103144. doi: 10.1016/j.agsy.2021.103144
Orbegozo, J., Solorzano, D., Cuellar, W. J., Bartolini, I., Roman, L. M., Ghislain, M., et al. (2016). Marker-free PLRV resistant potato mediated by Cre-loxP excision and RNAi. Transgenic Res. 25, 813–828. doi: 10.1007/s11248-016-9976-y
Pathirana, R., Mathew, L., Jibran, R., Hunter, D. A., and Morgan, E. R. (2019). Cryopreservation and cryotherapy research on horticultural crops in New Zealand. Acta Hortc. 1234, 29–36. doi: 10.17660/ActaHortic.2019.1234.4
Pathirana, R., McLachlan, A., Hedderley, D., Carra, A., Carimi, F., and Panis, B. (2015). Removal of leafroll viruses from infected grapevine plants by droplet vitrification. Acta Hortic. 1083, 491–498. doi: 10.17660/ActaHortic.2015.1083.64
Potatoes NZ. (2021). AGM papers and annual report 2021. Annual reports - Potatoes New Zealand. Available at: info@potatoesnz.co.nz (Accessed September 02, 2021).
Priegnitz, U., Lommen, W. J. M., van der Vlugt, R. A. A., and Struik, P. C. (2019). Impact of positive selection on incidence of different viruses during multiple generations of potato seed tubers in Uganda. Potato Res. 62, 1–30. doi: 10.1007/s11540-018-9394-z
Priegnitz, U., Lommen, W. J. M., van der Vlugt, R. A. A., and Struik, P. C. (2020). Potato yield and yield components as affected by positive selection during several generations of seed multiplication in southwestern Uganda. Potato Res. 63, 507–543. doi: 10.1007/s11540-020-09455-z
Quazi, M. H., and Martin, S. D. (1978). Pathogen-free potato plants regenerated from meristem-tip cultures. N. Z. J. Exp. Agric. 6, 305–307. doi: 10.1080/03015521.1978.10426293
Rubio, L., Galipienso, L., and Ferriol, I. (2020). Detection of plant viruses and disease management: relevance of genetic diversity and evolution. Front. Plant Sci. 11:1092. doi: 10.3389/fpls.2020.01092
Sakai, A., Kobayashi, S., and Oiyama, I. (1990). Cryopreservation of nucellar cells of navel orange (Citrus sinensis Osb. Var. brasiliensis Tanaka) by vitrification. Plant Cell Rep. 9, 30–33. doi: 10.1007/BF00232130
Souza, J. A., Bogo, A., Bettoni, J. C., Dalla Costa, M., da Silva, F. N., Casa, R. T., et al. (2020). Droplet-vitrification cryotherapy for eradication of apple stem grooving virus and apple stem pitting virus from “Marubakaido” apple rootstock. Trop. Plant Pathol. 45, 148–152. doi: 10.1007/s40858-019-00321-z
Tang, X., Zhang, N., Si, H., and Calderón-Urrea, A. (2017). Selection and validation of reference genes for RT-qPCR analysis in potato under abiotic stress. Plant Methods 13:85. doi: 10.1186/s13007-017-0238-7
Thomas-Sharma, S., Abdurahman, A., Ali, S., Andrade-Piedra, J. L., Bao, S., Charkowski, A. O., et al. (2016). Seed degeneration in potato: the need for an integrated seed health strategy to mitigate the problem in developing countries. Plant Pathol. 65, 3–16. doi: 10.1111/ppa.12439
Tian, Y.-P., and Valkonen, J. P. T. (2013). Genetic determinants of potato virus Y required to overcome or trigger hypersensitive resistance to PVY strain group O controlled by the gene Ny in potato. Mol. Plant-Microbe Interact. 26, 297–305. doi: 10.1094/MPMI-09-12-0219-R
Valkonen, J. P. T. (2007). “Potato viruses: economical losses and biotechnological potential,” in Potato Biology and Biotechnology: Advances and Perspectives. eds. D. Vreugdenhil, J. Bradshaw, C. Gebhardt, F. Govers, D. K. L. MacKerron, and M. A. Taylor, et al. (Netherlands: Elsevier Science), 619–641.
Valkonen, J. P. T. (2008). Novel resistances to four potyviruses in tuber-bearing potato species, and temperature-sensitive expression of hypersensitive resistance to potato virus Y. Ann. Appl. Biol. 130, 91–104. doi: 10.1111/j.1744-7348.1997.tb05785.x
Volk, G. M., Harris, J. L., and Rotindo, K. E. (2006). Survival of mint shoot tips after exposure to cryoprotectant solution components. Cryobiology 52, 305–308. doi: 10.1016/j.cryobiol.2005.11.003
Volk, G. M., Henk, A. D., Jenderek, M., and Richards, C. M. (2016). Probabilistic Viability Calculations for cryopreserving vegetatively propagated collections in genebanks. Genet. Resources Crop Evol. 64, 1613–1622. doi: 10.1007/s10722-016-0460-6
Volmer, R., Villagaray, R., Cárdenas, J., Castro, M., Chávez, O., Anglin, N., et al. (2017). A large-scale viability assessment of the potato cryobanks at the international potato center (CIP). In vitro cell. Dev. Biol. Plant 53, 309–317. doi: 10.1007/s11627-017-9846-1
Wakasa, T., Kasai, A., Yamazaki, M., Tabei, Y., Tsuyama, M., Igarashi, T., et al. (2020). Rapid analysis of GBSS1 and Vinv genes expressed in potato tubers using microtubers produced in liquid culture medium. Plant Cell Rep. 39, 1415–1424. doi: 10.1007/s00299-020-02572-6
Wang, M. R., Bi, W. L., Bettoni, J. C., Zhang, D., Volk, G. M., and Wang, Q. C. (2022b). Shoot tip cryotherapy for plant pathogen eradication. Plant Pathol. 1–14. doi: 10.1111/ppa.13565
Wang, Q., Cuellar, W. J., Rajamaki, M. L., Hirata, Y., and Valkonen, J. P. T. (2008). Combined thermotherapy and cryotherapy for efficient virus eradication: relation of virus distribution, subcellular changes, cell survival and viral RNA degradation in shoot tips. Mol. Plant Pathol. 9, 237–250. doi: 10.1111/j.1364-3703.2007.00456.x
Wang, M. R., Cui, Z. H., Li, J. W., Hao, X. Y., Zhao, L., and Wang, Q. C. (2018a). In vitro thermotherapy-based methods for plant virus eradication. Plant Methods 14:87. doi: 10.1186/s13007-018-0355-y
Wang, M. R., Hamborg, Z., Blystad, D. R., and Wang, Q. C. (2021). Combining thermotherapy with meristem culture for improved eradication of onion yellow dwarf virus and shallot latent virus from infected in vitro-cultured shallot shoots. Ann. Appl. Biol. 178, 442–449. doi: 10.1111/aab.12646
Wang, M. R., Hamborg, Z., Ma, X. Y., Blystad, D. R., and Wang, Q. C. (2022a). Double-edged effects of the cryogenic technique for virus eradication and preservation in shallot shoot tips. Plant Pathol. 71, 494–504. doi: 10.1111/ppa.13466
Wang, Q., Liu, Y., Xie, Y., and You, M. (2006). Cryotherapy of potato shoot tips for efficient elimination of potato leafroll virus (PLRV) and potato virus Y (PVY). Potato Res. 49, 119–129. doi: 10.1007/s11540-006-9011-4
Wang, B., Ma, Y., Zhang, Z., Wu, Z., Wu, Y., Wang, Q., et al. (2011). Potato viruses in China. Crop Prot. 30, 1117–1123. doi: 10.1016/j.cropro.2011.04.001
Wang, Q. C., Panis, B., Engelmann, F., Lambardi, M., and Valkonen, J. P. T. (2009). Cryotherapy of shoot tips: a technique for pathogen eradication to produce healthy planting materials and prepare healthy plant genetic resources for cryopreservation. Ann. Appl. Biol. 154, 351–363. doi: 10.1111/j.1744-7348.2008.00308.x
Wang, Q. C., and Valkonen, J. P. T. (2009). Cryotherapy of shoot tips: novel pathogen eradication method. Trends Plant Sci. 14, 119–122. doi: 10.1016/j.tplants.2008.11.010
Wang, M. R., Yang, W., Zhao, L., Li, J. W., Liu, K., Yu, J. W., et al. (2018b). Cryopreservation of virus: a novel biotechnology for long-term preservation of virus in shoot tips. Plant Methods 14:47. doi: 10.1186/s13007-018-0312-9
Wasilewska-Nascimento, B., Boguszewska-Mańkowska, D., and Zarzyńska, K. (2020). Challenges in the production of high-quality seed potatoes (Solanum tuberosum L.) in the tropics and subtropics. Agron. 10:260. doi: 10.3390/agronomy10020260
Waswa, M., Kakuhenzire, R., and Ochwo-ssemakula, M. (2017). Effect of thermotherapy duration, virus type and cultivar interactions on elimination of potato viruses X and S in infected seed stocks. African J. Plant Sci. 11, 61–70. doi: 10.5897/AJPS2016.1497
Wright, N. S. (1977). The effect of separate infections by potato viruses X and S on netted gem potato. Am. Potato J. 54, 147–149. doi: 10.1007/BF02852870
Yi, J. Y., Lee, G. A., Jeong, J. W., Lee, S. Y., and Lee, Y. G. (2014). Eliminating potato virus Y (PVY) and potato leaf roll virus (PLRV) using cryotherapy of in vitro-grown potato shoot tips. Korean J. Crop Sci. 59, 498–504. doi: 10.7740/KJCS.2014.59.4.498
Zhang, Z., Wang, Q. C., Spetz, C., and Blystay, D. R. (2019). In vitro therapies for virus elimination of potato-valuable germplasm in Norway. Sci. Hortic. 249, 7–14. doi: 10.1016/j.scienta.2019.01.027
Keywords: cryotherapy, thermotherapy, chemotherapy, high-health plants, shoot tips, cryopreservation, microtubers, Solanum tuberosum
Citation: Bettoni JC, Mathew L, Pathirana R, Wiedow C, Hunter DA, McLachlan A, Khan S, Tang J and Nadarajan J (2022) Eradication of Potato Virus S, Potato Virus A, and Potato Virus M From Infected in vitro-Grown Potato Shoots Using in vitro Therapies. Front. Plant Sci. 13:878733. doi: 10.3389/fpls.2022.878733
Edited by:
Walter Chitarra, Council for Agricultural and Economics Research (CREA), ItalyReviewed by:
Giorgio Gambino, Institute for Sustainable Plant Protection (CNR), ItalySvetlana Kushnarenko, Institute of Plant Biology and Biotechnology, Kazakhstan
Copyright © 2022 Bettoni, Mathew, Pathirana, Wiedow, Hunter, McLachlan, Khan, Tang and Nadarajan. This is an open-access article distributed under the terms of the Creative Commons Attribution License (CC BY). The use, distribution or reproduction in other forums is permitted, provided the original author(s) and the copyright owner(s) are credited and that the original publication in this journal is cited, in accordance with accepted academic practice. No use, distribution or reproduction is permitted which does not comply with these terms.
*Correspondence: Jean Carlos Bettoni, Jean.Bettoni@plantandfood.co.nz
†Present address: Ranjith Pathirana, Plant & Food Research Australia Pty Ltd., Waite Institute, Urrbrae, SA, Australia
‡These authors share first authorship