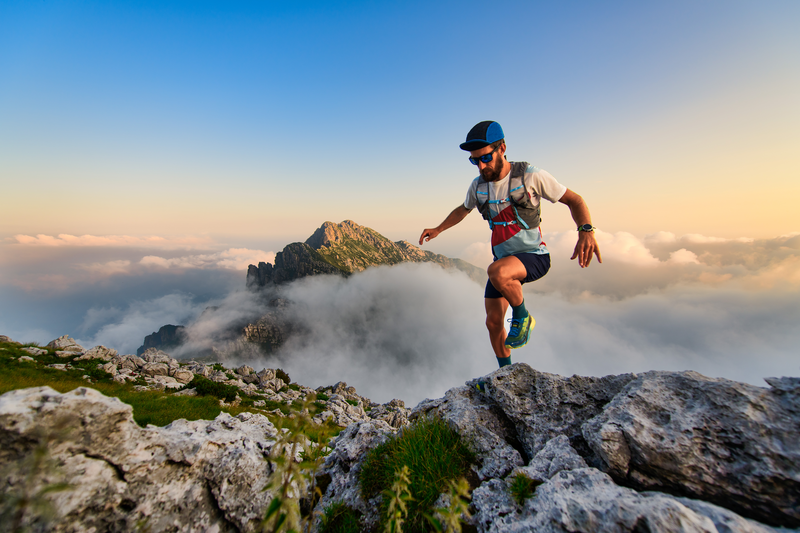
95% of researchers rate our articles as excellent or good
Learn more about the work of our research integrity team to safeguard the quality of each article we publish.
Find out more
EDITORIAL article
Front. Plant Sci. , 16 March 2022
Sec. Plant Abiotic Stress
Volume 13 - 2022 | https://doi.org/10.3389/fpls.2022.876432
This article is part of the Research Topic Multi-disciplinary Approaches to Plant Responses to Climate Change View all 5 articles
Editorial on the Research Topic
Multi-Disciplinary Approaches to Plant Responses to Climate Change
In August 2021, the Intergovernmental Panel on Climate Change (IPCC, 2021) released a new report on the anthropogenic effects of climate change. Climate change is causing a steady rise of global surface temperature and atmospheric CO2 concentration, increasing the frequency and intensity of temperature extremes, causing heat waves, heavy precipitation, and, in some regions, agricultural and ecological droughts (Schiermeier, 2018). Such knock-on effects of climate change have a dire impact on plants, affecting plant development and reproduction, impacting natural vegetation and agriculture. Hence, to overcome changes in their environment, plants must adapt swiftly and respond to changes in their natural settings or else face extinction.
Environmental cues, such as progressive changes in photoperiod, or in day and nighttime temperatures, allow the plant to make intricate developmental decisions to negotiate diverse environmental conditions through different seasons (Wilczek et al., 2009; Zhao et al., 2020). On the other hand, severe and sudden environmental changes lead to stresses that the plants must quickly respond and adapt to, in order to survive (Balasubramanian et al., 2006; Berg et al., 2019). Thus, it is crucial for us to continue to advance our overall understanding of how plants respond and adapt to environmental changes, and to work toward improving their resilience in face of the unpredictability of climate change.
Several decades of studies, especially in model plants such as Arabidopsis, have demonstrated the complexity of molecular mechanisms, that plants employ to perceive and respond to the ever-changing environments, highlighting the need for large-scale data analysis, integration, and comparison of multiple related data sets, as well as for the combination of multidisciplinary approaches to address such complex problems, e.g., Jung et al. (2016), Cortijo et al. (2017), Awlia et al. (2021), and Sriden and Charoensawan (2022). More recently, latest technological developments and improvements have allowed the acquisition of phenomics, physiological, transcriptomic, epigenomic, and microscopic data, as well as comprehensive data integration, in non-model crops and agricultural plants, as summarized in Zenda et al. in this Research Topic, and also other recent reviews (Wee and Dinneny, 2010; Scossa et al., 2021; Yang et al., 2021).
This Research Topic highlights multidisciplinary efforts that tackle responses to a wide variety of environmental changes associated with climate change, including cold (Wang et al.), drought (Wang et al.; Liu et al.) and even mineral deficiency (Zhang et al.). Interestingly, all the articles presented in this issue focus on non-model crop plants, highlighting the importance of applying omics and multi-omics techniques beyond conventional model plant species, as well as showing that omics techniques have been fully developed to be successfully translated in a wide variety of crop species.
In this Research Topic, Wang et al. combined transcriptomics and metabolomics to explore genes and metabolites that are involved in responses to cold and drought stresses in Poa crymophila Keng, a variation of gramineous forage grass widely distributed in the Qinghai-Tibet Plateau. The authors were able to identify the phenylpropanoid pathway, among a few others, as the key mechanism that allows the plant to adapt to these harsh environments. Using transcriptomics in combination with physiological analyses, Liu et al. have characterized morphological and physiological responses against chilling stress in 14 pumpkin (Cucurbita moschata) varieties, and identified differentially expressed genes in the leaves and the roots, notably, several in the α-linolenic acid biosynthesis pathway. Zhang et al. used a combination of phenomics, statistical methods and physiological measurements, including photosynthetic indicators to monitor the root architecture phenotypes of cotton (Gossypium hirsutum) under Phosphorus deficiency. The authors presented RhizoPot, an improvised in situ root phenotyping observation device, and found that several changes in root surface area are important indicators of cotton root phenotypes under low phosphorus. Finally, Zenda et al. reviewed the recent developments in omics that can help us toward crop improvement and breeding more resilient plants.
In summary, the articles presented in this Research Topic further reiterate that multidisciplinary approaches have already shown their importance, and are on their way to becoming indispensable in our quest to understand how the model plants as well as non-model crops respond to a wide range of stress conditions. This will in turn pave the way for the rational design and development of crops that are resilient to various stresses associated with climate change.
VC, SC, MD, and SN wrote and revised the article. All authors contributed to the article and approved the submitted version.
Work in the laboratory of VC was supported by the mid-career researcher grant from National Research Council of Thailand (NRCT) and Mahidol University (NRCT5-RSA63015-24), and Mahidol University's Postdoctoral Fellowship (MU-PD_2021_09). SN acknowledges the funding from Science Foundation Ireland through the SFI President of Ireland Future Research Leaders under Grant No. 18/FRL/6197.
The authors declare that the research was conducted in the absence of any commercial or financial relationships that could be construed as a potential conflict of interest.
All claims expressed in this article are solely those of the authors and do not necessarily represent those of their affiliated organizations, or those of the publisher, the editors and the reviewers. Any product that may be evaluated in this article, or claim that may be made by its manufacturer, is not guaranteed or endorsed by the publisher.
Awlia, M., Alshareef, N., Saber, N., Korte, A., Oakey, H., Panzarová, K., et al. (2021). Genetic mapping of the early responses to salt stress in Arabidopsis thaliana. Plant J. 107, 544–563. doi: 10.1111/tpj.15310
Balasubramanian, S., Sureshkumar, S., Lempe, J., and Weigel, D. (2006). Potent induction of Arabidopsis thaliana flowering by elevated growth temperature. PLoS Genet. 2:e106. doi: 10.1371/journal.pgen.0020106
Berg, C. S., Brown, J. L., and Weber, J. J. (2019). An examination of climate-driven flowering-time shifts at large spatial scales over 153 years in a common weedy annual. Am. J. Bot. 106, 1435–1443. doi: 10.1002/ajb2.1381
Cortijo, S., Charoensawan, V., Brestovitsky, A., Buning, R., Ravarani, C., Rhodes, D., et al. (2017). Transcriptional regulation of the ambient temperature response by H2A.Z nucleosomes and HSF1 transcription factors in arabidopsis. Mol. Plant. 10, 1258–1273. doi: 10.1016/j.molp.2017.08.014
IPCC (2021). “Climate change 2021: the physical science basis,” in Contribution of Working Group I to the Sixth Assessment Report of the Intergovernmental Panel on Climate Change, editors V. Masson-Delmotte, P. Zhai, A. Pirani, S. L. Connors, C. Péan, S. Berger, et al. Cambridge University Press.
Jung, J. H., Domijan, M., Klose, C., Biswas, S., Ezer, D., Gao, M., et al. (2016). Phytochromes function as thermosensors in Arabidopsis. Science 354, 886–889. doi: 10.1126/science.aaf6005
Schiermeier, Q. (2018). Droughts, heatwaves and floods: how to tell when climate change is to blame. Nature 560, 20–22. doi: 10.1038/d41586-018-05849-9
Scossa, F., Alseekh, S., and Fernie, A. R. (2021). Integrating multi-omics data for crop improvement. J. Plant Physiol. 257, 153352. doi: 10.1016/j.jplph.2020.153352
Sriden, N., and Charoensawan, V. (2022). Large-scale comparative transcriptomic analysis of temperature-responsive genes in Arabidopsis thaliana. Plant Mol. Biol. doi: 10.1007/s11103-021-01223-y. [Epub ahead of print].
Wee, C. W., and Dinneny, J. R. (2010). Tools for high-spatial and temporal-resolution analysis of environmental responses in plants. Biotechnol. Lett. 32, 1361–1371. doi: 10.1007/s10529-010-0307-8
Wilczek, A. M., Roe, J. L., Knapp, M. C., Cooper, M. D., Lopez-Gallego, C., Martin, L. J., et al. (2009). Effects of genetic perturbation on seasonal life history plasticity. Science 323, 930–934. doi: 10.1126/science.1165826
Yang, Y., Saand, M. A., Huang, L., Abdelaal, W. B., Zhang, J., Wu, Y., et al. (2021). Applications of multi-omics technologies for crop improvement. Front. Plant Sci. 12, 563953. doi: 10.3389/fpls.2021.563953
Keywords: climate change, systems biology, omics analyses, interdisciplinary research, bioinformatics
Citation: Charoensawan V, Cortijo S, Domijan M and Negrão S (2022) Editorial: Multi-Disciplinary Approaches to Plant Responses to Climate Change. Front. Plant Sci. 13:876432. doi: 10.3389/fpls.2022.876432
Received: 15 February 2022; Accepted: 21 February 2022;
Published: 16 March 2022.
Edited and reviewed by: Vicent Arbona, University of Jaume I, Spain
Copyright © 2022 Charoensawan, Cortijo, Domijan and Negrão. This is an open-access article distributed under the terms of the Creative Commons Attribution License (CC BY). The use, distribution or reproduction in other forums is permitted, provided the original author(s) and the copyright owner(s) are credited and that the original publication in this journal is cited, in accordance with accepted academic practice. No use, distribution or reproduction is permitted which does not comply with these terms.
*Correspondence: Varodom Charoensawan, dmFyb2RvbS5jaGFAbWFoaWRvbC5hYy50aA==; Sandra Cortijo, c2FuZHJhLmNvcnRpam9AY25ycy5mcg==; Mirela Domijan, TWlyZWxhLkRvbWlqYW5AbGl2ZXJwb29sLmFjLnVr; Sónia Negrão, c29uaWEubmVncmFvQHVjZC5pZQ==
Disclaimer: All claims expressed in this article are solely those of the authors and do not necessarily represent those of their affiliated organizations, or those of the publisher, the editors and the reviewers. Any product that may be evaluated in this article or claim that may be made by its manufacturer is not guaranteed or endorsed by the publisher.
Research integrity at Frontiers
Learn more about the work of our research integrity team to safeguard the quality of each article we publish.