- 1Department of Biological Resources, Centre for Agricultural Research, Eötvös Loránd Research Network, Martonvásár, Hungary
- 2Institute of Genetics and Biotechnology, Szent István Campus, MATE, Gödöllő, Hungary
- 3Centre of the Region Haná for Biotechnological and Agricultural Research, Institute for Experimental Botany of the Czech Academy of Sciences, Olomouc, Czechia
- 4Field Crops Research Institute, Agricultural Research Centre, Giza, Egypt
- 5John Innes Centre, Norwich, United Kingdom
Effective utilization of genetic diversity in wild relatives to improve wheat requires recombination between wheat and alien chromosomes. However, this is suppressed by the Pairing homoeologous gene, Ph1, on the long arm of wheat chromosome 5B. A deletion mutant of the Ph1 locus (ph1b) has been used widely to induce homoeologous recombination in wheat × alien hybrids. However, the original ph1b mutation, developed in Chinese Spring (CS) background has poor agronomic performance. Hence, alien introgression lines are first backcrossed with adapted wheat genotypes and after this step, alien chromosome segments are introduced into breeding lines. In this work, the ph1b mutation was transferred from two CSph1b mutants into winter wheat line Mv9kr1. Homozygous genotypes Mv9kr1 ph1b/ph1b exhibited improved plant and spike morphology compared to Chinese Spring. Flow cytometric chromosome analysis confirmed reduced DNA content of the mutant 5B chromosome in both wheat genotype relative to the wild type chromosome. The ph1b mutation in the Mv9kr1 genotype allowed wheat-alien chromosome pairing in meiosis of Mv9kr1ph1b_K × Aegilops biuncialis F1 hybrids, predominantly with the Mb-genome chromosomes of Aegilops relative to those of the Ub genome. High frequency of wheat-Aegilops chromosome interactions resulted in rearranged chromosomes identified in the new Mv9kr1ph1b × Ae. Biuncialis amphiploids, making these lines valuable sources for alien introgressions. The new Mv9kr1ph1b mutant genotype is a unique resource to support alien introgression breeding of hexaploid wheat.
Introduction
Bread wheat (Triticum aestivum L.) is an essential component of human nutrition. In terms of global production, it is the third most important crop after rice and maize (FAOSTAT, 2019). The annual wheat production area is 220.89 million hectares, which is ~30% of the total area used to cultivate cereals (FAOSTAT, 2018). Hexaploid wheat (2n = 6x = 42) comprises three subgenomes A, B, and D (Sears, 1952) which originated from three diploid species. Triticum urartu Tumanian ex Gandilyan (2n = 2x = 14, AuAu) is considered to be the donor of A genome, Aegilops speltoides Tausch (2n = 2x = 14, SS) is closely related to the putative B genome donor, while Ae. tauschii Coss. (2n = 2x = 14, DD) was the D genome donor (Sears, 1954; Okamoto, 1962). The hexaploid wheat genome resulted from two consecutive interspecific hybridizations and polyploidizations. The first of them occurred between T. urartu and a species similar to Ae. speltoides 0.3–0.5 million years ago and led to the origin of wild emmer wheat Triticum turgidum ssp. dicoccoides (Korn.) Thell. (AuAuBB, 2n = 4x = 28; Dvořák et al., 1993; Maestra and Naranjo, 2000). Cultivated emmer wheat T. turgidum ssp. dicoccon (Schrank) Thell, evolved from the wild emmer wheat due to human selection. Its hybridization with Ae. tauschii ~9,000 years ago gave rise to allohexaploid wheat, T. aestivum (Dvořák et al., 1998). However, because only a few genotypes were involved in these allopolyploidization events, genetic diversity of hexaploid wheat is narrow (Feldman and Levy, 2012). Also, domestication and 1,000 of years of cultivation narrowed down genetic variation of wheat (Lubbers et al., 1991; Cox, 1997; Xie et al., 2018; Cheng et al., 2019). One of the biggest challenges for breeders worldwide is to develop efficient allele combinations to produce high-yielding and stress-tolerant cultivars with good quality traits under changing global climate.
A powerful strategy to broaden genetic diversity of wheat is a transfer of new genes and alleles from primary, secondary, and tertiary genepools by interspecific or intergeneric hybridization (Friebe et al., 1996; Molnár-Láng et al., 2015). This approach was used to successfully introduce disease resistance as well as adaptive traits to abiotic stress such as heat, drought, and salinity (Schneider et al., 2008; Kishii, 2019; Darkó et al., 2020). However, the utilization of wild genetic diversity in wheat breeding has been hampered by several factors, including hybridization barriers, hybrid abnormalities, and sterility of F1 hybrids (Kishii, 2019). These could be overcome using biotechnological approaches such as hybrid embryo rescue and development of amphiploids after chromosome doubling (Taira et al., 1991; Wulff and Moscou, 2014; Kishii, 2019). Reduced pairing between wheat and alien chromosomes during meiosis brings another level of difficulty, especially in the case of gene transfer from tertiary genepool species (Qi et al., 2007).
Transferred alien chromosome segments can only be utilized in wheat cultivars if they are integrated into the wheat genome as wheat-alien translocations. Among various strategies for producing interspecific chromosome rearrangements (Jiang et al., 1993), the induction of homeologous recombination after the modification of meiotic chromosome pairing is the most preferred (Qi et al., 2007). The main advantage is the genetic compensation of transferred alien chromatin for the missing wheat segment (Jiang et al., 1993). However, chromosome pairing in hexaploid wheat is under strict genetic control, ensuring only the formation of bivalents of homologous chromosomes, while homoeologous chromosomes almost never pair (Okamoto, 1957; Riley and Chapman, 1958). This diploid-like meiotic behavior is a significant barrier against wheat-alien homeologous recombination.
Genetic control of chromosome pairing in wheat consists of suppressing and promoting pairing homoeologous (Ph) genes (Sears, 1977). Out of them, the Ph1 locus located on the long arm of chromosome 5B (Riley et al., 1968) has the strongest suppressing effect on homoeologue chromosome pairing. Another locus (Ph2) was mapped to the short arm of chromosome 3D (Mello-Sampayo and Lorente, 1968; Mello-Sampayo, 1971) and another suppressor element with a smaller effect was identified on the homoeologous locus on 3A (Driscoll, 1972; Mello-Sampayo and Canas, 1973). Two additional elements with minor suppressing effects were located on chromosomes 4D and 2D (Driscoll, 1973; Ceoloni et al., 1986). Genes promoting pairing of homoeologous chromosomes were identified on group 2, 3, and 5 chromosomes (Naranjo and Benavente, 2015).
The absence of Ph1 in 5B nullisomics results in a high frequency of associations between homoeologous chromosomes (Riley and Kempanna, 1963). The use of 5B nullisomic plants is not attractive in introgression breeding programs because of reduced fertility, and an attractive alternative is the use of mutants lacking the Ph1 locus. A Chinese Spring mutant genotype (ph1b) carrying a ~70 Mb deletion at the Ph1 locus (Dunford et al., 1995) was developed by Sears (1976). Later, other deletion mutants in the Ph1 locus were developed and utilized (Roberts et al., 1999; Al-Kaff et al., 2008). Apart from the ph1b mutation, Sears produced a ph2a mutation, which is located on the short arm of chromosome 3D at the position of the Ph2 locus (Sears, 1982). The pattern of chromosome pairing at meiotic metaphase I in the ph2b mutant was similar to that of wild type, and no multivalent formation was detected, while the ph1b mutant exhibited extensive multivalent formation (Naranjo and Benavente, 2015). In wheat-alien hybrids, the frequency of homoeologous chromosome associations at metaphase I was low, intermediate, and high in the wild type, ph2b, and ph1b hybrid genotypes, respectively (Naranjo and Benavente, 2015). Due to the ability of the ph1b mutation to induce wheat-alien homoeologous recombination, the Chinese Spring ph1b mutant has been applied widely in transferring alien genes from the genera Aegilops (Riley, 1968; Liu et al., 2011; Niu et al., 2011; Li et al., 2020), Secale (Lukaszewski, 2000), Hordeum (Rey et al., 2015), Haynaldia (Zhao et al., 2013); Leymus (Edet et al., 2018) and Agropyron (Copete-Parada et al., 2021).
A serious disadvantage of the ph1b mutation in the Chinese Spring background is its poor agronomic performance, such as high plant height, low strength of the stem, low yield, and poor quality traits. Because of this, several backcrosses with advanced wheat lines adapted to the local agro-climatic conditions are necessary before the real agronomic effect of the transferred alien chromosome segment can be evaluated (Li et al., 2020). This process could be avoided by development of new deletions for the Ph1 region in advanced adapted wheat cultivars. This approach was successfully applied by Al-Kaff et al. (2008) who used γ−irradiation of seeds from hexaploid wheat cultivar Paragon and the produced mutants were used for introgression of wild genetic diversity into wheat (Grewal et al., 2018, 2020; Devi et al., 2019).
The transfer of the ph1b deletion on chromosome 5B into an advanced wheat cultivar adapted to local agro-climatic conditions means another choice to eliminate unfavorable traits of Chinese Spring from introgression breeding programs. The winter wheat genotype Martonvásári 9 kr1 (Mv9kr1) is well adapted to the central European conditions and has better agronomic performance than Chinese Spring (Molnár-Láng et al., 1996). Moreover, it carries the kr1 and kr2 crossability genes in recessive homozygous form (kr1kr1kr2kr2), making this genotype an ideal crossing partner in alien gene introgression programs (Molnár-Láng et al., 2014). The use of this genotype could facilitate the utilization of wheat-alien recombinants. The present work reports on marker-assisted transfer of ph1b deletion chromosome 5B from two Chinese Spring genotypes into the wheat Mv9kr1 line. The resulting M9kr1ph1b lines were morphologically characterized and the presence of a chromosome 5B deletion was confirmed by flow cytometric analysis. The lack of the Ph1 locus and its effect on meiotic chromosome pairing was verified at meiotic metaphase I in F1 hybrids of the M9kr1ph1b mutant genotype and a tertiary genepool species Ae. biuncialis Vis. (UbUbMbMb) using genomic in situ hybridization (GISH). Finally, the presence of wheat-Aegilops chromosome rearrangements was confirmed by GISH in amphiploids obtained by colchicine treatment of the wheat-Aegilops F1 hybrids.
Materials and Methods
Plant Material
Winter wheat (T. aestivum L.) line Mv9kr1 containing the recessive crossability gene kr1 (Molnár-Láng et al., 1996) was used as a female parent with two variants of the Chinese Spring ph1b deletion line developed by Sears (1977) as pollinators. One deletion line, designated CSph1b_K, was provided by Professor Bernd Friebe (Kansas State University, Manhattan, KS, United States). The second deletion line, designated CSph1b_N, was provided by Dr. Steve Reader (John Innes Centre, Norwich, United Kingdom).
Production of Mv9kr1 ph1b Lines
The crossing program for transferring the ph1b mutant chromosome 5B from Chinese Spring into Mv9kr1 is summarized in Figure 1. Five spikes (160 florets) were pollinated with CSph1b_K line and another five spikes (148 florets) were pollinated with CSph1b_N line, producing 108 and 128 F1 seeds, respectively (Supplementary Table S1).
The Mv9kr1 × CSph1b crosses, as well as the backcrosses with the Mv9kr1, were carried out in the field nursery of MGI ELKH, Martonvásár, Hungary in the 2011–2012 vegetative season. For the self-pollination of marker selected homozygous mutant lines, each of the vernalized (at 4°C for 6 weeks under 20 μmolm−2 s−1 light intensity) seedlings were planted into 2 L pots filled with a 3:2:1 mixture of garden soil, compost and sand and were grown up in randomized complete block design in glasshouse (Global Glasshouse Venlo). The average day/night temperature was increased from the initial 13/10°C to 23/18°C over 16 weeks, while air humidity was maintained between 60% and 80% by ventilating the glasshouse air. The plants were irrigated weekly to keep the volumetric soil moisture content (VSMC) values between 30% and 35%. The maximum light intensity was gradually increased from the initial 500–700 μmol m−2 s−1.
The presence of the ph1b deletion was confirmed by molecular markers Xpsr128 and Xpsr574 specific for the deletion region (Roberts et al., 1999) and used for marker-assisted selection of homozygous ph1b plants in F3, BC1F1, and BC2F1 generations as described later.
The BC3F1 seeds of Mv9kr1ph1b_K and Mv9kr1ph1b_N genotypes have been deposited to the Genebank of the Agricultural Institute, ATK (Martonvásár, Hungary) and are available upon request.
Evaluation of Morphological Parameters
Morphological parameters (Plant height, Length of the main spike, Spikes per plant, Spikelets per main spike, Seeds per main spike, Seeds per plant) of the wheat line Chinese Spring (CS), parental lines Mv9kr1 and CSph1b_K and CSph1b_N were compared with the BC2F1 plants of Mv9kr1ph1b_K and Mv9kr1ph1b_N genotypes. For the morphological evaluation, plants were grown in a glasshouse in the 2020–2021 season. The data representing the mean ± standard deviation of 5–10 plants per genotype for each morphological parameter were compared by Tukey’s post-hoc test at p < 0.05 where different letters (a–c) indicate significant differences between the genotypes.
Wheat × Aegilops biuncialis Crosses
BC1F2 Mv9kr1ph1b_K genotypes homozygous for the deletion (ph1b/ph1b) were crossed with Ae. biuncialis Vis. (2n = 4x = 28, UbUbMbMb) accessions MvGB380, MvGB382, MvGB1714, MvGB1723, MvGB1733, MvGB1745 MvGB1987 (maintained in the Martonvásár Cereal Genebank) to produce T. aestivum × Ae. biuncialis F1 hybrids (2n = 5x = 35, ABDUbMb). As a control for the presence of Ph1 locus, we also developed Mv9kr1 × Ae. biuncialis MvGB1733 F1 seeds. The Mv9kr1 × Ae. biuncialis MvGB1733 (Ph1) and Mv9kr1ph1b_K × Ae. biuncialis MvGB1733 (ph1b) F1 hybrids have been used to confirm the positive effect of transferred ph1b mutation on wheat-alien homoeologouos chromosome pairing in meiosis. The wheat (Mv9kr1ph1b_K) × Ae. biuncialis amphiploids (2n = 10x = 70, AABBDD UbUbMbMb) developed by colchicine treatment of the F1 hybrids were checked for the presence of wheat-Ae. biuncialis chromosome rearrangements by GISH.
Colchicine Treatment of Mv9kr1 × Aegilops biuncialis Hybrids (F1 Plants)
The F1 seeds were germinated, the seedlings were planted in Jiffy pots with peat pellets of 3 cm in diameter. The young seedlings were vernalized (4°C for 6 weeks under a light intensity of 12 μmol m−2 s−1 and a day/night period of 10/14 h). Vernalized plants were grown in 2 L pots filled with a 2:1:1 mixture of garden soil, humus, and sand in a phytotron chamber (PGR15, Conviron) until tillering under an initial day/night temperature of 15°C/10°C and 12/12 h light/dark photoperiod. Seedlings at 3–4 leaf stage (Zadoks skale Z24) were removed from the pots and placed into 0.04% (w/v) colchicine for 16 h incubated at 15°C. After the colchicine treatment, the roots were washed under running water for 2 h and the plants were transferred into pots and grown up. Both the day and night temperatures were increased by 2°C after tillering (day length 14 h), stem elongation (16 h illumination), flowering, and 2 weeks after fertilization (Tischner et al., 1997; Türkösi et al., 2018).
Marker-Assisted Selection of Homozygous ph1b Deletion
The Xpsr128 and Xpsr574 PCR based markers (Supplementary Table S4), which map within the ph1b deletion region (Roberts et al., 1999) were used to confirm the presence of chromosome 5B deletions in Mv9kr1ph1b_K and Mv9kr1ph1b_N lines. Because the markers are dominant, the absence of their PCR fragments indicates the presence of the ph1b deletion in homozygous form, while the presence of their PCR amplicons indicates the presence of Ph1 locus in heterozygous or homozygous form. The cDNA-based XAWJL3 PCR marker, which maps to chromosome 2A (Roberts et al., 1999) was used as a positive PCR control (Supplementary Table S4).
Total genomic DNA was extracted from fresh young leaves (plants in the 2-leaf stage) from the wheat line Chinese Spring (CS), parental lines Mv9kr1 and CSph1b_K and CSph1b_N as well as from their F3, BC1F1, BC2F1, and BC3F1 progenies using Quick Gene-Mini80 device (FujiFilm, Japan) together with QuickGene DNA tissue kit (FujiFilm, Japan) according to the manufacturer’s instructions. The PCR reactions were performed in a volume of 15 μl containing 20 ng of template DNA, 1.5 μl of 10× key reaction buffer (MgCl2 final concentration of 1.5 mM), 200 μM of each dNTP, 0.2 μM of forward and reverse primers, and 0.375 U of TEMPase Hot Start DNA Polymerase (VWR International, Belgium). The PCR reaction was carried out in Eppendorf Mastercycler (Eppendorf, Hamburg, Germany). The PCR conditions and primer sequences of the three molecular markers were described by Roberts et al. (1999). PCR products were analyzed using a Fragment Analyzer™ Automated CE System equipped with a 96-Capillary Array Cartridge (Advanced Analytical Technologies, Ames, United States). The separated PCR products of all genotypes were analyzed and visualized as digital capillary electrophoretic gel images, using the PROsize v2.0 software (Advanced Analytical Technologies, Ames, United States).
Bivariate Flow Karyotyping
Suspensions of mitotic metaphase chromosomes were prepared from the Kansas and Norwich variants of ph1b mutant genotypes (CSph1b_K, CSph1b_N, Mv9kr1ph1b_K, Mv9kr1ph1b_N) together with wild-type Chinese Spring and Mv9kr1 genotypes according to Vrána et al. (2016). Prior the flow cytometric analysis, GAA microsatellites were labeled by fluorescent in situ hybridization in suspension (FISHIS) using 5′-FITC-GAA7-FITC-3′ oligonucleotides (Sigma, Saint Louis, United States) according to Giorgi et al. (2013) and the chromosomes were stained by DAPI (4′,6-diamidino 2-phenylindole) at 2 μg/ml. Chromosome analysis and sorting were carried out using FACSAria II SORP flow cytometer and sorter (Becton Dickinson Immunocytometry Systems, San José, United States) as described by Molnár et al. (2016) and Said et al. (2019). Bivariate flow karyotypes FITC-A vs. DAPI-A fluorescence were acquired for each sample. Approximately 3,000 chromosomes were flow-sorted from each 5B chromosome population identified on a flow karyotype onto a microscope slide into a 3.0 μl drop of PRINS buffer supplemented with 2.5% sucrose (Kubaláková et al., 1997). The slides were air-dried and used for fluorescence in situ hybridization (FISH). Chromosome identification in the flow-sorted fractions was done after FISH with probes for pSc119.2, Afa family repeat, and 45S rDNA according to Molnár et al. (2016) and Huang et al. (2018). The chromosomes were classified following the karyotype described by Huang et al. (2018).
Meiotic Chromosome Pairing Analysis
Meiotic chromosome pairing of Mv9kr1 × Ae. biuncialis MvGB1733 F1 hybrids (2n = 5x = 35, ABDUbMb) in the presence (Mv9kr1) and absence (Mv9kr1ph1b_K) of the Ph1 locus was investigated in metaphase I (MI) of meiosis by means of GISH as described by Molnár and Molnár-Láng (2010). Briefly, anthers containing PMCs at metaphase I were fixed in 1:3 (v/v) acetic acid:ethanol and stored at −20°C for 2 weeks. Then anthers were squashed in 45% acetic acid and slides were stored at 4°C until GISH using M- and U-genomic probes as described below. Images were captured with an AxioImager M2 fluorescence microscope equipped with an AxioCam MRm CCD camera (Zeiss, Oberkochen, Germany) and with appropriate filter sets for DAPI, Alexa Fluor488 and Rhodamine. The images were assembled with AXIOVISION v4.8 software (Zeiss).
In the frame of chromosome pairing analysis at meioic metaphase I, the frequency of meiotic pairing configurations (univalent, bivalent, trivalent, and quadrivalent) and those of scored chromosome associations (w-w, w-Mb, w-Ub, Mb-Ub) were compared between the wheat × Ae. biuncialis MvGB1733 F1 hybrids in the presence (Mv9kr1 × Ae. biuncialis MvGB1733) and absence (Mv9kr1ph1b_K × Ae. biuncialis MvGB1733) of the Ph1 locus. The calculated frequencies represent the percentage of PMCs in which a given pairing configuration or chromosome association was observed. Differences in the mean frequencies of pairing configurations or chromosome associations between the two F1 hybrids were investigated by t-tests at the p = 0.01 significance level.
Genomic in situ Hybridization
Root tips of germinating seeds from the Mv9kr1ph1b_K × Ae. biuncialis amphiploids containing chromatin introgressed from Ae. biuncialis accessions MvGB380, MvGB1714, MvGB1733, MvGB1987, and MvGB1723 were used for chromosome preparation as described by Lukaszewski et al. (2004). Genomic in situ hybridization (GISH) experiment was done as described by Molnár et al. (2009). Briefly, total genomic DNAs of Ae. umbellulata (UU) and Ae. comosa (MM), the diploid progenitors of Ae. biuncialis, were labeled with biotin (biotin-16-dUTP; Roche) and digoxigenin (digoxigenin-11-dUTP; Roche) by random priming and used as U- and M-genome probes, respectively. Unlabeled wheat genomic DNA was used as blocking DNA at a ratio of 30:1. Digoxigenin and biotin signals were detected using anti-digoxigenin-rhodamine Fab fragments and Alexa Fluor488, respectively. The slides were evaluated using the Zeiss fluorescence microscope system as described for the meiotic chromosome pairing analysis.
Results
Development of the Mv9kr1 ph1b Lines
To transfer the ph1b deletion chromosome 5B from Chinese Spring into a winter wheat genotype adapted to the Central European agro-climatic conditions, we crossed the CSph1b_K and CSph1b_N genotypes with the wheat line Mv9kr1 (Figure 1). After two self-pollinations, the F3 plants were screened for the presence of the ph1b deletion in homozygous state by PCR markers Xpsr128 and Xpsr574 specific for the deleted region (Roberts et al., 1999). Homozygous ph1b plants were then selected for the morphological characteristics of the Mv9kr1 genotype (small plant height, long spikes), backcrossed with Mv9kr1 (BC1 generation), and then self-pollinated to fix the deletion in homozygous state (BC1F1 generation). BC1F1 plants were also filtered using PCR for the homozygous ph1b deletion (Figure 2) and selected for the morphological traits of the Mv9kr1 parent (plant height, spike architecture). The backcrossing and selection cycle was repeated to produce BC2F1 plants. The information on the number of seeds analyzed in F3, BC1F1, and BC2F1 generations by molecular markers and those of carrying the ph1b deletion in homozygous form is summarized in Supplementary Table S2. The frequency of ph1b/ph1b individuals varied from 12% to 37%. No correlation was found between the frequency of the homozygous deletion and the generation analyzed, nor between the frequency of the deletion and their origin (CSph1b_K or CSph1b_N).
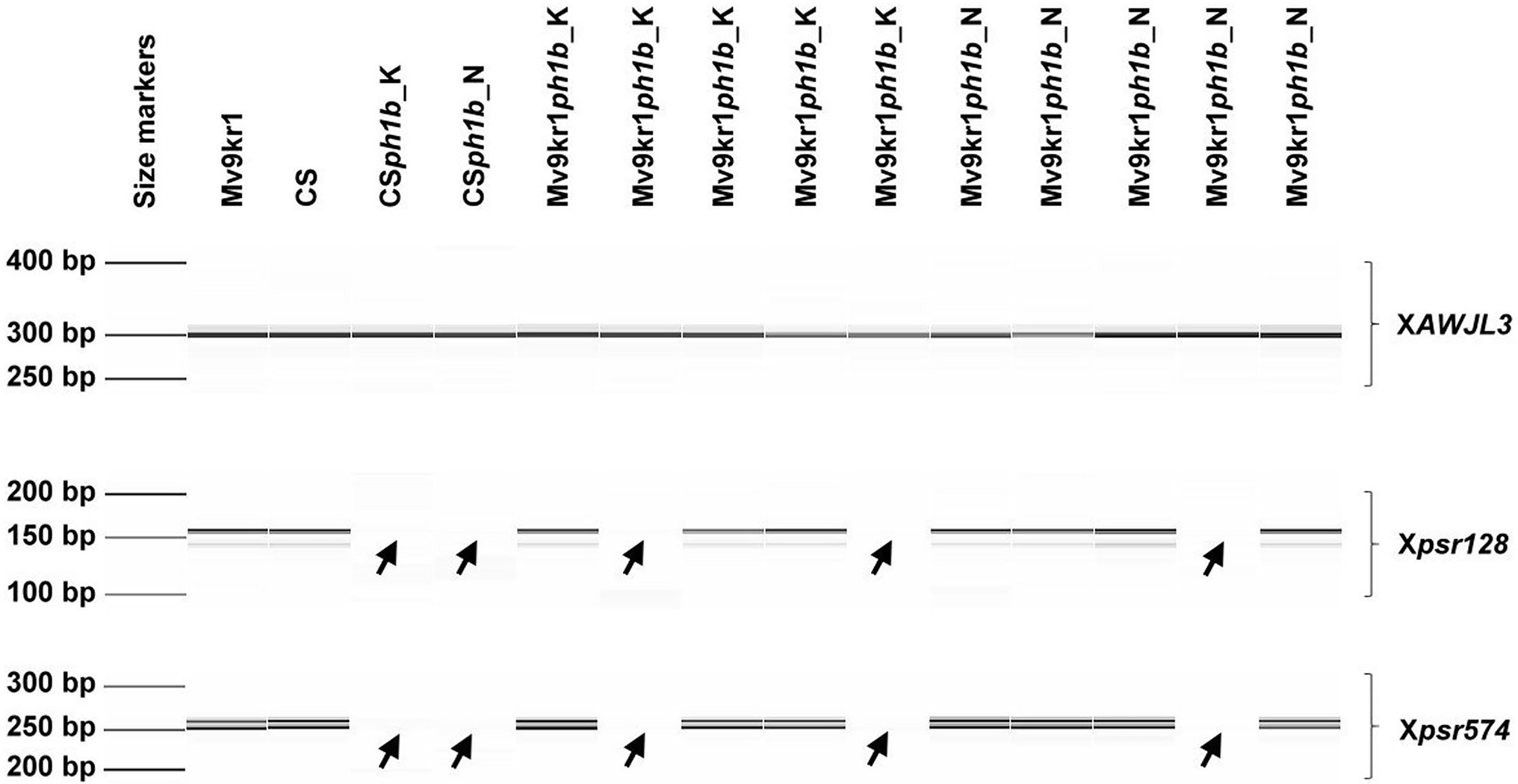
Figure 2. Marker-assisted selection of Mv9kr1ph1b mutant genotypes. On the capillary gel electrophoretogram, the presence of PCR amplicons of markers Xpsr128 and Xpsr574, which are specific for the deletion region of chromosome 5B indicate the presence of Ph1 locus in the wild type wheat genotypes CS and Mv9kr1 and some BC1F1 Mv9kr1ph1b_K and Mv9kr1ph1b_N genotypes, while the lack of these amplicons (highlighted by arrows) indicate the presence of ph1b deletion in homozygous form in the parental CSph1b_K and CSph1b_N genotypes and in several depicted BC1F1 genotypes. The marker XAWJL3, which mapped to chromosome 2A was used as positive control for the PCR assay.
Morphology of the newly developed BC2F1 Mv9kr1ph1b mutants (Mv9kr1ph1b_K, Mv9kr1ph1b_N) was compared with the wild type genotypes (Mv9kr1, Chinese Spring) and the parental Chinese Spring genotypes carrying the ph1b deletion (CSph1b_K, CSph1b_N; Table 1). Wild-type and mutant Mv9kr1 plants were shorter than the CS wheat lines (CS, CSph1b K, and CSph1b N). Apart from plant height, the mutant and wild type Mv9kr1 plants had longer spikes with more spikelets than Chinese Spring (Table 1), indicating that the morphological parameters of the plants carrying the ph1b deletion were improved after the transfer into the Mv9kr1 line.
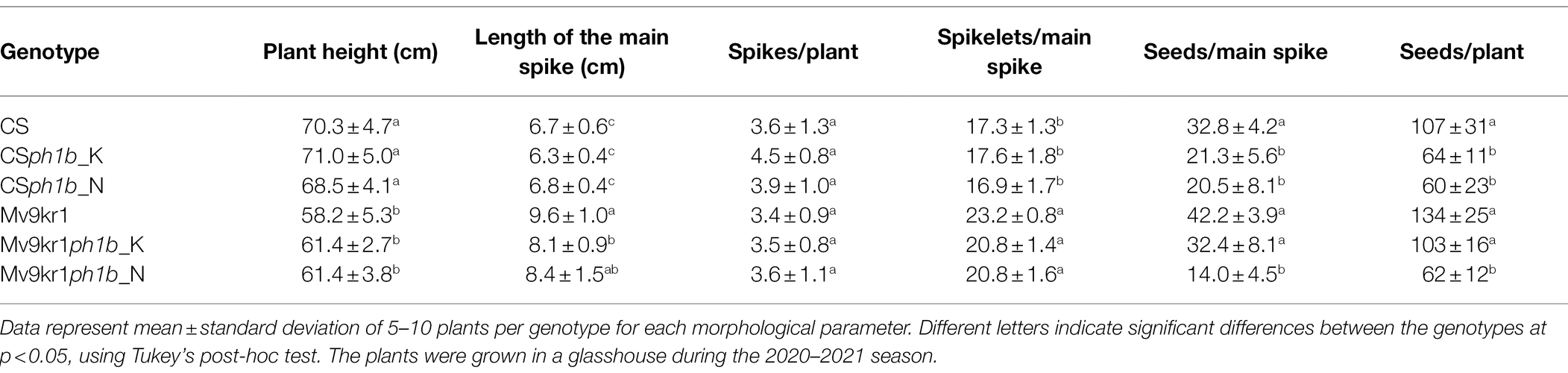
Table 1. Morphological parameters of the wheat cv. Chinese Spring (CS), wheat lines Mv9kr1 and CSph1b_K and N mutants and the BC2F1 Mv9kr1ph1b_K and Mv9kr1ph1b_N genotypes.
Interestingly, the genotype Mv9kr1ph1b_K exhibited significantly higher fertility as judged by the higher number of seeds per main spike and seeds per plant as compared to the two CSph1b mutant genotypes. In contrast, the Mv9kr1ph1b_N plants differed significantly from the wild type and the other ph1b mutant Mv9kr1 lines as they had lower seed set similar to the CSph1b wheat lines. The seed number data indicate that parallel with the plant and spike morphology, the fertility was also improved when the ph1b mutant 5B chromosome was transferred from the Kansas CSph1b genotype into Mv9kr1, while the fertility remained low when the mutant chromosome 5B was transferred from the CSph1b Norwich variant. Plant and spike morphology of the CS and Mv9kr1 genotypes carrying the Ph1 locus or ph1b deletion are shown in Figure 3.
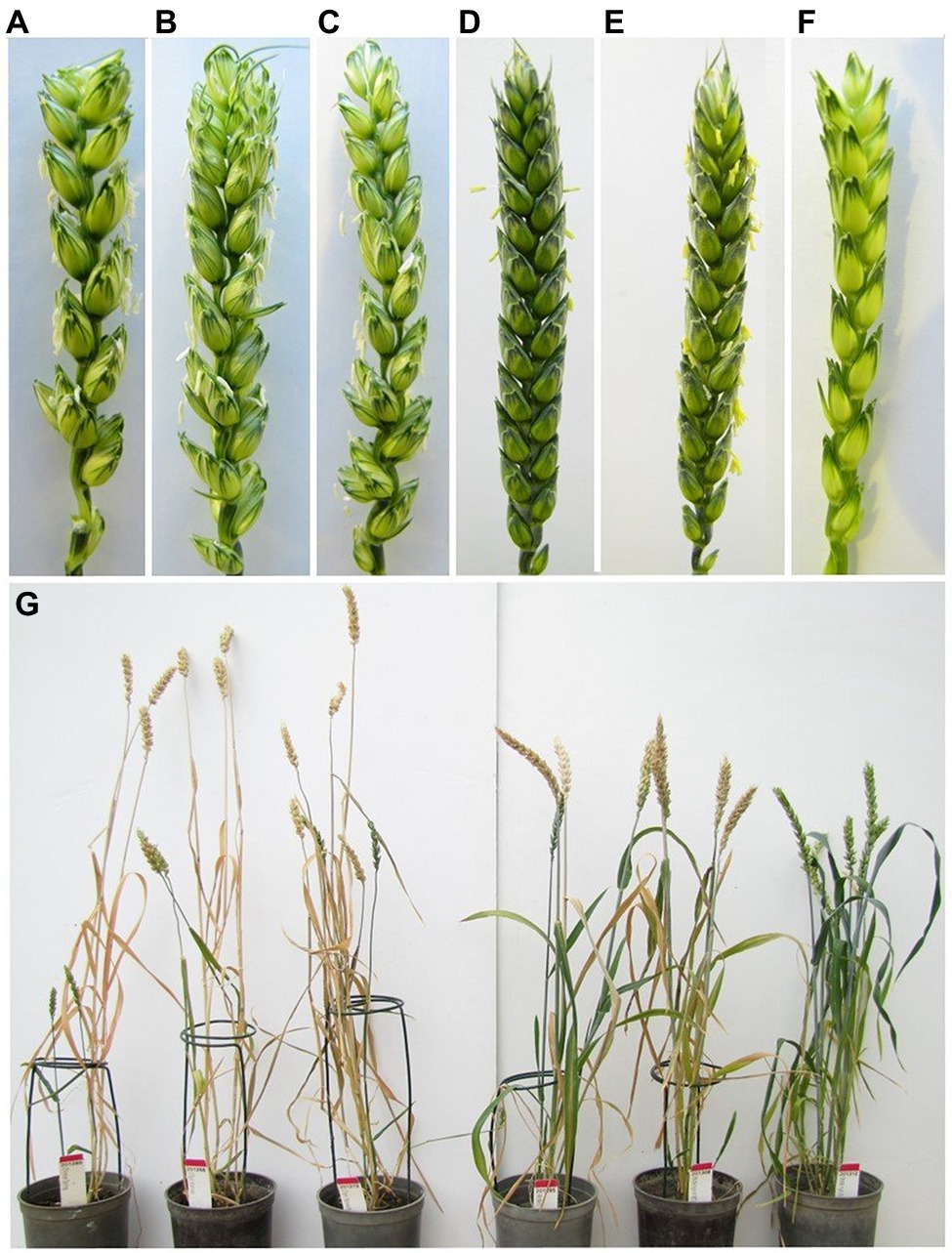
Figure 3. Spike morphology of the wild-type (Ph1) plants and of those carrying the ph1b deletion: (A) wild-type Chinese Spring; (B) Chinese Spring ph1b_K; (C) Chinese Spring ph1b_N; (D) wild-type Mv9kr1; (E) Mv9kr1 ph1b_K; (F) Mv9kr1 ph1b_N; (G) Whole plant morphology of the same genotypes (in the same order as it was shown for spike morphology).
Comparison of Chromosome 5B Size in the Wild Type and ph1b Mutant Lines
We used bivariate flow cytometric analysis of suspensions of isolated mitotic chromosomes to confirm the 70 Mb ph1b deletion on chromosome 5B in the Chinese Spring and Mv9kr1 lines. Simultaneous analysis of GAA-FITC and DAPI fluorescence permits discrimination of almost all 21 chromosomes of bread wheat, including chromosome 5B, and is sensitive enough to detect changes in chromosome DNA content (Doležel et al., 2021). To highlight changes in the position of chromosome 5B on a dot-plot (flow karyotype) GAA-FITC vs. DAPI, we used the position of chromosome 4A as a reference (Figure 4). Bivariate flow karyotyping of the wild type (Ph1/Ph1) Chinese Spring and Mv9kr1 wheat showed that the populations representing chromosome 5B were located close to other B-genome chromosomes (1B, 4B, 7B) which possess large clusters of GAA microsatellite (Figures 4A,B). The difference in DNA content between chromosomes 5B and 4A was small as reflected by small difference in relative DAPI fluorescence (Figures 4A,B). The identity of chromosome 5B population was confirmed by FISH on a chromosome fraction flow-sorted onto a microscope slide. Chromosome 5B was the most frequent in the sorted fraction (52.1% and 57.9% in CS and Mv9kr1, respectively), followed by 1B (39.3% and 39.4%), 4B and 7B (1%–5%; Supplementary Figure S1; Supplementary Table S3). The position of chromosome 5B population shifted to lower DAPI fluorescence intensity, resulting in a greater distance between chromosomes 5B and 4A on bivariate flow karyotypes of Chinese Spring ph1b mutant genotypes (CSph1b_K, CSph1b_N) relative to the wild-type plants. These changes reflected lower DNA content of the ph1b mutant 5B chromosome in these genotypes (Figures 4C,D).
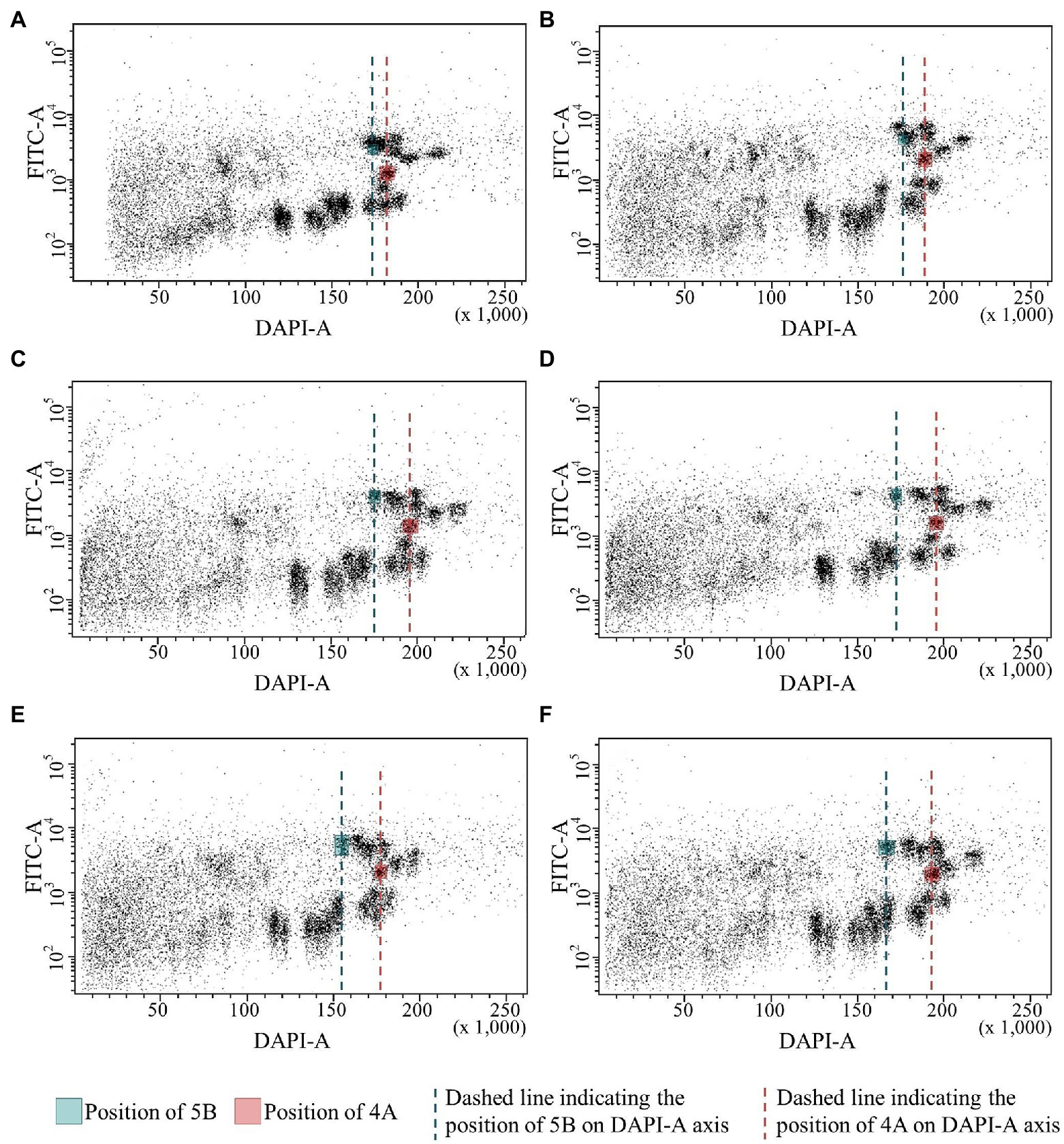
Figure 4. Bivariate flow cytometric analysis (flow karyotyping) of chromosomes isolated from wild-type and ph1b mutant wheat lines allows discrimination between the populations of wild-type and deleted chromosome 5B (green line) and chromosome 4A, which served as a reference (red line). Note that the populations of wild-type chromosome 5B in Chinese Spring (A) and Mv9kr1 (B) lines are closer to the position of 4A population on the DAPI axis. Chromosome 5B populations in CSph1b_K (C) and CSph1b_N (D) lines have lower DAPI fluorescence intensity and hence are more separated from chromosome 4A population. The same difference between the positions of 5B and 4A chromosomes was detected in Mv9kr1ph1b_K (E) and Mv9kr1ph1b_N (F) genotypes.
Interestingly, a bigger shift in the position of the 5B population on a flow karyotype was observed for the Norwich variant of CSph1b mutant as compared to CSph1b from Kansas. Due to this, the ph1b chromosome 5B could be discriminated better from the remaining B-genome chromosomes. This was reflected by higher purity in sorted chromosome fractions and the chromosome could be sorted in higher purity (97.2%) from the CSph1b_N genotypes than those from the Kansas variants (CSph1b_K: 89.1%; Supplementary Figure S1; Supplementary Table S3).
Difference in the DNA content between the Kansas and Norwich variants of ph1b deletion 5B chromosome were confirmed by flow karyotyping the Mv9kr1 mutants (Figures 4E,F). Similar to the CS mutants, a bigger shift in the position of chromosome 5B population on flow karyotype was observed for the Mv9kr1ph1b_N genotype. Consequently, the chromosome was sorted at higher purity (98.5%) as compared to Mv9kr1ph1b_K (85.8%).
Functional Verification of the Mv9kr1 ph1b Mutant
In order to verify that the promoting effect of ph1b deletion transferred to the Mv9kr1 genetic background on homoeologous chromosome pairing and recombination, we produced wheat × Ae. biuncialis F1 hybrids. Some of the F1 hybrids were used for chromosome pairing analysis at meiotic metaphase I, while other F1 hybrid plants were treated by colchicine to produce amphiploids. Because of higher fertility, only Mv9kr1ph1b_K genotype was used for the crosses with five accessions of Ae. biuncialis. The results of the Mv9kr1ph1b_K mutant × Ae. biuncialis crosses are summarized in Table 2.
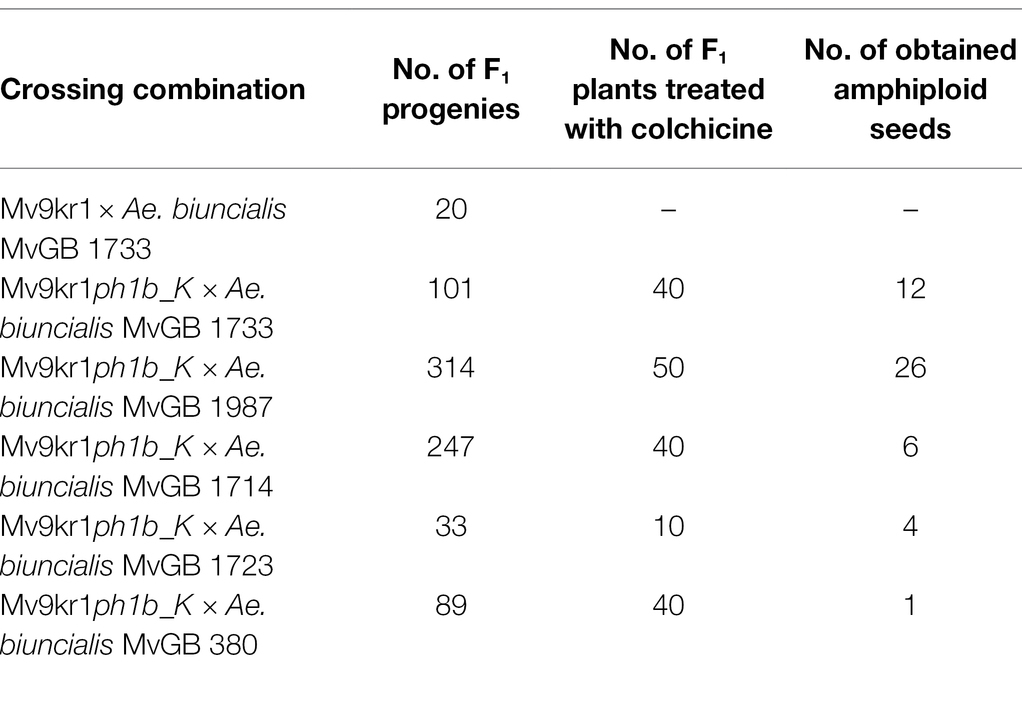
Table 2. Number of F1 progenies obtained from Mv9kr1ph1b_K × Aegilops biuncialis crosses and the amphiploid seeds obtained by colchicine treatment of the F1 hybrids.
We used GISH to investigate meiotic pairing behavior of the Mv9kr1 × Ae. biuncialis MvGB1733 F1 hybrids in the presence (Mv9kr1) or absence (Mv9kr1ph1b_K) of the Ph1 locus (Figure 5). The analysis of the pollen mother cells (PMCs) confirmed that the examined hybrids had 21 wheat and 7 Ub and 7 Mb Aegilops chromosomes, corresponding to genome composition of hexaploid wheat × Ae. biuncialis F1 hybrids (2n = 5x = 35, ABDUbMb). As expected, the level of MI chromosome paring was higher in Mv9kr1ph1b_K × Ae. biuncialis hybrids than in those obtained with wild-type Mv9kr1 genotype (Table 3). We observed significantly higher frequency of rod bivalents, trivalents, and multivalents in the presence of ph1b mutation and the increased frequency of chromosome pairing was manifested at the level of chromosome associations (Table 4). Four categories of chromosome associations were scored: associations between wheat chromosomes (w), interspecific associations between wheat and Aegilops chromosomes (Mb or Ub), and between Aegilops chromosomes. The results of the t-test (Table 5) showed that wheat chromosomes paired most frequently with each other, but there was no statistical difference between the wheat-wheat (w-w) chromosome associations and the associations between wheat and the Mb genome chromosomes of Aegilops (w-Mb; Tables 4, 5). The number of w-Ub and particularly Mb-Ub associations was significantly lower than w-w and w-Mb associations. The pairing frequency of Aegilops Mb and Ub genome chromosomes with those of wheat could thus be ranked as follows: w-w = w-Mb > w-Ub = Mb-Ub.
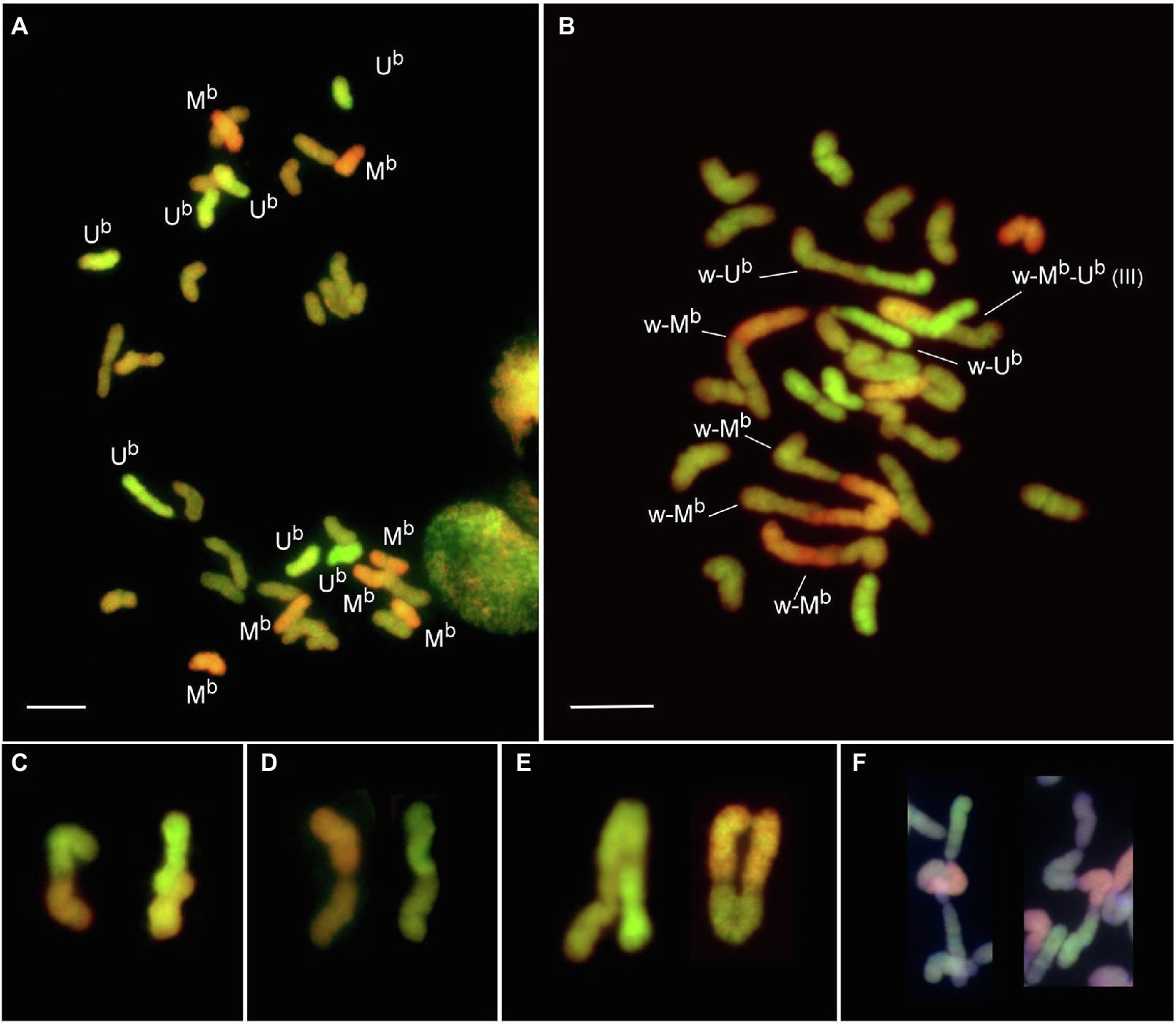
Figure 5. Genomic in situ hybridization of PMCs at MI of bread wheat × Aegilops biuncialis hybrids (2n = 5x = 35, ABDUbMb) in the presence (Mv9kr1) or absence (Mv9kr1ph1b_K) of the Ph1 locus. (A) MI cell of an Mv9kr1 × Ae. biuncialis MvGB1733 hybrid with functional Ph1 showing the whole chromosome complement, as seven Mb (red), seven Ub (green), and 21 unlabeled wheat (brown) univalents. (B) MI cell of an Mv9kr1ph1b_K × Ae. biuncialis MvGB1733 hybrid with seven rod bivalents; four of them involves wheat and Mb chromosomes (w-Mb), three involves wheat and Ub chromosomes (w-Ub) and one involves wheat chromosomes. A trivalent involving wheat, Mb and Ub chromosomes was also labeled (III). (C–F) Selected meiotic pairing configurations: Ub-Mb rod bivalents (C), wheat-Ub and wheat-Mb rod bivalents (D), trivalents involving wheat and Ub or Mb chromosomes (E), multivalents involving Ub, Mb, and wheat chromosomes (F) (in this figure, chromosomes were counterstained with DAPI “blue”). Scale bar 10 μm.
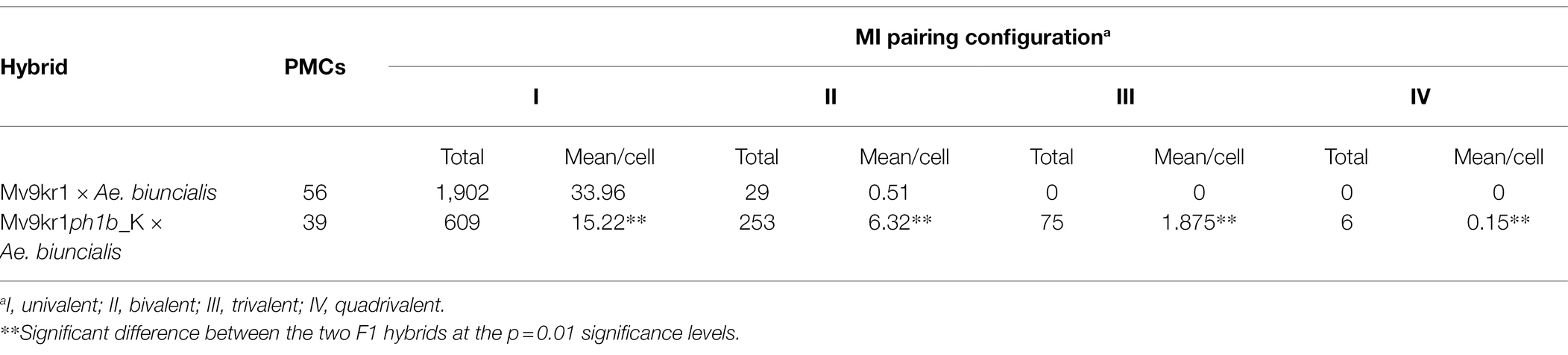
Table 3. Frequency of meiotic configurations at metaphase I in bread wheat (Mv9kr1) × Aegilops biuncialis MvGB1733 hybrids in the presence (Mv9kr1) and absence (Mv9kr1ph1b_K) of Ph1 locus.
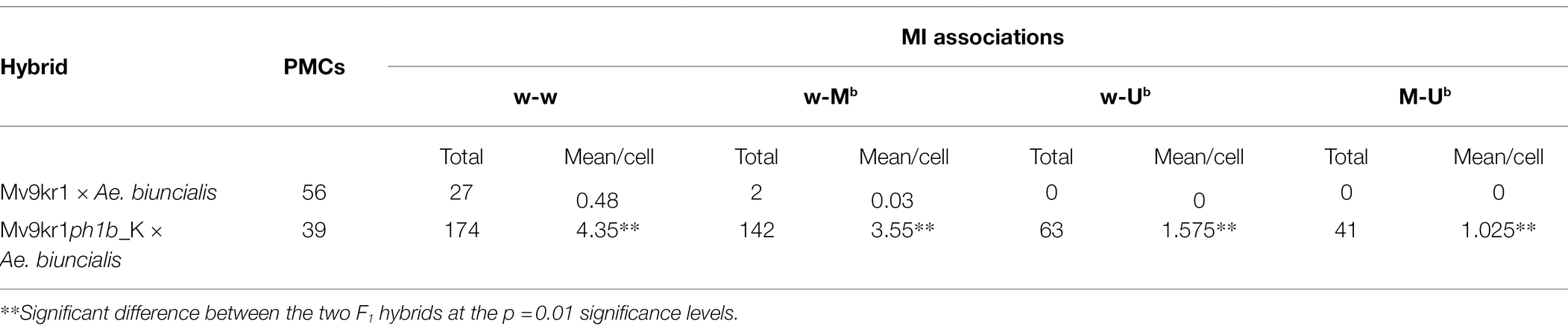
Table 4. Frequency of MI homoeologous associations in bread wheat (Mv9kr1) × Aegilops biuncialis MvGB1733 hybrids.
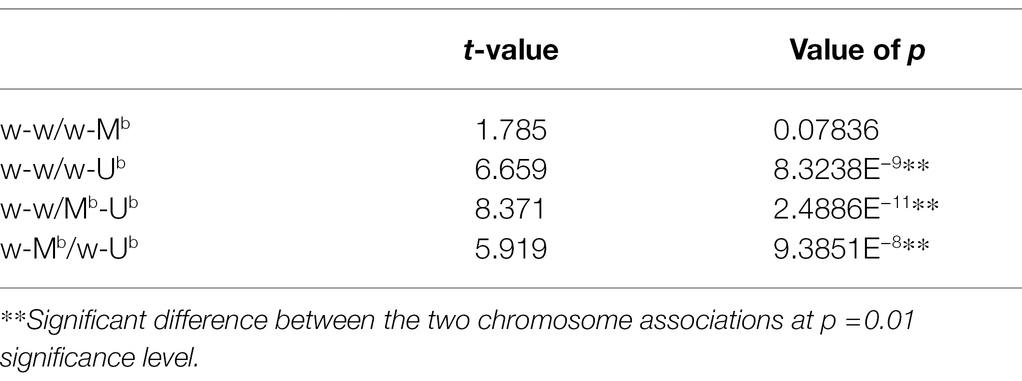
Table 5. Results of t-tests describing differences in the means of various associations involving wheat (w) and Aegilops (Mb, Ub) chromosomes in the Mv9kr1ph1b_K × Ae. biuncialis MvGB1733 F1 hybrid.
We also investigated mitotic chromosome spreads in 24 Mv9kr1ph1b_K × Ae. biuncialis amphiploids containing Aegilops genetic variation from five accessions (Table 2) by GISH in order to check if the increased level of wheat-Aegilops meiotic chromosome pairing resulted in interspecific translocations (Figure 6). The GISH analysis of the mitotic cells showed that chromosome number in most of the examined amphiploids were close to the maximum of 42 wheat and 14 Ub and 14 Mb Aegilops chromosomes, which corresponded to the genome composition of the hexaploid wheat × Ae. biuncialis amphiploids (2n = 10× = 70, AABBDDUbUbMbMb). Seven (29.16%) out of the 24 amphiploid genotypes investigated contained different types of translocations (Robertsonian, terminal and intercalary) between wheat and Aegilops chromosomes (Table 6).
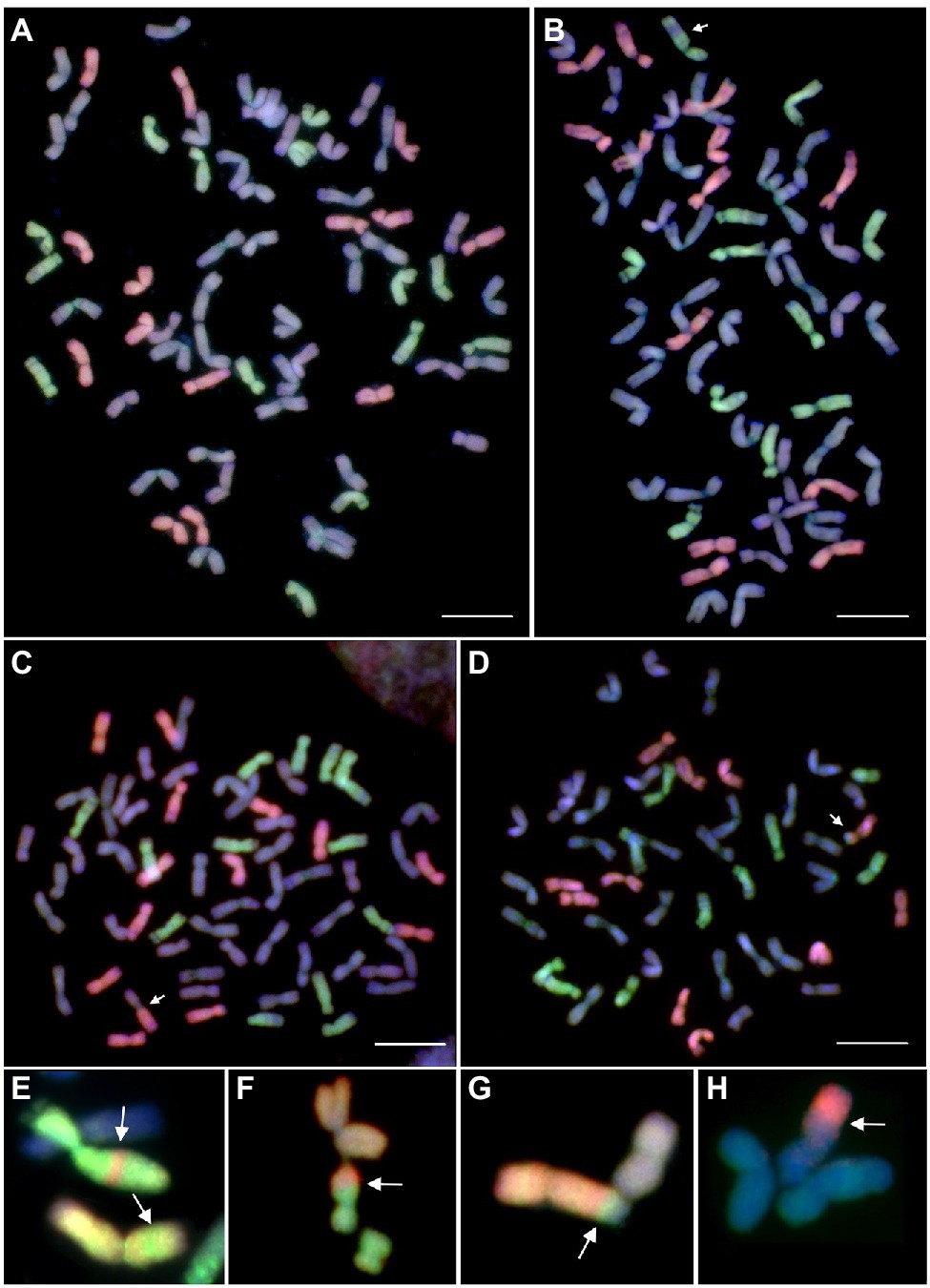
Figure 6. Mitotic metaphase plates of Mv9kr1ph1b_K × Aegilops biuncialis amphiploid after GISH with differentially labeled M- and U-genomic probes allowing the discrimination of Ae. biuncialis Mb—(red) and Ub—genome (green) chromosomes from those of unlabeled wheat chromosomes (blue). Partial amphiploid cell without intergeneric recombinant chromosomes (A), a partial cell of 201,226 amphiploid carrying an Ub-wheat intercalary translocation (B), a cell of genotype 201,246 carrying a wheat-Mb Robertsonian translocation (C), and a cell of genotype 201,216 carrying an Mb-wheat terminal translocation (D). Reciprocal intercalary (E) and terminal translocations (F-H) detected in additional amphiploids (201,225, 21,413, 201,245, and 21,407, respectively). The recombinant chromosomes are indicated by arrows. The chromosomes were counterstained with DAPI (blue). Scale bar = 10 μm.
Discussion
The Chinese Spring ph1b mutant produced by Sears (1977) has been used widely in homoeologous recombination-based chromosome engineering in wheat. However, due to poor agronomic performance of Chinese Spring, especially under Central European climatic conditions, the utilization of wheat-alien translocations requires several backcrosses with elite wheat genotypes adapted well to the local agro-climatic conditions. To overcome difficulties related to poor agronomic traits of Chinese Spring, wild type and newly developed ph1-mutant variants of hexaploid spring wheat cultivar “Paragon” (Al-Kaff et al., 2008), an elite line in United Kingdom environment, was chosen as key parent for a pre-breeding program in United Kingdom1 (Moore, 2015) to introgress chromatin of Thinopyrum bessarabicum, Triticum timopheevii, and Aegilops caudata into wheat (Grewal et al., 2018, 2020; Devi et al., 2019). Using an Axiom 35 K SNP array, the authors also demonstrated the effectivity of high resolution genotyping to detect alien introgressions in wheat (King et al., 2017).
Another approach is the transfer of original ph1b deletion from Chinese Spring into a wheat cultivar with better agronomic characters. Using this approach Li et al. (2020) transferred the ph1b deletion into a hexaploid spring wheat cultivar Shumai 126, indicating that morphological characters of the ph1b mutant lines could be improved by changing the wheat genetic background. Our work extended this this approach to a winter wheat genotype to develop a ph1b mutant genotype adapted to the Central European climate. We applied marker-assisted and phenotypic selection for morphological characters (low plant height, long spikes, and improved grain yield) to introduce the ph1b deletion into the winter wheat genotype Mv9kr1. Because of the good winter hardiness of Mv9kr1 (Molnár-Láng et al., 1996), the crossing programs with the Mv9kr1ph1b mutant plants can be performed under cost-effective field conditions. The wild type Mv9kr1 genotype has been used as crossing partner to introgress chromosome segments from barley (Szakács and Molnár-Láng, 2007, 2010), rye (Szakács et al., 2020), Thinopyrum (Kruppa and Molnár-Láng, 2016) and Aegilops (Schneider et al., 2005; Molnár et al., 2009; Farkas et al., 2014; Kruppa and Molnár-Láng, 2016) into wheat. The new wheat genotype will make it possible to use the ph1b mutant and wild type variants of the same (Mv9kr1) wheat genotype for interspecific hybridization programs to induce homoeologous recombination and later to stabilize the genome by elimination of the mutant 5B chromosome. The application of these genotypes will also avoid difficulties connected to multiple wheat genetic backgrounds during the agronomic evaluation of the introgression lines. The uniform wheat genetic background means further advantage when translocation chromosomes are used to map the introgressed alien chromosome segments for cloning genes with agronomical importance (Thind et al., 2017).
Morphological characterization showed that the plants containing the Norwich variant of ph1b mutant chromosome 5B in the Mv9kr1 background (Mv9kr1ph1b_N) had lower fertility than those of the Mv9kr1ph1b_K mutant, indicating that additional genetic modifications occurred in the Mv9kr1ph1b_N genotype. In line with this, flow cytometric chromosome analysis suggested that the chromosome 5B of the Norwich variant of Chinese Spring ph1b has lower DNA content as compared to the Kansas genotype. The size of the wild-type chromosome 5B in Chinese Spring was estimated as 870 Mbp (IWGSC International Wheat Genome Sequencing Consortium, 2014), and the population of this chromosome was located on a flow karyotype in a position typical for the chromosome 5B in hexaploid wheat with a wild-type karyotype (Doležel et al., 2021). Dunford et al. (1995) estimated the size of the 5B deletion in ph1b mutant as ~70 Mbp, and this region was further narrowed down to 59.3 Mbp with 1,187 genes by Martín et al. (2018). This ~6.8% reduction in the chromosome size resulted in the shift of the 5B population’s position toward a smaller DAPI fluorescence intensity (left of x-axis) on the flow karyotype. The fact that this shift was more pronounced in the Norwich variant ph1b mutant suggests that additional loss of 5B DNA content happened in this genotype. The smaller size of the Norwich variant of ph1b chromosome 5B was confirmed in the genotype Mv9kr1ph1b_N, which has a decreased fertility. These results are consistent with the previous observation that the inactivity of Ph1 locus may result in karyotype instability in the ph1b mutant wheat (Sánchez-Morán et al., 2001).
Due to homoeologous synapsis and crossovers, the ph1b mutant wheat exhibited an increased number of homoeologous metaphase I associations, most frequently between A and D genome chromosomes, which resulted in the formation of intergenomic chromosome rearrangements (Sánchez-Morán et al., 2001). These intergenomic exchanges have most likely been accumulated generation by generation resulting in decreased fertility of the ph1b mutants relative to the wild-type genotypes as was observed earlier (Sears, 1977) and by the present study. As a future research direction, it would be helpful to develop new wheat Ph1 mutant lines, with reduced homoeologous synapsis and crossover at meiosis, but which exhibit homoeologous crossovers in wheat-alien hybrids. The complex Ph1 locus affecting both synapsis and crossover, possesses CDK2-like and a ZIP4 paralogue (Tazip4-B2) genes. It has been proposed, that Ph1’s function on synapsis is related to CDK2-dependent chromatine phosphoryllation (Martín et al., 2017), while ZIP4 is involved in the effect of Ph1 on crossover formation (Martín et al., 2017; Rey et al., 2017). Recent improvements in CRISPR/Cas9 gene editing system allow the development of meiotically stable deletion mutants where the ZIP4 function is specifically knocked out to increase the crossover frequency without affecting the synapsis formation (Rey et al., 2018; Martín et al., 2021). Advances in wheat genetic transformation efficiencies makes it possible to achievable these goals (Hayta et al., 2021).
Flow karyotyping of the wild type and ph1b mutant wheat genotypes also indicated that a ~6.8% difference in the chromosome size allows discrimination of the deletion chromosome on a flow karyotype. This provides an opportunity for physical mapping of chromosomes based on the flow sorting of deletion chromosomes if deletion stocks for an entire chromosome are available (Svačina et al., 2019).
The increased frequency of wheat-alien chromosome associations and multivalent formation at meiotic metaphase I of wheat × alien F1 hybrids is a typical phenotype of the lines lacking Ph1 locus (Qi et al., 2007; Moore, 2014; Naranjo and Benavente, 2015). In the present study, we used Ae. biuncialis, which is considered a rich source of genes for alien introgression breeding of wheat (Schneider et al., 2005; Farkas et al., 2014), to produce wheat-alien F1 hybrids to validate the promoting effect on homoeologous chromosome pairing of the new Mv9kr1ph1b_K genotype.
Logojan and Molnár-Láng (2000) reported a low frequency of meiotic pairing between wheat and Ae. biuncialis chromosomes in wild-type Mv9kr1—Ae. biuncialis F1 hybrids (ABDUbMb). The present work showed that the ph1b mutation in Mv9kr1 genetic background significantly increases the frequency of homoeologous metaphase I associations as compared to the wild-type genotype. An increased level of wheat-Aegilops chromosome pairing was also observed by Cifuentes et al. (2006) who investigated meiotic chromosome pairing in durum wheat × Ae. geniculata interspecific hybrids (2n = 4x = 28, ABUgMg) in the presence or absence of Ph1 locus. Unfortunately, the genomic probes used by the authors did not allow discrimination between U and M genomes. In this study, we identified the Mb and Ub genome chromosomes by GISH and this allowed us to compare pairing affinity of constituent Aegilops genomes with the chromosomes of wheat. We found that the wheat chromosomes paired preferentially with the Mb genome chromosomes (3.55 w-Mb associations per cell) relative to Ub genome chromosomes (1.575 w-Ub associations per cell). Similar frequency of w-w and w-Mb homoeologous associations could be a consequence of high degree of homology between the Mb-genome chromosomes and the corresponding chromosomes of wheat. The predominant pairing affinity of wheat chromosomes with the Mb genome chromosomes relative to Ub chromosomes are consistent with the earlier meiotic pairing analysis of F1 hybrids obtained by the crossing Chinese Spring ph1b mutant and Mv9kr1-Ae. biuncialis disomic additions 2Mb, 3Mb, 7Mb, and 3Ub (Molnár and Molnár-Láng, 2010). Beside the fact that these monosomic wheat-Aegilops additions were heterozygous for the ph1b mutation and contained two copies of each wheat chromosomes, a tendency for increased level of wheat-Aegilops chromosome pairing were observed for 2Mb and 3Mb realtive to 3Ub chromosomes (Molnár and Molnár-Láng, 2010).
The chromosome pairing results are consistent with the previous investigations of the macro-level chromosome structure of wheat and M- and U-genomes of Aegilops by mapping conserved orthologous genes using single-gene FISH (Said et al., 2021) and COS markers (Molnár et al., 2013, 2016). These studies indicated close macrosyntenic relationships between the M-genome chromosomes with the corresponding chromosomes of wheat. On the other hand, the lower frequency of w-Ub metaphase I associations suggests larger structural differences between the Ub genome chromosomes and wheat. In fact, genetic mapping (Zhang et al., 1998; Edae et al., 2016, 2017), COS marker mapping on chromosome addition lines (Molnár et al., 2013) and single-gene FISH maps (Said et al., 2021) showed that U-genome of diploid Ae. umbellulata underwent multiple genome rearrangements during evolution resulting in synteny breaks in some chromosomes relative to wheat. While chromosomes 1 U, 2 U, 3 U, and 5 U remained more or less syntenic with wheat, chromosome 4 U contains regions homoeologous with wheat (w) chromosome groups 4, 5, and 6, 6 U homoeologous with w1, w2, w4, w6 and w7, while 7 U contains regions syntenic with w7 and w3 chromosomes. It is highly probable that these structural differences decreased the pairing affinity between Ub and wheat chromosomes in wheat- – Ae. biuncialis F1 hybrids.
Considering the differences in U- and M-genome chromosome structure, and their distinct affinity to pair with chromosomes of wheat, it may be concluded that ph-induced homeologous recombination is an effective strategy to transfer chromatin segments from all of the Mb chromosomes and from significant number of Ub chromosomes into wheat. In line with this, the feasibility of induced homoeologous chromosome pairing to transfer genes from U/M-genome Aegilops species into wheat has been demonstrated for Ae. umbellulata (Bansal et al., 2020) and Ae. geniculata (Kuraparthy et al., 2007).
The present study underlines the potential of the ph-based strategy when 29.16% of the Mv9kr1ph1b_K-Ae. biuncialis amphiploid plants contained intergenomic chromosome rearrangements. Wheat-Aegilops amphiploids thus obtained contain genetic variation from five Ae. biuncialis accessions originating from diverse geographical regions (Ivanizs et al., 2019) and presumably represent various allelic combinations for agronomically important genes. As these plants are homozygous for ph1b deletion, new wheat-Aegilops rearrangements can be produced in each new generation. These amphiploid genotypes may be used to generate new wheat-Ae. biuncialis chromosome translocations for wheat breeding through backcrossing with the wild type Mv9kr1 line. To conclude, the Mv9kr1ph1b mutant genotype developed in this work is an effective tool to facilitate alien gene introgression into hexaploid wheat.
Data Availability Statement
The original contributions presented in the study are included in the article/Supplementary Material, further inquiries can be directed to the corresponding author.
Author Contributions
IM: conceptualization, methodology, data curation, and project administration. IM, ET, LI, EG, AF, MS, PC, ÉS, KS-P, KK, and PK: investigation. IM and ÉS: resources. ET, IM, LI, and MS: visualization and writing—original draft preparation. IM, JD, and SG: writing—review. IM and JD: funding administration. All authors have read and approved the manuscript.
Funding
This work has been supported by the Hungarian National Research, Development and Innovation Office (K135057, K119387, TKP2021-NKTA-06, and 2019–2.1.11-TÉT-2019-00074), by ERDF project Plants as a Tool for Sustainable Global Development (no.CZ.02.1.01/0.0/0.0/16_019/0000827), and the Marie Curie Fellowship Grant award AEGILWHEAT (H2020-MSCA-IF-2016-746253).
Conflict of Interest
The authors declare that the research was conducted in the absence of any commercial or financial relationships that could be construed as a potential conflict of interest.
Publisher’s Note
All claims expressed in this article are solely those of the authors and do not necessarily represent those of their affiliated organizations, or those of the publisher, the editors and the reviewers. Any product that may be evaluated in this article, or claim that may be made by its manufacturer, is not guaranteed or endorsed by the publisher.
Acknowledgments
The authors would like to thank Fanni Tóth and Ildikó Lakner Könyvesné for the excellent technical assistance. Zdeňka Dubská, Romana Šperková, and Jitka Weiserová are also acknowledged for their assistance with chromosome sorting.
Supplementary Material
The Supplementary Material for this article can be found online at: https://www.frontiersin.org/articles/10.3389/fpls.2022.875676/full#supplementary-material
Footnotes
References
Al-Kaff, N., Knight, E., Bertin, I., Foote, T., Hart, N., Griffiths, S., et al. (2008). Detailed dissection of the chromosomal region containing the Ph1 locus in wheat Triticum aestivum: with deletion mutants and expression profiling. Ann. Bot. 101, 863–872. doi: 10.1093/aob/mcm252
Bansal, M., Adamski, N. M., Toor, P. I., Kaur, S., Molnár, I., Holušová, K., et al. (2020). Aegilops umbellulata introgression carrying leaf rust and stripe rust resistance genes Lr76 and Yr70 located to 9.47-Mb region on 5DS telomeric end through a combination of chromosome sorting and sequencing. Theor. Appl. Genet. 133, 903–915. doi: 10.1007/s00122-019-03514-x
Ceoloni, C., Strauss, I., and Feldman, M. (1986). Effect of different doses of group-2 chromosomes on homoeologous pairing in intergeneric wheat hybrids. Can. J. Genet. Cytol. 28, 240–246. doi: 10.1139/g86-033
Cheng, H., Liu, J., Wen, J., Nie, X., Xu, L., Chen, N., et al. (2019). Frequent intra- and inter-species introgression shapes the landscape of genetic variation in bread wheat. Genome Biol. 20:136. doi: 10.1186/s13059-019-1744-x
Cifuentes, M., Blein, M., and Benavente, E. (2006). A cytomolecular approach to assess the potential of gene transfer from a crop (Triticum turgidum L.) to a wild relative (Aegilops geniculata Roth.). Theor. Appl. Genet. 112, 657–664. doi: 10.1007/s00122-005-0168-z
Copete-Parada, A., Palomino, C., and Cabrera, A. (2021). Development and characterization of wheat-Agropyron cristatum introgression lines induced by gametocidal genes and wheat ph1b mutant. Agronomy 11:277. doi: 10.3390/agronomy11020277
Cox, T. S. (1997). Deepening the wheat gene pool. J. Crop. Prod. 1, 1–25. doi: 10.1300/J144v01n01_01
Darkó, É., Khalil, R., Dobi, Z., Kovács, V., Szalai, G., Janda, T., et al. (2020). Addition of Aegilops biuncialis chromosomes 2M or 3M improves the salt tolerance of wheat in different way. Sci. Rep. 10:22327. doi: 10.1038/s41598-020-79372-1
Devi, U., Grewal, S., Yang, C., Hubbart-Edwards, S., Scholefield, D., Ashling, S., et al. (2019). Development and characterisation of interspecific hybrid lines with genome-wide introgressions from Triticum timopheevii in a hexaploid wheat background. BMC Plant Biol. 19:183. doi: 10.1186/s12870-019-1785-z
Doležel, J., Lucretti, S., Molnár, I., Cápal, P., and Giorgi, D. (2021). Chromosome analysis and sorting. Cytometry A 99, 328–342. doi: 10.1002/cyto.a.24324
Driscoll, C. J. (1972). Genetic suppression of homoeologous chromosome pairing in hexaploid wheat. Can. J. Genet. Cytol. 14, 39–42. doi: 10.1139/g72-004
Driscoll, C. J. (1973). Minor genes affecting homoeologous pairing in hybrids between wheat and related genera. Genetics 74:S66.
Dunford, R. P., Kurata, N., Laurie, D. A., Money, T. A., Minobe, Y., and Moore, G. (1995). Conservation of fine-scale DNA marker order in the genomes of rice and the Triticeae. Nucleic Acids Res. 23, 2724–2728. doi: 10.1093/nar/23.14.2724
Dvořák, J., Luo, M. C., and Yang, Z. L. (1998). Restriction fragment length polymorphism and divergence in the genomic regions of high and low recombination in self-fertilizing and cross-fertilizing aegilops species. Genetics 148, 423–434. doi: 10.1093/genetics/148.1.423
Dvořák, J., Terlizzi, P., Zhang, H. B., and Resta, P. (1993). The evolution of polyploid wheats: identification of the A genome donor species. Genome 36, 21–31. doi: 10.1139/g93-004
Edae, E. A., Olivera, P. D., Jin, Y., Poland, J. A., and Rouse, M. N. (2016). Genotype-by-sequencing facilitates genetic mapping of a stem rust resistance locus in Aegilops umbellulata, a wild relative of cultivated wheat. BMC Genomics 17:1039. doi: 10.1186/s12864-016-3370-2
Edae, E. A., Olivera, P. D., Jin, Y., and Rouse, M. N. (2017). Genotyping-by-sequencing facilitates a high-density consensus linkage map for Aegilops umbellulata, a wild relative of cultivated wheat. G3. 7, 1551–1561. doi: 10.1534/g3.117.039966
Edet, O. U., Kim, J.-S., Okamoto, M., Hanada, K., Takeda, T., Kishii, M., et al. (2018). Efficient anchoring of alien chromosome segments introgressed into bread wheat by new Leymus racemosus genome-based markers. BMC Genet. 19:18. doi: 10.1186/s12863-018-0603-1
FAOSTAT (2018). Available at: http://www.fao.org/faostat/ (Accessed April 06, 2021).
FAOSTAT (2019). Available at: http://www.fao.org/faostat/ (Accessed April 06, 2021).
Farkas, A., Molnár, I., Dulai, S., Rapi, S., Oldal, V., Cseh, A., et al. (2014). Increased micronutrient content (Zn, Mn) in the 3M(b)(4B) wheat-Aegilops biuncialis substitution and 3M(b).4BS translocation identified by GISH and FISH. Genome 57, 61–67. doi: 10.1139/gen-2013-0204
Feldman, M., and Levy, A. A. (2012). Genome evolution due to allopolyploidization in wheat. Genetics 192, 763–774. doi: 10.1534/genetics.112.146316
Friebe, B., Jiang, J., Raupp, W. J., McIntosh, R. A., and Gill, B. S. (1996). Characterization of wheat-alien translocations conferring resistance to diseases and pests: current status. Euphytica 91, 59–87. doi: 10.1007/BF00035277
Giorgi, D., Farina, A., Grosso, V., Gennaro, A., Ceoloni, C., and Lucretti, S. (2013). FISHIS: fluorescence in situ hybridization in suspension and chromosome flow sorting made easy. PLoS One 8:e57994. doi: 10.1371/journal.pone.0057994
Grewal, S., Othmeni, M., Walker, J., Hubbart-Edwards, S., Yang, C., Scholefield, D., et al. (2020). Development of wheat-Aegilops caudata introgression lines and their characterization using genome-specific KASP markers. Front. Plant Sci. 11:606. doi: 10.3389/fpls.2020.00606
Grewal, S., Yang, C., Edwards, S. H., Scholefield, D., Ashling, S., Burridge, A. J., et al. (2018). Characterisation of Thinopyrum bessarabicum chromosomes through genome-wide introgressions into wheat. Theor. Appl. Genet. 131, 389–406. doi: 10.1007/s00122-017-3009-y
Hayta, S., Smedley, M. A., Clarke, M., Forner, M., and Harwood, W. A. (2021). An efficient Agrobacterium-mediated transformation protocol for hexaploid and tetraploid wheat. Curr. Protoc. 1:e58. doi: 10.1002/cpz1.58
Huang, X., Zhu, M., Zhuang, L., Zhang, S., Wang, J., Chen, X., et al. (2018). Structural chromosome rearrangements and polymorphisms identified in Chinese wheat cultivars by high-resolution multiplex oligonucleotide FISH. Theor. Appl. Genet. 131, 1967–1986. doi: 10.1007/s00122-018-3126-2
Ivanizs, L., Monostori, I., Farkas, A., Megyeri, M., Mikó, P., Türkösi, E., et al. (2019). Unlocking the genetic diversity and population structure of a wild gene source of wheat, Aegilops biuncialis Vis., and its relationship with the heading time. Front. Plant Sci. 10:1531. doi: 10.3389/fpls.2019.01531
IWGSC International Wheat Genome Sequencing Consortium (2014). A chromosome-based draft sequence of the hexaploid bread wheat (Triticum aestivum) genome. Science 345:1251788. doi: 10.1126/science.1251788
Jiang, J., Friebe, B., and Gill, B. S. (1993). Recent advances in alien gene transfer in wheat. Euphytica 73, 199–212. doi: 10.1007/BF00036700
King, J., Grewal, S., Yang, C. Y., Hubbart, S., Scholefield, D., Ashling, S., et al. (2017). A step change in the transfer of interspecific variation into wheat from Amblyopyrum muticum. Plant Biotechnol. J. 15, 217–226. doi: 10.1111/pbi.12606
Kishii, M. (2019). An update of recent use of Aegilops species in wheat breeding. Front. Plant Sci. 10:585. doi: 10.3389/fpls.2019.00585
Kruppa, K., and Molnár-Láng, M. (2016). Simultaneous visualization of different genomes (J, JSt and St) in a Thinopyrum intermedium × Thinopyrum ponticum synthetic hybrid (Poaceae) and in its parental species by multicolour genomic in situ hybridization (mcGISH). Comp. Cytogenet. 10, 283–293. doi: 10.3897/CompCytogen.v10i2.7305
Kubaláková, M., Macas, J., and Doležel, J. (1997). Mapping of repeated DNA sequences in plant chromosomes by PRINS and C-PRINS. Theor. Appl. Genet. 94, 758–763. doi: 10.1007/s001220050475
Kuraparthy, V., Chhuneja, P., Dhaliwal, H. S., Kaur, S., Bowden, R. L., and Gill, B. S. (2007). Characterization and mapping of cryptic alien introgression from Aegilops geniculata with new leaf rust and stripe rust resistance genes Lr57 and Yr40 in wheat. Theor. Appl. Genet. 114, 1379–1389. doi: 10.1007/s00122-007-0524-2
Li, Y., Li, Q., Lan, J., Tang, H., Qi, P., Ma, J., et al. (2020). Transfer of the ph1b gene of ‘Chinese spring’ into a common wheat cultivar with excellent traits. Cereal Res. Commun. 48, 283–291. doi: 10.1007/s42976-020-00048-7
Liu, W., Jin, Y., Rouse, M., Friebe, B., Gill, B., and Pumphrey, M. O. (2011). Development and characterization of wheat-Ae. Searsii Robertsonian translocations and a recombinant chromosome conferring resistance to stem rust. Theor. Appl. Genet. 122, 1537–1545. doi: 10.1007/s00122-011-1553-4
Logojan, A. A., and Molnár-Láng, M. (2000). Production of Triticum aestivum-Aegilops biuncialis chromosome additions. Cereal Res. Commun. 28, 221–228. doi: 10.1007/BF03543597
Lubbers, E. L., Gill, K. S., Cox, T. S., and Gill, B. S. (1991). Variation of molecular markers among geographically diverse accessions of Triticum tauschii. Genome 34, 354–361. doi: 10.1139/g91-057
Lukaszewski, A. J. (2000). Manipulation of the 1RS.1BL translocation in wheat by induced homoeologous recombination. Crop. Sci. 40, 216–225. doi: 10.2135/cropsci2000.401216x
Lukaszewski, A. J., Rybka, K., Korzun, V., Malyshev, S. V., Lapinski, B., and Whitkus, R. (2004). Genetic and physical mapping of homoeologous recombination points involving wheat chromosome 2B and rye chromosome 2R. Genome 47, 36–45. doi: 10.1139/g03-089
Maestra, B., and Naranjo, T. (2000). “Genome evolution in triticeae,” in Chromosomes Today. eds. E. Olmo and C. A. Redi (Basel: Birkhäuser Basel), 155–167.
Martín, A. C., Alabdullah, A. K., and Moore, G. (2021). A separation-of-function ZIP4 wheat mutant allows crossover between related chromosomes and is meiotically stable. Sci. Rep. 11:21811. doi: 10.1038/s41598-021-01379-z
Martín, A. C., Borrill, P., Higgins, J., Alabdullah, A., Ramírez-González, R. H., Swarbreck, D., et al. (2018). Genome-wide transcription during early wheat meiosis is independent of synapsis, ploidy level, and the Ph1 locus. Front. Plant Sci. 9:1791. doi: 10.3389/fpls.2018.01791
Martín, A. C., Rey, M. D., Shaw, P., and Moore, G. (2017). Dual effect of the wheat Ph1 locus on chromosome synapsis and crossover. Chromosoma 126, 669–680. doi: 10.1007/s00412-017-0630-0
Mello-Sampayo, T. (1971). Genetic regulation of meiotic chromosome pairing by chromosome 3D of Triticum aestivum. Nat. New Biol. 230, 22–23. doi: 10.1038/newbio230022a0
Mello-Sampayo, T., and Canas, A. P. (1973). “Suppressors of meiotic chromosome pairing in common wheat.” in Proceedings of 4th International Wheat Genetics Symposium. eds. E. R. Sears and L. M. S. Sears (Columbia, Missouri: University of Missouri), 709–713.
Mello-Sampayo, T., and Lorente, R. (1968). The role of chromosome 3D in the regulation of meiotic pairing in hexaploid wheat. EWAC Newsl. 2, 16–24.
Molnár, I., Benavente, E., and Molnár-Láng, M. (2009). Detection of intergenomic chromosome rearrangements in irradiated Triticum aestivum-Aegilops biuncialis amphiploids by multicolour genomic in situ hybridization. Genome 52, 156–165. doi: 10.1139/g08-114
Molnár, I., and Molnár-Láng, M. (2010). GISH reveals different levels of meiotic pairing with wheat for individual Aegilops biuncialis chromosomes. Biol. Plant. 54, 259–264. doi: 10.1007/s10535-010-0045-8
Molnár, I., Šimková, H., Leverington-Waite, M., Goram, R., Cseh, A., Vrána, J., et al. (2013). Syntenic relationships between the U and M genomes of Aegilops, wheat and the model species Brachypodium and rice as revealed by COS markers. PLoS One 8:e70844. doi: 10.1371/journal.pone.0070844
Molnár, I., Vrána, J., Burešová, V., Cápal, P., Farkas, A., Darkó, É., et al. (2016). Dissecting the U, M, S and C genomes of wild relatives of bread wheat (Aegilops spp.) into chromosomes and exploring their synteny with wheat. Plant J. 88, 452–467. doi: 10.1111/tpj.13266
Molnár-Láng, M., Ceoloni, C., and Doležel, J., eds (2015). Alien Introgression in wheat: Cytogenetics, Molecular Biology, and Genomics/Márta Molnár-Láng, Carla Ceoloni, Jaroslav Doležel, Editors. Cham: Springer Science + Business Media.
Molnár-Láng, M., Linc, G., and Sutka, J. (1996). Transfer of the recessive crossability allele kr1 from Chinese spring into the winter wheat variety Martonvásári 9. Euphytica 90, 301–305. doi: 10.1007/BF00027480
Molnár-Láng, M., Molnár, I., Szakács, É., Linc, G., and Bedö, Z. (2014). “Production and molecular cytogenetic identification of wheat-alien hybrids and introgression lines,” in Genomics of Plant Genetic Resources. eds. R. Tuberosa, A. Graner, and E. A. Frison (New York: Springer), 255–283.
Moore, G. (2014). The control of recombination in wheat by Ph1 and its use in breeding. Methods Mol. Biol. 1145, 143–153. doi: 10.1007/978-1-4939-0446-4_12
Moore, G. (2015). Strategic pre-breeding for wheat improvement. Nat. Plants 1:15018. doi: 10.1038/nplants.2015.18
Naranjo, T., and Benavente, E. (2015). “The mode and regulation of chromosome pairing in wheat–alien hybrids (Ph genes, an updated view),” in Alien introgression in wheat: Cytogenetics, molecular biology, and genomics/Márta Molnár-Láng, Carla Ceoloni, Jaroslav Doležel. eds. M. Molnár-Láng, C. Ceoloni, and J. Doležel (Cham: Springer Science + Business Media), 133–162.
Niu, Z., Klindworth, D. L., Friesen, T. L., Chao, S., Jin, Y., Cai, X., et al. (2011). Targeted introgression of a wheat stem rust resistance gene by DNA marker-assisted chromosome engineering. Genetics 187, 1011–1021. doi: 10.1534/genetics.110.123588
Okamoto, M. (1962). Identification of the chromosomes of common wheat belonging to the A and B genomes. Can. J. Genet. Cytol. 4, 31–37. doi: 10.1139/g62-005
Qi, L., Friebe, B., Zhang, P., and Gill, B. S. (2007). Homoeologous recombination, chromosome engineering and crop improvement. Chromosome Res. 15, 3–19. doi: 10.1007/s10577-006-1108-8
Rey, M.-D., Calderón, M. C., and Prieto, P. (2015). The use of the ph1b mutant to induce recombination between the chromosomes of wheat and barley. Front. Plant Sci. 6:160. doi: 10.3389/fpls.2015.00160
Rey, M.-D., Martín, A. C., Higgins, J., Swarbreck, D., Uauy, C., Shaw, P., et al. (2017). Exploiting the ZIP4 homologue within the wheat Ph1 locus has identified two lines exhibiting homoeologous crossover in wheat-wild relative hybrids. Mol Breeding 37:95. doi: 10.1007/s11032-017-0700-2
Rey, M.-D., Martín, A. C., Smedley, M., Hayta, S., Harwood, W., Shaw, P., et al. (2018). Magnesium increases homoeologous crossover frequency during meiosis in ZIP4 (Ph1 gene) mutant wheat-wild relative hybrids. Front. Plant Sci. 9:509. doi: 10.3389/fpls.2018.00509
Riley, R. (1968). “The basic and applied genetics of chromosome pairing.” in Proceedings of 3rd IInternational Wheat Genetics Symposium. eds. K. W. Finlay and K. W. Shepherd (Canberra: Australia Academy of Science), 185–195.
Riley, R., and Chapman, V. (1958). Genetic control of the cytologically diploid behaviour of hexaploid wheat. Nature 182, 713–715. doi: 10.1038/182713a0
Riley, R., Chapman, V., and Johnson, R. (1968). Introduction of yellow rust resistance of Aegilops comosa into wheat by genetically induced homoeologous recombination. Nature 217, 383–384. doi: 10.1038/217383a0
Riley, R., and Kempanna, C. (1963). The homoeologous nature of the non-homologous meiotic pairing in Triticum aestivum deficient for chromosome V (5B). Heredity 18, 287–306. doi: 10.1038/hdy.1963.31
Roberts, M. A., Reader, S. M., Dalgliesh, C., Miller, T. E., Foote, T. N., Fish, L. J., et al. (1999). Induction and characterization of Ph1 wheat mutants. Genetics 153, 1909–1918. doi: 10.1093/genetics/153.4.1909
Said, M., Holušová, K., Farkas, A., Ivanizs, L., Gaál, E., Cápal, P., et al. (2021). Development of DNA markers from physically mapped loci in Aegilops comosa and Aegilops umbellulata using single-gene FISH and chromosome sequences. Front. Plant Sci. 12:689031. doi: 10.3389/fpls.2021.689031
Said, M., Kubaláková, M., Karafiátová, M., Molnár, I., Doležel, J., and Vrána, J. (2019). Dissecting the complex genome of crested wheatgrass by chromosome flow sorting. Plant Genome 12:180096. doi: 10.3835/plantgenome2018.12.0096
Sánchez-Morán, E., Benavente, E., and Orellana, J. (2001). Analysis of karyotypic stability of homoeologous-pairing (ph) mutants in allopolyploid wheats. Chromosoma 110, 371–377. doi: 10.1007/s004120100156
Schneider, A., Linc, G., Molnár, I., and Molnár-Láng, M. (2005). Molecular cytogenetic characterization of Aegilops biuncialis and its use for the identification of 5 derived wheat-Aegilops biuncialis disomic addition lines. Genome 48, 1070–1082. doi: 10.1139/g05-062
Schneider, A., Molnár, I., and Molnár-Láng, M. (2008). Utilisation of Aegilops (goatgrass) species to widen the genetic diversity of cultivated wheat. Euphytica 163, 1–19. doi: 10.1007/s10681-007-9624-y
Sears, E. R. (1976). Genetic control of chromosome pairing in wheat. Annu. Rev. Genet. 10, 31–51. doi: 10.1146/annurev.ge.10.120176.000335
Sears, E. R. (1977). An induced mutant with homoeologous pairing in common wheat. Can. J. Genet. Cytol. 19, 585–593. doi: 10.1139/g77-063
Sears, E. R. (1982). A wheat mutation conditioning an intermediate level of homoeologous chromosome pairing. Can. J. Genet. Cytol. 24, 715–719. doi: 10.1139/g82-076
Svačina, R., Karafiátová, M., Malurová, M., Serra, H., Vítek, D., Endo, T. R., et al. (2019). Development of deletion lines for chromosome 3D of bread wheat. Front. Plant Sci. 10:1756. doi: 10.3389/fpls.2019.01756
Szakács, E., and Molnár-Láng, M. (2007). Development and molecular cytogenetic identification of new winter wheat-winter barley (“Martonvásári 9 kr1”-“Igri”) disomic addition lines. Genome 50, 43–50. doi: 10.1139/g06-134
Szakács, E., and Molnár-Láng, M. (2010). Identification of new winter wheat-winter barley addition lines (6HS and 7H) using fluorescence in situ hybridization and the stability of the whole “Martonvásári 9 kr1” – “Igri” addition set. Genome 53, 35–44. doi: 10.1139/g09-085
Szakács, É., Szőke-Pázsi, K., Kalapos, B., Schneider, A., Ivanizs, L., Rakszegi, M., et al. (2020). 1RS arm of Secale cereanum “Kriszta” confers resistance to stripe rust, improved yield components and high arabinoxylan content in wheat. Sci. Rep. 10:1792. doi: 10.1038/s41598-020-58419-3
Taira, T., Shao, Z. Z., Hamawaki, H., and Larter, E. N. (1991). The effect of colchicine as a chromosome doubling agent for wheat-rye hybrids as influenced by ph, method of application, and post-treatment environment. Plant Breed. 106, 329–333. doi: 10.1111/j.1439-0523.1991.tb00518.x
Thind, A. K., Wicker, T., Šimková, H., Fossati, D., Moullet, O., Brabant, C., et al. (2017). Rapid cloning of genes in hexaploid wheat using cultivar-specific long-range chromosome assembly. Nat. Biotechnol. 35, 793–796. doi: 10.1038/nbt.3877
Tischner, T., Koszegi, B., and Veisz, O. (1997). Climatic programmes used in the Martonvásár phytotron most frequently in recent years. Acta Agron. Hung. 45, 85–104.
Türkösi, E., Darko, É., Rakszegi, M., Molnár, I., Molnár-Láng, M., and Cseh, A. (2018). Development of a new 7BS.7HL winter wheat-winter barley Robertsonian translocation line conferring increased salt tolerance and (1,3;1,4)-β-D-glucan content. PLoS One 13:e0206248. doi: 10.1371/journal.pone.0206248
Vrána, J., Cápal, P., Šimková, H., Karafiátová, M., Čížková, J., and Doležel, J. (2016). Flow analysis and sorting of plant chromosomes. Curr. Protoc. Cytom. 78, 5.3.1–5.3.43. doi: 10.1002/cpcy.9
Wulff, B. B. H., and Moscou, M. J. (2014). Strategies for transferring resistance into wheat: from wide crosses to GM cassettes. Front. Plant Sci. 5:692. doi: 10.3389/fpls.2014.00692
Xie, Q., Li, N., Yang, Y., Lv, Y., Yao, H., Wei, R., et al. (2018). Pleiotropic effects of the wheat domestication gene Q on yield and grain morphology. Planta 247, 1089–1098. doi: 10.1007/s00425-018-2847-4
Zhang, H., Jia, J., Gale, M. D., and Devos, K. M. (1998). Relationships between the chromosomes of Aegilops umbellulata and wheat. Theor. Appl. Genet. 96, 69–75. doi: 10.1007/s001220050710
Zhao, R., Wang, H., Xiao, J., Bie, T., Cheng, S., Jia, Q., et al. (2013). Induction of 4VS chromosome recombinants using the CS ph1b mutant and mapping of the wheat yellow mosaic virus resistance gene from Haynaldia villosa. TAG. Theoretical and applied genetics. Theoretische und angewandte Genetik 126, 2921–2930. doi: 10.1007/s00122-013-2181-y
Keywords: bread wheat, Aegilops biuncialis, ph1b mutant, meiotic chromosome pairing, in situ hybridization, chromosome flow sorting, homoeologous recombination
Citation: Türkösi E, Ivanizs L, Farkas A, Gaál E, Kruppa K, Kovács P, Szakács É, Szőke-Pázsi K, Said M, Cápal P, Griffiths S, Doležel J and Molnár I (2022) Transfer of the ph1b Deletion Chromosome 5B From Chinese Spring Wheat Into a Winter Wheat Line and Induction of Chromosome Rearrangements in Wheat-Aegilops biuncialis Hybrids. Front. Plant Sci. 13:875676. doi: 10.3389/fpls.2022.875676
Edited by:
Pietro Gramazio, University of Tsukuba, JapanReviewed by:
Claudia E. Osorio, Instituto de Investigaciones Agropecuarias, ChileMatthew Rouse, Agricultural Research Service (USDA), United States
Copyright © 2022 Türkösi, Ivanizs, Farkas, Gaál, Kruppa, Kovács, Szakács, Szőke-Pázsi, Said, Cápal, Griffiths, Doležel and Molnár. This is an open-access article distributed under the terms of the Creative Commons Attribution License (CC BY). The use, distribution or reproduction in other forums is permitted, provided the original author(s) and the copyright owner(s) are credited and that the original publication in this journal is cited, in accordance with accepted academic practice. No use, distribution or reproduction is permitted which does not comply with these terms.
*Correspondence: András Farkas, YW5kcmFzLmZhcmthc0BhdGsuaHU=
†ORCID: Edina Türkösi, orcid.org/0000-0002-3689-8516
‡These authors have contributed equally to this work and share first authorship