- Agriculture and Agri-Food Canada, Morden Research and Development Centre, Morden, MB, Canada
The wheat multi-pest resistance genes Lr67 and Lr34 are similar in that they both condition resistance to many diseases, in a non-race-specific manner, and code for cellular transporters. Lr34 plays a critical role in breeding wheat for disease resistance in large part because it interacts with other resistance genes to result in effective and durable resistance. To determine if Lr67 interacts with other resistance genes in a similar manner as Lr34 six different doubled haploid populations were developed which segregated for either Lr67 or Lr34 along with a second resistance gene, either Lr13, Lr16, or Lr32. The presence or absence of each of these genes in the progeny lines was determined by molecular marker analysis. These six populations were tested for leaf rust field resistance in the same environments to compare the effects of Lr34 and Lr67 alone, and in combination with Lr13, Lr16 or Lr32. Lr67 and Lr34 significantly reduced the levels of rust severity, Lr34 showed a significant interaction with Lr13 but Lr67 did not. Both genes interacted with Lr16, and Lr67 had a significant interaction with Lr32. This analysis demonstrates the similar effect of Lr67, as seen with Lr34, on the interaction with other resistance genes to give a better level of resistance than with single resistance genes. While Lr67 is not widely deployed in agriculture, it could play an important role in disease resistance in future wheat cultivars.
Introduction
Wheat leaf rust is a very common and destructive disease of wheat internationally (Huerta-Espino et al., 2011) and in Canada (McCallum et al., 2016). Genetic resistance has proven effective in controlling this disease, however the Puccinia triticina Eriks. pathogen population has evolved virulence for most of the race-specific resistance genes widely deployed in wheat cultivars (McCallum et al., 2016). The race non-specific resistance gene Lr34 has been used widely over many years in wheat cultivars and has remained effective. Canadian wheat cultivars commonly carry Lr34 (McCallum et al., 2011) and it is frequently present in other wheat cultivars throughout the world. It also confers resistance to other diseases including stripe rust (Yr18, Singh, 1992), stem rust (Sr57, Dyck et al., 1985; Hiebert et al., 2010), powdery mildew (Pm38, Spielmeyer et al., 2005), and virus diseases (Bdv1, Singh, 1993).
One important feature of Lr34 is that it interacts with other leaf rust resistance genes to give better levels of resistance. Ezzahiri and Roelfs (1989) determined that the durable and effective adult plant resistance in Era wheat was controlled by the interaction of Lr13 and Lr34. These authors crossed plants of Era with the susceptible cultivar Baart, and they tested 473 and 367 F3 derived lines in the F4 generation in Minnesota USA and Morocco, respectively. They found that Lr34 significantly enhanced the level of resistance conditioned by Lr13 but the effect of Lr34 on its own was not detected.
German and Kolmer (1992) crossed the Thatcher-Lr34 near-isogenic line (NIL) with other Thatcher NILs containing different resistance genes. From F2 families ten to 16 plants with the lowest infection type when inoculated with P. triticina race 1 were selected and grown to maturity. Seed from these selected F2 plants was then grown in a rust nursery and the five or six most resistant F3 lines, homozygous for Lr34 were selected and harvested. Two homozygous F4 lines per cross were tested as seedlings in the greenhouse and three to four plants of one F4 line were tested as adults in greenhouse tests. Selected lines with Lr34 and a second leaf rust resistance gene were also field tested over two years. These authors found that Lr34 enhanced resistance, both at the seedling and adult plant stages, in combination with many effective resistance genes, and this also resulted in lower adult plant infection types and leaf rust severity levels in the field. However, combinations involving Lr34 with less effective or ineffective genes had the same level of resistance as Thatcher-Lr34.
Kloppers and Pretorius (1997) investigated two gene combinations of Lr13, Lr34, and Lr37. For glasshouse studies, they used a single F4 line from crosses between the pairs of Thatcher isolines that contained each of these resistance genes. These same F4 lines and six to eight sister lines from the same crosses were compared in field trials. They found that the two gene lines generally had better resistance as measured by latent period, field resistance, and the microscopic development of fungal structures. Interestingly, they also noted significant variation between sister lines for the Lr34 + Lr13 gene combination in which partial resistance was found in field trials. No variation was found among the sister lines from the gene combinations involving Lr37 since the level of resistance was nearly complete.
The wheat leaf rust resistance gene Lr67 is also race non-specific, is only effective at the adult plant stage, and confers multi-pest resistance to stripe rust (Hiebert et al., 2010; Herrera-Foessel et al., 2011) along with stem rust and powdery mildew like Lr34 (Herrera-Foessel et al., 2014). Both Lr34 and Lr67 have been cloned and code for different types of cellular transporters (Krattinger et al., 2009; Moore et al., 2015). Mutations in either gene resulted in mutants that were susceptible to leaf, stem, and stripe rust (Spielmeyer et al., 2013). With combinations of most leaf rust resistance genes, the severity of disease observed is similar to the most effective of the genes involved. However, Lr34 interacts with other leaf rust resistance genes, and combinations of genes involving Lr34 are more resistant than any of the genes involved.
Some of the most common leaf rust resistance genes in Canadian wheat are Lr2a, Lr10, Lr13, Lr14a, Lr16, Lr21, and Lr34 (McCallum et al., 2016). In this study, we choose to determine the interactions between both Lr67 and Lr34 with each of the genes; Lr13, Lr16, and Lr32. They represent a range of effectiveness from mostly ineffective (Lr13) to highly effective (Lr32). Both Lr13 and Lr16 are in many Canadian wheat cultivars, such as Carberry (Bokore et al., 2022), because popular wheat cultivars grown in the recent past like AC Barrie and AC Domain have either or both genes and donated these genes to the current generation of wheat cultivars. Lr13 is relatively ineffective against the Canadian population of P. triticina, as nearly all isolates are virulent to Lr13 (McCallum et al., 2021). However, it may still have an effect on reducing leaf rust severity in combination with other genes, such as Lr34 and Lr67. Complete virulence to Lr16 is rare in Canada (McCallum et al., 2021) but nearly all isolates have an intermediate level of virulence and combinations of Lr16 with genes, such as Lr34 and Lr46, are fairly effective at reducing leaf rust severity (Bokore et al., 2022). In contrast, Lr32 is a very effective leaf rust resistance gene in Canada with no virulence detected to date (McCallum et al., 2021); however, it has not yet been deployed in any Canadian wheat cultivars. The Thatcher near-isogenic lines containing Lr13 (RL4031), Lr16 (RL6005), Lr32 (RL6086), Lr34 (RL6058), and Lr13 + Lr34 (RL6114) had annual rust severity averages in inoculated nurseries in Manitoba Canada over the years 2003–2021 of 76.6, 65.6, 30.8, 23.2, and 10.3%, respectively, compare with Thatcher at 81.9% (B. McCallum unpublished).
Given the many similarities between Lr67 and Lr34, the objective of this study was to determine if Lr67 also interacts with other resistance genes. To test this we developed six doubled haploid populations from the crosses with either single gene lines with Lr34 or Lr67 and each of the near-isogenic lines with either Lr13, Lr16, or Lr32. Each progeny line was genotyped with molecular markers to determine if the line had the resistant or susceptible allele of each gene involved in the population, except for Lr13 which was determined by rust testing at the adult plant stage. Progeny from these crosses were field tested over four years to determine the resistance level of lines in each phenotypic class; susceptible, those having the resistant allele for either Lr34 or Lr67 alone, those only having the resistant allele of the second resistance gene (Lr13, Lr16 or Lr32), and those with both genes.
Materials and Methods
Populations
Doubled haploid (DH) populations were developed from the crosses between Thatcher near-isogenic lines with Lr34 (RL6058), Lr67 (RL6077), Lr13 (RL4031), Lr16 (RL6005), and a Katepwa backcross line with Lr32 (BW196R). The progeny populations consisted of 78 lines (Thatcher-Lr13/Thatcher-Lr34), 74 lines (Thatcher-Lr13/Thatcher-Lr67), 58 lines (Thatcher-Lr16/Thatcher-Lr34), 85 lines (Thatcher-Lr16/Thatcher-Lr67), 114 lines (Thatcher-Lr34/BW196R), and 113 lines (Thatcher-Lr67/BW196R). DH populations were generated using the maize pollination described by Thomas et al. (1997) except a single dicamba (100 ppm) treatment was used by placing a large drop with a syringe between the primary and secondary florets (all other florets were removed from each spikelet prior to emasculation) the day after pollination.
Marker Analysis
To classify the progeny for the presence or absence of the genes targeted in each population, DNA markers were used to classify Lr34, Lr32, Lr16, and Lr32. The Lr34 locus was genotyped using a PCR marker, caIND11, that targets an indel in the Lr34 gene sequence (Dakouri et al., 2010). Both Lr67 and Lr16 were classified based on closely linked SNP markers, csSNP856 (Forrest et al., 2014) and kwm742 (Kassa et al., 2017) respectively. SSR markers wmc43 and barc135 were used to detect the presence of Lr32 (Thomas et al., 2010). PCR products for caIND11 and SSR markers for Lr32 were resolved using an ABI 3100 genetic analyzer (Applied Biosystems) as described by Somers et al. (2004). To genotype the SNP markers for Lr67 and Lr16, KASP assays were performed as described by Kassa et al. (2016).
Given that current markers for Lr13 are not tightly linked, Lr13 was classified based on indoor leaf rust assays. For both populations that segregated for Lr13, two plants per line were grown to the adult plant stage in the greenhouse then inoculated with the Lr13 avirulent P. triticina isolate 1–1 BBBD. While these populations also segregated for Lr34 or Lr67, the presence of Lr13 resulted in a clear and highly resistant reaction phenotype (‘;1-‘infection type as described by McCallum et al., 2021) that was not seen in those lines with Lr34 or Lr67 or susceptible lines which had more susceptible pustule types. Therefore all lines could therefore be scored as having either the resistant or the susceptible allele for Lr13.
Leaf Rust Field Resistance
These populations were grown in leaf rust inoculated, irrigated, field nurseries at Morden Manitoba during four years 2012–2015, with two replications per year, except in 2012 in which a single row was planted for each line. Progeny from the populations lines Thatcher-Lr13/Thatcher-Lr34 and Thatcher-Lr13/Thatcher-Lr67 were tested for an additional two field seasons in 2016 and 2017, with two replicates per season. Each line was seeded in approximately 1 m rows. Spreader rows of susceptible wheat were planted at regular intervals to help the epidemic develop and infect the test lines. Spreader rows were inoculated a few times each year with a mixture of urediniospores in Soltrol mineral oil. The inoculum was a mixture of P. triticina virulence phenotypes, representative of those found in western Canada the previous year (McCallum et al., 2018, 2019, 2020, 2021). The lines were assessed for the level of leaf rust infection on the flag leaves using a 0–100% modified Cobb scale (Peterson et al., 1948). They were also assessed for pustule type (R-MR-MS-S) but only the severity data were used for analysis since this was a better measure of the proportion of the flag leaves infected with leaf rust.
Leaf rust severity percentage ratings were converted to proportions for analysis, then back to percentages for presentation. Data from each population were analyzed separately with SAS 9.3 (SAS Institute Inc.) using PROC GLIMMIX (beta distribution) with the presence or absence of the genes, and their interaction, in each progeny line of the population as dependent variables and replication within each year as the random variable. Within each population, the groups of lines with all the possible gene combinations were compared pairwise to each other using LSMEANS.
Results
The effects of both Lr13 and Lr34 were significant in the Lr13/Lr34 population, as was the interaction between Lr13 and Lr34 (Table 1). The lines that had both genes had the lowest level of leaf rust severity (17.7%), followed by lines with only Lr34 (23.8%), lines with only Lr13 (84.3%), and lines with neither gene (85.7%) (Table 1; Figure 1). When these four classes of lines were compared against each other, each class was significantly different from the others at the p < 0.01 level, except lines with only Lr13 which were not different from lines with neither gene (Table 2). In the Lr13/Lr67 population, the effect of Lr67 was significant, but not that of Lr13. In contrast to the Lr13/Lr34 population, there was no significant interaction between the two genes. In this population, lines with both genes had a similar level of leaf rust severity (36.2%) compared to lines with only Lr67 (37.0%) and lines that only had Lr13 were similar (80.5%) with lines that had neither gene (79.0%) (Tables 1, 2; Figure 2).
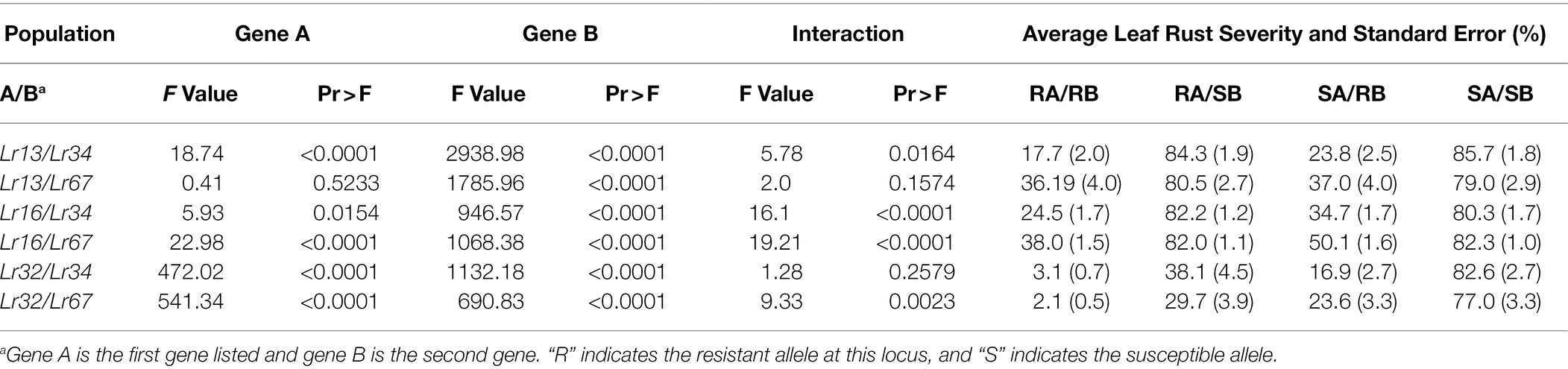
Table 1. Effect of each gene and their interaction on the severity of leaf rust and the average severity for each genotypic class.
Both Lr16 and Lr34 were significant in reducing leaf rust severity in the Lr16/Lr34 population, and the interaction between the genes was also significant (p < 0.01). Lines with both genes had the lowest leaf rust severity (24.6%), followed by lines with only Lr34 (34.7%), lines with only Lr16 however had a similar level of leaf rust severity (82.2%) as the lines with neither gene (80.3%) (Tables 1, 2; Figure 3). Similarly, in the Lr16/Lr67 population, both Lr16 and Lr67 were significant in reducing leaf rust severity, and their interaction was significant (p < 0.01). Again lines with both genes had the lowest level of severity (38.0%), followed by lines with only Lr67 (50.1%) and lines with only Lr16 were similar (82.0%) to lines with neither gene (82.3%) (Tables 1, 2; Figure 4).
In the Lr32/Lr34 population, both genes significantly reduced leaf rust severity (p < 0.01); however, their interaction was not significant. Lines with both genes were very resistant (3.1%), followed by lines with only Lr34 (16.9%) or only Lr32 (38.1%), compared to lines with neither gene (82.6%) (Table 1; Figure 5). However, each class of lines was significantly different from the other classes (Table 2). Similarly for the Lr32/Lr67 population both genes significantly reduced leaf rust severity (p < 0.01), their interaction was however significant (p < 0.01). Again lines with both genes had the lowest leaf rust severity (2.1%), followed by those with only Lr67 (23.6%), those with only Lr32 (29.7%), and those with neither gene (77.0%) (Table 1; Figure 6). Each class of line was also significantly different from all the other classes (Table 2).
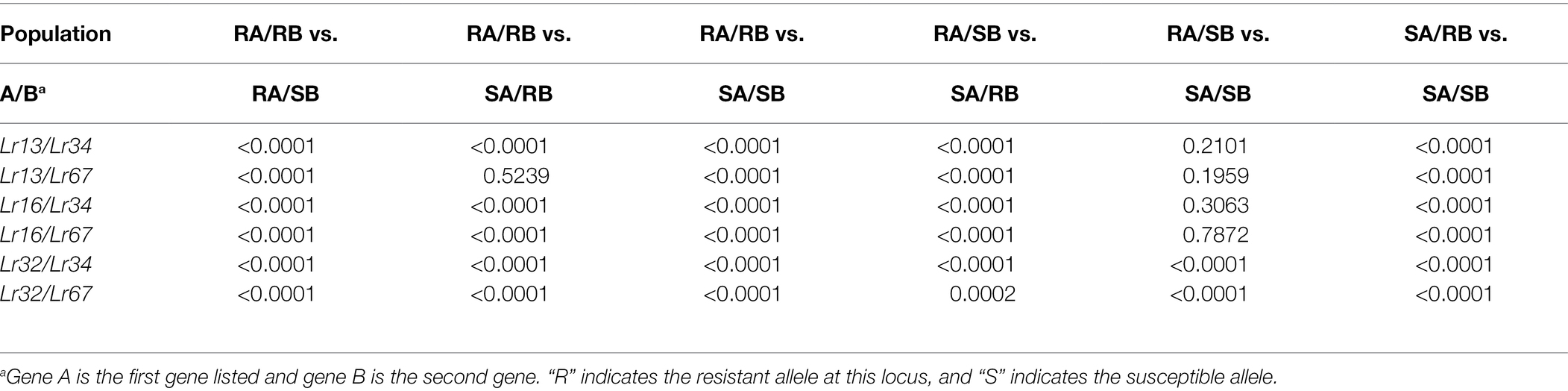
Table 2. Significance of the difference between groups of lines with different combinations of resistance genes.
Discussion
This study compared how Lr34 and Lr67 interact in combination with other resistance genes in progeny populations. They were each paired with Lr13, Lr16, or Lr32 in populations segregating for one of those genes and either Lr34 or Lr67. In the populations that segregated for Lr13, the effect of Lr13 was significant in the population with Lr34 but not with the population involving Lr67. There was a significant interaction between Lr13 and Lr34 whereas there was no significant interaction between Lr13 and Lr67. It appears that Lr34 and Lr67 differ in their interaction with Lr13. The lack of interaction with Lr67 may reflect the marginal resistance provided by Lr13 and that fact that most of the virulence phenotypes in Canada are virulent on Lr13. During the years of the field tests the frequency of virulence to Lr13 in the population was close to 100% (McCallum et al., 2018, 2019, 2020, 2021). Both Lr34 and Lr67 were effective in reducing the leaf rust severity, but Lr34 had a larger effect on reducing leaf rust compared to Lr67 which may reflect a different in their interactive magnitudes. The Lr13 + Lr34 gene combination appeared to be more effective than either gene alone in this study, and in previous studies (Ezzahiri and Roelfs, 1989; German and Kolmer, 1992; Kloppers and Pretorius, 1997).
The resistance gene Lr16 was more effective than Lr13 in reducing the severity of leaf rust. While the frequency of virulence to Lr16 is very low (near 0%) (McCallum et al., 2021), most isolates have an intermediate response to Lr16 and the Thatcher-Lr16 wheat line is fairly susceptible in field trials as the long term leaf rust severity average of the Thatcher isoline with Lr16 was 65.6% compared with Thatcher at 81.9% (B. McCallum unpublished). Overall Lr16 had a significant effect on leaf rust in these populations and had significant interactions with both Lr34 and Lr67 (Table 1). The effect of Lr16 is mainly in its interaction with either Lr34 or Lr67, because the lines with both Lr16 and either Lr34 or Lr67 were significantly more resistant than lines with just Lr34 or Lr67, however, the lines with Lr16 alone were not significantly different from lines with neither gene in this study (Table 2). Both Lr34 and Lr67 interacted with Lr16 to produce an enhanced resistance, even though lines with Lr16 alone were not significantly different from susceptible lines. This may reflect on the ability of Lr16 to interact with other resistance genes, as it has been shown previously to do with genes like Lr13 (Samborski and Dyck, 1982) and both Lr34 and Lr46 in Carberry (Bokore et al., 2022).
This effect of enhancement was also seen with the populations involving Lr32. Alone Lr32 was very effective in reducing the severity of leaf rust, as were both Lr34 and Lr67. The lines with two gene combinations of Lr32 + Lr34 and Lr32 + Lr67 were even more resistant than any of these genes alone. Virulence has not been detected in Canada to Lr32 and the Thatcher-Lr32 line is moderately resistant in field trials. Both Lr34 and Lr67 had the ability to enhance the resistance of Lr32 when in combination with this resistance gene. However, the interaction was only significant between Lr32 and Lr67 (Table 1). The parental line containing Lr32 in these crosses also contained Lr13, which segregated in both populations, although its presence or absence was not determined it would have been distributed evenly between phenotypic classes. The effects of Lr34 and Lr67 were stronger in these populations, than in the other populations analyzed, which could reflect the fact that Lr13 was segregating in these crosses and potentially interacting with the other leaf rust resistance genes.
Overall Lr67 behaved similarly to Lr34 in this study. Both genes consistently reduced the level of leaf rust in each population, Lr67 did not interact with Lr13, which was only effective on its own in the Lr34 population, however, both did have a significant interaction with Lr16. The effect of Lr16 was significant overall but it appeared to be effective only when in combination with either Lr34 or Lr67 to result in a significantly lower level of leaf rust than with either gene alone. Both genes also significantly reduced leaf rust when combined with the effective resistance gene Lr32.
Interactions between race-specific leaf rust resistance genes and Lr34 have been analyzed in previous studies. The studies by Kloppers and Pretorius (1997) and German and Kolmer (1992) both showed that lines carrying Lr34 plus a race-specific gene had lower disease severity than either gene singly. The data presented here show the same trend. The most direct comparison between these three studies is the interaction between Lr34 and Lr13 as this combination was present in all of the studies. German and Kolmer (1992) report what appears to be the strongest interaction between Lr13 and Lr34. However, there are some key differences between how these studies were conducted. German and Kolmer (1992) selected a single F4 line that was homozygous for Lr34 and Lr13 and they reportedly selected the most resistant homozygous line for field testing. This was also done for the other gene combinations in their study. Kloppers and Pretorius (1997) analyzed six lines with Lr34 and Lr13 and they found varying responses between lines. At the time of their final field rating, the severities of the six lines ranged from 10 to 50%. The interaction would look different if they had only used the most resistant line.
Similarly, in our study, DH lines carrying Lr34 and Lr13 had mean severities ranging from 7 to 37%. There was at least some range of severity levels among the progeny lines for all the various gene combinations generated in this study. While Thatcher near-isogenic lines were used primarily as the parental lines in this study, intercrossing these lines resulted in significant variation between lines with the same major gene combinations, similar to the variation found by Kloppers and Pretorius (1997) with the Lr13 + Lr34 sister lines. Using multiple lines or populations gives a more accurate representation of the interactions between genes as all of the other factors will also be segregating and correlated errors are minimized. In the present study, we also compared DH lines carrying each gene singly for the same reason as when the NILs were developed, the best phenotypes were selected which may not best represent the resistance conferred by the Lr gene in question.
Both Lr34 and Lr67, along with a third multi-pest non-race-specific adult plant resistance gene Lr46, are important components of resistance in CIMMYT and Mexican wheat cultivars (Singh et al., 2008; Huerta-Espino et al., 2020). Deployed alone these adult plant multi-pest resistance genes did not confer adequate resistance, but combinations of 4–5 genes usually results in near immunity levels of resistance (Singh et al., 2011). Interestingly, Lr67 was deployed in many Mexican wheat cultivars developed in the 1950s, but not in later cultivars due to chance parental selection in which only Lr34 was used. Since the donor lines for Lr34 (RL6058) and Lr67 (RL6077) showed similar resistance phenotypes, both lines were initially thought to have Lr34 and were used interchangeably as a source for Lr34 in the 1950s by the CIMMYT wheat breeding program (Huerta-Espino et al., 2020).
Gene pyramids involving Lr34 are also common in Canadian wheat cultivars (McCallum et al., 2011; Toth et al., 2018). The highly resistant Canadian cultivar Pasqua contains five resistance genes including Lr34 (Dyck, 1993). Lr34 appears to be key to its high level of resistance, as progeny lines derived from Pasqua with the four other resistance gene were fairly susceptible (McCallum and Thomas, 2014). Similarly, the high level of durable resistance in the cultivar Carberry is conditioned by the combination of Lr2a, Lr16, Lr23, Lr13, Lr34, and Lr46 (Bokore et al., 2022), in which the key is the interaction of Lr34 and Lr46 with the other resistance genes. These multi-pest resistance genes along with others, such as Sr2, appear to function very well in combinations with other genes to condition effective and durable resistance, often by boosting the effect of other resistance genes (Ellis et al., 2014). Randhawa et al. (2018) found that Lr34/Yr18/Sr57 interacted with Lr68 to reduce leaf rust and Sr2/Yr30 to reduce rust severity to leaf, stem, and stripe rust in a segregating population.
It appears that Lr67 could play a similar and important role in leaf rust resistance, like Lr34 or Lr46, if it was combined with other resistance genes, such as Lr16 and Lr32, in which it could interact to result in lower levels of leaf rust severity and improved durability of resistance. However, Lr67 failed to show the same significant interaction with Lr13 that Lr34 demonstrated, and the effect of Lr34 alone was stronger than that of Lr67 in each of the pairs of populations. In contrast, the interaction between Lr32 and Lr67 was significant whereas that between Lr32 and Lr34 was not. While Lr67 was deployed in many CIMMYT wheat cultivars from the 1950s (Huerta-Espino et al., 2020), it is not deployed in Canadian wheat cultivars to date. If it was deployed in Canada and other countries it could improve the rust resistance and durability of future wheat cultivars.
Data Availability Statement
The original contributions presented in the study are included in the article, further inquiries can be directed to the corresponding author.
Author Contributions
BM and CH planned the experiments, analyzed the data, and co-wrote the manuscript. CH developed the populations analyzed in this study and conducted the molecular marker analysis of all progeny lines. BM conducted the leaf rust phenotyping of the populations. All authors contributed to the article and approved the submitted version.
Funding
Funding for this project was from Agriculture and Agri-Food Canada.
Conflict of Interest
The authors declare that the research was conducted in the absence of any commercial or financial relationships that could be construed as a potential conflict of interest.
Publisher’s Note
All claims expressed in this article are solely those of the authors and do not necessarily represent those of their affiliated organizations, or those of the publisher, the editors and the reviewers. Any product that may be evaluated in this article, or claim that may be made by its manufacturer, is not guaranteed or endorsed by the publisher.
References
Bokore, F. E., Knox, R. E., Hiebert, C. W., Cuthbert, R. D., DePauw, R. M., Meyer, B., et al. (2022). A combination of leaf rust resistance genes, including Lr34 and Lr46, is the key to the durable resistance of the Canadian wheat cultivar. Carberry. Front. Plant Sci. 12:775383. doi: 10.3389/fpls.2021.775383
Dakouri, A., McCallum, B. D., Walichnowski, A. Z., and Cloutier, S. (2010). Fine-mapping of the leaf rust Lr34 locus in Triticum aestivum (L.) and characterization of large germplasm collections support the ABC transporter as essential for gene function. Theor. Appl. Genet. 121, 373–384. doi: 10.1007/s00122-010-1316-7
Dyck, P. L. (1993). The inheritance of leaf rust resistance in the wheat cultivar Pasqua. Can. J. Plant Sci. 73, 903–906. doi: 10.4141/cjps93-118
Dyck, P. L., Samborski, D. J., and Martens, J. W. (1985). Inheritance of resistance to leaf rust and stem rust in the wheat cultivar Glenlea. Can. J. Plant Pathol. 7, 351–354.
Ellis, J. G., Lagudah, E. S., Spielmeyer, W., and Dodds, P. N. (2014). The past, present and future of breeding rust resistant wheat. Fron Pl Sci. 5:641. doi: 10.3389/fpls.2014.00641
Ezzahiri, B., and Roelfs, A. P. (1989). Inheritance and expression of adult plant resistance to leaf rust in era wheat. Plant Dis. 73, 549–551. doi: 10.1094/PD-73-0549
Forrest, K., Pujol, V., Bulli, P., Pumphrey, M., Wellings, C., Herrera-Foessel, S., et al. (2014). Development of a SNP marker assay for the Lr67 gene of wheat using a genotyping by sequencing approach. Mol. Breeding 34, 2109–2118. doi: 10.1007/s11032-014-0166-4
German, S. E., and Kolmer, J. A. (1992). Effect of gene Lr34 in the enhancement of resistance to leaf rust of wheat. Theor. Appl. Genet. 84, 97–105. doi: 10.1007/BF00223987
Herrera-Foessel, S. A., Lagudah, E. S., Huerta-Espino, J., Hayden, M. J., Bariana, H. S., Singh, D., et al. (2011). New slow-rusting leaf rust and stripe rust resistance genes Lr67 and Yr46 in wheat are pleiotropic or closely linked. Theor. Appl. Genet. 122, 239–249. doi: 10.1007/s00122-010-1439-x
Herrera-Foessel, S. A., Singh, R. P., Lillemo, M., Huerta-Espino, J., Bhavani, S., Singh, S., et al. (2014). Lr67/Yr46 confers adult plant resistance to stem rust and powdery mildew in wheat. Theor. Appl. Genet. 127, 781–789. doi: 10.1007/s00122-013-2256-9
Hiebert, C. W., Fetch, T. G., Zegeye, T., Thomas, J. B., Somers, D. J., Humphreys, D. G., et al. (2010). Genetics and mapping of seedling resistance to Ug99 stem rust in Canadian wheat cultivars 'Peace' and 'AC Cadillac'. Theor. Appl. Genet. 122, 143–149. doi: 10.1007/s00122-010-1430-6
Hiebert, C. W., Thomas, J. B., McCallum, B. D., Humphreys, D. G., DePauw, R. M., Hayden, M. J., et al. (2010). An introgression on wheat chromosome 4DL in RL6077 (Thatcher*6/PI 250413) confers adult plant resistance to stripe rust and leaf rust (Lr67). Theor. Appl. Genet. 121, 1083–1091. doi: 10.1007/s00122-010-1373-y
Huerta-Espino, J., Singh, R., Crespo-Herrera, L. A., Villaseñor-Mir, H. E., Rodriguez-Garcia, M. F., Dreisigacker, S., et al. (2020). Adult plant slow rusting genes confer high levels of resistance to rusts in bread wheat cultivars from Mexico. Front Pl Sci. 11:824. doi: 10.3389/fpls.2020.00824
Huerta-Espino, J., Singh, R. P., Germán, S., McCallum, B. D., Park, R. F., Chen, W. Q., et al. (2011). Global status of wheat leaf rust caused by Puccinia triticina. Euphytica 179, 143–160. doi: 10.1007/s10681-011-0361-x
Kassa, M. T., You, F. M., Fetch, T. G., Fobert, P., Sharpe, A., Pozniak, C. J., et al. (2016). Genetic mapping of SrCad and SNP marker development for marker-assisted selection of Ug99 stem rust resistance in wheat. Theor. Appl. Genet. 129, 1373–1382. doi: 10.1007/s00122-016-2709-z
Kassa, M. T., You, F. M., Hiebert, C. W., Pozniak, C. J., Fobert, P. R., Sharpe, A. G., et al. (2017). Highly predictive SNP markers for efficient selection of the wheat leaf rust resistance gene Lr16. BMC Biol. 17:45. doi: 10.1186/s12870-017-0993-7(38785)
Kloppers, F. J., and Pretorius, Z. A. (1997). Effects of combinations amongst genes Lr13, Lr34 and Lr37 on components of resistance in wheat to leaf rust. Plant Pathol. 46, 737–750. doi: 10.1046/j.1365-3059.1997.d01-58.x
Krattinger, S. G., Lagudah, E. S., Spielmeyer, W., Singh, R. P., Huerta-Espino, J., McFadden, H., et al. (2009). A putative ABC transporter confers durable resistance to multiple fungal pathogens in wheat. Science 323, 1360–1363. doi: 10.1126/science.1166453
McCallum, B. D., Hiebert, C. W., Cloutier, S., Bakkeren, G., Rosa, S. B., Humphreys, D. G., et al. (2016). A review of wheat leaf rust research and the development of resistant cultivars in Canada. Can. J. Plant Pathol. 38, 1–18. doi: 10.1080/07060661.2016.1145598
McCallum, B. D., Humphreys, D. G., Somers, D. J., Dakouri, A., and Cloutier, S. (2011). Allelic variation for the rust resistance gene Lr34/Yr18 in Canadian wheat cultivars. Euphytica 183, 261–274. doi: 10.1007/s10681-011-0519-6
McCallum, B. D., Reimer, E., McNabb, W., Foster, A., Rosa, S., and Xue, A. (2021). Physiologic specialization of Puccinia triticina, the causal agent of wheat leaf rust, in Canada in 2015-2019. Can. J. Plant Pathol. 43, S333–S346. doi: 10.1080/07060661.2021.1888156
McCallum, B. D., Reimer, E., McNabb, W., Foster, A., and Xue, A. (2020). Physiologic specialization of Puccinia triticina, the causal agent of wheat leaf rust, in Canada in 2014. Can. J. Plant Pathol. 42, 520–526. doi: 10.1080/07060661.2020.1723705
McCallum, B. D., Seto-Goh, P., Foster, A., and Xue, A. (2018). Physiological specialization of Puccinia triticina, the causal agent of wheat leaf rust, in Canada in 2012. Can. J. Plant Pathol. 40, 434–441. doi: 10.1080/07060661.2018.1495267
McCallum, B. D., Seto-Goh, P., Reimer, E., Foster, A., and Xue, A. (2019). Physiological specialization of Puccinia triticina, the causal agent of wheat leaf rust, in Canada in 2013. Can. J. Plant Pathol. 42, 243–249. doi: 10.1080/07060661.2019.1653376
McCallum, B. D., and Thomas, J. (2014). Lr34 Is the Key to the Durable Leaf Rust Resistance in the Canadian Cultivar Pasqua. Borlaug Global Rust Initiative Technical Workshop. Obregon, Mexico.
Moore, J. W., Herrera-Foessel, S., Lan, C., Schnippenkoetter, W., Ayliffe, M., Huerta-Espino, J., et al. (2015). A recently evolved hexose transporter variant confers resistance to multiple pathogens in wheat. Nat. Genet. 47, 1494–1498. doi: 10.1038/ng.3439
Peterson, R. F., Campbell, A. B., and Hannah, A. E. (1948). A diagrammatic scale for estimating rust intensity on leaves and stems of cereals. Can. J. Res. 26c, 496–500. doi: 10.1139/cjr48c-033
Randhawa, M. S., Lan, C., Basnet, B. R., Bhavani, S., Huerta-Espino, J., Forrest, K. L., et al. (2018). Interactions among genes Sr2/Yr30, Lr34/Yr18/Sr57 and Lr68 confer enhanced adult plant resistance to rust diseases in common wheat (Triticum aestivum L.) line ‘Arula’. Aust. J. Crop. Sci. 12, 1023–1033. doi: 10.21475/ajcs.18.12.06.PNE1305
Samborski, D. J., and Dyck, P. L. (1982). Enhancement of resistance to Puccinia recondita by interactions of resistance genes in wheat. Can. J. Plant Pathol. 4, 152–156. doi: 10.1080/07060668209501317
Singh, R. P. (1992). Genetic association of leaf rust resistance gene Lr34 with adult plant resistance to stripe rust in bread wheat. Phytopathology 82, 835–838. doi: 10.1094/Phyto-82-835
Singh, R. P. (1993). Genetic association of gene Bdv1 for tolerance to barley yellow dwarf virus with genes Lr34 and Yr18 for adult plant resistance to rusts in bread wheat. Plant Dis. 77, 1103–1106. doi: 10.1094/PD-77-1103
Singh, R. P., Huerta-Espino, J., Bhavani, S., Herrera-Foessel, S. A., Singh, D., Singh, P. K., et al. (2011). Race non-specific resistance to rust diseases in CIMMYT spring wheats. Euphytica 179, 175–186. doi: 10.1007/s10681-010-0322-9
Singh, R. P., Huerta-Espino, J., and William, M. (2008). “Breeding for resistance to biotic stresses,” in Plant Breeding: The Arnel R. Hallauer International Symposium. eds. R. Kendall and M. L. Lamkey (Hoboken: Blackwell Publishing), 310–322. doi: 10.1002/9780470752708.ch22
Somers, D. J., Isaac, P., and Edwards, K. (2004). A high density microsatellite consensus map for bread wheat (Triticum aestivum L.). Theor. Appl. Genet. 109, 1105–1114. doi: 10.1007/s00122-004-1740-7
Spielmeyer, W., Mago, R., Wellings, C., and Ayliffe, M. (2013). Lr67 and Lr34 rust resistance genes have much in common - they confer broad spectrum resistance to multiple pathogens in wheat. BMC Plant Biol. 13:96.
Spielmeyer, W., McIntosh, R. A., Kolmer, J., and Lagudah, E. S. (2005). Powdery mildew resistance and Lr34/Yr18 genes for durable resistance to leaf and stripe rust cosegregate at a locus on the short arm of chromosome 7D of wheat. Theor. Appl. Genet. 111, 731–735. doi: 10.1007/s00122-005-2058-9
Thomas, J., Chen, Q., and Howes, N. (1997). Chromosome doubling of haploids of common wheat with caffeine. Genome 40, 552–558. doi: 10.1139/g97-072
Thomas, J., Nilmalgoda, S., Hiebert, C., McCallum, B., Humphreys, G., and DePauw, R. (2010). Genetic markers and leaf rust resistance of the wheat gene Lr32. Crop Sci. 50, 2310–2317. doi: 10.2135/cropsci2010.02.0065
Keywords: resistance, pyramid, gene, combinations, interaction, durable
Citation: McCallum BD and Hiebert CW (2022) Interactions Between Lr67 or Lr34 and Other Leaf Rust Resistance Genes in Wheat (Triticum aestivum). Front. Plant Sci. 13:871970. doi: 10.3389/fpls.2022.871970
Edited by:
Morten Lillemo, Norwegian University of Life Sciences, NorwayReviewed by:
Hanif Khan, Indian Institute of Wheat and Barley Research (ICAR), IndiaMichał Tomasz Kwiatek, Poznan University of Life Sciences, Poland
Volker Mohler, Bayerische Landesanstalt für Landwirtschaft (LfL), Germany
Copyright © 2022 McCallum and Hiebert. This is an open-access article distributed under the terms of the Creative Commons Attribution License (CC BY). The use, distribution or reproduction in other forums is permitted, provided the original author(s) and the copyright owner(s) are credited and that the original publication in this journal is cited, in accordance with accepted academic practice. No use, distribution or reproduction is permitted which does not comply with these terms.
*Correspondence: Brent D. McCallum, YnJlbnQubWNjYWxsdW1AYWdyLmdjLmNh