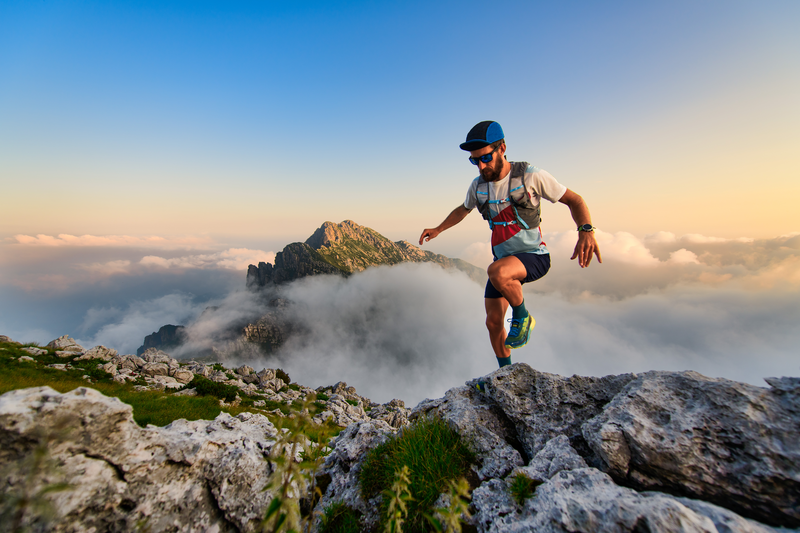
94% of researchers rate our articles as excellent or good
Learn more about the work of our research integrity team to safeguard the quality of each article we publish.
Find out more
REVIEW article
Front. Plant Sci. , 03 March 2022
Sec. Plant Metabolism and Chemodiversity
Volume 13 - 2022 | https://doi.org/10.3389/fpls.2022.866282
This article is part of the Research Topic Evolution of Pronouncing Chemodiversity and Biological Role of Secondary Metabolites in Lamiaceae Family View all 5 articles
Scutellaria baicalensis, is one of the most traditional medicinal plants in the Lamiaceae family, and has been widely used to treat liver and lung complaints and as a complementary cancer treatment in traditional Chinese medicine. The preparation from its roots, called “Huang Qin,” is rich in specialized flavones such as baicalein, wogonin, and their glycosides which lack a 4′-hydroxyl group on the B ring (4′-deoxyflavones), with anti-tumor, antioxidant, and antiviral activities. Baicalein has recently been reported to inhibit the replication of the COVID-19 virus. These 4′-deoxyflavones are found only in the order Lamiales and were discovered in the genus Scutellaria, suggesting that a new metabolic pathway synthesizing 4′-deoxyflavones evolved recently in this genus. In this review, we focus on the class of 4′-deoxyflavones in S. baicalensis and their pharmacological properties. We also describe the apparent evolutionary route taken by the genes encoding enzymes involved in the novel, root-specific, biosynthetic pathway for baicalein and wogonin, which provides insights into the evolution of specific flavone biosynthetic pathways in the mint family.
Scutellaria baicalensis Georgi (NCBI: txid65409) is a perennial herb belonging to the Lamiaceae family (Figure 1A). The dried root of S. baicalensis is known in China as “Huang (黄) Qin (芩)” (meaning golden herb) (Figure 1B), and was first recorded in Shennong Bencaojing (The Classic of Herbal Medicine) in around 200 AD. It is used for the treatment of bitter, cold, lung, and liver problems (Ma, 2013; Zhao et al., 2016a). It is indigenous to China, the Korean peninsula, Japan, Mongolia, and the Russian Federation, and has been cultivated worldwide as a medicinal plant (Shang et al., 2010). The potential medicinal activity of S. baicalensis was first recorded in Bencao Gangmu (Compendium of Materia Medica) published in 1593, with its root being used to alleviate the symptoms of diarrhea, dysentery, hypertension, hemorrhaging, insomnia, inflammation, and respiratory infections (Li, 1593). In recent decades, many studies have examined the potential hepato- and neuro-protective, antitumor, antimicrobial, anti-inflammatory, antioxidative, and antiviral effects of extracts of S. baicalensis (Wang et al., 2018).
Figure 1. The medicinal plant Scutellaria baicalensis and its major flavones. (A) S. baicalensis plant. (B) The dried roots of S. baicalensis used in traditional Chinese medicine. (C) Structure of flavones produced from naringenin. (D) Structure of 4′-deoxyflavones derived from pinocembrin.
Investigations into the pharmacological activities of S. baicalensis have focused mainly on the large amounts of specialized root-specific flavones (RSFs), such as baicalein, wogonin, and their glycosides baicalin and wogonoside. These RSFs lack a 4′-OH group on their B-rings (4′-deoxyRSFs) compared to the more typical 4′-hydroxyflavones such as scutellarein, which is widely distributed in aerial tissues of S. baicalensis (Figures 1C,D). The 4′-deoxyRSFs contribute most health benefits in S. baicalensis and specifically show a wide spectrum of antitumor activities (Table 1).
Scutellaria baicalensis is also an important ingredient of Qingfei Paidu Decoction (QPD). QPD is a new combined formula in traditional Chinese medicine (TCM) based on four classical formulae described in the Shang Han Zabing Lun (Treatise on Cold Pathogenic and Miscellaneous Diseases) (National Administration of Traditional Chinese Medicine, 2020). It is the only prescription in TCM recommended for treatment of mild, moderate, and severe cases of COVID-19 infection, and could also be used for critical patients according to their specific conditions (National Health Commission of the People’s Republic of China, 2021). Clinical data showed that COVID-19 related mortality was only 1.2% of the 2568 patients that received QPD compared with 4.8% of the mortality among 6371 patients that did not receive QPD (Zhang et al., 2021). This research also reported that the use of QPD could reduce 50% in-hospital, COVID-19-related mortality. Analysis of QPD shows it includes baicalin, the 4′-deoxyRSF from roots of S. baicalensis (Yang et al., 2020). Liu et al. (2021) reported that baicalein could suppress the replication of COVID-19 virus in Vero cells by inhibiting 3C-like protease, the main protease of severe acute respiratory syndrome coronavirus 2 (SARS-CoV-2). Other research gave similar results and confirmed the binding mode of baicalein with SARS-CoV-2 3C-like protease by X-ray protein crystallography (Su et al., 2020).
Flavonoids are large group of plant specialized metabolites and their biosynthetic pathways may evolve independently by convergent evolution which result in the production of these specialized bioactive flavones in widely diverged plant species (Wen et al., 2020). 4′-deoxyRSFs have a taxon-specific distribution and are also found sporadically in species outside the genus Scutellaria but in the order Lamiales, such as Andrographis paniculate, Oroxylum indicum, and Plantago major (Samuelsen, 2000; Chen et al., 2003; Li et al., 2007). They have also been reported in Anodendron affine and Cephalocereus senilis outside the order Lamiales (Qin et al., 1993; Tai et al., 2005). In recent years, taking advantage of newly developed genomic sequencing technologies, high-quality reference genome sequences for S. baicalensis have been published (Zhao et al., 2019; Xu et al., 2020). These were the first genome assemblies at chromosome-level resolution published for members of the family Lamiaceae. Based on the genomic information, notable progress has been made in elucidating the biochemical functions and evolutionary pathways of the genes encoding biosynthetic enzymes involved in the baicalein and wogonin metabolism in S. baicalensis (Zhao et al., 2016b; Zhao et al., 2018; Zhao et al., 2019; Xu et al., 2020).
There are two distinct pathways in S. baicalensis responsible for the synthesis of flavones (Figure 2). In the aerial parts of the plant, naringenin is used as the precursor of scutellarein and scutellarin biosynthesis. Naringenin is synthesized from phenylalanine by general phenylpropanoid metabolism. Phenylalanine is converted to naringenin by phenylalanine ammonialyase (PAL), followed by ring hydroxylation by cinnamoyl 4 hydroxylase (C4H), activation by p-coumaroyl CoA ligase (4CL), condensation with 3 molecules of malonyl CoA by chalcone synthase (CHS), and isomerization by chalcone isomerase (CHI) (Lepiniec et al., 2006). Naringenin is then oxidized by flavone synthase II-1 (FNSII-1) to form apigenin, which is further hydroxylated and glycosylated to form scutellarein and scutellarin (Nagashima et al., 2000; Zhao et al., 2016b). Alternatively, the 4′-deoxyRSFs from roots of S. baicalensis, which include baicalein and wogonin, and their glycosides, are synthesized from a newly evolved pathway where cinnamic acid is activated by cinnamate-CoA ligase-like 7 (CLL-7) to form cinnamoyl CoA, which is then condensed with three molecules of malonyl CoA by a specific isoform of chalcone synthase (CHS-2), and isomerized by chalcone isomerase (CHI) to form pinocembrin, a flavanone without a 4′-OH group. Pinocembrin is then converted by a specialized isoform of flavone synthase II-2 (FNSII-2) to form chrysin, which serves as the precursor of other 4′-deoxyRSFs (Zhao et al., 2016b). Chrysin is decorated by flavone 6-hydroxylase (F6H) to produce baicalein, or by flavone 8-hydroxylase (F8H) and phenylpropanoid and flavonoid O-methyltransferases (PFOMT) to produce wogonin (Zhao et al., 2018; Zhao et al., 2019). These two RSFs are further glucuronidated by flavonoid 7-O-glucuronosyltransferases (UBGAT) to produce baicalin and wogonoside (Nagashima et al., 2000).
Figure 2. Two pathways responsible for biosynthesis of flavones in Scutellaria baicalensis. The classic flavone pathway makes scutellarein in aerial tissues (left hand), while 4′-deoxyRSFs are produced by a newly evolved pathway in roots (right hand). PAL, phenylalanine ammonialyase; C4H, cinnamate 4-hydroxylase; CLL-7, cinnamate-CoA ligase; CLL-1, 4-coumarate CoA ligase; CHS, chalcone synthase; CHI, chalcone isomerase; FNSII, flavone synthase II; F6H, flavone 6-hydroxylase; F8H, flavone 8-hydroxylase; PFOMT, flavonoid O-methyltransferases; UBGAT, baicalein 7-O-glucuronosyltransferase.
Phenylalanine ammonialyase is one of the key enzymes acting between primary and secondary metabolism and catalyzes the deamination of L-phenylalanine to trans-cinnamic acid and ammonia (MacDonald and D’Cunha, 2007). Three full-length cDNAs encoding phenylalanine ammonialyase isoforms (SbPAL1, SbPAL2, and SbPAL3) were cloned from S. baicalensis using rapid amplification of cDNA ends (RACE) technology (Xu et al., 2010). Tissue-specific expression analysis indicated that the expression levels of SbPAL1, SbPAL2, and SbPAL3 were highest in stems, leaves and roots, respectively. Besides, the transcripts of SbPAL1, SbPAL2, and SbPAL3 were found to be up-regulated by MeJA and wounding treatments. To further confirm their function, SbPAL1, SbPAL2, and SbPAL3 were overexpressed in S. baicalensis hairy roots by Agrobacterium tumefaciens-mediated transformation, and the transgenic hairy root lines produced higher baicalin, baicalein, and wogonin than the control hairy root line (Park et al., 2012). Comparative genome analysis revealed that the PAL gene numbers (five) in S. baicalensis were expanded compared to those (four) in Scutellaria barbata, another medicinal plant of Scutellaria genus accumulating RSFs. The specific expansion of these genes might have occurred via tandem duplication following the speciation of S. baicalensis and S. barbata (<13.28 Mya) (Xu et al., 2020).
Cinnamate-CoA ligase-like are isoforms of 4CL which may activate cinnamic, benzoic, or fatty acid derivatives (Schneider et al., 2003; Shockey et al., 2003). Five full-length cDNAs of CLL genes were identified and cloned from S. baicalensis based on the root RNA-seq database (Zhao et al., 2016b). Phylogenetic analysis showed that SbCLL-1 and SbCLL-5 were grouped in the same clade as other known 4-CoA ligases and separated from the group of SbCLL-6, SbCLL-7, and SbCLL-8. Tissue-specific expression analysis suggested that SbCLL-7 was expressed most highly in roots. The purified recombinant proteins of SbCLL-1 and SbCLL-5 could add CoA to cinnamic acid, 4-coumaric acid, and caffeic acid, while SbCLL-7 could add CoA only to cinnamic acid. Besides, SbCLL-7 had a substantially lower apparent Km value and higher Vmax/Km value for cinnamic acid compared to SbCLL-1 and SbCLL-5. Silencing of SbCLL-7 in S. baicalensis hairy roots reduced the accumulation of baicalin, baicalein, and wogonoside compared to the empty vector controls. Comparative genome analysis revealed that SbCLL-7 had syntenic homologs in Salvia miltiorrhiza, Salvia splendens, and Sesamum indicum, but these syntenic CLL-7s from other species of the Lamiaceae had no activity with cinnamic acid, 4-coumaric acid, or caffeic acid, indicating that the specific activity of SbCLL-7 for cinnamic acid is not shared by syntenic genes in species closely related to S. baicalensis (Zhao et al., 2019). Protein modeling of CLLs revealed that a more hydrophilic cysteine residue (C393) substituting a hydrophobic tryptophan (W) or leucine (L) residue might be responsible for SbCLL-7 binding cinnamate as a substrate. These point mutations in the CLL gene likely occurred after the divergence of S. baicalensis, from S. miltiorrhiza and S. splendens (<32.7 Mya) (Zhao et al., 2019).
Two full-length cDNAs of CHS genes (SbCHS-1 and SbCHS-2) were identified and cloned from S. baicalensis based on the RNA-seq database (Zhao et al., 2016b). SbCHS-1 is expressed specifically in flowers which may be responsible for the biosynthesis of classic flavones and anthocyanins. SbCHS-2 is highly expressed in roots and encodes an enzyme involved in the formation of pinocembrin chalcone and responsible for RSF biosynthesis (Zhao et al., 2016b). Based on genome analysis, a tandem multiplication event occurred in the genomic region of SbCHS-2, which produced five adjacent gene copies (SbCHS2.1-SbCHS2.6). This example of gene amplification might increase transcript and protein dosage and drive stronger flux along the RSF biosynthetic pathway (Zhao et al., 2019). The neofunctionalization of SbCHS-1 and SbCHS-2 followed the divergence of the Salvia and Scutellaria lineages (∼32.7 Mya). The ancestral CHS gene was duplicated in S. baicalensis, with one copy (SbCHS-1) moving to pseudochromosome 3, while the other copy (SbCHS-2L) remained on pseudochromosome 9 (∼19 Mya). SbCHS-2L was further duplicated (SbCHS2.3) around 12 Mya, and was then amplified by tandem duplications to produce the other SbCHS-2 genes accompanied by neofunctionalization probably within the last 1 Mya (Figure 3A). In S. barbata, CHS-2 has not been amplified to the same extent as in S. baicalensis (Xu et al., 2020). This confirms that most of the amplifications of CHS-2 occurred very recently (Zhao et al., 2019), perhaps linked to domestication of S. baicalensis through its use in TCM.
Figure 3. Evolutionary paths of SbCHS2 and SbFNSII-2. (A) Syntenic analysis of SbCHS2s genes in Scutellaria baicalensis, Scutellaria barbata, and Salvia splendens. The multiplications of SbCHS2 are mostly late at the species level for S. baicalensis. (B) Syntenic analysis of SbFNSII-1 and SbFNSII-2 genes in S. baicalensis, S. barbata, and S. splendens. The duplication of SbFNSII-2 is earlier before the species divergence within Scutellaria.
One full-length cDNA encoding SbCHI was cloned from S. baicalensis using rapid amplification of cDNA ends (RACE) technology (Park et al., 2011). Tissue-specific expression analysis indicated that the expression levels of SbCHI were highest in roots, whereas lower expression levels were detected in the flowers, stems, and leaves. The transcripts of SbCHI were found to be up-regulated by MeJA and wounding in suspension cell cultures of S. baicalensis. Overexpression of SbCHI in S. baicalensis hairy roots enhanced the production of baicalein, baicalin, and wogonin, while SbCHI-silenced hairy root lines showed reduced accumulation of baicalein and baicalin (Park et al., 2011). There is only one locus encoding SbCHI and its activity is shared by both aerial and RSF biosynthetic pathways (Zhao et al., 2016b; Zhao et al., 2019).
Two different types of FNS enzyme (FNSI and FNSII) are responsible for the conversion of flavanones to flavones by introducing the double bond between the C2 and C3 positions in S. baicalensis. FNSI belongs to the 2-oxoglutarate-dependent dioxygenase (2OGD) superfamily and is found only in species of the Apiaceae (Umbelliferae) (Kawai et al., 2014), while FNSII is a cytochrome P450 (CYP450) monooxygenase widely distributed in angiosperms (Martens and Mithöfer, 2005). Generally, FNSI and FNSII catalyze the conversion of flavones with a 4′-OH group, such as naringenin, eriodictyol, and liquiritigen into flavones (Britsch, 1990; Fliegmann et al., 2010). Zhao et al. (2016b) reported a neo-functional FNSII from S. baicalensis (SbFNSII-2) which is required for the biosynthesis of specialized 4′-deoxyRSFs. SbFNSII-2 is highly expressed in roots and is induced by MeJA. It can convert only pinocembrin to chrysin in both in vivo and in vitro assays. RNAi silencing of SbFNSII-2 in hairy roots of S. baicalensis significantly reduced the accumulation of baicalin, wogonoside, and baicalein. S. baicalensis also produces an isoform of FNSII (SbFNSII-1) that converts naringenin to apigenin in aerial parts of the plant. In S. baicalensis there are two SbFNSII-1 loci which arose as part of a segmental duplication which occurred after the divergence of the Lamiaceae (<42.7 Mya). However, just one SbFNSII-2 locus is present in the S. baicalensis genome suggesting that SbFNSII-2 arose by tandem duplication of SbFNSII-1, and neofunctionalization following the divergence of genus Salvia and the genus Scutellaria (<32.7 Mya) (Zhao et al., 2019). In S. barbata, one gene orthologous to FNSII-2 can be detected in a tandem duplication of FNSII-1 and FNSII-2 in the region collinear between S. baicalensis and S. barbata (Figure 3B) (Xu et al., 2020). This would place the FNS-II duplication earlier than the species divergence within the genus Scutellaria and indicate the conserved evolution of 4′-deoxyflavones in the genus Scutellaria.
Two CYP450 family members in S. baicalensis are responsible for the hydroxylation of the 6- and 8-positions of the flavones (SbF6H and SbF8H, respectively). These two enzymes convert chrysin to baicalein and norwogonin, respectively (Zhao et al., 2018). SbF6H (CYP82D1.1) can use both 4′-hydroxyflavones and 4′-deoxyflavones (such as apigenin and chrysin, respectively) to produce scutellarein and baicalein in aerial parts and roots, respectively. RNAi silencing of SbF6H resulted in a significant reduction in the levels of baicalin and baicalein levels but promoted the accumulation of chrysin glycosides in hairy roots. SbF8H (CYP82D2) accepts only chrysin as its substrate to produce norwogonin, and exhibits only very minor 6-hydroxylation activity. Silencing of SbF8H reduced the levels of wogonin and wogonoside, but slightly increased baicalin levels. Structural modeling revealed that several amino acid substitutions contribute to the substrate binding in different orientations in the active site of SbF6H and SbF8H, resulting in their divergent catalytic activity (Zhao et al., 2018). In S. baicalensis there is only one SbF6H locus on pseudochromosome 5 which was likely derived from multiplication of CYP82D genes on pseudochromosome 1 and this gene acquired its new position by retrotransposition, while SbF8H underwent tandem multiplication and neofunctionalization (Zhao et al., 2019).
O-methyltransferases are responsible for the transformation of a methyl group from S-adenosyl-L-methionine (SAM) to a hydroxyl group on their substrate. O-methylation contributes to the structural diversity of flavonoids and modifies their solubility, stability, and bioactivity (Ibrahim and Anzellotti, 2003). In S. baicalensis, two types of OMT are involved in the biosynthesis of root-specific 4′-deoxyflavones. Type II OMTs are Mg2+-dependent and also known as phenylpropanoid and flavonoid OMTs (PFOMTs), which are reported to transfer methyl groups to flavones with adjacent hydroxyl groups on their aromatic rings. In S. baicalensis roots, SbPFOMT2 and 5 can efficiently O-methylate the C6, C8, and C3′ positions of flavones to form mono-methoxyflavones oroxylin A, wogonin, tenaxin II, and chrysoeriol, respectively (Zhao et al., 2019; Cui et al., 2021). Three type I OMTs from S. baicalensis, referred to as flavonoid OMTs (SbFOMTs), have been found to decorate hydroxyl 4′-deoxyflavones. SbFOMT3 is a 7-OMT that converts baicalein to 7-methoxybaicalein, while SbFOMT6 is a 7-OMT that can use both baicalein and norwogonin as substrates. SbFOMT5 can methylate hydroxyl groups of baicalein on the C5, C6, and C7 positions. Combination of SbPFOMT5 and SbFOMT6 or SbPFOMT5 plus SbFOMT5 can produce skullcapflavone I and tenaxin I, respectively, in yeast. These root methoxylated flavones exhibit stronger activities than baicalein in inducing apoptosis of human cancer cell lines (Cui et al., 2021). In addition, SbPFOMT5 has undergone recent tandem duplication and neofunctionalization (Zhao et al., 2019).
Glycosylation is an important decoration for flavones in the stabilization and enhancement of their solubility in water (Bowles et al., 2005). Baicalin is a 7-O glucuronide derived from baicalein, catalyzing by a UBGAT in S. baicalensis (Nagashima et al., 2000). This enzyme is also responsible for glucuronidation of the 7-OH group of flavones with ortho-substituents, such as wogonin and scutellarein (Nagashima et al., 2000).
In summary, the 4′-deoxyRSF biosynthetic pathway emerged after divergence of the genera Scutellaria and Salvia (< 32.7 Mya), and likely evolved by point mutation (SbCLL-7), tandem multiplication and neofunctionalization (SbCHS-2, SbFNSII-2, SbF8H, and SbPFOMT), segmental duplication (SbFNSII-2), and retroduplication (SbF6H). Specifically, SbCHS-2 underwent several rounds of amplification: the earlier duplications (SbCHS2L and SbCHS2.3) occurred before the species divergence within Scutellaria, while tandem multiplications (CHS-2.1, CHS-2.2, CHS-2.4, CHS-2.5, and CHS-2.6) occurred later at the species level, in S. baicalensis.
Specialized metabolites from plants have served in history as the main source of medicines for humans when challenged by pandemics (Weng, 2020), such as the COVID-19 virus, which has infected 280 million people and has killed over 5.4 million people at the time of writing this manuscript (World Health Organization1). Baicalein from the roots of S. baicalensis was reported to be an effective inhibitor of the COVID-19 virus (Su et al., 2020; Liu et al., 2021). So far, the complete biosynthetic pathway of baicalein has been elucidated, and the production of baicalein directly from glucose by Escherichia coli-fed batch fermentation can reach 214.1 mg/L (Ji et al., 2021). These studies provide a model for discovering and utilizing medicinal plants for development of therapies. Nevertheless, enzymes involved in the modification of the B-ring of some flavones found in S. baicalensis roots, such as tenaxin I, skullcapflavone I, rehderianin I, and viscidulin III, have not yet been identified. These flavones are hydroxylated on the 2′, 3′, 5′, and 6′ positions of their B-ring and some of them (tenaxin I and skullcapflavone I) exhibit stronger cytotoxicity than baicalein in cancer cells apoptosis assays (Cui et al., 2021), which has inspired us to explore further the biosynthetic pathways as well as their clinical applications.
Scutellaria includes about 350 species around the world, and plants of this genus have been widely used in traditional medicine for thousands of years. For example, S. barbata, a plant native to southern China, has been used to cure pain and swelling of throat, edema and hemorrhoids as well as its prescription for cancer treatment; Scutellaria galericulata and Scutellaria lateriflora are used as sedative/nerve tonics in Europe and North America; and Scutellaria indica has been employed for detoxification and promoting blood circulation effects in China, South Korea, and India. In addition to flavones, Scutellaria plants are rich in diterpenoids or diterpenoid alkaloids. These compounds exhibit excellent antitumor activities and their biosynthetic pathways are not fully understood (Shang et al., 2010). Rapidly developed genome sequencing, metabolite profiling, gene editing, and machine learning (AlphaFold) technologies will help us study the biosynthesis of active ingredients in Scutellaria plants as well as their diversification and evolution.
TP collected literatures and wrote the manuscript. MY performed the evolutionary analysis of SbCHS2 and SbFNSII-2. YH and YW provided the image of S. baicalensis plants. QZ and CM revised the manuscript. All authors read and approved the final manuscript.
This work was supported by National Key R&D Program of China (2018YFC1706200), National Natural Science Foundation of China (31870282), Special Fund for Scientific Research of Shanghai Landscaping and City Appearance Administrative Bureau (G212401 and G192419), and Youth Innovation Promotion Association of Chinese Academy of Sciences. QZ was also supported by the Shanghai Youth Talent Support Program and SANOFI-SIBS scholarship.
The authors declare that the research was conducted in the absence of any commercial or financial relationships that could be construed as a potential conflict of interest.
All claims expressed in this article are solely those of the authors and do not necessarily represent those of their affiliated organizations, or those of the publisher, the editors and the reviewers. Any product that may be evaluated in this article, or claim that may be made by its manufacturer, is not guaranteed or endorsed by the publisher.
Bonham, M., Posakony, J., Coleman, I., Montgomery, B., Simon, J., and Nelson, P. S. (2005). Characterization of chemical constituents in Scutellaria baicalensis with antiandrogenic and growth-inhibitory activities toward prostate carcinoma. Clin. Cancer Res. 11, 3905–3914. doi: 10.1158/1078-0432.CCR-04-1974
Bowles, D., Isayenkova, J., Lim, E.-K., and Poppenberger, B. (2005). Glycosyltransferases: managers of small molecules. Curr. Opin. Plant Biol. 8, 254–263. doi: 10.1016/j.pbi.2005.03.007
Britsch, L. (1990). Purification and characterization of flavone synthase I, a 2-oxoglutarate-dependent desaturase. Arch. Biochem. Biophys. 282, 152–160. doi: 10.1016/0003-9861(90)90099-K
Chan, F. L., Choi, H. L., Chen, Z. Y., Chan, P. S. F., and Huang, Y. (2000). Induction of apoptosis in prostate cancer cell lines by a flavonoid, baicalin. Cancer Lett. 160, 219–228. doi: 10.1016/S0304-3835(00)00591-7
Chen, L.-J., Games, D. E., and Jones, J. (2003). Isolation and identification of four flavonoid constituents from the seeds of Oroxylum indicum by high-speed counter-current chromatography. J. Chromatogr. A 988, 95–105. doi: 10.1016/S0021-9673(02)01954-4
Chen, M., Zhong, K., Tan, J., Meng, M., Liu, C. M., Chen, B., et al. (2021). Baicalein is a novel TLR4-targeting therapeutics agent that inhibits TLR4/HIF-1α/VEGF signaling pathway in colorectal cancer. Clin. Transl. Med. 11:e564. doi: 10.1002/ctm2.564
Chen, Y., Hui, H., Yang, H., Zhao, K., Qin, Y., Gu, C., et al. (2013). Wogonoside induces cell cycle arrest and differentiation by affecting expression and subcellular localization of PLSCR1 in AML cells. Blood 121, 3682–3691. doi: 10.1182/blood-2012-11-466219
Cheng, Y.-H., Li, L.-A., Lin, P., Cheng, L.-C., Hung, C.-H., Chang, N. W., et al. (2012). Baicalein induces G1 arrest in oral cancer cells by enhancing the degradation of cyclin D1 and activating AhR to decrease Rb phosphorylation. Toxicol. Appl. Pharmacol. 263, 360–367. doi: 10.1016/j.taap.2012.07.010
Chung, H., Jung, Y.-M., Shin, D.-H., Lee, J.-Y., Oh, M.-Y., Kim, H.-J., et al. (2008). Anticancer effects of wogonin in both estrogen receptor-positive and -negative human breast cancer cell lines in vitro and in nude mice xenografts. Int. J. Cancer 122, 816–822. doi: 10.1002/ijc.23182
Cui, M. Y., Lu, A. R., Li, J. X., Liu, J., Fang, Y. M., Pei, T. L., et al. (2021). Two types of O-methyltransferase are involved in biosynthesis of anticancer methoxylated 4′-deoxyflavones in Scutellaria baicalensis Georgi. Plant Biotechnol. J. 20, 129–142. doi: 10.1111/pbi.13700
Dong, P., Zhang, Y., Gu, J., Wu, W., Li, M., Yang, J., et al. (2011). Wogonin, an active ingredient of Chinese herb medicine Scutellaria baicalensis, inhibits the mobility and invasion of human gallbladder carcinoma GBC-SD cells by inducing the expression of maspin. J. Ethnopharmacol. 137, 1373–1380. doi: 10.1016/j.jep.2011.08.005
Fliegmann, J., Furtwängler, K., Malterer, G., Cantarello, C., Schüler, G., Ebel, J., et al. (2010). Flavone synthase II (CYP93B16) from soybean (Glycine max L.). Phytochemistry 71, 508–514. doi: 10.1016/j.phytochem.2010.01.007
Huang, S.-T., Wang, C.-Y., Yang, R.-C., Chu, C.-J., Wu, H.-T., and Pang, J.-H. S. (2010). Wogonin, an active compound in Scutellaria baicalensis, induces apoptosis and reduces telomerase activity in the HL-60 leukemia cells. Phytomedicine 17, 47–54. doi: 10.1016/j.phymed.2009.06.005
Ibrahim, R. K., and Anzellotti, D. (2003). “Chapter 1: The enzymatic basis of flavonoid biodiversity,” in Recent Advances in Phytochemistry, ed. J. T. Romeo (Amsterdam: Elsevier), 1–36.
Ji, D., Li, J., Xu, F., Ren, Y., and Wang, Y. (2021). Improve the biosynthesis of baicalein and scutellarein via manufacturing self-assembly enzyme reactor in vivo. ACS Synth. Biol. 10, 1087–1094. doi: 10.1021/acssynbio.0c00606
Kawai, Y., Ono, E., and Mizutani, M. (2014). Evolution and diversity of the 2-oxoglutarate-dependent dioxygenase superfamily in plants. Plant J. 78, 328–343. doi: 10.1111/tpj.12479
Lepiniec, L., Debeaujon, I., Routaboul, J.-M., Baudry, A., Pourcel, L., Nesi, N., et al. (2006). Genetics and biochemistry of seed flavonoids. Annu. Rev. Plant Biol. 57, 405–430. doi: 10.1146/annurev.arplant.57.032905.105252
Li, W., Xu, X., Zhang, H., Ma, C., Fong, H., van Breemen, R., et al. (2007). Secondary metabolites from Andrographis paniculata. Chem. Pharm. Bull. 55, 455–458. doi: 10.1248/cpb.55.455
Liu, H., Ye, F., Sun, Q., Liang, H., Li, C., Li, S., et al. (2021). Scutellaria baicalensis extract and baicalein inhibit replication of SARS-CoV-2 and its 3C-like protease in vitro. J. Enzyme Inhib. Med. Chem. 36, 497–503. doi: 10.1080/14756366.2021.1873977
Ma, J. X. (2013). Explanatory Notes to Shennong Bencaojing. Beijing: People’s Medical Publishing House.
MacDonald, M., and D’Cunha, G. (2007). A modern view of phenylalanine ammonia lyase. Biochem. Cell Biol. 85, 273–282. doi: 10.1139/o07-018
Martens, S., and Mithöfer, A. (2005). Flavones and flavone synthases. Phytochemistry 66, 2399–2407. doi: 10.1016/j.phytochem.2005.07.013
Mu, J., Liu, T., Jiang, L., Wu, X., Cao, Y., Li, M., et al. (2016). The traditional Chinese medicine baicalein potently inhibits gastric cancer cells. J. Cancer 7, 453–461. doi: 10.7150/jca.13548
Nagashima, S., Hirotani, M., and Yoshikawa, T. (2000). Purification and characterization of UDP-glucuronate: baicalein 7-O-glucuronosyltransferase from Scutellaria baicalensis Georgi. cell suspension cultures. Phytochemistry 53, 533–538. doi: 10.1016/S0031-9422(99)00593-2
National Administration of Traditional Chinese Medicine (2020). Qingfei Paidu Decoction. Available online at: http://www.satcm.gov.cn/hudongjiaoliu/guanfangweixin/2020-03-05/13622.html (accessed March 5, 2020).
National Health Commission of the People’s Republic of China (2021). Diagnosis and Treatment Protocol for COVID-19 (Trial Version 8.0). Available online at: http://www.nhc.gov.cn/cms-search/downFiles/a449a3e2e2c94d9a856d5faea2ff0f94.pdf (accessed April 14, 2021).
Park, N. I., Xu, H., Li, X., Kim, S.-J., and Park, S. U. (2011). Enhancement of flavone levels through overexpression of chalcone isomerase in hairy root cultures of Scutellaria baicalensis. Funct. Integr. Genomics 11, 491–496. doi: 10.1007/s10142-011-0229-0
Park, N. I., Xu, H., Li, X., Kim, Y. S., Lee, M. Y., and Park, S. U. (2012). Overexpression of phenylalanine ammonia-lyase improves flavones production in transgenic hairy root cultures of Scutellaria baicalensis. Process Biochem. 47, 2575–2580. doi: 10.1016/j.procbio.2012.09.026
Qin, L., Markham, K. R., Paré, P. W., Dixon, R. A., and Mabry, T. J. (1993). Flavonoids from elicitor-treated cell suspension cultures of Cephalocereus senilis. Phytochemistry 32, 925–928. doi: 10.1016/0031-9422(93)85230-O
Samuelsen, A. B. (2000). The traditional uses, chemical constituents and biological activities of Plantago major L. A review. J. Ethnopharmacol. 71, 1–21. doi: 10.1016/S0378-8741(00)00212-9
Schneider, K., Hövel, K., Witzel, K., Hamberger, B., Schomburg, D., Kombrink, E., et al. (2003). The substrate specificity-determining amino acid code of 4-coumarate:CoA ligase. Proc. Natl. Acad. Sci. U.S.A. 100, 8601–8606. doi: 10.1073/pnas.1430550100
Shang, X., He, X., He, X., Li, M., Zhang, R., Fan, P., et al. (2010). The genus Scutellaria an ethnopharmacological and phytochemical review. J. Ethnopharmacol. 128, 279–313. doi: 10.1016/j.jep.2010.01.006
Shockey, J. M., Fulda, M. S., and Browse, J. (2003). Arabidopsis contains a large superfamily of acyl-activating enzymes. Phylogenetic and biochemical analysis reveals a new class of acyl-coenzyme a synthetases. Plant Physiol. 132, 1065–1076. doi: 10.1104/pp.103.020552
Su, H. X., Yao, S., Zhao, W. F., Li, M. J., Liu, J., Shang, W. J., et al. (2020). Anti-SARS-CoV-2 activities in vitro of Shuanghuanglian preparations and bioactive ingredients. Acta Pharmacol. Sin. 41, 1167–1177. doi: 10.1038/s41401-020-0483-6
Tai, M. C., Tsang, S. Y., Chang, L. Y. F., and Xue, H. (2005). Therapeutic potential of wogonin: a naturally occurring flavonoid. CNS Drug Rev. 11, 141–150. doi: 10.1111/j.1527-3458.2005.tb00266.x
Wang, C. Z., Zhang, C. F., Chen, L., Anderson, S., Lu, F., and Yuan, C. S. (2015). Colon cancer chemopreventive effects of baicalein, an active enteric microbiome metabolite from baicalin. Int. J. Oncol. 47, 1749–1758. doi: 10.3892/ijo.2015.3173
Wang, Z. L., Wang, S., Kuang, Y., Hu, Z. M., Qiao, X., and Ye, M. (2018). A comprehensive review on phytochemistry, pharmacology, and flavonoid biosynthesis of Scutellaria baicalensis. Pharm. Biol. 56, 465–484. doi: 10.1080/13880209.2018.1492620
Wen, W., Alseekh, S., and Fernie, A. R. (2020). Conservation and diversification of flavonoid metabolism in the plant kingdom. Curr. Opin. Plant Biol. 55, 100–108. doi: 10.1016/j.pbi.2020.04.004
Weng, J. K. (2020). Plant solutions for the COVID-19 pandemic and beyond: historical reflections and future perspectives. Mol. Plant 13, 803–807. doi: 10.1016/j.molp.2020.05.014
Wu, J. Y., Tsai, K. W., Li, Y. Z., Chang, Y. S., Lai, Y. C., Laio, Y. H., et al. (2013). Anti-bladder-tumor effect of baicalein from Scutellaria baicalensis Georgi and its application in vivo. Evid. Based Complement. Alternat. Med. 2013:579751. doi: 10.1155/2013/579751
Xu, H., Park, N. I., Li, X., Kim, Y. K., Lee, S. Y., and Park, S. U. (2010). Molecular cloning and characterization of phenylalanine ammonia-lyase, cinnamate 4-hydroxylase and genes involved in flavone biosynthesis in Scutellaria baicalensis. Bioresour. Technol. 101, 9715–9722. doi: 10.1016/j.biortech.2010.07.083
Xu, X. F., Cai, B. L., Guan, S. M., Li, Y., Wu, J. Z., Wang, Y., et al. (2011). Baicalin induces human mucoepidermoid carcinoma Mc3 cells apoptosis in vitro and in vivo. Investig. New Drugs 29, 637–645. doi: 10.1007/s10637-010-9402-x
Xu, Z., Gao, R., Pu, X., Xu, R., Wang, J., Zheng, S., et al. (2020). Comparative genome analysis of Scutellaria baicalensis and Scutellaria barbata reveals the evolution of active flavonoid biosynthesis. Genomics Proteomics Bioinformatics 18, 230–240. doi: 10.1016/j.gpb.2020.06.002
Yang, R., Liu, H., Bai, C., Wang, Y., Zhang, X., Guo, R., et al. (2020). Chemical composition and pharmacological mechanism of Qingfei Paidu Decoction and Ma Xing Shi Gan Decoction against coronavirus disease 2019 (COVID-19): in silico and experimental study. Pharmacol. Res. 157:104820. doi: 10.1016/j.phrs.2020.104820
Zhang, D. Y., Wu, J., Ye, F., Xue, L., Jiang, S., Yi, J., et al. (2003). Inhibition of cancer cell proliferation and prostaglandin E2 synthesis by Scutellaria baicalensis. Cancer Res. 63, 4037–4043. doi: 10.1016/S0165-4608(03)00013-X
Zhang, L., Zheng, X., Bai, X., Wang, Q., Chen, B., Wang, H., et al. (2021). Association between use of Qingfei Paidu Tang and mortality in hospitalized patients with COVID-19: a national retrospective registry study. Phytomedicine 85:153531. doi: 10.1016/j.phymed.2021.153531
Zhao, Q., Chen, X. Y., and Martin, C. (2016a). Scutellaria baicalensis, the golden herb from the garden of Chinese medicinal plants. Sci. Bull. 61, 1391–1398. doi: 10.1007/s11434-016-1136-5
Zhao, Q., Zhang, Y., Wang, G., Hill, L., Weng, J. K., Chen, X. Y., et al. (2016b). A specialized flavone biosynthetic pathway has evolved in the medicinal plant, Scutellaria baicalensis. Sci. Adv. 2:1501780. doi: 10.1126/sciadv.1501780
Zhao, Q., Cui, M. Y., Levsh, O., Yang, D. F., Liu, J., Li, J., et al. (2018). Two CYP82D enzymes function as flavone hydroxylases in the biosynthesis of root-specific 4′-deoxyflavones in Scutellaria baicalensis. Mol. Plant 11, 135–148. doi: 10.1016/j.molp.2017.08.009
Keywords: Scutellaria baicalensis, 4′-deoxyflavones, flavonoids, biosynthetic pathways, evolutionary mechanisms
Citation: Pei T, Yan M, Huang Y, Wei Y, Martin C and Zhao Q (2022) Specific Flavonoids and Their Biosynthetic Pathway in Scutellaria baicalensis. Front. Plant Sci. 13:866282. doi: 10.3389/fpls.2022.866282
Received: 31 January 2022; Accepted: 14 February 2022;
Published: 03 March 2022.
Edited by:
Eirini Sarrou, Hellenic Agricultural Organization—ELGO, GreeceReviewed by:
Teemu Heikki Teeri, University of Helsinki, FinlandCopyright © 2022 Pei, Yan, Huang, Wei, Martin and Zhao. This is an open-access article distributed under the terms of the Creative Commons Attribution License (CC BY). The use, distribution or reproduction in other forums is permitted, provided the original author(s) and the copyright owner(s) are credited and that the original publication in this journal is cited, in accordance with accepted academic practice. No use, distribution or reproduction is permitted which does not comply with these terms.
*Correspondence: Cathie Martin, Y2F0aGllLm1hcnRpbkBqaWMuYWMudWs=; Qing Zhao, emhhb3FpbmdAY2VtcHMuYWMuY24=
Disclaimer: All claims expressed in this article are solely those of the authors and do not necessarily represent those of their affiliated organizations, or those of the publisher, the editors and the reviewers. Any product that may be evaluated in this article or claim that may be made by its manufacturer is not guaranteed or endorsed by the publisher.
Research integrity at Frontiers
Learn more about the work of our research integrity team to safeguard the quality of each article we publish.