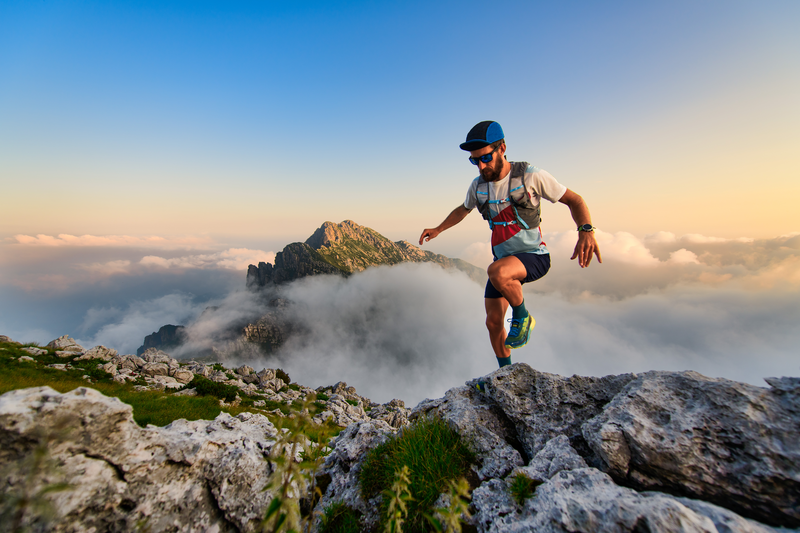
94% of researchers rate our articles as excellent or good
Learn more about the work of our research integrity team to safeguard the quality of each article we publish.
Find out more
REVIEW article
Front. Plant Sci. , 24 March 2022
Sec. Plant Physiology
Volume 13 - 2022 | https://doi.org/10.3389/fpls.2022.865302
This article is part of the Research Topic Methods in Phytohormone Detection and Quantification: 2022 View all 6 articles
The tubular-shaped unicellular extensions of plant epidermal cells known as root hairs are important components of plant roots and play crucial roles in absorbing nutrients and water and in responding to stress. The growth and development of root hair include, mainly, fate determination of root hair cells, root hair initiation, and root hair elongation. Phytohormones play important regulatory roles as signal molecules in the growth and development of root hair. In this review, we describe the regulatory roles of auxin, ethylene (ETH), jasmonate (JA), abscisic acid (ABA), gibberellin (GA), strigolactone (SL), cytokinin (CK), and brassinosteroid (BR) in the growth and development of plant root hairs. Auxin, ETH, and CK play positive regulation while BR plays negative regulation in the fate determination of root hair cells; Auxin, ETH, JA, CK, and ABA play positive regulation while BR plays negative regulation in the root hair initiation; Auxin, ETH, CK, and JA play positive regulation while BR, GA, and ABA play negative regulation in the root hair elongation. Phytohormones regulate root hair growth and development mainly by regulating transcription of root hair associated genes, including WEREWOLF (WER), GLABRA2 (GL2), CAPRICE (CPC), and HAIR DEFECTIVE 6 (RHD6). Auxin and ETH play vital roles in this regulation, with JA, ABA, SL, and BR interacting with auxin and ETH to regulate further the growth and development of root hairs.
The root system of a plant is an important structure in the organism. Its main functions are to fix the plant into the soil, obtain nutrients and water from the soil, and synthesize nitrogenous organic compounds such as amino acids. Root morphology and activity will directly affect the growth, development, and nutritional status of the whole plant (Yang et al., 2012). The root is composed of the root cap, the meristem zone, the elongation zone, and the mature zone (Bibikova and Gilroy, 2003). Root hairs are tubular-shaped unicellular extensions of epidermal cells, which serve to both expand the root surface area, facilitate the absorption and utilization of water and nutrients by the root system, and help plants respond to stress and interact with soil microorganisms (Peterson and Farquhar, 1996; Datta et al., 2011; Vissenberg et al., 2020).
The growth and development process of plant root hairs includes, mainly, root hair cell fate determination, root hair initiation, and root hair elongation (Bibikova and Gilroy, 2003). Root hair cells are derived from root epidermal cells, but not all epidermal cells can develop into root hairs. There are three types of root hair cell fate determination. The first type is random, in which all epidermal cells have the potential to differentiate into root hairs. Most dicotyledonous plants, ferns, and many monocotyledonous plants belong to this type (Clowes, 2000; Pemberton et al., 2001). The second type is asymmetrical cell differentiation, in which the asymmetric division of epidermal stem cells in the later meristems produces two different sizes of epidermal cells. Only the short epidermal cells can differentiate into root hairs. This type of differentiation mainly exists in some monocotyledons, primitive angiosperms, and nymphaeaceae plants (Kim and Dolan, 2011). The root hair cell fate determination method of Arabidopsis belongs to the third type, which is determined by the position effect. The epidermal cells in contact with two cortical cells (H-type cells) can develop to form root hairs. However, the epidermal cells connected to only one cortical cell (N-type cells) can often only develop into non-hair cells (Dolan et al., 1994).
The initiation of root hair refers to the process in which one side of the cell wall of the root epidermal cell with a special fate begins to expand, and the cell gradually widens and grows to form a tubular bulge. After the root hair cell completes the bulging process, it enters the elongation growth stage. When the root hair grows to a certain extent, the root hair will stop growing and enter the mature stage (Bibikova and Gilroy, 2003; Datta et al., 2011).
Root hair cell fate determination, root hair initiation, and root hair elongation are regulated by different genes. The molecular mechanism of root hair cell fate determination in the dicotyledonous model plant Arabidopsis has been relatively clear. The genes involved in root hair cell fate determination in root epidermal cells include TRANSPARENT TESTA GLABRA (TTG), GLABRA3 (GL3), ENHANCER OF GLABRA3 (EGL3), WEREWOLF (WER), GLABRA2 (GL2) and CAPRICE (CPC), and its homologs TRIPTYCHON (TRY) and ENHANCER OF TRY AND CPCs (ETC1; Figure 1; Table 1; Galway et al., 1994; Rerie et al., 1994; Wada et al., 1997; Lee and Schiefelbein, 1999; Bernhardt et al., 2003; Shibata and Sugimoto, 2019). WER, GL3, EGL3, and TTG in N-type cells form a transcription complex, WER-GL3/EGL3-TTG, which accumulates in large amounts and can induce the expression of GL2 and CPC. GL2 is a negative regulator of root hair growth and development and determines the differentiation of N-type cells (Rerie et al., 1994). CPC can move laterally from N-type cells to adjacent H-type cells (Wada et al., 1997). In H-type cells, CPC competes with WER to bind to the complex GL3/EGL3-TTG to inhibit the expression of GL2 and promote the differentiation of H-type cells (Shibata and Sugimoto, 2019). In addition, TRY and ETC1 play a functionally redundant role with CPC in H-type cells (Kirik et al., 2004; Simon et al., 2007). Downstream of GL2, bHLH transcription factors are involved in the regulation of root hair growth and development. ROOT HAIR DEFECTIVE 6 (RHD6; Figure 2; Table 1) is a class I member of the Group VIII subfamily of bHLH, and plays a key role in regulating root hair initiation. In addition, RHD6 and its homolog RHD6 LIKE1 (RSL1) form a complex to regulate the expression of RSL2 and RSL4, which are the secondary members of the Group VIII subfamily of bHLH. These secondary members play an active role in the process of root hair elongation (Masucci and Schiefelbein, 1994; Yi et al., 2010; Bruex et al., 2012; Shibata and Sugimoto, 2019). SCRAMBLED (SCM; Figure 1; Table 1) is a leucine-rich repeat receptor-like kinase (LRR-RLK), which can be specifically activated by as yet unidentified signals from the junction of two cortical cells. Activated SCM can promote the differentiation of H-type cells by reducing the accumulation of WER (Kwak and Schiefelbein, 2008). In contrast, only few advances in the molecular mechanism of root hair growth and development in crops have been made. It has been reported that rice genes, including OsEXPB2 (Zou et al., 2015), OsEXPA17 (Yu et al., 2011), OsCSLD1 (Kim et al., 2007), OsRHL1, and OsFH1 are involved in regulating rice root hair growth and development (Table 1; Ding et al., 2009; Huang et al., 2013). The RTH family genes in maize (Wen et al., 2005; Nestler et al., 2014) and the genes HvEXPB1 and HvEXPB7 in barely (Kwasniewski and Szarejko, 2006; He et al., 2015) are also involved in regulating the growth and development of root hair. However, there have been few studies on the mechanism of root hair growth and development in crop plants, including rice, maize, and barley, and the exact molecular regulatory network and regulatory mechanism of root hair growth and development are still unclear.
Figure 1. The regulation pattern of phytohormones on root hair cell fate determination in Arabidopsis. In N-type cells, WEREWOLF (WER), GLABRA3 (GL3), ENHANCER OF GLABRA3 (EGL3), and TRANSPARENT TESTA GLABRA (TTG) form a transcription complex WER-GL3/EGL3-TTG, which can induce the expression of GLABRA2 (GL2) and CAPRICE (CPC) in N-type cells. GL2 determines the differentiation of N-type cells, and CPC can move laterally from N-type cells to neighboring H-type cells. BR is able to upregulate the expression of WER. In H-type cells, SCRAMBLED (SCM) can be specifically activated by indeterminate signals from the junction of two cortical cells, and this activated SCM can inhibit the accumulation of WER. CPC competes with WER to bind the complex GL3/EGL3-TTG. CPC and its functionally redundant homologs TRIPTYCHON (TRY) and ENHANCER OF TRY AND CPCs (ETC1) inhibit the expression of GL2, which regulates the differentiation of H-type cells. Cytokinin (CK) and ethylene (ETH) promote CPC transcription through transcription factor ZINC FINGER PROTEIN 5 (ZFP5), auxin upregulates the expression of CPC, TRY, and ETC1, and BR promotes the expression of GL2 in H-type cells. ETH inhibits the transcription of GL2 in N-type cells. →, positive regulation; ┤, negative regulation; →, transmembrane transport; ·, unknown signal. N cell, N-type cell; H cell, H-type cell; SCM, SCRAMBLED; BR, brassinosteroid; ETH, ethylene; CK, cytokinin.
Table 1. The root hair growth and development-related genes in Arabidopsis and rice. –, negative regulation; +, positive regulation.
Figure 2. The regulation network of phytohormones on root hair initiation and elongation in Arabidopsis. GL2 inhibits the expression of ROOT HAIR DEFECTIVE 6 (RHD6) and RHD6 LIKE1 (RSL1), which are positive regulators of root hair initiation and elongation. RHD6 and RSL1 promote the expression of RSL2 and RSL4, which in turn promote root hair elongation by upregulating the expression of elongation genes. JAZ protein, a repressor of JA signal transduction, inhibits RHD6 transcription and interferes with the interaction between RHD6 and RSL1. JAZ protein also inhibits the transcription of EIN3 and EIL1 in the ethylene signaling pathway. EIN3/EIL1 can form a complex with RHD6/RSL1 to promote root hair initiation, and EIN3 binds to the promoter region of RSL4 to promote its transcription. The auxin response factor ARF5 also binds to the RSL4 promoter region to induce RSL4 expression, and the auxin response factor ARF7 and ARF19 bind to the promoter region of ERU to induce ERU expression. GA negatively regulates the expression of root hair elongation genes through DELLA-mediated signal transduction. ABA induces the accumulation of OBP4 protein, and OBP4 binds to the RSL2 promoter region to inhibit RSL2 expression. ABA also induces the expression of AtOXI1 and other root hair initiation-related genes by H2O2 production. CK can promote root hair elongation by promoting RSL4 expression. SL induces root hair elongation-related gene expression through the MAX2-mediated signal transduction pathway. BR negatively regulates the expression of root hair elongation-related genes. →, positive regulation; ┤, negative regulation. JA, jasmonate; ETH, ethylene; CK, cytokinin; GA, gibberellin; BR, brassinosteroid; SL, strigolactone; and ABA, abscisic acid.
Increasing evidences reveals that phytohormones play important roles in regulating the growth and development of plant root hair. Phytohormones are trace organic compounds produced by the plant that can regulate plant growth and development at very low concentrations. Phytohormones can function at the synthetic site or be transported via the vascular system to distal tissues or organs (Jaillais and Chory, 2010; Wang and Irving, 2011). This review introduces the current research regarding auxin, ethylene (ETH), jasmonic acid (JA), abscisic acid (ABA), gibberellin (GA), strigolactone (SL), cytokinin (CK), and brassinosteroid (BR) in regulating the growth and development of plant root hairs. In addition, this review also discusses some unsolved questions in the field of phytohormone regulation and the molecular regulatory network of phytohormones. Progress into this field will provide theoretical basis and technical guidance for cultivating plants with well-developed root hairs.
Auxin plays an essential role in the growth and development of plant root hair. Auxin is involved in regulating the determination of root hair cell fate and in promoting root hair initiation. Application of the exogenous auxin analogue naphthaleneacetic acid (NAA) can upregulate the expression of the CPC, TRY, and ETC1 genes, which can positively determine the fate of root hair cells (Figure 1; Niu et al., 2011). CPC, TRY, and ETC1 compete with WER to bind the complex GL3/EGL3-TTG, inhibit the expression of GL2, and thus produce more root hairs. The auxin transport inhibitor N-1-naphthylphthalamic acid (NPA) can increase the expression of the negative regulation genes (WER, GL3, GL2, and TTG) of root hair cell fate determination and reduce the expression of the TRY gene, which then inhibits root hair initiation (Niu et al., 2011). In addition, RHD6 positively regulates root hair initiation. Experimentally, the root hair density of the rhd6 mutant was determined to be significantly lower than that of the wild type, and exogenous auxin treatment increased the root hair density of rhd6, indicating that auxin acts downstream of RHD6 to regulate root hair initiation (Masucci and Schiefelbein, 1996).
Auxin is also involved in regulating the elongation of root hair. When compared to wild type Arabidopsis, the auxin-responsive mutant axr1-12 had no difference in the number of root hairs but had significantly diminished lengths of root hairs, which indicates that auxin is necessary for the elongation of root hairs (Pitts et al., 1998). Exogenous auxin treatment can induce the expression of RSL4 in roots and control the elongation of root hairs by regulating the expression of RSL4 (Yi et al., 2010). Mangano et al. (2017) demonstrated that AUXIN RESPONSE FACTOR 5 (ARF5) was able to bind directly to the promoter of RSL4 and induce the expression of RSL4 (Figure 2; Table 1). In addition, ERULUS (ERU), a member of the Catharanthus roseus RECEPTOR-LIKE KINASE1-LIKE subfamily, controls cell wall formation during the elongation of root hair. AUXIN RESPONSE FACTOR 7 (ARF7) and AUXIN RESPONSE FACTOR 19 (ARF19) are both able to bind to the promoter region of ERU and regulate its expression to control root hair elongation (Figure 2; Table 1; Schoenaers et al., 2018).
The regulation of auxin on the growth and development of root hair is regulated by auxin metabolism and polar auxin transport, which controls the local auxin level and forms a concentration gradient. The formation of this auxin concentration gradient is the main mechanism involved in regulating many life activities (Marchant et al., 2002; Cho et al., 2007). There are two pathways for indole-3-acetic acid (IAA) synthesis in plants: the tryptophan pathway and the non-tryptophan pathway. During the process of IAA synthesis through the tryptophan pathway, YUCCA is the rate-limiting enzyme for converting indole-3-pyruvate (IPA) into IAA and is involved in regulating the initiation and the elongation of root hair. Previous studies demonstrated that overexpression of yucca in Arabidopsis increased the content of the endogenous auxin compared with that in the wild type, and the root hair density and length of transgenic plants were higher than that of the wild type (Zhao et al., 2001; Zhao, 2010). In addition, overexpression of GmYUC2a, a member of YUCCA gene family of soybean, significantly increased soybean root hair density (Wang et al., 2019).
Previous studies demonstrated that the auxin influx carrier AUX1 plays an important regulatory role in regulating root hair elongation in rice. Although AUX1 is expressed in non-hair cells, but weakly or not at all in root hair cells (Jones et al., 2009), an AUX1 gene mutation led to a significant inhibition of root hair elongation (Yu et al., 2015). There are differences in the expression patterns of AUX1 in different plant roots. In addition, a mutation of the auxin efflux carrier gene PIN2 inhibited the transport of auxin from the root tip to the root hair zone and inhibited the transport of auxin from non-hair cells to root hair cells. This resulted in the lack of auxin in root hair cells and inhibited the initiation and the elongation of root hair in Arabidopsis (Cho et al., 2007). These results indicated that non-hair cells may supply auxin to root hair cells and transport auxin to root hair cells in an AUX1 and PIN2 dependent manner (Cho et al., 2007; Jones et al., 2009).
Ethylene is involved in regulating root hair cell fate determination and in promoting root hair initiation. The number of root hairs in ETH-insensitive Arabidopsis mutant etr1 is significantly lower than that in the wild type (Masucci and Schiefelbein, 1994). Exogenous application of the ETH biosynthesis inhibitor aminoethoxyvinylglycine (AVG) and the ETH action inhibitor Ag+ were able to significantly reduce the root hair density of the wild type, while the treatment of the ETH precursor 1-aminocyclopropane-1-carboxylic acid (ACC) increased the root hair density of the wild type (Masucci and Schiefelbein, 1994; Masucci and Schiefelbein, 1996; Dolan, 2001). Moreover, exogenous ACC treatment was also able to induce the formation of ectopic root hairs where non-treatment plants produced non-hair cells (Tanimoto et al., 1995). Recent study indicates that ETH induces the formation of ectopic root hairs by activating the transcription factor of ethylene signaling ETHYLENE INSENSITIVE 3 (EIN3)/EIN3-LIKE1 (EIL1) and reducing the transcription level of root hair growth inhibitor GL2 (Qiu et al., 2021). EIN3 affects the formation of the WER-GL3-TTG1 complex by competing with GL3 for binding to TTG1, which in turn reduces the transcription of GL2 by decreasing the formation of the WER-GL3-TTG1 complex (Qiu et al., 2021). These results suggest that ETH is involved in regulating the fate determination of root hair cells, but the underling molecular regulation mechanism remains unclear.
Previous studies have shown that ETH may participate in the regulation of root hair cell fate determination and initiation through the C2H2 zinc finger protein ZINC FINGER PROTEIN 5 (ZFP5; Figure 1; Table 1). ZFP5 is mainly expressed in the root and abundantly in root hair cells. The root hair density and root hair length of the ZFP5 mutant zfp5-4 were found to be lower than those of the wild type (An et al., 2012). Overexpression of CPC in the background of mutant zfp5-4 restored the root hair phenotype of zfp5-4 to the level of the wild type. The loss-of-function mutant cpc had nearly no root hair (An et al., 2012). However, overexpression of ZFP5 in the background of cpc was not able to restore the defective root hair phenotype of cpc, indicating that CPC acts downstream of ZFP5 to regulate root hair cell fate determination and root hair initiation. Further studies showed that ZFP5 could directly bind to the promoter region of CPC, promote CPC transcription, and then participate in the fate determination of root hair cells and regulate the initiation and the elongation of root hair (Figure 1; An et al., 2012).
It has been reported that the root hair density of ETH insensitive mutants etr1-1 and etr1-3 was lower than that of the wild type and that the root hair density of ETH overproduction mutant eto2 was higher than that of the wild type. In addition, the transcription of ZFP5 in etr1-1 and etr1-3 was downregulated, and the transcription of ZFP5 in eto2 was upregulated. These results suggest that ETH participates in the regulation of root hair cell fate determination and root hair initiation by inducing the expression of ZFP5 (An et al., 2012; Huang et al., 2020). Studies have also shown that when the fate determination mode of root hair cells is destroyed, the promoting effect of ETH on root hair initiation will be affected. When examining this, it was demonstrated that while the expression of CPC and TRY in root hair cells promoted the formation of root hairs, the number of root hairs of mutant cpc and double-mutant cpc try decreased significantly. Exogenous ETH treatment was able to significantly improve the root hair density of mutant cpc, but exogenous ETH treatment could not significantly improve the root hair density of double-mutant cpc try, indicating that ethylene can induce root hair initiation only when the CPC/TRY complex is functional or at least partially functional (Zhang et al., 2016).
Ethylene can promote the elongation of root hairs. The root hair length of the Arabidopsis ethylene-insensitive mutant etr1 was lower than that of the wild type, while the root hair length of the ethylene overproduction mutant eto1-1 was significantly longer than that of the wild type. Exogenous ACC treatment was able to increase the root hair length of the wild type and mutant etr1 (Masucci and Schiefelbein, 1994; Pitts et al., 1998). ZFP5 is also involved in the process of ETH-promoted root hair elongation. The root hair length of the mutant zfp5-4 was lower than that of the wild type. Exogenous ACC treatment was unable to restore the root hair length of zfp5-4, which indicates that ZFP5 is required for ETH to regulate Arabidopsis root hair elongation (An et al., 2012; Huang et al., 2020).
The key transcription factor EIN3 and its homologue EIL1 in the ethylene signaling pathway positively regulate the initiation and elongation of root hair (Figure 2; Table 1). The root hair density of the Arabidopsis double-mutant ein3 eil1 was lower than that of the wild type, indicating that EIN3/EIL1 is involved in regulating root hair initiation. The root hair length of ein3 eil1 was also shorter than that of the wild type, and it had no response to exogenous ACC treatment. These results indicate that EIN3/EIL1 is necessary for ETH to induce root hair elongation. EIN3 can directly bind to the promoter region of RSL4 and activate transcription. EIN3 can also interact with RHD6 to activate the expression of RSL4 and other related genes to promote root hair elongation (Feng et al., 2017).
In addition, MYB30, a member of MYB protein family, acts as a transcription factor in plants and negatively regulates the elongation of root hair (Table 1). MYB30 can directly bind to the promoter region of RSL4 and inhibit its transcription, which then inhibits root hair elongation. ETH can promote EIN3 and MYB30 to form a complex by reducing the association between MYB30 and the RSL4 promoter. This allows the transcription of RSL4 to be activated, which promotes root hair elongation (Xiao et al., 2021).
Ethylene can interact with auxin to regulate the initiation and elongation of root hair (Figure 2; Muday et al., 2012). Compared with the wild type, the root hair length of the auxin-insensitive mutant axr1 was significantly reduced, and exogenous ACC was able to restore the elongation of root hair. The ETH-insensitive mutant ein2-1 was inhibited in root hair elongation, and exogenous NAA treatment was able to effectively alleviate this inhibition. The ETH overproducing mutant eto1 had a long root hair phenotype, and the loss-of-function of AUX1 in the background of eto1 reduced its root hair length (Pitts et al., 1998; Strader et al., 2010; Muday et al., 2012). In addition, transcriptome analysis revealed that auxin and ETH were able to upregulate the expression of 90% of the genes related to root hair growth and development (Bruex et al., 2012). These results show that auxin and ETH can regulate the growth and development of root hair in an interactive manner.
Ethylene and auxin do not have a simple upstream and downstream regulatory relationship, but it may be a mutual regulation model. IAA promotes ETH synthesis. Exogenous ETH also promotes IAA synthesis and upregulates the expression of IAA transport related genes to promote auxin transport to epidermal cells in the root elongation zone (Tsuchisaka and Theologis, 2004; Růžička et al., 2007). However, auxin and ETH signals do not regulate root hair growth and development in a completely interdependent manner. For example, exogenous application of IAA can increase the percentage of root hair cells among all epidermal cells in the double-mutant aux1 etr1, but ACC treatment has no such effect (Masucci and Schiefelbein, 1996). The above results show that auxin and ETH regulate root hair growth and development of plants by complex interactions and that the mechanisms of these interactions need to be further studied.
Jasmonate plays a positive role in regulating the initiation and the elongation of root hair. It has been shown that exogenous treatment with appropriate concentration of JA and methyl jasmonate (MeJA) increased the root hair length and density in Arabidopsis. The increase in root hair density is induced by promoting more root hair cells to develop into root hair and by inducing the formation of branched hairs (Zhu et al., 2006). It has also been reported that exogenous application of MeJA significantly increased the root hair length. The COI1/JAZ-mediated JA signaling pathway is essential for the regulation of root hair elongation (Han et al., 2020). F-box protein COI1 is the receptor for JA, which positively regulates the JA response. JAZ proteins are repressors of JA signaling and can interact with a wide variety of transcription factors. The JAZ protein is degraded via the SCFCOI1-26S proteasome pathway. The JA signal is then activated by releasing downstream transcription factors (Xie et al., 1998; Chini et al., 2007). The root hairs of the loss-of-function mutation of COI1 and the JAZ overexpressed plants were much shorter than those of the wild type. The root hair length of the mutant containing five mutations in jazQ without JAZ function was longer than that of the wild type. The above results show that COI1/JAZ mediated JA signal transduction is necessary for root hair elongation. JAZ protein was able to interact with RHD6 and RSL1, inhibit the transcription of RHD6, and interfere with the interaction between RHD6 and RSL1 (Figure 2). The phenotypic analysis showed that JA was involved in the regulation of root hair growth and development in a RHD6 and RSL1 dependent manner (Han et al., 2020). In addition, overexpression of cotton GrTCP11 gene in Arabidopsis decreased the content of JA and the length of root hair compared with that of wild type. The results suggest that GrTCP11 may regulate root hair elongation by negatively regulating the biosynthesis of JA and the responses of Arabidopsis to JA (Hao et al., 2021). However, it remains elusive whether GrTCP11 regulates root hair elongation through a similar mechanism in cotton.
Jasmonate and ETH interact with each other to regulate the growth and development of root hairs. It has been reported that the promoting effect of JA on root hair initiation was weakened by ETH inhibitor (AVG or silver nitrate) and that the number of root hairs decreased significantly. Compared with the wild type, the promotion of MeJA and JA on root hair formation was also weakened in ETH insensitive mutants etr1-1 and etr1-3. In addition, JA biosynthesis inhibitors ibuprofen and salicylhydroxamic acid (SHAM) treatment were able to inhibit the induction effect of ACC on root hair formation and reduce the root hair density of the ETH overproduction mutant eto1-1. These results suggest that JA promotes root hair initiation through its interaction with ETH (Zhu et al., 2006).
The ETH-activated transcription factor EIN3 and its homologue EIL1 were also identified as direct downstream targets of JAZ protein in the JA signaling pathway (Figure 2). The root hair density and lengths of the ETH double-mutant ein3 eil1 were lower than those of the wild type. Exogenous application of JA was able to increase the root hair density and length of the wild type, but exogenous JA treatment was unable to increase the root hair density and length of ein3 eil1. Therefore, JA signal may positively regulate the fate determination and initiation of root hair cells through EIN3 and EIL1 (Zhu et al., 2011).
Previous research demonstrated that ABA positively regulates root hair cell fate determination and root hair initiation, but negative regulates root hair elongation. Exogenous ABA treatment increased root hair density not only by shortening the epidermal cells but also by inducing the formation of ectopic root hairs. However, the molecular mechanism by which ABA regulates root hair cell fate determination is still unclear (Lombardo and Lamattina, 2018). ABA plays a negative role in regulating root hair elongation. Exogenous ABA treatment leads to the shortening of root hair length of Arabidopsis seedlings. ABA induces the accumulation of OBF BINDING PROTEIN 4 (OBP4) protein, which directly inhibits the expression of RSL2 and inhibits root hair elongation (Figure 2; Table 1; Schnall and Quatrano, 1992; Rymen et al., 2017).
Abscisic acid regulating root hair initiation partially depends on the H2O2. Exogenous application of H2O2 has been found to induce the production of more root hairs. However, there were significantly fewer root hairs in Arabidopsis treated with ABA plus H2O2 scavenger ascorbic acid (ASA) than in those treated with ABA alone. This result indicates that H2O2 is involved in the regulation of ABA on the initiation of root hairs (Bai et al., 2007). Previous results revealed that H2O2 can directly induce the expression of AtOXI1, which can positively regulate root hair initiation and elongation (Figure 2; Table 1). The root hair density and length of the loss-of-function mutant atoxi1 were lower than those of the wild type. Exogenous ABA treatment was able to induce the expression of AtOXI1 and promote root hair initiation. These results indicate that ABA-induced root hair initiation is mediated by H2O2 through the regulation of AtOXI1 expression (Bai et al., 2007).
Previous studies demonstrated that ABA can positively regulate root hair initiation and root hair elongation in rice. Exogenous ABA treatment increased the area of the rice root hair zone, promoted the initiation of root hair, and then produced more root hair. ABA treatment also promoted rice root hair elongation (Chen et al., 2006; Wang et al., 2017). Further investigation indicated that ABA signal transduction may be involved in regulating rice root hair elongation. The OsABIL2 gene is a member of the PP2C family and is a negative regulator in the ABA signal transduction pathway in rice. Overexpression of OsABIL2 decreased the root hair length of transgenic rice compared with that of wild type rice (Table 1; Li et al., 2015a; Wang et al., 2017). SnRK2 is a positive regulator in the ABA signal transduction pathway, and OsSAPK10 is a member of SnRK2 subclass III. Overexpression of OsSAPK10 increased the root hair length of transgenic rice compared with that of wild type rice (Table 1; Wang et al., 2017; Yang et al., 2019).
Abscisic acid may participate in the regulation of rice root hair elongation by affecting auxin synthesis and polar auxin transport. It has been confirmed that ABA treatment can upregulate the expression of auxin synthesis genes including YUCCAs family members and auxin transport related genes such as OsAUX1 and OsPINs in rice roots (Table 1). ABA may regulate auxin content by regulating the dynamic balance of auxin synthesis and transport, which then regulates rice root hair elongation (Wang et al., 2017).
In conclusion, ABA interacts with auxin in regulating the growth and development of root hair. ABA regulation on root hair growth and development can differ between species. For instance, ABA positively regulates the root hair cell fate determination and root hair initiation, negatively regulates root hair elongation in Arabidopsis, and positively regulates root hair initiation and root hair elongation in rice.
Gibberellin positively regulates root hair initiation and negatively regulates root hair elongation. Previous results in Brassica campestris ssp. chinensis L. demonstrated that GA positively regulated root hair initiation. Exogenous GA3 treatment significantly increased the width of the root hair zone of Brassica campestris ssp. chinensis L. under saline alkali stress, and exogenous GA3 treatment increased the number of root hairs by promoting their initiation (Xu and Li, 2012).
Gibberellin negatively regulates root hair elongation (Figure 2). GA20-oxidase 2 (GA20ox2) is a rate-limiting enzyme of GA biosynthesis. The root hair length of transgenic Arabidopsis plants overexpressing the GA20ox2 gene was shorter than that of the wild type (Table 1). Moreover, under salt stress, the expression of GA20ox2 increased, GA4 accumulated, and root hair elongation was inhibited. The root hair length of transgenic plants with an overexpressed GA20ox2 gene was shorter than that of the wild type. A loss-of-function mutant ga20ox2-1 had longer root hairs than those of the wild type. These results suggest that GA20ox2 increases the content of endogenous active GA by promoting the synthesis of GA, which then negatively regulates the elongation of root hairs (Lv et al., 2018).
The inhibitory effect of GA on root hair elongation may be correlated to the DELLA protein. The DELLA protein is a negative regulator of GA signal transduction (Davière et al., 2008; Sun, 2011; Davière and Achard, 2013). The root hair length under low phosphorus conditions was much longer than that under normal culture conditions. Further investigation revealed that the content of bioactive GA decreased under low-phosphorus conditions, which leads to the accumulation of DELLA protein and inhibition of GA signal transduction (Jiang et al., 2007). These results indicate that GA negatively regulates root hair elongation through its biosynthesis and signal transduction.
Strigolactone has a positive regulatory effect on root hair elongation. MORE AXILLARY GROWTH2 (MAX2), an F-box protein, is an important component of the SL signal transduction pathway (Figure 2; Yao et al., 2017). Compared with the wild type, the root hair length of the SL signaling mutant max2-1 was significantly reduced. Application of GR24, a synthetic strigolactone analog, to the wild type and SL synthesis deficient mutants resulted in an increase in root hair length, but application of GR24 to SL-insensitive max2-1 did not restore its short root hair phenotype (Kapulnik et al., 2011a). These results indicated that SL promotes root hair elongation through MAX2-mediated signal transduction. In addition, overexpression of soybean MORE AXILLARY GROWTH3 (MAX3) in Arabidopsis orthologs’ mutants can restore the phenotype of the root hair and enhance root hair elongation (Ul Haq et al., 2017). It is still unclear whether SL is involved in regulating the fate determination and/or the initiation of root hair cells.
Strigolactone interacts with ETH to regulate root hair growth and development. An SL signal mutant is sensitive to ETH. Application of ETH precursor ACC to the SL-insensitive max2-1 was able to restore the short root hair of max2-1. In addition, the treatment of ETH biosynthesis inhibitor AVG was able to counteract the promoting effect of GR24 on root hair elongation of wild type plants. Therefore, ETH and SL may regulate root hair elongation in the same pathway, with ETH acting downstream of SL. ETH synthesis appears to be necessary for SL-mediated root hair elongation (Kapulnik et al., 2011b). Exogenous GR24 treatment can promote ETH biosynthesis by enhancing the transcription of ACS2, one of the rate-limiting enzymes of ETH biosynthesis, which then promotes root hair elongation (Table 1; Yamagami et al., 2003; Kapulnik et al., 2011b).
There are interactions between SL and auxin in regulating the growth and development of root hair. Application of IAA to the SL-insensitive mutant max2-1 restored its short root hair phenotype. The root hair length of the auxin-insensitive deficient mutant tir1-1 was shorter than that of the wild type. Exogenous GR24 treatment increased the root hair length of tir1-1. Simultaneous applications of IAA and GR24 to Arabidopsis resulted in a significant increase in the length of root hair, indicating that SL and auxin have an additive effect on promoting root hair elongation (Kapulnik et al., 2011b). The root hair density and length of tomato plant treated with excess GR24 were significantly lower than that of the control tomato plant. The inhibitory effect of excess GR24 on root hair density and length was counteracted by NPA, but not by exogenous IAA or NAA. Further investigation indicated that excessive SL in plants can promote the efflux of auxin, which decreases the auxin content of the plant, and thus inhibits the initiation and the elongation of root hair (Koltai et al., 2010). SL can also regulate the growth and development of root hair by regulating the expression of PINs (auxin polar transport genes) and TIR1 (auxin receptor gene; Table 1; Koltai et al., 2010; Mayzlish-Gati et al., 2012; Zhang et al., 2020).
Cytokinin plays a positive role in regulating the growth and development of root hair. Exogenous CK treatment can increase the density and the length of root hair (An et al., 2012; Zhang et al., 2016; Huang et al., 2020), but the underlying mechanism by which CK regulates root hair density and length is unclear. It has been reported that CK can regulate the fate determination, the initiation and the elongation of root hair cells through the C2H2 zinc finger protein ZFP5 (Figure 1; An et al., 2012). Exogenous synthetic CK 6-benzylaminopurine (6-BA) treatment was able to increase significantly the transcription level of ZFP5 and increase the density of root hair. CK may regulate the fate determination of root hair cells and promote root hair initiation by regulating the expression of ZFP5 (An et al., 2012). Exogenous CK treatment also caused an increase in the root hair length. Moreover, ZFP5 is involved in the process of CK promoting root hair elongation. The root hair length of the ZFP5 mutant zfp5-4 was lower than that of the wild type, and exogenous 6-BA treatment was unable to restore the root hair length of zfp5-4, indicating that ZFP5 is necessary for CK to regulate Arabidopsis root hair elongation (An et al., 2012). In addition, exogenous CK treatment was also able to significantly increase the root hair length and density of oilseed rape (Brassica napus L.) and alfalfa (Medicago sativa L.; Silverman et al., 1998; Li et al., 2014).
Cytokinin may regulate root hair elongation through auxin and the ETH-independent pathway. Application of 6-BA treatment to the ETH insensitive mutant etr1-1 and the auxin insensitive mutant axr1 resulted in significant increases in root hair length for the two mutants. These results indicated that CK can promote root hair elongation in the absence of auxin or ETH signal. CK is not necessary for root hair elongation, as auxin and ETH can maintain root hair elongation in its absence (Zhang et al., 2016). Treatment with CK, auxin, and ETH alone can regulate the expression of genes related to root hair growth and development, and then regulate root hair elongation. For example, treatment of the mutant rhd6 with CK, auxin, and ETH was able to induce the expression of RSL4, and then increase its root hair length (Masucci and Schiefelbein, 1994; Zhang et al., 2016).
Brassinosteroid plays a negative role in regulating root hair cell fate determination and initiation (Figures 1, 2). Compared with the wild type, the number of root hairs of a BR signal deficient mutant increased. Following BR signal enhancement, the number of root hairs decreased. The expression levels of WER and GL2 in the BR-insensitive mutant bri1 were lower than in the wild type, while exogenous BR treatment was able to induce the expression of WER and GL2 in bri1. BR can induce the expression of WER, which then promotes the formation of WER-GL3/EGL3-TTG complex, and consequently induces the expression of GL2 and the differentiation of N-type cells. Enhancing the BR signal can promote the expression of GL2 in root hair cells and inhibit the H-type cells from differentiating into root hair cells (Cheng et al., 2014; Wei and Li, 2016). However, the underlying molecular mechanism by which BR regulates the root hair cell fate determination is unclear and needs to be further studied.
Brassinosteroid regulation on root hair elongation differs among plant species. BR regulates root hair elongation in a concentration-dependent manner. It has been reported that BR negatively regulates root hair elongation and that the BR biosynthesis mutant det2 had longer root hair when compared to the wild type. Treatment with synthetic brassinosterol analogue 24-epibrassinolide (24-epiBL) reduced the root hair length of det2 to that of the wild type Arabidopsis. 24-epiBL treatment was able to significantly reduce the root hair length, while application of the BR synthesis inhibitor brassinazole (BRZ) promoted the elongation of root hair (Wang et al., 2010). Cell elongation has a great influence on the length of root hair, and the cell wall plasticity is an important determinant of cell elongation. Previous investigation revealed that, there, BR regulated root hair elongation in a cell-specific manner and that the overexpression of the BR receptor BRASSINOSTEROID INSENSITIVE1 (BRI1) in H-type cells promoted the elongation of root hair cells. The expression of BRI1 in non-hair cells inhibits cell elongation (Table 1). BRI1 in non-hair cells activates the expression of ETH biosynthesis gene, resulting in the increase of ETH and the accumulation of cellulose in the cell wall of non-hair cells, thus inhibiting cell elongation (Fridman et al., 2014). In rice, there is a dose effect of the regulation of BR on root hair elongation. The treatment with lower concentrations of 24-epiBL promoted root hair elongation of the wild type rice. Increasing the 24-epiBL treatment concentration reduced its promotion of root hair elongation. When the concentration of 24-epiBL treatment reaches 1 μmol/L, it inhibits root hair elongation (Wang et al., 2010; Zhao et al., 2016).
The difference of BR regulation of root hair elongation between Arabidopsis and rice may be due to species specificity. For Arabidopsis root hair elongation, the endogenous BR content may be superoptimal. Consequently, exogenous BR synthesis inhibitor treatment may promote root hair elongation by reducing BR content, while any exogenous BR treatment will lead to an excessive concentration of BR and inhibit Arabidopsis root hair elongation. In contrast, for rice root hair elongation, the endogenous BR content may be lower than the optimal concentration. Thus, treatment with low concentration of BR can promote the elongation of rice root hair; however, treatment with high concentration of BR may lead to a superoptimal concentration of BR within the rice plant, leading to inhibition of root hair elongation.
Brassinosteroid interacts with auxin in the regulation of root hair initiation and elongation. Exogenous application of 24-epiBL upregulated the expression level of AXR3/IAA17, which plays a repressive role in auxin signal transduction. The initiation and the elongation of root hairs of transgenic plants overexpressing AXR3/IAA17 were significantly inhibited (Table 1). In addition, the expression level of AXR3/IAA17 in the BR-insensitive mutant bri1 and the BR biosynthetic mutant det2 decreased. These results suggest that BR may inhibit auxin signal transduction by promoting the expression of AXR3/IAA17, which then inhibits Arabidopsis root hair initiation and elongation (Kim et al., 2006). Additionally, exogenous application of BR can increase the length and the density of root hair by enhancing the transport of auxin via the regulation of AcPIN and AcAUX1/LAX in kiwifruit (Wu et al., 2021). These results indicate that there are interactions between BR and auxin in regulating the initiation and elongation of root hair.
Plant root hair can not only increase the absorption efficiency of water and nutrients in plants but can also enhance stress resistance and play an important role in regulating plant growth and development (Peterson and Farquhar, 1996; Bibikova and Gilroy, 2003; Datta et al., 2011; Vissenberg et al., 2020). Previous studies revealed that enhanced auxin transport under salt stress is able to promote the root hair growth and improve salt resistance of Arabidopsis (Fu et al., 2021). ABA accumulation can enhance drought resistance of barely by promoting root hair elongation (Zhang et al., 2021). In addition, elevated auxin concentration can enhance the resistance of tomato plant to early blight by promoting root hair production (Abdel-Motaal et al., 2020). Although it has been reported that WER, TTG, GL3, GL2, and CPC genes are involved in regulating the growth and development of root hair (Figures 1, 2), other genes that can directly regulate the growth and development of root hair have not yet been reported. Therefore, it is of great significance to identify genes that are involved in regulating root hair growth and development, and to investigate the regulatory functions of these unknown genes on root hair growth and development. Further work into this will make an important contribution to our full understanding of the molecular regulatory mechanism of root hair growth and development.
Although many important research advances have been made in the regulation of phytohormones on root hair initiation and elongation, there have been few studies on the regulation of phytohormones on root hair cell fate determination. It has not yet been reported whether JA, GA, and SL can regulate the root hair cell fate determination. The regulatory mechanism and effect of these phytohormones on the fate determination of root hair cells are still unclear.
There are complex interactions between phytohormones, which serve to jointly regulate the growth and development of root hair. Although it has been reported that JA, ABA, SL, and BR can regulate root hair growth and development by interacting with auxin or ETH, the mechanism of interaction remains to be fully described. For example, although previous results demonstrated that JA is able to mediate lateral root formation and taproot growth by regulating auxin synthesis, polar auxin transport, and signal transduction (Sun et al., 2009, 2011; Hentrich et al., 2013), it is unclear whether JA can also mediate root hair growth and development by regulating auxin synthesis, polar transport, and signal transduction. The molecular mechanism of the interaction between JA and auxin that regulates root hair growth and development needs to be further studied.
The molecular mechanism of auxin, SL, and GA in regulating root hair growth and development needs to be further studied. Previous studies revealed that auxin can promote rice internode elongation by promoting GA synthesis and inhibiting GA inactivation, which maintains a high content of active GA (Yin et al., 2007). GA can regulate root growth by affecting auxin transport and signal transduction, and auxin can also promote root growth by regulating the response of the root to GA (Fu and Harberd, 2003; Li et al., 2015b). However, during the regulatory process of root hair growth and development, the effect of the interaction between auxin and GA has not been reported, and the mechanism of interaction between auxin and GA on root hair growth and development is unclear. SL can regulate stem elongation by mediating GA metabolism and signal transduction (Zou et al., 2019), but the molecular mechanism of the interaction between SL and GA on root hair growth and development is not clear.
It has been reported that CK can promote ETH synthesis by increasing the stability of the rate-limiting enzyme ACS for ethylene biosynthesis (Hansen et al., 2009). The CK-induced ETH plays an important role in regulating the elongation of rice seminal root (Zou et al., 2018). However, it is unclear whether CK and ETH can interact to regulate root hair growth and development. In addition, it is unclear whether there are any interactions between JA, ABA, SL, BR, GA, and/or other phytohormones during the process of root hair growth and development and, if so, the molecular mechanism of these phytohormone interactions on root hair growth and development need to be further studied.
In addition, most of the current studies focus on the dicotyledonous model plant Arabidopsis, and there have been few studies on the regulation of phytohormones on the growth and development of root hairs of rice and other crops. In future research, identifying novel genes involved in regulating the growth and development of crop root hairs, investigating the biological functions of these genes, and exploring the regulatory effect and regulatory mechanism of phytohormones on root hair growth and development will provide theoretical basis and technical guidance for cultivating crops with developed root hairs. After exploring the new functions of known genes and identifying novel genes, it is possible to cultivate crops with well-developed root hairs by using the technologies including gene editing and genetic improvement. Cultivating and planting crops with well-developed root hairs can increase the efficiency of water and nutrient absorption by crops and enhance crop stress resistance, thereby increasing crop yields and ensuring world food security.
ML and CY conceptualized this manuscript and designed the graphs and table. ML wrote the original draft. YZ helped to write and revise the manuscript. YZ and CY revised and reviewed the format. SL and WZ assisted with the edited version. CY obtained funding. CY and YL contributed to final approval of the manuscript. All authors contributed to the article and approved the submitted version.
This work was funded by the National Key Research and Development Program of China (No. 2016YFD0300102), the Science and Technology Major Projects of Guangxi Province of China (GuiKeChuang 010106), and the Postdoctoral Science Foundation of China (No. 2016T90705).
The authors declare that the research was conducted in the absence of any commercial or financial relationships that could be construed as a potential conflict of interest.
All claims expressed in this article are solely those of the authors and do not necessarily represent those of their affiliated organizations, or those of the publisher, the editors and the reviewers. Any product that may be evaluated in this article, or claim that may be made by its manufacturer, is not guaranteed or endorsed by the publisher.
Abdel-Motaal, F., Kamel, N., El-Zayat, S., and Abou-Ellail, M. (2020). Early blight suppression and plant growth promotion potential of the endophyte Aspergillus flavus in tomato plant. Ann. Agric. Sci. 65, 117–123. doi: 10.1016/j.aoas.2020.07.001
An, L., Zhou, Z., Sun, L., Yan, A., Xi, W., Yu, N., et al. (2012). A zinc finger protein gene ZFP5 integrates phytohormone signaling to control root hair development in Arabidopsis. Plant J. 72, 474–490. doi: 10.1111/j.1365-313X.2012.05093.x
Bai, L., Zhou, Y., Zhang, X., Song, C., and Cao, M. (2007). Hydrogen peroxide modulates abscisic acid signaling in root growth and development in Arabidopsis. Chin. Sci. Bull. 52, 1142–1145. doi: 10.1007/s11434-007-0179-z
Bernhardt, C., Lee, M. M., Gonzalez, A., Zhang, F., Lloyd, A., and Schiefelbein, J. (2003). The bHLH genes GLABRA3 (GL3) and ENHANCER OF GLABRA3 (EGL3) specify epidermal cell fate in the Arabidopsis root. Development 130, 6431–6439. doi: 10.1242/dev.00880
Bibikova, T., and Gilroy, S. (2003). Root hair development. J. Plant Growth Regul. 21, 383–415. doi: 10.1007/s00344-003-0007-x
Bruex, A., Kainkaryam, R. M., Wieckowski, Y., Kang, Y. H., Bernhardt, C., Xia, Y., et al. (2012). A gene regulatory network for root epidermis cell differentiation in Arabidopsis. PLoS Genet. 8, e1002446–e1002420. doi: 10.1371/journal.pgen.1002446
Chen, C.-W., Yang, Y.-W., Lur, H.-S., Tsai, Y.-G., and Chang, M.-C. (2006). A novel function of abscisic acid in the regulation of rice (oryza sativa L.) root growth and development. Plant Cell Physiol. 47, 1–13. doi: 10.1093/pcp/pci216
Cheng, Y., Zhu, W., Chen, Y., Ito, S., Asami, T., and Wang, X. (2014). Brassinosteroids control root epidermal cell fate via direct regulation of a MYB-bHLH-WD40 complex by GSK3-like kinases. Elife 3:e02525. doi: 10.7554/eLife.02525
Chini, A., Fonseca, S., Fernández, G., Adie, B., Chico, J. M., Lorenzo, O., et al. (2007). The JAZ family of repressors is the missing link in jasmonate signalling. Nature 448, 666–671. doi: 10.1038/nature06006
Cho, M., Lee, O. R., Ganguly, A., and Cho, H. T. (2007). Auxin-signaling: short and long. J. Plant Biol. 50, 79–89. doi: 10.1007/BF03030615
Clowes, F. A. L. (2000). Pattern in root meristem development in angiosperms. New Phytol. 146, 83–94. doi: 10.2307/2588748
Datta, S., Kim, C. M., Pernas, M., Pires, N. D., Proust, H., Tam, T., et al. (2011). Root hairs: development, growth and evolution at the plant-soil interface. Plant Soil 346, 1–14. doi: 10.1007/s11104-011-0845-4
Davière, J. M., and Achard, P. (2013). Gibberellin signaling in plants. Development 140, 1147–1151. doi: 10.1242/dev.087650
Davière, J.-M., de Lucas, M., and Prat, S. (2008). Transcriptional factor interaction: a central step in DELLA function. Curr. Opin. Genet. Dev. 18, 295–303. doi: 10.1016/j.gde.2008.05.004
Ding, W., Yu, Z., Tong, Y., Huang, W., Chen, H., and Wu, P. (2009). A transcription factor with a bHLH domain regulates root hair development in rice. Cell Res. 19, 1309–1311. doi: 10.1038/cr.2009.109
Dolan, L. (2001). The role of ethylene in root hair growth in Arabidopsis. J. Plant Nutr. Soil Sci. 164, 141–145. doi: 10.1002/1522-2624(200104)164:2<141::AID-JPLN141>3.3.CO;2-Q
Dolan, L., Duckett, C. M., Grierson, C., Linstead, P., Schneider, K., Lawson, E., et al. (1994). Clonal relationships and cell patterning in the root epidermis of Arabidopsis. Development 8, 2241–2255. doi: 10.1101/gad.8.18.2241
Feng, Y., Xu, P., Li, B., Li, P., Wen, X., An, F., et al. (2017). Ethylene promotes root hair growth through coordinated EIN3/EIL1 and RHD6/RSL1 activity in Arabidopsis. Proc. Natl. Acad. Sci. U.S.A. 114, 13834–13839. doi: 10.1073/pnas.1711723115
Fridman, Y., Elkouby, L., Holland, N., Vragovic, K., Elbaum, R., and Savaldi-Goldstein, S. (2014). Root growth is modulated by differential hormonal sensitivity in neighboring cells. Genes Dev. 28, 912–920. doi: 10.1101/gad.239335.114
Fu, X., and Harberd, N. P. (2003). Auxin promotes Arabidopsis root growth by modulating gibberellin response. Nature 421, 740–743. doi: 10.1038/nature01387
Fu, J., Zhu, C., Wang, C., Liu, L., Shen, Q., Xu, D., et al. (2021). Maize transcription factor ZmEREB20 enhanced salt tolerance in transgenic Arabidopsis. Plant Physiol. Biochem. 159, 257–267. doi: 10.1016/j.plaphy.2020.12.027
Galway, M. E., Masucci, J. D., Lloyd, A. M., Walbot, V., Davis, R. W., and Schiefelbein, J. W. (1994). The TTG gene is required to specify epidermal cell fate and cell patterning in the Arabidopsis root. Dev. Biol. 166, 740–754. doi: 10.1006/dbio.1994.1352
Han, X., Zhang, M., Yang, M., and Hu, Y. (2020). Arabidopsis JAZ proteins interact with and suppress RHD6 transcription factor to regulate jasmonate-stimulated root hair development. Plant Cell 32, 1049–1062. doi: 10.1105/tpc.19.00617
Hansen, M., Chae, H. S., and Kieber, J. J. (2009). Regulation of ACS protein stability by cytokinin and brassinosteroid. Plant J. 57, 606–614. doi: 10.1111/j.1365-313X.2008.03711.x
Hao, J., Lou, P., Han, Y., Chen, Z., Chen, J., Ni, J., et al. (2021). GrTCP11, a cotton TCP transcription factor, inhibits root hair elongation by down-regulating jasmonic acid pathway in Arabidopsis thaliana. Front. Plant Sci. 12:769675. doi: 10.3389/fpls.2021.769675
He, X., Zeng, J., Cao, F., Ahmed, I. M., Zhang, G., Vincze, E., et al. (2015). HvEXPB7, a novel β-expansin gene revealed by the root hair transcriptome of Tibetan wild barley, improves root hair growth under drought stress. J. Exp. Bot. 66, 7405–7419. doi: 10.1093/jxb/erv436
Hentrich, M., Bottcher, C., Duchting, P., Cheng, Y. F., Zhao, Y. D., Berkowitz, O., et al. (2013). The jasmonic acid signaling pathway is linked to auxin homeostasis through the modulation of YUCCA8 and YUCCA9 gene expression. Plant J. 74, 626–637. doi: 10.1111/tpj.12152
Huang, L., Jiang, Q., Wu, J., An, L., Zhou, Z., Wong, C., et al. (2020). Zinc finger protein 5 (ZFP5) associates with ethylene signaling to regulate the phosphate and potassium deficiency-induced root hair development in Arabidopsis. Plant Mol. Biol. 102, 143–158. doi: 10.1007/s11103-019-00937-4
Huang, J., Kim, C. M., Xuan, Y.-H., Liu, J., Kim, T. H., Kim, B.-K., et al. (2013). Formin homology 1 (OsFH1) regulates root-hair elongation in rice (Oryza sativa). Planta 237, 1227–1239. doi: 10.1007/s00425-013-1838-8
Jaillais, Y., and Chory, J. (2010). Unraveling the paradoxes of plant hormone signaling integration. Nat. Struct. Mol. Biol. 17, 642–645. doi: 10.1038/nsmb0610-642
Jiang, C., Gao, X., Liao, L., Harberd, N. P., and Fu, X. (2007). Phosphate starvation root architecture and anthocyanin accumulation responses are modulated by the gibberellin-DELLA signaling pathway in Arabidopsis. Plant Physiol. 145, 1460–1470. doi: 10.1104/pp.107.103788
Jones, A. R., Kramer, E. M., Knox, K., Swarup, R., Bennett, M. J., Lazarus, C. M., et al. (2009). Auxin transport through non-hair cells sustains root-hair development. Nat. Cell Biol. 11, 78–84. doi: 10.1038/ncb1815
Kapulnik, Y., Delaux, P.-M., Resnick, N., Mayzlish-Gati, E., Wininger, S., Bhattacharya, C., et al. (2011a). Strigolactones affect lateral root formation and root-hair elongation in Arabidopsis. Planta 233, 209–216. doi: 10.1007/s00425-010-1310-y
Kapulnik, Y., Resnick, N., Mayzlish-Gati, E., Kaplan, Y., Wininger, S., Hershenhorn, J., et al. (2011b). Strigolactones interact with ethylene and auxin in regulating root-hair elongation in Arabidopsis. J. Exp. Bot. 62, 2915–2924. doi: 10.1093/jxb/erq464
Kim, C. M., and Dolan, L. (2011). Root hair development involves asymmetric cell division in Brachypodium distachyon and symmetric division in Oryza sativa. New Phytol. 192, 601–610. doi: 10.1111/j.1469-8137.2011.03839.x
Kim, H., Park, P.-J., Hwang, H.-J., Lee, S.-Y., Oh, M.-H., and Kim, S.-G. (2006). Brassinosteroid signals control expression of the AXR3/IAA17 gene in the cross-talk point with auxin in root development. Biosci. Biotechnol. Biochem. 70, 768–773. doi: 10.1271/bbb.70.768
Kim, C. M., Park, S. H., Il Je, B., Park, S. H., Park, S. J., Piao, H. L., et al. (2007). OsCSLD1, a cellulose synthase-Like D1 gene, is required for root hair morphogenesis in rice. Plant Physiol. 143, 1220–1230. doi: 10.1104/pp.106.091546
Kirik, V., Simon, M., Huelskamp, M., and Schiefelbein, J. (2004). The ENHANCER OF TRY AND CPC1 gene acts redundantly with TRIPTYCHON and CAPRICE in trichome and root hair cell patterning in Arabidopsis. Dev. Biol. 268, 506–513. doi: 10.1016/j.ydbio.2003.12.037
Koltai, H., Dor, E., Hershenhorn, J., Joel, D. M., Weininger, S., Lekalla, S., et al. (2010). Strigolactones' effect on root growth and root-hair elongation may be mediated by auxin-efflux carriers. J. Plant Growth Regul. 29, 129–136. doi: 10.1007/s00344-009-9122-7
Kwak, S. H., and Schiefelbein, J. (2008). A feedback mechanism controlling SCRAMBLED receptor accumulation and cell-type pattern in Arabidopsis. Curr. Biol. 18, 1949–1954. doi: 10.1016/j.cub.2008.10.064
Kwasniewski, M., and Szarejko, I. (2006). Molecular cloning and characterization of β-expansin gene related to root hair formation in barley. Plant Physiol. 141, 1149–1158. doi: 10.2307/20205836
Lee, M. M., and Schiefelbein, J. (1999). WEREWOLF, a MYB-related protein in Arabidopsis, is a position-dependent regulator of epidermal cell patterning. Cell 99, 473–483. doi: 10.1016/S0092-8674(00)81536-6
Li, C., Shen, H., Wang, T., and Wang, X. (2015a). ABA regulates subcellular redistribution of OsABI-LIKE2, a negative regulator in ABA signaling, to control root architecture and drought resistance in Oryza sativa. Plant Cell Physiol. 56, 2396–2408. doi: 10.1093/pcp/pcv154
Li, D., Shi, T., Yuan, P., Feng, Y., and Shi, L. (2014). Differences in root microscopic structure of root mutants lrn1, prl1 and wild type in oilseed rape (Brassica napus L.). Plant Sci. J. 32, 406–412. doi: 10.3724/SP.J.1142.2014.40406
Li, G., Zhu, C., Gan, L., Ng, D., and Xia, K. (2015b). GA3 enhances root responsiveness to exogenous IAA by modulating auxin transport and signalling in Arabidopsis. Plant Cell Rep. 34, 483–494. doi: 10.1007/s00299-014-1728-y
Lombardo, M. C., and Lamattina, L. (2018). Abscisic acid and nitric oxide modulate cytoskeleton organization, root hair growth and ectopic hair formation in Arabidopsis. Nitric Oxide - Biol. Chem. 80, 89–97. doi: 10.1016/j.niox.2018.09.002
Lv, S., Yu, D., Sun, Q., and Jiang, J. (2018). Activation of gibberellin 20-oxidase 2 undermines auxin-dependent root and root hair growth in NaCl-stressed Arabidopsis seedlings. Plant Growth Regul. 84, 225–236. doi: 10.1007/s10725-017-0333-9
Mangano, S., Denita-Juarez, S. P., Choi, H.-S., Marzol, E., Hwang, Y., Ranocha, P., et al. (2017). Molecular link between auxin and ROS-mediated polar growth. Proc. Natl. Acad. Sci. U.S.A. 114, 5289–5294. doi: 10.1073/pnas.1701536114
Marchant, A., Bhalerao, R., Casimiro, I., Eklöf, J., Casero, P. J., Bennett, M., et al. (2002). AUX1 promotes lateral root formation by facilitating indole-3-acetic acid distribution between sink and source tissues in the Arabidopsis seedling. Plant Cell 14, 589–597. doi: 10.1105/tpc.010354.2
Masucci, J. D., and Schiefelbein, J. W. (1994). The rhd6 mutation of Arabidopsis thaliana alters root-hair initiation through an auxin- and ethylene-associated process. Plant Physiol. 106, 1335–1346. doi: 10.1104/pp.106.4.1335
Masucci, J. D., and Schiefelbein, J. W. (1996). Hormones act downstream of TTG and GL2 to promote root hair outgrowth during epidermis development in the Arabidopsis root. Plant Cell 8, 1505–1517. doi: 10.2307/3870246
Mayzlish-Gati, E., De-Cuyper, C., Goormachtig, S., Beeckman, T., Vuylsteke, M., Brewer, P. B., et al. (2012). Strigolactones are involved in root response to low phosphate conditions in Arabidopsis. Plant Physiol. 160, 1329–1341. doi: 10.1104/pp.112.202358
Muday, G. K., Rahman, A., and Binder, B. M. (2012). Auxin and ethylene: collaborators or competitors? Trends Plant Sci. 17, 181–195. doi: 10.1016/j.tplants.2012.02.001
Nestler, J., Liu, S., Wen, T.-J., Paschold, A., Marcon, C., Tang, H. M., et al. (2014). Roothairless5, which functions in maize (Zea mays L.) root hair initiation and elongation encodes a monocot-specific NADPH oxidase. Plant J. 79, 729–740. doi: 10.1111/tpj.12578
Niu, Y., Jin, C., Jin, G., Zhou, Q., Lin, X., Tang, C., et al. (2011). Auxin modulates the enhanced development of root hairs in Arabidopsis thaliana (L.) Heynh. Under elevated CO2. Plant Cell Environ. 34, 1304–1317. doi: 10.1111/j.1365-3040.2011.02330.x
Pemberton, L. M. S., Tsai, S.-L., Lovell, P. H., and Harris, P. J. (2001). Epidermal patterning in seedling roots of Eudicotyledons. Ann. Bot. 87, 649–654. doi: 10.1006/anbo.2001.1385
Peterson, R. L., and Farquhar, M. L. (1996). Root hairs: specialized tubular cells extending root surfaces. Bot. Rev. 62, 1–40. doi: 10.1007/bf02868919
Pitts, R. J., Cernac, A., and Estelle, M. (1998). Auxin and ethylene promote root hair elongation in Arabidopsis. Plant J. 16, 553–560. doi: 10.1046/j.1365-313x.1998.00321.x
Qiu, Y., Tao, R., Feng, Y., Xiao, Z., Zhang, D., and Peng, Y. (2021). EIN3 and RSL4 interfere with an MYB–bHLH–WD40 complex to mediate ethylene-induced ectopic root hair formation in Arabidopsis. Proc. Natl. Acad. Sci. U.S.A. 118:e2110004118. doi: 10.1073/pnas.2110004118
Rerie, W. G., Feldmann, K. A., and Marks, M. D. (1994). The GLABRA2 gene encodes a homeo domain protein required for normal trichome development in Arabidopsis. Genes Dev. 8, 1388–1399. doi: 10.1101/gad.8.12.1388
Růžička, K., Ljung, K., Vanneste, S., Podhorská, R., Beeckman, T., Friml, J., et al. (2007). Ethylene regulates root growth through effects on auxin biosynthesis and transport-dependent auxin distribution. Plant Cell 19, 2197–2212. doi: 10.1105/tpc.107.052126
Rymen, B., Kawamura, A., Schäfer, S., Breuer, C., Iwase, A., Shibata, M., et al. (2017). ABA suppresses root hair growth via the OBP4 transcriptional regulator. Plant Physiol. 173, 1750–1762. doi: 10.1104/pp.16.01945
Schnall, J. A., and Quatrano, R. S. (1992). Abscisic acid elicits the water-stress response in root hairs of Arabidopsis thaliana. Plant Physiol. 100, 216–218. doi: 10.1104/pp.100.1.216
Schoenaers, S., Balcerowicz, D., Breen, G., Hill, K., Zdanio, M., Mouille, G., et al. (2018). The auxin-regulated CrRLK1L kinase ERULUS controls cell wall vomposition during root hair tip growth. Curr. Biol. 28, 722–732.e6. doi: 10.1016/j.cub.2018.01.050
Shibata, M., and Sugimoto, K. (2019). A gene regulatory network for root hair development. J. Plant Res. 132, 301–309. doi: 10.1007/s10265-019-01100-2
Silverman, F. P., Assiamah, A. A., and Bush, D. S. (1998). Membrane transport and cytokinin action in root hairs of Medicago sativa. Planta 205, 23–31. doi: 10.2307/23385243
Simon, M., Lee, M. M., Lin, Y., Gish, L., and Schiefelbein, J. (2007). Distinct and overlapping roles of single-repeat MYB genes in root epidermal patterning. Dev. Biol. 311, 566–578. doi: 10.1016/j.ydbio.2007.09.001
Strader, L. C., Chen, G. L., and Bartel, B. (2010). Ethylene directs auxin to control root cell expansion. Plant J. 64, 874–884. doi: 10.1111/j.1365-313X.2010.04373.x
Sun, T. (2011). The molecular mechanism and evolution of the GA–GID1–DELLA signaling module in plants. Curr. Biol. 21, R338–R345. doi: 10.1016/j.cub.2011.02.036
Sun, J., Chen, Q., Qi, L., Jiang, H., Li, S., Xu, Y., et al. (2011). Jasmonate modulates endocytosis and plasma membrane accumulation of the Arabidopsis PIN2 protein. New Phytol. 191, 360–375. doi: 10.1111/j.1469-8137.2011.03713.x
Sun, J., Xu, Y., Ye, S., Jiang, H., Chen, Q., Liu, F., et al. (2009). Arabidopsis ASA1 is important for jasmonate-mediated regulation of auxin biosynthesis and transport during lateral root formation. Plant Cell 21, 1495–1511. doi: 10.1105/tpc.108.064303
Tanimoto, M., Roberts, K., and Dolan, L. (1995). Ethylene is a positive regulator of root hair development in Arabidopsis thaliana. Plant J. 8, 943–948. doi: 10.1046/j.1365-313X.1995.8060943.x
Tsuchisaka, A., and Theologis, A. (2004). Unique and overlapping expression patterns among the Arabidopsis 1-amino-cyclopropane-1-carboxylate synthase gene family members. Plant Physiol. 136, 2982–3000. doi: 10.1104/pp.104.049999
Ul Haq, B., Ahmad, M. Z., Rehman, N. U., Wang, J., Li, P., Li, D., et al. (2017). Functional characterization of soybean strigolactone biosynthesis and signaling genes in Arabidopsis MAX mutants and GmMAX3 in soybean nodulation. BMC Plant Biol. 17:259. doi: 10.1186/s12870-017-1182-4
Vissenberg, K., Claeijs, N., Balcerowicz, D., and Schoenaers, S. (2020). Hormonal regulation of root hair growth and responses to the environment in Arabidopsis. J. Exp. Bot. 71, 2412–2427. doi: 10.1093/jxb/eraa048
Wada, T., Tachibana, T., Shimura, Y., and Okada, K. (1997). Epidermal cell differentiation in Arabidopsis determined by a Myb homolog, CPC. Science 277, 1113–1116. doi: 10.1126/science.277.5329.1113
Wang, Y. H., and Irving, H. R. (2011). Developing a model of plant hormone interactions. Plant Signal. Behav. 6, 494–500. doi: 10.4161/psb.6.4.14558
Wang, T., Li, C., Wu, Z., Jia, Y., Wang, H., Sun, S., et al. (2017). Abscisic acid regulates auxin homeostasis in rice root tips to promote root hair elongation. Front. Plant Sci. 8:1121. doi: 10.3389/fpls.2017.01121
Wang, F., Shi, C., and Dong, J. (2010). The response of root hair on Brassinosteroids in Arabidopsis and rice. J. Agri. Univ. Hebei 33, 105–109. doi: 10.3969/j.issn.1000-1573.2010.06.021
Wang, Y., Yang, W., Zuo, Y., Zhu, L., Hastwell, A. H., Chen, L., et al. (2019). GmYUC2a mediates auxin biosynthesis during root development and nodulation in soybean. J. Exp. Bot. 70, 3165–3176. doi: 10.1093/jxb/erz144
Wei, Z., and Li, J. (2016). Brassinosteroids regulate root growth, development, and symbiosis. Mol. Plant 9, 86–100. doi: 10.1016/j.molp.2015.12.003
Wen, T.-J., Hochholdinger, F., Sauer, M., Bruce, W., and Schnable, P. S. (2005). The roothairless1 gene of maize encodes a homolog of sec3, which is involved in polar exocytosis. Plant Physiol. 138, 1637–1643. doi: 10.1104/pp.105.062174
Wu, Z., Gu, S., Gu, H., Cheng, D., Li, L., Guo, X., et al. (2021). Physiological and transcriptomic analyses of brassinosteroid function in kiwifruit root. Environ. Exp. Bot. 194:104685. doi: 10.1016/j.envexpbot.2021.104685
Xiao, F., Gong, Q., Zhao, S., Lin, H., and Zhou, H. (2021). MYB30 and ETHYLENE INSENSITIVE3 antagonistically modulate root hair growth in Arabidopsis. Plant J. 106, 480–492. doi: 10.1111/tpj.15180
Xie, D.-X., Feys, B. F., James, S., Nieto-Rostro, M., and Turner, J. G. (1998). COI1: An Arabidopsis gene required for jasmonate-regulated defense and fertility. Science 280, 1091–1094. doi: 10.1126/science.280.5366.1091
Xu, F., and Li, T. (2012). Effects of exogenous GA3 on seed germination of Brassica campestris ssp. chinensis L. under mixed salt stress. Chin. J. Bioprocess Engineer. 10, 56–59. doi: 10.3969/j.issn.1672-3678.2012.06.012
Yamagami, T., Tsuchisaka, A., Yamada, K., Haddon, W. F., Harden, L. A., and Theologis, A. (2003). Biochemical diversity among the 1-amino-cyclopropane-1-carboxylate synthase isozymes encoded by the Arabidopsis gene family. J. Biol. Chem. 278, 49102–49112. doi: 10.1074/jbc.M308297200
Yang, G., Yu, Z., Gao, L., and Zheng, C. (2019). SnRK2s at the crossroads of growth and stress responses. Trends Plant Sci. 24, 672–676. doi: 10.1016/j.tplants.2019.05.010
Yang, J.-C., Zhang, H., and Zhang, J.-H. (2012). Root morphology and physiology in relation to the yield formation of rice. J. Integr. Agric. 11, 920–926. doi: 10.1016/S2095-3119(12)60082-3
Yao, R., Li, J., and Xie, D. (2017). Recent advances in molecular basis for strigolactone action. Sci. China Life Sci. 61, 277–284. doi: 10.1007/s11427-017-9195-x
Yi, K., Menand, B., Bell, E., and Dolan, L. (2010). A basic helix-loop-helix transcription factor controls cell growth and size in root hairs. Nat. Genet. 42, 264–267. doi: 10.1038/ng.529
Yin, C., Gan, L., Ng, D., Zhou, X., and Xia, K. (2007). Decreased panicle-derived indole-3-acetic acid reduces gibberellin A1 level in the uppermost internode, causing panicle enclosure in male sterile rice Zhenshan 97A. J. Exp. Bot. 58, 2441–2449. doi: 10.1093/jxb/erm077
Yu, Z., Kang, B., He, X., Lv, S., Bai, Y., Ding, W., et al. (2011). Root hair-specific expansins modulate root hair elongation in rice. Plant J. 66, 725–734. doi: 10.1111/j.1365-313X.2011.04533.x
Yu, C., Sun, C., Shen, C., Wang, S., Liu, F., Liu, Y., et al. (2015). The auxin transporter, OsAUX1, is involved in primary root and root hair elongation and in Cd stress responses in rice (Oryza sativa L.). Plant J. 83, 818–830. doi: 10.1111/tpj.12929
Zhang, S., Huang, L., Yan, A., Liu, Y., Liu, B., Yu, C., et al. (2016). Multiple phytohormones promote root hair elongation by regulating a similar set of genes in the root epidermis in Arabidopsis. J. Exp. Bot. 67, 6363–6372. doi: 10.1093/jxb/erw400
Zhang, J., Mazur, E., Balla, J., Gallei, M., Kalousek, P., Medveďová, Z., et al. (2020). Strigolactones inhibit auxin feedback on PIN-dependent auxin transport canalization. Nat. Commun. 11:3508. doi: 10.1038/s41467-020-17252-y
Zhang, Y., Xu, F., Ding, Y., Du, H., Zhang, Q., Dang, X., et al. (2021). Abscisic acid mediates barley rhizosheath formation under mild soil drying by promoting root hair growth and auxin response. Plant Cell Environ. 44, 1935–1945. doi: 10.1111/pce.14036
Zhao, Y. (2010). Auxin biosynthesis and its role in plant development. Annu. Rev. Plant Biol. 61, 49–64. doi: 10.1146/annurev-arplant-042809-112308
Zhao, Y. D., Christensen, S. K., Fankhauser, C., Cashman, J. R., Cohen, J. D., Weigel, D., et al. (2001). A role for flavin monooxygenase-like enzymes in auxin biosynthesis. Science 291, 306–309. doi: 10.1126/science.291.5502.306
Zhao, X., Wang, Q., Yan, Q., Zhao, Y., Wang, F., and Dong, J. (2016). Function of brassinolide in the regulation of root development in rice. Chin. J. Cell Biol. 38, 1191–1198. doi: 10.11844/cjcb.2016.10.0109
Zhu, Z., An, F., Feng, Y., Li, P., Xue, L., Mu, A., et al. (2011). Derepression of ethylene-stabilized transcription factors (EIN3/EIL1) mediates jasmonate and ethylene signaling synergy in Arabidopsis. Proc. Natl. Acad. Sci. U.S.A. 108, 12539–12544. doi: 10.1073/pnas.1103959108
Zhu, C., Gan, L., Shen, Z., and Xia, K. (2006). Interactions between jasmonates and ethylene in the regulation of root hair development in Arabidopsis. J. Exp. Bot. 57, 1299–1308. doi: 10.1093/jxb/erj103
Zou, X., Shao, J., Wang, Q., Chen, P., Zhu, Y., and Yin, C. (2018). Supraoptimal cytokinin content inhibits rice seminal root growth by reducing root meristem size and cell length via increased ethylene content. Int. J. Mol. Sci. 19:4051. doi: 10.3390/ijms19124051
Zou, X., Wang, Q., Chen, P., Yin, C., and Lin, Y. (2019). Strigolactones regulate shoot elongation by mediating gibberellin metabolism and signaling in rice (Oryza sativa L.). J. Plant Physiol. 237, 72–79. doi: 10.1016/j.jplph.2019.04.003
Keywords: phytohormones, root hair cell fate determination, root hair initiation, root hair elongation, phytohormone interaction
Citation: Li M, Zhu Y, Li S, Zhang W, Yin C and Lin Y (2022) Regulation of Phytohormones on the Growth and Development of Plant Root Hair. Front. Plant Sci. 13:865302. doi: 10.3389/fpls.2022.865302
Received: 31 January 2022; Accepted: 03 March 2022;
Published: 24 March 2022.
Edited by:
Brad M. Binder, The University of Tennessee, Knoxville, United StatesReviewed by:
Arkadipta Bakshi, University of Wisconsin-Madison, United StatesCopyright © 2022 Li, Zhu, Li, Zhang, Yin and Lin. This is an open-access article distributed under the terms of the Creative Commons Attribution License (CC BY). The use, distribution or reproduction in other forums is permitted, provided the original author(s) and the copyright owner(s) are credited and that the original publication in this journal is cited, in accordance with accepted academic practice. No use, distribution or reproduction is permitted which does not comply with these terms.
*Correspondence: Changxi Yin, eWluY2hhbmd4aUBtYWlsLmh6YXUuZWR1LmNu
†These authors have contributed equally to this work and share first authorship
Disclaimer: All claims expressed in this article are solely those of the authors and do not necessarily represent those of their affiliated organizations, or those of the publisher, the editors and the reviewers. Any product that may be evaluated in this article or claim that may be made by its manufacturer is not guaranteed or endorsed by the publisher.
Research integrity at Frontiers
Learn more about the work of our research integrity team to safeguard the quality of each article we publish.