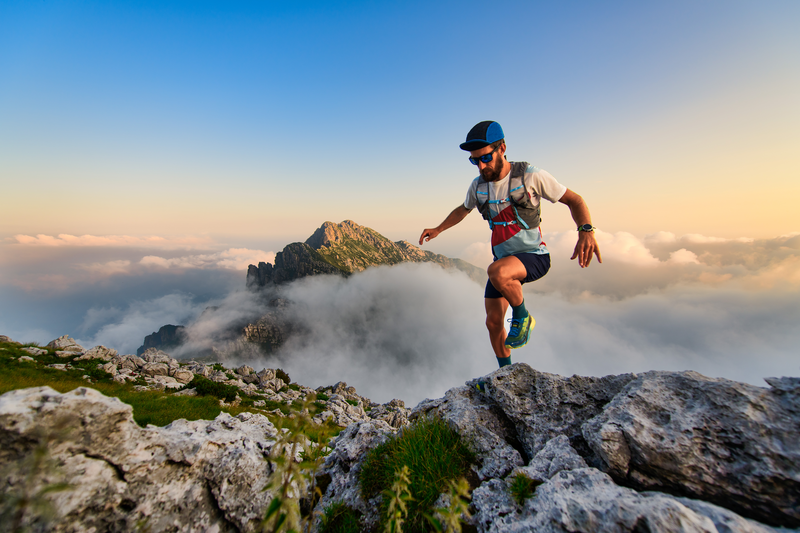
95% of researchers rate our articles as excellent or good
Learn more about the work of our research integrity team to safeguard the quality of each article we publish.
Find out more
ORIGINAL RESEARCH article
Front. Plant Sci. , 11 April 2022
Sec. Crop and Product Physiology
Volume 13 - 2022 | https://doi.org/10.3389/fpls.2022.863760
This article is part of the Research Topic Advances in Nitrogen Use Efficiency for Agriculture and Environment View all 36 articles
The aim of this study is to analyze the variations in the plant-available nitrogen (PAN) concentrations in the soil profile. Different fertilizers were applied for Chinese cabbage plantation (CCP) in the experimental fields of the Shunyi region. The treatments used for the comparative analysis are (i) no fertilizer and plantation (NVP), (ii) no fertilizer with CCP (CTP), (iii) fertilization as urea (URP), and (iv) potassium nitrate (KNP) and chicken manure (CMP) with CCP. It was concluded that the yield was significantly high in URP, CMP, and KNP as compared to CTP. In URP, maximum PAN in soil layers 0–60 cm was recorded during crop production and in 60–100 cm after harvesting as compared to other treatments. Significant variations in soil pH and electrical conductivity (EC) for the soil profile (0–100 cm) from the initial values with respect to time and treatments were observed. CMP showed maximum ammonium in the upper layers of 0–60 cm throughout the season, whereas minimum PAN was observed in NVP but increased in lower layers of 60–100 cm. In general, all fertilizers raised the PAN below the soil 60–100 cm which indicates their potential for nitrate leaching (NL).
Nitrogen (N) is the essential macro-nutrient for plants and is thereby added to agricultural soil to enhance crop production. Soil contains nitrogen in the form of organic and inorganic compounds which are usually in minute amounts with respect to crop demand, thus the application of N fertilizers is considered as a key factor to increase crop yield throughout the world. Globally, N is added in larger quantities, so more N losses transpire to the environment in the form of nitrate leaching (NL) and nitrous oxide emission (Cameron et al., 2013; Yousaf et al., 2016). Nitrate and ammonium ions are the two major forms of N that are consumed by the plants and the preference of one form over another depends upon plant species and environmental factors. These two inorganic pools describe the major portion of plant-available nitrogen (PAN) in soil for crop production and contribute to nitrogen losses (Britto and Kronzucker, 2013; Shah et al., 2017).
Every soil contains nitrate and ammonium concentrations that are the product of soil mineralization of organic matter and applied N fertilizers (Zhang et al., 2013). The mineralization rate in agricultural soil is directly linked with soil pH, temperature, and soil electrical conductivity (EC) (Curtin et al., 1998; Yan et al., 2015; Liu et al., 2016; Gubry-Rangin et al., 2017). Soil nitrate and ammonium are present mostly in lower amounts, hence they are supplemented as N fertilizer and their residence time in the soil root zone mainly depends on the soil texture (Bimüller et al., 2014). Furthermore, N addition more than plant demands may build a peak concentration layer of inorganic N in the soil profile and depends on the fertilizer application and move down with time along with water (Wang et al., 2014; Dai et al., 2015; Van Meter et al., 2016). The gradual leaching of residual nitrate into the layers below the root zone is a major N loss in cropping systems (Chen et al., 2014; Li et al., 2016). Total organic N and inorganic N present in the different horizons of soil from the topsoil to bedrock is a function of depth from the upper surface, time, drainage quantity, and quality passing through the soil profile (Johnsson et al., 1987; Negm et al., 2014). After N fertilization, ammonium ions in upper soil layers increase up to a threshold and then start to reduce with an associated increase in nitrate concentration (Kraft et al., 2014). According to Sullivan et al. (2014), the amount of biologically fixed atmospheric nitrogen added to soil is smaller as compared to the amount of fertilizer added for a specific period of time. Therefore, fertilizer addition is the real cause of NL in many regions of the world.
Nitrate is highly soluble in water and does not bind well with the soil particles because of its negative charge, similar to clay particles. Thus, water movement carries nitrate from the soil medium to surface water (runoff) or groundwater via NL (Zhou and Butterbach-Bahl, 2014). Therefore, nitrate is the most important ion related to groundwater pollution due to its high solubility in water and potential for NL (Basso et al., 2013). Higher N application favors the higher mineralization with an ultimate abundance of nitrate ions in the soil causes higher nitrate accumulation in the different parts of the plant body, particularly in vegetables (Colla et al., 2018). Subsequently, this connection may generate peroxynitrite (ONOO–) through nitrate reductase in portions of the plant, which is highly toxic to human beings and animals (Islam et al., 2015; Schwertz et al., 2016).
Riley and Decker (2000) found significant variations in the soil nitrate and ammonium levels in the soil profile from 0 to 60 cm under the different types of fertilizers during the wheat cropping season. In addition, the most commonly used fertilizer rate of 250 kg N/ha with irrigation had the potential to raise the nitrate level in soil layers and subsequently cause NL to the deeper layers in the soil profile. Moreover, they concluded that the most commonly used fertilization practices (irrigation and 250 kg N/ha) had the potential to increase the N accumulation in soil layers as well as NL. Zhongjiang et al. (2014) found a significant decrease in ammonium levels from the top 10–15 cm soil with an increase in the nitrate level under different fertilization. Similarly, Yang et al. (2013) studied dry land soil in Shanxi, China and found that the peak nitrate concentration layer is developed at 80 cm in soil profile after longtime straw application. In fact, PAN accumulation in the soil profile below the root zone is the main cause of groundwater nitrate contamination in the Chinese semi-humid croplands because heavy rainfall in the monsoon season or irrigation water moves mineral N deeper in soil layers (Yang et al., 2015; Zhou et al., 2016). A recent study on higher nitrate concentration in the filtered groundwater of Shaanxi and Shandong provinces showed the adverse effects on the resident population (Zhai et al., 2017).
Increased food demand increased chemical fertilization throughout the world, especially in the large populated Asian countries, such as China and India (Liu et al., 2014). According to the results of the Rapid Diagnostic Appraisal survey, vegetables in the Shunyi District were heavily fertilized approximately 2,250 kg N/ha (150 kg N/μ) of organic manure and sometimes combined use with potassium fertilizers (Quan et al., 2015). Poultry industries have been increased multi-fold times since 1980, especially by small farmers as the family-run farming system in China (Fan et al., 2010). These farmers are incorporating the poultry manures abundantly without being concerned about the crop requirements, resulting in the accumulation of inorganic N in the soil profile and ultimately causing to increase NL (Lu et al., 2012; Zheng et al., 2017). Different types of seedbeds are prepared in the crop rotation system, such as a row cropping to cultivate the vegetable and the hill drop or broadcasting for cereal crops. These cultivation practices may leave few places fallow in the agricultural fields. In a similar way, fields are left fallow without crop cultivation in the crop rotation to maintain soil fertility or for any other purpose. These fallow soils in agricultural land have higher N mineralization and nitrate in the upper soil layers. Eventually, this increase in the nitrate level contributes to building a peak concentration deeper in sub-soil or causes to contaminate the groundwater. During seedbed preparation, a bare land section may construct when cereal cropping fields are prepared to grow row cropping vegetables. Similarly, during crop rotation seasons without plantation and fertilization (fallow period) are adjusted to maintain fertility and for any other purpose. According to Chen et al. (2016), bare land may also contribute to significant NL in the agricultural soil in China because of the previous fertilization and rainfall effects (Zheng et al., 2017).
This study was planned to analyze the variations in the PAN with 25–27%, pH, and EC in the soil profile under different fertilization during and after the cropping season of Chinese cabbage (Brassica rapa subsp. pekinensis) in the experimental fields. The variations in soil parameters were observed with respect to time (cropping season) and layers (0–100 cm) under fertilizer treatments including chicken manure, potassium nitrate and urea, CMP, KNP, and URP, non-fertilization with crop cultivation, and bare land without cropping. Moreover, the comparative yield analyses were also made among the treatments in Shunyi District, China.
Shunyi District (40.1303°N, 116.6547°E) is situated in the Northeast of Beijing, having a total area of 1,021 km2, including 5.0 × 105 ha agricultural lands under cereal crops, vegetable farms, fruit gardens, and grasslands for animal husbandry. The mean annual temperature is 11.5°C with hot and humid summer and with cold and dry winter. Mean annual precipitation is 600 mm but mostly occurs during monsoon (July–August) and thereby, irrigation is essential for crop production (Ṙuz̆ic̆ and Hansen, 1975; Geng et al., 2015).
The rainfall events and daily temperature for the experiment year (2016) are presented in Figure 1 and soil texture details from 0 to 100 (0–20, 20–40, 40–60, 60–80, and 80–100) cm soil profile are given in Table 1. The experimental field had installed a drip irrigation system and scheduled on the demand basis but the same for all treatments.
Figure 1. The average daily temperature (°C) and rainfall event (mm) recorded in the Shunyi District in the year 2016 (source: Weather Underground Online Record).
Repeat Soil Core Method (RSCM) is used in this study to evaluate the ammonium, nitrate, pH, EC, and total PAN differences with respect to the cropping season and soil profile (0–100 cm). RSCM was used to evaluate the surface and subsoil physical and chemical properties as the number of soil profile samples was taken at different locations from the experimental field and the same procedure was repeated after a pre-defined time of 1 month. The number of samples taken in a single collection from treatment was considered as repetitions for a parameter. In this study, nine repetitions were taken for a single layer in a plot. The changes in the properties of the soil were analyzed with respect to time, treatment, and soil profile depth. Moreover, this method is easy to handle and broader regions can be covered in actual field conditions. Any fertilization in soil may significantly affect the plant available N within a soil profile of 0–100 cm (Rayment and Higginson, 1992) and provides realistic analysis if distributed in small sectional layers, such as 0–20, 20–40, and 40–60.
This experiment was conducted in the field, having five plots of equal size 25 m × 5.25 m and had three rows of 1.75 m spacing as the split plot design. Five treatments were applied in five plots, including bare soil with no fertilizer and no crop planted (NVP), Chinese cabbage plantation with either no fertilizer (CTP), urea at a rate of 300 kg N/ha (URP), potassium nitrate at a rate of 300 kg N/ha (KNP), or chicken manure at a rate of 300 kg N/ha (CMP). Soil samples were collected and divided into five equal sections 0–20, 20–40, 40–60, 60–80, and 80–100 cm along with the Gouge Auger (6 cm diameter and 100 cm length). Chinese cabbage (B. rapa subsp. pekinensis) was transplanted as 0.25 m plant to plant and 1.75 m row to row distance after the 1 week of fertilization. A single plot contained 300 plants sowed in three rows. NVP plot was left without any interference after secondary tillage. Soil samples were collected at the time of pre-planting and fertilization (t0), during cropping season as 1 month after fertilization (t1), 2 months after fertilization (t2), and 3 months after fertilization (t3) just after harvesting. At each time point, nine soil cores for 1 m soil depth in each plot was collected at three repetitions from each row and overall nine repetitions. Samples were marked and transferred in an ice-filled container from the field site to the laboratory. Nitrate and ammonium analyses were made using Lachat flow injection (QuikChem® 8500 Series 2 FIA System, United States with 0.5% accuracy and reproducibility) (Butterly et al., 2013). For nitrate and ammonium analysis, the soil was extracted using 2 M KCl at a 5:1 ratio. For soil, pH, and EC, air-dried soil samples were passed through a 2 mm sieve, mixed with de-ionized water solution at a ratio of 5:1, and shaken for 1 h at 15 rpm (McLaughlin, 2009). A digital pH and EC meter (Mettler Toledo™ S230 SevenCompact™ with ±0.5% accuracy) were calibrated using a standard solution of 1,413 μS/cm at 25°C for EC and buffer solution was used to calibrate at pH 4, 6, and 7 Electrodes were washed with distilled water and wiped with tissue after each reading.
The data were adjusted by subtracting each value for the soil pH, EC, nitrate, ammonium, and PAN at t1, t2, and t3 from the baseline value collected at pre-plant (t0). Positive values represent an increase from the baseline (t0) and negative values represent a decrease relative to the baseline (t0). Data were analyzed by two-way analysis of variance (ANOVA) using Statistix v.8.1 software. Multiple comparisons were made using the Tukey HSD with 0.05 alpha (α = 0.05) for the time, treatment, and time-treatment interaction. The yield data analysis was also made by the one-way ANOVA. The graphical presentation was being made using GraphPad Prism v.6.01 and SigmaPlot v.13.
In the study area of Shunyi District, soil pH ranges from 7.0 to 8.5 which is a suitable range for crop production. The pH variations were analyzed more in the above soil layers from 0 to 80 cm than in 80–100 cm for all treatments as shown in Figure 2. CTP, NVP, and CMP showed an increasing trend, while URP and KNP showed a decreasing trend relative to the baseline t0 values. Soil layer from 80 to 100 cm showed minimum variations in NVP, CTP, and CMP as compared with URP and KNP. In soil profile, the mean variations for all treatments were not significantly different at the time t1, t2, and t3 in the soil profile 0–100 cm, whereas treatments were significantly different among each other as given in Table 1.
Figure 2. Mean values with standard error bar of soil pH in soil profile (0–100 cm) for the treatments NVP, CTP, CMP, KNP, and URP before (t0) and after fertilization at t1 (1 month), t2 (2 months), and t3 (3 months) in the experimental fields of Shunyi District, China.
The mean EC variations in soil profile from 0 to 60 cm showed a decreasing trend in NVP and CTP while increasing trend in CMP and KNP after the pre-planted t0 up to the harvest time t3. URP represented a different result from the other treatments as the mean EC increased up to t2 and thereafter decreased at t3. Maximum variations were recorded in the upper layers from 0 to 40 cm than 60 to 100 cm for all the treatments. In soil layers from 60 to 100 cm, uneven addition was found in all treatments from the baseline t0 as shown in Figure 3. Statistically, variations in the fertilized plots (CMP, KNP, and URP) were significantly different from non-fertilized plots (CTP and NVP) with maximum variations in KNP and minimum in CTP in the soil profile 0–100 cm as given in Table 1. In addition, the mean variations for all treatments were significantly different with respect to the time as maximum recorded at t3.
Figure 3. Soil electrical conductivity (EC) mean values with standard error bar in soil profile (0–100 cm) at t0, t1, t2, and t3 for the treatments during the Chinese cabbage production in Shunyi District, China.
Nitrate concentrations were recorded in the declined order from surface to bottom layers in the soil profile (0–100 cm) in all treatment plots at the baseline time t0, whereas at t3 (after harvest) CTP, NVP, and CMP represented the incline but URP and KNP decline trends as shown in Figure 4. It can be analyzed that nitrate level increased in layers 60–100 cm for all fertilized plots while decreased in non-fertilized plots. After harvesting, nitrate was recorded in CMP more than KNP and URP in the upper soil layer of 0–40 cm but not during the cropping season t1 and t2. The mean variations in the soil layers 0–40 cm were higher in URP as compared with KNP and CMP. In the soil profile 0–100 cm, the mean variations in nitrate were significantly different in all plots with respect to time and treatment as given in Table 2. The mean negative values of the CTP indicated that nitrate was decreased in the soil profile (0–100 cm) from the values recorded at the baseline t0, while the maximum increase was found in the URP. The mean values for all treatments recorded at t2 and t3 were less than t1 which presented the reduction in nitrate concentrations with the passing time.
Figure 4. Nitrate mean and standard bar values in the different layers of soil profile 0–100 cm in the treatment plots of Chinese cabbage for the time periods t0, t1, t2, and t3 in the cropping season at Shunyi District, China.
Table 2. Presents the two-way ANOVA (split plot design) with the Tukey HSD (α = 0.05) for nitrate and ammonium ions in soil profile 0–100 cm.
Ammonium concentrations were increased from the baseline t0 in the fertilized plots (CMP, KNP, and URP) in the soil layers from 0 to 60 cm, while decreased in non-fertilized plots (NVP and CTP) at t1, t2, and t3. The mean values at t3 in CMP and URP were higher in the soil layers 60–100 cm from baseline t0 values which represented the accumulation of ammonium in these layers after the cropping season as shown in Figure 5. In CMP, the ammonium level increased significantly in t1, gained peak level at t2 and declined at t3, whereas URP and KNP gained peak levels in t1 and thereafter showed declining trends lasting up to the harvesting period (t3) in soil layer 0–40 cm. The maximum increase in mean values was observed in lower layers (40–100 cm) for CMP and URP as compared with KNP in t1. In soil layers up to 60 cm, CMP had shown maximum increase but the deeper layers of URP showed peak values. The negative mean values in non-fertilized plots (NVP and CTP) represented loss, whereas positive values in fertilized plots (CMP, KNP, and URP) represented the gain of ammonium in the soil layers as given in Table 2. Statistically, ammonium concentrations were significantly different with respect to the treatment and time in the soil profile 0–100 cm.
Figure 5. Ammonium levels in different soil layers (0–100 cm) for the treatments at the time periods t0, t1, t2, and t3 in the cropping season of Chinese cabbage production at Shunyi District, China.
Plant-available nitrogen represented the declining trend in soil layer 0–20 cm from t0 to t3 in CTP, t1 to t3 in KNP and NVP, while inclined to the maximum level at t2 in CMP and URP as shown in Figure 6. Statistically, it was recorded that all treatments showed significant variations with respect to time, treatments, and their interaction as shown in Table 3. PAN was significantly increased in all fertilizer treatments and decreased for a non-fertilizer plot in the whole soil profile. After harvesting, peak PAN levels were observed in soil profiles 60–80 cm for all treatments. Urea presented the maximum for the soil layers 0–40 cm at t1 and for 20–80 cm at t3 than KNP and CMP. The negative mean values were recorded in only CTP which showed decreased PAN in the soil profile 0–100 cm from the baseline t0 values.
Figure 6. Plant-available nitrogen (PAN) (nitrate + ammonium) variations in soil profile (0–100 cm) for the treatments NVP, CTP, CMP, KNP, and URP before fertilization (t0) and after 1 month (t1), 2 months (t2), and 3 months (t3) of fertilization during the Chinese cabbage production in Shunyi District, China.
Table 3. Presents the two-way ANOVA (split plot design) with the Tukey HSD (α = 0.05) for the plant-available nitrogen (PAN) in soil profile 0–100 cm.
Every plot (except NVP) had 300 plants at the time of harvest. From every row, two average plants were taken as representative values for yield analysis. Thus, every plot had 6 values and these values were used to convert yield per hectare. Overall, the yield was significantly higher in all fertilizer plants than in control. KNP and URP (chemical fertilizers) presented significantly higher yields than CMP (organic fertilizer) as shown in Figure 7. Chinese cabbage yield followed the order from maximum to minimum as URP > KNP > CMP > CTP.
Figure 7. Chinese cabbage yield in different treatments with standard error bar and letters (a, b, and c) presenting the statistical significant variation (p < 0.05).
A cabbage root zone was spread over 100 cm of soil and rapidly decreased with the increasing soil depth that was able to effectively absorb the part of soil nutrients in the root zone. As a result, we selected soil depths from 0 to 40 cm in this study. The results showed that the soil nitrogen content increased with an increasing soil depth from 0 to 30 cm in 25 days after planting. In extremely well-drained soils, the content was mainly distributed in the topsoil (0-20 cm) 43 days after planting, and the soil nitrogen content was reached to 71.98 mg kg–1. The same result was obtained when the soil nitrogen concentration was reduced from 30 cm. Most parts of the soil nitrogen content remained in the topsoil (0–50 cm), which was associated with several root parameters (Yousaf et al., 2016). Soil pH temporal variation in a particular cropping system depends upon the plant species or crop grown (Curtin and Trolove, 2013), nitrogen fertilization source (Bird and Rapport, 1986), and the buffering capacity of the soil (Min et al., 2016). In many regions of the world, it was observed that NL after inorganic N fertilizers may cause considerable acidification in the soil such as in Atlantic Canada, 74% acidic effects came from N fertilizers and 26% from acidic rain (Kemmitt et al., 2008). An increase in the nitrate ions may decrease pH in a soil profile exponentially. Hence, nitrate accumulation in the soil profile is a substantial factor affecting soil acidification (Duruigbo et al., 2007). Soil pH is the main regulating factor of soil microbial activities and the rate of soil carbon and N cycling that may be disturbed by urea acidification (Eigenberg et al., 2002). In this study, the same results were found in the urea plot as pH declined in the first month and thereafter, returned to the initial values. In previous results, the poultry manure has the ability to increase the pH level of soil when it was treated as 15 ton/ha (300 kg N/ha with 2% N) (Ruter, 1992).
Statistically, overall temporal EC variations in the soil profile before, during, and after plantation and fertilization were observed significantly in all plots but the maximum increase was observed in KNP. Potassium nitrate is considered to be the salt fertilizer and has the ability to change the soil EC (Djurhuus and Olsen, 1997). Thus, significant variation in soil EC might be occurred due to the potassium nitrate fertilization. Organic and inorganic fertilization in agricultural soil raises its EC (Sims et al., 1995; Sainju et al., 2019) which might be the reason for high EC in KNP, URP, and CMP as compared to CTP and NVP.
The nitrate levels in the first 2 months were increasing in NVP and thereafter declined to initial values in the soil profile which might be due to the increasing mineralization of bare tilled soil. The same results were noted by Sainju et al. (2019) in Piarco Series Soil (West Indies) while analyzing the temporal differences between nitrate concentrations in the soil profile from 0 to 90 cm in the early and late rainfall seasons. Nitrate in CTP increased in the first month but declined up to the harvest period, such as the lowest concentrations were measured in all layers of the soil profile at the end of the cropping season. It might be happened due to the plant growth which might increase the nitrate uptake from the root zone as well as decreased the total N budget and mineralization. It was recorded that nitrate concentration was increased in all the layers of soil profile as compared with the initial values in CMP, particularly after 2 months of chicken manure application. It was observed that chicken manure contained a high rate of available N and a larger fraction in organic form which needed further mineralization to release ammonium or/and nitrate ions. The time rate of poultry manure mineralization was not fixed, such as Azeez and Van Averbeke (2010) found that 48% of the organic N in poultry manure was released within 10 weeks during the laboratory experiment, whereas Bitzer and Sims (1988) reported 40% during 18 weeks. Fan et al. (2003) reported maximum nitrate available in the soil about 10–15 weeks after broiler manure application (Rong and Xuefeng, 2011). In this study, higher nitrate concentration after cropping season might be happened due to the slow mineralization rate. In KNP soil layers from 0 to 60 cm showed that the nitrate level increased to a certain limit in the first 2 months and thereafter decreased up to nearly initial concentration whereas, in soil layers 60–100 cm, the nitrate level increased up to the end of the cropping season. Few researchers reported that the balance amount of potassium in the soil might withhold nitrate ions, increase nitrate uptake in the plant body, and decrease NL (Gabriel et al., 2012; Zhou et al., 2016). Sainju et al. (2019) worked out the adsorption effects of potassium ions with nitrate ions and found minimum adsorption in the soil surface layers from 0 to 15 cm as compared with subsoil layers. Furthermore, subsoil layers from 0 to 90 cm showed maximum adsorption effects as withholding the maximum amount of nitrate in the field experiment. Therefore, it might be the reason why subsoil layers from 60 to 100 cm presented nitrate in raising trend up to the harvesting time. In soil profile from 0 to 40 cm in URP treatment, the nitrate level increased after the first 2 months and turned back to the initial level thereafter, in the soil layers from 40 to 60 cm inclined up to the harvesting period as shown in Figure 5. Rong and Xuefeng (2011) affirmed in their experiment that urea fertilization had a positive relation with nitrate accumulation in soil profiles from 0 to 100 cm. In this study, nitrate incremented in soil layers of 0–40 cm and lasting up to 2 months, was previously found, such as Sainju et al. (2019) reported non-significant variation in soil profile within 4 weeks subsequent to urea fertilization but thereafter, found a significant increase in the nitrate level.
It was obvious from the previous studies that the maximum mineralization of organic matters and the nitrification of residual nitrogen occurred in the fallow period or bare soil without vegetation after harvesting (Bishop and Manning, 2010), which might be the cause of ammonium level raised in CTP and NVP at t1 in the soil profile. In Shunyi District, clay level was found in increasing order with the soil depth as presented in Table 4, which might be constructed the plow pan in the subsoil and raised the ammonium level in lower layers. The temporal variations in ammonium levels before and after cropping season in CTP showed that the final values were three times lesser than the initial values in the 0–60 cm soil profile which might be happened due to lower soil mineralization rate as compared with ammonium utilization (nitrate conversion, volatilization, and plant uptake). In KNP, it was recorded that the ammonium level was decreased in 0–60 cm soil layers before and after cropping season. Meanwhile, a little increment was noted in 60–100 cm soil layers that might be due to the potassium nitrate which promotes the nitrate concentration in soil, not ammonium. Thus, the overall ammonium in soil decreased during the cropping season due to the plant consumption and utilization as nitrate conversion and volatilization. Soil layers 0–60 cm in CMP made available higher ammonium concentration throughout the cropping season as compared with initial values (before fertilization) whereas, soil layers from 60 to 100 cm showed negligible variation. Similar results were observed in the study of Perego et al. (2012). Temporal increment in ammonium level in URP treatment was disappeared in soil profile from 0 to 60 cm but lasted in 60–100 cm after harvesting. As urea addition might be the cause of significantly increased ammonium (Xiong et al., 2012) up to the maximum limit within 4 weeks after fertilization in the presence of moisture, whereas subsequent rainfall or irrigation might drag down the urea concentration in the soil profile (Gai et al., 2016). In this study, it was observed that the total PAN in the soil profile (0–100 cm) increased to maximum level after 1 month (September) of fertilization, not only in fertilized plot but also in without fertilized (NVP and CTP) plots which might be due to the heavy rainfall occurred during this month as shown in Figure 1. It was proved that heavy rainfall events increased nitrate and ammonium in the soil profile (0–100 cm) (Zhang et al., 2013).
It was observed that the maximum Chinese cabbage yield was produced in fertilized plots than control plots but significant variations were also observed among the fertilizer treated plots as well. Maximum yield was observed in URP than KNP but both have significantly higher values as compared to CMP. It might be possible that because poultry manure had the slow N release at the time of high plant requirement as previously reported in a similar study (Li et al., 2021).
Chinese cabbage produced maximum yields in fertilizer treated plot because of optimum PAN level during the cropping season. Chinese cabbage had shallow root depth, required frequent irrigation, and optimum PAN in the upper soil layer 0–40 cm for optimum production. Therefore, total PAN concentration and PAN lasting time in a root zone (0–30 cm) are important while selecting fertilizer type and crop type. In our experiment, the variations in soil pH, EC, nitrate, ammonia, and PAN in soil profile 0–60 cm with respect to initial values for all treatments were larger as compared to variations in soil profile 60–100 cm. PAN level as ammonium was found maximum in CMP as compared to the other treatments. CMP showed a higher ammonium concentration in the upper soil layer during the cropping season and increased the potential use of PAN and decreased the potential NL as compared to KNP and URP. Bare land without plantation also caused to increase in PAN concentration in lower soil profile 0–60 cm. Higher PAN levels as compared to initial values in soil profile 60–100 cm showed the N losses occurred after all treatments and had the potential to contaminate the groundwater resources except for the CTP. Furthermore, Chinese cabbage productions without fertilization significantly dragged down the PAN level in soil layers 0–60 cm as well as reduced crop yield. For a single cropping season, pH variations in the soil profile of 0–100 cm were significant during and after cropping season with respect to time and treatments, although these were very minute as compared to the initial values. Similar results were observed for EC as well. Overall, the soil EC and pH values were in the decreasing trend for KNP and URP, whereas in increasing trend in NVP, CMP, and CTP. Therefore, a single cropping season may vary notable differences in nitrate, ammonium, and PAN levels in the soil (Shunyi District, Beijing, China) profile with smaller differences in soil pH and EC.
The raw data supporting the conclusions of this article will be made available by the authors, without undue reservation.
RA designed the experiment methodology and wrote the manuscript. YL was the supervisor and project administrator, assisted to correct the methodology, and validate the design. JD, WL, and SA supported data curation and laboratory work. LM helped in reviewing and editing. RA made the software analysis with the assistance of LM, SA, and WL. WA and AA have done the data analysis and curated and helped in manuscript writing. All authors contributed to the article and approved the submitted version.
This work is supported by the Natural Science Foundation of China (Grant No. 52161145104).
The authors declare that the research was conducted in the absence of any commercial or financial relationships that could be construed as a potential conflict of interest.
All claims expressed in this article are solely those of the authors and do not necessarily represent those of their affiliated organizations, or those of the publisher, the editors and the reviewers. Any product that may be evaluated in this article, or claim that may be made by its manufacturer, is not guaranteed or endorsed by the publisher.
We extend our thanks to the National Natural Science Foundation of China, Agricultural and Water Productivity and Water Environment Team for their grant support for this Ph.D. research work at Graduate School of Chinese Academy of Agricultural Sciences, Beijing China. We are also grateful for the reviewers who suggested improvements in this manuscript.
Azeez, J., and Van Averbeke, O. W. (2010). Nitrogen mineralization potential of three animal manures applied on a sandy clay loam soil. Bioresour. Technol. 101, 5645–5651. doi: 10.1016/j.biortech.2010.01.119
Basso, A. S., Miguez, F. E., Laird, D. A., Horton, R., and Westgate, M. (2013). Assessing potential of biochar for increasing water-holding capacity of sandy soils. Glob. Change Biol. Bioenergy 5, 132–143. doi: 10.1111/gcbb.12026
Bimüller, C., Mueller, C. W., Von-Lützow, M., Kreyling, O., Kölbl, A., Haug, S., et al. (2014). Decoupled carbon and nitrogen mineralization in soil particle size fractions of a forest topsoil. Soil Biol. Biochem. 78, 263–273. doi: 10.1016/j.soilbio.2014.08001
Bird, P. M., and Rapport, D. (1986). State of the Environment Report for Canada. Environment Canada:PE8.1 CENSA I.D. 116978. Ottawa, ON: Environment Canada.
Bishop, P., and Manning, M. (2010). Urea Volatilisation the Risk Managements and Mitigation Strategies. 2010, No. Figure 1, 1–13. Palmerston North: Fertilizer and Lime Research Centre, Massey University.
Bitzer, C. C., and Sims, J. T. (1988). Estimating the availability of nitrogen in poultry manure through laboratory and field studies. J. Environ. Qual. 17:47. doi: 10.2134/jeq1988.00472425001700010007x
Britto, D. T., and Kronzucker, H. J. (2013). Ecological significance and complexity of N-source preference in plants. Ann. Bot. 112, 957–963. doi: 10.1093/aob/mct157
Butterly, C. R., Baldock, J. A., and Tang, C. (2013). The contribution of crop residues to changes in soil pH under field conditions. Plant Soil 366, 185–198.
Cameron, K. C., Di, H. J., and Moir, J. L. (2013). Nitrogen losses from the soil / plant system : a review. Ann. Appl. Biol. 162, 145–173. doi: 10.1111/aab.12014
Chen, X., Cui, Z., Fan, M., Vitousek, P., Zhao, M., Ma, W., et al. (2014). Producingmore grain with lower environmental costs. Nature 514, 486–489. doi: 10.1038/nature13609
Chen, Y., Wang, J. H., Xiao, W. H., Zhou, Y. Y., Wang, L. T., and Song, X. Y. (2016). Spatiotemporal variation of precipitation based on three indicators in the flood season in Beijing. IOP Conf. Ser. Earth Environ. Sci. 39:012060. doi: 10.1088/1755-1315/39/1/012060
Colla, G., Kim, H. J., Kyriacou, M. C., and Rouphael, Y. (2018). Nitrate in fruits and vegetables. Sci. Hortic. 237, 221–238. doi: 10.1016/j.scienta.2018.04.016
Curtin, D., and Trolove, S. (2013). Predicting pH buffering capacity of New Zealand soils from organic matter content and mineral characteristics. Soil Res. 51:494. doi: 10.1071/SR13137
Curtin, D., Campbell, C. A., and Jalil, A. (1998). Effects of acidity on mineralization, pH- dependence of organic matter mineralization in weakly acidic soils. Soil Biol. Biochem. 30, 57–64. doi: 10.1016/S0038-0717(97)00094-1
Dai, J., Wang, Z., Li, F., He, G., Wang, S., Li, Q., et al. (2015). Optimizing nitrogen input by balancing winter wheat yield and residual nitrate-N in soil in a long-term Dryland field experiment in the loess plateau of China. Field Crops Res. 181, 32–41. doi: 10.1016/j.fcr.2015.06.014
Djurhuus, J., and Olsen, P. (1997). Nitrate leaching after cut grass/clover leys as affected by time of ploughing. Soil Use Manag. 13, 61–67. doi: 10.1111/j.1475-2743.1997.tb00558.x
Duruigbo, C. I., Obiefuna, J. C., and Onweremadu, E. (2007). Effect of poultry manure rates on soil acidity in an ultisol. Int. J. Soil Sci. 2, 154–158. doi: 10.3923/ijss.2007.154.158
Eigenberg, R., Doran, J., Nienaber, J., Ferguson, R., and Woodbury, B. (2002). Electrical conductivity monitoring of soil condition and available N with animal manure and a cover crop. Agric. Ecosyst. Environ. 88, 183–193. doi: 10.1016/S0167-8809(01)00256-0
Fan, J., Hao, M. D., and Shao, M. A. (2003). Nitrate accumulation in soil profile of dry land farming in Northwest China. Pedosphere 13, 367–374.
Fan, J., Hao, M., and Malhi, S. S. (2010). Accumulation of nitrate N in the soil profile and its implications for the environment under Dryland agriculture in Northern China: a Review. Can. J. Soil Sci. 90, 429–440. doi: 10.4141/CJSS09105
Gabriel, J. L., Mu, R., and Quemada, M. (2012). The role of cover crops in irrigated systems, water balance, nitrate leaching and soil mineral nitrogen accumulation. Agric. Ecosyst. Environ. 155, 50–61. doi: 10.1016/j.agee.2012.03.021
Gai, X., Liu, H., Zhai, L., Tan, G., Liu, J., Ren, T., et al. (2016). Vegetable yields and soil biochemical properties as influenced by fertilization in Southern China. Appl. Soil Ecol. 107, 170–181. doi: 10.1016/j.apsoil.2016.06.001
Geng, J., Sun, Y., Zhang, M., Li, C., Yang, Y., Liu, Z., et al. (2015). Long-term effects of controlled release urea application on crop yields and soil fertility under rice-oilseed rape rotation system. Field Crops Res. 184, 65–73. doi: 10.1016/j.fcr.2015.09.003
Gubry-Rangin, C., Novotnik, B., Mandič-Mulec, I., Nicol, G. W., and Prosser, J. I. (2017). Temperature responses of soil ammonia-Oxidising Archaea depend on pH. Soil Biol. Biochem. 106, 61–68. doi: 10.1016/j.soilbio.2016.12.007
Islam, B., Habib, S., Ahmad, P., Allarakha, S., Moinuddin, and Ali, A. (2015). Pathophysiological role of peroxynitrite induced DNA damage in human diseases, A special focus on Poly(ADP-Ribose) Polymerase (PARP). Indian J. Clin. Biochem. 30, 368–385. doi: 10.1007/s12291-014-0475-8
Johnsson, H., Bergstrom, L., Jansson, P. E., and Paustian, K. (1987). Simulated nitrogen dynamics and losses in a layered agricultural soil. Agric. Ecosyst. Environ. 18, 333–356. doi: 10.1016/0167-8809(87)90099-5
Kemmitt, S. J., Lanyon, C. V., Waite, I. S., Wen, Q., Addiscott, T. M., Bird, N. R. A. O., et al. (2008). Mineralization of native soil organic matter is not regulated by the size, activity or composition of the soil microbial biomass, a new perspective. Soil Biol. Biochem. 40, 61–73. doi: 10.1016/j.soilbio.2007.06.021
Kraft, B., Tegetmeyer, H. E., Sharma, R., Klotz, M. G., Ferdelman, T. G., Hettich, R. L., et al. (2014). The environmental controls that govern the end product of bacterial nitrate respiration. Science 345, 676–679. doi: 10.1126/science.1254070
Li, B., Dai, F., Xiao, Q., Yang, L., Shen, J., Zhang, C., et al. (2016). Nitrogen-doped activated carbon for a high energy hybrid supercapacitor. Energy Environ. Sci. 9, 102–106. doi: 10.1039/C5EE03149
Li, N., Zhang, Z., Gao, S., Lv, Y., Chen, Z., Cao, B., et al. (2021). Different responses of two Chinese cabbage (Brassica rapa L. ssp. pekinensis) cultivars in photosynthetic characteristics and chloroplast ultrastructure to salt and alkali stress. Planta 254:102.
Liu, Q., Sun, X., Hu, A. Y., Zhang, Y. A., and Cao, Z. H. (2014). Characteristics of toxic metal accumulation in farmland in relation to long-term chicken manure application, A case study in the Yangtze river delta region, China. Bull. Environ. Contam. Toxicol. 92, 279–284. doi: 10.1007/s00128-014-1204
Liu, Y., He, N., Wen, X., Yu, G., Gao, Y., and Jia, Y. (2016). Patterns and regulating mechanisms of soil nitrogen mineralization and temperature sensitivity in Chinese terrestrial ecosystems. Agric. Ecosyst. Environ. 215, 40–46. doi: 10.1016/j.agee.2015.09.012
Lu, A., Wang, J., Qin, X., Wang, K., Han, P., and Zhang, S. (2012). Science of the total environment multivariate and geostatistical analyses of the spatial distribution and origin of heavy metals in the agricultural soils in shunyi, The Center of Beijing, China. Sci. Total Environ. 425, 66–74. doi: 10.1016/j.scitotenv.2012.03.003
McLaughlin, M. (2009). Technical Bulletin, Fertilisers and Soil Acidity. No. Davidson, 4. Adelaide SA: The University of Adelaide.
Min, J., Lu, K., Sun, H., Xia, L., Zhang, H., and Shi, W. (2016). Global warming potential in an intensive vegetable cropping system as affected by crop rotation and nitrogen rate. CLEAN Soil Air Water 44, 766–774. doi: 10.1002/clen.201400785
Negm, L. M., Youssef, M. A., Skaggs, R. W., Chescheir, G. M., and Jones, J. (2014). DRAINMOD–DSSAT model for simulating hydrology, soil carbon and nitrogen dynamics, and crop growth for drained crop land. Agric. Water Manag. 137, 30–45. doi: 10.1016/j.agwat.2014.02.001
Perego, A., Basile, A., Bonfante, A., De Mascellis, R., Terribile, F., Brenna, S., et al. (2012). Nitrate leaching under maize cropping systems in Po Valley (Italy). Agric. Ecosyst. Environ. 147, 57–65. doi: 10.1016/j.agee.2011.06.014
Quan, Z., Huang, B., Lu, C., Shi, Y., Cao, Y., Wang, Y., et al. (2015). Nitrogen accumulation and loss in a high-input greenhouse vegetable cropping system elevated by application of manures. HortScience 50, 1688–1693.
Rayment, G. E., and Higginson, F. R. (1992). Australian Laboratory Handbook of Soil and Water Chemical Methods. Melbourne, VIC: Inkata Press Pty Ltd.
Riley, S. J., and Decker, D. J. (2000). Risk perception as a factor in wildlife stakeholder acceptance capacity for cougars in Montana. Hum. Dimens. Wildl. 5, 50–62.
Rong, Y., and Xuefeng, W. (2011). Effects of nitrogen fertilizer and irrigation rate on nitrate present in the profile of a sandy farmland in Northwest China. Procedia Environ. Sci. 11, 726–732. doi: 10.1016/j.proenv.2011.12.113
Ruter, J. M. (1992). Leachate Nutrient Content and Growth of two hollies as influenced by controlled release fertilizers. J. Environ. Hortic. 10, 162–166.
Sainju, U. M., Ghimire, R., and Pradhan, G. P. (2019). “Nitrogen fertilization I: impact on crop, soil, and environment,” in Nitrogen Fixation, eds E. C. Rigobelo and A. P. Serra (London: IntechOpen). doi: 10.5772/intechopen.86028
Schwertz, C. I., Do Carmo, G. M., Bottari, N. B., da Silva, E. S., Gabriel, M. E., Lucca, N. J., et al. (2016). Relationship between pathological findings and cholinesterase activity and nitric oxide levels in cattle infected naturally by Eurytrema coelomaticum. J. Comp. Pathol. 154, 150–156. doi: 10.1016/j.jcpa.2016.01.009
Shah, A. N., Iqbal, J., Tanveer, M., Yang, G., Hassan, W., Fahad, S., et al. (2017). Nitrogen fertilization and conservation tillage: a review on growth, yield, and greenhouse gas emissions in cotton. Environ. Sci. Pollut. Res. 24, 2261–2272. doi: 10.1007/s11356-016-7894-4
Sims, G. K., Ellsworth, T. R., and Mulvaney, R. L. (1995). Microscale determination of inorganic nitrogen in water and soil extracts. Commun. Soil Sci. Plant Anal. 26, 303–316. doi: 10.2136/sssaj1981.03615995004500020025
Sullivan, B. W., Smith, W. K., Townsend, A. R., Nasto, M. K., Reed, S. C., Chazdon, R. L., et al. (2014). Spatially robust estimates of biological nitrogen (N) fixation imply substantial human alteration of the tropical N cycle. Proc. Natl. Acad. Sci. U.S.A. 111, 8101–8106. doi: 10.1073/pnas.1320646111
Van Meter, K. J., Basu, N. B., Veenstra, J. J., and Burras, C. L. (2016). The nitrogen legacy, emerging evidence of nitrogen accumulation in anthropogenic landscapes. Environ. Res. Lett. 11:35014. doi: 10.1088/1748-9326/11/3/035014
Wang, J., Wang, D., Zhang, G., Wang, Y., Wang, C., Teng, Y., et al. (2014). Nitrogen and phosphorus leaching losses from intensively managed paddy fields with straw retention. Agric. Water Manag. 141:73. doi: 10.1016/j.agwat.2014.04.008
Xiong, J., Fu, G., Yang, Y., Zhu, C., and Tao, L. (2012). Tungstate, Is it really a specific nitrate reductase inhibitor in plant nitric oxide research. J. Exp. Bot. 63, 33–41. doi: 10.1093/jxb/err268
Yan, N., Marschner, P., Cao, W., Zuo, C., and Qin, W. (2015). Influence of salinity and water content on soil microorganisms. Int. Soil Water Conserv. Res. 3, 316–323. doi: 10.1016/j.iswcr.2015.11.003
Yang, X., Lu, Y., Tong, Y., and Yin, X. (2015). A 5-Year lysimeter monitoring of nitrate leaching from wheat–maize rotation system, Comparison between optimum N fertilization and conventional farmer N fertilization. Agric. Ecosyst. Environ. 199, 34–42. doi: 10.1016/j.agee.2014.08.019
Yang, Z. X., Zhou, H. P., Guan, C. L., and Xie, W. Y. (2013). Effect of long-term straw returning on distribution and accumulation of nitrate nitrogen in dryland soil. Acta Agric. Boreali Sin. 3, 32–39. doi: 10.1038/s41598-017-18152
Yousaf, M., Li, X., Zhang, Z., Ren, T., Cong, R., Ata-Ul-Karim, S. T., et al. (2016). Nitrogen fertilizer management for enhancing crop productivity and nitrogen use efficiency in a rice- oilseed rape rotation system in China. Front. Plant Sci. 7:1496. doi: 10.3389/fpls.2016.01496
Zhai, Y., Lei, Y., Wu, J., Teng, Y., Wang, J., Zhao, X., et al. (2017). Does the groundwater nitrate pollution in China pose a risk to human health. A critical review of published data. Environ. Sci. Pollut. Res. 24, 3640–3653. doi: 10.1007/s11356-016-8088-9
Zhang, J., Zhu, T., Meng, T., Zhang, Y., Yang, J., Yang, W., et al. (2013). Agricultural land use affects nitrate production and conservation in humid subtropical soils in China. Soil Biol. Biochem. 62, 107–114. doi: 10.1016/j.soilbio.2013.03.006
Zheng, H., Liu, Z., Zuo, J., Wang, L., and Nie, X. (2017). Characteristics of nitrogen loss through surface-subsurface flow on red soil slopes of Southeast China. Eurasian Soil Sci. 50, 1506–1514. doi: 10.1134/S1064229317130063
Zhongjiang, W., Kangni, C., Lili, W., Wenzhe, L., and Guangyuan, W. (2014). Influence of Surface application of biogas slurry on ammonia volatilization and dynamic distribution of soil nitrogen. Trans. Chin. Soc. Agric. Mach. 45, 139–143.
Zhou, J., Gu, B., Schlesinger, W. H., and Ju, X. (2016). Significant accumulation of nitrate in Chinese semi-humid croplands. Sci. Rep. 6:25088. doi: 10.1038/srep25088
Keywords: nitrate, ammonia, pH, EC, Chinese cabbage, PAN
Citation: Ahmed R, Mao L, Li Y, Ding J, Lin W, Ahmed S, Abbas A and Ahmed W (2022) Effect of Different Fertilizations on the Plant-Available Nitrogen in Soil Profile (0–100 cm): A Study on Chinese Cabbage. Front. Plant Sci. 13:863760. doi: 10.3389/fpls.2022.863760
Received: 27 January 2022; Accepted: 07 March 2022;
Published: 11 April 2022.
Edited by:
Adnan Noor Shah, Khwaja Fareed University of Engineering and Information Technology (KFUEIT), PakistanReviewed by:
Hanifeh Seyed Hajizadeh, Dr. Yashwant Singh Parmar University of Horticulture and Forestry, IndiaCopyright © 2022 Ahmed, Mao, Li, Ding, Lin, Ahmed, Abbas and Ahmed. This is an open-access article distributed under the terms of the Creative Commons Attribution License (CC BY). The use, distribution or reproduction in other forums is permitted, provided the original author(s) and the copyright owner(s) are credited and that the original publication in this journal is cited, in accordance with accepted academic practice. No use, distribution or reproduction is permitted which does not comply with these terms.
*Correspondence: Lili Mao, bWFvbGlsaUBjYWFzLmNu; Waseem Ahmed, d2FzZWVtdWFmMTJAZ21haWwuY29t
Disclaimer: All claims expressed in this article are solely those of the authors and do not necessarily represent those of their affiliated organizations, or those of the publisher, the editors and the reviewers. Any product that may be evaluated in this article or claim that may be made by its manufacturer is not guaranteed or endorsed by the publisher.
Research integrity at Frontiers
Learn more about the work of our research integrity team to safeguard the quality of each article we publish.