- 1Key Laboratory of Grassland Livestock Industry Innovation, Ministry of Agriculture and Rural Affairs, College of Pastoral Agriculture Science and Technology, Lanzhou University, Lanzhou, China
- 2School of Agriculture and Environment, Massey University, Palmerston North, New Zealand
The Qinghai-Tibetan Plateau is a vast geographic area currently subject to climate warming. Improved knowledge of the CO2 respiration dynamics of the Plateau alpine meadows and of the impact of grazing on CO2 fluxes is highly desirable. Such information will assist land use planning. We measured soil and vegetation CO2 efflux of alpine meadows using a closed chamber technique over diurnal cycles in winter, spring and summer. The annual, combined soil and plant respiration on ungrazed plots was 28.0 t CO2 ha−1 a−1, of which 3.7 t ha−1 a−1occurred in winter, when plant respiration was undetectable. This suggests winter respiration was driven mainly by microbial oxidation of soil organic matter. The winter respiration observed in this study was sufficient to offset the growing season CO2 sink reported for similar alpine meadows in other studies. Grazing increased herbage respiration in summer, presumably through stimulation of gross photosynthesis. From limited herbage production data, we estimate the sustainable yield of these meadows for grazing purposes to be about 500 kg herbage dry matter ha−1 a−1. Addition of photosynthesis data and understanding of factors affecting soil carbon sequestration to more precisely determine the CO2 balance of these grasslands is recommended.
Introduction
The rise in global human population during the latter half of the 20th century, and continuing at the present time, has sparked a wide range of studies aimed at defining human impact on the environment and mitigating those impacts likely to result in detrimental future change (IPCC, 2013). One of the key questions moving forward into the 21st century is how to minimize the environmental footprints of food production systems, such as release of greenhouse gases. The main greenhouse gas (GHG) present in the atmosphere is CO2, which is estimated to be responsible for 60% of the anthropogenic greenhouse effect (Rodhe, 1990). There has been a well-documented rise in atmospheric CO2 concentration from approximately 315 parts per million (ppm) in 1960 to over 410 ppm in 2020, and this rise is ongoing. Grasslands of the world are estimated to account for 40.5% of the terrestrial land area excluding Greenland and Antarctica (Reynolds, 2005) and are potentially important to managing global atmospheric CO2 concentration. Hence, a keen interest is developing to better understand ecological processes in grasslands such as carbon cycling. This understanding is expected to provide tools for planning land use at a national level, to enhance sustainability of grassland ecosystems and to maximize delivery to the population of ecosystem services to the population, such as food supply, recreational opportunity, biodiversity maintenance and climate regulation while at the same time enhancing sustainability of the grassland ecosystems providing those services.
For carbon cycling of an ecosystem the basic equation to describe the major components and their interrelationship is:
where NEE is net ecosystem exchange, GPP is gross primary production representing photosynthetic CO2 capture, and Re is the ecosystem respiration. NEE is primarily comprised of vegetation-related fluxes including net photosynthesis but can include a range of other components such as the impact of herbivores. Re can be further partitioned into above- and below-ground components (Rea and Reb, respectively) and Rea and Reb can be further separated into autotrophic and heterotrophic contributions, with heterotrophic Reb including the contribution of the soil microbial community. Hence, respiration associated with vegetation cover, (i.e., the autotrophic component of Re), is an important component of global carbon cycling and CO2 emissions to the atmosphere. The most common method for measuring NEE is eddy covariance (EC). However, EC data for NEE provides only the sum of GPP and Re without directly measuring either of them. To resolve the components of NEE, Re is often estimated from temperature data or GPP from solar radiation data. Since both of these approaches involve major assumptions, directly measured Re (and associated soil temperature data) are valuable complementary data to assist with interpretation of NEE data obtained by EC. Soil respiration over an annual cycle (i.e., Reb) was reported in one major study aggregating data of many forest ecosystems across Europe to be approximately 60% of gross aboveground primary production (GAPP; Janssens et al., 2001). A synopsis of the major components of the global carbon cycle by Rustad et al. (2000), also highlighted the importance of soil respiration but noted that there are technical difficulties and high errors when measuring it, and therefore a knowledge gap in this area. We are not aware of a comparable compilation of grassland data but a first expectation would be that soil respiration in grassland would be a similar or higher proportion of GAPP. These authors considered it likely that global warming will generally increase soil respiration, so releasing more CO2 and further increasing global warming. In another review of the global carbon cycle directed at informing policymakers, Schlesinger and Andrews (2000) identified the ratio of soil respiration: GAPP as 75:120 (62.5%) and stated that “nearly all models of global climate change predict a loss of carbon from soils as a result of global warming,” and note that the response is greatest in soils in cold climates. It is indicative of the difficulties measuring the contribution of soil respiration to ecosystem carbon cycling, that almost a decade after these two reviews, a European study referred to the potential for C sequestration by world grasslands as “speculative,” and of “uncertain quantitative importance” (Rogiers et al., 2008). That particular study demonstrated that a mountain hay meadow ecosystem studied in Switzerland was a net source of CO2 over a 3-year study period from 2002 to 2005 (1.2–2.6 t C ha−1 a−1) and that losses were increased by grazing and by snow cover in winter. The sustained release of CO2 in these alpine meadows was attributed to ongoing oxidation of soil carbon as a consequence of drainage that had been carried out around 1940. Elsewhere, a 2-year study of the growing-season carbon balance in natural Miscanthus sinensis grassland in Japan (Toma et al., 2011) was unable to determine with certainty if the site studied was a net source or sink for CO2 on an annual basis.
These uncertainties from earlier studies highlight a general need for additional insight into the Re contribution to C cycling and C source-sink status of grasslands, and Chinese grasslands are an ideal “model system” for such a study. In China, the steppe grasslands and alpine meadows of Inner Mongolia and the Qinghai-Tibetan Plateau cover a total area of some 240 million ha, excluding the more extreme desert environments. On the Qinghai-Tibetan plateau the prevailing cold climate predisposes to formation of dark soils with high organic matter content and a mollic epipedon. For one of these soils, Yuan and Hou (2015) reported soil organic C contents >12% at sites with lower historic grazing intensity and reducing to 4%–5% under sustained intensive grazing. Estimates of the carbon storage of Qinghai-Tibetan Plateau grassland soils vary but range from 6.5 to 21.4 kg C m−2 and 7.4–35.4 Pg total C stock (Fang et al., 2010). In one study of alpine meadow grassland on the Tibetan Plateau, grazing exclusion for three or 5 years increased NEE by 47.37% and 15.84%, respectively, and increased growing season carbon sequestration accordingly, compared with a free grazing treatment (Chen et al., 2015). With China’s large population of 1.4 billion in 2020, there is now wide recognition that these extensive grassland ecosystems will have an important role in sustainable support of the human population in future. Therefore, more information and good contextualization of that research is needed in order to position the pastoral industry in this geographically large area for optimal contribution of ecosystem services to the human population and for formulation of C budgets and definition of the global contribution to greenhouse gas cycling.
Here, we present data on soil-to-atmosphere CO2 fluxes directly measured using the closed chamber technique in a botanically diverse alpine grassland meadow typical of mid-altitude localities on the Qinghai-Tibetan plateau. Our study was designed to quantify both diurnal and seasonal change in the respiration components of ecosystem CO2 flux, Rea and Reb, and how these fluxes respond to sheep stocking rate (The sum of Rea + Reb would be estimated by measurements on intact herbage while Reb would be estimated by measurement of an adjacent area with herbage removed at ground level, and Rea found by difference.). These data will complement those available from eddy covariance studies where Rea and Reb are seldom measured directly and separately and where alternative grazing managements are seldom compared because of scale factors implicit in the eddy covariance technique. We also measured soil microbial biomass, which may allow some inference about the contribution of heterotrophic soil microorganisms to Reb under different sheep stocking rates. Our hypothesis was that with warming climatic conditions and increasing animal numbers on the Qinghai-Tibetan plateau during the last 30 years, soil respiration losses of C from the ecosystem may be increasing as predicted in the European literature cited above and may be now larger than generally realized. We wished to quantify the current C-respiration losses in order to provide data for future C-balance determinations for these alpine meadows.
Experimental
Study Site
The study was conducted on a 20 ha, botanically diverse, fenced alpine meadow located on the eastern Qinghai-Tibetan Plateau at the Maqu County Azi Livestock Breeding Base (Lat. 35°58′N, 101°53′E; 3,750 m elevation) in Gansu Province, People’s Republic of China. This area is classified as alpine humid grassland (alpine meadow), with a frost-free period of 90–100 days, an annual mean air temperature of 1.2°C, and monthly mean maximum 11.7°C in July and minimum of −10 in January. The mean annual precipitation of approximately 620 mm is distributed unevenly among seasons, primarily falling as rain during the short, cool summer (Table 1). The annual cloud-free sunshine is about 2,580 h. Inter-annual variation in temperature and rainfall for the previous 45 years is shown in Figure 1 and time trends for this data are evaluated in “Maqu County Climate Data” section below. The soils at the experiment site are well drained so accumulation of excess soil moisture should not have been a major factor in determining respiration responses we measured.

Table 1. Seasonal variation in mean monthly temperature (°C) and monthly precipitation (mm) for Maqu County where the experiment was located.
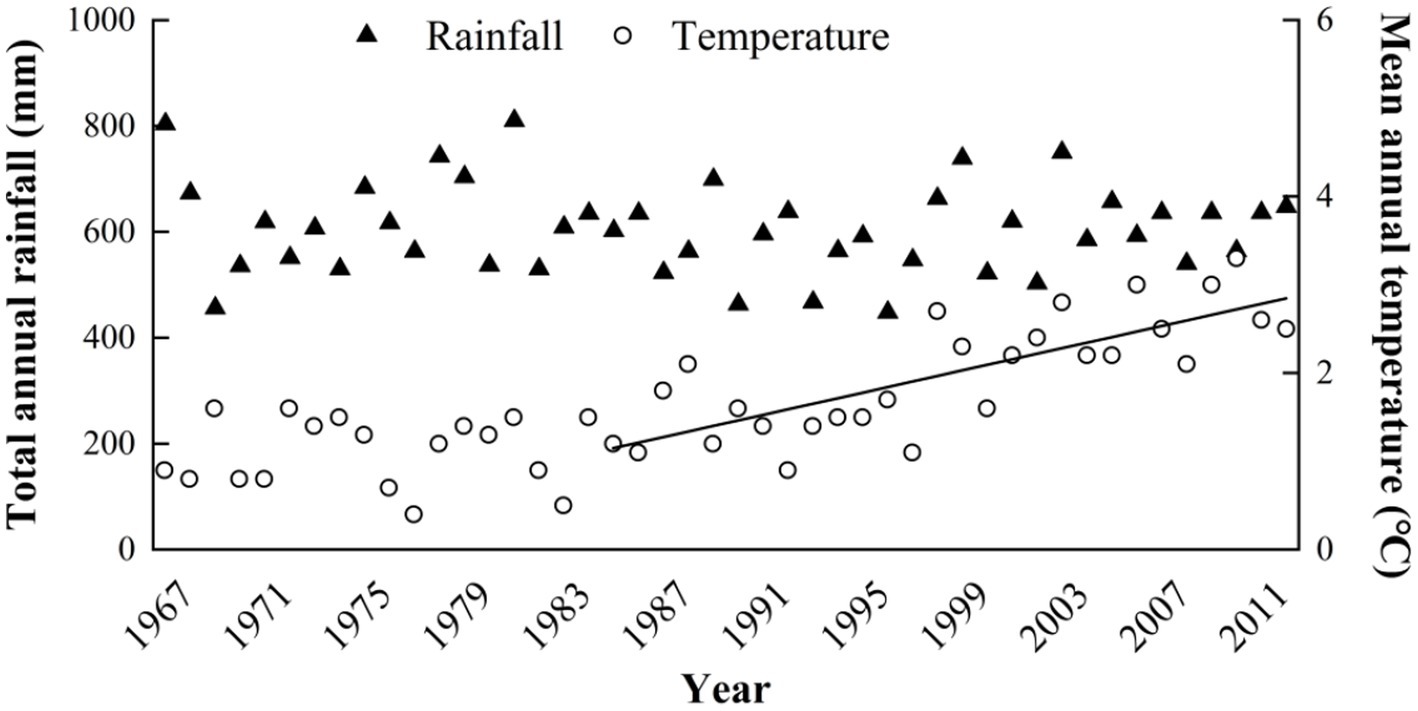
Figure 1. Data for annual precipitation and annual mean temperature (AMT), 1967–2012 provided by the Gansu Meteorological Bureau for Maqu County, Tibet, where the experiment was located. Equation for Regression of mean annual temperature (MAT) on year was (taking 1967 as year zero): MAT = 1.09 + 0.0027 (SE + 0.0148) °C per year (NS) for the 20-year period 1967–1986 and MAT = 0.094 + 0.0637 (SE + 0.0090) °C per year (p < 0.001) for the 30-year period 1983–2012 (as indicated by the trend line).
Grazing Management and Herbage Intake of Animals
The experiment was set up as a lamb production system with lambs purchased at 6 months of age in late May and sold for slaughter in late December of each year, commencing in 2010. Two visually homogeneous areas located about 300 m apart on a high alluvial plain were selected for July to September (summer) and October to December (winter) grazing and fenced to provide four replicate plots sized 100 × 100 m (low stocking rate) and four further plots sized 100 × 50 m (high stocking rate), in both the summer and winter grazing areas. In each of the 16 plots created in this way, a 10 × 10 m sub-plot was fenced so samples from ungrazed pasture could be collected for pairwise comparison with data from the grazed pastures. All plots were stocked with eight sheep and rotationally grazed. For grazing, each paddock was divided into three strips of equal area and the eight sheep spent 10 days on each strip. The low and high stocking rates therefore involved the equivalent of 1.97 or 3.95 lambs ha−1 continuously stocked during daylight hours, but with sheep housed at night. Three 30-day cycles were completed in this way on both the summer and the winter grazing areas. However, for simplicity we will refer to the grazing treatments in terms of animals per unit area during grazing of plots: 8 and 16 sheep ha−1, respectively. Animals were weighed monthly and herbage consumed by the animals was estimated based on energy requirements of the animals, as described by Chen et al. (2010).
Our animal night-housing facility is of a standard design used in China and conforms to the national standard “Laboratory Animals Environment and Housing Facilities” (GB 14925-2010), the care of animals during the experiment conformed to relevant Chinese government protocols.
CO2 Efflux
The soil-atmosphere CO2 fluxes in the field were measured by a static closed chamber method (Levy et al., 2011). Two identical cube-shaped chambers (40 × 40 × 40 cm) were constructed from stainless steel. The chambers were sheathed in foam plastic for improved temperature stability and fitted with an internal fan to ensure complete gas mixing and with a port with a septum for gas sampling. For a gas seal at the soil surface, the bottom edge of each chamber was seated into a water filled gas flux barrier collar inserted to a depth of 7 cm into the soil and protruding 2 cm above the soil surface. Gas sampling was performed with plastic syringes (20 ml capacity) fitted with three-way stopcocks and connected to multilayer foil/plastic sampling bags commercially available in China for storing gas samples collected for research purposes (Dalian Delin Gas Packaging Co. Ltd.). At collection, samples were withdrawn into the syringe and then, after switching the stopcock, injected immediately into the plastic gas sampling bags. Samples were analyzed within 12 h by a DLT-100 Greenhouse Gas Analyzer (GGA) with syringe injection (Model 908-0011). During gas sampling, two chambers were normally installed about 1 m apart in the same plot, one with herbage intact and one with herbage clipped to expose bare soil. High and low stocking rate plots in each replicate were sampled simultaneously, thus utilizing the four chambers. Grazed and ungrazed partitions of each plot were sampled on successive days. For logistical reasons, collar placement and clipping of herbage to ground level occurred the day before gas flux measurement. Each time the chamber collars were installed on a new plot, any dung inside the perimeter was removed by hand before closing the chamber. In each sampling “run” after a chamber was placed into its collar, four gas samples were collected 0, 10, 20, and 30 min after closing the chambers. Soil temperature (5 cm depth) inside the chamber at the start of each sampling run and chamber air temperature at the start and end of each sampling run were recorded. After collars were placed in a plot, sampling runs were carried out at 4:00, 8:00, 10:00, 12:00, 14:00, 16:00, 18:00, 20:00, and 24:00 h for each plot, without moving the collars, to define diurnal changes in gas flux. Thus, a complete seasonal measurement cycle of four grazed plots and their ungrazed partitions required 288 runs: (high or low stocking rate) x (intact vegetation or bare soil) x (the diurnal cycle of nine sampling times) x (grazed or ungrazed) x (four replicates). Gas sampling was carried out in winter (late November to early December 2010), in spring (late April to early May 2011) and in the summer growing season (late July to early August 2011). For spring and summer samplings the number of chamber runs was reduced from 288 to 216 by sampling only one of the two ungrazed control partitions in each replicate of high and low stocking rate treatments. Each seasonal set of 288 or 216 sampling runs was completed within 14–21 days.
CO2 gas concentration changes in the chamber were determined by regression analysis of concentration on time (see below) with units of ppm min−1 using the equation:
Where: F = gas flux (mg m−2 h−1), ρ = gas density at 273°K and 1 atmosphere pressure (kg m−3), V = chamber volume (m3), A = soil contact area of the chamber (m2), P = measurement pressure (for Tibet ~67 kPa), P0 = reference pressure (1 atmosphere, 101.3 kPa), T0 = reference temperature (273°K), T = measurement temperature (°K), dCt/dt = rate of change of gas concentration in the chamber (ppm min−1). From Eq. 2, assuming a CO2 density of 1.902 kg m−3 at 280°K and 100 kPa pressure, an internal chamber height of 42 cm including the seating channel, and further assuming a temperature and pressure of 5°C and 67 kPa, respectively (typical of average conditions on the Qinghai-Tibetan plateau), then 1 ppm min−1 CO2 flux in the chamber used in this research = 32.46 mg m−2 h−1 CO2 flux from an ecosystem perspective.
To scale up from plot scale to annual fluxes a weighted average of diurnal fluxes was calculated for each season and winter values assumed for months November to March, spring values for months April to June and summer values for months July to October. When performing these calculations, temperature response coefficients from regression analysis as described below were used to adjust CO2 flux data for the measured temperature difference between bare soil and soil with vegetation intact (Li and Sun, 2011).
Herbage Mass
Biomass change between the start and end of grazing in the summer grazing plots in 2011 was measured by cutting, drying and weighing herbage from four 0.25 m2 quadrats in each plot. Herbage mass data were not collected from winter grazing plots in 2010 but were collected when identical grazing management treatments were imposed in late 2011.
Microbial Biomass Determination
Soil samples were collected from each plot in conjunction with gas sampling in winter 2010 and spring and summer 2011. The microbial biomass fraction of soil C was determined using a chloroform fumigation extraction method (Oren et al., 2018). Briefly, organic C was extracted from fumigated and unfumigated soil samples using 0.5 M K2SO4 solution, and the extract passed through standard filter paper, and vacuum-filtered with 0.45 μm millipore filters to remove particulate matter. Organic carbon in solution was then oxidized using a known quantity of a K2Cr2O7/H2SO4 reagent, and the unused oxidizing reagent quantified by back-titration with Fe2SO4 solution and phenanthroline as a redox indicator to determine the titration endpoint, as described by Fan et al. (2013). Soil microbial biomass C was calculated as 2.64 × the difference in soil organic C between fumigated and unfumigated soil samples, implying an assumption that 38% of microbial biomass C had been evolved during incubation following fumigation (Oren et al., 2018).
Statistical Analysis
A multiple regression analysis using Type III sums of squares in the GLM procedure of SAS (SAS Institute 2011) was employed to analyse the data. For each of the three seasons separately, the gas concentration data for CO2 measured in the chambers (1,152, 864 and 864 data points in winter, spring and summer, respectively, accumulated across the measurement cycles in each season) were regressed on minutes since chamber closing to give a regression slope representing the rate of CO2 accumulation inside the chamber in ppm min−1. Terms for the interaction between “min” (minutes since chamber closure) and other experiment treatment factors were added to the model to evaluate the change in rate of CO2 efflux for intact vegetation versus bare soil, the slope difference associated with grazing intensity, and for variation in air or soil temperature. The SAS model used for the combined data set in each season can be represented as follows:
where PPMct denotes the measured CO2 concentration (ppm) in the chamber at t minutes (0, 10, 20, or 30) from chamber closing, Ambient is the regression intercept representing measured CO2 concentration at chamber closing (t = 0 min), Temp is chamber temperature during the measurement run, PPM.min–1 SOIL is the contribution of soil respiration in ppm min−1 to PPMct (estimating Reb), PPM.min−1 HERB is the contribution of herbage respiration in ppm min−1 to PPMct (estimating Rea), PPM.min−1 GRAZ is the effect of grazing intensity on PPMct in units of ppm min−1 (sheep/ha)−1, and a, b, c, and d are parameters estimated by the multiple regression.
A model including a term for hour of the day was also evaluated. Because slope coefficients in a multiple regression can be affected in complex ways by correlations between experimental factors such as air temperature and soil temperature, we examined the impact of air and soil temperature on CO2 flux in separate runs of the SAS model. Separately from the full model described above, we also performed regression analyses to determine the linear and quadratic responses of CO2 efflux to temperature for bare soil and intact herbage, averaged over grazing intensity effects. For the purposes of obtaining coefficients for scaling up from the experiment to the ecosystem level, weighted averages of slope coefficients for individual gas flux measurement runs for time periods of interest were also calculated where statistical significance had been confirmed by multiple regression analysis as described above.
Results
Maqu County Climate Data
Visual inspection of climate data (Figure 1) suggested a warming trend for mean annual temperature (MAT), beginning in the mid 1980s. This was statistically tested by linear regression. For the arbitrary 20-year period 1967–1986 the linear regression equation was (taking 1967 as year zero): MAT = 1.09 + 0.0027 (SE ± 0.0148) °C year−1 (NS). However, for the 30-year period 1983–2012 (a standard 30-year climate evaluation cycle), the equation was MAT = 0.094 + 0.0637 (SE ± 0.0090) °C year−1 (p < 0.001).
Diurnal Temperature Ranges During Gas Flux Measurement
The diurnal range of air temperature recorded in the chambers during the three measurement cycles was wide and the daily temperature peak was of short duration. For the three seasons, maximum, minimum and mean air temperatures, respectively, were: summer, 33.0, 10.0, and 16.9°C; winter, 14.8, −13.2, and −2.4°C; spring, 25.6, −2.1, and 8.0°C. The diurnal range of soil temperature was much less then for air temperature. Notably, mean winter soil temperature averaged exactly 0°C, with midday temperatures often above freezing (Figure 2).
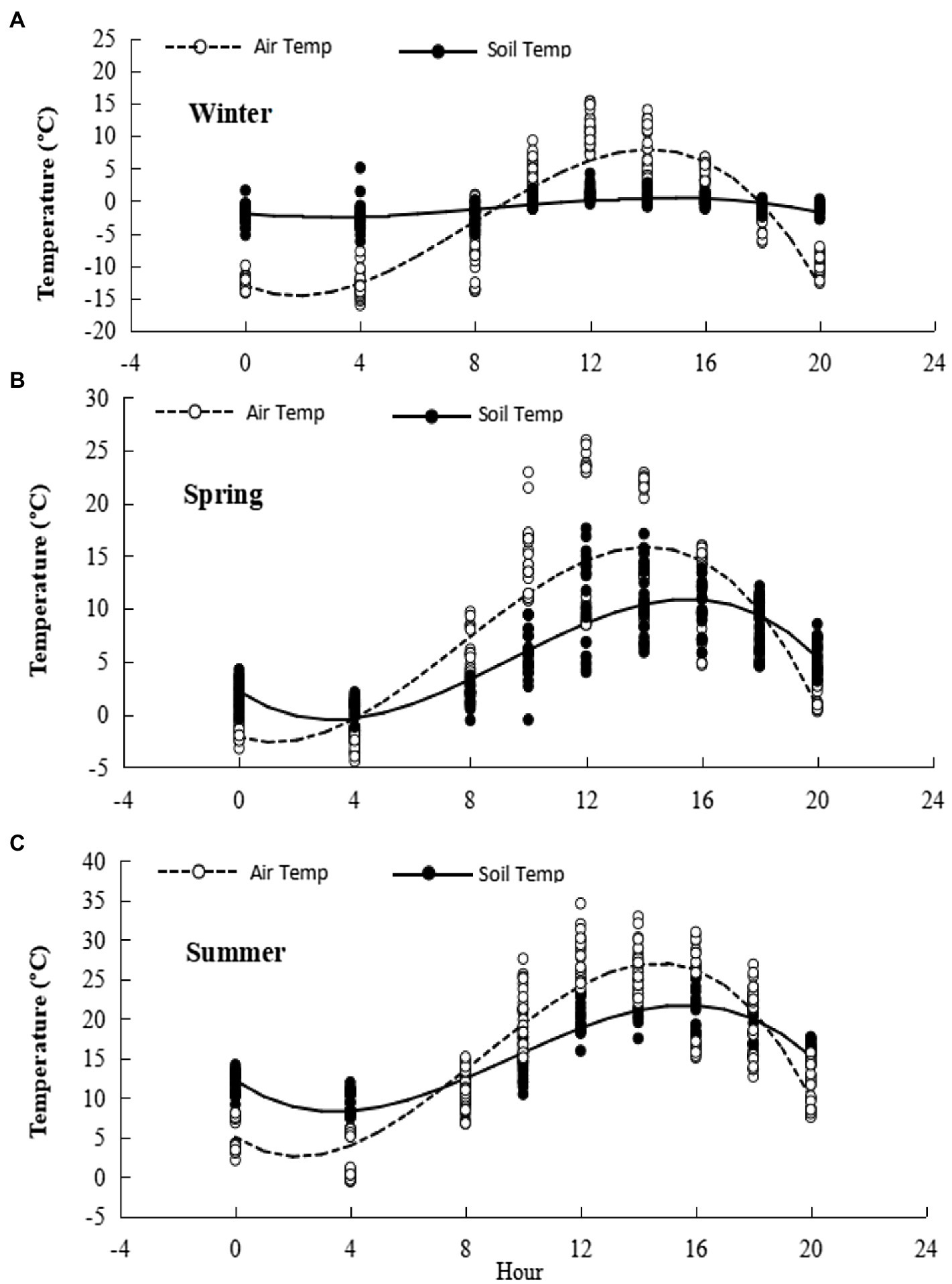
Figure 2. Diurnal temperature ranges during gas flux measurement. Cubic equations for fitted diurnal trends are presented in Supplementary Table S1. (A) Winter, (B) Spring, (C) Summer.
CO2 Fluxes From Soil and Herbage Respiration
Seasonal changes in the temperature effects on rate of CO2 efflux from bare soil (filled circles) and soil with intact vegetation (hollow circles) are shown in Figure 3. Notably, the multiple regression coefficient for bare soil respiration at 0°C was relatively constant over the seasons and indicated “background” respiration occurring in winter at a rate approximately 50% of that in spring and summer. However there was seasonal variation in the response of respiration rate to temperature, with a very small but statistically significant negative response to temperature increase in winter, a small positive response to temperature increase in spring, and a much larger response to temperature increase in summer. When quadratic curves were fitted to the respiration response to temperature, the quadratic coefficient was significantly positive in spring and summer but not winter (Table 2). Herbage respiration was statistically undetectable in winter, small in spring, and was the dominant contribution to respiration in summer (Figure 3; Table 2). Fluxes of CO2 were statistically more strongly correlated with air temperature than soil temperature in winter, but air and soil temperature correlated equally well with CO2 flux in spring and summer.
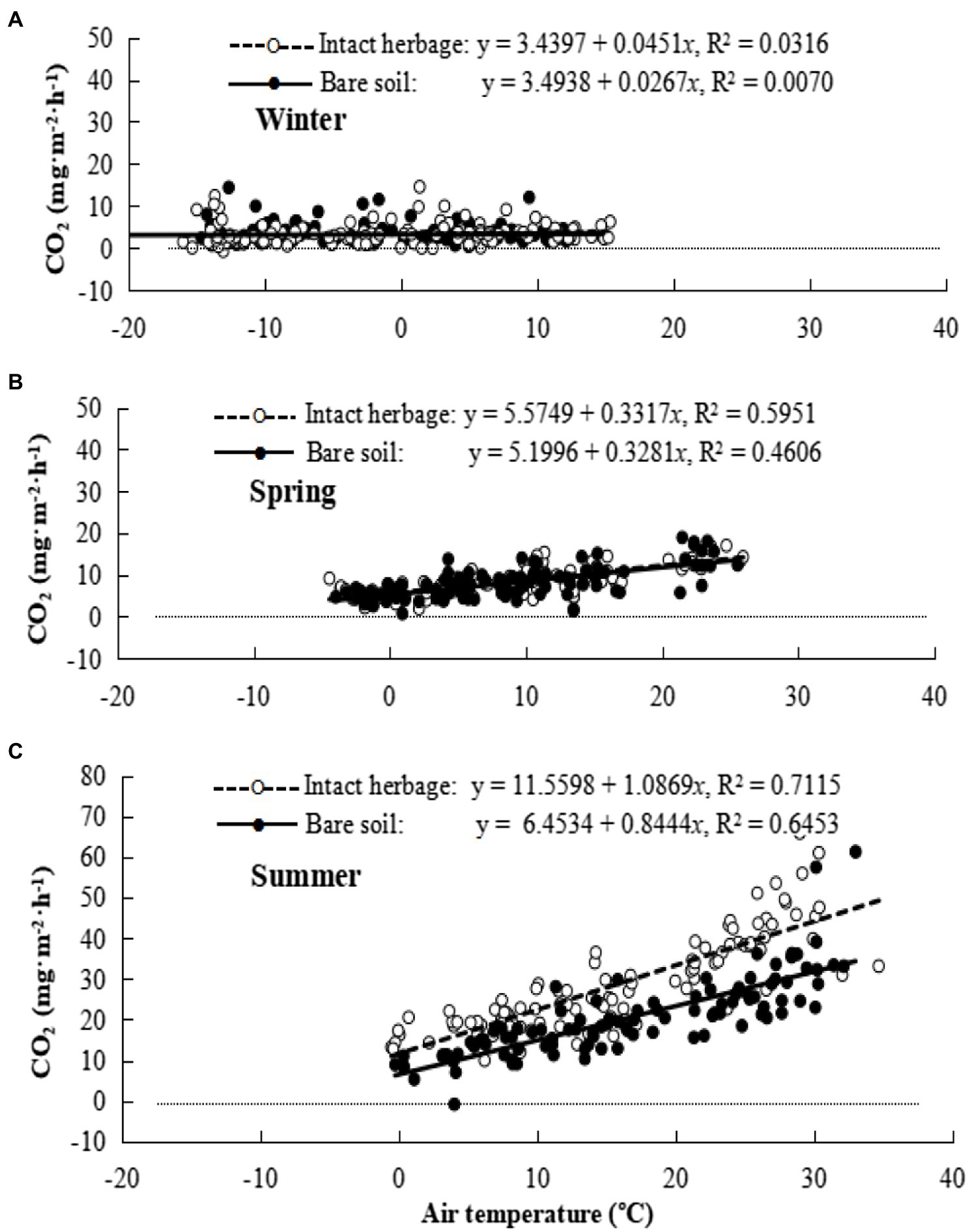
Figure 3. Seasonal carbon dioxide (CO2) emission for bare soil and intact herbage. (A) Winter, (B) Spring, (C) Summer.
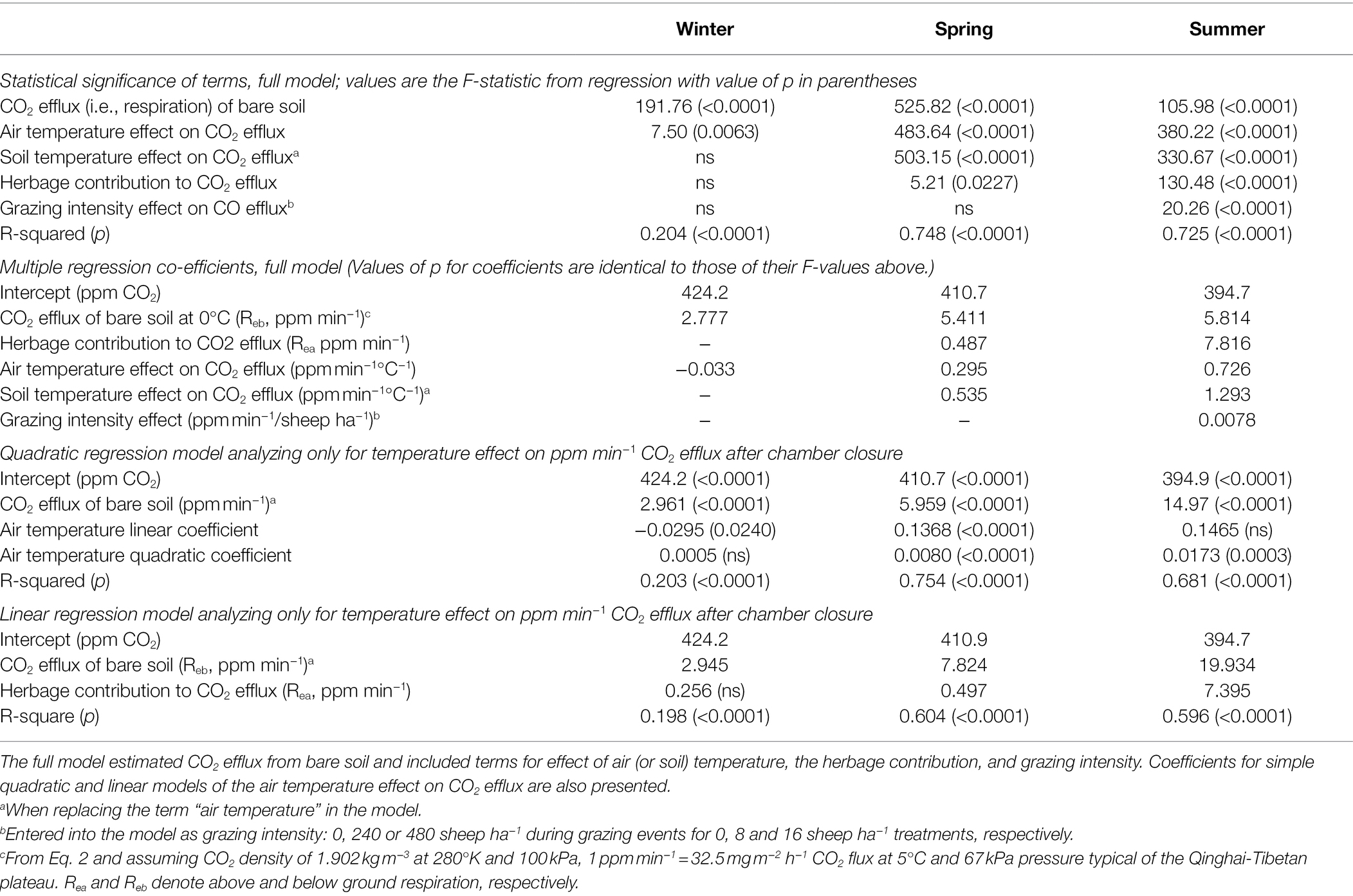
Table 2. Statistical significance (F-statistic from regression analysis and value of p) of terms and multiple regression coefficients for GLM analysis of CO2 efflux measured by a chamber technique.
A statistically significant positive correlation between grazing intensity and soil respiration was detected in summer (Table 2), but not winter or spring. Inclusion of a model term for hour of the day (results not presented) indicated a diurnal variation of intercept consistent with higher CO2 concentration at chamber closure during the night than during the day, but otherwise did not substantively change the results presented in Table 2.
Using coefficients in Table 2, soil respiration rates at this site without grazing and with air temperature at 5°C are estimated to be 223 and 307 mg CO2 m−2 h−1 in spring and summer, respectively, and 96 mg CO2 m−2 h−1 with soil and air temperatures at −5°C in winter. Herbage respiration increased CO2 emission (compared to bare soil) by 16 and 254 mg CO2 m−2 h−1 in spring and summer, respectively, but not unexpectedly was negligible in winter. The diurnal pattern of respiration for herbage and bare soil for the three measurement periods is shown in Figure 4. Notably, winter soil and herbage respiration fluxes were nonzero while spring fluxes more resembled those of winter than summer. The mean CO2 effluxes across the diurnal cycle measured for bare soil were 98, 214 and 566 mg CO2 m−2 h−1 in winter, spring and summer, respectively, while values for chambers with intact herbage were, respectively, 10, 31 and 209 mg CO2 m−2 h−1 greater than for bare soil. For comparison with other published studies, the seasonal values for Re (i.e., Rea + Reb) calculated from CO2 fluxes illustrated in Figure 4 were 3.9, 5.4, and 22.9 t CO2 ha−1 for winter, spring, and summer seasons, respectively, with the proportion of Re derived from Reb being, respectively, 91%, 87%, and 73% in the same seasons. Herbage respiration was significantly increased on grazed plots, compared to ungrazed plots in summer only (Table 2).
Herbage Mass at the Start and End of Grazing
Herbage mass averaged approximately 750 kg DM ha−1 at the start of summer grazing and was approximately maintained under grazing but increased in the same period to a little over 1,000 kg DM ha−1 on Control plots. In winter-grazed plots herbage mass at start of grazing was similar to that in control plots at the end of summer grazing but was greatly reduced by grazing to approximately 400 kg DM ha−1 (Figure 5).
Seasonal Data for Soil Microbial Biomass
Soil microbial biomass C values averaged approximately 2.5 g kg−1 dry soil with no observed response to grazing in the winter and summer measurements, but were higher and exhibited a grazing intensity response in spring (p = 0.015), ranging from 3.19 g kg−1 dry soil on control plots to 4.39 g kg−1 dry soil (SEM 0.39 g k−1 dry soil) in plots grazed at 16 sheep ha−1 (Table 3).
Scaling Up to Ecosystem Level and Impact of Grazing
Calculated herbage removal by grazing animals was greater than the combined total of decline in herbage mass during grazing and herbage accumulation on ungrazed plots for both stocking rates in both summer and winter, indicating either reduced senescence of herbage on grazed plots or a possible stimulatory effect of grazing on herbage accumulation. The estimated energy yield of these grazing systems was a modest 5–10 GJ ha−1 a−1. Further investigation of the data used for the regression analysis in Table 2 showed that the increased CO2 efflux on grazed plots in summer was largely caused by grazing related increase in herbage respiration, not soil respiration (Table 3). From these data, the annualized estimate for soil + herbage respiration rate was 28.0 t CO2 ha−1 a−1, and the increase in respiration associated sheep grazing estimated by the model, while statistically significant, was a modest 0.075 and 0.149 t CO2 ha−1 for the summer period for the stocking rates of 8 and 16 sheep ha−1, respectively (Tables 2 and 3). Combining NEE data of Figure 5 in Kato et al. (2004b) for similar vegetation 60 km distant with our own Rea and Reb estimates we arrived at a tentative estimate of herbage GPP of 25.3 t CO2 ha−1 a−1 (Table 3).
Discussion
Seasonal Characteristics of Respiration Fluxes in Tibetan Plateau Alpine Meadow Pastures
Our study collected respiration data for intact vegetation and bare soil in ungrazed and grazed plots across three seasons in alpine meadows on the Qinghai-Tibetan Plateau in order to quantify seasonal and grazing-induced variation in soil and herbage respiration.
For winter, our salient finding was that CO2 efflux was not zero as might be intuitively supposed from the low winter temperatures, but was consistently positive, and amounted to 3.7 t CO2 ha−1 a−1 for the winter period. The fact that our regression model found no detectable herbage contribution to CO2 efflux in winter (Table 2) implies that plant leaf tissues were physiologically inactive, that photosynthesis in winter would also have been negligible, and root systems likely also dormant. This is also supported by Kato et al. (2004a) where it is shown that the temperature sensitivity (Q10) of CO2 efflux of a Kobresia meadow on the Qinghai-Tibetan Plateau showed a marked transition around −1.0°C. This suggests that winter Reb activity may be coming mainly from microbial decomposition of soil C, rather than from plant root respiration. Subsequent to our study, others (e.g., Song et al., 2020) have confirmed the positive Re values of alpine meadow pasture in winter and that grasslands on the Qinghai-Tibetan Plateau are CO2 sinks in the growing season and CO2 sources in winter.
Our data from spring are notable for the lag between the rise in Rea and rise in Reb as temperatures rose in spring. For example, mean soil temperatures over the winter, spring and summer measurement periods for which diurnal temperature curves are shown in Figure 2 were, respectively, −0.8, 6.4, and 16.5°C while model estimates (Table 2) of winter, spring and summer Reb were, respectively, 2.8, 5.4, and 5.8 ppm CO2 min−1 (i.e., spring values were close to summer values). By contrast winter, spring and summer estimates of Rea were, respectively, 0, 0.49, and 7.8 ppm CO2 min−1 (i.e., spring values were close to winter values). This implies that not even the whole growing season is available for carbon sequestration through leaf litter and root deposition to replace soil C respired during the comparatively long winter, and raises an interest in extending the current measurements to explore C balance factors in future research.
A relevant earlier study with which to compare our data is that of Kato et al. (2004b). These authors used the EC technique to determine NEE for an alpine meadow with botanical composition similar to that at our study site and in the same region. Two of four species listed as dominant by Kato et al. (2004b) (Kobresia humilis and Kobresia tibetica) were also present at our site and a third Kobresia species (Kobresia graminifolia) was one of the dominant species at our site (Sun et al., 2018). Kato et al. (2004b) note that the alpine Kobresia meadow ecosystem is one of the most widely distributed vegetation types on the vast Qinghai-Tibetan Plateau and occurs from 3,200 to 5,200 m altitude. They suggested that carbon budgets depend more on vegetation type than local geological conditions, so that their results may be taken as indicative for large areas of the Plateau. Their reporting focuses on diurnal fluxes and provides little clarity as to components of NEE identified in Eq. 1 above. They concluded from their EC NEE data that during the growing season from late May to the end of September there was a “small” net CO2 sequestration which we determined by extracting data from their Figure 5 to be 5.1 t CO2 ha−1.
It is interesting to note that the calculated winter Reb from our study of 3.9 t CO2 ha−1 (apparently from oxidation of soil C as there was no herbage activity at this time), was only marginally smaller than the growing season sequestration of 5.1 t ha−1 recorded by Kato et al. (2004b) and that in late May those authors recorded very low daytime CO2 uptake, suggesting a herbage dormancy like that which we observed in our spring sampling in early May remained a feature of the ecosystem behavior at that point in the growing season. Further, neither our study nor that of Kato et al. (2004b) provide data for the months of April and October at the shoulders of the growing season, where based on present data there may also be a negative ecosystem carbon balance.
Another of our research objectives was elucidation of how Rea and Reb respond to imposition of sheep grazing. The only significant grazing effect on Re detected by the regression analysis presented in Table 2 occurred in summer and further analysis (Table 3) showed that this effect primarily involved grazing stimulation of herbage respiration (Rea), with little change in Reb, and a tendency for grazing to decrease, rather than increase Reb. This finding was corroborated by comparatively small grazing-intensity-related changes in soil microbial biomass. Studies in North American rangeland (Thomas, 2012), Inner Mongolian steppe grassland (Tang et al., 2015), and a previous study in the Tibetan Plateau (Unteregelsbacher et al., 2011) have all reported reduced soil CO2 efflux under grazing, compared to ungrazed pastures, in common with the trend in our own data (Table 3).
A range of factors are likely involved in the apparent stimulation in herbage production under grazing and the associated summer increase in Rea. Firstly, the concentration of nutrients in dung and urine patches by grazing animals would have been expected to decrease soil respiration and increase herbage production (Wu et al., 2009; Kaštovská et al., 2010). Secondly, increased grazing intensity alters the temperature dependence of soil CO2 efflux, increasing soil CO2 efflux at a given temperature, and also increases soil temperature (Unteregelsbacher et al., 2011). Soil respiration response to increasing temperature should be enhanced at elevated temperatures (Yuste et al., 2009). The temperature sensitivity of organic matter decomposition decreases with increasing temperature, as indicated by the Q10 decreasing with temperature to be about 3.2 at 12°C and 1.4 at 24°C (Zhang et al., 2015), the logarithmic soil temperature-CO2 efflux relationship of Unteregelsbacher et al. (2011), and the significant quadratic relationship in the present study (Table 2; Figure 3C) also demonstrated this point. Thirdly, Fterich et al. (2012) demonstrated that microbial biomass of soil is maximal at low-to-intermediate levels of grazing influence and that the phenotypic evenness of the microbial community declines as the intensity of grazing increases, and soil microbial communities of heavily grazed sites are dominated by bacterial-based channels of decomposition. Lastly, a recent study of differentially expressed genes in Stipa grandis in steppe grassland in Inner Mongolia has shown that increased grazing pressure promotes expression of the Calvin-Benson cycle (Dang et al., 2021). Others have also noted that herbivory has a generally positive feedback effect, and can promote plant regrowth as well as energy and nutrient flows in grazed landscapes (Zhang Y. J. et al. 2014). All such effects on soil microbial dynamics feedback to soil respiration indirectly (Guo et al., 2012; Li et al., 2016).
Implications for C-Balance and Sustainable Farming of Tibetan Alpine Meadow Pastures
A question of high topical interest with respect to Rea and Reb data like those from the present study, is whether or not there is evidence that increased grazing intensity of alpine meadows on the Qinghai-Tibetan plateau coupled with climate warming may be tipping these farming systems from C-sink to C-source status. In general herbivores consume plant tissue that would otherwise be cycled to the soil and exhale much of that directly to the atmosphere as CO2 from respiration. In addition, excreta returns are concentrated in small patches rather than distributed evenly across the grazed area, implying higher losses than when leaves senesce and die in situ (Parsons et al., 2013). Therefore, grazed plots might be expected to show a decline in soil C over time through lack of replenishment, compared to ungrazed plots. As noted above, Schlesinger and Andrews (2000) broadly predicted such effects, and Yuan and Hou (2015) documented evidence grazing-related soil C decline over time in deer pastures in the Qilian mountains. Alpine meadow soils of the Tibetan plateau typically contain about 7% C (Pei et al., 2008) with this high carbon content extending to 30–40 cm in depth. These soils are reported by Rui et al. (2011) to cover an area of about 110 million ha. Hence, there is potential for any change in their response to have a global impact on greenhouse gas accounting. A point of great interest at the present time is whether or not these soils remain a CO2 sink as they have been historically, or have become a net source.
Ultimately, such questions are likely to be answered by studies that incorporate both EC measurements of NEE, and direct measurements of one or more NEE components such as respiration or photosynthesis. Such studies are currently rare or non-existent because of logistical challenges. Our very tentative attempt to make such a calculation by combining our Re data with NEE data extracted from Figure 5 of Kato et al. (2004b) and the estimate for GPP of 25.3 t CO2 ha−1 a−1 would indicate these ecosystems to now be CO2 sources, not CO2 sinks, but this estimate requires confirmation using data collected at the same site. Meanwhile, some comments are relevant on other approaches to determining C-balance of these ecosystems and issues that might arise based on findings of this study.
An approach, used by Wang et al. (2019) among others, is to estimate Re from published relationships with soil temperature. These authors also did not conduct measurements in winter. However, it is clear from discussion in “Seasonal Characteristics of Respiration Fluxes in Tibetan Plateau Alpine Meadow Pastures” section above and from data of Figure 5 of Kato et al. (2004b) that in Tibetan Plateau Kobresia meadows there is a burst of herbage metabolic activity within a comparatively narrow time window during the months of April to October within the growing season so that activity predictions based on temperature, while they might capture soil microbial dynamics, would not capture associated herbage dynamics well. Hence, more data to define Rea and Reb and differentiate their heterotrophic and autotrophic components on the shoulders of the growing season in the months of May and October, in particular, are required for a complete understanding.
Another line of investigation for characterizing ecosystem source sink status is inventory of change in soil C content across time. Using this approach, Yang et al. (2010) concluded that soils on the Tibetan plateau have been carbon neutral for the last 20 years. Such results can be quite influential in causing redirection of research resources to other areas deemed a higher priority. However, this methodology would only detect accumulated change over time and would not be expected to detect an emerging trend in its early stages. Yang et al. (2010) indicated 127 ± 9 t ha−1 soil organic C to 100 cm, while the CO2 efflux indicated in Table 3, building linearly from zero over the 5 years prior to our study, would reduce soil C by about 7 t ha−1, or less than the measurement error for soil C in the study of Yang et al. (2010). Hence, their findings do not necessarily disprove the above conclusions that there is a concerning level of winter Reb in these meadows, that may signal a shift toward a C-balance tipping point and will certainly be exacerbated by warming and any reduction in soil moisture associated with climate change.
There is also a precedent from a recent study of alpine soils in Europe, Rogiers et al. (2008) observed net annual CO2 efflux from alpine soils in Switzerland, and attributed the CO2 release to ongoing soil organic matter oxidation following drainage 40 years earlier. The Qinghai-Tibetan Plateau appears to be experiencing a similar soil-drying trend associated with the temperature increase indicated in Figure 1, despite there being no evidence of change in precipitation. For example, the total lake area of the Yamzhog Yumcoin southern Tibet decreased by about 67 km2 during 1980–1990 (Ye et al., 2008) and these authors concluded this was most likely a result of change in balance between precipitation and evaporation in the valley resulting from climate change. Study to determine if trends toward reduced soil moisture content might also be a factor determining soil-atmosphere CO2 fluxes on the Qinghai-Tibetan Plateau might also be rewarding.
Livestock Carrying Capacity of the Environment
The above discussion leads us to conceptualize the Qinghai Tibetan Plateau farming systems as characterized by soils with a large historic accumulation of soil C from slow decomposition of returned above- and below-ground plant material in the prevailing cold climate. To develop sustainable farming practices in this context it is highly relevant to ask how much of the net primary production (NPP) can be harvested, and how much should be allowed to return to the soil to replace C lost by winter respiration, here indicated to be largely microbial oxidation of soil C. The above-ground NPP of Kobresia alpine meadows was stated by Zhang Q. et al. (2014) in their Table 1 to be about 1.5 t DM ha−1 a−1 and the recommended stocking rate is 1–5 animals ha−1. However, their discussion provides no clarification about classes or weights of animals and quantities of herbage removed at a particular stocking rate. Hence, in order to develop meaningful discussion on sustainable farming practices for the region it will be necessary to develop systems for recording animal numbers that correlate with animal feed demand. We provide further comment in “Methodology Considerations” section below on this point.
Intuitively, a grazing system similar to our eight sheep ha−1 grazing treatment with herbage removed estimated at 376 kg DM ha−1 with little change in herbage mass (Table 3) should be sustainable in the long term but more targeted data collection on herbage accumulation and animal intake through the growing season is required to confirm this. Our higher grazing intensity of 16 sheep ha−1 for 3 months would appear to have increased above-ground NPP (730 kg DM ha−1 herbage consumed with only 108 kg DM ha−1 decline in biomass, Table 3), but this may well have been at a cost to below-ground deposition. A crude estimate of reduction in litter deposition through removal of leaves before their senescence in summer grazed plots is obtained by adding the herbage mass reduction on grazed plots during the grazing season to the estimate of herbage eaten and comparing with herbage mass increase on Control plots (Table 3). Values obtained in this way are 655 kg DM ha−1 for the low stocking rate plots and 1,104 kg DM ha−1 for the high stocking rate plots. Inspection of Figure 5 shows that at the start of winter grazing in 2011 the herbage had not fully recovered from the previous year’s grazing activity and that the herbage mass reduction was greater during winter grazing than summer grazing. This is to be expected since in winter animals were heavier and their intake would have been enhanced by cold conditions, while herbage accumulation during grazing would have been less. Based on our data, we tentatively suggest that from an ecological sustainability perspective animal intake in grazed systems should be not more than 500 kg DM ha−1 a−1.
Methodology Considerations
It has been pointed out (Li and Sun, 2011) that in the chamber method of measuring soil and herbage respiration, the elevated temperatures on bare soil after clipping may result in overestimation of soil respiration and underestimation of herbage respiration. We do not consider this would have been a problem in the current experiment for two reasons: Firstly the analysis presented in Table 2 considers the temperature inside the chamber during each measurement run as one of the variables affecting CO2 efflux rate; secondly we checked the data for evidence of change in CO2 efflux rate between successive 10 min intervals of chamber closure and found that any such trends in the data were usually small and not statistically significant. In fact, in our data for winter this “bare-soil-heating” effect following clipping was reversed. Bare soil tended to show reducing, not rising soil respiration rates with time since chamber closure in winter, indicating that clipped soil loses heat faster than a vegetation-covered surface in winter. In addition, in our calculations reported in Table 3, the CO2 fluxes were adjusted to correct for elevated temperature on bare soil.
The units of sheep stocking rate used here and based on body weight (kg0.75. grazing days; Table 3) will be unfamiliar to many readers. These units were chosen because body maintenance energy of an animal is proportional to (body weight)0.75, and hence units of kg0.75 animal body weight are widely used in farm systems research to represent the energy needs of different animal species in a single common formula (Ramírez-Restrepo et al., 2010). If future studies are reported in these units, it should be possible to compare data for animals of differing weight, and animal densities from different experiments using sheep, goats, yak, deer and other herbivores, without further adjustment. However, in such comparisons, factors such as pregnancy or body weight change during the experiment would need to be accounted for in the calculations in order to accurately estimate energy requirements of animals and herbage consumed. In that respect GJ ha−1 y−1 energy harvested from a grazing system is probably the ultimate unit of commonality when comparing systems and performing calculations to determine sustainability guidelines for graziers, once sustainable limitations have been experimentally determined. By contrast with the 4–10 GJ ha−1 a−1 reported in Table 3, more intensive temperate pastoral systems may yield energy contributions of over 150 GJ ha−1 a−1 to animal production (Matthew et al., 2010).
The estimation of herbage intakes of animals by feed budgeting based on their energy requirements underpins farm consultancy and industry regulation in Australasia and the United Kingdom and elsewhere. This is generally believed to be more accurate than direct measurement techniques like sampling of herbage to determine herbage disappearance at grazing or use of alkane markers (Narvaez et al., 2012). In fact, direct measurement of herbage intake tends to be resource intensive and often such data have a coefficient of variation >10%. The main requirement for use of energy budgeting methodology to infer herbage consumption by animals is recording of animal body weights at intervals during the experiment. Because collection of such data is not resource-intensive, data inferences are available in this study that have not been available from previous studies.
Conclusion
Key insights emerging from our study of diurnal and seasonal CO2 efflux in an alpine meadow pasture in Maqu county on the Qinghai-Tibetan Plateau were: (1) soil respiration over winter was unexpectedly large. In sub-zero mean air temperatures of winter Re continued at approximately 110 mg CO2 m−2 h−1. The winter respiration appears to be from microbial sources and not from plant roots. From a carbon accounting perspective the winter respiration and any warming-related increase is important as it tips the ecosystem from CO2 sink status toward CO2 source status; (2) herbage metabolic activity, as indicated by estimates of Rea from our data was low in spring, indicating that substantive soil C sequestration by vegetation can happen only in a comparatively narrow time window within the growing season from June to September. More data on ecosystem CO2 fluxes for the months of May and September at the shoulders of the growing season are needed to gain a clear picture of annual C cycles; (3) decisions on stocking rate in farming systems on the Qinghai-Tibetan plateau should consider the need for return of plant litter to replace soil C lost through heterotrophic respiration; and (4) much greater clarity on the current source-sink status of these grasslands could be obtained if EC determinations of NEE were coupled with either direct measurements of components of Re or direct measurements of GPP and NPP but initial indications from the current data are that warming temperatures and recent animal number increases may be tipping these alpine meadows to carbon-source status over an annual cycle.
Data Availability Statement
The raw data supporting the conclusions of this article will be made available by the authors, without undue reservation.
Author Contributions
FH: conceptualization, resources, project administration, and funding acquisition. FH, CM, XH, and HY: methodology. XH: software. FH, CM, and HY: validation. CM and XH: formal analysis. HY, YS, YL, TZ, XG, and CY: investigation. HY: data curation, writing—original draft preparation, and visualization. FH and CM: writing—review and editing. FH and JL: supervision. All authors contributed to the article and approved the submitted version.
Funding
This research was financially supported by the National Natural Science Foundation of China, grant/award number: 32161143028 and U21A20242; the Program of National Science and Technology Assistance, grant/award number: KY202002011; the Program for Innovative Research Team of Ministry of Education, grant/award number: IRT17R50; and “Lanzhou City’s Scientific Research Funding Subsidy to Lanzhou University.” National Key Research and Development Program of China (2021YFD1300504).
Conflict of Interest
The authors declare that the research was conducted in the absence of any commercial or financial relationships that could be construed as a potential conflict of interest.
Publisher’s Note
All claims expressed in this article are solely those of the authors and do not necessarily represent those of their affiliated organizations, or those of the publisher, the editors and the reviewers. Any product that may be evaluated in this article, or claim that may be made by its manufacturer, is not guaranteed or endorsed by the publisher.
Supplementary Material
The Supplementary Material for this article can be found online at: https://www.frontiersin.org/articles/10.3389/fpls.2022.860739/full#supplementary-material
References
Chen, X. J., Hou, F. J., Matthew, C., and He, X. Z. (2010). Stocking rate effects on metabolizable energy intake and grazing behaviour of tan sheep in steppe grassland on the loess plateau of Northwest China. J. Agric. Sci. 148, 709–721. doi: 10.1017/S0021859610000511
Chen, J., Shi, W. Y., and Cao, J. J. (2015). Effects of grazing on ecosystem CO2 exchange in a meadow grassland on the Tibetan plateau during the growing season. Environ. Manag. 55, 347–359. doi: 10.1007/s00267-014-0390-z
Dang, Z. H., Jia, Y. Y., Tian, Y. Y., Li, J. B., Zhang, Y. N., Huang, L., et al. (2021). Transcriptome-wide gene expression plasticity in Stipa grandis in response to grazing intensity differences. Int. J. Mol. Sci. 22:11882. doi: 10.3390/ijms222111882
Fan, D., Hu, Y. L., Li, L. J., Li, A., Shi, S., Lian, P. Y., et al. (2013). Changes in soil organic carbon and total nitrogen stocks after conversion of meadow to cropland in Northeast China. Plant Soil 373, 659–672. doi: 10.1007/s11104-013-1827-5
Fang, J. Y., Yang, Y. H., Ma, W. H., Mohammat, A., and Shen, H. H. (2010). Ecosystem carbon stocks and their changes in China’s grasslands. Sci. China Life Sci. 53, 757–765. doi: 10.1007/s11427-010-4029-x
Fterich, A., Mahdhi, M., and Mars, M. J. (2012). Impact of grazing on soil microbial communities along a chronosequence of Acacia tortilis subsp. raddiana in arid soils in Tunisia. Eur. J. Soil Biol. 50, 56–63. doi: 10.1016/j.ejsobi.2011.12.002
Guo, M. Y., Zhao, K. T., You, J. C., Xu, L. J., Wang, L. J., Jia, S. J., et al. (2012). Soil microbial characteristic and soil respiration in grassland under different use patterns. Acta Agrestia Sinica 20, 42–48.
IPCC (2013). “Climate change 2013: the physical science basis.” in Contribution of Working Group I to the Fifth Assessment Report of the Intergovernmental Panel on Climate Change. eds. T. F. Stocker, D. Qin, G. K. Plattner, M. Tignor, and S. K. Allen, et al. (Cambridge, United Kingdom and New York, NY, USA: Cambridge University Press).
Janssens, I. A., Lankreijer, H., Matteucci, G., Kowalski, A. S., Buchmann, N., Epron, D., et al. (2001). Productivity overshadows temperature in determining soil and ecosystem respiration across European forests. Glob. Chang. Biol. 7, 269–278. doi: 10.1046/j.1365-2486.2001.00412.x
Kaštovská, E., Santruckova, H., Picek, T., Vašková, M., and Edwards, K. R. (2010). Direct effect of fertilization on microbial carbon transformation in grassland soils in dependence on the substrate quality. J. Plant Nutr. Soil Sci. 173, 706–714. doi: 10.1002/jpln.200900013
Kato, T., Hirota, M., Tang, Y. H., Cui, X. Y., Li, Y. N., Zhao, X. Q., et al. (2004a). Strong temperature dependence and no moss photosynthesis in winter CO2 flux for a Kobresia meadow on the Qinghai-Tibetan plateau. Soil Biol. Biochem. 37, 1966–1969. doi: 10.1016/j.soilbio.2005.02.018
Kato, T., Tang, Y. H., Gu, S., Cui, X. Y., Hirota, M., Du, M. Y., et al. (2004b). Carbon dioxide exchange between the atmosphere and an alpine meadow ecosystem on the Qinghai–Tibetan Plateau, China. Agric. For. Meteorol. 124, 121–134. doi: 10.1016/j.agrformet.2003.12.008
Levy, P. E., Gray, A., Leeson, S. R., Gaiawyn, J., Kelly, M. P. C., Cooper, M. D. A., et al. (2011). Quantification of uncertainty in trace gas fluxes measured by the static chamber method. Eur. J. Soil Sci. 62, 811–821. doi: 10.1111/j.1365-2389.2011.01403.x
Li, Y., Liu, Y. H., Wu, S. M., Niu, L., and Tian, Y. Q. (2016). Microbial properties explain temporal variation in soil respiration in a grassland subjected to nitrogen addition. Sci. Rep. 5:18496. doi: 10.1038/srep18496
Li, G. Y., and Sun, S. C. (2011). Plant clipping may cause overestimation of soil respiration in a Tibetan alpine meadow, Southwest China. Ecol. Res. 26, 497–504. doi: 10.1007/s11284-011-0806-7
Matthew, C., Horne, D. J., and Baker, R. D. (2010). Nitrogen loss: an emerging issue for the ongoing evolution of New Zealand dairy farming systems. Nutr. Cycl. Agroecosyst. 88, 289–298. doi: 10.1007/s10705-010-9358-4
Narvaez, N., Brosh, A., and Pittroff, W. (2012). Use of n-alkanes to estimate seasonal diet composition and intake of sheep and goats grazing in California chaparral. Small Rumin. Res. 104, 129–138. doi: 10.1016/j.smallrumres.2011.10.002
Oren, A., Rotbart, N., Borisover, M., and Bar-Tal, A. (2018). Chloroform fumigation extraction for measuring soil microbial biomass: the validity of using samples approaching water saturation. Geoderma 319, 204–207. doi: 10.1016/j.geoderma.2018.01.007
Parsons, A. J., Thornley, J. H. M., Newton, P., Rasmussen, S., and Rowarth, J. S. (2013). Soil carbon dynamics: the effects of nitrogen input, intake demand and off-take by animals. Sci. Total Environ. 465, 205–215. doi: 10.1016/j.scitotenv.2013.02.019
Pei, S. F., Hua, F., and Wan, C. G. (2008). Changes in soil properties and vegetation following exclosure and grazing in degraded Alxa desert steppe of Inner Mongolia, China. Agric. Ecosyst. Environ. 124, 33–39. doi: 10.1016/j.agee.2007.08.008
Ramírez-Restrepo, C. A., Barry, T. N., Marriner, A., López-Villalobos, N., McWilliam, E. L., Lassey, K. R., et al. (2010). Effects of grazing willow fodder blocks upon methane production and blood composition in young sheep. Anim. Feed Sci. Technol. 155, 33–43. doi: 10.1016/j.anifeedsci.2009.10.003
Reynolds, S. G. (2005). “Introduction,” in Grasslands of the World. eds. J. M. Suttie, S. G. Reynolds, and C. Batello (Rome: Food and Agriculture Organisation of the United Nations), 1–18.
Rodhe, H. (1990). A comparison of the contribution of various gases to the greenhouse effect. Science 248, 1217–1219. doi: 10.1126/science.248.4960.1217
Rogiers, N., Conen, F., Furger, M., Stoeckli, R., and Eugster, W. (2008). Impact of past and present land-management on the C-balance of a grassland in the Swiss Alps. Glob. Chang. Biol. 14, 2613–2625. doi: 10.1111/j.1365-2486.2008.01680.x
Rui, Y. C., Wang, S. P., Xu, Z. H., Wang, Y. F., Chen, C. R., Zhou, X. Q., et al. (2011). Warming and grazing affect soil labile carbon and nitrogen pools differently in an alpine meadow of the Qinghai-Tibet plateau in China. J. Soils Sediments 11, 903–914. doi: 10.1007/s11368-011-0388-6
Rustad, L. E., Huntington, T. G., and Boone, R. D. (2000). Controls on soil respiration: implications for climate change. Biogeochemistry 48, 1–6. doi: 10.1023/A:1006255431298
Schlesinger, W. H., and Andrews, J. A. (2000). Soil respiration and the global carbon cycle. Biogeochemistry 48, 7–20. doi: 10.1023/A:1006247623877
Song, C. L., Wang, G. X., Hua, Z. Y., Zhang, T., Huang, K. W., Chen, X. P., et al. (2020). Net ecosystem carbon budget of a grassland ecosystem in Central Qinghai-Tibet plateau: integrating terrestrial and aquatic carbon fluxes at catchment scale. Agric. For. Meteorol. 290:108021. doi: 10.1016/j.agrformet.2020.108021
Sun, Y., He, X. Z., Hou, F. J., Wang, Z. F., and Chang, S. H. (2018). Grazing increases litter decomposition rate but decreases nitrogen release rate in an alpine meadow. Biogeosciences 15, 4233–4243. doi: 10.5194/bg-15-4233-2018
Tang, S., Zhang, Y., Guo, Y., Zhai, X., Wilkes, A., Han, G., et al. (2015). Changes of soil CO 2 flux under different stocking rates during spring-thaw period in a northern desert steppe, China. Atmos. Environ. 122, 343–348. doi: 10.1016/j.atmosenv.2015.09.073
Thomas, A. D. (2012). Impact of grazing intensity on seasonal variations in soil organic carbon and soil CO2 efflux in two semiarid grasslands in southern Botswana. Philos. Trans. R. Soc. Lond., B, Biol. Sci. 367, 3076–3086. doi: 10.1098/rstb.2012.0102
Toma, Y., Fernández, F. G., Sato, S., Izumi, M., Hatano, R., Yamada, T., et al. (2011). Carbon budget and methane and nitrous oxide emissions over the growing season in a Miscanthus sinensis grassland in Tomakomai, Hokkaido, Japan. GCB Bioenergy 3, 116–134. doi: 10.1111/j.1757-1707.2010.01070.x
Unteregelsbacher, S., Hafner, S., Guggenberger, G., Miehe, G., Xu, X., Liu, J., et al. (2011). Response of long-, medium- and short-term processes of the carbon budget to overgrazing-induced crusts in the Tibetan plateau. Biogeochemistry 111, 187–201. doi: 10.1007/s10533-011-9632-9
Wang, Q. F., Jin, H. J., Wen, J., Yuan, Z. Q., Jin, X. Y., and Ma, Q. (2019). Hydro-meteorological influences on the growing season CO2 exchange of an alpine meadow in the northeastern Tibetan plateau permafrost region: observations using eddy covariance method. Theor. Appl. Climatol. 138, 1063–1073. doi: 10.1007/s00704-019-02861-5
Wu, G. L., Du, G. Z., Liu, Z. H., and Thirgood, S. J. (2009). Effect of fencing and grazing on a Kobresia-dominated meadow in the Qinghai-Tibetan plateau. Plant Soil 319, 115–126. doi: 10.1007/s11104-008-9854-3
Yang, Y. H., Fang, J. Y., Ma, W. H., Smith, P., Mohammat, A., Wang, S. P., et al. (2010). Soil carbon stock and its changes in northern China’s grasslands from 1980s to 2000s. Glob. Chang. Biol. 16, 3036–3047. doi: 10.1111/j.1365-2486.2009.02123.x
Ye, Q. H., Yao, T. D., and Naruse, R. (2008). Glacier and lake variations in the Mapam Yumco basin, western Himalaya of the Tibetan plateau, from 1974 to 2003 using remote-sensing and GIS technologies. J. Glaciol. 54, 933–935. doi: 10.3189/002214308787779997
Yuan, H., and Hou, F. J. (2015). Grazing intensity and soil depth effects on soil properties in alpine meadow pastures of Qilian Mountain in Northwest China. Acta Agric. Scand., B: Soil Plant Sci. 65, 222–232. doi: 10.1080/09064710.2014.992940
Yuste, J. C., Ma, S., and Baldocchi, D. D. (2009). Plant-soil interactions and acclimation to temperature of microbial-mediated soil respiration may affect predictions of soil CO2 efflux. Biogeochemistry 98, 127–138. doi: 10.1007/s10533-009-9381-1
Zhang, Q., Ding, Y., Ma, W., Kang, S., Li, X., Niu, J., et al. (2014). Grazing primarily drives the relative abundance change of C4 plants in the typical steppe grasslands across households at a regional scale. Rangel. J. 36, 565–572. doi: 10.1071/RJ13050
Zhang, C. P., Li, X. D., Wen, H. Y., Wan, C. G., and Fu, H. (2015). Variation of Q10 values in a fenced and a grazed grassland on the loess plateau, northwestern China. Soil Sci. Plant Nutr. 61, 629–640. doi: 10.1080/00380768.2015.1036307
Keywords: carbon cycle, carbon sequestration, Kobresia, grazing, metabolisable energy
Citation: Yuan H, Matthew C, He XZ, Sun Y, Liu Y, Zhang T, Gao X, Yan C, Chang S and Hou F (2022) Seasonal Variation in Soil and Herbage CO2 Efflux for a Sheep-Grazed Alpine Meadow on the North-East Qinghai-Tibetan Plateau and Estimated Net Annual CO2 Exchange. Front. Plant Sci. 13:860739. doi: 10.3389/fpls.2022.860739
Edited by:
Huakun Zhou, Key Laboratory of Restoration Ecology in Cold Regions, Northwest Institute of Plateau Biology (CAS), ChinaReviewed by:
Amber Churchill, University of Minnesota, United StatesRoxana Vidican, University of Agricultural Sciences and Veterinary Medicine of Cluj-Napoca, Romania
Copyright © 2022 Yuan, Matthew, He, Sun, Liu, Zhang, Gao, Yan, Chang and Hou. This is an open-access article distributed under the terms of the Creative Commons Attribution License (CC BY). The use, distribution or reproduction in other forums is permitted, provided the original author(s) and the copyright owner(s) are credited and that the original publication in this journal is cited, in accordance with accepted academic practice. No use, distribution or reproduction is permitted which does not comply with these terms.
*Correspondence: Fujiang Hou, cyhoufj@lzu.edu.cn