- 1College of Horticulture and Plant Protection, Yangzhou University, Yangzhou, China
- 2Department of Floriculture, Faculty of Agriculture, Alexandria University, Alexandria, Egypt
Floral scents and fruit aromas are crucial volatile organic compounds (VOCs) in plants. They are used in defense mechanisms, along with mechanisms to attract pollinators and seed dispersers. In addition, they are economically important for the quality of crops, as well as quality in the perfume, cosmetics, food, drink, and pharmaceutical industries. Floral scents and fruit aromas share many volatile organic compounds in flowers and fruits. Volatile compounds are classified as terpenoids, phenylpropanoids/benzenoids, fatty acid derivatives, and amino acid derivatives. Many genes and transcription factors regulating the synthesis of volatiles have been discovered. In this review, we summarize recent progress in volatile function, composition, biosynthetic pathway, and metabolism regulation. We also discuss unresolved issues and research perspectives, providing insight into improvements and applications of plant VOCs.
Introduction
Floral scents and fruit aromas are volatile organic compounds (VOCs) released by plants. These VOCs are lipophilic and characterized by low molecular weights and high melting points. Biosynthesis of VOCs occurs in all plant organs, including seeds, roots, stems, leaves, fruits, and flowers; floral scents and fruit aromas are relevant to our daily lives and have substantial economic value.
Floral VOCs are important traits of floral scents. The differences and abundances of these VOCs in scents vary widely among flowering plants (Farré-Armengol et al., 2017). According to their origin, biosynthesis, and function, floral scents are classified as terpenoids, phenylpropanoids, fatty acid derivatives and amino acids.
Fruit aroma is a key contributor to fruit quality and acceptance by animal and human. Fruit aroma consists of various chemical compounds (e.g., aldehydes, alcohols, ketones, esters, lactones, and terpenes) (Riu-Aumatell et al., 2004); the presence or absence of certain compounds determines differences among fruit aromas. In a wide range of fruits, the production of volatile compounds beginning from fruit-set to late-ripening is modulated by the accumulation of fruity esters, terpenes, and other compounds.
In the past decade, numerous studies of floral scents and fruit aromas have improved our understanding of their functions, components, biosynthesis, and regulation. In addition, previous reviews focused on floral scents or fruit aromas separately, whereas both have important volatile components contributing to the economic value of horticultural plants. Therefore, we review recent progress regarding floral scents and fruit aromas, focusing on the functions and compositions of volatiles, the factors and biosynthetic pathways that affect volatiles, and the regulation of their biosynthesis and metabolism.
Functions
Floral Scent Volatiles
Floral volatiles attract effective pollinators for sexual reproduction (Schiestl, 2010; Gross et al., 2016). Although some floral VOCs attract a variety of pollinators (broad pollinators) (Schiestl et al., 2003), flowers release volatiles to signal to specific pollinators (Schiestl, 2015). Their selection behavior depends on differences in composition, amount, and emission of floral volatile compounds (Muhlemann et al., 2014). For instance, emitted terpenoids and benzenoids can attract pollinators and repel elective visitors (Farré-Armengol et al., 2013), while the same terpenoid compound may attract one animal but repel another (Eilers et al., 2021). Many flowers offer floral rewards as nectar, pollen or oil products, on which visitors depend (Steiner et al., 2011) but some plants attract pollinators without offering nectar by mimicking the scents and colors of neighboring plants (Kunze, 2001; Schiestl, 2005). High production of VOCs is necessary to guide pollinators to flowers, particularly to overcome the decreased conspicuousness of flowers at night (Muhlemann et al., 2014). β-ocimene is implicated in the attraction of pollinators. For example, in Mirabilis jalapa flowers, the emitted floral scent is dominated by trans-β-ocimene and its emission peaks in the evening, which match flower opening and hawkmoth pollinator activity (Effmert et al., 2005).
Other adaptive roles for floral scents include repellents (Piechulla and Pott, 2003; Kessler et al., 2008; Raguso, 2008) and the provision of physiological protection against biotic stresses in plants (Dudareva et al., 2006; Knudsen and Gershenzon, 2006). In plant allelopathy, different VOCs can function as cues to other plants (Caruso and Parachnowitsch, 2016). For instance, in plant-plant communication, plants use volatiles emitted by their neighbors to evaluate their environment, including the presence of herbivores (Heil and Karban, 2010) and competitors (Kegge and Pierik, 2010). Generally, exposure of higher plants to biotic stresses (e.g., herbivore damage) can result in the emission of VOCs to repulse the attack (Arimura and Pearse, 2017) and enhance the response to future attacks (Morrell and Kessler, 2014). Similarly, some flower VOCs can be used in plant allelopathy. For example, the olfactory cues of some non-host plants attract the pollinators of other plants (e.g., Malva moschata and Geranium sanguineum attract Chelostoma rapuncul bees, the pollinator of Campanula spp.) (Burger et al., 2021). However, the compositions of floral scents that attract pollinators or repel herbivores are unclear.
Some volatiles have antibacterial properties and function in defense against microbial pathogens (Junker et al., 2011; Huang et al., 2012). Certain defensive functions rely on a single compound, which is more effective than a mixture of compounds (Gershenzon and Dudareva, 2007). A single floral volatile can have multiple roles in flowers. For example, (E)-b-caryophyllene from Arabidopsis thaliana promotes defense against pathogens and enhances pollinator attraction (Huang et al., 2012). In rose geranium (Pelargonium graveolens), β-citronellol is an abundant compound with extensive antibacterial activity (Boukhris et al., 2015). The reported functions of floral scents are listed in Table 1.
Fruit Aroma Volatiles
Fruit aroma is a major contributor to fruit quality (color, texture, flavor, and aroma). In the wild, the aroma VOCs released from fruits influence herbivore behavior and attract animal dispersers (Dudareva et al., 2013). For example, fruit bats recognize ripe and non-ripe fruits based on the emitted volatiles (Hodgkison et al., 2007). VOCs have biological activities against bacteria, fungi, and insects. Volatiles extracted from citrus peels (Citrus reticulata Blanco) exhibit significant antifungal and antibacterial activities against pathogenic strains (Sultana et al., 2012). In another citrus species (Citrus hystrix), the essential oils extracted from fruit peels possess antibacterial activity against respiratory bacteria; the most effective components are α-terpineol, terpinene-4-ol, and limonene (Srisukh et al., 2012). The antibacterial activities of fruit VOCs in extracts of lemon (Citrus limonium), sweet lime (Citrus limetta), pomegranate (Punica granatum), apple (Malus domestica), and tomato (Solanum lycopersicum) against pathogenic bacteria isolated from a wound have been reported (Unnisa et al., 2012). Essential oils extracted from Alchornea cordifolia fruit have shown antibacterial activity against Staphylococcus aureus and antifungal activity against Aspergillus niger, while essential oils extracted from Canthium subcordatum fruit have shown antibacterial activity against Bacillus cereus, S. aureus, and A. niger (Essien et al., 2015). In strawberry fruit, the antifungal activity of fruit VOCs against Colletotrichum acutatum is contributed by (E)-hex-2-enal (Arroyo et al., 2007). Essential oil components from pepper fruit significantly inhibited the germination of Colletotrichum gloeosporioides; the active compounds were carvacrol, cinnamon oil, citral, trans-cinnamaldehyde, p-cymene, and linalool (Hong et al., 2015). Volatiles isolated from leaves, flowers, and fruits of three opuntia species had antifungal activity against fungal species such as Alternaria solani (Bergaoui et al., 2007). Although fruit VOCs have antibacterial and antifungal activities, the most important function of fruit aroma in horticulture is to attract humans. Indeed, fruit aromas have been selected by horticulture breeding to improve their edible quality and economic value.
Volatile Composition
Floral Scents
Floral scents consist of a mixture of compounds; they are categorized as terpenoids, phenylpropanoids/benzenoids, fatty acids, and amino acids. Terpenoids are the largest class of volatiles and comprise more than 40,000 structures derived from five-carbon isoprene units as monoterpenoids, sesquiterpenoids, apocarotenoids, and others. Phenylpropanoids comprise more than 8,000 metabolites (Dong and Lin, 2021); benzenoids are the second class of VOCs derived from the amino acid phenylalanine. Fatty acids and amino acids are important VOCs present in floral scents and fruit aromas (Negre-Zakharov et al., 2009).
Rose (Rosa spp.)
Rose is one of the most important ornamental plants for the production of cut flowers and perfumes. Therefore, floral scent is the main economic trait of rose, and some cultivars are classified according to their fragrant components (Du et al., 2019). Rose scents are composed of mixtures of organic compounds. In Bulgarian rose (Rosa damascene) essential oil, the most abundant components are phenethyl alcohol, citronellol, heneicosane, pentadecane, eugenol, methyleugenol, and geraniol (Won et al., 2009; Kiralan, 2015; Koksal et al., 2015). The first rose water (R. damascene) comprised benzoic acid 2-hydroxy-3-methyl butyl ester, geranyl acetate, carbamic acid methyl ester, linalool, eucalyptol, citronellol, geraniol, and methyleugenol (Koksal et al., 2015). In other rose (Rosa L.) species (R. dumalis, R. canina, R. dumalis subsp. boissieri, R. gallica, and R. hirtissima), the major compounds are aldehydes, alcohols, monoterpenes, and sesquiterpenes (Demir et al., 2014). Moreover, the fragrant rose cultivar “Fragrant Cloud” contains monoterpene alcohols, terpene hydrocarbons, and acetates (Shalit et al., 2004). The predominant scent compounds in the petals of six Hybrid Rugosa roses are phenyl ethyl alcohol, β-citronellol, nerol, and geraniol (Sparinska and Rostoks, 2015). Rose-breeding programs are underway to produce new varieties of floral scents.
Orchid
Orchid is the largest family (Orchidaceae) of flowering plants (Julsrigival et al., 2013), comprising 20,000–30,000 species (Khuraijam et al., 2017). Approximately 75% of orchids are fragrant (De et al., 2019). In an economic context, they can be used to produce cut flowers and as potted plants (Khuraijam et al., 2017). The family Orchidaceae is divided into five subfamilies. Epidendroideae is the largest subfamily, comprising approximately 76% of the family (Freudenstein and Chase, 2015). In Epidendroideae, the major aromatic compounds of Thai fragrant orchid species (Rhynchostylis gigantea Ridl., R. gigantea var. harrisonianum Holtt., Vanda coerulea, and Dendrobium parishii Rchb. f.) are nerol, 2,3-dihydrofarnesol, nonanal, and 2-pentadecanone (Julsrigival et al., 2013). The emitted volatiles of Zygopetalum maculatum (an orchid species) are enriched in benzenoids, including O-diethylbenzene, p-diethylbenzene, benzyl acetate, and methyl salicylate; 2-phenylethylacetate is the major phenylpropanoid component (Bera et al., 2018). Cymbidium spp. flowers are rich in cineole, isoeugenol, and (-) selinene (Ramya et al., 2020). Phalaenopsis orchids contain monoterpenes, including linalool and geraniol (Chuang et al., 2017). The scent compounds in Vanda Mimi Palmer (an orchid hybrid) are ocimene, linalool, linalool oxide, and nerolidol as the major terpenoids; the major benzenoids and phenylpropanoids are benzyl acetate, methylbenzoate, phenylethyl acetate, and phenylethanol (Mohd-Hairul et al., 2010). The major components of the floral scents of Ophrys sphegodes, Ophrys bertolonii, and Neotinea tridentate are hydrocarbons, alcohols, aldehydes, and terpenes (Manzo et al., 2014).
Tulip (Tulipa spp.)
The few fragrant tulip cultivars produce a range of floral scents (Oyama-Okubo and Tsuji, 2019). The major scent compounds of tulips (Tulipa L.) cultivars are monoterpenoids (eucalyptol, d-limonene, linalool, trans-β-ocimene, and α-pinene), sesquiterpenoids (α-farnesene, caryophyllene, geranyl acetone, and β-ionone), benzenoids (benzaldehyde, acetophenone, 3,5-dimethoxytoluene, benzyl alcohol, methyl salicylate, and 2-phenyl ethanol), and fatty acid derivatives (decanal, cis-3-hexenol, cis-3-hexenyl acetate, 2-hexenal, and octanal) (Oyama-Okubo and Tsuji, 2013).
Peony (Paeonia spp.)
Peony (tree and herbaceous peony) includes many Paeoniaceae species and cultivars, which are important ornamental plants with rich fragrances. The major flavoring substances in peony are citronellol, geraniol, and linalool (Song et al., 2018; Wang et al., 2021). In 30 herbaceous peony cultivars, the main compounds are phenyl ethyl alcohol, β-caryophyllene, linalool, nerol, and (R)-citronellol (Song et al., 2018).
Lily (Lilium spp.)
Lilies are commercially important ornamental plants because of their attractive colors and scents (Du et al., 2019). Volatile-emitting lily cultivars (Lilium spp.) release various scent compounds, predominantly three monoterpenoids (1.8-cineole, (E)-b-ocimene and linalool) and one benzenoid (methyl benzoate) (Kong et al., 2017). β-cis-ocimene represents the major component in Lilium spp. “Sweetness” (Aros et al., 2020).
Water Lily (Family: Nymphaeaceae)
Water lily flowers (Nymphaea colorata) release 11 volatiles, comprising terpenoids (sesquiterpenes), fatty acid derivatives (methyl decanoate), and benzenoids (Zhang et al., 2020).
Carnation (Dianthus spp.)
Benzenoids are the principal components of carnation flowers in addition to terpenoids and fatty acid derivatives (Kishimoto, 2020). Some scents are described as spicy because they contain eugenols (Kishimoto, 2020). In Dianthus rupicola Biv. (cliffs carnation), phenolic monoterpenes are the predominant components of the essential oil, followed by monoterpene hydrocarbons and oxygen-containing sesquiterpenes (Casiglia et al., 2014). In a carnation species (D. elymaiticus), the essential oil of flowers contains high levels of fatty acid derivatives and terpenoids; the major compounds are (Z)-3-hexenyl acetate, methyl benzoate, β-caryophyllene, and decanal (Azadi and Entezari, 2016).
Lavender (Lavandula spp.)
Lavender plants have excellent medicinal and aromatic properties (Guo and Wang, 2020). The major volatiles extracted from lavender essential oil (Lavandula angustifolia Mill) are linalool, linalyl acetate (Venskutonis et al., 1997; Won et al., 2009; Guo and Wang, 2020), 1,8-cineole, and α-terpineol (Tschiggerl and Bucar, 2010; Śmigielski et al., 2013), along with oxygenated derivatives of monoterpenes and monoterpene alcohols (Śmigielski et al., 2013). In L. officinalis, linalyl acetate was the predominant volatile in the essential oils (Xiao et al., 2017). Lavender flower (L. angustifolia) vapor consists of terpene hydrocarbons, oxygenated terpenes, and sesquiterpenes; linalool, terpin-4-ol, and linalyl acetate are predominant (An et al., 2001).
Jasmine (Jasminum spp.)
Jasmine flowers are well-known for their pleasant fragrances. In Jasminum species (J. sambac, J. auriculatum, J. grandiflorum, and J. multiflorum), linalool and (3E, 6E)-a-farnesene are the major monoterpene and sesquiterpene, respectively (Bera et al., 2015). J. sambac is a fragrant flower species distributed worldwide. The major compounds of the floral scent are methyl anthranilate, linalool, 4-nonanolide, 4-hexanolide, (E)-2-hexenyl hexanoate, 4-hydroxy-2,5-dimethyl-3(2 H)-furanone (Ito et al., 2002), eugenol, benzyl alcohol, benzyl acetate, benzyl benzoate, methyl salicylate, methyl anthranilate, (Z) 3-hexenyl benzoate, indole, and α-farnesene (Lin et al., 2013; Chen et al., 2017, 2020).
Daffodil (Narcissus spp.)
Narcissus floral volatiles and their essential oils are used in the perfume industry (Terry et al., 2021). Chinese daffodil flowers (Narcissus tazetta) contain acetic acid phenethyl ester, E-ocimene, acetic acid benzyl ester, neo-allo-ocimene, allo-ocimene, α-linalool, 1,8 cineole, benzenepropyl acetate, and 3-methyl-2-buten-1-ol acetate as the major VOCs (Melliou et al., 2007; Song et al., 2007; Chen et al., 2013). Monoterpenes are the major VOCs in daffodil (N. pseudonarcissus); β-ocimene and β-myrcene are predominant (Li et al., 2018). In simple and double flower cultivars of Narcissus, the major scent compounds are monoterpenes and benzenoids, particularly cis-β-ocimene and benzyl acetate (Ruíz-Ramón et al., 2014). In the essential oil of N. serotinus, the major component is benzyl acetate; linalool oxides are the main characteristics of this species (Melliou et al., 2007). In two varieties of European Daffodil (N. pseudonarcissus L.), “Isha” and “Acropolis,” the major categories of VOCs are terpenes and ethers; the characteristic aromatic component is β-ocimene (Wang et al., 2013).
Hyacinth (Hyacinths spp.)
In Hyacinth (Hyacinths orientals) varieties, the major components of floral scents are terpenes, esters, and alcohols; the characteristic aromatic components are acetoxytoluene, β-ocimene, β-myrcene, and β-phenethyl alcohol (Wang et al., 2013).
Most studies of floral scents have focused on VOC profiles. However, the components of specific species (cultivars) should be evaluated by mass spectrometry and a sensory evaluation of floral fragrances. The chemical compositions of floral scents of major flower species are listed in Supplementary Table 1.
Fruit Aroma
According to the modes of fruit development, there are three main groups (botanical classification): simple fruits, aggregate fruits, and multiple (or composite) fruits (Singh, 2004). However, based on the yield and commercial values, the fruits are normally classified into berries, melons, citrus fruits, drupes (stone fruits), pomes (apples and pears), and tropical fruits. Most fruits release a wide range of VOCs, which determine their aroma profiles. The VOCs released from fruits are esters, ketones, aldehydes, lactones, alcohols, and terpenoids (Ahmed et al., 2013). C10 monoterpenes and C15 sesquiterpenes are abundant and key determinants of the characteristic aroma of fruits (Ahmed et al., 2013). Each fruit species has a distinctive aroma based on the mixture of fruit VOCs (Tucker, 1993; Baietto and Wilson, 2015).
Banana (Musa spp.)
Banana is the main fruit traded globally (Alam, 2014). Almost 200 volatile components are found in banana fruit (Alam, 2014). Hexanal is the major volatile compound in most banana cultivars. The typical volatile compounds are (E)-2-hexenal and acetoin (Cavendish), (E)-2-hexenal and hexanal (Plantain), and 2,3-butanediol (Frayssinette) (Aurore et al., 2011). In addition, VOC composition may change during fruit maturation. During the ripening of two banana cultivars (“Brazilian” and “Fenjiao”), the predominant volatile components are isoamyl acetate, butanoic acid, 3-methyl-3-methylbutyl ester, hexanal, trans-2-hexenal, and 1-hexanol. However, octanoic acid and propanoic acid 2-methylbutyl ester are only detected in Fenjiao (Zhu et al., 2018a).
Apple (Malus domestica)
More than 300 aromatic compounds have been identified in apples, including esters, alcohols, aldehydes, acids, ketones, and terpenoids (Yang S. et al., 2021). Esters are the most important determinant of the aroma of ripe apples, followed by alcohols (Espino-Díaz et al., 2016). Although the aroma is cultivar-specific, eight common VOCs are detected in 40 apple cultivars: esters (hexyl butyrate, hexyl 2-methylbutyrate and hexyl hexanoate), hexanal, (E)-2-hexenal, 1-hexanol, estragole, and α-farnesene (Yang S. et al., 2021). In addition, esters such as butyl acetate, hexyl acetate, and 2-methyl butyl acetate influence the aroma profiles of the apple cultivars “Discovery” and “Prima” (Dunemann et al., 2009). There are more than 7,500 cultivars of culinary and eating apples (Elzebroek and Wind, 2008); thus, more aroma components must be identified.
Grape (Vitis vinifera)
Grape is of great commercial significance worldwide; it is divided into table (fresh consumption), juice, wine, and dried (raisins) types. The components of three varieties (Merlot, Cabernet Sauvignon, and Feteasca Neagra) of wine grape are: butanoic acid, tropilidene, methyl ester, 2-ethyl heptanoic acid, 2,4-dimethyl heptane, 2,4-dimethyl-1-heptene, n-nonane, 4-methyl octane, 2-propyl-1-pentanol, 6-methyl tridecane, 3,5-dimethyl octane, n-decane, O-cymene, terpinen-4-ol, undecane, linalool, and estragole (Palade and Popa, 2016). During development of muscadine grape (Vitis rotundifolia), myrcenol, β-ocimene, and L-limonene are common components at the first stage (green). Nonanal, decanal, and β-citronellol are detected at the second stage (soft and translucent and skin pink/red); at the third stage (purple to black), butyl-2-butenoate, propyl acetate, hexyl acetate, hexyl-2-butenoate, ethyl trans-2-butenoate, ethyl acetate, 1-octanol, butyl acetate, ethyl hexanoate, and β-citral are detected (Lee et al., 2016). The most common VOCs in white “Albariño” grapes are (E)-2-hexenal, (Z)-2-hexanol, 1-hexanol, benzaldehyde, phenylethanal, 2-phenylethanol, and cis pyran linalool oxide (Ripoll et al., 2017). In table grape cultivars (Centennial Seedless, Italia, Italia Rubi, Chasselas, Alphonse Lavallée, and Muscat de Hambourg), (E)-2-hexenal and hexanal are the two major volatiles, whereas monoterpenols are specific to Muscat varieties (Aubert and Chalot, 2017).
Strawberry (Fragaria spp.)
Several compounds are found at high concentrations (such as ketones and long-chain acids) in VOCs of strawberry, whereas several characteristic VOCs such as furanones (particularly 4-methoxy-2,5-dimethyl-3(2H)-furanone), esters (ethyl butanoate, ethyl hexanoate, methyl butanoate, and methyl hexanoate), terpenes (linalool and nerolidol) and sulfur compounds (methanethiol) are present at low levels (Yan et al., 2018). In white strawberry (Fragaria chiloensis), the major aroma compounds are ethyl butanoate, 2-hexenal, ethyl hexanoate, hexyl acetate, 2-hexen-1-ol acetate, furfuryl acetate, linalool, mesifuran, ethyl decanoate, benzyl alcohol, 2-phenylethyl acetate, 2,5-dimethyl-4-hydroxy-3(2H)-furanone, hydrocinnamyl alcohol, γ-decalactone, cinnamyl acetate, (E)-2,6-dimethylocta-2,7-dien-1,6-diol, (Z)-2,6-dimethylocta-2,7-dien-1,6-diol, and hexadecanoic acid (Prat et al., 2013).
Citrus (Citrus spp.)
Citrus fruits (e.g., orange and lemon) have high nutritional and economical value because of their contents of vitamin C, flavonoids, pectin, carotenoids, and calcium (Abobatta, 2019). Global production reached 98 million tons in 2021, according to the United States Department of Agriculture. The aromatic compounds in citrus fruits are present in peels and juices; their essential oils are used in the food, cosmetics, and pharmaceutical industries. Terpenes and terpenols are the major volatiles in orange juice. In orange beverage, limonene is the main volatile compound, followed by myrcene, ethyl butyrate, γ-terpinene, linalool, 3-carene, decanal, ethyl acetate and low levels of 1-octanol, geranial, β-pinene, octanal, α-pinene, and neral (Mirhosseini et al., 2007). Similarly, the main aromatic compounds in orange essence oil (from juice) are limonene followed by linalool, octanal, decanal, myrcene, and ethyl butyrate (Högnadóttir and Rouseff, 2003). In sweet orange (Citrus sinensis) juice, ethyl butanoate, nootkatone, linalool, and limonene are predominant (Kelebek and Selli, 2011; Herrera et al., 2016); the aroma profile of Jinchen sweet orange juice and peel oil comprises the same VOCs (Qiao et al., 2008).
The volatile oil of Citrus limon peels consists mainly of monoterpenes; limonene is the most abundant component (Ayedoun et al., 1996; Mahalwal and Ali, 2003), followed by camphenes, α-terpineol, α-phellandrene, and 4-terpineol, along with α-selinene (a predominant sesquiterpene), caryophyllene oxide, t-nerolidol, and valencene (Mahalwal and Ali, 2003). In eureka lemon, terpenoids are the main aromatic components; d-limonene is the major component in lemon juice and peel, followed by aldehydes and esters (Zhong et al., 2014).
Mango (Mangifera indica)
Mango is a tropical fruit and a good source of vitamins, minerals, and fiber. According to the latest report of global fruit production in 20191, mango ranked sixth among the major fruits. The predominant aroma compounds in mango are (E)-β-damascenone, (E,Z)-nonadienal, (E)-2-nonenal, (E)-β-ionone, terpinolene, ethyl 2-methylpropanoate, ethyl butanoate, ethyl 2-methylbutanoate, limonene, myrcene, linalool, δ-3-carene, β-caryophyllene, γ-octalactone, nonanal, methyl benzoate, 2,5-dimethyl-4-methoxy-3(2H)-furanone, and hexanal (Pino, 2012). In addition to ethyl-2-methylpropanoate, ethyl butanoate, and methyl benzoate, the most abundant aromatic compounds in 20 mango cultivars are (E)-2-nonenal, (E,Z)-2,6-nonadienal, decanal, (E)-β-ionone, and 2,5-dimethyl-4-methoxy-3(2H)-furanone (Pino and Mesa, 2006). Some cultivars have typical aromas; examples include Colombian mangoes (α-pinene, α-phellandrene and terpinolene) (Quijano et al., 2007) and Harumanis mango (β-ocimene, transβ-ocimene, and allo-ocimene) (Zakaria et al., 2018). Mango skin produces glycosidically bound aromatic volatile compounds, the levels of which are strongly influenced by fruit part and maturity (Lalel et al., 2003a).
Peach (Prunus persica)
Peach is an important stone fruit; it contains vitamins, minerals, and sugars. The volatile levels in white-fleshed peach skin are significantly higher than in other parts of the fruit. Unsaturated lactones and C6-compounds are detected mainly in the top and bottom mesocarp; benzaldehyde content is highest close to the stone (Aubert and Milhet, 2007). The essential oils of six peaches comprise aldehydes, lactones, alcohols, terpenes, esters, acids, norisoprenoids, phenylalanine derivates, and ketones (Eduardo et al., 2010). The characteristic volatiles and their contents in peach cultivars depend on the genotypic background and germplasm origin. For example, the highest contents of terpenoids and esters are present in Chinese wild peaches and “Wutao,” lactones are present in “Ruipan 14” and “Babygold 7,” and linalool is present in seven cultivars of American or European origin (Wang et al., 2009).
Apricot (Prunus armeniuca)
The major volatiles in apricot are aldehydes, alcohols, esters, acetates, terpenes, and acids. The most abundant compounds are ethanol, hexanal, hexyl acetate, (Z)-3-hexenyl acetate, (E)-2-hexenyl acetate, (Z)-3-hexenol, 1-hexanol, and (E)-2-hexen-1-ol (Gokbulut and Karabulut, 2012). The major compounds in other apricot species are heptyl isobutyrate, citronellyl propionate, geranyl acetate, γ-hexalactone, δ-undecalactone, 5-hydroxy-7-decenoic acid lactone, and 5-hydroxy-2,4-decadienoic acid lactone (Zhang et al., 2008). In some apricot cultivars, ethyl acetate, hexyl acetate, limonene, β-cyclocitral, γ-decalactone, 6-methyl-5-hepten-2-one, linalool, β-ionone, menthone, and (E)-hexen-2-al are the most important aromatics (Guillot et al., 2006). In the apricot cultivar “Xinshiji,” the predominant compounds are hexyl acetate, β-ionone, butyl acetate, linalool, limonene, γ-decalactone, (E)-2-hexenal, and hexanal (Meixia et al., 2004; Chen et al., 2006). Aldehydes and terpenes decrease significantly, whereas lactones and apocarotenoids increase significantly, with apricot ripening. β-ionone, γ-decalactone, sucrose, and citrate are key flavor characteristics influencing consumer acceptance (Xi et al., 2016). In ripe “Xinshiji” and “Hongfeng” apricots, shared constituents include ionone, hexanal, hexenal, hexanol, hexenol, lactones, and terpenic alcohols (Meixia et al., 2004). In Japanese apricot (Prunus mume Sieb. et Zucc.), benzaldehyde, isolongifololyl acetate, linalool, butyl acetate, and palmitic acid are the dominant compounds (Miyazawa et al., 2009).
Pineapple (Ananas comosus)
Pineapple is an important tropical fruit in which the most common VOCs are ethyl 2-methylbutyrate, methyl-2-methylbutyrate, ethyl 2-methylbutanoate, methyl 2-methylbutanoate, methyl hexanoate, ethyl hexanoate, decanal, and 2,5-dimethyl-4-hydroxy-3(2H)-furanone (Montero-Calderón et al., 2010; Wei et al., 2011; Zheng et al., 2012; Žemlička et al., 2013). Other VOCs include furaneol, 3-(methylthio) propanoic acid ethyl ester, 3-(methylthio) propanoic acid methyl ester, δ-octalactone (Zheng et al., 2012), and methyl butanoate (Montero-Calderón et al., 2010). The dominant compounds in pineapple at all growth stages are esters, terpenes, alcohols, 2-ketones, aldehydes, free fatty acids, and γ- and δ-lactones (Steingass et al., 2015).
Pineapple juice comprises mainly esters, decanal, acetic acid, ethyl octanoate, 1-hexanol, γ-octalactone, δ-octalactone, γ-hexalactone, γ-decalactone, and γ-dodecalactone (de Barretto et al., 2013). The major esters are methyl 2-methylbutanoate, methyl 3-(methylthio)-propanoate, methyl butanoate, methyl hexanoate, ethyl hexanoate, ethyl 3-(methylthio)-propanoate, 2,5-dimethyl-4-methoxy-3(2H)-furanone (mesifurane), and 2,5-dimethyl-4-hydroxy-3(2H)-furanone (furaneol) (Elss et al., 2005). In pineapple wine, the main components are ethyl octanoate, ethyl acetate, ethyl decanoate, and 3-methyl-1-butanol (Pino and Queris, 2010).
Durian (Durio zibethinus)
Durian is a tropical fruit popular in southeast Asia (Chin et al., 2008); it is well-known for its strong aroma and unique taste. Durian produces various VOCs, including esters (ethyl ester and propanoic acid), aldehydes (acetaldehyde), and sulfur compounds (di-ethyl disulfide, di-ethyl trisulfide, and ethyl-propyl disulfide) (Ali et al., 2020).
The VOCs of most major fruits have been identified. The chemical compositions of fruit aromas are listed in Supplementary Table 2.
Biosynthetic Pathways
Terpenoid Biosynthetic Pathway
Terpenoids are the largest group of volatile compounds in plants (Muhlemann et al., 2014; Ramya et al., 2018). Based on C5 isoprenoid units, terpenoids are classified as C5 (hemiterpenes), C10 (monoterpenes), C15 (sesquiterpenes), C20 (diterpenes), C25 (sesterpenes), C30 (triterpenes), C40 (tetraterpenes), and > C40 (polyterpenes) (Martin et al., 2003; Ashour et al., 2010). Terpenoids are derived from isopentenyl diphosphate (IPP) or dimethylallyl diphosphate (DMAPP). Terpenoids are classified based on the molar ratio of IPP to DMAPP: monoterpenes (1:1); sesquiterpenes and sterols (2:1); and diterpenes, carotenoids, and polyterpenes (3:1) (Gershenzon and Kreis, 1999). In higher plants, the C5 unit is generated by the mevalonic acid (MVA) and 2-c-methylerythritol 4-phosphate (MEP) pathways. In the cytosol, IPP is generated from the mevalonic acid (MVA) pathway, beginning with the condensation of acetyl-CoA (Newman and Chappell, 1999). In plastids, IPP is formed by the MEP pathway, beginning with pyruvate and glyceraldehyde-3-phosphate. In cytosol and plastid, isopentenyl diphosphate isomerase is responsible for the reversible conversion of IPP to DMAPP (Phillips et al., 2008; Jin et al., 2020).
Monoterpenes constitute an important class of aromatic compounds; they are the principal constituents of the scents of flowers and fruits (Croteau et al., 2000). Monoterpenes include limonene, (E)-β-ocimene, myrcene, linalool, and α- and β-pinene (Piechulla and Effmert, 2010; Dudareva et al., 2013; Oyama-Okubo et al., 2013). In plants, monoterpenes are synthesized in plastids (Dudareva and Pichersky, 2000; Chen and Tholl, 2011). IPP is the precursor of geranyl diphosphate (GPP) and geranylgeranyl pyrophosphate (GGPP). Monoterpenes are derived from geranyl diphosphate (GPP, C10); GPP synthase catalyzes the conversion of one IPP and one DMAPP molecule to GPP through a head-to-tail condensation reaction (Poulter and Rilling, 1981; Ogura and Koyama, 1998). Sesquiterpenes are synthesized in the cytosol by the MVA pathway. In this pathway, two IPP molecules and one DMAPP molecule are condensed to produce farnesyl diphosphate by farnesyl pyrophosphate synthase. Finally, cytosolic sesquiterpene synthases catalyze the conversion of farnesyl diphosphate to sesquiterpenes (Chen and Tholl, 2011; Monson, 2013). Notably, in snapdragon flowers, sesquiterpenes are synthesized via the MEP pathway (Vranová et al., 2013).
Similar to other isoprenoids, carotenoids share a biosynthetic pathway with mono- and diterpenoids; they are derived from IPP. In plastids (Lichtenthaler et al., 1997), the sequential addition of three IPP molecules to one DMAPP molecule, catalyzed by geranylgeranyl diphosphate synthase, leads to synthesis of the 20-carbon molecule GGPP. The first step in the carotenoid pathway is the condensation of two GGPP molecules to produce phytoene, the primary carotenoid, which is catalyzed by phytoene synthase. Phytoene undergoes several enzymatic reactions to carotenoid compounds (Figure 1; Zhou and Pichersky, 2020).
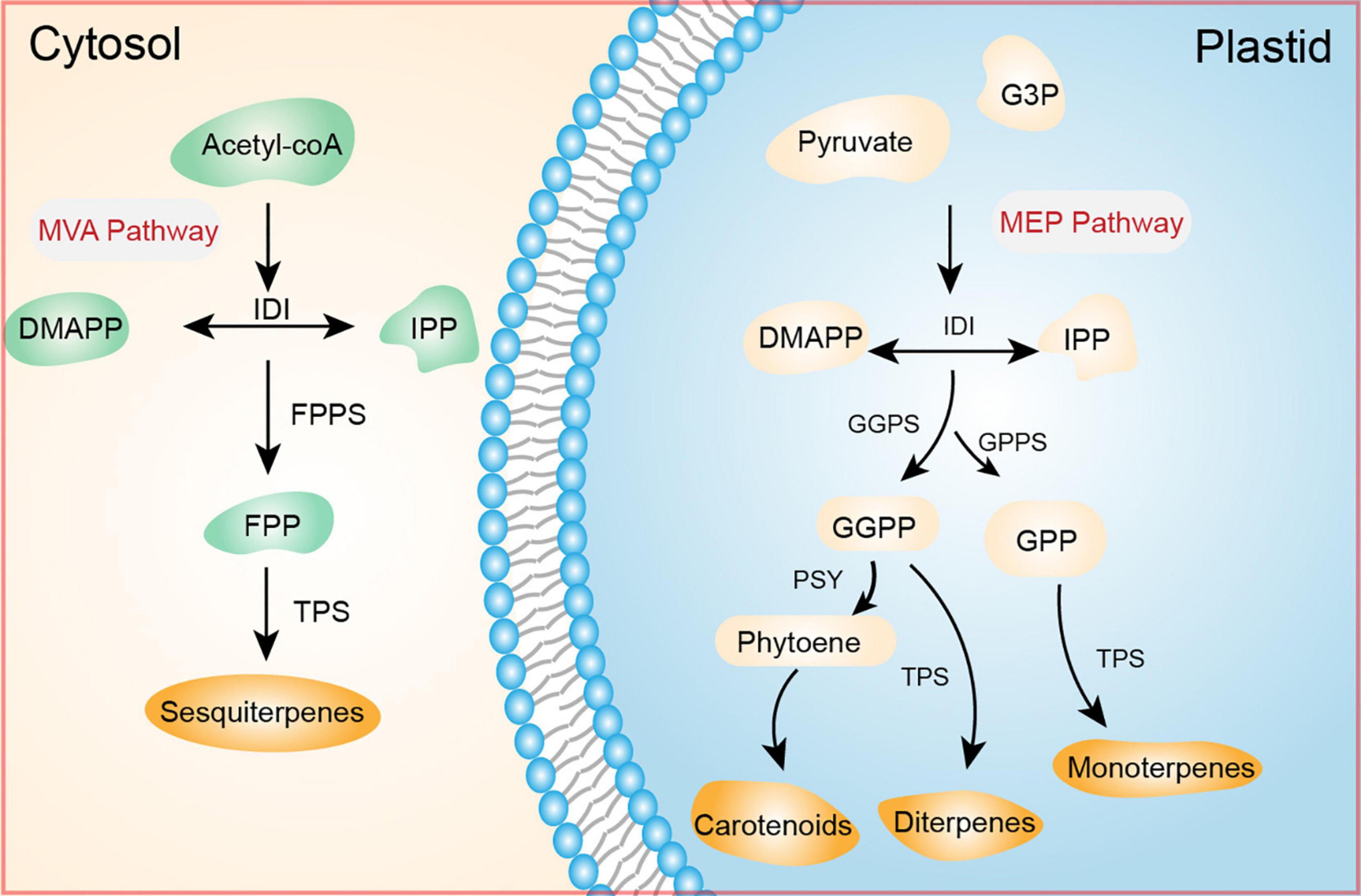
Figure 1. Terpenoid biosynthetic pathway. Terpenoid precursors (acetyl-CoA and pyruvate) enter the MVA pathway in the cytosol to produce sesquiterpenes or the MEP pathway in plastids to generate monoterpenes, diterpenes, and carotenoids. The enzymes and intermediates of both pathways are shown. DMAPP, dimethylallyl diphosphate; FPP, farnesyl diphosphate; FPPS, farnesyl pyrophosphate synthase; GGPP, geranylgeranyl pyrophosphate; GGPS, geranylgeranyl pyrophosphate synthase; GPP, geranyl diphosphate; GPPS, geranyl diphosphate synthase; IDI, isopentenyl diphosphate isomerase; IPP, isopentenyl diphosphate; PSY, phytoene synthase; TPS, terpenoid synthase.
Terpenoid concentrations in flowers and fruits vary according to developmental stage. For example, in berries, terpenes accumulate during the early stages of development. Terpene compounds synthesized during this stage might be precursors for the final products (Kalua and Boss, 2009). In lavender (L. angustifolia), terpenoid quantities are closely related to flower maturity (Li et al., 2019).
Carotenoids are important components of fruit aroma and important precursors of volatile norisoprenoids, which influence the aroma profile of fruits despite their presence at low levels (Watanabe et al., 2009). In some tomato and watermelon varieties, the degradation of carotenoids into lycopene pigment (red) produces geranial, a lemon-scented monoterpene aldehyde (Azulay et al., 2005; Crops et al., 2005). Furthermore, the degradation of β-carotene and lycopene in “Sui hong” papaya fruit results in a pleasant aromatic odor (Jing et al., 2015). In cashew apple (Anacardium occidentale L.) juice, the thermal degradation of carotenoids produces an aroma profile of 33 active odor volatiles, such as 1,2,3,5-tetramethylbenzene, naphthalene, and p-xylene (Queiroz et al., 2014). Carotenoids are also involved in floral scent in Osmanthus fragrans (Baldermann et al., 2010; Xi et al., 2021). β-ionone, a carotenoid derivative, is produced by the flowering plants Rosa moschata, Thymus vulgaris, Viola tricolor, Medicago marina, and Myrtus communis (Paparella et al., 2021).
Phenylpropanoid/Benzenoid Pathway
Phenylpropanoids and benzenoids are the second largest class of plant VOCs (Knudsen and Gershenzon, 2006). Only phenylpropanoids containing a reduced carboxyl group at C9 (i.e., aldehydes, alcohols, or alkenes) and/or alkyl addition to hydroxyl groups of the benzyl ring or to the carboxyl group (i.e., ethers and esters) are considered volatiles (Dudareva and Pichersky, 2000). According to the side-chain length, aromatic compounds are classified as phenylpropanoids (C6–C3), phenylpropanoid-related (C6–C2), and benzenoids (C6–C1) (Muhlemann et al., 2014; Lackus et al., 2021; Figure 2B). Phenylpropanoids and benzenoids are produced from the aromatic amino acid phenylalanine (Phe) via the shikimate pathway (Maeda and Dudareva, 2012; Yoo et al., 2013). The first step in phenylpropanoid and benzenoid biosynthesis is catalyzed by phenylalanine ammonia-lyase (PAL), the entry-point enzyme of the general phenylpropanoid biosynthetic pathway, which converts Phe to trans-cinnamate (CA) (Bate et al., 1994; Boatright et al., 2004; Orlova et al., 2006).
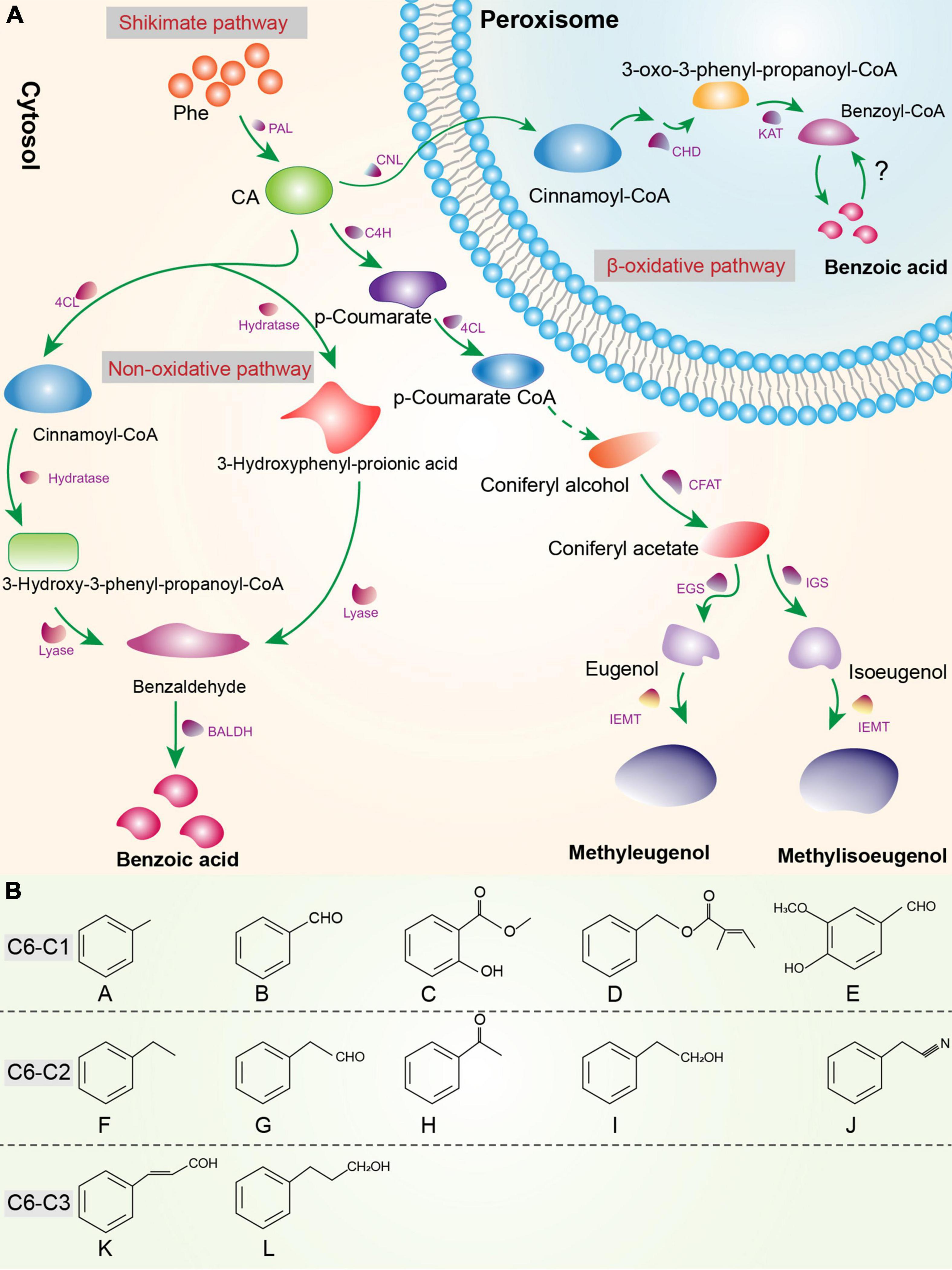
Figure 2. (A) Phenylpropanoid and benzenoid biosynthesis. All shikimate pathway enzymes and intermediates in the cytosol and peroxisome are shown. BALDH, benzaldehyde dehydrogenase; CA, trans-cinnamate; CHD, cinnamoyl-CoA hydratase/dehydrogenase; CFAT, coniferyl alcohol acyltransferase; C4H, cinnamate 4-hydroxylase; CNL, cinnamate-CoA ligase; 4CL, 4-hydroxycinnamoyl CoA ligase; EGS, eugenol synthase; IEMT, isoeugenol O-methyltransferase; IGS, isoeugenol synthase; PAL, phenylalanine ammonia lyase; KAT, 3-ketoacyl thiolase 1.(B) Chemical structures of benzenoid (C6–C1) compounds. A, methylbenzene; B, benzaldehyde; C, methyl 2-hydroxybenzoate (methyl salicylate); D, benzyl (£)-2-methyl-2-butenoate (benzyl tiglate); E, 4-hydroxy-3-methoxybenzaldehyde (vanillin). Phenylpropanoid-related (C6–C2) compounds: F, ethylbenzene; G, phenylacetaldehyde; H, acetophenone; I, 2-phenylethanol; J, 2-phenylacetonitrile. Phenylpropanoid (C6–C3) compounds: K (£)-cinnamic aldehyde; L, phenylpropanol.
To synthesize phenylpropanoids, CA is hydroxylated by cinnamate 4-hydroxylase (C4H), producing p-coumarate. Consequently, 4-coumaroyl CoA ligase catalyzes the conversion of p-coumarate into p-coumaroyl-CoA (Deng and Lu, 2017). A series of enzymatic reactions is required for the formation of coniferyl alcohol, which is acetylated by coniferyl alcohol acyltransferase and converted to coniferyl acetate. The NADPH-dependent reductases eugenol synthase and isoeugenol synthase (IGS) catalyze the conversion of coniferyl acetate to eugenol and isoeugenol, respectively (Ferrer et al., 2008; Huang et al., 2020). Eugenol and isoeugenol are methylated by isoeugenol O-methyltransferases to produce the volatiles methyleugenol and methyl isoeugenol (Gang et al., 2002; Dudareva et al., 2013; Figure 2A).
To synthesize benzenoids, the propyl side chain of CA is shortened by two carbons. Multiple chain-shortening pathways have been proposed: two nonoxidative pathways in the cytosol (CoA-dependent or CoA-independent), and a β-oxidative pathway in peroxisomes (Widhalm and Dudareva, 2015). The CoA-dependent non-oxidative pathway involves the conversion of CA to cinnamoyl-CoA by 4-hydroxycinnamoyl CoA ligase. Thereafter, hydratase catalyzes cinnamoyl-CoA to 3-hydroxy-3-phenyl-propanoyl-CoA, from which benzaldehyde is produced by lyase. In the CoA-independent non-oxidative pathway, CA is converted by hydratase to 3-hydroxyphenyl-propionic acid, which is reduced by lyase to benzaldehyde (Widhalm and Dudareva, 2015). The last step is the production of benzoic acid by benzaldehyde dehydrogenase, as characterized in Snapdragon flowers (Anthirrhinum majus) (Long et al., 2009) and proposed in petunia flowers (Kim et al., 2019).
The β-oxidative pathway has been described in petunia (Petunia hybrida) flowers and Arabidopsis (A. thaliana) (Moerkercke et al., 2009; Qualley et al., 2012). The β-oxidative pathway includes shortening of the propyl side chain in three enzymatic steps. First, CA is converted by cinnamate-CoA ligase to cinnamoyl-CoA. The bifunctional cinnamoyl-CoA hydratase/dehydrogenase, which is responsible for two intermediate steps (hydration and oxidation), converts cinnamoyl-CoA to 3-oxo-3-phenyl-propanoyl-CoA (Qualley et al., 2012). Finally, 3-oxo-3-phenyl-propanoyl-CoA is converted by 3-keto-acyl CoA-thiolase to benzoyl-CoA (Moerkercke et al., 2009). Benzoyl-CoA is a precursor of volatile and nonvolatile benzenoids (Lackus et al., 2021). Unfortunately, the steps and several enzymes of the benzenoid pathway are unknown.
The phenylpropanoid and benzenoid biosynthetic pathways compete for Phe (opposite relationship). For example, in Phlox subulata cultivars, the emission of larger quantities of benzenoids will produce little or no phenylpropanoid volatiles and vice versa (Majetic and Sinka, 2013). Because phenylpropanoids and their derivatives are widely distributed in flowers (Ramya et al., 2017) and fruits (Neelam et al., 2020), further investigations of their synthesis mechanisms are warranted.
Fatty Acid Derivatives Pathway
Fatty acids are the major precursors of volatile compounds in floral scents and fruit aromas; they are catabolized via the lipoxygenase (LOX) pathway (Sanz et al., 1997). The LOX pathway is important for the production of aromatic compounds. Polyunsaturated fatty acids—linoleic (C18:2) and linolenic (C18:3) acids—are the main substrates of LOX in plant tissues (Espino-Díaz et al., 2016). Linoleic and linolenic acids are produced from phospholipids, triacylglycerols, and glycolipids by acyl hydrolases. Soluble cytosolic LOXs are divided into two major subfamilies: 9- and 13-LOXs (Baysal and Demirdöven, 2007; Viswanath et al., 2020). LOXs catalyze the addition of oxygen to polyunsaturated fatty acids at the 9 or 12 position, yielding unsaturated 9- or 13-hydroperoxides. Thereafter, 9-hydroperoxide lyase and 13-hydroperoxide lyase convert 9- and 13-hydroperoxides to C9 and C6 aldehydes, respectively. These C9 and C6 aldehydes are reduced by alcohol dehydrogenases (ADH) to C9 and C6 alcohols, whereas C6 alcohols are converted into esters by alcohol acyltransferase (Jerković et al., 2019). The divergence of esters depends on substrate availability, enzyme specificity, and ATT gene variation (Sanz et al., 1997; Espino-Díaz et al., 2016). Jasmonic acid can be generated from 13-hydroperoxy through a separated branch via production of an unstable epoxide by allene oxide synthase, followed by a series of cyclization reduction reactions (Muhlemann et al., 2014; Ramya et al., 2018; Figure 3).
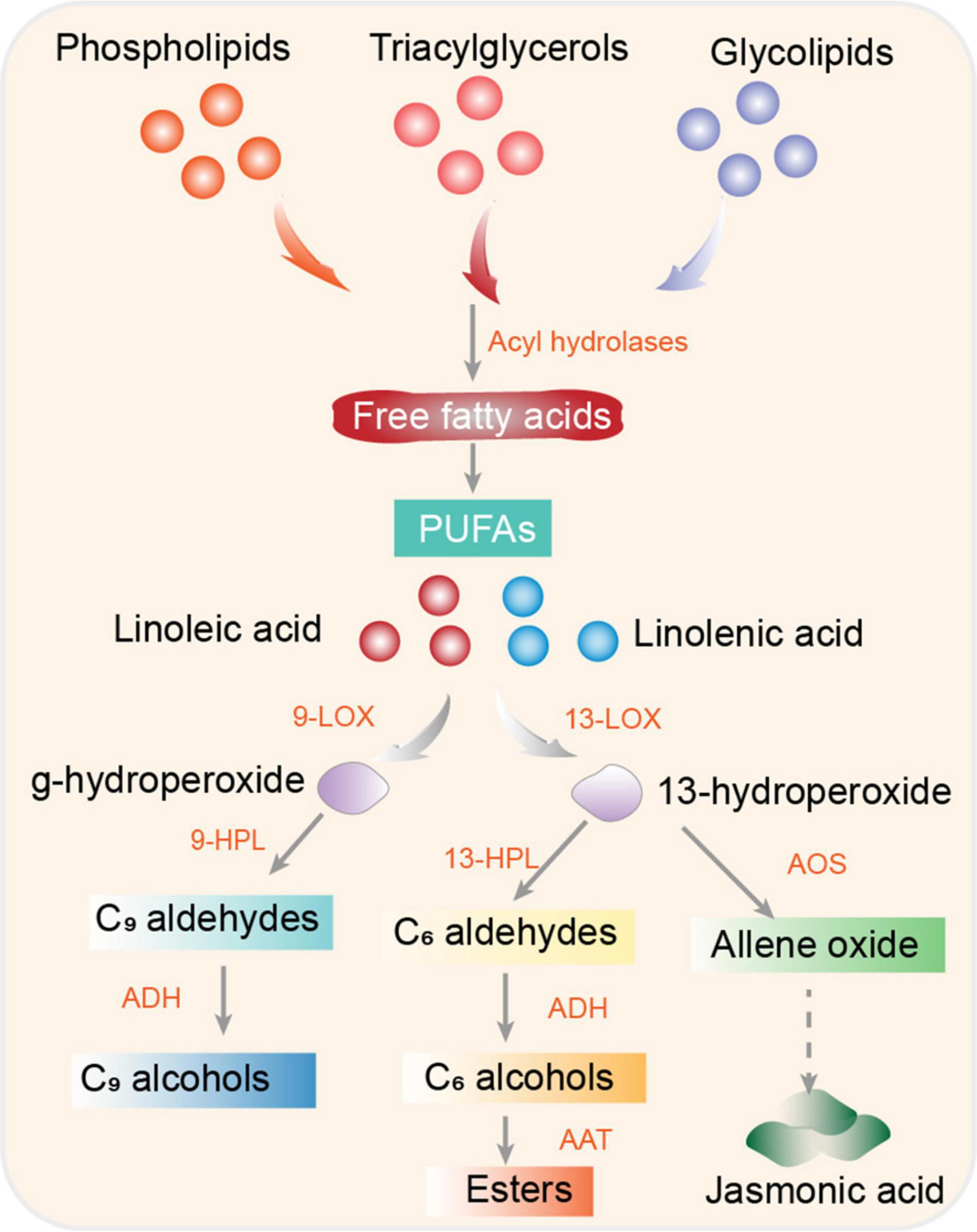
Figure 3. Fatty acid derivative biosynthetic pathway. Fatty acid precursors (linoleic and linolenic acids) enter the LOX pathway and are converted to 9- and 13-hydroperoxide, which are oxidized and converted to volatiles by hydroperoxide lyase (HPL) and alcohol dehydrogenase (ADH). AAT, alcohol acyltransferase; ADH, alcohol dehydrogenase; AOS, allene oxide synthase; HPL, hydroperoxide lyase; LOX, lipoxygenase; PUFAs, polyunsaturated fatty acids.
Amino Acid Derivatives
Amino acids are precursors for various plant aromatic secondary metabolites (Vogt, 2010), such as aldehydes, alcohols, acids, and esters. There are three main aromatic amino acids in plants, which are the precursors for various secondary metabolites: Phe, tyrosine (Tyr), and tryptophan (Trp). The amino acid Phe is a precursor for multiple functional secondary metabolites: phenylpropanoids, flavonoids, cell wall lignin, anthocyanins, and various other compounds (Tzin and Galili, 2010b; Qian et al., 2019). Chorismate is converted by chorismate mutase (CM) to prephenate as the first step in Phe biosynthesis (Mobley et al., 1999). The conversion of chorismate to Phe (via prephenate and arogenate) is catalyzed by prephenate aminotransferase and arogenate dehydratase, respectively (Cho et al., 2007; Yamada et al., 2008; Maeda et al., 2010). Furthermore, plants can produce Phe through a microbe-like phenylpyruvate pathway involving prephenate dehydratase; flux is increased when the entry point to the arogenate pathway is limiting (Yoo et al., 2013). The volatile Tyr has the same biosynthetic pathway as Phe, involving derivation from chorismate and arogenate. The last step is conversion by arogenate dehydrogenase (TyrA) to Tyr (Schenck et al., 2020; Yokoyama et al., 2021). This pathway has been explained in plants such as tobacco (Gaines et al., 1982), sorghum (Connelly and Conn, 1986), and Arabidopsis (Rippert and Matringe, 2002; Rippert et al., 2009). Alternatively, Tyr biosynthesis can involve prephenate conversion to 4-hydroxyphenylpyruvate by prephenate dehydrogenase and possibly TyrA2 (Rippert and Matringe, 2002). Thereafter, 4-hydroxyphenylpyruvate is converted to Tyr by aminotransferases (e.g., 4-hydroxyphenylpyruvate amino transferase) (Tzin and Galili, 2010a).
The first committed step of volatile Trp biosynthesis is the transfer of an amino group of glutamine to chorismate to produce anthranilate and pyruvate; this is performed by anthranilate synthase (ASα and ASβ) (Tzin and Galili, 2010a). Thereafter, anthranilate phosphoribosylanthranilate transferase (AnPRT) converts anthranilate and phosphoribosyl pyrophosphate into phosphoribosylanthranilate and inorganic pyrophosphate. The next step is conversion of phosphoribosylanthranilate into L-(O-carboxyphenylamino)-L-deoxyribulose-5-phosphate (CdRP); this is performed by phosphoribosylanthranilate isomerase. Furthermore, indole-3-glycerol phosphate synthase catalyzes the conversion of CdRP to indole-3-glycerol phosphate (Li et al., 1995). The last two steps in the Trp biosynthetic pathway are catalyzed by Trp synthase (TS), which has alpha (TSα) and beta (TSβ) subunits. Indole-3-glycerol phosphate is converted by TSα into indole and glyceraldehyde-3-phosphate (α-reaction). Finally, indole is transported to TSβ, which catalyzes its condensation with serine (β-reaction) to generate Trp (Miles, 2001; Weber-Ban et al., 2001; Parthasarathy et al., 2018; Figure 4).
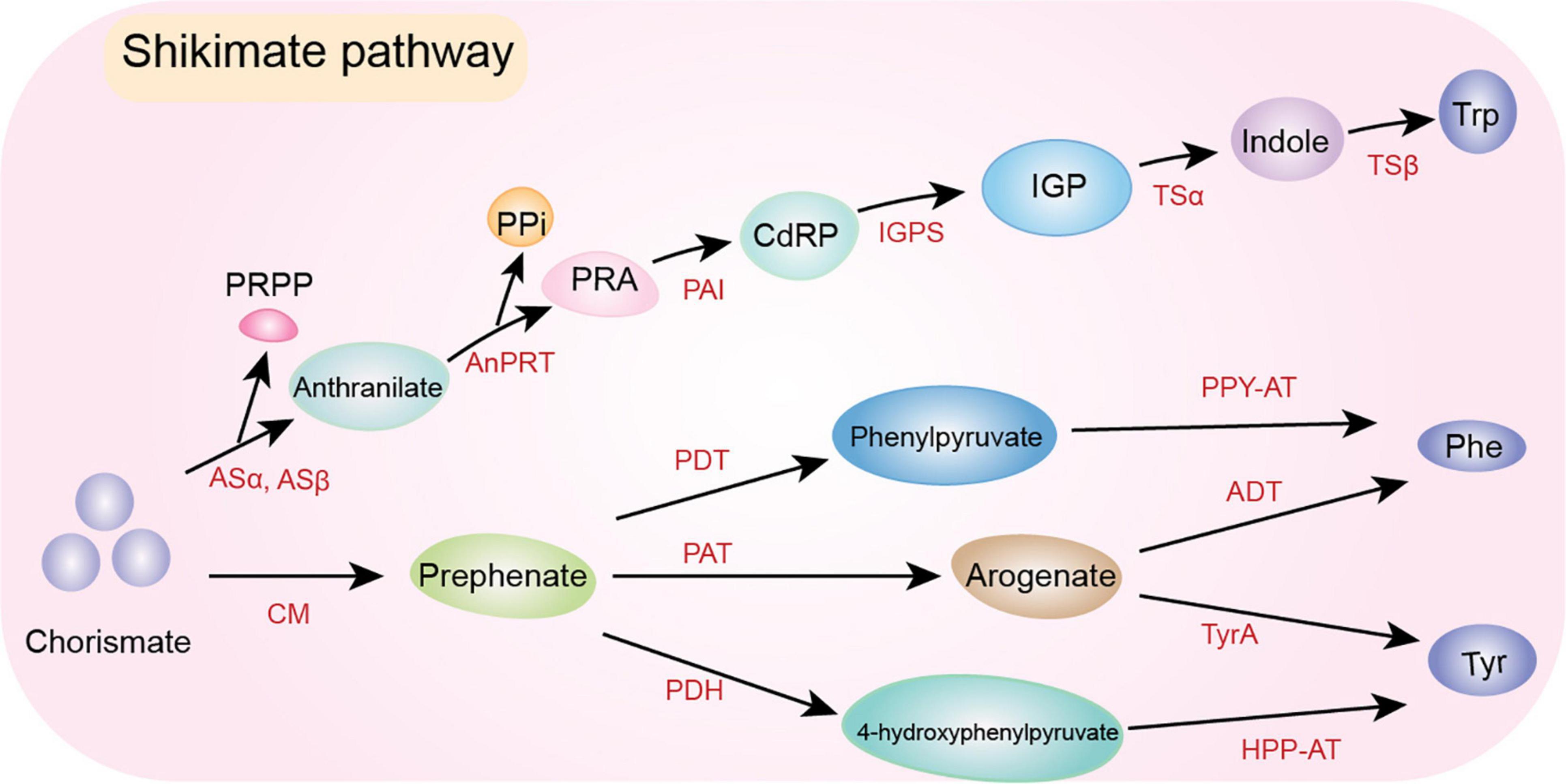
Figure 4. Phe, Tyr, and Trp biosynthetic pathways. Chorismate is the precursor of Phe, Tyr and Trp. Two distinctive pathways starting from the same precursor, Chorismate, where Phe and Tyr share some common intermediates (i.e., prephenate and arogenate) and Trp is produced from a separated branched-chain. ADT, arogenate dehydratase; AnPRT, anthranilate phosphoribosylanthranilate transferase; ASα; ASβ, anthranilate synthase; CdRP, L-(O-carboxyphenylamino)-l-deoxyribulose-5-phosphate; CM, chorismate mutase; HPP-AT, 4-hydroxyphenylpyruvate aminotransferase; IGP, indole-3-glycerol phosphate; IGPS, indole-3-glycerol phosphate synthase; PAI, phosphoribosylanthranilate isomerase; PAT, prephenate aminotransferase; PDH, prephenate dehydrogenase; PDT, prephenate dehydratase; Phe, phenylalanine; PPi, inorganic pyrophosphate; PPY-AT, phenylpyruvate aminotransferase; PRA, phosphoribosylanthranilate; PRPP, phosphoribosyl pyrophosphate; Trp, tryptophan; TSα, tryptophan synthase alpha subunit; TSβ, tryptophan synthase beta subunit; Tyr, tyrosine; TyrA, arogenate dehydrogenase.
Amino acids undergo deamination or transamination, forming the corresponding α-keto acid, a key intermediate in the conversion of amino acids to volatiles (Gonda et al., 2010; Pott et al., 2019); this reversible step is catalyzed by branched-chain aminotransferases (Klee, 2010). For example, in tomato, six of these enzymes are present in chloroplasts, mitochondria, and the cytoplasm (Kochevenko et al., 2012). Decarboxylation is the most likely route for conversion of α-keto acids to aldehyde volatiles, followed by SDR-mediated synthesis of alcohols (Klee, 2010).
Various synthesis mechanisms of Phe-derived volatiles, such as phenylacetaldehyde and 2-phenylethanol, which contribute to fruit flavors and floral scents have been studied. For instance, in tomato plants, aromatic L-amino acid decarboxylases convert Phe to phenethylamine, the first step in phenylacetaldehyde and 2-phenylethanol synthesis (Tieman et al., 2006). Conversion of phenylacetaldehyde to 2-phenylethanol by phenylacetaldehyde reductases is the last step in the pathway (Tieman et al., 2007). Notably, P. hybrida contains a bi-functional decarboxylase/amine oxidase enzyme that directly converts Phe to phenylacetaldehyde (Kaminaga et al., 2006). Additionally, the synthesis of 2-phenylethanol (which has a pleasant fragrance) has been studied in rose (Sakai et al., 2007). Branched-chain alcohols, carbonyls, and esters are produced by metabolism of the amino acids leucine, isoleucine, valine, alanine, and aspartic acid (Heath and Reineccius, 1986; Sanz et al., 1997). Volatile esters are the largest group of volatile compounds produced by fruits. Fruit esters are formed by the esterification of alcohols and acyl CoA, which are derived from fatty acid and amino acid metabolism. This reaction is catalyzed by alcohol acyltransferase (Sanz et al., 1997). In post-climacteric banana slices, amino acids are converted to branched-chain alcohols and esters by aminotransferases, decarboxylases, and ADH (Sanz et al., 1997). The biosynthesis of higher alcohols is linked to amino acid deamination (Herrero et al., 2006).
Regulation of Floral and Fruit Volatile Compounds
Factors Affecting Volatile Organic Compound Emission
Floral Scent Volatiles
The emission of floral scents is regulated by time of release, environmental variables (light and temperature), tissue type, and plant status (age, physiological state, and sex). These factors stimulate olfactory communication (Raguso, 2008).
The circadian rhythm controls the emission patterns of floral scents in response to the day/night cycle (Picazo-Aragonés et al., 2020). Diurnal and nocturnal emission patterns have been reported in plant species. Some VOCs are emitted independently of environmental conditions; they are influenced by the endogenous biological clock (Zeng et al., 2017). The floral scents emitted by Myrtaceae species at night attract nocturnal bees (Cordeiro et al., 2019). In Hoya carnosa, the circadian rhythm is also expressed during exposure to continuous dark (Fenske and Imaizumi, 2016). The impact of the circadian clock on the release patterns of volatile terpenoids and phenylpropanoids/benzenoids has been reported; however, few studies have focused on volatile fatty acid derivatives (Zeng et al., 2017). For example, the circadian clock and light promote the diurnal emission of monoterpenes from orchid (Phalaenopsis violacea) flowers (Chuang et al., 2017). The endogenous circadian clock in petunia flower is proposed to regulate the rhythmic emission of volatile phenylpropanoids and benzenoids (Cheng et al., 2016). Moreover, an important fatty acid derivative, jasmonic acid, is controlled by the circadian clock (Zhang Y. et al., 2019).
Volatile compounds are synthesized and emitted from petunia petal limbs during the evening and shortly after anthesis; such emission is drastically decreased when limbs are not supplied with exogenous Phe, and post-pollination-derived ethylene causes the same effect (Dexter et al., 2007). In a single snapdragon flower, the upper and lower petal lobes release high levels of myrcene, ocimene, linalool, and nerolidol (Dudareva et al., 2003; Nagegowda et al., 2008). The cuticle of petunia flowers prevents the release of almost 50% of internal VOCs; a decrease in cuticle thickness influences the synthesis, cellular distribution, and mass transfer resistance of VOCs (Liao et al., 2021).
Floral VOCs respond to changes in environmental conditions, such as temperature, light, water availability, and soil nutrients. As demonstrated by Dötterl et al. (2005), volatile emissions by Silene latifolia decrease during hot and dry summer months, whereas low temperatures decrease scent emission in Pyrola grandiflora (Knudsen, 1994). Moreover, abiotic stresses influence plant-pollinator and plant-herbivore interactions through different floral VOCs (Campbell et al., 2019).
The developmental stage of flowers affects VOC emission patterns. The changes that occur in petal cells from budding to full blooming, along with the intercellular spaces, modulate VOC evaporation in jasmine (J. sambac) flowers (Chen et al., 2020).
Fruit Aroma Volatiles
Many factors regulate aroma emission by fruits. Fruit genotype influences flavor (Kongor et al., 2016). The final flavor profile is affected by environmental conditions such as climate, sunlight, soil, fruit ripening, harvesting time, and post-harvesting processes (Kongor et al., 2016). Environmental stresses (e.g., temperature and drought) influence fruit metabolism and aromatic compound content (Romero et al., 2021).
The VOC profiles of fruit varieties change according to maturation stage. Terpenoids dominate the aroma profile in some fruits during ripening, such as apple (Liu et al., 2021), apricot (Karabulut et al., 2018), and peach (Wei et al., 2021). In grape, some phenylpropanoids increase with maturation (Kambiranda et al., 2016). Furthermore, fatty acid and amino acid-related compounds increase during the maturation of apple (Liu et al., 2021) and apricot (Karabulut et al., 2018). Therefore, maturation is vital for VOC emission in fruits and affects commercial production.
Pre-harvest factors (water, sunlight, fertilization, and chemical application) influence fruit quality characteristics, including flavor (Ahmed et al., 2013). For example, the use of biofertilizers influences the fruit aroma profile and can improve flavors. Biofertilizer treatment promotes the production of volatile compounds in strawberry (Duan et al., 2021). Treatment of pomegranate and peach with salicylic acid, a natural plant phenolic, improves their quality, including flavor (Erogul and Özsoydan, 2020). Pesticides (e.g., triazole pesticides) can negatively affect fruit flavor compounds (Xiao et al., 2020).
Post-harvest techniques (e.g., chemical applications, radiation, cold, heat, and controlled storage atmospheres) are used to suppress disease and enhance fruit quality during storage (Ahmed et al., 2013). Nitric oxide, salicylic acid, 1-methylcyclopropene, and oxalic acid are used for fruit crops, altering their VOC contents (Bambalele et al., 2021). The application of chemical treatments, individually or in combination, can also alter VOC contents. Controlled atmosphere (CA) treatment of fruit aims to prevent the development of bitter pit disease by altering the aromatic contents (Ahmed et al., 2013). The application of CA treatment in combination with 1-methylcyclopropene to “Fuji” apples stored at room temperature promotes the emission of volatiles (Lu et al., 2018). Ethephon increases and 1-methylcyclopropene decreases the production of volatile compounds in apple (Kondo et al., 2005). Intriguingly, the combination of ethephon with methyl jasmonate decreases or increases VOCs in apple cultivars (Kondo et al., 2005). A combination of methyl jasmonate and ethanol significantly decreases the levels of volatiles in raspberry and enhances the aroma of strawberry (Blanch et al., 2011).
The flavor properties of fruits and their products are affected by ultraviolet radiation in grape (Del-Castillo-Alonso et al., 2021), mango (Wang et al., 2020), strawberry (Warner et al., 2021) and pepper fruit (Ma et al., 2021). Cold storage is used for fruit preservation worldwide. However, chilling injury and aroma loss are problems for commercial fruit production. During cold storage, VOC levels are significantly reduced because of precursor metabolism suppression; this occurs in banana (Zhu et al., 2018b), grape (Matsumoto and Ikoma, 2015), mango (Singh et al., 2004), tomato (Bai et al., 2022), and avocado (García-Rojas et al., 2016). CA storage of fruits is a common preservation technology involving the control of O2 and CO2 levels. This method increases the levels of some volatile compounds, while decreasing the levels of other volatile compounds. The storage of mango in a high level of CO2 reduces the monoterpene and sesquiterpene levels, while increasing the levels of esters and norisoprenoids (Lalel et al., 2003b). High-pressure and decompression technology, ethylene scavengers, ozone (O3), active packaging, plasma treatment, high-voltage electrostatic field, and pulse-CA with modified atmosphere preservation have been evaluated for fruit preservation (Fang and Wakisaka, 2021).
Heat treatment is performed after harvesting to control insects, inhibit ripening, and induce resistance to chilling injury (Lu et al., 2007). Heat processing influences fruit aromatic compound contents (e.g., it significant increases off-flavors in watermelon juice) (Aboshi et al., 2020). Drying is used for fruit conservation; it also influences their VOC contents. In banana, ester and aldehyde levels decrease rapidly in response to drying, whereas alcohol levels initially increase then decrease (Saha et al., 2018). The volatile profiles of strawberry subjected to different drying methods have been reported (Abouelenein et al., 2021). Most commercial fruits undergo post-harvest treatments or preservation; however, the effects on fruit aromas are unclear.
Glycosides, Ethylene, and Enzymes Influencing Volatile Organic Compound Emission
Glycosides control volatile emission by flowers and fruits (Schwab et al., 2015). Glycoside volatiles are odorless and release free aroma volatiles upon hydrolysis by β-glucosidase (Yauk et al., 2014; Schwab et al., 2015). Glycosides enhance the flavor of grape wine and tea; they also modify fruit aroma during maturation, storage, and processing (Birtic et al., 2009; Garcia et al., 2013; Yauk et al., 2014; Ohgami et al., 2015).
Ethylene production accelerates the release of VOCs during fruit ripening. For example, the overexpression of a ripening inhibitor (rin) gene led to decreased ethylene production in tomato fruit, thereby reducing volatile levels. Fruits with antisense aminocyclopropanecarboxylic acid synthase have low levels of several volatiles. Fruits with downregulated phytoene synthase have lower levels of carotenoid-derived volatiles, whereas fruits with antisense pectin methylesterase have a lowered level of methanol (Baldwin et al., 2000).
Genetic and Epigenetic Regulation of Volatile Organic Compound Biosynthesis
Genes
Discovery of important genes in volatile biosynthetic pathways has revealed the molecular mechanisms underlying regulation of floral scent and fruit aroma. Multiple studies have focused on isolating terpenoid-related genes in various plant species. For example, in Lilium “Siberia,” three terpenoid-related genes (LoTPS2, LoTPS4 and LoTPS5) have been isolated and characterized. Among them, LoTPS2 catalyzes (E, E)-α-farnesene, LoTPS4 generates D-limonene and β-myrcene, and LoTPS5 produces squalene (Abbas et al., 2020a,b). Regarding phenylpropanoids and benzenoids, only the genes responsible for the biosynthesis of phenylacetaldehyde and 2-phenylethanol have been discovered (Picazo-Aragonés et al., 2020) (e.g., RhPAAS in rose) (Roccia et al., 2019). Little is known regarding the genes responsible for the synthesis of fatty acid and amino acid derivatives.
Transcriptome analysis is used to identify genes involved in the metabolism of floral scents and fruit aromas. There have been reports of transcriptome and genes underlying the regulation of floral scents and fruit aromas of rose (R. chinensis “Old Blush” and R. odorata var. gigantean) (Guo et al., 2017), orchid (C. sinense) (Yang F. X. et al., 2021), orchid (Vanda Mimi Palmer) (Toh et al., 2017), peony (Paeonia suffruticosa Andr.) (Zhang et al., 2021), Lilium “Siberia” (Hu et al., 2017), water lily (Zhang et al., 2020), carnation (D. caryophyllus) (Tanase et al., 2012), lavender (L. angustifolia) (Li et al., 2019), jasmine (J. sambac) (Chen et al., 2020), Polianthes tuberosa (Fan et al., 2018) and the Chinese narcissus (He et al., 2020), banana (Kaur et al., 2021), apple “Ruixue” (Liu et al., 2021), grape (V. vinifera) (Sun et al., 2019), mango (Xin et al., 2021), and apricot (Zhang Q. et al., 2019). The identification of sets of genes involved in VOC synthesis will provide insight into the corresponding metabolic pathways.
Epigenetics
The formation of VOCs is modulated by DNA methylation, histone protein modifications, and chromatin structure remodeling (Picazo-Aragonés et al., 2020). Cold storage of tomato plant reduces its flavor content; it also causes DNA methylation changes (Zhang et al., 2016). Chromatin-based regulatory mechanisms in petunia flower affect genes involved in phenylpropanoid metabolism, implicating histone acetylation in VOC synthesis (Patrick et al., 2021).
Transcription Factors
Numerous transcription factors (TFs) regulate the production of volatile compounds. In petunia (P. hybrida) flowers, TFs regulate the phenylpropanoid/benzenoid network. For example, ODORANT1 (ODO1), a member of the R2R3-type MYB family, regulates the synthesis of precursors in the shikimate pathway; it also controls the transcription of entry points to the Phe and phenylpropanoid pathways. Suppression of ODO1 expression reduces the transcript levels of multiple genes in this pathway: DAHPS, EPSPS, PAL, CM, and SAMS (Verdonk et al., 2005). ODO1 activates the ABC transporter promoter (ATP-binding cassette transporter), which is localized to the plasma membrane (Van Moerkercke et al., 2012); it also regulates the emission of volatile compounds (Adebesin et al., 2017). The TF Lilium hybrid ODO1 (LhODO1) was isolated from Oriental and Asiatic hybrid lilies; it regulates the production of phenylpropanoids/benzenoids (Yoshida et al., 2018).
EMISSION OF BENZENOIDS II (EOBII), a member of the R2R3-type MYB family, regulates ODO1 and activates the promoter of the biosynthetic gene isoeugenol synthase (Spitzer-Rimon et al., 2010; Colquhoun et al., 2011a; Van Moerkercke et al., 2011). Petunia EOBI (R2R3-MYB–like) interacts with regulatory genes; it acts both downstream of EOBII and upstream of ODO1 (Spitzer-Rimon et al., 2012). Numerous genes in the shikimate and phenylpropanoid pathways [5-enolpyruvylshikimate-3-phosphate synthase (EPSPS), 3-deoxy-D-arabinoheptulosonate 7-phosphate synthase (DAHP synthase), chorismate synthase, CM, arogenate dehydratase, and PAT), and scent-related genes (PAL, isoeugenol synthase, and benzoic acid/salicylic acid carboxyl methyltransferase] are downregulated by the silencing of EOBI expression (Spitzer-Rimon et al., 2012). The interaction between PhERF6 and EOBI negatively regulates benzenoid biosynthesis in petunia (Liu et al., 2017). Moreover, in petunia flower and tobacco, the overexpression of PAP1 (R2R3 MYB-type TF) increases the levels of anthocyanins and volatile compounds (phenylpropanoids/benzenoids) (Xie et al., 2006; Zvi et al., 2008). In rose, overexpression of PAP1 increases the levels of volatile phenylpropanoids/benzenoids and volatile terpenoids (Zvi et al., 2012). Furthermore, MYB4TF represses cinnamate-4-hydroxylase in the phenylpropanoid pathway, influencing the production of phenylpropanoid compounds in petunia (Colquhoun et al., 2011b). In the Cymbidium cultivar “Sael Bit,” CsMYB1 positively regulates genes responsible for the synthesis of phenylpropanoids/benzenoids and esters. CsMYB1 is highly expressed in petals and columns, particularly at the fully open flower stage, when compared with sepals and labella (Ramya et al., 2019).
Six TF families are implicated in the regulation of terpenoid biosynthesis: AP2/ERF, ARF, bHLH, bZIP, MYB, and WRKY (Picazo-Aragonés et al., 2020). MYC2 (bHLH family) induces the expression of two sesquiterpene synthase genes (TPS11 and TPS21) in Arabidopsis (Hong et al., 2012). In rose flowers, RhMYB1 is involved in floral scent biosynthesis (Yan et al., 2011). In Syringa oblata flowers, two R2R3-MYB TFs are upregulated at the bud and flowering developmental stages; they are involved in the biosynthesis of terpenoids and monoterpenoids (Zheng et al., 2015). In Hedychium coronarium, HcMYB3-6 (scent related R2R3-MYB TFs) is highly expressed at full flowering stages, but its expression levels are low in other plant organs (Yue et al., 2015). MYBs from H. coronarium and S. oblata control the biosynthesis of terpenoid volatile compounds (Ramya et al., 2017). In H. coronarium, several HcMYB genes regulate terpenoid and benzenoid biosynthesis (Abbas et al., 2021b); HcARF5 regulates β-ocimene production (Abbas et al., 2021a). Some monoterpenes in sweet osmanthus (O. fragrans) are regulated by OfWRKY genes (Ding et al., 2020), and OfERF61 regulates β-ionone biosynthesis (Han et al., 2019). In Phalaenopsis orchids, multiple TFs (e.g., PbbHLH4, PbbHLH6, PbbZIP4, PbERF1, PbERF9, and PbNAC1) regulate the expression of PbGDPS and its downstream putative monoterpene synthases genes, PbTPS5 and PbTPS10 (Chuang et al., 2017). CrWRKY1 positively regulates the biosynthesis of terpenoid indole alkaloid in Catharanthus roseus (Suttipanta et al., 2011). Artemisinin, a sesquiterpene, is regulated by AabZIP1 in Artemisia annua (Zhang et al., 2015). Linalool synthesis in Cinnamomum osmophloeum is controlled by CoWRKY (Lin et al., 2014). The overexpression of CpMYC2 increases the linalool level, while overexpression of CpbHLH13 increases the β-caryophyllene level, in wintersweet (Chimonanthus praecox L.) flowers (Aslam et al., 2020).
Several TFs linked to fruit VOCs have been characterized. For example, in wine grape, VviWRKY40 regulates monoterpenoid glycosylation (Li et al., 2020). E-geraniol synthesis is positively regulated by CitERF71 in sweet orange (Li et al., 2017). In ripe kiwifruit (Actinidia arguta), NAC and ETHYLENE-INSENSITIVE3-like TF binding sites in the terpene synthase-1 protein (AaTPS1) promoter activate its expression; the absence of NAC (AaNAC2, AaNAC3 and AaNAC4) reduces the AcTPS1 transcript and protein levels, as well as the monoterpene volatile content (Nieuwenhuizen et al., 2015). Further studies of TFs will provide insights into the mechanisms underlying regulation of fruit aroma production. The major TFs that regulate VOCs are listed in Table 2.
Perspectives
Studies of floral and fruit aromas have generated extensive information concerning their volatile compounds, functions, biosynthesis, and regulation. Moreover, new techniques and methods have accelerated the discovery of VOCs and their synthetic enzymes, genes, and TFs, thereby enhancing the broader understanding of VOC biosynthesis in plants. However, several unresolved issues warrant further investigation.
The aromas of flowers and fruits serve as signals to pollinators or fruit eaters; however, most horticultural varieties and cultivars are selected by human preference. The identification of VOCs relevant to human sensory preference is important to ensure that consumer demand is met. Additionally, biotechnological modification of the aromatic characteristics of plants or engineering of synthesis pathways in microbial cell factories could increase aromatic metabolite production for commercial exploitation.
Studies of the composition, synthesis, and regulation of floral scents and fruit aromas have focused on major plants, such as petunia (floral scents), tomato, apple (fruit aromas), and model plants. Little information is available concerning other aromatic plant species, possibly because of scarce genome information and difficulties with genetic transformation. The rapid development of genomic techniques, particularly gene editing, enables research regarding genome functions and regulatory mechanisms in a range of plant species. Flowers differ from edible fruits and may be less restricted by concerns involving public opinion and governmental regulation. Thus, genome editing can be applied to produce flowering plants with novel aromas and phenotypes, as well as improved sensory properties.
Most studies concerning the functions of fruit VOCs have focused on the biological activities of VOCs against microorganisms and insects, or on the attraction of animal dispersers; investigations of flower VOCs have focused on pollinator attraction. However, the effects of flower and fruit VOCs on human health are unknown. While VOCs improve fruit/flower edible/aromatic qualities, they also have potential for improving human physical and mental health. The utility of flower fragrances and fruit aromas as natural adjuvant therapies for chronic diseases or other health problems warrants further research.
Author Contributions
SM and BJ led the writing of this manuscript, contributed in the writing and reviewing for the manuscript. YW draw the figures. WZ reviewed the manuscript. All authors contributed to the article and approved the submitted version.
Conflict of Interest
The authors declare that the research was conducted in the absence of any commercial or financial relationships that could be construed as a potential conflict of interest.
Publisher’s Note
All claims expressed in this article are solely those of the authors and do not necessarily represent those of their affiliated organizations, or those of the publisher, the editors and the reviewers. Any product that may be evaluated in this article, or claim that may be made by its manufacturer, is not guaranteed or endorsed by the publisher.
Supplementary Material
The Supplementary Material for this article can be found online at: https://www.frontiersin.org/articles/10.3389/fpls.2022.860157/full#supplementary-material
Footnotes
References
Abbas, F., Ke, Y., Zhou, Y., Ashraf, U., Li, X., Yu, Y., et al. (2020a). Molecular cloning, characterization and expression analysis of LoTPS2 and LoTPS4 involved in floral scent formation in oriental hybrid Lilium variety “Siberia”. Phytochemistry 173:112294. doi: 10.1016/j.phytochem.2020.112294
Abbas, F., Ke, Y., Zhou, Y., Waseem, M., Yu, Y., Ashraf, U., et al. (2020b). Cloning, functional characterization and expression analysis of LoTPS5 from Lilium “Siberia”. Gene 756:144921. doi: 10.1016/j.gene.2020.144921
Abbas, F., Ke, Y., Zhou, Y., Yu, Y., Waseem, M., Ashraf, U., et al. (2021b). Genome-wide analysis reveals the potential role of MYB transcription factors in floral scent formation in Hedychium coronarium. Front. Plant Sci. 12:623742. doi: 10.3389/fpls.2021.623742
Abbas, F., Ke, Y., Zhou, Y., Yu, Y., Waseem, M., Ashraf, U., et al. (2021a). Genome-wide analysis of ARF transcription factors reveals HcARF5 expression profile associated with the biosynthesis of β-ocimene synthase in Hedychium coronarium. Plant Cell Rep. 40, 1269–1284. doi: 10.1007/s00299-021-02709-1
Abobatta, F. W. (2019). Nutritional benefits of citrus fruits. Am. J. Biomed. Sci. Res. 3, 303–306. doi: 10.34297/ajbsr.2019.03.000681
Aboshi, T., Musya, S., Sato, H., and Ohta, T. (2020). Changes of volatile flavor compounds of watermelon juice by heat treatment. Biosci. Biotech. Biochem. 84, 2157–2159. doi: 10.1080/09168451.2020.1787814
Abouelenein, D., Mustafa, A. M., Angeloni, S., Borsetta, G., Vittori, S., Maggi, F., et al. (2021). Influence of freezing and different drying methods on volatile profiles of strawberry and analysis of volatile compounds of strawberry commercial jams. Molecules 26:4153. doi: 10.3390/molecules26144153
Adebesin, F., Widhalm, J. R., Boachon, B., Lefèvre, F., Pierman, B., Lynch, J. H., et al. (2017). Emission of volatile organic compounds from petunia flowers is facilitated by an ABC transporter. Science 356, 1386–1388. doi: 10.1126/science.aan0826
Ahmed, M., El, M., Zhang, F., Wu, F., Zhou, C., and Tao, J. (2013). Advances in fruit aroma volatile research. Molecules 18, 8200–8229. doi: 10.3390/molecules18078200
Alam, E. A. (2014). Volatile components of banana peels tea; a new pharmaceutical product. Int. J. Pharm. Pharm. Sci. 6, 16–17.
Ali, M. M., Hashim, N., Aziz, S. A., and Lasekan, O. (2020). Exploring the chemical composition, emerging applications, potential uses, and health benefits of durian: a review. Food Control 113:107189. doi: 10.1016/j.foodcont.2020.107189
An, M., Haig, T., and Hatfield, P. (2001). On-site field sampling and analysis of fragrance from living Lavender (Lavandula angustifolia L.) flowers by solid-phase microextraction coupled to gas chromatography and ion-trap mass spectrometry. J. Chromatogr. A 917, 245–250. doi: 10.1016/S0021-9673(01)00657-4
Androutsopoulou, C., Christopoulou, S. D., Hahalis, P., Kotsalou, C., Lamari, F. N., and Vantarakis, A. (2021). Evaluation of essential oils and extracts of rose geranium and rose petals as natural preservatives in terms of toxicity, antimicrobial, and antiviral activity. Pathogens 10:494. doi: 10.3390/pathogens10040494
Arimura, G., and Pearse, I. S. (2017). From the lab bench to the forest: ecology and defence mechanisms of volatile-mediated “Talking Trees.”. Adv. Bot. Res. 82, 3–17. doi: 10.1016/bs.abr.2016.08.001
Aros, D., Garrido, N., Rivas, C., Medel, M., Müller, C., Rogers, H., et al. (2020). Floral scent evaluation of three cut flowers through sensorial and gas chromatography analysis. Agronomy 10:131. doi: 10.3390/agronomy10010131
Arroyo, F. T., Moreno, J., Daza, P., Boianova, L., and Romero, F. (2007). Antifungal activity of strawberry fruit volatile compounds against Colletotrichum acutatum. J. Agric. Food Chem. 55, 5701–5707. doi: 10.1021/jf0703957
Ashour, M., Wink, M., and Gershenzon, J. (2010). “Biochemistry of terpenoids: monoterpenes, sesquiterpenes and diterpenes,” in Annual Plant Reviews: Biochemistry of Plant Secondary Metabolism, ed. M. Wink (New York, NY: Wiley).
Aslam, M. Z., Lin, X., Li, X., Yang, N., and Chen, L. (2020). Molecular cloning and functional characterization of CpMYC2 and CpBHLH13 transcription factors from wintersweet (Chimonanthus praecox L.). Plants 9:785. doi: 10.3390/plants9060785
Aubert, C., and Chalot, G. (2017). Chemical composition, bioactive compounds, and volatiles of six table grape varieties (Vitis vinifera L.). Food Chem. 240, 524–533. doi: 10.1016/j.foodchem.2017.07.152
Aubert, C., and Milhet, C. (2007). Distribution of the volatile compounds in the different parts of a white-fleshed peach (Prunus persica L. Batsch). Food Chem. 102, 375–384. doi: 10.1016/j.foodchem.2006.05.030
Aurore, G., Ginies, C., Ganou-Parfait, B., Renard, C. M., and Fahrasmane, L. (2011). Comparative study of free and glycoconjugated volatile compounds of three banana cultivars from French West Indies: Cavendish, Frayssinette and Plantain. Food Chem. 129, 28–34. doi: 10.1016/j.foodchem.2011.01.104
Ayedoun, A. M., Sossou, P. V., Mardarowicz, M., and Leclercq, P. A. (1996). Volatile constituents of the peel and leaf oils of Citrus limon L. Burm. f. from Benin. J. Essent. Oil Res. 8, 441–444. doi: 10.1080/10412905.1996.9700661
Azadi, B., and Entezari, M. (2016). Floral scent composition of Dianthus elymaiticus Hausskn. and Bornm. ex Bornm. J. Essent. Oil Bear. Plants 19, 1830–1833. doi: 10.1080/0972060X.2014.962622
Azulay, Y., Ibdah, M., Meir, A., Yosef, E., and Zamir, D. (2005). Not just colors — carotenoid degradation as a link between pigmentation and aroma in tomato and watermelon fruit. Trends Food Sci. Tech. 16, 407–415. doi: 10.1016/j.tifs.2005.04.004
Bai, C., Wu, C., Ma, L., Fu, A., Zheng, Y., Han, J., et al. (2022). Transcriptomics and metabolomics analyses provide insights into postharvest ripening and senescence of tomato fruit under low temperature. Hortic. Plant J. 8. doi: 10.1016/j.hpj.2021.09.001
Baietto, M., and Wilson, A. D. (2015). Electronic-nose applications for fruit identification, ripeness and quality grading. Sensors 15, 899–931. doi: 10.3390/s150100899
Baldermann, S., Kato, M., Kurosawa, M., Kurobayashi, Y., Fujita, A., Fleischmann, P., et al. (2010). Functional characterization of a carotenoid cleavage dioxygenase 1 and its relation to the carotenoid accumulation and volatile emission during the floral development of Osmanthus fragrans Lour. J. Exp. Bot. 61, 2967–2977. doi: 10.1093/jxb/erq123
Baldwin, E. A., Scott, J. W., Shewmaker, C. K., and Schuch, W. (2000). Flavor trivia and tomato aroma: biochemistry and possible mechanisms for control of important aroma components. Hortscience 35, 1013–1021. doi: 10.21273/HORTSCI.35.6.1013
Bambalele, N. L., Mditshwa, A., Magwaza, L. S., and Tesfay, S. Z. (2021). Recent advances on postharvest technologies of mango fruit: a review. Int. J. Fruit Sci. 21, 565–586. doi: 10.1080/15538362.2021.1918605
Barretto, L. C., de, O., Moreirade, J., de, J., da, S., dos Santos, J. A. B., et al. (2013). Characterization and extraction of volatile compounds from pineapple (Ananas comosus L. Merril) processing residues. Food Sci. Tech. 33, 638–645. doi: 10.1590/S0101-20612013000400007
Bate, N. J., Orr, J., Ni, W., Meromi, A., Nadler-Hassar, T., Doerner, P. W., et al. (1994). Quantitative relationship between phenylalanine ammonia-lyase levels and phenylpropanoid accumulation in transgenic tobacco identifies a rate-determining step in natural product synthesis. P. Natl. Acad. Sci. U.S.A. 91, 7608–7612. doi: 10.1073/pnas.91.16.7608
Baysal, T., and Demirdöven, A. (2007). Lipoxygenase in fruits and vegetables: a review. Enzyme Microb. Tech. 40, 491–496. doi: 10.1016/j.enzmictec.2006.11.025
Bera, P., Chakrabarti, S., Gaikwad, N. K., Kutty, N. N., Barman, M., and Mitra, A. (2018). Developmental variation in floral volatiles composition of a fragrant orchid Zygopetalum maculatum (Kunth) Garay. Nat. Prod. Res. 33, 435–438. doi: 10.1080/14786419.2018.1451999
Bera, P., Kotamreddy, J. N. R., Samanta, T., Maiti, S., and Mitra, A. (2015). Inter-specific variation in headspace scent volatiles composition of four commercially cultivated jasmine flowers. Nat. Prod. Res. 29, 1328–1335. doi: 10.1080/14786419.2014.1000319
Bergaoui, A., Boughalleb, N., Jannet, H. B., Harzallah-Shiric, F., El Mahjoub, M., and Mighri, Z. (2007). Chemical composition and antifungal activity of volatiles from three Opuntia species growing in Tunisia. Pak. J. Biol. Sci. 10, 2485–2489. doi: 10.3923/pjbs.2007.2485.2489
Bernhardt, P., Meier, R., and Vance, N. (2013). Pollination ecology and floral function of brown’s Peony (Paeonia Brownii) in the blue mountains of northeastern oregon. J. Pollinat. Ecol. 2, 9–20. doi: 10.26786/1920-760320132
Birtic, S., Ginies, C., Causse, M., Renard, C. M., and Page, D. (2009). Changes in volatiles and glycosides during fruit maturation of two contrasted tomato (Solanum lycopersicum) lines. J. Agric. Food Chem. 57, 591–598. doi: 10.1021/jf8023062
Blanch, G. P., Flores, G., and del Castillo, M. L. R. (2011). Influence of methyl jasmonate in conjunction with ethanol on the formation of volatile compounds in berries belonging to the Rosaceae. Postharvest Biol. Tec. 62, 168–178. doi: 10.1016/j.postharvbio.2011.05.003
Boatright, J., Negre, F., Chen, X., Kish, C. M., Wood, B., Peel, G., et al. (2004). Understanding in vivo benzenoid metabolism in petunia petal tissue. Plant Physiol. 135, 1993–2011. doi: 10.1104/pp.104.045468
Boukhris, M., Hadrich, F., Chtourou, H., Dhouib, A., Bouaziz, M., and Sayadi, S. (2015). Chemical composition, biological activities and DNA damage protective effect of Pelargonium graveolens L’Hér. essential oils at different phenological stages. Ind. Crop Prod. 74, 600–606. doi: 10.1016/j.indcrop.2015.05.051
Brandt, K., Machado, I. C., do Amaral Ferraz Navarro, D. M., Dötterl, S., Ayasse, M., and Milet-Pinheiro, P. (2020). Sexual dimorphism in floral scents of the neotropical orchid Catasetum arietinum and its possible ecological and evolutionary significance. AoB Plants 12:laa030. doi: 10.1093/AOBPLA/PLAA030
Burger, H., Joos, N., and Ayasse, M. (2021). Floral cues of non-host plants attract oligolectic Chelostoma rapunculi bees. Front. Ecol. Evol. 9:682960. doi: 10.3389/fevo.2021.682960
Campbell, D. R., Sosenski, P., and Raguso, R. A. (2019). Phenotypic plasticity of floral volatiles in response to increasing drought stress. Ann. Bot. 123, 601–610. doi: 10.1093/aob/mcy193
Caruso, C. M., and Parachnowitsch, A. L. (2016). Do plants eavesdrop on floral scent signals? Trends Plant Sci. 21, 9–15. doi: 10.1016/j.tplants.2015.09.001
Casiglia, S., Bruno, M., and Senatore, F. (2014). Volatile constituents of Dianthus rupicola Biv. from sicily: activity against microorganisms affecting cellulosic objects. Nat. Prod. Res. 28, 1739–1746. doi: 10.1080/14786419.2014.945087
Chen, F., and Tholl, D. (2011). The family of terpene synthases in plants?: a mid-size family of genes for specialized metabolism that is highly diversified throughout the kingdom. Plant J. 66, 212–229. doi: 10.1111/j.1365-313X.2011.04520.x
Chen, G., Mostafa, S., Lu, Z., Du, R., Cui, J., Wang, Y., et al. (2020). The jasmine (Jasminum sambac) genome and flower fragrances. bioRxiv [Preprint]. doi: 10.1101/2020.12.17.420646
Chen, H. C., Chi, H. S., and Lin, L. Y. (2013). Headspace solid-phase microextraction analysis of volatile components in Narcissus tazetta var. chinensis Roem. Molecules 18, 13723–13734. doi: 10.3390/molecules181113723
Chen, M., Zhu, Y., Liu, B., Chen, Z., Zheng, J., Guan, M., et al. (2017). Changes in the volatiles, chemical components, and antioxidant activities of Chinese jasmine tea during the scenting processes. Int. J. Food Prop. 20, 681–693. doi: 10.1080/10942912.2016.1177542
Chen, M. X., Chen, X. S., Wang, X. G., Ci, Z. J., Liu, X. L., He, T. M., et al. (2006). Comparison of headspace solid-phase microextraction with simultaneous steam distillation extraction for the analysis of the volatile constituents in Chinese apricot. Agric. Sci. China 5, 879–884. doi: 10.1016/S1671-2927(06)60139-9
Cheng, S., Fu, X., Mei, X., Zhou, Y., Du, B., Watanabe, N., et al. (2016). Regulation of biosynthesis and emission of volatile phenylpropanoids/benzenoids in Petunia hybrida flowers by multi-factors of circadian clock, light, and temperature. Plant Physiol. Bioch. 107, 1–8. doi: 10.1016/j.plaphy.2016.05.026
Chin, S. T., Nazimah, S. A. H., Quek, S. Y., Che Man, Y. B., Abdul Rahman, R., and Mat Hashim, D. (2008). Changes of volatiles’ attribute in durian pulp during freeze- and spray-drying process. LWT Food Sci. Technol. 41, 1899–1905. doi: 10.1016/j.lwt.2008.01.014
Cho, M. H., Corea, O. R. A., Yang, H., Bedgar, D. L., Laskar, D. D., Anterola, A. M., et al. (2007). Phenylalanine biosynthesis in Arabidopsis thaliana: identification and characterization of arogenate dehydratases. J. Biol. Chem. 282, 30827–30835. doi: 10.1074/jbc.M702662200
Chuang, Y. C., Lee, M. C., Chang, Y. L., Chen, W. H., and Chen, H. H. (2017). Diurnal regulation of the floral scent emission by light and circadian rhythm in the Phalaenopsis orchids. Bot. Stud. 58:50. doi: 10.1186/s40529-017-0204-8
Singh, Z., Lalel, H. J. D., and Nair, S. (2004). A review of mango fruit aroma volatile compounds – state of the art research zora. Acta Hortic. 645, 519–527. doi: 10.17660/ActaHortic.2004.645.68
Colquhoun, T. A., Kim, J. Y., Wedde, A. E., Levin, L. A., Schmitt, K. C., Schuurink, R. C., et al. (2011a). PhMYB4 fine-tunes the floral volatile signature of Petunia hybrida through PhC4H. J. Exp. Bot. 62, 1133–1143. doi: 10.1093/jxb/erq342
Colquhoun, T. A., Schwieterman, M. L., Wedde, A. E., Schimme, B. C. J., Marciniak, D. M., Verdonk, J. C., et al. (2011b). EOBII controls flower opening by functioning as a general transcriptomic switch. Plant Physiol. 156, 974–984. doi: 10.1104/pp.111.176248
Connelly, J. A., and Conn, E. E. (1986). Tyrosine biosynthesis in Sorghum bicolor: isolation and regulatory properties of arogenate dehydrogenase. Z. Naturforsch. C J. Biosci. 41, 69–78. doi: 10.1515/znc-1986-1-212
Cordeiro, G. D., Fernandes dos Santos, I. G., Silva, C. I., da, Schlindwein, C., Alves-dos-Santos, I., et al. (2019). Nocturnal floral scent profiles of myrtaceae fruit crops. Phytochemistry 162, 193–198. doi: 10.1016/j.phytochem.2019.03.011
Crops, V., Yishay, R., and Crops, V. (2005). Carotenoid pigmentation affects the volatile composition of tomato and watermelon fruits, as revealed by comparative genetic analyses. J. Agric. Food Chem. 53, 3142–3148. doi: 10.1021/jf047927t
Croteau, R., Kutchan, T. M., and Lewis, N. G. (2000). “Natural products (secondary metabolites),” in Biochemistry and Molecular Biology of Plants, eds B. Buchanan, W. Gruissem, and R. Jones (Rockville, MD: American Society of Plant Physiologists), 1250–1319. doi: 10.1128/mSystems.00186-17
De, L. C., Rao, A. N., Rajeevan, P. K., Promila, P., and Singh, D. R. (2019). Medicinal and aromatic orchids -an overview. Int. J. Curr. Res. 7, 19931–19935.
Del-Castillo-Alonso, M. Á, Monforte, L., Tomás-Las-Heras, R., Ranieri, A., Castagna, A., Martínez-Abaigar, J., et al. (2021). Secondary metabolites and related genes in Vitis vinifera L. cv. Tempranillo grapes as influenced by ultraviolet radiation and berry development. Physiol. Plant. 173, 709–724. doi: 10.1111/ppl.13483
Demir, N., Yildiz, O., Alpaslan, M., and Hayaloglu, A. A. (2014). Evaluation of volatiles, phenolic compounds and antioxidant activities of rose hip (Rosa L.) fruits in Turkey. LWT Food Sci. Technol. 57, 126–133. doi: 10.1016/j.lwt.2013.12.038
Deng, Y., and Lu, S. (2017). Biosynthesis and regulation of phenylpropanoids in plants. Crit. Rev. Plant Sci. 36, 257–290. doi: 10.1080/07352689.2017.1402852
Dexter, R., Underwood, B., and Clark, D. (2007). “Ethylene-regulated floral volatile synthesis in Petunia hybrida,” in Advances in Plant Ethylene Research, eds A. Ramina, C. Chang, J. Giovannoni, H. Klee, P. Perata, and E. Woltering (Dordrecht: Springer), 141–146.
Ding, W., Ouyang, Q., Li, Y., Shi, T., Li, L., Yang, X., et al. (2020). Genome-wide investigation of WRKY transcription factors in sweet osmanthus and their potential regulation of aroma synthesis. Tree Physiol. 40, 557–572. doi: 10.1093/treephys/tpz129
Dong, N. Q., and Lin, H. X. (2021). Contribution of phenylpropanoid metabolism to plant development and plant–environment interactions. J. Integr. Plant Biol. 63, 180–209. doi: 10.1111/jipb.13054
Dötterl, S., Wolfe, L. M., and Jürgens, A. (2005). Qualitative and quantitative analyses of flower scent in Silene latifolia. Phytochemistry 66, 203–213. doi: 10.1016/j.phytochem.2004.12.002
Du, F., Wang, T., Fan, J. M., Liu, Z. Z., Zong, J. X., Fan, W. X., et al. (2019). Volatile composition and classification of lilium flower aroma types and identification, polymorphisms, and alternative splicing of their monoterpene synthase genes. Hortic. Res. 6:110. doi: 10.1038/s41438-019-0192-9
Duan, W., Peng, L., Zhang, H., Han, L., and Li, Y. (2021). Microbial biofertilizers increase fruit aroma content of Fragaria ananassa by improving photosynthetic efficiency. Alex. Eng. J. 60, 5323–5330. doi: 10.1016/j.aej.2021.04.014
Dudareva, N., Klempien, A., Muhlemann, J. K., and Kaplan, I. (2013). Biosynthesis, function and metabolic engineering of plant volatile organic compounds. New Phytol. 198, 16–32. doi: 10.1111/nph.12145
Dudareva, N., Martin, D., Kish, C. M., Kolosova, N., Gorenstein, N., Fäldt, J., et al. (2003). (£)-β-ocimene and myrcene synthase genes of floral scent biosynthesis in snapdragon: function and expression of three terpene synthase genes of a new terpene synthase subfamily. Plant Cell 15, 1227–1241. doi: 10.1105/tpc.011015.2
Dudareva, N., Negre, F., Nagegowda, D. A., and Orlova, I. (2006). Plant volatiles: recent advances and future perspectives. Crit. Rev. Plant Sci. 25, 417–440. doi: 10.1080/07352680600899973
Dudareva, N., and Pichersky, E. (2000). Biochemical and molecular genetic aspects of floral scents. Plant Physiol 122, 627–633. doi: 10.1104/pp.122.3.627
Dunemann, F., Ulrich, D., Boudichevskaia, A., Grafe, C., and Weber, W. E. (2009). QTL mapping of aroma compounds analysed by headspace solid-phase microextraction gas chromatography in the apple progeny “Discovery” × “Prima.”. Mol. Breed. 23, 501–521. doi: 10.1007/s11032-008-9252-9
Eduardo, I., Chietera, G., Bassi, D., Rossini, L., and Vecchietti, A. (2010). Identification of key odor volatile compounds in the essential oil of nine peach accessions. J. Sci. Food Agric. 90, 1146–1154. doi: 10.1002/jsfa.3932
Effmert, U., Große, J., Röse, U. S. R., Ehrig, F., Kägi, R., and Piechulla, B. (2005). Volatile composition, emission pattern, and localization of floral scent emission in Mirabilis jalapa (Nyctaginaceae). Am. J. Bot. 92, 2–12. doi: 10.3732/ajb.92.1.2
Eilers, E. J., Kleine, S., Eckert, S., Waldherr, S., and Müller, C. (2021). Flower production, headspace volatiles, pollen nutrients, and florivory in Tanacetum vulgare chemotypes. Front. Plant Sci. 11:611877. doi: 10.3389/fpls.2020.611877
Elss, S., Preston, C., Hertzig, C., Heckel, F., Richling, E., and Schreier, P. (2005). Aroma profiles of pineapple fruit (Ananas comosus [L.] Merr.) and pineapple products. LWT Food Sci. Technol. 38, 263–274. doi: 10.1016/j.lwt.2004.07.014
Elzebroek, A. T. G., and Wind, K. (2008). “Edible fruits and nuts,” in Guide to Cultivated Plants, ed. A. T. G. Elzebroek (Wallingford: CAB International), 27. doi: 10.1038/s41597-019-0350-9
Erogul, D., and Özsoydan, I. (2020). Effect of pre-harvest salicylic acid treatments on the quality and shelf life of the “Cresthaven” peach cultivar. Folia Hortic. 32, 221–227. doi: 10.2478/fhort-2020-0020
Espino-Díaz, M., Sepúlveda, D. R., González-Aguilar, G., and Olivas, G. (2016). Biochemistry of apple aroma: a review. Food Technol. Biotech. 54, 375–394. doi: 10.17113/ftb.54.04.16.4248
Essien, E. E., Newby, J. S., Walker, T. M., and Setzer, W. N. (2015). Characterization and antimicrobial activity of volatile constituents from fresh fruits of Alchornea cordifolia and Canthium subcordatum. Medicines 3:1. doi: 10.3390/medicines3010001
Fan, R., Chen, Y., Ye, X., Wu, J., Lin, B., and Zhong, H. (2018). Transcriptome analysis of Polianthes tuberosa during floral scent formation. PLoS One 13:e0199261. doi: 10.1371/journal.pone.0199261
Fang, Y., and Wakisaka, M. (2021). A review on the modified atmosphere preservation of fruits and vegetables with cutting-edge technologies. Agriculture 11:992. doi: 10.3390/agriculture11100992
Farré-Armengol, G., Filella, I., Llusia, J., and Peñuelas, J. (2013). Floral volatile organic compounds: between attraction and deterrence of visitors under global change. Perspect. Plant Ecol. 15, 56–67. doi: 10.1016/j.ppees.2012.12.002
Farré-Armengol, G., Filella, I., Llusià, J., and Peñuelas, J. (2017). β-Ocimene, a key floral and foliar volatile involved in multiple interactions between plants and other organisms. Molecules 22:1148. doi: 10.3390/molecules22071148
Fenske, M. P., and Imaizumi, T. (2016). Circadian rhythms in floral scent emission. Front. Plant Sci. 7:462. doi: 10.3389/fpls.2016.00462
Ferrer, J., Austin, C. S. Jr., and Noel, J. P. (2008). Structure and function of enzymes involved in the biosynthesis of phenylpropanoids. Plant Physiol. Biochem. 46, 356–370. doi: 10.1016/j.plaphy.2007.12.009
Freudenstein, J. V., and Chase, M. W. (2015). Phylogenetic relationships in Epidendroideae (Orchidaceae), one of the great flowering plant radiations: progressive specialization and diversification. Ann. Bot. 115, 665–681. doi: 10.1093/aob/mcu253
Fu, J., Hou, D., Zhang, C., Bao, Z., Zhao, H., and Hu, S. (2017). The emission of the floral scent of four Osmanthus fragrans cultivars in response to different temperatures. Molecules 22:430. doi: 10.3390/molecules22030430
Gaines, C. G., Byng, G. S., Whitaker, R. J., and Jensen, R. A. (1982). L-tyrosine regulation and biosynthesis via arogenate dehydrogenase in suspension-cultured cells of Nicotiana silvestris Speg. et Comes. Planta 156, 233–240. doi: 10.1007/BF00393730
Gang, D. R., Lavid, N., Zubieta, C., Chen, F., Beuerle, T., Lewinsohn, E., et al. (2002). Characterization of phenylpropene O-methyltransferases from sweet basil: facile change of substrate specificity and convergent evolution within a plant O-methyltransferase family. Plant Cell 14, 505–519. doi: 10.1105/tpc.010327
Garcia, C. V., Stevenson, R. J., Atkinson, R. G., Winz, R. A., and Quek, S.-Y. (2013). Changes in the bound aroma profiles of “Hayward”and “Hort16A” kiwifruit (Actinidia spp.) during ripening and GC-olfactometry analysis. Food Chem. 137, 45–54. doi: 10.1016/j.foodchem.2012.10.002
García-Rojas, M., Morgan, A., Gudenschwager, O., Zamudio, S., Campos-Vargas, R., González-Agüero, M., et al. (2016). Biosynthesis of fatty acids-derived volatiles in “Hass” avocado is modulated by ethylene and storage conditions during ripening. Sci. Hortic. Amst. 202, 91–98. doi: 10.1016/j.scienta.2016.02.024
Gershenzon, J., and Dudareva, N. (2007). The function of terpene natural products in the natural world. Nat. Chem. Biol. 3, 408–414. doi: 10.1038/nchembio.2007.5
Gershenzon, J., and Kreis, W. (1999). “Biochemistry of terpenoids: Monoterpenes, sesquiterpenes, diterpenes, sterols, cardiac glycosides and steroid saponins,” in Annual Plant Reviews: Biochemistry of Plant Secondary Metabolism, ed. M. Wink (Sheffield: Sheffield Academic Press), 222–299.
Ghavam, M., Manca, M. L., Manconi, M., and Bacchetta, G. (2020). Chemical composition and antimicrobial activity of essential oils obtained from leaves and flowers of Salvia hydrangea DC. ex Benth. Sci. Rep. 10:15647. doi: 10.1038/s41598-020-73193-y
Gokbulut, I., and Karabulut, I. (2012). SPME-GC-MS detection of volatile compounds in apricot varieties. Food Chem. 132, 1098–1102. doi: 10.1016/j.foodchem.2011.11.080
Gonda, I., Bar, E., Portnoy, V., Lev, S., Burger, J., Schaffer, A. A., et al. (2010). Branched-chain and aromatic amino acid catabolism into aroma volatiles in Cucumis melo L. fruit. J. Exp. Bot. 61, 1111–1123. doi: 10.1093/jxb/erp390
Gross, K., Sun, M., and Schiestl, F. P. (2016). Why do floral perfumes become different? Region-specific selection on floral scent in a terrestrial orchid. PLoS One 11:e0147975. doi: 10.1371/journal.pone.0147975
Guillot, S., Peytavi, L., Bureau, S., Boulanger, R., Lepoutre, J. P., Crouzet, J., et al. (2006). Aroma characterization of various apricot varieties using headspace-solid phase microextraction combined with gas chromatography-mass spectrometry and gas chromatography-olfactometry. Food Chem. 96, 147–155. doi: 10.1016/j.foodchem.2005.04.016
Guo, X., and Wang, P. (2020). Aroma characteristics of lavender extract and essential oil from Lavandula angustifolia mill. Molecules 25:5541. doi: 10.3390/molecules25235541
Guo, X., Yu, C., Luo, L., Wan, H., Li, Y., Wang, J., et al. (2017). Comparative transcriptome analysis of the floral transition in Rosa chinensis “Old Blush” and R. odorata var. gigantea. Sci. Rep. 7:6068. doi: 10.1038/s41598-017-05850-8
Han, Y., Wang, H., Wang, X., Li, K., Dong, M., Li, Y., et al. (2019). Mechanism of floral scent production in Osmanthus fragrans and the production and regulation of its key floral constituents, β-ionone and linalool. Hortic. Res. 6:106. doi: 10.1038/s41438-019-0189-4
He, Y., Xu, M., and Chen, X. (2020). De novo transcriptomics analysis of the floral scent of Chinese narcissus. Trop. Plant Biol. 13, 172–188. doi: 10.1007/s12042-020-09253-4
Heath, H. B., and Reineccius, G. (1986). Flavor Chemistry and Technology. Westport, Conn.: AVI Publishing Company Westport.
Heil, M., and Karban, R. (2010). Explaining evolution of plant communication by airborne signals. Trends Ecol. Evol. 25, 137–144. doi: 10.1016/j.tree.2009.09.010
Herrera, C., Castro, R., García-Barroso, C., and Durán-Guerrero, E. (2016). Development of a stir bar sorptive extraction method for the determination of volatile compounds in orange juices. J. Sep. Sci. 39, 3586–3593. doi: 10.1002/jssc.201600590
Herrero, M., García, L. A., and Díaz, M. (2006). Volatile compounds in cider: inoculation time and fermentation temperature effects. J. I. Brewing 112, 210–214. doi: 10.1002/j.2050-0416.2006.tb00715.x
Hodgkison, R., Ayasse, M., Kalko, E. K. V., Häberlein, C., Schulz, S., Mustapha, W. A. W., et al. (2007). Chemical ecology of fruit bat foraging behavior in relation to the fruit odors of two species of paleotropical bat-dispersed figs (Ficus hispida and Ficus scortechinii). J. Chem. Ecol. 33, 2097–2110. doi: 10.1007/s10886-007-9367-1
Högnadóttir, Á, and Rouseff, R. L. (2003). Identification of aroma active compounds in orange essence oil using gas chromatography-olfactometry and gas chromatography-mass spectrometry. J. Chromatogr. A 998, 201–211. doi: 10.1016/S0021-9673(03)00524-7
Hong, G. J., Xue, X. Y., Mao, Y. B., Wang, L. J., and Chen, X. Y. (2012). Arabidopsis MYC2 interacts with DELLA proteins in regulating sesquiterpene synthase gene expression. Plant Cell 24, 2635–2648. doi: 10.1105/tpc.112.098749
Hong, J. K., Yang, H. J., Jung, H., Yoon, D. J., Sang, M. K., and Jeun, Y.-C. (2015). Application of volatile antifungal plant essential oils for controlling pepper fruit anthracnose by Colletotrichum gloeosporioides. Plant Pathol. J. 31, 269–277. doi: 10.5423/PPJ.OA.03.2015.0027
Hu, Z., Tang, B., Wu, Q., Zheng, J., Leng, P., and Zhang, K. (2017). Transcriptome sequencing analysis reveals a difference in monoterpene biosynthesis between scented Lilium “Siberia” and unscented Lilium “Novano.”. Front. Plant Sci. 8:1351. doi: 10.3389/fpls.2017.01351
Huang, L., Ho, C., and Wang, Y. (2020). Biosynthetic pathways and metabolic engineering of spice flavors. Crit. Rev. Food Sci. 61, 2047–2060. doi: 10.1080/10408398.2020.1769547
Huang, M., Sanchez-Moreiras, A. M., Abel, C., Sohrabi, R., Lee, S., Gershenzon, J., et al. (2012). The major volatile organic compound emitted from Arabidopsis thaliana flowers, the sesquiterpene (E)-β-caryophyllene, is a defense against a bacterial pathogen. New Phytol. 193, 997–1008. doi: 10.1111/j.1469-8137.2011.04001.x
Ito, Y., Sugimoto, A., Kakuda, T., and Kubota, K. (2002). Identification of potent odorants in Chinese jasmine green tea scented with flowers of Jasminum sambac. J. Agric. Food Chem. 50, 4878–4884. doi: 10.1021/jf020282h
Jerković, I., Kuś, P. M., and Carbonell-Barrachina, ÁA. (2019). Volatile organic compounds as artefacts derived from natural phytochemicals sourced form plants and honey. Phytochem. Rev. 18, 871–891. doi: 10.1007/s11101-019-09621-3
Jin, J., Zhang, S., Zhao, M., Jing, T., Zhang, N., Wang, J., et al. (2020). Scenarios of genes-to-terpenoids network led to the identification of a novel α/β-farnesene/β-ocimene synthase in Camellia sinensis. Int. J. Mol. Sci. 21:655. doi: 10.3390/ijms21020655
Jing, G., Li, T., Qu, H., Yun, Z., Jia, Y., Zheng, X., et al. (2015). Postharvest biology and technology carotenoids and volatile profiles of yellow and red fleshed papaya fruit in relation to the expression of carotenoid cleavage dioxygenase genes. Postharvest Biol. Tech. 108, 114–119. doi: 10.1016/j.postharvbio.2015.06.006
Julsrigival, J., Songsak, T., Kirdmanee, C., and Chansakaow, S. (2013). Determination of volatile constituents of thai fragrant orchids by gas chromatography-mass spectrometry with solid-phase microextraction. J. Nat. Sci. 12, 43–57. doi: 10.12982/CMUJNS.2013.0005
Junker, R. R., Loewel, C., Gross, R., Dötterl, S., Keller, A., and Blüthgen, N. (2011). Composition of epiphytic bacterial communities differs on petals and leaves. Plant Biol. 13, 918–924. doi: 10.1111/j.1438-8677.2011.00454.x
Kalske, A., Shiojiri, K., Uesugi, A., Sakata, Y., Morrell, K., and Kessler, A. (2019). Insect herbivory selects for volatile-mediated plant-plant communication. Curr. Biol. 29, 3128–3133. doi: 10.1016/j.cub.2019.08.011
Kalua, C. M., and Boss, P. K. (2009). Evolution of volatile compounds during the development of cabernet sauvignon grapes (Vitis vinifera L.). J. Agric. Food Chem. 57, 3818–3830. doi: 10.1021/jf803471n
Kambiranda, D., Basha, S. M., Singh, R. K., He, H., Calvin, K., and Mercer, R. (2016). In depth proteome analysis of ripening muscadine grape berry cv. carlos reveals proteins associated with flavor and aroma compounds. J. Proteome Res. 15, 2910–2923. doi: 10.1021/acs.jproteome.5b01064
Kaminaga, Y., Schnepp, J., Peel, G., Kish, C. M., Ben-Nissan, G., Weiss, D., et al. (2006). Plant phenylacetaldehyde synthase is a bifunctional homotetrameric enzyme that catalyzes phenylalanine decarboxylation and oxidation. J. Biol. Chem. 281, 23357–23366. doi: 10.1074/jbc.M602708200
Karabulut, I., Gokbulut, I., Bilenler, T., and Sislioglu, K. (2018). Effect of fruit maturity level on quality, sensory properties and volatile composition of two common apricot (Prunus armeniaca L.) varieties. J Food Sci. Tech. 55, 2671–2678. doi: 10.1007/s13197-018-3189-8
Kaur, K., Awasthi, P., and Id, S. T. (2021). Comparative transcriptome analysis of unripe and ripe banana (cv. Nendran) unraveling genes involved in ripening and other related processes. PLoS One 16:e0254709. doi: 10.1371/journal.pone.0254709
Kegge, W., and Pierik, R. (2010). Biogenic volatile organic compounds and plant competition. Trends Plant Sci. 15, 126–132. doi: 10.1016/j.tplants.2009.11.007
Kelebek, H., and Selli, S. (2011). Determination of volatile, phenolic, organic acid and sugar components in a Turkish cv. Dortyol (Citrus sinensis L. Osbeck) orange juice. J. Sci. Food Agric. 91, 1855–1862. doi: 10.1002/jsfa.4396
Kessler, D., Gase, K., and Baldwin, I. T. (2008). Field experiments with transformed plants reveal the sense of floral scents. Science 321, 1200–1202. doi: 10.1126/science.1160072
Khuraijam, J. S., Sharma, S. C., and Roy, R. K. (2017). Orchids: potential ornamental crop in north India. J. Essent. Oil Bear. Plants 7, 1–8.
Kim, J. Y., Swanson, R. T., Alvarez, M. I., Johnson, T. S., Cho, K. H., Clark, D. G., et al. (2019). Down regulation of p-coumarate 3-hydroxylase in petunia uniquely alters the profile of emitted floral volatiles. Sci. Rep. 9:8852. doi: 10.1038/s41598-019-45183-2
Kiralan, M. (2015). Use of headspace solid-phase microextraction in rose (Rosa damescena Mill) products for volatile compounds. J. Essent. Oil Bear. Plants 18, 1266–1270. doi: 10.1080/0972060X.2015.1044571
Kishimoto, K. (2020). “The characteristics of flower scents in carnations,” in The Carnation Genome. Compendium of Plant Genomes, eds T. Onozaki and M. Yagi (Singapore: Springer), 147–157. doi: 10.1007/978-981-15-8261-5_11
Klee, H. J. (2010). Improving the flavor of fresh fruits: genomics, biochemistry, and biotechnology. New Phytol. 187, 44–56. doi: 10.1111/j.1469-8137.2010.03281.x
Knudsen, J. T. (1994). Floral scent variation in the Pyrola rotundifolia complex in Scandinavia and Western Greenland. Nord. J. Bot. 14, 277–282. doi: 10.1111/j.1756-1051.1994.tb00599.x
Knudsen, J. T., and Gershenzon, J. (2006). “The chemical diversity of floral scent,” in Biology of Floral Scent, eds N. Dudareva and E. Pichersky (Boca Raton, FL: CRC Press), 27–52.
Kochevenko, A., Araújo, W. L., Maloney, G. S., Tieman, D. M., Do, P. T., Taylor, M. G., et al. (2012). Catabolism of branched chain amino acids supports respiration but not volatile synthesis in tomato fruits. Mol. Plant 5, 366–375. doi: 10.1093/mp/ssr108
Koksal, N., Saribas, R., Kafkas, E., Aslancan, H., and Sadighazadi, S. (2015). Determination of volatile compounds of the first rose oil and the first rose water by HS-SPME/GC/MS techniques. Afr. J. Tradit. Complem. 12, 145–150. doi: 10.4314/ajtcam.v12i4.21
Kondo, S., Setha, S., Rudell, D., Buchanan, D., and Mattheis, J. (2005). Aroma volatile biosynthesis in apples affected by 1-MCP and methyl jasmonate. Postharvest Biol. Tech. 36, 61–68. doi: 10.1016/j.postharvbio.2004.11.005
Kong, Y., Bai, J., Lang, L., Bao, F., Dou, X., Wang, H., et al. (2017). Variation in floral scent compositions of different lily hybrid groups. J. Am. Soc. Hortic. Sci. 142, 175–183. doi: 10.21273/JASHS03934-16
Kongor, J. E., Hinneh, M., de Walle, D., Van Afoakwa, E. O., Boeckx, P., and Dewettinck, K. (2016). Factors influencing quality variation in cocoa (Theobroma cacao) bean flavour profile - a review. Food Res. Int. 82, 44–52. doi: 10.1016/j.foodres.2016.01.012
Kunze, J. (2001). The combined effect of color and odor on flower choice behavior of bumble bees in flower mimicry systems. Behav. Ecol. 12, 447–456. doi: 10.1093/beheco/12.4.447
Lackus, N. D., Schmidt, A., Gershenzon, J., and Köllner, T. G. (2021). A peroxisomal b-oxidative pathway contributes to the formation of C6–C1 aromatic volatiles in poplar. Plant Physiol. 186, 891–909. doi: 10.1093/PLPHYS/KIAB111
Lalel, H. J. D., Singh, Z., and Tan, S. C. (2003a). Glycosidically-bound aroma volatile compounds in the skin and pulp of “Kensington Pride”mango fruit at different stages of maturity. Postharvest Biol. Tech. 29, 205–218. doi: 10.1016/S0925-5214(02)00250-8
Lalel, H. J. D., Singh, Z., and Tan, S. C. (2003b). Elevated levels of CO2 in controlled atmosphere storage affects shelf life, fruit quality and aroma volatiles of mango. Acta Hortic. 628, 407–413. doi: 10.17660/actahortic.2003.628.51
Lee, B., Lin, P. C., Cha, H. S., Luo, J., and Chen, F. (2016). Characterization of volatile compounds in Cowart muscadine grape (Vitis rotundifolia) during ripening stages using GC-MS combined with principal component analysis. Food Sci. Biotechnol. 25, 1319–1326. doi: 10.1007/s10068-016-0207-3
Li, H., Li, J., Dong, Y., Hao, H., Ling, Z., Bai, H., et al. (2019). Time-series transcriptome provides insights into the gene regulation network involved in the volatile terpenoid metabolism during the flower development of lavender. BMC Plant Biol. 19:313. doi: 10.1186/s12870-019-1908-6
Li, J., Chen, S., Zhu, L., and Last, R. L. (1995). Isolation of cDNAs encoding the tryptophan pathway enzyme indole-3-glycerol phosphate synthase from Arabidopsis thaliana. Plant Physiol. 108, 877–878. doi: 10.1104/pp.108.2.877
Li, X., He, L., An, X., Yu, K., Meng, N., and Duan, C. (2020). VviWRKY40, a WRKY transcription factor, regulates glycosylated monoterpenoid production by VviGT14 in grape berry. Genes 11:485. doi: 10.3390/genes11050485
Li, X., Tang, D., and Shi, Y. (2018). Volatile compounds in perianth and corona of Narcissus pseudonarcissus cultivars. Nat. Prod. Res. 33, 2281–2284. doi: 10.1080/14786419.2018.1499632
Li, X., Xu, Y., Shen, S., Yin, X., Klee, H., Zhang, B., et al. (2017). Transcription factor CitERF71 activates the terpene synthase gene CitTPS16 involved in the synthesis of e-geraniol in sweet orange fruit. J. Exp. Bot. 68, 4929–4938. doi: 10.1093/jxb/erx316
Liao, P., Ray, S., Boachon, B., Lynch, J. H., Deshpande, A., McAdam, S., et al. (2021). Cuticle thickness affects dynamics of volatile emission from petunia flowers. Nat. Chem. Biol. 17, 138–145. doi: 10.1038/s41589-020-00670-w
Lichtenthaler, H. K., Schwender, J., Disch, A., and Rohmer, M. (1997). Biosynthesis of isoprenoids in higher plant chloroplasts proceeds via a mevalonate-independent pathway. FEBS Lett. 400, 271–274. doi: 10.1016/S0014-5793(96)01404-4
Lin, J., Chen, Y., Zhang, P., Ren, M., Xu, H., and Wang, X. (2013). A Novel quality evaluation index and strategies to identify scenting quality of jasmine tea based on headspace volatiles analysis. Food Sci. Biotechnol. 22, 331–340. doi: 10.1007/s10068-013-0085-x
Lin, Y. L., Lee, Y. R., Huang, W. K., Chang, S. T., and Chu, F. H. (2014). Characterization of S-(+)-linalool synthase from several provenances of Cinnamomum osmophloeum. Tree Genet. Genom. 10, 75–86. doi: 10.1007/s11295-013-0665-1
Liu, F., Xiao, Z., Yang, L., Chen, Q., Shao, L., Liu, J., et al. (2017). PhERF6, interacting with EOBI, negatively regulates fragrance biosynthesis in petunia flowers. New Phytol. 215, 1490–1502. doi: 10.1111/nph.14675
Liu, X., Hao, N., Feng, R., Meng, Z., Li, Y., and Zhao, Z. (2021). Transcriptome and metabolite profiling analyses provide insight into volatile compounds of the apple cultivar “Ruixue” and its parents during fruit development. BMC. Plant Biol. 21:231. doi: 10.1186/s12870-021-03032-3
Long, M. C., Nagegowda, D. A., Kaminaga, Y., Ho, K. K., Kish, C. M., Schnepp, J., et al. (2009). Involvement of snapdragon benzaldehyde dehydrogenase in benzoic acid biosynthesis. Plant J. 59, 256–265. doi: 10.1111/j.1365-313X.2009.03864.x
Lu, J., Vigneault, C., Marie, T. C., and Raghavan, V. G. S. (2007). Heat treatment application to increase fruit and vegetable quality. Stewart Postharvest Rev. 3, 1–7. doi: 10.2212/spr.2007.3.4
Lu, X., Meng, G., Jin, W., and Gao, H. (2018). Effects of 1-MCP in combination with Ca application on aroma volatiles production and softening of ‘Fuji’ apple fruit. Sci. Hortic. 229, 91–98. doi: 10.1016/j.scienta.2017.10.033
Ma, L., Wang, Q., Li, L., Grierson, D., Yuan, S., Zheng, S., et al. (2021). UV-C irradiation delays the physiological changes of bell pepper fruit during storage. Postharvest Biol. Tech. 180:111506. doi: 10.1016/j.postharvbio.2021.111506
Maeda, H., and Dudareva, N. (2012). The shikimate pathway and aromatic amino acid biosynthesis in plants. Annu. Rev. Plant Biol. 63, 73–105. doi: 10.1146/annurev-arplant-042811-105439
Maeda, H., Shasany, A. K., Schnepp, J., Orlova, I., Taguchi, G., Cooper, B. R., et al. (2010). RNAi suppression of arogenate dehydratase1 reveals that phenylalanine is synthesized predominantly via the arogenate pathway in petunia petals. Plant Cell 22, 832–849. doi: 10.1105/tpc.109.073247
Mahalwal, V. S., and Ali, M. (2003). Volatile constituents of the fruits peels of Citrus lemon (linn) burm. f. J. Essent. Oil Bear. Plants 6, 31–35. doi: 10.1080/0972-060X.2003.10643325
Majetic, C. J., and Sinka, B. N. (2013). Diverging pathways: differential benzenoid and phenylpropanoid volatile production in Phlox subulata L. cultivars. Biochem. Syst. Ecol. 50, 75–81. doi: 10.1016/j.bse.2013.03.047
Manzo, A., Panseri, S., Vagge, I., and Giorgi, A. (2014). Volatile fingerprint of Italian populations of orchids using solid phase microextraction and gas chromatography coupled with mass spectrometry. Molecules 19, 7913–7936. doi: 10.3390/molecules19067913
Martin, J. J., Pitera, D. J., Withers, S. T., Newmann, J. D., and Keasling, J. D. (2003). Engineering a mevalonate pathway in Escherichia coli for production of terpenoids. Nat. Biotechnol. 21, 796–802. doi: 10.1038/nbt833
Matsumoto, H., and Ikoma, Y. (2015). Effect of postharvest temperature on the muscat flavor and aroma volatile content in the berries of “Shine Muscat” (Vitis labruscana Baily × V. vinifera L.). Postharvest Biol. Tech. 112, 256–265. doi: 10.1016/j.postharvbio.2015.09.004
Meixia, C., Xuesen, C., and Baochun, F. (2004). GC-MS analysis of fruit aroma components of two apricot cultivars. Acta Hortic. Sin. 31, 663–665.
Melliou, E., Kalpoutzakis, E., Tsitsa, E., and Magiatis, P. (2007). Composition of the essential oils of Narcissus tazetta and Narcissus serotinus from Greece. J. Essent. Oil Bear. Plants 10, 101–103. doi: 10.1080/0972060X.2007.10643526
Miles, E. W. (2001). Tryptophan synthase: a multienzyme complex with an intramolecular tunnel. Chem. Rec. 1, 140–151. doi: 10.1002/tcr.4
Mirhosseini, H., Salmah, Y., Nazimah, S. A. H., and Tan, C. P. (2007). Solid-phase microextraction for headspace analysis of key volatile compounds in orange beverage emulsion. Food Chem. 105, 1659–1670. doi: 10.1016/j.foodchem.2007.04.039
Miyazawa, M., Shirakawa, N., Utsunomiya, H., Inada, K. I., and Yamada, T. (2009). Comparision of the volatile components of unripe and ripe Japanese apricot (Prunus mume Sieb. et Zucc.). Nat. Prod. Res. 23, 1567–1571. doi: 10.1080/14786410500462926
Mobley, E. M., Kunkel, B. N., and Keith, B. (1999). Identification, characterization and comparative analysis of a novel chorismate mutase gene in Arabidopsis thaliana. Gene 240, 115–123. doi: 10.1016/S0378-1119(99)00423-0
Moerkercke, A., Van Schauvinhold, I., Pichersky, E., Haring, M. A., and Schuurink, R. C. (2009). A plant thiolase involved in benzoic acid biosynthesis and volatile benzenoid production. Plant J. 60, 292–302. doi: 10.1111/j.1365-313X.2009.03953.x
Mohd-Hairul, A. R., Namasivayam, P., Lian, G. E. C., and Abdullah, J. O. (2010). Terpenoid, benzenoid, and phenylpropanoid compounds in the floral scent of Vanda Mimi Palmer. J. Plant Biol. 53, 358–366. doi: 10.1007/s12374-010-9123-x
Monson, R. K. (2013). “Metabolic and gene expression controls on the production of biogenic volatile organic compounds,” in Biology, Controls and Models of Tree Volatile Organic Compound Emissions, eds Ü Niinemets and R. Monson (Berlin: Springer), 153–179. doi: 10.1007/978-94-007-6606-8_6
Montero-Calderón, M., Rojas-Graü, M. A., and Martín-Belloso, O. (2010). Aroma profile and volatiles odor activity along gold cultivar pineapple flesh. J. Food Sci. 75, 506–512. doi: 10.1111/j.1750-3841.2010.01831.x
Morrell, K., and Kessler, A. (2014). The scent of danger: volatile-mediated information transfer and defence priming in plants. Biochemist 36, 26–31. doi: 10.1042/BIO03605026
Muhlemann, J. K., Klempien, A., and Dudareva, N. (2014). Floral volatiles: from biosynthesis to function. Plant Cell Environ. 37, 1936–1949. doi: 10.1111/pce.12314
Nagegowda, D. A., Gutensohn, M., Wilkerson, C. G., and Dudareva, N. (2008). Two nearly identical terpene synthases catalyze the formation of nerolidol and linalool in snapdragon flowers. Plant J. 55, 224–239. doi: 10.1111/j.1365-313X.2008.03496.x
Neelam, Khatkar, A., and Sharma, K. K. (2020). Phenylpropanoids and its derivatives: biological activities and its role in food, pharmaceutical and cosmetic industries. Crit. Rev. Food Sci. 60, 2655–2675. doi: 10.1080/10408398.2019.1653822
Negre-Zakharov, F., Long, M. C., and Dudareva, N. (2009). “Floral scents and fruit aromas inspired by nature,” in Plant-Derived Natural Products Synthesis, Function, and Application, eds A. Osbourn and V. Lanzotti (New York, NY: Springer), 405–431. doi: 10.1007/978-0-387-85498-4_19
Newman, J. D., and Chappell, J. (1999). Isoprenoid biosynthesis in plants: carbon partitioning within the cytoplasmic pathway. Crit. Rev. Biochem. Mol. 34, 95–106. doi: 10.1080/10409239991209228
Nieuwenhuizen, N. J., Chen, X., Wang, M. Y., Matich, A. J., Perez, R. L., Allan, A. C., et al. (2015). Natural variation in monoterpene synthesis in kiwifruit: transcriptional regulation of terpene synthases by NAC and ETHYLENE-INSENSITIVE3-like transcription factors. Plant Physiol. 167, 1243–1258. doi: 10.1104/pp.114.254367
Obenland, D., Collin, S., Sievert, J., Negm, F., and Arpaia, M. L. (2012). Influence of maturity and ripening on aroma volatiles and flavor in “Hass” avocado. Postharvest Biol. Tech. 71, 41–50. doi: 10.1016/j.postharvbio.2012.03.006
Ogura, K., and Koyama, T. (1998). Enzymatic aspects of isoprenoid chain elongation. Chem. Rev. 98, 1263–1276. doi: 10.1021/cr9600464
Ohgami, S., Ono, E., Horikawa, M., Murata, J., Totsuka, K., Toyonaga, H., et al. (2015). Volatile glycosylation in tea plants: sequential glycosylations for the biosynthesis of aroma β-primeverosides are catalyzed by two Camellia sinensis glycosyltransferases. Plant Physiol. 168, 464–477. doi: 10.1104/pp.15.00403
Orlova, I., Marshall-Colón, A., Schnepp, J., Wood, B., Varbanova, M., Fridman, E., et al. (2006). Reduction of benzenoid synthesis in petunia flowers reveals multiple pathways to benzoic acid and enhancement in auxin transport. Plant Cell 18, 3458–3475. doi: 10.1105/tpc.106.046227
Oyama-Okubo, N., Sakai, T., Ando, T., Nakayama, M., and Soga, T. (2013). Metabolome profiling of floral scent production in Petunia axillaris. Phytochemistry 90, 37–42. doi: 10.1016/j.phytochem.2013.03.006
Oyama-Okubo, N., and Tsuji, T. (2013). Analysis of floral scent compounds and classification by scent quality in tulip cultivars. J. Jpn. Soc. Hortic. Sci. 82, 344–353. doi: 10.2503/jjshs1.82.344
Oyama-Okubo, N., and Tsuji, T. (2019). Diversity of floral scent of tulips. Acta Hortic. 1237, 259–267. doi: 10.17660/ActaHortic.2019.1237.34
Palade, M., and Popa, M. E. (2016). Differentiation of three grape varieties by using sensory analysis and characterization of the volatile compounds profile of their musts. Rom. Biotech. Lett. 22, 12005–12009.
Paparella, A., Shaltiel-harpaza, L., and Ibdah, M. (2021). β-Ionone: its occurrence and biological function and metabolic engineering. Plants 10:754. doi: 10.3390/plants10040754
Parthasarathy, A., Cross, P. J., Dobson, R. C. J., Adams, L. E., Savka, M. A., and Hudson, A. O. (2018). A three-ring circus: metabolism of the three proteogenic aromatic amino acids and their role in the health of plants and animals. Front. Mol. Biosci. 5:29. doi: 10.3389/fmolb.2018.00029
Patrick, R. M., Huang, X. Q., Dudareva, N., and Li, Y. (2021). Dynamic histone acetylation in floral volatile synthesis and emission in petunia flowers. J. Exp. Bot. 72, 3704–3722. doi: 10.1093/jxb/erab072
Phillips, M. A., Gershenzon, J., and Pichersky, E. (2008). The Arabidopsis thaliana type I isopentenyl diphosphate isomerases are targeted to multiple subcellular compartments and have overlapping functions in isoprenoid biosynthesis. Plant Cell 20, 677–696. doi: 10.1105/tpc.107.053926
Picazo-Aragonés, J., Terrab, A., and Balao, F. (2020). Plant volatile organic compounds evolution: transcriptional regulation, epigenetics and polyploidy. Int. J. Mol. Sci. 21:8956. doi: 10.3390/ijms21238956
Piechulla, B., and Effmert, U. (2010). “Biosynthesis and regulation of flower scent,” in Plant Developmental Biology - Biotechnological Perspectives, eds E. Pua and M. Davey (Berlin: Springer), 189–205. doi: 10.1007/978-3-642-04670-4_10
Piechulla, B., and Pott, M. B. (2003). Plant scents—mediators of inter-and intraorganismic communication. Planta 217, 687–689. doi: 10.1007/s00425-003-1047-y
Pino, J. A. (2012). Odour-active compounds in mango (Mangifera indica L. cv. Corazón). Int. J. Food Sci. 47, 1944–1950. doi: 10.1111/j.1365-2621.2012.03054.x
Pino, J. A., and Mesa, J. (2006). Contribution of volatile compounds to mango (Mangifera indica L.) aroma. Flav. Frag. J. 21, 207–213. doi: 10.1002/ffj.1703
Pino, J. A., and Queris, O. (2010). Analysis of volatile compounds of pineapple wine using solid-phase microextraction techniques. Food Chem. 122, 1241–1246. doi: 10.1016/j.foodchem.2010.03.033
Pott, D. M., Osorio, S., and Vallarino, J. G. (2019). From central to specialized metabolism: an overview of some secondary compounds derived from the primary metabolism for their role in conferring nutritional and organoleptic characteristics to fruit. Front. Plant Sci. 10:835. doi: 10.3389/fpls.2019.00835
Poulter, C. D., and Rilling, H. C. (1981). “Prenyl transferases and isomerase,” in Biosynthesis of Isoprenoid Compounds, eds J. W. Porter and S. L. Spurgeon (New York, NY: John Wiley and Sons), 161–224.
Prat, L., Espinoza, M. I., Agosin, E., and Silva, H. (2013). Identification of volatile compounds associated with the aroma of white strawberries (Fragaria chiloensis). J. Sci. Food Agric. 94, 752–759. doi: 10.1002/jsfa.6412
Qian, Y., Lynch, J. H., Guo, L., Rhodes, D., Morgan, J. A., and Dudareva, N. (2019). Completion of the cytosolic post-chorismate phenylalanine biosynthetic pathway in plants. Nat. Commun. 10:15. doi: 10.1038/s41467-018-07969-2
Qiao, Y., Bi, J. X., Zhang, Y., Zhang, Y., Fan, G., Xiao, L. Y., et al. (2008). Characterization of aroma active compounds in fruit juice and peel oil of Jinchen sweet orange fruit (Citrus sinensis (L.) Osbeck) by GC-MS and GC-O. Molecules 13, 1333–1344. doi: 10.3390/molecules13061333
Qualley, A. V., Widhalm, J. R., Adebesin, F., Kish, C. M., and Dudareva, N. (2012). Completion of the core β-oxidative pathway of benzoic acid biosynthesis in plants. Proc. Natl. Acad. Sci. U.S.A. 109, 16383–16388. doi: 10.1073/pnas.1211001109
Queiroz, L., Garruti, D. S., Sampaio, K. L., Zerlotti, A., Aparecida, M., and Silva, A. P. D. (2014). Aroma compounds derived from the thermal degradation of carotenoids in a cashew apple juice model. Food Res. Int. 56, 108–114. doi: 10.1016/j.foodres.2013.12.015
Quijano, C. E., Salamanca, G., and Pino, J. A. (2007). Aroma volatile constituents of Colombian varieties of mango (Mangifera indica L.). Flav. Frag. J. 22, 401–406. doi: 10.1002/ffj.1812
Raguso, R. A. (2008). Wake up and smell the roses: the ecology and evolution of floral scent. Annu. Rev. Ecol. Evol. Sci. 39, 549–569. doi: 10.1146/annurev.ecolsys.38.091206.095601
Ramya, M., An, H. R., Baek, Y. S., and Reddy, K. E. (2018). Orchid floral volatiles?: biosynthesis genes and transcriptional regulations. Sci. Hortic. Amst. 235, 62–69. doi: 10.1016/j.scienta.2017.12.049
Ramya, M., Jang, S., An, H. R., Lee, S. Y., Park, P. M., and Park, P. H. (2020). Volatile organic compounds from orchids: from synthesis and function to gene regulation. Int. J. Mol. Sci. 21:1160. doi: 10.3390/ijms21031160
Ramya, M., Kwon, O. K., An, H. R., Park, P. M., Baek, Y. S., and Park, P. H. (2017). Floral scent: regulation and role of MYB transcription factors. Phytochem. Lett. 19, 114–120. doi: 10.1016/j.phytol.2016.12.015
Ramya, M., Lee, S. Y., An, H. R., Park, P. M., Kim, N. S., and Park, P. H. (2019). MYB1 transcription factor regulation through floral scent in Cymbidium cultivar “Sael Bit.”. Phytochem. Lett. 32, 181–187. doi: 10.1016/j.phytol.2019.06.007
Ripoll, G., Vazquez, M., and Vilanova, M. (2017). Ultraviolet - visible - near infrared spectroscopy for rapid determination of volatile compounds in white grapes during ripening. Cienc. Tech. Vitivinic. 32, 53–61. doi: 10.1051/ctv/20173201053
Rippert, P., and Matringe, M. (2002). Molecular and biochemical characterization of an Arabidopsis thaliana arogenate dehydrogenase with two. Plant Mol. Biol. 48, 361–368. doi: 10.1023/A:1014018926676
Rippert, P., Puyaubert, J., Grisollet, D., Derrier, L., and Matringe, M. (2009). Tyrosine and phenylalanine are synthesized within the plastids in arabidopsis. Plant Physiol. 149, 1251–1260. doi: 10.1104/pp.108.130070
Riu-Aumatell, M., Castellari, M., López-Tamames, E., Galassi, S., and Buxaderas, S. (2004). Characterisation of volatile compounds of fruit juices and nectars by HS/SPME and GC/MS. Food Chem. 87, 627–637. doi: 10.1016/j.foodchem.2003.12.033
Roccia, A., Oyant, L. H., Saint Cavel, E., Caissard, J. C., Machenaud, J., Thouroude, T., et al. (2019). Biosynthesis of 2-phenylethanol in rose petals is linked to the expression of one allele of rhpaas. Plant Physiol. 179, 1064–1079. doi: 10.1104/pp.18.01468
Romero, H., Pott, D. M., Vallarino, J. G., and Osorio, S. (2021). Metabolomics-based evaluation of crop quality changes as a consequence of climate change. Metabolites 11:461. doi: 10.3390/metabo11070461
Ruíz-Ramón, F., Águila, D. J., Egea-Cortines, M., and Weiss, J. (2014). Optimization of fragrance extraction: daytime and flower age affect scent emission in simple and double narcissi. Ind. Crop Prod. 52, 671–678. doi: 10.1016/j.indcrop.2013.11.034
Sagae, M., Oyama-Okubo, N., Ando, T., Marchesi, E., and Nakayama, M. (2008). Effect of temperature on the floral scent emission and endogenous volatile profile of Petunia axillaris. Biosci. Biotech. Biochem. 72, 110–115. doi: 10.1271/bbb.70490
Saha, B., Bucknall, M. P., Arcot, J., and Driscoll, R. (2018). Profile changes in banana flavour volatiles during low temperature drying. Food Res. Int. 106, 992–998. doi: 10.1016/j.foodres.2018.01.047
Sakai, M., Hirata, H., Sayama, H., Sekiguchi, K., Itano, H., Asai, T., et al. (2007). Production of 2-phenylethanol in roses as the dominant floral scent compound from L-phenylalanine by two key enzymes, a PLP-dependent decarboxylase and a phenylacetaldehyde reductase. Biosci. Biotech. Biochem. 71, 2408–2419. doi: 10.1271/bbb.70090
Santhanam, J., Abd Ghani, F. N., and Basri, D. F. (2014). Antifungal activity of Jasminum sambac against Malassezia sp. and Non- Malassezia sp. isolated from human skin samples. J. Mycol. 2014, 359630. doi: 10.1155/2014/359630
Sanz, C., Olı’as, J. M., and Pe’rez, A. G. (1997). “Aroma biochemistry of fruits and vegetables,” in Phytochemistry of Fruits and Vegetables, eds F. A. Toma’;s, U. G. Barbera’n, and R. J. Robins (Oxford: Clarendon Press), 125–155.
Schenck, C. A., Westphal, J., Jayaraman, D., Garcia, K., Wen, J., Mysore, K. S., et al. (2020). Role of cytosolic, tyrosine-insensitive prephenate dehydrogenase in Medicago truncatula. Plant Direct. 4:e00218. doi: 10.1002/pld3.218
Schiestl, F. P. (2005). On the success of a swindle: pollination by deception in orchids. Sci. Nat. 92, 255–264. doi: 10.1007/s00114-005-0636-y
Schiestl, F. P. (2010). The evolution of floral scent and insect chemical communication. Ecol. Lett. 13, 643–656. doi: 10.1111/j.1461-0248.2010.01451.x
Schiestl, F. P. (2015). Ecology and evolution of floral volatile- mediated information transfer in plants. New Phytol. 206, 571–577. doi: 10.1111/nph.13243
Schiestl, F. P., Peakall, R., Mant, J. G., Ibarra, F., Schulz, C., Franke, S., et al. (2003). The chemistry of sexual deception in an orchid-wasp pollination system. Science 302, 437–438. doi: 10.1126/science.1087835
Schwab, W., Fischer, T. C., Giri, A., and Wüst, M. (2015). Potential applications of glucosyltransferases in terpene glucoside production: impacts on the use of aroma and fragrance. Appl. Microbiol. Biot. 99, 165–174. doi: 10.1007/s00253-014-6229-y
Shalit, M., Shafir, S., Larkov, O., Bar, E., Kaslassi, D., Adam, Z., et al. (2004). Volatile compounds emitted by rose cultivars: fragrance perception by man and honeybees. Isr. J. Plant Sci. 52, 245–255. doi: 10.1560/P7G3-FT41-XJCP-1XFM
Shohayeb, M., Abdel-Hameed, E. S. S., Bazaid, S. A., and Maghrabi, I. (2014). Antibacterial and antifungal activity of Rosa damascena MILL. essential oil, different extracts of rose petals. Glob. J. Pharmacol. 8, 1–7. doi: 10.5829/idosi.gjp.2014.8.1.81275
Singh, G. (2004). Plants Systematics: An Integrated Approach. New Hampshire: Science Publishers Inc, 83.
Śmigielski, K. B., Prusinowska, R., Krosowiak, K., and Sikora, M. (2013). Comparison of qualitative and quantitative chemical composition of hydrolate and essential oils of lavender (Lavandula angustifolia). J. Essent. Oil Res. 25, 291–299. doi: 10.1080/10412905.2013.775080
Solis-Solis, H. M., Calderon-Santoyo, M., Gutierrez-Martinez, P., Schorr-Galindo, S., and Ragazzo-Sanchez, J. A. (2007). Discrimination of eight varieties of apricot (Prunus armeniaca) by electronic nose, LLE and SPME using GC-MS and multivariate analysis. Sens. Actuat. B Chem. 125, 415–421. doi: 10.1016/j.snb.2007.02.035
Song, C., Wang, Q., Teixeira da Silva, J. A., and Yu, X. (2018). Identification of floral fragrances and analysis of fragrance patterns in herbaceous peony cultivars. J. Am. Soc Hortic. Sci. 143, 248–258. doi: 10.21273/JASHS04420-18
Song, G., Xiao, J., Deng, C., Zhang, X., and Hu, Y. (2007). Use of solid-phase microextraction as a sampling technique for the characterization of volatile compounds emitted from Chinese daffodil flowers. J. Anal. Chem. 62, 674–679. doi: 10.1134/S1061934807070118
Sparinska, A., and Rostoks, N. (2015). Volatile organic compounds of Hybrid Rugosa roses in Latvia. Proc. Latv. Acad. Sci. B Nat. Exact Appl. Sci. 69, 57–61. doi: 10.1515/prolas-2015-0007
Spitzer-Rimon, B., Farhi, M., Albo, B., Cna’ani, A., Ben Zvi, M. M., Masci, T., et al. (2012). The R2R3-MYB-like regulatory factor EOBI, acting downstream of EOBII, regulates scent production by activating ODO1 and structural scent-related genes in Petunia. Plant Cell 24, 5089–5105. doi: 10.1105/tpc.112.105247
Spitzer-Rimon, B., Marhevka, E., Barkai, O., Marton, I., Edelbaum, O., Masci, T., et al. (2010). EOBII, a gene encoding a flower-specific regulator of phenylpropanoid volatiles’ biosynthesis in Petunia. Plant Cell 22, 1961–1976. doi: 10.1105/tpc.109.067280
Srisukh, V., Tribuddharat, C., Nukoolkarn, V., Bunyapraphatsara, N., Chokephaibulkit, K., Phoomniyom, S., et al. (2012). Antibacterial activity of essential oils from Citrus hystrix (makrut lime) against respiratory tract pathogens. Scienceasia 38, 212–217. doi: 10.2306/scienceasia1513-1874.2012.38.212
Steiner, K. E., Kaiser, R., and Dötterl, S. (2011). Strong phylogenetic effects on floral scent variation of oil-secreting orchids in South Africa. Am. J. Bot. 98, 1663–1679. doi: 10.3732/ajb.1100141
Steingass, C. B., Carle, R., and Schmarr, H. G. (2015). Ripening-dependent metabolic changes in the volatiles of pineapple (Ananas comosus (L.) Merr.) fruit: I. Characterization of pineapple aroma compounds by comprehensive two-dimensional gas chromatography-mass spectrometry. Anal. Bioanal. Chem. 407, 2591–2608. doi: 10.1007/s00216-015-8474-z
Sultana, H. S., Ali, M., and Panda, B. P. (2012). Influence of volatile constituents of fruit peels of Citrus reticulata Blanco on clinically isolated pathogenic microorganisms under in-vitro. Asian Pac. J. Trop. Biol. 2, S1299–S1302. doi: 10.1016/S2221-1691(12)60404-3
Sun, L., Zhu, B., Zhang, X., Zhang, G., Yan, A., and Wang, H. (2019). Transcriptome profiles of three Muscat table grape cultivars to dissect the mechanism of terpene biosynthesis. Sci. Data 6:89. doi: 10.1038/s41597-019-0101-y
Suttipanta, N., Pattanaik, S., Kulshrestha, M., Patra, B., Singh, S. K., and Yuan, L. (2011). The transcription factor CrWRKY1 positively regulates the terpenoid indole alkaloid biosynthesis in Catharanthus roseus. Plant Physiol. 157, 2081–2093. doi: 10.1104/pp.111.181834
Tanase, K., Nishitani, C., Hirakawa, H., Isobe, S., Tabata, S., Ohmiya, A., et al. (2012). Transcriptome analysis of carnation (Dianthus caryophyllus L.) based on next-generation sequencing technology. BMC Genom. 13:292. doi: 10.1186/1471-2164-13-292
Terry, M. I., Ruiz-Hernández, V., Águila, D. J., Weiss, J., and Egea-Cortines, M. (2021). The effect of post-harvest conditions in Narcissus sp. cut flowers scent profile. Front. Plant Sci. 11:540821. doi: 10.3389/fpls.2020.540821
Tieman, D., Taylor, M., Schauer, N., Fernie, A. R., Hanson, A. D., and Klee, H. J. (2006). Tomato aromatic amino acid decarboxylases participate in synthesis of the flavor volatiles 2-phenylethanol and 2-phenylacetaldehyde. Proc. Natl. Acad. Sci. U.S.A. 103, 8287–8292. doi: 10.1073/pnas.0602469103
Tieman, D. M., Loucas, H. M., Kim, J. Y., Clark, D. G., and Klee, H. J. (2007). Tomato phenylacetaldehyde reductases catalyze the last step in the synthesis of the aroma volatile 2-phenylethanol. Phytochemistry 68, 2660–2669. doi: 10.1016/j.phytochem.2007.06.005
Toh, C., Mohd-Hairul, A. R., Ain, N. M., Namasivayam, P., Go, R., Abdullah, N. A. P., et al. (2017). Floral micromorphology and transcriptome analyses of a fragrant Vandaceous Orchid, Vanda Mimi Palmer, for its fragrance production sites. BMC Res. Notes 10:554. doi: 10.1186/s13104-017-2872-6
Tschiggerl, C., and Bucar, F. (2010). Volatile fraction of lavender and bitter fennel infusion extracts. Nat. Prod. Commun. 5, 1431–1436. doi: 10.1177/1934578x1000500917
Tucker, G. (1993). “Introduction in biochemistry of fruit ripening,” in Biochemistry of Fruit Ripening, eds G. Seymour, J. Taylor, and G. Tucker (London: Chapman & Hall), 1–51. doi: 10.1007/978-94-011-1584-1_1
Tzin, V., and Galili, G. (2010b). New insights into the shikimate and aromatic amino acids biosynthesis pathways in plants. Mol. Plant 3, 956–972. doi: 10.1093/mp/ssq048
Tzin, V., and Galili, G. (2010a). The biosynthetic pathways for shikimate and aromatic amino acids in Arabidopsis thaliana. Arabidopsis Book 8:e0132. doi: 10.1199/tab.0132
Unnisa, N., Tabassum, H., Ali, M. N., and Ponia, K. (2012). Evaluation of antibacterial activity of five selected fruits on bacterial wound isolates. Int. J. Pharma. Bio. Sci. 3, 531–546.
Van Moerkercke, A., Galván-Ampudia, C. S., Verdonk, J. C., Haring, M. A., and Schuurink, R. C. (2012). Regulators of floral fragrance production and their target genes in petunia are not exclusively active in the epidermal cells of petals. J. Exp. Bot. 63, 3157–3171. doi: 10.1093/jxb/ers034
Van Moerkercke, A., Haring, M. A., and Schuurink, R. C. (2011). The transcription factor EMISSION of BENZENOIDS II activates the MYB ODORANT1 promoter at a MYB binding site specific for fragrant petunias. Plant J. 67, 917–928. doi: 10.1111/j.1365-313X.2011.04644.x
Venskutonis, P. R., Dapkevicius, A., and Baranauskiene, M. (1997). Composition of the essential oil of lavender (Lavandula angustifolia Mill.) from Lithuania. J. Essent. Oil Res. 9, 107–110. doi: 10.1080/10412905.1997.9700727
Verdonk, J. C., Haring, M. A., Van Tunen, A. J., and Schuurink, R. C. (2005). Odorant1 regulates fragrance biosynthesis in petunia flowers. Plant Cell 17, 1612–1624. doi: 10.1105/tpc.104.028837
Viswanath, K. K., Varakumar, P., Pamuru, R. R., Basha, S. J., Mehta, S., and Rao, A. D. (2020). Plant lipoxygenases and their role in plant physiology. J. Plant Biol. 63, 83–95. doi: 10.1007/s12374-020-09241-x
Vranová, E., Coman, D., and Gruissem, W. (2013). Network analysis of the MVA and MEP pathways for isoprenoid synthesis. Annu. Rev. Plant Biol. 64, 665–700. doi: 10.1146/annurev-arplant-050312-120116
Wang, J., Liu, Q., Xie, B., and Sun, Z. (2020). Effect of ultrasound combined with ultraviolet treatment on microbial inactivation and quality properties of mango juice. Ultrason. Sonochem. 64:105000. doi: 10.1016/j.ultsonch.2020.105000
Wang, J., Yu, L., Qi, X., Gao, H., and Yang, J. (2013). Difference in aromatic components between hyacinths and European daffodil by GC-MS. J. Beijing Uni. Agric. 28, 46–49.
Wang, T., Xie, A., Zhang, D., Liu, Z., Li, X., Li, Y., et al. (2021). Analysis of the volatile components in flowers of Paeonia lactiflora Pall. and Paeonia lactiflora Pall. var. Trichocarpa. Am. J. Plant Sci. 12, 146–162. doi: 10.4236/ajps.2021.121009
Wang, Y., Yang, C., Li, S., Yang, L., Wang, Y., Zhao, J., et al. (2009). Volatile characteristics of 50 peaches and nectarines evaluated by HP-SPME with GC-MS. Food Chem. 116, 356–364. doi: 10.1016/j.foodchem.2009.02.004
Warner, R., Wu, B. S., MacPherson, S., and Lefsrud, M. (2021). A review of strawberry photobiology and fruit flavonoids in controlled environments. Front. Plant Sci. 12:611893. doi: 10.3389/fpls.2021.611893
Watanabe, N., Susanne, B., Yang, Z., Sakai, M., and Fleischmann, P. (2009). Volatile constituents in the scent of roses. Floric. Ornament. Biotech. 3, 89–97.
Weber-Ban, E., Hur, O., Bagwell, C., Banik, U., Yang, L. H., Miles, E. W., et al. (2001). Investigation of allosteric linkages in the regulation of tryptophan synthase: the roles of salt bridges and monovalent cations probed by site-directed mutation, optical spectroscopy, and kinetics. Biochemistry 40, 3497–3511. doi: 10.1021/bi002690p
Wei, C., Liu, H., Cao, X., Zhang, M., Li, X., Chen, K., et al. (2021). Synthesis of flavour-related linalool is regulated by PpbHLH1 and associated with changes in DNA methylation during peach fruit ripening. Plant Biotechnol. J. 19, 2082–2096. doi: 10.1111/pbi.13638
Wei, C. B., Liu, S. H., Liu, Y. G., Lv, L. L., Yang, W. X., and Sun, G. M. (2011). Characteristic aroma compounds from different pineapple parts. Molecules 16, 5104–5112. doi: 10.3390/molecules16065104
Widhalm, J. R., and Dudareva, N. (2015). A familiar ring to it: biosynthesis of plant benzoic acids. Mol. Plant 8, 83–97. doi: 10.1016/j.molp.2014.12.001
Won, M. M., Cha, E. J., Yoon, O. K., Kim, N. S., Kim, K., and Lee, D. S. (2009). Use of headspace mulberry paper bag micro solid phase extraction for characterization of volatile aromas of essential oils from Bulgarian rose and Provence lavender. Anal. Chim. Acta 631, 54–61. doi: 10.1016/j.aca.2008.10.013
Xi, W., He, Y., Zhu, L., Hu, S., Xiong, S., Zhang, Y., et al. (2021). CPTA treatment reveals potential transcription factors associated with carotenoid metabolism in flowers of Osmanthus fragrans. Hortic. Plant J. 7, 479–487. doi: 10.1016/j.hpj.2021.03.002
Xi, W., Zheng, H., Zhang, Q., and Li, W. (2016). Profiling taste and aroma compound metabolism during apricot fruit development and ripening. Int. J. Mol. Sci. 17:998. doi: 10.3390/ijms17070998
Xiao, O., Li, M., Chen, J., Li, R., Quan, R., Zhang, Z., et al. (2020). Influence of triazole pesticides on wine flavor and quality based on multidimensional analysis technology. Molecules 25:5596. doi: 10.3390/molecules25235596
Xiao, Z., Li, Q., Niu, Y., Zhou, X., Liu, J., Xu, Y., et al. (2017). Odor-active compounds of different lavender essential oils and their correlation with sensory attributes. Ind. Crop Prod. 108, 748–755. doi: 10.1016/j.indcrop.2017.07.040
Xie, D. Y., Sharma, S. B., Wright, E., Wang, Z. Y., and Dixon, R. A. (2006). Metabolic engineering of proanthocyanidins through co-expression of anthocyanidin reductase and the PAP1 MYB transcription factor. Plant J. 45, 895–907. doi: 10.1111/j.1365-313X.2006.02655.x
Xin, M., Li, C., Khoo, H. E., Li, L., He, X., Yi, P., et al. (2021). Dynamic analyses of transcriptome and metabolic profiling: revealing molecular insight of aroma synthesis of mango (Mangifera indica L. Var. Tainong). Front. Plant Sci. 12:666805. doi: 10.3389/fpls.2021.666805
Yamada, T., Matsuda, F., Kasai, K., Fukuoka, S., Kitamura, K., Tozawa, Y., et al. (2008). Mutation of a rice gene encoding a phenylalanine biosynthetic enzyme results in accumulation of phenylalanine and tryptophan. Plant Cell 20, 1316–1329. doi: 10.1105/tpc.107.057455
Yan, H., Zhang, H., Wang, Q., and Rhmyb, Á (2011). Isolation and identification of a putative scent-related gene RhMYB1 from rose. Mol. Biol. Rep. 38, 4475–4482. doi: 10.1007/s11033-010-0577-1
Yan, J. W., Ban, Z. J., Lu, H. Y., Li, D., Poverenov, E., Luo, Z. S., et al. (2018). The aroma volatile repertoire in strawberry fruit: a review. J. Sci. Food Agric. 98, 4395–4402. doi: 10.1002/jsfa.9039
Yang, F. X., Gao, J., Wei, Y. L., Ren, R., Zhang, G. Q., Lu, C. Q., et al. (2021). The genome of Cymbidium sinense revealed the evolution of orchid traits. Plant Biotechnol. J. 19, 2501–2516. doi: 10.1111/pbi.13676
Yang, S., Hao, N., Meng, Z., Li, Y., and Zhao, Z. (2021). Identification, comparison and classification of volatile compounds in peels of 40 apple cultivars by HS–SPME with GC–MS. Foods 10:1051. doi: 10.3390/foods10051051
Yauk, Y., Ged, C., Wang, M. Y., Matich, A. J., Tessarotto, L., Cooney, J. M., et al. (2014). Manipulation of flavour and aroma compound sequestration and release using a glycosyltransferase with specificity for terpene alcohols. Plant J. 80, 317–330. doi: 10.1111/tpj.12634
Yokoyama, R., de Oliveira, M. V. V., Kleven, B., and Maeda, H. A. (2021). The entry reaction of the plant shikimate pathway is subjected to highly complex metabolite-mediated regulation. Plant Cell 33, 671–696. doi: 10.1093/plcell/koaa042
Yoo, H., Widhalm, J. R., Qian, Y., Maeda, H., Cooper, B. R., Jannasch, A. S., et al. (2013). An alternative pathway contributes to phenylalanine biosynthesis in plants via a cytosolic tyrosine:phenylpyruvate aminotransferase. Nat. Commun. 4:2833. doi: 10.1038/ncomms3833
Yoshida, K., Oyama-Okubo, N., and Yamagishi, M. (2018). An R2R3-MYB transcription factor ODORANT1 regulates fragrance biosynthesis in lilies (Lilium spp.). Mol. Breed. 38:144. doi: 10.1007/s11032-018-0902-2
Yue, Y., Yu, R., and Fan, Y. (2015). Transcriptome profiling provides new insights into the formation of floral scent in Hedychium coronarium. BMC Genom. 16:470. doi: 10.1186/s12864-015-1653-7
Zakaria, S. R., Saim, N., Osman, R., Haiyee, Z. A., and Juahir, H. (2018). Combination of sensory, chromatographic, and chemometrics analysis of volatile organic compounds for the discrimination of authentic and unauthentic harumanis mangoes. Molecules 23:2365. doi: 10.3390/molecules23092365
Žemlička, L., Fodran, P., Kolek, E., and Prónayová, N. (2013). Analysis of natural aroma and flavor of MD2 pineapple variety (Ananas comosus [L.] Merr.). Acta Chim. Slov. 6, 123–128. doi: 10.2478/acs-2013-0019
Zeng, L., Wang, X., Kang, M., Dong, F., and Yang, Z. (2017). Regulation of the rhythmic emission of plant volatiles by the circadian clock. Int. J. Mol. Sci. 18:2408. doi: 10.3390/ijms18112408
Zeng, X., Liu, C., Zheng, R., Cai, X., Luo, J., Zou, J., et al. (2016). Emission and accumulation of monoterpene and the key terpene synthase (TPS) associated with monoterpene biosynthesis in Osmanthus fragrans Lour. Front. Plant Sci. 6:1232. doi: 10.3389/fpls.2015.01232
Zhang, B., Han, S., Jiang, Y., Bi, Y., and Zhu, X. (2008). Analysis of volatile compounds in apricot fruit. Food Sci. 29, 559–563. doi: 10.1016/S1872-2075(08)60071-0
Zhang, B., Tieman, D. M., Jiao, C., Xu, Y., Chen, K., Fe, Z., et al. (2016). Chilling-induced tomato flavor loss is associated with altered volatile synthesis and transient changes in DNA methylation. Proc. Natl. Acad. Sci. U.S.A. 113, 12580–12585. doi: 10.1073/pnas.1618362113
Zhang, F., Fu, X., Lv, Z., Lu, X., Shen, Q., Zhang, L., et al. (2015). A basic leucine zipper transcription factor, AabZIP1, connects abscisic acid signaling with artemisinin biosynthesis in Artemisia annua. Mol. Plant 8, 163–175. doi: 10.1016/j.molp.2014.12.004
Zhang, L., Chen, F., Zhang, X., Li, Z., Zhao, Y., Lohaus, R., et al. (2020). The water lily genome and the early evolution of flowering plants. Nature 577, 79–84. doi: 10.1038/s41586-019-1852-5
Zhang, Q., Feng, C., Li, W., Qu, Z., Zeng, M., and Xi, W. (2019). Transcriptional regulatory networks controlling taste and aroma quality of apricot (Prunus armeniaca L.) fruit during ripening. BMC Genom. 20:45. doi: 10.1186/s12864-019-5424-8
Zhang, Y., Bo, C., and Wang, L. (2019). Novel crosstalks between circadian clock and jasmonic acid pathway finely coordinate the tradeoff among plant growth, senescence and defense. Int. J. Mol. Sci. 20:5254. doi: 10.3390/ijms20215254
Zhang, Y., Li, C., Wang, S., Yuan, M., Li, B., Niu, L., et al. (2021). Transcriptome and volatile compounds profiling analyses provide insights into the molecular mechanism underlying the floral fragrance of tree peony. Ind. Crop Prod. 162:113286. doi: 10.1016/j.indcrop.2021.113286
Zheng, J., Hu, Z., Guan, X., Dou, D., Bai, G., Wang, Y., et al. (2015). Transcriptome analysis of Syringa oblata lindl. inflorescence identifies genes associated with pigment biosynthesis and scent metabolism. PLoS One 10:e0142542. doi: 10.1371/journal.pone.0142542
Zheng, L. Y., Sun, G. M., Liu, Y. G., Lv, L. L., Yang, W. X., Zhao, W. F., et al. (2012). Aroma volatile compounds from two fresh pineapple varieties in China. Int. J. Mol. Sci. 13, 7383–7392. doi: 10.3390/ijms13067383
Zhong, S., Ren, J., Chen, D., Pan, S., Wang, K., Yang, S., et al. (2014). Free and bound volatile compounds in juice and peel of Eureka lemon. Food Sci. Technol. Res. 20, 167–174. doi: 10.3136/fstr.20.167
Zhou, F., and Pichersky, E. (2020). More is better: the diversity of terpene metabolism in plants. Curr. Opin. Plant Biol. 55, 1–10. doi: 10.1016/j.pbi.2020.01.005
Zhu, X., Li, Q., Li, J., Luo, J., Chen, W., and Li, X. (2018a). Comparative study of volatile compounds in the fruit of two banana cultivars at different ripening stages. Molecules 23:1017. doi: 10.3390/molecules23102456
Zhu, X., Luo, J., Li, Q., Li, J., Liu, T., Wang, R., et al. (2018b). Low temperature storage reduces aroma-related volatiles production during shelf-life of banana fruit mainly by regulating key genes involved in volatile biosynthetic pathways. Postharvest Biol. Tech. 146, 68–78. doi: 10.1016/j.postharvbio.2018.08.015
Zvi, M. M. B., Negre-Zakharov, F., Masci, T., Ovadis, M., Shklarman, E., Ben-Meir, H., et al. (2008). Interlinking showy traits: co-engineering of scent and colour biosynthesis in flowers. Plant Biotechnol. J. 6, 403–415. doi: 10.1111/j.1467-7652.2008.00329.x
Keywords: flower scent, fruit aroma, volatile function, VOC, biosynthetic pathway, transcription factor
Citation: Mostafa S, Wang Y, Zeng W and Jin B (2022) Floral Scents and Fruit Aromas: Functions, Compositions, Biosynthesis, and Regulation. Front. Plant Sci. 13:860157. doi: 10.3389/fpls.2022.860157
Received: 22 January 2022; Accepted: 09 February 2022;
Published: 10 March 2022.
Edited by:
Tong Chen, Institute of Botany (CAS), ChinaReviewed by:
Jinhua Zuo, Beijing Vegetable Research Center, ChinaZhong Qi Fan, Fujian Agriculture and Forestry University, China
Copyright © 2022 Mostafa, Wang, Zeng and Jin. This is an open-access article distributed under the terms of the Creative Commons Attribution License (CC BY). The use, distribution or reproduction in other forums is permitted, provided the original author(s) and the copyright owner(s) are credited and that the original publication in this journal is cited, in accordance with accepted academic practice. No use, distribution or reproduction is permitted which does not comply with these terms.
*Correspondence: Biao Jin, YmppbkB5enUuZWR1LmNu