- 1State Key Laboratory of Arid Land Crop Science, Lanzhou, China
- 2College of Agronomy, Gansu Agricultural University, Lanzhou, China
- 3Council for Scientific and Industrial Research (CSIR)-Plant Genetic Resources Research Institute, Bunso, Ghana
- 4Department of Crop Science, Faculty of Agriculture, Food and Consumer Sciences, University for Development Studies, Tamale, Ghana
- 5Biocontrol Engineering Laboratory of Crop Diseases and Pests of Gansu Province, College of Plant Protection, Gansu Agricultural University, Lanzhou, China
- 6Action for Climate and Environment Program, Dr. Reddy’s Foundation, Hyderabad, India
Wheat grain yield and nitrogen (N) content are influenced by the amount of N remobilized to the grain, together with pre-anthesis and post-anthesis N uptake. Isotopic techniques in farmed areas may provide insight into the mechanism underlying the N cycle. 15N-labeled urea was applied to microplots within five different fertilized treatments 0 kg ha–1 (N1), 52.5 kg ha–1 (N2), 105 kg ha–1 (N3), 157.5 kg ha–1 (N4), and 210 kg ha–1 (N5) of a long-term field trial (2003–2021) in a rainfed wheat field in the semi-arid loess Plateau, China, to determine post-anthesis N uptake and remobilization into the grain, as well as the variability of 15N enrichment in aboveground parts. Total N uptake was between 7.88 and 29.27 kg ha–1 for straw and 41.85 and 95.27 kg ha–1 for grain. In comparison to N1, N fertilization increased straw and grain N uptake by 73.1 and 56.1%, respectively. Nitrogen use efficiency (NUE) and harvest index were altered by N application rates. The average NUE at maturity was 19.9% in 2020 and 20.01% in 2021; however, it was usually higher under the control and low N conditions. The amount of 15N excess increased as the N rate increased: N5 had the highest 15N excess at the maturity stage in the upper (2.28 ± 0.36%), the middle (1.77 ± 0.28%), and the lower portion (1.68 ± 1.01%). Compared to N1, N fertilization (N2–N5) increased 15N excess in the various shoot portions by 50, 38, and 35% at maturity for upper, middle, and lower portions, respectively. At maturity, the 15N excess remobilized to the grain under N1–N5 was between 5 and 8%. Our findings revealed that N had a significant impact on yield and N isotope discrimination in spring wheat that these two parameters can interact, and that future research on the relationship between yield and N isotope discrimination in spring wheat should take these factors into account.
Introduction
Nitrogen (N) is the most important nutrient for plant growth, productivity, and grain quality (Hussain et al., 2020). Optimal use of N fertilizers is essential to improve yield, increase profitability, and minimize environmental consequences from nitrate leaching and nitrous oxide (N2O, a greenhouse gas) emissions from denitrification by soil bacteria (Lazcano et al., 2021). The global use of N fertilizer is expected to reach 240 million tons by 2050 (Zhou et al., 2018; Randive et al., 2021). However, plants only use about 40% of the fertilizer N, with the rest escaping into the atmosphere as nitrogenous emissions or getting into groundwater via leaching (Nations, 2015).
During crop growth, the plant remobilizes a part of the stored N from the vegetative stem and leaves to reproductive organs such as panicles and grains (Yan et al., 2018; Broberg et al., 2021). N remobilization is genetically controlled (Alpuerto et al., 2021) but could also be influenced by environmental factors (Zheng et al., 2020) and N fertilizer application. The accumulation and relocation of N are considered very significant processes in determining grain yield and grain quality (Gaju et al., 2011; Prey et al., 2019).
The use of 15N stable isotope labeling is an excellent method that enables a less biased and more precise estimation of N uptake after anthesis and remobilization of N accumulated during the pre-anthesis period in various organs. N isotope discrimination is controlled by the link between N supply and demand when the N environment is held at a steady-state similar to how carbon isotopes are discriminated during photosynthesis (Dong et al., 2017; Hu and Guy, 2020; Qian et al., 2021). The employment of specialized methodologies, especially for tracking aboveground N dynamics in plants, is required for the precise quantification of N cycling in plant-soil systems (Shan et al., 2012; Meng et al., 2017; Zheng et al., 2018; Xu et al., 2019). Fertilizers can be isotopically labeled with 15N to follow its evolution movement in soil-plant systems. Under field conditions, labeling with 15N shows promise in analyzing N dynamics from crop residues, which are influenced by the chemical composition and quantity of the crop residue (Berg et al., 1991; Tahir et al., 2018). Handley et al. (2020) defined nitrogen fluxes and 15N remobilization during plant development. During the post-anthesis period, the partitioning of the 15N assimilated during the pre-anthesis period and remobilized after showed that leaves, stalks, ear, chaff, and sheath serve successively as N sinks and sources. Along with physiological data obtained from model plants (Masclaux-Daubresse and Chardon, 2011; Hawkesford, 2017), N remobilization was shown to be involved in N fluxes from leaf to leaf during the vegetative phase (Wendler, 2018) and from leaves to grains during the reproductive phase. Maintaining yield and profit while minimizing environmental effects requires better fertilizer N management that harmonizes N supply with crop demand (Cui et al., 2010).
Studies have shown that there is a possible interaction between grain isotope discrimination in the aboveground dry matter and grain yield; yet studies on this interaction are few, especially during the crop’s main growth stages (Liang et al., 2013; Chen et al., 2016; Sun et al., 2019). As a result, further information about N isotope discrimination during N uptake and absorption is needed to effectively track the remobilization of N that had been accumulated during the early crop growth stages and the post-anthesis phase under field conditions. However, little has been done in terms of using 15N tracing under field conditions to study the effect of N fertilizers on N accumulation and partitioning in spring wheat in the study area. Fewer research efforts have been conducted on how N fertilization affects plant 15N (Wang et al., 2016), with previous studies yielding contradicting results (Khalil et al., 2020; Agisho and Hairat, 2021) along with the comparison of N sources being emphasized more frequently (Asif et al., 2020). We hypothesized that the long-term application of inorganic nitrogen fertilizers would impact the relationship between N supply and crop N uptake, and thereby N use efficiency and grain yield. To test this hypothesis, we conducted a study with 15N-labeled fertilizer applied to microplots within a long-term trial with five N fertilization rates to study N remobilization, uptake, use efficiency, yield, and post-anthesis losses in spring wheat under rainfed conditions in the semi-arid loess plateau. For determining N remobilization, 15N labeling has proven to be a reliable and quantitative method.
Materials and Methods
Site Description
This study was conducted in the Gansu Agricultural University’s Rainfed Experimental Station in Dingxi, Gansu Province, China (35°28′N, 104°44′E, elevation 1971 m above sea level) from 2003 to date, but the current study focused on 2020 to 2021 growing seasons (March to July each year). In crop season, the average minimum and maximum air temperatures at the research location (2020 and 2021) were −22 and 38°C, respectively, while the average precipitation was 390.7 mm yr–1. With 2,480 h of sunshine for the crop season, the average crop season cumulative air temperature > 10°C was 2240°C, and the average annual radiation was 5,930 MJ m–2. The site experienced a rise in the amount of rainfall from May and peaked in August and declined in September (Figure 1). During the growing season, the highest amount of rainfall was recorded in August with 124.9 and 113.5 mm in 2020 and 2021, respectively (Figure 1). Evaporation was three to four times higher than precipitation, with an average of 1,531 mm (coefficient of variation: 24.3%) for the crop season. The soil type at the site is a Huangmian sandy loam (Hocking and Stapper, 2001) and is classified as a Calcaric Cambisol (Hu et al., 2013). Flax (Linum usitatissimum L.) had been the previous crop, and the field has a long history of traditional farming with wheat-pea rotation system. The chemical characteristics of the soil (at 0–30 cm depth) were found to be 3.88 kg ha–1 of total nitrogen (TN), 24.92 kg ha–1 NH4-N, 12.72 kg ha–1 NO3-N, 8.33 pH, 8.3 kg ha–1 total phosphorus (P), 4.53 kg ha–1 accessible P, and 82.68 kg ha–1 total potassium (K).
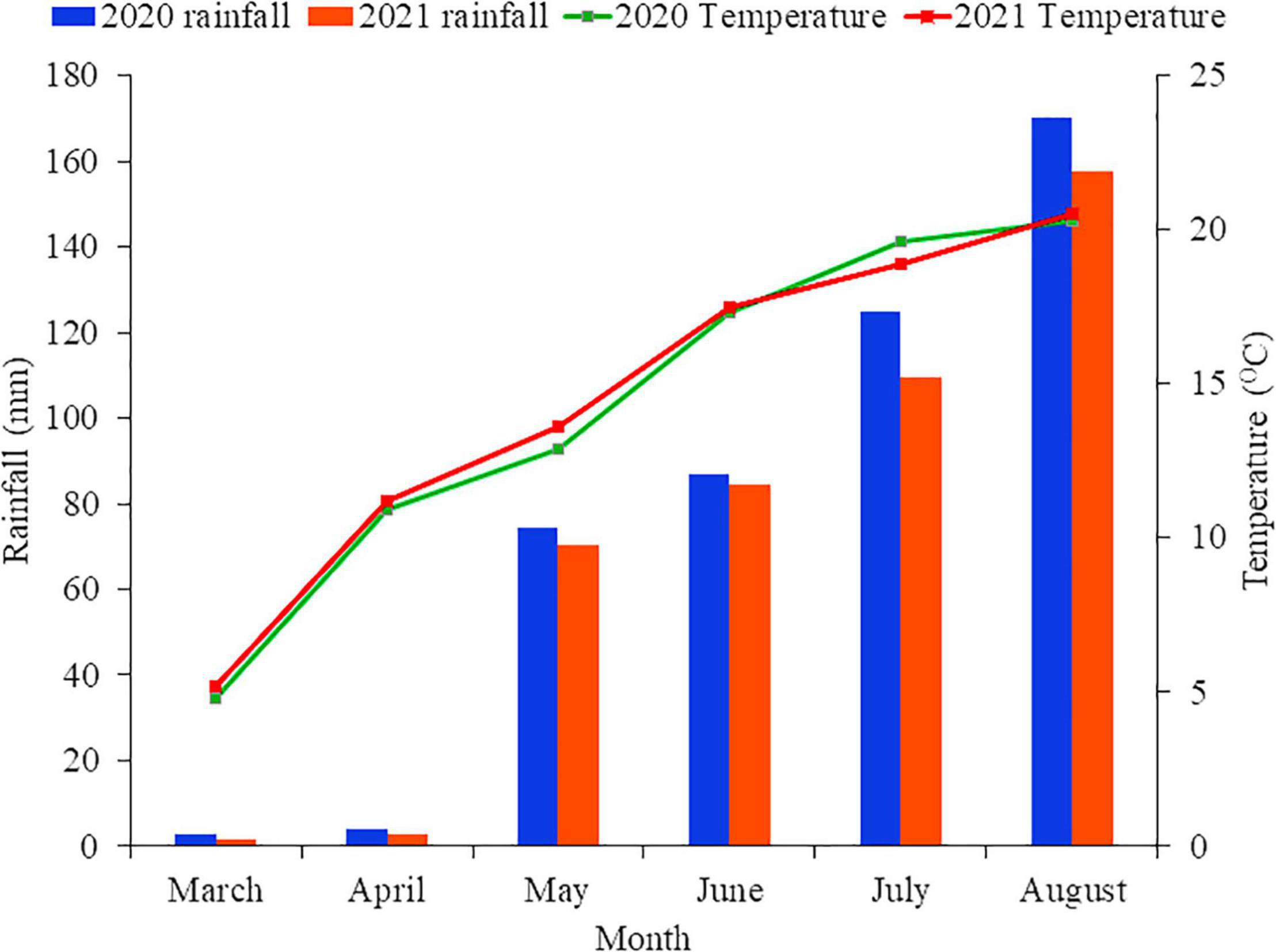
Figure 1. The monthly mean rainfall and temperature during the growing seasons (2020 and 2021) at the experimental site.
Experimental Design and Treatments
The experiment was laid in a randomized complete block design with three replications. The treatments consisted of five N fertilizer (urea) application rates: 0, 52.5, 105, 157.5, and 210 kg ha–1, designated as N1, N2, N3, N4, and N5, respectively. In addition, calcium superphosphate to give 105 kg P2O5 ha–1 was applied. The fertilizers were broadcasted across the entire plot area before planting and subsequently mixed into the 0–20 cm soil layer using rotary tillage.
In mid-March, the high-yielding spring wheat (Triticum aestivum L.) cultivar “Dingxi 38” was planted in rows 20 cm apart at a rate of 187.5 kg seeds ha–1 and was harvested in late July to early August. Each test plot measured 30 m2 (3 m × 10 m). Within each experimental plot, microplots (0.045 m2, 5 plants) were created after seeding. Each plot contained six of these microplots. The microplot was created with a 0.20 m diameter and 0.35 m long polyvinyl chloride (PVC) column that was pushed 0.30 m into the soil at one end of each plot. The microplots, which were spaced 0.5 m apart along the row and between the rows, were used to apply 15N-labeled fertilizer and to measure total growth and nitrogen content. During the growing season, weeds were manually removed, and during the fallow periods, after harvesting, Roundup® (glyphosate, 10%) was used to control weeds according to the manufacturer’s instructions. Pests and diseases were monitored and controlled using best practices in the area.
Plant Sampling and Chemical Analysis
The total growth period was 130 days during 2020 and 128 days during 2021, with six developmental stages recognized (germination, tillering, stem elongation, booting, heading/flowering, and grain-fill/ripening). Ten plants were harvested at 14 days after anthesis (DAA) and separated into ear, leaves, and stem. At maturity, plants were harvested and the biomass was divided into shoots (with chaff) and grains. Dry matter was measured on the different organs of the 10 plants after oven drying for 48 h at 80°C. Before analysis, all samples were ground to a fine powder (<20 mm) in a ball mill. Using the Vapodest 50s (Gerhardt, Königswinter, Germany), the total N concentration in the aboveground biomass was assessed using the Kjeldahl method. Grain yields were recorded for all the treatment after reducing the moisture content to 14%. The N content was determined by multiplying the N concentration with the total biomass (g). The crop’s N uptake was measured at harvest. The amount of N in the straw and grain was multiplied by the dry weight to determine nitrogen uptake.
where Nf is the N derived from fertilizer, Ns is N derived from soil, and 0.3663 atom % is the natural abundance of 15N (Høgh-Jensen and Schjoerring, 2000).
l5N Labeling and Analysis
Separate 15N enrichment analyses were performed on the ear, leaf, and stem portions at anthesis and additionally on the grain and chaff at maturity. At flowering (June 23, each season), we harvested two of the microplots and 1.68 g m–2 N at 10% 15N-excess as urea diluted in 1.5 L water were applied on the soil surface of the four remaining microplots. These micro plots were then cut at ground level 14 DAA, and at final harvest (July 29, each season). The N isotope ratio was measured on the different organs using an automatic element analyzer Sercon Control“Callisto CF-IRMS” Version 30.0.11 (Sercon integra 2) coupled to a mass spectrometer (Elemental microanalysis LTD, United Kingdom). The 15N enrichment of 15N-labeled plant parts fertilized with labeled urea was calculated as an atom percent 15N excess adjusted for background abundance (i.e., 0.366%) (Coplen, 2011).
A simple isotopic mass balance mixing equation was used to estimate the average-weight 15N values of the aboveground portions of spring wheat plants (Thompson, 1996):
where 15N aboveground, 15N ear, 15N leaves, and 15N stem represent the 15N excess (in atom %) for aboveground parts, ear, leaves, and stem of spring wheat, respectively; and F ear, F leaves, and F stem are the total dry weight of spring wheat plant parts ear, leaves, and stem fractions, respectively.
where 15Ni portion is the 15N excess (in atom %) for the upper, middle, and lower portion of the shoot of spring wheat plants, respectively, and Fi leaves and Fi stem are the respective leaves and stem fractions in the same portion of the shoot.
The N harvest index (NHI; kg kg–2) is the ratio of N in grain to total plant N. The ratio of total dry matter to total nitrogen content was used to calculate total nitrogen utilization efficiency (NUE).
Statistical Analysis
The data were analyzed using two-way analysis of variance (ANOVA) as implemented in the SPSS software package (Version 17.0) (SPSS Inc., Chicago, IL, United States) to test whether significant differences existed between the treatments and years. Post hoc mean separations were done with Duncan Multiple Range Test (DMRT) at a 5% probability level. The results are presented in tables and graphs.
Results
Variation in 15N Enrichment in Aboveground Components
The total 15N surplus of leaves and stems was equal among diverse portions of the three shoot portions in both growing seasons. The 15N excess of stems and leaves was highest in the upper portion and lowest in the lower portion at maturity (Figure 2). The 15N excess of stem and leaf increased with increasing N rate, with N5 recording the highest percentage at the maturity stage for the upper portion (23%), middle portion (24%), and lower portion (22%). However, there were no significant differences between N5, N4, and N3. Compared to the control (N1), N fertilization increased 15N excess in the various portions by 50, 38, and 35% at maturity for upper, middle, and lower, respectively (Table 1). Year x N rate interaction had no significant effect on 15N excess on the various portions. The percentage 15N content of the grain, leaves, stem, and chaff was higher in both growing seasons for all N fertilizer rates compared to the control (Figure 2). In all the wheat plant organs studied, increasing N fertilizer rates resulted in a considerable rise in percentage of 15N content. The amount of 15N in plant organs increased at an increasing rate at 14 DAA. However, this trend changed at maturity where there was no significant difference between treatments N3 and N5 in terms of 15N content of the same organs. The control (N1) obtained the least grain 15N content (5%) in wheat at maturity (Figure 2G).
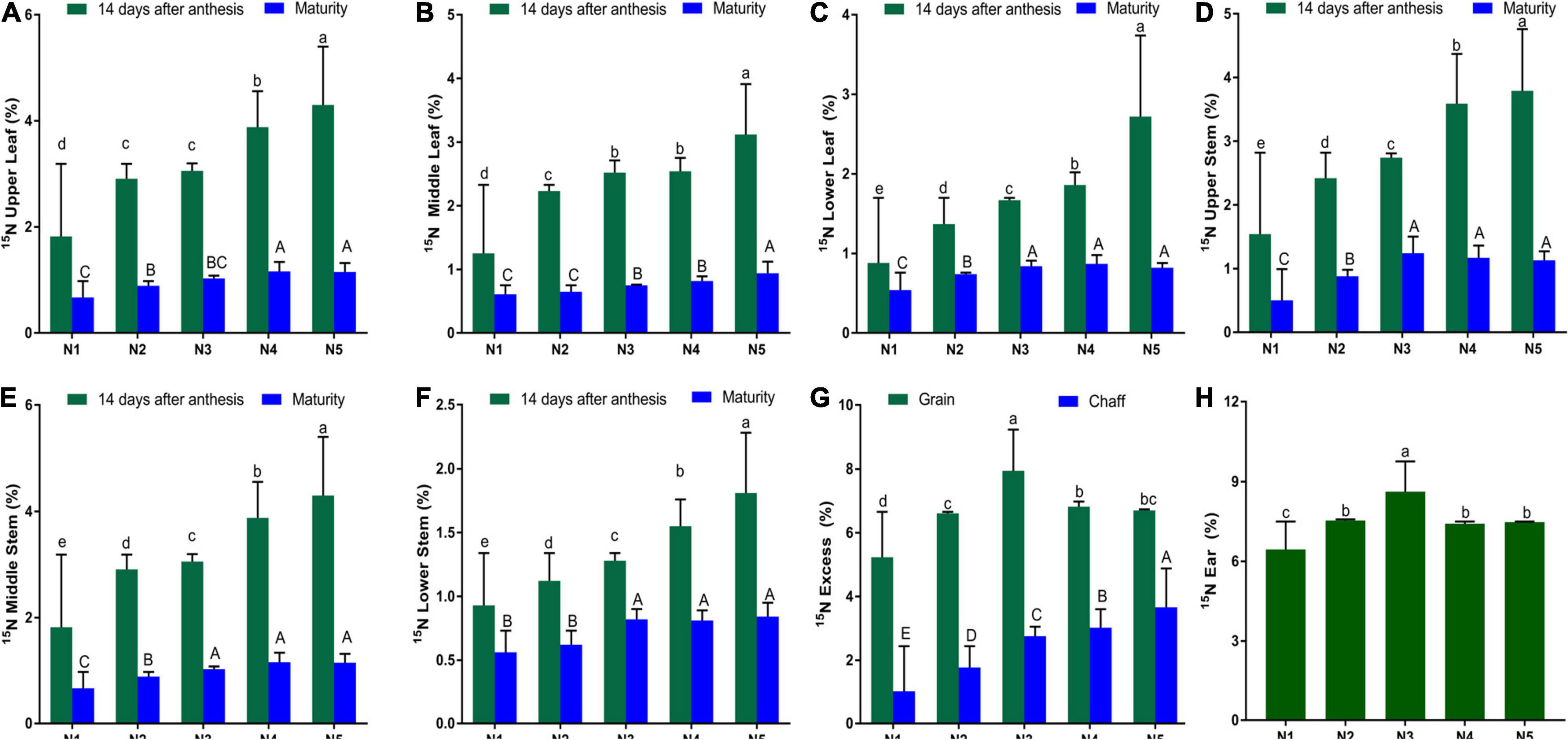
Figure 2. Effect of N fertilization on 15N excess (in atom %) of different spring wheat organs with 15N labeling. (A) Upper leaf. (B) Middle leaf. (C) Lower leaf. (D) Upper stem. (E) Middle stem. (F) Lower stem. (G) Grain and chaff. (H) Ear at 14 days after anthesis. Bars with the same color with the same/a common alphabet indicates no significant difference with Duncan Multiple Range Test at 5% probability level and those with different alphabets mean otherwise. Error bars indicate the standard error.
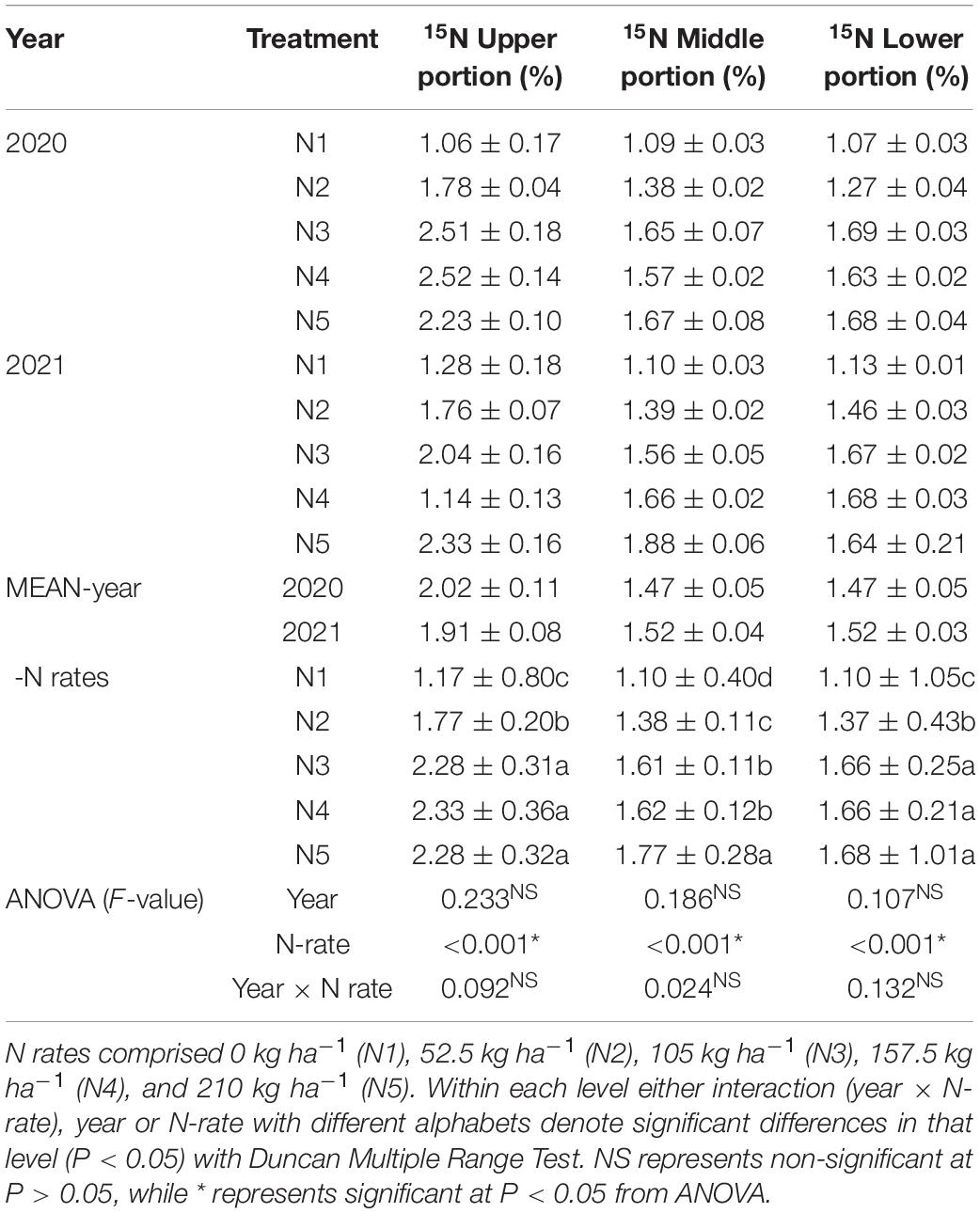
Table 1. Effect of N fertilizer on isotope values of 15N excess (in atom percent) in the upper, middle, and lower portions of shoots in spring wheat.
Effect of N Fertilizer on Crop Biomass and Yield
Spring wheat grain, straw, and aboveground biomass were all affected by the N rate (Table 2). Straw, grain, and aboveground biomass were all higher in the N3 treatment (5.526 ± 0.62, 4.85 ± 0.93, and 10.371 ± 1.55 t ha–1). This was followed by N4 (5.172 ± 0.27, 4.26 ± 0.34, and 9.428 ± 0.61 t ha–1, respectively). The least straw, grain, and above ground biomass were as recorded in the control with values of 4.155 ± 0.75, 2.58 ± 1.34, and 6.738 ± 2.08 t ha–1, respectively (Table 2). Compared to the control, N fertilization in treatments N3–N5 increased straw, grain, and aboveground biomass by 24.77, 48.04, and 35.01%, respectively. The N rate had a considerable impact on the wheat harvest index (HI). The highest HI was recorded with N3 (0.47 ± 0.03 t ha–1) which was not significantly different from N4 and N2 (Table 2). The control (N1) recorded the lowest HI (0.381 ± 0.06 t ha–1). Straw, grain, and total aboveground biomass all increased with an increase in the rate of N fertilization. Overall, aboveground dry-matter production was 9.07 ± 0.49 t ha–1 in 2020 and 8.58 ± 0.13 t ha–1 in 2021 (Table 2). Regardless of the N treatment, the grain yield from fertilized treatments was not significantly different from each other (Figure 3). Although treatment N3 recorded the highest grain yield, the same was not significantly different from the other treatments. When compared to the control, increasing N supply beyond N3 resulted in a negligible yield gain (Figure 3). This suggests that increasing N beyond N3 may not be economical.
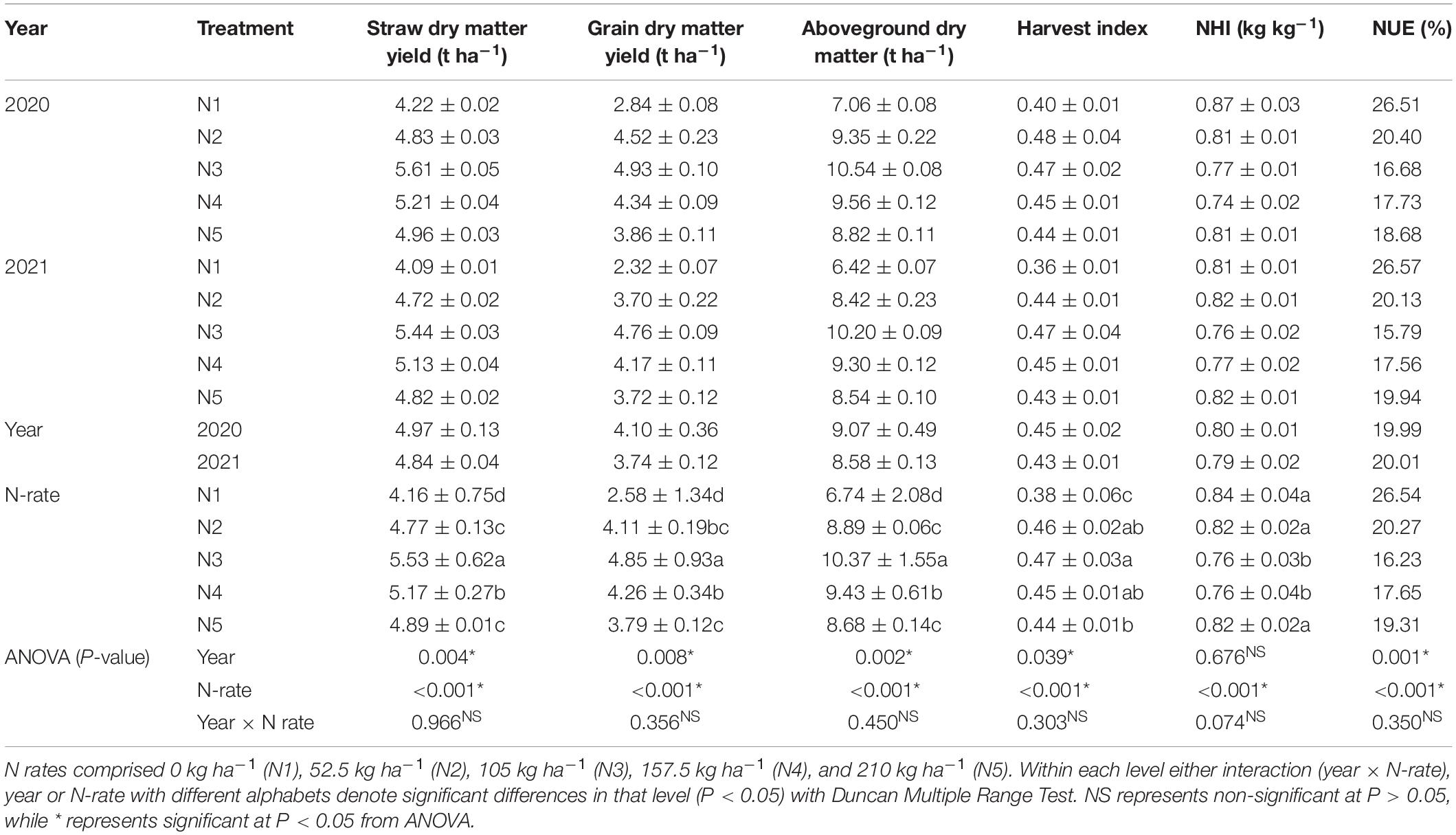
Table 2. Effects of N rate on grain, straw, and aboveground biomass; harvest index (HI), nitrogen harvest index (NHI), and use efficiency (NUE) of spring wheat cultivar.
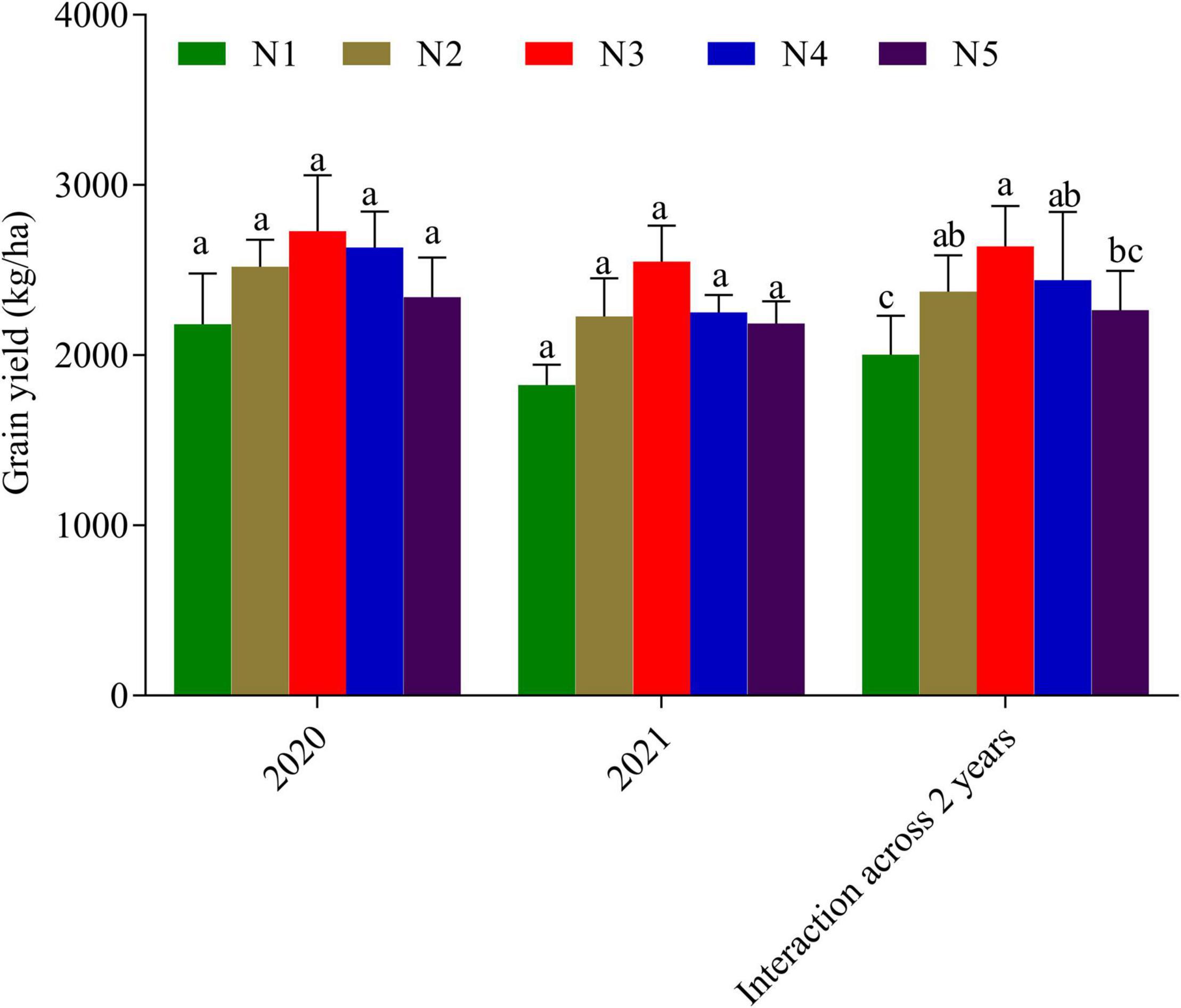
Figure 3. Yield of wheat cultivar fertilized with 0 kg N ha–1 (N1), 52.5 kg N ha–1 (N2), 105 kg N ha–1 (N3), 157 kg N ha– 1 (N4), and 210 kg N ha–1 (N5). Bars in year/interaction across 2 years with the same/a common alphabet indicates no significant difference with Duncan Multiple Range Test at 5% probability level and those with different alphabets mean otherwise. Error bars indicate the standard error.
Effect of N Rates on N Concentration and Uptake
The grain N concentration was higher in the high N treatments at N3, N4, and N5 (19.66 ± 1.57, 18.65 ± 0.55, and 18.00 ± 0.10 g kg–1, respectively) than in N1 (16.25 g kg–1) (Table 3). A similar trend was observed for the concentration of N in straw 5.30 ± 1.55, 4.91 ± 1.17, and 3.12 ± 0.62 g kg–1, respectively, for N3, N4, and N5. With increasing N rate, wheat N concentration and uptake increased (Table 3). The N rate had a significant effect on wheat N uptake for both grain and straw. The N3 treatment had the highest N uptake in straw (29.27 ± 10.38 kg ha–1) and in grain (95.27 ± 23.49 kg ha–1) compared to the other treatments. Wheat uptake of total nitrogen ranged between 7.88 and 29.27 kg ha–1 for straw and 41.85 and 95.27 kg ha–1 for grain (Table 3). Relative to N1, N fertilization increased the N content in straw by 73.1% and in grain by 56.1%.
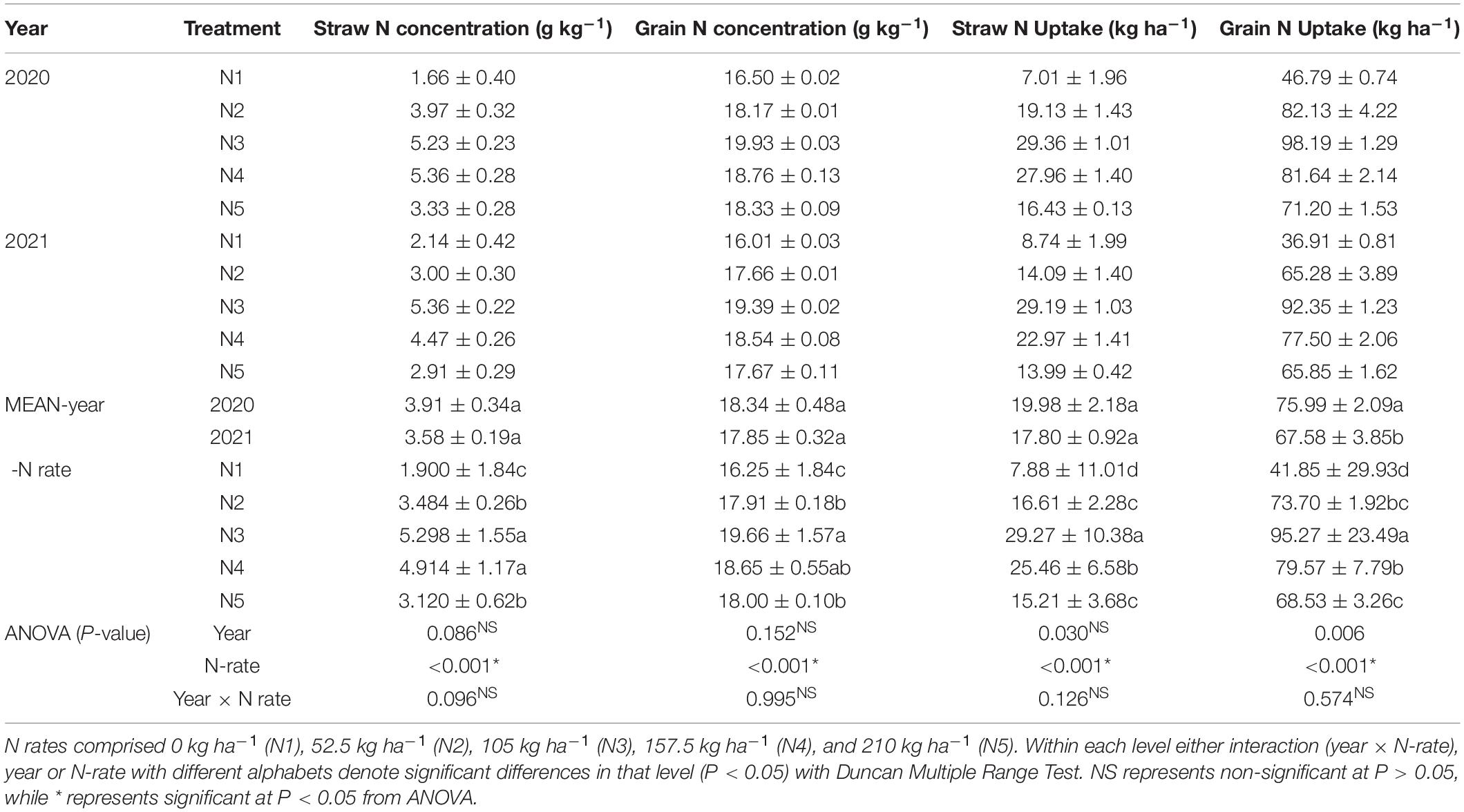
Table 3. Effects of N fertilizer rate on N accumulation and uptake in grain and straw of spring wheat cultivar.
Delivery of 15N and Soil N in Wheat Cultivar
In both seasons, Nf in wheat grain and straw increased while Ns declined as the N rate was increased. The total grain of wheat N derived from 15N fertilizer (44.19–73.84%) increased with the increased N application rate (P < 0.001) (Table 4). Total straw N derived from fertilizer ranged between 7.14 and 15.80%. Wheat grain N derived from soil N ranged between 21.43 and 28.57%, while that of straw N derived from soil N ranged between 6.78 and 13.47%. There was a significant difference between years by N rate interaction for all parameters (Nf Grain, Ns Grain, and Ns straw) except Ns straw (Table 4).
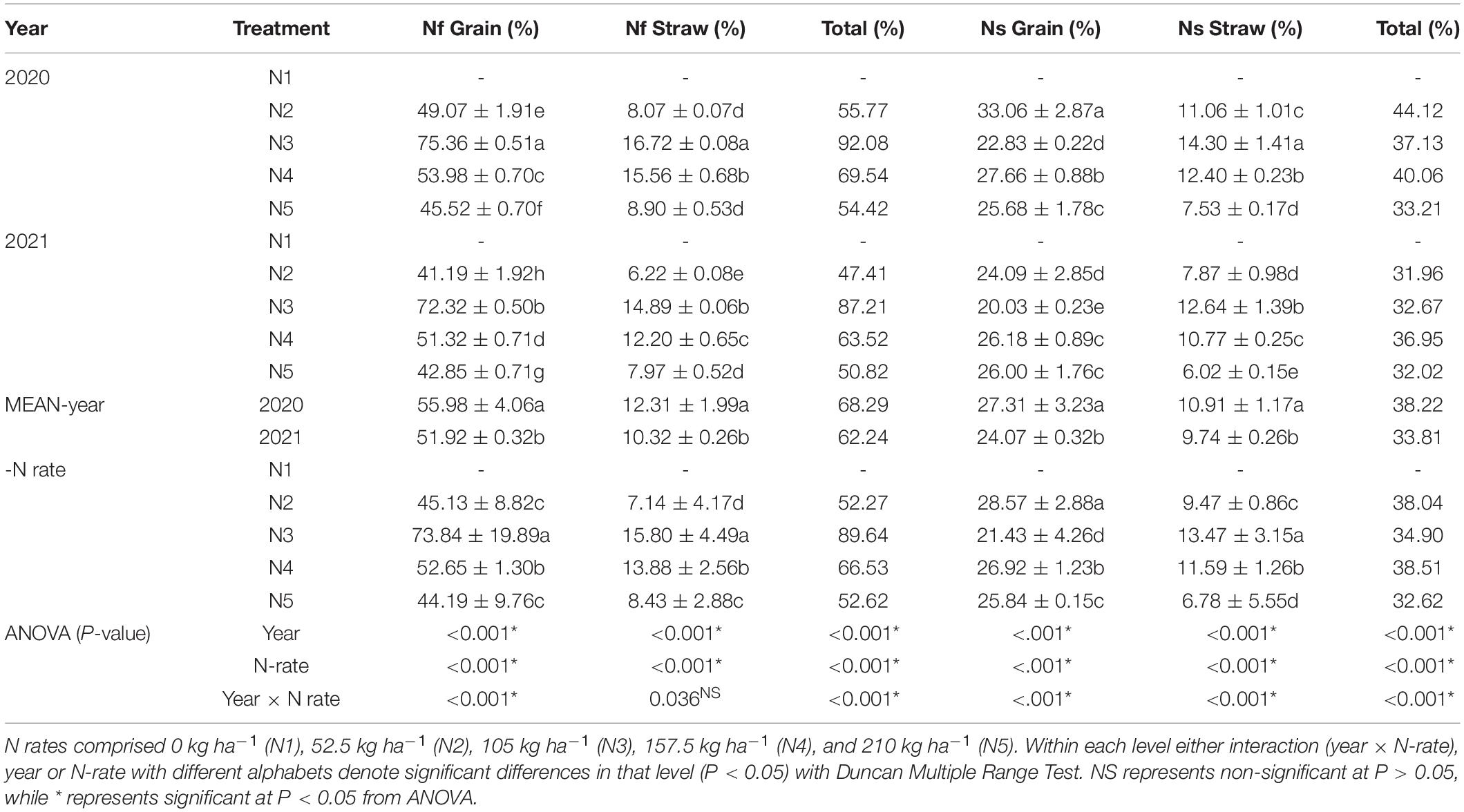
Table 4. Effect of N fertilizer rates on N derived from fertilizer and soil at maturity in 2020–2021.
Remobilization of Labeled Nitrogen Fertilizer (Urea)
The remobilization of labeled N fertilizer differed significantly among the treatments and was affected by N fertilization. The highest remobilization of 15N excess to the grain was recorded by N3 (7.95%), which was significantly different from the other treatments. At maturity, the percentage of 15N excess remobilized to the grain was 5.23, 6.61, 7.95, 6.82, and 6.69% for the treatments N1, N2, N3, N4, and N5, respectively (Table 4). Remobilization of 15N to the ears (grain and chaff) accounted for 48.33% of post-anthesis stored N in N3. Compared to the control N, fertilization increased 15N by 34.2% (Table 4). 15N fertilizer remobilization did not differ from year to year. This implies that the results were relatively consistent in each year. A large amount of early-accumulated 15N was unaccounted for, and it was presumed that it had been lost. Loss of accumulated 15N at 14 DAA increased with increasing N fertilization (Table 4). The highest loss occurred in N5 and N4 with 10.03 ± 3.01 and 7.98 ± 0.97, respectively, representing 38.4 and 34.1% of the total accumulated 15N at 14 DAA.
Discussion
The N distribution in different plant parts, grain yield as well as N uptake of spring wheat fertilized with different amounts of N were studied in this work. Application of N fertilizer increased the 15N content of the various aboveground component of spring wheat cultivar under field growing conditions. During the grain filling stage and maturity, there were considerable differences in 15N excess among wheat aboveground components; the most significant difference, between N1 and N5, was 57.67% for upper leaf and 34.29% for grain (Figure 2). These findings imply that N fertilization was more important for grain filling in wheat plants during the grain filling stage. Our findings are consistent with that of Ba et al. (2020) who reported that the redistribution of labeled N from source organs (flag leaves and stem) to sink organs (ears) was more pronounced in high N wheat plants. These differences were caused by the fact that N remobilization begins earlier in plants under low N fertilization conditions than it does under high N fertilization conditions (Aranjuelo et al., 2013). In spring wheat, the 15N enrichment of shoots and ears varied according to developmental stages.
In our present study, when compared to other aboveground components, spring wheat ears accumulate more 15N during the grain filling stage (14 DAA) (Figure 2G). The concentration of 15N in the ear did not decrease much with time (14 DAA-maturity) as it did in stem and leaves, except under N1 and N2 (Figure 2). Similar effects have been observed in sorghum (He et al., 2022). The ears serve as a strong N sink, impacting N accumulation in the ears throughout grain filling, whereas the shoots provide nutrients to the ears (Aranjuelo et al., 2013; Zhou et al., 2016; Sun et al., 2018).
In our study, the grain yield of the spring wheat cultivar was 11.52–24.13% greater with the N treatment (N2–N5) than with the control (N1). N treatments, on the other hand, had no significant effect on grain yield (Figure 3). When the N rate was increased from 105 to 210 kg ha–1, wheat grain yield did not differ significantly (Figure 3). This could be explained by the fact that the study site has been fertilized continuously for a long time. In some studies, N fertilizer application has proven to boost crop yield (Abad et al., 2004), while excessive and long-term N fertilizer use has resulted in yield reduction (Garrido-Lestache et al., 2005; Wang et al., 2011; Ierna et al., 2015; Agegnehu et al., 2016).
Since water availability is the major factor limiting grain yield, the overall biomass yield in 2020 was higher than in 2021, a result that was linked to less rainfall throughout the growing period in 2021 (Figure 1; Selles and Zentner, 2001). The current study found that increasing the N rate enhanced grain, straw, and aboveground biomass of spring wheat (P < 0.001), as found by other researchers (Wang et al., 2010; Zhao et al., 2014). The spring wheat cultivar had a lower HI in the control. However, there were no significant differences between the N treatments. This may be attributed to the fact that increased N-rate-enhanced wheat development in the early stages than the control treatment (Mahler et al., 1994). Our results appeared to agree with those of Mahjourimajd et al. (2016), who observed that a different N rate supply did not influence HI in an Australian wheat mapping population.
Increasing N application rates improved both grain (17.91–19.66 g kg–1) and straw (3.12–5.29 g kg–1) N concentration of spring wheat in our present field experiment, with the best effect observed in N3 in both growing seasons (Table 3). Chen et al. (2016) reported a similar pattern that while increasing the N application rate from 60 to 240 kg ha–1, the N concentration increased from 2 to 4 and 15 to 22 g kg–1 for straw and grain, respectively. Post-anthesis nitrogen uptake is an important parameter for identifying higher-yield wheat varieties (Monaghan et al., 2001), which allowed researchers to distinguish the contrasting behavior between wheat varieties under varying N supply. Results from our study showed that the N fertilizer rate had a significant effect on N uptake by grain and straw. This may be attributed to enhanced biomass yield and N concentration of the N treatments when compared to the control (Table 3). The lower NUE values recorded at maturity (Table 2) in our present study is an indication that excessive and continuous application of N fertilizer does not only promote vegetative growth but also reduces plants’ ability to utilize nutrients under field conditions. According to the “law of diminishing returns,” a high N application rate indicates a low NUE under normal conditions. Crop N uptake, in addition to fertilizer N rate, is another direct factor that affects NUE (Zhang et al., 2021).
Our findings revealed that, when the rate of applied N increased, the amount of early stored 15N remobilized to both ears and grain increased as also observed by previous research efforts (Palta and Fillery, 1995; Zhou et al., 2018). They suggested that the rate of applied N increased the demand for early stored N, probably because the increased early availability of N increased the size of the sink. The rate at which N was applied affected the loss of pre-anthesis stored nitrogen during post-anthesis. Between 31 and 38% of the 15N in the crop at the grain filling stage (14 DAA) had been lost by maturity at N1–N5, and the entire excess N that remained in the crop was remobilized to the grain, resulting in an absolute increase in pre-anthesis stored N transferred to the grain. Another important finding of this study was that increasing the rate of applied N increased post-anthesis N losses in spring wheat (Table 4). However, N3 compared to the other treatments (N2, N4, and N5) recorded the least N loss, indicating that at this rate the plant can remobilize a greater portion of the applied N (Table 5). Shivay et al. (2020) observed that N application rate and year have an effect on N losses in spring wheat and suggested ammonia volatilization from the aerial parts of the plants as the major source. Higher N losses from the plant are attributable to higher N expenditure during the remobilization process (O’Deen, 1989; Reining et al., 1995; Sra et al., 2004). Losses from the crop need to be explored further, and attention must be drawn to the crop as more than simply a sink for N. The N recovery for wheat in the Middle and Lower Yangtze River Region was reported to be between 33.0 and 49.0% by some researchers (Zhao et al., 2009; Shi et al., 2012). Xiao-Tang et al. (2007) found that the N recovery in wheat was 54% at 120 kg N ha–1 and 32% at 360 kg N ha–1, and it is affected by wheat cultivars, soil fertility, and climate conditions (Wang et al., 2011), a result that is similar to our present study.
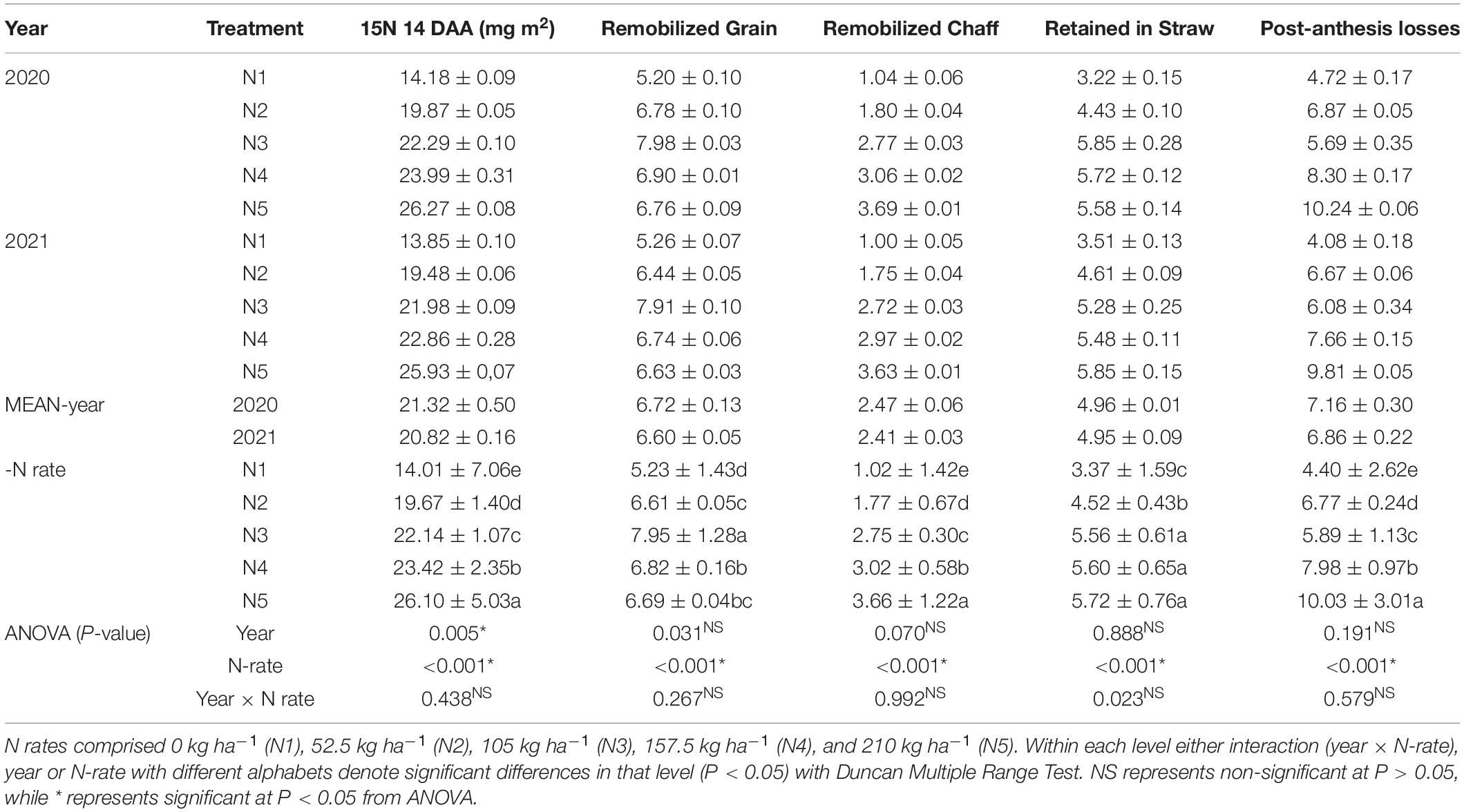
Table 5. Post-anthesis effect of N fertilization on 15N accumulated by the grain filling stage (14 DAA), losses and remobilization to the ears and grain between 14 DAA and maturity for spring wheat cultivar.
Conclusion
In conclusion, this study found that NUE strength in the study area is relatively low and that it has to be improved in spring wheat cultivation by using appropriate fertilization strategies. Our results showed that soil with low fertility (N1 and N2) treatments had the lowest grain yield and straw and grain dry matter which is an indication that N fertilization affects yield and its attributes. Long-term and excessive N application (210 kg ha–1) reduces grain yield and increases N loss in a wheat crop under the conditions of this study. The upper portions of leaves and stem were more strongly enriched with 15N than the lower (aged) ones at both 14 DAA and maturity which is an indication that the upper portion of the leaves, in particular, maybe a great sink and storage for N in the plant during development, as well as a major sink for N freshly taken up post-anthesis. The importance of the ear as a sink for nitrogen is shown by the fact that a greater percentage of total plant nitrogen at 14 DAA was found in the ear, which accounted for a chunk of total dry matter. The results from this study offer useful insights for N application in spring-cultivated wheat in an attempt to reduce the quantity of N fertilizer inputs, thereby costs, and in producing wheat in an environmentally sustainable manner.
Data Availability Statement
The original contributions presented in the study are included in the article/supplementary material, further inquiries can be directed to the corresponding author.
Author Contributions
LL: funding acquisition, conceptualization, and supervision. JW: resources and project administration. ZE: investigation and writing—original draft. JX: methodology. ZE, JW, and MZ: data collection. ZE, SB, and BK: formal analysis. LL, BK, and JP: writing—review and editing. All authors read and approved the final manuscript.
Funding
This research was supported by the Education Science and Technology Innovation Project of Gansu Province (GSSYLXM-02), the National Natural Science Foundation of China (31761143004), and the Young Instructor Fund Project of Gansu Agricultural University (GAU-QDFC-2020-03).
Conflict of Interest
The authors declare that the research was conducted in the absence of any commercial or financial relationships that could be construed as a potential conflict of interest.
Publisher’s Note
All claims expressed in this article are solely those of the authors and do not necessarily represent those of their affiliated organizations, or those of the publisher, the editors and the reviewers. Any product that may be evaluated in this article, or claim that may be made by its manufacturer, is not guaranteed or endorsed by the publisher.
References
Abad, A., Lloveras, J., and Michelena, A. (2004). Nitrogen fertilization and foliar urea effects on durum wheat yield and quality and on residual soil nitrate in irrigated Mediterranean conditions. Field Crops Res. 87, 257–269.
Agegnehu, G., Nelson, P. N., and Bird, M. I. (2016). Crop yield, plant nutrient uptake and soil physicochemical properties under organic soil amendments and nitrogen fertilization on Nitisols. Soil Tillage Res. 160, 1–13.
Agisho, H. A., and Hairat, S. (2021). Understanding drought stress in plants for facing challenges and management in wheat breeding: a review. Plant Cell Biotechnol. Mol. Biol. 22, 140–156.
Alpuerto, J. B., Brasier, K. G., Griffey, C. A., Thomason, W. E., and Fukao, T. (2021). Accelerated senescence and nitrogen remobilization in flag leaves enhance nitrogen use efficiency in soft red winter wheat. Plant Prod. Sci. 24, 490–504. doi: 10.3390/plants10010165
Aranjuelo, I., Cabrera-Bosquet, L., Araus, J. L., and Nogués, S. (2013). Carbon and nitrogen partitioning during the post-anthesis period is conditioned by N fertilisation and sink strength in three cereals. Plant Biol. (Stuttg) 15, 135–143. doi: 10.1111/j.1438-8677.2012.00593.x
Asif, M., Zora, S., Ceylan, Y., Rehman, R., and Ozturk, L. (2020). Nitrogen supply in combination of nitrate and ammonium enhances harnessing of elevated atmospheric CO2 through improved nitrogen and carbon metabolism in wheat (Triticum aestivum). Crop Pasture Sci. 71, 101–112.
Ba, Q., Zhang, L., Chen, S., Li, G., and Wang, W. (2020). Effects of foliar application of magnesium sulfate on photosynthetic characteristics, dry matter accumulation and its translocation, and carbohydrate metabolism in grain during wheat grain filling. Cereal Res. Commun. 48, 157–163. doi: 10.1007/s42976-020-00026-z
Berg, J., Hendrix, P., Cheng, W., and Dillard, A. (1991). A labeling chamber for 13C enrichment of plant tissue for decomposition studies. Agric. Ecosyst. Environ. 34, 421–425. doi: 10.1016/0167-8809(91)90125-h
Broberg, M. C., Xu, Y., Feng, Z., and Pleijel, H. (2021). Harvest index and remobilization of 13 elements during wheat grain filling: experiences from ozone experiments in China and Sweden. Field Crops Res. 271:108259. doi: 10.1016/j.fcr.2021.108259
Chen, Z., Wang, H., Liu, X., Liu, Y., Gao, S., and Zhou, J. (2016). The effect of N fertilizer placement on the fate of urea-15N and yield of winter wheat in southeast China. PLoS One 11:e0153701. doi: 10.1371/journal.pone.0153701
Coplen, T. B. (2011). Guidelines and recommended terms for expression of stable-isotope-ratio and gas-ratio measurement results. Rapid Commun. Mass Spectr. 25, 2538–2560. doi: 10.1002/rcm.5129
Cui, Z., Chen, X., and Zhang, F. (2010). Current nitrogen management status and measures to improve the intensive wheat–maize system in China. Ambio 39, 376–384. doi: 10.1007/s13280-010-0076-6
Dong, Y.-P., Liu, X.-Y., Sun, X.-C., Song, W., Zheng, X.-D., Li, R., et al. (2017). Inter-species and intra-annual variations of moss nitrogen utilization: implications for nitrogen deposition assessment. Environ. Pollut. 230, 506–515. doi: 10.1016/j.envpol.2017.06.058
Gaju, O., Allard, V., Martre, P., Snape, J., Heumez, E., Legouis, J., et al. (2011). Identification of traits to improve the nitrogen-use efficiency of wheat genotypes. Field Crops Res. 123, 139–152. doi: 10.1016/j.fcr.2011.05.010
Garrido-Lestache, E., López-Bellido, R. J., and López-Bellido, L. (2005). Durum wheat quality under Mediterranean conditions as affected by N rate, timing and splitting, N form and S fertilization. Eur. J. Agron. 23, 265–278. doi: 10.1016/j.eja.2004.12.001
Handley, L. L., Scrimgeour, C., and Raven, J. (2020). “15N at natural abundance,” in Stable Isotopes: The Integration of Biological, Ecological and Geochemical Processes, ed. H. Griffiths (New York, NY: Garland Science), 464, 89.
Hawkesford, M. J. (2017). Genetic variation in traits for nitrogen use efficiency in wheat. J. Exp. Bot. 68, 2627–2632. doi: 10.1093/jxb/erx079
He, X., Zhang, L., Hu, Y., Liang, Z., Zhang, W., Meng, Q., et al. (2022). Pursuing sustainable high-yield winter wheat via pre-anthesis dry matter and nitrogen accumulation by optimizing nitrogen management. Agron. J. 1–17. doi: 10.1002/agj2.20967 [Epub ahead of print].
Hocking, P., and Stapper, M. (2001). Effects of sowing time and nitrogen fertiliser on canola and wheat, and nitrogen fertiliser on Indian mustard. I. Dry matter production, grain yield, and yield components. Australian J. Agric. Res. 52, 623–634. doi: 10.1071/ar00113
Høgh-Jensen, H., and Schjoerring, J. K. (2000). Below-ground nitrogen transfer between different grassland species: direct quantification by 15N leaf feeding compared with indirect dilution of soil 15N. Plant Soil 227, 171–183.
Hu, H., Ning, T., Li, Z., Han, H., Zhang, Z., Qin, S., et al. (2013). Coupling effects of urea types and subsoiling on nitrogen–water use and yield of different varieties of maize in northern China. Field Crops Res. 142, 85–94. doi: 10.1016/j.fcr.2012.12.001
Hu, Y., and Guy, R. D. (2020). Isotopic composition and concentration of total nitrogen and nitrate in xylem sap under near steady-state hydroponics. Plant Cell Environ. 43, 2112–2123. doi: 10.1111/pce.13809
Hussain, S., Khaliq, A., Noor, M. A., Tanveer, M., Hussain, H. A., Hussain, S., et al. (2020). “Metal toxicity and nitrogen metabolism in plants: an overview,” in Carbon and Nitrogen Cycling in Soil, eds R. Datta, R. Meena, S. Pathan, and M. Ceccherini (Singapore: Springer), 221–248. doi: 10.1007/978-981-13-7264-3_7
Ierna, A., Lombardo, G., and Mauromicale, G. (2015). Yield, nitrogen use efficiency and grain quality in durum wheat as affected by nitrogen fertilization under a mediterranean environment. Exp. Agric. 52, 314–329. doi: 10.1017/s0014479715000113
Khalil, A., Murchie, E., and Mooney, S. (2020). Quantifying the influence of water deficit on root and shoot growth in wheat using X-ray Computed Tomography. AoB Plants 12:laa036. doi: 10.1093/aobpla/plaa036
Lazcano, C., Zhu-Barker, X., and Decock, C. (2021). Effects of organic fertilizers on the soil microorganisms responsible for N2O emissions: a review. Microorganisms 9:983. doi: 10.3390/microorganisms9050983
Liang, B., Yang, X., Murphy, D. V., He, X., and Zhou, J. (2013). Fate of 15 N-labeled fertilizer in soils under dryland agriculture after 19 years of different fertilizations. Biol. Fertil. Soils 49, 977–986. doi: 10.1007/s00374-013-0789-3
Mahjourimajd, S., Kuchel, H., Langridge, P., and Okamoto, M. (2016). Evaluation of Australian wheat genotypes for response to variable nitrogen application. Plant Soil 399, 247–255. doi: 10.1007/s11104-015-2694-z
Mahler, R. L., Koehler, F. E., and Lutcher, L. (1994). Nitrogen source, timing of application, and placement: effects on winter wheat production. Agron. J. 86, 637–642. doi: 10.2134/agronj1994.00021962008600040010x
Masclaux-Daubresse, C., and Chardon, F. (2011). Exploring nitrogen remobilization for seed filling using natural variation in Arabidopsis thaliana. J. Exp. Bot. 62, 2131–2142. doi: 10.1093/jxb/erq405
Meng, F., Dungait, J. A., Xu, X., Bol, R., Zhang, X., and Wu, W. (2017). Coupled incorporation of maize (Zea mays L.) straw with nitrogen fertilizer increased soil organic carbon in Fluvic Cambisol. Geoderma 304, 19–27. doi: 10.1016/j.geoderma.2016.09.010
Monaghan, J. M., Snape, J. W., Chojecki, A. J. S., and Kettlewell, P. S. (2001). The use of grain protein deviation for identifying wheat cultivars with high grain protein concentration and yield. Euphytica 122, 309–317. doi: 10.1016/j.fcr.2020.107778
O’Deen, W. (1989). Wheat volatilized ammonia and resulting nitrogen isotopic fractionation. Agron. J. 81, 980–985. doi: 10.2134/agronj1989.00021962008100060027x
Palta, J., and Fillery, I. (1995). N application enhances remobilization and reduces losses of pre-anthesis N in wheat grown on a duplex soil. Australian J. Agric. Res. 46, 519–531.
Prey, L., Hu, Y., and Schmidhalter, U. (2019). Temporal dynamics and the contribution of plant organs in a phenotypically diverse population of high-yielding winter wheat: evaluating concepts for disentangling yield formation and nitrogen use efficiency. Front. Plant Sci. 10:1295. doi: 10.3389/fpls.2019.01295
Qian, J., Jin, W., Hu, J., Wang, P., Wang, C., Lu, B., et al. (2021). Stable isotope analyses of nitrogen source and preference for ammonium versus nitrate of riparian plants during the plant growing season in Taihu Lake Basin. Sci. Total Environ. 763:143029. doi: 10.1016/j.scitotenv.2020.143029
Randive, K., Raut, T., and Jawadand, S. (2021). An overview of the global fertilizer trends and India’s position in 2020. Mineral Econ. 34, 371–384.
Reining, E., Merbach, W., and Knof, G. (1995). 15N distribution in wheat and chemical fractionation of root-borne 15N in the soil. Isotopes Env. Health Stud. 31, 345–349.
Selles, F., and Zentner, R. (2001). Grain protein as a post-harvest index of N sufficiency for hard red spring wheat in the semiarid prairies. Canadian J. Plant Sci. 81, 631–636.
Shan, H., Lu, C., Zhang, J., Wang, J., and Xu, M. (2012). Effect of maize straw applied with N fertilizer on nitrogen adsorption of winter wheat under different soil fertility. Plant Nutr. Fertil. Sci. 18, 35–41.
Shi, Z., Jing, Q., Cai, J., Jiang, D., Cao, W., and Dai, T. (2012). The fates of 15N fertilizer in relation to root distributions of winter wheat under different N splits. Eur. J. Agron. 40, 86–93.
Shivay, Y. S., Prasad, R., and Mandi, S. (2020). Plants, fertilizer nitrogen, and environment: an overview. Int. J. Plant Environ. 6, 98–102.
Sra, A. K., Hu, Y., Martin, G. E., Snow, D. D., Ribbe, M. W., and Kohen, A. (2004). Competitive 15N kinetic isotope effects of nitrogenase-catalyzed dinitrogen reduction. J. Am. Chem. Soc. 126, 12768–12769. doi: 10.1021/ja0458470
Sun, Z., Chen, Q., Han, X., Bol, R., Qu, B., and Meng, F. (2018). Allocation of photosynthesized carbon in an intensively farmed winter wheat-soil system as revealed by (14)CO(2) pulse labelling. Sci. Rep. 8:3160. doi: 10.1038/s41598-018-21547-y
Sun, Z., Wu, S., Zhu, B., Zhang, Y., Bol, R., Chen, Q., et al. (2019). Variation of 13C and 15N enrichments in different plant components of labeled winter wheat (Triticum aestivum L.). PeerJ 7:e7738. doi: 10.7717/peerj.7738
Tahir, M. M., Recous, S., Aita, C., Pfeifer, I. C., Chaves, B., and Giacomini, S. J. (2018). Field 13C pulse labeling of pea, wheat, and vetch plants for subsequent root and shoot decomposition studies. Revista Brasileira de Ciência do Solo 42:e0160449.
Thompson, R. (1996). Pulse-labelling a cover crop with 13 C to follow its decomposition in soil under field conditions. Plant Soil 180, 49–55. doi: 10.1007/bf00015410
Wang, D., Xu, Z., Zhao, J., Wang, Y., and Yu, Z. (2011). Excessive nitrogen application decreases grain yield and increases nitrogen loss in a wheat–soil system. Acta Agric. Scandinavica Section B-Soil Plant Sci. 61, 681–692. doi: 10.1080/09064710.2010.534108
Wang, Q., Li, F., Zhao, L., Zhang, E., Shi, S., Zhao, W., et al. (2010). Effects of irrigation and nitrogen application rates on nitrate nitrogen distribution and fertilizer nitrogen loss, wheat yield and nitrogen uptake on a recently reclaimed sandy farmland. Plant Soil 337, 325–339. doi: 10.1007/s11104-010-0530-z
Wang, S., Luo, S., Yue, S., Shen, Y., and Li, S. (2016). Fate of 15 N fertilizer under different nitrogen split applications to plastic mulched maize in semiarid farmland. Nutr. Cycl. Agroecosyst. 105, 129–140. doi: 10.1007/s10705-016-9780-3
Wendler, N. (2018). “The genomes of the secondary and tertiary gene pools of barley,” in The Barley Genome, eds N. Stein and G. J. Muehlbauer (Berlin: Springer), 337–344. doi: 10.1007/978-3-319-92528-8_18
Xiao-Tang, J., Xue-Jun, L., Jia-Rong, P., and Zhang, F.-S. (2007). Fate of 15N-labeled urea under a winter wheat-summer maize rotation on the North China Plain. Pedosphere 17, 52–61.
Xu, X., An, T., Zhang, J., Sun, Z., Schaeffer, S., and Wang, J. (2019). Transformation and stabilization of straw residue carbon in soil affected by soil types, maize straw addition and fertilized levels of soil. Geoderma 337, 622–629. doi: 10.1016/j.geoderma.2018.08.018
Yan, B.-F., Nguyen, C., Pokrovsky, O., Candaudap, F., Coriou, C., Bussiere, S., et al. (2018). Contribution of remobilization to the loading of cadmium in durum wheat grains: impact of post-anthesis nitrogen supply. Plant Soil 424, 591–606.
Zhang, X., Li, F., Ding, Y., Ma, Q., Yi, Y., Zhu, M., et al. (2021). Transcriptome analysis of two near-isogenic lines with different NUE under normal nitrogen conditions in wheat. Biology 10:787. doi: 10.3390/biology10080787
Zhao, S., Qiu, S., Cao, C., Zheng, C., Zhou, W., and He, P. (2014). Responses of soil properties, microbial community and crop yields to various rates of nitrogen fertilization in a wheat–maize cropping system in north-central China. Agric. Ecosyst. Environ. 194, 29–37.
Zhao, X., Xie, Y.-X., Xiong, Z.-Q., Yan, X.-Y., Xing, G.-X., and Zhu, Z.-L. (2009). Nitrogen fate and environmental consequence in paddy soil under rice-wheat rotation in the Taihu lake region, China. Plant Soil 319, 225–234.
Zheng, L., Pei, J., Jin, X., Schaeffer, S., An, T., and Wang, J. (2018). Impact of plastic film mulching and fertilizers on the distribution of straw-derived nitrogen in a soil-plant system based on 15N–labeling. Geoderma 317, 15–22.
Zheng, X., Yu, Z., Zhang, Y., and Shi, Y. (2020). Nitrogen supply modulates nitrogen remobilization and nitrogen use of wheat under supplemental irrigation in the North China Plain. Sci. Rep. 10:3305. doi: 10.1038/s41598-020-59877-5
Zhou, B., Serret, M. D., Elazab, A., Bort Pie, J., Araus, J. L., Aranjuelo, I., et al. (2016). Wheat ear carbon assimilation and nitrogen remobilization contribute significantly to grain yield. J. Integr. Plant Biol. 58, 914–926. doi: 10.1111/jipb.12478
Keywords: N remobilization, harvest index, 15N labeling, nitrogen uptake, sustainable production
Citation: Effah Z, Li L, Xie J, Karikari B, Wang J, Zeng M, Wang L, Boamah S and Padma Shanthi J (2022) Post-anthesis Relationships Between Nitrogen Isotope Discrimination and Yield of Spring Wheat Under Different Nitrogen Levels. Front. Plant Sci. 13:859655. doi: 10.3389/fpls.2022.859655
Received: 21 January 2022; Accepted: 21 February 2022;
Published: 17 March 2022.
Edited by:
Sajid Fiaz, The University of Haripur, PakistanReviewed by:
Sumera Anwar, University of Lahore, PakistanAyman El Sabagh, Kafrelsheikh University, Egypt
Copyright © 2022 Effah, Li, Xie, Karikari, Wang, Zeng, Wang, Boamah and Padma Shanthi. This is an open-access article distributed under the terms of the Creative Commons Attribution License (CC BY). The use, distribution or reproduction in other forums is permitted, provided the original author(s) and the copyright owner(s) are credited and that the original publication in this journal is cited, in accordance with accepted academic practice. No use, distribution or reproduction is permitted which does not comply with these terms.
*Correspondence: Lingling Li, bGlsbEBnc2F1LmVkdS5jbg==