- 1Hubei Collaborative Innovation Center for Grain Industry, Yangtze University, Jingzhou, China
- 2Agriculture College, Yangtze University, Jingzhou, China
- 3Tasmanian Institute of Agriculture, University of Tasmania, Burnie, TAS, Australia
- 4Hainan Key Laboratory for Sustainable Utilization of Tropical Bioresource, College of Tropical Crops, Hainan University, Haikou, China
- 5Department of Agronomy, The University of Haripur, Haripur, Pakistan
Rice–wheat (RW) cropping systems are integral to global food security. Despite being practiced for decades, Chinese RW cropping systems often suffer from low productivity and poor nitrogen use efficiency (NUE), reflecting management approaches that are not well-contextualized to region and season. Here, we develop the best management guides for N fertilizer in RW systems that are designed to help raise the productivity, NUE, and environmental sustainability of winter wheat over the long term. 2-year field experiments were conducted with four N fertilizer rates (0, 135, 180, and 225 kg N ha–1), allowing contrasts of yields, soil moisture, and NUE of wheat in RW in the humid climates zones on the Jianghan Plain. We compared RW systems with soybean/maize dryland wheat (DW) systems that are similarly endemic to China: after soybean/maize is harvested, soils are often drier compared with moisture content following rice harvest. With high seasonal N application rates (180–225 kg N ha–1), wheat crop yields increased by 24% in RW which were greater than comparable yields of wheat in DW, mainly due to greater kernels per spike in the former. Across treatments and years, N accumulation in plant tissue and kernel dry matter of DW was higher than that in RW, although mean agronomic efficiency of nitrogen (AEN) and physiological efficiency of nitrogen (PEN) of RW systems were greater. As N application rates increased from 135 to 225 kg ha–1, AEN and PEN of DW decreased but changed little for RW. Soil ammonium N was much lower than that of nitrate N; changes in NH4+ and NO3– as a consequence of increasing N fertilization were similar for RW and DW. We recommend that tactical application of N fertilizer continue seasonally until midgrain filling for both the DW and RW systems. At fertilization rates above 180 kg N ha–1, yield responses disappeared but nitrate leaching increased significantly, suggesting declining environmental sustainability above this N ceiling threshold. Collectively, this study elicits many functional and agronomic trade-offs between yields, NUE, and environmental sustainability as a function of N fertilization. Our results show that yield and NUE responses measured as part of crop rotations are both more robust and more variable when derived over multiple seasons, management conditions, and sites.
Introduction
Wheat cropping systems endemic to the Jianghan Plain include dryland wheat (DW)–soybean- and rice paddy-dryland rotations, wheat being sown in winter and rice in summer. The Jianghan Plain belongs to the subtropical monsoon humid climate zone, and while rainfall can be spuriously distributed throughout the wheat growing season, probabilistically, rainfall is more prevalent between jointing and maturity (Wang et al., 2013; Li et al., 2021). Driven by differences in climate, soil, geography, agronomy, and other socio-cultural practices, yields of rice stubble wheat and DW systems vary widely (Yang et al., 2021; Yan et al., 2022). In view of the diversity in management systems across the Jianghan Plain, the development of practicable, productive, and environmentally responsible fertilization strategies is of utmost importance to ensuring the long-term sustainability of agri-food systems in the region.
In itself, crop fertilization with nitrogenous compounds is one of the most powerful tactical tools farmers have at their disposal for substantially increasing growth and yield (Rawnsley et al., 2019; Shah et al., 2021a). Double-cropping and high-intensity crop rotations conducted on a year-round basis (Zhao et al., 2021) can contribute to excessive nitrogen use and depletion, resulting in N mining and suboptimal yields (Kodzwa et al., 2020). On the other hand, excessive and/or poorly timed nitrogen fertilizer application results in both the lower nitrogen use efficiency (NUE) and significant N losses to the environment (Fageria and Baligar, 2005; Yousaf et al., 2016; Christie et al., 2018, 2020), including nitrous oxide emissions (a potent greenhouse gas), nitrate runoff, and leaching into groundwater (Harrison et al., 2014, 2016; Alcock et al., 2015; Shah et al., 2017). Scientific nitrogen fertilizer management advice should carefully consider soil nitrogen supply as a function of crop type and rotation, soil type, existing N levels, and organic matter (Bilotto et al., 2021), as well as climatic and whole farm management (Harrison et al., 2012a,b). Collectively, these factors determine the level of farm intensification and profitability (Ho et al., 2014; Harrison et al., 2017). Bu et al. (2019) contend that in rice-rapeseed rotations, soil nitrogen supply in the early stage of rapeseed is low; however, nitrogen supply of the cotton oil rotation soil at the later stage is greater: optimal N management approaches should thus increase toward later phases for these crop types, considering soil nitrogen supply and crop N demand. In actual production, the supply of soil nitrogen to crop nutrients is affected by climatic conditions (rainfall and temperature), soil (texture and pH), and cultivation management methods (rotation and tillage) (Harrison et al., 2009; Christie et al., 2014).
Both the seasonal rainfall distribution and the quantum of individual rainfall events have a large bearing on agronomic outcomes (Chang-Fung-Martel et al., 2017). Under humid climatic conditions, a single high rainfall event or continuous rainfall for multiple days will cause the nitrate in the soil to runoff with the water surface or leach into the deep soil layer, resulting in the loss of nitrogen (Christie et al., 2014; Rawnsley et al., 2019; Hess et al., 2020; Smith et al., 2021). Soil moisture is one of the foremost abiotic factors limiting wheat yield potential and nitrogen absorption (Harrison et al., 2011). Seasonal alternation of water and drought increases soil organic matter and soil water retention performance, although excess soil water will affect the nitrification of ammonium nitrogen in the soil (Yang et al., 2019; Smith et al., 2021). Paddy-upland rice rotations promote the transformation of nitrogen, influencing subsequent crop nutrient absorption; choice of crop rotation, therefore, affects the response of the collective system to nitrogen fertilization (Ibrahim et al., 2018; Ren et al., 2019). The actual effect of optimized nitrogen fertilizer management varies with soil and climatic conditions depending on contextual genotype by environment by management interactions (Liu et al., 2020; Shah et al., 2021b).
While much research has focused on approaches for improving wheat yield and nitrogen-use efficiency (NUE), this research has primarily focused on DW or rice stubble wheat single planting system. There are few comprehensive comparisons of the yield and NUE of the two. Therefore, this study carried out 2 years field experiment in the humid climatic of the Jianghan Plain to analyze the differences in soil moisture in the growing season of DW and RW and to analyze the difference in response of DW and RW yield, NUE, and soil nitrogen content to increasing nitrogen fertilizer.
Materials and Methods
Field Experimental Details
Field experiments were conducted at the experimental farm at Yangtze University (30°36′ N, 112°08′ E, 34 m asl) in Jingzhou, Hubei Province, China in 2017–2018 and 2020–2021.
This experiment was conducted with two cropping rotation systems in both the cases, we only focused on the wheat within each rotation (Figure 1):
Dryland crop rotation system: The summer crops are dryland crops, mainly corn and soybeans, and winter wheat is DW.
Rice-wheat rotation system: The summer crop is rice and winter wheat is rice stubble wheat (RW). This cropping rotation system has a unique dry–wet cycle soil regime.
The daily mean temperature and rainfall during the wheat-growing season are shown in Figure 2. Meteorological data were collected during each growing season using an automatic weather station adjacent to experimental trials conducted here.
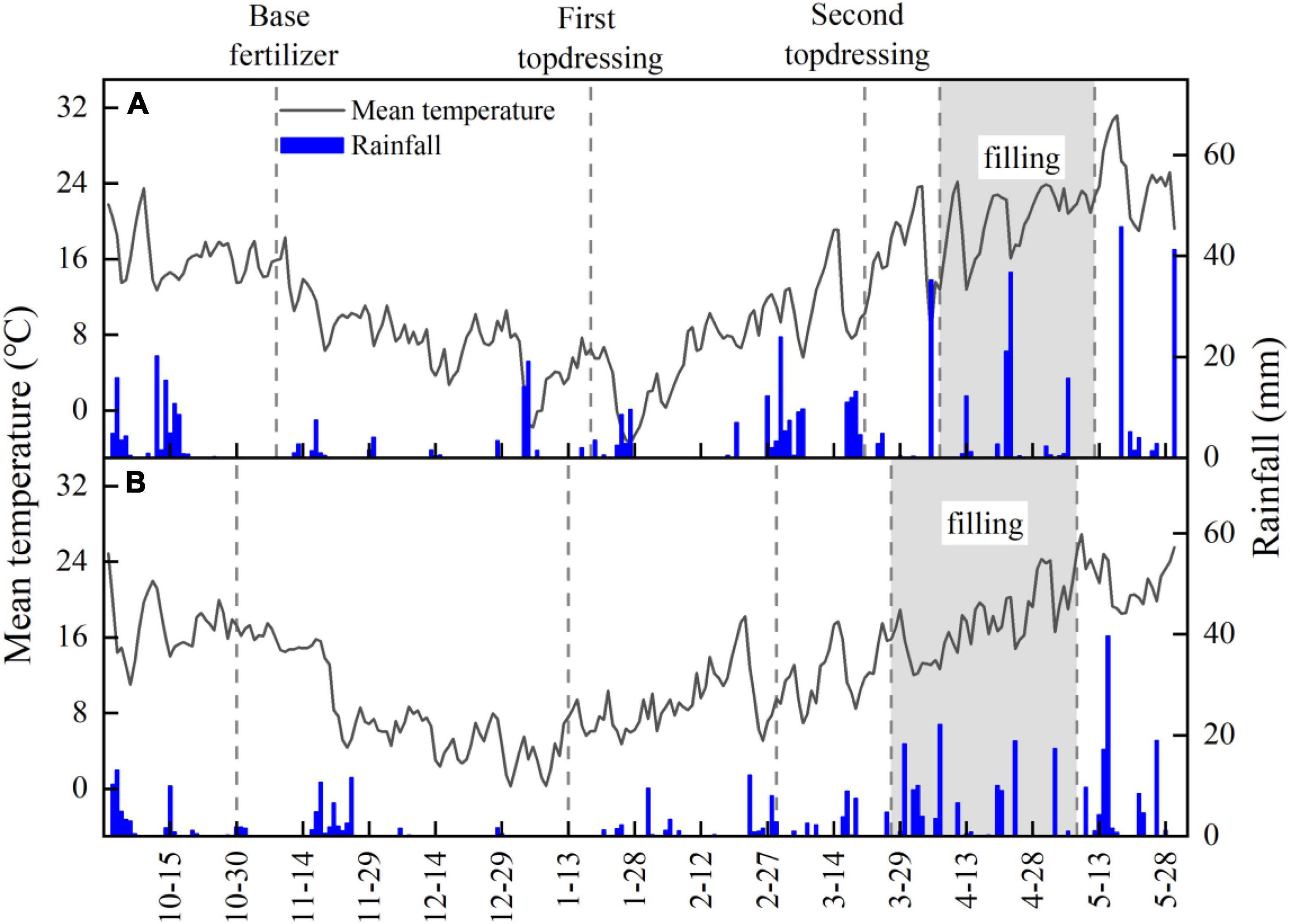
Figure 2. 2017–2018 (A) and 2020–2021 (B) the daily mean temperature and rainfall during the whole growth period of wheat in the experimental area.
Before beginning the experiment, both the cropping systems had been used primarily for wheat production for more than 10 years. The tillage soil layer (0–20 cm) of the two cropping systems was as follows. Soil organic matter 11.00 g kg–1, available nitrogen 82.03 mg kg–1, available phosphorus 15.20 mg kg–1, available potassium 51.11 mg kg–1, and pH 7.8 in DW, and soil organic matter 12.37 g kg–1, available nitrogen 51.22 mg kg–1, available phosphorus 12.07 mg kg–1, available potassium 52.74 mg kg–1, and pH 7.79 in RW.
The treatments were arranged in a split-plot design with a cropping system as the main plots and N treatment as the subplots. Nitrogen fertilization treatments (timing and amount) were designed in line with local management. The four N treatments were applied as follows: 0 kg N ha–1 (N0), 135 kg N ha–1 (N135), 180 kg N ha–1 (N180), and 225 kg N ha–1 (N225), of which 180 kg N ha–1 is the local conventional N application rate. N fertilizer was applied at the basal, tillering, and jointing initiation stages in a ratio of 1: 1: 1, being 45 kg N ha–1 for the N135 treatment, 60 kg N ha–1 for the N180 treatment, and 75 kg N ha–1 for the N225 treatment, respectively. Each plot is repeated four times, arranged in random block groups, with a plot area of 12 m2 (2 m × 6 m). The experimental design and wheat growth process are shown in Figure 1. In the experiment, “Zhengmai 9023” was used as the tested variety, and the planting density was 2.25 million plants ha–1. N fertilizer was applied in the form of urea (46% N) that was ploughed into the soil during sowing and was spread as top dressing. Plots were supplied with P (105 kg P2O5 ha–1, calcium superphosphate) and K (105 kg K2O ha–1 potassium sulfate) fertilizer during the sowing period. We did not need to irrigate during the two growing seasons due to the abundant rainfall. Herbicides, pesticides, and fungicides were sprayed according to standard growing practices to avoid yield loss.
Measurement of Yield and Yield Components
We selected mature plants from a 2 m2 harvest area in the middle of each plot to determine the yield and yield components. The spike number was enumerated in each plot and recorded prior to harvest. All spikes were manually harvested, threshed, and weighed to calculate grain yield. The average number of kernels per spike was enumerated from 30 spikes. One thousand random kernels from each harvested grain were weighed to calculate a 1,000-kernel weight. Grain moisture was measured using a grain analyzer (InfratecTM, Foss, Denmark). Grain yield and 1,000-kernel weight were adjusted to 13% moisture.
Measurement of N Accumulation and Nitrogen Use Efficiency
The shoot biomass was harvested from twenty mature plants in each plot at the flowering and maturity. Samples were oven-dried at 65°C for at least 48 h until they were a constant weight. Then, pulverized with a plant grinder, followed by digestion in sulfuric acid at 400°C. Then, the N contents in the plant were measured with an automatic Kjeldahl analyzer (Kjeltec-8400, FOSS, Denmark) and the values were used to calculate the agronomic efficiency of nitrogen (AEN) and physiological efficiency of nitrogen (PEN).
AEN = (Grain yield in the plot that received N applied–grain yield in the zero-N control)/(amount of N applied).
Which represents the amount of yield increased per unit N fertilizer, reflecting the economic performance of N fertilizer in terms of grain production.
PEN = (Grain yield in the plot that received N applied–grain yield in the zero-N control)/(total aboveground plant N accumulation in the plot received N applied–total aboveground plant N accumulation in the zero-N control).
Which represents the yield increase per kg increase in N uptake from fertilizer. It expresses the efficiency with which the plant utilizes N taken up to produce fruit dry biomass.
Measurement of Soil Moisture Content, Nitrate, and Ammonium Nitrogen
Soil samples were collected from each plot at the flowering (March 28, 2021), midfilling (April 14, 2021), and maturity (May 8, 2021) of wheat. Soil samples to a depth of 60 cm were obtained in triplicate by a soil auger (5-cm diameter) at 20-cm intervals. In total, 10 g of each sample was taken for determinations of nitrate (NO3–) and ammonium (NH4+), and the remaining dose was used for a gravimetric water content determination. The 10 g of the soil sample was extracted with 50 ml of 1 mol l–1 KCl to obtain the nitrate and ammonium concentrations in the soil. All nitrate and ammonium concentrations in various solutions were determined using A SMARTCHEN automatic intermittent chemical analyzer. The gravimetric soil moisture content of the samples was subsequently measured using the oven-drying method (105°C for 24 h).
Statistical Analysis
Data were analyzed using a three-way (year, cropping system, and nitrogen management) ANOVA with SAS 9.2 (SAS Institute, Cary, NC, United States). Treatment means were compared using the least significant differences (LSD) at P < 0.05.
Results
Climate Conditions During the Wheat Growing Season
The daily mean temperatures for the wheat filling period were 20.4°C in 2017–2018 and 17.6°C in 2020–2021. The reduced temperature in the post-flowering period extends the length of the grain filling period in 2020–2021(Figure 2). The total rainfall for the wheat growing season was 351.1 mm in 2017–2018 and 263.8 mm in 2020–2021. In 2017–2018, the rainfall from wintering to jointing of wheat was 149.4 mm, accounting for 43% of the total rainfall during the growth period. In 2020–2021, the rainfall from the winter to the jointing of wheat was only 44.5 mm. The rainfall in this year was mainly concentrated in the filling period reached 132.6 mm, accounting for 50% of the rainfall in the entire growth period of wheat, 39% more than that in the same period in 2017–2018. Compared with 2017–2018, the frequency of rainfall in the filling period in 2020–2021 is higher. Rainy days accounted for 44% of the total filling days. The daily mean rainfall of the rainy days in the filling period was 7.0 mm, of which 57% was concentrated in the early stage of filling.
Soil Moisture Content (%)
Figure 3 shows the 0–60 cm soil moisture content during the grain filling period of DW and rice stubble wheat (RW) in 2020–2021. The soil moisture content of RW was significantly (P < 0.05) higher than DW (except for 0–20 cm at maturity), higher 0.6–3.2% at 0–20 cm, 2.2–4.1% at 20–40 cm, and 2.1–6.9% at 40–60 cm soil depth than DW.
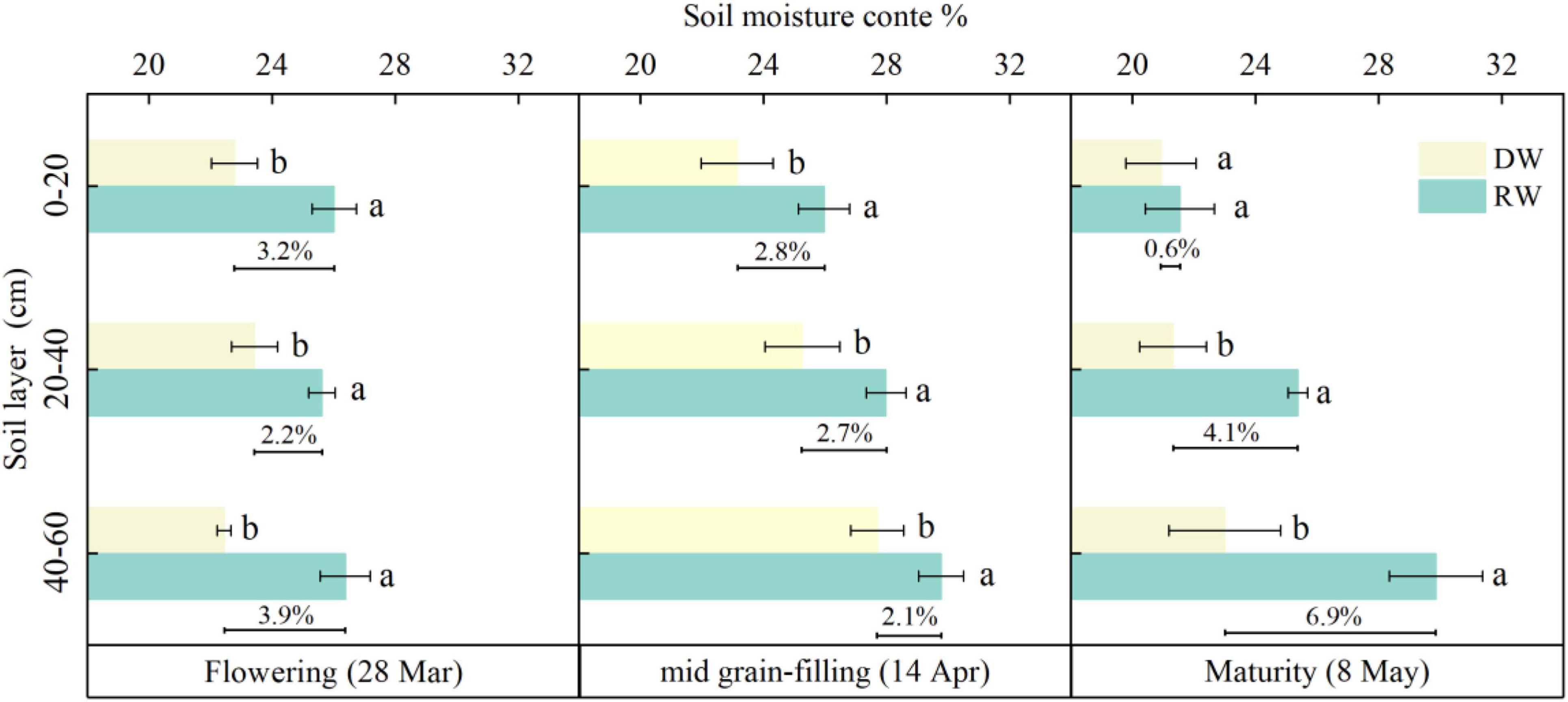
Figure 3. Soil moisture content in different rotation wheat seasons in 2020–2021 (0–60 cm). Different lowercase letters indicate a significant difference (P < 0.05) between dryland wheat vs. rice stubble wheat.
Yield and Yield Components
Increasing N significantly improved grain yield in DW and RW, with significant differences between years (Table 1). Compared with N0, the yield of N supply increased by 1.2–1.4 times (RW), 0.5–0.7 times (DW) in 2017–2018; the yield increased by 2.0–2.5 times (RW), 1.6–1.7 times (DW) in 2020–2021, increased N supply has a greater effect on the increase in RW. The yield of DW was higher than that of RW, which was more pronounced in the N0 and N135 treatment in 2020–2021. When the N supply was increased from 135 to 180 kg ha–1, the yield increase of DW and RW will only reach significant levels in 2020–2021. When the N supply was increased from 180 to 225 kg ha–1, the yield increase of DW and RW was not significant in 2017–2018 and 2020–2021.
The yield components of wheat were both significantly (P < 0.05) affected by planting patterns and N treatments (Table 1). The spike number of DW was 4–26.3% higher than that of RW under the same treatment, while RW has an advantage in the kernels per spike. Increased N supply has a difference in the increase of the spike number and kernels per spike of DW and RW. The highest increase in the spike number of DW and RW by increasing N supply by an average of 65.3 and 63.8%, respectively, over the two wheat growing seasons. The highest increase in the kernels per spike of DW was 28.3% on average, while RW could reach 87.8%. The results showed that increased N supply has a greater effect on increasing the kernels per spike in RW. Affected by the low rainfall from the tillering to the jointing of wheat and the excessive rainfall during the wheat filling in 2020–2021 (Figure 2), the spike number and kernels per spike of wheat under the same treatment in 2020–2021 was significantly lower than that in 2017–2018, and the 1,000-grain weight was not significant differences.
N Accumulation and Nitrogen-Use Efficiency
There were significant differences in the N accumulation and NUE between DW and RW (Table 2). Under the same N supply, the N accumulation in plant tissue and kernel dry matter of DW in 2017–2018 was 9.2–168.9% and 31.2–199.4% higher than at RW. There were significant differences only under N0 treatment in 2020–2021. The agronomic efficiency of nitrogen (AEN) and physiological efficiency of nitrogen (PEN) at RW were significantly higher than at DW by 11.4–17.4 and 15.3–25.6 kg kg–1. RW was also higher than DW in 2020–2021, but the difference was not significant. The plant N accumulation and NUE of DW and RW were different in years. The N accumulation in 2017–2018 was higher than that in 2020–2021, and there was a significant difference between DW and RW in the first year. The second year also showed the same regular difference, but the difference did not reach a significant level.
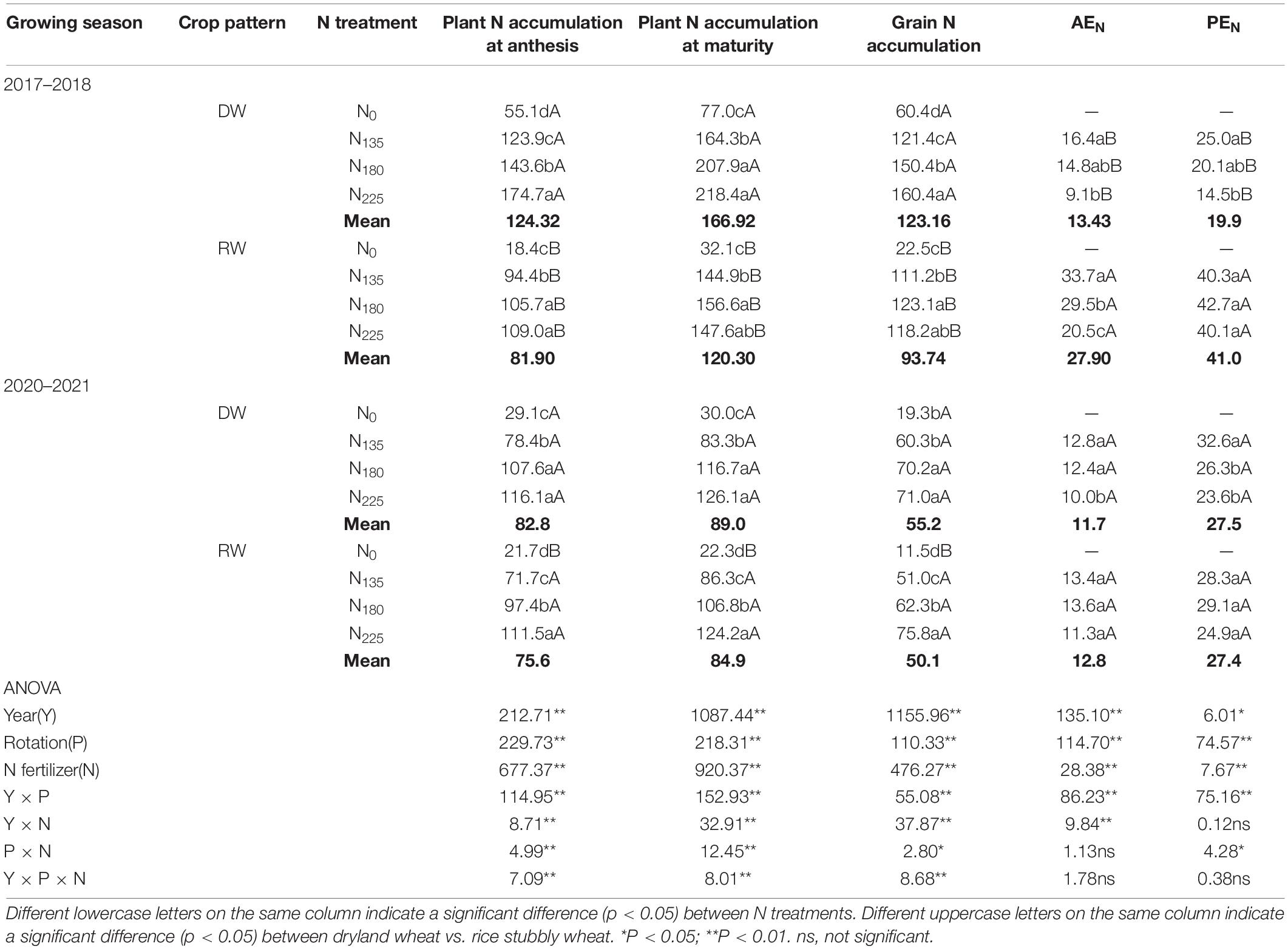
Table 2. Responses of nitrogen accumulation and nitrogen use efficiency of wheat under dryland wheat (DW) and rice stubble wheat (RW) to increased nitrogen fertilizer application.
Increased N supply has a significant impact on the plant N accumulation and NUE at DW and RW (Table 2). With the increase of N supply, the N accumulation in plant tissue and kernel dry matter of DW at flowering and maturity increased significantly. Compared with N135 treatment, the N accumulation at flowering and maturity of N180 treatment increased by an average of 26.6 and 23.9%, respectively, over the two wheat growing seasons, and RW increased by 33.3 and 15.9%. The corresponding N accumulation in the kernel of DW and RW increased by 20.2 and 16.4%, respectively. The increase of N225 treatment compared with N180 treatment reached a significant level only in DW in 2017–2018 and RW in 2020–2021. With the increase of N supply, AEN and PEN of DW decreased significantly, but AEN and PEN of RW had no significant difference between nitrogen treatment (except for AEN in 2017–2018).
Soil NO3–-N and NH4+-N
In the flowering, both DW and RW 0–20 cm soil NO3–-N increased with the increase of N supply, and both reached the maximum under N225 treatment (Figure 4). Which were significantly increased by 4.3–7.3 mg kg–1 at DW, 3.6–4.9 mg kg–1 at RW, compared with other treatments; But at 20–40 cm soil layer, the increase of N supply gradually reduced the soil NO3––N content. The DW soil NO3––N content of N225 treatment increased 2.4–3.6 mg kg–1 compared with other n treatments, while RW Only increased by 0.4–0.5 mg kg–1. With the deepening of the soil layer, the difference in the soil NO3––N content at DW and RW between N treatments gradually decreased. In the middle of filling, only the 0–20 cm soil NO3––N content at DW has significant differences between N treatments, while at RW was not significant; There was no significant difference in the NO3––N content at DW in 20–60 cm soil between N treatments, but the N225 treatment significantly increased 0.8–2.7 mg kg–1 compared with other N treatments in RW. In the maturity, the 0–40 cm soil NO3––N content at DW and RW had no significant difference between N treatments.
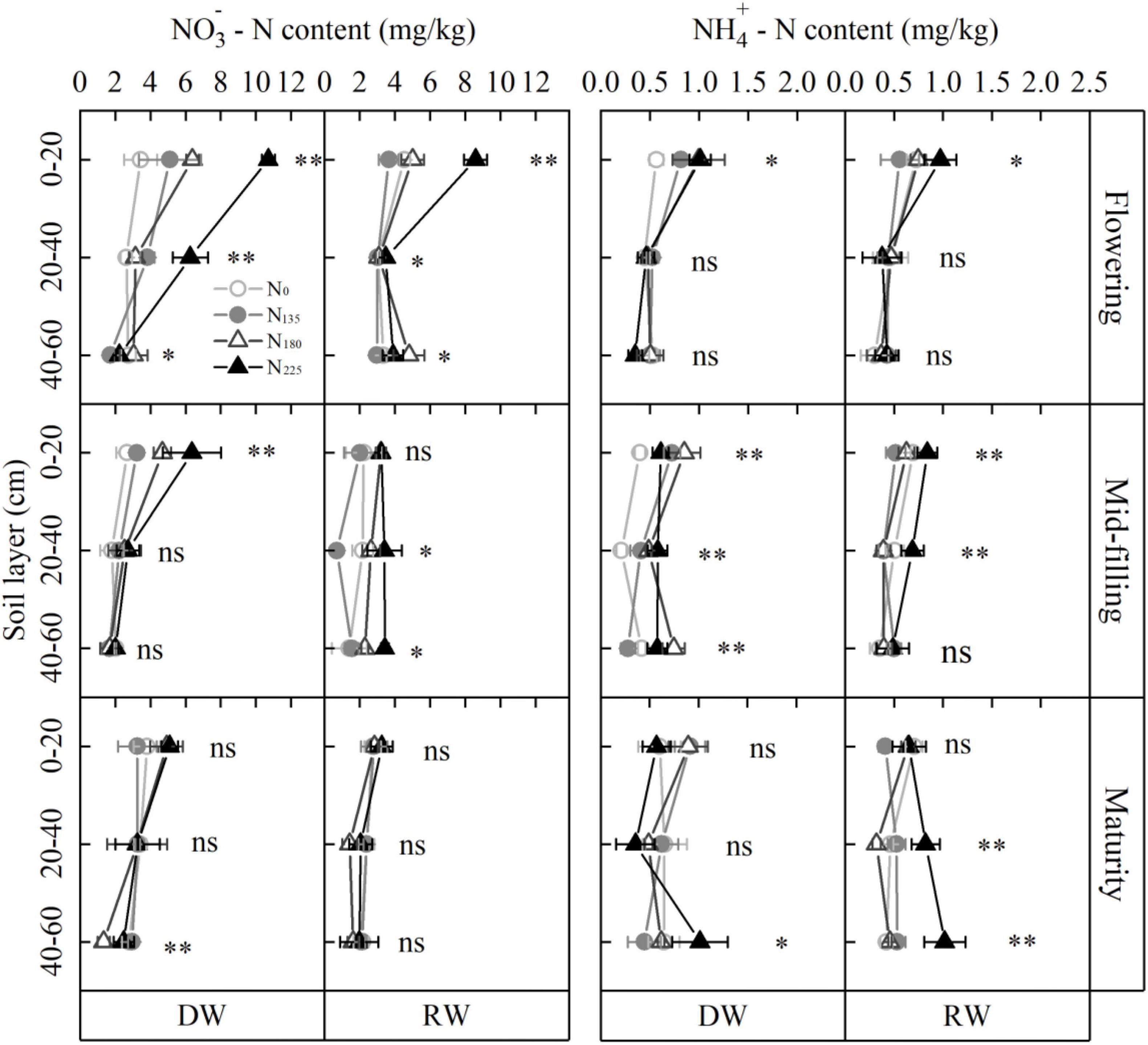
Figure 4. Changes in soil NO3––N and NH4+–N content during the late growth stage of wheat under dryland wheat (DW) and rice stubble wheat (RW) in 2020–2021. *P < 0.05; **P < 0.01; ns, non-significant.
The content of NH4+–N in the soil is low, only 7.4–46.2% of the corresponding nitrate-nitrogen content (Figure 4). In the flowering, increased N supply increased the 0–20 cm soil NH4+–N content at DW and RW. Compared with other N treatments, N225 treatment increased by 0.02–0.45 mg kg–1 under DW and RW. The NH4+–N in 20–60 cm soil was not significantly different between N treatments. In the middle of filling, there were significant differences in the NH4+–N at DW in 0–60 cm soil between N treatments, while the difference in RW was only in the 0–40 cm soil layer. At maturity, there were no significant differences in NH4+–N between DW and RW in the 0–20 cm soil layer, but there were significant differences between the 40 and 60 cm soil layer.
Discussion
This study compared the nitrogen accumulation and yield of DW and rice stubble wheat (RW) in response to different nitrogen levels. Our research results show that there were differences in nitrogen accumulation and yield of wheat between years, and the effect of nitrogen fertilizer on RW is better than DW, and RW has a higher NUE.
Differences in Soil Moisture Content and Mineral Nitrogen Between Dryland Wheat and Rice-Wheat
The type of soil texture and the depth of the soil layer are the main factors that affect the soil moisture pattern. From the perspective of the vertical change characteristics of soil water content, the water content of the 0–60 cm soil layer increases with the increase of depth (Figure 3). Because there are more residual straws in the soil surface layer, the decomposed humus promotes the formation of soil porosity (Turmel et al., 2015), and the wheat root system is mainly distributed in the surface layer (Yang et al., 2021). The soil surface layer has a large infiltration rate, which is not conducive to the preservation of soil moisture. Therefore, the soil moisture content of the soil surface layer is likely to fluctuate with rainfall. There was a big difference in soil moisture between DW and RW. Rotation methods will change the physical properties of the soil. Long-term paddy-rice rotation promotes soil granulation. The soil texture of RW was stickier and its soil moisture content is 2.2–4.3% higher than that of DW on average (Figure 3). This may be mainly because the rice stubble wheat soil with fine texture and high field water holding capacity will more effectively store rainwater with high rainfall (Yoo et al., 1998).
The concentration of mineral nitrogen is an important index to evaluate the available nitrogen supply capacity of the soil, and the change of soil moisture is one of the most important environmental factors affecting nitrogen mineralization and nitrification. Studies have suggested that nitrification is more sensitive to water than ammonification, and the nitrification rate will be inhibited when the soil moisture is high (Schimel and Parton, 1986; Wang et al., 2021). This is mainly because the increase in rainfall results in a large soil moisture content, which is not conducive to the diffusion of oxygen in the soil. Nitrifying bacteria prefer aerobic conditions. Excessive rainfall makes clay loam soils with higher field water holding capacity more likely to form an anaerobic environment, inhibiting nitrifying bacteria and nitrification (Cameron et al., 2013). Soil available nitrogen supply was affected by soil water content, and rice stubble wheat has a greater decrease in nitrate-nitrogen content in the middle of grain filling when the soil water content was higher. Armstrong et al. (1996) found that the response of dryland sorghum to nitrogen application largely depends on the soil moisture content, and the response to nitrogen increase depends on the time of rainfall. The results of this study can also prove this point. Due to the influence of soil water content and high rainfall frequency, the nitrate-nitrogen content of the surface soil of rice stubble wheat is less responsive to increased nitrogen fertilizer application than DW. This is because of the three ways of soil nutrient loss (gas loss, leaching loss and runoff, and erosion loss), both leaching loss and runoff loss are highly susceptible to rainfall intensity. The Jianghan Plain has abundant rainfall, with a total rainfall of 132.6 mm during the wheat filling period in 2020–2021, which is a humid climate zone (Figure 2). The soil nitrogen loss pathways of rice stubble wheat and DW have a great relationship with soil texture and soil moisture. When the rainfall of the fine-textured paddy soil exceeds the infiltration amount of the soil, the field water holding capacity will reach a saturated state, and the field water is more likely to form surface runoff (Zhao et al., 2012); The rough soil has strong water conductivity, fast soil infiltration rate, and more serious leaching loss (Sugita and Nakane, 2007). We suggest that the interplay between soil water and soil nitrogen in crop rotations warrants further attention: part of this could include direct quantification of the association between soil moisture and soil nitrogen at the beginning of the cropping phase and subsequent implications for both growth and NUE.
Differences in Nitrogen Accumulation Between Dryland Wheat and Rice-Wheat
This study adds significant novelty to the scientific literature as we compare: (1) cropping systems rotational effects (RW or soybean/corn–wheat) on soil nitrogen and crop NUE and (2) our contrast between RW and DW systems allows direct comparison of the effects of residual soil moisture on subsequent crop growth and NUE. Indeed, soil mineral nitrogen concentration is often linked with the vegetative growth of wheat. Some studies have shown that high levels of mineral N (NO3– and NH4+) stimulate shoot biomass accumulation of fertilized treatments, neither seed yield nor seed nitrogen concentration was affected by soil mineral N availability (Voisin et al., 2002). In contrast, this study has shown that the yield and nitrogen accumulation of RW were related to nitrogen application rate and soil mineral nitrogen content at flowering (Table 2). Increasing nitrogen application significantly increased the soil mineral nitrogen content of rice stubble and wheat, and increased plant nitrogen accumulation. Compared to the RW, plant N accumulation at maturity was greater at the DW. Only when the N accumulation at DW at the flowering stage was greater than at RW, the N accumulation of at DW at maturity was greater at RW (Table 2).
Excessive rainfall at the time of fertilization may cause loss of external nitrogen sources at RW soil because the amount of fertilizer-N applied before flowering initiation was similar at the two systems. Therefore, in areas with high rainfall, RW may be more suitable for small and multiple fertilization, especially to avoid the high-frequency rainfall period (March–May). Even though Ney et al. (1997) stressed that any diagnosis using the critical nitrogen concentration was best done before seed filling. However, the more rainfall from March to May in Jianghan Plain makes the nitrogen supply in different wheat growing environments different, so we believe that it is equally important to understand the soil nitrogen supply in the later stages of wheat growth.
Differences in Yield and Nitrogen-Use Efficiency Between Dryland Wheat and Rice-Wheat
Under low nitrogen (0–135 kg ha–1), the yield of DW was higher than that of RW, and under conditions of sufficient nitrogen supply, RW can also obtain the same yield as DW. The results show that the soil of DW has a higher basic nitrogen supply capacity. In this experiment, DW has a higher spike number and nitrogen accumulation at flowering. The soil of DW provides more nitrogen in the early growth period and has more tillers at wintering and spike number at harvest (Yin et al., 2018). This may be the reason for the higher yield of DW and the higher accumulation of nitrogen at flowering. Compared with DW, the conversion of paddy fields to dryland causes changes in the physical and chemical properties of the soil. Poor soil aeration has an adverse effect on the emergence of RW and the growth of the seedling stage (Nawaz et al., 2016). Therefore, the spike number at maturity of RW is less than that of DW. The yield increase effect of RW was mainly that the number of kernels per spike was increased by 71.2–84.4% (Table 1). Due to decreased shoots per unit area and alleviated competition for resources between shoots (Guo and Schnurbusch, 2015).
Prior to this study, the greater root length density is an important root trait for the crop with high grain yield and higher NUE under N-limited conditions or at lower N rates (Ju et al., 2015). Our previous results indicated that the root length density at DW was greater at RW, especially in deep soil layers. This may also be the reason why the N accumulation at DW was greater at RW (Yang et al., 2021). AEN refers to the amount of yield increased per unit N fertilizer, reflecting the economic performance of N fertilizer in terms of grain production. Although the N accumulation at RW was lower than at DW, it can reach a similar yield level at DW. Therefore, RW has greater N use efficiency. Higher nitrogen accumulation level or NUE can achieve high yield.
Conclusion
The effect of increasing nitrogen fertilizer on the yield at rice stubble wheat was greater, and the main reason for the increase was the increase in the number of kernels per spike. The nitrogen accumulation at rice stubble wheat was not as good as at DW, but it can reach the same yield level. The agronomic nitrogen efficiency and physiological use efficiency at rice stubble wheat were better than at DW. From the perspective of soil nitrogen levels, higher rainfall and higher field water holding capacity can easily lead to the loss of soil nitrogen at rice stubble wheat. Overall, based on the humid climatic conditions and different soil environmental characteristics of the Jianghan Plain, the nitrogen fertilizer application rates for both DW and rice stubble wheat should not exceed 180 kg ha–1.
Data Availability Statement
The original contributions presented in the study are included in the article/supplementary material, further inquiries can be directed to the corresponding authors.
Author Contributions
RY and XW initiated and designed the research. RY and ZW performed the experiments. MH, MZ, KL, SF, and XW revised and edited the manuscript and also provided advice on the experiments. All authors contributed to the article and approved the submitted version.
Funding
This study was funded by the National Science Foundation of China (Grant Number 31871578), the National Key Research & Development Program of China during the 13th Five-year Period (Grant Number 2016YFD0300107), and Engineering Research Center of Ecology and Agricultural Use of Wetland (Grant Number KFT202102).
Conflict of Interest
The authors declare that the research was conducted in the absence of any commercial or financial relationships that could be construed as a potential conflict of interest.
Publisher’s Note
All claims expressed in this article are solely those of the authors and do not necessarily represent those of their affiliated organizations, or those of the publisher, the editors and the reviewers. Any product that may be evaluated in this article, or claim that may be made by its manufacturer, is not guaranteed or endorsed by the publisher.
References
Alcock, D. J., Harrison, M. T., Rawnsley, R. P., and Eckard, R. J. (2015). Can animal genetics and flock management be used to reduce greenhouse gas emissions but also maintain productivity of wool-producing enterprises? Agric. Syst. 132, 25–34. doi: 10.1016/j.agsy.2014.06.007
Armstrong, R. D., Halpin, N. V., Mccosker, K., Standley, J., and Lisle, A. T. (1996). Applying nitrogen to grain sorghum in central Queensland: residual value and effect of fallowing and tillage practice. Aust. J. Agric. Res. 47, 81–95.
Bilotto, F., Harrison, M. T., Migliorati, M. D. A., Christie, K. M., Rowlings, D. W., Grace, P. R., et al. (2021). Can seasonal soil N mineralisation trends be leveraged to enhance pasture growth? Sci. Total Environ. 772:145031. doi: 10.1016/j.scitotenv.2021.145031
Bu, R., Ren, T., Liao, S., Li, X., Cong, R., Zhang, Y., et al. (2019). Difference of soil nitrogen supply and rapeseed nitrogen uptake under different rotation systems and seasonal distribution of nitrogen fertilizer. J. Plant Nutr. Fertilizers 25, 412–420.
Cameron, K. C., Di, H. J., and Moir, J. L. (2013). Nitrogen losses from the soil/plant system: a review. Ann. Appl. Biol. 162, 145–173. doi: 10.1111/j.1469-8137.2012.04300.x
Chang-Fung-Martel, J., Harrison, M. T., Rawnsley, R., Smith, A. P., and Meinke, H. (2017). The impact of extreme climatic events on pasture-based dairy systems: a review. Crop Pasture Sci. 68, 1158–1169. doi: 10.1071/cp16394
Christie, K. M., Rawnsley, R. P., Harrison, M. T., and Eckard, R. J. (2014). Using a modelling approach to evaluate two options for improving animal nitrogen use efficiency and reducing nitrous oxide emissions on dairy farms in southern Australia. Anim. Prod. Sci. 54, 1960–1970. doi: 10.1071/an14436
Christie, K. M., Smith, A. P., Rawnsley, R. P., Harrison, M. T., and Eckard, R. J. (2018). Simulated seasonal responses of grazed dairy pastures to nitrogen fertilizer in SE Australia: pasture production. Agric. Syst. 166, 36–47. doi: 10.1016/j.agsy.2018.07.010
Christie, K. M., Smith, A. P., Rawnsley, R. P., Harrison, M. T., and Eckard, R. J. (2020). Simulated seasonal responses of grazed dairy pastures to nitrogen fertilizer in SE Australia: N loss and recovery. Agric. Syst. 182:102847. doi: 10.1016/j.agsy.2020.102847
Fageria, N. K., and Baligar, V. C. (2005). “Enhancing nitrogen use efficiency in crop plants. Adv. Agron. 88, 97–185. doi: 10.1016/s0065-2113(05)88004-6
Guo, Z., and Schnurbusch, T. (2015). Variation of floret fertility in hexaploid wheat revealed by tiller removal. J. Exp. Bot. 66, 5945–5958. doi: 10.1093/jxb/erv303
Harrison, M. T., Cullen, B. R., and Armstrong, D. (2017). Management options for dairy farms under climate change: effects of intensification, adaptation and simplification on pastures, milk production and profitability. Agric. Syst. 155, 19–32. doi: 10.1016/j.agsy.2017.04.003
Harrison, M. T., Cullen, B. R., Tomkins, N. W., McSweeney, C., Cohn, P., and Eckard, R. J. (2016). The concordance between greenhouse gas emissions, livestock production and profitability of extensive beef farming systems. Anim. Prod. Sci. 56, 370–384.
Harrison, M. T., Edwards, E. J., Farquhar, G. D., Nicotra, A. B., and Evans, J. R. (2009). Nitrogen in cell walls of sclerophyllous leaves accounts for little of the variation in photosynthetic nitrogen-use efficiency. Plant Cell Environ. 32, 259–270. doi: 10.1111/j.1365-3040.2008.01918.x
Harrison, M. T., Evans, J. R., and Moore, A. D. (2012a). Using a mathematical framework to examine physiological changes in winter wheat after livestock grazing: 1. model derivation and coefficient calibration. Field Crops Res. 136, 116–126. doi: 10.1016/j.fcr.2012.06.015
Harrison, M. T., Evans, J. R., and Moore, A. D. (2012b). Using a mathematical framework to examine physiological changes in winter wheat after livestock grazing: 2. model validation and effects of grazing management. Field Crops Res. 136, 127–137.
Harrison, M. T., Evans, J. R., Dove, H., and Moore, A. D. (2011). Recovery dynamics of rainfed winter wheat after livestock grazing 2. Light interception, radiation-use efficiency and dry-matter partitioning. Crop Pasture Sci. 62, 960–971.
Harrison, M. T., Jackson, T., Cullen, B. R., Rawnsley, R. P., Ho, C., Cummins, L., et al. (2014). Increasing ewe genetic fecundity improves whole-farm production and reduces greenhouse gas emissions intensities: 1. sheep production and emissions intensities. Agric. Syst. 131, 23–33.
Hess, L. J. T., Hinckley, E.-L. S., Robertson, G. P., and Matson, P. A. (2020). Rainfall intensification increases nitrate leaching from tilled but not no-till cropping systems in the U.S. Midwest. Agric. Ecosyst. Environ. 290:106747. doi: 10.1016/j.agee.2019.106747
Ho, C. K. M., Jackson, T., Harrison, M. T., and Eckard, R. J. (2014). Increasing ewe genetic fecundity improves whole-farm production and reduces greenhouse gas emissions intensities: 2. economic performance. Anim. Prod. Sci. 54, 1248–1253.
Ibrahim, A., Harrison, M., Meinke, H., Fan, Y., Johnson, P., and Zhou, M. (2018). A regulator of early flowering in barley (Hordeum vulgare L.). PLoS One 13:e0200722. doi: 10.1371/journal.pone.0200722
Ju, C., Buresh, R. J., Wang, Z., Zhang, H., Liu, L., Yang, J., et al. (2015). Root and shoot traits for rice varieties with higher grain yield and higher nitrogen use efficiency at lower nitrogen rates application. Field Crops Res. 175, 47–55. doi: 10.7717/peerj.7294
Kodzwa, J. J., Gotosa, J., and Nyamangara, J. (2020). Mulching is the most important of the three conservation agriculture principles in increasing crop yield in the short term, under sub humid tropical conditions in Zimbabwe. Soil and Tillage Res. 197, 104515. doi: 10.1016/j.still.2019.104515
Li, W., Shao, D., Gu, W., and Miao, D. (2021). Regional agroclimate characteristic and its multiple teleconnections: a case study in the Jianghan Plain (JHP) Region. Water 13:2789.
Liu, K., Harrison, M. T., Hunt, J., Angessa, T. T., Meinke, H., Li, C., et al. (2020). Identifying optimal sowing and flowering periods for barley in Australia: a modelling approach. Agric. For. Meteorol. 282:107871. doi: 10.1016/j.agrformet.2019.107871
Nawaz, A., Farooq, M., Ahmad, R., Basra, S. M. A., and Lal, R. (2016). Seed priming improves stand establishment and productivity of no till wheat grown after direct seeded aerobic and transplanted flooded rice. Eur. J. Agron. 76, 130–137. doi: 10.1016/j.eja.2016.02.012
Ney, B., Doré, T., and Sagan, M. (1997). “The nitrogen requirement of major agricultural crops: Grain Legumes,” in Diagnosis of the Nitrogen Status in Crops, ed. G. Lemaire (Berlin: Springer), 107–118. doi: 10.1007/978-3-642-60684-7_6
Rawnsley, R. P., Smith, A. P., Christie, K. M., Harrison, M. T., and Eckard, R. J. (2019). Current and future direction of nitrogen fertiliser use in Australian grazing systems. Crop Pasture Sci. 70, 1034–1043. doi: 10.1071/cp18566
Ren, T., Bu, R., Liao, S., Zhang, M., Li, X., Cong, R., et al. (2019). Differences in soil nitrogen transformation and the related seed yield of winter oilseed rape (Brassica napus L.) under paddy-upland and continuous upland rotations. Soil Tillage Res. 192, 206–214.
Schimel, D. S., and Parton, W. J. (1986). Microclimatic controls of nitrogen mineralization and nitrification in shortgrass steppe soils. Plant Soil 93, 347–357. doi: 10.1007/bf02374285
Shah, A. N., Iqbal, J., Tanveer, M., Yang, G., Hassan, W., and Fahad, S. (2017). Nitrogen fertilization and conservation tillage: a review on growth, yield, and greenhouse gas emissions in cotton. Environ. Sci. Pollut. Res. 24, 2261–2272. doi: 10.1007/s11356-016-7894-4
Shah, A. N., Wu, Y., Iqbal, J., Tanveer, M., Bashir, S., and Rahman, S. U. (2021a). Nitrogen and plant density effects on growth, yield performance of two different cotton cultivars from different origin. J. King Saud Univers. Sci. 33:101512. doi: 10.1016/j.jksus.2021.101512
Shah, A. N., Wu, Y., Tanveer, M., Hafeez, A., Tung, S. A., and Ali, S. (2021b). Interactive effect of nitrogen fertilizer and plant density on photosynthetic and agronomical traits of cotton at different growth stages. Saudi J. Biol. Sci. 28, 3578–3584. doi: 10.1016/j.sjbs.2021.03.034
Smith, A. P., Christie, K. M., Harrison, M. T., and Eckard, R. J. (2021). Ammonia volatilisation from grazed, pasture based dairy farming systems. Agric. Syst. 190:103119.
Sugita, F., and Nakane, K. (2007). Combined effects of rainfall patterns and porous media properties on nitrate leaching. Vadose Zone J. 6, 548–553. doi: 10.2136/vzj2006.0095
Turmel, M. S., Speratti, A., Baudron, F., Verhulst, N., and Govaerts, B. (2015). Crop residue management and soil health: a systems analysis. Agric. Syst. 134, 6–16.
Voisin, A. S., Salon, C., Munier-Jolain, N. G., and Ney, B. (2002). Effect of mineral nitrogen on nitrogen nutrition and biomass partitioning between the shoot and roots of pea (Pisum sativum L.). Plant Soil 242, 251–262.
Wang, N., Li, L., Dannenmann, M., Luo, Y., Xu, X., Zhang, B., et al. (2021). Seasonality of gross ammonification and nitrification altered by precipitation in a semi-arid grassland of Northern China. Soil Biol. Biochem. 154:108146. doi: 10.1016/j.soilbio.2021.108146
Wang, X., Gao, C., Lu, B., and Su, R. (2013). Characteristics of rainfall before anthesis and corresponding effects of waterlogging on grain yield in Jianghan plain. Resour. Environ. Yangtze Basin 22, 1642–1647.
Yan, H., Harrison, M. T., Liu, K., Wang, B., Feng, P., and Fahad, S. (2022). Crop traits enabling yield gains under more frequent extreme climatic events. Sci. Total Environ. 808:152170. doi: 10.1016/j.scitotenv.2021.152170
Yang, R., Liu, K., Geng, S., Zhang, C., Yin, L., and Wang, X. (2021). Comparison of early season crop types for wheat production and nitrogen use efficiency in the Jianghan plain in China. PeerJ 9:e11189. doi: 10.7717/peerj.11189
Yang, Y., Zhang, H., Shan, Y., Wang, J., Qian, X., Meng, T., et al. (2019). Response of denitrification in paddy soils with different nitrification rates to soil moisture and glucose addition. Sci. Total Environ. 651, 2097–2104. doi: 10.1016/j.scitotenv.2018.10.066
Yin, L., Dai, X., and He, M. (2018). Delayed sowing improves nitrogen utilization efficiency in winter wheat without impacting yield. Field Crops Res. 221, 90–97. doi: 10.1002/jsfa.11775
Yoo, C., Valdés, J. B., and North, G. R. (1998). Evaluation of the impact of rainfall on soil moisture variability. Adv. Water Resour. 21, 375–384.
Yousaf, M., Li, X., Zhang, Z., Ren, T., Cong, R., Ata-Ul-Karim, S. T., et al. (2016). Nitrogen fertilizer management for enhancing crop productivity and nitrogen use efficiency in a rice-oilseed rape rotation system in China. Front. Plant Sci. 7:1496. doi: 10.3389/fpls.2016.01496
Zhao, X., Zhou, Y., Min, J., Wang, S., Shi, W., and Xing, G. (2012). Nitrogen runoff dominates water nitrogen pollution from rice-wheat rotation in the Taihu Lake region of China. Agric. Ecosyst. Environ. 156, 1–11.
Keywords: dryland wheat, rice stubble wheat, yield, nitrogen use efficiencies, soil
Citation: Yang R, Liu K, Harrison MT, Fahad S, Wang Z, Zhou M and Wang X (2022) How Does Crop Rotation Influence Soil Moisture, Mineral Nitrogen, and Nitrogen Use Efficiency? Front. Plant Sci. 13:854731. doi: 10.3389/fpls.2022.854731
Received: 14 January 2022; Accepted: 07 February 2022;
Published: 17 March 2022.
Edited by:
Mohsin Tanveer, University of Tasmania, AustraliaReviewed by:
Yu Shi, Shandong Agricultural University, ChinaChengyan Zheng, Institute of Crop Sciences (CAAS), China
Copyright © 2022 Yang, Liu, Harrison, Fahad, Wang, Zhou and Wang. This is an open-access article distributed under the terms of the Creative Commons Attribution License (CC BY). The use, distribution or reproduction in other forums is permitted, provided the original author(s) and the copyright owner(s) are credited and that the original publication in this journal is cited, in accordance with accepted academic practice. No use, distribution or reproduction is permitted which does not comply with these terms.
*Correspondence: Ke Liu, ke.liu@utas.edu.au; Shah Fahad, shah_fahad80@yahoo.com; Xiaoyan Wang, wamail_wang@163.com