- 1School of Life Sciences, Nantong University, Nantong, China
- 2Xuzhou Institute of Agricultural Sciences in Xuhuai District, Sweet Potato Research Institute, Xuzhou, China
Sclerotinia stem rot, which is caused by the fungal pathogen Sclerotinia sclerotiorum, is a soybean disease that results in enormous economic losses worldwide. The control of S. sclerotiorum is a difficult task due to the pathogen’s wide host range and its persistent structures, called sclerotia. In addition, there is lack of soybean cultivars with medium to high levels of resistance to S. sclerotiorum. In this work, kojic acid (KA), a natural bioactive compound commonly used in cosmetic industry, was evaluated for the management of Sclerotinia stem rot. Interestingly, KA showed strong antifungal activity against S. sclerotiorum by inhibiting chitin and melanin syntheses and, subsequently, sclerotia formation. The antifungal activity of KA was not obviously affected by pH, but was reduced in the presence of metal ions. Treatment with KA reduced the content of virulence factor oxalic acid in S. sclerotiorum secretions. Preventive applications of 50 mM KA (7.1 mg/ml) completely inhibited S. sclerotiorum symptoms in soybean; whereas, in curative applications, the combination of KA with prochloraz and carbendazim improved the efficacy of these commercial fungicides. Taken together, the antifungal activity of KA against S. sclerotiorum was studied for the first time, revealing new insights on the potential application of KA for the control of Sclerotinia stem rot in soybean.
Introduction
Soybean, Glycine max (Fabaceae: Phaseoleae), is extensively cultivated in China, United States, Brazil, and Argentina (Ding et al., 2021; Hu et al., 2021). The global soybean production in 2017 was 359.5 million tons; however, soybean production declined to 333.7 million tons in 2019 [Food and Agricultural Organization of the United Nations (FAO), 2019]. This decrease has been mainly attributed to yield losses produced by soybean diseases, such as Sclerotinia stem rot, which is caused by Sclerotinia sclerotiorum (Willbur et al., 2018; Zhang et al., 2019). Sclerotinia sclerotiorum is known to adhere to the host surface and to penetrate the plant cuticle (Liu et al., 2018; Xu et al., 2018). This fungal pathogen is able to invade different soybean tissues, such as stem, root, and pod, at different plant growth stages (Gao et al., 2020). It was estimated that the losses produced by S. sclerotiorum in soybean in 1997, 2004, and 2009 were approximately in 953 million, 1.63, and 1.61 billion kg, which correspond to 227, 344, and 560 million dollars, respectively (Peltier et al., 2012).
Currently, there is lack of soybean cultivars with medium to high levels of resistance to S. sclerotiorum, and crop rotation is not fully effective for the control of this pathogen (Gracia-Garza et al., 2002; Ranjan et al., 2019). At present, the management of S. sclerotiorum in soybean strongly relies on the use of chemical fungicides, such as carbendazim, fluazinam, prochloraz, and procymidone (Miorini et al., 2021). However, none of them offer complete control and several resistant strains have been reported (Mao et al., 2020; Zhou et al., 2020). Apart from the low efficacy of commercial fungicides, the management of S. sclerotiorum is a difficult task due to the pathogen’s wide host range, and its persistent resting structures, called sclerotia. These are known to play important roles in fungal life and disease development cycles (Xia et al., 2020; Tian et al., 2021). Sclerotia can produce apothecia and release spores, which can be transmitted by wind and rain, to infect plants under appropriate temperature and humidity conditions (Clarkson et al., 2014; Becka et al., 2016). Sclerotia can also germinate and directly infect the host plant. Moreover, sclerotial exudates have been reported to help in the development of sclerotia, and facilitate host cell necrosis (Bolton et al., 2006). The process of mycelial germination from sclerotia is controlled by melanin, which induces resistance to adverse environmental conditions (Huang, 1991). The melanin in S. sclerotiorum is mainly composed by 1,8-dihydroxynaphthalene (DHN) and is deposited in peripheral rind cells during sclerotia formation (Butler et al., 2009; Eisenman and Casadevall, 2012; Ordonez-Valencia et al., 2015).
In order to develop efficient methods for the management of Sclerotinia stem rot, several research groups have developed biocontrol strategies using Bacillus spp., and have achieved generally low–moderate control efficacies (46–76% inhibition rate; Radhakrishnan et al., 2017; Lopes et al., 2018; Massawe et al., 2018). Among the biocontrol strains Coniothyrium minitans CON/M/91–08, Streptomyces lydicus WYEC 108, Trichoderma harzianum T-22, and Bacillus subtilis QST 713 screened for the control of Sclerotinia stem rot, the highest biocontrol efficacy was obtained when using C. minitans (68.5% disease severity inhibition; Zeng et al., 2012; Zhao et al., 2020). Similarly, Clonostachys rosea, Stachybotrys levispora, Trichoderma asperelloides, and Sporidesmiun sclerotivorum have also been evaluated for the management of S. sclerotiorum in soybean, and in this case, S. sclerotivorum reduced Sclerotinia stem rot by 56–100% in commercial fields (Del Rio et al., 2002; Rodriguez et al., 2011; Ribeiro et al., 2018; Sumida et al., 2018). Wang et al. (2019) discovered two mycoviruses in S. sclerotiorum SZ-150, while Bacillus amyloliquefaciens VB7 was reported to inhibit S. sclerotiorum mycelial growth by 45% and sclerotial production by 100% (Vinodkumar et al., 2017). The volatiles produced by Pseudomonas brassicacearum, brassicacearum putida, and Bacillus megaterium altered the cytoplasm and organelle organization in S. sclerotiorum (Giorgio et al., 2015).
On the other hand, kojic acid (KA, 5-hydroxy-2-hydroxymethyl-γ-pyrone) is a common secondary metabolite produced by numerous fungal strains and is widely used in cosmetic industry (Saeedi et al., 2019; Song et al., 2021). The accumulation of tyrosinase in human cells involves the biosynthesis of melanin, leading to skin hyperpigmentation. Tyrosinase contains copper ion in the active site, and KA forms a complex with it thereby reducing enzymatic activity. For this reason, KA is used as a skin lightener, protecting the skin from the UV radiation (Cabanes et al., 1994). KA has been also used as a food additive to preserve postharvest fruit and vegetables from browning (Cabezudo et al., 2021). The antioxidant and chelating properties of KA, together with its natural safety, non-significant environmental side effects, and the ability for large-scale production, have stimulated the research on the availability and possible applications of KA during recent years, with 179 articles published in 2020. Although the ability of KA to inhibit melanin biosynthesis in human cells suggests that KA may be able to inhibit melanin biosynthesis and sclerotia formation in S. sclerotiorum, the potential application of KA for the control of plant pathogens in vivo remains completely unexplored. In this work, the antifungal properties of KA were evaluated as a new low cost alternative for S. sclerotiorum disease management.
Materials and Methods
Fungal Strains
Sclerotinia sclerotiorum strain NJC09 was isolated from soybean plants showing Sclerotinia stem rot symptoms in Xuzhou, China (117.30° E, 34.28° N). Isolation and identification of the pathogen are described in Supplementary Figure S1. The isolation of Valsa pyri strain Vp297, Colletotrichum brevisporum strain GM1, and Botryosphaeria dothidea isolate SBL303 was previously reported by our research group (Chen et al., 2020; Shi et al., 2020; Song et al., 2020). Fungal strains were cultured on potato–dextrose–agar (PDA) medium (200 g potato, 20 g dextrose, and 15 g agar in 1 L water) at 28°C.
Antifungal Activity Assay
Fungal plant pathogens B. dothidea, C. brevisporum, S. sclerotiorum, and V. pyri were used in the antifungal assay. KA was purchased from Macklin (China). The antifungal activity of KA was evaluated in PDA medium containing 5, 10, and 15 mM KA (0.71, 1.42, and 2.13 mg/ml KA), respectively. The control experiments were performed in the absence of KA. The cultures were incubated under darkness at 28°C for 3 days. The colony diameter was measured using a ruler (Tremarin et al., 2015), and the antifungal effect was determined according to the mycelial growth. Experiments were repeated five times.
To calculate the half maximal effective concentrations (EC50), KA, carbendazim, and prochloraz were used. S. sclerotiorum was grown on PDA plates containing 0.5, 1, 2, 3, 5, 10, and 15 mM of each fungicide (KA: 0.071, 0.14, 0.28, 0.43, 0.71, 1.42, and 2.13 mg/ml; carbendazim: 0.096, 0.19, 0.38, 0.57, 0.96, 1.91, and 2.87 mg/ml; prochloraz: 0.19, 0.38, 0.75, 1.13, 1.88, 3.77, and 5.65 mg/ml). The negative control experiment was performed by culturing S. sclerotiorum on PDA in the absence of fungicides. The dishes were incubated at 28°C for 3 days. Experiments were repeated five times. Prism 7.0 (GraphPad Software, United States) was used to calculate the EC50 values.
Effect of pH and Metals on the Inhibitory Activity of KA
Sclerotinia sclerotiorum was cultured on PDA medium under darkness at 28°C for 5 days. Three plugs (7 mm diameter) of mycelium were inoculated into 40 ml honey–barley–tryptone medium (600 mg honey, 100 mg barley, and 200 mg tryptone in 40 ml water) and placed at 28°C and 200 rpm for 12 h. After centrifugation at 8,000 rpm and 4°C, the produced mycelial fragments were washed twice with 20 ml sterilized distilled deionized water (ddH2O). Harvested cells were suspended in 200 μl yeast extract–peptone–dextrose medium (YEPD; 0.15 g yeast extract, 0.5 g peptone, and 1 g glucose in 50 ml water; 1 × 106 mycelial fragments/ml) containing 0 and 10 mM KA (0 and 1.42 mg/ml KA). The number of mycelial fragments was calculated using a Leica DM2500 microscope (Germany) at ×40 magnifications and adjusted using sterilized ddH2O.
The effect of pH was calculated by adjusting the YEPD medium to pH values 3, 4, 5, 6, 7, and 8. The effect of iron(II), nickel(II), zinc(II), cobalt(II), and copper(II) was examined by adding the corresponding metal chlorides (1 mM final concentration) into the YEPD medium (pH 4). After 12 h of culture at 28°C and 200 rpm, fungal growth was detected using a Leica DM2500 microscope at ×20 magnifications. The antifungal activity was calculated according to the number of sclerotial aggregates per microliter. Experiments were repeated three times.
Fluorescent Live-Cell Imaging
Sclerotinia sclerotiorum mycelial fragments were cultured in YEPD medium with 0 and 10 mM KA (0 and 1.42 mg/ml KA) as described in “Effect of pH and Metals on the Inhibitory Activity of KA” section. After 12 h at 28°C and 200 rpm, scanning electron microscope (SEM Gemini 300 Instrument, United States) and fluorescent images (Olympus BX51, Japan) were acquired. For the preparation of SEM samples, fungal cells were centrifuged and immobilized in 4% paraformaldehyde. The samples were dehydrated with 65, 75, 85, 95, and 100% (twice) ethanol at room temperature (each dehydration condition was carried out for 10 min). Then, the samples were stored in a desiccator for 6 h. Dried samples were placed on the sample holder, and coated with gold. Images were obtained with an acceleration voltage of 20 kV. In the analysis using the fluorescent microscope, 10 μl YEPD medium was stained with 10 μl 4,6-diamino-2-phenylindole (DAPI, 10 μg/ml solution, Solarbio, China). The resulting suspension was kept in darkness during 5 min before the analysis. Images were collected using a DAPI-selective filter (excitation wavelength: 377 nm; emission wavelength: 447) at ×40 magnifications.
Determination of DHN Content
Two 7-mm-diameter PDA plugs containing S. sclerotiorum mycelium were inoculated into 50 ml YEPD medium with 0, 2, 5, and 10 mM KA (0, 0.28, 0.71, and 1.42 mg/ml KA). After 15 days at 28°C and 200 rpm, DHN was extracted following the standard procedure (Kogej et al., 2004) and DHN content was determined by analytical HPLC-MS using a QTRAP 5500 system (AB SCIEX, United States), equipped with an Agilent C18 column (250 × 4.6 mm, United States). The system was maintained at 30°C. The mobile phase was 0.1% formic acid aqueous solution/acetonitrile 30:70. The injection volume was 5 μl and the flow rate was 0.2 ml/min. The peak corresponding to DHN was detected at 1.26 min. The concentration of DHN was calculated according to the peak area in MS using the transition from 161.0 to 143.3 [(M + H)+ calcd. For C10H9O2, 161.0603; found, 161.0]. Experiments were repeated three times.
Detection of mRNA Levels (qRT-PCR) in Sclerotinia sclerotiorum
Freshly harvested cells prepared as described above were resuspended in YEPD medium (8 ml, 1 × 106 mycelial fragments/ml) with 0 and 10 mM KA (0 and 1.42 mg/ml KA), and shake at 28°C and 200 rpm for 24 h. After centrifugation at 8,000 rpm and 4°C, the formed aggregates were collected. Total RNA was extracted using TRIzol reagent (Ambion, United States). cDNA was obtained by reverse transcription using the Transcript All-in-One First-Strand DNA Synthesis SuperMix for qPCR (OneStep gDNA Removal) Kit (Tsingke, China). qRT-PCR was performed using a set of two PCR primers with SYBR Green I Real-Time PCR (Solarbio, China) using a 7500 Real-time PCR system (Applied Biosystems, United States). Genes related to cell wall formation (CHS1, CHS2, CHS3, and GSH) and melanin synthesis (PKS12 and PKS13) were examined. Actin transcript was used as the internal reference gene (Melo et al., 2019). Primers are shown in Supplementary Table S1. The relative gene expression was calculated by the 2−ΔΔCt method. Experiments were repeated five times.
Determination of Chitin Content
After suspension of S. sclerotiorum mycelial fragments in 50 ml YEPD medium (1 × 106 mycelial fragments/mL) containing 0 and 10 mM KA (0 and 1.42 mg/ml KA), the cells were shaken at 28°C and 200 rpm for 0, 1, 2, and 3. Chitin content was determined using the procedure described by Song et al. (2020). Briefly, after centrifugation (8,000 rpm, 4°C, 10 min) of the cells contained in the 50 ml YEPD medium, the fungal cells were hydrolyzed in 1 ml 6 M HCl at 100°C for 17 h. After evaporation of the solvent using a freeze-drier (FreeZone 2.5 Plus, LABCONCO, United States), the samples were dissolved in 1 ml water. Then, 0.75 M Na2CO3 solution (100 μl) was added to 100 μl sample. The mixture was incubated at 100°C for 20 min. Then, 95% ethanol (0.7 ml) and solution A (100 μl; solution A: 1.6 g p-dimethylaminobenzaldehyde in 30 ml concentrated HCl and 30 ml ethanol) were added. The absorbance was measured at 420 nm and compared with the standard curve from 0.1 to 20 mg/ml glucosamine. Experiments were repeated three times.
Determination of Oxalic Acid Content
Two 7-mm-diameter PDA plugs containing S. sclerotiorum mycelium were inoculated into 50 ml YEPD medium with 0, 2, 5, and 10 mM KA (0, 0.28, 0.71, and 1.42 mg/ml KA). Samples were collected after 0, 1, 2, and 3 days. The content of oxalic acid was measured using the Oxalic Acid Content Detection (Visible Spectrophotometry) Kit (Solarbio, China). Briefly, 50 μl of culture medium were mixed with reagents 1, 2, and 3 following the manufacturer’s instructions, and the resulting solution was kept at room temperature for 20 min. The concentration of oxalic acid was calculated according to the absorbance at 510 nm. Experiments were repeated three times.
Curative and Preventive Efficacies of KA for the Management of Sclerotinia sclerotiorum in Soybean Pods
Sclerotinia sclerotiorum was cultured on PDA medium at 28°C for 5 days, and the mycelium was divided into 7-mm-diameter plugs. For the preventive assay, 2-mm-diameter wounds were made on the surface of soybean pods directly detached from soybean plants. After spraying 50 ml aqueous solutions (pH 5) containing 0, 10, 20, and 50 mM KA (0, 1.42, 2.84, and 7.11 mg/ml KA), the soybean pods were dried, and a mycelial plug was kept in contact with the wound. Carbendazim and prochloraz at 20 and 50 mM (carbendazim: 3.82 and 9.56 mg/ml; prochloraz: 7.53, and 18.84 mg/ml) were applied, instead of KA, as positive controls (4 kg/ha carbendazim and 0.45 kg/ha prochloraz are the recommended rates in fields trials). After 3 days at 28°C and 70% humidity, the preventive efficacy was measured according to the lesion length (necrotic area) caused by S. sclerotiorum.
For the curative assay, 2-mm-diameter wounds were made on the soybean pods. After inoculation using a mycelial plug, the inoculated soybean pods were incubated at 28°C and 70% humidity for 1 day and the mycelial plugs were removed. Then, 50 ml aqueous solutions containing KA, prochloraz, or carbendazim at 20 and 50 mM concentration were sprayed. Sterilized ddH2O (50 ml) was sprayed in the control experiment. Treatments with combinations of fungicides were carried out using a 50 ml solution containing the fungicides at 10 mM concentration (KA: 1.42 mg/ml; carbendazim: 1.91 mg/ml; and prochloraz: 3.77 mg/ml).
Twenty soybean pods were used for each treatment condition. Experiments were repeated three times. The brownish-black color of the lesions caused by S. sclerotiorum, covered in some cases by white hyphae, was easily observed with the naked eye. All the lesion length measurements were pooled to calculate the mean value and deviation.
Data Analysis
The statistical analyses were performed using SPSS (Statistical Package, Version 20.0). The variables were subjected to student’s t-test and were tested for significance at p < 0.05 (*), p < 0.01 (**), p < 0.001 (***), and p < 0.0001 (****) levels (ns = no significance). The SD, which was calculated using Microsoft Excel 2010, was used to quantify the dispersion.
Results
KA Shows Antifungal Activity Against Sclerotinia sclerotiorum and Inhibits Sclerotia Formation
Although KA at 15 mM slightly reduced the mycelial growth of B. dothidea, C. brevisporum, and V. pyri, this concentration of KA inhibited completely the growth of S. sclerotiorum (Figure 1A), whereas 5 and 10 mM KA reduced S. sclerotiorum growth by 29 and 56%, respectively (Supplementary Figure S2). These results indicated that S. sclerotiorum is more sensitive to KA in comparison to the other fungal species used in the assay. After 15 days of incubation, no mycelial growth was detected when treating S. sclerotiorum with 15 mM KA. When comparing the antifungal activity of KA with that of commercial fungicides carbendazim and prochloraz, it was found that the commercial fungicides showed higher antifungal activity in vitro than KA. The EC50 value for KA was 6.3 ± 0.7 mM (0.89 ± 0.09 mg/ml), while the EC50 values for carbendazim and prochloraz were 2.8 ± 0.3 (0.51 ± 0.06 mg/ml) and 0.7 ± 0.2 mM (0.26 ± 0.07 mg/ml), respectively.
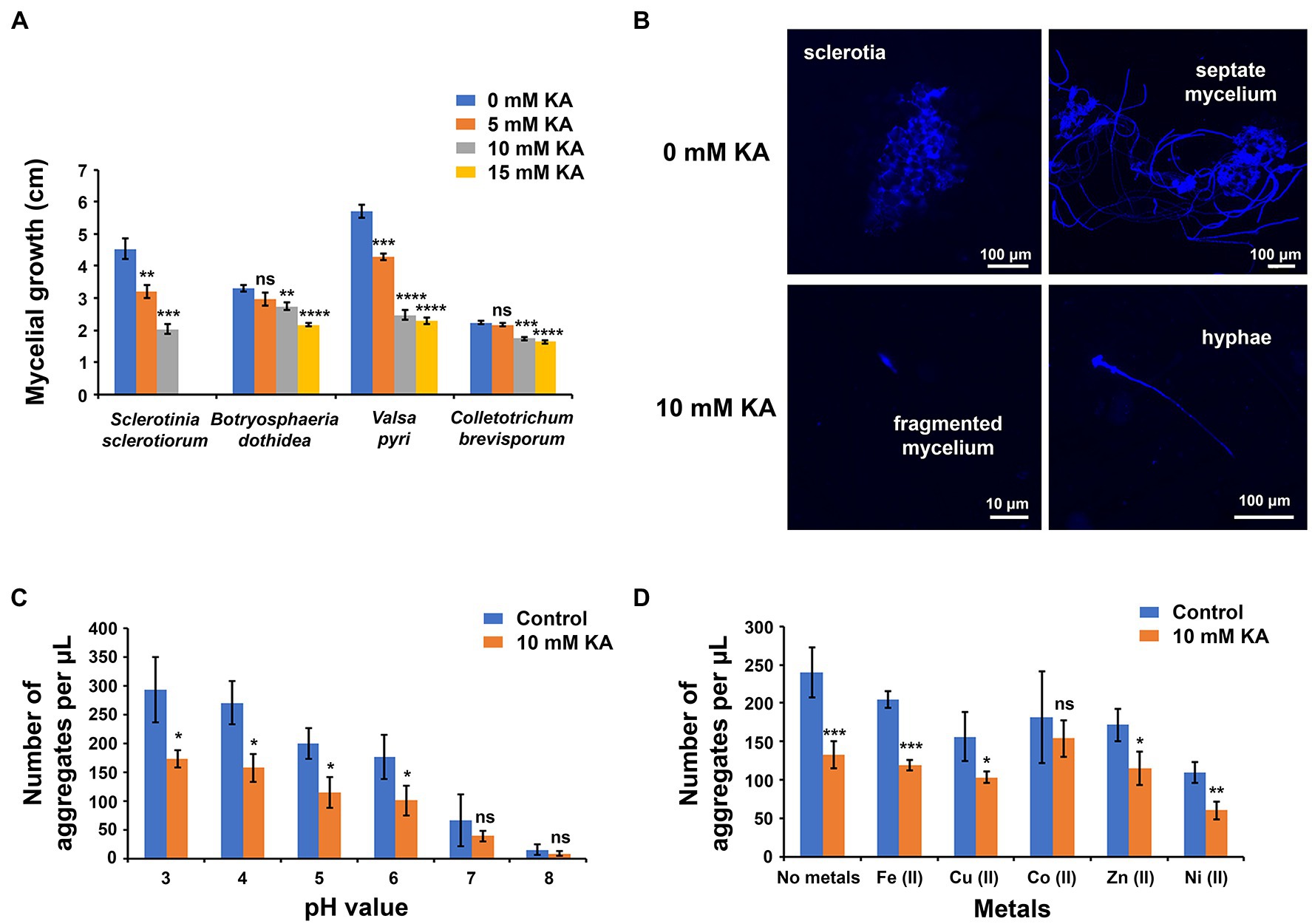
Figure 1. Antifungal properties of kojic acid (KA). (A) Antifungal activity of KA against fungal pathogens Sclerotinia sclerotiorum, Botryosphaeria dothidea, Valsa pyri, and Colletotrichum brevisporum. The antifungal activity was measured according to the inhibition of mycelial growth (each treatment mean represents the average of five repetitions). (B) Fluorescent microscope observations of S. sclerotiorum growth with and without KA treatment (10 mM; 1.42 mg/ml). (C) Effect of pH on the antifungal activity of 10 mM KA against S. sclerotiorum (each treatment mean represents the average of three repetitions). (D) Effect of metal ions (1 mM) on the antifungal activity of 10 mM KA against S. sclerotiorum at pH 4 (each treatment mean represents the average of three repetitions). Significance levels at *p < 0.05, **p < 0.01, ***p < 0.001, ****p < 0.0001, and no significance (ns). Bars represent the SD.
Fluorescent microscope observations revealed that, in the absence of KA, S. sclerotiorum mycelial fragments formed sclerotial aggregates (number of observed sclerotia: 64), and only a few non-attached hyphae were detected (number of observed hyphae: 26; Figure 1B; Supplementary Figure S3). In some cases, hyphal structures were observed growing from the sclerotial aggregates (number of observed sclerotia with hyphae: 53; number of sclerotia without hyphae: 11). In contrast, after treatment with 10 mM KA, most S. sclerotiorum mycelial fragments remained in the original state (number of observed mycelial fragments: 112), and the number of hyphae increased in comparison with that observed in the absence of KA (number of observed hyphae: 53). After treatment with KA, no sclerotial aggregates were observed, indicating that KA was able to inhibit the formation of sclerotia and, subsequently, to temporarily halt S. sclerotiorum cell cycle.
The antifungal activity of KA was screened at different pH values, from 3 to 8. Although S. sclerotiorum could grow at all pH values, the formation of sclerotial aggregates was higher in an acidic environment than under alkaline conditions. The number of aggregates per microliter was 270 at pH 3, whereas the number of aggregates decreased to 15 at pH 8. In all tested pH values, the inhibitory activity of KA against S. sclerotiorum varied from 40 to 45%, revealing that the antifungal activity of KA was not obviously affected by pH (Figure 1C).
The presence of metals inhibited the formation of sclerotial aggregates (Figure 1D). In this sense, nickel(II) and copper(II) reduced the number of aggregates by 53 and 32%, respectively, in comparison to the control experiment. When KA treatment was combined with metal ions, the antifungal effect of KA was significantly reduced, with cobalt(II) and zinc(II) producing the greatest effect. KA, in the presence of cobalt(II) and zinc(II), reduced the number of aggregates per μl by 28 and 33%, respectively; whereas KA, without metal addition, reduced the number of aggregates by 45%.
KA Inhibits Melanin Biosynthesis in Sclerotinia sclerotiorum
Cultivation of S. sclerotiorum on PDA plates allowed the formation of sclerotia after 15 days (96 ± 19 sclerotia per plate), which could be easily observed due to the dark color produced by melanin (Figure 2A; Sousa Melo et al., 2019). Instead, the presence of 10 mM KA inhibited the formation of sclerotia (6 ± 3 sclerotia per plate), and cultures were completely white showing only non-melanized structures.
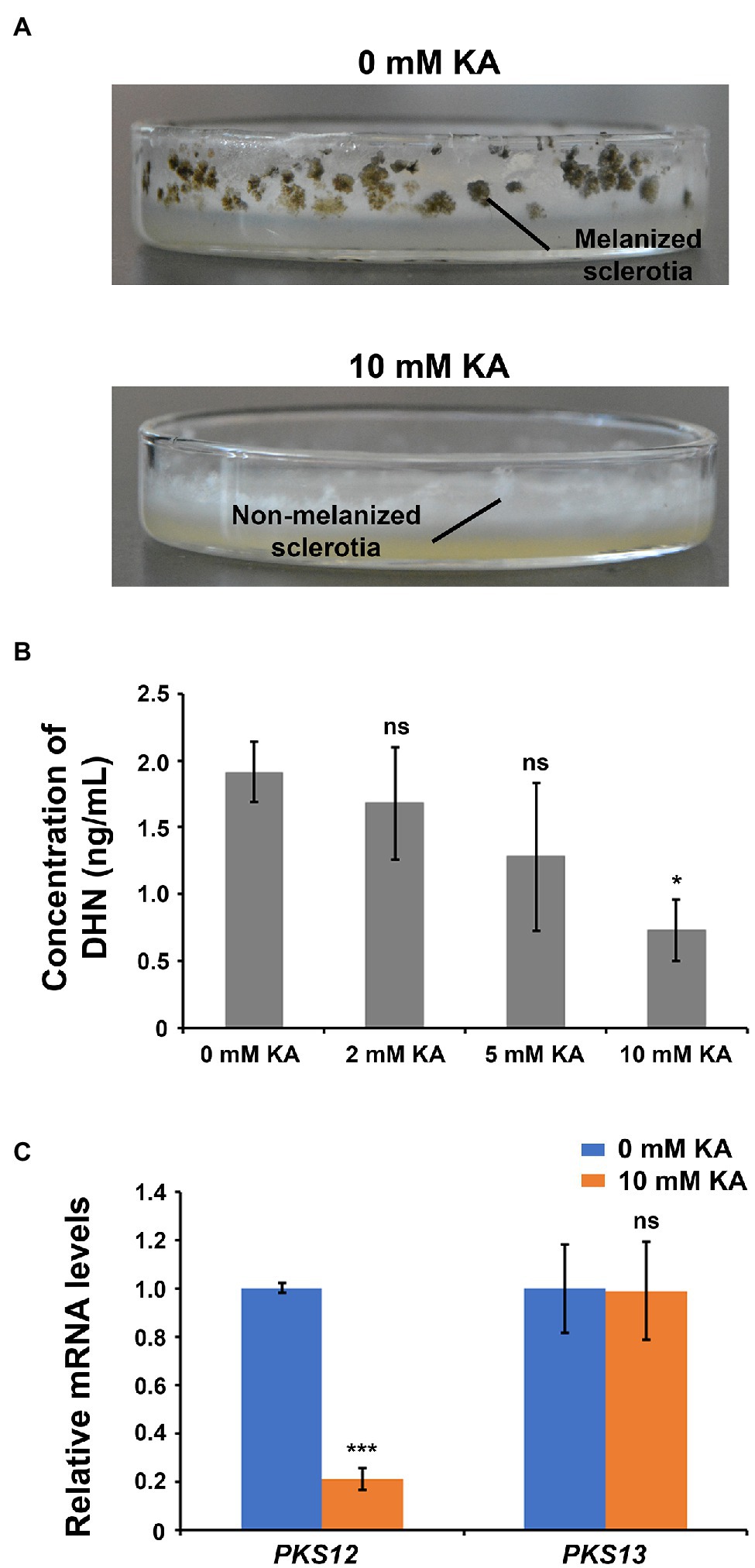
Figure 2. Kojic acid inhibits melanin biosynthesis and sclerotia formation in Sclerotinia sclerotiorum. (A) Images showing sclerotia formation after treatment with 10 mM KA (1.42 mg/ml KA). (B) Concentration of 1,8-dihydroxynaphthalene (DHN) in S. sclerotiorum cells after treatment with 10 mM KA (each treatment mean represents the average of three repetitions). (C) Relative mRNA levels of key genes involved in the biosynthesis of DHN and other melanin compounds after treatment with 10 mM KA (each treatment mean represents the average of five repetitions). Significance levels at *p < 0.05, ***p < 0.001, and no significance (ns). Bars represent the SD.
In order to confirm that KA was inhibiting the biosynthesis of melanin in S. sclerotiorum, the content of DHN, a kind of melanin and a key intermediate involved in biosynthesis of other melanin compounds in S. sclerotiorum (Supplementary Figure S4; Butler et al., 2009), was examined after application of different concentrations of KA. In agreement with the lack of dark sclerotia in the plates, 2, 5, and 10 mM KA reduced DHN content by 12% (no significant difference), 32% (no significant difference), and 62% (significant difference), respectively (Figure 2B); confirming that KA is inhibiting the biosynthesis of melanin. Consistently, the expression of polyketide synthase PKS12, which is involved in melanin biosynthesis, was found 4.8-fold downregulated in the presence of 10 mM KA, whereas no significant difference was observed in the mRNA level of polyketide synthase PKS13 after KA treatment (Figure 2C).
KA Inhibits Chitin Biosynthesis and Alters Cell Wall Integrity in Sclerotinia sclerotiorum
The antifungal activity assay indicated that KA can not only inhibit the formation of sclerotia, but can also inhibit S. sclerotiorum mycelial growth. SEM observations revealed that KA was altering cell wall integrity (Figure 3A). KA-treated cells (10 mM KA) showed numerous holes and irregularities in the cell wall that were not observed in the non-treated cells.
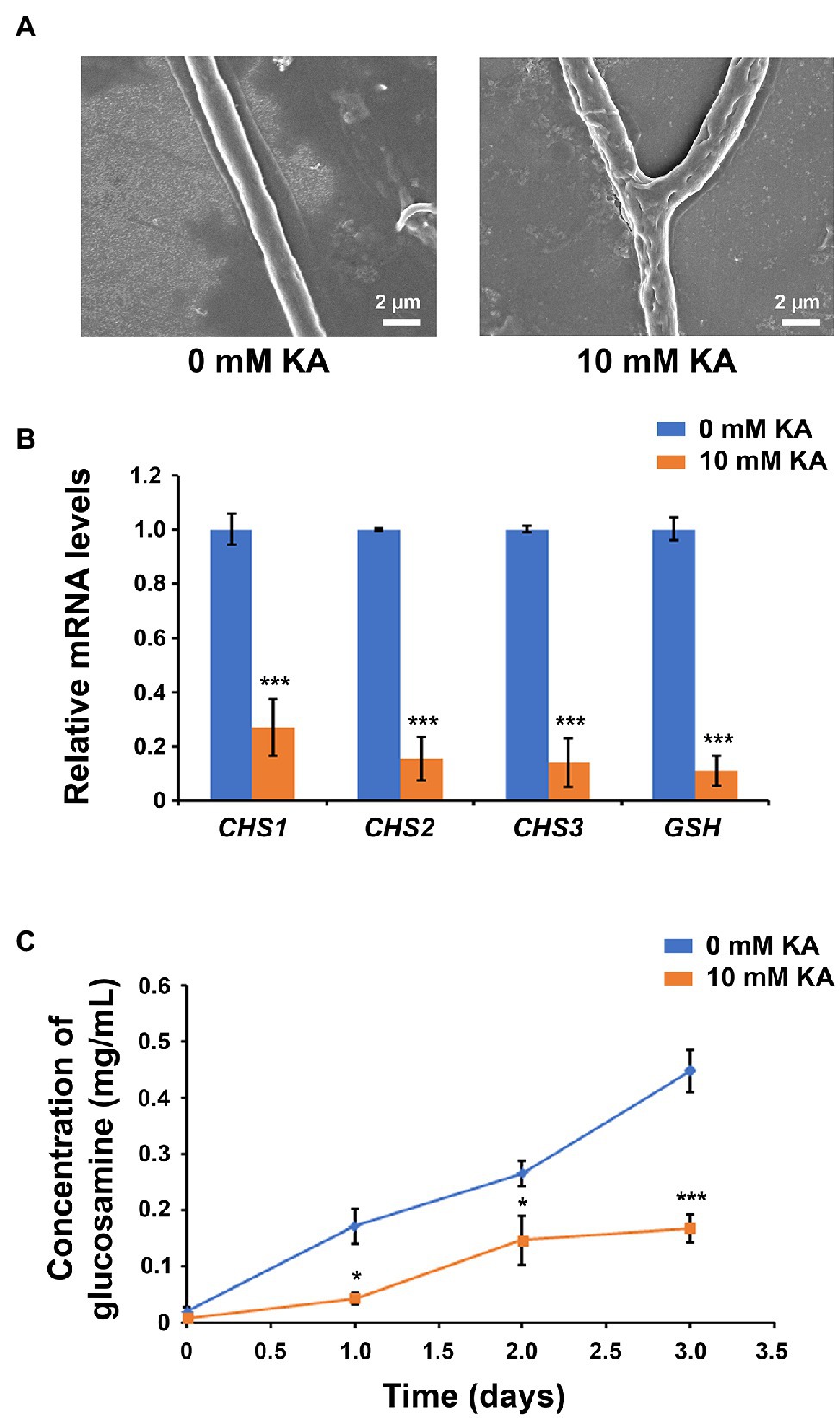
Figure 3. Kojic acid inhibits chitin synthesis and alters cell wall integrity in Sclerotinia sclerotiorum. (A) Scanning electron microscope (SEM) observations of S. sclerotiorum cell wall after treatment with 10 mM KA (1.42 mg/ml KA). (B) Relative mRNA levels of chitin synthase genes CHS1, CHS2, and CHS3, and β-1,3-glucan synthase gene GSH, in S. sclerotiorum after treatment with 10 mM KA (each treatment mean represents the average of five repetitions). (C) Concentration of chitin, measured by the concentration of glucosamine after hydrolysis, in S. sclerotiorum cells after treatment with 10 mM KA (each treatment mean represents the average of three repetitions). Significance levels at *p < 0.05 and ***p < 0.001. Bars represent the SD.
In order to understand the metabolic alterations caused by KA in S. sclerotiorum cell wall, the mRNA levels of three chitin synthase genes (CHS1, CHS2, and CHS3) and one β-1,3-glucan synthase gene (GSH) were measured after KA treatment (10 mM KA; Figure 3B). qRT-PCR analysis revealed that the gene expression of these genes was significantly reduced under KA treatment. The gene expression of GSH was 9.3-fold lower in KA-treated cells compared to that detected without KA treatment, while the gene expression of CHS1, CHS2, and CHS3 decreased by 73, 85, and 87%, respectively. These results suggested that KA is not only inhibiting melanin biosynthesis but also inhibiting the biosynthesis of chitin and β-1,3-glucans.
To confirm that KA was able to inhibit chitin biosynthesis, the concentration of chitin in the KA-treated cells was measured after 0, 1, 2, and 3 days (Figure 3C). Although chitin content increased steadily in non-treated cells, 10 mM KA significantly decreased chitin content, which is consistent with the mRNA levels of the chitin synthase genes after treatment with KA. The concentration of chitin decreased by 75, 45, and 63% in the KA-treated cells after 1, 2, and 3 days, respectively.
KA Reduces Oxalic Acid Content
The concentration of virulence factor oxalic acid when culturing S. sclerotiorum in the absence of KA was 3.13 ± 0.76, 3.38 ± 0.67, and 4.05 ± 0.73 μM after 1, 2, and 3 days, respectively (Figure 4). The concentration of oxalic acid was reduced by 12% (no significant difference) and 91% after application of 2 and 5 mM KA, respectively. When treating S. sclerotiorum cells with 10 mM KA, no oxalic acid was observed. These results demonstrated that KA is able to inhibit oxalic acid production in S. sclerotiorum.
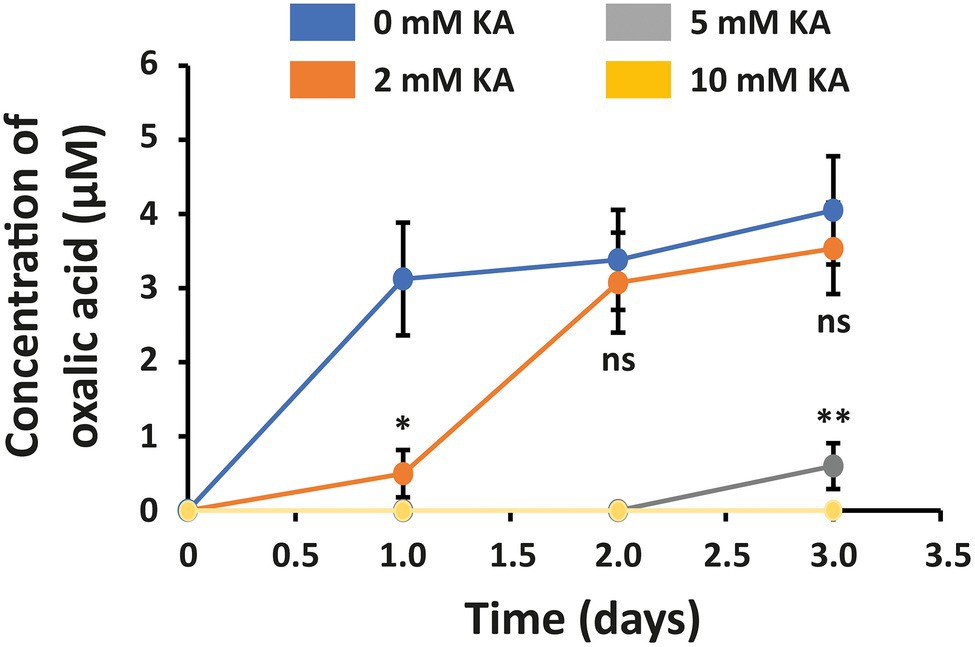
Figure 4. Concentration of oxalic acid in Sclerotinia sclerotiorum secretions after treatment with 2, 5, and 10 mM KA (0.28, 0.71, and 1.42 mg/ml KA). The control experiment was carried out in the absence of KA. Each treatment mean represents the average of three repetitions. Significance levels at *p < 0.05 and **p < 0.01. Bars represent the SD.
KA Is Able to Reduce the Symptoms of Sclerotinia sclerotiorum in Soybean Pods
Kojic acid showed both curative and preventive abilities, reducing the symptoms of S. sclerotiorum in soybean (Figures 5A,B). In preventive applications (Table 1), the lesion length inhibition was proportional to the concentration of KA, observing complete inhibition with 50 mM KA. This, carbendazim and prochloraz at 20 mM provided 64, 65, and 53% lesion length inhibition, respectively, indicating that KA shows similar antifungal activity in preventive applications in comparison with commonly used fungicides.

Figure 5. Efficacy of KA and some commercial fungicides for the control of Sclerotinia sclerotiorum in soybean pods. (A) Preventive applications. (B) Curative applications.
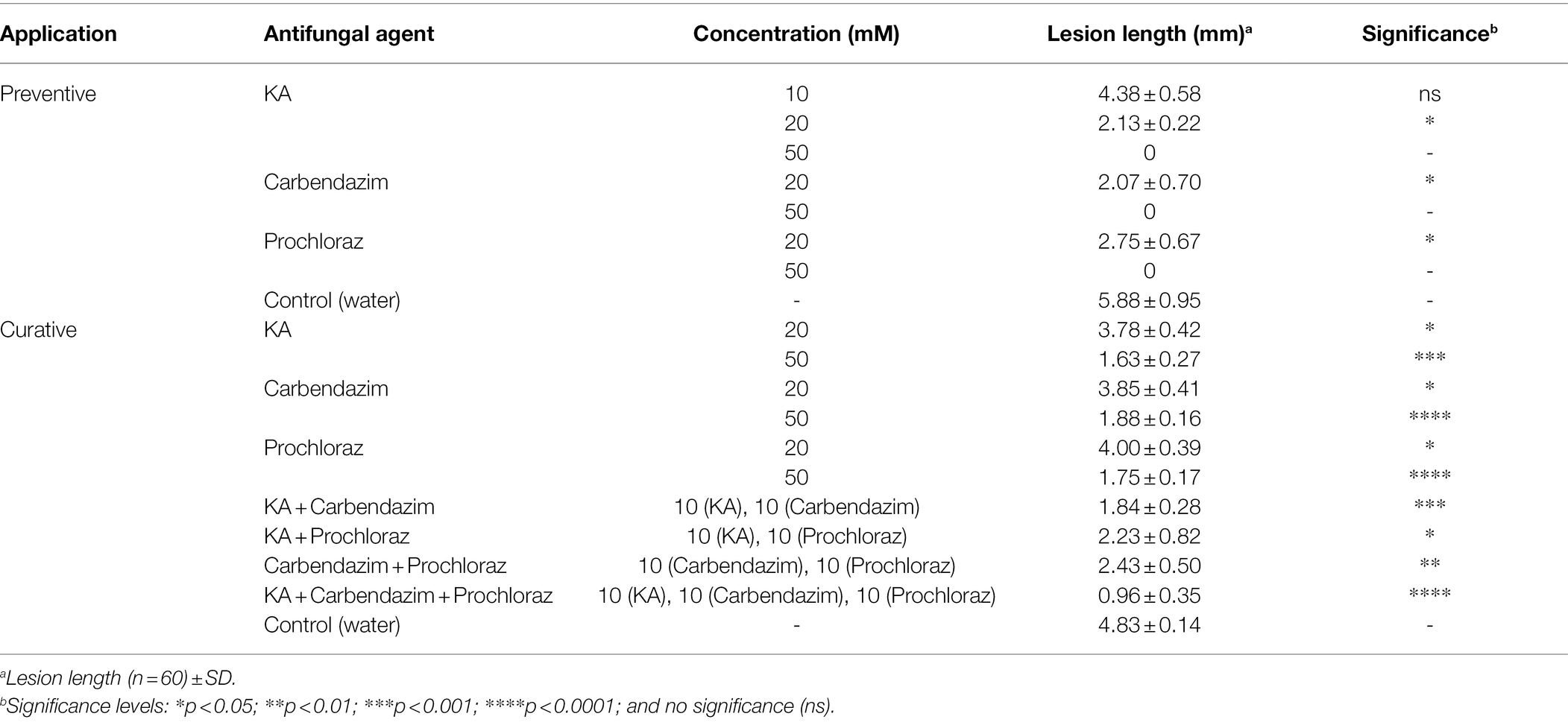
Table 1. Efficacy of kojic acid (KA; MW: 142.1 g/mol) and commercial fungicides carbendazim (MW: 191.2 g/mol), and prochloraz (MW: 376.7 g/mol) for the control of Sclerotinia sclerotiorum in soybean pods.
In curative applications, the lesion length inhibition of 20 mM KA was slightly higher than those of carbendazim and prochloraz at the same concentration (KA: 22%; carbendazim: 20%; and prochloraz: 17% lesion length inhibition), and the same trend was also observed when using 50 mM concentrations (KA: 66%; carbendazim: 61%; prochloraz: 64% lesion length inhibition). Comparison between the curative and preventive abilities revealed that the three antifungal agents used in this study showed higher preventive ability in comparison with curative ability on S. sclerotiorum. After combining 10 mM KA with 10 mM carbendazim or 10 mM KA with 10 mM prochloraz, the inhibitory effect was higher than that detected with the treatment with single fungicides at 20 mM concentration (KA + carbendazim: 62%; KA + prochloraz: 54% lesion length inhibition). When 10 mM KA was applied together with 10 mM carbendazim and 10 mM prochloraz, the inhibitory effect was enhanced up to 80% lesion length inhibition.
Discussion
In order to evaluate the antifungal activity of KA, this was screened against four fungal plant pathogens, including B. dothidea, C. brevisporum, S. sclerotiorum, and V. pyri. These species were selected for the screening because they were recently isolated by our research group, and suppose a relevant threat in China. Botryosphaeria dothidea and C. brevisporum were found causing stem canker and anthracnose on soybean, whereas V. pyri was isolated from pear trunk (Chen et al., 2020; Shi et al., 2020; Song et al., 2020). The obtained results indicated that KA shows much stronger antifungal activity against S. sclerotiorum than against the other strains and, for this reason, the rest of the study was performed using S. sclerotiorum. As it is common in the studies using S. sclerotiorum, only one strain was isolated and this strain was used in the experiments (Clarkson et al., 2014; Ordonez-Valencia et al., 2015; Ranjan et al., 2019).
Regarding the antifungal activity of KA, this was previously reported to show weak antifungal activity in vitro against Glomerella cingulata and Botrytis cinerea (Wei and Ji, 2016), and to inhibit the growth of some human pathogenic yeasts, including Candida albicans (MIC = 4.5 M), Cryptococcus neoformans (MIC = 0.56 M), and Trichophyton rubrum (MIC = 1.13 M; Chee and Lee, 2003). When comparing the antifungal activity of KA to S. sclerotiorum and mentioned yeasts, it can be concluded that S. sclerotiorum (MIC = 15 mM) is much more sensitive than other fungal strains, which is in agreement with the antifungal assay carried out in this work. The antifungal activity of KA in combination with H2O2 was screened against Penicillium species and Aspergillus fumigatus (Kim et al., 2012; Kim and Chan, 2014). Carbendazim and prochloraz showed higher ability to inhibit mycelial growth than KA. The EC50 value obtained for prochloraz in this work was in agreement with the EC50 values observed for prochloraz against other S. sclerotiorum strains (Zhang et al., 2018).
Microscope observations revealed that the agglutination of the fragmented mycelia formed sclerotia; however, the treatment with KA inhibited sclerotia formation. As in the case of KA, Soylu et al. (2007) reported that essential oils from oregano blocked the formation of sclerotia in S. sclerotiorum. SEM observations of S. sclerotiorum sclerotia revealed that essential oils from oregano were able to cause important morphological alterations. The sclerotia of S. sclerotiorum can show different size and shape (Ordonez-Valencia et al., 2015; Nahar et al., 2020). As indicated in the “Materials and Methods” section, experiments were carried out at 28°C. Although the optima temperatures for mycelial growth and sclerotia germination are between 18 and 22°C, the production of sclerotia, which was the main target in this work, is usually induced at slightly higher temperatures, between 24 and 30°C (Abdullah et al., 2008; Euteneuer et al., 2021).
When evaluating the inhibitory activity of KA at different pH conditions, it was observed that the formation of sclerotia was higher at acidic pH values than at alkaline conditions. In agreement, Xu et al. (2015) indicated that the formation of sclerotia by S. sclerotiorum was mainly produced at low pH values. The synthesis of oxalic acid by S. sclerotiorum has been reported to be involved in the reduction of environmental pH, facilitating the formation of sclerotia. The treatment of S. sclerotiorum with KA inhibited the production of acidifying agent oxalic acid, which is consistent with the observed inhibition of sclerotia formation. In this work, it was found that the formation of sclerotia was reduced by some metal ions, such as nickel(II) and copper(II). As far as we know, this is the first study of the effect of metals on sclerotia formation. The addition of metal ions reduced the inhibitory activity of KA, which can be explained considering the ability of KA to coordinate with metals in solution. This fact suggests that KA should not be combined with metal-based fungicides to achieve a high efficacy.
Kojic acid inhibited the formation of melanin DHN in S. sclerotiorum, which is necessary for sclerotia formation. In agreement with our result, Lujan et al. (2016) reported the use of KA, phthalide, and tricyclazole to avoid the pigmentation in S. sclerotiorum. As indicated in the “Introduction” section, KA is a copper chelator and is known to inhibit tyrosinase activity in human cells reducing the content of melanin. Sclerotinia sclerotiorum also contains a copper-dependent tyrosinase that is known to be involved in melanin formation using L-dopa as an intermediate (Melo et al., 2019). However, S. sclerotiorum contains another biosynthetic pathway responsible for the synthesis of DHN melanin, which seems to be more important for the formation of sclerotia than the L-dopa-related pathway (Melo et al., 2019). The DHN melanin pathway does not involve the participation of the tyrosinase but involves the participation of the polyketide synthase PKS12, which gene expression was found to be downregulated after KA treatment, and a copper-dependent laccase. Although the obtained results demonstrated that KA is able to inhibit DHN biosynthesis and sclerotia formation in S. sclerotiorum, further studies are necessary in order to understand the target enzymes that are affected by KA. Disruption of SCD1 or THR1, which are involved in melanin biosynthesis in S. sclerotiorum, did not change the pathogenicity of the fungus but resulted in slower radial growth, less biomass, wider angled hyphal branches, and impaired sclerotial development (Liang et al., 2018).
In this study, KA was found to inhibit chitin and β-1,3-glucan biosynthesis in S. sclerotiorum, altering fungal cell wall. Both structures, chitin and β-1,3-glucans, are known to play an important role in cell wall formation and mycelial growth in S. sclerotiorum and have been associated with virulence (Ding et al., 2019). Fungicide prochloraz, which also shows chelating properties, was reported to inhibit chitin biosynthesis in S. sclerotiorum (Zhang et al., 2018). The expression of chitin and β-1,3-glucan synthases is known to occur along with the formation of melanin in S. sclerotiorum, and this effect must be produced due to chitin and β-1,3-glucans serve as a scaffold to which the melanin granules are cross-linked (Melo et al., 2019). In agreement with that report, the treatment with KA simultaneously downregulated the biosyntheses of melanin, chitin, and β-1,3-glucans, suggesting that there must be some connections between these processes.
As indicated in the “Results” section, the treatment with KA reduced the content of oxalic acid in S. sclerotiorum secretions. Oxalic acid is one of the major virulence factors of S. sclerotiorum and is involved in programmed cell death (PCD) in plants (Cessna et al., 2000; Williams et al., 2011; Jiao et al., 2021). These results suggest that KA is reducing the pathogenicity of S. sclerotiorum, which is in agreement with the ability of KA to reduce the lesions of S. sclerotiorum in vivo. Although there are several reports confirming the antifungal activity of KA against plant fungal pathogens in vitro, this is the first study on the use of KA for the management of a fungal pathogen in vivo.
As mentioned in “KA is able to reduce the symptoms of S. sclerotiorum in soybean pods” section, KA showed similar, or slightly higher, efficacy in comparison with commercial fungicides carbendazim and prochloraz. Interestingly, combinations of KA with mentioned commercial fungicides improved the efficacy of the commercial fungicides in curative applications. These results can be explained considering the ability of KA to modify cell wall composition, which may improve the antifungal effects of the commercial fungicides. The mode of action of carbendazim is based on the inhibition of microtubule polymerization in cells by acting with β-tubulin, which leads to impaired segregation of chromosomes during cell division (Wang et al., 2020); while prochloraz, which is an imidazole fungicide, can block the biosynthesis of ergosterol in fungus by inhibiting the enzymatic activity of iron(II)-dependent lanosterol 14-α demethylase (Zhang et al., 2018). This study reveals an interesting strategy to enhance the activity of commercial agents for the management of S. sclerotiorum, and provides new insights on the use of KA for the control of fungal plant diseases.
Data Availability Statement
The datasets presented in this study can be found in online repositories. The names of the repository/repositories and accession number(s) can be found in the article/Supplementary Material.
Author Contributions
PL, X-CS, and S-YW designed the experiments. G-YZ and BW performed the experiments. PL and X-CS analyzed the data. PL, S-YW, and G-YZ drafted the manuscript. All authors contributed to the article and approved the submitted version.
Funding
This study was supported by the National Natural Science Foundation of China (81803407, 3201101306, and 32172441), the Nantong Applied Research Program (JC2020103), the Social and Livelihood Project of Nantong (MS12020069), and the Large Instruments Open Foundation of Nantong University (KFJN2130 and KFJN2135).
Conflict of Interest
The authors declare that the research was conducted in the absence of any commercial or financial relationships that could be construed as a potential conflict of interest.
Publisher’s Note
All claims expressed in this article are solely those of the authors and do not necessarily represent those of their affiliated organizations, or those of the publisher, the editors and the reviewers. Any product that may be evaluated in this article, or claim that may be made by its manufacturer, is not guaranteed or endorsed by the publisher.
Supplementary Material
The Supplementary Material for this article can be found online at: https://www.frontiersin.org/articles/10.3389/fpls.2022.845698/full#supplementary-material
References
Abdullah, M. T., Ali, N. Y., and Suleman, P. (2008). Effect of salinity, temperature and carbon source on the growth and development of sclerotia of Sclerotinia sclerotiorum isolated from semi-arid environment. Plant Pathol. J. 24, 407–416. doi: 10.5423/PPJ.2008.24.4.407
Becka, D., Prokinova, E., Simka, J., Cihlar, P., Beckova, L., Bokor, P., et al. (2016). Use of petal test in early-flowering varieties of oilseed rape (Brassica napus L.) for predicting the infection pressure of Sclerotinia sclerotiorum (Lib.) de Bary. Crop Prot. 80, 127–131. doi: 10.1016/j.cropro.2015.11.006
Bolton, M. D., Thomma, B., and Nelson, B. D. (2006). Sclerotinia sclerotiorum (Lib.) de Bary: biology and molecular traits of a cosmopolitan pathogen. Mol. Plant Pathol. 7, 1–16. doi: 10.1111/J.1364-3703.2005.00316.X
Butler, M. J., Gardiner, R. B., and Day, A. W. (2009). Melanin synthesis by Sclerotinia sclerotiorum. Mycologia 101, 296–304. doi: 10.3852/08-120
Cabanes, J., Chazarra, S., and Garcia-Carmona, F. (1994). Kojic acid, a cosmetic skin whitening agent, is a slow-binding inhibitor of catecholase activity of tyrosinase. J. Pharm. Pharmacol. 46, 982–985. doi: 10.1111/j.2042-7158.1994.tb03253.x
Cabezudo, I., Ramallo, I. A., Alonso, V. L., and Furlan, R. L. E. (2021). Effect directed synthesis of a new tyrosinase inhibitor with anti-browning activity. Food Chem. 341:128232. doi: 10.1111/j.2042-7158.1994.tb03253.x
Cessna, S. G., Sears, V. E., Dickman, M. B., and Low, P. S. (2000). Oxalic acid, a pathogenicity factor for Sclerotinia sclerotiorum, suppresses the oxidative burst of the host plant. Plant Cell 12, 2191–2199. doi: 10.1105/tpc.12.11.2191
Chee, H. Y., and Lee, E. H. (2003). Fungistatic activity of kojic acid against human pathogenic fungi and inhibition of melanin production in Cryptococcus neoformans. Mycobiology 31, 248–250. doi: 10.4489/MYCO.2003.31.4.248
Chen, T., Shi, X., Wang, S., and Laborda, P. (2020). First report of Botryosphaeria dothidea causing stem canker on soybean in China. Plant Dis. 105:1216. doi: 10.1094/PDIS-11-20-2398-PDN
Clarkson, J. P., Fawcett, L., Anthony, S. G., and Young, C. (2014). A model for Sclerotinia sclerotiorum infection and disease development in lettuce, based on the effects of temperature, relative humidity and ascospore density. PLoS One 9:e94049. doi: 10.1371/journal.pone.0094049
Del Rio, L. E., Martison, C. A., and Yang, X. B. (2002). Biological control of Sclerotinia stem rot of soybean with Sporidesmium sclerotivorum. Plant Dis. 86, 999–1004. doi: 10.1094/PDIS.2002.86.9.999
Ding, Y., Chen, Y., Yan, B., Liao, H., Dong, M., Meng, X., et al. (2021). Host-induced gene silencing of a multifunction gene Sscnd1 enhances plant resistance against Sclerotinia sclerotiorum. Front. Microbiol. 12:693334. doi: 10.3389/fmicb.2021.693334
Ding, Y. J., Mei, J. Q., Chai, Y. R., Yu, Y., Shao, C. G., Wu, Q. A., et al. (2019). Simultaneous transcriptome analysis of host and pathogen highlights the interaction between Brassica oleracea and Sclerotinia sclerotiorum. Phytopathology 109, 542–550. doi: 10.1094/PHYTO-06-18-0204-R
Eisenman, H. C., and Casadevall, A. (2012). Synthesis and assembly of fungal melanin. Appl. Microbiol. Biotechnol. 93, 931–940. doi: 10.1007/s00253-011-3777-2
Euteneuer, P., Wagentristl, H., Pauer, S., Keimerl, M., Schachinger, C., Bodner, G., et al. (2021). Field investigation of topsoil moisture and temperature as drivers for decomposition or germination of sclerotia (Sclerotinia sclerotiorum) under winter-killed cover crops. Acta Agric. Scand. B Soil Plant Sci. doi: 10.1080/09064710.2021.2018034 (in press).
Food and Agricultural Organization of the United Nations (FAO) (2019). Available at: http://www.fao.org/faostat/en/#data/QC/visualize (Accessed December 25, 2021).
Gao, Y. Y., He, L. F., Zhu, J. M., Cheng, J. G., Li, B. X., Liu, F., et al. (2020). The relationship between features enabling SDHI fungicide binding to the Sc-Sdh complex and its inhibitory activity against Sclerotinia sclerotiorum. Pest Manag. Sci. 76, 2799–2808. doi: 10.1002/ps.5827
Giorgio, A., De Stradis, A., Lo Cantore, P., and Iacobellis, N. S. (2015). Biocide effects of volatile organic compounds produced by potential biocontrol rhizobacteria on Sclerotinia sclerotiorum. Front. Microbiol. 6:1056. doi: 10.3389/fmicb.2015.01056
Gracia-Garza, J. A., Neumann, S., Vyn, T. J., and Boland, G. J. (2002). Influence of crop rotation and tillage on production of apothecia by Sclerotinia sclerotiorum. Can. J. Plant Pathol. 24, 137–143. doi: 10.1080/07060660309506988
Hu, K. X., Shi, X. C., Xu, D., Laborda, P., Wu, G. C., Liu, F. Q., et al. (2021). Antibacterial mechanism of Biochanin A and its efficacy for the control of Xanthomonas axonopodis pv. glycines in soybean. Pest Manag. Sci. 77, 1668–1673. doi: 10.1002/ps.6186
Huang, H. C. (1991). Induction of myceliogenic germination of sclerotia of Sclerotinia sclerotiorum by exposure to sub-freezing temperatures. Plant Pathol. 40, 621–625. doi: 10.1111/j.1365-3059.1991.tb02427.x
Jiao, W., Liu, X., Li, Y., Li, B., Du, Y., Zhang, Z., et al. (2021). Organic acid, a virulence factor for pathogenic fungi, causing postharvest decay in fruits. Mol. Plant Pathol. 23, 304–312. doi: 10.1111/mpp.13159
Kim, J. H., and Chan, K. L. (2014). Augmenting the antifungal activity of an oxidizing agent with kojic acid: control of Penicillium strains infecting crops. Molecules 19, 18448–18464. doi: 10.3390/molecules191118448
Kim, J. H., Chang, P. K., Chan, K. L., Faria, N. C. G., Mahoney, N., Kim, Y. K., et al. (2012). Enhancement of commercial antifungal agents by kojic acid. Int. J. Mol. Sci. 13, 13867–13880. doi: 10.3390/ijms131113867
Kogej, T., Wheeler, M. H., Rizner, T. L., and Gunde-Cimerman, N. (2004). Evidence for 1,8-dihydroxynaphthalene melanin in three halophilic black yeasts grown under saline and non-saline conditions. FEMS Microbiol. Lett. 232, 203–209. doi: 10.1016/S0378-1097(04)00073-4
Liang, Y., Xiong, W., Steinkellner, S., and Feng, J. (2018). Deficiency of the melanin biosynthesis genes SCD1 and THR1 affects sclerotial development and vegetative growth, but not pathogenicity, in Sclerotinia sclerotiorum. Mol. Plant Pathol. 19, 1444–1453. doi: 10.1111/mpp.12627
Liu, L., Wang, Q., Zhang, X., Liu, J., Zhang, Y., and Pan, H. (2018). Ssams2, a gene encoding GATA transcription factor, is required for appressoria formation and chromosome segregation in Sclerotinia sclerotiorum. Front. Microbiol. 9:3031. doi: 10.3389/fmicb.2018.03031
Lopes, K. B. D., Carpentieri-Pipolo, V., Fira, D., Balatti, P. A., Lopez, S. M. Y., Oro, T. H., et al. (2018). Screening of bacterial endophytes as potential biocontrol agents against soybean diseases. J. Appl. Microbiol. 125, 1466–1481. doi: 10.1111/jam.14041
Lujan, P., Sanogo, S., Puppala, N., and Randall, J. (2016). Factors affecting mycelium pigmentation and pathogenicity of Sclerotinia sclerotiorum on Valencia peanut. Can. J. Plant Sci. 96, 461–473. doi: 10.1139/cjps-2015-0258
Mao, X. W., Wang, Y. F., Hou, Y. P., and Zhou, M. G. (2020). Activity of the succinate dehydrogenase inhibitor fungicide penthiopyrad against Sclerotinia sclerotiorum. Plant Dis. 104, 2696–2703. doi: 10.1094/PDIS-10-19-2253-RE
Massawe, V. C., Hanif, A., Farzand, A., Mburu, D. K., Ochola, S. O., Wu, L. M., et al. (2018). Volatile compounds of endophytic Bacillus spp. have biocontrol activity against Sclerotinia sclerotiorum. Phytopathology 108, 1373–1385. doi: 10.1094/PHYTO-04-18-0118-R
Melo, B. S., Voltan, A. R., Arruda, W., Lopes, F. A. C., Georg, R. C., and Ulhoa, C. J. (2019). Morphological and molecular aspects of sclerotial development in the phytopathogenic fungus Sclerotinia sclerotiorum. Microbiol. Res. 229:126326. doi: 10.1016/j.micres.2019.126326
Miorini, T. J. J., Raetano, C. G., Negrisoli, M. M., and Perez-Hernandez, O. (2021). Determination of the protection period of fungicides used for control of Sclerotinia stem rot in soybean through bioassay and chromatography. Eur. J. Plant Pathol. 159, 877–889. doi: 10.1007/s10658-021-02212-z
Nahar, M. S., Naher, N., Alam, M. J., Hussain, M. J., Yasmin, L., Mian, M. Y., et al. (2020). Survey, morphology and white mold disease of country bean (Lablab purpureus L.) caused by Sclerotinia sclerotiorum (Lib.) de Bary in-relation to soil physico-chemical properties and weather conditions in Bangladesh. Crop Prot. 135:104825. doi: 10.1016/j.cropro.2019.05.019
Ordonez-Valencia, C., Ferrera-Cerrato, R., Quintanar-Zuniga, R. E., Flores-Ortiz, C. M., Marquez Guzman, G. J., Alarcon, A., et al. (2015). Morphological development of sclerotia by Sclerotinia sclerotiorum: a view from light and scanning electron microscopy. Ann. Microbiol. 65, 765–770. doi: 10.1007/s13213-014-0916-x
Peltier, A. J., Bradley, C. A., Chilvers, M. I., Malvick, D. K., Mueller, D. S., Wise, K. A., et al. (2012). Biology, yield loss and control of Sclerotinia stem rot of soybean. J. Integr. Pest Manag. 3, B1–B7. doi: 10.1603/IPM11033
Radhakrishnan, R., Hashem, A., and Abd Allah, E. F. (2017). Bacillus: A biological tool for crop improvement through bio-molecular changes in adverse environments. Front. Physiol. 8:667. doi: 10.3389/fphys.2017.00667
Ranjan, A., Westrick, N. M., Jain, S., Piotrowski, J. S., Ranjan, M., Kessens, R., et al. (2019). Resistance against Sclerotinia sclerotiorum in soybean involves a reprogramming of the phenylpropanoid pathway and up-regulation of antifungal activity targeting ergosterol biosynthesis. Plant Biotechnol. J. 17, 1567–1581. doi: 10.1111/pbi.13082
Ribeiro, A. I., Costa, E. S., Thomasi, S. S., Brandao, D. F. R., Vieira, P. C., Fernandes, J. B., et al. (2018). Biological and chemical control of Sclerotinia sclerotiorum using Stachybotrys levispora and its secondary metabolite griseofulvin. J. Agric. Food Chem. 66, 7627–7632. doi: 10.1021/acs.jafc.7b04197
Rodriguez, M. A., Cabrera, G., Gozzo, F. C., Eberlin, M. N., and Godeas, A. (2011). Clonostachys rosea BAFC3874 as a Sclerotinia sclerotiorum antagonist: mechanisms involved and potential as a biocontrol agent. J. Appl. Microbiol. 110, 1177–1186. doi: 10.1111/j.1365-2672.2011.04970.x
Saeedi, M., Eslamifar, M., and Khezri, K. (2019). Kojic acid applications in cosmetic and pharmaceutical preparations. Biomed. Pharmacother. 110, 582–593. doi: 10.1016/j.biopha.2018.12.006
Shi, X., Wang, S., Duan, X., Gao, X., Zhu, X., and Laborda, P. (2020). First report of Colletotrichum brevisporum causing soybean anthracnose in China. Plant Dis. 105:707. doi: 10.1094/PDIS-09-20-1910-PDN
Song, X. G., Han, M. H., He, F., Wang, S. Y., Li, C. H., Wu, G. C., et al. (2020). Antifungal mechanism of dipicolinic acid and its efficacy for the biocontrol of pear Valsa canker. Front. Microbiol. 11:958. doi: 10.3389/fmicb.2020.00958
Song, X., Ni, M. T., Zhang, Y., Zhang, G. W., Pan, J. H., and Gong, D. M. (2021). Comparing the inhibitory abilities of epigallocatechin-3-gallate and gallocatechin gallate against tyrosinase and their combined effects with kojic acid. Food Chem. 349:129172. doi: 10.1016/j.foodchem.2021.129172
Soylu, S., Yigitbas, H., Soylu, E. M., and Kurt, S. (2007). Antifungal effects of essential oils from oregano and fennel on Sclerotinia sclerotiorum. J. Appl. Microbiol. 103, 1021–1030. doi: 10.1111/j.1365-2672.2007.03310.x
Sumida, C. H., Daniel, J. F. S., Araujod, A. P. C. S., Peitl, D. C., Abreu, L. M., Dekker, R. F. H., et al. (2018). Trichoderma asperelloides antagonism to nine Sclerotinia sclerotiorum strains and biological control of white mold disease in soybean plants. Biocontrol Sci. Tech. 28, 142–156. doi: 10.1080/09583157.2018.1430743
Tian, J., Chen, C., Sun, H., Wang, Z., Steinkellner, S., Feng, J., et al. (2021). Proteomic analysis reveals the importance of exudates on sclerotial development in Sclerotinia sclerotiorum. J. Agric. Food Chem. 69, 1430–1440. doi: 10.1021/acs.jafc.0c06685
Tremarin, A., Longhi, D. A., Salomao, B. C. M., and Aragao, G. M. F. (2015). Modeling the growth of Byssochlamys fulva amd Neosartorya fischeri on solidified apple juice by measuring colony diameter and ergosterol content. Int. J. Food Microbiol. 193, 23–28. doi: 10.1016/j.ijfoodmicro.2014.10.006
Vinodkumar, S., Nakkeeran, S., Renukadevi, P., and Malathi, V. G. (2017). Biocontrol potentials of antimicrobial peptide producing Bacillus species: multifaceted antagonists for the management of stem rot of carnation caused by Sclerotinia sclerotiorum. Front. Microbiol. 8:446. doi: 10.3389/fmicb.2017.00446
Wang, Q., Cheng, S., Xiao, X., Cheng, J., Fu, Y., Chen, T., et al. (2019). Discovery of two mycoviruses by high-throughput sequencing and assembly of mycovirus-derived small silencing RNAs from a hypovirulent strain of Sclerotinia sclerotiorum. Front. Microbiol. 10:1415. doi: 10.3389/fmicb.2019.01415
Wang, S. Y., Shi, X. C., Liu, F. Q., and Laborda, P. (2020). Chromatographic methods for detection and quantification of carbendazim in food. J. Agric. Food Chem. 68, 11880–11894. doi: 10.1021/acs.jafc.0c04225
Wei, S., and Ji, Z. (2016). Isolation, synthesis, and antifungal activity of kojic acid and its derivatives. Chem. Nat. Compd. 52, 123–124. doi: 10.1007/s10600-016-1565-z
Willbur, J. F., Fall, M. L., Bloomingdale, C., Byrne, A. M., Chapman, S. A., Isard, S. A., et al. (2018). Weather-based models for assesing the risk of Sclerotinia sclerotiorum apothecial presence in soybean (Glycine max) fields. Plant Dis. 102, 73–84. doi: 10.1094/PDIS-04-17-0504-RE
Williams, B., Kabbage, M., Kim, H. J., Britt, R., and Dickman, M. B. (2011). Tipping the balance: Sclerotinia sclerotiorum secreted oxalic acid suppresses host defenses by manipulating the host redox environment. PLoS Pathog. 7:e1002107. doi: 10.1371/journal.ppat.1002107
Xia, Z., Wang, Z., Kav, N. N. V., Ding, C., and Liang, Y. (2020). Characterization of microRNA-like RNAs associated with sclerotial development in Sclerotinia sclerotiorum. Fungal Genet. Biol. 144:103471. doi: 10.1016/j.fgb.2020.103471
Xu, T., Li, J., Yu, B., Liu, L., Zhang, X., Liu, J., et al. (2018). Transcription factor SsSte12 was involved in mycelium growth and development in Sclerotinia sclerotiorum. Front. Microbiol. 9:2476. doi: 10.3389/fmicb.2018.02476
Xu, L., Xiang, M., White, D., and Chen, W. (2015). pH dependency of sclerotial development and pathogenicity revealed by using genetically defined oxalate-minus mutants of Sclerotinia sclerotiorum. Environ. Microbiol. 17, 2896–2909. doi: 10.1111/1462-2920.12818
Zeng, W., Kirk, W., and Hao, J. (2012). Field management of Sclerotinia stem rot of soybean using biological control agents. Biol. Control 60, 141–147. doi: 10.1016/j.biocontrol.2011.09.012
Zhang, J., Wang, Y., Du, J., Huang, Z., Fang, A., Yang, Y., et al. (2019). Sclerotinia sclerotiorum thioredoxin reductase is required for oxidative stress tolerance, virulence, and sclerotial development. Front. Microbiol. 10:233. doi: 10.3389/fmicb.2019.00233
Zhang, R., Xu, Q., Zhang, Y., and Zhu, F. (2018). Baseline sensitivity and toxic actions of prochloraz to Sclerotinia sclerotiorum. Plant Dis. 102, 2149–2157. doi: 10.1094/PDIS-01-18-0148-RE
Zhao, H., Zhou, T., Xie, J., Cheng, J., Jiang, D., and Fu, Y. (2020). Host transcriptional response of Sclerotinia sclerotiorum induced by the mycoparasite Coniothyrium minitans. Front. Microbiol. 11:183. doi: 10.3389/fmicb.2020.00183
Keywords: kojic acid, Sclerotinia sclerotiorum, antifungal activity, melanin biosynthesis, soybean pathogens
Citation: Zhu G-Y, Shi X-C, Wang S-Y, Wang B and Laborda P (2022) Antifungal Mechanism and Efficacy of Kojic Acid for the Control of Sclerotinia sclerotiorum in Soybean. Front. Plant Sci. 13:845698. doi: 10.3389/fpls.2022.845698
Edited by:
Prem Lal Kashyap, Indian Institute of Wheat and Barley Research (ICAR), IndiaReviewed by:
Elsherbiny A. Elsherbiny, Mansoura University, EgyptSanjay Goswami, Indian Institute of Sugarcane Research (ICAR), India
Marcelo Anibal Carmona, University of Buenos Aires, Argentina
Copyright © 2022 Zhu, Shi, Wang, Wang and Laborda. This is an open-access article distributed under the terms of the Creative Commons Attribution License (CC BY). The use, distribution or reproduction in other forums is permitted, provided the original author(s) and the copyright owner(s) are credited and that the original publication in this journal is cited, in accordance with accepted academic practice. No use, distribution or reproduction is permitted which does not comply with these terms.
*Correspondence: Pedro Laborda, cGVkcm9AbnR1LmVkdS5jbg==; Su-Yang Wang, d2FuZ3N1eWFuQG50dS5lZHUuY24=; Xin-Chi Shi, c2h4Y2gwMzAxQG50dS5lZHUuY24=