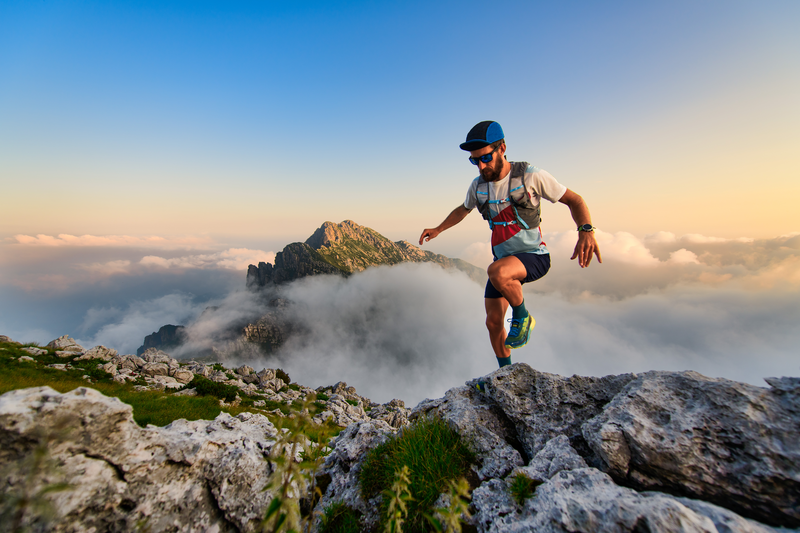
94% of researchers rate our articles as excellent or good
Learn more about the work of our research integrity team to safeguard the quality of each article we publish.
Find out more
ORIGINAL RESEARCH article
Front. Plant Sci. , 21 March 2022
Sec. Plant Proteomics and Protein Structural Biology
Volume 13 - 2022 | https://doi.org/10.3389/fpls.2022.845107
This article is part of the Research Topic Regulation of Proteolysis and Proteome Composition in Plant Response to Environmental Stress View all 11 articles
As one of the serious environmental problems worldwide, acid rain (AR) has always caused continuous damage to the forestry ecosystem. Studies have shown that AR can leach calcium ions from plants and soil. Calcium (Ca) is also a crucial regulator of the plant stress response, whereas there are few reports on how Ca regulates the response of AR-resistant woody plants to AR stress. In this study, by setting different exogenous Ca levels, we study the physiological and molecular mechanism of Ca in regulating the Taxus wallichiana var. mairei response to AR stress. Our results showed that low Ca level leads to photosynthesis, and antioxidant defense system decreases in T. wallichiana var. mairei leaves; however, these negative effects could be reversed at high Ca level. In addition, proteomic analyses identified 44 differentially expressed proteins in different Ca level treatments of T. wallichiana var. mairei under AR stress. These proteins were classified into seven groups, which include metabolic process, photosynthesis and energy pathway, cell rescue and defense, transcription and translation, protein modification and degradation, signal transduction, etc. Furthermore, the study found that low Ca level leads to an obvious increase of Ca-related gene expression under AR stress in T. wallichiana var. mairei using qRT-PCR analyses and however can be reversed at high Ca level. These findings would enrich and extend the Ca signaling pathways of AR stress in AR-resistant woody plants and are expected to have important theoretical and practical significance in revealing the mechanism of woody plants tolerating AR stress and protecting forestry ecosystem in soil environment under different Ca levels.
As a serious environmental problem, acid rain (AR) affects seriously normal growth and development of forest tree species, which includes balance of leaf nutrient, growth indices, chlorosis, and necrosis in leaves, chlorophyll content, restrict photosynthesis, and the antioxidant enzyme activity (Liu and Diamond, 2005; Larssen et al., 2006; Hu et al., 2014a,2016, 2021; Huang et al., 2019; Liu M. et al., 2019; Grennfelt et al., 2020; Zhang et al., 2020). Furthermore, AR can interfere with the material metabolism, transcriptional factors, and secondary metabolites genes, further cause metabolic disorders and inhibit the plant growth and development, and even cause plant death in severe cases (Lee et al., 2006; Hu et al., 2016; Debnath and Ahammed, 2020; Debnath et al., 2020, 2021). In addition, recent studies have found that AR severely affects woody plant vegetation growth through leaching away calcium (Ca) element from plant and soil (Likens et al., 1996; Malakoff, 2010; Liu et al., 2011c). Further studies have also revealed that AR can disturb mineral ion absorption, deplete soil base Ca ion from pools, and limit the Ca uptake in woody plant (Liu et al., 2011c; Hu et al., 2014c; Shu et al., 2019). Many studies have demonstrated that Ca plays important roles in plants responding to environmental stresses, and exogenous Ca level regulates cytosolic Ca concentration and enhances antioxidant defense, thus serving as a source of nutrition and structure, regulatory agent to further modulate signaling functions (Reddy and Reddy, 2004; Hepler, 2005; Juice et al., 2006; Zhang et al., 2021). On the other hand, our previous studies also indicated that exogenous Ca level played a key role in the response process of AR stress in AR-sensitive woody plants (Hu et al., 2014c,2016). Ma et al. (2021) also found that exogenous Ca enhances antioxidant defense to simulated AR stress in rice. However, the AR-resistant woody plants in response to AR stress at different Ca levels have not been reported in forest tree species.
Taxus wallichiana var. mairei is a well-known gymnosperm with great ornamental and medicinal value (Gao et al., 2007; Cheng et al., 2021; Xiong et al., 2021). As an AR-resistant tree species, T. wallichiana var. mairei is distributed over large areas of southern China, where AR is relatively serious (Gao et al., 2007; Hu et al., 2014a). Some physiological and biochemical changes and growth response to simulated AR have been reported in T. wallichiana var. mairei (Liu K. et al., 2007; Liu et al., 2012). Our previous study also found that T. wallichiana var. mairei is an AR-tolerant species under normal Ca condition (Hu et al., 2014a). However, the molecular mechanisms of AR resistance remain poorly understood in T. wallichiana var. mairei under different Ca conditions.
As responses of different Ca levels to AR stress are very complex processes, and further investigations are needed to clarify the molecular mechanism in AR-resistant species. Although our recent study revealed that there are rescue effects of exogenous Ca against AR stress in AR-sensitive species (Hu et al., 2014c,2016), the underlying mechanisms remain unclear in AR-tolerant species at various Ca levels. To better understand the extent to which biological and environmental factors shape AR resistance of T. wallichiana var. mairei at different Ca levels, proteomics and Ca-related gene expression in T. wallichiana var. mairei response to AR stress at different Ca levels is one of powerful ways to identify the molecular mechanism. A recent study has presented a reference genome of T. wallichiana var. mairei, which will provide genetic resources and serve as a platform for identification and decoding of the AR resistance pathway in various Ca conditions in the future (Cheng et al., 2021; Xiong et al., 2021). In this study, we conduct proteomic study to clarify the molecular mechanisms of various Ca levels in T. wallichiana var. mairei under AR treatment. The objective of this study was to characterize the AR-responsive proteins in T. wallichiana var. mairei at different Ca levels, combined with the physiological and gene expression data, and further to establish the molecular metabolism in Ca-mediated AR resistance in AR-resistant woody plants.
In our experiment, the substrate soil was lateritic soil, and the soil samples were collected from in southern forest areas of China where AR is much too harmful. Ca content in the soil was leached for 6 months of simulated AR, and the specific soil leaching process is referred to Liu J. X. et al. (2007) and Liu et al. (2011c). According to Hu et al. (2014c), Ca content was analyzed using ICP-MS (PerkinElmer Inc., Elan DRC-e, Waltham, MA, United States). Soil nutrients were recovered by a Hoagland nutrient solution, which contains one of the three Ca concentrations (20.0, 2.0, or 0.1 mmol L–1), respectively (Liu et al., 2011c). The final soil exchangeable Ca level was high Ca level (107.08 mmol kg–1), medium Ca level (19.65 mmol kg–1), and low Ca level (1.85 mmol kg–1).
The 6-month-old and size-identical T. wallichiana var. mairei seedlings (the aerial part length was 6.3 ± 0.5 cm) were transplanted into plastic pots. The seedlings were grown in a greenhouse with a light–dark regime of 16/8 h, temperature of 27/21°C (day/night), relative humidity of 60–70%, and photosynthetically active radiation of 210 μmol m–2 s–1. After 2 weeks of recovery, the T. wallichiana var. mairei seedlings were sprayed once each day with simulated AR solution (pH 3.0) with medium Ca level as control group and low or high Ca level for treatment group as described by the previous study (Hu et al., 2014c). After 2-month AR treatment, the fresh leaves of T. wallichiana var. mairei seedlings were collected for further experiments, which include physiological and proteomic research and qRT-PCR analysis.
Chlorophyll in T. wallichiana var. mairei leaves was extracted using ice-cold 80% v/v acetone according to the study by Hu et al. (2014c). Net photosynthetic rate (Pn) was performed with a portable photosynthesis system (Li-6400, Li-Cor, Lincoln, NE, United States) as described by Chen et al. (2013). At least eight saplings were randomly selected from the control group or the treatment group for Pn measurements. For Ca element analysis, T. wallichiana var. mairei leaf tissue was dried at 80°C for 72 h. Ca concentration in the leaves was measured using ICP-MS (PerkinElmer Inc., Elan DRC-e, Waltham, MA, United States), following the study by Hu et al. (2014c).
Soluble protein, proline, H2O2, and O2•– content were measured as described by Chen et al. (2013). According to Liu et al. (2011a), lipid peroxidation in leaves was measured by estimating the content of malondialdehyde (MDA) using thiobarbituric acid (TBA) reaction. Superoxide dismutase (SOD) activity, ascorbic peroxidase (APX) activity, peroxidase (POD) activity, and catalase (CAT) activity were measured following the methods of Hu et al. (2014b).
Protein in T. wallichiana var. mairei leaves was extracted using the method of phenol extraction according to the study of Hu et al. (2014a). Three independent biological repetitions were performed for each treatment. According to the manufacturer’s instructions of GE Healthcare Amersham Bioscience, protein concentration of the lysates was measured using a 2-D Quant Kit.
Two-dimensional electrophoresis (2-DE) was conducted according to the study of Hu et al. (2014a). Ettan IPGphor isoelectric focusing system (GE Healthcare Amersham Bioscience, Little Chalfont, United Kingdom) was used for isoelectric focusing (IEF). After IEF, gel strips were equilibrated as described by Hu et al. (2014c). For the second-dimension electrophoresis, it was conducted using a protein apparatus (Bio-Rad), the proteins were separated on 12.5% SDS polyacrylamide gels according to the manufacturer’s instructions (Hu et al., 2014c). The 2-DE gels were stained using Coomassie Brilliant Blue R-250, and an image scanner (Uniscan M3600, China) was used for gel images at 600 dots per inch resolution. The 2-D gel images were analyzed using PDQuest software (Version 8.01, Bio-Rad, Hercules, CA, United States). The intensity of protein spots changed more than twofold and passed the Student’s t-test (p < 0.05), which were considered for mass spectrometry analysis.
Protein digestion and protein identification in T. wallichiana var. mairei was performed according to the study of Hu et al. (2014a). The identified tryptic peptide masses in T. wallichiana var. mairei were searched against the National Center for Biotechnology Information non-redundant (NCBInr) database, and the taxonomy of green plants was selected using the MASCOT interface (Version 2.5; Matrix Science, London, United Kingdom). The following parameters were used for database search: no molecular weight restriction, permitting one missed cleavage, fixed modification of cysteine by carbamidomethylation, oxidation (Met) as a variable modification, the peptide tolerance of 100 ppm, and fragment ion mass tolerance of ±0.3 Da. At least three peptides were matched for protein identification, and the protein scores of MOWSE threshold were set greater than 73 with the NCBInr database (p < 0.05). As described by Hu et al. (2014a), the functions and subcellular localization of identified proteins were searched for the NCBI protein database,1 UniProt,2 and published literature.
Hierarchical clustering was performed on density value of differentially expressed proteins according to the study of Hu et al. (2014c). Input value was calculated by dividing volume percentage of each protein spot at the high Ca-AR level and low Ca-AR level by the corresponding protein spot at the medium Ca-AR level. Complete linkage algorithm was enabled, and the results of hierarchical cluster were plotted using TreeView software version 1.1.3.
Total RNA from T. wallichiana var. mairei leaves (0.1 g) was extracted using RNA purification reagent (Invitrogen Inc., CA, United States) in liquid nitrogen according to the study of Hu et al. (2014c). The M-MLV reverse transcriptase (TaKaRa, Dalian, China) was used for the synthesis of the first-strand cDNAs as described by the previous study (Hu et al., 2014c). Gene primers were designed for cloning the fragments of Ca-related genes in T. wallichiana var. mairei (Supplementary Table 1). The Ca-related gene abundance was analyzed using the Rotor-gene-6000 real-time PCR system (Corbett Research, Mortlake, NSW, Australia) as described by Hu et al. (2014c) with minor modifications. The following temperature program was used for qRT-PCR analysis: 94°C for 10 min, followed 94°C for 30 s by 40 cycles, primer annealing at 52–56°C for 30 s (Supplementary Table 2), and extension at 72°C for 20 s. The glyceraldehyde-3-phosphate dehydrogenase gene (GAPDH) in T. wallichiana var. mairei was used as the internal control for each sample. Three independent biological replicates were performed for each sample.
All data were presented as the mean ± SE of three replicated samples. The statistical significance was analyzed using a univariate analysis of variance (one-way ANOVA; SPSS, version 22.0, Inc., Chicago, IL, United States). Statistical significance was considered at p < 0.05.
To study the responses of woody plant to AR stress at different Ca levels, T. wallichiana var. mairei was treated with simulated AR (pH 3.0) for 2 months. Physiological changes are shown in Figure 1, and Ca content of the leaf, chlorophyll content, and photosynthetic activity (Pn) were also tested and analyzed. After 2-month AR treatment, Ca content and Pn were significantly decreased in T. wallichiana var. mairei leaves at low Ca level; however, high Ca treatment can reverse the decline of the physiological indicators.
Figure 1. Effects of different Ca treatments on morphological and physiological parameters of T. wallichiana var. mairei leaves under AR stress. (A) Phenotype on plant leaves. (B) Leaf Ca content. (C) Chlorophyll content. (D) Net photosynthetic rate (Pn). Different letters above columns indicate significant difference at p < 0.05.
As shown in Figures 2A,B, there was an obvious increase in soluble protein content and proline content in AR-treated T. wallichiana var. mairei at high Ca level. We found that the levels of MDA, H2O2, and O2•– were significantly stimulated by low Ca treatment (Figures 2C–E). It found that SOD, APX, POD, and CAT showed a significant increase in T. wallichiana var. mairei leaves at high Ca level (Figures 2F–I).
Figure 2. Effects of different Ca treatments on lipid peroxidation, ROS production, and antioxidant enzyme activity under simulated AR stress in T. wallichiana var. mairei leaves. The soluble protein content (A), proline content (B), MDA content (C), H2O2 content (D), O2•– content (E), SOD activity (F), APX activity (G), POD activity (H), and CAT activity (I). Columns labeled by different letters indicate significant differences at p < 0.05.
To further explore the proteome changes in Pinus massoniana and T. wallichiana var. mairei leaves under AR treatment, 2-DE was performed in this study.
A total of 44 protein spot in T. wallichiana var. mairei leaves response to AR stress with different Ca treatments (Figure 3A and Table 1). Close-up views of representative different protein spots are shown in Figure 3B. To analyze which biological processes the identified differential proteins are involved in, the identified 44 proteins were analyzed in appropriate functional pathways (Figure 4). The differentially expressed proteins were divided into seven groups based on their biological functions. The largest protein group was sorted to photosynthesis and energy pathway (27.2%), followed by transcription and translation (20.5%), cell rescue and defense (18.2%), signal transduction (11.4%), metabolic process (9.1%), and protein modification and degradation (4.5%) (Figure 4A). As shown in Figure 4B, the subcellular localization analysis found that 44 differentially expressed proteins were located in the cytoplasm (25.0%), chloroplast (22.7%), mitochondrion (11.4%), membrane (11.4%), nucleus (9.0%), extracellular (4.5%), endoplasmic reticulum (2.3%), and vacuole (2.3%).
Figure 3. Two-dimensional electrophoresis analysis of proteins extracted from the leaves of T. wallichiana var. mairei treated at different Ca levels under AR stress. The numbers are assigned to the protein spots correspond to those listed in Table 1. (A) Representative CBB R250-stained 2D gel of total proteins. Arrows indicate 44 spots showing at least twofold changes (p < 0.05) analyzed by MALDI-TOF/TOF MS. (B) The enlarged window represents some differentially expressed protein spots after AR treatment at different Ca levels.
Table 1. Differentially expressed proteins of Taxus wallichiana var. mairei in response to AR stress under different calcium levels.
Figure 4. (A) Functional category distribution of the identified proteins in AR-treated T. wallichiana var. mairei at low, medium, and high Ca levels. (B) Protein subcellular locations of all 44 identified and quantified proteins in AR-treated T. wallichiana var. mairei at low, medium, and high Ca levels.
In addition, we used a hierarchical cluster analysis to find the regular pattern of different protein expression changes during AR stress in T. wallichiana var. mairei leaves with different Ca treatments. As shown in Figure 5, the differentially expressed proteins are mainly distributed into two branches in T. wallichiana var. mairei. We found that the expression abundance of cell defense-related proteins (spots 21, 22, and 24) showed a downward trend with the increase of Ca concentration under AR stress. Signal transduction-related proteins (spots 3, 37, and 39) showed a significant upregulation trend under low Ca-AR treatment. The expression trend of these proteins was reversed to downregulated with the increase in Ca concentration. More interestingly, we found that a protein (spot 17, light-harvesting complex II protein Lhcb1) related to photosynthesis process was significantly downregulated in low Ca condition, but turned to upregulated in high Ca condition, which indicates that high Ca treatment helps to improve T. wallichiana var. mairei leaves photosynthesis, which is one of the ways to enhance plant resistance to AR stress. The specific information of the differential proteins is listed in Table 1.
Figure 5. Hierarchical clustering analyses of the 44 AR-responsive proteins expression profiles at different Ca levels in T. wallichiana var. mairei.
The proteomics results revealed that the abundance of ribulose-1, 5-bisphosphate carboxylase–oxygenase large subunit (spot 16), and ATP synthase (spots 7, 8, and 10) was increased in high Ca-AR-treated T. wallichiana var. mairei (Table 1). As shown in Figure 6, protein abundance levels of rubulose-1, 5-bisphoshate carboxylase–oxygenase large subunit (Rubisco LSU), and ATP synthase (ATPase) were significantly increased with high Ca treatment.
Figure 6. Effects of different Ca levels on rubulose-1, 5-bisphoshate carboxylase–oxygenase large subunit (Rubisco LSU) (A), ATP synthase (ATPase) (B) in T. wallichiana var. mairei under AR stress using western blot. Relative expression levels of Rubisco LSU (C) and ATPase (D) were analyzed with the Quantity One software. β-Actin was used as the internal control. Bars with different letters are significantly different from each other (p < 0.05).
To evaluate the expression abundances of the Ca-related genes at different Ca levels in AR-treated T. wallichiana var. mairei, we further analyze calmodulin gene (CaM1), touch 3 gene (TCH3), calreticulin 3 gene (CRT3), CDPK-related kinase gene (CDPK1), glutamate dehydrogenase 2 gene (GDH2), calcineurin B-like calcium sensor protein 1 gene (CBL1), calnexin 1 gene (CNX1), and respiratory burst oxidase homolog A gene (RbohA). As shown in Figure 7, under AR stress, the expression abundance of most Ca-related genes increased obviously at low Ca level; however, this expression trend can be reversed at high Ca level.
Figure 7. Expression analysis by qRT-PCR of eight Ca-related genes in T. wallichiana var. mairei treated at different Ca levels under AR stress. (A) Calmodulin 1 (CaM1), (B) touch 3 gene (TCH3), (C) calreticulin 3 gene (CRT3), (D) calcium-dependent protein kinase 1 (CDPK1), (E) glutamate dehydrogenase 2 gene (GDH2), (F) calcineurin B-like calcium sensor protein 1 gene (CBL1), (G) calnexin 1 gene (CNX1), (H) respiratory burst oxidase homolog A gene (RbohA). Bars represent the mean value ± SE (n = 3). Bars with different letters are significantly different from each other (p < 0.05).
The environmental stresses severely affect the material metabolism of plants (Liu et al., 2011a; Chen et al., 2014). UDP-glucose pyrophosphorylase is the precursor of carbohydrate formation (Chen et al., 2019). Our results showed that Ca application reduced AR-induced increases in protein abundance of UDP-glucose pyrophosphorylase (spot 2) in T. wallichiana var. mairei (Table 1). Under AR stress, our previous study indicates that high Ca level enhances the carbohydrate metabolism in Liquidambar formosana (Hu et al., 2016). It has been showed that woody plants against environmental stresses with a complex manner, which results in similar protein expressions (Hu et al., 2014c; Liu Y. et al., 2019). In this study, our finding is consistent with the results from the study of how exogenous Ca affects other woody plants to AR stress. These results showed that Ca application helps in alleviating the adverse effects of AR stress by accelerating the carbohydrate metabolism. On the other hand, Chen et al. (2019) found that UDP-glucose pyrophosphorylase plays an important role in cell wall metabolism and the synthesis of cellulase and hemicellulose. Carbohydrates act as a cytoskeleton and compose the cell wall as the first physical barrier defending against all types of AR stress (Liu et al., 2011b). We speculate that the protecting role of Ca can be explained by the upregulated carbohydrate metabolism-related protein under AR stress. S-adenosylmethionine synthase catalyzes the formation of S-adenosylmethionine from methionine and ATP, which is the critical enzymes in ethylene biosynthetic process (Peleman et al., 1989; Wang et al., 2002). In this study, protein abundances of S-adenosylmethionine synthase (spot 4) increased after low Ca treatment (Figure 5), which indicates that Ca-mediated ethylene biosynthetic process might make effect in AR tolerance in T. wallichiana var. mairei. Additionally, the abundance of S-adenosylmethionine synthase increases in high Ca-AR-treated L. formosana (Hu et al., 2016). These findings indicate that exogenous Ca plays different roles in AR-resistant tree species (T. wallichiana var. mairei) and AR-sensitive tree species (L. formosana and P. massoniana) in response to AR stress. Further study is needed to clarify the different metabolic processes at various Ca levels in AR-tolerance woody plants.
Aspartyl aminopeptidase is likely to play an important role in intracellular protein proteolysis and peptide metabolism (Park et al., 2017). In addition, F-box/kelch-repeat protein is involved in the pathway of protein ubiquitination, which is a part of protein modification. F-box/kelch-repeat protein may mediate the ubiquitination and subsequent proteasomal degradation of target proteins (González-Carranza et al., 2007). In this study, our proteomic analysis has detected increased abundance of probable aspartyl aminopeptidase (spot 6) and F-box/kelch-repeat protein (spot 36) at low Ca level in T. wallichiana var. mairei response to AR stress; however, this expression trend can be reversed at high Ca level (Table 1). It should be noted that the previous proteome analysis reveals the significantly elevated abundance of F-box family protein in AR-treated woody plant, which suggests that AR stress affects the biosynthesis and refolding of proteins and exacerbated protein degradation (Chen et al., 2014; Hu et al., 2014a). The findings provide informative clues regarding the protein modification and degradation mechanisms for the negative regulation of exogenous Ca level during T. wallichiana var. mairei response to AR stress. It is reasonable to speculate that AR stress leads to more serious protein degradation in low Ca condition, and high expression of degraded proteins system needs to be activated to maintain the stability of the protein metabolism in T. wallichiana var. mairei; high Ca condition has effective protection mechanism, low expression, or without degradation of protein fragments damaged by AR stress. AR stress can damage the homeostasis of protein metabolism between biosynthesis and degradation (Hu et al., 2014a). Most of these proteins play important roles in linking change of Ca concentration and the subsequent metabolic adaptation to AR stress in woody plants (Hu et al., 2014c,2016), but the specific details of the mechanism need to be further studied in the future.
Photosynthesis is a key metabolic process of woody plants, which is sensitive to AR stress (Liu et al., 2011a; Chen et al., 2013). In addition, our previous studies found that photosynthesis is greatly affected by simulated AR stress under low Ca level (Hu et al., 2014c,2016). In this study, physiological analysis found that the photosynthesis is inhibited with low Ca-AR treatment, but significantly increased with high Ca-AR treatment in T. wallichiana var. mairei leaves (Figure 1C). Consistent with the results of physiological data, our proteomic data analysis showed that high Ca level can remarkably improve photosynthetic capacity of T. wallichiana var. mairei with AR treatment. For instance, we found that chlorophyll a/b-binding protein (spot 15), ribulose-1,5-bisphosphate carboxylase–oxygenase large subunit (spot 16), light-harvesting complex II protein Lhcb1 (spot 17), and ferredoxin-NADP reductase (spot 18) were decreased in protein expression abundance with low Ca-AR treatment and increased with high Ca-AR treatment in T. wallichiana var. mairei (Table 1). These results indicated that high Ca level could restore the impaired photosynthetic function in AR-treated T. wallichiana var. mairei seedlings.
The protective role of exogenous Ca may lie in the increased upregulation of photosynthesis and energy pathway, which enhances AR tolerance in T. wallichiana var. mairei. This inference is supported by our previous studies, in which we demonstrated that exogenous Ca supply improved photosynthesis in woody plants against AR stress (Hu et al., 2014c,2016).
On the other hand, sufficient ATP is necessary for T. wallichiana var. mairei responding to AR stress in plants. ATP synthase and ATPase are the key enzymes in energy production and conversion (Liu et al., 2011b; Hu et al., 2014a). Previous evidence indicates that enough ATP is necessary for plants in response to environmental stress (Jiang et al., 2007). Our results found that a large number of enzymes were involved in this process that include ATP synthase beta subunit (spots 7, 8), F1-ATP synthase beta subunit (spot 9), and ATP synthase beta chain (spot 10). Our previous studies found that AR-sensitive tree species (L. formosana and P. massoniana) have the increased abundance of energy production-related proteins with high Ca-AR treatment (Hu et al., 2014c,2016). In this study, we also find a similar pattern in T. wallichiana var. mairei. Additionally, western blot analysis showed that relative abundance levels of the Rubisco LSU and ATPase were increased with high Ca treatment (Figure 6), and western blot result is consistent with the selected protein of our proteomic data at various Ca levels in AR-treated T. wallichiana var. mairei. Besides, as a membrane-bound protein located in thylakoids, ATP-dependent zinc metalloprotease-related proteins involve in the removal of a damaged D1 protein from PSII in plants (Hu et al., 2014a). Expression abundance of ATP-dependent zinc metalloprotease ThFtsH8 (spot 13) and ATP-dependent zinc metalloprotease FTSH (spot 14) was downregulated in high Ca-AR-treated T. wallichiana var. mairei. This change meant that less damaged protein is produced in high Ca condition, by which we furthermore find that the recovered photosynthesis decline caused by AR stress. Moreover, H+-ATPase plays an important role in the maintenance of ion homeostasis and nutrient uptake in plants (Liu et al., 2011b; Chen et al., 2019). Our study also showed that plasma membrane H+-ATPase (spot 11) was upregulated by high Ca level with AR treatment. Based on these results, high Ca condition may stimulate the resistance of T. wallichiana var. mairei to AR stress by modulating special energy-related pathway. These findings indicate that with high Ca-AR treatment, the mechanisms of energy pathway in AR-resistant tree species (T. wallichiana var. mairei) are different from AR-sensitive tree species (L. formosana and P. massoniana). Further investigations are needed to clarify the different energy pathways in AR-tolerance woody plants.
Acid rain stress has negative effects on plant growth and development, and also the internal functional components. Low Ca-AR treatment increased the content of MDA and reactive oxygen radicals [reactive oxygen species (ROS), e.g., H2O2 and O2•–], which suggests the ROS enrichment in T. wallichiana var. mairei; however, high Ca supplementation can reverse these effects (Figure 2). In addition, we observed that soluble protein content, proline content, SOD, APX, POD, and CAT activity showed a significant increase in T. wallichiana var. mairei leaves with high Ca treatment, which implies that antioxidant defense system was provoked by high Ca supplementation in AR-treated T. wallichiana var. mairei. These changes of physiological and biochemical findings indicated the protective role in exogenous Ca being against AR stress in T. wallichiana var. mairei.
To further reveal the different strategies to cope with AR stress at various Ca levels, many cell rescue and defense-related proteins were also identified by proteomic analysis in this study. As stress response proteins and heat shock proteins can be induced by many abiotic stresses (Wang et al., 2004, 2013), our study showed that exogenous Ca addition enhanced the abundance of heat shock protein (spot 19) and heat shock protein 70 (spot 20). Additionally, heat shock proteins are also considered as an important part in stabilizing protein folding and play important roles in the cellular stress response (Chen et al., 2019). The increased abundance of the heat shock-related proteins in high Ca-AR treatment set indicated the multidimensional role of external Ca supply in the alleviation of AR stress in T. wallichiana var. mairei.
In this study, decreased expressions of thioredoxin-related protein (spot 21) and glutathione S-transferase (spot 24) were also observed at high Ca levels. It was reported that thioredoxin can reduce intramolecular disulfide bridges of target proteins, maintain suppression of apoptosis, and supply reducing equivalents to the antioxidant systems (Tada et al., 2008; Liu et al., 2011b). Under both low and high Ca levels, elevated abundance of thioredoxin superfamily protein was described in L. formosana seedlings in response to AR stress (Hu et al., 2016). In addition, glutathione S-transferases are associated with stress tolerance, which can catalyze the addition of glutathione to electrophilic compounds and resist various cellular damages (Frova, 2003; Aloui et al., 2009). Previous studies reported the enhanced expressions of glutathione S-transferase in Arabidopsis response to AR stress and low Ca-AR-treated P. massoniana seedlings (Hu et al., 2014c,2016). As an antioxidative protein, glutathione S-transferase can be strongly induced by biotic and abiotic stresses (Dixon et al., 2002). In this study, downregulation of these proteins indicates that high Ca level has the potential to protect T. wallichiana var. mairei from oxidative stress caused by AR stress, which suggests that AR-resistant tree species has developed different defense strategies against AR stress under high Ca condition.
Transcription and translation regulate the expression level of stress responsive genes, which are important for plants response to environmental stresses (Jiang et al., 2007; Chen et al., 2014; Hu et al., 2014c). In this study, we have successfully identified nine proteins related to transcription and translation. In plants, maturase K catalyzes intron RNA binding and affects gene expression at the transcriptional level (Ji et al., 2009). The expression level of maturase K is complex in plants response to abiotic stress, which depends on plant species and the type of environmental stresses (Pandey et al., 2008; Chen et al., 2014). In this study, the abundance of maturase K (spot 27) increases in both low Ca-AR and high Ca-AR-treated T. wallichiana var. mairei, which suggests that expression changes in maturase K may play a part in linking change of Ca level under AR stress. Moreover, Chen et al. (2019) showed that a transcription factor displayed an elevated expression pattern in Arabidopsis of exogenous Ca-alleviated Al toxicity. We also found that exogenous high Ca enhances the abundance of transcription factor JUNGBRUNNEN 1 (spot 32) in T. wallichiana var. mairei. In addition, Hu et al. (2016) found that an increase in ribosomal protein S1 was detected in high Ca-AR-treated woody plant. Consistent with previous research, protein synthesis machinery was also affected by high Ca treatment as increased expression level of ribosomal protein L14 (spot 29) and small subunit ribosomal protein S1 (spot 35) has been detected in T. wallichiana var. mairei under AR stress. Transcription and translation control the expression of stress responsive genes, which play crucial roles in plants in response to various abiotic stresses (Amme et al., 2006). We detected increased abundance of series transcription and translation-related proteins in T. wallichiana var. mairei under high Ca-AR treatment, and the results indicated that Ca-regulated transcription and translation mediate T. wallichiana var. mairei adaptation to AR stress. The complex AR-responsive signaling pathways are associated with stress sensing and activation of defense pathways, which involves in calcium-regulated proteins crosstalk among various transcription factors. Under AR stress, future work on these transcription and translation-related proteins are needed to clarify the specific functions in various Ca conditions.
Under environmental stresses, woody plants initiate multiple signaling pathways through sensing and transducting several stress signals, further regulate the expression level of many functional proteins, and finally exhibit corresponding physiological response to against the abiotic stresses (Hu et al., 2014a; Liu Y. et al., 2019). Membrane steroid-binding protein 1 (MSBP1) can bind steroids and negatively regulates cell elongation and expansion, and also photomorphogenesis and brassinosteroid signaling (Yang et al., 2005; Song et al., 2009; Shi et al., 2011). In this study, we found that the abundance of membrane steroid-binding protein 1 (spot 37) was significantly increased at low Ca level; however, it can reverse the increased abundance of MSBP1 in T. wallichiana var. mairei at high Ca level, which provides informative hints on interactions between Ca and membrane steroid-binding protein. This finding suggested the specific relationship between Ca and steroid-binding protein in the BR signaling pathway. Protein phosphatase 2A plays an important role in plant development and growth through a functional link with the target of rapamycin (TOR) signaling pathway (Ahn et al., 2015). The TOR signaling pathway integrates multiple signals transduction, such as energy, nutrients, growth factors, and environmental conditions, to regulate plant cell metabolism and growth (Wullschleger et al., 2006; Xiong et al., 2013). We found that the expression level of 2A phosphatase-associated protein of 46 kDa (spot 38) was significantly upregulated in T. wallichiana var. mairei with high Ca-AR treatment. On the other hand, it should be noted that the abundance of 2A phosphatase-associated protein of 46 kDa was decreased in P. massoniana under high Ca-AR treatment (Hu et al., 2014c), which suggests that 2A phosphatase-associated protein of 46 kDa could play a specific role in woody plants response to AR stress. These results implied the different expression patterns and coping response strategies between AR-sensitive (P. massoniana) and AR-resistant (T. wallichiana var. mairei) tree species under high Ca condition; however, further studies are needed to elucidate the specific function of the signal transduction-related proteins in AR-stressed tree species.
Moreover, Ca plays an important role in plants coping with a series of environmental stresses (Reddy et al., 2011). In the cytoplasm, free cytosolic Ca2+ level is a universal second messenger of signal transduction in plants in response to environmental stresses (Kudla et al., 2018). Ca-binding-related proteins regulated modulation of intracellular Ca2+ levels (Hu et al., 2014a,2016). In this study, expression level of calcium-binding protein KIC-like (spot 40) showed declines in T. wallichiana var. mairei with high Ca-AR treatment. It has been observed that Ca-binding proteins are regarded as crosstalk key nodes for environmental stress signaling pathways (Hepler, 2005; Liu Y. et al., 2019). These findings suggest that calcium-binding proteins participate in adaptation responses to AR stress in T. wallichiana var. mairei, which provides informative hints on interactions between Ca-associated signal transduction and AR tolerance. In Figure 7, our result provided additional evidence that the expression abundance of eight Ca-related genes (CaM1, TCH3, CRT3, CDPK1, GDH2, CBL1, CNX1, and RbohA) increased obviously at low Ca level, whereas this expression trend can be reversed at high Ca level under AR stress. Plants respond to environmental stresses through altering gene expression and adaptive responses associated with stress sensing and activation of defense pathways, such as expression of stress adaptive proteins, synthesis of oxidative stress protectors, calcium-regulated proteins, and signal pathway (Hasegawa et al., 2000; Hu et al., 2014a). In this study, these findings indicate that Ca-dependent signal transduction could induce upregulated expression of Ca-related genes and further interact with various biological processes to against AR stress in T. wallichiana var. mairei.
In this study, physiological and proteomic evidence is carried out to clarify that Ca plays an important role in AR-resistant tree species T. wallichiana var. mairei response to simulated AR treatment. Our data reveal that low Ca treatment activated the cell rescue- and defense-related proteins and Ca-related gene level to respond to AR stress in T. wallichiana var. mairei. These proteins might operate in a dynamic network in the response of AR-resistant tree species under AR stress, and these identified proteins might be useful in revealing insights into the different defense mechanisms of AR-resistant tree species to AR stress. In addition, exogenous Ca supply against AR stress by increasing the abundance of the proteins involved in photosynthesis and energy pathway, translational factors, which suggest that AR-tolerant woody plants can equip themselves better to respond to AR stress by provoking related proteins and gene expression. As shown in Figure 8, we proposed a scheme associated with different defense mechanisms of T. wallichiana var. mairei responding to AR damage at different Ca levels. These results would deepen insights into the adaptation strategies of AR-resistant tree species under AR stress at different Ca levels and furthermore provide theoretical support for the scientific prevention of AR stress.
Figure 8. An adaptive strategy of T. wallichiana var. mairei leaves response to AR stress under different Ca levels.
The original contributions presented for this study are included in the article/supplementary material. For details, please refer to Supplementary Table S3 and Supplementary Datasheet.
H-LZ, W-JH, and G-XS: conceptualization and funding acquisition. W-JH and QW: methodology. T-WL: software and visualization. C-KJ: validation. JW: formal analysis. QW and H-LL: investigation. LC: resources. C-QZ: data curation. W-JH and C-QZ: writing – original draft preparation. H-LZ and G-XS: writing, reviewing, and editing, and project administration. H-LZ: supervision. All authors have read and agreed to the published version of the manuscript.
This research was funded by the Key Research and Development Program of Zhejiang Province (No. 2021C02002), the Key Technologies R&D Program for Crop Breeding of Zhejiang Province (No. 2021C02072-6), the National Natural Science Foundation of China (Nos. 32001104, 31870581, 32171740, and 30930076), the Open Project of State Key Laboratory of Rice Biology of China (No. 20190402), and the Project of Science and Technology Program of Quzhou (No. 2021K34).
The authors declare that the research was conducted in the absence of any commercial or financial relationships that could be construed as a potential conflict of interest.
All claims expressed in this article are solely those of the authors and do not necessarily represent those of their affiliated organizations, or those of the publisher, the editors and the reviewers. Any product that may be evaluated in this article, or claim that may be made by its manufacturer, is not guaranteed or endorsed by the publisher.
The Supplementary Material for this article can be found online at: https://www.frontiersin.org/articles/10.3389/fpls.2022.845107/full#supplementary-material
Ahn, C. S., Ahn, H.-K., and Pai, H.-S. (2015). Overexpression of the PP2A regulatory subunit Tap46 leads to enhanced plant growth through stimulation of the TOR signalling pathway. J. Exp. Bot. 66, 827–840. doi: 10.1093/jxb/eru438
Aloui, A., Recorbet, G., Gollotte, A., Robert, F., Valot, B., Gianinazzi-Pearson, V., et al. (2009). On the mechanisms of cadmium stress alleviation in Medicago truncatula by arbuscular mycorrhizal symbiosis: a root proteomic study. Proteomics 9, 420–433. doi: 10.1002/pmic.200800336
Amme, S., Matros, A., Schlesier, B., and Mock, H. P. (2006). Proteome analysis of cold stress response in Arabidopsis thaliana using DIGE-technology. J. Exp. Bot. 57, 1537–1546. doi: 10.1093/jxb/erj129
Chen, J., Duan, R.-X., Hu, W.-J., Zhang, N.-N., Lin, X.-Y., Zhang, J.-H., et al. (2019). Unravelling calcium-alleviated aluminium toxicity in Arabidopsis thaliana: insights into regulatory mechanisms using proteomics. J. Proteomics 199, 15–30. doi: 10.1016/j.jprot.2019.02.013
Chen, J., Hu, W.-J., Wang, C., Liu, T.-W., Chalifour, A., Chen, J., et al. (2014). Proteomic analysis reveals differences in tolerance to acid rain in two broad-leaf tree species, Liquidambar formosana and Schima superba. PLoS One 9:e102532. doi: 10.1371/journal.pone.0102532
Chen, J., Wang, W.-H., Liu, T.-W., Wu, F.-H., and Zheng, H.-L. (2013). Photosynthetic and antioxidant responses of Liquidambar formosana and Schima superba seedlings to sulfuric-rich and nitric-rich simulated acid rain. Plant Physiol. Biochem. 64, 41–51. doi: 10.1016/j.plaphy.2012.12.012
Cheng, J., Wang, X., Liu, X., Zhu, X., Li, Z., Chu, H., et al. (2021). Chromosome-level genome of Himalayan yew provides insights into the origin and evolution of the paclitaxel biosynthetic pathway. Mol. Plant 14, 1199–1209. doi: 10.1016/j.molp.2021.04.015
Debnath, B., and Ahammed, G. J. (2020). “Effect of acid rain on plant growth and development: physiological and molecular interventions,” in Contaminants in Agriculture, eds M. Naeem, A. Ansari, and S. Gill (Berlin: Springer), 103–114.
Debnath, B., Li, M., Liu, S., Pan, T., Ma, C., and Qiu, D. (2020). Melatonin-mediate acid rain stress tolerance mechanism through alteration of transcriptional factors and secondary metabolites gene expression in tomato. Ecotox. Environ. Saf. 200:110720. doi: 10.1016/j.ecoenv.2020.110720
Debnath, B., Sikdar, A., Islam, S., Hasan, K., Li, M., and Qiu, D. (2021). Physiological and molecular responses to acid rain stress in plants and the impact of melatonin, glutathione and silicon in the amendment of plant acid rain stress. Molecules 26:862. doi: 10.3390/molecules26040862
Dixon, D. P., Lapthorn, A., and Edwards, R. (2002). Plant glutathione transferases. Genome Biol. 3:REVIEWS3004.
Frova, C. (2003). The plant glutathione transferase gene family: genomic structure, functions, expression and evolution. Physiol. Plant. 119, 469–479. doi: 10.1046/j.1399-3054.2003.00183.x
Gao, L., Möller, M., Zhang, X. M., Hollingsworth, M., Liu, J., Mill, R., et al. (2007). High variation and strong phylogeographic pattern among cpDNA haplotypes in Taxus wallichiana (Taxaceae) in China and North Vietnam. Mol. Ecol. 16, 4684–4698. doi: 10.1111/j.1365-294X.2007.03537.x
González-Carranza, Z. H., Rompa, U., Peters, J. L., Bhatt, A. M., Wagstaff, C., Stead, A. D., et al. (2007). HAWAIIAN skirt: an F-box gene that regulates organ fusion and growth in Arabidopsis. Plant Physiol. 144, 1370–1382. doi: 10.1104/pp.106.092288
Grennfelt, P., Engleryd, A., Forsius, M., Hov, O., Rodhe, H., and Cowling, E. (2020). Acid rain and air pollution: 50 years of progress in environmental science and policy. Ambio 49, 849–864. doi: 10.1007/s13280-019-01244-4
Hasegawa, P. M., Bressan, R. A., Zhu, J. K., and Bohnert, H. J. (2000). Plant cellular and molecular responses to high salinity. Annu. Rev. Plant Biol. 51, 463–499. doi: 10.1146/annurev.arplant.51.1.463
Hepler, P. K. (2005). Calcium: a central regulator of plant growth and development. Plant Cell 17, 2142–2155. doi: 10.1105/tpc.105.032508
Hu, H., Hua, W., Shen, A., Zhou, H., Sheng, L., Lou, W., et al. (2021). Photosynthetic rate and chlorophyll fluorescence of barley exposed to simulated acid rain. Environ. Sci. Pollut. R. 28, 42776–42786. doi: 10.1007/s11356-021-13807-8
Hu, W. J., Chen, J., Liu, T. W., Simon, M., Wang, W. H., Chen, J., et al. (2014a). Comparative proteomic analysis of differential responses of Pinus massoniana and Taxus wallichiana var. mairei to simulated acid rain. Int. J. Mol. Sci. 15, 4333–4355. doi: 10.3390/ijms15034333
Hu, W. J., Chen, J., Liu, T. W., Wu, Q., Wang, W. H., Liu, X., et al. (2014c). Proteome and calcium-related gene expression in Pinus massoniana leaves in response to acid rain under different calcium levels. Plant Soil 380, 285–303. doi: 10.1007/s11104-014-2086-9
Hu, W. J., Chen, J., Liu, T. W., Liu, X., Wu, F. H., Wang, W. H., et al. (2014b). Comparative proteomic analysis on wild type and nitric oxide-overproducing mutant (nox1) of Arabidopsis thaliana. Nitric Oxide 36, 19–30. doi: 10.1016/j.niox.2013.10.008
Hu, W. J., Wu, Q., Liu, X., Shen, Z., Chen, J., Liu, T., et al. (2016). Comparative proteomic analysis reveals the effects of exogenous calcium against acid rain stress in Liquidambar formosana Hance leaves. J. Proteome Res. 15, 216–228. doi: 10.1021/acs.jproteome.5b00771
Huang, J., Wang, H., Zhong, Y., Huang, J., Fu, X., Wang, L., et al. (2019). Growth and physiological response of an endangered tree, Horsfieldia hainanensis merr., to simulated sulfuric and nitric acid rain in southern China. Plant Physiol. Biochem. 144, 118–126. doi: 10.1016/j.plaphy.2019.09.029
Ji, X. L., Gai, Y. P., Zheng, C. C., and Mu, Z. M. (2009). Comparative proteomic analysis provides new insights into mulberry dwarf responses in mulberry (Morus alba L.). Proteomics 9, 5328–5339. doi: 10.1002/pmic.200900012
Jiang, Y., Yang, B., Harris, N. S., and Deyholos, M. K. (2007). Comparative proteomic analysis of NaCl stress-responsive proteins in Arabidopsis roots. J. Exp. Bot. 58, 3591–3607. doi: 10.1093/jxb/erm207
Juice, S. M., Fahey, T. J., Siccama, T. G., Driscoll, C. T., Denny, E. G., Eagar, C., et al. (2006). Response of sugar maple to calcium addition to northern hardwood forest. Ecology 87, 1267–1280. doi: 10.1890/0012-9658(2006)87[1267:rosmtc]2.0.co;2
Kudla, J., Becker, D., Grill, E., Hedrich, R., Hippler, M., Kummer, U., et al. (2018). Advances and current challenges in calcium signaling. New Phytol. 218, 414–431. doi: 10.1111/nph.14966
Larssen, T., Lydersen, E., Tang, D., He, Y., Gao, J., Liu, H., et al. (2006). Acid rain in China. Environ. Sci. Technol. 40, 418–425.
Lee, Y., Park, J., Im, K., Kim, K., Lee, J., Lee, K., et al. (2006). Arabidopsis leaf necrosis caused by simulated acid rain is related to the salicylic acid signaling pathway. Plant Physiol. Biochem. 44, 38–42. doi: 10.1016/j.plaphy.2006.01.003
Likens, G. E., Driscoll, C. T., and Buso, D. C. (1996). Long-term effects of acid rain: response and recovery of a forest ecosystem. Science 272, 244–245. doi: 10.1016/j.scitotenv.2021.149626
Liu, J. X., Zhou, G. Y., and Zhang, D. Q. (2007). Effects of acidic solutions on element dynamics in monsoon evergreen broad-leaved forest at Dinghushan, China. Environ. Sci. Pollut. R. 14, 123–129. doi: 10.1065/espr2006.07.325
Liu, J., and Diamond, J. (2005). China’s environment in a globalizing world. Nature 435, 1179–1186. doi: 10.1038/4351179a
Liu, J., Yang, C., and Wang, M. (2012). Effect of simulated acid rain on physiological characteristics in Taxus chinensis var. Mairei. Chinese J. Trop. Crop. 33, 1046–1050.
Liu, K., Yu, F., Peng, S., Fang, Y., and Li, F. (2007). Effects of simulated acid on saplings of Taxus species. Ecol. Env. 16, 309–312.
Liu, M., Huang, X., Song, Y., Tang, J., Cao, J., Zhang, X., et al. (2019). Ammonia emission control in China would mitigate haze pollution and nitrogen deposition, but worsen acid rain. Proc. Natl. Acad. Sci. U. S. A. 116, 7760–7765. doi: 10.1073/pnas.1814880116
Liu, T. W., Wu, F. H., Wang, W. H., Chen, J., Li, Z. J., Dong, X. J., et al. (2011c). Effects of calcium on seed germination, seedling growth and photosynthesis of six forest tree species under simulated acid rain. Tree Physiol. 31, 402–413. doi: 10.1093/treephys/tpr019
Liu, T. W., Fu, B., Niu, L., Chen, J., Wang, W.-H., He, J.-X., et al. (2011a). Comparative proteomic analysis of proteins in response to simulated acid rain in Arabidopsis. J. Proteome Res. 10, 2579–2589. doi: 10.1021/pr200056a
Liu, T. W., Jiang, X., Shi, W., Chen, J., Pei, Z., and Zheng, H. (2011b). Comparative proteomic analysis of differentially expressed proteins in β-aminobutyric acid enhanced Arabidopsis thaliana tolerance to simulated acid rain. Proteomics 11, 2079–2094. doi: 10.1002/pmic.201000307
Liu, Y., Ji, D., Turgeon, R., Chen, J., Lin, T., Huang, J., et al. (2019). Physiological and proteomic responses of Mulberry Trees (Morus alba. L.) to combined salt and drought stress. Int. J. Mol. Sci. 20:2486. doi: 10.3390/ijms20102486
Ma, Y., Ren, X., and Liang, C. (2021). Exogenous Ca2+ enhances antioxidant defense in rice to simulated acid rain by regulating ascorbate peroxidase and glutathione reductase. Planta 254, 1–16. doi: 10.1007/s00425-021-03679-0
Malakoff, D. (2010). Taking the sting out of acid rain. Science 330, 910–911. doi: 10.1126/science.330.6006.910
Pandey, A., Chakraborty, S., Datta, A., and Chakraborty, N. (2008). Proteomics approach to identify dehydration responsive nuclear proteins from chickpea (Cicer arietinum L.). Mol. Cell Proteomics 7, 88–107. doi: 10.1074/mcp.M700314-MCP200
Park, S. Y., Scranton, M. A., Stajich, J. E., Yee, A., and Walling, L. L. (2017). Chlorophyte aspartyl aminopeptidases: ancient origins, expanded families, new locations, and secondary functions. PLoS One 12:e0185492. doi: 10.1371/journal.pone.0185492
Peleman, J., Boerjan, W., Engler, G., Seurinck, J., Botterman, J., Alliotte, T., et al. (1989). Strong cellular preference in the expression of a housekeeping gene of Arabidopsis thaliana encoding S-adenosylmethionine synthetase. Plant Cell 1, 81–93. doi: 10.1105/tpc.1.1.81
Reddy, A. S., Ali, G. S., Celesnik, H., and Day, I. S. (2011). Coping with stresses: roles of calcium-and calcium/calmodulin-regulated gene expression. Plant Cell 23, 2010–2032. doi: 10.1105/tpc.111.084988
Reddy, V. S., and Reddy, A. S. (2004). Proteomics of calcium-signaling components in plants. Phytochemistry 65, 1745–1776. doi: 10.1016/j.phytochem.2004.04.033
Shi, Q. M., Yang, X., Song, L., and Xue, H. W. (2011). Arabidopsis MSBP1 is activated by HY5 and HYH and is involved in photomorphogenesis and brassinosteroid sensitivity regulation. Mol. Plant 4, 1092–1104. doi: 10.1093/mp/ssr049
Shu, X., Zhang, K., Zhang, Q., and Wang, W. (2019). Ecophysiological responses of Jatropha curcas L. seedlings to simulated acid rain under different soil types. Ecotox. Environ. Saf. 185:109705. doi: 10.1016/j.ecoenv.2019.109705
Song, L., Shi, Q.-M., Yang, X.-H., Xu, Z.-H., and Xue, H.-W. (2009). Membrane steroid-binding protein 1 (MSBP1) negatively regulates brassinosteroid signaling by enhancing the endocytosis of BAK1. Cell Res. 19, 864–876. doi: 10.1038/cr.2009.66
Tada, Y., Spoel, S. H., Pajerowska-Mukhtar, K., Mou, Z., Song, J., Wang, C., et al. (2008). Plant immunity requires conformational charges of NPR1 via S-nitrosylation and thioredoxins. Science 321, 952–956. doi: 10.1126/science.1156970
Wang, K. L., Li, H., and Ecker, J. R. (2002). Ethylene biosynthesis and signaling networks. Plant Cell 14, 131–151. doi: 10.1105/tpc.001768
Wang, W., Vinocur, B., Shoseyov, O., and Altman, A. (2004). Role of plant heat-shock proteins and molecular chaperones in the abiotic stress response. Trends Plant Sci. 9, 244–252.
Wang, X., Liu, Z., Niu, L., and Fu, B. (2013). Long-term effects of simulated acid rain stress on a staple forest plant, Pinus massoniana Lamb: a proteomic analysis. Trees 27, 297–309. doi: 10.1007/s00468-012-0799-z
Wullschleger, S., Loewith, R., and Hall, M. N. (2006). TOR signaling in growth and metabolism. Cell 124, 471–484.
Xiong, X., Gou, J., Liao, Q., Li, Y., Zhou, Q., Bi, G., et al. (2021). The Taxus genome provides insights into paclitaxel biosynthesis. Nat. Plants 7, 1026–1036. doi: 10.1038/s41477-021-00963-5
Xiong, Y., Mccormack, M., Li, L., Hall, Q., Xiang, C., and Sheen, J. (2013). Glucose–TOR signalling reprograms the transcriptome and activates meristems. Nature 496, 181–186. doi: 10.1038/nature12030
Yang, X. H., Xu, Z. H., and Xue, H.-W. (2005). Arabidopsis membrane steroid binding protein 1 is involved in inhibition of cell elongation. Plant Cell 17, 116–131. doi: 10.1105/tpc.104.028381
Zhang, C., Yi, X., Gao, X., Wang, M., Shao, C., Lv, Z., et al. (2020). Physiological and biochemical responses of tea seedlings (Camellia sinensis) to simulated acid rain conditions. Ecotox. Environ. Saf. 192:110315. doi: 10.1016/j.ecoenv.2020.110315
Keywords: plant proteomics, acid rain, calcium, Taxus wallichiana var. mairei, soil, tree species
Citation: Hu W-J, Liu T-W, Zhu C-Q, Wu Q, Chen L, Lu H-L, Jiang C-K, Wei J, Shen G-X and Zheng H-L (2022) Physiological, Proteomic Analysis, and Calcium-Related Gene Expression Reveal Taxus wallichiana var. mairei Adaptability to Acid Rain Stress Under Various Calcium Levels. Front. Plant Sci. 13:845107. doi: 10.3389/fpls.2022.845107
Received: 29 December 2021; Accepted: 07 February 2022;
Published: 21 March 2022.
Edited by:
Mateusz Labudda, Warsaw University of Life Sciences – SGGW, PolandReviewed by:
Juan Chen, Northwest A&F University, ChinaCopyright © 2022 Hu, Liu, Zhu, Wu, Chen, Lu, Jiang, Wei, Shen and Zheng. This is an open-access article distributed under the terms of the Creative Commons Attribution License (CC BY). The use, distribution or reproduction in other forums is permitted, provided the original author(s) and the copyright owner(s) are credited and that the original publication in this journal is cited, in accordance with accepted academic practice. No use, distribution or reproduction is permitted which does not comply with these terms.
*Correspondence: Wen-Jun Hu, Z3V5dWV4aW5nc2hpQDE2My5jb20=; Guo-Xin Shen, Z3VveGluLnNoZW5AdHR1LmVkdQ==; Hai-Lei Zheng, emhlbmdobEB4bXUuZWR1LmNu
Disclaimer: All claims expressed in this article are solely those of the authors and do not necessarily represent those of their affiliated organizations, or those of the publisher, the editors and the reviewers. Any product that may be evaluated in this article or claim that may be made by its manufacturer is not guaranteed or endorsed by the publisher.
Research integrity at Frontiers
Learn more about the work of our research integrity team to safeguard the quality of each article we publish.