- 1Technology Center, China Tobacco Hunan Industrial Co., Ltd., Changsha, China
- 2Key Laboratory for Tobacco Gene Resources, Tobacco Research Institute, Chinese Academy of Agricultural Sciences, Qingdao, China
- 3Hunan Key Laboratory of Plant Functional Genomics and Developmental Regulation, College of Biology, Hunan University, Changsha, China
- 4Hunan Tobacco Research Institute, Changsha, China
The Catharanthus roseus RLK1-like (CrRLK1L) family is involved in the regulation of plant reproduction, growth and development, cell wall integrity sensing, as well as responses to both biotic and abiotic stress conditions. Extraordinary progress has been made in elucidating the CrRLK1L family receptor kinases–mediated signaling pathway, while limited research addressed the functions of CrRLK1L proteins in tobacco. In this study, we identified and analyzed 48 NtCrRLK1L members from the tobacco genome. The newly identified NtCrRLK1L members were divided into seven groups together with the Arabidopsis CrRLK1L members. The syntenic analysis revealed that four pairs of NtCrRLK1L genes were predicted to have arisen from segmental duplication events. Expression profiling showed that the NtCrRLK1L genes were expressed in various tissues, and most NtCrRLK1L genes were induced by salt and drought stress conditions. Notably, NtCrRLK1L47 was upregulated under drought and salinity stresses, and the NtCrRLK1L47-GFP fusion protein was located in the cell membrane. Furthermore, overexpression of the NtCrRLK1L47 gene enhanced the salt tolerance in tobacco seedlings.
Introduction
In the plant kingdom, the individuals live in a complex environment and are constantly threatened by various stresses. Hence, perceiving external signals and responding accordingly are vital to development and survival. The receptor-like kinase (RLK) family is one of the largest families, which can sense extracellular signals and then activate intracellular signaling pathways (Morillo and Tax, 2006; Lindner et al., 2012; Dievart et al., 2020; Wang et al., 2020). Among them, CrRLK1L proteins are the subfamily of RLKs, and might probably be unique to plants (Han et al., 2011; Lindner et al., 2012). Since the first CrRLK1L protein was discovered from the Catharanthus roseus cell cultures (Schulze-Muth et al., 1996), the family members have been identified in many species (Niu et al., 2016; Kou et al., 2017; Wang et al., 2021). Generally, the CrRLK1L family members possess three typical domains, including the intercellular cytoplasmic kinase domain, transmembrane domain, and extracellular ligands-binding domain (Dievart et al., 2020). Previous studies have shown that the extracellular domain is highly similar to the glycoprotein in Xenopus, suggesting that the ligands of the CrRLK1L family member may be modified by glycosylation (Schallus et al., 2008; Boisson-Dernier et al., 2011; Takeda et al., 2014). The CrRLK1L family members exhibited diverse expression patterns by triggering different biological processes (Hématy and Höfte, 2008; Lindner et al., 2012). The rapid alkalinization factor (RALF) peptides were identified to serve as the ligands, which could be perceived by the CrRLK1L members (Lindner et al., 2012; Xiao et al., 2019; Zhang et al., 2020b).
The CrRLK1L family members were reported to be involved in a variety of processes, including plant growth and development (Haruta et al., 2014), hormone signaling (Guo et al., 2009), stress responses (Ye et al., 2017), reproduction (Nibau and Cheung, 2011), energy production (Li et al., 2016), and RNA metabolism (Wang et al., 2020).
In Arabidopsis, a total of 17 CrRLK1L members were identified, and most of them were studied in detail to reveal their functions (Feng et al., 2019; Duan et al., 2020). As the founding member, FERONIA (FER) was first isolated from the semi sterile, female gametophytic mutant (Huck et al., 2003). In addition, the FERONIA was widely expressed in all vegetative tissues and developmental stages, which indicates that its role is not limited to the regulation of fertilization (Stegmann et al., 2017). With the remarkable progress, the FER was reported to act as the receptor of RALF1, RALF17, RALF23, RALF32, and RALF33, and also regulate the plant development and biotic/abiotic stress responses (Yu et al., 2018; Ge et al., 2019a; Xiao et al., 2019). Notably, FER was found to inhibit the abscisic acid (ABA) signaling pathway through activation of ABA Insensitive 2 (ABI2) (Chen et al., 2016). Furthermore, FER could confer the JA signaling pathway by phosphorylating MYC2 and is involved in regulating plant immunity (Guo et al., 2018). Furthermore, the THESEUS1 (THE1) was reported to play a key role in cell wall synthesis, cell expansion, and cell wall integrity (Van Der Does et al., 2017; Gonneau et al., 2018). Besides, ANX1/2 and BUPS1/2 were shown to work as a heteromeric receptor complex to coordinate the maintenance of pollen tube integrity and sperm cell discharge (Franck et al., 2018; Feng et al., 2019; Ge et al., 2019b). In addition, MDS1, MDS2, MDS3, and MDS4 were reported to have redundant roles in the metal ion stress response (Richter et al., 2018). In other species, several CrRLK1L members are identified. OsFLR1 and OsFLR2 are necessary for maintaining architecture, fertility, and seed yield (Li et al., 2016; Pu et al., 2017). In apples, MdFERL1 and MdFERL6 were reported to influence fruit ripening (Jia et al., 2017). PbrCrRLK1L3 and PbrCrRLK1L26 could regulate the pollen tube rupture and growth in pears (Kou et al., 2017).
Tobacco is not only a considerable economic crop but also a well-studied model organism. However, the extreme environment and diseases have always been a potential threat to tobacco yield and quality. The CrRLK1L members have been identified in Arabidopsis (Shiu et al., 2004), rice (Shiu et al., 2004), soybeans (Wang et al., 2021), cotton (Niu et al., 2016), pear (Kou et al., 2017), and apple (Zuo et al., 2018). The study of CrRLK1L members in tobacco is found to be limited. Therefore, the identification of the tobacco CrRLK1L is of great significance for the research of tobacco development and in response to biotic/abiotic stresses. With access to the tobacco genome, a systematic analysis by integrating bioinformatics and molecular biology was performed in the current study. The clues provided in this study would present the foundation for a further study focusing on these tobacco CrRLK1L members.
Materials and Methods
Identification and Phylogenetic Analysis
The version 4.5 of the genome sequence annotations of tobacco (Nicotiana tabacum L.) was downloaded from the SGN database (https://solgenomics.net/). All of the reported Arabidopsis CrRLK1L full-length protein sequences (Zhang et al., 2020c) were obtained from The Arabidopsis Information Resource (TAIR, https://www.arabidopsis.org/), and these sequences were used as queries to perform a BLASTP search against the tobacco protein database (E-value < 0.01). Then, these resulting sequences were used as new queries to conduct a BLASTP search again, to avoid missing potential members. The remaining members were named by their physical locations on the chromosome/scaffold. The amino acid number, molecular weights, and isoelectric points were predicted by using the ProtParam online tool (Garg et al., 2016).
The multiple sequence alignment of the new NtCrRLK1Ls and reported AtCrRLK1Ls were performed by using MAFFT v5.3 under default settings (Katoh and Standley, 2013). Then, the neighbor-joining phylogenetic analysis was conducted by MAGE X based on the alignment of full-length protein sequences with the bootstrap method of 1,000 replicates, substitution with the Poisson model, and pairwise deletion (Kumar et al., 2018).
Motif and Gene Structure Analysis
The CrRLK1L motifs were identified by MEME online toolbox (http://meme-suite.org/) (Bailey et al., 2015). The GSDS tool (http://gsds.cbi.pku.edu.cn/) was adopted to visualize exon–intron organizations of the tobacco CrRLK1L genes by comparing their coding sequences (CDSs) and genomic sequences (Hu et al., 2015).
Chromosomal Localization Analysis
The chromosomal localization information of NtCrRLK1L genes was obtained from the SGN database and was illustrated by Perl. The segmental duplications of NtCrRLK1L genes were predicted by the MCScanX program and were visualized by Circos of TBtools (Chen et al., 2020). The synteny relationship of the orthologous genes from tobacco and other plant species (Arabidopsis, tomato, potato, grape, and rice) was identified by the Synteny Plotter of Tbtools (Chen et al., 2020). The non-synonymous (ka) and synonymous (ks) substitutions of each duplicated gene pair were calculated by DnaSP v5 (Rozas et al., 2017).
Promoter Analysis of Tobacco CrRLK1L Genes
The 2,000 bp region upstream of the NtCrRLK1L genes start site was selected to perform the promoter analysis as previously described (Cao et al., 2016). These promoter sequences were extracted from the genome sequence database. Then, the PlantCARE database was adopted for the cis-elements investigation (Lescot et al., 2002). The results were visualized by R.
Expression Profiling of NtCrRLK1Ls
The reported RNA-seq data of tobacco tissues (Edwards et al., 2017) were downloaded from the GEO database (accession number: GSE95717). Then, the expression data of NtCrRLK1L genes were extracted and transformed with log2 to normalize the raw data. The pHeatmap R package was adopted to illustrate Heatmap under the default parameters.
Tobacco Plants Preparation and Treatment
Cultivated tobacco K326 was used to analyze the expression pattern of NtCrRLK1L genes in this study. The roots, stems, leaves, and flowers were collected when tobacco grew to the budding stage, and the samples were stored in the refrigerator at −80°C for later use. The tobacco seedlings were germinated in Murashige and Skoog (MS) solid medium after disinfection, then they were transferred to a liquid MS medium and adapted for 5 days. Based on the previous reference, the seedlings were treated with 150 mmol/L NaCl liquid MS medium or placed on absorbent filter paper for salt and drought treatment, respectively, and the samples were harvested at 0, 1, 3, or 6 h after treatment (Li et al., 2021). All the samples were frozen in liquid nitrogen and transferred to −80°C for later use. Triple biological replicates were performed for each sample.
RNA Extraction and qRT-PCR
Ultrapure RNA Kit (Cwbiotech, Beijing, China) was used to extract the total RNA of each sample, and the cDNA was synthesized using Evo M-MLV Mix Kit with gDNA Clean for qPCR (Accurate Biotechnology, Changsha, China). The qRT-PCR was performed on Roche LightCyclerR 480 by using SYBR Green Premix Pro Taq HS qPCR Kit (Accurate Biotechnology, Changsha, China). The tobacco ribosomal protein-encoding gene L25 (GenBank No. L18908) was used as a control (Li et al., 2021). Three independent experiments were carried out with three technical replicates, and the average value was taken for analysis based on the 2−ΔΔCt method (Livak and Schmittgen, 2001). The primers used are listed in Supplementary Table 1.
Subcellular Localization Analysis of NtCrRLK1L47
The coding sequence of NtCrRLK1L47 and AtCLV1 (as cell membrane control) excluding the termination codon was amplified by Phanta Max Master Mix (Vazyme, Nanjing, China). Then, these sequences and green fluorescent protein (GFP) fragments were inserted into the pCHF3 vector separately, which was driven by the CaMV35S promoter. Furthermore, the CDS of AtCLV1 and yellow fluorescent protein (YFP) fragments were inserted into the pCHF3 vector to construct 35S:: AtCLV1-YFP vector. The reconstructed vectors were confirmed by sequencing (Sangon Biotech, Shanghai, China). The recombinant constructs and control were transiently transformed and expressed in Nicotiana benthamiana leaf epidermal cells for 3 days. Then, the GFP and YFP fluorescence signals were captured using a confocal microscope (TCS-SP8 Leica, Wetzlar, Germany).
Generation of Transgenic Tobacco Plants and Analysis of Salt Stress Tolerance
To construct the overexpression vector, the CDS of NtCrRLK1L47 was amplified by PCR and inserted into the pCHF3 vector by infusion (Clontech, Bejing, China). The recombinant plasmid was transformed into tobacco leaves by the Agrobacterium-mediated method (Buschmann, 2016). The T0 generation plants were screened on the MS medium containing 50 mg/L kanamycin. At T1 generation, the separation ratio was detected on the kanamycin medium to identify the homozygous lines. For salt stress assays, two homozygous lines and wild-type (K326) seeds were surface-sterilized and germinated in a vertical MS medium for 7 days. The seedlings with the same growth stage were transferred to MS medium with 0 or 150 mM NaCl, respectively, to observe changes in root length. The significant difference analysis was calculated by SPSS v18.0 with the t-test.
Statistical Analysis
Statistical significance was determined by conducting Student's t-test or one-way ANOVA using SPSS 23.0 software (SPSS).
Results
Identification of NtCrRLK1L Genes
The BLASTP searches were used to identify NtCrRLK1L genes. A total of 48 NtCrRLK1L genes were identified. Then, the 48 NtCrRLK1L genes were renamed from NtCrRLK1L01 to NtCrRLK1L48 according to their physical locations on each chromosome/scaffold. Characteristics of the NtCrRLK1L proteins, including the number of amino acids, molecular weight (MW), and isoelectric point (pI) were analyzed. The length of 48 NtCrRLK1L proteins ranged from 641 (NtCrRLK1L42) to 1,842 amino acids (NtCrRLK1L33). The molecular weight of NtCrRLK1L proteins ranged from 71.34 (NtCrRLK1L42) to 205.29 (NtCrRLK1L33) kDa, and isoelectric points ranged from 6.24 (NtCrRLK1L42) to 8.41 (NtCrRLK1L05). The detailed information could be explored in Supplementary Table 2.
Multiple Sequence Alignment and Phylogenetic Analysis
To explore the phylogenetic relationship of the NtCrRLK1L proteins, the neighbor-joining tree was constructed based on the multiple sequence alignment of the tobacco CrRLK1L members and reported Arabidopsis members. As a result, those CrRLK1L members were classified into seven groups (Figure 1). Generally, most of the groups contained CrRLK1L members from tobacco and Arabidopsis, indicating that the expansion of CrRLK1L members might appear before the divergence of those species. Among the 7 groups, groups VII and IV contained the largest (18) and smallest (3) numbers of genes, respectively. In Group VI, only NtCrRLK1L48 was divided with AtFER, while AtTHE1 was clustered with multiple NtCrRLK1Ls in group III, which implied that the CrRLK1L members experienced duplication event after species differentiation. Intriguingly, it was found that only the tobacco CrRLK1L proteins fall in Group VII, indicating that the generation of these NtCrRLK1L genes might be caused by duplication events after the differentiation of tested species.
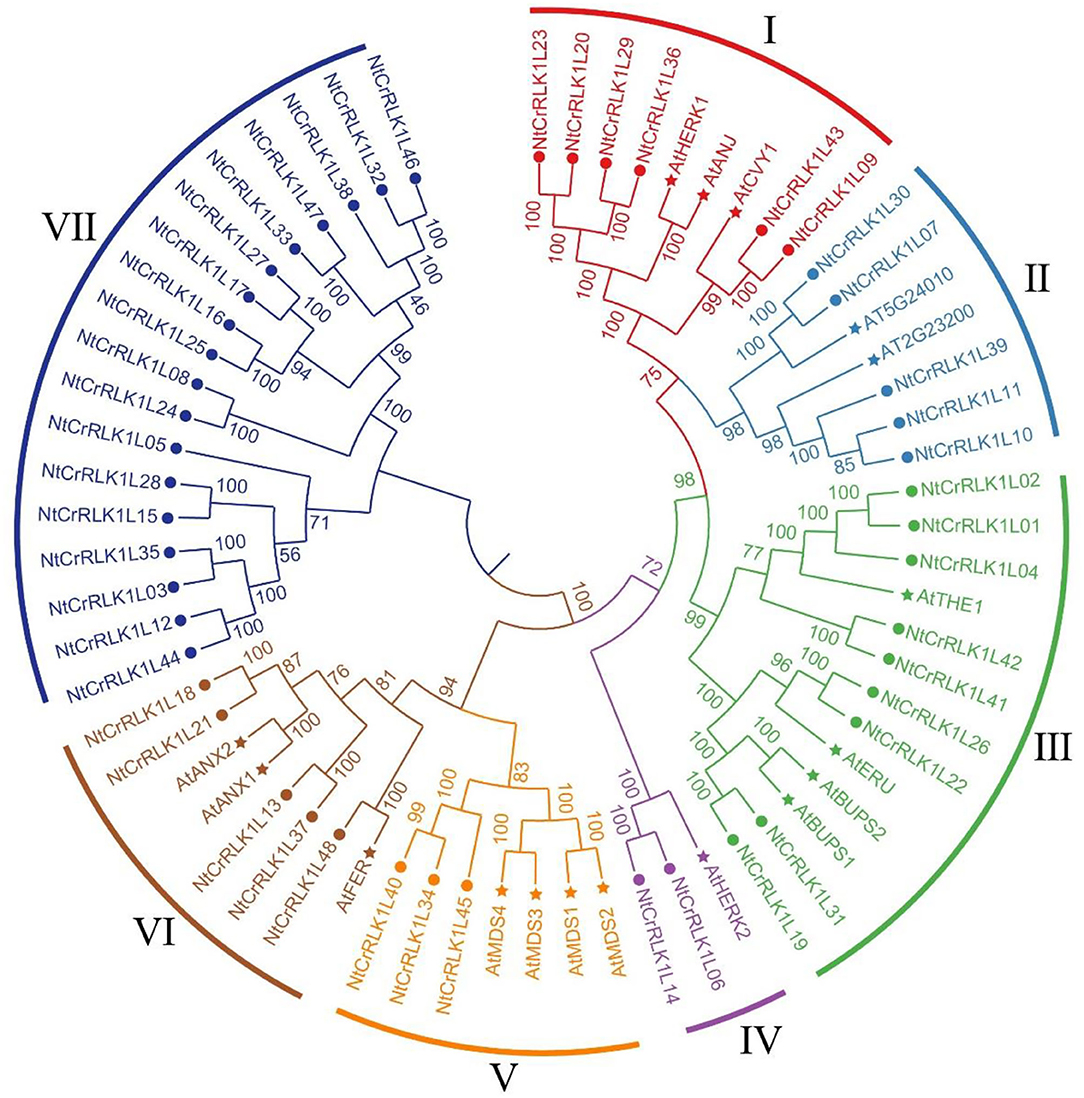
Figure 1. Phylogenetic analysis of tobacco NtCrRLK1L family members. The phylogenetic tree was generated from the alignment of the full-length protein sequence of CrRLK1L proteins from tobacco and Arabidopsis by the neighbor-joining (NJ) method. The tobacco CrRLK1L members together with their Arabidopsis homologs were classified into 7 groups, and distinct groups were marked by different colors.
Gene Structure and Conserved Motif Analysis
The GSDS was adopted to analyze the tobacco CrRLK1L gene structure. As a result, the intron number of studied genes was found to range from 0 to 28 (Supplementary Figure 2). Interestingly, 14 (29.17%) NtCrRLK1L genes were interrupted by one or two introns, whereas 15 (31.25%) NtCrRLK1L genes did not hold any introns. Furthermore, in Group VII, 18 NtCrRLK1L members contained an average of 18.5 introns, of which NtCrRLK1L33 harbored the maximum number of introns, as high as 28 introns. In particular, the AtCrRLK1L members were also analyzed together with NtCrRLK1L, and the result showed that CrRLK1L genes from tobacco and Arabidopsis shared similar gene structures in the same group.
In addition, the CrRLK1L protein sequences were analyzed by the MEME toolbox. As a result, a total of 10 motifs were identified, namely, motif 1–10 (Supplementary Figure 1). As displayed in Supplementary Figure 2, the lengths of these conserved motifs ranged from 21 to 50 aa. Among them, motifs 1, 4, and 6 could be found in all studied CrRLK1L members. Inconsistent with the results of gene structure analysis, the CrRLK1L proteins in the same group usually have similar motifs' types and orders. Notably, the motif compositions of the Group VII members are not as consistent as the other group. For example, only 6 motifs were found in the protein sequence of NtCrRLK1L12 and NtCrRLK1L44, while in NtCrRLK1L33, there were 18 motifs detected. The similarities of characteristic motifs in each group may reflect functional similarities.
NtCrRLK1L Genes Syntenic Analysis
To further study the evolutionary relationship among the tobacco CrRLK1L genes, syntenic analysis was carried out between tobacco and five plant species, including four dicots (Arabidopsis, tomato, potato, and grape) and one monocot species (rice). The result showed that there was a syntenic relationship between 15 of the NtCrRLK1L genes with CrRLK1L genes in tomato, 14 NtCrRLK1L genes in grape, 7 NtCrRLK1L genes in Arabidopsis, 15 NtCrRLK1L genes in potato, and 1 NtCrRLK1L gene in rice. The numbers of predicted collinear pairs between tobacco and tomato, grape, Arabidopsis, potato, and rice were 17, 15, 9, 17, and 1, respectively (Figure 2A).
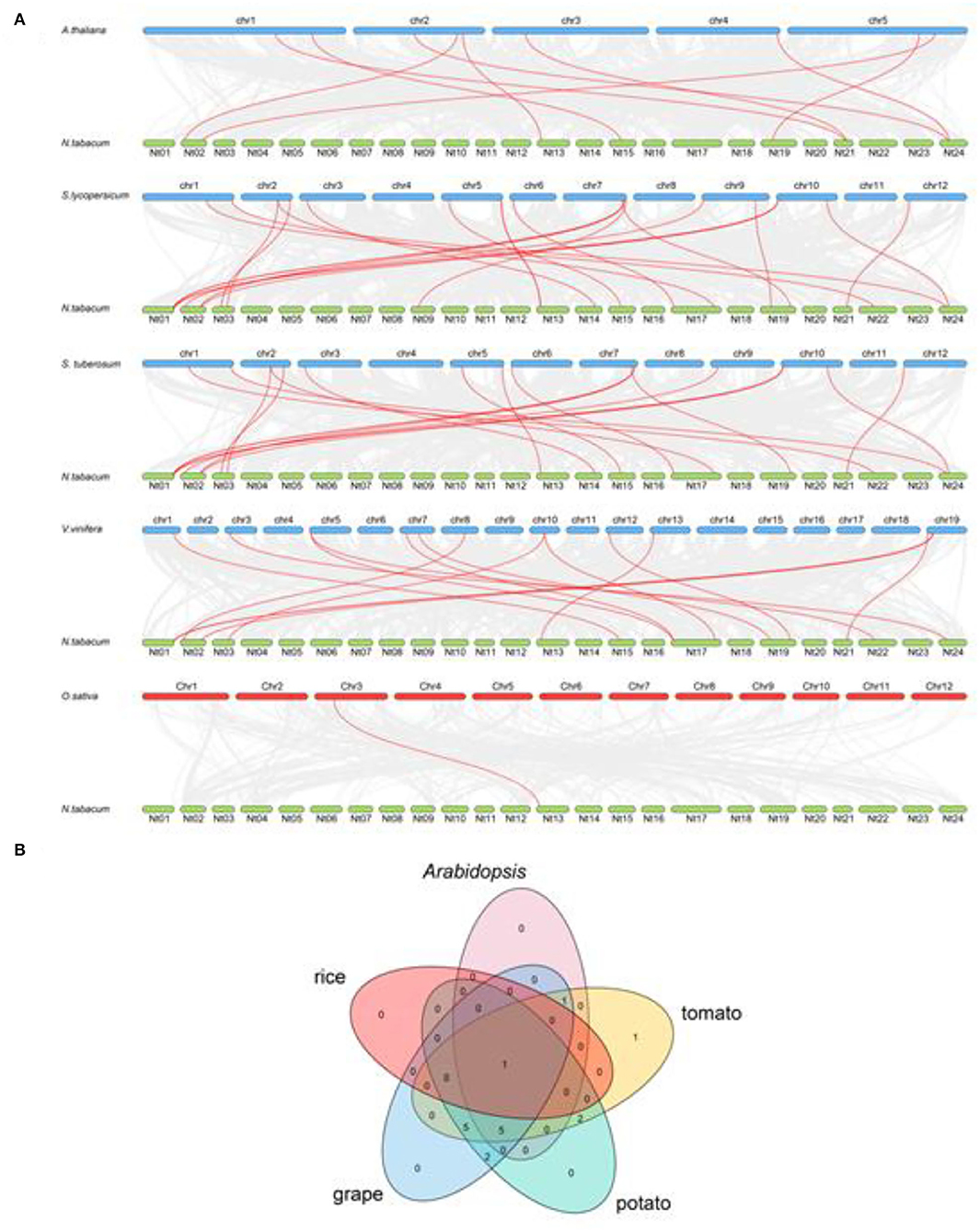
Figure 2. The synteny analysis of CrRLK1L members between tobacco and five other species. (A) The gray line represents all the collinear blocks between tobacco and five other species, while the syntenic CrRLK1L gene pairs were marked by the red line. (B) The CrRLK1L members formed the syntenic pairs between tobacco and five other species, which were analyzed and visualized by the Venn plot.
Particularly, NtCrRLK1L09 were predicted to form collinear pairs with CrRLK1L genes from the five tested species, indicating that this gene may arise before the separation of these species (Figure 2B). Notably, a total of six collinear gene pairs were identified within tobacco and tomato/grape/Arabidopsis/potato members, indicating that those pairs may appear after the divergence of dicot and monocot species except NtCrRLK1L09.
Chromosomal Distribution and Duplication Events
The chromosomal location information of newly identified NtCrRLK1L genes was obtained from the SGN database and visualized using the R. As a result, 20 of the 48 NtCrRLK1L genes were mapped onto 13 tobacco chromosomes (Figure 3), while the remaining NtCrRLK1L genes were located on scaffolds. There were two tobacco CrRLK1L genes found on chromosomes 1, 2, 3, 13, 17, 19, and 24, while chromosomes 4, 9, 14, 15, 21, and 22 only harbored one CrRLK1L gene. It has been reported that a chromosome region possessing two or more genes within 200 kb is defined as a cluster (Li et al., 2019). The location information showed that NtCrRLK1L32 and NtCrRLK1L33 formed one cluster on scaffold Nitab4.5_0005453 (Supplementary Table 2).
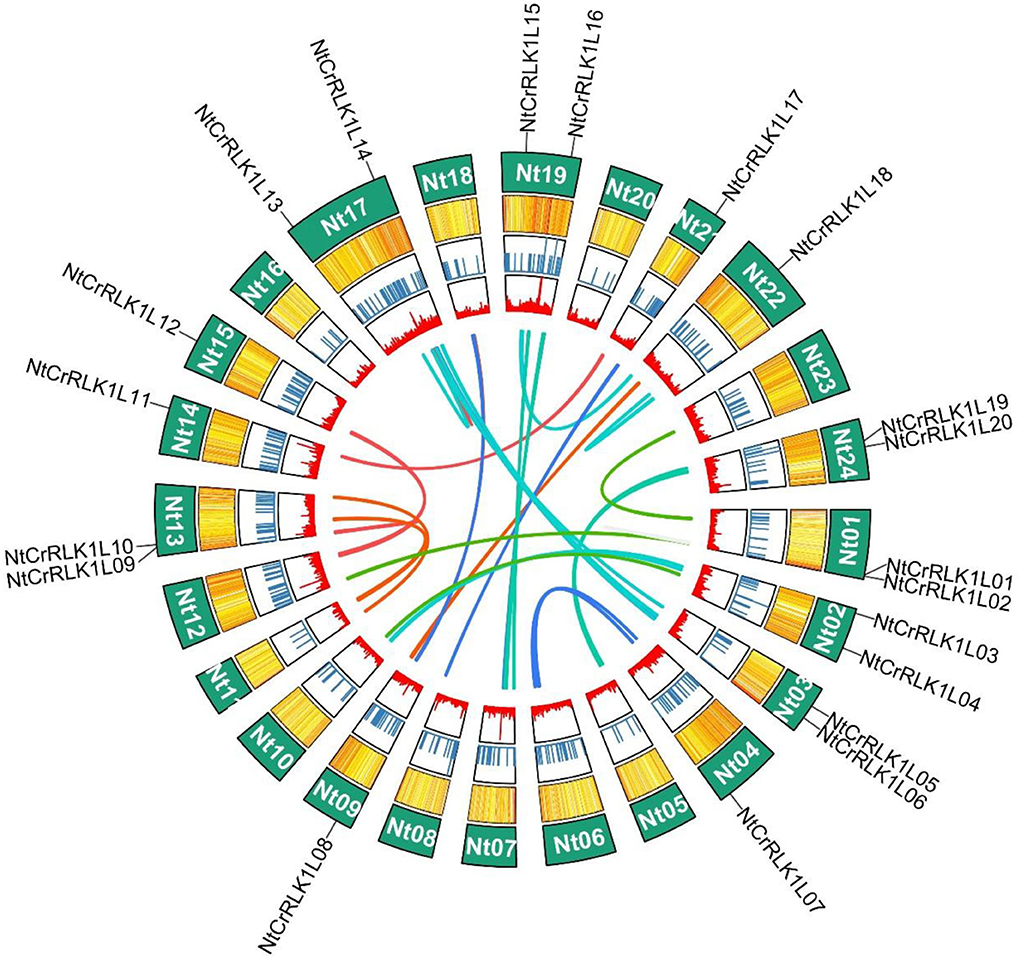
Figure 3. Distribution of NtCrRLK1L genes on tobacco chromosomes. In tobacco, 20 CrRLK1L genes were mapped to 13 tobacco chromosomes, the remaining members were located on scaffolds (not shown in this figure).
The segmental duplication was reported to play important role in the expansion of the gene family. In this study, the MCScanX was used to analyze the segmental duplication of NtCrRLK1L members. As a result, a total of five NtCrRLK1L genes were identified to form 4 segmental duplication pairs (Figure 4). As the reference, the ratio of non-synonymous (Ka) and synonymous (Ks) is one of the important parameters for evolution analysis (Cao et al., 2016). In the current study, the Ka/Ks values of 4 segmental duplication pairs were <1 (Supplementary Table 4), implying that those 4 NtCrRLK1L duplication pairs might have experienced the purifying selective pressure. On the other hand, this result also suggested that the purifying selection occurs in the evolution of the tobacco CrRLK1L family and might play an important role in the conservative function of NtCrRLK1L members.
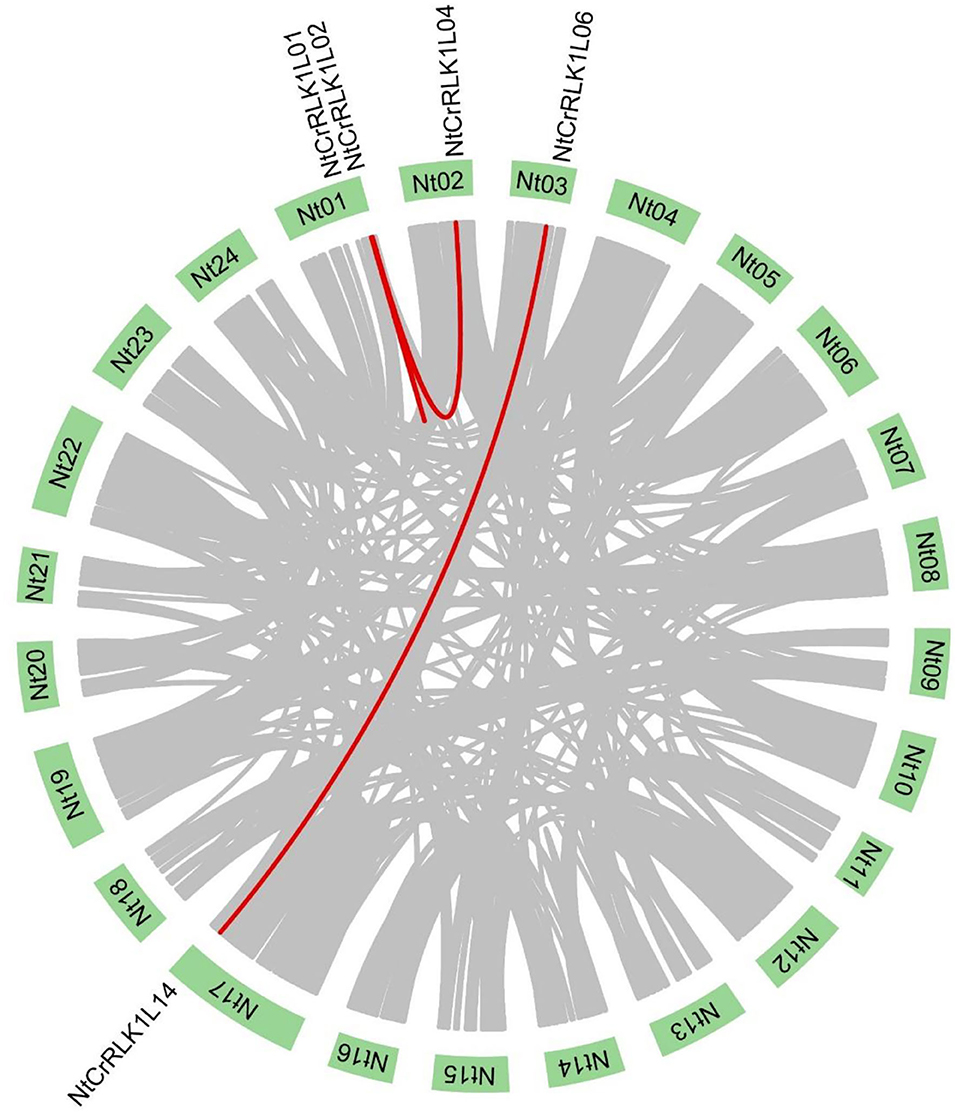
Figure 4. Segmental duplication analysis of the NtCrRLK1L genes. In the tobacco genome, all the putative segmental duplication pairs were marked by gray color, and the red lines represented the NtCrRLK1L segmental duplication pairs.
Analysis of NtCrRLK1L Gene Promoters
To study the regulatory features of the family genes globally, the promoter regions of 48 NtCrRLK1L genes were analyzed by the PlantCARE Online toolbox. Results showed that many cis-elements were detected in the promoter regions of NtCrRLK1L genes, and 14 cis-elements were selected from the PLACE database for visualization (Figure 5). The cis-elements related to stress responses including W-box, MBS, TC-rich repeats, Wun-motif, LTR, and ARE were detected in the promoter regions of many NtCrRLK1L genes, indicating that these genes might be involved in various stress responses. In addition, several hormone-response elements were also identified in promoter regions of NtCrRLK1L genes, including ABRE, TCA-element, ERE, and the CGTCA-motif. In particular, the observation of the ABRE element in the promoter regions of all NtCrRLK1L genes suggests that those genes might be involved in ABA-mediated stress responses.
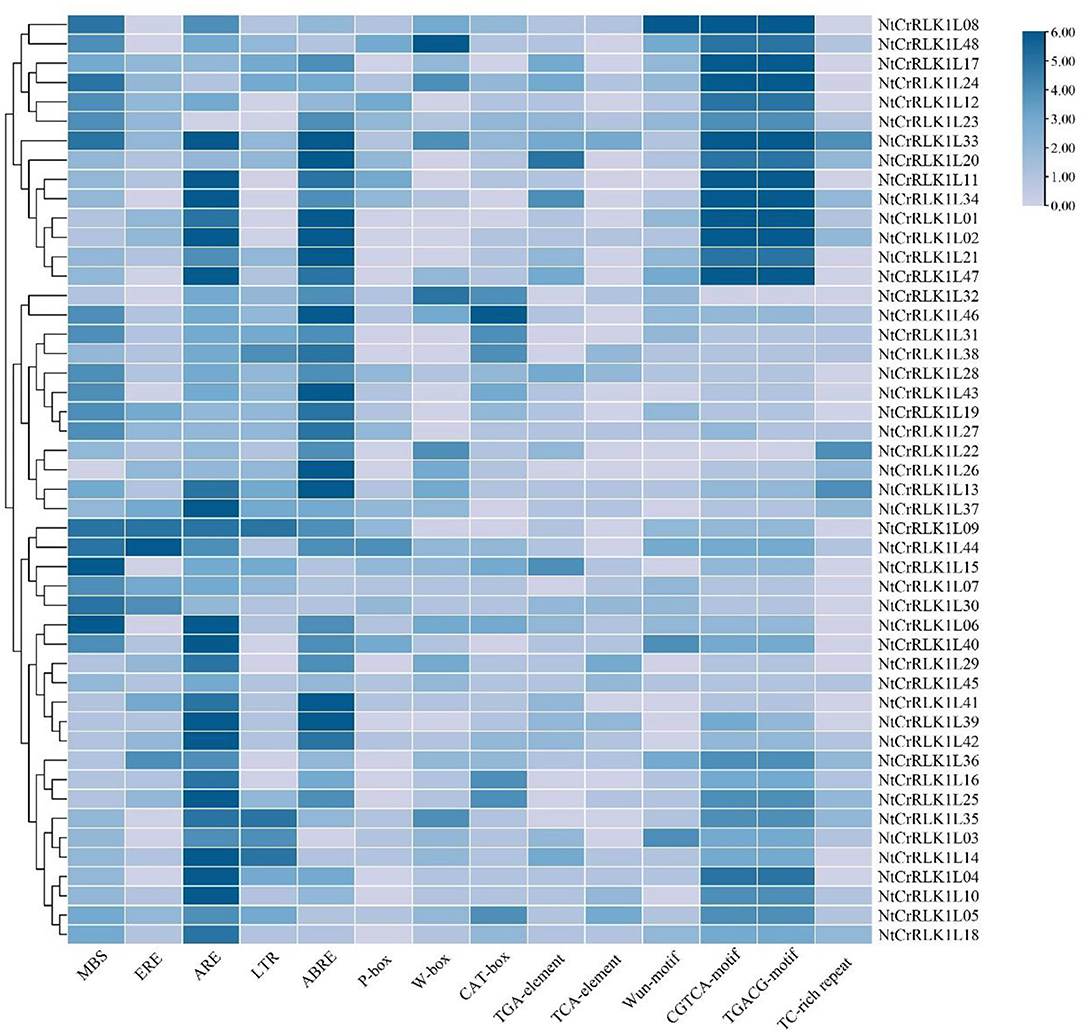
Figure 5. Statistics diagram of cis-elements in the promoter region of NtCrRLK1L genes. The color represents the numbers of cis-element in NtCrRLK1L gene promoters.
Expression Profile of NtCrRLK1L Genes
To preliminarily elucidate the roles of NtCrRLK1L genes in tobacco growth and development, the RNA-seq data about NtCrRLK1L genes in three different tissues were obtained from the NCBI database and analyzed (Edwards et al., 2017). As the result (Figure 6), among 48 NtCrRLK1L genes, 40 were detected to be expressed in at least one of the three tested tissues, and eight were highly expressed in all three tissues, especially NtCrRLK1L48. Furthermore, several genes exhibited tissue-specific expression patterns. For example, the expression of NtCrRLK1L05 in Group VII was detected in the roots only. In Group III, NtCrRLK1L02 and NtCrRLK1L42 were detected exclusively in the roots. Similarly, NtCrRLK1L09 was detected exclusively in the shoot apex. Notably, expressions of eight NtCrRLK1L genes were not detected in those three test tissues.
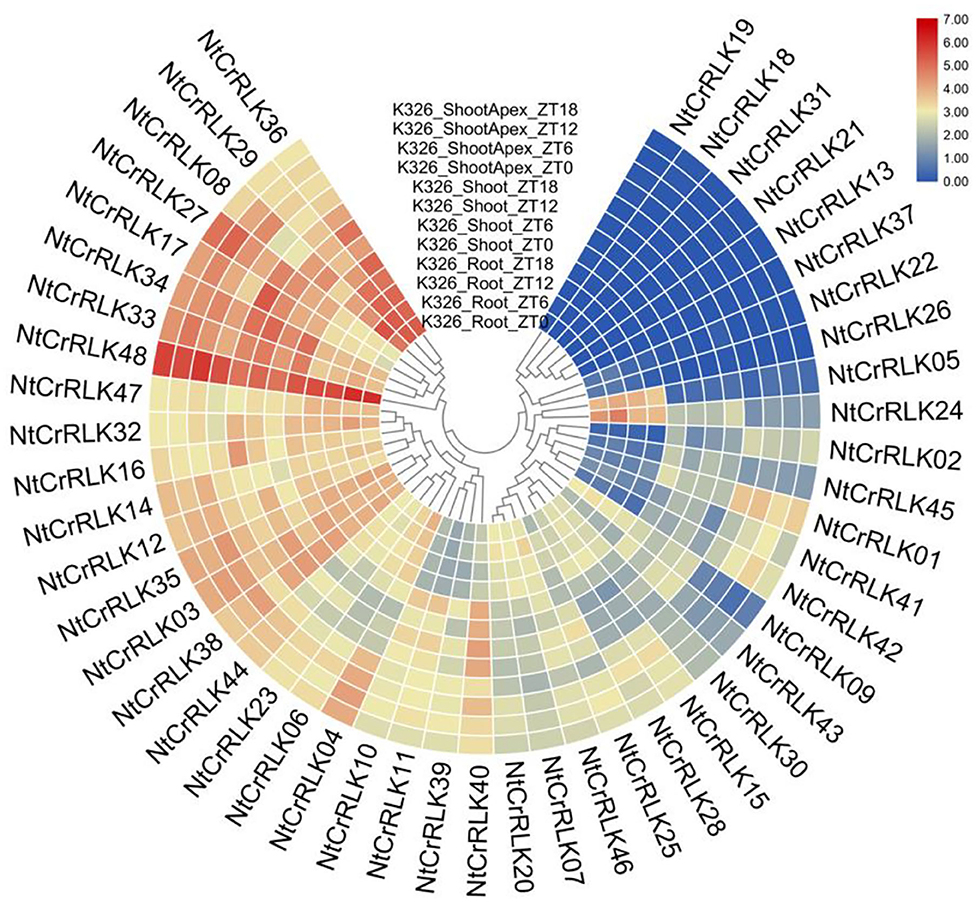
Figure 6. Expression patterns of the NtCrRLK1L genes in the tested tissues (root, shoot, and shoot apex). The data were retrieved from transcriptome data and visualized by R.
Validation and Enrichment of Expression Patterns by qRT-PCR
To confirm and enrich the expression patterns of the NtCrRLK1L genes from RNA-seq analysis, representative NtCrRLK1L genes from different groups were selected to perform qRT-PCR analysis. The tobacco tissues including root, stem, flower, upper leaf, middle leaf, and lower leaf were obtained to detect the expression changes of NtCrRLK1L genes. As a result (Figure 7), the selected NtCrRLK1L genes exhibit multiple expression patterns. For instance, NtCrRLK1L02 was highly expressed in the other five tissues exclusively in the roots, and NtCrRLK1L47 and NtCrRLK1L48 were detected in all six tested tissues, which was consistent with the RNA-seq analysis. In Group II, NtCrRLK1L07 exhibited a tissue-specific expression pattern and was expressed exclusively in roots, while NtCrRLK1L39 was expressed in all tested tissues. This result suggested that the functional differentiation may occur in the same group of NtCrRLK1L.
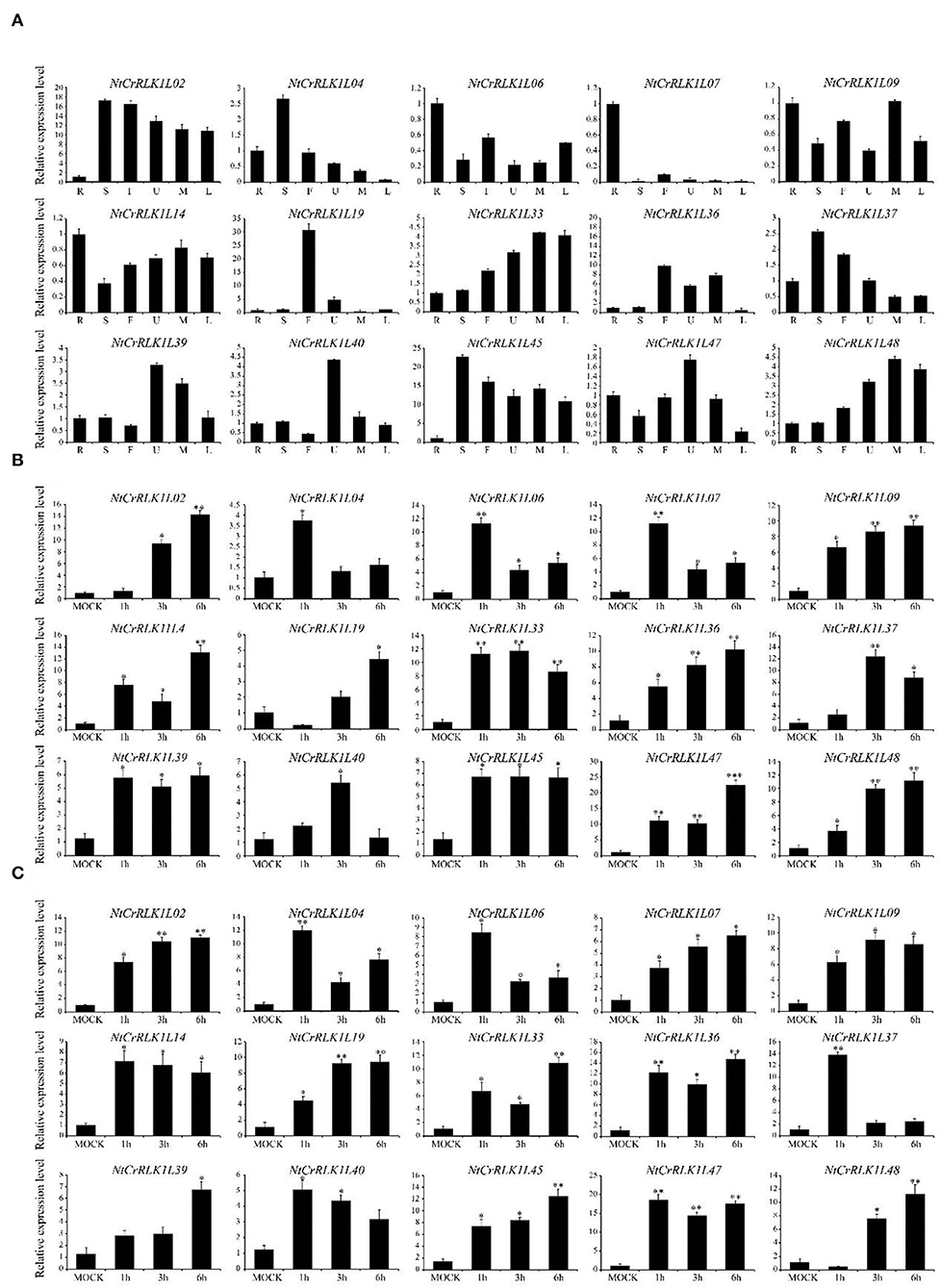
Figure 7. qRT-PCR analysis of representative NtCrRLK1L genes from different groups. (A) Expression patterns of the NtCrRLK1L genes in different tissues. R (root), S (stem), F (flower), U (upper leaf), M (middle leaf), and L (lower leaf). To verify the tissue specificity expression of the selected NtCrRLK1L genes, the expression level of each gene was calculated relative to the root tissue. (B) The expression level of the selected NtCrRLK1L genes under salt stress treatments. (C) The expression level of selected NtCrRLK1L genes under drought stress treatments. The data were means ±SD from three independent replications. *p < 0.05; **p < 0.01.
Furthermore, several NtCrRLK1L genes were selected to test whether they could respond to salt and drought stresses. As a result, the expression patterns of most NtCrRLK1L genes under salt or drought stress were time-specific. For example, the expression level of NtCrRLK1L04, NtCrRLK1L06, and NtCrRLK1L07 reached the peak at 1 h of salt treatment, then dropped sharply. Notably, NtCrRLK1L19 was inhibited after 1 h of salt treatment, while its expression level reached a peak (~4-fold) after 6 h of salt treatment, and the expression of the NtCrRLK1L19 gene continued to rise under drought treatment. Among them, NtCrRLK1L47 had the highest expression level no matter the salt and drought treatment. Its expression level reached the peak after 6 h by the salt treatment (22-fold) and 1 h by drought treatment (18-fold). Thence, the NtCrRLK1L47 gene was selected for further study.
Subcellular Localization Analysis of NtCrRLK1L47
To further explore the subcellular localization of the NtCrRLK1L genes, the subcellular localization of the NtCrRLK1L47 gene was selected for analysis (Figure 8). As revealed by confocal microscopy, the green fluorescence of the AtCLV1-GFP is only distributed on the cell membrane as previously reported (Stahl et al., 2013), whereas the signal of GFP protein was found to distribute throughout the whole cell. In addition, the signal of NtCrRLK1L47-GFP fusion protein was specifically confined to the membrane. Besides, the co-localization assay was carried out, and the fluorescence signal of the NtCrRLK1L47-GFP fusion protein was found to be consistent with AtCLV1-YFP (Supplementary Figure 3). Considering these clues, NtCrRLK1L47 protein was proved to localize on the membrane.
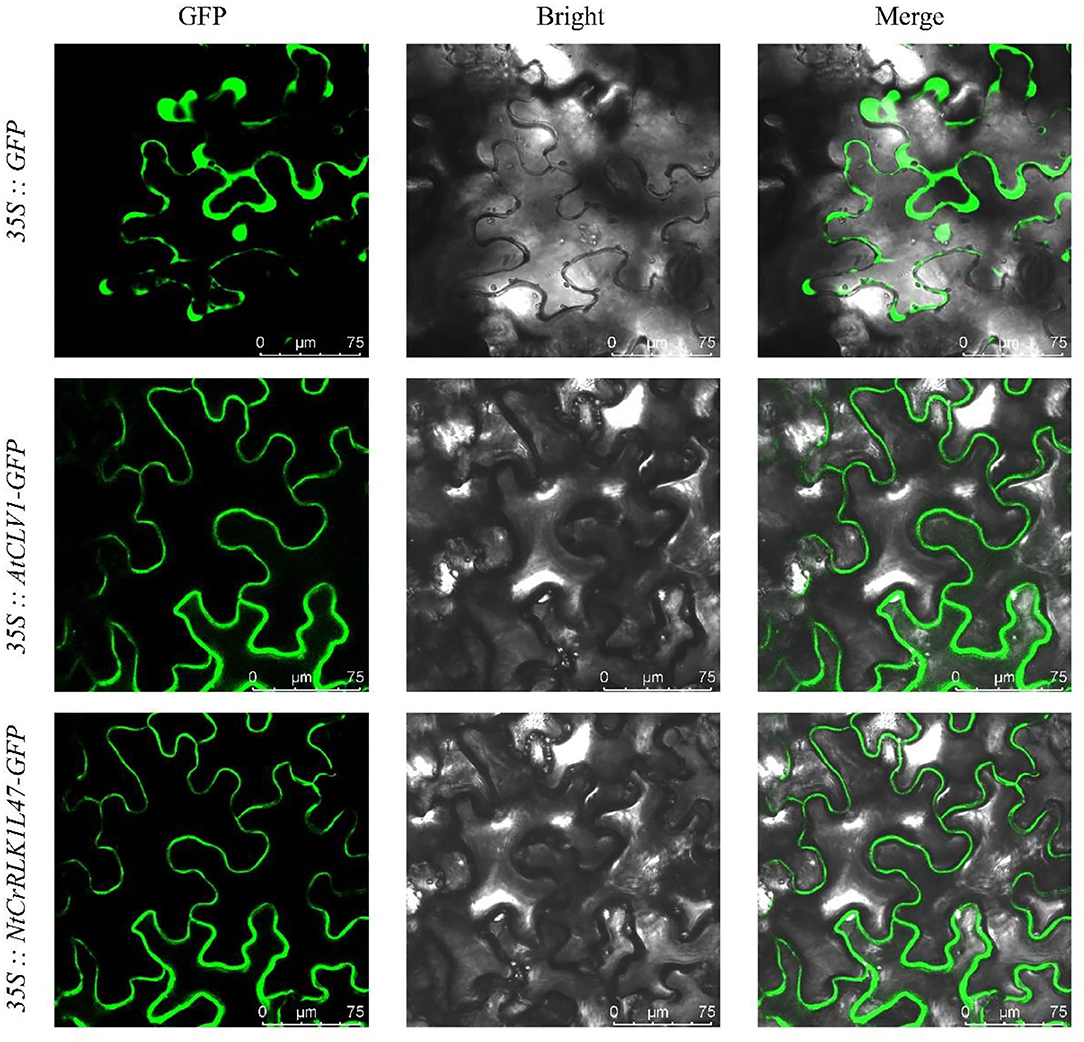
Figure 8. Subcellular localization of NtCrRLK1L47 protein within the cell by GFP assays. The 35::GFP, 35::AtCLV1-GFP, and 35::NtCrRLK1L47-GFP constructs were transiently transformed and expressed in Nicotiana benthamiana leaf epidermal cells; the GFP signals were visualized on a confocal laser microscope.
Overexpression of NtCrRLK1L47 Enhanced the Salt Tolerances
The genetic experiments were performed to explore the potential function of the NtCrRLK1L47 genes in salt tolerance, and we obtained two overexpression lines of the NtCrRLK1L47 gene, NtCrRLK1L47-OE4 and NtCrRLK1L47-OE5. Then, the root elongation assay was carried out and no obvious differences were observed between wildtype and NtCrRLK1L47-OE lines under normal growth conditions. However, the NtCrRLK1L47-OE4 and NtCrRLK1L47-OE5 lines showed better growth performance than wildtype plants under salt treatments. The root elongation assay showed that significantly longer roots of the two independent overexpression lines were measured compared to the wildtype on 150 mM NaCl. This result indicated that NtCrRLK1L47 conferred salt tolerance on transgenic tobacco seedlings.
Discussion
The CrRLK1L gene family has been reported to play important roles in plant development, metabolism, and stress responses. In the current study, a comprehensive analysis of the CrRLK1L family in tobacco was carried out. As a result, a total of 48 tobacco CrRLK1L members were identified. Furthermore, the phylogenetic and syntenic analyses were integrated to identify the homologs of tobacco CrRLK1L genes with reported members and infer their potential functions. Furthermore, to verify these inferences, several genes were selected to perform the expression and function analysis.
In the current study, CrRLK1L family members of tobacco and Arabidopsis were clustered within seven groups. In Group III, NtCrRLK1L19 was clustered together with AtBUPS1 and AtBUPS2 (Figure 1), and their coding genes were predicted to form the collinear pairs (Figure 2), suggesting that the NtCrRLK1L19 might be the homolog of AtBUPS1 and AtBUPS2. Interestingly, it was indicated that the reactive oxygen species (ROS) served downstream of the BUPS/ANX receptor complex, which might confer stress response complex (Zhang et al., 2020a; Kim et al., 2021). Meanwhile, our results revealed that the promoter of the NtCrRLK1L19 gene contains many stress-response elements, and this gene was proved to be induced by salt stress. These clues implied, as the homolog of AtBUPS1 and AtBUPS2, the NtCrRLK1L19 gene might be involved in the stress responses. Furthermore, in the same group, AtTHE1 was reported to function in plant cell wall synthesis and cell expansion and detected to express in root and shoot (Hématy et al., 2007; Merz et al., 2017). In our study, NtCrRLK1L01, NtCrRLK1L02, and NtCrRLK1L04 were clustered together with AtTHE1 (Figure 1), and their encoding genes were predicted to form collinear pairs with AtTHE1, respectively (Figure 2), proving that these tobacco genes might be homologous genes of AtTHE1. Furthermore, NtCrRLK1L01, NtCrRLK1L02, and NtCrRLK1L04 were identified to form two segmental duplication pairs, implying that duplication events were raised after the segregation of these two species (Figure 4). Interestingly, the expression analysis showed that the expression patterns of these genes were discrepant. NtCrRLK1L01 and NtCrRLK1L02 were detected to mainly express in the shoot apex, while NtCrRLK1L04 expressed in all detected tissues, especially more in the stem (Figures 6, 7). The results presented here indicated that these duplicated genes might undergo subfunctionalization.
In Group I, NtCrRLK1L09 was clustered with AtCVY1, and the encoding gene was predicted to form collinear pairs with AtCVY1 and OsFLR13, respectively (Figures 1, 2). Interestingly, OsFLR13 was clustered with AtCVY1 in a previous study (Yang et al., 2020), which implies that NtCrRLK1L09 acts as the homolog of OsFLR13 and AtCVY1 in tobacco. AtCVY1 was reported to regulate cell morphogenesis, flowering, and seed production. In the current study, NtCrRLK1L09 was detected to express in flowers (Figure 7), suggesting this gene might undertake the conserved function in tobacco.
Furthermore, the CrRLK1L genes in Group VII were unique to tobacco (Figure 1), suggesting that this family might appear after the differentiation of these two species. In addition, NtCrRLK1L32 and NtCrRLK1L33 were found to fall into the same cluster (Supplementary Table 2), indicating that the duplication event might drive the expansion of this group. Considering the unsatisfactory quality of the tobacco genome, most members from this group could not map the chromosomes, and there might be chances to identify duplication events of this group in the future. Furthermore, various stress-response cis-elements were found to attend in the promoter region of this group members, including W-box, MBS, and ARE (Figure 5), indicating that the members of this group might be involved in stress responses. Then, several representative genes were investigated with the expression profile under drought and salt stresses (Figure 7). Notably, NtCrRLK1L47 could be highly induced by these two treatments (Figure 7). The subcellular localization analysis showed that NtCrRLK1L47-GFP fusion protein was localized on the membrane (Figure 8). Not surprisingly, it was found that the overexpression of the NtCrRLK1L47 gene could enhance the salt tolerance in tobacco seedlings (Figure 9), suggesting that NtCrRLK1L47 may act as a membrane receptor regulating stress responses.
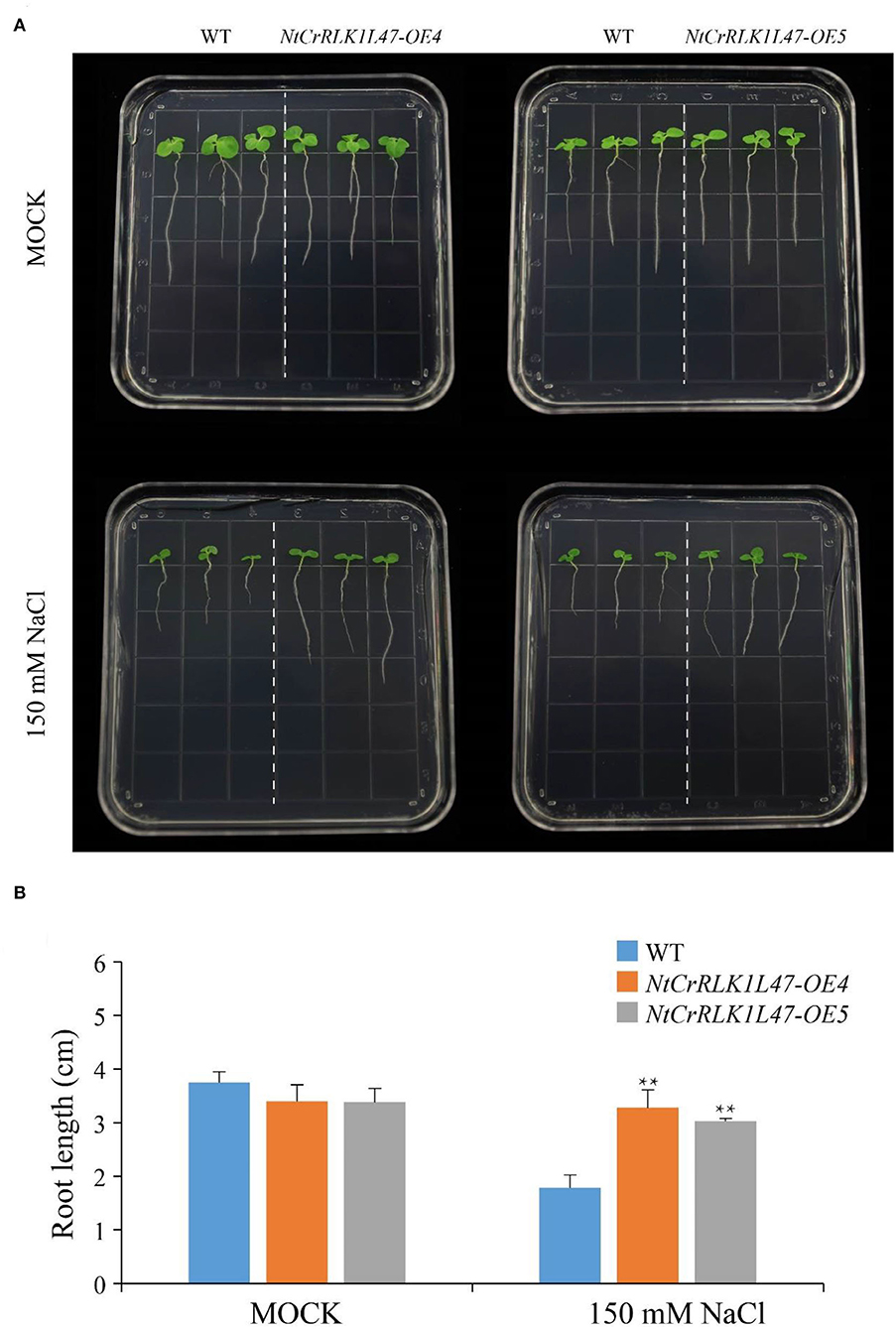
Figure 9. Phenotype and function analysis of NtCrRLK1L47 gene in transgenic tobacco under salt stress conditions. (A) Root growth experiment of wild type and NtCrRLK1L47 overexpression lines under 0 or 150 mM NaCl treatment. (B) The quantification of tobacco root length and the data were collected from more than 15 plants per genotype with three biological replicates. WT, wild type. Values represent means ± SD. **p < 0.01 (t-tests).
Conclusion
In this study, we identified 48 CrRLK1L genes in the tobacco genome, and comprehensive and systematic analyses were performed to interpret the evolutionary relationship of CrRLK1L family members. Furthermore, the promoter cis-element and expression pattern analysis showed that many cis-elements related to stress responses existed in the promoter regions of the NtCrRLK1L47 gene, and NtCrRLK1L47 was significantly up-regulated under salt stress conditions. Furthermore, the genetic experiments also confirmed that NtCrRLK1L47 provides effective resistance against salt stressors. These results provide insight into understanding the evolutionary mechanism of NtCrRLK1L genes and could be helpful to explore the biological functions of NtCrRLK1L members in the future.
Data Availability Statement
The datasets presented in this study can be found in online repositories. The names of the repository/repositories and accession number(s) can be found in the article/Supplementary Material.
Author Contributions
XL and CG conducted the research and drafted the manuscript. QW, ZL, JC, DW, YL, AY, YG, and JG assisted in data collection and analysis. LW and WP conceived the research and drafted the manuscript. All authors read and approved this final manuscript.
Funding
This research was financially supported by the China Tobacco Genome Project [110202001029(JY-12) and 110202101003(JY-03)], the China Tobacco Hunan Industrial Co., Ltd. Technology Project (KY2021YC0001), and the Agricultural Science and Technology Innovation Program (ASTIP-TRIC01 & 02).
Conflict of Interest
XL, JG, and WP were employed by China Tobacco Hunan Industrial Co., Ltd.
The remaining authors declare that the research was conducted in the absence of any commercial or financial relationships that could be construed as a potential conflict of interest.
Publisher's Note
All claims expressed in this article are solely those of the authors and do not necessarily represent those of their affiliated organizations, or those of the publisher, the editors and the reviewers. Any product that may be evaluated in this article, or claim that may be made by its manufacturer, is not guaranteed or endorsed by the publisher.
Supplementary Material
The Supplementary Material for this article can be found online at: https://www.frontiersin.org/articles/10.3389/fpls.2022.838857/full#supplementary-material
Supplementary Table 1. qRT-PCR primers and sequences used in this study.
Supplementary Table 2. Features of NtCrRLK1L genes identified in tobacco.
Supplementary Table 3. Synteny blocks of NtCrRLK1L genes between tobacco and other five plant species.
Supplementary Table 4. The detailed information of segmental duplication of gene pairs within the tobacco genome.
Supplementary Figure 1. Conserved motifs and exon–intron structure organizations of the NtCrRLK1L members.
Supplementary Figure 2. The detailed sequence information for each motif in the NtCrRLK1L proteins.
Supplementary Figure 3. The co-localization assay of NtCrRLK1L47 with AtCLV1.
Supplementary Figure 4. The relative expression level of the NtCrRLK1L47 gene in wild type and two overexpression lines; the relative expression level of each genotype was calculated relative to the wild type.
References
Bailey, T. L., Johnson, J., Grant, C. E., and Noble, W. S. (2015). The MEME suite. Nucl. Acids Res. 43, W39–W49. doi: 10.1093/nar/gkv416
Boisson-Dernier, A., Kessler, S. A., and Grossniklaus, U. (2011). The walls have ears: the role of plant CrRLK1Ls in sensing and transducing extracellular signals. J. Exp. Bot. 62, 1581–1591. doi: 10.1093/jxb/erq445
Buschmann, H.. (2016). Plant cell division analyzed by transient agrobacterium-mediated transformation of tobacco BY-2 Cells. Methods Mol. Biol. 1370, 17–25. doi: 10.1007/978-1-4939-3142-2_2
Cao, Y., Han, Y., Li, D., Lin, Y., and Cai, Y. (2016). MYB transcription factors in chinese pear (Pyrus bretschneideri Rehd.): genome-wide identification, classification, and expression profiling during fruit development. Front. Plant Sci. 7, 577. doi: 10.3389/fpls.2016.00577
Chen, C., Chen, H., Zhang, Y., Thomas, H. R., Frank, M. H., He, Y., et al. (2020). TBtools: an integrative toolkit developed for interactive analyses of big biological data. Mol. Plant 13, 1194–1202. doi: 10.1016/j.molp.2020.06.009
Chen, J., Yu, F., Liu, Y., Du, C., Li, X., Zhu, S., et al. (2016). FERONIA interacts with ABI2-type phosphatases to facilitate signaling cross-talk between abscisic acid and RALF peptide in Arabidopsis. Proc. Natl. Acad. Sci. U. S. A. 113, E5519–E5527. doi: 10.1073/pnas.1608449113
Dievart, A., Gottin, C., Périn, C., Ranwez, V., and Chantret, N. (2020). Origin and diversity of plant receptor-like kinases. Annu. Rev. Plant Biol. 71, 131–156. doi: 10.1146/annurev-arplant-073019-025927
Duan, Q., Liu, M. C. J., Kita, D., Jordan, S. S., Yeh, F. L. J., Yvon, R., et al. (2020). FERONIA controls pectin-and nitric oxide-mediated male–female interaction. Nature 579, 561–566. doi: 10.1038/s41586-020-2106-2
Edwards, K., Fernandez-Pozo, N., Drake-Stowe, K., Humphry, M., Evans, A., Bombarely, A., et al. (2017). A reference genome for Nicotiana tabacum enables map-based cloning of homeologous loci implicated in nitrogen utilization efficiency. BMC Genom. 18, 1–14. doi: 10.1186/s12864-017-3791-6
Feng, H., Liu, C., Fu, R., Zhang, M., Li, H., Shen, L., et al. (2019). LORELEI-LIKE GPI-ANCHORED PROTEINS 2/3 regulate pollen tube growth as chaperones and coreceptors for ANXUR/BUPS receptor kinases in Arabidopsis. Mol. Plant 12, 1612–1623. doi: 10.1016/j.molp.2019.09.004
Franck, C. M., Westermann, J., and Boisson-Dernier, A. (2018). Plant malectin-like receptor kinases: from cell wall integrity to immunity and beyond. Annu. Rev. Plant Biol. 69, 301–328. doi: 10.1146/annurev-arplant-042817-040557
Garg, V. K., Avashthi, H., Tiwari, A., Jain, P. A., Ramkete, P. W., Kayastha, A. M., et al. (2016). MFPPI–multi FASTA ProtParam interface. Bioinformation 12, 74. doi: 10.6026/97320630012074
Ge, Z., Dresselhaus, T., and Qu, L.-J. (2019a). How CrRLK1L receptor complexes perceive RALF signals. Trends Plant Sci. 24, 978–981. doi: 10.1016/j.tplants.2019.09.002
Ge, Z., Zhao, Y., Liu, M.-C., Zhou, L.-Z., Wang, L., Zhong, S., et al. (2019b). LLG2/3 are co-receptors in BUPS/ANX-RALF signaling to regulate Arabidopsis pollen tube integrity. Curr. Biol. 29, 3256–3265. e3255. doi: 10.1016/j.cub.2019.08.032
Gonneau, M., Desprez, T., Martin, M., Doblas, V. G., Bacete, L., Miart, F., et al. (2018). Receptor kinase THESEUS1 is a rapid alkalinization factor 34 receptor in Arabidopsis. Curr. Biol. 28, 2452–2458. e2454. doi: 10.1016/j.cub.2018.05.075
Guo, H., Li, L., Ye, H., Yu, X., Algreen, A., and Yin, Y. (2009). Three related receptor-like kinases are required for optimal cell elongation in Arabidopsis thaliana. Proc. Natl. Acad. Sci. U. S. A. 106, 7648–7653. doi: 10.1073/pnas.0812346106
Guo, H., Nolan, T. M., Song, G., Liu, S., Xie, Z., Chen, J., et al. (2018). FERONIA receptor kinase contributes to plant immunity by suppressing jasmonic acid signaling in Arabidopsis thaliana. Curr. Biol. 28, 3316–3324. e3316. doi: 10.1016/j.cub.2018.07.078
Han, Y. F., Yang, Q., Zhang, S. W., Sun, D. Y., and Sun, Y. (2011). Receptor-like kinase CrRLK1-L subfamily: novel motifs in extracellular domain and biological functions in plants. Prog. Biochem. Biophys. 38, 891–899. doi: 10.3724/SP.J.1206.2011.00085
Haruta, M., Sabat, G., Stecker, K., Minkoff, B. B., and Sussman, M. R. (2014). A peptide hormone and its receptor protein kinase regulate plant cell expansion. Science 343, 408–411. doi: 10.1126/science.1244454
Hématy, K., and Höfte, H. (2008). Novel receptor kinases involved in growth regulation. Curr. Opin. Plant Biol. 11, 321–328. doi: 10.1016/j.pbi.2008.02.008
Hématy, K., Sado, P.-E., Van Tuinen, A., Rochange, S., Desnos, T., and Balzergue, S. (2007). A receptor-like kinase mediates the response of Arabidopsis cells to the inhibition of cellulose synthesis. Curr. Biol. 17, 922–931. doi: 10.1016/j.cub.2007.05.018
Hu, B., Jin, J., Guo, A. Y., Zhang, H., Luo, J., and Gao, G. (2015). GSDS 2.0: an upgraded gene feature visualization server. Bioinformatics 31, 1296–1297. doi: 10.1093/bioinformatics/btu817
Huck, N., Moore, J. M., Federer, M., and Grossniklaus, U. (2003). The Arabidopsis mutant feronia disrupts the female gametophytic control of pollen tube reception. Development. 130, 2149–2159. doi: 10.1242/dev.00458
Jia, M., Du, P., Ding, N., Zhang, Q., Xing, S., Wei, L., et al. (2017). Two FERONIA-like receptor kinases regulate apple fruit ripening by modulating ethylene production. Front. Plant Sci. 8, 1406. doi: 10.3389/fpls.2017.01406
Katoh, K., and Standley, D. M. (2013). MAFFT multiple sequence alignment software version 7: improvements in performance and usability. Mol. Biol. Evol. 30, 772–780. doi: 10.1093/molbev/mst010
Kim, Y. J., Kim, M. H., Hong, W. J., Moon, S., Tae Kim, S., Ki Park, S., et al. (2021). OsMTD2-mediated reactive oxygen species (ROS) balance is essential for intact pollen-tube elongation in rice. Plant J. 107, 1131–1147. doi: 10.1111/tpj.15373
Kou, X., Qi, K., Qiao, X., Yin, H., Liu, X., Zhang, S., et al. (2017). Evolution, expression analysis, and functional verification of Catharanthus roseus RLK1-like kinase (CrRLK1L) family proteins in pear (Pyrus bretchneideri). Genomics 109, 290–301. doi: 10.1016/j.ygeno.2017.05.003
Kumar, S., Stecher, G., Li, M., Knyaz, C., and Tamura, K. (2018). MEGA X: molecular evolutionary genetics analysis across computing platforms. Mol. Biol. Evol. 35, 1547. doi: 10.1093/molbev/msy096
Lescot, M., Déhais, P., Thijs, G., Marchal, K., Moreau, Y., Van De Peer, Y., et al. (2002). PlantCARE, a database of plant cis-acting regulatory elements and a portal to tools for in silico analysis of promoter sequences. Nucl. Acids Res. 30, 325–327. doi: 10.1093/nar/30.1.325
Li, C., Wang, L., Cui, Y., He, L., Qi, Y., Zhang, J., et al. (2016). Two FERONIA-like receptor (FLR) genes are required to maintain architecture, fertility, and seed yield in rice. Mol. Breed. 36, 1–11. doi.org/10.1007/s11032-016-0580-x doi: 10.1007/s11032-016-0580-x
Li, X., Guo, C., Ahmad, S., Wang, Q., Yu, J., Liu, C., et al. (2019). Systematic analysis of MYB family genes in potato and their multiple roles in development and stress responses. Biomolecules 9, 317. doi: 10.3390/biom9080317
Li, Z., Chao, J., Li, X., Li, G., Song, D., Guo, Y., et al. (2021). Systematic Analysis of the bZIP Family in Tobacco and Functional Characterization of NtbZIP62 Involvement in Salt Stress. Agronomy 11, 148. doi: 10.3390/agronomy11010148
Lindner, H., Müller, L. M., Boisson-Dernier, A., and Grossniklaus, U. (2012). CrRLK1L receptor-like kinases: not just another brick in the wall. Curr. Opin. Plant Biol. 15, 659–669. doi: 10.1016/j.pbi.2012.07.003
Livak, K. J., and Schmittgen, T. D. (2001). Analysis of relative gene expression data using real-time quantitative PCR and the 2−ΔΔCT method. Methods 25, 402–408. doi: 10.1006/meth.2001.1262
Merz, D., Richter, J., Gonneau, M., Sanchez-Rodriguez, C., Eder, T., Sormani, R., et al. (2017). T-DNA alleles of the receptor kinase THESEUS1 with opposing effects on cell wall integrity signaling. J. Exp. Bot. 68, 4583–4593. doi: 10.1093/jxb/erx263
Morillo, S. A., and Tax, F. E. (2006). Functional analysis of receptor-like kinases in monocots and dicots. Curr. Opin. Plant Biol. 9, 460–469. doi: 10.1016/j.pbi.2006.07.009
Nibau, C., and Cheung, A. (2011). New insights into the functional roles of CrRLKs in the control of plant cell growth and development. Plant Signal Behav. 6, 655–659. doi: 10.4161/psb.6.5.14951
Niu, E., Cai, C., Zheng, Y., Shang, X., Fang, L., and Guo, W. (2016). Genome-wide analysis of CrRLK1L gene family in Gossypium and identification of candidate CrRLK1L genes related to fiber development. Mol. Genet Genom. 291, 1137–1154. doi: 10.1007/s00438-016-1169-0
Pu, C. X., Han, Y. F., Zhu, S., Song, F. Y., Zhao, Y., Wang, C. Y., et al. (2017). The rice receptor-like kinases DWARF AND RUNTISH SPIKELET1 and 2 repress cell death and affect sugar utilization during reproductive development. Plant Cell 29, 70–89. doi: 10.1105/tpc.16.00218
Richter, J., Watson, J. M., Stasnik, P., Borowska, M., Neuhold, J., Berger, M., et al. (2018). Multiplex mutagenesis of four clustered CrRLK1L with CRISPR/Cas9 exposes their growth regulatory roles in response to metal ions. Sci. Rep. 8, 1–14. doi: 10.1038/s41598-018-30711-3
Rozas, J., Ferrer-Mata, A., Sánchez-Delbarrio, J. C., Guirao-Rico, S., Librado, P., Ramos-Onsins, S. E., et al. (2017). DnaSP 6: DNA sequence polymorphism analysis of large data sets. Mol. Biol. Evol. 34, 3299–3302. doi: 10.1093/molbev/msx248
Schallus, T., Jaeckh, C., Fehér, K., Palma, A. S., Liu, Y., Simpson, J. C., et al. (2008). Malectin: a novel carbohydrate-binding protein of the endoplasmic reticulum and a candidate player in the early steps of protein N-glycosylation. Mol. Biol. Cell 19, 3404–3414. doi: 10.1091/mbc.e08-04-0354
Schulze-Muth, P., Irmler, S., Schröder, G., and Schröder, J. (1996). Novel type of receptor-like protein kinase from a higher plant (Catharanthus roseus): cDNA, gene, intramolecular autophosphorylation, and identification of a threonine important for auto-and substrate phosphorylation. J. Biol. Chem. 271, 26684–26689. doi: 10.1074/jbc.271.43.26684
Shiu, S.-H., Karlowski, W. M., Pan, R., Tzeng, Y.-H., Mayer, K. F., and Li, W.-H. (2004). Comparative analysis of the receptor-like kinase family in Arabidopsis and rice. Plant Cell 16, 1220–1234. doi: 10.1105/tpc.020834
Stahl, Y., Grabowski, S., Bleckmann, A., Kühnemuth, R., Weidtkamp-Peters, S., Pinto, K. G., et al. (2013). Moderation of Arabidopsis root stemness by CLAVATA1 and Arabidopsis CRINKLY4 receptor kinase complexes. Curr. Biol. 23, 362–371. doi: 10.1016/j.cub.2013.01.045
Stegmann, M., Monaghan, J., Smakowska-Luzan, E., Rovenich, H., Lehner, A., Holton, N., et al. (2017). The receptor kinase FER is a RALF-regulated scaffold controlling plant immune signaling. Science 355, 287–289. doi: 10.1126/science.aal2541
Takeda, K., Qin, S.-Y., Matsumoto, N., and Yamamoto, K. (2014). Association of malectin with ribophorin I is crucial for attenuation of misfolded glycoprotein secretion. Biochem. Biophys. Res. Commun. 454, 436–440. doi: 10.1016/j.bbrc.2014.10.102
Van Der Does, D., Boutrot, F., Engelsdorf, T., Rhodes, J., Mckenna, J. F., Vernhettes, S., et al. (2017). The Arabidopsis leucine-rich repeat receptor kinase MIK2/LRR-KISS connects cell wall integrity sensing, root growth and response to abiotic and biotic stresses. PLoS Genet. 13, e1006832. doi: 10.1371/journal.pgen.1006832
Wang, L., Yang, T., Wang, B., Lin, Q., Zhu, S., Li, C., et al. (2020). RALF1-FERONIA complex affects splicing dynamics to modulate stress responses and growth in plants. Sci. Adv. 6, eaaz1622. doi: 10.1126/sciadv.aaz1622
Wang, Z. Q., Yu, T. F., Sun, G. Z., Zheng, J. C., Chen, J., Zhou, Y. B., et al. (2021). Genome-wide Analysis of the Catharanthus roseus RLK1-like in Soybean and GmCrRLK1L20 Responds to Drought and Salt Stresses. Front. Plant Sci. 12, 614909. doi: 10.3389/fpls.2021.614909
Xiao, Y., Stegmann, M., Han, Z., Defalco, T. A., Parys, K., Xu, L., et al. (2019). Mechanisms of RALF peptide perception by a heterotypic receptor complex. Nature 572, 270–274. doi: 10.1038/s41586-019-1409-7
Yang, Z., Xing, J., Wang, L., Liu, Y., Qu, J., Tan, Y., et al. (2020). Mutations of two FERONIA-like receptor genes enhance rice blast resistance without growth penalty. J. Exp. Bot. 71, 2112–2126. doi: 10.1093/jxb/erz541
Ye, Y., Ding, Y., Jiang, Q., Wang, F., Sun, J., and Zhu, C. (2017). The role of receptor-like protein kinases (RLKs) in abiotic stress response in plants. Plant Cell Rep. 36, 235–242. doi: 10.1007/s00299-016-2084-x
Yu, Y., Chakravorty, D., and Assmann, S. M. (2018). The G protein β-subunit, AGB1, interacts with FERONIA in RALF1-regulated stomatal movement. Plant Physiol. 176, 2426–2440. doi: 10.1104/pp.17.01277
Zhang, M. J., Zhang, X. S., and Gao, X.-Q. (2020a). ROS in the male–female interactions during pollination: function and regulation. Front. Plant Sci. 11, 177. doi: 10.3389/fpls.2020.00177
Zhang, X., Peng, H., Zhu, S., Xing, J., Li, X., Zhu, Z., et al. (2020b). Nematode-encoded RALF peptide mimics facilitate parasitism of plants through the FERONIA receptor kinase. Mol. Plant 13, 1434–1454. doi: 10.1016/j.molp.2020.08.014
Zhang, X., Yang, Z., Wu, D., and Yu, F. (2020c). RALF–FERONIA signaling: linking plant immune response with cell growth. Plant Commun. 1, 100084. doi: 10.1016/j.xplc.2020.100084
Keywords: FERONIA receptor kinase, CrRLK1L family, environmental stresses, NtCrRLK1L47, tobacco
Citation: Li X, Guo C, Wang Q, Li Z, Cai J, Wu D, Li Y, Yang A, Guo Y, Gao J, Wen L and Pu W (2022) Systematic Analysis of Tobacco CrRLK1L Family Genes and Functional Identification of NtCrRLK1L47 in Environmental Stresses. Front. Plant Sci. 13:838857. doi: 10.3389/fpls.2022.838857
Received: 18 December 2021; Accepted: 17 May 2022;
Published: 17 June 2022.
Edited by:
Luisa M. Sandalio, Spanish National Research Council (CSIC), SpainReviewed by:
Weibing Yang, Shanghai Institutes for Biological Sciences (CAS), ChinaDongping Lu, Chinese Academy of Sciences (CAS), China
Copyright © 2022 Li, Guo, Wang, Li, Cai, Wu, Li, Yang, Guo, Gao, Wen and Pu. This is an open-access article distributed under the terms of the Creative Commons Attribution License (CC BY). The use, distribution or reproduction in other forums is permitted, provided the original author(s) and the copyright owner(s) are credited and that the original publication in this journal is cited, in accordance with accepted academic practice. No use, distribution or reproduction is permitted which does not comply with these terms.
*Correspondence: Liuying Wen, d2VubGl1eWluZyYjeDAwMDQwO2NhYXMuY24=; Wenxuan Pu, cHV3eDA2MDUmI3gwMDA0MDtobmd5dG9iYWNjby5jb20=
†These authors have contributed equally to this work