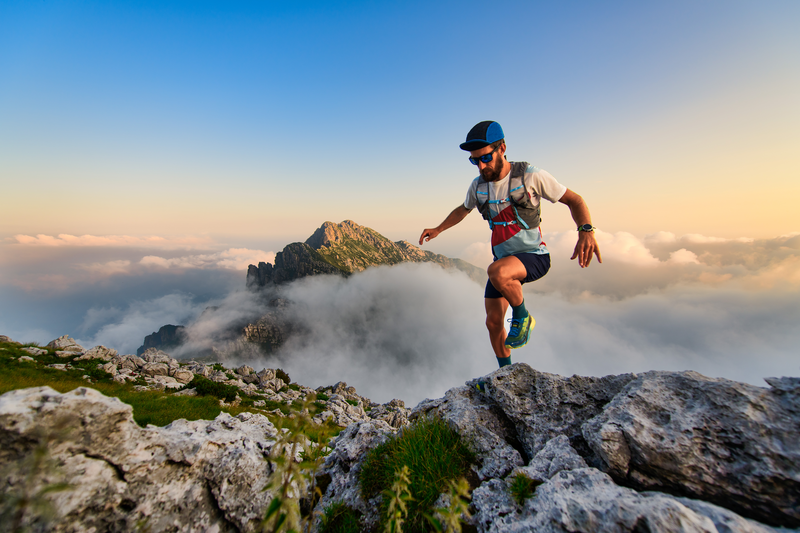
95% of researchers rate our articles as excellent or good
Learn more about the work of our research integrity team to safeguard the quality of each article we publish.
Find out more
REVIEW article
Front. Plant Sci. , 04 April 2022
Sec. Plant Physiology
Volume 13 - 2022 | https://doi.org/10.3389/fpls.2022.835921
This article is part of the Research Topic Plant Hydraulics Under Climate Change View all 10 articles
A correction has been applied to this article in:
Corrigendum: Unlocking drought-induced tree mortality: Physiological mechanisms to modelling
Drought-related tree mortality has become a major concern worldwide due to its pronounced negative impacts on the functioning and sustainability of forest ecosystems. However, our ability to identify the species that are most vulnerable to drought, and to pinpoint the spatial and temporal patterns of mortality events, is still limited. Model is useful tools to capture the dynamics of vegetation at spatiotemporal scales, yet contemporary land surface models (LSMs) are often incapable of predicting the response of vegetation to environmental perturbations with sufficient accuracy, especially under stressful conditions such as drought. Significant progress has been made regarding the physiological mechanisms underpinning plant drought response in the past decade, and plant hydraulic dysfunction has emerged as a key determinant for tree death due to water shortage. The identification of pivotal physiological events and relevant plant traits may facilitate forecasting tree mortality through a mechanistic approach, with improved precision. In this review, we (1) summarize current understanding of physiological mechanisms leading to tree death, (2) describe the functionality of key hydraulic traits that are involved in the process of hydraulic dysfunction, and (3) outline their roles in improving the representation of hydraulic function in LSMs. We urge potential future research on detailed hydraulic processes under drought, pinpointing corresponding functional traits, as well as understanding traits variation across and within species, for a better representation of drought-induced tree mortality in models.
The survival of forests around the globe is increasingly threatened by climatic extremes (Brodribb et al., 2020; Bennett et al., 2021; Gora and Esquivel-Muelbert, 2021). Massive forest dieback induced by climate change type drought has been recorded on every vegetated land within the past few decades (Adams et al., 2009; Allen et al., 2010, 2015). These events will become more common given that climate projections indicate that future drought episodes will be typified by increased frequency, severity, and duration (Adams et al., 2009; Carnicer et al., 2011; Dai, 2013). Forests cover approximately 30% of the land surface area and provide numerous crucial ecological, economic and social benefits. Globally, forests store ca. 45% of the carbon in terrestrial ecosystems and sequester approximately 25% of annual anthropogenic carbon emissions each year (Bonan, 2008; Pan et al., 2011). In addition, forests can regulate the terrestrial energy budget and hydrological cycle by modifying surface albedo and evapotranspiration, which exert strong control over global carbon cycle. Forest mortality can therefore significantly influence ecosystem structure and function, resulting in cascading negative effects on biochemical and biophysical cycles, consequently generating a positive feedback to the climate (Adams et al., 2010; Anderegg et al., 2013).
Elucidating the spatial-temporal pattern of imminent mortality events is essential for ameliorating the detrimental consequences of tree death triggered by drought. Land surface models (LSMs) with mechanistic representations of vegetation and soil processes are an efficacious tool for predicting future plant dynamics (Geary et al., 2020). These models are capable of simulating growth, mortality, and reproduction of vegetation and are often coupled with climate models to predict the dynamics of biosphere-atmosphere interactions at large scales (Fisher et al., 2014). Early LSMs often poorly performed when simulating tree mortality events caused by drought, as evidenced by the existence of offsets between model outcomes and field observations (McDowell et al., 2013; Bugmann et al., 2019; Thrippleton et al., 2021). While subsequent progress in physiology research has led to updated model structure and components, thereby enabling better predictions of ecosystem fluxes as well as vegetation dynamics under drought with improved accuracy (De Kauwe et al., 2020; Sabot et al., 2020; Wang et al., 2021), predicting drought-related tree mortality remains a significant shortcoming of current LSMs (Trugman, 2021).
More accurate simulation of drought-induced tree mortality events requires detailed knowledge of the mechanisms through which drought affects plant physiology and the cascading effects on plant carbon and water status. Empirical models exist, but they often lack theoretical underpinnings; thus their predictive power for novel conditions or subjects is limited. Process-based models can overcome these weaknesses by representing biological processes in detail. Current experimental evidence points to the crucial role of plant hydraulic traits in explaining the pattern of drought-induced tree mortality (Anderegg et al., 2016; Zhu et al., 2018; Chen et al., 2019, 2021a; Powers et al., 2020; Nolan et al., 2021), which subsequently motivated the development and integration of plant hydraulic modules into LSMs (De Kauwe et al., 2020; Eller et al., 2020; Sabot et al., 2020; López et al., 2021). Nonetheless, physiological processes driving hydraulic dysfunction are incompletely integrated into these models, mainly because of knowledge gaps surrounding the physiological mechanisms of drought response, as well as inadequate empirical datasets enabling adequate model parameterization (Meir et al., 2015; Hartmann et al., 2018). Hence, modelers must employ largely unvalidated assumptions such as leaf phenology when drought strikes, lethal threshold during drought or post-drought recovery of hydraulic function, which consequently produce unrealistic outcomes (McDowell et al., 2013; Xu et al., 2016; De Kauwe et al., 2020), e.g., model suggests that trees in South-East Australia forests did not approach lethal water potential (De Kauwe et al., 2020), despite large-scale forest mortality was substantiated by field observation (Nolan et al., 2021).
In light of the modeling need, this review attempts to provide a brief overview of the current understanding of mechanisms leading to tree mortality under drought stress, with particular emphasis on carbon and hydraulic functions. Next, the development of hydraulic failure under drought stress, together with current understanding about key traits modulating this process, is outlined and discussed. We suggest that incorporating these hydraulic traits would improve the predictability in terms of mortality events during drought of process-based models. Finally, coordination among traits is discussed in the context of facilitating model parameterization. Here, we emphasize that this review does not attempt to provide a comprehensive summary of the gaps between data and models; such a summary must be interdisciplinary and requires iterative collaborations between modelers and experimentalists. Rather, our aim is to facilitate such an advancement by providing a physiological interpretation of the merit of functional traits, especially hydraulic traits involved in drought tolerance, to improve the predictive power of vegetation models under climate change. Finally, the matter of scale is a crucial but challenging consideration when incorporating plant hydraulic traits to predict drought-induced mortality in LSMs. While tree death at the individual level can be quantitatively determined via better representation of plant physiology, mortality events at the larger scales (e.g., population and landscape) are much more complex, involving interactions among individuals within and across species, heterogeneous plant-environment feedback across the landscape, and stochasticity to account for other co-variates that are difficult to capture in a typical LSM (e.g., insect and disease effects). Given that our overall objective is to frame a pathway linking plant hydraulic trait with improved predictive power in the models, the current review will focus exclusively on attributes at organ or canopy scale, while characteristics at other spatial scales are beyond the scope of discussion.
Four decades ago, Manion (1981) proposed a slow decline hypothesis to explain the progress of tree mortality. In this theory, tree death begins with a moderate long-term stress that predisposes trees to mortality risk, which is intensified by a short-term severe stress, and death eventually occurs due to a contributing factor. Early modeling often builds on this original framework, with particular emphasis being placed on the role of carbon balance in tree mortality (McDowell et al., 2011; Sevanto and Xu, 2016). Based on the many physiological processes documented in trees approaching death under drought stress, McDowell et al. (2008) put forward two physiological hypotheses that explain and generalize mechanisms underlying this phenomenon. The carbon starvation (CS) hypothesis suggests that downregulated stomatal conductance during chronic, moderate drought stress will lead to reduced carbon assimilation. As plants still require photosynthate to fuel metabolic processes such as respiration and osmoregulation, limited carbon supply, and the continued demand for it will eventually deplete plant carbon storage (e.g., non-structural carbohydrates, NSCs), which in turn causing tree death due to carbon starvation. Alternatively, the hydraulic failure (HF) hypothesis suggests that water transport failure-induced desiccation is the primary cause of tree mortality under drought stress. According to the air-seeding hypothesis (Zimmermann, 1983), when xylem water transport is operating under greater tension during acute drought due to high evaporative demand and/or decreased soil water availability, air will be aspirated from air-filled vessels into adjacent water-filled, functional vessels through inter-conduit pits, forming air bubbles, and consequently creating emboli that break the continuity of water transport in xylem. Embolized conduits are unable to transport water to distal organs and are unlikely to recover over short periods (Brodribb et al., 2010; Choat et al., 2015; Li et al., 2018b; Choat et al., 2019), eventually leading to desiccation and death at multiple organizational levels (i.e., cell, tissue, organ, and whole plant). It is worth noting that hydraulic failure does not exclusively occur in the vascular system of plants, but can also arise at the plant-soil interface as the hydraulic pathway connecting roots and soil is interrupted by air (Sperry et al., 1998). However, mortality under drought is still finalized by hydraulic failure of vascular system in this scenario.
Given the intimate link between carbon and water metabolism, the binary theory of tree mortality mechanisms during drought stress is apparently oversimplified. In recognition of the interdependency between CS and HF, an integrated mechanism was further proposed (McDowell et al., 2011). In this updated theory, CS and HF are synergistic and interrelated through a “carbon-hydraulic feedback loop.” During drought stress, rates of carbon assimilation progressively decrease due to stomatal closure, consequently reducing the tissue non-structural carbohydrates content, which act as osmolytes facilitating water absorption by maintaining the water potential gradient between plant and soil. Inadequate water supply will result in increased xylem tension and degree of embolism, which diminishes hydraulic conductivity and leads to intensified physiological water deficit. This will ultimately feedback to leaves, causing enhanced stomatal closure or leaf shedding, which in turn exacerbates the NSCs deficiency. This integrated framework is generally supported, as both shifted tissue NSCs and xylem conductivity are often observed concomitantly in plants killed by drought (Adams et al., 2017). Therefore, pure CS or HF may not exist. Rather, CS and HF are likely the two endpoints of the “mortality mechanism spectrum”. However, it is still necessary to identify the primary contributing factors for drought-induced tree mortality given that models require quantitative information on the tipping point where recovery is impossible, so as to accurately predict the dynamics of tree mortality under different environmental conditions. Incorporating both mechanisms will likely add extra complexity to computation without much improvement of predictive power.
Carbohydrate may be involved in the process of drought-related tree mortality through its effects on plant hydraulics, particularly by lowering the risk of xylem embolism. In support of this theoretical expectation, a strong relationship between the percentage loss of xylem conductivity (PLC) and the extent of NSC depletion has been observed (Trifilò et al., 2017; Tomasella et al., 2019). It has long been acknowledged that NSCs contribute to osmotic regulation, which may lower the risk of hydraulic failure by increasing plant water uptake (De Roo et al., 2020). For example, by manipulating tissue NSCs, O’Brien et al. (2014) showed that trees fed by exogenous NSCs exhibited higher survival rate during drought stress. Trees with enriched NSCs typically exhibited less negative water potential, which in turn reduced PLC, assuming xylem vulnerability to embolism was not shifted by the treatment. Alternatively, NSCs may lower the risk of xylem embolism by restraining the movement of air bubbles within the vascular system under negative pressure (De Baerdemaeker et al., 2017). It has been suggested that several carbon-based organic compounds, such as choline, amphiphilic lipids, or proteins, may act as surfactants that increase the stability of nanobubbles against increased tensile force, thereby lowering xylem vulnerability to embolism (Schenk et al., 2017). In addition, NSCs have been suggested to facilitate the repair of embolism by generating osmotic force that helps draw water from the reservoir to air-filled conduits (Liu et al., 2019) or change the embolism resistance by mediating the structure of vessels during the ontogenesis of xylem. Note that both NSC-mediated xylem refilling, and structural change requires sufficient time to occur and thus may not alter the fate for trees exposed to short-term pules of lethal drought.
The interplay between CS and HF is intricate, hence validating these mechanisms can be complex. In many studies, partial or complete loss of hydraulic conductivity in dead trees has been observed with little or no change in tissue carbohydrate concentration. A multi-species synthesis shows trees that died from drought typically exhibit more than 60% embolism with various degrees of carbon depletion, indicating hydraulic failure is a universal phenomenon for trees exposed to lethal drought stress (Adams et al., 2017). The role of plant hydraulics in tree death under drought is further supported by studies showing correlative relationships between hydraulic traits related to embolism resistance and mortality patterns at both regional and global scales (Anderegg et al., 2016; Li et al., 2018a; Nolan et al., 2021; Chen et al., 2021a). In comparison, experimental evidence supporting carbon starvation as the main mechanism of mortality is relatively scarce. In Sevanto et al. (2014), tissue carbohydrate content decreased by nearly 70% in dead Pinus edulis treated with light exclusion, while both water potential and xylem conductivity were comparable to non-stressed controls. These results indicate that protracted carbon deficiency can generate tree death independent of hydraulic impairment. However, in the same study, trees killed by drought stress showed identical loss of xylem conductivity but different degrees of carbon depletion depending on the duration of drought exposure; hence, hydraulic failure remained the decisive factor generating tree mortality during drought stress.
Incorporating hydraulic failure into models requires its process to be elaborated. According to Choat et al. (2018), the development and progression of hydraulic failure under drought can be divided into two major phases (Figure 1A). During the initial phase (Phase I), drought stress develops as soil water availability declines due to decreased precipitation or depth to groundwater and can be exacerbated by an increase in the soil-atmosphere moisture gradient (i.e., vapor pressure deficit). Xylem tension becomes greater, as indicated by the continuously decreasing (i.e., more negative) maximum water potential (Ψ) of plants (e.g., predawn Ψ). Plants minimize water loss by reducing stomatal conductance until complete stomatal closure, either driven by the loss of turgor in guard cells and/or accumulation of cellular hormones (Buckley, 2019). It has been shown in many studies that the occurrence of complete stomatal closure precedes the inception of xylem embolism (Hochberg et al., 2017; Martin-StPaul et al., 2017; Creek et al., 2020). Therefore, the xylem water transport system remains largely intact during this stage, with no significant change in the hydraulic conductivity.
Figure 1. Key physiological processes following reductions in plant water potential as outlined by the biphasic framework of drought-related tree mortality (panel A). Physiological functions including stomatal conductance (gs, cyan), percentage loss of hydraulic conductivity in leaves (PLCLeaf, red) and stems (PLCStem, blue), as well as branch relative water content (RWC, orange) are shown as percentage of maximum. Vertical dashed line indicates the leaf turgor loss point (TLP). Lethal water potential thresholds (PLethal) for leaves and stems are indicated by red and blue circles. Transition from Phase I to Phase II occurs when stomata are fully closed, which theoretically coincides with the turgor loss (broken dashed line). Panel (B) shows the observed variation of these physiological processes from Eucalyptus sideroxylon during a dry-down experiment conducted in a common garden (Blackman et al., 2019), with shaded regions surrounding the lines denoting the 95% confidence interval of fitted curves. Similarity in the two panels indicate that the biphasic framework is generally supported by the experimental evidence. Note that TLP in panel (B) occurred prior to complete stomatal closure, and leaf shedding was initiated when leaf xylem was completely embolized, indicating these traits may not be robust for predicting the timing of these physiological adjustments (see text for detail).
As drought stress persists, plant water potential slowly decreases. Roots can no longer extract water from soil due to xylem cavitation or disconnection between roots and soil, leading to decoupled water potentials between soil and plants. Once stomata are fully closed, water leaves the plant mainly through the leaf cuticle, leaky stomata, or other tissues, although with markedly reduced rates (Phase II; Duursma et al., 2019). Water will be extracted from intercellular spaces, apoplast, or living tissues (i.e., hydraulic capacitance), to meet the evaporation demand and maintain cellular water status. In some species, water loss during this stage can be further prevented by leaf shedding (Pivovaroff et al., 2014; Levionnois et al., 2020). If drought remains unalleviated, xylem water potential will ultimately exceed the threshold of embolism. Air bubbles rapidly propagate and spread within the vascular system until all vessels are embolized, with the spatial pattern of propagation depending on the vessel arrangement and connectivity (Johnson et al., 2020; Wason et al., 2021). Complete loss of water transport capacity results in desiccation of living tissue and cellular death, including meristematic cells that govern post-drought resilience, finally resulting in whole tree mortality (Li et al., 2016; Mantova et al., 2021).
The timing of inception and progression of hydraulic failure can be complicated given it may be driven by impacts associated with plant morphology, biochemistry, and physiology. Nonetheless, the biphasic hydraulic failure framework offers a simplified yet mechanistic approach for locating key traits involved in this process given that this framework is generally supported by empirical evidence (Figure 1B). Below, we list a few key aspects and corresponding traits with a brief discussion about their significance regarding plant drought response as well as current understanding and uncertainties. Of note, one implicit assumption for the development of xylem embolism during this phase is that plants and soil have become hydraulically disconnected prior to the occurrence of embolism in leaves and stems. This assumption is supported by some early studies showing that roots are often more vulnerable to embolism than stems or leaves (Johnson et al., 2016; Wason et al., 2018). However, some recent findings suggest that roots are comparably or even more tolerant to drought-induced embolism compared with other organs in some species (Rodriguez-Dominguez et al., 2018; Peters et al., 2020; Lübbe et al., 2021). Clearly, more studies are required to confirm the spatial pattern of vulnerability to embolism within plants, particularly within the root system and rhizosphere.
Rooting depth delimits the root zone extension of plants in the vertical direction and largely determines plant water acquisition capacity and the availability of water resources to plants. Therefore, rooting depth can significantly affect the response and resilience of plants to drought stress (Canadell et al., 1996), and has long been explicitly considered in most vegetation models, albeit using relatively simplistic mathematical representations (Zeng, 2001). Intuitively, species with deeper rooting systems have a greater chance to capitalize on relatively stable water resources such as deep soil water or groundwater, so there will be less risk of experiencing negative water potential during protracted drought stress. This suggests that the rooting depth trait can be informative in predicting species-specific mortality vulnerability, especially for co-occurring species or individuals showing contrasting drought response (Johnson et al., 2018b; Li et al., 2018b; Jiang et al., 2020). Interestingly, in a study conducted by Wu et al. (2018), the authors noted that the legacy effects of drought, as represented by the offsets between observed growth and predicted growth, persisted longer in deep-rooted trees compared with shallow-rooted functional types such as grasses or shrubs, indicating that deeper roots may compromise drought resilience, therefore questioning the role of deeper roots in facilitating overall fitness. Very few studies have related rooting depth to observed whole plant drought response, mainly due to lack of relevant information regarding this trait. It is well known that plant rooting depth is controlled by many climatic, edaphic, hydrological, and biological factors (Canadell et al., 1996; Fan et al., 2017). However, due to the great difficulty in studying roots, especially roots in the deep soil layer, and the high dynamics of root growth, information regarding rooting depth and its environmental drivers is still very scarce for many species, limiting our ability to better constrain the models. In a synthesis of 2,200 root observations for more than 1,000 species around the globe, Fan et al. (2017) established a hydrologic framework to interpret the spatial variation of plant rooting depth and demonstrated that soil hydrology is a globally common force driving rooting depth patterns from the landscape to global scale. This provides a useful approach to predict plant rooting depth based on the long-term characteristic of soil water profile, which is determined by both precipitation and groundwater level. Yet, the relationship between rooting depth and plant water absorption could become decoupled as the deep soil layer desiccates or the groundwater level declines beyond the capture extent of roots (Xi et al., 2018). Therefore, identifying the environmental and biological drivers for the variation of rooting depth warrants further study.
Strictly speaking, stomatal regulation during drought stress is not a trait, although it can be defined by quantitative metrics, which are the dynamics of stomatal conductance (gs) in response to organ water status (i.e., leaf Ψ; Figure 1, cyan line). However, several key traits can be derived from this response curve and can encompass important implications to model drought-induced tree mortality (Klein, 2014; Blackman et al., 2019; Chen et al., 2019; De Kauwe et al., 2020). Current studies commonly measure gs as a function of leaf Ψ, with the sensitivity of gs to drought being quantified by the Ψ at the inception of complete stomatal closure (Pgs). Theoretically, stomatal closure at less negative water potentials during drought stress would significantly prevent water loss, which in turn reduces the risk of xylem embolism. However, given that stomatal closure also prevents the entry of CO2 for carbon assimilation, closing stomata at the expense of carbon gain in the absence of embolism risk will negatively impact the plant and increase the probability of carbon starvation (McDowell et al., 2008). Therefore, water potential at stomatal closure per se would be less informative about overall species drought tolerance (Garcia-Forner et al., 2016; Martínez-Vilalta and Garcia-Forner, 2017). In a meta-analysis conducted by Martin-StPaul et al. (2017), the water potential triggering stomatal closure ranges between −1 MPa and approximately −4 MPa across a wide range of species; however, hydraulic safety is largely conferred by more negative water potential thresholds of xylem cavitation, especially for species in arid regions. Therefore, combining stomatal regulation with other traits can often yield metrics that are more informative to plant drought response. For example, by combining water potential thresholds at stomatal closure and xylem cavitation, Skelton et al. (2015) found that the risk of hydraulic failure can be well predicted by the safety margin defined by these two traits. Likewise, in the study of Chen et al. (2019), the percentage of tree survival was correlated with the safety margin based on the Pgs. Other typical examples include the index of desiccation time proposed by Blackman et al. (2016), which integrates stomatal regulation with many other traits potentially affecting plant drought response and shows great promise in estimating the duration from stomatal closure and mortality. Clearly, quantifiable information on hydraulic safety margin, together with stomatal regulation, is useful to develop better models, yet traits delineating stomatal regulation (e.g., Pgs) are available for only a few species. Given the difficulties in measuring the response of stomata to drought, extrapolating these values from proper proxies will be instrumental for model parameterization (see below).
Leaf minimum conductance (gmin) presumably becomes the main route for water leaving the plants, after complete stomatal closure during drought, and hence directly relates to the drought response strategy and survival of the plant (Duursma et al., 2019; Carignato et al., 2020; Lanning et al., 2020). Note that gmin characterizes the overall water loss rate after stomatal closure under field conditions; therefore, it can be higher than the conductance specifically measured for the adaxial side of leaves (i.e., cuticular conductance, gcut) and is lower than the conductance value measured from leaves that are dark-adapted or with minimal photosynthetic rate (Duursma et al., 2019). Lower gmin should confer longer times for dehydration, but studies testing the functional significance of gmin in the process of drought-related tree mortality are scare. In an experiment designed to test the model described above, Blackman et al. (2019) found no correlation between observed gmin and observed time to desiccation. However, this does not mean that gmin plays no role in controlling water loss during dehydration. Instead, water loss during Phase II might be controlled by the conductance of other tissues such as bark. In support, Gleason et al. (2014) showed that desiccation time was significantly correlated with lower rates of transpiration in detached branches, which was the sum of water loss from both leaves and bark. It is also possible that the lack of a relationship was masked by the plasticity of gmin. It has been shown that gmin is highly responsive to various environmental stimuli, including temperature and water availability (Schuster et al., 2017; Duursma et al., 2019). In Blackman et al. (2019), gmin may have acclimated to the environment in the common garden, showing only 2-fold variation despite the distinctly different times to mortality across species. More generalizable information regarding the variation in gmin is required to facilitate model advancement.
Relative water content (RWC) is the classic metric describing the water status of plants. The amount of water in cells governs cell turgor, the maintenance of which is essential for many cell functions. With respect to drought stress, water content in plants directly determines the duration of drought stress that plants can sustain, especially in Phase II when exogenous water supply is no longer available (Blackman et al., 2019). Furthermore, RWC is more than a simple indicator of cell hydration state. Under drought stress, plants need to retain a minimum amount of water to prevent complete cell desiccation. At this stage, the water balance of plants is co-regulated by the capacity of water supply via the vascular system, as well as the capacity to retain water in the cell by osmotic regulation; the former depends on xylem embolism resistance, while the latter primarily relies on NSCs (Martinez-Vilalta et al., 2019). The variation is therefore thought to reflect the integration of CS and HF. This concept is highly intriguing as it provides a tool for identifying mortality risk and also allows monitoring tree health at large scales given that RWC can be detected through remote sensing, thus enabling large-scale model evaluation (Konings et al., 2019). However, few studies have tested this concept to date. In Sapes et al. (2019), plant water content scaled linearly with PLC at both the organ and whole plant level in Pinus ponderosa, and water content could reflect NSCs content at a given soil water availability. Importantly, a threshold-like function was observed between population mortality risk and plant water content, suggesting the usefulness of this metric in ascertaining the probability of tree death under drought stress.
Plant hydraulic capacitance (Cp) refers to the water that can be extracted per unit change in water potential. The significance of water storage to whole plant water balance varies among species, and it has been suggested that Cp could contribute up to 50% of daily water loss from evapotranspiration (Pfautsch et al., 2015). During short-term drought, water stored in the apoplastic, intracellular capillary space or in living cells can be discharged, radially transported to xylem through ray parenchyma, thereby buffering the increasing tension of xylem sap to limit the development of embolism (Richards et al., 2014). In line with this suggestion, a negative correlation between Cp and the steepness of the xylem vulnerability curve, as represented by the slope of the rapidly increasing PLC phase, has been observed across species (Meinzer et al., 2009). During later phases of drought stress, when Cp becomes the only water source once water can no longer be taken up from the soil, a larger Cp should therefore allow plants to live longer. However, experimental evidence supporting this hypothesis is rare and equivocal (Gleason et al., 2014; Blackman et al., 2019). The inconsistent observations among different studies might be attributed to the scale (branch vs. whole plant) or plasticity of the response. For example, there are reports that the Cp of roots contains a comparable amount of extractable water as stems in some species, and Cp is also reportedly responsive to environmental moisture (Salomón et al., 2020). In addition, it is also possible that the water provided by Cp is not utilized to prevent xylem embolism but to sustain the vitality of other tissues such as the cambium (Knipfer et al., 2017, 2019). Nevertheless, Cp is a measurable trait that can potentially buffer the impact of drought on plant hydraulic status and therefore warrants further studies.
Plant water loss primarily occurs through leaves; therefore, adjustments in canopy leaf area have significant influence on water balance of plants. Drought deciduous plants undergo annual cycles of leaf shedding and flushing in response to regular seasonal drought; this phenological strategy allows deciduous trees to avoid water stress associated with the dry season. However, even in evergreen species prolonged drought stress can trigger leaf shedding (Figure 1, dashed yellow line). This phenomenon, commonly termed as hydraulic segmentation, is hypothesized to reduce the risk of xylem embolism given that water loss can be significantly minimized once leaves have been hydraulically isolated from the plant. Studies of hydraulic segmentation commonly focus on revealing its underlying mechanisms by identifying differences in vulnerability to embolism or hydraulic resistance across organs (Pivovaroff et al., 2014; Levionnois et al., 2020). It has been shown that embolism resistance or hydraulic resistance varies across organs in some species, but not in others, indicating that leaf shedding is not a universal strategy (Zhu et al., 2018). On the other hand, the effects of leaf shedding on plant water status are less well understood. Incorporating a leaf shedding function into models significantly increased the time to mortality and reduced the offset between predicted and observed values, suggesting that leaf area adjustment does facilitate water retention during drought stress (Blackman et al., 2019). However, the functional significance of this strategy may be debatable for some species exhibiting leaf shedding during drought stress. For instance, Wolfe et al. (2016) reported that the occurrence of leaf shedding coincided with a 50% loss of maximum xylem conductivity. Of note, one of the studied species, Genipa americana, showed continuously decreasing water potential after leaf shedding, indicating that leaf area adjustments may not always stabilize plant water status, if water loss with continuous drying is dominated by other organs. Similarly, in the study of Blackman et al. (2019), leaf shedding was initiated at water potentials triggering 50% loss of hydraulic conductivity in stems (P50), suggesting that reducing canopy leaf area may have limited capacity to prevent the occurrence of xylem embolism (Figure 2).
Figure 2. Conceptual diagram summarizing current understanding regarding the relationship among functional traits and illustrating the general approach for simulating of tree dynamics in response to water availability with hydraulic traits in process-based models. Light blue boxes indicate traits that are directly involved in the occurrence of hydraulic failure during drought stress but are not well represented in current TBMs, either due to lack of trait values or insufficient knowledge regarding the variation at spatial or temporal scales. Traits presented in the diagram are maximum rooting depth (RDmax), water potential of soil, root, stem, and leaf (Ψsoil, Ψroot, Ψstem, and Ψleaf, respectively), relative water content (RWC), hydraulic capacitance (Cp), minimum conductance (gmin), water potential threshold of stomata respond to drought (Pgs), hydraulic conductivity (K), percentage loss of hydraulic conductivity (PLC), stomatal conductance (gs), evaporation (E), leaf area index (LAI), leaf photosynthesis (A), non-structural carbohydrates (NSCs), lethal threshold (Plethal), gross (GPP), and net primary productivity (NPP).
Given that hydraulic failure occurs due to massive xylem cavitation, it is intuitive that there is a lethal threshold in xylem vulnerability that generates a fatal degree of embolism. By subjecting plants to drought and then a re-watering treatment, Brodribb and Cochard (2009) showed that once the xylem of several gymnosperms lost 50% of the maximum hydraulic conductivity, recovery did not occur upon re-watering. Based on this finding, they suggested that the P50 of stems can be used as the lethal water potential threshold (Plethal) for this functional group. Using the same approach, Urli et al. (2013) found that the Plethal in angiosperms corresponds to the water potential threshold triggering 88–100% loss of xylem hydraulic conductivity (P88). These thresholds are generally valid for whole plants and organs such as leaves (Blackman et al., 2009; Figure 1, Plethal). However, uncertainty remains regarding the commonality of these water potential thresholds. Common approaches for determining Plethal are often time-consuming; therefore, these threshold water potentials are derived from limited species. By compiling existing Plethal data, Liang et al. (2021) recently showed that stem P50 and P88 explained 75 and 43% of the variation in Plethal across gymnosperm and angiosperm species, respectively. In particular, lethal water potentials of some angiosperm species are much lower than P88, as have been observed by Li et al. (2016). Tree death occurs under drought because living cells are dehydrated and therefore, trees are unable to regain metabolic function by reconstructing damaged tissues even after drought stress has been alleviated. Given the observed discrepancy between embolism threshold and Plethal, it is likely that drought tolerance of some species can be organization level-specific, such that cell vitality can persist longer than the integrity of vascular water transport under drought stress. Therefore, assigning Plethal based on cellular hydraulic failure represents a more mechanistic and robust approach, yet the vulnerability of cell vitality to dehydration remains unclear.
Providing a mechanistic underpinning to better simulate drought mortality in trees is not an easy endeavor, as described above, but data-driven evidence is emerging and has led to an increasing momentum toward incorporating plant hydraulic traits in LSMs. Previously, predictive models often relied on empirical relationships to introduce soil moisture stress on plants and to induce the subsequent tree death (De Kauwe et al., 2015; Medlyn et al., 2015, 2016; Christoffersen et al., 2016; Trugman et al., 2018). Clearly this approach is not very applicable for novel conditions, which often change rapidly over space and time with global climate change. The abovementioned hydraulic traits and the associated ecophysiological processes offer a framework through which a more mechanistic simulation of drought mortality can be realized.
Indeed, many LSMs have incorporated some of these hydraulic traits and processes into their modeling framework. These models are often conceptually similar, i.e., plant hydraulics exert control on growth through leaf gas exchange, and tree mortality occurs once Plethal is approached (Figure 2) but can differ in model structure and parameter. McDowell et al. (2013) evaluated six models of different scales using a data-model inter-comparison and highlighted that the capacity to simulate internal hydraulic and carbohydrate dynamics is crucial to capture observed tree mortality in the models. More recent modeling exercises showed a similar role for plant hydraulics. For example, De Kauwe et al. (2015) replaced the empirical scalar parameter (i.e., beta) for drought stress with a new expression for drought sensitivity of gas exchange that depends on leaf water potentials (i.e., Zhou et al., 2013, 2014) in the CABLE model. This new model feature led to improved simulation of gross primary production during the drought period, especially at the more xeric sites along a rainfall gradient (De Kauwe et al., 2015). Similarly, Xu et al. (2016) introduced a trait-driven plant hydraulic module into the ED2 model, which led to more realistic predictions of plant hydraulic dynamics, such as leaf water potential and stem sap-flow and better spatial predictions of leaf area index. Kennedy et al. (2019) implemented a plant hydraulic stress configuration to the Community Land Model (CLM5) that better connects stomatal conductance to water stress via the influence of vegetation water potential, allowing hydraulic redistribution and compensatory root water uptake to buffer shortfalls in rainfall (Kennedy et al., 2019). All these abovementioned model developments regarding plant hydraulics could have significant implications for the simulated land-climate feedback, thereby providing crucial benefits to better anticipate cascading climate change consequences. Nonetheless, few LSMs have explicitly integrated current state-of-art knowledge regarding the development of hydraulic dysfunction into modeling due to existing data uncertainties for many traits mentioned above. We suggest that advancing our mechanistic understanding and building sufficient datasets, that allow traits variation at spatial or temporal scales to be predicted, will help to reduce these uncertainties (Table 1). Overall, this will facilitate data-model integration and improve the representation of drought-induced tree mortality in LSMs.
Table 1. Key summary of plant hydraulic traits and/or variables and their model integration recommendations.
A critical pre-requisite of process-based modeling is the information regarding traits values and the pattern of variation. Global databases of functional traits are becoming increasingly available but are often constrained to limited trait types and species (Kattge et al., 2020). This poses a major obstacle to its application, especially when predicting drought-induced tree mortality, the occurrence of which is likely ubiquitous across diverse tree species and is often a function of various traits (Blackman et al., 2016; Choat et al., 2018; Blackman et al., 2019; Chen et al., 2021a). With regard to plant hydraulics, many of the fundamental traits engaged in the process of hydraulic failure are only available for a few hundred species (Bartlett et al., 2012; Choat et al., 2012; Klein, 2014; Bartlett et al., 2016), which accounts for a trivial portion of the vast plant taxa. One important reason for the lack of sufficient data is that traits representing plant hydraulics are usually difficult to measure. Assessments of key functional traits (e.g., thresholds for stomatal closure, xylem embolism, or tree death) are often time- and labor-consuming, and can often be hard to realize under field conditions. Although new techniques are emerging to address these issues (Gauthey et al., 2020; Chen et al., 2021b), building substantial datasets still requires considerable time.
In plants, many functional traits are mathematically correlated across species. Positive correlation can arise because of shared ancestry, functional convergence, or subjected to co-selection, while negative correlative relationships can result from functional or structural trade-offs (Wright et al., 2007; Reich, 2014; Bartlett et al., 2016). Such correlative relationships not only provide insights into many key ecological questions, such as principles governing community assembly or species coexistence, but also offer the opportunity to rapidly gain information regarding traits variation across diverse species. Using easily measured traits (i.e., soft traits), as proxies of traits that are difficult to measure (i.e., hard traits), has become a common approach in traits-based ecology, especially when TBMs are parameterized (Rogers et al., 2017). Importantly, these relationships often reflect mechanistic implications rather than demonstrated causality (Reich et al., 1998).
With respect to plant water relations, coordination and trade-offs have been found among various hydraulic or structural traits. Among others, woody density (WD), which is thought to integrate a suite of plant functions, has been shown to be a strong predictor of xylem vulnerability to embolism (Markesteijn et al., 2011; Li et al., 2018a; Liang et al., 2021). Furthermore, wood density is correlated with sapwood hydraulic capacitance, which may facilitate both drought resistance and resilience (Li et al., 2018a; Santiago et al., 2018). It has been observed that species with high wood density are more resistant to drought-induced xylem embolism. Mechanisms underpinning this observation may be due to reinforced vessel walls, which can resist xylem implosion and therefore cavitation (Hacke et al., 2001), although collapse of xylem vessels is seldomly reported in stems. The correlation between WD and xylem embolism resistance has been adopted by some models. For instance, in the work of Xu et al. (2016), WD was used to predict the water potential threshold of xylem embolism, and the latter was then used to parameterize the hydraulic module of ED2. However, caution should be taken when extrapolating this correlation to assess mortality risk for field-grown trees given that the two traits are not always related (Figure 3A). For example, some species characterized by high WD tend to exhibit a higher mortality ratio during drought stress (Hoffmann et al., 2011). One possible reason is that WD is not consistently correlated with embolism resistance. Indeed, high WD can be supported by features that are unrelated to plant hydraulics, such as fiber traits (Russo et al., 2010). Additionally, the occurrence of hydraulic failure, as discussed above, is a function of multiple traits. A species characterized by embolism-resistant xylem can still die rapidly during drought stress due to a profligate water regulation strategy or water acquisition ability (Hoffmann et al., 2011; Fu et al., 2019).
Figure 3. The relationships between stem water potential at 50% loss of xylem hydraulic conductivity (P50) and two easily measured traits, sapwood density (WD, panel A), and leaf turgor loss point (TLP, panel B), at global scale. P50 data were obtained from Choat et al. (2012). Data for WD were sourced from Zanne et al. (2009), and TLP data were compiled from Bartlett et al. (2012) and Zhu et al. (2018). Regression formula for panel (B) y = 1.34x + 0.25 (R2 = 0.22, p < 0.001).
Another valuable trait is leaf water potential at turgor loss point (TLP), which has been used to assess drought tolerance in plant physiology for decades (Bartlett et al., 2012). TLP is often correlated with water potential thresholds triggering stomatal closure and xylem embolism (Figure 3B), either because of mechanistic linkages given that stomatal closure is structurally driven by the turgor loss of guard cells, or functional linkages as less resistance xylem typically requires early stomatal closure to minimize water loss (Buckley, 2019). Recent findings also suggest that TLP integrates a wide range of hydraulic traits and thereby describes the overall water use strategy of plants (Meinzer et al., 2016; Fu et al., 2019; Li et al., 2019). Furthermore, it has been shown that TLP can also be correlated with economic traits such as specific leaf area, photosynthetic rate, or leaf longevity, although the correlations can be much weaker (Zhu et al., 2018), suggesting that diverse aspects of plant strategy can probably converge to a simple trait (Reich, 2014). Indeed, several meta-analysis studies have shown that TLP varies systematically with site water availability within and across biomes, indicating that TLP does provide some adaptive advantages (Bartlett et al., 2012; Zhu et al., 2018). However, the correlation between TLP and traits such as xylem embolism threshold is still surprising as TLP is known to be highly plastic (Farrell et al., 2017; Johnson et al., 2018a), while embolism threshold is more conservative within and across species (Lamy et al., 2014; Li et al., 2018a). Again, one should be cautious when using TLP as a surrogate given that it can easily become decoupled from other hydraulic traits due to growth conditions. Overall, soft traits like WD or TLP provide a rapid tool for generating other traits essential for model parameterization, as well as assessing plant strategy for a considerable number of species. Nonetheless, “true” values of hard traits are warranted to increase the precision of model outcomes.
The elusive nature of physiological mechanisms underpinning drought-induced tree mortality suggest that detailed biological processes and their interactions from the beginning of drought response to complete desiccation can be difficult to model. Nonetheless, the identification of hydraulic dysfunction as a major driver of tree death during drought stress, together with the biphasic framework depicting the development of xylem embolism, allows us to locate key functional traits involved in this complicated phenomenon and to predict its occurrence using a mechanistic approach. To better represent hydraulic failure in process-based models, we suggest that the following areas require future attention.
Firstly, physiological mechanisms for plant water regulation strategy during drought stress need to be better elucidated. Drought-induced tree death occurs when plant water potential approaches a critical threshold where xylem water transport is largely blocked by embolism. To prevent water potential from reaching this tipping point, plants would adopt diverse regulation strategies to maintain a reasonable water status. However, many of these strategies are not well represented in current models. For example, hydraulic segmentation has long been thought to mitigate the decline of water potential. Yet, the physiological basis of this phenomenon remains unclear. Hypotheses explaining leaf shedding during drought stress include differences in vulnerability to embolism or hydraulic resistance across organs, both of which are supported by experimental evidence, but can generate distinct ecological consequences. A similar example is xylem refilling, which still represents a research frontier in the field of plant hydraulics. Whether an embolized vessel can hydraulically recover after drought is still controversial. Several mechanisms have been proposed, but it remains unknown whether it is common in species, the nature of the circumstances leading to xylem refilling, and potential impacts on water transport. Identification of these physiological mechanisms is crucial because it determines how this strategy will be represented and parameterized in models.
Secondly, functional traits influencing water transport of plants need to be fully examined, especially for traits governing belowground hydraulic processes. Although data of key hydraulic traits such as vulnerability to embolism are rapidly accumulating, information regarding other functional traits affecting plant hydraulics is limited. With respect to aboveground water relations, traits, such as cuticular conductance, hydraulic capacitance, or water potential threshold for stomatal closure, are only available for a relatively small number of species compared with embolism thresholds. In addition, trait variation with the environment is also largely unknown. Likewise, belowground traits affecting water uptake and conductance are limited. Root functional traits that can be measured with relatively simple approaches should be the focus, such as maximum rooting depth, vertical root distribution, proportions of fine (absorption) and coarse (transport) roots, and water uptake vulnerability to soil desiccation. In addition, the variability of root traits with tree age should be determined to facilitate assessment of impacts in a dynamic way.
Patterns and sources of intraspecific trait variation at spatial and temporal scales require further investigation. The inter-specific variation of traits related to water regulation has been extensively studied and many of these traits vary systematically along an aridity gradient. However, the pattern of intraspecific trait variation is less clear, with the environmental drivers somewhat inconclusive. In some species, the variation of hydraulic traits among populations shares a similar pattern as variation across species, i.e., traits generally shift toward an increased drought tolerance as habitat water availability becomes limited. However, in other species, hydraulic traits can be highly conservative despite variation in environmental dryness. In addition, traits may also vary over time for a given population, but studies focusing on this aspect are infrequent. It has been shown that some hydraulic traits, including vulnerability to embolism or hydraulic capacitance, can shift seasonally or interannually (Guo et al., 2020; Sorek et al., 2021; Wu et al., 2021), leading to temporally altered overall water regulation strategy. This type of variation can be due to differences in ontogeny or shifts in environmental conditions over time. A useful framework for understanding observed differences in traits within species is to separate the observed difference into genotype variation and phenotypic plasticity and to unravel the source of variation using common garden experiments. If trait expression is governed by genetics, then we would expect small differences in targeted traits across populations, and we could apply a single trait value in models, regardless of the population-specific growth conditions. However, if phenotypic plasticity is dominant, then controlled environment experiments or transect studies in the field will be needed to uncover the environmental driver and then calculate the trait value as a function of environmental variables in models.
In conclusion, drought-related tree mortality is a ramification of complex interactions, consisting of multiple physiological processes associated with carbon and hydraulic dynamics. Recent advancements in plant physiology indicate the crucial role of hydraulic failure in determining tree mortality under drought, which offers a pathway for LSMs to represent mortality events with a simplified, but efficacious approach. In particular, the biphasic framework describing the development of hydraulic failure is generally supported by current experimental evidence and is therefore instrumental in locating key traits. The incorporation of these key traits will have the potential to boost the predictability of tree mortality under drought, without introducing much complexity to the algorithms and burdening the computational capacity. Nonetheless, many hydraulic processes within this framework are not mechanistically understood or remain controversial, which represents the priority of future research in the field of plant hydraulics. In addition, traits coordination and variation at different levels (e.g., intra- and inter-specific) and scales (i.e., spatial and temporal) also warrant further study, which would facilitate model parameterization by providing and constraining trait values.
XL drafted the early version of this manuscript, with inputs from BX on root hydraulics and MJ on ecosystem models. DT provided critical edits. All authors contributed to the article and approved the submitted version.
XL is financially supported by research initializing funding from Minzu University of China (nos. 0210040217 and 2020CXTD). BX is supported by the National Natural Science Foundation of China (no. 32171763). JF receives funds from the interdisciplinary research program of Minzu University of China (nos. 2020MDJC09 and 2021XSTD02). MJ acknowledges funding from the Australia Research Council (DE210101654).
The authors declare that the research was conducted in the absence of any commercial or financial relationships that could be construed as a potential conflict of interest.
All claims expressed in this article are solely those of the authors and do not necessarily represent those of their affiliated organizations, or those of the publisher, the editors and the reviewers. Any product that may be evaluated in this article, or claim that may be made by its manufacturer, is not guaranteed or endorsed by the publisher.
The authors appreciate the advice from Belinda Medlyn on the modeling section of this manuscript. This manuscript is adapted from the first chapter of XL’s PhD thesis at Western Sydney University. XL therefore thanks Peter Franks from University of Sydney and Patrick Meir from Australian National University, who were examiners of XL’s PhD thesis, for their valuable comments on the prototype of this paper.
Adams, H. D., Guardiola-Claramonte, M., Barron-Gafford, G. A., Villegas, J. C., Breshears, D. D., Zou, C. B., et al. (2009). Temperature sensitivity of drought-induced tree mortality portends increased regional die-off under global-change-type drought. Proc. Natl. Acad. Sci. U. S. A. 106, 7063–7066. doi: 10.1073/pnas.0901438106
Adams, H. D., Macalady, A. K., Breshears, D. D., Allen, C. D., Stephenson, N. L., Saleska, S. R., et al. (2010). Climate-induced tree mortality: earth system consequences. EOS Trans. Am. Geophys. Union 91, 153–154. doi: 10.1029/2010EO170003
Adams, H. D., Zeppel, M. J., Anderegg, W. R., Hartmann, H., Landhäusser, S. M., Tissue, D. T., et al. (2017). A multi-species synthesis of physiological mechanisms in drought-induced tree mortality. Nat. Ecol. Evol. 1, 1285–1291. doi: 10.1038/s41559-017-0248-x
Allen, C. D., Breshears, D. D., and Mcdowell, N. G. (2015). On underestimation of global vulnerability to tree mortality and forest die-off from hotter drought in the Anthropocene. Ecosphere 6, art129–art155. doi: 10.1890/ES15-00203.1
Allen, C. D., Macalady, A. K., Chenchouni, H., Bachelet, D., Mcdowell, N., Vennetier, M., et al. (2010). A global overview of drought and heat-induced tree mortality reveals emerging climate change risks for forests. For. Ecol. Manag. 259, 660–684. doi: 10.1016/j.foreco.2009.09.001
Anderegg, W. R., Kane, J. M., and Anderegg, L. D. (2013). Consequences of widespread tree mortality triggered by drought and temperature stress. Nat. Clim. Chang. 3, 30–36. doi: 10.1038/nclimate1635
Anderegg, W. R., Klein, T., Bartlett, M., Sack, L., Pellegrini, A. F., Choat, B., et al. (2016). Meta-analysis reveals that hydraulic traits explain cross-species patterns of drought-induced tree mortality across the globe. Proc. Natl. Acad. Sci. U. S. A. 113, 5024–5029. doi: 10.1073/pnas.1525678113
Bartlett, M. K., Klein, T., Jansen, S., Choat, B., and Sack, L. (2016). The correlations and sequence of plant stomatal, hydraulic, and wilting responses to drought. Proc. Natl. Acad. Sci. U. S. A. 113, 13098–13103. doi: 10.1073/pnas.1604088113
Bartlett, M. K., Scoffoni, C., and Sack, L. (2012). The determinants of leaf turgor loss point and prediction of drought tolerance of species and biomes: a global meta-analysis. Ecol. Lett. 15, 393–405. doi: 10.1111/j.1461-0248.2012.01751.x
Bennett, A. C., Dargie, G. C., Cuni-Sanchez, A., Mukendi, J. T., Hubau, W., Mukinzi, J. M., et al. (2021). Resistance of African tropical forests to an extreme climate anomaly. Proc. Natl. Acad. Sci. U. S. A. 118:e2003169118. doi: 10.1073/pnas.2003169118
Blackman, C. J., Brodribb, T. J., and Jordan, G. J. (2009). Leaf hydraulics and drought stress: response, recovery and survivorship in four woody temperate plant species. Plant Cell Environ. 32, 1584–1595. doi: 10.1111/j.1365-3040.2009.02023.x
Blackman, C. J., Li, X., Choat, B., Rymer, P. D., De Kauwe, M. G., Duursma, R. A., et al. (2019). Desiccation time during drought is highly predictable across species of Eucalyptus from contrasting climates. New Phytol. 224, 632–643. doi: 10.1111/nph.16042
Blackman, C. J., Pfautsch, S., Choat, B., Delzon, S., Gleason, S. M., and Duursma, R. A. (2016). Toward an index of desiccation time to tree mortality under drought. Plant Cell Environ. 39, 2342–2345. doi: 10.1111/pce.12758
Bonan, G. B. (2008). Forests and climate change: forcings, feedbacks, and the climate benefits of forests. Science 320, 1444–1449. doi: 10.1126/science.1155121
Brodribb, T. J., Bowman, D. J., Nichols, S., Delzon, S., and Burlett, R. (2010). Xylem function and growth rate interact to determine recovery rates after exposure to extreme water deficit. New Phytol. 188, 533–542. doi: 10.1111/j.1469-8137.2010.03393.x
Brodribb, T. J., and Cochard, H. (2009). Hydraulic failure defines the recovery and point of death in water-stressed conifers. Plant Physiol. 149, 575–584. doi: 10.1104/pp.108.129783
Brodribb, T. J., Powers, J., Cochard, H., and Choat, B. (2020). Hanging by a thread? Forests and drought. Science 368, 261–266. doi: 10.1126/science.aat7631
Buckley, T. N. (2019). How do stomata respond to water status? New Phytol. 224, 21–36. doi: 10.1111/nph.15899
Bugmann, H., Seidl, R., Hartig, F., Bohn, F., Brůna, J., Cailleret, M., et al. (2019). Tree mortality submodels drive simulated long-term forest dynamics: assessing 15 models from the stand to global scale. Ecosphere 10:e02616. doi: 10.1002/ecs2.2616
Canadell, J., Jackson, R., Ehleringer, J., Mooney, H., Sala, O., and Schulze, E. D. (1996). Maximum rooting depth of vegetation types at the global scale. Oecologia 108, 583–595. doi: 10.1007/BF00329030
Carignato, A., Vázquez-Piqué, J., Tapias, R., Ruiz, F., and Fernández, M. (2020). Variability and plasticity in cuticular transpiration and leaf permeability allow differentiation of Eucalyptus clones at an early age. Forests 11:9. doi: 10.3390/f11010009
Carnicer, J., Coll, M., Ninyerola, M., Pons, X., Sanchez, G., and Penuelas, J. (2011). Widespread crown condition decline, food web disruption, and amplified tree mortality with increased climate change-type drought. Proc. Natl. Acad. Sci. U. S. A. 108, 1474–1478. doi: 10.1073/pnas.1010070108
Chen, Y. J., Choat, B., Sterck, F., Maenpuen, P., Katabuchi, M., Zhang, S. B., et al. (2021a). Hydraulic prediction of drought-induced plant dieback and top-kill depends on leaf habit and growth form. Ecol. Lett. 24, 2350–2363. doi: 10.1111/ele.13856
Chen, Z., Li, S., Luan, J., Zhang, Y., Zhu, S., Wan, X., et al. (2019). Prediction of temperate broadleaf tree species mortality in arid limestone habitats with stomatal safety margins. Tree Physiol. 39, 1428–1437. doi: 10.1093/treephys/tpz045
Chen, Y. J., Maenpuen, P., Zhang, Y. J., Barai, K., Katabuchi, M., Gao, H., et al. (2021b). Quantifying vulnerability to embolism in tropical trees and lianas using five methods: can discrepancies be explained by xylem structural traits? New Phytol. 229, 805–819. doi: 10.1111/nph.16927
Choat, B., Brodersen, C. R., and McElrone, A. J. (2015). Synchrotron X-ray microtomography of xylem embolism in Sequoia sempervirens saplings during cycles of drought and recovery. New Phytol. 205, 1095–1105. doi: 10.1111/nph.13110
Choat, B., Brodribb, T. J., Brodersen, C. R., Duursma, R. A., López, R., and Medlyn, B. E. (2018). Triggers of tree mortality under drought. Nature 558, 531–539. doi: 10.1038/s41586-018-0240-x
Choat, B., Jansen, S., Brodribb, T. J., Cochard, H., Delzon, S., Bhaskar, R., et al. (2012). Global convergence in the vulnerability of forests to drought. Nature 491, 752–755. doi: 10.1038/nature11688
Choat, B., Nolf, M., Lopez, R., Peters, J. M., Carins-Murphy, M. R., Creek, D., et al. (2019). Non-invasive imaging shows no evidence of embolism repair after drought in tree species of two genera. Tree Physiol. 39, 113–121. doi: 10.1093/treephys/tpy093
Christoffersen, B. O., Gloor, M., Fauset, S., Fyllas, N. M., Galbraith, D. R., Baker, T. R., et al. (2016). Linking hydraulic traits to tropical forest function in a size-structured and trait-driven model (TFS v. 1-Hydro). Geosci. Model Dev. 9, 4227–4255. doi: 10.5194/gmd-9-4227-2016
Creek, D., Lamarque, L. J., Torres-Ruiz, J. M., Parise, C., Burlett, R., Tissue, D. T., et al. (2020). Xylem embolism in leaves does not occur with open stomata: evidence from direct observations using the optical visualization technique. J. Exp. Bot. 71, 1151–1159. doi: 10.1093/jxb/erz474
Dai, A. (2013). Increasing drought under global warming in observations and models. Nat. Clim. Chang. 3, 52–58. doi: 10.1038/nclimate1633
De Baerdemaeker, N. J., Salomón, R. L., De Roo, L., and Steppe, K. (2017). Sugars from woody tissue photosynthesis reduce xylem vulnerability to cavitation. New Phytol. 216, 720–727. doi: 10.1111/nph.14787
De Kauwe, M. G., Medlyn, B. E., Ukkola, A. M., Mu, M., Sabot, M. E., Pitman, A. J., et al. (2020). Identifying areas at risk of drought-induced tree mortality across South-Eastern Australia. Glob. Chang. Biol. 26, 5716–5733. doi: 10.1111/gcb.15215
De Kauwe, M. G., Zhou, S. X., Medlyn, B. E., Pitman, A. J., Wang, Y. P., Duursma, R. A., et al. (2015). Do land surface models need to include differential plant species responses to drought? Examining model predictions across a mesic-xeric gradient in Europe. Biogeosciences 12, 7503–7518. doi: 10.5194/bg-12-7503-2015
De Roo, L., Salomón, R. L., Oleksyn, J., and Steppe, K. (2020). Woody tissue photosynthesis delays drought stress in Populus tremula trees and maintains starch reserves in branch xylem tissues. New Phytol. 228, 70–81. doi: 10.1111/nph.16662
Duursma, R. A., Blackman, C. J., Lopéz, R., Martin-Stpaul, N. K., Cochard, H., and Medlyn, B. E. (2019). On the minimum leaf conductance: its role in models of plant water use, and ecological and environmental controls. New Phytol. 221, 693–705. doi: 10.1111/nph.15395
Eller, C. B., Rowland, L., Mencuccini, M., Rosas, T., Williams, K., Harper, A., et al. (2020). Stomatal optimization based on xylem hydraulics (SOX) improves land surface model simulation of vegetation responses to climate. New Phytol. 226, 1622–1637. doi: 10.1111/nph.16419
Fan, Y., Miguez-Macho, G., Jobbágy, E. G., Jackson, R. B., and Otero-Casal, C. (2017). Hydrologic regulation of plant rooting depth. Proc. Natl. Acad. Sci. U. S. A. 114, 10572–10577. doi: 10.1073/pnas.1712381114
Farrell, C., Szota, C., and Arndt, S. K. (2017). Does the turgor loss point characterize drought response in dryland plants? Plant Cell Environ. 40, 1500–1511. doi: 10.1111/pce.12948
Fisher, J. B., Huntzinger, D. N., Schwalm, C. R., and Sitch, S. (2014). Modeling the terrestrial biosphere. Annu. Rev. Environ. Resour. 39, 91–123. doi: 10.1146/annurev-environ-012913-093456
Fu, X., Meinzer, F. C., Woodruff, D. R., Liu, Y. Y., Smith, D. D., Mcculloh, K. A., et al. (2019). Coordination and trade-offs between leaf and stem hydraulic traits and stomatal regulation along a spectrum of isohydry to anisohydry. Plant Cell Environ. 42, 2245–2258. doi: 10.1111/pce.13543
Garcia-Forner, N., Adams, H. D., Sevanto, S., Collins, A. D., Dickman, L. T., Hudson, P. J., et al. (2016). Responses of two semiarid conifer tree species to reduced precipitation and warming reveal new perspectives for stomatal regulation. Plant Cell Environ. 39, 38–49. doi: 10.1111/pce.12588
Gauthey, A., Peters, J., Carins-Murphy, M., Rodriguez-Dominguez, C., Li, X., Delzon, S., et al. (2020). Evaluating methods used to measure cavitation resistance in seven woody species with differing xylem anatomy: a comparison of visual and hydraulic techniques. New Phytol. 228, 884–897. doi: 10.1111/nph.16746
Geary, W. L., Bode, M., Doherty, T. S., Fulton, E. A., Nimmo, D. G., Tulloch, A. I., et al. (2020). A guide to ecosystem models and their environmental applications. Nat. Ecol. Evol. 4, 1459–1471. doi: 10.1038/s41559-020-01298-8
Gleason, S. M., Blackman, C. J., Cook, A. M., Laws, C. A., and Westoby, M. (2014). Whole-plant capacitance, embolism resistance and slow transpiration rates all contribute to longer desiccation times in woody angiosperms from arid and wet habitats. Tree Physiol. 34, 275–284. doi: 10.1093/treephys/tpu001
Gora, E. M., and Esquivel-Muelbert, A. (2021). Implications of size-dependent tree mortality for tropical forest carbon dynamics. Nat. Plants 7, 384–391. doi: 10.1038/s41477-021-00879-0
Guo, J. S., Hultine, K. R., Koch, G. W., Kropp, H., and Ogle, K. (2020). Temporal shifts in iso/anisohydry revealed from daily observations of plant water potential in a dominant desert shrub. New Phytol. 225, 713–726. doi: 10.1111/nph.16196
Hacke, U. G., Stiller, V., Sperry, J. S., Pittermann, J., and Mcculloh, K. A. (2001). Cavitation fatigue. Embolism and refilling cycles can weaken the cavitation resistance of xylem. Plant Physiol. 125, 779–786. doi: 10.1104/pp.125.2.779
Hartmann, H., Moura, C. F., Anderegg, W. R., Ruehr, N. K., Salmon, Y., Allen, C. D., et al. (2018). Research frontiers for improving our understanding of drought-induced tree and forest mortality. New Phytol. 218, 15–28. doi: 10.1111/nph.15048
Hochberg, U., Windt, C. W., Ponomarenko, A., Zhang, Y. J., Gersony, J., Rockwell, F. E., et al. (2017). Stomatal closure, basal leaf embolism, and shedding protect the hydraulic integrity of grape stems. Plant Physiol. 174, 764–775. doi: 10.1104/pp.16.01816
Hoffmann, W. A., Marchin, R. M., Abit, P., and Lau, O. L. (2011). Hydraulic failure and tree dieback are associated with high wood density in a temperate forest under extreme drought. Glob. Chang. Biol. 17, 2731–2742. doi: 10.1111/j.1365-2486.2011.02401.x
Jiang, P., Meinzer, F. C., Wang, H., Kou, L., Dai, X., and Fu, X. (2020). Below-ground determinants and ecological implications of shrub species’ degree of isohydry in subtropical pine plantations. New Phytol. 226, 1656–1666. doi: 10.1111/nph.16502
Johnson, D. M., Berry, Z. C., Baker, K. V., Smith, D. D., Mcculloh, K. A., and Domec, J. C. (2018a). Leaf hydraulic parameters are more plastic in species that experience a wider range of leaf water potentials. Funct. Ecol. 32, 894–903. doi: 10.1111/1365-2435.13049
Johnson, K. M., Brodersen, C., Carins-Murphy, M. R., Choat, B., and Brodribb, T. J. (2020). Xylem embolism spreads by single-conduit events in three dry forest angiosperm stems. Plant Physiol. 184, 212–222. doi: 10.1104/pp.20.00464
Johnson, D. M., Domec, J. C., Carter Berry, Z., Schwantes, A. M., Mcculloh, K. A., Woodruff, D. R., et al. (2018b). Co-occurring woody species have diverse hydraulic strategies and mortality rates during an extreme drought. Plant Cell Environ. 41, 576–588. doi: 10.1111/pce.13121
Johnson, D. M., Wortemann, R., Mcculloh, K. A., Jordan-Meille, L., Ward, E., Warren, J. M., et al. (2016). A test of the hydraulic vulnerability segmentation hypothesis in angiosperm and conifer tree species. Tree Physiol. 36, 983–993. doi: 10.1093/treephys/tpw031
Kattge, J., Bönisch, G., Díaz, S., Lavorel, S., Prentice, I. C., Leadley, P., et al. (2020). TRY plant trait database-enhanced coverage and open access. Glob. Chang. Biol. 26, 119–188. doi: 10.1111/gcb.14904
Kennedy, D., Swenson, S., Oleson, K. W., Lawrence, D. M., Fisher, R., Lola da Costa, A. C., et al. (2019). Implementing plant hydraulics in the community land model, version 5. J. Adv. Model. Earth Syst. 11, 485–513. doi: 10.1029/2018MS001500
Klein, T. (2014). The variability of stomatal sensitivity to leaf water potential across tree species indicates a continuum between isohydric and anisohydric behaviours. Funct. Ecol. 28, 1313–1320. doi: 10.1111/1365-2435.12289
Knipfer, T., Cuneo, I. F., Earles, J. M., Reyes, C., Brodersen, C. R., and Mcelrone, A. J. (2017). Storage compartments for capillary water rarely refill in an intact woody plant. Plant Physiol. 175, 1649–1660. doi: 10.1104/pp.17.01133
Knipfer, T., Reyes, C., Earles, J. M., Berry, Z. C., Johnson, D. M., Brodersen, C. R., et al. (2019). Spatiotemporal coupling of vessel cavitation and discharge of stored xylem water in a tree sapling. Plant Physiol. 179, 1658–1668. doi: 10.1104/pp.18.01303
Konings, A. G., Rao, K., and Steele-Dunne, S. C. (2019). Macro to micro: microwave remote sensing of plant water content for physiology and ecology. New Phytol. 223, 1166–1172. doi: 10.1111/nph.15808
Lamy, J. B., Delzon, S., Bouche, P. S., Alia, R., Vendramin, G. G., Cochard, H., et al. (2014). Limited genetic variability and phenotypic plasticity detected for cavitation resistance in a Mediterranean pine. New Phytol. 201, 874–886. doi: 10.1111/nph.12556
Lanning, M., Wang, L., and Novick, K. A. (2020). The importance of cuticular permeance in assessing plant water-use strategies. Tree Physiol. 40, 425–432. doi: 10.1093/treephys/tpaa020
Levionnois, S., Ziegler, C., Jansen, S., Calvet, E., Coste, S., Stahl, C., et al. (2020). Vulnerability and hydraulic segmentations at the stem-leaf transition: coordination across Neotropical trees. New Phytol. 228, 512–524. doi: 10.1111/nph.16723
Li, X., Blackman, C. J., Choat, B., Duursma, R. A., Rymer, P. D., Medlyn, B. E., et al. (2018a). Tree hydraulic traits are coordinated and strongly linked to climate-of-origin across a rainfall gradient. Plant Cell Environ. 41, 646–660. doi: 10.1111/pce.13129
Li, X., Blackman, C. J., Peters, J. M., Choat, B., Rymer, P. D., Medlyn, B. E., et al. (2019). More than iso/anisohydry: hydroscapes integrate plant water use and drought tolerance traits in 10 eucalypt species from contrasting climates. Funct. Ecol. 33, 1035–1049. doi: 10.1111/1365-2435.13320
Li, X., Blackman, C. J., Rymer, P. D., Quintans, D., Duursma, R. A., Choat, B., et al. (2018b). Xylem embolism measured retrospectively is linked to canopy dieback in natural populations of Eucalyptus piperita following drought. Tree Physiol. 38, 1193–1199. doi: 10.1093/treephys/tpy052
Li, S., Feifel, M., Karimi, Z., Schuldt, B., Choat, B., and Jansen, S. (2016). Leaf gas exchange performance and the lethal water potential of five European species during drought. Tree Physiol. 36, tpv117–tpv192. doi: 10.1093/treephys/tpv117
Liang, X., Ye, Q., Liu, H., and Brodribb, T. J. (2021). Wood density predicts mortality threshold for diverse trees. New Phytol. 229, 3053–3057. doi: 10.1111/nph.17117
Liu, J., Gu, L., Yu, Y., Huang, P., Wu, Z., Zhang, Q., et al. (2019). Corticular photosynthesis drives bark water uptake to refill embolized vessels in dehydrated branches of Salix matsudana. Plant Cell Environ. 42, 2584–2596. doi: 10.1111/pce.13578
Liu, Y. Y., Wang, A. Y., An, Y. N., Lian, P. Y., Wu, D. D., Zhu, J. J., et al. (2018). Hydraulics play an important role in causing low growth rate and dieback of aging Pinus sylvestris var. mongolica trees in plantations of Northeast China. Plant Cell Environ. 41, 1500–1511. doi: 10.1111/pce.13160
López, R., Cano, F. J., Martin-Stpaul, N. K., Cochard, H., and Choat, B. (2021). Coordination of stem and leaf traits define different strategies to regulate water loss and tolerance ranges to aridity. New Phytol. 230, 497–509. doi: 10.1111/nph.17185
Lübbe, T., Lamarque, L. J., Delzon, S., Torres Ruiz, J. M., Burlett, R., Leuschner, C., et al. (2021). High variation in hydraulic efficiency but not xylem safety between roots and branches in four temperate broad-leaved tree species. Funct. Ecol. 36, 699–712. doi: 10.1111/1365-2435.13975
Mantova, M., Herbette, S., Cochard, H., and Torres-Ruiz, J. M. (2021). Hydraulic failure and tree mortality: from correlation to causation. Trends Plant Sci 27, 335–345. doi: 10.1016/j.tplants.2021.10.003
Markesteijn, L., Poorter, L., Paz, H., Sack, L., and Bongers, F. (2011). Ecological differentiation in xylem cavitation resistance is associated with stem and leaf structural traits. Plant Cell Environ. 34, 137–148. doi: 10.1111/j.1365-3040.2010.02231.x
Martinez-Vilalta, J., Anderegg, W. R., Sapes, G., and Sala, A. (2019). Greater focus on water pools may improve our ability to understand and anticipate drought-induced mortality in plants. New Phytol. 223, 22–32. doi: 10.1111/nph.15644
Martínez-Vilalta, J., and Garcia-Forner, N. (2017). Water potential regulation, stomatal behaviour and hydraulic transport under drought: deconstructing the iso/anisohydric concept. Plant Cell Environ. 40, 962–976. doi: 10.1111/pce.12846
Martin-Stpaul, N., Delzon, S., and Cochard, H. (2017). Plant resistance to drought depends on timely stomatal closure. Ecol. Lett. 20, 1437–1447. doi: 10.1111/ele.12851
McDowell, N. G., Beerling, D. J., Breshears, D. D., Fisher, R. A., Raffa, K. F., and Stitt, M. (2011). The interdependence of mechanisms underlying climate-driven vegetation mortality. Trends Ecol. Evol. 26, 523–532. doi: 10.1016/j.tree.2011.06.003
McDowell, N. G., Fisher, R. A., Xu, C., Domec, J. C., Hölttä, T., Mackay, D. S., et al. (2013). Evaluating theories of drought-induced vegetation mortality using a multimodel-experiment framework. New Phytol. 200, 304–321. doi: 10.1111/nph.12465
McDowell, N., Pockman, W. T., Allen, C. D., Breshears, D. D., Cobb, N., Kolb, T., et al. (2008). Mechanisms of plant survival and mortality during drought: why do some plants survive while others succumb to drought? New Phytol. 178, 719–739. doi: 10.1111/j.1469-8137.2008.02436.x
Medlyn, B. E., De Kauwe, M. G., and Duursma, R. A. (2016). New developments in the effort to model ecosystems under water stress. New Phytol. 212, 5–7. doi: 10.1111/nph.14082
Medlyn, B. E., Zaehle, S., De Kauwe, M. G., Walker, A. P., Dietze, M. C., Hanson, P. J., et al. (2015). Using ecosystem experiments to improve vegetation models. Nat. Clim. Chang. 5, 528–534. doi: 10.1038/nclimate2621
Meinzer, F. C., Johnson, D. M., Lachenbruch, B., Mcculloh, K. A., and Woodruff, D. R. (2009). Xylem hydraulic safety margins in woody plants: coordination of stomatal control of xylem tension with hydraulic capacitance. Funct. Ecol. 23, 922–930. doi: 10.1111/j.1365-2435.2009.01577.x
Meinzer, F. C., Woodruff, D. R., Marias, D. E., Smith, D. D., McCulloh, K. A., Howard, A. R., et al. (2016). Mapping ‘hydroscapes’ along the iso‐to anisohydric continuum of stomatal regulation of plant water status. Ecol. Lett. 19, 1343–1352. doi: 10.1111/ele.12670
Meir, P., Mencuccini, M., and Dewar, R. C. (2015). Drought-related tree mortality: addressing the gaps in understanding and prediction. New Phytol. 207, 28–33. doi: 10.1111/nph.13382
Nolan, R. H., Gauthey, A., Losso, A., Medlyn, B. E., Smith, R., Chhajed, S. S., et al. (2021). Hydraulic failure and tree size linked with canopy die-back in eucalypt forest during extreme drought. New Phytol. 230, 1354–1365. doi: 10.1111/nph.17298
O’Brien, M. J., Leuzinger, S., Philipson, C. D., Tay, J., and Hector, A. (2014). Drought survival of tropical tree seedlings enhanced by non-structural carbohydrate levels. Nat. Clim. Chang. 4, 710–714. doi: 10.1038/nclimate2281
Pan, Y., Birdsey, R. A., Fang, J., Houghton, R., Kauppi, P. E., Kurz, W. A., et al. (2011). A large and persistent carbon sink in the world’s forests. Science 333, 988–993. doi: 10.1126/science.1201609
Peters, J. M., Gauthey, A., Lopez, R., Carins-Murphy, M. R., Brodribb, T. J., and Choat, B. (2020). Non-invasive imaging reveals convergence in root and stem vulnerability to cavitation across five tree species. J. Exp. Bot. 71, 6623–6637. doi: 10.1093/jxb/eraa381
Pfautsch, S., Renard, J., Tjoelker, M. G., and Salih, A. (2015). Phloem as capacitor: radial transfer of water into xylem of tree stems occurs via symplastic transport in ray parenchyma. Plant Physiol. 167, 963–971. doi: 10.1104/pp.114.254581
Pivovaroff, A. L., Sack, L., and Santiago, L. S. (2014). Coordination of stem and leaf hydraulic conductance in southern California shrubs: a test of the hydraulic segmentation hypothesis. New Phytol. 203, 842–850. doi: 10.1111/nph.12850
Powers, J. S., Vargas, G. G., Brodribb, T. J., Schwartz, N. B., Pérez-Aviles, D., Smith-Martin, C. M., et al. (2020). A catastrophic tropical drought kills hydraulically vulnerable tree species. Glob. Chang. Biol. 26, 3122–3133. doi: 10.1111/gcb.15037
Reich, P. B. (2014). The world-wide ‘fast-slow’ plant economics spectrum: a traits manifesto. J. Ecol. 102, 275–301. doi: 10.1111/1365-2745.12211
Reich, P., Ellsworth, D., and Walters, M. (1998). Leaf structure (specific leaf area) modulates photosynthesis-nitrogen relations: evidence from within and across species and functional groups. Funct. Ecol. 12, 948–958. doi: 10.1046/j.1365-2435.1998.00274.x
Richards, A. E., Wright, I. J., Lenz, T. I., and Zanne, A. E. (2014). Sapwood capacitance is greater in evergreen sclerophyll species growing in high compared to low-rainfall environments. Funct. Ecol. 28, 734–744. doi: 10.1111/1365-2435.12193
Rodriguez-Dominguez, C. M., Carins Murphy, M. R., Lucani, C., and Brodribb, T. J. (2018). Mapping xylem failure in disparate organs of whole plants reveals extreme resistance in olive roots. New Phytol. 218, 1025–1035. doi: 10.1111/nph.15079
Rogers, A., Medlyn, B. E., Dukes, J. S., Bonan, G., Von Caemmerer, S., Dietze, M. C., et al. (2017). A roadmap for improving the representation of photosynthesis in earth system models. New Phytol. 213, 22–42. doi: 10.1111/nph.14283
Russo, S. E., Jenkins, K. L., Wiser, S. K., Uriarte, M., Duncan, R. P., and Coomes, D. A. (2010). Interspecific relationships among growth, mortality and xylem traits of woody species from New Zealand. Funct. Ecol. 24, 253–262. doi: 10.1111/j.1365-2435.2009.01670.x
Sabot, M. E., De Kauwe, M. G., Pitman, A. J., Medlyn, B. E., Verhoef, A., Ukkola, A. M., et al. (2020). Plant profit maximization improves predictions of European forest responses to drought. New Phytol. 226, 1638–1655. doi: 10.1111/nph.16376
Salomón, R. L., Steppe, K., Ourcival, J. M., Villers, S., Rodríguez-Calcerrada, J., Schapman, R., et al. (2020). Hydraulic acclimation in a Mediterranean oak subjected to permanent throughfall exclusion results in increased stem hydraulic capacitance. Plant Cell Environ. 43, 1528–1544. doi: 10.1111/pce.13751
Santiago, L. S., De Guzman, M. E., Baraloto, C., Vogenberg, J. E., Brodie, M., Hérault, B., et al. (2018). Coordination and trade-offs among hydraulic safety, efficiency and drought avoidance traits in Amazonian rainforest canopy tree species. New Phytol. 218, 1015–1024. doi: 10.1111/nph.15058
Sapes, G., Roskilly, B., Dobrowski, S., Maneta, M., Anderegg, W. R., Martinez-Vilalta, J., et al. (2019). Plant water content integrates hydraulics and carbon depletion to predict drought-induced seedling mortality. Tree Physiol. 39, 1300–1312. doi: 10.1093/treephys/tpz062
Schenk, H. J., Espino, S., Romo, D. M., Nima, N., Do, A. Y., Michaud, J. M., et al. (2017). Xylem surfactants introduce a new element to the cohesion-tension theory. Plant Physiol. 173, 1177–1196. doi: 10.1104/pp.16.01039
Schuster, A. C., Burghardt, M., and Riederer, M. (2017). The ecophysiology of leaf cuticular transpiration: are cuticular water permeabilities adapted to ecological conditions? J. Exp. Bot. 68, 5271–5279. doi: 10.1093/jxb/erx321
Sevanto, S., Mcdowell, N. G., Dickman, L. T., Pangle, R., and Pockman, W. T. (2014). How do trees die? A test of the hydraulic failure and carbon starvation hypotheses. Plant Cell Environ. 37, 153–161. doi: 10.1111/pce.12141
Sevanto, S., and Xu, C. (2016). Towards more accurate vegetation mortality predictions. Tree Physiol. 36, 1191–1195. doi: 10.1093/treephys/tpw082
Skelton, R. P., West, A. G., and Dawson, T. E. (2015). Predicting plant vulnerability to drought in biodiverse regions using functional traits. Proc. Natl. Acad. Sci. U. S. A. 112, 5744–5749. doi: 10.1073/pnas.1503376112
Sorek, Y., Greenstein, S., Netzer, Y., Shtein, I., Jansen, S., and Hochberg, U. (2021). An increase in xylem embolism resistance of grapevine leaves during the growing season is coordinated with stomatal regulation, turgor loss point and intervessel pit membranes. New Phytol. 229, 1955–1969. doi: 10.1111/nph.17025
Sperry, J. S., Adler, F., Campbell, G., and Comstock, J. (1998). Limitation of plant water use by rhizosphere and xylem conductance: results from a model. Plant Cell Environ. 21, 347–359. doi: 10.1046/j.1365-3040.1998.00287.x
Thrippleton, T., Hülsmann, L., Cailleret, M., and Bugmann, H. (2021). An evaluation of multi-species empirical tree mortality algorithms for dynamic vegetation modelling. Sci. Rep. 11:19845. doi: 10.1038/s41598-021-98880-2
Tomasella, M., Casolo, V., Aichner, N., Petruzzellis, F., Savi, T., Trifilò, P., et al. (2019). Non-structural carbohydrate and hydraulic dynamics during drought and recovery in Fraxinus ornus and Ostrya carpinifolia saplings. Plant Physiol. Biochem. 145, 1–9. doi: 10.1016/j.plaphy.2019.10.024
Trifilò, P., Casolo, V., Raimondo, F., Petrussa, E., Boscutti, F., Gullo, M. A. L., et al. (2017). Effects of prolonged drought on stem non-structural carbohydrates content and post-drought hydraulic recovery in Laurus nobilis L.: the possible link between carbon starvation and hydraulic failure. Plant Physiol. Biochem. 120, 232–241. doi: 10.1016/j.plaphy.2017.10.003
Trugman, A. T. (2021). Integrating plant physiology and community ecology across scales through trait‐based models to predict drought mortality. New Phytol. 234, 21–27. doi: 10.1111/nph.17821
Trugman, A. T., Detto, M., Bartlett, M. K., Medvigy, D., Anderegg, W. R. L., Schwalm, C., et al. (2018). Tree carbon allocation explains forest drought-kill and recovery patterns. Ecol. Lett. 21, 1552–1560. doi: 10.1111/ele.13136
Urli, M., Porté, A. J., Cochard, H., Guengant, Y., Burlett, R., and Delzon, S. (2013). Xylem embolism threshold for catastrophic hydraulic failure in angiosperm trees. Tree Physiol. 33, 672–683. doi: 10.1093/treephys/tpt030
Wang, Y., Köhler, P., He, L., Doughty, R., Braghiere, R. K., Wood, J. D., et al. (2021). Testing stomatal models at the stand level in deciduous angiosperm and evergreen gymnosperm forests using CliMA land (v0.1). Geosci. Model Dev. 14, 6741–6763. doi: 10.5194/gmd-14-6741-2021
Wason, J. W., Anstreicher, K. S., Stephansky, N., Huggett, B. A., and Brodersen, C. R. (2018). Hydraulic safety margins and air-seeding thresholds in roots, trunks, branches and petioles of four northern hardwood trees. New Phytol. 219, 77–88. doi: 10.1111/nph.15135
Wason, J., Bouda, M., Lee, E. F., Mcelrone, A. J., Phillips, R. J., Shackel, K. A., et al. (2021). Xylem network connectivity and embolism spread in grapevine (Vitis vinifera L.). Plant Physiol. 186, 373–387. doi: 10.1093/plphys/kiab045
Wolfe, B. T., Sperry, J. S., and Kursar, T. A. (2016). Does leaf shedding protect stems from cavitation during seasonal droughts? A test of the hydraulic fuse hypothesis. New Phytol. 212, 1007–1018. doi: 10.1111/nph.14087
Wright, I. J., Ackerly, D. D., Bongers, F., Harms, K. E., Ibarra-Manriquez, G., Martinez-Ramos, M., et al. (2007). Relationships among ecologically important dimensions of plant trait variation in seven Neotropical forests. Ann. Bot. 99, 1003–1015. doi: 10.1093/aob/mcl066
Wu, G., Guan, K., Li, Y., Novick, K. A., Feng, X., Mcdowell, N. G., et al. (2021). Interannual variability of ecosystem iso/anisohydry is regulated by environmental dryness. New Phytol. 229, 2562–2575. doi: 10.1111/nph.17040
Wu, X., Liu, H., Li, X., Ciais, P., Babst, F., Guo, W., et al. (2018). Differentiating drought legacy effects on vegetation growth over the temperate northern hemisphere. Glob. Chang. Biol. 24, 504–516. doi: 10.1111/gcb.13920
Xi, B., Di, N., Liu, J., Zhang, R., and Cao, Z. (2018). Hydrologic regulation of plant rooting depth: pay attention to the widespread scenario with intense seasonal groundwater table fluctuation. Proc. Natl. Acad. Sci. U. S. A. 115, E3863–E3864. doi: 10.1073/pnas.1803987115
Xu, X., Medvigy, D., Powers, J. S., Becknell, J. M., and Guan, K. (2016). Diversity in plant hydraulic traits explains seasonal and inter-annual variations of vegetation dynamics in seasonally dry tropical forests. New Phytol. 212, 80–95. doi: 10.1111/nph.14009
Zeng, X. (2001). Global vegetation root distribution for land modeling. J. Hydrometeorol. 2, 525–530. doi: 10.1175/1525-7541(2001)002<0525:GVRDFL>2.0.CO;2
Zhou, S., Duursma, R. A., Medlyn, B. E., Kelly, J. W., and Prentice, I. C. (2013). How should we model plant responses to drought? An analysis of stomatal and non-stomatal responses to water stress. Agric. For. Meteorol. 182–183, 204–214. doi: 10.1016/j.agrformet.2013.05.009
Zhou, S., Medlyn, B., Sabaté, S., Sperlich, D., and Prentice, I. C. (2014). Short-term water stress impacts on stomatal, mesophyll and biochemical limitations to photosynthesis differ consistently among tree species from contrasting climates. Tree Physiol. 34, 1035–1046. doi: 10.1093/treephys/tpu072
Zhu, S. D., He, P. C., Li, R. H., Fu, S. L., Lin, Y. B., Zhou, L. X., et al. (2018). Drought tolerance traits predict survival ratio of native tree species planted in a subtropical degraded hilly area in South China. For. Ecol. Manag. 418, 41–46. doi: 10.1016/j.foreco.2017.09.016
Zanne, A. E., Lopez-Gonzalez, G., Coomes, D. A., Ilic, J., Jansen, S., Lewis, S. L., et al. (2009). Data from: Towards a worldwide wood economics spectrum. Dryad, Dataset. doi: 10.5061/dryad.234
Keywords: drought, tree mortality, hydraulic failure, carbohydrates, functional traits, plant hydraulics, land surface models
Citation: Li X, Xi B, Wu X, Choat B, Feng J, Jiang M and Tissue D (2022) Unlocking Drought-Induced Tree Mortality: Physiological Mechanisms to Modeling. Front. Plant Sci. 13:835921. doi: 10.3389/fpls.2022.835921
Received: 15 December 2021; Accepted: 04 March 2022;
Published: 04 April 2022.
Edited by:
Amanda A. Cardoso, Federal University of Alfenas, BrazilReviewed by:
Christoforos Pappas, Université TÉLUQ, CanadaCopyright © 2022 Li, Xi, Wu, Choat, Feng, Jiang and Tissue. This is an open-access article distributed under the terms of the Creative Commons Attribution License (CC BY). The use, distribution or reproduction in other forums is permitted, provided the original author(s) and the copyright owner(s) are credited and that the original publication in this journal is cited, in accordance with accepted academic practice. No use, distribution or reproduction is permitted which does not comply with these terms.
*Correspondence: Ximeng Li, bGl4aW1lbmcyMDA5QGhvdG1haWwuY29t; David Tissue, ZC50aXNzdWVAd2VzdGVybnN5ZG5leS5lZHUuYXU=
Disclaimer: All claims expressed in this article are solely those of the authors and do not necessarily represent those of their affiliated organizations, or those of the publisher, the editors and the reviewers. Any product that may be evaluated in this article or claim that may be made by its manufacturer is not guaranteed or endorsed by the publisher.
Research integrity at Frontiers
Learn more about the work of our research integrity team to safeguard the quality of each article we publish.