- 1Limpopo Department of Agriculture and Rural Development, Agriculture Regulatory and Technology Development Directorate, Crop Science Division, Towoomba Research Station, Bela-Bela, South Africa
- 2African Centre for Crop Improvement, University of KwaZulu-Natal, Pietermaritzburg, South Africa
- 3Vegetable, Industrial and Medicinal Plants, Agricultural Research Council, Pretoria, South Africa
Fruit quality traits of sweet watermelon (Citrullus lanatus var. lanatus) are crucial for new product development and commercialization. Sweet watermelon fruit quality traits are affected by the compositions of phytochemical compounds, phytohormones, and fruit flesh firmness which are affected by genes, the growing environment and their interaction. These compositions determine fruit ripening, eating quality, and postharvest shelf-life. Knowledge of the genetic profile and analyses of quality traits in watermelon is vital to develop improved cultivars with enhanced nutritional compositions, consumer-preferred traits, and extended storage life. This review aims to present the opportunities and progress made on the genetic analysis of fruit quality traits in watermelon as a guide for quality breeding based on economic and end-user attributes. The first section of the review highlights the genetic mechanisms involved in the biosynthesis of phytochemical compounds (i.e., sugars, carotenoids, amino acids, organic acids, and volatile compounds), phytohormones (i.e., ethylene and abscisic acid) and fruit flesh structural components (i.e., cellulose, hemicellulose, and pectin) elicited during watermelon fruit development and ripening. The second section pinpoints the progress on the development of molecular markers and quantitative trait loci (QTL) analysis for phytochemical compounds, phytohormones and fruit quality attributes. The review presents gene-editing technology and innovations associated with fruit quality traits for selection and accelerated cultivar development. Finally, the paper discussed gene actions conditioning fruit ripening in citron watermelon (C. lanatus var. citroides [L. H. Bailey] Mansf. ex Greb.) as reference genetic resources to guide current and future breeding. Information presented in this review is useful for watermelon variety design, product profiling and development to serve the diverse value chains of the crop.
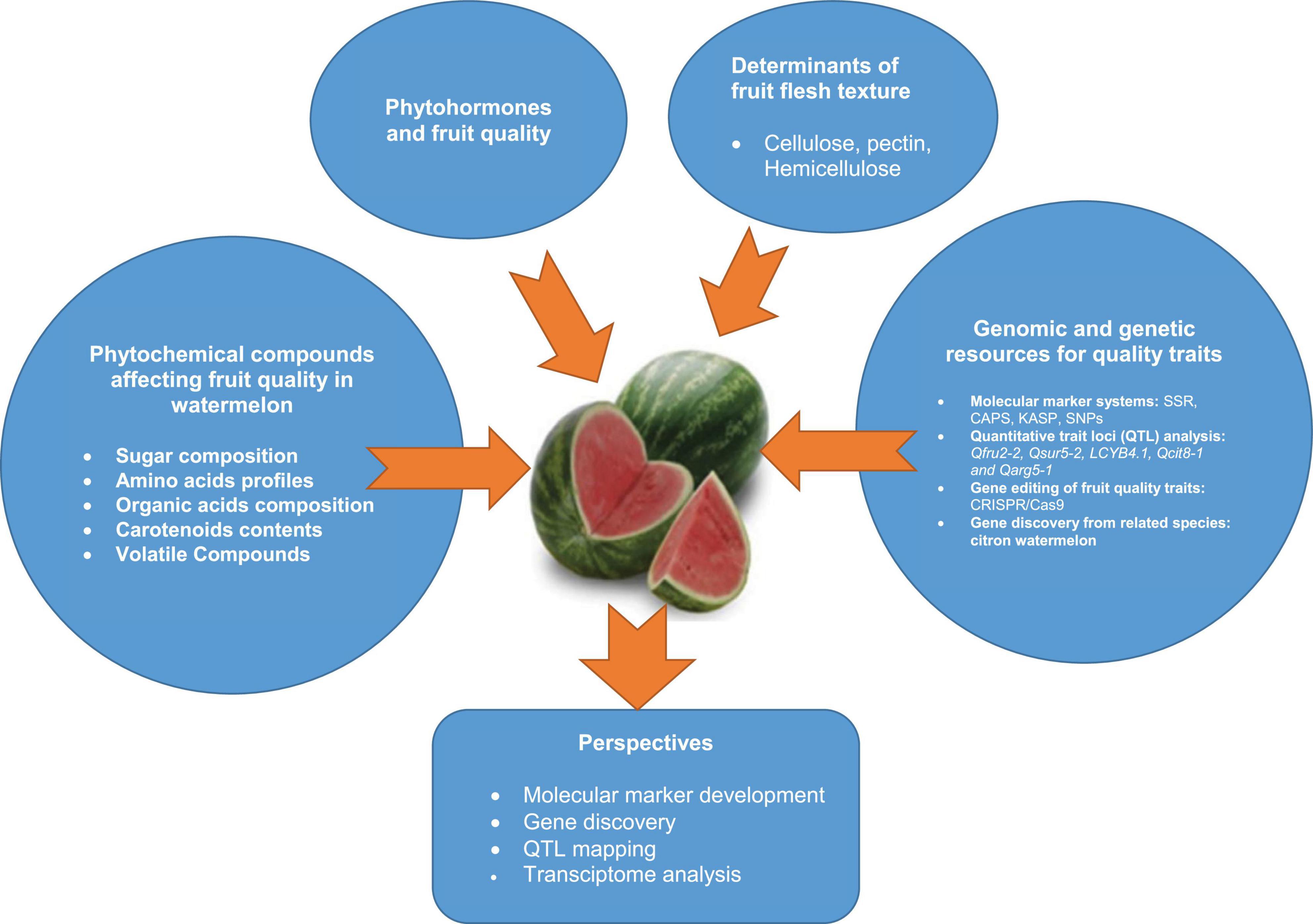
Graphical Abstract. An overview of phytochemical compounds, phytohormones and flesh textural properties influencing fruit quality of watermelon fruit, and developed genomic and genetic resources for fruit quality breeding.
Introduction
Sweet watermelon [Citrullus lanatus (Thunb.) Matsum. and Nakai var. lanatus; 2n = 2x = 22] belonging to the family Cucurbitaceae is predominantly grown for its fresh and nutritious fruit. The genus Citrullus comprises several species: C. lanatus var. citroides, C. mucosospermus, C. colocynthis, C. ecirrhosus, C. rehmii, and C. naudinianus (Chomicki and Renner, 2015). The center of origin and genetic diversity of most Citrullus species is continental Africa (Robinson and Decker-Walters, 1997; Rubatzky, 2001; Dane and Lang, 2004).
With an estimated total annual production of ∼90 million tons, watermelon ranks in the top 10 fruit crops widely produced globally (FAOSTAT, 2020). China is the largest producer of watermelons, accounting for 60 million tons per annum from an estimated agricultural land of 1.4 million ha (FAOSTAT, 2020). The following leading global producers of watermelons are Turkey and Iran, with an estimated annual total production of 3.4 and 2.7 million tons, respectively. Brazil and Egypt rank fourth and fifth with total production of 2.1 and 1.4 million tons per annum, respectively. The United States ranks sixth with 1.74 million tons total production annually. The economic and nutritional values of watermelon have been recognized in recent years, creating the opportunity to develop and commercialize new varieties combining high fruit yield and quality (Yang et al., 2016).
Watermelon fruit is a rich source of water and human health-benefiting nutrients. The fruit consists of sugars (i.e., fructose, sucrose, and glucose), carotenoids (i.e., lycopene and β-carotene), amino acids (i.e., citrulline and arginine), organic acids (i.e., citric and glutamic acids) and volatile compounds (VOCs) (Perkins-Veazie et al., 2006; Davis et al., 2011; Liu et al., 2012; Yoo et al., 2012; Ren et al., 2014; Jawad et al., 2020). The fruit sugar content determines the sweetness, while the VOCs influence aroma and flavor, and the organic acids enhance fruit acidity (Liu et al., 2012, 2013, 2018; Dima et al., 2014). Reportedly, carotenoid accumulation are associated with flesh color development (Tadmor et al., 2005; Bang et al., 2007, 2010). The aforementioned phytochemical compounds condition the overall nutritional compositions, eating quality, and fruit ripening (Liu et al., 2012; Dima et al., 2014; Ramirez et al., 2020; Wang C. et al., 2021). Fruit hormones such as ethylene and abscisic acid, and flesh composition (i.e., cellulose, hemicellulose, and pectin) determine fruit ripening, shelf-life, and chemical compositions influencing product quality such as fruit firmness, flesh- chewiness and -gumminess, and maturity (Gao et al., 2020; Sun et al., 2020; Anees et al., 2021).
Watermelon’s fruit quality are affected by polygenes, the growing environment and their interaction. Furthermore, fruit quality is a highly complex trait governed by physiological, biochemical and molecular processes (Yu et al., 2012; Guo et al., 2015; Fang et al., 2020; Sun et al., 2020). The genetic mechanism of fruit quality in watermelon was revealed through comparative transcriptome analysis (Grassi et al., 2013; Guo et al., 2015; Fang et al., 2020). The reports indicated that multiple genes are involved and differentially expressed, influencing fruit quality (Wechter et al., 2008; Kang et al., 2010; Guo et al., 2015; Wang et al., 2017; Zhu et al., 2017; Yue et al., 2021). Quantitative trait loci (QTL) analysis revealed that fruit quality influencing genes in watermelon are located across the 11 chromosomes (Sandlin et al., 2012; Ren et al., 2014; Cheng et al., 2016; Branham et al., 2017; Fall et al., 2019; Yang et al., 2021). The QTL were successfully used in marker-assisted breeding, including the parental selection and development of novel progenies of watermelon (Cheng et al., 2016; Shang et al., 2016; Branham et al., 2017; Zhang et al., 2018; Jiang et al., 2019; Jin et al., 2019; Yang et al., 2021).
Fruit traits of sweet watermelon are crucial for new product development and commercialization. The novel and value-added genotypes will serve the fresh market, processing and pharmaceutical industry. Value-added products (i.e., pickles, jam, fruit puree, popsicles, and watermelon juice) are derived from newly designed cultivars with good quality attributes. The new cultivars should possess balanced bioactive compounds of nutritive and therapeutic significance (Tlili et al., 2011a,b; Rico et al., 2020; Zamuz et al., 2021; Zia et al., 2021).
Watermelon breeders have relied on conventional selection methods (e.g., single plant, pure line, mass selection) to develop cultivars with excellent fruit quality traits (Davis and King, 2007; Davis et al., 2008; Gao et al., 2018a). However, the low genetic base of sweet watermelon (Levi et al., 2001a,2004; Che et al., 2003; Kwon et al., 2010; Solmaz et al., 2010; Hwang et al., 2011; Minsart et al., 2011; Sheng et al., 2012; Yang et al., 2016; Zhang et al., 2016; Singh et al., 2017; Stone et al., 2019) poses challenges for quality breeding. Also, in many watermelon improvement programs, a limited number of watermelon genotypes are utilized as breeding parents (Levi et al., 2001a,2004; Zhang et al., 2012; Pal et al., 2020). Most parental genotypes exhibit excellent agronomic and horticultural traits but share similar genetic backgrounds presenting genetic bottlenecks for breeding (Levi et al., 2001a; Guo et al., 2012; Wang et al., 2015; Zhang et al., 2016). Non-conventional breeding using gene-editing technologies presents a viable and alternative approach for speed breeding and release of watermelon varieties possessing suitable agronomic and quality traits to serve the value chains of the crop. For example, targeted editing of genes associated with agronomic traits in the watermelon using the clustered regularly interspaced short palindromic repeats (CRISPR/Cas9) enabled the selection of novel cultivars (Tian et al., 2017; Zhang et al., 2020a; Wang Y. et al., 2021). Furthermore, the gene editing and associated technologies will deliver knowledge on the genetic regulation of fruit quality traits in watermelon that will aid in the development of improved cultivars with enhanced nutritional compositions, market-preferred traits and extended storage life.
In light of the above background, this review aims to present the opportunities and progress made on the genetic analysis of fruit quality traits in watermelon as a guide for quality breeding based on economic and end-user attributes. The first section of the review highlights the genetic mechanisms involved in the biosynthesis of phytochemical compounds, phytohormones and fruit flesh structural components during watermelon fruit development and ripening. This is followed by a summary of the progresses made on molecular markers and QTL analysis for fruit quality attributes. The review presents the new generation genetic and breeding technologies and innovations for accelerated cultivar development with excellent fruit quality traits. The paper presented possible gene actions conditioning fruit ripening in citron watermelon genetic resources to guide current and future breeding. Information presented in this review is useful for watermelon variety design, product profiling and development to serve diverse value chains of the crop.
Genetic Analysis of Fruit Quality in Sweet Watermelon
Sugar Composition of Sweet Watermelon Fruit
Flesh sweetness is an important attribute that determines the eating quality of ripe watermelon fruit (Yativ et al., 2010; Liu et al., 2013). Sweetness is determined by the composition of the following sugars, namely: sucrose, fructose, and glucose (Ren et al., 2014; Zhu et al., 2017). The most important enzymes that regulate sugar metabolism of watermelon are sucrose synthase and sucrose phosphate synthase (Yativ et al., 2010; Guo et al., 2015). Also, the expression of sugar biosynthetic gene clusters including α-galactosidase, invertase and Urease diphosphate (UDP)-galactose/glucose pyrophosphorylase (UDP-Gal/Glc PPase) increase during watermelon fruit ripening. Reportedly, the gene designated as Cla013902 influence sugar metabolism in watermelon (Guo et al., 2015). A total of nine α-galactosidase genes were identified in the watermelon genome. The nine genes are reportedly involved in the hydrolyzes of stachyose and raffinose (Guo et al., 2012).
Raffinose family oligosaccharides (RFOs), including raffinose and stachyose are the main photo-assimilates translocated in the phloem of cucurbit crops (Zhang et al., 2010). The hydrolysis of RFOs is crucial for sugar accumulation and transportation in the fruit. RFOs are hydrolyzed rapidly by α-galactosidase genes in the phloem. Sugar accumulation in the phloem and sucrose translocation to fruits require the action of insoluble acid invertase (Guo et al., 2012). Five insoluble acid invertase (IAI) genes are involved in sugar accumulation in watermelon (Guo et al., 2015). Of these, the IAI gene Cla020872 is involved in the extracellular sucrose degeneration and thus facilitate fructose and glucose translocation and the intercellular sugar accumulation in watermelon fruit (Liu et al., 2013; Guo et al., 2015). Another gene Cla013902, also contribute to sugar metabolism in watermelon. Two major sugar transporter genes, namely: Cla015835 and Cla015836 are associated with sugar transportation and accumulation in watermelon fruit (Liu et al., 2013). Recently, the alkaline α-galactosidase gene ClAGA2 expressed in the vascular bundle was identified as a major gene controlling stachyose and raffinose hydrolysis in watermelon. ClAGA2 controls fruit raffinose hydrolysis and reduces sugar content in ripened watermelon fruits (Ren et al., 2021). Sugar Transporter 3 gene designed as ClSWEET3 and Tonoplast Sugar Transporter gene (i.e., ClTST2) influence sugar transport and storage in watermelon fruit cell vacuoles (Ren et al., 2021). Sugar transporters genes denominated as EDR6 (Cla97C05G087120) and STP (Cla97C01G018840) were highly expressed during watermelon fruit ripening simultaneously influencing sugar accumulation and transportation (Umer et al., 2020b). A tonoplast sugar transporter gene designated as ClTST2 (gene ID: Cla000264) was highly expressed during fruit ripening associating with tonoplast uptake and accumulation of sugars in watermelon fruit flesh cells (Ren et al., 2018). The gene WMFBPA-2 was found to be influencing fructose breakdown (Umer et al., 2020a). Fructokinase (FK), fructose bisphosphate aldolase (FBA), and fructose 1-6, bisphosphate (FBP) genes regulate fructose metabolism of watermelon. The FK gene designated as Cla97C05G094630, FBA (Cla97C04G076620) and FBP (Cla97C08G147060) were the most important regulators of fructose metabolism in watermelon fruit (Umer et al., 2020b). The following genes: Cla97C01G000640 (SWEET), Cla97C05G087120 (EDR6), and Cla97C01G018840 identified from sweet and sweet-and-sour genotypes reportedly regulate glucose biosynthesis, while Cla97C03G064990 condition sucrose biosynthesis in watermelon (Umer et al., 2020b). A vacuolar sugar transporter gene named ClVST1 was highly expressed during the ripening of watermelon fruit associated with sucrose accumulation (Ren et al., 2020). Genes involved in sugar biosynthesis can be up- or down-regulated during the breeding of desired genotypes. The sweetness product profile influences the needs and preferences of consumers and the industry during the development of value-added products.
Amino Acids Profiles of Sweet Watermelon Fruit
Citrulline is the most abundant amino acid present in ripened watermelon fruit (Joshi et al., 2019; Zhong et al., 2019). Citrulline is a non-essential amino acid and is biosynthesized as a metabolic intermediate compound during the urea cycle (Bahri et al., 2013). This amino acid is a precursor of arginine, which is another major amino acid in watermelon fruit (Joshi et al., 2019; Song et al., 2020). Several genes involved in citrulline metabolism have been identified in watermelon. Of these, ornithine carbamoyltransferase (OTC; ClCG05G018820), N-acetylornithine aminotransferase (N-AOA; ClCG09G003180), N-acetylornithine-glutamate acetyltransferase (N-AOGA), -1 (ClCG11G013120) and CPS-2 (ClCG09G021680), N-acetylornithine deacetylase (AOD-1), N-acetylornithine deacetylase (AOD-3), and Nitric-oxide Synthase gene (ClCG01G004960) are associated with citrulline biosynthesis in watermelon (Joshi et al., 2019). In addition, genes namely: ASS-1,2,3-Argininosuccinate synthase; ASL-1,2-Argininosuccinate lyase; ARG – Arginase; ODC – Ornithine decarboxylase; and ADC-Arginine decarboxylase are reportedly associated with citrulline catabolism (Joshi et al., 2019). The genes Cla022154 (Argininosuccinate lyase), Cla022273 (Nacetylglutamate Kinase), and Cla022392 (Ornithine decarboxylase) are involved in citrulline biosynthesis, whereas candidate genes Cla020511, Cla020512, and Cla020781 (Ornithine carbamoyltransferase) are involved in the biosynthesis and accumulation of arginine (Fall et al., 2019).
Organic Acids Composition of Sweet Watermelon Fruit
The composition of organic acids enhances flesh acidity and improve the taste of ripe watermelon fruit. Organic acids including malic, quinic, citric, oxalic, and tartaric acids, accumulate in watermelon fruit (Liu et al., 2012; Gao et al., 2018a,b). Of these, citric and malic acids are abundantly present (Gao et al., 2018a,b). Differentially expressed genes, including the NAD-dependent malate dehydrogenase (NAD-cyt MDH), aluminum-activated malate transporter (ALMT), and citrate synthase (CS) influence the accumulation of organic acids in watermelon (Gao et al., 2018a). NAD-dependent malate dehydrogenase (NAD-cyt MDH) gene catalyzes the reversible conversion of malate into oxaloacetate (OAA) (Yao et al., 2011), while citrate synthase (CS) regulates the biosynthesis of citric acid. Aluminum activated malate transporters, and malate dehydrogenase genes regulate malate regulation and degradation (Umer et al., 2020a). The citrate synthase gene (Cla97C03G068240) and isocitrate dehydrogenase gene (Cla97C01G008870) are reportedly involved in citric acid synthesis and degradation (Umer et al., 2020b). Higher expression of malate dehydrogenases genes (i.e., Cla008235 and Cla011268) and citrate synthases gene (Cla013500) are associated with enhanced accumulation of malic and citric acids (Gao et al., 2018a). Also, three other genes, namely: WMALMT-3, WMMDH-1, and WMMDH-2, which are highly expressed during watermelon fruit development and ripening reportedly regulate malate biosynthesis and catabolism (Umer et al., 2020a). WMALMT-3 is the major gene regulating malic acid biosynthesis (Umer et al., 2020a). The gene denoted as WMCS is believed to affect the production and accumulation of citric acid (Umer et al., 2020a). The malate synthase gene Cla97C03G054690 conditions malate synthesis and found to be more highly expressed in a sweet-and-sour watermelon genotype than in sweet watermelon genotype (Umer et al., 2020b). Also, the malate dehydrogenase genes, namely: Cla97C06G125760 and Cla97C05G103110, and transmembrane malate transport gene (Cla97C02G049340), probably influence malate biosynthesis in watermelon (Umer et al., 2020b). Pyruvate kinase genes Cla97C04G076530 and Cla97C11G218660 are reportedly associated with malate breakdown during watermelon fruit ripening (Umer et al., 2020b). Gao et al. (2018a) bred sweet-and-sour watermelon cultivar designed as “SW” through a conventional breeding approach involving the crossing of sweet and citron watermelon genotypes. This finding suggests the possibility of improving organic acid content through targeted mutagenesis using gene-editing technologies such as Targeting Induced Local Lesions in Genomes (TILLING) and through gene editing using the clustered regularly interspaced short palindromic repeats (CRISPR)/CRISPR-associated system 9 (CRISPR/Cas9).
Carotenoids Contents of Sweet Watermelon Fruit
The development of watermelon fruit flesh color is determined by the accumulation of various carotenoids. The fruit flesh exhibits a wide range of colors, including canary yellow, salmon-yellow, orange, red, coral-red, and white (Henderson et al., 1998; Guner and Wehner, 2003, 2004). Lycopene is the major carotenoid present in red- and pink-fleshed watermelon types (Tadmor et al., 2005; Kang et al., 2010). Yellow-fleshed watermelon contains mainly violaxanthin and neoxanthin, whereas orange-fleshed watermelon contains mainly β-carotene (Tadmor et al., 2005; Lewinsohn et al., 2006; Bang et al., 2007, 2010; Liu et al., 2012). White-fleshed watermelons accumulate the colorless phytofluene and light-yellow ζ-carotene (Kang et al., 2010; Grassi et al., 2013). The genetic variation in watermelon fruit flesh color suggests the presence of different mechanisms regulating carotenoid metabolism. Hence, understanding the mechanism of carotenoid inheritance allows for cultivar design with enhanced compositions of phytochemical compounds. The genome-wide comparative expression analysis of the carotenoid biosynthesis and metabolism pathway genes revealed complex gene expression and regulatory networks resulting in various carotenoids accumulation in watermelon fruit (Kang et al., 2010; Guo et al., 2015; Lv et al., 2015; Dou et al., 2017; Fang et al., 2020). Guo et al. (2015) identified a total of 26 genes responsible for carotenoid metabolism in watermelon, of which the phytoene synthase, β-carotene hydroxylase, 9-cis-epoxycarotenoid dioxygenase and carotenoid cleavage dioxygenase genes were the key biosynthesis genes. The phytoene synthase gene Cla009122 is associated with white-flesh color development (Grassi et al., 2013; Guo et al., 2015). A chromoplast phosphate transporter gene ClPHT4;2 (gene ID: Cla017962) plays a dominant role in flesh color formation in watermelon (Zhang et al., 2017). β-carotene hydroxylase genes, namely: Cla006149 and Cla011420 regulate β-carotene concentration. 9-cis-epoxy-carotenoid dioxygenase (NCED) is involved in the catabolic process, which converts 9-cis-violaxanthin or 9-cis-neoxanthin to xanthoxin (Schwartz et al., 2003). NCED genes, namely: Cla005404 and Cla009779, facilitate carotenoid catabolism to initiate the biosynthesis of abscisic acid [ABA] (Li et al., 2012; Guo et al., 2015). Carotenoid cleavage dioxygenase (CCD) catalyses the oxidative cleavage of carotenoids and leads to the production of apocarotenoids (Guo et al., 2015). Apocarotenoids, including β-cyclocitral, β-ionone, geranial, geranyl acetone, theaspirone, α-damascenone and β-damascenone contribute to fruit flavor and aroma. The CCD gene Cla015245 serves as an important link between flesh pigmentation and flavor (Guo et al., 2015). A recent study indicated that the gene Cla018406 is responsible for β-carotene accumulation in orange flesh-colored fruit in watermelon (Wang et al., 2021). Also, the gene Cla007686 was reportedly highly expressed in the red flesh-colored watermelon, serving as a key regulator of lycopene accumulation. The genes designated as Cla018767, Cla018768, Cla018769, Cla018770, and Cla018771 were linked to controlling red-flesh color development in watermelon for being involved in lycopene biosynthesis (Li et al., 2020). The genes Cla003760 and Cla021635 conferred yellow flesh color development (Yuan et al., 2021). Carotenoid metabolic pathway genes namely: ClPSY1 (Cla009122), ClZDS (Cla003751), ClNCED-7 (Cla005404), ClCRTISO (Cla017593), ClCHYB (Cla006149), ClLCYB (Cla005011), ClPDS (Cla010898), ClNCED-1 (Cla009779), and ClCHXE (Cla011420) are involved in the development of white and pale-yellow fruit flesh color of watermelon (Wang et al., 2021). Of these genes, ClPSY, ClLCYB, ClCHYB, ClZEP, and ClNCED regulate the biosynthesis of lycopene, carotene, lutein, zeaxanthin and neoxanthin, respectively (Kang et al., 2010; Lv et al., 2015; Dou et al., 2017; Wang C. et al., 2021). ClPsy1 (gene ID Cla97C01G008760) was recently identified as the major gene conditioning the golden flesh color in watermelon (Liu et al., 2022). Previous studies also identified the lycopene β-cyclase gene ClLCYB (i.e., Cla005011) for being the main gene conditioning the development of red-flesh color in watermelon (Wang et al., 2016, 2019; Li et al., 2020), and in the development of canary yellow flesh-color (Bang et al., 2007, 2014). The down-regulation of ClLCYB (gene ID: Cla97C04G070940) is associated with changes from pale-yellow to red flesh, whereas overexpression of ClLCYB causes color change from red to orange (Zhang et al., 2020b). Also, phytoene synthase gene ClPSY1 promotes phytofluene accumulation, thus influencing the total carotenoid content composition of ripened watermelon fruit (Fang et al., 2020). High contents of phytoene were found to be highly correlated with high β-carotene contents in watermelon (Fang et al., 2020), suggesting that phytoene is a key compound for carotenoid metabolism in watermelon. Plastid lipid-associated genes (i.e., ClPAPs, Cla006670, Cla010946, Cla008831, Cla014416, Cla021506, Cla003468, and Cla003198) are reportedly involved in the formation of plastoglobules, globular and crystalloid chromoplasts (Fang et al., 2020). Watermelon breeders aiming to improve carotenoid profiles should select progenies with genes conditioning the carotenoid biosynthesis pathway.
Volatile Compounds of Sweet Watermelon Fruit
Volatile compounds determine the aroma and flavor of the ripened watermelon fruit (Dima et al., 2014; Fredes et al., 2017; Liu et al., 2018; Bianchi et al., 2020). VOCs are synthesized via the methylerythritol phosphate or mevalonate pathways which result in the accumulation of terpenoids, and C6 and C9 alcohols/aldehydes (ADHs), ethyl acetate, acetaldehyde, tetradecanoic acid and methyl acetate (Pino et al., 2003; Dudareva et al., 2013; Wei et al., 2016). Various VOCs, including monoterpenes, apo-carotenoids, ketones, aldehydes, alcohols, acids, olefins, and furans, accumulate in the watermelon fruit (Lewinsohn et al., 2006; Liu et al., 2012; Bianchi et al., 2020). A recent study by Bianchi et al. (2020) indicated that C-9 aldehydes and alcohols were the prevalent VOCs present in ripened watermelon fruit. VOCs such as 4-oxononanal and 2- hydroxy-, 2- pentyloxy-, 2- hexyloxy-, 2- octyloxy-, 2- nonyloxy-, 2-[(Z)-3- noneyloxy]-, 2-[(Z)-2- nonenyloxy]-, 2-[(Z,Z)-3,6- nonadienyloxy]-, 2-benzyl- oxy-, and 2-phenetyloxy-5-pentyltetrahydrofurans were identified as key flavor influencing compounds in watermelon fruit (Yajima et al., 1985). Also, β-Ionone, dihydroactinodiolide, and β-cyclocitral were found to be prevalent in ripened watermelon fruit (Lewinsohn et al., 2006). VOCs such as 2-pentyl-furan, (E)-2-(2-pentenyl) furan, 2-ethyl-1-hexanol; nonanal, (E)-2-nonenal, nonanol, 2-nonen-1-ol; Nol-3, (Z)-3-nonen-1-ol, 2,6-nonadien-1-ol, (E,Z)-3,6-nonadien-1-ol, nonanoic acid, 1,10-undecadiene; (E)-geranyl acetone; limonene, and β-ionone were reported to be accumulated in watermelon fruit (Liu et al., 2012). VOCs such hexanal, 6-methyl-5-hepten-2-one, (E)-2-octenal, 4-nonenal, (Z)-6-nonenal, Nonanal, (Z)-3-Nonen-1-ol and (E,Z)-2,6-nonadienal, (Z,Z)-3,6-nonadien-1-ol, (E)-2-nonenal and 1-nonanol were identified in seedless watermelon fruit, with 3-nonen-1-ol/(E,Z)-2,6-nonadienal (16.5-28.2%), (E)-2-nonenal (10.6–22.5%), (Z)-6-nonenal (2.0-11.3%) and hexanal (37.7%) being the abundant flavor and aroma enhancing compounds (Beaulieu and Lea, 2006). (Z)-3-nonen-1-ol, (E)-2-nonenal and (Z,Z)-3,6-nonadien-1-ol were also the predominant VOCs in mini-watermelon fruits (Dima et al., 2014). Additionally, (Z)-3-Nonen-1-ol, 2-ethyl-1-hexanol, nonanal, (E,Z)-3,6-nonadien-1-ol, and nonanol were reportedly dominant VOCs accounting for 81–87% in red-fleshed watermelon and 75–79% in yellow and orange-fleshed watermelon (Liu et al., 2012). Off-flavor VOCs were reported in watermelon, including (E)-2-heptenal, decanal, octanol, diisopropyl disulfide, hexanol, (E)-2-decenal, and (E)-2-octenol (Yang et al., 2020).
Sensory evaluation revealed that flavor is a vital fruit quality attribute relating to the refreshing perception of watermelon, an attribute linked with VOCs (Ramirez et al., 2020; Mandha et al., 2021). Comparative transcriptome analysis revealed ADHs genes: Cla97C01G013600, Cla97C05G089700, Cla97C01G001290 Cla97C05G095170, and Cla97C06G118330 enhanced flavor and aroma formation of ripened watermelon fruit (Gong et al., 2021). The reported genes condition the biosynthesis of VOCs 2,6-nonadienal, (E,Z); 2-non-enal, (E)-; 3,6-nonadien-1-ol, (E,Z)-; and 3-nonen-1-ol, (Z)-, respectively (Gong et al., 2021). Previous findings pinpointed that VOCs are main flavor-forming compounds in watermelon (Liu et al., 2012; Dima et al., 2014). Other ADHs genes such as Cla97C09G172570, Cla97C05G092340, Cla97C01G001290, and Cla97C08G152400 and lipoxygenase (LOX) gene (Cla97C06G115570) are reportedly linked to aroma and flavor development of the ripened watermelon fruit (Gong et al., 2021). Understanding the genes involved and its expressions in aroma and flavor development of watermelon fruit is useful in variety development.
Phytohormones of Sweet Watermelon Fruit
Phytohormones such as ethylene and ABA regulate watermelon fruit ripening and tissue degradation, thus influencing fruit quality and shelf life (Zhou et al., 2016; Wang et al., 2017). Transcriptome analysis revealed 22 genes conditioning ethylene biosynthesis and signaling pathway including ACC synthase (ACS), ACC oxidase (ACO), ethylene receptor (ETR), and ethylene-responsive factor (ERF). The genes regulate the production and accumulation of phytohormones in watermelon (Guo et al., 2015). ACS and ACO are key genes regulating ethylene biosynthesis (Guo et al., 2015). The ACS gene denoted as Cla011522 is highly expressed during watermelon fruit development and ripening, making it the most important gene regulating ethylene biosynthesis. The following ACS genes: Cla000483, Cla006245, Cla006634, Cla011230, Cla014057, Cla014652, and Cla022653 showed a relatively low expression levels playing vital roles in ethylene biosynthesis (Guo et al., 2015). ACO genes namely: Cla018620 and Cla018621 are reportedly involved in regulating endogenous ethylene biosynthesis (Guo et al., 2015). ACS (i.e., Cla022653 and Cla011522), and ACO (i.e., Cla014827 and Cla016287) genes induce increased ethylene accumulation in watermelon fruit (Zhou et al., 2016). An ethylene receptor gene Cla021550 with low expression during fruit development and ripening is associated with decreased ethylene biosynthesis (Guo et al., 2015).
Watermelon flesh firmness is influenced by the levels of phytohormones, especially ABA (Wang et al., 2017). ABA concentrations in the flesh of sweet dessert watermelon genotype “97103” and egusi watermelon (C. mucosospermus) line “PI179878” increased during fruit development and ripening, but low concentrations were observed in citron watermelon accession “PI 296341-FR” (Wang et al., 2017). Reduced ABA concentrations is believed to be associated with hard-flesh, reduced tissue breakdown and extended shelf and storage life in watermelon. The following genes are involved in ABA biosynthesis in watermelon: Cla009779 (NCED), Cla005404 (NCED), Cla020673 (CYP707A), Cla006655 (UGT), and Cla020180 (SnRK2) (Wang et al., 2017). Of these, Cla009779, Cla005404, and Cla005457 enhanced ABA accumulation. A recent report indicated that the genes Cla016033 and Cla012507 controlled ABA biosynthesis in watermelon (Sun et al., 2020). The high correlation between ABA and total sugar content suggests the involvement of ABA in regulating watermelon fruit quality via sugar metabolism (Wang et al., 2017).
Fruit Flesh Texture of Sweet Watermelon Fruit
Fruit flesh texture properties, especially flesh firmness, influence sensory quality and affect taste, flavor, and the shelf life of ripened watermelon fruit (Gao et al., 2020; Sun et al., 2020). A hard flesh results in reduced juice and poor flavor, whereas a soft flesh shows poor eating quality, storage and reduced shelf life (Gao et al., 2020). Changes in flesh firmness are mainly attributed to changes in fruit flesh structural components. Cellulose, pectin and hemicellulose are the main components of the cell wall, which largely determine the firmness and sensory qualities of watermelon fruit flesh (Guo et al., 2015; Gao et al., 2020; Sun et al., 2020; Anees et al., 2021). The catabolism of fruit flesh structural components (i.e., pectin, cellulose, and hemicellulose) cause watermelon fruit softening (Gao et al., 2020). Comparative transcriptome analysis revealed the gene Cla016033 influencing the components of the cell wall and flesh firmness. Also, the gene designated as Cla012507 is responsible for hard flesh texture of watermelon fruit (Gao et al., 2020). Transcriptome analysis based on citron watermelon accession “PI186490” and sweet watermelon ‘Sanbai’ revealed 85 genes including 10 pectinesterase genes, 2 polygalacturonase genes, 4 pectate lyase genes, 10 glycosyltransferase genes, 9 cellulose synthase genes, 8 β-galactosidase genes, 9 xyloglucan transferase genes, and 2 lipoxygenase genes were up-regulated. The listed genes influence flesh texture by strengthening the cell wall structure of watermelon fruit (Gao et al., 2020). Cla11211, Cla10077, Cla14927, Cla18814, Cla12351, and Cla002537 were highly expressed during ripening, enhancing watermelon flesh textural properties (Gao et al., 2020). Peroxidases (i.e., Cla003188, Cla003189, Cla014013, Cla019382, and Cla003187), transcription factors genes (i.e., Cla009283, Cla012465, Cla015515, Cla020969, Cla022083, Cla013612, Cla016224, Cla009263, Cla013099, Cla007307, Cla007656, and Cla018059) and phytohormone biosynthesis genes (i.e., Cla006604, Cla004102, Cla014808, Cla019806, Cla020976, and Cla021550) strengthened the cell wall structure of the ripe watermelon fruit (Gao et al., 2020). Gene expression analysis between watermelon hard flesh firmness lines (HWF) and soft flesh firmness lines provided three main gene networks, namely water-soluble pectin, cellulose, hemicellulose, and protopectin, responsible in the structural integrity of watermelon fruit (Anees et al., 2021). The genes Cla012351 (Cellulose synthase) and Cla004251 (Pectinesterase) influence cellulose and protopectin contents, whereas Cla004120, Cla009966 (Cellulose synthase), and Cla006648 (Galactosyltransferase) enhanced the accumulation of water-soluble pectin, cellulose, and protopectin in the watermelon flesh. Also, the following genes: Cla007092, Cla004119, and Cla018816, influence the composition of hemicellulose, cellulose, and protopectin, respectively (Anees et al., 2021). Ethylene accumulation influence fruit softening in watermelon, making the fruit susceptible to cracking and rotting. Ethylene-responsive transcription factor 4 gene ClERF4 conditioned hard watermelon rind (Liao et al., 2020). The textural quality of watermelon fruit is also influenced by rind hardness/firmness (Yang et al., 2021). Genes conditioning rind hardness are not co-localized with genes involved in fruit cracking (Sun et al., 2020). The following genes: MDP, DHDDS, POD1, and GST are involved in reduced fruit cracking, whereas CHDH, GPAT, POD2, SMT, KCS, XET1, XET2, and DHCR24 promote watermelon fruit cracking (Jiang et al., 2019). Genes influencing rind hardness and fruit cracking should be integrated in watermelon breeding to improve quality and shelf life of ripened watermelon fruit.
Molecular Markers and Quantitative Trait Loci Analysis of Phytochemical Compounds, Phytohormones and Fruit Flesh-Structural Compounds in Sweet Watermelon
Molecular Markers Reported in Genetic Analysis of Watermelon
Molecular markers are complementary tools to phenotyping, allowing accelerated cultivar development and deployment. Molecular markers such as simple sequence repeat (SSR) or microsatellites, insertion-deletion (InDel), single nucleotide polymorphism (SNP), kompetitive allele-specific polymorphism (KASP) and cleaved amplified polymorphic sequence (CAPS) have been developed to genotype watermelon populations for fruit quality breeding (Liu et al., 2015; Jin et al., 2019; Subburaj et al., 2019; Li et al., 2020). For example, InDel markers InDel27_fc6 and InDel28_fc6 were developed for marker-assisted selection for scarlet red flesh color (Li et al., 2020). A SNP marker linked to the lycopene b-cyclase gene (LCYB) has been developed for genotype selection (Bang et al., 2007). Kompetitive allele-specific polymorphism markers co-segregating with pale flesh color phenotype have been developed for genetic analysis of watermelon (Pei et al., 2021). The KASP marker (chr06_7040350) effectively distinguished white and pale-yellow fleshed watermelons useful for marker-assisted breeding (Wang et al., 2017). A cleaved amplified polymorphic sequence marker (CAPS-C1976) was developed for distinguishing yellow and orange-fleshed watermelon genotypes (Jin et al., 2019). A CAPS marker was also developed to effectively select watermelon with canary yellow and red fruit colors (Bang et al., 2007). Simple sequence repeat marker (BVWS00954) was identified on chromosome 6 conditioning watermelon flesh firmness (Gao et al., 2016). There are limited molecular markers developed and reported for fruit quality traits in sweet watermelon genetic resources. It is essential to develop diagnostic molecular markers to aid efficient screening and selection of desirable parents for fruit quality improvement and complement phenotypic selection.
Quantitative Trait Loci Analysis of Sweet Watermelon Fruit Quality
Next-generation sequencing (NGS) technologies have allowed genome sequencing and identification of QTL associated with watermelon fruit quality and other important agronomic and horticultural traits (Table 1). Sugar transporter genes Cla015835 and Cla015836 are located at the region of the major sugar content QTL Qbrix2-2 on chromosome 2 in watermelon (Ren et al., 2014). Two QTL (Qbrix2-1 and Qsuc2-1) linked to sucrose content were mapped on chromosome 2 (Ren et al., 2014). Fructose QTL Qfru2-1 and Qfru2-2 are co-localized with QTL associated with total sugar content, namely: Qbrix2-1 and Qbrix2-2 (Ren et al., 2014). The glucose QTL Qglu6 was co-localized with the QTL conditioning fructose content (Ren et al., 2014). Two QTL controlling sucrose content, namely: Qsur2-1 and Qsur2-2 were mapped on chromosomal 2 linked with brix influencing QTL Qbrix2-1 and Qbrix2-2 and influencing fructose levels such as QTLs Qfru2-1 and Qfru2-2 (Ren et al., 2014). Two QTL, Qsur5-2 and Qsur8-1 localized on chromosomes 5 and 8, respectively, influence sucrose content in watermelon (Fall et al., 2019). Cheng et al. (2016) identified 4 QTL linked to sugar content in watermelon (Table 1). The following genes: Cla021522 (phosphate permease), Cla021325 (pectinesterase), Cla021640, Cla021693 (membrane transporters), Cla021639, Cla021641, Cla021642, Cla021643 (sugar/inositol transporters), Cla021807 (galactokinase), and Cla021809 (neutral invertase) condition sugar biosynthesis. These genes were located on chromosome 5 (Guo et al., 2012; Zhu et al., 2017; Fall et al., 2019). A QTL affecting sugar content (QBRX2-1) correlated with a tonoplast sugar transporter 2 ClTST2 and mapped on chromosome 2 (Ren et al., 2018). Major QTL affecting citrulline biosynthesis are localized on chromosomes 1, 8, and 11 of watermelon (Fall et al., 2019). The reported genes involved were Cla022154 (Argininosuccinate lyase), Cla022273 (Nacetylglutamate Kinase), and Cla022392 (Ornithine decarboxylase). These genes were located on chromosome 8, while the additional gene Cla006553 (Pyrroline-5-carboxylate synthase) was mapped on chromosome 11 (Fall et al., 2019). QTL conditioning arginine was located on chromosomes 2 and 5 of watermelon (Fall et al., 2019). The arginine candidate genes Cla020511, Cla020512, and Cla020781 (Ornithine carbamoyltransferase) were localized on chromosome 5 (Fall et al., 2019). A QTL LCYB4.1 related to lycopene content was mapped on chromosome 4 (Liu et al., 2015). Recently, the genomic region Yscr associated with the scarlet-red flesh color was mapped on chromosome 6 of watermelon (Li et al., 2020). A major QTL (qFC.1) linked to β-carotene in watermelon was identified on chromosome 1 (Branham et al., 2017). Also, the β-carotene hydroxylase CHYB gene (gene ID: Cla011420) was mapped on chromosome 1 (Jin et al., 2019). The QTL qfc10.1 located on chromosome 10 provides the pale flesh color of watermelon (Pei et al., 2021). Carotenoid biosynthesis enzymes carotenoid isomerase gene (CRTISO; gene ID: Cla017593) is located on chromosome 10 (Jin et al., 2019). Primary genes controlling watermelon flesh hardness are located on chromosomes 2 and 8 (Sun et al., 2020). Chromosome 10 harbor QTL associated with rind hardness (Yang et al., 2021). QTL associated with rind hardness was mapped to chromosome 4 of watermelon (Hashizume et al., 2003).
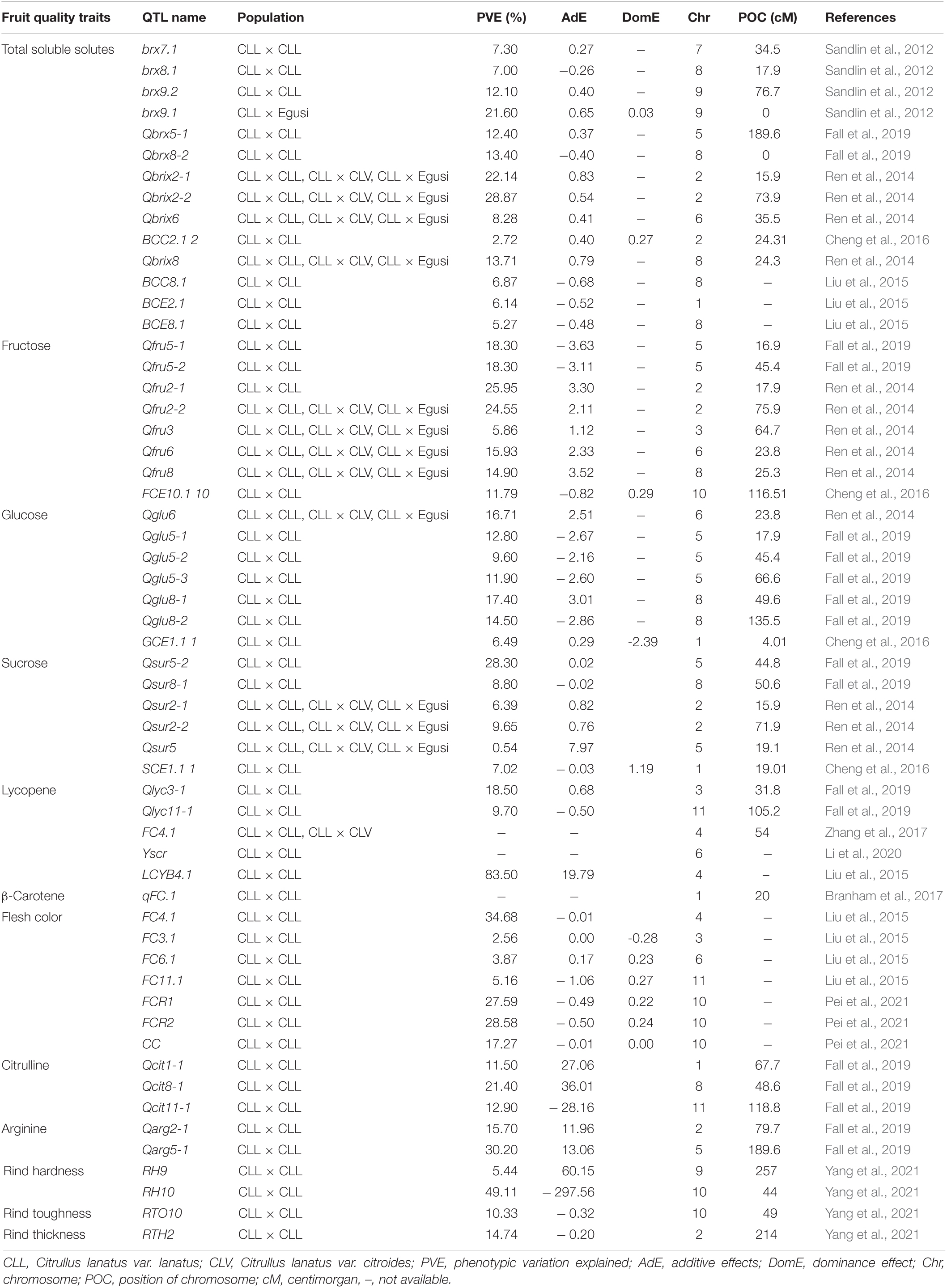
Table 1. Reported quantitative trait loci (QTLs) associated with fruit quality traits in watermelon.
Quantitative trait loci conditioning organic acids, VOCs, flesh and cell-wall structural compounds are yet to be discovered and mapped in watermelon. NGS technology combined with sequencing-based gene mining strategies such as bulk segregant analysis (BSA), bulked segregant analysis sequencing (BSA-seq), and genome-wide association study (GWAS) have been successfully used in gene discovery associated with agronomic and horticultural traits in watermelon (Wei et al., 2017; Dong et al., 2018, 2021; Ren et al., 2018; Zhu et al., 2019; Gimode et al., 2020; Cho et al., 2021; Liu et al., 2021; Wang C. et al., 2021; Wang et al., 2022). These technologies are useful for mining QTL linked to organic acids, VOCs, flesh and cell-wall structural compounds in watermelon. QTL mapping is based on whole-genome re-sequencing of DNA bulk samples of F2 generation derived from watermelon genotypes with the contrasting expression of organic acids, VOCs and cell-wall structural compounds. Genome-wide and bulk segregant analysis analyses can then be applied to detect genomic regions harboring genes associated with these compounds in watermelon. These will also facilitate the development of molecular markers for marker-assisted selection for developing watermelon genotypes with excellent fruit quality attributes. Overall, QTL linked to fruit quality traits provides opportunities for marker-assisted selection to breed watermelon genotypes with desired end-user quality attributes required by growers, consumers, and the industry.
Gene Editing for Fruit Quality Traits in Sweet Watermelon
The genetic regulation of watermelon fruit quality has been unraveled through comparative transcriptome analysis involving comparative cultivars (Guo et al., 2012; Grassi et al., 2013; Gao et al., 2020; Sun et al., 2020). Previous studies revealed a complex gene network influencing watermelon fruit quality. Conventional breeding approaches have been widely used in watermelon breeding programs to develop cultivars with desirable fruit quality traits. For example, a watermelon cultivar “SW” with high total soluble sugar and organic acid contents was developed by crossing the sweet watermelon inbred line “203Z” and citron watermelon accession “PI 271769-FR” (Gao et al., 2018a). Sweet watermelon breeding lines “USVL-370” and “USVL-380” with low total soluble solutes (TSS) were developed from an F2 population through crosses of the citron watermelon accession PI 595203 and C. lanatus var. lanatus variety “New Hampshire Midget” (Levi et al., 2016; Levi and Ling, 2017). USVL-220 with TSS ranging from 8.5 to 11.0% was selected from an F2 population derived from a cross involving F1 hybrid sweet watermelon cultivar “New Hampshire Midget” × “Griffin 14113” (C. lanatus var. citroides) with C. colocynthis accession “PI386015” (Levi et al., 2011). In some instances, the development of sweet watermelon cultivars with desirable fruit quality attributes were derived from uncontrolled pollination. For example, a sweet watermelon cultivar “MSW-28” exhibiting medium sugar content, high lycopene content, and crisp flesh texture was selected from an F6 population derived from half-sib families of different varieties of commercial sweet watermelon and citron watermelon lines (Davis and King, 2007). Also, watermelon cultivars “LSW-177” and “LSW-194” with low fruit TSS content and firm texture and intense yellow flesh color were selected from the crosses of C. lanatus var. lanatus and C. lanatus var. citroides accessions (Davis et al., 2008).
Sweet dessert watermelon has a narrow genetic base attributed to many years of domestication and selection by growers for fruit quality traits such as red-scarlet flesh color and sweetness (Levi et al., 2001b; Hwang et al., 2011; Wu et al., 2019). These, in turn have resulted in limited genetic improvement of fruit quality attributes in modern cultivars. Genome editing has the potential to develop watermelon genotypes with desired fruit quality attributes (Tian et al., 2017). The clustered regularly interspaced short palindromic repeats (CRISPR)/CRISPR-associated system 9 (CRISPR/Cas9) is the widely used genome editing platform allowing for substantial improvement of economic traits. CRISPR/Cas9 was used for targeted mutagenesis of the phytoene desaturase gene CIPDS, which confers the albino phenotype in watermelon. CRISPR/Cas9 was used to induce mutations on the ClPDS gene sequences ATGCCGCTAGAGTGGTGCC and CGAGATGCTGTTCTCCATT. Transgenic watermelon plants developed using the technology harbored the ClPDS mutations and expressed the albino phenotype (Tian et al., 2017). CRISPR/Cas9 system was successfully employed to knockout the CLPSK1 gene, encoding the PSK precursor, to confer enhanced resistance to Fusarium oxysporum f.sp. niveum in watermelon (Zhang et al., 2020b). Gynoecious (i.e., female flowers only) watermelon lines were derived using CRISPR/Cas9 system by targeted mutations of the gene sequences CTACTACTGGACTTAATAAGG and CCTTTGGACATGGCCATGCAGC (i.e., ATG and TGA arms, respectively) associated with the ClWIP1 (gene ID Cla008537) (Zhang et al., 2020b). Also, CRISPR/Cas9-mediated mutagenesis of the ClBG1 gene sequences (i.e., GAGGAAGATTCGCCACCGTTGG and CCGAGCGGAATTTGTTTTCGGAA) influencing size resulted in decreased watermelon seed size of transgenic plants (Wang Y. et al., 2021). Targeted mutagenesis of the watermelon acetolactate synthase (ALS) gene designated as ClALS (gene ID Cla019277) involved in the biosynthesis of the amino acidsvaline, leucine and isoleucine aided in the development of herbicide-resistance lines (Tian et al., 2018). This was achieved by CRISPR/Cas9-editing of the sequence GAAGTTCCGAGAAGAATGAT harboring the ClALS gene. Recently, Ren et al. (2021) reported that knocking out of sugar transporter genes ClAGA2, ClSWEET3, and ClTST2 affected fruit sugar accumulation in watermelon. These reports indicate that CRISPR/Cas9 may offer opportunities for effective genome editing to develop watermelon genotypes with desired fruit quality attributes. Watermelon genotypes with desired fruit quality attributes can be developed through QTL analysis influencing the contents of sugars, lycopene, β-carotene, citrulline and arginine biosynthesis. These QTLs could be targeted for CRISPR/Cas9-mediated gene-editing to create a genetic variation for the selection and advancement of new progenies. Recently, editing of sucrose transporter gene ClVST1 using CRISPR/Cas9 gene editing platform resulted in the development of watermelon lines with decreased sugar content, whereas overexpression of ClVST1 increased sucrose content in the mutant lines (Ren et al., 2020). The development of an efficient CRISPR/Cas9 protocol is required for effective mutagenesis to improve watermelon fruit quality traits to facilitate product development for commercialization.
Utilization of Citron Watermelon for Gene Discovery Associated With Fruit Quality
Citron watermelon belongs to the family Cucurbitaceae, a closely related species of sweet watermelon (Chomicki and Renner, 2015; Renner et al., 2017). Both species display similar phenotypic traits, including growth habit, flowering habit (i.e., monoecious) and fruit traits (i.e., fruit shape and rind stripe patterns). Flesh colors are similar in both species (i.e., white, yellow, and orange) except the deep red flesh color absent in citron watermelon (Levi et al., 2012; Ngwepe et al., 2019, 2021). Regarding flesh texture, sweet watermelon has a relatively soft flesh (1.0–1.8 kg/cm2) compared to citron watermelon, which has a medium-hard and crispy flesh (∼6.00 kg/cm2) texture (Fredes et al., 2017; García-Mendívil et al., 2019; Anees et al., 2021). This is attributed to the low expression levels of flesh texture related genes during fruit development (Guo et al., 2015). The mechanisms of fruit ripening at physiological and molecular levels differ between the two species (Guo et al., 2011, 2015; Zhou et al., 2016; Wang et al., 2017). Comparative transcriptome analysis between C. lanatus var. lanatus and C. lanatus var. citroides provided insights on genetic regulation of watermelon fruit quality development (Ren et al., 2014, 2018, 2020; Guo et al., 2015; Wang et al., 2017). However, most transcriptome studies have utilized the white-fleshed citron watermelon line PI 296341-FR for comparisons (Sandlin et al., 2012; Ren et al., 2014, 2018, 2020; Guo et al., 2015; Zhou et al., 2016; Wang et al., 2017; Zhang et al., 2017, 2020b; Anees et al., 2021). White-fleshed citron watermelon is non-sweet type because of accumulating low concentrations of sugars. Also, these genotypes show no changes in flesh color development during ripening (Ren et al., 2014, 2018; Guo et al., 2015; Anees et al., 2021) attributed to the low expression of genes involved in sugar and carotenoid metabolism (Guo et al., 2015).
There is limited comparative transcriptome analysis involving yellow and orange-fleshed citron watermelon genotypes. Compared to white-fleshed types, yellow and orange-fleshed types show extensive variations in flesh color development at various fruit developmental stages attributable to changes in carotenoid metabolism. The ripened fruit is slightly sweet- and- sour (TSS ∼4.0) (Ngwepe et al., 2021), probably attributable to high expression levels of genes involved in the biosynthesis of organic acids and sugars (Umer et al., 2020a). Also, the ripened citron watermelon fruit have a longer shelf life of up to 12 months under room temperature and up to 6 months in the open field (Personal observation). The extended shelf life of citron watermelon may be linked to low expression of ethylene and ABA biosynthesis genes leading to low accumulation of these phytohormones, and the high contents of cellulose, pectin and hemicellulose attributed to the high expression levels genes involved in cell-wall structural components (Guo et al., 2015; Zhou et al., 2016; Sun et al., 2020; Anees et al., 2021). These probably leads to the improved structural integrity of the rind and flesh, and low flesh degradation resulting in slow tissue breakdown increasing flesh firmness and extended shelf life (Sun et al., 2020; Anees et al., 2021). Therefore, comparative transcriptome analysis of gene expression involved in the regulation of fruit quality may reveal novel genes involved in watermelon fruit quality development. Therefore, we propose comparative transcriptome analysis as follows: (1) comparisons of yellow vs. orange-fleshed genotypes of C. lanatus var. lanatus and C. lanatus var. citroides, (2) yellow vs. orange-fleshed genotypes of C. lanatus var. citroides and (3) white vs. yellow vs. orange-fleshed genotypes of C. lanatus var. lanatus and C. lanatus var. citroides. These may reveal new insights on genetic regulation of watermelon fruit ripening and lead to molecular breeding strategies to improve sugars, carotenoids, organic acids, amino acid contents, and flavor and aroma (i.e., VOCs) and shelf-life of watermelon. These will result in new cultivar and product development to serve the diverse markets of the crop.
Perspective
Watermelon fruit and flesh quality are affected by the composition of phytochemical compounds, phytohormones, and fruit flesh firmness which are affected by genes, the growing environment and their interaction. These attributes determine fruit ripening, eating quality, and postharvest shelf-life. For accelerated cultivar and product development to serve the changing needs of end-users, genes associated with fruit quality can be targeted for mutagenesis using gene editing platforms. Comparative transcriptome studies using yellow and orange-fleshed citron watermelon genetic resources is recommended to improve our understanding of genes involved in ripening and fruit quality development of watermelon fruit. Studies on QTL mapping and molecular marker development linked to organic acids, VOCs and fruit flesh structural components is proposed for marker-assisted breeding for accelerated product development, release and commercialization.
Author Contributions
All authors listed have made a substantial, direct, and intellectual contribution to the work, and approved it for publication.
Funding
The University of KwaZulu-Natal (UKZN) and the Limpopo Department of Agriculture and Rural Development are acknowledged for financial support of this study.
Conflict of Interest
The authors declare that the research was conducted in the absence of any commercial or financial relationships that could be construed as a potential conflict of interest.
Publisher’s Note
All claims expressed in this article are solely those of the authors and do not necessarily represent those of their affiliated organizations, or those of the publisher, the editors and the reviewers. Any product that may be evaluated in this article, or claim that may be made by its manufacturer, is not guaranteed or endorsed by the publisher.
References
Anees, M., Gao, L., Umer, M. J., Yuan, P., Zhu, H., Lu, X., et al. (2021). Identification of key gene networks associated with cell wall components leading to flesh firmness in watermelon. Front. Plant Sci. 12:243. doi: 10.3389/fpls.2021.630243
Bahri, S., Zerrouk, N., Aussel, C., Moinard, C., Crenn, P., Curis, E., et al. (2013). Citrulline: from metabolism to therapeutic use. Nutr. J. 29, 479–484. doi: 10.1016/j.nut.2012.07.002
Bang, H., Davis, A. R., Kim, S., Leskovar, D. I., and King, S. R. (2010). Flesh color inheritance and gene interactions among canary yellow, pale yellow, and red watermelon. J. Am. Soc. Hortic. Sci. 135, 362–368. doi: 10.21273/jashs.135.4.362
Bang, H., Kim, S., Leskovar, D., and King, S. (2007). Development of a codominant CAPS marker for allelic selection between canary yellow and red watermelon based on SNP in lycopene b-cyclase (LCYB) gene. Mol. Breed. 20, 63–72.
Bang, H., Yi, G., Kim, S., Leskovar, D., and Patil, B. S. (2014). Watermelon lycopene β-cyclase: promoter characterisation leads to the development of a PCR marker for allelic selection. Euphytica 200, 363–378.
Beaulieu, J. C., and Lea, J. M. (2006). Characterization and semiquantitative analysis of volatiles in seedless watermelon varieties using solid-phase microextraction. J. Agric. Food Chem. 54, 7789–7793. doi: 10.1021/jf060663l
Bianchi, G., Provenzi, L., and Rizzolo, A. (2020). Evolution of volatile compounds in ‘Cuoredolce®’ and ‘Rugby’ mini- watermelons (Citrullus lanatus (Thunb.) Matsumura and Nakai) in relation to ripening at harvest. J. Sci. Food Agric. 100, 945–952. doi: 10.1002/jsfa.10023
Branham, S. E., Vexler, L., Meir, A., Tzuri, G., Frieman, Z., Levi, A., et al. (2017). Genetic mapping of a major codominant QTL associated with β-carotene accumulation in watermelon. Mol. Breed. 37:146.
Che, K., Liang, C., Wang, Y., Jin, D., Wang, B., Xu, Y., et al. (2003). Genetic Assessment of Watermelon Germplasm using the AFLP Technique. Sci. Hortic. 38, 81–84.
Cheng, Y., Luan, F., Wang, X., Gao, P., Zhu, Z., Liu, S., et al. (2016). Construction of a genetic linkage map of watermelon (Citrullus lanatus) using CAPS and SSR markers and QTL analysis for fruit quality traits. Sci. Hortic. 202, 25–31. doi: 10.1016/j.scienta.2016.01.004
Cho, Y., Lee, S., and Park, J. (2021). Identification of a candidate gene controlling semi-dwarfism in watermelon, Citrullus lanatus, using a combination of genetic linkage mapping and QTL-seq. Hortic. Environ. Biotechnol. 62, 447–459. doi: 10.1007/s13580-020-00330-x
Chomicki, G., and Renner, S. S. (2015). Watermelon origin solved with molecular phylogenetics including Linnaean material: another example of museomics. New Phytol. 205, 526–532. doi: 10.1111/nph.13163
Dane, F., and Lang, P. (2004). Sequence variation at cpDNA regions of watermelons and related wild species: implications for evolution of Citrullus haplotypes. Am. J. Bot. 91, 1922–1929. doi: 10.3732/ajb.91.11.1922
Davis, A., Perkins-Veazie, P., Collins, J., and Levi, A. (2008). LSW-177 and LSW-194: Red-fleshed watermelon lines with low total soluble solids. J. Am. Soc. Hortic. Sci. 43, 538–539. doi: 10.21273/hortsci.43.2.538
Davis, A. R., and King, S. R. (2007). MSW-28, a full-flavor crisp watermelon line with high lycopene and medium brix. J. Am. Soc. Hortic. Sci. 42, 1715–1716.
Davis, A. R., Webber, C. L., Fish, W. W., Wehner, T. C., King, S., and Perkins-Veazie, P. (2011). L-Citrulline levels in watermelon cultigens tested in two environments. J. Am. Soc. Hortic. Sci. 46, 1572–1575. doi: 10.21273/hortsci.46.12.1572
Dima, G., Tripodi, G., Condurso, C., and Verzera, A. (2014). Volatile constituents of mini-watermelon fruits. Essent. Oil Res. 26, 323-327. doi: 10.1080/10412905.2014.933449
Dong, W., Dewei, W., Chen, Y., and Defeng, W. (2021). Mapping and analysis of a novel genic male Sterility Gene in Watermelon (Citrullus lanatus). Front. Plant Sci. 12:611. doi: 10.3389/fpls.2021.639431
Dong, W., Wu, D., Li, G., Wu, D., and Wang, Z. (2018). Next-generation sequencing from bulked segregant analysis identifies a dwarfism gene in watermelon. Sci. Rep. 8:2908. doi: 10.1038/s41598-018-21293-1
Dou, J., Yuan, P., Zhao, S., He, N., Zhu, H., Gao, L., et al. (2017). Effect of ploidy level on expression of lycopene biosynthesis genes and accumulation of phytohormones during watermelon (Citrullus lanatus) fruit development and ripening. J. Integr. Agric. 16, 1956–1967. doi: 10.1016/s2095-3119(16)61618-0
Dudareva, N., Klempien, A., Muhlemann, J. K., and Kaplan, I. (2013). Biosynthesis, function and metabolic engineering of plant volatile organic compounds. New Phytol. 198, 16–32. doi: 10.1111/nph.12145
Fall, L. A., Perkins-Veazie, P., and Ma, G. (2019). QTLs associated with flesh quality traits in an elite × elite watermelon population. Euphytica 215:30. doi: 10.1007/s10681-019-2356-y
Fang, X., Liu, S., Gao, P., Liu, H., Wang, X., Luan, F., et al. (2020). Expression of CLPAP and CLPSY1 in watermelon correlates with chromoplast differentiation, carotenoid accumulation, and flesh color formation. Sci. Hortic. 270, 10943.
FAOSTAT (2020). Available online at: http://www.fao.org/faostat/en/#data/QC (accessed January 10, 2022).
Fredes, A., Roselló, S., Beltrán, J., Cebolla-Cornejo, J., Pérez-de-Castro, A., and Gisbert, C. (2017). Fruit quality assessment of watermelons grafted onto citron melon rootstock. J. Sci. Food Agric. 97, 1646–1655. doi: 10.1002/jsfa.7915
Gao, L., Zhao, S., Lu, X., He, N., and Liu, W. (2018a). ‘SW’, a new watermelon cultivar with a sweet and sour flavor. J. Am. Soc. Hortic. Sci. 53, 895–896. doi: 10.21273/hortsci12857-18
Gao, L., Zhao, S., Lu, X., He, N., and Zhu, H. (2018b). Comparative transcriptome analysis reveals key genes potentially related to soluble sugar and organic acid accumulation in watermelon. PLoS One 13:e0190096. doi: 10.1371/journal.pone.0190096
Gao, L., Zhao, S. J., Lu, X. Q., He, N., Zhu, H. J., and Dou, J. L. (2016). Linkage analysis on flesh firmness of watermelon [Citrullus lanatus (Thunb.) Mansf.] by SSR molecular marker. J. Plant Genet. Resour. 17, 866–870.
Gao, Y., Guo, Y., Su, Z., Yu, Y., Zhu, Z., Gao, P., et al. (2020). Transcriptome analysis of genes related to fruit texture in watermelon. Sci. Hortic. 262:109075. doi: 10.1016/j.scienta.2019.109075
García-Mendívil, H. A., Munera, M., Giné, A., Escudero, N., Picó, M. B., Gisbert, C., et al. (2019). Response of two Citrullus amarus accessions to isolates of three species of Meloidogyne and their graft compatibility with watermelon. J. Crop Prot. 119, 208–213. doi: 10.1016/j.cropro.2019.02.005
Gimode, W., Clevenger, J., and McGregor, C. (2020). Fine-mapping of a major quantitative trait locus Qdff3-1 controlling flowering time in watermelon. Mol. Breed. 40:3. doi: 10.1007/s11032-019-1087-z
Gong, C., Diao, W., Zhu, H., Umer, M. J., Zhao, S., He, N., et al. (2021). Metabolome and transcriptome integration reveals insights into flavor formation of ‘crimson’ watermelon flesh during fruit development. Front. Plant Sci. 12:704. doi: 10.3389/fpls.2021.629361
Grassi, S., Piro, G., Lee, J. M., Zheng, Y., Fei, Z., Dalessandro, G., et al. (2013). Comparative genomics reveals candidate carotenoid pathway regulators of ripening watermelon fruit. BMC Genomics 14:781. doi: 10.1186/1471-2164-14-781
Guner, N., and Wehner, T. C. (2003). Gene list for watermelon. Cucurbit Genet. Coop. Rep. 26, 76–92.
Guner, N., and Wehner, T. C. (2004). The genes of watermelon. J. Am. Soc. Hortic. Sci. 6, 1175-1182.
Guo, S., Liu, J., Zheng, Y., Huang, M., Zhang, H., Gong, G., et al. (2011). Characterisation of transcriptome dynamics during watermelon fruit development: sequencing, assembly, annotation and gene expression profiles. BMC Genomics 12:454. doi: 10.1186/1471-2164-12-454
Guo, S., Sun, H., Zhang, H., Liu, J., Ren, Y., Gong, G., et al. (2015). Comparative transcriptome analysis of cultivated and wild watermelon during fruit development. PLoS One 10:e0130267. doi: 10.1371/journal.pone.0130267
Guo, S., Zhang, J., Sun, H., Salse, J., Lucas, W. J., Zhang, H., et al. (2012). The draft genome of watermelon (Citrullus lanatus) and resequencing of 20 diverse accessions. Nat. Genet. 45, 51–58. doi: 10.1038/ng.2470
Hashizume, T., Shimamoto, I., and Hirai, M. (2003). Construction of a linkage map and QTL analysis of horticultural traits for watermelon [Citrullus lanatus (THUNB.) MATSUM & NAKAI] using RAPD, RFLP and ISSR markers. Theor. Appl. Genet. 106, 779–785.
Henderson, W. R., Scott, G. H., and Wehner, T. C. (1998). Interaction of flesh colour genes in watermelon. J. Hered. 89, 50–53. doi: 10.1093/abbs/gmw132
Hwang, J. H., Ahn, S. G., Oh, J. Y., Choi, Y. W., Kang, J. S., and Park, Y. H. (2011). Functional characterisation of watermelon (Citrullus lanatus L.) EST–SSR by gel electrophoresis and high resolution melting analysis. Sci. Hortic. 130, 715–724. doi: 10.1016/j.scienta.2011.08.014
Jawad, U. M., Gao, L., Gebremeskel, H., Safdar, L. B., Yuan, P., Zhao, S., et al. (2020). Expression pattern of sugars and organic acids regulatory genes during watermelon fruit development. Sci. Hortic. 265:109102. doi: 10.1016/j.scienta.2019.109102
Jiang, H., Tian, H., Yan, C., Jia, L., Wang, Y., Wang, M., et al. (2019). RNA-seq analysis of watermelon (Citrullus lanatus) to identify genes involved in fruit cracking. Sci. Hortic. 248, 248–255.
Jin, B., Lee, J., and Kweon, S. (2019). Analysis of flesh color-related carotenoids and development of a CRTISO gene-based DNA marker for prolycopene accumulation in watermelon. Hortic. Environ. Biotechnol. 60, 399–410. doi: 10.1007/s13580-019-00139-3
Joshi, V., Joshi, M., Silwal, D., Noonan, K., Rodriguez, S., and Penalos, A. (2019). Systematised biosynthesis and catabolism regulate citrulline accumulation in watermelon. Phytochemistry 162, 129–140. doi: 10.1016/j.phytochem.2019.03.003
Kang, B., Zhao, W., Hou, Y., and Tian, P. (2010). Expression of carotenogenic genes during the development and ripening of watermelon fruit. Sci. Hortic. 124, 368–375.
Kwon, Y. S., Oh, Y. H., and Yi, S. I. (2010). Informative SSR markers for commercial variety discrimination in watermelon (Citrullus lanatus). Genes Genomics 32, 115–122. doi: 10.1007/s13258-008-0674-x
Levi, A., Harris-Shultz, K. R., and Ling, K. (2016). USVL-370, a Zucchini yellow mosaic virus–resistant watermelon breeding line. J. Am. Soc. Hortic. Sci. 51, 107–109. doi: 10.21273/hortsci.51.1.107
Levi, A., and Ling, K. (2017). USVL-380, a Zucchini yellow mosaic virus-resistant watermelon breeding line. J. Am. Soc. Hortic. Sci. 52, 1448–1450. doi: 10.21273/hortsci12292-17
Levi, A., Thies, J. A., Simmons, A. M., Harrison, H., Hassell, R., and Keinath, A. (2011). USVL-220, a novel watermelon breeding line. J. Am. Soc. Hortic. Sci. 46, 135–138. doi: 10.1093/jhered/esj023
Levi, A., Thies, J. A., Wechter, W. P., Harrison, H. F. J., Simmons, A. M., Reddy, U., et al. (2012). High frequency oligonucleotides-Targeting active gene (HFO-TAG) markers reveal wide genetic diversity among Citrullus spp. accessions useful for enhancing disease or pest resistance in watermelon cultivars. Genet. Resour. Crop Evol. 60, 427–440.
Levi, A., Thomas, C. E., Keinath, A. P., and Wehner, T. C. (2001b). Genetic diversity among watermelon (Citrullus lanatus and Citrullus colocynthis) accessions. Genet. Resour. Crop. Evol. 48, 559–566. doi: 10.1023/A:1013888418442
Levi, A., Thomas, C. E., Newman, M., Reddy, O. K., Zhang, X., and Xu, Y. (2004). ISSR and AFLP markers differ among American watermelon cultivars with limited genetic diversity. J. Am. Soc. Hortic. Sci. 129, 553–558. doi: 10.21273/jashs.129.4.0553
Levi, A., Thomas, C. E., Wehner, T. C., and Zhang, X. (2001a). Low genetic diversity indicates the need to broaden the genetic base of cultivated watermelon. J. Am. Soc. Hortic. Sci. 36, 1096–1101. doi: 10.21273/hortsci.36.6.1096
Lewinsohn, E., Sitrit, Y., Bar, E., Azulay, Y., Meir, A., Zamir, D., et al. (2006). Carotenoid pigmentation affects the volatile composition of tomato and watermelon fruits, as revealed by comparative genetic analyses. J. Agric. Food Chem. 53, 3142–3148. doi: 10.1021/jf047927t
Li, N., Shang, J., Wang, J., Zhou, D., Li, N., and Ma, S. (2020). Discovery of the genomic region and candidate genes of the scarlet red flesh color (Yscr) locus in watermelon (Citrullus Lanatus L.). Front. Plant Sci. 11:116. doi: 10.3389/fpls.2020.00116
Li, Q., Li, P., Sun, L., Wang, Y., Ji, K., Sun, Y., et al. (2012). Expression analysis of beta-glucosidase genes that regulate abscisic acid homeostasis during watermelon (Citrullus lanatus) development and under stress conditions. J. Plant Physiol. 169, 78–85. doi: 10.1016/j.jplph.2011.08.005
Liao, N., Hu, Z., Li, Y., Hao, J., Chen, S., Xue, Q., et al. (2020). Ethylene-responsive factor 4 is associated with the desirable rind hardness trait conferring cracking resistance in fresh fruits of watermelon. Plant Biotechnol. J. 18, 1066–1077. doi: 10.1111/pbi.13276
Liu, C., Zhang, H., Dai, Z., Liu, Y., Deng, X., Chen, F., et al. (2012). Volatile chemical and carotenoid profile in watermelon [Citrullus vulgaris (Thunb.) Schrad (Cucurbitaceae)] with different flesh colors. Food Sci. Biotechnol. 21, 531–541. doi: 10.1007/s10068-012-0068-3
Liu, D., Sun, D., Liang, J., Dou, J., Yang, S., Zhu, H., et al. (2021). Characterisation and bulk segregant analysis of ‘moon and star’ appearance in watermelon. Sci. Hortic. 285, 110-140. doi: 10.1016/j.scienta.2021.110140
Liu, J., Guo, S., He, H., Zhang, H., Gong, G., Ren, Y., et al. (2013). Dynamic characteristics of sugar accumulation and related enzyme activities in sweet and non-sweet watermelon fruits. Acta Physiol. Plant. 35, 3213–3222. doi: 10.1007/s11738-013-1356-0
Liu, S., Gao, P., Wang, X., Davis, A., Baloch, A. M., and Luan, F. (2015). Mapping of quantitative trait loci for lycopene content and fruit traits in Citrullus lanatus. Euphytica 202, 411–426. doi: 10.1007/s10681-014-1308-9
Liu, S., Gao, Z., Wang, X., Luan, F., Dai, Z., Yang, Z., et al. (2022). Nucleotide variation in the phytoene synthase (ClPsy1) gene contributes to golden flesh in watermelon (Citrullus lanatus L.). Theor. Appl. Genet. 135, 185–200. doi: 10.1007/s00122-021-03958-0
Liu, Y., He, C., and Song, H. (2018). Comparison of fresh watermelon juice aroma characteristics of five varieties based on gas chromatography-olfactometry-mass spectrometry. Food Res. Int. 107, 119–129. doi: 10.1016/j.foodres.2018.02.022
Lv, P., Li, N., Liu, H., Gu, H. H., and Zhao, W. E. (2015). Changes in carotenoid profiles and in the expression pattern of the genes in carotenoid metabolisms during fruit development and ripening in four watermelon cultivars. Food Chem. 174, 52–59. doi: 10.1016/j.foodchem.2014.11.022
Mandha, J., Shumoy, H., Devaere, J., Schouteten, J. J., Gellynck, X., de Winne, A., et al. (2021). Effect of lactic acid fermentation of watermelon juice on its sensory acceptability and volatile compounds. Food Chem. 358:129809. doi: 10.1016/j.foodchem.2021.129809
Minsart, L. A., Zoro Bi, I. A., and Djè, Y. (2011). Set up of simple sequence repeat markers and first investigation of the genetic diversity of West-African watermelon (Citrullus lanatus ssp. Vulgaris oleaginous type). Genet. Resour. Crop Evol. 58, 805–814. doi: 10.1007/s10722-010-9617-x
Ngwepe, R. M., Mashilo, J., and Shimelis, H. (2019). Progress in genetic improvement of citron watermelon (Citrullus lanatus var. citroides): a review. Genet. Resour. Crop Evol. 66, 735–758. doi: 10.1007/s10722-018-0724-4
Ngwepe, R. M., Shimelis, H., and Mashilo, J. (2021). Variation in South African citron watermelon (Citrullus lanatus var. citroides [L.H. Bailey] Mansf. Ex Greb.) Landraces assessed through qualitative and quantitative phenotypic traits. Genet. Resour. Crop. Evol. 68, 2495–2520. doi: 10.1007/s10722-021-01145-0
Pal, S., Revadi, M., Thontadarya, R., Reddy, D., Varalakshmi, B., Pandey, C., et al. (2020). Understanding genetic diversity, population structure and development of a core collection of Indian accessions of watermelon (Citrullus lanatus (Thunb.) Matsum. and Nakai). Plant Genet. Resour. 18, 359–368. doi: 10.1017/s1479262120000386
Pei, S., Liu, Z., Wang, X., Luan, F., Dai, Z., Yang, Z., et al. (2021). Quantitative trait loci and candidate genes responsible for pale green flesh colour in watermelon (Citrullus lanatus). Plant Breed. 140, 349–359. doi: 10.1111/pbr.12908
Perkins-Veazie, P., Collins, J. K., Davis, A. R., and Roberts, W. (2006). Carotenoid content of 50 watermelon cultivars. J. Agric. Food Chem. 54, 2593–2597. doi: 10.1021/jf052066p
Pino, J. A., Marbot, R., and Aguero, J. (2003). Volatile components of watermelon (Citrullus Ianatus [Thunb.] Matsum. et Nakai) fruit. J. Essent. Oil Res. 15, 379–380. doi: 10.1080/10412905.2003.9698616
Ramirez, J. L., Du, X., and Wallace, R. W. (2020). Investigating sensory properties of seven watermelon varieties and factors impacting refreshing perception using quantitative descriptive analysis. Food Res. Int. 138:109681. doi: 10.1016/j.foodres.2020.109681
Ren, Y., Guo, S., Zhang, J., He, H., Sun, H., Tian, S., et al. (2018). A tonoplast sugar transporter underlies a sugar accumulation QTL in watermelon. Plant Physiol. 176, 836–850. doi: 10.1104/pp.17.01290
Ren, Y., Li, M., Guo, S., Sun, H., Zhao, J., Zhang, J., et al. (2021). Evolutionary gain of oligosaccharide hydrolysis and sugar transport enhanced carbohydrate partitioning in sweet watermelon fruits. Plant Cell 33, 1554–1573. doi: 10.1093/plcell/koab055
Ren, Y., Mcgregor, C., Zhang, Y., Gong, G., Zhang, H., Guo, S., et al. (2014). An integrated genetic map based on four mapping populations and quantitative trait loci associated with economically important traits in watermelon (Citrullus lanatus). BMC Plant Biol. 14:33. doi: 10.1186/1471-2229-14-33
Ren, Y., Sun, H., Zong, M., Guo, S., Ren, Z., Zhao, J., et al. (2020). Localisation shift of a sugar transporter contributes to phloem unloading in sweet watermelons. New Phytol. 227, 1858–1871. doi: 10.1111/nph.16659
Renner, S. S., Sousa, A., and Chomicki, G. (2017). Chromosome numbers, Sudanese wild forms, and classification of the watermelon genus Citrullus, with 50 names allocated to seven biological species. Taxon 66, 1393–1405. doi: 10.12705/666.7
Rico, X., Gullón, B., Alonso, J. L., and Yáñez, R. (2020). Recovery of high value-added compounds from pineapple, melon, watermelon and pumpkin processing by-products: an overview. Food Res. Int. 132:109086. doi: 10.1016/j.foodres.2020.109086
Rubatzky, V. E. (2001). Watermelons: Characteristics, Production and Marketing. Origin, Distribution and Uses. Alexandria, VA: ASHS Press.
Sandlin, K., Prothro, J., Heesacker, A., Khalilian, N., Okashah, R., Xiang, W., et al. (2012). Comparative mapping in watermelon (Citrullus lanatus (Thunb.) Matsum. Et Nakai). Theor. Appl. Genet. 125, 1603–1618. doi: 10.1007/s00122-012-1938-z
Schwartz, S. H., Qin, X., and Zeevaart, J. A. (2003). Elucidation of the indirect pathway of abscisic acid biosynthesis by mutants, genes, and enzymes. Plant Physiol. 131, 1591–1601. doi: 10.1104/pp.102.017921
Shang, J., Li, N., Li, N., Xu, Y., Ma, S., and Wang, J. (2016). Construction of a high-density genetic map for watermelon (Citrullus lanatus L.) based on large-scale SNP discovery by Specific Length Amplified Fragment sequencing (SLAF-seq). J. Am. Soc. Hortic. Sci. 203, 38–46. doi: 10.1016/j.scienta.2016.03.007
Sheng, Y., Luan, F., Zhang, F., and Davis, A. R. (2012). Genetic diversity within Chinese watermelon ecotypes compared with germplasm from other countries. J. Am. Soc. Hortic. Sci. 137, 144–151. doi: 10.21273/jashs.137.3.144
Singh, D., Singh, R., Sandhu, J. S., and Chunneja, P. (2017). Morphological and genetic diversity analysis of Citrullus landraces from India and their genetic inter relationship with continental watermelons. Sci. Hortic. 218, 240–248. doi: 10.1016/j.scienta.2017.02.013
Solmaz, I., Sari, N., and Aka-Kacar, Y. (2010). The genetic characterisation of Turkish watermelon (Citrullus lanatus) accessions using RAPD markers. Genet. Resour. Crop Evol. 57, 763–771. doi: 10.1007/s10722-009-9515-2
Song, Q., Joshi, M., dipiazza, J., and Joshi, V. (2020). Functional relevance of citrulline in the vegetative tissues of watermelon during abiotic stresses. Front. Plant Sci. 11:512. doi: 10.3389/fpls.2020.00512
Stone, S., Boyhan, G., and Macgregor, C. (2019). Inter- and intracultivar variation of heirloom and open-pollinated watermelon cultivars. J. Am. Soc. Hortic. Sci. 54, 212–220. doi: 10.21273/hortsci13638-18
Subburaj, S., Lee, K., Jeon, Y., Tu, L., and Son, G. (2019). Whole genome resequencing of watermelons to identify single nucleotide polymorphisms related to flesh color and lycopene content. PLoS One 14:e0223441. doi: 10.1371/journal.pone.0223441
Sun, L., Zhang, Y., Cui, H., Zhang, L., Sha, T., Wang, C., et al. (2020). Linkage Mapping and comparative transcriptome analysis of firmness in watermelon (Citrullus lanatus). Front. Plant Sci. 11:831. doi: 10.3389/fpls.2020.00831
Tadmor, Y., King, S., Levi, A., Davis, A., Meir, A., Wasserman, B., et al. (2005). Comparative fruit colouration in watermelon and tomato. Food Res. Int. 38, 837–841. doi: 10.1016/j.foodres.2004.07.011
Tian, S., Jiang, L., and Cui, X. (2018). Engineering herbicide-resistant watermelon variety through CRISPR/Cas9-mediated base-editing. Plant Cell Rep. 37, 1353–1356. doi: 10.1007/s00299-018-2299-0
Tian, S., Jiang, L., and Gao, Q. (2017). Efficient CRISPR/Cas9-based gene knockout in watermelon. Plant Cell Rep. 36, 399–406. doi: 10.1007/s00299-016-2089-5
Tlili, I., Hdider, C., Lenucci, M. S., Riadh, R. I., Jebari, H., and Dalessandro, G. (2011a). Bioactive compounds and antioxidant activities of different watermelon (Citrullus lanatus (Thunb.) Mansfeld) cultivars as affected by fruit sampling area. J. Food Comp. Anal. 24, 307–314. doi: 10.1016/j.jfca.2010.06.005
Tlili, I., Hdider, C., Lenucci, M. S., Riadh, R. I., Jebari, H., and Dalessandro, G. (2011b). Bioactive compounds and antioxidant activities during fruit ripening of watermelon cultivars. J. Food Comp. Anal. 24, 923–928. doi: 10.1016/j.jfca.2011.03.016
Umer, M. J., Bin Safdar, L., and Gebremeskel, H. (2020b). Identification of key gene networks controlling organic acid and sugar metabolism during watermelon fruit development by integrating metabolic phenotypes and gene expression profiles. Hortic. Res. 7:193. doi: 10.1038/s41438-020-00416-8
Umer, M. J., Gao, L., Gebremeskel, H., Bin Safdar, L., Yuan, P., Zhao, S., et al. (2020a). Expression pattern of sugars and organic acids regulatory genes during watermelon fruit development. Sci. Hortic. 265:109102.
Wang, C., Luan, F., Liu, H., Davis, A. R., Zhang, Q., Dai, Z., et al. (2021). Mapping and predicting a candidate gene for flesh color in watermelon. J. Integr. Agric. 20, 2100–2111. doi: 10.1016/s2095-3119(20)63487-6
Wang, C., Qiao, A., Fang, X., Sun, L., Gao, P., Angela, R. D., et al. (2019). Fine mapping of lycopene content and flesh color related gene and development of molecular marker assisted selection for flesh. Front. Plant Sci. 10:1240. doi: 10.3389/fpls.2019.01240
Wang, D., Zhang, M., Xu, N., Yang, S., Dou, J., Liu, D., et al. (2022). Fine mapping a CLGS gene controlling dark-green stripe rind in watermelon. Sci. Hortic. 291:110583. doi: 10.1016/j.scienta.2021.110583
Wang, N., Liu, S., Gao, P., Luan, F., and Davis, A. R. (2016). Developmental changes in gene expression drive accumulation of lycopene and beta-carotene in watermelon. J. Am. Soc. Hortic. Sci. 141, 1–10. doi: 10.9734/bbj/2016/23865
Wang, P., Li, Q., Hu, J., and Su, Y. (2015). Comparative analysis of genetic diversity among Chinese watermelon germplasms using SSR and SRAP markers, and implications for future genetic improvement. Turk. J. Agric. For. 39, 322–331. doi: 10.3906/tar-1407-29
Wang, W., Guo, S., Tian, S., Zhang, J., Ren, Y., Sun, H., et al. (2017). Abscisic acid pathway involved in the regulation of watermelon fruit ripening and quality trait evolution. PLoS One 12:e0179944. doi: 10.1371/journal.pone.0179944
Wang, Y., Wang, J., and Guo, S. (2021). CRISPR/Cas9-mediated mutagenesis of CLBG1 decreased seed size and promoted seed germination in watermelon. Hortic. Res. 8:70. doi: 10.1038/s41438-021-00506-1
Wechter, W. P., Levi, A., Harris, K. R., Davis, A. R., Fei, Z., and Katzir, N. (2008). Gene expression in developing watermelon fruit. BMC Genomics 9:275. doi: 10.1186/1471-2164-9-275
Wei, C., Chen, X., Wang, Z., Liu, Q., Li, H., Zhang, Y., et al. (2017). Genetic mapping of the LOBED LEAF 1 (CLLL1) gene to a 127.6-kb region in watermelon (Citrullus lanatus L.). PLoS One 12:e0180741. doi: 10.1371/journal.pone.0180741
Wei, G., Tian, P., Zhang, F., Qin, H., Miao, H., and Chen, Q. (2016). Integrative analyses of nontargeted volatile profiling and transcriptome data provide molecular insight into VOC diversity in cucumber plants (Cucumis sativus). Plant Physiol. 172, 603–618. doi: 10.1104/pp.16.01051
Wu, S., Wang, X., Reddy, U., Sun, H., Bao, K., Gao, L., et al. (2019). Genome of ‘Charleston Gray’, the principal American watermelon cultivar, and genetic characterisation of 1,365 accessions in the U.S. National Plant Germplasm System watermelon collection. Plant Biotechnol. J. 17, 2246-2258. doi: 10.1111/pbi.13136
Yajima, I., Sakakibara, H., Ide, J., Yanai, T., and Kazuo, H. (1985). Volatile flavor components of watermelon (Citrullus vulgaris). Agric. Biol. Chem. 49, 3145-3150. doi: 10.1080/00021369.1985.10867246
Yang, T., Amanullah, S., and Pan, J. (2021). Identification of putative genetic regions for watermelon rind hardness and related traits by BSA-seq and QTL mapping. Euphytica 217:19.
Yang, X., Ren, R., Ray, R., Xu, J., Li, P., Zhang, M., et al. (2016). Genetic diversity and population structure of core watermelon (Citrullus lanatus) genotypes using dartseq based SNPs. Plant Genet. Resour. 14, 226–233. doi: 10.1017/S1479262115000659
Yang, X., Yang, F., Liu, Y., Li, J., and Song, H. L. (2020). Identification of key off-flavor compounds in thermally treated watermelon juice via gas chromatography–olfactometry–mass spectrometry, aroma recombination, and omission experiments. Foods 9:227. doi: 10.3390/foods9020227
Yao, Y. X., Li, M., Zhai, H., You, C. X., and Hao, Y. J. (2011). Isolation and characterisation of an apple cytosolic malate dehydrogenase gene reveal its function in malate synthesis. J. Plant Physiol. 168, 474–480. doi: 10.1016/j.jplph.2010.08.008
Yativ, M., Harary, I., and Wolf, S. (2010). Sucrose accumulation in watermelon fruits: genetic variation and biochemical analysis. J. Plant Physiol. 167, 589–596. doi: 10.1016/j.jplph.2009.11.009
Yoo, K. S., Bang, H., Lee, E. J., Crosby, K., and Patil, B. S. (2012). Variation of carotenoid, sugar, and ascorbic acid concentrations in watermelon genotypes and genetic analysis. Hortic. Environ. Biotechnol. 53, 552–560. doi: 10.1007/s13580-012-0014-6
Yu, K., Xu, Q., Da, X., Guo, F., Ding, Y., and Deng, X. (2012). Transcriptome changes during fruit development and ripening of sweet orange (Citrus sinensis). BMC Genomics 13:10. doi: 10.1186/1471-2164-13-10
Yuan, P., Umer, M. J., and He, N. (2021). Transcriptome regulation of carotenoids in five flesh-colored watermelons (Citrullus lanatus). BMC Plant Biol. 21:203. doi: 10.1186/s12870-021-02965-z
Yue, Z., Ma, R., Cheng, D., Yan, X., He, Y., Wang, C., et al. (2021). Candidate gene analysis of watermelon stripe pattern locus ClSP ongoing recombination suppression. Theor. Appl. Genet. 134, 3263–3277. doi: 10.1007/s00122-021-03891-2
Zamuz, S., Munekata, P. E. S., Gullón, B., Rocchetti, G., Montesano, D., and Lorenzo, J. M. (2021). Citrullus lanatus as source of bioactive components: an up-to-date review. Trends Food Sci. Technol. 111, 208-222. doi: 10.1016/j.tifs.2021.03.002
Zhang, B., Tolstikov, V., Turnbull, C., Hicks, L. M., and Fiehn, O. (2010). Divergent metabolome and proteome suggest functional independence of dual phloem transport systems in cucurbits. Proc. Natl. Acad. Sci. U.S.A. 107, 13532–13537. doi: 10.1073/pnas.0910558107
Zhang, H., Fan, J., Guo, S., Ren, Y., Gong, G., Zhang, J., et al. (2016). Genetic diversity, population structure, and formation of a core collection of 1197 citrullus accessions. J. Am. Soc. Hortic. Sci. 51, 23–29. doi: 10.21273/hortsci.51.1.23
Zhang, H., Wang, H., and Guo, S. (2012). Identification and validation of a core set of microsatellite markers for genetic diversity analysis in watermelon, Citrullus lanatus Thunb. Matsum. & Nakai. Euphytica 186, 329–342. doi: 10.1007/s10681-011-0574-z
Zhang, J., Guo, S., Ji, G., Zhao, H., Sun, H., Ren, Y., et al. (2020a). A unique chromosome translocation disrupting CLWIP1 leads to gynoecy in watermelon. Plant J. 101, 265–277. doi: 10.1111/tpj.14537
Zhang, J., Sun, H., Guo, S., Ren, Y., Li, M., Wang, J., et al. (2020b). Decreased protein abundance of lycopene β-cyclase contributes to red flesh in domesticated watermelon. Plant Physiol. 183, 1171–1183. doi: 10.1104/pp.19.01409
Zhang, J., Guo, S., Ren, Y., Zhang, H., Gong, G., Zhou, M., et al. (2017). High-level expression of a novel chromoplast phosphate transporter CLPHT4;2 is required for flesh color development in watermelon. New Phytol. 213, 1208–1221. doi: 10.1111/nph.14257
Zhang, Z., Zhang, Y., Sun, L., Qiu, G., Sun, Y., Zhu, Z., et al. (2018). Construction of a genetic map for Citrullus lanatus based on CAPS markers and mapping of three qualitative traits. Sci. Hortic. 233, 532–538. doi: 10.1016/j.scienta.2017.10.029
Zhong, Y., Shi, J., Zheng, Z., Nawaz, M. A., Chen, C., Cheng, F., et al. (2019). NMR-based fruit metabonomic analysis of watermelon grafted onto different rootstocks under two potassium levels. Sci. Hortic. 258:108793. doi: 10.1016/j.scienta.2019.108793
Zhou, M., Guo, S., Zhang, J., Zhang, H., Li, C., Tang, X., et al. (2016). Comparative dynamics of ethylene production and expression of the ACS and ACO genes in normal-ripening and non-ripening watermelon fruits. Acta Physiol. Plant. 38, 228–241.
Zhu, H., Zhang, M., Sun, S., Yang, S., Li, J., Li, H., et al. (2019). A single nucleotide deletion in an ABC transporter gene leads to a dwarf phenotype in watermelon. Front. Plant Sci. 10:1399. doi: 10.3389/fpls.2019.01399
Zhu, Q., Gao, P., Liu, S., Zhu, Z., Amanullah, S., Davis, A. R., et al. (2017). Comparative transcriptome analysis of two contrasting watermelon genotypes during fruit development and ripening. BMC Genomics 18:3. doi: 10.1186/s12864-016-3442-3
Keywords: C. lanatus, flesh texture, gene editing, molecular markers, phytochemical compounds, phytohormones, transcriptome analysis, quantitative trait loci
Citation: Mashilo J, Shimelis H, Ngwepe RM and Thungo Z (2022) Genetic Analysis of Fruit Quality Traits in Sweet Watermelon (Citrullus lanatus var. lanatus): A Review. Front. Plant Sci. 13:834696. doi: 10.3389/fpls.2022.834696
Received: 13 December 2021; Accepted: 25 January 2022;
Published: 22 March 2022.
Edited by:
Sanghyeob Lee, Sejong University, South KoreaReviewed by:
Yong Suk Chung, Jeju National University, South KoreaO. New Lee, Sejong University, South Korea
Copyright © 2022 Mashilo, Shimelis, Ngwepe and Thungo. This is an open-access article distributed under the terms of the Creative Commons Attribution License (CC BY). The use, distribution or reproduction in other forums is permitted, provided the original author(s) and the copyright owner(s) are credited and that the original publication in this journal is cited, in accordance with accepted academic practice. No use, distribution or reproduction is permitted which does not comply with these terms.
*Correspondence: Jacob Mashilo, amFjb2JtYXNoaWxvQHlhaG9vLmNvbQ==