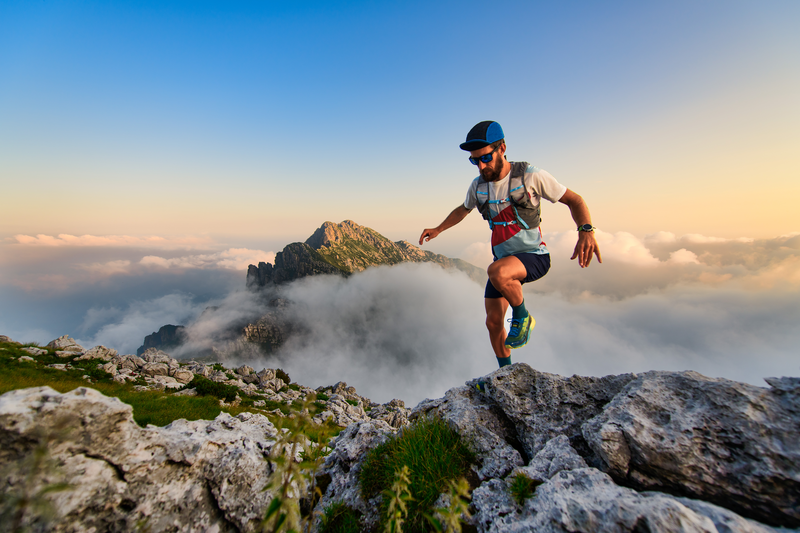
94% of researchers rate our articles as excellent or good
Learn more about the work of our research integrity team to safeguard the quality of each article we publish.
Find out more
ORIGINAL RESEARCH article
Front. Plant Sci. , 17 June 2022
Sec. Plant Metabolism and Chemodiversity
Volume 13 - 2022 | https://doi.org/10.3389/fpls.2022.822956
Volatile compounds could affect the flavor and ornamental quality of cut flowers, but the flavor change occurring during the vase period of the cut flower is unclear. To clarify the dynamic changes during the vase period of cut lily (Lilium spp. ‘Manissa’) flowers, comprehensive flavor profiles were characterized by the electronic nose (E-nose) and headspace solid-phase microextraction gas chromatography-mass spectrometry (HS-SPME/GC-MS). The response value of sensor W2W was significantly higher than other sensors, and its response value reached the highest on day 4. A total of 59 volatiles were detected in cut lilies by HS-SPME/GC-MS, mainly including aldehydes, alcohols, and esters. There were 19 volatiles with odor activity values (OAVs) greater than 1. Floral and fruity aromas were stronger, followed by a pungent scent. Principal component analysis (PCA) and hierarchical cluster analysis (HCA) could effectively discriminate lily samples derived from different vase times on the basis of E-nose and HS-SPME-GC-MS. In summary, our study investigates the flavor change profile and the diversity of volatile compounds during the vase period of cut lilies, and lilies on day 4 after harvest exhibited excellent aroma and flavor taking into consideration of the flavor intensity and diversity. This provided theoretical guidance for the assessment of scent volatiles and flavor quality during the vase period of cut lily flowers and will be helpful for the application of cut lilies during the postharvest process.
Cut lilies (Lilium spp.) are bulbous plants with large, trumpet-shaped, and typically fragrant flowers and derived from interspecific crosses of species belonging to the Archelirion section (Liao et al., 2013). Additionally, lilies are well-known ornamental plants with a diversity of fragrant types (Du et al., 2019). Nowadays, cut lilies are in demand worldwide due to their superior commercial and ornamental value (Zhang et al., 2018a). The Netherlands dominated the world’s total trade in lily bulbs, with a production area of 5,280 hectares in 2020 (Kang et al., 2021). More than 600 cultivars of lily bulbs were produced in the Netherlands every year and exported to other countries (Asjes, 2000; Wani et al., 2016). In addition to the ornamental value, lily flowers have also extensive edible and medicinal value (Francis et al., 2004; Mlcek and Rop, 2011). Lily flowers have been cultivated for the production of lily oil and lily water for the treatment of skin and articular diseases (Pieroni, 2000; Zaccai et al., 2020). In recent years, the essential oil extracted from lily flowers has become more popular, which can effectively eliminate body odor and promote metabolism and detoxification. Since lily essential oil contains natural plant protein and polysaccharides, it also has an effect on moisturizing, soothing, and skin elasticity, accelerating cell growth and blood circulation (Bruni et al., 1997; Zaccai et al., 2020).
The postharvest vase process of cut lily flowers involves a complex process of maturation and senescence. In general, cut lily flowers are harvested at the tight and green bud (puffy bud) stage, which is also called as commercial harvest stage of cut lily flowers (Zhang et al., 2018a). From a physiological perspective, the development of cut lily flowers is a metabolic process, which involves the macromolecules, including protein, hormone, starch, lipid, and related inorganic ions (Zhang et al., 2021). Previously, various studies have also indicated the key regulatory mechanism of developmental processes in cut flowers. The flower opening, senescence, dynamics in various physiological and biochemical levels, and changes in organelle and cell levels have been elucidated (Ma et al., 2018; Zhang et al., 2018b). Interestingly, various previous results in our group also indicated that key signal molecules, such as hydrogen gas (Ren et al., 2017), nitric oxide, calcium, and hydrogen peroxide (Zhang et al., 2018a), not only regulated the metabolic process of cut lily after postharvest but also improved the vase life and ornamental quality.
Recently, electronic nose (E-nose) technology and headspace solid-phase microextraction combined with gas chromatography-mass spectrometry (HS-SPME/GC-MS) are applied in the food industry for quality control. E-nose is sensitive to smell information from samples, and slight changes in volatile compounds could cause the different responses of sensors (Emre and Bekir, 2017). Moreover, E-nose is designed as an apparatus to mimic the human olfactory perception within an instrument designed to obtain repeatable measurements, allowing identifications and classifications of aroma mixtures while eliminating the common occurrence of olfactory fatigue in people (Wilson and Ba Ietto, 2011). For example, E-nose analysis was generally supplied to comprehensively evaluate the overall information scent, thereby achieving fresh grading and quality control of vegetables, fruits, beverages, cereals, and meats (Wang et al., 2017; Mirzaee-Ghaleh et al., 2019). The HS-SPME/GC-MS technology has been widely applied in the identification of volatile compounds, which was characterized by easy control, good repeatability, low threshold, solvent-free, and sensitive approach (Wei et al., 2021).
Lilium, a famous and significant cut flower, emits a variety of volatile organic compounds (Zhang et al., 2018b). The volatile substances in different tissues of lily might be different. Johnson et al. (2016) indicated that floral volatile emission of Lilium was differential between tepals and reproductive organs, and tepal tissues (two petal whirls) emitted the vast majority of total volatile molecules compared with the reproductive organs of the flower. Moreover, for Murraya koenigii L., the stems and flowers have a strong aroma compared with the leaves (Walde et al., 2006). In addition, Shi et al. (2018) captured the changes in flower volatile emissions at four flower developmental stages of Lilium ‘Siberia’ and found that most of the flower scent compounds had the highest emission rate at the full-flowering stage. The accumulation levels of 80 out of 100 volatiles of Rosa damascena were significantly influenced by the flower developmental stages (Rusanov et al., 2011). These results reveal that the biosynthesis of floral volatiles was controlled developmentally in flowers. However, no literature is available on the assessment and change of scent volatiles and flavor quality during the vase period of cut flowers to date.
Thus, the objectives of this study were as follows: (1) to explore the overall flavor profile by E-nose during the vase period of lilies; (2) to identify and quantify the volatile compounds of cut lilies derived from different vase times by HS-SPME/GC–MS technology; (3) to further seek the characteristic odor compositions (OAVs greater than 1) in cut lilies and determine the main aroma types; (4) to distinguish the cut lilies derived from different vase times through radar fingerprint chart, hierarchical cluster analysis (HCA), and principal component analysis (PCA) on the basis of E-nose and HS-SPME-GC-MS; and (5) to evaluate and compare the odor compounds, aromatic profile and aroma, and flavor of lily flowers during the vase period. These results made a significant contribution to assess scent volatiles and ornamental quality during the vase period of cut lily flowers. By extension, it also powerfully provided a theoretical foundation for the development and application of cut lilies based on the volatile components of the commercial harvest stage.
The experiment was conducted using lilies (Lilium spp.‘Manissa’). Flowers were obtained from a local commercial grower (Meilan Florist, Lanzhou, China) and harvested early in the morning. Cut lily flowers were harvested at the tight and green bud (puffy bud) stage (commercial harvest stage) (Zhang et al., 2018a). Buckets containing the flower stems were covered with a plastic film shroud to minimize moisture loss and physical damage during transportation. After reaching the laboratory for approximately 30 min, the stems were cut under water to a length of 60–70 cm, which avoided air embolism, reduced water loss, and maintained the water balance of cut flowers. These flowers were then held in the laboratory. The laboratory was maintained at a temperature of 25 ± 3°C, a relative humidity of 60 ± 5%, and a photon irradiance of 15 μmol m–2 s–1. The flowers with an identical size, color, and no mechanical damage or infections were selected to observe the postharvest senescence of flowers. Each treatment was carried out in three replicates, with each replication consisting of five flowering stems. The whole flowers (including distal and proximal tepals, stamens, and carpels) were then sampled every 2 days and randomly mixed for E-nose and GC-MS detection (Figure 1).
Figure 1. Physical phenotype of cut lily flowers after postharvest. Photographs were taken after postharvest 0, 2, 4, 6, and 8 days, respectively.
Chromatographic 2-octanol obtained from Sigma Aldrich (United States) was used as an internal standard for quantitative analysis. SPME fibers and injection handles were purchased from Supelco of the US. The 7890B–7000C GC–MS detector with automatic deconvolution system (AMDIS) and standard mass spectrometry library (NIST 2014) workstation was purchased from the United States Agilent. DB-WAX elastic quartz capillary column (30 m × 0.25 mm, 0.25 μm) was also purchased from the United States Agilent. The 15-ml screw-top headspace bottle with black opening screw cap and silicone septum and constant temperature metal magnetic stirrer are all from Shanghai AnPu Experimental Technology Co., Ltd.
The E-nose analysis was conducted according to the procedure described by Li et al. (2017) with modifications. The headspace analysis was performed with a commercial PEN3.5 E-nose (Airsense Analytics, GmBH, Schwerin, Germany). The system contained 10 metal oxide sensors as shown in Supplementary Table 1. Prior to detection, each sample (5 g of flower samples from 5 flowers) was placed in an airtight glass vial and closely capped with a PTFE-silicon stopper. Then, the samples were kept at 25 ± 1°C for approximately 40 min (headspace-generation time). The detection time of the sample was 120 s, the cleaning time of the sensor was 60 s, and the adjustment time of automatic zero was 10 s. All samples were run with three repetitions.
The GC-MS conditions were conducted according to the procedure described by Wei et al. (2021) with some modifications. For that purpose, the SPME fiber used was DVB/CAR/PDMS (50/30 mm) (Supelco, Bellefonte, PA, United States). The lily sample was taken out of liquid nitrogen and quickly ground to the homogenized state, then the homogenate (5 g of flower samples) was added in a 15-ml screw-head headspace vial containing a magnetic stirring rotor and 2 ml ultrapure water to fully stir. Later, a certain amount of NaCl (1 g) and 10 μl of 2-octanol (41.05 mg l–1) internal standard were added. The sample vial was then tightened and fitted with a PTFE silicon stopper quickly. Later, the headspace bottle was equilibrated at a certain time (15 min) for a certain temperature (60°C) on a metal heating agitation platform at 500 rpm. Then, the extraction and adsorption were carried out by inserting the pretreated SPME fibers into the headspace bottle for a certain time (30 min) with consecutive heating and agitation. After extraction, the fiber was desorbed into the GC injection port for a certain time (5 min) and then subjected to the GC-MS analysis.
The volatiles were separated on a DB-WAX elastic quartz capillary column (30 m × 0.25 mm, 0.25 μm) with helium (≥99.999% purity) at a flow rate of 1.0 ml min–1 as the carrier gas. Splitless injection mode was adopted during volatile insertion at 250°C. The oven temperature program was as follows: initial temperature 40°C (held for 3 min) and increased to 150°C at a rate of 4°C min–1, then a ramp of 8°C min–1 to 210°C, and held for 3 min, which took 41 min in the entire procedure. The mass spectrometer was operated by the electron impact (EI) method with an ionization energy of 70 eV and a source temperature of 230°C. The acquisition was full-scan mode and mass acquisition range of 33–550 m⋅–1. The filament current and the quadrupole temperature were 150 μA and 250°C, respectively.
Every composition was analyzed by the computer workstation’s automatic deconvolution system (AMDIS) and mass spectrometry library (NIST 2014) according to its mass fragmentation pattern from the spectral database after the GC-MS analysis. Volatile components with a matching degree greater than 80% were maintained. The concentration of each individual compound in the cut lilies was calculated using the internal standard method, and the formula is as follows: the content of each composition (μg⋅tkg–1) = (A1/A2) × (M1/M2) × 1,000 (A1: the component area of the detected composition; A2: the component area of the internal standard; M1: the amount of the internal standard; and M2: the amount of the sample).
Odor activity values (OAVs) are classical coefficients commonly used in the evaluation of aromatic substances. The calculation formula is as follows: OAVs = Ci/OTi (Ci: the actual concentration of a certain volatile in lily samples and OTi: odor olfaction threshold) (Liu et al., 2018). When the OAVs are equal to or greater than 1, components contribute significantly to the overall aroma of cut lilies since they have reached the olfactory threshold of the human nasal cavity, while components with OAVs smaller than 1 may not play a direct role (Zhu et al., 2020a).
The Excel 2010 software was conducted for statistical analysis and charting of data. The data were analyzed using SPSS 22.0 (SPSS Inc., Chicago, IL, United States). Contents of different components were presented as the mean ± SE of three independent experiments with three replicated measurements. Differences among treatments were analyzed using the Duncan’s multiple range test at a P < 0.05 level.
In this study, the comprehensive flavor characterization of cut lilies was analyzed using an E-nose equipped with 10 types of sensors. According to Figure 2, the response value of sensors W3C, W6S, W5C, W1S, W1W, W2S, and W3S to all samples was small and almost unchanged. Subsequently, the response value of sensor W2W was the highest, and sensors W1C and W5S were also slightly responded for lilies during the postharvest process (Figure 2). Interestingly, W2W, W1W, and W5C sensors also contributed significantly to the flavor discrimination of shrimp paste during the process of discrimination of shrimp paste (Fan et al., 2017). In addition, the response values of sensors W2W, W1C, and W5S on day 4 were significantly higher than those of the other sensors, and the response of W2W for 4-day samples was significantly different from those of the other samples (Figure 2), implying the emergence of flavor intensity on day 4 after lily harvest. Undeniably, the sensor W2W contributed significantly to the flavor discrimination during the vase period of cut lily flowers.
Figure 2. Response values of electronic nose (E-nose) sensors for cut lily flowers during the postharvest process. The values (means ± SE) are the averages of three independent experiments (n = 5). Bars not sharing the same letters on the same sensors indicate statistically significant differences using the Duncan’s multiple range test (P < 0.05).
The PCA is a broad statistical procedure by applying an orthogonal transformation to transform a set of observations of possibly correlated variables into a set of values of linearly uncorrelated variables called principal components (PCs). PCA explains the relationship of objects and the correlation structure of the variables (Wei et al., 2021). PCA was performed on the dataset of the response values of E-nose to evaluate the influence of different postharvest times on the grouping of lily samples. The two-dimensional bi-plots of score and loading of the lily samples are presented in Figure 3. The PCs, namely, PC1 and PC2 represented 69.36 and 11.43% of the total variance, respectively, with the cumulative contribution rate of the first two PCs accounting for 80.70%, which indicated that they were sufficient to explain the total variance in the dataset (Dong et al., 2019), and could be used to distinguish lily samples at different vase times. Satisfactorily, it could be observed that the grouping of the samples could be divided into three categories through the first PCs. The lily samples on days 2 and 4 are clustered into one category, being located to the right of the X-axis. The second category is the 6- and 8-day lily samples, which are located to the left of the X-axis. The third category is the 0-day-cut lilies, the day of harvest when the first PC is near 0 (Figure 3A). Furthermore, lily samples on days 2 and 4 could be divided into two categories through the second PCs. In combination with Figure 3B, it can be seen that W5S, W1C, and W2W are significantly positively correlated with the first PC and are the main sensors for distinguishing different samples. Sensors W5C and W1S have the largest positive and negative effects in the second PC, respectively. Thus, the sensor W1S contributed more to the 2-day lily samples, and the response values of W5C might be more correlated with the 4-day lily samples.
Figure 3. Principal component analysis (PCA) of cut lilies during the postharvest process based on E-nose date. (A) Score plot; (B) loading plot.
Unsupervised data processing as a visualization protocol was usually used to evaluate clustering tendency when some indicators were applied to discriminate different samples (Lv et al., 2015). stocktickerHCA was a numerical data consolidation method that could accurately describe the otherness among different cut lily samples during the vase period and was conducive to locate the homogeneity of cut lily flowers to some extent in accordance with similar characteristic volatiles. We referred to the system clustering model characterized through the inter-group connection method with the measurement standard of Euclidean distance employed by Chen et al. (2018). The coalescent data were conducted as a hierarchical dendrogram, where the horizontal axis showed the Euclidean distance among groups and the vertical axis displayed the similarity and diverse degree of flavor in lily flowers. stocktickerHCA can not only judge the repeatability within the group, but more importantly, it can distinguish the differences between different treatments. Three lily samples at each postharvest time were very closely related and could be grouped into a small category (Figure 4), indicating that the E-nose has good reproducibility when evaluating the cut lily aroma at different postharvest times. It could be obtained on the basis of Figure 4, and five different lily flowers were evidently classified into 3 groups at the Euclidean distance of 7.5. The 0-day-cut lilies (1, 2, 3) belonged to one group, the lily samples on days 2 (4, 5, 6) and 4 (7, 8, 9) belonged to one group, and 6-day (10, 11, 12) and 8-day (13, 14, 15) cut lilies belonged to another group. In addition, the relationship between 0-day lilies and 6- and 8-day lilies is relatively close. When the Euclidean distance continues to expand, they could also be clustered into one category, which is completely consistent with the results of PCA. However, the 2- and 4-day lily samples were relatively distant from 0-day-cut lilies. It could be speculated that the main aroma types might change at this time, and the response intensity of sensors W2W and W5S is significantly enhanced. The results of PCA and stocktickerHCA in distinguishing the aroma of lily flowers at different vase times are completely consistent, indicating that the combination of the two methods is feasible and accurate in detecting the flavor changes during the vase period of cut lilies with E-nose. This can not only identify the type of characteristic sensor but also distinguish and categorize cut lily flowers during the vase period.
Figure 4. Hierarchical cluster analysis (HCA) of cut lilies during the postharvest process based on E-nose date. Note: cut lilies on day 0 (1, 2, 3), on day 2 (4, 5, 6), on day 4 (7, 8, 9), on day 6 (10, 11, 12) and on day 8 (13, 14, 15).
The volatile profile of cut flowers constitutes a very important organoleptic characteristic for the distinguishing and application of cut flowers during the vase period. To evaluate the flavor change of cut lily flowers after postharvest, volatile compounds were monitored using HS-SPME/GC–MS to provide the volatile profile of each sample at different postharvest times. In this study, a total of 59 different volatile compounds were detected in cut lily flowers. The volatile compounds were classified into 8 groups based on their chemical properties, including 10 aldehydes, 14 alcohols, 13 esters, 6 phenols, 5 hydrocarbons, 2 ketones, 5 acids, and 4 others (Table 1). During the Tremella aurantialba fermentation, the identified compounds consisted of 5 families, 10 aldehydes, 7 esters, 8 alcohols, 2 ketones, and 16 heterocyclic and aromatic compounds (Dai et al., 2018), resulting in a predominant flavor during the T. aurantialba fermentation. Moreover, Yin et al. (2015) reviewed that volatile compounds of water lily flowers comprised several classes, which was in accordance with this study, thereby implying the diversity of volatile substances of lilies. Approximately 40 volatile compounds from flowers reported in the literature (Ike Da et al., 2010; Gaytán et al., 2013; Yang et al., 2017) could also be authenticated in our samples. Approximately 10 kinds of aroma compounds were identified for the first time in cut lilies, which presumably attributed to many other factors, including genotype, cultivation region, harvest maturity, and cultivar differences. However, some of these compounds had been reported in cabbages (Wei et al., 2021), grapes (Wang et al., 2017), and Chinese jujubes (Chen et al., 2018).
Table 1. The composition and content of volatile compounds in cut lily during the postharvest process by headspace solid-phase microextraction gas chromatography-mass spectrometry (HS-SPME/GC–MS) methodology.
According to Table 1, it was clear that 22 compounds were detected on all days. Among 22 volatile compounds, both (E)-2-hexenal and linalool accounted for approximately 10% of the total content, occupying the most abundant of lilies. Linalool was found as a prominent constituent of the floral scent in Magnolia kobus (Azuma et al., 2001) and Clarkia breweri (Pichersky et al., 1994). Moreover, Dhandapani et al. (2017) identified that linalool synthase was essential for the production of major volatile organic compounds of champak flowers by metabolite-guided transcriptomics. Thus, this implies that linalool could be a key substance for the floral fragrance of cut lilies, which was also reported in the previous literature (Du et al., 2019; Melo et al., 2021).
Four volatile compounds only existed in 0-day-cut lilies after harvest (Table 1). It is worth mentioning that (E)-2-hexen-1-ol was reported exclusively in lilies by our group. Vanillin, farnesol, and methyl salicylate were identified previously in honeysuckle flowers (Ike Da et al., 2010) and Hybrid Lilium (Johnson et al., 2016). Previously, vanillin was widely used in edible spices, which could be found in vanilla seeds (Dong et al., 2014) and H. Lilium petals (Johnson et al., 2016). Interestingly, in a recent study, vanillin has been found to have appetite-enhancing effects on vanilla flavors (Ogawa et al., 2018). Since farnesol acts as a solvent to make the fragrance stronger and more uniform when it is decomposed, it is usually used to enhance the fragrance of floral perfumes or aromatherapy oils (Chen and Viljoen, 2010).
Volatile compounds of 5-indanol and p-menth-1-en-8-ol were only identified in cut lilies on day 2. Previously, Johnson et al. (2016) found that Lilium cv. ‘Conca d’Or’ accumulated the most indole and the lowest 5-indanol unlike ‘Robina’, which has the inverse accumulation profile, implying that the content of nitrogen-containing volatile compounds in lilies in different varieties or at different postharvest times is different. On day 4, 5 volatile compounds existed in cut lilies (Table 1), among them, 3-methyl-1, 6-heptadien-3-ol, and p-allylphenol were first identified in flower petals, and methyl palmitoleate was previously found in honeysuckle (Lonicera japonica Thunb.) flowers (Ike Da et al., 2010).
Non-anoic acid was found in only 6-day-cut lilies (Table 1), which were also identified in Cymbidium sp. (Gaytán et al., 2013). Moreover, nonanoic acid, as a main volatile compound, was found in ten different varieties of Chinese jujubes (Chen et al., 2018). Furthermore, another acid, p-(benzyloxy) benzoic acid, only existed in 8-day-cut lily flowers (Table 1). A recent study revealed a new mechanism of acid for the promotion of cell senescence (Chen et al., 2021), which indicated that cut lilies on day 8 after harvest gradually showed a state of aging. These results further indicated the difference and diversity of volatile compounds during the vase period of cut lily flowers.
From a quantitative perspective, the total content of volatiles identified in cut lilies were analyzed, ranging from 5,897.79 (0 day), 7,953.47 (2 days), 10,156.39 (4 days), 4,961.12 (6 days) to 3,018.15 μg kg–1 (8 days) (Figure 5A). Approximately 39, 40, 49, 39, and 32 kinds of volatile compounds were identified in cut lily flowers at 0, 2, 4, 6, and 8 days, respectively (Figure 5A). The amount of alcohols, phenols, and esters first increased and then decreased, and the amount of these volatile compounds reached the highest on day 4. Interestingly, the amount of aldehydes and acids first declined, then rose, and then fell (Figure 5B). That is to say, cut lilies during the postharvest process might exhibit significantly different kinds and amounts of aroma compounds, which might be partly dependent on the growth period (Zhang et al., 2019).
Figure 5. Analysis of the total content and amount (A) of volatile compounds of cut lily flowers and the amount (B) and content (C) of various category volatile compounds of cut lily flowers and during the postharvest process. Different lowercase letters in the figure indicate significant differences at the level of P < 0.05.
During the vase period of lily, alcohol compounds that contributed to the aroma of cut lilies over 20% (25.41% on day 2 and 25.53% on day 4) were the most abundant in cut lily flowers. Among 8 alcohol compounds, 46.1 and 21.6% of the compounds were linalool and alpha-terpineol on day 4, respectively, which existed on all days and were the predominant volatile compounds in alcohols. Linalool and alpha-terpineol were also identified in other flower petals, such as Magnolia champaca (Dhandapani et al., 2017), fresh flowers of Cymbidium sp. (Gaytán et al., 2013), and Lavandula angustifolia (Smigielski et al., 2018). In addition, linalool and alpha-terpineol have been also identified as the main volatile components of essential oils of lavender flowers (Smigielski et al., 2018). Thus, we speculated that 4-day-cut lilies have the main volatile components of essential oils, which might be suitable for producing lily essential oil. The total content of aldehydes gradually decreased during the postharvest process, ranging from 0 day (2,363.18 μg kg–1) to 8 days (610.99 μg kg–1) (Figure 5C). This may be due to aldehydes that were converted into alcohols by dehydrogenase (Zhou et al., 2019). Among 10 aldehyde compounds, (E)-2-hexenal had the highest content, accounting for 46% of the total content of aldehydes, which were the main aroma components with contributions that over 47.4% on day 0. It is noteworthy that (E)-non-2-enal, (Z)-tetradec-9-enal, (E)-2-octenal, and (Z)-9-hexadecenal have not been reported in flowers before.
Esters synthesized through the oxidation of fatty acids (Chen et al., 2018) contribute to fruit and floral flavor. In our study, cut lilies on day 4 had the highest total content of esters (2,346.70 μg kg–1). Additionally, 2-hydroxybenzoic acid phenylmethyl ester and benzyl benzoate were the main compounds of esters and had the highest contents on day 4 (680.24 and 909.55 μg kg–1, respectively) (Figure 5C and Table 1), which was also identified from honeysuckle flowers in previous studies (Ike Da et al., 2010). Similarly, the total content of phenols showed the highest value (1,421.15 μg⋅kg–1) on day 4 (Figure 5C), and p-cresol and 3-hydroxy-4-methoxytoluene were the main compounds of phenols in lilies, which were also reported in the previous study (Johnson et al., 2016). Edible flowers are rich sources of phenolic compounds with antioxidants, DPPH radical-scavenging activity, and reducing power. Thus, this implies that 4-day lily flowers might have strong antioxidant capacity and flavor quality. Notably, 4-day-cut lily flowers might facilitate the extraction of phenols.
Ketone is one of the key aroma compounds for flavor. Although there were few amounts of ketones, it had higher contents in cut lily flowers on days 4 (747.40 μg kg–1) and 6 (1,102.60 μg kg–1) (Figure 5C and Table 1). Geranyl acetone and 6-methyl-5-hepten-2-one were the main compounds of ketones with contributions that were over 13%. In our study, acids showed lower contents (0.3–1.5%) (Figure 5C), and oleic acid and linoleic acid were first reported in this study. Since some alcohols and aldehydes undergo oxidation and other changes to form acid, the content of acid will gradually increase with the decrease of aldehydes and alcohols on days 6 and 8 after lily harvest. Therefore, cut lilies from different vase times might exhibit various kinds and quantities of the volatiles, leading to flavor change during the postharvest process. Thus, cut lily flowers on the 4th day of the vase could exhibit excellent flavor and ornamental quality.
Odor activity values refer to the ratio of the actual concentration of volatile compounds in the matrix to its odor threshold, which means the contribution of a volatile to the comprehensive flavor (Zhu et al., 2020b). Usually, when its OAVs are greater than 1, volatile components are considered to make a practical contribution. In our study, there were 19 volatiles with the OAVs over 1 that made contributions to the cut lily’s flavor, which were divided into eight categories of aroma, including floral, pungent, fruity, fatty, sweet, herbal, and vegetable (Table 2). The radar fingerprint chart (RFC) composed of cut lily flowers during the postharvest process is depicted in Figure 6, which makes the changes in the comprehensive odor of cut lilies clearer. The floral and fruity odors were the strongest scent, followed by the spicy odor. A previous study indicated that lily accessions were classified into six groups according to the composition of major scent components: faint-scented, fruity, cool, musky, fruity-honey, and lily (Du et al., 2019). In our study, the floral and fruity odors of cut lilies were richer, mainly including the floral of clove, carnation, rosy, and narcissus, and fruity of apples, oranges, melons, and citrus (Table 2), which make great contributions to the aroma of lilies.
Table 2. Odor activity values (OAVs) of the 19 most potent volatile compounds in cut lilies during the postharvest process.
Figure 6. Radar fingerprint chart of the 19 most potent volatile compounds in cut lily flowers during the postharvest process.
The floral odor first strengthened and then gradually weakened, reaching its strongest scent on day 4 (Figure 6). Due to a lower threshold of (E)-2-hexenal (4 μg kg–1) and its high concentration, it contributed greatly to the fruity and floral aromas of cut lilies with the OAVs above 200 on day 4. Under the action of alcohol dehydrogenase, aldehyde substrates were metabolized into alcohols, which were used as direct substrates to participate in the synthesis of ester aroma substances, then promoting the fragrance emission of volatile compounds (León-Sánchez et al., 2009). That may be the main reason why 4-day-cut lilies were stronger than 0- and 2-day-cut lilies in floral aroma intensity. However, OAVs of (E)-2-hexenal were between 1 and 7 in green cabbages (Wei et al., 2021), which may be due to the different activity and contribution of volatile compounds on different species. Subsequently, linalool also had a great contribution to the floral aroma of lilies and had the highest OAV (78.98 μg kg–1) on day 4. A previous study showed that (E)-2-hexenal had a negative correlation with the aroma quality of black tea, and the ratio of linalool to (E)-2-hexenal could be used to judge the quality of black tea aroma (Fernando and Roberts, 2010). That is to say, in our study, linalool with higher OAV and (E)-2-hexenal with lower OAV on day 4 might contribute greatly to lily flavor.
The fruity fragrances of lilies gradually weakened during the vase period of cut lilies (Figure 6). Wang et al. (2017) suggested that drying or thermal treatment could induce changes in some novel volatile substances and then change flavor quality. Thus, the weakness of the fruity flavor of lilies might be due to the water loss-induced drying after the postharvest process. In addition to (E)-2-hexenal, linalool, and 3-hydroxy-4-methoxytoluene, (E)-non-2-enal with a threshold value of 1.5 μg kg–1 significantly contributed to the fruity flavor. Notably, ketones (geranyl acetone and 6-methyl-5-hepten-2-one) with OAVs greater than 1 also contributed greatly to the fragrance of cut lilies (Table 2). Previously, 6-methyl-5-hepten-2-one had also been found in sun-dried raisins, whereas its OAVs were less than 1, thereby hardly contributing to the aroma of raisins (Wang et al., 2017). Interestingly, Smigielski et al. (2018) indicated that the essential oil from fresh lavender flowers received the highest marks among the distinguishing features: floral, fresh, and green. This implies that the floral and fruity might be the main odors for essential oil isolated from lily flowers, which needs to be further explored. Thus, the potential use of lily oil as cosmetics and food additives might be an important research topic in the future.
It is worth noting that spicy odor was a stronger scent of cut lilies, mainly including (E)-2-hexenal with higher OAVs and phenols of eugenol with lower OAVs. Eugenol was also identified in lily bulbs as a clove-like compound with a lower OAV (Chiang, 2016). Recently, Wei et al. (2021) indicated that the spicy odor played an indispensable role in the fragrance of cabbages and might be related to the regulation of the anthocyanin metabolism pathway. The green aroma was mainly comprised of hexanal, (E)-non-2-enal, and P-cresol and gradually weakened during the postharvest process (Figure 6), which might be the main reason why cut lilies were weaker on day 8 than those on other days. In addition, cut lilies after harvest also exhibited fatty, sweet, herbal, and vegetable aromas, which were low-intensity aromas relatively and were not toilless to be smelled in cut lilies for the masking effect when the floral, fruity, and pungent odors were strong. Thus, the overall OAVs of cut lilies on day 4 were universally much higher than those on other days, and the floral odor was the strongest scent, which hypothesized that the activities of related metabolic enzymes and the content of volatile precursors in cut lilies on day 4 were relatively higher.
The PCA was performed to observe possible sample distribution according to different postharvest times of cut lilies. The PCA plot presented in Figures 7A,B explained 76.74% of the total cumulative variance contribution. PC1 and PC2 accounted for 44.80 and 31.94% of the total variance, respectively, as shown in the score plot of PCA (Figure 7A). Similar to the results of the PCA of the E-nose, all lily samples in different postharvest times were clustered into three distinct groups in the dimensional graph based on the PC1 × PC2 score. The 0-day-cut lily samples were all located in the second quadrant of the graph, whereas samples on days 2 and 4 were in the first quadrant, and 6- and 8-day lily samples were localized in the third quadrant of the graph. Furthermore, Figure 7B shows the relationship of lily samples in different postharvest times with volatile compounds. It could be observed that volatile substances, namely, benzeneacetaldehyde (V8), pentadecane (V45), nerol (V20), and ethyl palmitate (V29) are highly and positively related to PC1, whereas (E)-2-hexenal (V1), (E)-hex-3-en-1-ol (V13), D-Limonene (V47), and benzyl alcohol (V23) have more contribution in PC2. In addition, it could also be found that unique volatile compounds of vanillin (V10), (E)-2-hexen-1-ol (V16), and farnesol (V17) and higher content compounds of (Z)-tetradec-9-enal (V4), (E)-2-Octenal (V5), and methyleugenol (V40) mainly distinguished 0 day-lily samples from other samples. The volatile compounds that contributed greatly to the separation of lily flowers on days 2 and 4 were benzyl benzoate (V28), benzoic acid, 2-phenylethyl ester (V31), and eugenol (V42). Finally, 6- and 8-day samples were characterized by benzyl alcohol (V23) and D-limonene (V47), which were correlated with PC1.
Figure 7. Principal component analysis of cut lilies in different days after harvest and 59 volatile compounds. (A) Score plot; (B) loading plot. The number code after “V” in (7B) also corresponds to the volatile code in Table 1.
The 59 kinds of volatile compounds and corresponding contents were the elements of this new data matrix, and the matrix with dimensions of 5 samples × 59 variables (volatiles) was constructed to perform HCA (Figure 8). The Euclidean distance of the GC-MS cluster map classification was consistent with the E-nose at 7.5. It could be observed from Figure 8 that cut lilies were evidently classified into 3 groups. Cut lilies on day 0 belonged to one group, 2- and 4-day lilies belonged to one group, and 6- and 8-day samples belonged to one another group. These classification groups were consistent with the PCA results, in which all lily samples were fully distinguished according to their vase time. In addition, three replicate samples at each postharvest time were closely related, which further proved the great repeatability within the group. Interestingly, the clustering results of GC-MS were also consistent with the E-nose. Therefore, it could be speculated that the E-nose and GC-MS are highly correlated in distinguishing the aroma changes of lily flowers during the postharvest process, which could be confirmed by PCA and HCA. In conclusion, the E-nose combined with GC-MS applied to the dynamic profile analysis of cut flower aroma was matching and corresponding, and it was highly efficient and meaningful in the flavor research and characteristic substance screening of cut flowers.
Figure 8. Hierarchical cluster analysis of cut lily flowers during the postharvest process based on the composition and content of their volatile compounds. Note: cut lilies on day 0 (1, 2, 3), on day 2 (4, 5, 6), on day 4 (7, 8, 9), on day 6 (10, 11, 12), and on day 8 (13, 14, 15).
The volatile profile of cut lilies during the vase period was identified by HS-SPME-GC-MS coupled with E-nose. E-nose analysis found that the response values of sensors W2W, W1C, and W5S were significantly higher than those of the other sensors on day 4, implying the emergence of flavor intensity on day 4 after lily harvest. A total of 59 volatiles were identified and quantified in cut lilies using HS-SPME-GC-MS methods, mainly including alcohols, aldehydes, esters, and phenols. The total content of volatiles was ranging from 5,897.79 (0 day), 7,953.47 (2 days), 10,156.39 (4 days), 4,961.12 (6 days) to 3,018.15 μg⋅kg–1 (8 days). Approximately 39, 40, 49, 39, and 32 kinds of volatile compounds were identified in lily flowers at 0, 2, 4, 6, and 8 days, respectively. Among them, there were 19 characteristic volatiles (OAVs > 1), making large contributions to the lily flavor. Floral and fruity aromas in lilies were stronger, followed by pungent aromas. Furthermore, E-nose and HS-SPME-GC-MS are highly correlated in distinguishing the aroma changes of lily flowers during the postharvest process, which could be confirmed by PCA and HCA. In summary, cut lily flowers on the 4th day of the vase might exhibit excellent flavor and aroma quality taking into consideration of the flavor intensity and diversity. These give a new insight into the mechanisms of flavor change and establish theoretical guidance for commercial application of cut flowers and extraction of characteristic volatiles during postharvest periods. Thus, further investigations are required focusing on the metabolic regulation of characteristic volatiles during the vase period of cut lily flowers.
The original contributions presented in this study are included in the article/Supplementary Material, further inquiries can be directed to the corresponding author.
LW: data curation, investigation, formal analysis, writing—original draft, and writing—review and editing. SW: investigation and formal analysis. DH and LF: formal analysis, investigation, and methodology. YL and HL: project administration. WL: conceptualization, funding acquisition, project administration, supervision, conceptualization, data curation, funding acquisition, project administration, and supervision. All authors contributed to the article and approved the submitted version.
This study was supported by the National Key Research and Development Program (2018YFD1000800), the National Natural Science Foundation of China (Nos. 32072559, 31860568, 31560563, and 31160398), the Research Fund of Higher Education of Gansu, China (Nos. 2018C-14 and 2019B-082), the Post-Doctoral Foundation of China (Nos. 20100470887 and 2012T50828), and the Natural Science Foundation of Gansu Province, China (Nos. 1606RJZA073 and 1606RJZA077).
The authors declare that the research was conducted in the absence of any commercial or financial relationships that could be construed as a potential conflict of interest.
All claims expressed in this article are solely those of the authors and do not necessarily represent those of their affiliated organizations, or those of the publisher, the editors and the reviewers. Any product that may be evaluated in this article, or claim that may be made by its manufacturer, is not guaranteed or endorsed by the publisher.
The Supplementary Material for this article can be found online at: https://www.frontiersin.org/articles/10.3389/fpls.2022.822956/full#supplementary-material
Asjes, C. J. (2000). Control of aphid-borne lily symptomless virus and lily mottle virus in lilium in the netherlands. Virus Res. 71, 23–32. doi: 10.1016/S0168-1702(00)00185-4
Azuma, H., Toyota, M., and Asakawa, Y. (2001). Intraspecific variation of floral scent chemistry in Magnolia kobus DC. (Magnoliaceae). J. Plant Res. 114, 411–422. doi: 10.1007/PL00014006
Bruni, A., Ballero, M., and Poli, F. (1997). Quantitative ethnopharmacological study of the campidano valley and urzulei district, Sardinia, Italy. J. Ethnopharmacol. 57, 97–124. doi: 10.1016/S0378-8741(97)00055-X
Chen, Q. Q., Song, J. X., Bi, J. F., Meng, X. J., and Wu, X. Y. (2018). Characterization of volatile profile from ten different varieties of Chinese jujubes by HS-SPME/GC-MS coupled with E-nose. Food Res. Int. 105, 605–615. doi: 10.1016/j.foodres.2017.11.054
Chen, W. P., Yu, X. L., Wu, Y. S., Tang, J., Yu, Q., Lv, X. D., et al. (2021). The SESAME complex regulates cell senescence through the generation of acetyl-CoA. Nat. Metab. 3, 983–1000. doi: 10.1038/s42255-021-00412-9
Chen, W., and Viljoen, A. M. (2010). Geraniol-a review of a commercially important fragrance material. S. Afr. J. Bot. 76, 643–651. doi: 10.1016/j.sajb.2010.05.008
Chiang, N. (2016). Determination of Key Aroma Active Compounds in Raw and Roasted Lily Bulbs (BAI HE) - an Ingredient in Chinese Cuisine. Chapter1. New Jersey, NJ: Rutgers, The State University of New Jersey.
Dai, C., Huang, X., Lv, R., Zhang, Z., and Aheto, J. H. (2018). Analysis of volatile compounds of Tremella aurantialba fermentation via electronic nose and HS-SPME-GC-MS. J. Food Saf. 38:e12555. doi: 10.1111/jfs.12555
Dhandapani, S., Jin, J. J., Sridhar, V., Sarojam, R., Chua, N. H., and Jang, I. C. (2017). Integrated metabolome and transcriptome analysis of Magnolia champaca identifies biosynthetic pathways for floral volatile organic compounds. BMC Genom. 18:463. doi: 10.1186/s12864-017-3846-8
Dong, W., Hu, R., Long, Y., Li, H., Zhang, Y., Zhu, K., et al. (2019). Comparative evaluation of the volatile profiles and taste properties of roasted coffee beans as affected by drying method and detected by electronic nose, electronic tongue, and HS-SPME-GC-MS. Food Chem. 272, 723–731. doi: 10.1016/j.foodchem.2018.08.068
Dong, Z., Gu, F., Xu, F., and Wang, Q. (2014). Comparison of four kinds of extraction techniques and kinetics of microwave-assisted extraction of vanillin from Vanilla planifolia Andrews. Food Chem. 15, 54–61. doi: 10.1016/j.foodchem.2013.10.052
Du, F., Wang, T., Fan, J. M., Liu, Z. Z., Zong, J. X., and Fan, W. X. (2019). Flower aroma types and identification, polymorphisms, and alternative splicing of their monoterpene synthase genes. Hortic. Res. 6:110. doi: 10.1038/s41438-019-0192-9
Emre, O., and Bekir, K. (2017). Quality control of olive oils using machine learning and electronic nose. J. Food Qual. 2017, 1–7. doi: 10.1155/2017/9272404
Fan, Y., Yin, L., Xue, Y., Li, Z. J., Hou, H., and Xue, C. (2017). Analyzing the flavor compounds in Chinese traditional fermented shrimp pastes by HS-SPME-GC/MS and electronic nose. J. Ocean Univ. China 16, 311–318. doi: 10.1007/s11802-017-3194-y
Fernando, V., and Roberts, G. R. (2010). The effect of process parameters on seasonal development of flavour in black tea. J. Sci. Food Agr. 35, 71–76. doi: 10.1002/jsfa.2740350112
Francis, J. A., Rumbeiha, W., and Nair, M. G. (2004). Constituents in easter lily flowers with medicinal activity. Life Sci. 76, 671–683. doi: 10.1016/j.lfs.2004.10.001
Gaytán, V. G., Mendoza, M., Hernández, M. S., Trejo-Téllez, L. I., Santos, M., and Ponce, G. V. (2013). Volatile components in the flower, pedicellate ovary and aqueous residue of Cymbidium sp. (orchidaceae). J. Anal. Sci. Methods Instrum. 3, 212–218. doi: 10.4236/jasmi.2013.34027
Ike Da, N., Ishihara, M., Tsuneya, T., Kawakita, M., and Inui, M. (2010). Volatile components of honeysuckle (Lonicera japonica thunb.) flowers. Flavour Frag. J. 9, 325–331. doi: 10.1002/ffj.2730090609
Johnson, T., Schwieterman, M. L., Kim, J. Y., Cho, K. H., and Colquhoun, T. A. (2016). Lilium floral fragrance: a biochemical and genetic resource for aroma and flavor. Phytochemistry 122, 103–112. doi: 10.1016/j.phytochem.2015.11.010
Kang, Y. I., Choi, Y. J., Lee, Y. R., Seo, K. H., Suh, J. N., and Lee, H. R. (2021). Cut Flower characteristics and growth traits under Salt Stressin Lily Cultivars. Plants 10:1435. doi: 10.3390/plants10071435
León-Sánchez, F. D. D., Pelayo-Zaldívar, C., Rivera-Cabrera, F., Ponce-Valadez, M., Ávila-Alejandre, X., Fernández, F. J., et al. (2009). Effect of refrigerated storage on aroma and alcohol dehydrogenase activity in tomato fruit. Postharvest Biol. Tec. 54, 93–100. doi: 10.1016/j.postharvbio.2009.07.003
Li, Q., Yu, X. Z., Xu, L. R., and Gao, J. M. (2017). Novel method for the producing area identification of Zhongning Goji berries by electronic nose. Food Chem. 211, 1113–1119. doi: 10.1016/j.foodchem.2016.11.049
Liao, W. B., Zhang, M. L., and Yu, J. H. (2013). Role of ntric oxide in delaying senescence of cut rose flowers and its interaction with ethylene. Sci. Hortic. 155, 30–38. doi: 10.1016/j.scienta.2013.03.005
Liu, Y., He, C., and Song, H. (2018). Comparison of fresh watermelon juice aroma characteristics of five varieties based on gas chromatography-olfactometry-mass spectrometry. Food Res. Int. 107, 119–129. doi: 10.1016/j.foodres.2018.02.022
Lv, S. D., Wu, Y. S., Song, Y. Z., Zhou, J. S., Lian, M., Wang, C., et al. (2015). Multivariate analysis based on GC-MS fingerprint and volatile composition for the quality evaluation of Pu-erh green tea. Food Anal. Method 8, 321–333. doi: 10.1007/s12161-014-9900-0
Ma, G., Shi, X., Zou, Q., Tian, D., An, X., and Zhu, K. (2018). iTRAQ-based quantitative proteomic analysis reveals dynamic changes during daylily flower senescence. Planta 248, 859–873. doi: 10.1007/s00425-018-2943-5
Melo, A. S., Leite, W., Kato, M. T., and Barros, K. K. (2021). Essential oil and linalool contents in basil (Ocimum basilicum) irrigated with reclaimed water. J. Water Supply 70, 773–782. doi: 10.2166/aqua.2021.057
Mirzaee-Ghaleh, E., Taheri-Garavand, A., Ayari, F., and Lozano, J. (2019). Identification of fresh-chilled and frozen-thawed chicken meat and estimation of their shelf life using an E-nose machine coupled fuzzy knn. Food Anal. Method 13, 678–689. doi: 10.1007/s12161-019-01682-6
Mlcek, J., and Rop, O. (2011). Fresh edible flowers of ornamental plants – a new source of nutraceutical foods. Trends Food Sci. Tech. 22, 561–569. doi: 10.1016/j.tifs.2011.04.006
Ogawa, K., Tashima, A., Sadakata, M., and Osamu, M. (2018). Appetite-enhancing effects of vanilla flavours such as vanillin. J. Nat. Med. 72, 798–802. doi: 10.1007/s11418-018-1206-x
Pichersky, E., Raguso, R. A., Lewinsohn, E., and Croteau, R. (1994). Floral scent production in Clarkia (Onagraceae) (I. Localization and developmental modulation of monoterpene emission and linalool synthase activity). Plant Physiol. 106, 1533–1540. doi: 10.1104/pp.114.1.213
Pieroni, A. (2000). Medicinal plants and food medicines in the folk traditions of the upper Lucca province. Italy J. Ethnopharmacol. 70, 235–273. doi: 10.1016/S0378-8741(99)00207-X
Pino, J. A., and Mesa, J. (2010). Contribution of volatile compounds to mango (Mangifera indica) aroma. Flavour Frag. J. 21, 207–213. doi: 10.1002/ffj.1703
Ren, P. J., Jin, X., Liao, W. B., Wang, M., Niu, L. J., and Li, X. P. (2017). Effect of hydrogen-rich water on vase life and quality in cut lily and rose flowers. Hortic. Environ. Biotechnol. 58, 576–584. doi: 10.1007/s13580-017-0043-2
Rusanov, K., Kovacheva, N., Rusanova, M., and Atanassov, I. (2011). Traditional Rosa damascena flower harvesting practices evaluated through gc/ms metabolite profiling of flower volatiles. Food Chem. 129, 1851–1859. doi: 10.1016/j.foodchem.2011.05.132
Shi, S., Duan, G., Li, D., Wu, J., Liu, X., and Hong, B. (2018). Two-dimensional analysis provides molecular insight into flower scent of lilium ‘siberia’. Sci. Rep. 8:5352. doi: 10.1038/s41598-018-23588-9
Smigielski, K., Prusinowska, R., Stobiecka, A., Kunicka-Styczyska, A., and Gruska, R. (2018). Biological properties and chemical composition of essential oils from flowers and aerial parts of lavender (Lavandula angustifolia). J. Essent. Oil bearing Plants 21, 1–12. doi: 10.1080/0972060X.2018.1503068
van Gemert, L. J. (2003). Compilations of Odour Threshold Values in Air, Water and Other Media. The Netherlands: Oliemans Punter & Partners.
Walde, S. G., Jyothirmayi, T., Rao, P. G. P., and Srinivas, P. (2006). Flavour volatiles of flowers and stalks of Murraya koenigii L. Flavour Frag. J. 21, 581–584.
Wang, D., Duan, C. Q., Shi, Y., Zhu, B. Q., Umer, J. H., and Wang, J. (2017). Free and glycosidically bound volatile compounds in sun-dried raisins made from different fragrance intensities grape varieties using a validated HS-SPME with GC-MS method. Food Chem. 228, 125–135. doi: 10.1016/j.foodchem.2017.01.153
Wani, M. A., Nazki, I. T., Din, A., Malik, S. A., and Rather, Z. A. (2016). Photosynthate partitioning in asiatic lilies under ammoniacal and nitrate sources of nitrogen. Agr. Res. 5, 230–235. doi: 10.1007/s40003-016-0222-x
Wei, S. H., Xiao, X. M., Wei, L. J., Li, L. S., Li, G. C., Liu, F. H., et al. (2021). Development and comprehensive HS-SPME/GC-MS analysis optimization, comparison, and evaluation of different cabbage cultivars (Brassica oleracea L. var. capitata L.) volatile components. Food Chem. 340:128166. doi: 10.1016/j.foodchem.2020.128166
Wilson, A. D., and Ba Ietto, M. (2011). Advances in electronic-nose technologies developed for biomedical applications. Sensors 11, 1105–1176. doi: 10.3390/s110101105
Yang, L., Nuerbiye, A., Cheng, P., Wang, J. H., and Li, H. (2017). Analysis of floral volatile components and antioxidant activity of different varieties of Chrysanthemum morifolium. Molecules 22:1790. doi: 10.3390/molecules22101790
Yin, D. D., Yuan, R. Y., Wu, Q., Li, S. S., Shao, S., Xu, Y. J., et al. (2015). Assessment of flavonoids and volatile compounds in tea infusions of water lily flowers and their antioxidant activities. Food Chem. 187, 20–28. doi: 10.1016/j.foodchem.2015.04.032
Zaccai, M., Yarmolinsky, L., Khalfin, B., Budovsky, A., Gorelick, J., Dahan, A., et al. (2020). Medicinal Properties of Lilium candidum L. and Its Phytochemicals. Plants 9:959. doi: 10.3390/plants9080959
Zhang, J., Fang, H., Huo, J. Q., Huang, D. J., Wang, B., and Liao, W. B. (2018a). Involvement of calcium and calmodulin in nitric oxide-regulated senescence of cut lily flowers. Front. Plant Sci. 9:1284. doi: 10.3389/fpls.2018.01284
Zhang, T., Ming, S., Guo, Y., Shi, X., and Ahmad, S. (2018b). Overexpression of lidxs and lidxr from lily (Lilium ‘siberia’) enhances the terpenoid content in tobacco flowers. Front. Plant Sci. 9:909. doi: 10.3389/fpls.2018.00909
Zhang, W., Tan, S., Xi, W., Yang, J., Liao, Q., Lan, J., et al. (2019). Comparison of volatile components in fresh and dried Zanthoxylum bungeanum maxim. Food Sci. Biotechnol. 28, 1083–1092. doi: 10.1007/s10068-019-00562-3
Zhang, Y., Zhong, D., Liu, Z., and Gao, J. (2021). Study on the physiological, cellular, and morphological aspects of the postharvest development of cut lily flowers. Hortic. Plant J. 7, 149–158. doi: 10.1016/j.hpj.2021.02.005
Zhou, Z., Wu, Q., Yao, Z., Deng, H., and Sun, Y. (2019). Dynamics of ADH and related genes responsible for the transformation of C6-aldehydes to C6-alcohols during the postharvest process of oolong tea. Food Sci. Nutr. 8, 104–113. doi: 10.1002/fsn3.1272
Zhu, C., Lu, Q., Zhou, X., Li, J., Yue, J., Wang, Z., et al. (2020a). Metabolic variations of organic acids, amino acids, fatty acids and aroma compounds in the pulp of different pummelo varieties. LWT-Food Sci. Technol. 130:109445. doi: 10.1016/j.lwt.2020.109445
Zhu, J. C., Niu, Y., and Xiao, Z. B. (2020b). Characterization of the key aroma compounds in laoshan green teas by application of odour activity value (OAV), gas chromatography-mass spectrometry-olfactometry (GC-MS-O) and comprehensive two-dimensional gas chromatography mass spectrometry (gc×gc-qms). Food Chem. 339, 128–136. doi: 10.1016/j.foodchem.2020.128136
Keywords: cut lily flowers, postharvest, volatile components, E-nose, HS-SPME/GC-MS, OAVs
Citation: Wei L, Wei S, Hu D, Feng L, Liu Y, Liu H and Liao W (2022) Comprehensive Flavor Analysis of Volatile Components During the Vase Period of Cut Lily (Lilium spp. ‘Manissa’) Flowers by HS-SPME/GC–MS Combined With E-Nose Technology. Front. Plant Sci. 13:822956. doi: 10.3389/fpls.2022.822956
Received: 29 November 2021; Accepted: 23 May 2022;
Published: 17 June 2022.
Edited by:
Jens Rohloff, Norwegian University of Science and Technology, NorwayReviewed by:
Xiaohong Kou, Tianjin University, ChinaCopyright © 2022 Wei, Wei, Hu, Feng, Liu, Liu and Liao. This is an open-access article distributed under the terms of the Creative Commons Attribution License (CC BY). The use, distribution or reproduction in other forums is permitted, provided the original author(s) and the copyright owner(s) are credited and that the original publication in this journal is cited, in accordance with accepted academic practice. No use, distribution or reproduction is permitted which does not comply with these terms.
*Correspondence: Weibiao Liao, bGlhb3diQGdzYXUuZWR1LmNu
Disclaimer: All claims expressed in this article are solely those of the authors and do not necessarily represent those of their affiliated organizations, or those of the publisher, the editors and the reviewers. Any product that may be evaluated in this article or claim that may be made by its manufacturer is not guaranteed or endorsed by the publisher.
Research integrity at Frontiers
Learn more about the work of our research integrity team to safeguard the quality of each article we publish.