- College of Grassland Science and Technology, Sichuan Agricultural University, Chengdu, China
Alteration of ploidy in one particular plant species often influences their environmental adaptation. Warm-season bermudagrass is widely used as forage, turfgrass, and ground-cover plant for ecological remediation, but exhibits low shade tolerance. Adaptive responses to shade stress between triploid hybrid bermudagrass cultivars [“Tifdwarf” (TD), “Tifsport” (TS), and “Tifway” (TW)] and tetraploid common bermudagrass cultivar “Chuanxi” (CX) were studied based on changes in phenotype, photosynthesis, and secondary metabolites in leaves and stems. Shade stress (250 luminance, 30 days) significantly decreased stem diameter and stem internode length, but did not affect the leaf width of four cultivars. Leaf length of CX, TD, or TW showed no change in response to shade stress, whereas shade stress significantly elongated the leaf length of TS. The CX and the TS exhibited significantly higher total chlorophyll (Chl), Chl a, carotenoid contents, photosynthetic parameters [PSII photochemical efficiency (Fv/Fm), transpiration rate, and stomatal conductance] in leaves than the TW and the TD under shade stress. The CX also showed a significantly higher performance index on absorption basis (PIABS) in leaf and net photosynthetic rate (Pn) in leaf and stem than the other three cultivars under shade stress. In addition, the TS maintained higher proantho cyanidims content than the TW and the TD after 30 days of shade stress. Current results showed that tetraploid CX exhibited significantly higher shade tolerance than triploid TD, TS, and TW mainly by maintaining higher effective photosynthetic leaf area, photosynthetic performance of PSI and PSII (Pn and Fv/Fm), and photosynthetic pigments as well as lower Chl a/b ratio for absorption, transformation, and efficient use of light energy under shade stress. For differential responses to shade stress among three triploid cultivars, an increase in leaf length and maintenance of higher Fv/Fm, gas exchange, water use efficiency, carotenoid, and proanthocyanidin contents in leaves could be better morphological and physiological adaptations of TS to shade than other hybrid cultivars (TD and TW).
Introduction
Light is an indispensable ecological factor for plant growth and development. Weak or intense light affects plant morphogenesis and photosynthesis depending on different species, such as C3 and C4 plants. As compared to C3 plants, the C4 plants need more lights in their life cycle. Photosynthesis provides energy for plant life activities and also controls plant morphogenesis. However, net photosynthetic rate (Pn) always decreases with increasing shade, especially in C4 plants (Kubásek et al., 2013; Xie et al., 2020). The shade also affects other photosynthetic characteristics, such as inconsistent light saturation and compensation points, as well as declines in non-photochemical burst capacity and electron transfer rate (Huang et al., 2011; Kim et al., 2011). In addition to physiological changes including declines in photosynthesis, transpiration rate (Tr), and carbohydrate accumulation, shade induces morphological alterations, as demonstrated by thinner leaves and stems, longer leaves and internodes, lower shoot density, and more upright growth (Fankhauser and Batschauer, 2016). In nature, shade also modifies microenvironmental conditions leading to high air relative humidity, less air movement, and high soil moisture content, which promotes the possibility of disease occurrence. For most fruit trees and vegetables, the shade limits their yield and quality (Allan and Carlson, 2003; Stier, 2001). Horticultural plants including various flowers, ornamental grasses, and turfgrasses often suffer from more shade situations than trees since they have a lower ecological niche (Pires et al., 2011; Fan et al., 2019). Shade stress caused by tress or buildings decreases turf quality and also increases turf maintenance costs. Insufficient light affects crop growth and development resulting in a significant reduction in crop dry matter accumulation and yield (Demotes-Mainard and Jeuffroy, 2004), such as 20% reduction in rice yield, under low light (Estrada-Campuzano et al., 2008). Thus, the breeding and application of shade-enduring plants are of great significance to agriculture and horticulture.
Reactive oxygen species (ROS) caused by shade oxidize plant cell membranes leading to an increase in cell membrane permeability. To cope with weak light environments, plants have evolved multiple adaptive pathways involved in the regulation of plant growth, physiology, and metabolic changes (Xie et al., 2017; Esmailpourmoghadam and Salehi, 2021). An enhanced antioxidant defense system is one of the most effective pathways to remove ROS, thereby reducing oxidative damage under shade stress. Previous studies have reported that higher activities of antioxidant enzymes, such as superoxide dismutase (SOD), peroxidase (POD), ascorbate peroxidase (APX), and catalase (CAT), were associated with better shade tolerance in buffalograss (Buchloe dactyloides) (Ren et al., 2017) and centipedegrass (Eremochloa ophiuroides) (Zhou and Cao, 2006). Except for antioxidant enzymes, some of the organic metabolites including carotenoids (CR), flavonoids (FA), and proantho cyanidins (PC) with stronger antioxidant properties play vital roles in scavenging ROS to alleviate oxidative damage to cells in plants. CR has the ability to absorb and dissipate excess light energy, which is an important mechanism of photoprotection in plants (Takahashi and Badger, 2011; Son et al., 2021). In addition, the CR is also an excellent scavenging agent of single-linear oxygen (Zakar et al., 2016). It has been shown that the FA exhibits a stronger scavenging capacity for ROS than vitamin C and E analogs under stress conditions (Agati and Tattini, 2010). Plants that grow under low light increased CR and chlorophyll (Chl) b contents as compared to those plants that grow under normal conditions (Huang et al., 2011). The PC is a key component of phytochrome and is also known as a condensed tannin involved in defensive adaptation against biotic and abiotic stresses (Debeaujon et al., 2003). Plants accumulated more PC in the upper sunny leaves than that in the lower shady leaves (Mole et al., 1988). In addition, it has been shown that enhanced UV radiation-induced PC accumulation in birch (Betula platyphylla) seedlings (Anu, 1998).
During the process of plant evolution, polyploidization alters botanical morphology, which is characterized by taller shoots, bigger leaves, and improved biomass (Abdolinejad et al., 2021; Hu et al., 2021). Polyploidization also could be beneficial for plants to develop better adaption to various environmental stresses. For example, tetraploid sea barley (Hordeum marinum) materials exhibited better drought tolerance than their diploid ancestors (Zhou et al., 2019). Bermudagrass (Cynodon dactylon) is one of the most important and popular warm-season C4 grass species for landscape greening and sports turf (Li et al., 2011). Common bermudagrass is rich in wild germplasms with a coarse texture and aggressive rhizomes. Triploid hybrid bermudagrass has been developed by crossbreeding tetraploid common bermudagrass with diploid African bermudagrass (Cynodon transvaalensis), which produces many genotypes, such as “Tifdwarf,” “Tifsport,” and “Tifway,” with a finer texture and lower mowing height than common bermudagrass. These hybrid cultivars are more suitable for use in golf course and other athletic fields. However, one of the main disadvantages of hybrid bermudagrass cultivars is a weak tolerance to shade stress (Huang et al., 2019). It has been reported that approximately one-quarter of ground cover plants in the city suffer from shade stress, which is derived from tall buildings, broad-leaved trees, conifers, etc. (Bell et al., 2000). Richardson et al. (2019) concluded that the shade degraded the turf quality of bermudagrass and also increased the cost of management and maintenance of bermudagrass turf in football court. The study of Trappe et al. (2011) found that there were significant variations among hybrid bermudagrasses because “Princess 77” and “Riviera” showed higher coverage than “Patriot,” “Tifsport,” and “Tifway” when these cultivars were grown under some shade condition. However, differential responses to shade between leaf and stem, as well as a potential mechanism of shade tolerance between tetraploid and triploid bermudagrasses, are worth further investigation.
After more than 10 years of selective breeding, a new common bermudagrass cultivar “Chuanxi” (CX) was developed. The new cultivar exhibits a finer texture, lower mowing height, and longer green period than other common bermudagrass and could be used for landscaping and ecological remediation. Most of the previous studies mainly focused on leaves in response to shade, but the adaptive mechanism of stems was not fully understood under low-light conditions. The purpose of this study is (1) to evaluate shade tolerance of one tetraploid common cultivar CX and three triploid hybrid cultivars “Tifdwarf” (TD), “Tifsport” (TS), and “Tifway” (TF) and (2) to further reveal the mechanism of shade tolerance between common and hybrid bermudagrasses associated with phenotypic alteration, photosynthetic performance, and secondary metabolites. Current findings will provide effective information about the differential mechanism of shade tolerance between tetraploid and triploid plant species.
Materials and Methods
Plant Materials and Treatment
Tetraploid common bermudagrass (Cynodon dactylon) cultivar “Chuanxi” (CX) and three triploid hybrid bermudagrass (Cynodon transvaalensis × Cynodon dactylon) cultivars “Tifdwarf” (TD), “Tifsport” (TS), and “Tifway” (TW) were selected as experimental materials. Stoloniform stems were obtained from the resource nursery in the Research Farm of Sichuan Agricultural University (Chongzhou, Sichuan, China). Stoloniform stems were planted in white PVC tubes (33 cm high and 11 cm diameter) containing sands and soils (1:1, v:v). Each tube included six independent stems and each stem had three internodes. All the materials were cultivated and managed regularly including sand covering, pruning, watering, and fertilizing once a week in the greenhouse (average 29/25°C (day/night), 60% relative humidity, and 700–800 μmol⋅m–2⋅s –1 PAR). To form a dense lawn, the mowing height of all materials was maintained at 1.5 cm. After two and a half months of establishment in the greenhouse, all materials were moved into growth chambers for 7 days of acclimation to growth chamber condition before stress treatment [30/26°C (day/night), 60% relative humidity, and 750 μmol⋅m–2⋅s –1 PAR]. Then, half of the materials of each cultivar were transferred to low-light growth chambers for shade stress [30/26°C (day/night), 60% relative humidity, and 250 μmol⋅m–2⋅s –1 PAR]. After 30 days of cultivation under normal conditions or shade stress, leaf and stem samples were taken for determination and data analysis. Each treatment included four independent biological replications (four tubes).
Determination of Plant Growth and Water Status
Leaf length (LL) and leaf width (LW) of the first fully expanded leaf and stem internode length (SIL) of the third stem node were measured by using a ruler (accuracy of 1 mm); the stem diameter (SD) was measured by using a vernier caliper (accuracy of 0.02 mm). Stoloniferous stems were selected randomly from each pot for determination. The relative water content in the leaves was calculated by the formula RWC (%) = [(FW-DW)/(TW-DW)] × 100, where FW is the fresh weight, TW is the saturated fresh weight, and DW is the dry weight (Barrs and Weatherley, 1962). For the determination of osmotic potential (OP), collected fresh leaves and stems were immediately soaked in distilled water for 8 h. the water-saturated leaves and stems were immediately frozen in liquid nitrogen for 8 min and then completely thawed at 4°C. The OP of saps in leaves was measured by an osmotic potential instrument (Wescor, Logan, UT, United States). The OP was calculated based on OP (MPa) = −c × 2.58 × 10–3.
Determination of Chlorophyll Content and Photosynthetic Parameters
For Chl content, 0.1 g fresh leaves or stems were soaked in a 10 ml extraction solution (95% ethanol: 80% acetone, volume 1:1) in the dark until all tissues turned white. The absorbance value of the extraction solution was determined at 470, 645, and 663 nm with a spectrophotometer (Spectronic Instruments, Rochester, NY) (Arnon, 1949). For the determination of Chl fluorescence parameters including photochemical efficiency of PSII (Fv/Fm) and performance index on absorption basis (PIABS), leaves were placed in the dark for 30 min by using the leaf clamping. Fv/Fm and PIABS were recorded by a Chl fluorescence system (Pocket PEA, Hansatech, Norfolk, United Kingdom). Photosynthetic parameters including net photosynthetic rate (Pn), Tr, stomatal conductance (Gs), and water use efficiency (WUE) were measured by a portable photosynthesis system (CIRAS-3, PP Systems, Norfolk, United Kingdom). Leaves or stems were placed in the leaf chamber (400 μl CO2 L –1 and 800 μmol photon m –2 red and blue light) for determination. Leaf temperature or relative humidity was maintained at 25°C or 60%, respectively. Leaf samples were then cut from plants and scanned with Magic W and TM Portable Scanner (PDS-ST415-VPS, VuPoint Solutions) to measure a leaf or stem area which was used to calculate Pn, Tr, Gs, and WUE (Liang et al., 2021).
Determination of Organic Metabolites
Water-soluble carbohydrate (WSC) content was measured according to the method of Li et al. (2015). Briefly, dry leaves or stems were ground into fine powders and 0.05 g of the powders were extracted with 2 ml of 80% (V/V) methanol in 80°C water bath for 40 min. The extract was centrifuged at 5,000 g for 10 min, and the supernatant was taken for determining the WSC. The reaction mixture (1 ml of supernatant, 4 ml of 98% sulfuric acid, and 1 ml of 5% phenol) was heated to 100°C in a water bath for 10 min. The cooled reaction mixture was determined at 490 nm with D-glucose as the standard. Flavonids (FA) content (Art. No. G00118W) and proantho cyanidins (PC) content (Art. No. G0120W) were determined by using test kits (Suzhou Comin Biotechnology Company) according to the manufacturer’s instructions.
Data Analysis
SPSS 25 (IBM, Armonk, NY, United States) was used for all data and PCA analyses. Significant differences among TD, TS, TW, and CX varieties were tested at p ≤ 0.05. Stress index (SI) was used to evaluate stress tolerance (SI = stress parameter/normal parameter × 100) (Zhang et al., 2020), which presented a relative change of one particular parameter between stress condition and normal conditions. Heat map data were processed as log2 (SI/100).
Results
Changes in Phenotype and Growth Parameters in Response to Shade
Phenotypic changes showed that the leaf color of four different cultivars turned yellow after 30 days of shade stress (Figure 1A). Under normal conditions, TS had significantly the smallest LL and LW than the other three cultivars (Figures 1B,C). However, the LW and SIL of CX were significantly higher than the other cultivars under normal conditions (Figures 1C,E). There was no significant difference in LL among TD, TW, TS, and CX under shade stress (Figure 1B). Under shade stress, the LW, SD, and SIL of CX were significantly higher than those of the other three cultivars (Figures 1C–E). The LW of TS and the SD of TW were lowest than those of other cultivars under shade stress (Figures 1C,D). The decrement of SD and SIL was significant in all cultivars in response to shade stress (Figures 1D,E).
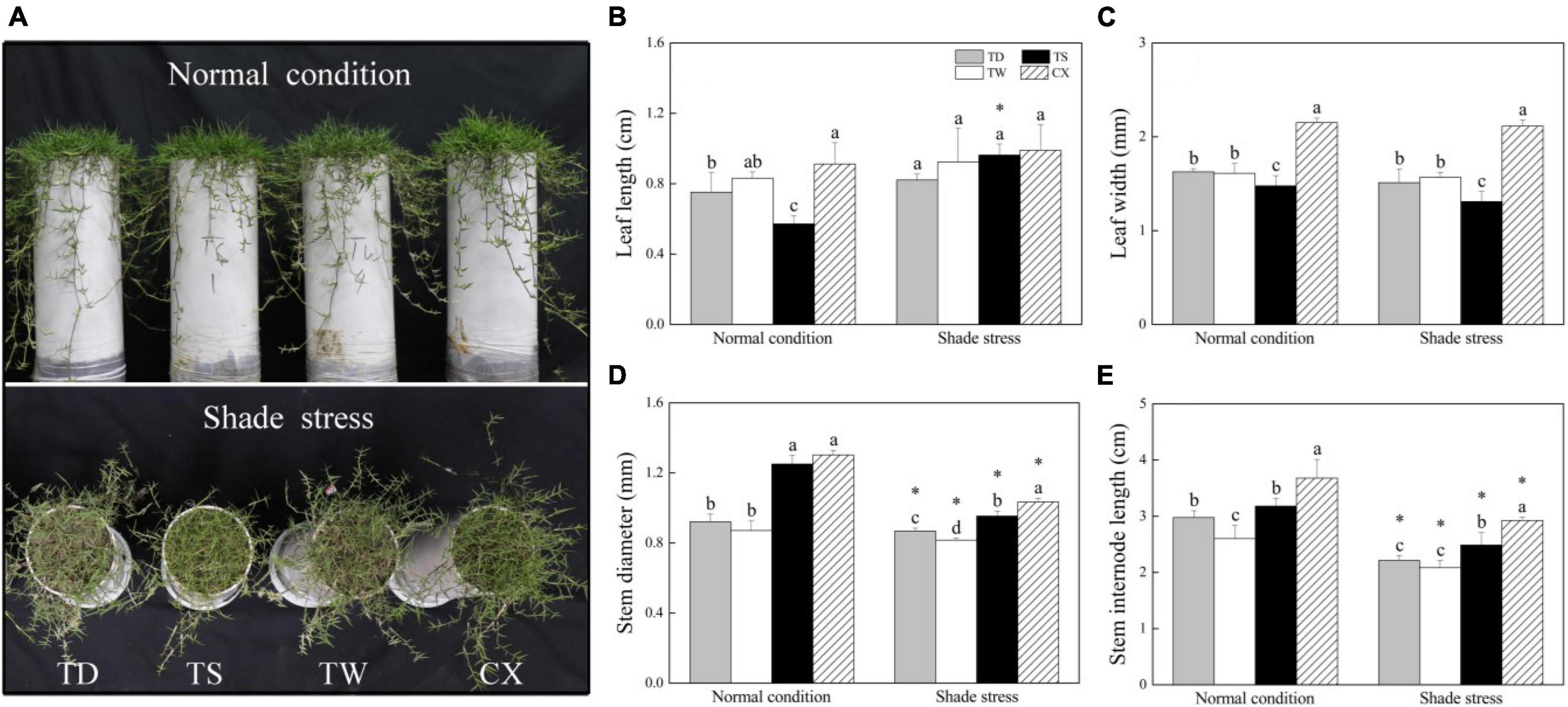
Figure 1. Effects of shade stress on (A) phenotype, (B) leaf length, (C) leaf width, (D) stem diameter, and (E) stem internode length of four bermudagrass cultivars. Vertical bars indicate the ± SE of mean (n = 5). Different letters above columns indicate significant differences (p ≤ 0.05) among the four cultivars under normal conditions or shade stress. The asterisk (*) above the letter indicates a significant difference (p ≤ 0.05) in one particular cultivar between normal conditions and shade stress.
Changes in Leaf Relative Water Content and Osmotic Potential in Response to Shade
Under normal conditions, the RWC in leaves of TD and TW was significantly higher than that in the leaves of CX, but CX exhibited significantly higher leaf RWC than the other three cultivars under shade stress (Figure 2A). The RWC in stems was not significantly different among four cultivars under normal conditions, and the TS showed significantly higher RWC than other cultivars in stems under shade stress (Figure 2B). There was no significant difference in OP between TW and TD under normal conditions and shade stress, and the OP in leaves of TW and TD was significantly lower than that in leaves of TS and CX under normal and shade stress conditions (Figure 2C). The shade stress significantly decreased OP in the leaves of all cultivars (Figure 2C). In stems, there were no significant differences in OP among the four cultivars under normal conditions (Figure 2D). Under shade stress, the TS maintained the lowest OP than the other three cultivars in stems (Figure 2D).
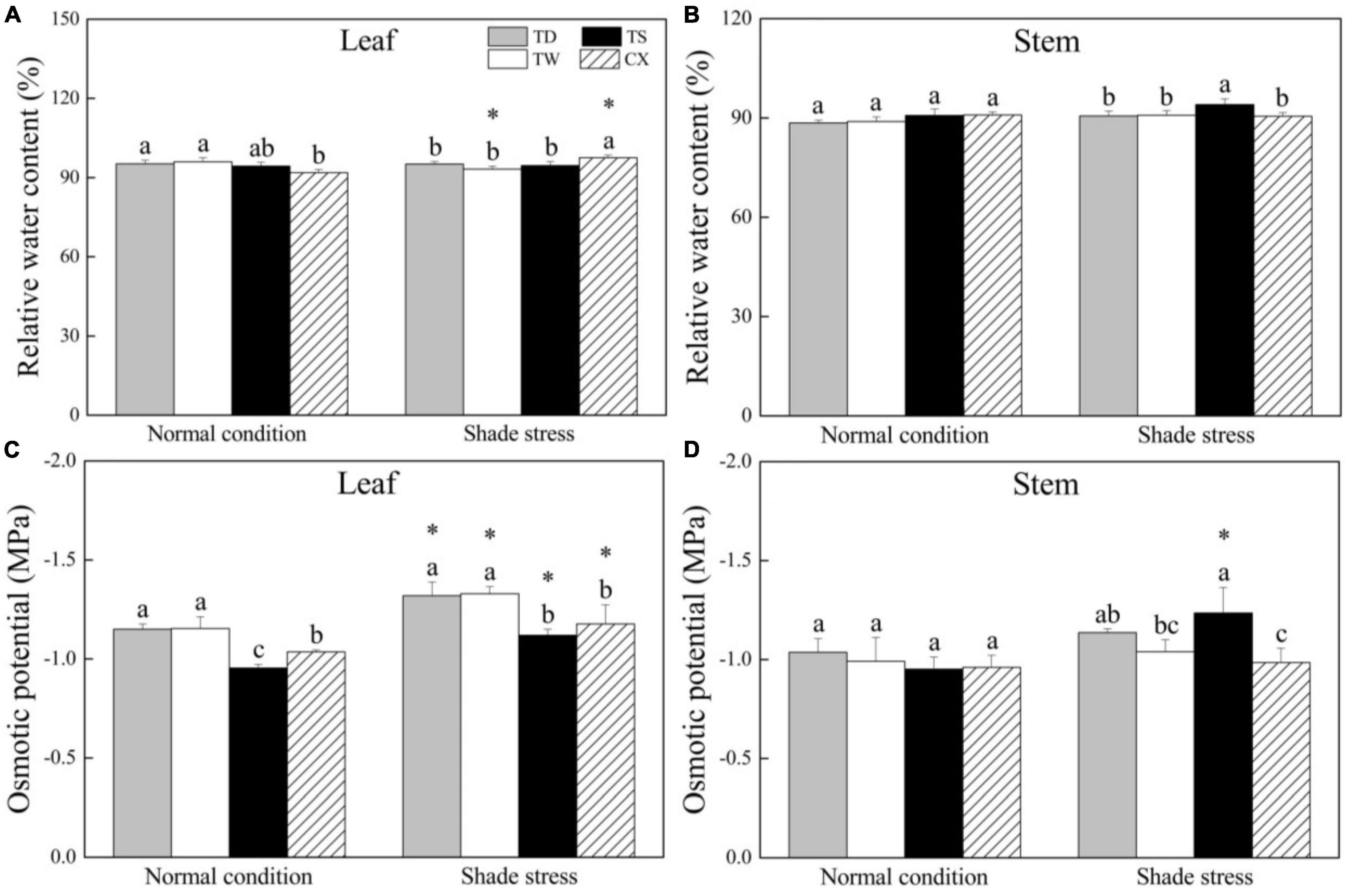
Figure 2. Effects of shade stress on (A) relative water content in leaf, (B) relative water content in stem, (C) osmotic potential in leaf, and (D) osmotic potential in the stem of four bermudagrass cultivars. Vertical bars indicate the ± SE of mean (n = 4). Different letters above columns indicate significant differences (p ≤ 0.05) among the four cultivars under normal conditions or shade stress. The asterisk (*) above letter indicates a significant difference (p ≤ 0.05) in one particular cultivar between normal conditions and shade stress.
Changes in Chlorophyll and Carotenoid Content in Response to Shade
Shade stress significantly decreased total Chl in leaves of TD, TW, and TS, but had no significant effect on total Chl content in the CX. The TS and CX maintained significantly higher total Chl content in leaves as compared to the TD and TW under shade stress (Figure 3A). The TS or CX had higher or the lowest total Chl content in stems than TD and TW under normal and shade conditions, respectively (Figure 3B). Shade stress reduced the Chl a content in leaves of common and hybrid cultivars (Figure 3C). The CX and TS had significantly higher Chl a content in leaves than the TD and TW under shade condition (Figure 3C). The Chl a content in stems of the TS was highest under normal conditions, and the TD, TW, and TS exhibited significantly higher Chl a content in stems than the CX under shade stress (Figure 3D). Shade stress significantly decreased Chl b content in leaves of TD, TW, and TS by 46.06, 64.85, and 43.81%, but did not affect the Chl b content in leaves of CX (Figure 3E). For changes in Chl b content in stems, shade stress decreased its content in TD and TW by 25.35 and 15.66%, but did not significantly affect its content in TS and CX (Figure 3F). Under normal conditions, the CX or TS had the highest or the lowest Chl a/b ratio in leaves, respectively (Figure 3G). Under stress conditions, the highest Chl a/b ratio was found in stems of TW (Figure 3G). There were no significant differences in Chl a/b ratio in stems among four cultivars under shade stress (Figure 3H).
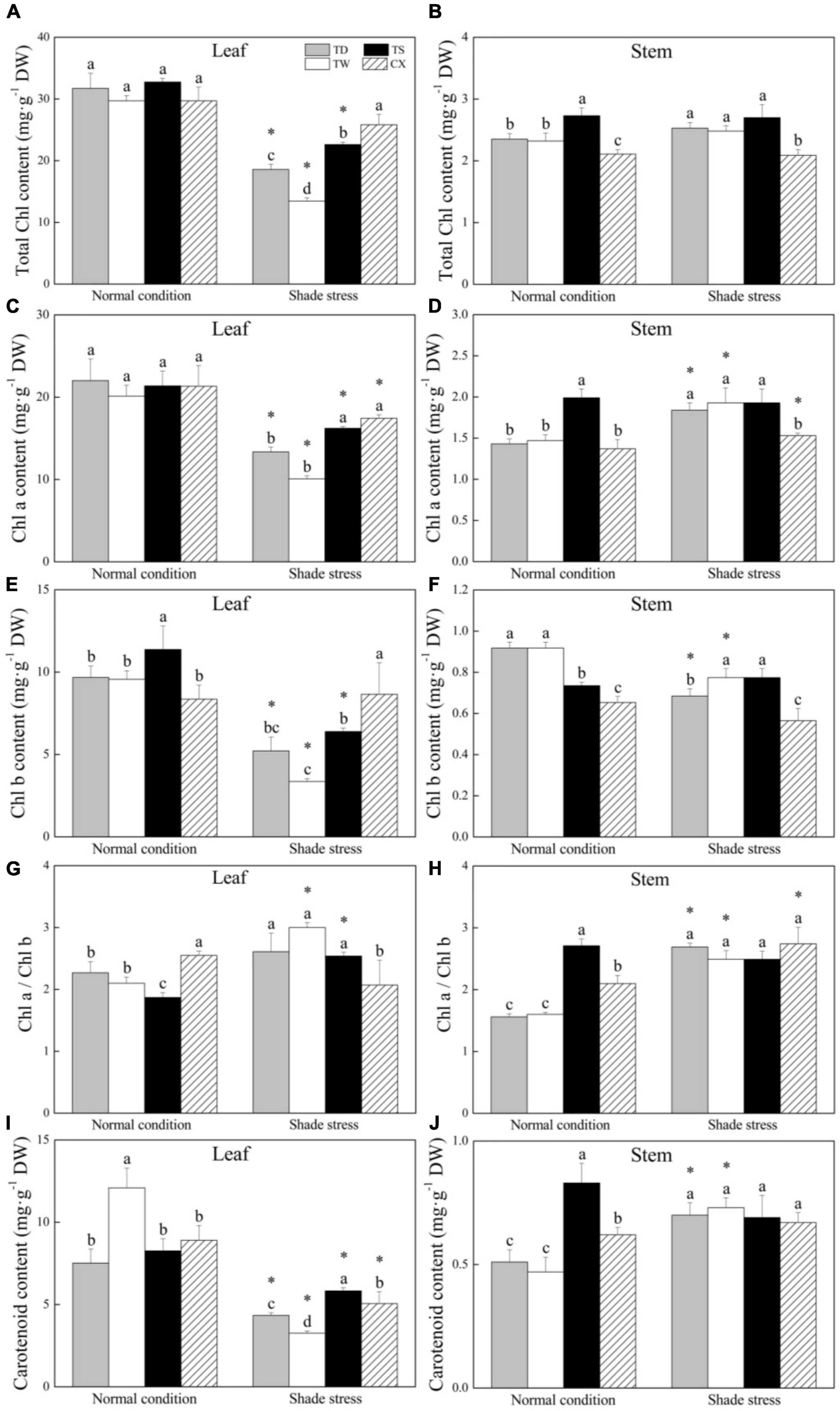
Figure 3. Effects of shade stress on (A) total chlorophyll (Chl) a content in leaf, (B) total Chl a content in stem, (C) Chl a content in leaf, (D) Chl a content in stem, (E) Chl b content in leaf, (F) Chl b in stem, and (G) the ratio of Chl a to Chl b in leaf, (H) the ratio of Chl a to Chl b in stem, (I) carotenoid content in leaf, and (J) carotenoid content in stem of four bermudagrass cultivars. Vertical bars indicate the ± SE of mean (n = 4). Different letters above columns indicate significant differences (p ≤ 0.05) among four cultivars under normal conditions or shade stress. The asterisk (*) above letter indicates a significant difference (p ≤ 0.05) in one particular cultivar between normal conditions and shade stress.
The TW had the highest CR content in leaves than the other three cultivars under normal conditions and the CR content significantly declined in all the cultivars after 30 days of shade stress (Figure 3I). The decrement of CR content in leaves was 72.99% in the TW, 42.27% in the TD, 43.12% in the CX, or 29.33% in the TS, respectively (Figure 3I). In stems, the TS maintained significantly higher CR content than other cultivars under normal conditions, and the shade stress led to a significant increase in CR content in the TD and TW, but did not significantly affect CR content in the TS and CX (Figure 3J).
Changes in Photosynthetic Parameters in Response to Shade
The PIABS in leaves of the TD was significantly different from the other three cultivars under normal conditions. Shade stress induced a significant decrease in PIABS of four cultivars, and the CX maintained the highest PIABS than the other cultivars under shade stress (Figure 4A). There was no significant change in Fv/Fm of TS or CX, but the Fv/Fm of TD and TW significantly declined after 30 days of shade stress (Figure 4B). The Pn in leaves and stems of the TD, TW, and TS significantly decreased by 41.60, 46.68, and 48.69% after 30 days of shade stress, but the Pn in leaves of the CX was not significantly changed by the shade stress. The Pn of CX was the highest in leaves and stems under shade stress (Figures 4C,D). Under normal conditions, there were no significant differences in the Tr and Gs in leaves and stems among common and hybrid cultivars (Figures 4E–H). In the leaves, the CX and TS maintained significantly higher Tr and Gs than other cultivars under shade stress (Figures 4E,G). There was no significant difference in Gs among the four cultivars under shade stress in the stems (Figure 4H). The CX had the minimum WUE in both leaves and stems under normal conditions (Figures 4I,J). The TS had significantly higher WUE in leaves as compared with the other three cultivars under shade stress (Figure 4I). The WUE significantly declined by 39.74% or increased by 88.76% in stems of TS or CX after shade stress, respectively (Figure 4J).
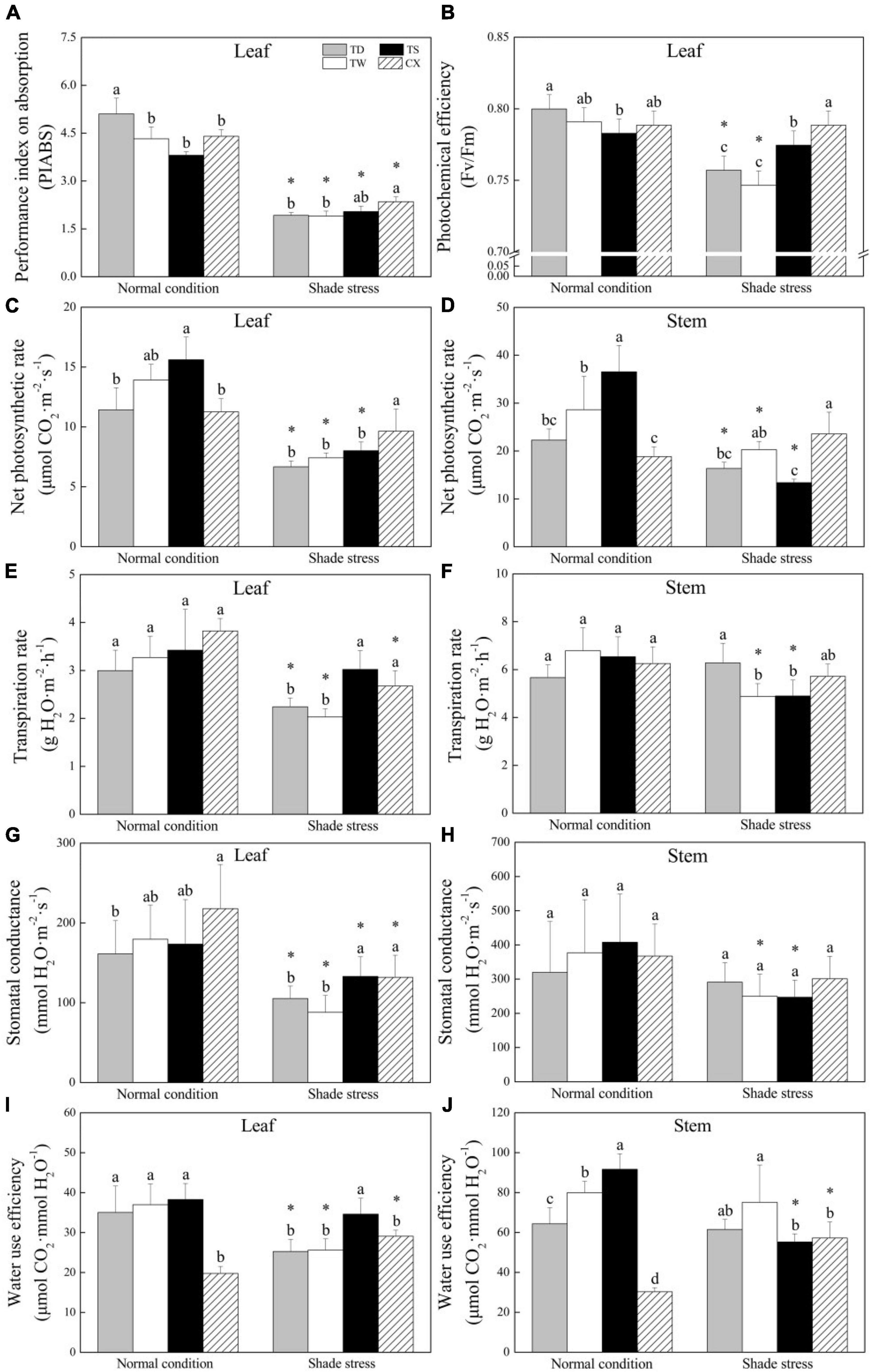
Figure 4. Effects of shade stress on (A) performance index on absorption in leaf, (B) photochemical efficiency of PS II in leaf, (C) net photosynthetic rate in leaf, (D) net photosynthetic rate in stem, (E) transpiration rate in leaf, (F) transpiration rate in stem, (G) stomatal conductance in leaf, (H) stomatal conductance in stem, and (I) water use efficiency in leaf, and (J) water use efficiency in stem of four bermudagrass cultivars. Vertical bars indicate the ± SE of mean (n = 4). Different letters above columns indicate significant differences (p ≤ 0.05) among four cultivars under normal conditions or shade stress. The asterisk (*) above letter indicates a significant difference (p ≤ 0.05) in one particular cultivar between normal conditions and shade stress.
Changes in Organic Metabolites in Response to Shade
In leaves, the WSC content in CX was the highest and in TD was the lowest among the four cultivars under normal conditions (Figure 5A). Shade stress led to a significant decrease in the WSC content of CX or TS by 57.33 or 15.90%, and a significant increase in TD. The TD and TW exhibited significantly higher WSC in leaves than the TS and CX under shade stress (Figure 5A). In stems, the TW or TS had the highest or lowest WSC content than other cultivars under normal conditions (Figure 5B). Shade stress caused a significant decrease in WSC content in the stems of TW or CX by 37.85 or 35.17%, but a significant increase in TD by 23.4% (Figure 5B). In leaves, the FA content of the TS or the CX was significantly higher than that of the TD and the TW under normal conditions. Under shade stress, the FA content of CX and TD maintained a significantly higher level as compared to the TW and TS (Figure 5C). Shade stress significantly increased the FA content of TD, TW, or TS (Figure 5D). As for PC content, shade stress significantly decreased its content in the leaves of all the cultivars, and the decrement was the biggest in leaves of the TW by 71.11% (Figure 5E). The content of PC in TD, TW, and CX decreased significantly because of shade stress, but there were no significant differences in PC content in the stems of all the cultivars under shade stress (Figure 5F).
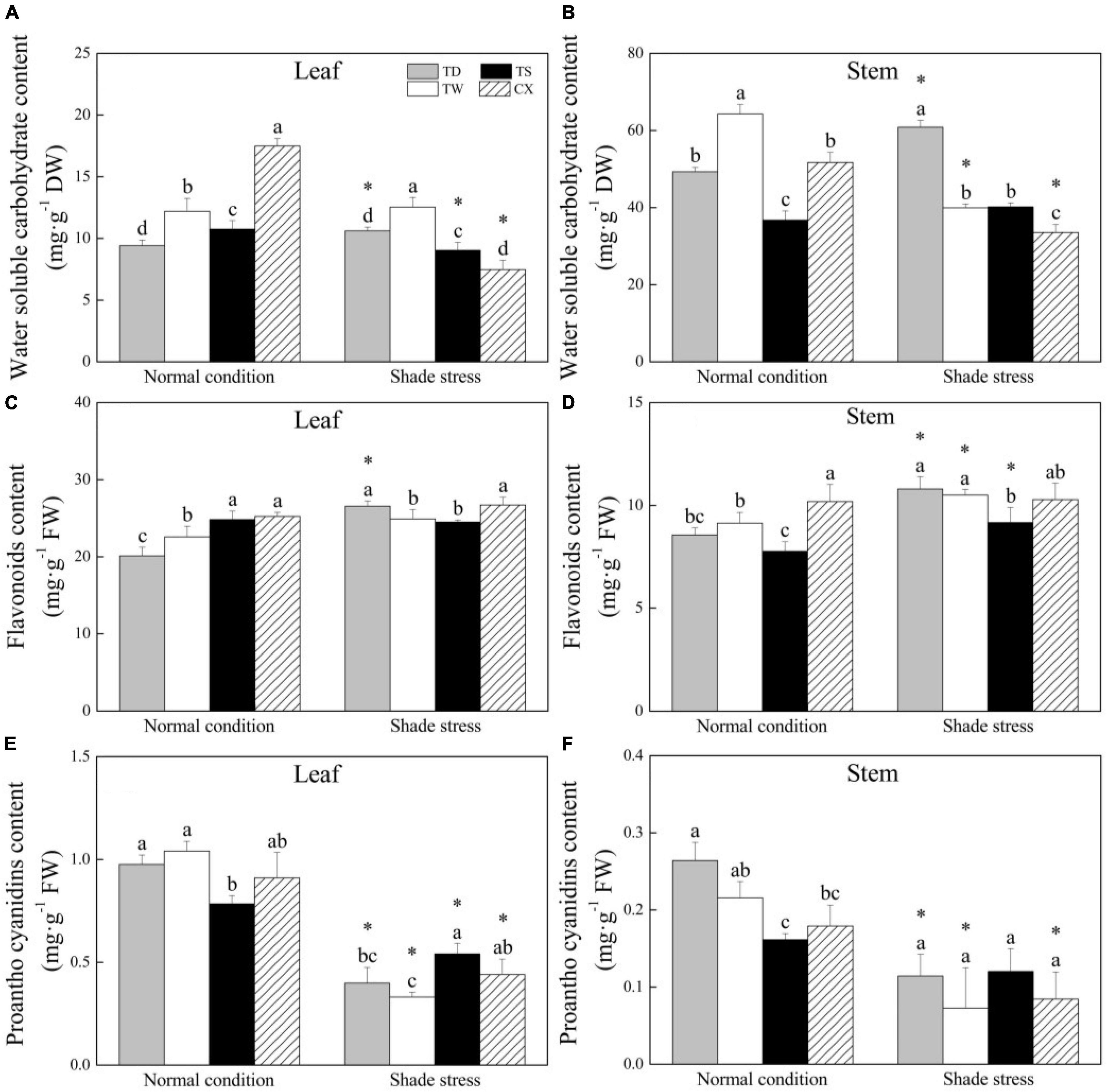
Figure 5. Effects of shade stress on (A) water-soluble carbohydrate content in leaf, (B) water-soluble carbohydrate content in stem, (C) flavonoids content in leaf, (D) flavonoids content in stem, and (E) proantho cyanidims content in leaf, and (F) proantho cyanidims content in stem of four bermudagrass cultivars. Vertical bars indicate the ± SE of mean (n = 4). Different letters above columns indicate significant differences (p ≤ 0.05) among the four cultivars under normal conditions or shade stress. The asterisk (*) above letter indicates a significant difference (p ≤ 0.05) in one particular cultivar between normal conditions and shade stress.
Stress Index, Hierarchical Clustering, and Principal Component Analysis of Four Cultivars in Response to Shade
The SI of LL was highest in the TS as compared to that in other cultivars and no significant difference was detected in the SI of LW or OP among four cultivars in leaves (Figure 6A). The CX exhibited significantly higher SI of RWC in leaves than other cultivars (Figure 6A). The SI of SD was significantly higher in the TD and TW compared with the SI of TS and CX (Figure 6B). The CX exhibited significantly higher the SI of OP in stems than other cultivars (Figure 6B). The SI of Chl, Chl a, and Chl b in leaves of CX was the highest than the other three cultivars, while the SI of Chl a/b was the lowest (Figure 6C). The TS exhibited the highest or the lowest the SI of Chl b or Chl a/b in stems than other cultivars, respectively (Figure 6D). The SI of Pn and WUE was highest in the leaves of CX as compared to that in other cultivars, and the SI of Tr and Gs was the highest in the leaves of TS (Figure 6E). The CX had the highest SI of Pn and WUE in stems than other cultivars (Figure 6F). The SI of WSC was significantly lower in leaves of CX than that in other cultivars, and the FA of TD was the highest in leaves among four cultivars (Figure 6G). The SI of WSC was significantly lower in the stems of TW and CX than that in the stems of TS and TD, and the SI of FA was the lowest in the stem of CX than other cultivars (Figure 6H). The TS showed the highest SI of PC than other cultivars both in the leaves and stems (Figures 6G,H).
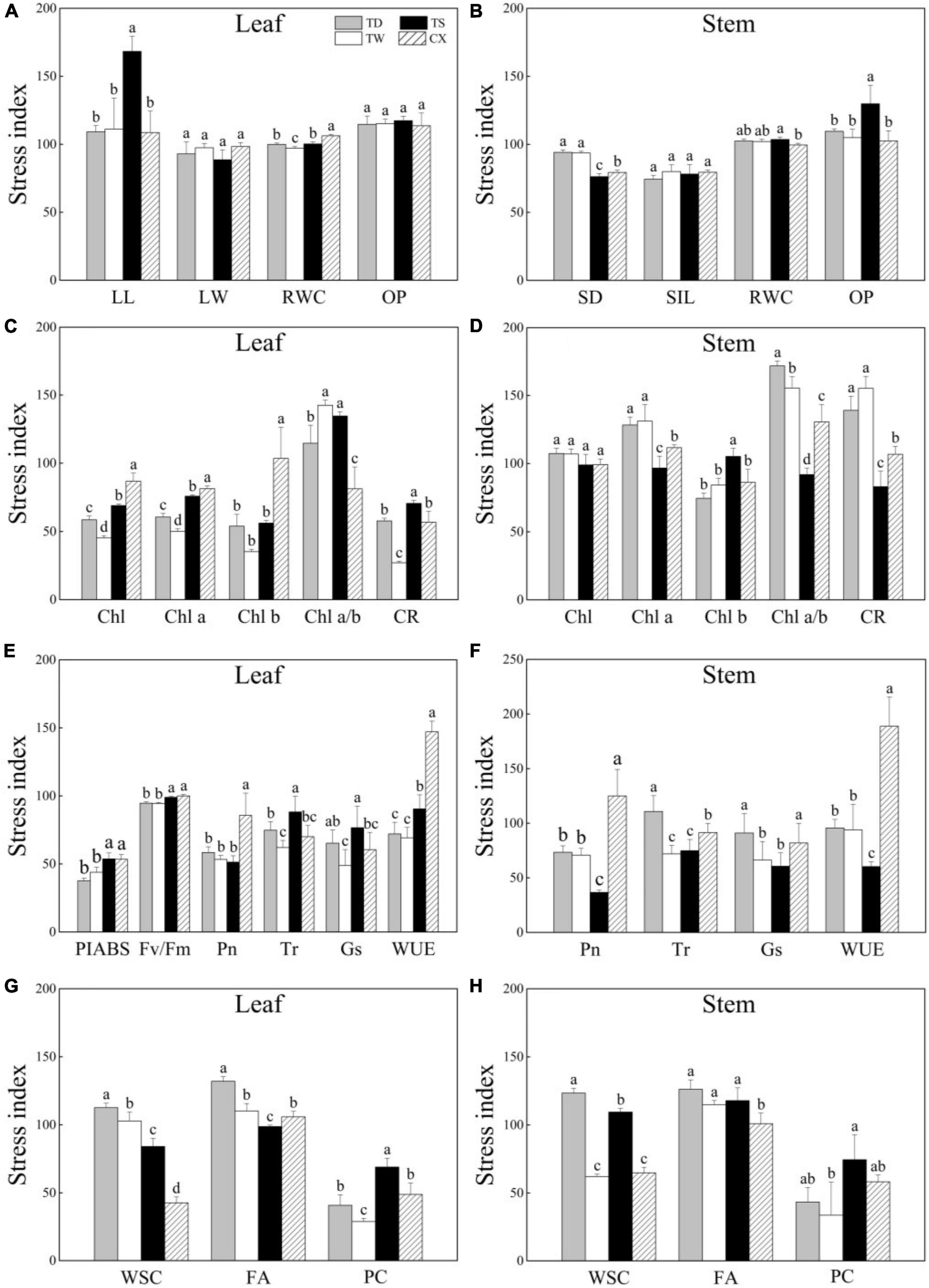
Figure 6. (A–H) Effects of shade stress on stress index (SI) of four bermudagrass cultivars. Different letters above columns indicate significant differences (p ≤ 0.05). Vertical bars indicate the ± SE of mean (n = 4).
Hierarchical clustering analysis of 20 parameters in leaves or 14 parameters in stems is shown in the heat map (Figures 7A,B). In leaves, the TS had a more similar variation profile with the CX than TD and TW (Figure 7A). The TD and TW showed similar changes in leaves and stems in response to shade stress (Figures 7A,B). For the PCA, PC1 or PC2 explained 52.37 or 24.64% of the total variance in the leaves, respectively (Figure 8A). OP, SIL, LW, PC, and Pn showed lower variation in leaves than other parameters under shade stress (Figure 8A). In the stems, the PC1 or PC2 explained 39.47 or 28.13% of the total variance, respectively (Figure 8B). Ch a, Chl b, Chl a/b, PC, OP, CC, WUE, and Pn exhibited a bigger variation in stems than other parameters in response to shade stress (Figure 8B). Figure 9 showed differential responses to shade stress between common and hybrid bermudagrasses (CX vs. TW&TD&TS) or among three different hybrid bermudagrasses (TS vs. TD and TW).
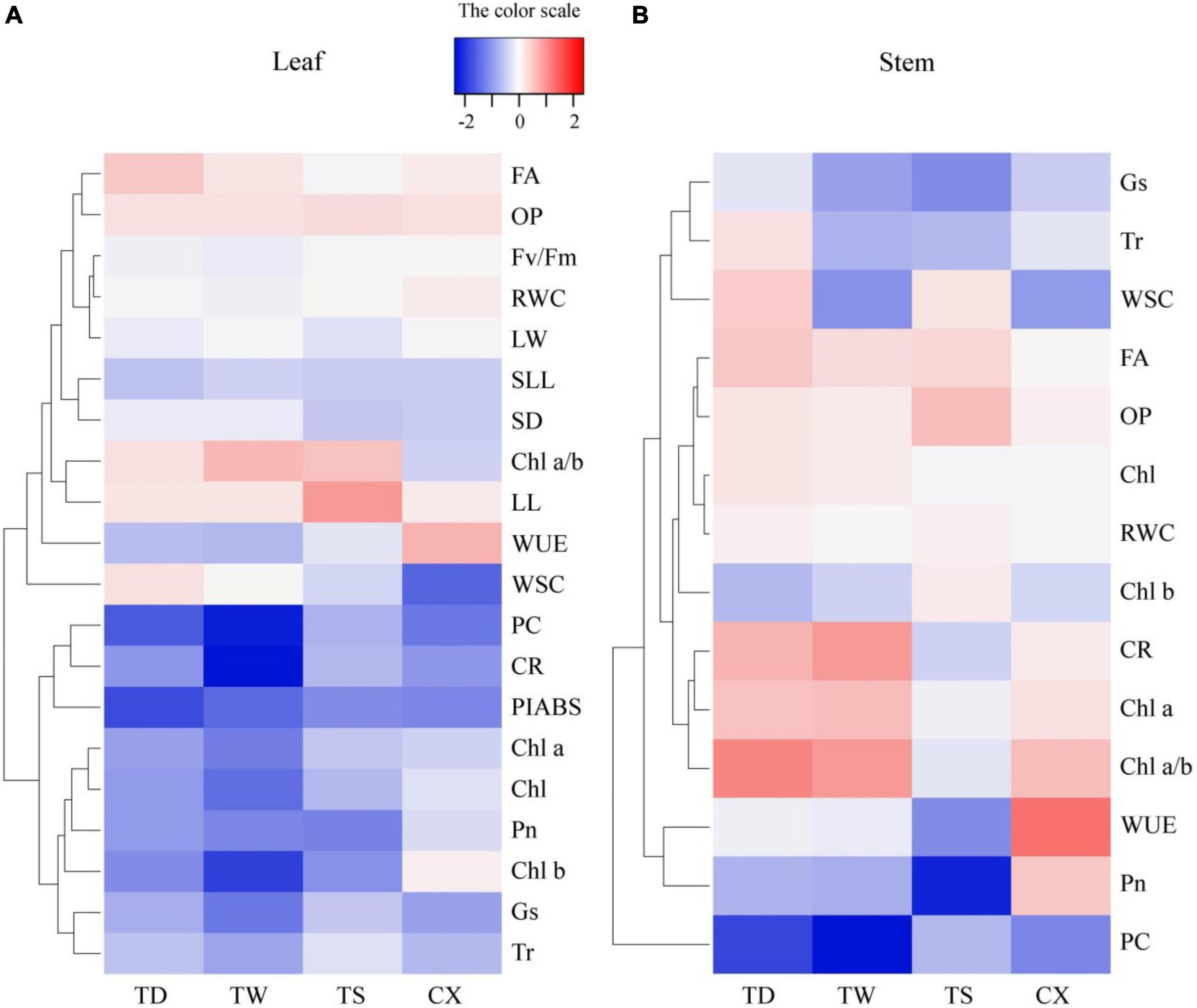
Figure 7. Heatmap and hierarchical clustering for physiological and morphological parameters in (A) leaf and (B) stem of common and hybrid bermudagrasses.
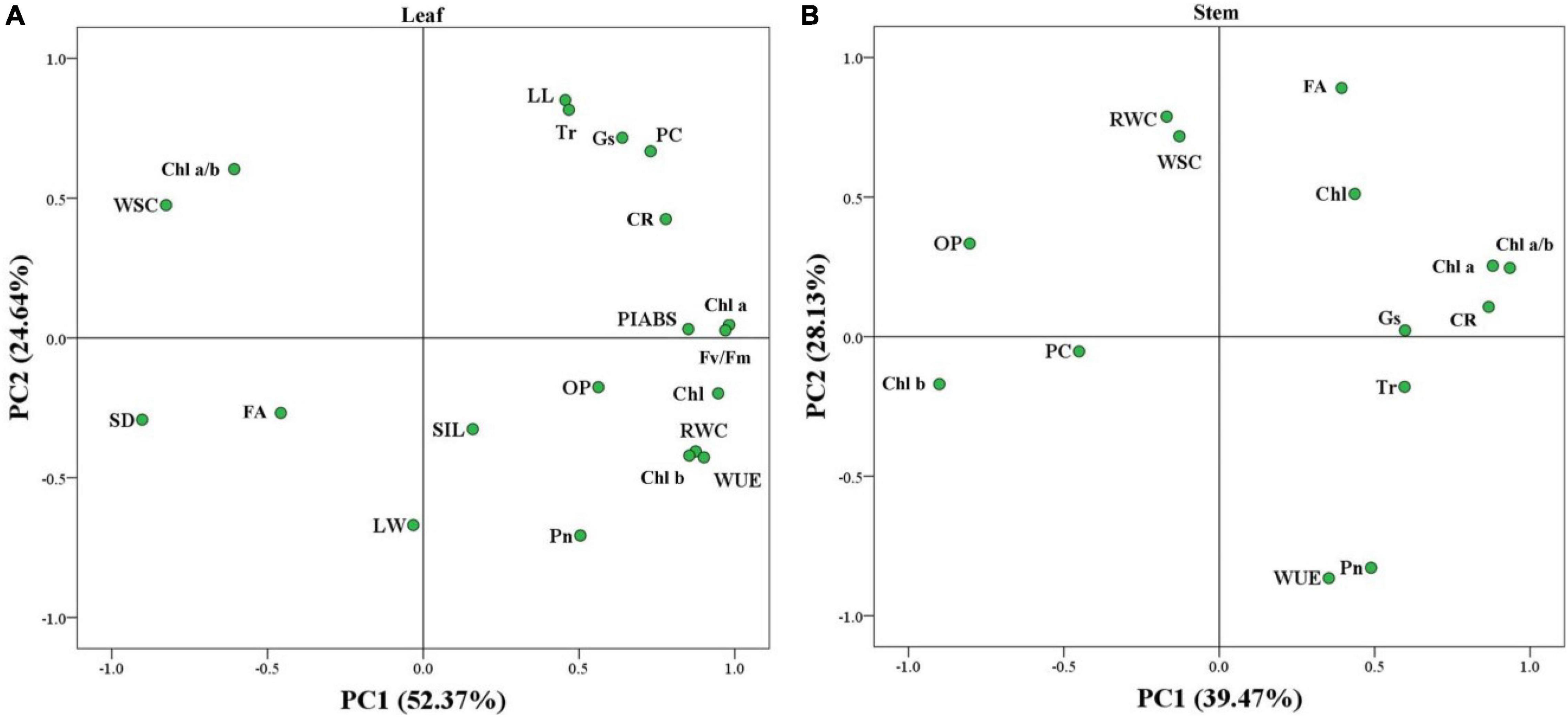
Figure 8. Principal component analysis of the stress index (SI) in (A) leaf and (B) stem of common and hybrid bermudagrasses.
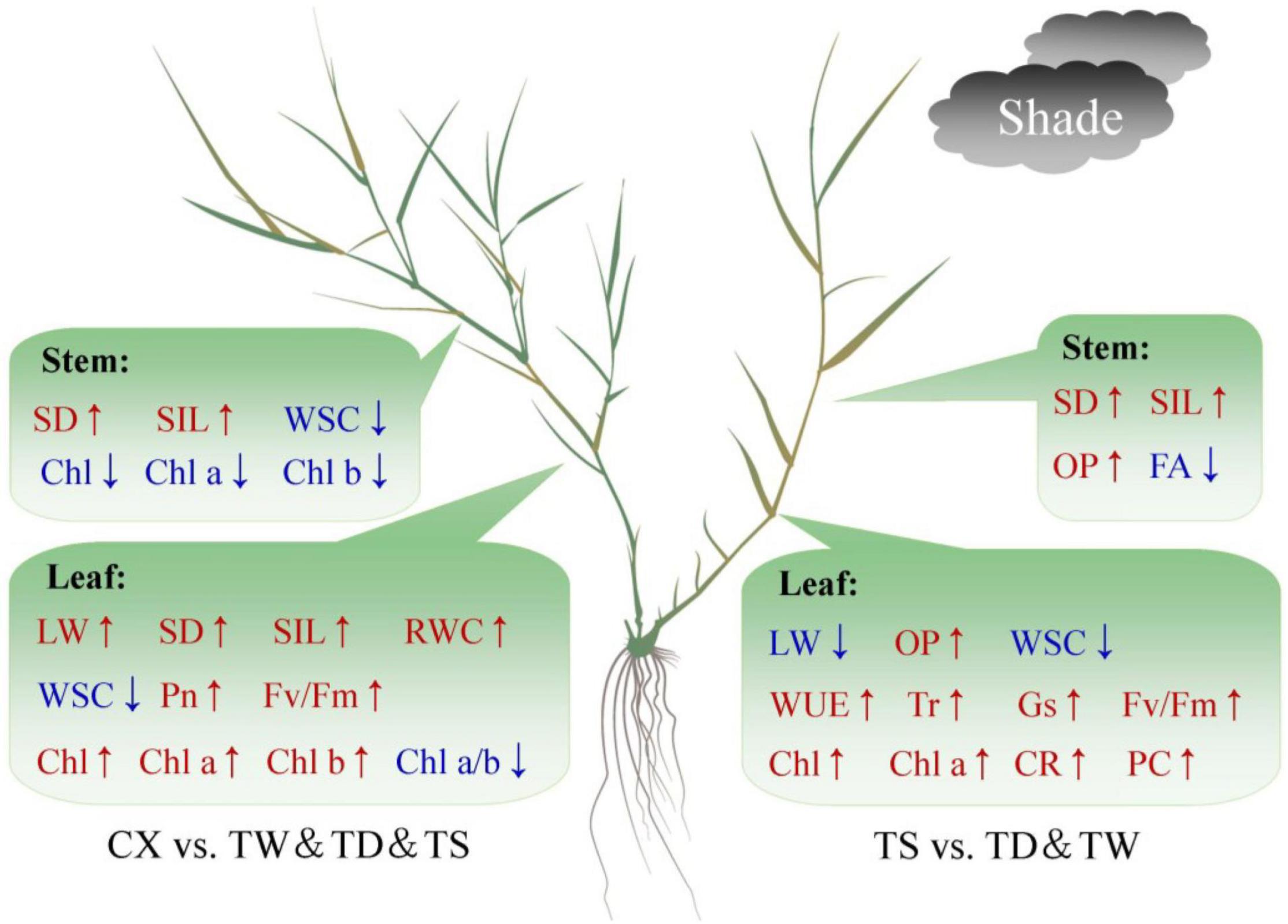
Figure 9. Differential responses to shade stress between common and hybrid bermudagrasses (CX vs. TW&TD&TS) or among three different hybrid bermudagrasses (TS vs. TD&TW).
Discussion
Prolonged low-light conditions affect a range of morphological and physiological changes, such as elongated stems and leaves, thinner leaf thickness, decline in OP, and increased tissue water content (Fankhauser and Batschauer, 2016). Accelerated elongation and upward growth as well as increased leaf area were considered to be important adaptive strategies when plants suffer from shade stress, which is known as shadeavoidance syndrome (SAS). These responses provide more possibilities for plants to intercept more light resources for photosynthesis under shade conditions (Carriedo et al., 2016; Fankhauser and Batschauer, 2016). In the current study, shade improved the LL of four bermudagrass cultivars, especially in the TS which showed elongated leaves in response to shade stress. However, the LW of four bermudagrass cultivars was not affected significantly by the shade. Elongated LL could provide more effective areas for light-harvesting and absorption, which was beneficial to maintain higher photosynthesis in the TS under shade stress. Interestingly, the SD and SIL decreased in four bermudagrass cultivars after a prolonged period of shade stress (30 days). It is well known that shade leads to thin and elongated erect stems and tillers in many plant species (Huylenbroeck and Bockstaele, 2001). An earlier study has shown that warm-season grasses are more morphologically restricted by the shade-stress, leading to an overall decrease in turf quality (Xie et al., 2020). Stoloniferous stems of bermudagrasses exhibit creeping habit that improves their aggressiveness for rapid thatch accumulation (Fry and Huang, 2004). The stoloniferous stems are also the main energy storage organ for plantlets regeneration or energy supply and transfer for stress defense (He et al., 2021). This is why stoloniferous stems of four bermudagrass cultivars demonstrated different responses to shade from erect stems in other plant species. In addition, shade stress resulted in a significant decline in OP in leaves of four cultivars, which could be conducive for the maintenance of water balance and turgid cells under stress conditions. Higher RWC and lower OP in stems of the TS and higher RWC in leaves of the CX could be associated with better unfolding of stems or leaves in response to shade stress.
In addition to morphological changes, physiological adaptation (e.g., increased chlorophyll content, decreased ratio of chla/b, or increased ratio of light response center II to light response center I) is also an important strategy for shade-tolerant plants to cope with shade stress (Melis and Harvey, 1981). A short-term relatively lower light intensity can effectively stimulate Chl formation; however, a prolonged period of shade condition inhibits Chl biosynthesis and also accelerates Chl degradation leading to leaf chlorosis (Pires et al., 2011; Huylenbroeck and Bockstaele, 2001). The maintenance of a lower ratio of Chl a/b has been found to be one of the important indicators of stress adaptability in plants (Zeng et al., 2021). Under shade conditions, the proportion of red light decreases and more blue–violet lights are available to the plants. Therefore, the ability to capture and utilize more diffuse light (blue-violet light) will help plants to achieve a dominant position under shade conditions (Dai et al., 2009). As compared to Chl a, the Chl b is capable of absorbing and using more blue-violet lights. Many shade-tolerant plant species decrease the ratio of Ch a/b in response to shade stress. The study of Terashima et al. (2021) showed that the ratio of Chl a/b in leaves of Alocasia odora gradually decreased with constantly increasing shade. In our present study, the CX and the TS maintained significantly higher total Chl content and Chl a content than the TD and the TW in leaves. Interestingly, the tetraploid CX exhibited a significantly higher Chl b and a lower ratio of Chl a/b in leaves than triploid three cultivars after 30 days of shade stress. This could be one of the critical adaptive mechanisms in tetraploid bermudagrass as compared to triploid cultivars suffering shade conditions. The previous study has also demonstrated that the Chl a/b ratio in leaves of shade-tolerant warm-season seashore paspalum (Paspalum vaginatum) was not affected significantly by low light condition, but it significantly increased in the shade-intolerant TS (Jiang et al., 2005).
Under shade condition, leaf chlorosis and less available long-wavelength lights cause a decline in photosynthesis. Fv/Fm represents the maximum photochemical quantum yield of PSII and is commonly used to illustrate the photochemical efficiency of PSII in plant leaves. PIABS, a key indicator of maximum photochemical efficiency and total numbers of activated photochemical reaction centers of PS II, reflects health status of chloroplasts under stress conditions, such as high-temperature stress, drought, salt stress, and shade stress (Geng et al., 2021; Li et al., 2021; Zeng et al., 2021). Alteration in the PIABS is more sensitive than the Fv/Fm in response to environmental stresses (Yang and Chen, 2015). Although a significant decrease in PIABS was observed in the leaves of all the four bermudagrass cultivars under shade stress, the CX exhibited higher PIABS than the TD, the TW, and the TS. However, shade induced a significant decline in the Fv/Fm in leaves of the TD and the TW, but not in the TS and the CX. A similar result was demonstrated in the study of Jiang et al. (2005) who reported that the Fv/Fm in leaves of TS was not significantly affected after 35 days of low-light stress (60–100 μmol m–2 s–1).
Photosynthetic capacity, as demonstrated by a change in Pn, is closely related to the maintenance of plant growth and stress tolerance since photosynthesis provides energy supply for plants through CO2 assimilation (Pires et al., 2011). In response to environmental stresses, plants adjust stomatal closure and opening to maintain water and gaseous interchange homeostasis (Bharath et al., 2021). Tr often shows a consistent trend with Gs, which is an important indicator of water evaporation and gas exchange (Borel et al., 2010). Our current study found that both Tr and Gs were decreased significantly in four bermudagrass cultivars under shade stress, but the TS and the CX maintained significantly higher Tr and Gs as compared to the TD and the TW, indicating that TS and CX had more frequent gas exchange for photosynthetic maintenance under shade stress. It is noteworthy that a significant decrease in the Pn induced by shade stress was only observed in leaves and stems of triploid hybrid bermudagrass cultivars (TD, TW, and TS). The CX maintained a significantly higher Pn in leaves than TD, TW, and TS under shade stress, which could be related to the maintenance of lower Chl a/b in leaves. In addition, improved WUE in leaves and stems was only observed in the tetraploid CX after 30 days of shade stress. The higher WUE indicates greater energy conversion efficiency associated with dry matter accumulation and water consumption in plants. Zhang et al. (2017) found that the 44% light could improve WUE of walnut (Juglans regia L.). The shade-induced significant decrease in WSC accumulation has been found in the bermudagrass and other warm-season grass species (Jiang et al., 2005). Interestingly, the CX and TS did not accumulate more WSC in leaves and stems than the TD and TW under shade stress. The WSC is the main energy donor for maintaining plant growth and development (Liu et al., 2021). These findings suggested that maintenance of higher photosynthesis and WUE were important physiological adaptions to shade stress in the tetraploid CX. The utilization of WSC for maintenance of normal plant growth could be more important than WSC accumulation when bermudagrass species suffered from a prolonged period of shade stress.
Adverse stresses including shade stress induce a large accumulation of ROS, which oxidizes cell membranes and attacks organelles, leading to lipid peroxidation, protein degradation, and impaired function of cytoplasmic organoids (Gill and Tuteja, 2010). CRs are fat-soluble pigments that, to some extent, determine the coloration of organisms. In higher plants, the main role of CRs in photosynthetic organisms is to transmit light energy and quench excess light energy especially under a high light condition (Zakar et al., 2016). CRs also have strong antioxidants that can efficiently kill singlet oxygen and protect the photosynthetic membrane system against oxidative damage (Dewanjee et al., 2021). PC is a member of a large group of FA compounds in plants. Both PC and FA exhibit strong antioxidant capacity and free radical (superoxide anion and hydroxyl radicals) elimination effects for protecting lipids from ROS attack (Fini et al., 2011; Li et al., 2017). A previous study has demonstrated that FA and PC have the ability to improve antioxidant activity and scavenge superoxide anion radicals and hydroxyl radicals to slow down the oxidative damage caused by shade stress (Winkel-Shirley, 2002; Treutter, 2006). As compared to the TD and the TW, the TS and the CX had significantly higher CR content in leaves, which might provide better antioxidant and photoprotection capacities under shade stress. In addition, the maintenance of significantly higher PC content in leaves of the TS could be propitious to adapt to shade environment. However, the tetraploid CX did not have the advantage of the accumulation of FA and PC than triploid hybrid cultivars in response to shade stress.
Conclusion
In conclusion, common (CX) and hybrid (TW, TS, and CX) bermudagrasses exhibited some common and differential responses to shade stress. In leaves, the TS had a more similar variation profile with CX than TD and TW, whereas TD and TW showed similar changes in leaves and stems under shade stress. Among the three hybrids (TW, TS, and CX) bermudagrasses, an increase in LL and maintenance of higher photosynthetic performance (higher Fv/Fm, total Chl content, and Chl a content), gas exchange (higher Tr and Gs), and WUE in leaves could be better morphological and physiological adaptations of TS to shade than other hybrid bermudagrass (TD and TW). The TS also had significantly higher CR and PC contents than the TD and the TW, which indicated a potentially better antioxidant capacity in leaves of the TS under shade stress (Figure 9). In addition, tetraploid common CX showed excellent shade tolerance than triploid hybrid TD, TW, and TS mainly by maintaining higher effective photosynthetic leaf area (higher LW), photosynthetic performance of PSI and PSII (higher Pn and Fv/Fm), and photosynthetic pigments and ratio (lower Chl a/b ratio, higher total Chl, Chl a, and Chl b content) for efficient use of light energy under shade stress (Figure 9). Furthermore, OP, SIL, LW, PC, and Pn showed lower variation in leaves than other parameters, and Ch a, Chl b, Chl a/b, PC, OP, CC, WUE, and Pn exhibited bigger variation in stems than other parameters under shade stress. Leaves showed more variations than stems among the four bermudagrasses in response to shade stress, which indicated the physiological importance of leaves when warm-season bermudagrasses suffered from the shade.
Data Availability Statement
The raw data supporting the conclusions of this article will be made available by the authors, without undue reservation.
Author Contributions
ZL conceived and designed the research. YC and KY conducted the experiments. ZL and YC evaluated the data and completed the manuscript writing. YP provided different chemical reagents and experimental material. GF, WL, and YP reviewed and edited the manuscript. All authors contributed to the article and approved the submitted version.
Funding
This research was supported by the Sichuan Forage Innovation Team Project of the Industrial System Construction of Modern Agriculture of China (sccxtd-2020-16).
Conflict of Interest
The authors declare that the research was conducted in the absence of any commercial or financial relationships that could be construed as a potential conflict of interest.
Publisher’s Note
All claims expressed in this article are solely those of the authors and do not necessarily represent those of their affiliated organizations, or those of the publisher, the editors and the reviewers. Any product that may be evaluated in this article, or claim that may be made by its manufacturer, is not guaranteed or endorsed by the publisher.
References
Abdolinejad, R., Shekafandeh, A., and Jowkar, A. (2021). In vitro tetraploidy induction creates enhancements in morphological, physiological and phytochemical characteristics in the fig tree (Ficus Carica L.). Plant Physiol. Biochem. 166, 191–202. doi: 10.1016/j.plaphy.2021.05.047
Agati, G., and Tattini, M. (2010). Multiple functional roles of flavonoids in photoprotection. New Phytol. 186, 786–793. doi: 10.1111/j.1469-8137.2010.03269.x
Allan, P., and Carlson, C. (2003). Effects of shade level on kiwifruit leaf efficiency in a marginal area. Acta Hortic. 610, 509–516. doi: 10.17660/actahortic.2003.610.68
Anu, L. (1998). Accumulation of flavonoids and related compounds in birch induced by UV-B irradiance. Tree Physiol. 18, 53–58. doi: 10.1093/treephys/18.1.53
Arnon, D. I. (1949). Copper Enzymes in Isolated Chloroplasts. Polyphenoloxidase in Beta vulgaris. Plant Physiol. 24, 1–15. doi: 10.1104/pp.24.1.1
Barrs, H. D., and Weatherley, P. E. (1962). A re-examination of the relative turgidity technique for estimating water deficit in leaves. Aust. J. Biol. Sci. 15, 413–428. doi: 10.1071/bi9620413
Bell, G. E., Danneberger, T. K., and Mcmahon, M. J. (2000). Spectral irradiance available for turfgrass growth in sun and shade. Crop Sci. 40, 189–195. doi: 10.2135/cropsci2000.401189x
Bharath, P., Gahir, S., and Raghavendra, A. S. (2021). Abscisic acid-induced stomatal closure: an important component of plant defense against abiotic and biotic stress. Front. Plant Sci. 12:615114. doi: 10.3389/fpls.2021.615114
Borel, C., Frey, A., Marion-Poll, A., Tardieu, F., and Simonneau, T. (2010). Does engineering abscisic acid biosynthesis in Nicotiana plumbaginifolia modify stomatal response to drought? Plant Cell Environ. 24, 477–489. doi: 10.1046/j.1365-3040.2001.00698.x
Carriedo, L. G., Maloof, J. N., and Brady, S. M. (2016). Molecular control of crop shade avoidance. Curr. Opin. Plant Biol. 30, 151–158. doi: 10.1016/j.pbi.2016.03.005
Dai, Y., Shen, Z., Liu, Y., Wang, L., Hannaway, D., and Lu, H. (2009). Effects of shade treatments on the photosynthetic capacity, chlorophyll fluorescence, and chlorophyll content of Tetrastigma hemsleyanum Diels et Gilg. Environ. Exp. Bot. 65, 177–182. doi: 10.1016/j.envexpbot.2008.12.008
Debeaujon, I., Nesi, N., Perez, P., Devic, M., Grandjean, O., Caboche, M., et al. (2003). Proanthocyanidin-accumulating cells in Arabidopsis testa: regulation of differentiation and role in seed development. Plant Cell 15, 2514–2531. doi: 10.1105/tpc.014043
Demotes-Mainard, S., and Jeuffroy, M. (2004). Effects of nitrogen and radiation on dry matter and nitrogen accumulation in the spike of winter wheat. Field Crops Res. 87, 221–233. doi: 10.1016/j.fcr.2003.11.014
Dewanjee, S., Bhattacharjee, N., Chakraborty, P., and Bhattacharjee, S. (2021). “Carotenoids as antioxidants,” in Carotenoids: Structure and Function in the Human Body, eds M. Zia-Ul-Haq, S. Dewanjee, and M. Riaz (Cham: Springer).
Esmailpourmoghadam, E., and Salehi, H. (2021). Tall fescue is a superturfgrass: tolerance to shade conditions under deficit irrigation. J. Saudi Soc. Agric. Sci. 20, 290–301. doi: 10.1016/j.jssas.2021.03.001
Estrada-Campuzano, G., Miralles, D. J., and Slafer, G. A. (2008). Yield determination in triticale as affected by radiation in different development phases. Eur. J. Agron. 28, 597–605. doi: 10.1016/j.eja.2008.01.003
Fan, J., Lou, Y., Shi, H., Chen, L., and Cao, L. (2019). Transcriptomic analysis of dark-induced senescence in bermudagrass (Cynodon dactylon). Plants 8:614. doi: 10.3390/plants8120614
Fankhauser, C., and Batschauer, A. (2016). Shadow on the plant: a strategy to exit. Cell 164, 15–17. doi: 10.1016/j.cell.2015.12.043
Fini, A., Brunetti, C., Ferdinando, M. D., Ferrini, F., and Tattini, M. (2011). Stress-induced flavonoid biosynthesis and the antioxidant machinery of plants. Plant Signal. Behav. 6, 709–711. doi: 10.4161/psb.6.5.15069
Fry, J., and Huang, B. (2004). Applied Turfgrass Science and Physiology. Hoboken, NJ: John Wiley & Sons.
Geng, W., Qiu, Y., Peng, Y., Zhang, Y., and Li, Z. (2021). Water and oxidative homeostasis, Na+/K+ transport, and stress-defensive proteins associated with spermine-induced salt tolerance in creeping bentgrass. Environ. Exp. Bot. 192:104659. doi: 10.1016/j.envexpbot.2021.104659
Gill, S. S., and Tuteja, N. (2010). Reactive oxygen species and antioxidant machinery in abiotic stress tolerance in crop plants. Plant Physiol. Biochem. 48, 909–930. doi: 10.1016/j.plaphy.2010.08.016
He, L., Xiao, X., Zhang, X., Jin, Y., Pu, Z., Lei, N., et al. (2021). Clonal fragments of stoloniferous invasive plants benefit more from stolon storage than their congeneric native species. Flora 281:151877. doi: 10.1016/j.flora.2021.151877
Hu, Y., Sun, D., Hu, H., Zuo, X., and Xie, J. (2021). A comparative study on morphological and fruit quality traits of diploid and polyploid carambola (Averrhoa carambola L.) genotypes. Sci. Hortic. 277:109843. doi: 10.1016/j.scienta.2020.109843
Huang, D., Wu, L., Chen, J. R., and Dong, L. (2011). Morphological plasticity, photosynthesis and chlorophyll fluorescence of Athyrium pachyphlebium at different shade levels. Photosynthetica 49, 611–618. doi: 10.1007/s11099-011-0076-1
Huang, S., Jiang, S., Liang, J., Chen, M., and Shi, Y. (2019). Current knowledge of bermudagrass responses to abiotic stresses. Breed. Sci. 69, 215–226. doi: 10.1270/jsbbs.18164
Huylenbroeck, J., and Bockstaele, E. V. (2001). Effects of shading on photosynthetic capacity and growth of turfgrass species. Int. Turfgrass Soc. Res. J. 9, 353–359.
Jiang, Y., Carrow, R. N., and Duncan, R. R. (2005). Physiological acclimation of seashore paspalum and bermudagrass to low light. Sci. Hortic. 105, 101–115. doi: 10.1016/j.scienta.2004.11.004
Kim, S. J., Yu, D. J., Kim, T. C., and Lee, H. J. (2011). Growth and photosynthetic characteristics of blueberry (Vaccinium corymbosum cv. Bluecrop) under various shade levels. Sci. Hortic. 129, 486–492. doi: 10.1016/j.scienta.2011.04.022
Kubásek, J., Urban, O., and Šantrùèek, J. (2013). C4 plants use fluctuating light less efficiently than do C3 plants: a study of growth, photosynthesis and carbon isotope discrimination. Physiol. Plant. 149, 528–539. doi: 10.1111/ppl.12057
Li, H., Liu, L., Lou, Y., Hu, T., and Fu, J. (2011). Genetic diversity of Chinese natural bermudagrass (Cynodon dactylon) germplasm using ISSR markers. Sci. Hortic. 127, 555–561. doi: 10.1016/j.scienta.2010.12.001
Li, Z., Jing, W., Peng, Y., Zhang, X. Q., Ma, X., Huang, L. K., et al. (2015). Spermine alleviates drought stress in white clover with different resistance by influencing carbohydrate metabolism and Dehydrins Synthesis. PLoS One 10:e0120708. doi: 10.1371/journal.pone.0120708
Li, Z., Tang, M., Hassan, M. J., Zhang, Y., Han, L., and Peng, Y. (2021). Adaptability to High Temperature and Stay-Green Genotypes Associated With Variations in Antioxidant, Chlorophyll Metabolism, and γ-Aminobutyric Acid Accumulation in Creeping Bentgrass Species. Front. Plant Sci. 12:750728. doi: 10.3389/fpls.2021.750728
Li, Z., Zhang, Y., Zhang, X., Merewitz, E., Peng, Y., Ma, X., et al. (2017). Metabolic pathways regulated by chitosan contributing to drought resistance in white clover. J. Proteome Res. 16, 3039–3052. doi: 10.1021/acs.jproteome.7b00334
Liang, L. L., Cao, Y. Q., Wang, D., Peng, Y., Zhang, Y., and Li, Z. (2021). Spermine alleviates heat-induced senescence in creeping bentgrass by regulating water and oxidative balance, photosynthesis, and heat shock proteins. Biol. Plant. 65, 184–192. doi: 10.32615/bp.2021.008
Liu, Z., Shi, Y., Xue, Y., Wang, X., Huang, Z., Xue, J., et al. (2021). Non-structural carbohydrates coordinate tree peony flowering both as energy substrates and as sugar signaling triggers, with the bracts playing an essential role. Plant Physiol. Biochem. 159, 80–88. doi: 10.1016/j.plaphy.2020.12.012
Melis, A., and Harvey, G. W. (1981). Regulation of photosystem stoichiometry, chlorophyll a and chlorophyll b content and relation to chloroplast ultrastructure. Biochim. Biophys. Acta 637, 138–145. doi: 10.1016/0005-2728(81)90219-x
Mole, S., Ross, J. A., and Waterman, P. G. (1988). Light-induced variation in phenolic levels in foliage of rain-forest plants: I. Chemical changes. J. Chem. Ecol. 14, 1–21. doi: 10.1007/BF01022527
Pires, M. V., Almeida, A. A. F., Figueiredo, A. L., Gomes, F. P., and Souza, M. M. (2011). Photosynthetic characteristics of ornamental passion flowers grown under different light intensities. Photosynthetica 49, 593–602. doi: 10.1007/s11099-011-0075-2
Ren, Y. C., Liu, J., Mao, L. I., Chen, C., and Sun, X. L. (2017). Effects of Shading Stress on Antioxidant System of Two Buffalograss Varieties. Acta Agrestia Sin. 25, 1345–1351.
Richardson, M. D., Mattina, G., Sarno, M., McCalla, J. H., Karcher, D. E., Thoms, A. W., et al. (2019). Shade effects on overseeded bermudagrass athletic fields: II. Rooting, species composition, and traction. Crop Sci. 59, 2856–2865. doi: 10.2135/cropsci2019.05.0311
Son, M., Hart, S. M., and Schlau-Cohen, G. S. (2021). Investigating carotenoid photophysics in photosynthesis with 2D electronic spectroscopy. Trends Chem. 3, 733–746. doi: 10.1016/j.trechm.2021.05.008
Takahashi, S., and Badger, M. R. (2011). Photoprotection in plants: a new light on photosystem II damage. Trends Plant Sci. 16, 53–60. doi: 10.1016/j.tplants.2010.10.001
Terashima, I., Matsuo, M., Suzuki, Y., Yamori, W., and Kono, M. (2021). Photosystem I in low light-grown leaves of Alocasia odora, a shade-tolerant plant, is resistant to fluctuating light-induced photoinhibition. Photosynth. Res. 149, 69–82. doi: 10.1007/s11120-021-00832-4
Trappe, J. M., Karcher, D. E., Richardson, M. D., and Patton, A. J. (2011). Shade and traffic tolerance varies for bermudagrass and zoysiagrass cultivars. Crop Sci. 51, 870–877. doi: 10.2135/cropsci2010.05.0248
Treutter, D. (2006). Significance of flavonoids in plant resistance: a review. Environ. Chem. Lett. 4:147. doi: 10.1007/s10311-006-0068-8
Winkel-Shirley, B. (2002). Biosynthesis of flavonoids and effects of stress. Curr. Opin. Plant Biol. 5, 218–223. doi: 10.1016/s1369-5266(02)00256-x
Xie, F., Shi, Z., Zhang, G., Zhang, C., Sun, X., Yan, Y., et al. (2020). Quantitative leaf anatomy and photophysiology systems of C3 and C4 turfgrasses in response to shading. Sci. Hortic. 274:109674.
Xie, Y., Liu, Y., Wang, H., Ma, X., Wang, B., Wu, G., et al. (2017). Phytochrome-interacting factors directly suppress MIR156 expression to enhance shade-avoidance syndrome in Arabidopsis. Nat. Commun. 8:348. doi: 10.1038/s41467-017-00404-y
Yang, X. S., and Chen, G. X. (2015). Diurnal changes in gas exchange and chlorophyll fluorescence in ginkgo leaves under field conditions. J. Anim. Plant Sci. 25, 309–313.
Zakar, T., Laczko Dobos, H., Toth, T. N., and Zoltan, G. (2016). Carotenoids Assist in Cyanobacterial Photosystem II Assembly and Function. Front. Plant Sci. 7:295. doi: 10.3389/fpls.2016.00295
Zeng, W. H., Hassan, M., Kang, D. F., Peng, Y., and Li, Z. (2021). Photosynthetic maintenance and heat shock protein accumulation relating to γ-aminobutyric acid (GABA)-regulated heat tolerance in creeping bentgrass (Agrostis stolonifera). S. Afr. J. Bot. 141, 405–413.
Zhang, J., Li, H., Jiang, Y., Li, H., Zhang, Z., Xu, Z., et al. (2020). Natural variation of physiological traits, molecular markers, and chlorophyll catabolic genes associated with heat tolerance in perennial ryegrass accessions. BMC Plant Biol. 20:520. doi: 10.1186/s12870-020-02695-8
Zhang, S., Chongzhi, X. U., Zhang, R., Zhou, W., Gao, S., and Guo, L. (2017). Effect of shading on photosynthetic characteristics of ‘Wen 185’ walnut. China Fruits. 3, 22–27.
Zhou, K., Liu, B., Wang, Y., Zhang, X., and Sun, G. (2019). Evolutionary mechanism of genome duplication enhancing natural autotetraploid sea barley adaptability to drought stress. Environ. Exp. Bot. 159, 44–54.
Keywords: polyploidization, morphology, chlorophyll, photochemical efficiency, flavonoids, proanthocyanidin
Citation: Cao Y, Yang K, Liu W, Feng G, Peng Y and Li Z (2022) Adaptive Responses of Common and Hybrid Bermudagrasses to Shade Stress Associated With Changes in Morphology, Photosynthesis, and Secondary Metabolites. Front. Plant Sci. 13:817105. doi: 10.3389/fpls.2022.817105
Received: 17 November 2021; Accepted: 01 February 2022;
Published: 03 March 2022.
Edited by:
Ajoy Kumar Roy, Indian Council of Agricultural Research (ICAR), IndiaReviewed by:
M. Baig, National Rice Research Institute (ICAR), IndiaMiao Chen, Sher-e-Kashmir University of Agricultural Sciences and Technology of Kashmir, India
Copyright © 2022 Cao, Yang, Liu, Feng, Peng and Li. This is an open-access article distributed under the terms of the Creative Commons Attribution License (CC BY). The use, distribution or reproduction in other forums is permitted, provided the original author(s) and the copyright owner(s) are credited and that the original publication in this journal is cited, in accordance with accepted academic practice. No use, distribution or reproduction is permitted which does not comply with these terms.
*Correspondence: Zhou Li, bGl6aG91MTk4NjgxNEAxNjMuY29t
†These authors have contributed equally to this work