- 1Berries Genetics and Breeding Unit, Research and Innovation Centre, Fondazione Edmund Mach, Trento, Italy
- 2Sensory Quality Unit, Research and Innovation Centre, Fondazione Edmund Mach, Trento, Italy
Ethylene, produced endogenously by plants and their organs, can induce a wide array of physiological responses even at very low concentrations. Nevertheless, the role of ethylene in regulating blueberry (Vaccinium spp.) ripening and storability is still unclear although an increase in ethylene production has been observed in several studies during blueberry ripening. To overcome this issue, we evaluated the endogenous ethylene production of a Vaccinium germplasm selection at different fruit ripening stages and after cold storage, considering also textural modifications. Ethylene and texture were further assessed also on a bi-parental full-sib population of 124 accessions obtained by the crossing between “Draper” and “Biloxi”, two cultivars characterized by a different chilling requirement and storability performances. Our results were compared with an extensive literature research, carried out to collect all accessible information on published works related to Vaccinium ethylene production and sensitivity. Results of this study illustrate a likely role of ethylene in regulating blueberry shelf life. However, a generalisation valid for all Vaccinium species is not attainable because of the high variability in ethylene production between genotypes, which is strictly genotype-specific. These differences in ethylene production are related with blueberry fruit storage performances based on textural alterations. Specifically, blueberry accessions characterized by the highest ethylene production had a more severe texture decay during storage. Our results support the possibility of tailoring ad hoc preharvest and postharvest strategies to extend blueberry shelf life and quality according with the endogenous ethylene production level of each cultivar.
Introduction
Blueberry became, in the last decade, the second most important cultivated soft fruit species with a worldwide production that increased by almost 80% (Romo-Muñoz et al., 2019). The postharvest behavior knowledge specific for different blueberry accessions, including information on prolonged storage, is fundamental to guarantee year-round supply to cover the rising market demand, with shipped fruit that should ensure the best conditions upon delivery.
Despite a longer storability than other soft berries, blueberry’s susceptibility to decay, caused for the most part by Botrytis cinerea infection (Rivera et al., 2013), can enhance fruit perishability and greatly reduces shelf life (Cappellini et al., 1982). Blueberry shelf-life and organoleptic quality can be also negatively affected by fruit weight loss that enhances softening and skin wrinkling (Chiabrando and Giacalone, 2011; Paniagua et al., 2013; Perkins-Veazie, 2016). Indeed, a firm texture is one of the consumers’ most appreciated features, being associated with the general concept of blueberry freshness and quality (Ehlenfeldt and Martin, 2002; Gilbert et al., 2014; Daniels et al., 2018). In addition, blueberry fruit is susceptible to loss of integrity in the internal tissues (Allan-Wojtas et al., 2001), leading to discoloration as a dark blue or cell death as a red-brown. Therefore, appropriate postharvest techniques, properly tailored to extend storage of blueberry accessions, are needed.
Fruit storability and marketability are highly affected by an elevate ripening heterogeneity among fruit at harvest. During development, blueberry fruit mature at different rates, resulting in a non-uniform ripening period extending over two to 3 weeks (Suzuki et al., 1997b). Berries are considered ready to pick when they turn 100% blue, but since they do not ripen uniformly on a cluster (Lobos et al., 2014) growers usually wait several days for blue color to accumulate in the plant in order to optimize harvesting labor management and reduce production costs (Lobos et al., 2018). This heterogeneity in fruit ripening might negatively impact both fruit storability and organoleptic quality (Retamales and Hancock, 2012). A feasible solution to improve both harvest efficiency and fruit storability, can be the application of plant growth regulators. However, this application has to be tailored to the distinct ripening behavior of each accession.
Fruit ripening is for the most part regulated by multiple plant hormones such as ethylene, abscisic acid, auxins, and jasmonates, whose production rate and interactions are regulated by both genetic and environmental factors (McAtee et al., 2013). However, there are only few studies about the physiological and biochemical regulation of Vaccinium spp. fruit ripening in comparison with other climacteric and nonclimacteric model fruit species, like apple or strawberry. Thus, the active role of ethylene in regulating blueberry ripening and development is still doubtful. Although a rise in respiration and ethylene production has been observed in several studies during Vaccinium spp. fruit ripening (Windus et al., 1976; Shimura et al., 1986; Suzuki et al., 1997b; Goto et al., 2013), this was not conclusive in many others (Frenkel, 1972; Abdallah et al., 1989; Özgen et al., 2002). Proofs of the non-climacteric blueberry behavior are supported by the active role of abscisic acid (ABA) on fruit ripening, coloration and anthocyanins accumulation (Buran et al., 2012; Zifkin et al., 2012; Karppinen et al., 2013; Wang et al., 2018b). Similar inconsistencies regard the role of ethylene in regulating blueberry storability. Available results about increasing the fruit exposure to ethylene, for instance with ethephon or ethylene application (Poole et al., 1998; Costa et al., 2018; Wang et al., 2018b; Xu et al., 2020), or reducing the possible effect of ethylene applying ethylene scrubbers (Wang et al., 2018a; Álvarez-Hernández et al., 2019), 1-MCP (DeLong et al., 2003; Brackmann et al., 2010; MacLean et al., 2011; Blaker and Olmstead, 2014; Deng et al., 2014; Tao et al., 2017; Xu and Liu, 2017; Xu et al., 2020; Xue et al., 2020), or ozone (Song et al., 2003), indicated contrasting results and did not elucidate the role of ethylene on blueberry ripening.
The genotypes used in these published studies might considerably differ based on ethylene level production and/or ethylene sensitivity. Thus, the objectives of this work are (i) to elucidate the role of ethylene in blueberry fruit ripening with an extensive literature research, carried out to collect all accessible information on published works related to Vaccinium ethylene production and sensitivity; (ii) to verify the variability in ethylene production among a Vaccinium genotype collection during fruit ripening and storage; (iii) to verify a possible relation between the endogenous ethylene production and fruit textural variations during storage.
Materials and Methods
Literature Research About the Role of Ethylene in Regulating Quality and Storability of Vaccinium Species
An extensive literature search was carried out to collect all accessible information on published works related to Vaccinium ethylene production and sensitivity in Scopus and Web of Science databases. The name of the specific fruit (“Blueberr*” OR “Bilberr*” OR “Cranberr*”) and its botanical genus (“Vaccinium”) along with some keywords (“climacteric” OR “non-climacteric” OR “ethylene” OR “ethephon” OR “1-methylcyclopropene” OR “1-MCP”) were applied. Keywords were used in the fields [Topic] and [Article Title/Abstract/Keywords] for Web of Science and Scopus, respectively. All searches were carried out in April 2021. Only papers with, at least, the abstract in English were considered eligible with no restriction on the date of publication. A first selection of papers was performed according to abstract and title relevance. Full texts were obtained for the selected articles, and they were further assessed for appropriateness according to their relevance in determining a possible role of ethylene on Vaccinium fruit ripening and storability. If possible, recorded values of ethylene production rates were converted into ul kg−1 h−1.
Plant Material and Fruit Sampling
Twenty-four Vaccinium accessions (Supplementary Table 1) were chosen from the collection experimental field of Fondazione Edmund Mach at Pergine (Trento), located in the North of Italy (Trentino Alto Adige region). At the time of the analysis, plants were in the full production phase, between 7 and 10 years old. Bushes were maintained following standard pruning and surface bark mulching renewal. In the plot, each of the accessions was represented at least by five plants.
Fruit were harvested at the commercial harvest maturity stage (Dark blue; DB). Homogeneous fruit of each cultivar, free from external damages or irregularities, were sampled immediately at harvest and divided into two batches of around 40 fruit each. Ethylene analyses were carried out at harvest, after 4 weeks of storage, at 2°C (RH 85%), and after one additional day of storage at 21°C.
Twelve of these 24 blueberry accessions (Supplementary Table 1) were chosen for an additional ethylene assessment during fruit ripening. Approximately twenty fruit for each cultivar., free from external damages or irregularities, were harvested at five ripening stages based on berry color: green (Gr), breaker (Br), red (Rd), blue (Bl), and dark-blue (DB). Ethylene analyses were carried after 3 h from harvest, with fruit preserved at 21°C.
Ethylene was further assessed on a bi-parental full-sib population of 124 accessions obtained by the crossing between “Draper” and “Biloxi”, two cultivars characterized, respectively, by a high and low -chill requirement. In this experimental design, seedlings from the progeny are represented by single plant of 3 years old. Bushes, grown on 35-L fertigated pots, were maintained following standard pruning and without disease control. Fruit were harvested at the commercial harvest maturity stage (Dark blue; DB) when the 75% of fruit, of the individual accession, were ripe. Homogeneous fruit of each accession, free from external damages or irregularities, were sampled immediately after harvest and divided into two batches of about 40 fruit each. Ethylene analyses were carried out for 4 h after harvest and after 4 weeks of storage at 2°C (RH 85%).
Ethylene Analysis by PTR/SRI-ToF-MS
Ethylene measurements were performed with a commercial PTR-ToF-MS 8000 apparatus [Ionicon Analytik GmbH, Innsbruck (Austria)] equipped with switchable reagent ion system (SRI-ToF-MS) that allowed the operation of the instrument in H3O+ and O2+ modes, as described by Cappellin et al. (2014). The drift tube conditions were as follows: 110°C drift tube temperature, 2.25 mbar drift pressure, 550 V drift voltage. This leads to an E/N ratio of about 140 Townsend (Td), with E corresponding to the electric field strength and N to the gas number density (1 Td = 10−17 Vcm2). The sampling time per channel of ToF acquisition was 0.1 ns, amounting to 350,000 channels for a mass spectrum ranging up to m/z = 400. The sample headspace was withdrawn through SRI-ToF-MS inlet with 40 ml min−1 flow for 60 cycles resulting in an analysis time of 60 s/sample.
A custom built calibration system based on flow controllers (MKS, Andover, MA) was used to dilute ethylene standard (1 ppmv) with zero air (generated by a Gas Calibration Unit, Ionicon Analytik GmbH). Measurements were done with the SRI-ToF-MS in O2+ mode.
Ethylene Assessment on Intact Berries
In order to measure the ethylene production of intact fruit during ripening and storage, three berries, after 4 h of equilibrium at 21°C, were put into a closed glass vial of 40 ml volume equipped with PTFE/silicone septa (Agilent, Cernusco sul Naviglio, Italy) and incubated at 21°C for 30 min. Each vial was then connected to the GCU zero air provider and to the SRI-ToF-MS operated in O2+mode. Concentration values of the masses m/z 26.012, m/z 28.031, m/z 29.034, m/z 42.011 were summed and converted into ethylene production rate (ul kg−1 h−1) based on the calibration curve (Supplementary Figure 1). Each measurement was done in four replicates.
High Throughput Assessment of Ethylene on Powdered Frozen Material
Ethylene content was additionally estimated on powdered frozen material by setting the SRI-ToF-MS in H3O+mode (PTR-ToF-MS mode, Cappellin et al., 2014; Busatto et al., 2022). According with the recent publication about the application of a high-throughput methodology to assess blueberry VOC profile based on PTR-ToF-MS (Farneti et al., 2020), we analyzed the possibility to apply that methodology to measure ethylene, together with all other VOCs. Three replicates of 0.5 g of powdered frozen sample, each obtained by three fruit, were inserted into 20 ml glass vials equipped with PTFE/silicone septa (Agilent, Cernusco sul Naviglio, Italy) and mixed with 0.5 ml of deionized water, 200 mg of sodium chloride, 2.5 mg of ascorbic acid, and 2.5 mg of citric acid (Farneti et al., 2020). Sample headspace was withdrawn through SRI-ToF-MS (in H3O+mode) inlet with 40 ml min−1 flow for 60 cycles resulting in an analysis time of 60 s/sample. Pure nitrogen was flushed continuously through the vial to prevent any pressure drop. Each measurement was performed automatically after 20 min of sample incubation at 40°C and 2 min between each measurement was applied in order to prevent any memory effect. All measurement steps were automated by an adapted GC autosampler (MPS Multipurpose Sampler, GERSTEL) coupled to SRI-ToF-MS. Ethylene content was expressed as concentration (ul L−1) of the mass m/z 28.031.
This methodology, based on the analysis of powdered frozen material, was firstly applied on the same fruit that were previously measured non-destructively using the O2+ mode (blueberry cultivars assessed at different ripening stages and after storage; section Literature Research About the Role of Ethylene in Regulating Quality and Storability of Vaccinium Species). Secondly, it was applied on 124 accessions of the bi-parental population. Each accession was measured in triplicate.
Texture Analysis
Texture was assessed immediately after storage on 10 homogenous fruit of each accession of both cultivars selection and bi-parental population by a texture analyzer (Zwick Roell, Ulm, Germany) equipped with a 5 kg loading cell and a cylindrical flat head probe with a diameter of 4 mm entering into the berry flesh from the sagittal side (Giongo et al., 2013, 2022; Farneti et al., 2020). The mechanical profile was defined by two variables: force (N) and distance (strain, %). The force was measured with the following instrumental settings: test speed of 100 mm min−1, post-test speed of 300 mm min−1, auto force trigger of 2 g, and stop plot at target position. Each berry was compressed until reaching the deformation of 90%. Based on the force displacement profile, three parameters were here considered: maximum force, deformation at maximum force and gradient (or Apparent Young’s module). All data were processed by TaxtExpertII software (Zwick Roell, Ulm, Germany).
Data Analysis
The analysis of SRI-ToF–MS spectra proceeded as described in Cappellin et al. (2011, 2012). Peak intensities of each mass spectra were converted into concentrations (ul L−1) according to Lindinger et al. (1998). The data acquisition and extraction carried out on the texture mechanical profiles was operated by the software Exponent v.4 (Stable MicroSystem, Ltd., Godalming, United Kingdom) provided with the Zwick instrument. Data analysis was performed with R.4.0.2 software using internal functions and the external package “ggplot2” for graphic representations, and “splines” for polynomial regression model. For the polynomial regression model between ethylene and texture traits, knots were places at the lower, median quartile, and upper quartile of ethylene content/production. ANOVA levels of significance were set at p < 0.05 (*) and p < 0.01 (**).
Results
Bibliographic Research on Vaccinium Ethylene Production
The bibliographic research, carried out without any limitation about publication date, allowed to select 41 articles concerning the possible role of ethylene in regulating the ripening and senescence of Vaccinium fruit (Table 1). In total, 45 cultivars of different Vaccinium species were considered: V. virgatum (seven cv.), V. corymbosum (31 cv.), V. corymbosum x V. darrowii (three cv.) and V. macrocarpon (four cv.). For one article (Frenkel, 1972), neither the cultivar name nor the species were indicated. Within these studies, the number of trials carried out during pre-harvest and post-harvest phases were almost equivalent (35 and 39, respectively; as a “trial” we mean the combination of each treatment and each cultivars). More in detail, treatments with ethephon or ethylene were used to study the possible physiological and biochemical effects in 18 trials, while 19 trials addressed the effect of ethylene reduction by using 1-MCP post-harvest treatments, ozone application, or ethylene scrubbers (Table 1).
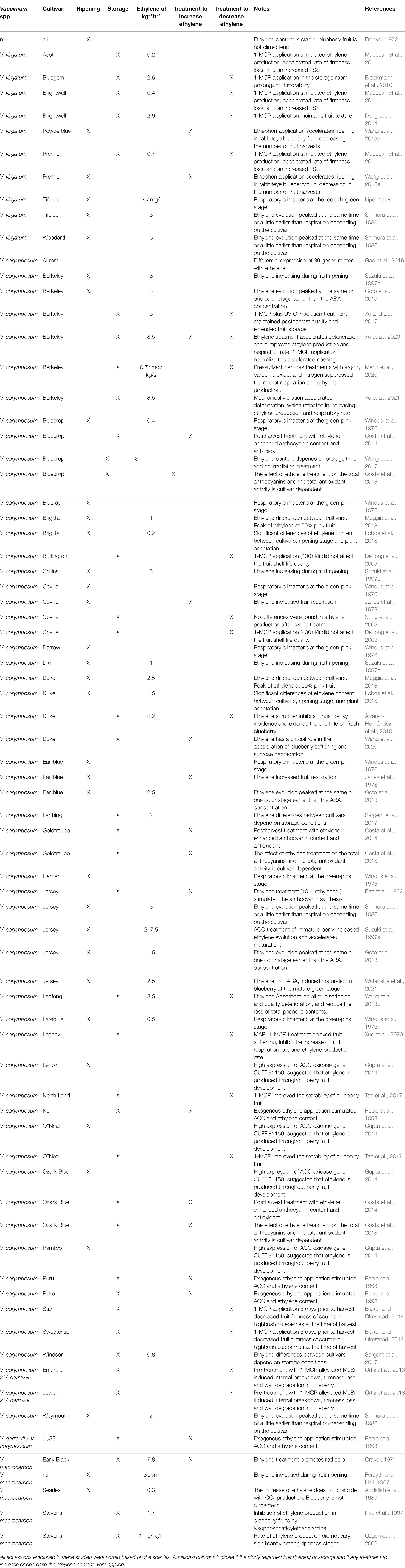
Table 1. Bibliographic research about published studies related to ethylene production and sensitivity of Vaccinium accessions.
In all studies, the most employed cultivars were “Berkeley” (six trials), “Jersey” (five trials), “Bluecrop” (four trials), “Coville” (four trials), “Duke” (four trials), “Ozark Blue” and “Earliblue” (three trials). Overall, trials carried out using the same cultivars (i.e., “Berkeley,” “Duke”, and “Jersey”) showed consistent results regarding both ethylene concentrations and physiological effect on ripening and storability, for instance on color and texture changes. Unfortunately, the exact ethylene production level was reported only on a limited number of articles (25 articles; 38 trials). The comparison between the ethylene production rates, reported in these papers, was not always possible since measurement units were not comparable (Forsyth and Hall, 1967; Lipe, 1978; Özgen et al., 2002; Meng et al., 2020). The high variability in both ethylene production levels and physiological responses of berries to ethylene treatment could be related with the high heterogeneity of species and cultivars employed in all these studies.
These studies indicated that, independently of the species, the Vaccinium accessions characterized by a significant increase in ethylene production during maturation and storage are also more sensitive to treatments applied to reduce and /or increase fruit exposure to ethylene. In particular, texture, color and nutraceutical content are the most influenced fruit quality traits.
Variability in Ethylene Production During Fruit Ripening
Ethylene emission rate of 12 blueberry cultivars (“Atlantic,” “Berkeley,” “Biloxi,” “Bluechip,”“Bluegold,” Brigitta Blue,” “Chandler,” “Emerald,” “Jersey,” “Jubilee,” “Legacy,” and “Misty”; Supplementary Table 1) was evaluated at different fruit ripening stages (GR, green; BR, breaker; RD, red; BL, blue; DB, dark blue). The ethylene produced by each berry was measured using the SRI-ToF-MS applied in O2 + mode. The concentration of masses related with ethylene molecule (m/z 26.012, m/z 28.031, m/z 29.034, m/z 42.011) were quantified through a calibration curve obtained with a gas reference standard (Supplementary Figure 1).
Ethylene production significantly differs (p < 0.01) among cultivars and fruit ripening stages (Figure 1). For most cultivars, maximum ethylene production corresponds with the berry veraison matching with RD and/or BL ripening stages. Next to the accomplishment of ripening (stage DB), the ethylene production tended to decrease and it returned to the values measured at the beginning of fruit veraison (stage BR). The only exception concerned “Jersey” which showed a high production of ethylene (about 4.2 ul kg−1 h−1) already at breaker stage (BR) which remained statistically unchanged until the complete ripening of the berry. “Jersey” and “Atlantic,” being the first parent of the second, were the two cultivars with the maximum ethylene rates recorded during ripening, respectively of 4.2 ul kg−1 h−1 and 5.8 ul kg−1 h−1. For the remaining cultivars, the maximum values were around 1 ul kg−1 h−1. There was no significant effect of type of blueberry cultivars (Northern high bush, NHB; Southern high bush, SHB; High bush, HB) on the production trend of ethylene during berry ripening (Supplementary Figure 2).
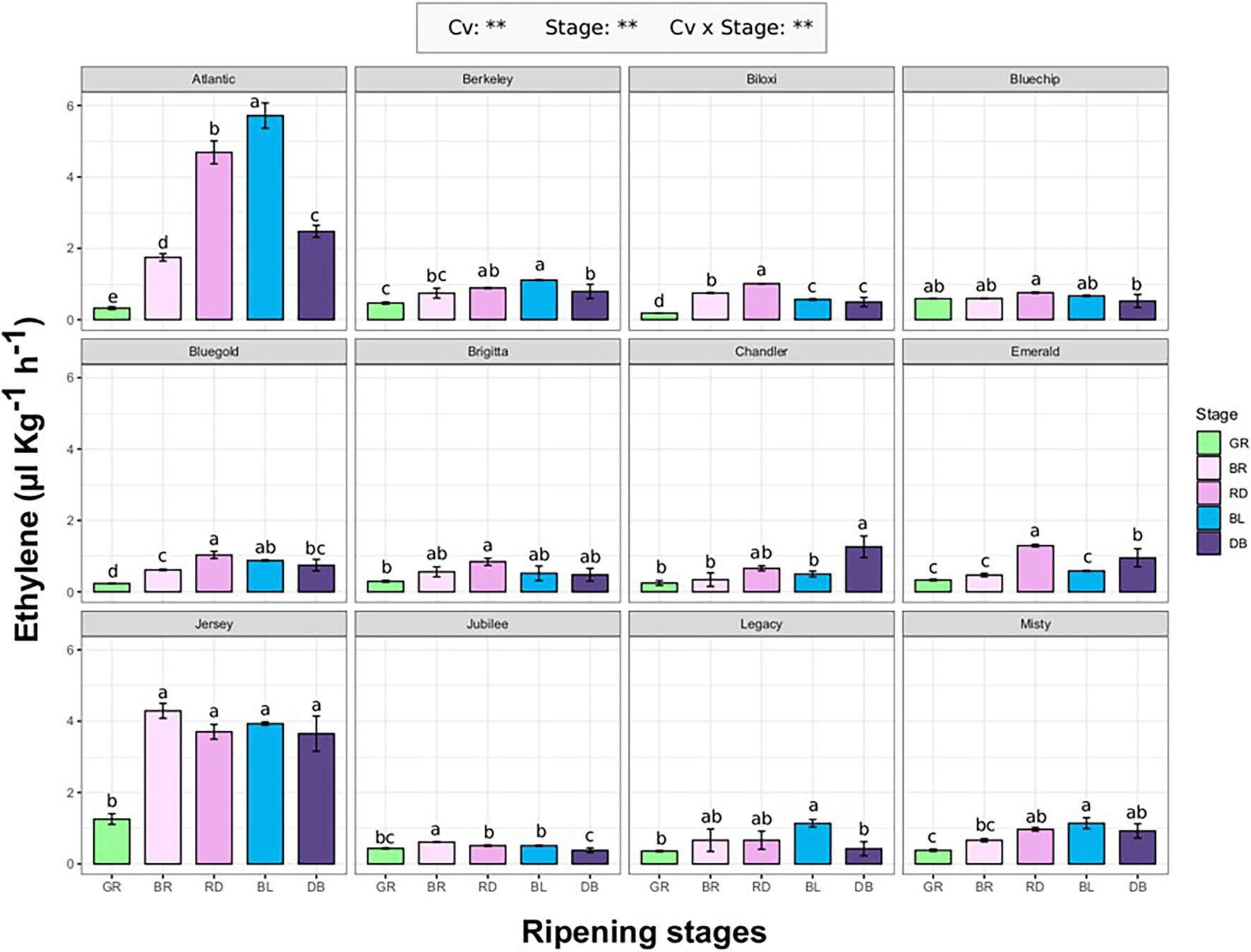
Figure 1. Ethylene production rate of 12 blueberry cultivars assessed at five fruit ripening stages: green (Gr), breaker (Br), red (Rd), blue (Bl), and dark-blue (DB). Each measurement was done in four biological replicates. Ethylene measurement was assessed on intact berries by using an PTR/SRI-ToF-MS set in O2+mode. ANOVA levels of significance (p < 0.05 and p < 0.01) are represented by * and **, respectively. Different letters indicate significant differences between treatments (p ≤ 0.05) according to Tukeyʼs post-hoc test.
Variability in Ethylene Production During Blueberry Storage
Ethylene Production of Commercial Blueberry Cultivars
The ethylene production of 24 blueberry cultivars (Supplementary Table 1) was evaluated at harvest (HR) when berries reached commercial maturity, and after 4 weeks of storage at 2°C, both immediately after storage (SL_0d) and after an additional 1 day of shelf life at 21°C (SL_1d). As previously reported on berries assessed at different ripening stages, ethylene production significantly (p < 0.01) differed among both cultivars and berry storage stages (Figure 2). It is possible to identify two cultivar groups: cultivars with an increased ethylene production during storage (13 cv. out of 24) and cultivars without any significant effect of storage on ethylene production. Among cultivars with a significant ethylene boost during storage, some accessions showed a remarkable increase of ethylene only after 1 day of shelf life such as “Bluechip” (about 12 ul kg−1 h−1), “Jersey” (about 10 ul kg−1 h−1), “Misty” (about 10 ul kg−1 h−1), “Emerald” (about 5 ul kg−1 h−1), “Toro” (about 4ul kg−1 h−1), “Chandler” (about 4ul kg−1 h−1). There was no significant effect of the earliness/lateness of the cultivars (harvest times, Supplementary Figure 3) on the detected ethylene production levels.
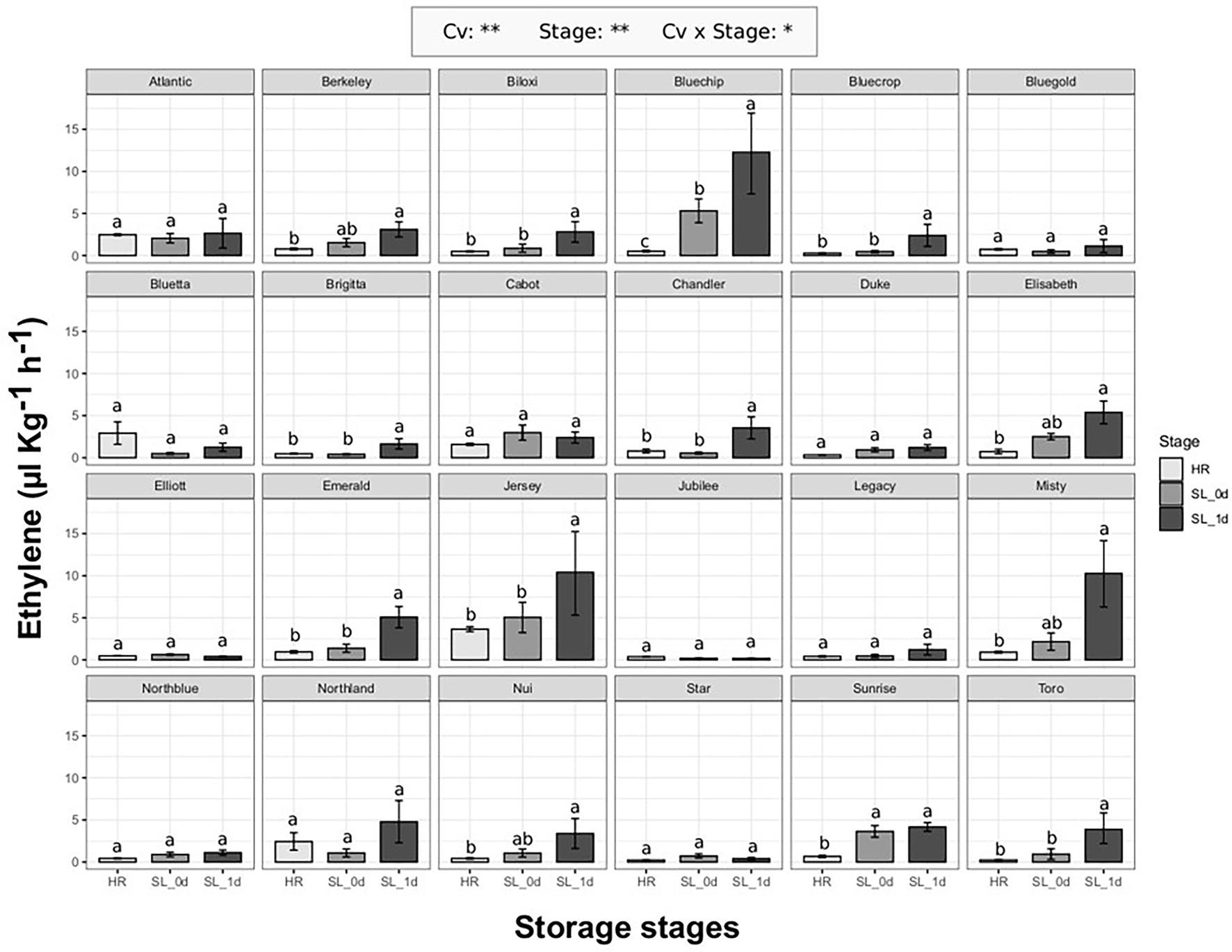
Figure 2. Ethylene production rate of 24 blueberry cultivars assessed at harvest (HR), after 4 weeks of storage at 2°C (SL_0d), and after one additional day of shelf life at 21°C (SL_1d). Each measurement was done in four replicates. Ethylene measurement was assessed on intact berries by using an PTR/SRI-ToF-MS set in O2+mode. ANOVA levels of significance (p < 0.05 and p < 0.01) are represented by * and **, respectively. Different letters indicate significant differences between treatments (p ≤ 0.05) according to Tukeyʼs post-hoc test
The production of ethylene during storage did not depend on ethylene release at harvest. Some cultivars, as “Atlantic,” “Bluetta” or “Cabot,” produced moderate values of ethylene at harvest that remained stable during storage. Other cultivars, like “Jersey,” further increased the ethylene production during storage despite the high levels detected at harvest. On the other hand, there were cultivars, such as “Bluegold,” “Elliott,” “Jubilee” or “Star,” characterized by a stable low ethylene production both at harvest and storage, and cultivars, as “Bluechip,” “Misty,” “Sunrise” or “Toro,” showing a rising ethylene content during storage despite the low levels assessed at harvest.
Results of this trial were further applied to test the use of SRI-ToF-MS (at H3O+ mode) combined with a multipurpose auto-sampler to evaluate the ethylene content on an aliquot of frozen and powdered fruit instead of the whole fruit. This high throughput methodology, previously developed for the analysis of blueberry volatilome (Farneti et al., 2020), aimed on a more qualitative characterization of the ethylene production, rather than a precise and absolute quantification. The ethylene content of the 24 blueberry cultivars, assessed on the headspace of intact berries at harvest and after storage, was correlated with the concentration of mass m/z 28.031 measured, destructively, on the same powdered frozen fruit (Supplementary Figure 4). The high correlation between the ethylene values measured with both methodologies suggested the possibility of using the concentration of the mass peak 28.031 (measured with the SRI-ToF-MS in H3O+ mode) to make a quick and qualitative estimation of ethylene content, even in combination with the entire fruit volatilome (data not shown). With this method, up to 350 samples a day can be automatically measured assessing both ethylene release and VOC fingerprint.
Ethylene Variability Between Accessions of a Biparental Population
The ethylene production of a bi-parental full-sib population obtained from the crossing of “Draper” x “Biloxi” (124 accessions), was evaluated on ripe berries at harvest and after 4 weeks of storage at 2°C, by using the high throughput methodology based on SRI-ToF-MS (at H3O+ mode), that we have previously described. For an additional validation of this high throughput methodology, the ethylene produced by the parental cultivars “Draper” and “Biloxi,” at different stages of ripening and storage, was measured applying both methodologies (Supplementary Figure 5).
Data obtained on this segregating population (Figure 3) were coherent with the data collected on the 24 blueberry cultivars. These results confirmed a high genotype variability in the ethylene production of fruit analyzed both at harvest and after storage. In agreement with the above-mentioned results obtained on blueberry cultivars, the correlation between the ethylene content at harvest and after storage was quite low (r2 = 0.21) due to two distinct behavior patterns among all accessions: (i) accessions with similar ethylene content both at harvest and after storage, like “Draper”; (ii) accessions with an enhanced content of ethylene after storage, like “Biloxi.” especially for accessions characterized by a limited ethylene production at harvest. This correlation improved considering also the accessions characterized by a high production of ethylene at harvest (greater than 1 ul L−1). The ethylene production of the two parental cultivars, “Draper” and “Biloxi,” was similar at harvest. However, they showed two distinct behaviors after storage: the ethylene production was stable in “Draper” while it increased in “Biloxi.” This trend is also confirmed by the non-destructive analysis of the fruit headspace on these two parental cultivars (Supplementary Figure 5).
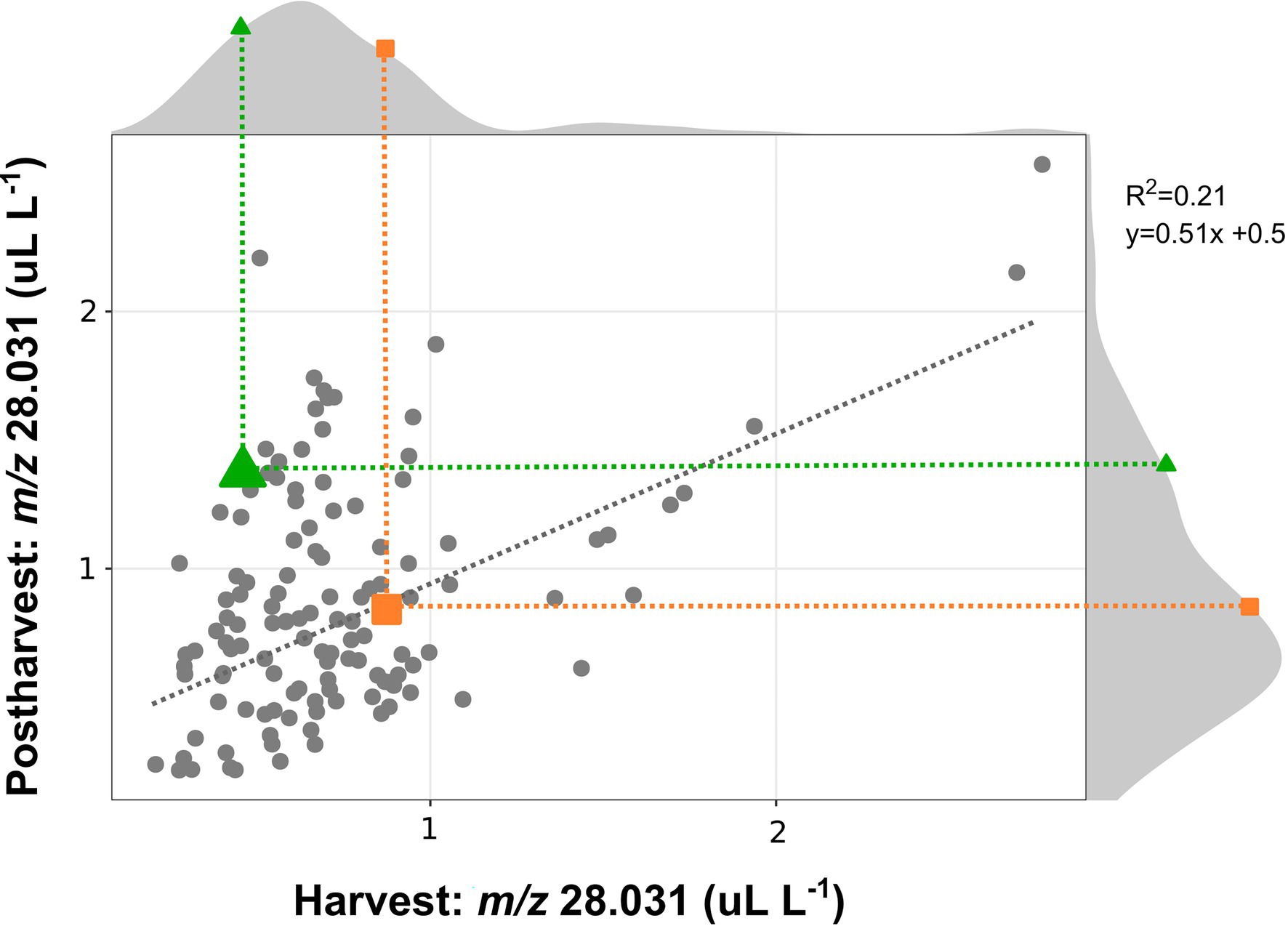
Figure 3. Correlation plot and density plot of the ethylene content determined at harvest and after 4 weeks of storage of 124 accessions of a bi-parental full-sib population obtained by the crossing between the cultivars “Biloxi” (green triangle) and “Draper” (orange square). Each measurement was done in three replicates. Ethylene measurement was assessed on frozen powdered material by using an PTR/SRI-ToF-MS in H3O+mode. Ethylene content was estimated taking into account the concentration of the mass m/z 28.031.
Role of Ethylene in Regulating Berry Softening
To verify the role of ethylene in regulating fruit softening, we evaluated the link between ethylene content and fruit texture, before and after storage. At the time of harvest, no significant correlation was found between texture parameters (maximum force, Young’s modulus and deformation at maximum force) and ethylene content (data not shown). Immediately after storage (SL_0d), no significant linear correlations were highlighted for most accessions; however, six varieties (“Bluechip,” “Jersey,” “Sunrise,” “Cabot,” “Misty,” and “Atlantic”) defined by high ethylene productions (above 2 ul kg−1 h−1) were also characterized by the lowest maximum force values (lower than 3.5 N, Figure 4). No significant correlation was found between ethylene production and Young’s modulus and deformation at maximum force (Supplementary Figure 6).
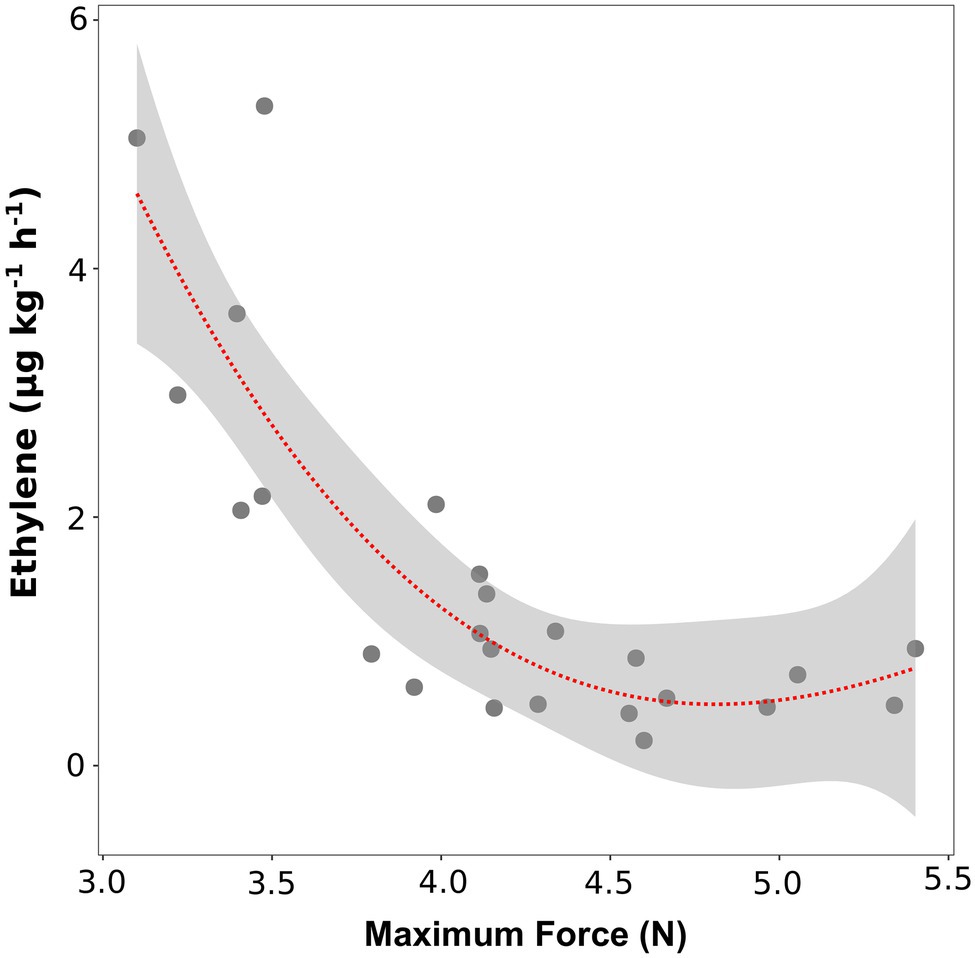
Figure 4. Correlation plot and polynomial regression model between the ethylene production and the textural value of maximal force of 24 blueberry cultivars assessed after 4 weeks of storage. Ethylene measurement was assessed in four replicates on intact berries by using an PTR/SRI-ToF-MS set in O2+mode. Texture analysis was assessed on 10 berries by using a texture analyzer.
These results about texture parameters (maximum force) and ethylene concentrations (m/z 28.031) were coherent with results of the recent article by Farneti et al. (2020), in which the volatilome and texture of 46 Vaccinium cultivars were analyzed both at harvest and after 6 weeks of storage. Results obtained on berries assessed after storage confirm a possible relation between texture (maximum force values) and ethylene content (Figure 5A). However, after storage only six out of 46 cultivars (“Jersey,” “Jewell,” “Emerald,” “Safir,” “Azur,” “Atlantic”) showed, very low values of texture (maximum strength less than 3 N) and, at the same time, high values of ethylene (m/z 28.031 greater than 1.5 ul L−1).
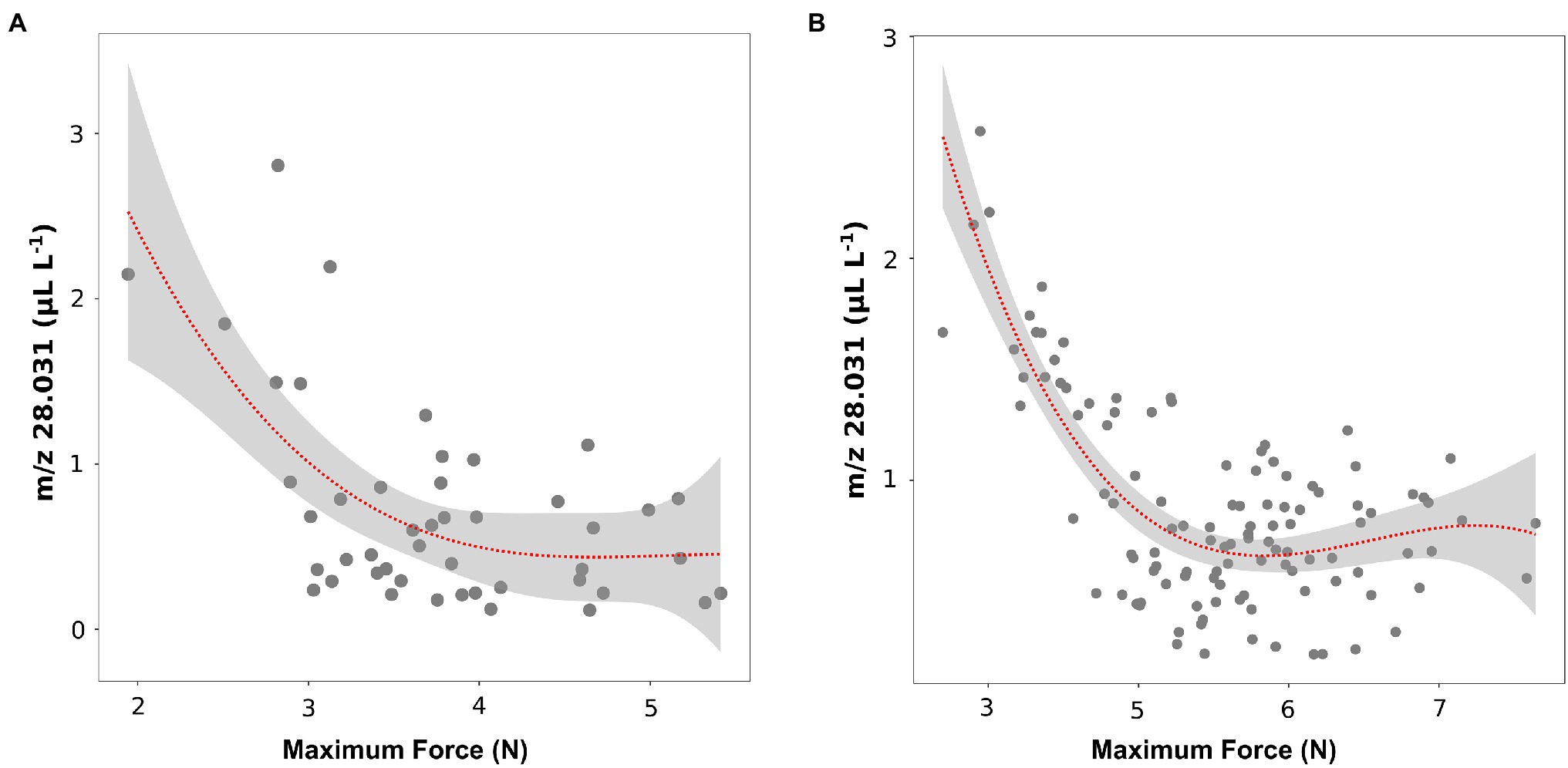
Figure 5. Correlation plot and polynomial regression model between the ethylene content and the textural value of maximal force of 46 blueberry cultivars (A) and 124 accessions of a bi-parental full-sib population (B) both assessed after storage. Ethylene measurement was assessed on frozen powdered material by using an PTR/SRI-ToF-MS set in H3O+mode. Texture analysis was assessed on 10 berries by using a texture analyzer. Results of plot A were extrapolated from the raw data of Farneti et al. (2020).
These outcomes were further confirmed by the analysis of ethylene (m/z 28.031) and texture (maximum force) of the 124 accessions of the bi-parental full-sib segregating population (Figure 5B). Similar to the above-mentioned results, no significant linear correlation between ethylene and texture was observed considering the entire pool of genotypes. However, we identified a group of accessions characterized by a lower texture performance, with maximum strength values below 3.5 N, and, at the same time, a higher production of ethylene (greater than 1 ul L−1 of m/z 28.031).
Discussion
Endogenous ethylene production by plants, can induce relevant physiological responses at concentrations as low as 0.1 μl L−1 (Watada, 1986). In particular, mechanisms of fruit ripening and senescence could involve both ethylene-dependent and ethylene-independent processes, which may differ in extent and/or manner depending on the species (Paul et al., 2012). Monitoring and manipulating fruit ethylene production, along with respiration levels, is therefore of paramount importance in establishing the optimal storage strategy.
Among soft berry species (i.e., strawberry, raspberry or blackberry), for blueberry there are some more scientific evidences on a possible active role of ethylene in both phases of ripening and storage (Table 1). However, the role of ethylene has not been yet fully clarified based on the very contrasting results reported in literature (Table 1), still keeping the dispute open whether the blueberry is climacteric or not. One of the causes of this controversy could lie in the use of different cultivars and species of Vaccinium (V. virgatum, V. corymbosum, V. corymbosum x V. darrowii, and V. macrocarpon; Table 1) in all the studies regarding fruit maturation and storage. Studies, in which the same cultivars were taken into consideration in different years and/or cultivation locations, show indeed comparable results (Table 1).
Results obtained in our study confirmed the high variability in ethylene production and release that can be found within the blueberry germplasm, and how this variability seems more related to genotype rather than species dependent. For instance, no differences in ethylene production were associated with the distinction between Northern highbush and Southern highbush (Figures 1, 2), as it has also been confirmed by the bibliographic research (Table 1). Results obtained by the analysis of a bi-parental full-sib population generated by the cross between “Draper” and “Biloxi” (Figure 3) suggested a direct genetic control of fruit ethylene production both at harvest and after storage.
Genotype related differences in ethylene production were evident during both fruit ripening and storage (Figures 1–3). Accessions characterized by a non-basal production of ethylene (such as “Atlantic,” “Jersey,” “Bluegold,” or “Biloxi”), showed an increase of ethylene production during fruit veraison, which tends to decrease with the progress of maturation, as previously confirmed by several studies (Windus et al., 1976; Shimura et al., 1986; Suzuki et al., 1997b; Goto et al., 2013). However, despite a regular ripening development of the fruit, this effect cannot be found on the accessions characterized by a constant trace production of ethylene (such as “Jubilee,” “Legacy,” “Bluechip,” or “Chandler”). This result suggests a subordinate role of ethylene in regulating blueberry fruit ripening. Another hypothesis, already suggested for non-climacteric fruits, such as grape (Chervin et al., 2004), supports the requirement of traces amounts of ethylene to start the ripening process. Such ethylene concentrations might be below the sensitivity threshold of the analytical technique used so far to quantify ethylene.
In several studies the colour and metabolic changes characterizing blueberry ripening are associated with a physiological role of abscisic acid (Karppinen et al., 2018; Oh et al., 2018) as for many other fruit species classified as non-climacteric (Bai et al., 2021). However, this hypothesis does not exclude a possible interaction between ethylene and abscisic acid, as it has already been found on strawberry fruit (Li et al., 2020; Tosetti et al., 2020). The colour changes of blueberries coincide with several biochemical changes related with the anthocyanin synthesis (Günther et al., 2020), the sugar/acid ratio change (Forney et al., 2012) and also with the variations in VOC profile (Farneti et al., 2017a). Such biochemical changes are associated with the up-regulation of genes involved in enzymatic activity, such as UDP-glucosyltransferases, an alcohol dehydrogenase, and hormone biosynthetic genes (Gupta et al., 2015). These metabolic changes related with fruit ripening are mostly independent from the production of ethylene, since they occur indistinctly from the level of ethylene produced by the fruit. However, this does not exclude a possible secondary role of ethylene in catalyzing these physiological processes. The often-conflicting results obtained after applying ethephon or 1-MCP during blueberry ripening, underline the need to develop chemical/agronomic strategies for a more precise control of fruit ripening, specific for each accession of blueberry.
Results of our study (Figures 2, 3) demonstrated that many blueberry accessions have a second post-harvest ethylene production increase that is probably associated with the senescence of the fruit or with an effect of exposure to cold temperature. For several accessions, the ethylene concentration, both at the time of release from the cold room and after 24 h of shelf life at room temperature, has fairly high values comparable to other fruit species commonly considered as climacteric, such as banana (around 1.5–2 ul kg−1 h−1; de Martino et al., 2007), tomato (around 5–8 ul kg−1 h−1; Yokotani et al., 2009) and kiwifruit (around 8–10 ul kg−1 h−1; Antunes and Sfakiotakis, 2002; Minas et al., 2018).
The magnitude of this second post-harvest ethylene increase seems to be inversely related to the blueberry texture, especially to the maximum force value (Figures 4, 5). Based on results obtained both on the varietal collection and on the segregating population (“Draper” x “Biloxi”), this correlation is evident only for the accessions characterised by a high ethylene production rate (higher than 2 ul kg−1 h−1). On the contrary, ethylene production was not significantly correlated with the values of Young’s modulus and deformability (Supplementary Figure 6). While blueberry fruit elasticity is a consequence of fruit turgor and cell wall strength (Fava et al., 2006; Rivera et al., 2021), fruit softening is a result of hemicellulosic depolymerization and pectic polysaccharides change (Vicente et al., 2007). Mechanical changes associated with berry softening observed during blueberry ripening and storage differ among species and cultivars and they are likely determined by modification in the content of different cell wall polymers (Vicente et al., 2007; Angeletti et al., 2010; Chen et al., 2015; Liu et al., 2019).
Ethylene regulates fruit softening of several climacteric plant species (Ireland et al., 2014). However, the role of ethylene in blueberry softening is still not clear as it has been confirmed by contrasting results of several studies (Table 1). Few articles (DeLong et al., 2003; Blaker and Olmstead 2014; Costa et al., 2014) do not show any significant effect of ethylene on fruit texture during ripening and storage, while others (Ortiz et al., 2018; Wang et al., 2020) suggest an active role of ethylene in regulating fruit softening rate. Postharvest technologies aimed at reducing environmental ethylene (i.e., KmNO4 scrubber) and its perception (i.e., 1-MCP) resulted in textural improvements (Chiabrando and Giacalone, 2011; Ortiz et al., 2018; Wang et al., 2018a; Xue et al., 2020; Xu et al., 2020). In particular, 1-MCP treatment delayed the hemicelluloses solubilization in blueberry fruit (cv. “Brightwell”) over the whole storage, and it decreased the Endo-beta-glucanase (EGase) activity (Deng et al., 2014). On the opposite, exogenous ethylene treatments can significantly promote the softening of blueberry fruit (cv. “Duke”) by promoting cell wall degradation via stimulation of PE, PG, and β-gal enzyme activities and VcPE and VcPG expression (Wang et al., 2020). However, blueberry fruit softening might be also regulated by other hormones, especially ABA. Indeed, post-harvest application of ABA enhanced the expression of many genes involved in cell wall disassembly in bilberry (Vaccinium myrtillus) fruit (Karppinen et al., 2018).
In addition to the possible regulation of blueberry texture, ethylene could also have an indirect role in controlling the fruit shelf life by inducing the development of fungal pathogens (i.e., B. cinerea). The active role of ethylene on regulating the growth of certain fungal and bacterial pathogens is well known (Cristescu et al., 2002; Zhu et al., 2012). Fungal (i.e., B. cinerea) germination and hyphal growth might be also promoted in blueberries due to ethylene presence (Chiabrando and Giacalone, 2011). Fungal (B. cinerea) decay incidence, on fresh blueberry stored under modified atmosphere packaging (MAP) conditions was significantly inhibited by using ethylene scrubbers consisting of a protonated montmorillonite loaded with KMnO4 (Álvarez-Hernández et al., 2019). However, it is not possible from these studies to establish whether any enhanced mold growth induced by ethylene is a secondary effect arising from increased cell permeability that facilitates germination and growth of fungal spores, or any inhibition of mold growth is due to a defence response by tissues to ethylene. Since botrytis is one of the main post-harvest pathogens of blueberry, results of our study recommend a greater attention in storage especially for blueberry accessions characterized by a more abundant production of ethylene. The application of ethylene scrubbers, especially in MAP, or treatments to reduce the concentration of ethylene (i.e., ozone or 1-MCP application) could have the synergic positive effect of slowing the decay of the berry texture and reducing the possible attack of fungal infections and will be further investigated.
This study illustrates a likely role of ethylene in regulating blueberry shelf life. However, a solid generalisation valid for all Vaccinium species is not attainable because of the high variability in ethylene production between genotypes, which is strictly genotype-specific. Up to these results, this high variability is the main cause of the discordant literature data on the role of ethylene in regulating blueberry ripening and storability. This does not exclude a possible role of other hormones, especially ABA, in fruit ripening and senescence. In addition, texture data, both on varietal collection and segregated population, highlight a possible active role of ethylene which, however, does not fully explain the high variability in texture between genotypes. The extreme variability in ethylene production between genotypes is common for many species considered strictly climacteric, such as apple (Farneti et al., 2017b), tomato (Zhao et al., 2021), melon (Zheng and Wolff, 2000; Pereira et al., 2020), or peach (Guo et al., 2018). This must be a warning about the excessive approximation in classifying the various fruit and vegetable species in climacteric and non-climacteric based exclusively on the ethylene and respiration average values extrapolated from few reference cultivars. To optimize pre- and post-harvest cultivation practices, suitable for a detailed and precise control of fruit ripening, it is recommended to obtain all possible information on ethylene production values of each accession of commercial interest. This can help to develop specific cultivation and storage strategies for each genotype in order to maximize the sustainability of the entire production chain focusing on a lower energy input, higher organoleptic quality of the product and a reduced product loss during storage. To this end, future breeding programs focused on prolonged fruit post-harvest storage need to also consider ethylene production. This can be achieved only with a more informed and precise identification of the best performing cultivars to be used as superior parental lines in combination with reliable molecular markers and high throughput phenotyping techniques, such as the SRI-ToF-MS methodologies presented in this study.
Data Availability Statement
The raw data supporting the conclusions of this article will be made available by the authors, without undue reservation.
Author Contributions
BF: conceptualization, investigation, visualization, data analysis, and writing–original draft. IK: investigation, data analysis, and writing–review and editing. MA: texture analysis. FE: investigation and sample preparation. FB: supervision and writing–review and editing. LG: supervision, funding acquisition, and writing–review and editing. All authors contributed to the article and approved the submitted version.
Funding
This work was financially supported by the AdP of the PAT (Provincia Autonoma di Trento) and partially by the project AppleBerry (L6/99 of the PAT).
Conflict of Interest
The authors declare that the research was conducted in the absence of any commercial or financial relationships that could be construed as a potential conflict of interest.
Publisher’s Note
All claims expressed in this article are solely those of the authors and do not necessarily represent those of their affiliated organizations, or those of the publisher, the editors and the reviewers. Any product that may be evaluated in this article, or claim that may be made by its manufacturer, is not guaranteed or endorsed by the publisher.
Supplementary Material
The Supplementary Material for this article can be found online at: https://www.frontiersin.org/articles/10.3389/fpls.2022.813863/full#supplementary-material
References
Abdallah, A. Y., and Palta, J. P. (1989). Changes in biophysical and biochemical properties of cranberry (Vaccinium macrocarpon ait.) fruit during growth and development. Hortic. 241, 360–365. doi: 10.17660/actahortic.1989.241.62
Allan-Wojtas, P. M., Forney, C. F., Carbyn, S. E., and Nicholas, K. U. K. G. (2001). Microstructural indicators of quality-related characteristics of blueberries—An integrated approach. LWT 34, 23–32. doi: 10.1006/fstl.2000.0738
Álvarez-Hernández, M. H., Martínez-Hernández, G. B., Avalos-Belmontes, F., Rodríguez-Hernández, A. M., Castillo-Campohermoso, M. A., and Artés-Hernández, F. (2019). An innovative ethylene scrubber made of potassium permanganate loaded on a protonated Montmorillonite: a case study on blueberries. Food Bioprocess Technol. 12, 524–538. doi: 10.1007/s11947-018-2224-0
Angeletti, P., Castagnasso, H., Miceli, E., Terminiello, L., Concellon, A., Chaves, A., et al. (2010). Effect of preharvest calcium applications on postharvest quality, softening and cell wall degradation of two blueberry (Vaccinium corymbosum) varieties. Postharvest Biol. Technol. 58, 98–103. doi: 10.1016/j.postharvbio.2010.05.015
Antunes, M. D. C., and Sfakiotakis, E. M. (2002). Ethylene biosynthesis and ripening behavior of “Hayward” kiwifruit subjected to some controlled atmospheres. Postharvest Biol. Technol. 26, 167–179. doi: 10.1016/S0925-5214(02)00040-6
Bai, Q., Huang, Y., and Shen, Y. (2021). The physiological and molecular mechanism of abscisic acid in regulation of fleshy fruit ripening. Front. Plant Sci. 11:619953. doi: 10.3389/fpls.2020.619953
Blaker, K. M., and Olmstead, J. W. (2014). Effects of preharvest applications of 1-methylcyclopropene on fruit firmness in southern highbush blueberry. Acta Hortic. 1017, 71–75. doi: 10.17660/actahortic.2014.1017.5
Brackmann, A., Weber, A., Giehl, R. F. H., Eisermann, A. C., Sautter, C. K., Gonçalves, E. D., et al. (2010). Armazenamento de mirtilo “Bluegem” em atmosfera controlada e refrigerada com absorção e inibição do etileno. Revista Ceres. 57, 6–11. doi: 10.1590/s0034-737x2010000100002
Buran, T. J., Sandhu, A. K., Azeredo, A. M., Bent, A. H., Williamson, J. G., and Gu, L. (2012). Effects of exogenous abscisic acid on fruit quality, antioxidant capacities, and phytochemical contents of southern high bush blueberries. Food Chem. 2012, 1375–1381. doi: 10.1016/j.foodchem.2011.11.124
Busatto, N., Vittani, L., Farneti, B., Khomenko, I., Caffini, M., Faccini, S., et al. (2022). Physiological and molecular characterization of the late ripening stages in Mangifera indica cv Keitt. Postharvest Biol. Technol. 183:111746. doi: 10.1016/j.postharvbio.2021.111746
Cappellin, L., Aprea, E., Granitto, P., Wehrens, R., Soukoulis, C., Viola, R., et al. (2012). Linking GC-MS and PTR-TOF-MS fingerprints of food samples. Chemometr. Intell. Lab. Syst. 118, 301–307. doi: 10.1016/j.chemolab.2012.05.008
Cappellin, L., Biasioli, F., Granitto, P. M., Schuhfried, E., Soukoulis, C., Costa, F., et al. (2011). On data analysis in PTR-TOF-MS: from raw spectra to data mining. Sensors Actuators B Chem. 155, 183–190. doi: 10.1016/j.snb.2010.11.044
Cappellin, L., Makhoul, S., Schuhfried, E., Romano, A., Sanchez del Pulgar, J., Aprea, E., et al. (2014). Ethylene: absolute real-time high-sensitivity detection with PTR/SRI-MS. The example of fruits, leaves and bacteria. Int. J. Mass Spectrom. 365–366, 33–41. doi: 10.1016/j.ijms.2013.12.004
Cappellini, R. A., Ceponis, M. J., and Koslow, G. (1982). Nature and extent of losses in consumer-grade samples of blueberries in greater New York. HortScience 17, 55–56.
Chen, H. J., Cao, S. F., Fang, X. J., Mu, H. L., Yang, H. L., Wang, X., et al. (2015). Changes in fruit firmness, cell wall composition and cell wall degrading enzymes in postharvest blueberries during storage. Sci. Hortic. 188, 44–48. doi: 10.1016/j.scienta.2015.03.018
Chervin, C., El-Kereamy, A., Roustan, J. P., Latché, A., Lamon, J., and Bouzayen, M. (2004). Ethylene seems required for the berry development and ripening in grape, a non- climacteric fruit. Plant Sci. 167, 1301–1305. doi: 10.1016/j.plantsci.2004.06.026
Chiabrando, V., and Giacalone, G. (2011). Shelf-life extension of highbush blueberry using 1-methylcyclopropene stored under air and controlled atmosphere. Food Chem. 126, 1812–1816. doi: 10.1016/j.foodchem.2010.12.032
Costa, D. V. T. A., Almeida, D. P. F., and Pintado, M. (2018). Effect of postharvest application of ethylene on the profile of phenolic acids and anthocyanins in three blueberry cultivars (Vaccinium corymbosum). J. Sci. Food Agric. 98, 5052–5061. doi: 10.1002/jsfa.9042
Costa, D. V. T. A., Pintado, M., and Almeida, D. P. F. (2014). Postharvest ethylene application affects anthocyanin content and antioxidant activity of blueberry cultivars. Acta Hortic. 1017, 525–530. doi: 10.17660/ActaHortic.2014.1017.65
Craker, L. E. (1971). Postharvest color promotion in cranberry with ethylene. HortScience 6, 137–139.
Cristescu, S. M., De Martinis, D., Hekkert, S.t. L., Parker, D. H., and Harren, F. J. M. (2002). Ethylene production by Botrytis cinerea in vitro and in tomatoes. Appl. Environ. Microbiol. 68, 5342–5350. doi: 10.1128/AEM.68.11.5342-5350.2002
Daniels, M., Simonne, A. H., Jensen, S., Diehl, D., Shelnutt, K. P., Bruhn, C., et al. (2018). Consumer perceptions of quality and price of high-value specialty crops in supermarkets in the United States: lessons for producers, handlers and educators. Acta Hortic. 1213, 21–30. doi: 10.17660/ActaHortic.2018.1213.3
DeLong, J. M., Prange, R. K., Bishop, C., and Harrison, P. A. (2003). The influence of 1-MCP on shelf-life quality of highbush blueberry. HortScience 38, 417–418. doi: 10.21273/HORTSCI.38.3.417
de Martino, G., Fabio Mencarelli, F., and Golding, J. B. (2007). Preliminary investigation into the uneven ripening of banana (Musa sp.) peel. N. Z. J. Crop. Hortic. Sci. 35, 193–199. doi: 10.1080/01140670709510185
Deng, J., Shi, Z., Li, X., and Liu, H. (2014). Effects of cold storage and 1-methylcyclopropene treatments on ripening and cell wall degrading in rabbiteye blueberry (Vaccinium ashei) fruit. Food Sci. Technol. Int. 20, 287–298. doi: 10.1177/1082013213483611
Ehlenfeldt, M. K., and Martin, R. B. (2002). A survey of fruit firmness in Highbush blueberry and species-introgressed blueberry cultivars. Hortscience 37, 386–389. doi: 10.21273/HORTSCI.37.2.386
Farneti, B., Di Guardo, M., Khomenko, I., Cappellin, L., Biasioli, F., Velasco, R., and Costa, F. (2017b). Genome-wide association study unravels the genetic control of the apple volatilome and its interplay with fruit texture. J. Exp. Bot. : 68 1467–1478. doi: 10.1093/jxb/erx018
Farneti, B., Emanuelli, F., Khomenko, I., Ajelli, M., Biasioli, F., and Giongo, L. (2020). Development of a novel phenotypic roadmap to improve blueberry quality and storability. Front. Plant Sci 11:1140. doi: 10.3389/fpls.2020.01140
Farneti, B., Khomenko, I., Grisenti, M., Ajelli, M., Betta, E., Algarra, A. A., et al. (2017a). Exploring blueberry aroma complexity by chromatographic and direct-injection spectrometric techniques. Front. Plant Sci. 8:617. doi: 10.3389/fpls.2017.00617
Fava, J., Alzamora, S. M., and Castro, M. A. (2006). Structure and nanostructure of the outer tangential epidermal cell wall in Vaccinium corymbosum L. (blueberry) fruits by blanching, freezing–thawing and ultrasound. Food Sci. Technol. Int. 12, 241–251. doi: 10.1177/1082013206065702
Forney, C. F., Kalt, W., Jordan, M., Vinqvist-Tymchuk, M. R., and Fillmore, S. A. E. (2012). Blueberry and cranberry fruit composition during development. J. Berry Res. 2, 169–177. doi: 10.3233/JBR-2012-034
Forsyth, F. R., and Hall, I. V. (1967). Oxygen absorption and ethylene production by developing cranberry fruit. Can. J. Plant Sci. 47, 153–156. doi: 10.4141/cjps67-026
Frenkel, C. (1972). Involvement of peroxidase and indole-3-acetic acid oxidase isozymes from pear, tomato, and blueberry fruit in ripening. Plant Physiol. 49, 757–763. doi: 10.1104/pp.49.5.757
Gao, X., Walworth, A. E., MacKie, C., and Song, G. Q. (2016). Overexpression of blueberry FLOWERING LOCUS T is associated with changes in the expression of phytohormone-related genes in blueberry plants. Horticulture Research. 3:16053. doi: 10.1038/hortres.2016.53
Gilbert, J. L., Olmstead, J. W., Colquhoun, T. A., Levin, L. A., Clark, D. G., and Moskowitz, H. R. (2014). Consumer-assisted selection of blueberry fruit quality traits. HortScience 49, 864–873. doi: 10.21273/HORTSCI.49.7.864
Giongo, L., Ajelli, M., Pottorff, M., Perkins-Veazie, P., and Iorizzo, M. (2022). Comparative multi-parameters approach to dissect texture subcomponents of highbush blueberry cultivars at harvest and postharvest. Postharvest Biol. Technol. 183:111696. doi: 10.1016/j.postharvbio.2021.111696
Giongo, L., Poncetta, P., Loretti, P., and Costa, F. (2013). Texture profiling of blueberries (Vaccinium spp.) during fruit development, ripening and storage. Postharvest Biol. Technol. 76, 34–39. doi: 10.1016/j.postharvbio.2012.09.004
Goto, R., Watanabe, M., Murakami, M., Sagawa, S., Komori, S., and Suzuki, A. (2013). The changes in IAA and ABA concentrations and ethylene evolution during fruit development in blueberry. Horticultural Research 12, 165–171. doi: 10.2503/hrj.12.165
Günther, C. S., Dare, A. P., McGhie, T. K., Deng, C., Lafferty, D. J., Plunkett, B. J., et al. (2020). Spatiotemporal modulation of flavonoid metabolism in blueberries. Front. Plant Sci. 11:545. doi: 10.3389/fpls.2020.00545
Guo, S., Iqbal, S., Ma, R., Song, J., Yu, M., and Gao, Z. (2018). High-density genetic map construction and quantitative trait loci analysis of the stony hard phenotype in peach based on restriction-site associated DNA sequencing. BMC Genomics 19:612. doi: 10.1186/s12864-018-4952-y
Gupta, V., Estrada, A. D., Blakley, I. C., Reid, R., Patel, K., Meyer, M., Andersen, S. U., Brown, A., Lila, M. A., and Loraine, A. (2014). RNA-Seq analysis and annotation of a draft blueberry genome assembly identifies candidate genes involved in fruit ripening, biosynthesis of bioactive compounds, and stage-specific alternative splicing. BioRxiv [Preprint]. doi: 10.1101/010116
Gupta, S., Radhakrishnan, A., Raharja-Liu, P., Lin, G., Steinmetz, L. M., Gagneur, J., et al. (2015). Temporal expression profiling identifies pathways mediating effect of causal variant on phenotype. PLoS Genet. 11:e1005195. doi: 10.1371/journal.pgen.1005195
Ireland, H.S., Gunaseelan, K., and Muddumage, R., Tacken, E. J, Putterill, J., Johnston, J.W., and Schaffer, R.J. (2014). Ethylene regulates apple (Malus× domestica) fruit softening through a dose× time-dependent mechanism and through differential sensitivities and dependencies of cell wall-modifying genes. Plant Cell Physiol., 55, 1005–1016. doi: 10.1093/pcp/pcu034
Janes, H. W., Chin, C.-K., and Frenkel, C. (1978). Respiratory upsurge in blueberries and strawberries as influenced by ethylene and acetaldehyde. Bot. Gaz. 139, 50–52. doi: 10.1086/336966
Karppinen, K., Hirvelä, E., Nevala, T., Sipari, N., Suokas, M., and Jaakola, L. (2013). Changes in the abscisic acid levels and related gene expression during fruit development and ripening in bilberry (Vaccinium myrtillus L.). Phytochemistry 95, 127–134. doi: 10.1016/j.phytochem.2013.06.023
Karppinen, K., Tegelberg, P., Häggman, H., and Jaakola, L. (2018). Abscisic acid regulates anthocyanin biosynthesis and gene expression associated with cell wall modification in ripening bilberry (Vaccinium myrtillus L.) fruits. Front. Plant Sci. 9:1259. doi: 10.3389/fpls.2018.01259
Li, Z., Wang, Z., Wang, K., Liu, Y., Hong, Y., Chen, C., et al. (2020). Co-expression network analysis uncovers key candidate genes related to the regulation of volatile esters accumulation in woodland strawberry. Planta 252:55. doi: 10.1007/s00425-020-03462-7
Lindinger, W., Hansel, A., and Jordan, A. (1998). On-line monitoring of volatile organic compounds at pptv levels by means of proton-transfer-reaction mass spectrometry (PTR-MS) medical applications, food control and environmental research. J. Mass Spectrom. Ion Processes 173, 191–241. doi: 10.1016/S0168-1176(97)00281-4
Lipe, J. A. (1978). Ethylene in fruits of blackberry and rabbiteye blueberry. J. Am. Soc. Hortic. Sci. 103, 76–77.
Liu, B. H., Wang, K. F., Shu, X. G., Liang, J., Fan, X. L., and Sun, L. (2019). Changes in fruit firmness, quality traits and cell wall constituents of two Highbush blueberries (Vaccinium corymbosum L.) during postharvest cold storage. Acta Hortic. 246, 557–562. doi: 10.1016/j.scienta.2018.11.042
Lobos, G. A., Bravo, C., Valdés, M., Graell, J., Ayala, I. L., Beaudry, R. M., et al. (2018). Within-plant variability in blueberry (Vaccinium corymbosum L.): maturity at harvest and position within the canopy influence fruit firmness at harvest and postharvest. Postharvest Biol. Technol. 146, 26–35. doi: 10.1016/j.postharvbio.2018.08.004
Lobos, G. A., Callow, P., and Hancock, J. F. (2014). The effect of delaying harvest date on fruit quality and storage of late highbush blueberry cultivars (Vaccinium corymbosum L). Postharvest Biol. Technol. 87, 133–139. doi: 10.1016/j.postharvbio.2013.08.001
MacLean, D. D., and Nesmith, D. S. (2011). Rabbiteye blueberry postharvest fruit quality and stimulation of ethylene production by 1-methylcyclopropene. HortScience 46, 1278–1281. doi: 10.21273/hortsci.46.9.1278
McAtee, P., Karim, S., Schaffer, R., and David, K. (2013). A dynamic interplay between phytohormones is required for fruit development, maturation, and ripening. Front. Plant Sci. 4:79. doi: 10.3389/fpls.2013.00079
Meng, X., Song, T., Chen, C., Zhang, H., Pan, Z., and Wang, J. (2020). Evaluation of pressurized inert gas treatments on the postharvest quality of blueberries. Food Bioprocess Technol. 13, 1918–1928. doi: 10.1007/s11947-020-02525-7
Minas, I. S., Tanou, G., Krokida, A., Karagiannis, E., Belghazi, M., Vasilakakis, M., et al. (2018). Ozone-induced inhibition of kiwifruit ripening is amplified by 1-methylcyclopropene and reversed by exogenous ethylene. BMC Plant Biol. 18:358. doi: 10.1186/s12870-018-1584-y
Moggia, C., González, C., Lobos, G. A., Bravo, C., Valdés, M., Lara, I., et al. (2018). Changes in quality and maturity of Duke and Brigitta blueberries during fruit development: postharvest implications. Acta Hortic. 1194, 1495–1501. doi: 10.17660/ActaHortic.2018.1194.209
Oh, H. D., Yu, D. J., Chung, S. W., Chea, S., and Lee, H. J. (2018). Abscisic acid stimulates anthocyanin accumulation in Jersey highbush blueberry fruits during ripening. Food Chem. 244, 403–407. doi: 10.1016/j.foodchem.2017.10.051
Ortiz, C. M., Franceschinis, F., Gergoff Grozeff, G. E., Chan, H., Labavitch, J. M., Crisosto, C., et al. (2018). Pre-treatment with 1-methylcyclopropene alleviates methyl bromide-induced internal breakdown, softening and wall degradation in blueberry. Postharvest Biol. Technol. 146, 90–98. doi: 10.1016/j.postharvbio.2018.08.018
Özgen, M., Palta, J. P., and Smith, J. D. (2002). Ripeness stage at harvest influences postharvest life of cranberry fruit: physiological and anatomical explanations. Postharvest Biol. Technol. 24, 291–299. doi: 10.1016/S0925-5214(01)00154-5
Paniagua, A. C., East, A. R., Hindmarsh, J. P., and Heyes, J. A. (2013). Moisture loss is the major cause of firmness change during postharvest storage of blueberry. Postharvest Biol. Technol. 79, 13–19. doi: 10.1016/j.postharvbio.2012.12.016
Paul, V., Pandey, R., and Srivastava, G. C. (2012). The fading distinctions between classical patterns of ripening in climacteric and non-climacteric fruit and the ubiquity of ethylene—an overview. J. Food Sci. Technol. 49, 1–21. doi: 10.1007/s13197-011-0293-4
Paz, O., Janes, H. W., Prevost, B. A., and Frenkel, C. (1982). Enhancement of fruit sensory quality by post-harvest applications of acetaldehyde and ethanol. J. Food Sci. 47, 270–273. doi: 10.1111/j.1365-2621.1982.tb11076.x
Pereira, L., Santo Domingo, M., Ruggieri, V., Argyris, J., Phillips, M. A., Zhao, G., et al. (2020). Genetic dissection of climacteric fruit ripening in a melon population segregating for ripening behavior. Hortic. Res. 7:187. doi: 10.1038/s41438-020-00411-z
Perkins-Veazie, P. (2016). “Blueberry,” in The Commercial Storage of Fruits, Vegetables, and Florist and Nursery Stocks. eds. K. C. Gross, C. Y. Wang, and M. Saltveit (Washington, DC: U.S. Dept. of Agriculture), 240–242.
Poole, P. R., Boyd, A. J., Wahrlich, S. M., and Patel, N. (1998). Ethylene biosynthesis in ripening and stored highbush blueberry cultivars. Acta Hortic. 464:505. doi: 10.17660/actahortic.1998.464.97
Retamales, J. B., and Hancock, J. F. (2012). Blueberries. Crop Production Science in Horticulture. CABI Publ. Co. Oxfordshire, UK. Series; no. 21.
Rivera, S., Kerckhoffs, H., Sofkova-Bobcheva, S., Hutchins, D., and Eas, t, A. (2021). Influence of water loss on mechanical properties of stored blueberries. Postharvest Biol. Technol. 176:111498. doi: 10.1016/j.postharvbio.2021.111498
Rivera, S. A., Zoffoli, J. P., and Latorre, B. A. (2013). Infection risk and critical period for the postharvest control of gray mold (Botrytis cinerea) on blueberry in Chile. Plant Dis. 97, 1069–1074. doi: 10.1094/PDIS-12-12-1112-RE
Romo-Muñoz, R. R., Dote-pardo, J. D., and Garrido-henrı́quez, H. G. (2019). Blueberry consumption and healthy lifestyles in an emerging market. Span. J. Agric. Res. 17:e0111. doi: 10.5424/sjar/2019174-14195
Ryu, S. B., Karlsson, B. H., Özgen, M., and Palta, J. P. (1997). Inhibition of phospholipase D by lysophosphatidylethanolamine, a lipid-derived senescence retardant. Proc. Natl. Acad. Sci. U. S. A. 94, 12717–12721. doi: 10.1073/pnas.94.23.12717
Sargent, S. A., Berry, A. D., Brecht, J. K., Santana, M., Zhang, S., and Ristow, N. (2017). Studies on quality of southern highbush blueberry cultivars: effects of pulp temperature, impact and hydrocooling. Acta Hortic. 1180, 497–502. doi: 10.17660/ActaHortic.2017.1180.70
Shimura, I., Kobayashi, M., and Ishikawa, S. (1986). Characteristics of fruit growth and development in Highbush and Rabbiteye blueberries (Vaccinium corymbosum L. and V. ashei Reade) and the differences among their cultivars. Journal of the Japanese Society for Horticultural Science 55, 46–50. doi: 10.2503/jjshs.55.46
Song, J., Fan, L., Forney, C. F., Jordan, M. A., Hildebrand, P. D., Kalt, W., et al. (2003). Effect of ozone treatment and controlled atmosphere storage on quality and phytochemicals in highbush blueberries. Acta Hortic. 600, 417–423. doi: 10.17660/ActaHortic.2003.600.62
Suzuki, A., Kikuchi, T., and Aoba, K. (1997a). Effects of ethylene on fruit set and maturation of highbush blueberry (Vaccinium corymbosum L.). J. Jap. Soc. Hortic. Sci. 66, 267–272. doi: 10.2503/jjshs.66.267
Suzuki, A., Kikuchi, T., and Aoba, K. (1997b). Changes of ethylene evolution, ACC content, ethylene forming enzyme activity and respiration in fruits of highbush blueberry. J. Jap. Soc. Hortic. Sci. 66, 23–27. doi: 10.2503/jjshs.66.23
Tao, S., Chu, H., Chen, X., Yuan, H., Qiu, L., Zhao, L., et al. (2017). Study of the effects of 1-MCP to blueberry under cold storage. IOP Conference Series: Earth Environ. Sci. 61:012041. doi: 10.1088/1755-1315/61/1/012041
Tosetti, R., Elmi, F., Pradas, I., Cools, K., and Terry, L. A. (2020). Continuous exposure to ethylene differentially affects senescence in receptacle and achene tissues in strawberry fruit. Front. Plant Sci. 11:174. doi: 10.3389/fpls.2020.00174
Vicente, A.R., Ortugno, C., Rosli, H., Powell, A.L.T, Greve, C.L., and Labavitch, J.M. (2007). Temporal sequence of cell wall disassembly events in developing fruits. 2. Analysis of blueberry (Vaccinium species). J. Agric. Food Chem., 55, 4125–4130. doi: 10.1021/jf063548j
Wang, C., Gao, Y., Tao, Y., Wu, X., and Zhibo, C. (2017). Influence of γ-irradiation on the reactive-oxygen metabolism of blueberry fruit during cold storage. Innov. Food Sci. Emerg. Technol. 41, 397–403. doi: 10.1016/j.ifset.2017.04.007
Wang, Y. W., Malladi, A., Doyle, J. W., Scherm, H., and Nambeesan, S. U. (2018b). The effect of ethephon, abscisic acid, and methyl jasmonate on fruit ripening in rabbiteye blueberry (Vaccinium virgatum). Horticulturae. 1–13. doi: 10.3390/horticulturae4030024
Wang, S., Zhou, Q., Zhou, X., Wei, B., and Ji, S. (2018a). The effect of ethylene absorbent treatment on the softening of blueberry fruit. Food Chem. 246, 286–294. doi: 10.1016/j.foodchem.2017.11.004
Wang, S., Zhou, Q., Zhou, X., Zhang, F., and Ji, S. (2020). Ethylene plays an important role in the softening and sucrose metabolism of blueberries postharvest. Food Chem. 310:125965. doi: 10.1016/j.foodchem.2019.125965
Watada, A. E. (1986). Effects of ethylene on the quality of fruits and vegetables. Food Technol. 40, 82–85.
Watanabe, M., Goto, R., Murakami, M., Komori, S., and Suzuki, A. (2021). Interaction between ethylene and abscisic acid and maturation in Highbush blueberry. Horticulture Journal. 90, 14–22. doi: 10.2503/hortj.UTD-210
Windus, N. D., Shutak, V. G., and Gough, R. E. (1976). CO2 and C2H4 evolution by highbush blueberry fruit. HortScience 11, 515–517.
Xu, F., and Liu, S. (2017). Control of postharvest quality in blueberry fruit by combined 1-methylcyclopropene (1-MCP) and UV-C irradiation. Food Bioprocess Technol. 10, 1695–1703. doi: 10.1007/s11947-017-1935-y
Xu, F., Liu, Y., Dong, S., and Wang, S. (2020). Effect of 1-methylcyclopropene (1-MCP) on ripening and volatile compounds of blueberry fruit. J. Food Process. Preserv. 44, 1–11. doi: 10.1111/jfpp.14840
Xu, F., Liu, S., Liu, Y., and Wang, S. (2021). Effect of mechanical vibration on postharvest quality and volatile compounds of blueberry fruit. Food Chem. 349:129216. doi: 10.1016/j.foodchem.2021.129216
Xue, Y. L., Yu, H. T., Zhang, P., and Li, J. K. (2020). Comparison of shelf quality of different treatments on the blueberries. Mod. Food Sci. Technol. 36, 113–121. doi: 10.13982/j.mfst.1673-9078.2020.5.016
Yokotani, N., Nakano, R., Imanishi, S., Nagata, M., Inaba, A., and Kubo, Y. (2009). Ripening-associated ethylene biosynthesis in tomato fruit is autocatalytically and developmentally regulated. J. Exp. Bot. 60, 3433–3442. doi: 10.1093/jxb/erp185
Zhao, T., Nakano, A., and Iwasaki, Y. (2021). Differences between ethylene emission characteristics of tomato cultivars in tomato production at plant factory. J. Agric. Food Res. 5:100181. doi: 10.1016/j.jafr.2021.100181
Zheng, X., and Wolff, D. (2000). Ethylene production, shelf-life and evidence of RFLP polymorphisms linked to ethylene genes in melon (Cucumis melo L.). Theor. Appl. Genet. 101, 613–624. doi: 10.1007/s001220051523
Zhu, P., Xu, L., Zhang, C., Toyoda, H., and Gan, S. S. (2012). Ethylene produced by Botrytis cinerea can affect early fungal development and can be used as a marker for infection during storage of grapes. Postharvest Biol. Technol. 66, 23–29. doi: 10.1016/j.postharvbio.2011.11.007
Zifkin, M., Jin, A., Ozga, J. A., Zaharia, L. I., Schernthaner, J. P., Gesell, A., et al. (2012). Gene expression and metabolite profiling of developing Highbush blueberry fruit indicates transcriptional regulation of flavonoid metabolism and activation of abscisic acid metabolism. Plant Physiol. 158, 200–224. doi: 10.1104/pp.111.180950
Keywords: Vaccinium spp., SRI-ToF-MS, texture analyzer, germplasm, biparental population
Citation: Farneti B, Khomenko I, Ajelli M, Emanuelli F, Biasioli F and Giongo L (2022) Ethylene Production Affects Blueberry Fruit Texture and Storability. Front. Plant Sci. 13:813863. doi: 10.3389/fpls.2022.813863
Edited by:
Ernst Woltering, Wageningen University and Research, NetherlandsReviewed by:
Bastiaan Brouwer, Umeå University, SwedenGeorge Manganaris, Cyprus University of Technology, Cyprus
Copyright © 2022 Farneti, Khomenko, Ajelli, Emanuelli, Biasioli and Giongo. This is an open-access article distributed under the terms of the Creative Commons Attribution License (CC BY). The use, distribution or reproduction in other forums is permitted, provided the original author(s) and the copyright owner(s) are credited and that the original publication in this journal is cited, in accordance with accepted academic practice. No use, distribution or reproduction is permitted which does not comply with these terms.
*Correspondence: Brian Farneti, brian.farneti@fmach.it