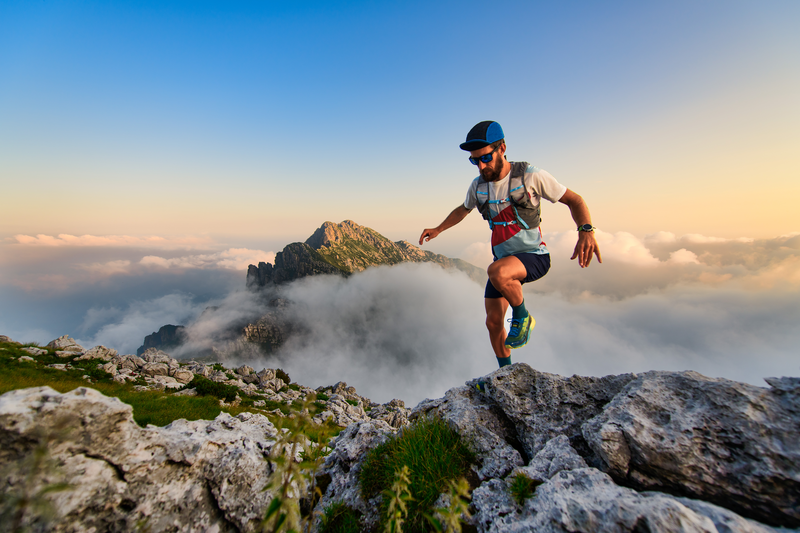
94% of researchers rate our articles as excellent or good
Learn more about the work of our research integrity team to safeguard the quality of each article we publish.
Find out more
REVIEW article
Front. Plant Sci. , 11 February 2022
Sec. Plant Abiotic Stress
Volume 13 - 2022 | https://doi.org/10.3389/fpls.2022.807844
The 21st century presents many challenges to mankind, including climate change, fast growing human population, and serious concerns over food security. Wheat is a leading cereal crop that largely fulfills the global food needs. Low temperature stress accompanied by nutrient-starved soils is badly disrupting the source–sink relationship of wheat, thus causing an acute decline in final yield and deteriorating the grain quality. This review paper aimed to understand how low temperature stress affects wheat source–sink organs (i.e., leaves, roots, and spikes) and how phosphorus application reliefs in alleviating its harmful consequences. Also, we discussed mitigation strategies to enhance wheat capacity to adapt to varying temperature extremes and made rational recommendations based on modern agronomic and breeding approaches. Therefore, this study is likely to establish a solid foundation for improving the tolerance to low temperature stress and to improve its phosphorus utilization efficiency in wheat.
To meet the dietary needs of 10 billion people by 2050, current food productivity will have to rise by 60% (Hickey et al., 2019). Wheat (Triticum aestivum L.) is an important cereal crop consumed as a staple food across the globe (Muhammad et al., 2021), which occupies about 220 million hectares of cropland worldwide (Erenstein et al., 2021). As a vital source of plant protein, wheat is easy to be processed into various types of food products, consumed by billions of people, playing an important role in reducing hunger (Subedi et al., 2019; Wojtowicz et al., 2020). According to the latest IPCC report, the global temperature rise is expected to reach or exceed 1.5°C by the end of the 21st century (IPCC, 2021). Global warming increases the instability of the climate system, and extreme low and high temperature events occur frequently (Chen et al., 2019a). Low temperature stress (LTS) causes substantial decline in wheat yield in major wheat-growing regions of the world (e.g., Europe, China, the United States, and Australia; Table 1).
Global warming conditions accelerate growth and development of wheat, resulting in significant advancement of the low-temperature-sensitive stage of wheat, thus increasing the risks of chilling stress (0 ~ 15°C) or freezing stress (<0°C; Ji et al., 2017; Xue et al., 2019; Liu et al., 2020a). Sub-zero temperatures induce extracellular ice, which severely damages the membranous structures and dehydrates the cell (Roman-Figueroa et al., 2021). It stimulates the dry conditions in roots, leaves, and spikes (Aroca et al., 2012), consequently shortens the plant height, and reduces leaf area and spike size (Valluru et al., 2012). LTS also induces photoinhibition by reducing plant capability to assimilate light energy (Mattila et al., 2020). Generally, the imbalanced source–sink relationship deteriorates the wheat yield and quality by reducing root absorption capacity, declining photosynthetic activity, poor spike differentiation, and delayed grain filling under LTS (Li et al., 2014; Zhang et al., 2019; Kul et al., 2020).
Soil degradation also greatly interferes with wheat productivity (Alewell et al., 2020; Raimondo et al., 2020). Phosphorus (P) is an essential nutrient for wheat growth and development, and about 40% agricultural lands are deficient in P worldwide (Lynch, 2011; Rafiullah et al., 2020). The deficiency of P not only limits the crop growth but also reduces the plant capacity to withstand adverse impacts of LTS (Gong et al., 2020). In soil, P exists in both organic and inorganic form, while plants generally intake the inorganic phosphate (Pi: H2PO4− and HPO42−) through the adsorption process (Shen et al., 2011). Inadequate P uptake inhibits the development of plant organs, resulting in more prone to the LTS, eventually lower down grain yield (Cong et al., 2020; Oliverio et al., 2020). Many studies described that P application enhanced the drought and salt tolerance in field crops (Bargaz et al., 2016; Karimzadeh et al., 2021). Nevertheless, fewer studies depicted the relationships and interactions between LTS and P application (Cordell and Neset, 2014).
This review article abridged the damaging effects of LTS on wheat source–sink organs and highlighted the prominent breeding and agronomic approaches to enhance the wheat LTS tolerance and PUE by implying recommended crop husbandry practices. Further, various doable measures are proposed to conserve the subsoil phosphate resources, increase the availability of Pi to the roots, and to improve the PUE and LTS tolerance capacities in wheat. This review intended to open-up new research directions for crop stress resistance and mineral resource management.
LTS undermines the plant’ ability to smoothly acquire water and nutrients, resulting in chlorotic leaves, stunted growth, disrupted ROS metabolism, floret abortions, and poor seed setting. This section mainly discusses the damaging effects of LTS in wheat from morphological, physiological and molecular perspectives (Tables 2, 3).
Roots are considered vital plant organs, which are involved in active nutrient transport from soil to other plant parts (Kim et al., 2020). The root growth is depicted as an ecologically regulated phenomenon (Buriro et al., 2011; Kul et al., 2020). Despite clearly visible detrimental effects of LTS in the aboveground portion of the plant, it also severely disrupts active functioning of root system (Kul et al., 2020).
Development patterns and morphological structures of roots are considerably affected by LTS (Shibasaki et al., 2009; Koevoets et al., 2016). LTS restricts the active root growth, particularly upon continuous low temperature exposure, root surface area decreased, branching angle, and contact area narrowed (Nagel et al., 2009). Lateral root formation slower down under LTS (≤10°C), and root volume decreased by 60% than that at 25°C (Nagel et al., 2009). In an experimental investigation, spring wheat and winter wheat were subjected to LTS at 5°C; subsequently, root growth diminished by 60 and 75% as compared to control at 25°C, respectively (Equiza, 2001). Similar results were found in rice, where root length and biomass significantly decreased by 51% under LTS exposure (Hsu and Hsu, 2019; Kul et al., 2020).
The active absorption capacity of root systems is vital for maintaining an adequate root–shoot ratio and optimum crop productivity (Equiza, 2001). Sub-optimal conditions disrupt the root water uptake, causing water scarcity in the stem that leads to drought stress; and in the meantime, decreased root absorption capacity and hydraulic conductivity are anticipated with decreased leaf transpiration rate under low temperature and drought conditions (Aroca et al., 2012). Subsequently, drought-induced imbalanced water relations decline the nutrient intake and restrict its transport, ultimately reducing wheat plant growth and development (Nezhadahmadi et al., 2013; Hussain et al., 2018). In an experiment, rice seedlings were subjected to LTS, which led to increased electrolyte leakage, elevated release of hydrogen peroxide and malondialdehyde contents that severely damaged root cells (Hsu and Hsu, 2019). Moreover, LTS also influences the soil’s physio-chemical characteristics that affect the soil microbial activity; later, followed by poor plant–nutrient relations (Yan et al., 2003).
LTS hinders the biosynthesis of auxins (IAA) by suppressing the gene expression of ARR1/12 and the gene expression of PIN1/3/7 that reduces the rootward flow of IAA; subsequently decreased accumulation of IAA in root cells restricts the active cell division of root’s meristematic tissues (Zhu et al., 2015). Thus, poor IAA accumulation is a primary reason for the reduced branching of lateral roots. Sub-optimal conditions give rise to imbalanced osmotic potential, which greatly inhibits active osmoregulation and triggers osmotic and oxidative stress (Sawant et al., 2021). The molecular expression of TaSnRK2.7 is considered a multifunctional regulatory factor involved in carbohydrate metabolism and reducing osmotic potential in wheat plants under sub-optimal temperature conditions (Zhang et al., 2011). Likewise, other factors, such as phytohormone regulation, expression of PIP-Aquaporins, rate of evapotranspiration, and osmotic balance, are thought to be vital for alleviating oxidative stress and active uptake of water and nutrients by roots under low temperature conditions (Aroca et al., 2012; Balliu et al., 2021).
It is briefly concluded that LTS causes a significant reduction in root surface area and lateral branching; subsequently, it interrupts the water and nutrient relations that limits the plant’s capacity to explore more water and mineral resources. There have been few studies that investigated root activity and its molecular expressions under LTS.
Plant leaves are known as food factories because they play an essential role in the process of photosynthesis (PS) and dry matter production. LTS not only instigates root dehydration but leaves as well.
Dehydration conditions accompanied by LTS, accelerates the outer membrane destruction, electrolyte leakage in leaf cells, leading to chlorosis, wilting, and eventually necrosis (Dikilitas et al., 2021). Besides, LTS shrinks the leaf area, reduces chlorophyll concentration, alters leaf pigment ratio, and damages the leaf sponge structure (Chinnusamy et al., 2007; Wang et al., 2016b; Zhang et al., 2021b). These morphological changes in leaf area, size, and color are the first visible symptoms of LTS induced damage, and the degree of damage is determined by intensity and duration of LTS (Valluru et al., 2012; Keramidas et al., 2020). In an experimental investigation, wheat plants are exposed to −5°C for 3 d, leaf wilting starts after 1 d and are wholly drooped after 3 d (Han et al., 2013). Fuller et al. (2007) documented that wheat exposure to −3°C for 2 h directly damages the flag leaves, and the damage magnitude intensifies if the temperature drops to −5°C. It is reported that the leaf area index of winter wheat reduced by 43.8% on exposure to below 0°C for 24 h duration, as compared to control (mean temperature: 11°C) treatment (Liu et al., 2019a).
A plant cell under cold stress experiences severe physiological and biochemical disturbances, which exhibits in leaf chlorosis, wilting, and even necrosis (Ruelland and Zachowski, 2010; Muhammad et al., 2021). The primary sources of wheat grain production are PS and biomass accumulations; these physiological processes are highly vulnerable to LTS (Sharma et al., 2019; Zhang et al., 2020). LTS adversely affects the chloroplast development, chlorophyll biosynthesis, electron transport chain, photophosphorylation, Rubisco efficiency, and carbohydrates transport, resulting in a declining rate of PS and biomass accumulation (Venzhik et al., 2011; Li et al., 2014). Phosphate cycling and photophosphorylation are restricted because LTS inhibits starch and sucrose biosynthesis, resulting in feedback-limited PS (Savitch et al., 2010). Xu et al. (2013) revealed that excessive soluble carbohydrate accumulation inhibits PS and photosynthetic electron transport efficiency by downregulating the level of photosynthetic carbon reduction cycle enzymes. A study has documented an 18% decrease in PS when wheat seedlings were exposed to a low temperature (4°C) in a controlled chamber for 7 d (Cvetkovic et al., 2017). Frost burning of flag leaves resulted in complete restriction of photosynthetic activity (Nevyl and Battaglia, 2021). In cold-sensitive cultivars, photosynthetic activity is more susceptible to cold stress than in cold-tolerant cultivars (Yamori et al., 2009).
ROS is an important indicator for plants to respond to LTS, and its dynamic balance maintains the cell stability and normal plant growth (Sharma et al., 2012). LTS instigates the ROS imbalance by reducing molecular oxygen and producing ROS, and this imbalance is extremely damaging to metabolic processes (Muhammad et al., 2021). With the increase of low temperature frequency, intensity, and duration, extreme LTS occurred that led to excessive accumulation of ROS and membrane lipid peroxidation and subsequently caused significant damage to the chloroplast structure, photosynthetic apparatus, and accelerated the leaf senescence (Huang et al., 2019; Liu et al., 2020b).
Environmental variations enforced crop plants to evolve diverse adaptation approaches (Chong et al., 2021). The accumulation of soluble sugars, several amino acids, and the expression of antifreeze proteins (PS and electron transfer related protein) in wheat leaves contribute in maintenance of cell turgidity through osmotic balance and the increased capacity of frost tolerance (Markovskaya et al., 2010; Yang et al., 2011; Xu et al., 2013). Many transcriptional studies of Arabidopsis thaliana and wheat shows that LTS significantly inhibits the expression of PS-related genes (Gulick et al., 2005; Gan et al., 2019); in which C-repeat/dehydration-responsive element binding transcription factors mediates low temperature signal transduction pathway (Arshad et al., 2016; Jiang et al., 2020). Abscisic acid (ABA) regulation genes, such as TaMYB33, are involved in the production of antioxidants, which are beneficial to ROS scavenging, proline accumulation, and osmotic balance (Qin et al., 2012). Likewise, in another experimental study, TaSAG3 and TaSAG5 have expressed naturally in wheat leaf senescence; followed by low temperature treatment exhibited stable expressions for TaSAG5 and highly instable expressions for TaSAG3 at a low level (Zhao et al., 2012). At this point, ROS transforms from second messengers to cell killers. The mechanism of how abiotic stress affects plant redox states is not clear yet, needs more experimental studies to explore (Huang et al., 2019).
In brief, LTS has drastic impacts on leaf morphology and interior cell structure. Excessive production of ROS disrupts the photosynthetic activity by hindering active electron transfer and accelerated leaf senescence. The decrease of leaf area and net photosynthetic rate resulted in the decrease of assimilates and other photosynthetic products.
LTS hinders the wheat growth at all stages, from seedling to maturity, but the intensity of the damage varies (Muhammad et al., 2021). Many experimental studies established that wheat is more sensitive to LTS at the reproductive stage than vegetative, particularly its susceptibility higher from heading to anthesis, that leads to significant yield losses (Barlow et al., 2015; Zhang et al., 2021d). The top spikelets are considered more sensitive to LTS, followed by the basal and central spikelets (Liu et al., 2020a). The negative effects of LTS not only depends on intensity and duration of LTS but also on crop cultivars and soil fertility (Soares et al., 2019).
The negative impacts of LTS on spike development are well documented; most crucial growth period, associated with LTS, begins after pollen development enters meiosis phase prior to anthesis (Slafer et al., 2001). LTS results in short statured spikes, reduced number of spikelets, pollination failure, poor grain filling, and inadequate seed setting (Liu et al., 2019a). Wheat plant’s upper spikelets turns pale yellow or partially dies on exposure to LTS at booting stage (Kodra et al., 2011; Sanghera et al., 2011). Dehydration accompanied by LTS results in abnormal pollen and ovule development, leading to pollen inactivation, floret sterility, and eventually cause abortion (Ali and Malik, 2021). Denatured spikelets, reduced assimilate transport, decreased dry matter accumulation, and poor grain yield are all consequences of LTS (Whaley et al., 2004).
LTS interrupts the balance of source–sink relationship by limiting key physiological process of sucrose accumulation to wheat spikes (Zhang et al., 2019). LTS induced excessive ROS production limits the photosynthetic activity as well as respiration activity that subsequently leads to oxidative damage to sink organs (i.e., spike; anther’s desiccation). In previous studies, LTS induced short statured spikes were attributed to the overexpression of miRNA156 (Liu et al., 2017). Furthermore, LTS induced ABA pathway affects the sucrose metabolism and related gene expressions in spikelets, subsequently delaying floret and spikelet development (Zhang et al., 2019). The similar trends were found in rice (Oryza sativa L.) in which acid invertase (AI) activity was inhibited under LTS, and the quantity of sucrose transportation to the pollen grains and tapetum severely decreased, thus leading to pollen abortion (Oliver et al., 2007). However, it is not clear whether the frost damage in wheat at anthesis is due to the lack of acclimation expressions or the insufficient environmental stimulus to upregulate the acclimation genes (Fuller et al., 2007). Recently, a research finding revealed that wheat spike development under cold conditions in early spring was accelerated due to increased ABA contents and the upregulation of encoding PP2Cs, SnRK2s, and bZIP transcription factors (Yu et al., 2021).
Worldwide, researchers are working hard to improve the wheat’s yield, quality, and adaptability to various environmental conditions (Osman et al., 2016; Fleitas et al., 2020). The adverse effects of LTS on yield of wheat plants before heading stage can be compensated by the development of newly formed tillers (Frederiks et al., 2012), whereas yield damage caused by late spring coldness to wheat tillers and newly formed spikes cannot be rectified. This is due to reduction in grain number per plant (GNPP), spike number per plant (SNPP), and grains number per spike (GNPP = SNPP × GNPS; Liu et al., 2020a). The yield of two cultivars (with varying temperature tolerance capacity) decreased by 4.6 and 13.9% under LTS (2°C/0°C, day/night) at jointing stage for 24 h, respectively; and the damages to tillers and spikes are the main reason for reduction in grain yield per plant (GYPP; Li et al., 2015). The grain yield per plant of spring wheat and semi-winter wheat decreased between 4.6–56.4% and 3.1–44.6% under LTS (the mean temperature in the range of −1 to 3°C) at jointing stage, respectively, while decreased by 13.9–85.2% and 3.2–85.9% under LTS at booting stage, respectively (Liu et al., 2019b).
LTS considerably deteriorates the grain quality. Study has shown that sub-optimal temperature conditions have a detrimental impacts on assimilate accumulation during grain filling, leading to deterioration of grain quality and quantity (Yang and Zhang, 2006). Since LTS inhibited both the absorption and distribution of beneficial nutrient elements, nitrogen (N) compounds and non-structural carbohydrates did not reach spikes (Zhang et al., 2021a). Additionally, the decreased canopy temperature resulted in reduced starch and protein accumulation, followed by deformed grain appearance (grain length and size; Liu et al., 2019b).
In summary, LTS limits the normal spike development, which causes severe reduction in grain yield and quality. The effects of LTS on yield loss at early growth stages (vegetative: emergence, tillering, etc.) are not as detrimental as those of later growth stages (reproductive: booting, grain filling, etc.). The impact of LTS on wheat spikes varies with varying temperature intensity and duration, growth phases, and cultivars.
It is evident from various experimental investigations that optimum mineral nutrition is vital in combating abiotic stresses, that is, LTS, salinity stress, and drought stress (Waraich et al., 2011; Bouain et al., 2019). P as an essential plant nutrient, not only take part in various metabolic activities (i.e., PS, carbohydrates accumulation, assimilate transportation and distribution, and electron transport), but also vital in strengthening the plant’s adaptability to the external environmental factors via taking part in various biological pathways (i.e., signal transduction, energy kinetics, enzymatic catalysis; Soetan et al., 2009). In general, P absorption is primarily affected by temperature change; therefore, in cold weather conditions, P application could significantly increase wheat growth and development. In this section, we discussed how deficient soil conditions coupled with LTS adversely affect the active growth of source–sink organs in wheat.
Fertilizer consumptions have been steadily increasing since the green revolution, but wheat productivity has remained stagnant in last decade. Since use of different fertilizer application methods and varying soil nutrients supplying capacities, there were large variations noted in wheat nutrient use efficiencies (Jat and Gerard, 2014). In modern-day crop cultivation, P is an integral part of plant metabolism, ranked second essential nutrient after N (Nedelciu et al., 2020). It is expected that global P demand will exceed the supply in 2045 (Nedelciu et al., 2020). Despite the high input of chemical fertilizer, global P shortage is intensified by soil erosion (Alewell et al., 2020). Nowadays, about 50% of agricultural lands across the world are facing P deficiency, which is becoming a serious threat to crop production (Lynch, 2011). To meet the food demands of increasing population, it requires effective measures to prevent fertile agricultural lands from P starvation.
It is known that many abiotic factors are simultaneously influencing the depletion of soil mineral resources, and there is already a shortage of available phosphate in soil (Lynch, 2011). P is relatively easy to be fixed in the soil and becomes immovable. The P-deficient soil accelerates the increase in sucrose transport through phloem toward roots, where Pi transporters cause outward efflux and cause secretions of organic phosphatic acids (Hammond and White, 2007). The P deficiency symptoms are clearly visible in wheat plants: short stature, slender stems/peduncles, yellowish spots on leaves, delayed flowering, poor grain filling, and late maturity (Gross et al., 2021). It further hinders the primary root growth (Linkohr et al., 2002). Moreover, P deficiency directly influences the normal functioning of biochemical and physiological activities, that is, disrupted cell division and protein nucleation (Linkohr et al., 2002; Péret et al., 2011).
Nevertheless, plants have tendency to improve the uptake and utilization of P by changing root system architecture (RSA) and inducing the expression of P starvation response genes (Baek et al., 2017; Cho et al., 2021). It is verified from research investigations that protein expression of TaPHT2; 1 is a significant factor in P signaling, and its upregulated expression under P-deficient conditions is beneficial in increasing Pi concentration in chloroplasts, enhancing photosynthetic capacity, and P accumulation in wheat plants (Guo et al., 2013; Aziz et al., 2014; De et al., 2019).
The optimal dose of P is needed in wheat plants to enhance primary root growth, improve water–nutrient relations and osmotic stress tolerance, and enhance photosynthetic activity facilitated by increased leaf chlorophyll content and dry matter accumulation (Figure 1). These traits are critical in defining the production quantity and quality in wheat. In this section, we briefly discussed the role of P in low temperature resistance in combination with the source–sink pools of wheat.
Figure 1. LTS tolerance mechanism by optimizing P application in wheat. Optimizing P application improves soil–plant nutrient relationships, maintains source-sink balance and membrane stability, relieves dehydration and oxidative stress, and enhances crop stress resistance and productivity in wheat. And root surface area and root activity increase in roots; functional leaf area, PS and photoproduct increase in leaves; flower abortion decreases, but pollen activity, grain yield and quality increase in spikes by P application, respectively. AMF: arbuscular mycorrhizal fungi, ATP: adenosine triphosphate [Here, ↓ indicates a decrease and ↑ indicates an increase/improvement].
Many studies have focused on the aboveground part and ignored the belowground part (roots) of plants, but root systems play a vital role in mitigating the adverse effects of LTS (Ambroise et al., 2020). Beneficial microorganisms and mineral nutrients are helpful to improve the cold tolerance of wheat roots (Zhou et al., 2021). Although P is relatively easy to be fixed in the soil and becomes immovable, but soil microbial activities are critical in converting insoluble phosphates into soluble phosphates, phosphate solubilizing bacteria (PSB) play a key role in this process (Tabassum et al., 2017; Chatterjee et al., 2021). The P fertilization is recommended to alleviate the negative impacts of LTS and rectify the deficiency symptoms in wheat. It also enhances the belowground microbial activity which is mutually beneficial for healthy root growth and development. Increased microbial activity excites soil temperature to a certain extent that contributes in alleviating the adverse impacts of LTS.
Further, P plays an important role in regulating root system responses to drought stress (Jin et al., 2015; Attarzadeh et al., 2020), because P application prevents crop plants from oxidative stress and improve chloroplast structure, while excessive P application trigger ROS toxicity (Shibli et al., 2006; Noor et al., 2021). Combined with N and potassium (K), P application promotes the secondary root growth, proliferation of growing root tips, and root hair differentiation; subsequently root surface area increased that supports in active nutrient–water uptake (Gahoonia et al., 1999).
An efficient RSA is vital for adequate utilization of mineral and water resources under LTS. Dynamically developed RSA favors the active uptake of available phosphate and other mineral nutrients for the optimal growth of aboveground plant organs (Shen et al., 2011; Dijkstra et al., 2016). Adequate P supply accelerates root growth and its spread in deeper soil layers (Jin et al., 2015). It is reported that beneficial relationship between arbuscular mycorrhizal fungi (AMF) promote P uptake and utilization. AMF is vital in stabilizing soil aggregates and regulating the non-nutritional functioning in field crops that enhance the LTS tolerance by preventing membrane lipid peroxidation (Zhu et al., 2010; Latef and He, 2011; Gerz et al., 2018). Similarly, strigolactones (SLs) secretions from root play a significant role in active N and P uptake by establishing the symbiotic relationship between plants and soil microbes, and it is also crucial in tolerating various biotic and abiotic stresses (Halouzka et al., 2020; Jamil et al., 2020). According to De et al. (2019), two wheat cultivars with different PUEs on fertilization exhibited 60 and 80% increases, respectively, while their PUEs varies by 17% upon no fertilization; this increase in PUE is due to SLs-induced regulation of PHO2 activity.
In brief, application of P not only improves the soil P deficiency, but also improves the RSA of wheat that is key factor in active nutrient uptake and mitigating biotic and abiotic stresses. In addition, AMF, PSB, and SLs promote the active P absorption from soil.
Leaves are considered as primary source organs of the plant. Several environmental factors negatively influence leaf functioning and hinder optimal crop growth (Gong et al., 2020). PS is the key physiological process that mainly takes place in the leaf. P fertilization is essential for the development of healthy wheat leaf and maintains the normal PS process (Sharma et al., 2019). LTS repercussions (i.e., membrane impairment, imbalanced osmoregulation, and excessive ROS production) substantially reduced the rate of PS. In this aspect, P fertilization is a handy tactic in alleviating the negative impacts of LTS.
The optimal dose of P is not only helpful in sustaining membrane stability and increasing leaf surface area, but also enhances the leaf chlorophyll and carotenoid contents; subsequently, it increases the rate of PS, enhances the dry matter production, improves the stomatal conductivity and improved the plant–water relations (Waraich et al., 2011; Shirmohammadi et al., 2020). Optimal allocation of P in plants can prolong and improve the P utilization in PS (Veneklaas et al., 2012). It has been reported that foliar application of plant growth regulators (i.e., SLs, salicylic acid, and ABA) significantly enhanced the activities of antioxidant enzymes, which facilitates in preventing membrane peroxidation under drought and LTS (Sun et al., 2009; Ma et al., 2017; Saleem et al., 2021). Accumulation of fructans in cereal crops upon experiencing low temperature environmental conditions is another preventive approach in maintaining membrane stability (Livingston et al., 2009). In a field experiment, combined P (200 mg P2O5 kg−1 soil) and molybdenum fertilizer at the tillering stage of winter wheat upshots the leaf soluble sugars contents by 9.7% and reduced the malondialdehyde content by 28.4% (Nie et al., 2015). In addition, P application under abiotic stress also increases the uptake and utilization of N, increases the concentration of soluble sugar and Pi in plants, and high Pi availability for carbon assimilation maximizes leaves photosynthetic activity (Jin et al., 2015).
P supply relieved the symptoms of LTS through modulating the activities of antioxidant enzymes and maintaining the regular osmotic homeostasis in leaves. Normal morphology and physiology of wheat leaves can maintain the sustainability of PS, the vital ingredient for plant growth and total biomass production.
Wheat spikes are known as the primary sink pool of wheat, which accumulates the maximum number of photosynthates. LTS induce deformities in spike shape and appearance and badly influences SNPP, GNPP, spike length, and 1,000-grain weight. These basic agronomic parameters are key components of the final wheat yield (Chen et al., 2019b). P deficiency combined with LTS causes a drastic reduction in the number of spikes and productive tillers (El Mazlouzi et al., 2020a). Application of P under LTS maintains the source–sink balance through promoting photosynthetic activity and improving grain filling rate (Engels et al., 2012; Zangani et al., 2021). The vegetative organs (i.e., leaves, stems, and roots) in wheat used as P reserves, which later was transported to harvest organs (i.e., spikes), after anthesis, to promote grain filling and ripening, as P deposition in the grains was mainly brought through remobilization of internal P sources stored before anthesis (El Mazlouzi et al., 2020b). The transport and accumulation of starch and protein to ear improves wheat quality (Kizilgeci, 2019). This enhanced relocation and accumulation of P from source organs to sink organs supported through Pi transporters, H+-ATPase, phospholipids, and carbon-metabolism gene expressions (Aziz et al., 2014). Therefore, it is important to supplement sufficient P at the vegetative growth stage to safeguard optimal spike and grain development and stress tolerance in wheat.
It is described that P fertilization significantly increased the spike length, spike number, grains per spike, and 1,000-grain weight, which directly influenced the wheat yield (Saqib et al., 2015; Arshad et al., 2016). In a field trial of wheat broadcasted phosphate fertilizer at the rate of 90 kg P2O5 ha−1, substantial increase was noted in plant height, spike length, GNPP, 1000-grain weight, P concentration, P uptake, and GYPP by 12.8, 7.7, 13.7, 22.4, 24.0, 85.5, and 60.6%, respectively, as compared to control treatment with no P application (Shafi et al., 2020). Moreover, increased accumulation of SS was also observed in various reproductive organs; augmented sugar contents improved the pollen activity, which significantly prevented the floret abortion and spike damage under temperature stress (Zhang et al., 2017).
Currently, little research is being conducted on the role of P fertilization in sustaining source–sink balance and plant–nutrient relations for LTS. Although its actual mechanism is not fully explored yet, a comprehensive and systematic approach study is required to unleash the role of P in the alleviation of risks anticipated with LTS.
To strengthen the wheat’s capacity in combating the negative effects of LTS, a number of integrated management approaches are being implemented. It includes modern crop breeding techniques (gene mapping, inducing LTS-tolerant genes, omics, etc.), improved crop husbandry practices (seed enhancements, fertilizer management, adequate sowing techniques, timely sowing irrigation management, etc.), and crop modeling approaches (for optimizing the resources and estimating the risks of temperature variability). In this section, we briefly discussed the above-mentioned crop management strategies with regard to improved P regulation.
Every year, breeders work hard to create cultivars that are better adapted to changing climates. The ongoing research work on wheat LTS tolerance mechanism needs to be combined with modern breeding techniques (i.e., fast breeding, space breeding, and CRISPR-Cas9) for the development of efficient and temperature resilient crop varieties (Hristov et al., 2007; Zhao et al., 2020; Li et al., 2021). For efficient utilization of P, more research is needed into the genetic and molecular mechanisms related to LTS.
To develop a new variety, traditional breeding techniques take more than 10 years, which are not enough to meet the present problems of climatic fluctuations and nutrient deficient soil conditions (Muhammad et al., 2021). The current complex ecological system and abnormal climatic variations posing a severe threat to normal crop growth that restraining the efficiency of existing crop cultivars for longer duration. Therefore, in future, along with opting genotypic marker-assisted and high flux phenotypic selection techniques (Mickelbart et al., 2015; Younis et al., 2020), employing crop simulation models (e.g., CERES-Wheat and DSAT) for predicting the lifespan of particular cultivars in different climatic zones is quite useful (Koç, 2020).
Genome-wide association studies (GWAS) thought to be pragmatic in identifying the genetic factors controlling the phenotypic variability of PUE in wheat (Bin Safdar et al., 2021). In the meantime, GWAS is useful in understanding the genetic basis of LTS tolerance and in developing LTS-resistant wheat cultivars. GWAS-based research reported that PHO2 and zinc finger transcription factors are involved in many functional and regulatory processes in plants, such as P homeostasis and ROS metabolism (Devaiah et al., 2007; De et al., 2019). Later, the identified genes sources subjected to the site-specific by CRISPR-Cas9 analysis for promoting the development of the efficient P utilization and improved LTS-resistant wheat varieties (Doudna and Charpentier, 2014). The response of different wheat genotypes to LTS and P nutrient was significantly varies, and so, the effective combination of LTS-tolerant and P-efficient genotypes is good way to develop LTS-resistant cultivars; then later followed by domestication of newly developed P-efficient and LTS-tolerant wheat cultivars (Wang et al., 2016a). Recently, a new wheat-Thinopyrum intermedium introgression line with tolerance to P deficiency was identified, which provides, which really a new insight into wheat germplasm resources and aids in developing high P-efficient-stress tolerant cultivars (Zhang et al., 2021c).
Only breeding high P-efficient cultivars is not enough to counter low temperature repercussions, but it is also crucial to implement optimal crop husbandry practices. According to the sustainable development goals of the United Nations, P management is a crucial element in determining final grain yield (Griggs et al., 2014; Rafiullah et al., 2020). Therefore, it is needed to pay attention to the adequate fertilizer application, appropriate timing, and suitable method of fertilization. During the vegetative stage of wheat, P absorption rate is maximum and healthy source organs are needed to combat the negative impacts of LTS at sensitive reproductive (jointing and booting) stages; therefore, adequate P supply must be ensured before anthesis (Liu et al., 2020a).
There are many P application methods, including broadcasting, top dressing, fertigation, foliar application, and P-amendments of biochar. The efficiency of each application method varies. Foliar application of potassium dihydrogen phosphate effectively combat the LTS at critical jointing and booting stages and enhanced the final wheat yield (Rafiullah et al., 2020). Drip fertigation of phosphate fertilizer allows nutrients to reach the root zone directly and improve the P concentration in plant tissue and PUE (Mikkelsen, 1989). Biochar is an emerging soil amendment approach; biochar as P-amendment significantly increased the source of soil organophosphorus, improved the soil quality, and enhanced the efficiency of plant nutrient uptake (Manolikaki et al., 2016). Biochar amendments enables the mobilization soil P through altering soil pH and supporting AMF in developing symbiotic relationships with plant roots (Shen et al., 2016). Combined applications of organic and inorganic fertilizers play a similar role in ensuring P availability for sustainable crop production (Yadav et al., 2021).
Conservation agricultural practices are very handy in improving soil health and ensuring bioavailability of essential nutrients in soil (Yang et al., 2020). In South Asia, farmers have already started implementing conservation farming practices, such as reduced tillage and residue retention (Jat and Gerard, 2014). After paddy harvesting, the incorporation of straw residues is an important tillage practice, termed as straw retention. Straw residues are rich in organic carbon, mineral nutrients, and rich biochar amendment for next wheat crop (Arif et al., 2017). Alternatively, inoculation of PSB and mutually beneficial AMF associations effectively improve the bioavailability of phosphate. Further, it promotes active plant growth and minimize the negative implications of stress by establishing a positive relationship with soil mineral nutrients (Khan et al., 2009; Etesami et al., 2021). It is known that PSB plays a vital role in reducing soil pH, solubilizing Pi, and chelating P from iron and aluminum oxides (Khan et al., 2009). And bioavailability of P and soil P-nutrition greatly enhanced by inoculating PSB combined with application of organic manures (Adnan et al., 2017). Further, applying Silicon (Si) fertilizers (Na2SiO3) stimulates the wheat root exudation and changed the soil pH, as a result improved the Pi availability in rhizosphere; subsequently, upon Si-fertilization the upregulating expression of Pi transporters (TaPHT1.1 and TaPHT1.2) significantly increases the P uptake (Kostic et al., 2017).
The organic combination of P management strategies and modern cultivation practices is equally important to treasure the soil resources and maintain plant health under adverse environmental conditions. Enhanced P management does not mean more P fertilizer application but efficient utilization of mineral resources with regard to the potential of agricultural productivity and ecological stability (Alewell et al., 2020).
Low temperature is one of the major abiotic factors that limits the wheat productivity under global climate change. In this review, we summarized the damaging impacts of LTS to wheat source–sink organs with respect to morphological, physiological, and molecular attributes (Tables 2, 3). Recently, under the continuous efforts of scientists around the world, great progress has been made in the research on the damaging mechanism of low temperature in wheat. LTS decreases the active leaf area and photosynthetic capacity, severely inhibits the expression of PS-related genes, resulting in reduced production of assimilates (Gan et al., 2019). LTS induced ABA pathways affect the sucrose metabolism and related gene expressions in spikelets, subsequently, sucrose transportation significantly diminished, thus leading to pollen abortion (Zhang et al., 2019).
As an essential plant nutrient, P can increase soil AP contents and improve the soil–plant nutrient and source–sink relationship, that resulted in enhanced LTS tolerance through reducing oxidative stress and increasing pollen activity. To enhance the PUE and cold tolerance of wheat, the crop husbandry practices, such as top dressing, mulched drip irrigation, deep application, and foliar spraying, should be opted according to different climatic zones (Li et al., 2019; Bindraban et al., 2020; Rafiullah et al., 2020). Further, it is needed to adopt conservation agricultural practices, especially application of P activators that promote the release of phosphate from soil particles (Zhu et al., 2018). Despite the ongoing developments in research field, some aspects still needs to be explored further and requires prospective studies, that is, stress sensing and signaling, molecular studies of the P regulation mechanism, the use of efficient breeding technologies, reducing gap between research and applied sectors through bridging research institutes and farmer communities (Zhang et al., 2021a). Hence, for better understanding of the stress responses, laboratory experiments should be combined with field experiments, and wheat plants should be focused than model plants (Dresselhaus and Huckelhoven, 2018; Hussain et al., 2018). Apart from this, breeding P-efficient and LTS-tolerant cultivars are also a pragmatic approach for future dealings with LTS and nutrient scarce soil conditions. Multidisciplinary cooperation is essential to build a platform for cooperative research concerning macro and micronutrient utilization and confronting abiotic stresses, thus mitigating the looming global P and food crisis.
HX and MAH conceived the concept of the review and prepared an outline of the review. HX, MAH, XC, DS, HF, and GJ compiled the literature and wrote different sections. BL, QN, WY, and ZW aided in designing figures and arranging references. XC and JL provided technical assistance and editing support. All authors contributed to the article and approved the submitted version.
This work was supported by the Natural Science Foundation of Anhui Province (2008085QC122) and Major Science and Technology Projects in Anhui Province (202003b06020021).
The authors declare that the research was conducted in the absence of any commercial or financial relationships that could be construed as a potential conflict of interest.
All claims expressed in this article are solely those of the authors and do not necessarily represent those of their affiliated organizations, or those of the publisher, the editors and the reviewers. Any product that may be evaluated in this article, or claim that may be made by its manufacturer, is not guaranteed or endorsed by the publisher.
The authors would like to thank Zhaoliang Zhang (State Key Laboratory of Tea Plant Biology and Utilization, Anhui Agricultural University) for proofreading and rectifying the manuscript. Moreover, the authors want to dedicate this article to the newly married HX and his wife Ms. Zhen Wu (College of Marxism, Anhui Agricultural University).
Adnan, M., Shah, Z., Fahad, S., Arif, M., Alam, M., Khan, I. A., et al. (2017). Phosphate-solubilizing bacteria nullify the antagonistic effect of soil calcification on bioavailability of phosphorus in alkaline soils. Sci. Rep. 7, 1–13. doi: 10.1038/s41598-017-16537-5
Alewell, C., Ringeval, B., Ballabio, C., Robinson, D. A., Panagos, P., and Borrelli, P. (2020). Global phosphorus shortage will be aggravated by soil erosion. Nat. Commun. 11:4546. doi: 10.1038/s41467-020-18326-7
Ali, Q., and Malik, A. (2021). Genetic response of growth phases for abiotic environmental stress tolerance in cereal crop plants. Genetika. Belgrade 53, 419–456. doi: 10.2298/GENSR2101419A
Ambroise, V., Legay, S., Guerriero, G., Hausman, J. F., Cuypers, A., and Sergeant, K. (2020). The roots of plant frost hardiness and tolerance. Plant Cell Physiol. 61, 3–20. doi: 10.1093/pcp/pcz196
Arif, M., Ilyas, M., Riaz, M., Ali, K., Shah, K., Haq, I. U., et al. (2017). Biochar improves phosphorus use efficiency of organic-inorganic fertilizers, maize-wheat productivity and soil quality in a low fertility alkaline soil. Field Crop Res. 214, 25–37. doi: 10.1016/j.fcr.2017.08.018
Aroca, R., Porcel, R., and Ruiz-Lozano, J. M. (2012). Regulation of root water uptake under abiotic stress conditions. J. Exp. Bot. 63, 43–57. doi: 10.1093/jxb/err266
Arshad, M., Adnan, M., Ahmed, S., Khan, A., Ali, I., Ali, M., et al. (2016). Integrated effect of phosphorus and zinc on wheat crop. Am. Eurasian J. Agric. Environ. Sci. 16, 455–459. doi: 10.5829/idosi.aejaes.2016.16.3.12887
Attarzadeh, M., Balouchi, H., Rajaie, M., Dehnavi, M. M., and Salehi, A. (2020). Improving growth and phenolic compounds of Echinacea purpurea root by integrating biological and chemical resources of phosphorus under water deficit stress. Ind. Crop. Prod. 154:112763. doi: 10.1016/j.indcrop.2020.112763
Aziz, T., Finnegan, P. M., Lambers, H., and Jost, R. (2014). Organ-specific phosphorus-allocation patterns and transcript profiles linked to phosphorus efficiency in two contrasting wheat genotypes. Plant Cell Environ. 37, 943–960. doi: 10.1111/pce.12210
Baek, D., Chun, H. J., Yun, D. J., and Min, C. K. (2017). Cross-talk between phosphate starvation and other environmental stress signaling pathways in plants. Mol. Cells 40, 697–705. doi: 10.14348/molcells.2017.0192
Balliu, A., Zheng, Y. B., Sallaku, G., Fernandez, J. A., Gruda, N. S., and Tuzel, Y. (2021). Environmental and cultivation factors affect the morphology, architecture and performance of root systems in soilless grown plants. Horticulturae 7:243. doi: 10.3390/horticulturae7080243
Bargaz, A., Nassar, R. M. A., Rady, M. M., Gaballah, M. S., Thompson, S. M., Brestic, M., et al. (2016). Improved salinity tolerance by hosphorus fertilizer in two phaseolus vulgaris recombinant inbred lines contrasting in their P-efficiency. J. Agron. Crop Sci. 202, 497–507. doi: 10.1111/jac.12181
Barlow, K. M., Christy, B. P., O’Leary, G. J., Riffkin, P. A., and Nuttall, J. G. (2015). Simulating the impact of extreme heat and frost events on wheat crop production: A review. Field Crop Res. 171, 109–119. doi: 10.1016/j.fcr.2014.11.010
Bin Safdar, L., Umer, M. J., Almas, F., Uddin, S., Safdar, Q. T. A., Blighe, K., et al. (2021). Identification of genetic factors controlling phosphorus utilization efficiency in wheat by genome-wide association study with principal component analysis. Gene 768:145301. doi: 10.1016/j.gene.2020.145301
Bindraban, P. S., Dimkpa, C. O., and Pandey, R. (2020). Exploring phosphorus fertilizers and fertilization strategies for improved human and environmental health. Biol. Fertil. Soils 56, 299–317. doi: 10.1007/s00374-019-01430-2
Bouain, N., Krouk, G., Lacombe, B., and Rouached, H. (2019). Getting to the root of plant mineral nutrition: combinatorial nutrient stresses reveal emergent properties. Trends Plant Sci. 24, 542–552. doi: 10.1016/j.tplants.2019.03.008
Buriro, M., Oad, F. C., Keerio, M. I., Tunio, S., and Oad, S. M. (2011). Wheat seed germination under the influence of temperature regimes. Sarhad J. Agric. 27, 539–543.
Chatterjee, D., Nayak, A. K., Mishra, A., Swain, C. K., Kumar, U., Bhaduri, D., et al. (2021). Effect of long-term organic fertilization in flooded rice soil on phosphorus transformation and phosphate solubilizing microorganisms. J. Soil Sci. Plant Nutr. 21, 1368–1381. doi: 10.1007/s42729-021-00446-8
Chen, J. H., Liu, J. B., Zhang, X. J., Chen, S. Q., Huang, W., Chen, J., et al. (2019a). Unstable little ice age climate revealed by high-resolution proxy records from northwestern China. Clim. Dyn. 53, 1517–1526. doi: 10.1007/s00382-019-04685-5
Chen, X. X., Zhang, W., Liang, X. Y., Liu, Y. M., Xu, S. J., Zhao, Q. Y., et al. (2019b). Physiological and developmental traits associated with the grain yield of winter wheat as affected by phosphorus fertilizer management. Sci. Rep. 9:16580. doi: 10.1038/s41598-019-53000-z
Chinnusamy, V., Zhu, J., and Zhu, J. K. (2007). Cold stress regulation of gene expression in plants. Trends Plant Sci. 12, 444–451. doi: 10.1016/j.tplants.2007.07.002
Cho, H., Bouain, N., Zheng, L., and Rouached, H. (2021). Plant resilience to phosphate limitation: current knowledge and future challenges. Crit. Rev. Biotechnol. 41, 63–71. doi: 10.1080/07388551.2020.1825321
Chong, L., Shi, X. N., and Zhu, Y. F. (2021). Signal integration by cyclin-dependent kinase 8 (CDK8) module and other mediator subunits in biotic and abiotic stress responses. Int. J. Mol. Sci. 22:354. doi: 10.3390/ijms22010354
Cong, W. F., Suriyagoda, L. D. B., and Lambers, H. (2020). Tightening the phosphorus cycle through phosphorus-efficient crop genotypes. Trends Plant Sci. 25, 967–975. doi: 10.1016/j.tplants.2020.04.013
Cordell, D., and Neset, T. S. S. (2014). Phosphorus vulnerability: A qualitative framework for assessing the vulnerability of national and regional food systems to the multidimensional stressors of phosphorus scarcity. Glob. Environ. Change-Human Policy Dimens. 24, 108–122. doi: 10.1016/j.gloenvcha.2013.11.005
Cvetkovic, J., Muller, K., and Baier, M. (2017). The effect of cold priming on the fitness of Arabidopsis thaliana accessions under natural and controlled conditions. Sci. Rep. 7, 1–16. doi: 10.1038/srep44055
De, S., Pablo, C., Carlos, R., Fernando, B., Varela, R. M., Alex, S., et al. (2019). Phosphate acquisition efficiency in wheat is related to root: shoot ratio, strigolactone levels, and PHO2 regulation. J. Exp. Bot. 70, 5631–5642. doi: 10.1093/jxb/erz349
Devaiah, B. N., Nagarajan, V. K., and Raghothama, K. G. (2007). Phosphate homeostasis and root development in Arabidopsis are synchronized by the zinc finger transcription factor ZAT6. Plant Physiol. 145, 147–159. doi: 10.1104/pp.107.101691
Dijkstra, F. A., Carrillo, Y., Aspinwall, M. J., Maier, C., Canarini, A., Tahaei, H., et al. (2016). Water, nitrogen and phosphorus use efficiencies of four tree species in response to variable water and nutrient supply. Plant Soil 406, 187–199. doi: 10.1007/s11104-016-2873-6
Dikilitas, M., Karakas, S., Simsek, E., and Yadav, A. N. (2021). “Microbes from cold deserts and their applications in mitigation of cold stress in plants,” in Microbiomes of Extreme Environments. eds. A. N. Yadav, A. A. Rastegari, and N. Yadav (Boca Raton, Florida: CRC Press), 126–152.
Doudna, J. A., and Charpentier, E. (2014). The new frontier of genome engineering with CRISPR-Cas9. Science 346:3213. doi: 10.1126/science.1258096
Dresselhaus, T., and Huckelhoven, R. (2018). Biotic and abiotic stress responses in crop plants. Agronomy 8:267. doi: 10.3390/agronomy8110267
El Mazlouzi, M., Morel, C., Chesseron, C., Robert, T., and Mollier, A. (2020a). Contribution of external and internal phosphorus sources to grain P loading in durum wheat (Triticum durum L.) grown under contrasting P levels. Front. Plant Sci. 11:870. doi: 10.3389/fpls.2020.00870
El Mazlouzi, M., Morel, C., Robert, T., Yan, B. F., and Mollier, A. (2020b). Phosphorus uptake and partitioning in two durum wheat cultivars with contrasting biomass allocation as affected by different P supply during grain filling. Plant Soil 449, 179–192. doi: 10.1007/s11104-020-04444-0
Engels, C., Kirkby, E., and White, P. (2012). “Mineral nutrition, yield and source-sink relationships,” in Mineral Nutrition of Higher Plants. 3rd Edn. ed. P. Marschner (Netherlands: Elsevier), 85–131.
Equiza, M. (2001). Morphological, anatomical and physiological responses related to differential shoot vs. root growth inhibition at low temperature in spring and winter wheat. Ann. Bot. 87, 67–76. doi: 10.1006/anbo.2000.1301
Erenstein, O., Chamberlin, J., and Sonder, K. (2021). Estimating the global number and distribution of maize and wheat farms. Glob. Food Secur. Agric. Policy 30:100558. doi: 10.1016/j.gfs.2021.100558
Etesami, H., Jeong, B. R., and Glick, B. R. (2021). Contribution of arbuscular mycorrhizal fungi, phosphate–solubilizing bacteria, and silicon to P uptake by plant. Front. Plant Sci. 12:699618. doi: 10.3389/fpls.2021.699618
Fleitas, M. C., Mondal, S., Gerard, G. S., Hernandez-Espinosa, N., Prakash Singh, R., Crossa, J., et al. (2020). Identification of CIMMYT spring bread wheat germplasm maintaining superior grain yield and quality under heat-stress. J. Cereal Sci. 93:102981. doi: 10.1016/j.jcs.2020.102981
Frederiks, T. M., Christopher, J., and Borrell, A., (2004). “Investigation of post head-emergence frost resistance in several CIMMYT synthetic and Queensland wheats.” in 4th International Crop Science Congress. eds. T. N. Fischer, J. Angus, L. McIntyre, M. Robertson, A. Borrell, and D. Lloyd September, 2004; Brisbane, Australia (Australia: Regional Institute Ltd.).
Frederiks, T. M., Christopher, J. T., and Borrell, A. K., (2008). “Low temperature adaption of wheat post head-emergence in northern Australia.” in The 11th International Wheat Genetics Symposium proceedings. eds. E. R. R. Appels, E. Lagudah, P. Langridge, M. Mackay, L. McIntyre, and P. Sharp Brisbane, Australia (Australia: Sydney University Press), August 24–29, 2008.
Frederiks, T. M., Christopher, J. T., Harvey, G. L., Sutherland, M. W., and Borrell, A. K. (2012). Current and emerging screening methods to identify post-head-emergence frost adaptation in wheat and barley. J. Exp. Bot. 63, 5405–5416. doi: 10.1093/jxb/ers215
Fuller, M. P., Fuller, A. M., Kaniouras, S., Christophers, J., and Fredericks, T. (2007). The freezing characteristics of wheat at ear emergence. Eur. J. Agron. 26, 435–441. doi: 10.1016/j.eja.2007.01.001
Gahoonia, T. S., Nielsen, N. E., and Lyshede, O. B. (1999). Phosphorus (P) acquisition of cereal cultivars in the field at three levels of P fertilization. Plant Soil 211, 269–281. doi: 10.1023/A:1004742032367
Gan, P., Liu, F., Li, R. B., Wang, S. K., and Luo, J. J. (2019). Chloroplasts-beyond energy capture and carbon fixation: tuning of photosynthesis in response to chilling stress. Int. J. Mol. Sci. 20:5046. doi: 10.3390/ijms20205046
Gerz, M., Guillermo Bueno, C., Ozinga, W. A., Zobel, M., Moora, M., and van der Heijden, M. (2018). Niche differentiation and expansion of plant species are associated with mycorrhizal symbiosis. J. Ecol. 106, 254–264. doi: 10.1111/1365-2745.12873
Gong, Z. Z., Xiong, L. M., Shi, H. Z., Yang, S. H., Herrera-Estrella, L. R., Xu, G. H., et al. (2020). Plant abiotic stress response and nutrient use efficiency. Sci. China Life Sci. 63, 635–674. doi: 10.1007/s11427-020-1683-x
Griggs, D., Smith, M. S., Rockstrom, J., Ohman, M. C., Gaffney, O., Glaser, G., et al. (2014). An integrated framework for sustainable development goals. Ecol. Soc. 19:49. doi: 10.5751/es-07082-190449
Gross, A., Tiwari, S., Shtein, I., and Erel, R. (2021). Direct foliar uptake of phosphorus from desert dust. New Phytol. 230, 2213–2225. doi: 10.1111/nph.17344
Gulick, P. J., Drouin, S., Yu, Z., Danyluk, J., Poisson, G., Monroy, A. F., et al. (2005). Transcriptome comparison of winter and spring wheat responding to low temperature. Genome 48, 913–923. doi: 10.1139/g05-039
Guo, C., Zhao, X., Liu, X., Zhang, L., Gu, J., Li, X., et al. (2013). Function of wheat phosphate transporter gene TaPHT2; 1 in pi translocation and plant growth regulation under replete and limited pi supply conditions. Planta 237, 1163–1178. doi: 10.1007/s00425-012-1836-2
Halouzka, R., Zeljkovic, S. C., Klejdus, B., and Tarkowski, P. (2020). Analytical methods in strigolactone research. Plant Methods 16, 1–13. doi: 10.1186/s13007-020-00616-2
Hammond, J. P., and White, P. J. (2007). Sucrose transport in the phloem: integrating root responses to phosphorus starvation. J. Exp. Bot. 59, 93–109. doi: 10.1093/jxb/erm221
Han, Q., Kang, G., and Guo, T. (2013). Proteomic analysis of spring freeze-stress responsive proteins in leaves of bread wheat (Triticum aestivum L.). Plant Physiol. Biochem. 63, 236–244. doi: 10.1016/j.plaphy.2012.12.002
Hickey, L. T., Hafeez, A. N., Robinson, H., Jackson, S. A., Leal-Bertioli, S. C. M., Tester, M., et al. (2019). Breeding crops to feed 10 billion. Nat. Biotechnol. 37, 744–754. doi: 10.1038/s41587-019-0152-9
Holman, J. D., Schlegel, A. J., Thompson, C. R., and Lingenfelser, J. E. (2011). Influence of precipitation, temperature, and 56 years on winter wheat yields in western Kansas. Crop Manag. 10, 1–10. doi: 10.1094/cm-2011-1229-01-rs
Hristov, N., Mladenov, N., and Kondić-Šipka, A. (2007). Breeding aspects of low temperature tolerance in wheat. Genetika 39, 375–386. doi: 10.2298/GENSR0703375H
Hsu, C. H., and Hsu, Y. T. (2019). Biochemical responses of rice roots to cold stress. Bot. Stud. 60, 1–12. doi: 10.1186/s40529-019-0262-1
Huang, H., Ullah, F., Zhou, D., Yi, M., and Zhao, Y. (2019). Mechanisms of ROS regulation of plant development and stress responses. Front. Plant Sci. 10:800. doi: 10.3389/fpls.2019.00800
Hussain, H. A., Hussain, S., Khaliq, A., Ashraf, U., Anjum, S. A., Men, S., et al. (2018). Chilling and drought stresses in crop plants: implications, cross talk, and potential management opportunities. Front. Plant Sci. 9:393. doi: 10.3389/fpls.2018.00393
Jamil, M., Kountche, B. A., Wang, J. Y., Haider, I., Jia, K.-P., Takahashi, I., et al. (2020). A new series of carlactonoic acid based strigolactone analogs for fundamental and applied research. Front. Plant Sci. 11:434. doi: 10.3389/fpls.2020.00434
Jat, M. L., and Gerard, B. (2014). Nutrient management and use efficiency in wheat systems of South Asia. Adv. Agron. 125, 171–259. doi: 10.1016/B978-0-12-800137-0.00005-4
Ji, H., Xiao, L., Xia, Y., Song, H., Liu, B., Tang, L., et al. (2017). Effects of jointing and booting low temperature stresses on grain yield and yield components in wheat. Agric. For. Meteorol. 243, 33–42. doi: 10.1016/j.agrformet.2017.04.016
Jiang, B. C., Shi, Y. T., Peng, Y., Jia, Y. X., Yan, Y., Dong, X. J., et al. (2020). Cold-induced CBF-PIF3 interaction enhances freezing tolerance by stabilizing the phyB thermosensor in Arabidopsis. Mol. Plant 13, 894–906. doi: 10.1016/j.molp.2020.04.006
Jin, J., Lauricella, D., Armstrong, R., Sale, P., and Tang, C. (2015). Phosphorus application and elevated CO2 enhance drought tolerance in field pea grown in a phosphorus-deficient vertisol. Ann. Bot. 116, 975–985. doi: 10.1093/aob/mcu209
Karimzadeh, G. (2000). Low temperature-induced accumulation of protein is sustained both in root meristems and in callus in winter wheat but not in spring wheat. Ann. Bot. 85, 769–777. doi: 10.1006/anbo.2000.1137
Karimzadeh, J., Alikhani, H. A., Etesami, H., and Pourbabaei, A. A. (2021). Improved phosphorus uptake by wheat plant (Triticum aestivum L.) with rhizosphere fluorescent pseudomonads strains under water-deficit stress. J. Plant Growth Regul. 40, 162–178. doi: 10.1007/s00344-020-10087-3
Keramidas, M. E., Kolegard, R., and Eiken, O. (2020). Hypoxia gradually augments metabolic and thermoperceptual responsiveness to repeated whole-body cold stress in humans. Exp. Physiol. 105, 2123–2140. doi: 10.1113/EP089070
Khan, A. A., Jilani, G., Akhtar, M. S., Naqvi, S., and Rasheed, M. (2009). Phosphorus solubilizing bacteria: occurrence, mechanisms and their role in crop production. J. Agri. Biol. Sci. 1, 48–58.
Kim, Y., Chung, Y. S., Lee, E., Tripathi, P., Heo, S., and Kim, K. H. (2020). Root response to drought stress in rice (Oryza sativa L.). Int. J. Mol. Sci. 21:1513. doi: 10.3390/ijms21041513
Kizilgeci, F. (2019). Physiological, agronomical and quality response of bread wheat to phosphorus application under dryland condtion. Appl. Ecol. Environ. Res. 17, 1979–1987. doi: 10.15666/aeer/1702_19791987
Koç, E. (2020). “Assessing climate change impacts on wheat production in Turkey and various adaptation strategies,” in Climate Change and Food Security with Emphasis on Wheat. eds. M. Ozturk and A. Gul (New York: Elsevier), 43–54.
Kodra, E., Steinhaeuser, K., and Ganguly, A. R. (2011). Persisting cold extremes under 21st-century warming scenarios. Geophys. Res. Lett. 38:L08705. doi: 10.1029/2011gl047103
Koevoets, I. T., Venema, J. H., Elzenga, J. T. M., and Testerink, C. (2016). Roots withstanding their environment: exploiting root system architecture responses to abiotic stress to improve crop tolerance. Front. Plant Sci. 7:1335. doi: 10.3389/fpls.2016.01335
Koo, B. C., Bushman, B. S., and Mott, I. W. (2008). Transcripts associated with non-acclimated freezing response in two barley cultivars. Plant Genome 1, 21–32. doi: 10.3835/plantgenome2008.06.0344
Kostic, L., Nikolic, N., Bosnic, D., Samardzic, J., and Nikolic, M. (2017). Silicon increases phosphorus (P) uptake by wheat under low P acid soil conditions. Plant Soil 419, 447–455. doi: 10.1007/s11104-017-3364-0
Kul, R., Ekinci, M., Turan, M., Ors, S., and Yildirim, E. (2020). “How abiotic stress conditions affects plant roots,” in Plant Roots. ed. E. Yildirim (London: Intech Open), 6–10.
Latef, A. A. H. A., and He, C. (2011). Arbuscular mycorrhizal influence on growth, photosynthetic pigments, osmotic adjustment and oxidative stress in tomato plants subjected to low temperature stress. Acta Physiol. Plant. 33, 1217–1225. doi: 10.1007/s11738-010-0650-3
Li, X., Cai, J., Liu, F., Dai, T., Cao, W., and Jiang, D. (2014). Cold priming drives the sub-cellular antioxidant systems to protect photosynthetic electron transport against subsequent low temperature stress in winter wheat. Plant Physiol. Biochem. 82, 34–43. doi: 10.1016/j.plaphy.2014.05.005
Li, Y. B., Hou, R. X., and Tao, F. L. (2021). Wheat morpho-physiological traits and radiation use efficiency under interactive effects of warming and tillage management. Plant Cell Environ. 44, 2386–2401. doi: 10.1111/pce.13933
Li, X., Pu, H., Liu, F., Zhou, Q., Cai, J., Dai, T., et al. (2015). Winter wheat photosynthesis and grain yield responses to spring freeze. Agron. J. 107, 1002–1010. doi: 10.2134/agronj14.0460
Li, Q., Xu, C., Yin, C. X., Kong, L. L., Qin, Y. B., Hou, Y. P., et al. (2019). Evaluation of fertigation technique for phosphorus application of maize in the semi-arid region of Northeast China. Plant Soil Environ. 65, 401–407. doi: 10.17221/287/2019-PSE
Linkohr, B. I., Williamson, L. C., Fitter, A. H., and Leyser, H. M. O. (2002). Nitrate and phosphate availability and distribution have different effects on root system architecture of Arabidopsis. Plant J. 29, 751–760. doi: 10.1046/j.1365-313X.2002.01251.x
Liu, J., Cheng, X., Liu, P., and Sun, J. (2017). miR156-targeted SBP-box transcription factors interact with DWARF53 to regulate TEOSINTE BRANCHED1 and BARREN STALK1 expression in bread wheat. Plant Physiol. 174, 1931–1948. doi: 10.1104/pp.17.00445
Liu, L., Ji, H., An, J., Shi, K., Ma, J., Liu, B., et al. (2019a). Response of biomass accumulation in wheat to low-temperature stress at jointing and booting stages. Environ. Exp. Bot. 157, 46–57. doi: 10.1016/j.envexpbot.2018.09.026
Liu, L. L., Song, H., Shi, K. J., Liu, B., Zhang, Y., Tang, L., et al. (2019b). Response of wheat grain quality to low temperature during jointing and booting stages-On the importance of considering canopy temperature. Agric. For. Meteorol. 278:107658. doi: 10.1016/j.agrformet.2019.107658
Liu, L. L., Xia, Y. M., Liu, B., Chang, C. Y., Xiao, L. J., Shen, J., et al. (2020a). Individual and combined effects of jointing and booting low-temperature stress on wheat yield. Eur. J. Agron. 113:125989. doi: 10.1016/j.eja.2019.125989
Liu, T., Ye, X. L., Li, M., Li, J. M., Qi, H. Y., and Hu, X. H. (2020b). H2O2 and NO are involved in trehalose-regulated oxidative stress tolerance in cold-stressed tomato plants. Environ. Exp. Bot. 171:103961. doi: 10.1016/j.envexpbot.2019.103961
Livingston, D. P., Hincha, D. K., and Heyer, A. G. (2009). Fructan and its relationship to abiotic stress tolerance in plants. Cell. Mol. Life Sci. 66, 2007–2023. doi: 10.1007/s00018-009-0002-x
Lynch, J. P. (2011). Root phenes for enhanced soil exploration and phosphorus acquisition: tools for future crops. Plant Physiol. 156, 1041–1049. doi: 10.1104/pp.111.175414
Ma, N., Hu, C., Wan, L., Hu, Q., and Xiong, J. L. (2017). Strigolactones improve plant growth, photosynthesis, and alleviate oxidative stress under salinity in rapeseed (Brassica napus L.) by regulating gene expression. Front. Plant Sci. 8:1671. doi: 10.3389/fpls.2017.01671
Manolikaki, I. I., Mangolis, A., and Diamadopoulos, E. (2016). The impact of biochars prepared from agricultural residues on phosphorus release and availability in two fertile soils. J. Environ. Manag. 181, 536–543. doi: 10.1016/j.jenvman.2016.07.012
Markovskaya, E. F., Sherudilo, E. G., Galibina, N. A., and Sysoeva, M. I. (2010). The role of carbohydrates in the responses of chilling-sensitive plants to short- and long-term low-temperature treatments. Russ. J. Plant Physiol. 57, 641–647. doi: 10.1134/S1021443710050067
Mattila, H., Mishra, K. B., Kuusisto, I., Mishra, A., Novotna, K., Sebela, D., et al. (2020). Effects of low temperature on photoinhibition and singlet oxygen production in four natural accessions of Arabidopsis. Planta 252, 1–17. doi: 10.1007/s00425-020-03423-0
Mickelbart, M. V., Hasegawa, P. M., and Bailey-Serres, J. (2015). Genetic mechanisms of abiotic stress tolerance that translate to crop yield stability. Nat. Rev. Genet. 16, 237–251. doi: 10.1038/nrg3901
Mikkelsen, R. L. (1989). Phosphorus fertilization through drip irrigation. J. Prod. Agri. 2, 279–286. doi: 10.2134/jpa1989.0279
Muhammad, A. H., Chen, X., Muhammad, F., Noor, M., Zhang, Y., Xu, H., et al. (2021). Cold stress in wheat: plant acclimation responses and management strategies. Front. Plant Sci. 12:676884. doi: 10.3389/fpls.2021.676884
Nagel, K. A., Kastenholz, B., Jahnke, S., Dusschoten, D. V., Aach, T., Mühlich, M., et al. (2009). Temperature responses of roots: impact on growth, root system architecture and implications for phenotyping. Funct. Plant Biol. 36, 947–959. doi: 10.1071/FP09184
Nedelciu, C. E., Ragnarsdottir, K. V., Schlyter, P., and Stjernquist, I. (2020). Global phosphorus supply chain dynamics: assessing regional impact to 2050. Glob. Food Secur. Agric. Policy 26:100426. doi: 10.1016/j.gfs.2020.100426
Nevyl, S. F., and Battaglia, M. E. (2021). Developmental plasticity in Arabidopsis thaliana under combined cold and water deficit stresses during flowering stage. Planta 253, 1–3. doi: 10.1007/s00425-021-03575-7
Nezhadahmadi, A., Prodhan, Z. H., and Faruq, G. (2013). Drought tolerance in wheat. Sci. World J. 2013:610721. doi: 10.1155/2013/610721
Nie, Z., Li, S., Hu, C., Sun, X., Tan, Q., and Liu, H. (2015). Effects of molybdenum and phosphorus fertilizers on cold resistance in winter wheat. J. Plant Nutr. 38, 808–820. doi: 10.1080/01904167.2014.939289
Noor, I., Sohail, H., Hasanuzzaman, M., Hussain, S., Li, G., and Liu, J. (2021). Phosphorus confers tolerance against manganese toxicity in Prunus persica by reducing oxidative stress and improving chloroplast ultrastructure. Chemosphere :132999. doi: 10.1016/j.chemosphere.2021.132999 [Epub Ahead of Print]
Oliver, S. N., Dennis, E. S., and Dolferus, R. (2007). ABA regulates apoplastic sugar transport and is a potential signal for cold-induced pollen sterility in rice. Plant Cell Physiol. 48, 1319–1330. doi: 10.1093/pcp/pcm100
Oliver, S. N., Dongen, J., Alfred, S. C., Mamun, E. A., and Dolferus, R. (2010). Cold-induced repression of the rice anther-specific cell wall invertase gene OSINV4 is correlated with sucrose accumulation and pollen sterility. Plant Cell Environ. 28, 1534–1551. doi: 10.1111/j.1365-3040.2005.01390.x
Oliverio, A. M., Bissett, A., McGuire, K., Saltonstall, K., Turner, B. L., and Fierer, N. (2020). The role of phosphorus limitation in shaping soil bacterial communities and their metabolic capabilities. MBio 11:e01718-20. doi: 10.1128/mBio.01718-20
Osman, T., Psyche, S. S., Kamal, M. R., Tamanna, F., Haque, F., and Rahman, R. M., (2016). “Predicting early crop production by analysing prior environment factors.” in International Conference on Advances in Information and Communication Technology, December 12, 2016.
Péret, B., Clément, M., Nussaume, L., and Desnos, T. (2011). Root developmental adaptation to phosphate starvation: better safe than sorry. Trends Plant Sci. 16, 442–450. doi: 10.1016/j.tplants.2011.05.006
Qin, Y., Wang, M., Tian, Y., He, W., Lu, H., and Xia, G. (2012). Over-expression of TaMYB33 encoding a novel wheat MYB transcription factor increases salt and drought tolerance in Arabidopsis. Mol. Biol. Rep. 39, 7183–7192. doi: 10.1007/s11033-012-1550-y
Rafiullah, K. M. J., Muhammad, D., Fahad, S., and Siddiqui, M. H. (2020). Phosphorus nutrient management through synchronization of application methods and rates in wheat and maize crops. Plan. Theory 9:1389. doi: 10.3390/plants9101389
Raimondo, M., Nazzaro, C., Marotta, G., and Caracciolo, F. (2020). Land degradation and climate change: global impact on wheat yields. Land Degrad. Dev. 32, 387–398. doi: 10.1002/ldr.3699
Roman-Figueroa, C., Bravo, L., Paneque, M., Navia, R., and Cea, M. (2021). Chemical products for crop protection against freezing stress: A review. J. Agron. Crop Sci. 207, 391–403. doi: 10.1111/jac.12489
Ruelland, E., and Zachowski, A. (2010). How plants sense temperature. Environ. Exp. Bot. 69, 225–232. doi: 10.1016/j.envexpbot.2010.05.011
Saleem, M., Fariduddin, Q., and Janda, T. (2021). Multifaceted role of salicylic acid in combating cold stress in plants: A review. J. Plant Growth Regul. 40, 464–485. doi: 10.1007/s00344-020-10152-x
Sanghera, G. S., Wani, S. H., Hussain, W., and Singh, N. B. (2011). Engineering cold stress tolerance in crop plants. Curr. Genomics 12, 30–43. doi: 10.2174/138920211794520178
Saqib, B., Shazma, A., Bashir, A., Qamar, S., Wajid, K., and Muhamad, I. (2015). Response of wheat crop to phosphorus levels and application methods. J. Environ. Earth Sci. 5, 151–155.
Savitch, L. V., Harney, T., and Huner, N. (2010). Sucrose metabolism in spring and winter wheat in response to high irradiance, cold stress and cold acclimation. Physiol. Plant. 108, 270–278. doi: 10.1034/j.1399-3054.2000.108003270.x
Sawant, N., Singh, H., and Appukuttan, D. (2021). Overview of the cellular stress responses involved in fatty acid overproduction in E. coli. Mol. Biotechnol. 18, 1–15. doi: 10.1007/s12033-021-00426-4
Shafi, M. I., Adnan, M., Fahad, S., Wahid, F., Khan, A., Yue, Z., et al. (2020). Application of single superphosphate with humic acid improves the growth, yield and phosphorus uptake of wheat (Triticum aestivum L.) in calcareous soil. Agronomy 10:1224. doi: 10.3390/agronomy10091224
Sharma, P., Jha, A. B., Dubey, R. S., and Pessarakli, M. (2012). Reactive oxygen species, oxidative damage, and antioxidative defense mechanism in plants under stressful conditions. J. Botany 2012, 1–26. doi: 10.1155/2012/217037
Sharma, A., Kumar, V., Shahzad, B., Ramakrishnan, M., Singh Sidhu, G. P., Bali, A. S., et al. (2019). Photosynthetic response of plants under different abiotic stresses: A review. J. Plant Growth Regul. 39, 509–531. doi: 10.1007/s00344-019-10018-x
Shen, Q., Hedley, M., Camps Arbestain, M., and Kirschbaum, M. (2016). Can biochar increase the bioavailability of phosphorus? J. Soil Sci. Plant Nutr. 16, 268–286. doi: 10.4067/S0718-95162016005000022
Shen, J., Yuan, L., Zhang, J., Li, H., Bai, Z., Chen, X., et al. (2011). Phosphorus dynamics: From soil to plant. Plant Physiol. 156, 997–1005. doi: 10.1104/pp.111.175232
Shibasaki, K., Uemura, M., Tsurumi, S., and Rahman, A. (2009). Auxin response in Arabidopsis under cold stress: underlying molecular mechanisms. Plant Cell 21, 3823–3838. doi: 10.1105/tpc.109.069906
Shibli, R. A., Sawwan, J., Swaidat, I., and Tahat, M. (2006). Increased phosphorus mitigates the adverse effects of salinity in tissue culture. Commun. Soil Sci. Plant Anal. 32, 429–440. doi: 10.1081/css-100103019
Shirmohammadi, E., Alikhani, H. A., Pourbabaei, A. A., and Etesami, H. (2020). Improved phosphorus (P) uptake and yield of rainfed wheat fed with P fertilizer by drought-tolerant phosphate-solubilizing fluorescent pseudomonads strains: a field study in drylands. J. Soil Sci. Plant Nutr. 20, 2195–2211. doi: 10.1007/s42729-020-00287-x
Slafer, G. A., Abeledo, L. G., Miralles, D. J., Gonzalez, F. G., and Whitechurch, E. M. (2001). Photoperiod sensitivity during stem elongation as an avenue to raise potential yield in wheat. Euphytica 119, 191–197. doi: 10.1023/A:1017535632171
Soares, J. C., Santos, C. S., Carvalho, S. M. P., Pintado, M. M., and Vasconcelos, M. W. (2019). Preserving the nutritional quality of crop plants under a changing climate: importance and strategies. Plant Soil 443, 1–26. doi: 10.1007/s11104-019-04229-0
Soetan, K. O., Olaiya, C. O., and Oyewole, O. E. (2009). The importance of mineral elements for humans, domestic animals and plants: A review. Afr. J. Food Sci. 4, 200–222. doi: 10.5897/AJFS.9000287
Subedi, S., Ghimire, Y. N., Adhikari, S. P., Devkota, D., Shrestha, J., Poudel, H. K., et al. (2019). Adoption of certain improved varieties of wheat (Triticum aestivum L.) in seven different provinces of Nepal. Archives Agri. Environ. Sci. 4, 404–409. doi: 10.26832/24566632.2019.040406
Sun, X. C., Hu, C. X., Tan, Q. L., Liu, J. S., and Liu, H. G. (2009). Effects of molybdenum on expression of cold-responsive genes in abscisic acid (ABA)-dependent and ABA-independent pathways in winter wheat under low-temperature stress. Ann. Bot. 104, 345–356. doi: 10.1093/aob/mcp133
Tabassum, B., Khan, A., Tariq, M., Ramzan, M., Khan, M. S. I., Shahid, N., et al. (2017). Bottlenecks in commercialisation and future prospects of PGPR. Appl. Soil Ecol. 121, 102–117. doi: 10.1016/j.apsoil.2017.09.030
Thakur, P., Kumar, S., Malik, J. A., Berger, J. D., and Nayyar, H. (2010). Cold stress effects on reproductive development in grain crops: An overview. Environ. Exp. Bot. 67, 429–443. doi: 10.1016/j.envexpbot.2009.09.004
Valluru, R., Link, J., and Claupein, W. (2012). Consequences of early chilling stress in two Triticum species: plastic responses and adaptive significance. Plant Biol. 14, 641–651. doi: 10.1111/j.1438-8677.2011.00540.x
Veneklaas, E. J., Lambers, H., Bragg, J., Finnegan, P. M., Lovelock, C. E., Plaxton, W. C., et al. (2012). Opportunities for improving phosphorus-use efficiency in crop plants. New Phytol. 195, 306–320. doi: 10.1111/j.1469-8137.2012.04190.x
Venzhik, Y. V., Titov, A. F., Talanova, V. V., Frolova, S. A., Talanov, A. V., and Nazarkina, Y. A. (2011). Influence of lowered temperature on the resistance and functional activity of the photosynthetic apparatus of wheat plants. Biol. Bull. 38, 132–137. doi: 10.1134/S1062359011020142
Wang, H. F., Huo, Z. G., Zhou, G. S., Liao, Q. H., Feng, H. K., and Wu, L. (2016b). Estimating leaf SPAD values of freeze-damaged winter wheat using continuous wavelet analysis. Plant Physiol. Biochem. 98, 39–45. doi: 10.1016/j.plaphy.2015.10.032
Wang, F., Rose, T., Jeong, K., Kretzschmar, T., and Wissuwa, M. (2016a). The knowns and unknowns of phosphorus loading into grains, and implications for phosphorus efficiency in cropping systems. J. Exp. Bot. 67, 1221–1229. doi: 10.1093/jxb/erv517
Wang, S. G., Wang, Z. L., Wang, P., Wang, H. W., Li, F., Huang, W., et al. (2011). Evaluation of wheat freezing resistance based on the responses of the physiological indices to low temperature stress. Acta Ecol. Sin. 31, 1064–1072. doi: 10.1007/s00220-011-1336-4
Waraich, E. A., Ahmad, R., Saifullah, A., and Ehsanullah, M. Y. (2011). Role of mineral nutrition in alleviation of drought stress in plants. Aust. J. Crop. Sci. 5, 764–777. doi: 10.3316/informit.282340708899391
Whaley, J. M., Kirby, E. J. M., Spink, J. H., Foulkes, M. J., and Sparkes, D. L. (2004). Frost damage to winter wheat in the UK: the effect of plant population density. Eur. J. Agron. 21, 105–115. doi: 10.1016/S1161-0301(03)00090-X
Wojtowicz, A., Oniszczuk, A., Kasprzak, K., Olech, M., Mitrus, M., and Oniszczuk, T. (2020). Chemical composition and selected quality characteristics of new types of precooked wheat and spelt pasta products. Food Chem. 309:125673. doi: 10.1016/j.foodchem.2019.125673
Xu, J., Li, Y., Sun, J., Du, L., Zhang, Y., Yu, Q., et al. (2013). Comparative physiological and proteomic response to abrupt low temperature stress between two winter wheat cultivars differing in low temperature tolerance. Plant Biol. 15, 292–303. doi: 10.1111/j.1438-8677.2012.00639.x
Xue, M., Guo, T., Ren, M., Wang, Z., Tang, K., Zhang, W., et al. (2019). Constitutive expression of chloroplast glycerol-3-phosphate acyltransferase from Ammopiptanthus mongolicus enhances unsaturation of chloroplast lipids and tolerance to chilling, freezing and oxidative stress in transgenic Arabidopsis. Plant Physiol. Biochem. 143, 375–387. doi: 10.1016/j.plaphy.2019.07.019
Yadav, G. S., Babu, S., Das, A., Datta, M., Mohapatra, K. P., Singh, R., et al. (2021). Productivity, soil health, and carbon management index of Indian Himalayan intensified maize-based cropping systems under live mulch based conservation tillage practices. Field Crop Res. 264:108080. doi: 10.1016/j.fcr.2021.108080
Yamori, W., Noguchi, K., Hikosaka, K., and Terashima, I. (2009). Cold-tolerant crop species have greater temperature homeostasis of leaf respiration and photosynthesis than cold-sensitive species. Plant Cell Physiol. 50, 203–215. doi: 10.1093/pcp/pcn189
Yan, J., Jing, W., Tissue, D., Holaday, A. S., and Hong, Z. (2003). Photosynthesis and seed production under water-deficit conditions in transgenic tobacco plants that overexpress an Arabidopsis ascorbate peroxidase gene. Crop Sci. 43, 1477–1483. doi: 10.2135/cropsci2003.1477
Yang, W., Liu, X. D., Chi, X. J., Wu, C. A., Li, Y. Z., Song, L. L., et al. (2011). Dwarf apple MbDREB1 enhances plant tolerance to low temperature, drought, and salt stress via both ABA-dependent and ABA-independent pathways. Planta 233, 219–229. doi: 10.1007/s00425-010-1279-6
Yang, T., Siddique, K. H. M., and Liu, K. (2020). Cropping systems in agriculture and their impact on soil health-A review. Glob. Ecol. Conserv. 23:e01118. doi: 10.1016/j.gecco.2020.e01118
Yang, J. C., and Zhang, J. H. (2006). Grain filling of cereals under soil drying. New Phytol. 169, 223–236. doi: 10.1111/j.1469-8137.2005.01597.x
Younis, A., Ramzan, F., Ramzan, Y., Zulfiqar, F., Ahsan, M., and Lim, K. B. (2020). Molecular markers improve abiotic stress tolerance in crops: A review. Plants-Basel 9:1374. doi: 10.3390/plants9101374
Yu, X., Jiang, Y., Yao, H., Ran, L., Zang, Y., and Xiong, F. (2021). Cytological and molecular characteristics of delayed spike development in wheat under low temperature in early spring. Crop J. doi: 10.1016/j.cj.2021.08.008
Zangani, E., Afsahi, K., Shekari, F., Mac Sweeney, E., and Mastinu, A. (2021). Nitrogen and phosphorus addition to soil improves seed yield, foliar stomatal conductance, and the photosynthetic response of rapeseed (Brassica napus L.). Agriculture 11:483. doi: 10.3390/agriculture11060483
Zhang, X., Cui, C. H., Bao, Y. G., Wang, H. G., and Li, X. F. (2021c). Molecular cytogenetic characterization of a novel wheat-Thinopyrum intermedium introgression line tolerant to phosphorus deficiency. Crop J. 9, 816–822. doi: 10.1016/j.cj.2020.08.014
Zhang, J. Y., Ding, J. P., Ibrahim, M., Jiao, X. C., Song, X. M., Bai, P., et al. (2021b). Effects of the interaction between vapor-pressure deficit and potassium on the photosynthesis system of tomato seedlings under low temperature. Sci. Hortic. 283:110089. doi: 10.1016/j.scienta.2021.110089
Zhang, C. X., Feng, B. H., Chen, T. T., Zhang, X. F., Tao, L. X., and Fu, G. F. (2017). Sugars, antioxidant enzymes and IAA mediate salicylic acid to prevent rice spikelet degeneration caused by heat stress. Plant Growth Regul. 83, 313–323. doi: 10.1007/s10725-017-0296-x
Zhang, Z. B., Hu, M. H., Xu, W. W., Wang, Y., Huang, K., Zhang, C., et al. (2021d). Understanding the molecular mechanism of anther development under abiotic stresses. Plant Mol. Biol. 105, 1–10. doi: 10.1007/s11103-020-01074-z
Zhang, H., Mao, X., Jing, R., Chang, X., and Xie, H. (2011). Characterization of a common wheat (Triticum aestivum L.) TaSnRK2.7 gene involved in abiotic stress responses. J. Exp. Bot. 62, 975–988. doi: 10.1093/jxb/erq328
Zhang, W., Wang, J., Huang, Z., Mi, L., Xu, K., Wu, J., et al. (2019). Effects of low temperature at booting stage on sucrose metabolism and endogenous hormone contents in winter wheat spikelet. Front. Plant Sci. 10:498. doi: 10.3389/fpls.2019.00498
Zhang, Z. W., Wu, P., Zhang, W. B., Yang, Z. F., Liu, H. Y., Ahammed, G. J., et al. (2020). Calcium is involved in exogenous NO-induced enhancement of photosynthesis in cucumber (Cucumis sativus L.) seedlings under low temperature. Sci. Hortic. 261:108953. doi: 10.1016/j.scienta.2019.108953
Zhang, H. M., Zhu, J. H., Gong, Z. Z., and Zhu, J. K. (2021a). Abiotic stress responses in plants. Nat. Rev. Genet. 23, 1–16. doi: 10.1038/s41576-021-00413-0
Zhao, Y., Li, J. H., Zhao, R. L., Xu, K., Xiao, Y. R., Zhang, S. H., et al. (2020). Genome-wide association study reveals the genetic basis of cold tolerance in wheat. Mol. Breed. 40, 1–13. doi: 10.1007/s11032-020-01115-x
Zhao, L., Zhang, H., Zhang, B., Bai, X., and Zhou, C. (2012). Physiological and molecular changes of detached wheat leaves in responding to various treatments. J. Integr. Plant Biol. 54, 567–576. doi: 10.1111/j.1744-7909.2012.01139.x
Zhou, Y., Sommer, M. L., and Hochholdinger, F. (2021). Cold response and tolerance in cereal roots. J. Exp. Bot. 72, 7474–7481. doi: 10.1093/jxb/erab334
Zhu, J., Li, M., and Whelan, M. (2018). Phosphorus activators contribute to legacy phosphorus availability in agricultural soils: A review. Sci. Total Environ. 612, 522–537. doi: 10.1016/j.scitotenv.2017.08.095
Zhu, X. C., Song, F. B., and Xu, H. W. (2010). Arbuscular mycorrhizae improves low temperature stress in maize via alterations in host water status and photosynthesis. Plant Soil 331, 129–137. doi: 10.1007/s11104-009-0239-z
Zhu, J., Zhang, K. X., Wang, W. S., Gong, W., Liu, W. C., Chen, H. G., et al. (2015). Low temperature inhibits root growth by reducing auxin accumulation via ARR1/12. Plant Cell Physiol. 56, 727–736. doi: 10.1093/pcp/pcu217
Keywords: wheat, low temperature stress, source–sink damage, phosphorus, mitigation strategies
Citation: Xu H, Hassan MA, Sun D, Wu Z, Jiang G, Liu B, Ni Q, Yang W, Fang H, Li J and Chen X (2022) Effects of Low Temperature Stress on Source–Sink Organs in Wheat and Phosphorus Mitigation Strategies. Front. Plant Sci. 13:807844. doi: 10.3389/fpls.2022.807844
Received: 02 November 2021; Accepted: 11 January 2022;
Published: 11 February 2022.
Edited by:
Yingfang Zhu, Henan University, ChinaCopyright © 2022 Xu, Hassan, Sun, Wu, Jiang, Liu, Ni, Yang, Fang, Li and Chen. This is an open-access article distributed under the terms of the Creative Commons Attribution License (CC BY). The use, distribution or reproduction in other forums is permitted, provided the original author(s) and the copyright owner(s) are credited and that the original publication in this journal is cited, in accordance with accepted academic practice. No use, distribution or reproduction is permitted which does not comply with these terms.
*Correspondence: Xiang Chen, 15056921642@163.com; Jincai Li, ljc5122423@126.com
†These authors have contributed equally to this work
Disclaimer: All claims expressed in this article are solely those of the authors and do not necessarily represent those of their affiliated organizations, or those of the publisher, the editors and the reviewers. Any product that may be evaluated in this article or claim that may be made by its manufacturer is not guaranteed or endorsed by the publisher.
Research integrity at Frontiers
Learn more about the work of our research integrity team to safeguard the quality of each article we publish.