- 1Department of Plant Physiology and Biochemistry, Agricultural University, Plovdiv, Bulgaria
- 2Institute of Botany, University of Punjab, Lahore, Pakistan
- 3Senior Superintendent Garden, RO-II Office, University of Punjab, Lahore, Pakistan
- 4Department of Botany, Division of Science and Technology, University of Education, Lahore, Pakistan
- 5Department of Botany and Microbiology, King Saud University, Riyadh, Saudi Arabia
- 6Department of Environmental Sciences, University of Narowal, Punjab, Pakistan
- 7Key Laboratory of Ministry of Education for Genetics, Breeding and Multiple Utilization of Crops, Centre of Legume Crop Genetics and Systems Biology/College of Agriculture, Oil Crop Research Institute, Fujian Agriculture and Forestry University (FAFU), Fuzhou, China
- 8College of Agriculture, Fujian Agriculture and Forestry University, Fuzhou, China
- 9Agronomy, Horticulture, and Plant Science Department, South Dakota State University, Brookings, SD, United States
The application of nanoparticles (NPs) has been proved as an efficient and promising technique for mitigating a wide range of stressors in plants. The present study elucidates the synergistic effect of iron oxide nanoparticles (IONPs) and silicon nanoparticles (SiNPs) in the attenuation of Cd toxicity in Phaseolus vulgaris. Seeds of P. vulgaris were treated with IONPs (10 mg/L) and SiNPs (20 mg/L). Seedlings of uniform size were transplanted to pots for 40 days. The results demonstrated that nanoparticles (NPs) enhanced growth, net photosynthetic rate, and gas exchange attributes in P. vulgaris plants grown in Cd-contaminated soil. Synergistic application of IONPs and SiNPs raised not only K+ content, but also biosynthesis of polyamines (PAs), which alleviated Cd stress in P. vulgaris seedlings. Additionally, NPs decreased malondialdehyde (MDA) content and electrolyte leakage (EL) in P. vulgaris plants exposed to Cd stress. These findings suggest that stress alleviation was mainly attributed to the enhanced accumulation of K+ content, improved antioxidant defense system, and higher spermidine (Spd) and putrescine (Put) levels. It is suggested that various forms of NPs can be applied synergistically to minimize heavy metal stress, thus increasing crop production under stressed conditions.
Introduction
Mining operation, industrial waste, and pollutants emitted during agricultural and industrial operations (Palansooriya et al., 2020; Raghib et al., 2020) release substantial quantities of toxic heavy metals (As, Cd, Hg, Pb) into the environment. The quantity of harmful heavy metals in the soil environment has steadily increased over time, which produces negative effects on plant growth and their developmental process, which is a great challenge for the sustainability of agriculture (Afzal et al., 2019). Among the numerous heavy metals, Cd content in the environment has risen owing to excessive use of chemical fertilizers and pesticides besides mining sources (Seshadri et al., 2016; Manzoor et al., 2019). Although the concentration of Cd reported in the environment is low, it is considered extremely toxic due to its higher mobility in media and living cells (Rahman and Singh, 2019; Kaya et al., 2020a), which is raising concerns for agriculture. It was reported that Cd exposure reduces seed sprouting, root elongation, shoot development, and the number of leaves per plant (Kaya et al., 2020b; Seifikalhor et al., 2020). In addition, it also results in the overproduction of reactive oxygen species (ROS) (Moradi-Marjaneh et al., 2019), which influence plant physiological, biochemical, and molecular characteristics (Raza et al., 2020). Plants can avoid, tolerate, and immobilize heavy metals in soil (Kumar and Prasad, 2018; Mei et al., 2020) by activating signaling molecules that regulate ROS production, phytohormone synthesis, and calcium calmodulin pathways (Bali et al., 2019). The activated pathways include the production of nitric oxide (NO), glutathione, phytohormones, and antioxidant enzymes (Dias et al., 2019; Rady et al., 2019), which lead to Cd chelation and reduced oxidative damage.
Nanoparticles (NPs) with a size of 100 nm exhibit unique properties such as increased reaction site, high surface activity, better catalytic efficiency, and unique magnetic characteristics (Yang et al., 2017, 2018; Wang et al., 2019), which have assisted in agriculture promotion by alleviating abiotic stress in plants (Adeel et al., 2019). Many researchers have reported that NPs improve seed germination, rhizome development, and quality of crops cultivated in stressed conditions (Ghafariyan et al., 2013; Palchoudhury et al., 2018; Kah et al., 2019). Nanotechnology research offers a new pathway for soil pollution remediation (Liu et al., 2021), since it has the potential to enhance plants’ antioxidative defense systems, thus reducing the bioaccumulation of ROS in plants (Usman et al., 2020; Wang et al., 2020). As documented, NPs when applied in soil (Li et al., 2021) or sprayed via foliar (Toumey, 2020), results in a reduction of Cd and Pd toxicity in rice seedlings (Hussain et al., 2020). It was observed that the Si improved hydraulic conductivity in applied plants through enhancing K+ concentration in xylem sap resulting in improved xylem hydraulic conductivity and osmotic gradient (Chen et al., 2016). Similarly, exogenously applied Fe modulates K+ uptake and translocation in crop plants (Okturen Asri and Sonmez, 2014).
Potassium is a crucial phytonutrient required by plants for the maintenance of growth (Jaiswal et al., 2016), stomatal conductance, gaseous exchange (Hasanuzzaman et al., 2018), photosynthate production, and antioxidative defense system (Xu et al., 2020). Approximately 10% of plant biomass is composed of K, which not only acts as a co-factor for various enzymes involved in photosynthesis and protein stabilization, but it also hinders Cd-translocation and escalates the production of crucial amino acids, carbohydrates, and nitrogenous compounds. Potassium improves plant stress tolerance by regulating the biosynthesis of polyamines (Karimi, 2017). Polyamines regulate physiochemical procedures of plants under normal conditions and also induce modification in the expression level of stress-responsive genes besides detoxifying metals by vacuolar compartmentalization (Spormann et al., 2021).
Considering the background information, we hypothesized that the synergistic effect of NPs can mitigate Cd stress; and regulate polyamine synthesis, K+ metabolism, and antioxidant enzymes. Therefore, the objective of the present study was to explore the individual and combined role of IONPs and SiNPs application in mitigation of Cd toxicity through regulation of K+ metabolism and antioxidant enzymes in P. vulgaris exposed to Cd-contaminated conditions.
Materials and Methods
A total of 85 soil samples were collected (0–30 cm) from an agricultural field (2-ha area) in the vicinity of the campus site. The soil was sieved through a 4 mm mesh to remove plant parts, debris, then thoroughly mixed and conditioned for 1 week at 35% of water holding capacity (WHC) before the experiment. The physicochemical properties of the soil such as pH, electrical conductivity (EC), organic matter, and metal content (Cd, Zn, Fe, Ni, Pb) were measured using standard protocols (Bouyoucos, 1962; Page et al., 1982). The physicochemical properties of the soil were as follows; pH (7.13), EC (1.87), and organic content (0.45%). The total concentration of Cd, Zn, Fe, Ni, and Pb in soil was quantified as 5.87, 34.98, 37.91, 13.28, and 35.18 mg/kg, respectively. The soil was contaminated by mixing CdCl2. Cadmium concentrations in the soil were kept as 0 mM CdCl2, 1 mM CdCl2, 1.5 mM CdCl2, and 2 mM CdCl2. Both IONPs and SiNPs were purchased from Alfa Aesar. A pilot project was carried out to find out the toxic concentration of Cd that affects the growth of P. vulgaris. A 10 mg/L IONPs and 20 mg/L SiNPs were found to be effective against selected Cd toxic levels. Iron oxide nanoparticles (IONPs) obtained from Alfa Aesar were having 99% purity, size 15–25 nm, and 4.67 density. Silicon nanoparticles obtained from Alfa Aesar were having 98% purity, size 40–100 nm, and 4.7 density. The solution used during the experiment was prepared using deionized H2O.
Seed Priming With Iron Oxide Nanoparticles and Silicon Nanoparticles
Seeds of P. vulgaris were surface sterilized using 2.5% sodium hypochlorite solution for 2 min and then washed with deionized H2O to remove chlorophyll (Chl) contents. IONPs and SiNPs were weighed and added in deionized H2O followed by ultra-sonication for half-hour and their desired concentrations (IONPs = 10 mg/L) and (SiNPs = 20 mg/L) were achieved. After that sterilized seeds of P. vulgaris were soaked in an NP solution. In the case of the control treatment, seeds were treated with deionized H2O. In the next step, soaked seeds were dried and stored at 4°C for further experiments.
Seed Sowing and Greenhouse Conditions
The current study was executed at the university site (32°′N, 74°′E, 236m altitude). Ten seeds were sown in plastic pots of 40 × 45 cm containing 20kg treated soil. These pots were kept in the greenhouse condition with an average temperature of 20/15°C (day/night), average humidity of 50/70% late afternoon/morning, and a natural photoperiod. After germination, thinning was done and 8 plants were retained per pot. Regular weeding was done and plants were watered daily to uphold 70% of the field maximum moisture capacity throughout the growth period.
Determination of Growth and Leaf Relative Water Content
Plants were uprooted after 40 days and growth characteristics (root and shoot fresh weight, root and shoot dry weight) were estimated. Leaf relative water content (LRWC) values from P. vulgaris leaf samples were estimated using the following equation as given by Smart and Bingham (1974);
Where LRWC = Leaf relative water content
LFW = Leaf fresh weight
LDW = Leaf dry weight
LTW = Leaf turgid weight
Estimation of Photosynthetic Pigments
Arnon method (1949) was used for the estimation of photosynthetic pigments. Approximately 100 mg leaf extract was mixed with 80% acetone and centrifuged for 5 min at 10,000 rpm. The optical density (OD) of filtrates was then measured using a spectrophotometer (Hitachi U-2001, Tokyo, Japan).
Determination of Malondialdehyde Content and Electrolyte Leakage
Malondialdehyde (MDA) content was determined according to the method of Cavalcanti et al. (2004). Briefly, 0.5 g samples were homogenized using mortar and pestle in 4 mL of trichloroacetic acid (TCA) (1% w/v) at 4°C. The homogenous mixture was then centrifuged for 20 min at 12,000 rpm to collect the supernatant. One milliliter of this supernatant was added to 3 mL of reaction mixture containing TCA (20% w/v) and thiobarbituric acid (0.5% w/v). The reaction mixture was incubated at 95°C for half-hour and the reaction was stopped by placing it in an ice bath. The absorbance value of the fraction was measured at 440, 532, and 600 nm.
The method of Blum and Ebercon (1981) was used for the determination of electrolyte leakage. A dry leaf sample (0.2 g) was floated in deionized H2O (50 mL) for 24 h at room temperature with shaking. The electrolyte content in the solution was quantified and recorded as CO. After 20 min of boiling the sample, the electrolyte content in the solution was recorded as C1. Electrolyte leakage was measured in percentage as per the following formula:
Determination of Antioxidant Enzymes
About 1 g leaf samples were homogenized in a solution of potassium phosphate buffer solution (100 mM), polyvinyl pyrrolidone (1% w/v), ethylenediamine tetraacetic acid (0.1 mM) and triton X-100 (0.5%). The homogenate was filtered through cheesecloth and centrifuged at 18,000 rpm for 20 min at 4°C. The resultant supernatant was used for the estimation of antioxidative enzymes and was stored at –80°C for analysis. The method of Aebi (1974) was used for the determination of catalase (CAT) activity. Briefly, potassium phosphate buffer (50 mM) and plant extract were used in the reaction (3 mL). To start the reaction, 10 mM H2O2 was added. 1 unit of CAT is defined as the number of enzymes, which release 1/2 of peroxide oxygen from 10 mM H2O2 solution in 100 s at 25°C. Superoxide dismutase (SOD) activity was measured according to the method of Beyer and Fridovich (1987). The reaction mixture (30 mL) for the determination of SOD was composed of potassium phosphate buffer (50 mM), methionine (9.9 mM), nitroblue tetrazolium (57 mM), and plant extract. The reaction mixture was started by light illumination. One unit of SOD is defined as the enzyme which causes a 50% decrease of SOD inhabitable reduction.
Determination of Proline Content
To determine proline content, dry leaf samples (0.5 g) were extracted in sulfosalicylic acid (3%) and then filtered (Bates et al., 1973). Afterward, 2 mL of leaf extract was mixed in ninhydrin solution (2 mL) and glacial acetic acid (2 mL). Plant samples were incubated at 100°C for 1 h and cooled in an ice bath. Four milliliters of toluene was vigorously added to the mixture. The absorbance value was calibrated at 520 nm using a spectrophotometer. Proline content was estimated using a standard curve.
Determination of Cadmium Content
Following Cd treatment, P. vulgaris seedlings were rinsed with deionized H2O. Seedlings were dried at 80°C for 24 h. The plant material was digested in HNO3:HCLO4. About 1 g plant sample was homogenized in 50 mM Tris (hydroxymethyl) aminomethane (Tris–HCl), 250 mM sucrose, and 1 mM DL-dithiothreitol. The homogenous mixture obtained was centrifuged at 3,000 rpm for 5 min for the isolation of the cell wall. The supernatant obtained was centrifuged at 20,000 rpm for 45 min. Cd was determined using ICP (ICP-AES, Thermo Elemental, United States) (Wang et al., 2009).
Determination of Nutritional Content
A flame photometer (Jenway PFP-7) was used for the determination of nutritional content (Mo+, Ca+, K+, Mn+) from the digested plant samples. K+ content was quantified with the help of a standard solution.
Determination of Gaseous Exchange
Assessments of gaseous exchange attributes, net CO2 uptake, net photosynthesis (Pn), stomatal conductance (Gs), and transpiration rate (E), were performed at 10:00 a.m. at 27°C during the daytime on completely expanded leaves using a portable gas exchange system (Holá et al., 2010).
Determination of Polyamine Activity
The concentration of hydrogen peroxide produced during polyamine oxidation assisted in the estimation of polyamine oxidase (PAO) activity. The foliage plant sample was mixed with potassium phosphate buffer (0.1 mM at pH 6.5 in an pre-chilled mortar and pestle. The resultant supernatant (0.5 mL) was obtained by centrifugation of this mixture at 10,000 g at 4°C for 20 min followed by mixing with 4-aminoantipyrine/N,N-dimethylaniline solutions (0.4 mL), 0.2 mL horseradish POD (250 U/mL), and 5 mL of K3PO4 buffer (100 mM at pH 6.5). For the determination of polyamine oxidase, putrescine (30 mL of 20 mM) was homogenized in enzyme extract to initiate reaction according to Zhao et al. (2004).
Determination of Nitric Oxide Content
Nitric oxide activity was quantified using the nitric oxide detection kit (Solarbio Life Science, Beijing, China) following the manufacturer’s instructions.
Estimation of Soluble Protein Content
Leaf samples (1 g) were extracted using KH2PO4 (4 mL at pH 6.8) following centrifugation at 3,600 rpm for 30 min. The resultant supernatant was collected and a 20 μL aliquot of the extract was collected and homogenized with the Bradford color reagent (1 mL). The spectrophotometer calibration was carried out at 595 nm as per the procedure described by Bradford (1976).
Statistical Analysis
Data reported in the experiment was mean of 5 replicates. Statistical package XL-STAT was carried out for analysis of variance (ANOVA). Subsequently, Tukey’s test was conducted to determine the significant differences among the values.
Results
Effect of Iron Oxide Nanoparticles and Silicon Nanoparticles on Growth Attributes of Phaseolus vulgaris
Table 1 shows the role of IONPs and SiNPs, alone or in combination, on the growth of P. vulgaris seedlings grown in normal and Cd-contaminated soil. Synergistic application of NPs improved shoot fresh weight and root fresh weight by 39 and 40%, respectively, as compared to IONPs-only treated P. vulgaris seedlings grown in non-contaminated soil. When P. vulgaris seedlings were exposed to 2 mM CdCl2, combined treatment of IONPs and SiNPs increased shoot fresh weight and root fresh weight by 45 and 19%, respectively, as compared to SiNP-treated P. vulgaris seedlings grown in Cd-contaminated media. Likewise, combined and synergistic treatments involving NPs enhanced root dry weight and shoot dry weight in P. vulgaris seedlings grown in normal and Cd-contaminated conditions (Table 1).
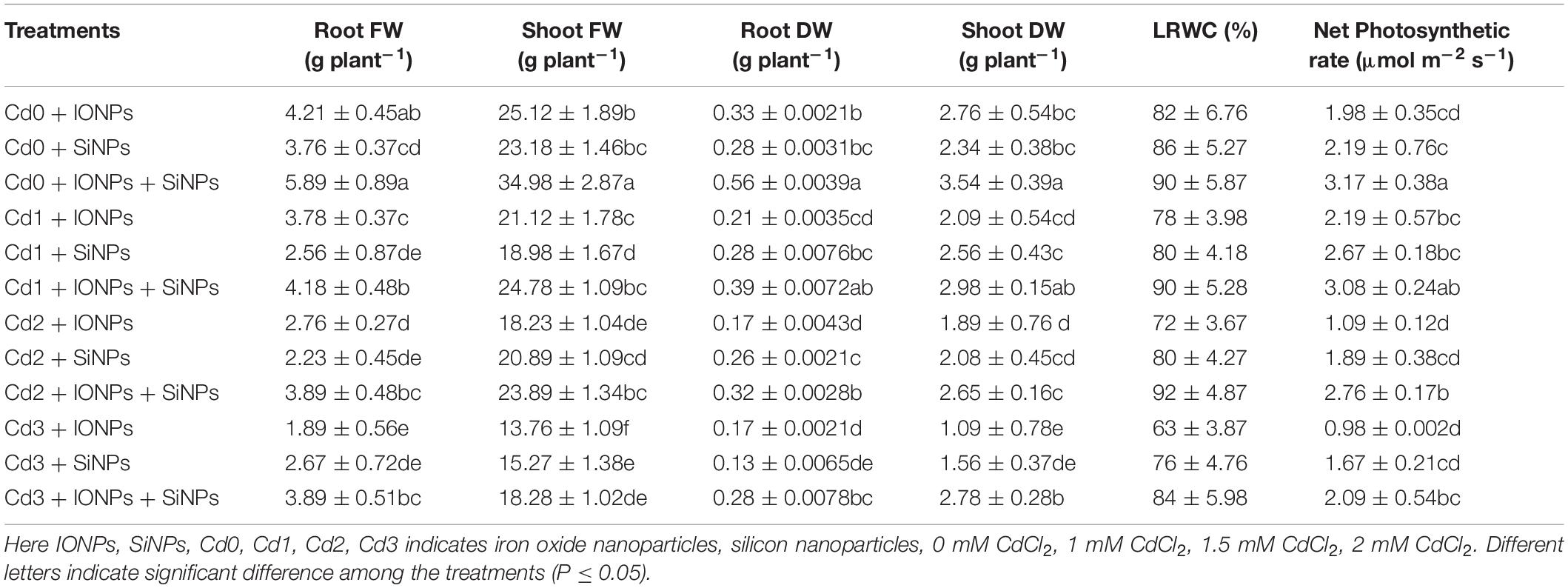
Table 1. Effect of IONPs and SiNPs on root fresh weight, shoot fresh weight, root dry weight, shoot dry weight, leaf relative water content, and net photosynthetic rate of Phaseolus vulgaris grown in different concentrations of Cd.
Effect of Iron Oxide Nanoparticles and Silicon Nanoparticles on Leaf Relative Water Content and Net Photosynthetic Rate of Phaseolus vulgaris
When P. vulgaris seedlings were grown in non-contaminated media, combined treatment of IONPs and SiNPs enhanced LRWC content by 9 and 4% in comparison with IONPs-only and SiNPs-only treatment, respectively. A similar trend of net photosynthetic rate was observed in P. vulgaris seedlings exposed to different concentrations of CdCl2 (Table 1). IONPs and SiNPs also orchestered pigments content in P. vulgaris seedlings (Table 3).
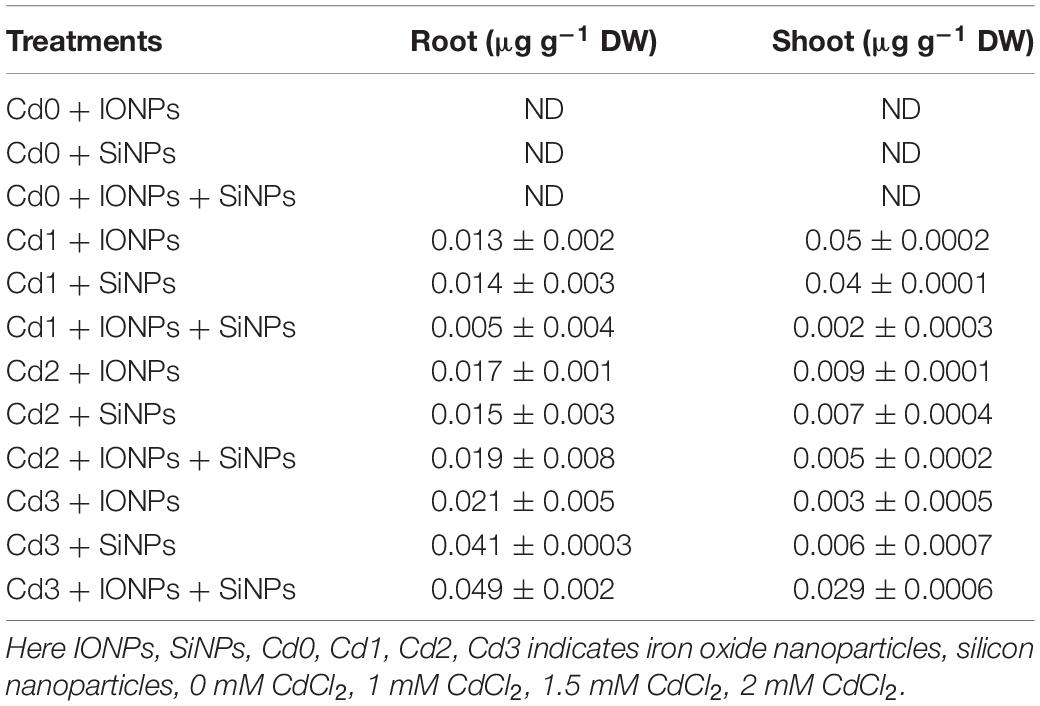
Table 2. Effect of IONPs and SiNPs on root Cd content, shoot Cd content, translocation factor, and metal tolerance index of Phaseolus vulgaris grown in different concentrations of Cd.
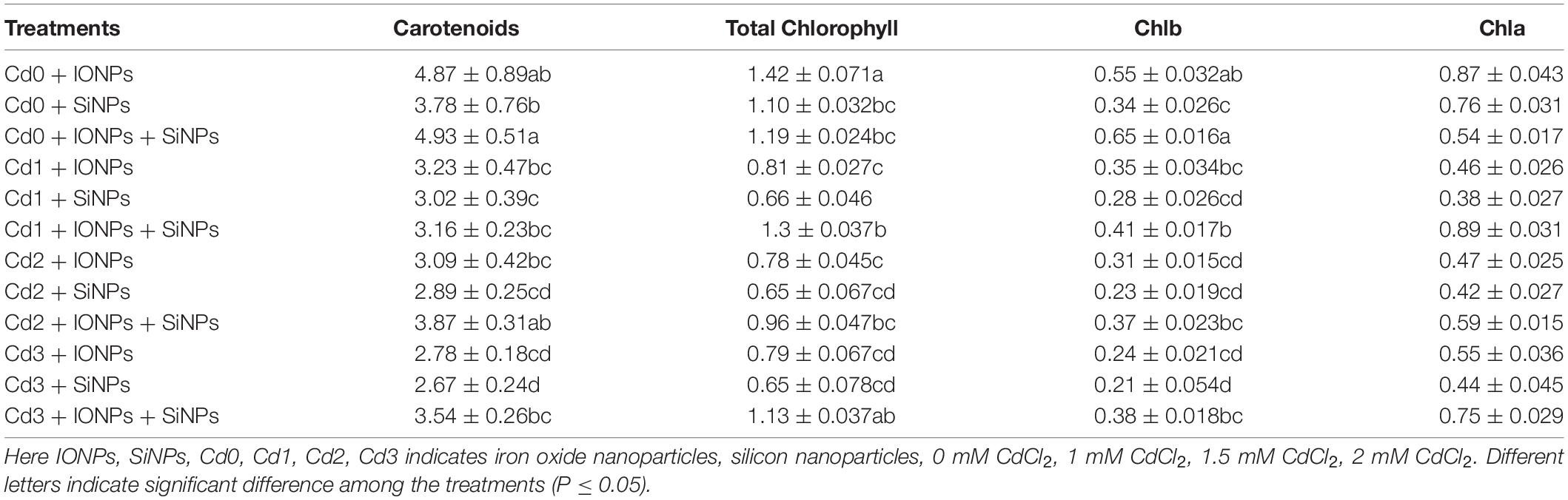
Table 3. Effect of IONPs and SiNPs on carotenoids, total chlorophyll content, Chlb, and Chla of Phaseolus vulgaris grown in different concentrations of Cd.
Effect of Iron Oxide Nanoparticles and Silicon Nanoparticles on Gas Exchange Parameters of Phaseolus vulgaris
In the case of P. vulgaris seedlings grown in non-contaminated soil, synergistic application of IONPs and SiNPs increased intercellular CO2 concentration and stomatal conductance by 8 and 18%, respectively, in comparison with alone application of IONP and SiNP treatment. When P. vulgaris seedlings were treated with 1 mM CdCl2, 1.5 mM CdCl2, and 2 mM CdCl2, intercellular CO2 concentration decreased by 20, 29, and 43%, respectively, as compared to Cd0 treatment. Synergistic application of IONP and SiNP increased intercellular CO2 concentration in P. vulgaris seedlings grown in 1.5 mM CdCl2 by 22 and 7%, respectively, in comparison with alone treatments of IONPs and SiNPs. Likewise, synergistic application of IONP and SiNP enhanced stomatal conductivity in P. vulgaris seedlings grown in Cd0-treatment and Cd-contaminated soil (Figure 1).
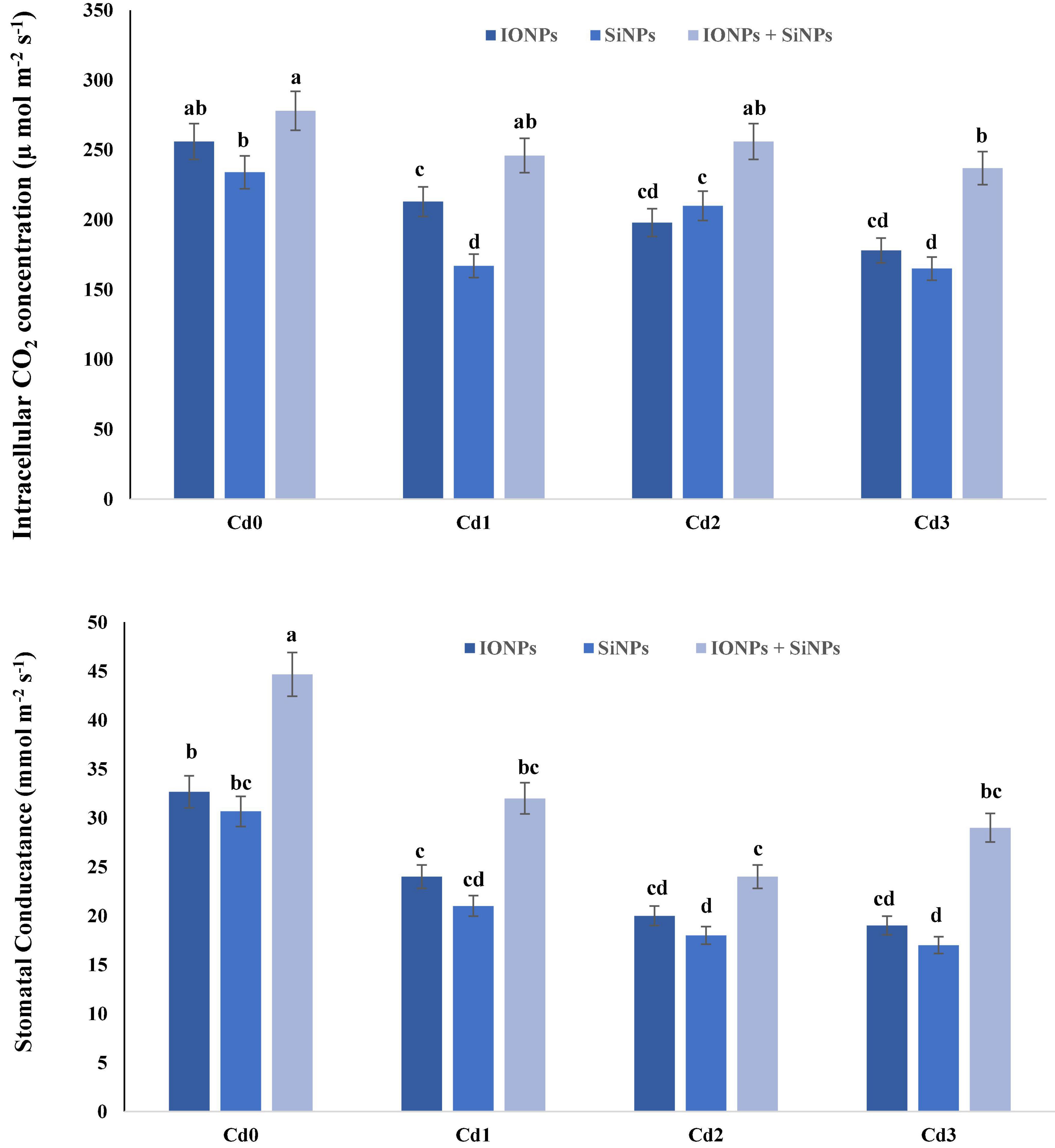
Figure 1. Effect of IONPs and SiNPs on intercellular CO2 concentration and stomatal conductance of Phaseolus vulgaris grown in different concentrations of Cd. Here IONPs, SiNPs, Cd0, Cd1, Cd2, and Cd3 indicate iron oxide nanoparticles, silicon nanoparticles, 0 mM CdCl2, 1 mM CdCl2, 1.5 mM CdCl2, and 2 mM CdCl2. Different letters indicate significant difference among the treatments (P ≤ 0.05).
Effect of Iron Oxide Nanoparticles and Silicon Nanoparticles on Malondialdehyde Content and Electrolyte Leakage in Phaseolus vulgaris
Figure 2 shows that the application of IONPs and SiNPs reduced MDA content and EL leakage in P. vulgaris seedlings grown in non-contaminated and Cd-polluted soil. Synergistic application of IONPs and SiNPs reduced MDA content by 50 and 20% as compared to individual application of IONPs and SiNPs, respectively, in P. vulgaris seedlings grown in 1.5 mM CdCl2-contaminated soil.
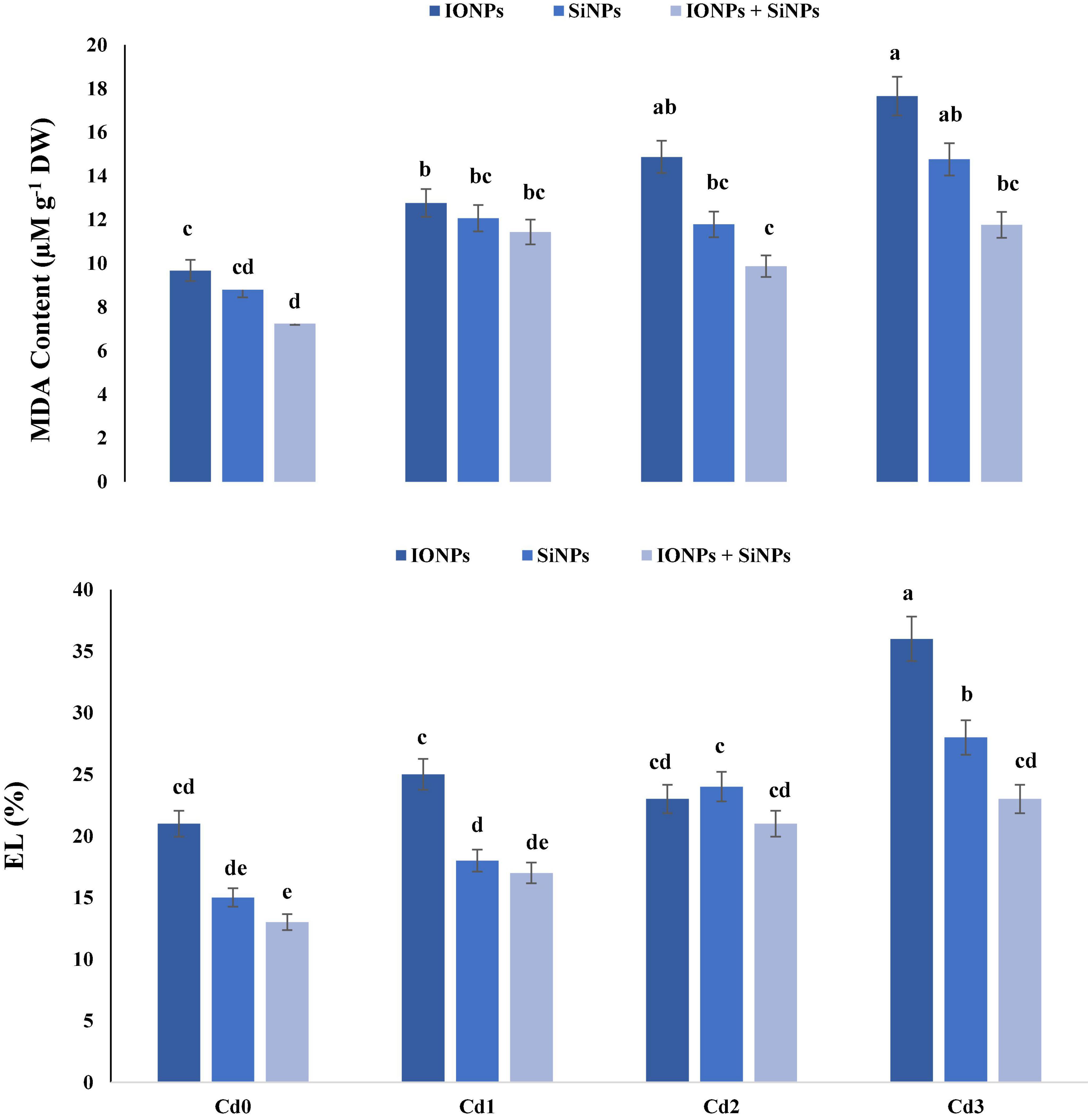
Figure 2. Effect of IONPs and SiNPs on MDA content and EL of Phaseolus vulgaris grown in different concentrations of Cd. Here IONPs, SiNPs, Cd0, Cd1, Cd2, and Cd3 indicate iron oxide nanoparticles, silicon nanoparticles, 0 mM CdCl2, 1 mM CdCl2, 1.5 mM CdCl2, and 2 mM CdCl2. Different letters indicate significant difference among the treatments (P ≤ 0.05).
Electrolyte leakage was also reduced in P. vulgaris seedlings treated with combined application of IONPs and SiNPs, in comparison with individual treatments. In the case of P. vulgaris seedlings grown in 2 mM CdCl2, combined application of IONPs and SiNPs reduced EL by 56 and 21%, respectively, in comparison with individual treatments of IONPs and SiNPs (Figure 2).
Effect of Iron Oxide Nanoparticles and Silicon Nanoparticles on Polyamine Content in Phaseolus vulgaris Seedlings
Figure 3 shows the effect of IONPs and SiNPs on spermidine (Spd) and putrescine (Put) content of P. vulgaris seedlings grown in normal and Cd-contaminated soil. Application of IONPs and SiNPs, alone or in combination, escalated Spd and Put content in P. vulgaris. Combined treatment of IONPs and SiNPs enhanced Spd content by 91% as compared to individual application of SiNPs in P. vulgaris seedlings grown in 1 mM CdCl2. Likewise, Put content also increased when P. vulgaris seedlings were treated with IONPs and SiNPs in combination. The combined application of IONPs and SiNPs enhanced Put content by more than onefold in comparison with SiNPs-only treatment in P. vulgaris seedlings grown in 2 mM CdCl2.
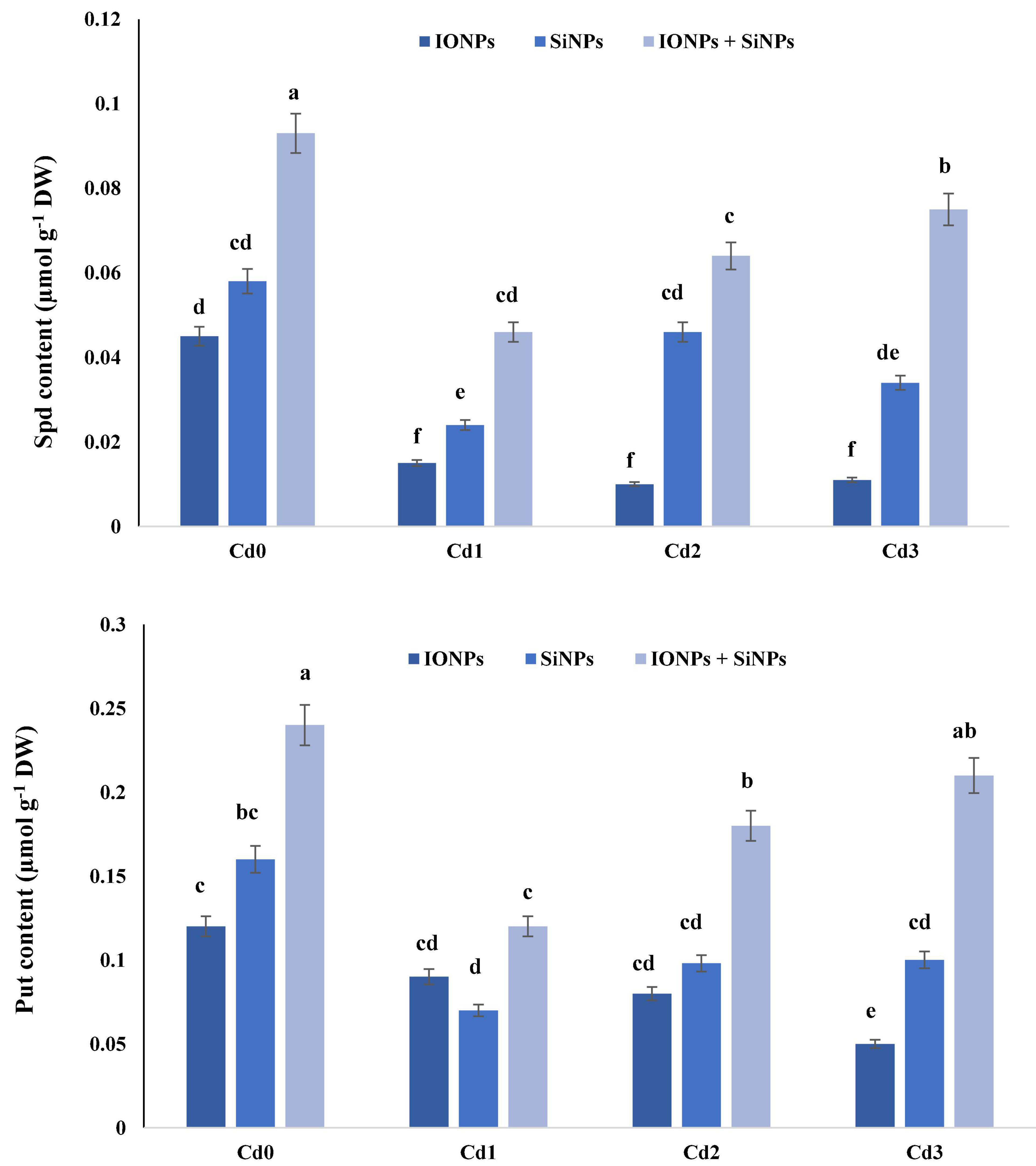
Figure 3. Effect of IONPs and SiNPs on PAs content of Phaseolus vulgaris grown in different concentrations of Cd. Here IONPs, SiNPs, Cd0, Cd1, Cd2, and Cd3 indicate iron oxide nanoparticles, silicon nanoparticles, 0 mM CdCl2, 1 mM CdCl2, 1.5 mM CdCl2, and 2 mM CdCl2. Different letters indicate significant difference among the treatments (P ≤ 0.05).
Effect of Iron Oxide Nanoparticles and Silicon Nanoparticles on Nitric Oxide and Proline Content of Phaseolus vulgaris
Nitric oxide and proline (Pro) content are considered as stress markers in plants. During the current study, IONPs and SiNPs enhanced nitric oxide and proline content in P. vulgaris seedlings grown in normal and Cd-contaminated soil. In the case of P. vulgaris seedlings grown in control conditions, synergistic application of IONPs and SiNPs enhanced NO content by 44 and 50%, respectively, in comparison with individual application of IONPs and SiNPs. When P. vulgaris seedlings were exposed to 2 mM CdCl2, combined application of IONPs and SiNPs enhanced NO content by more than onefold and 60% as compared to IONPs-only and SiNPs-only treatment. Similarly, IONPs and SiNPs also enhanced proline content in P. vulgaris seedlings grown in normal and Cd-contaminated conditions (Figure 4).
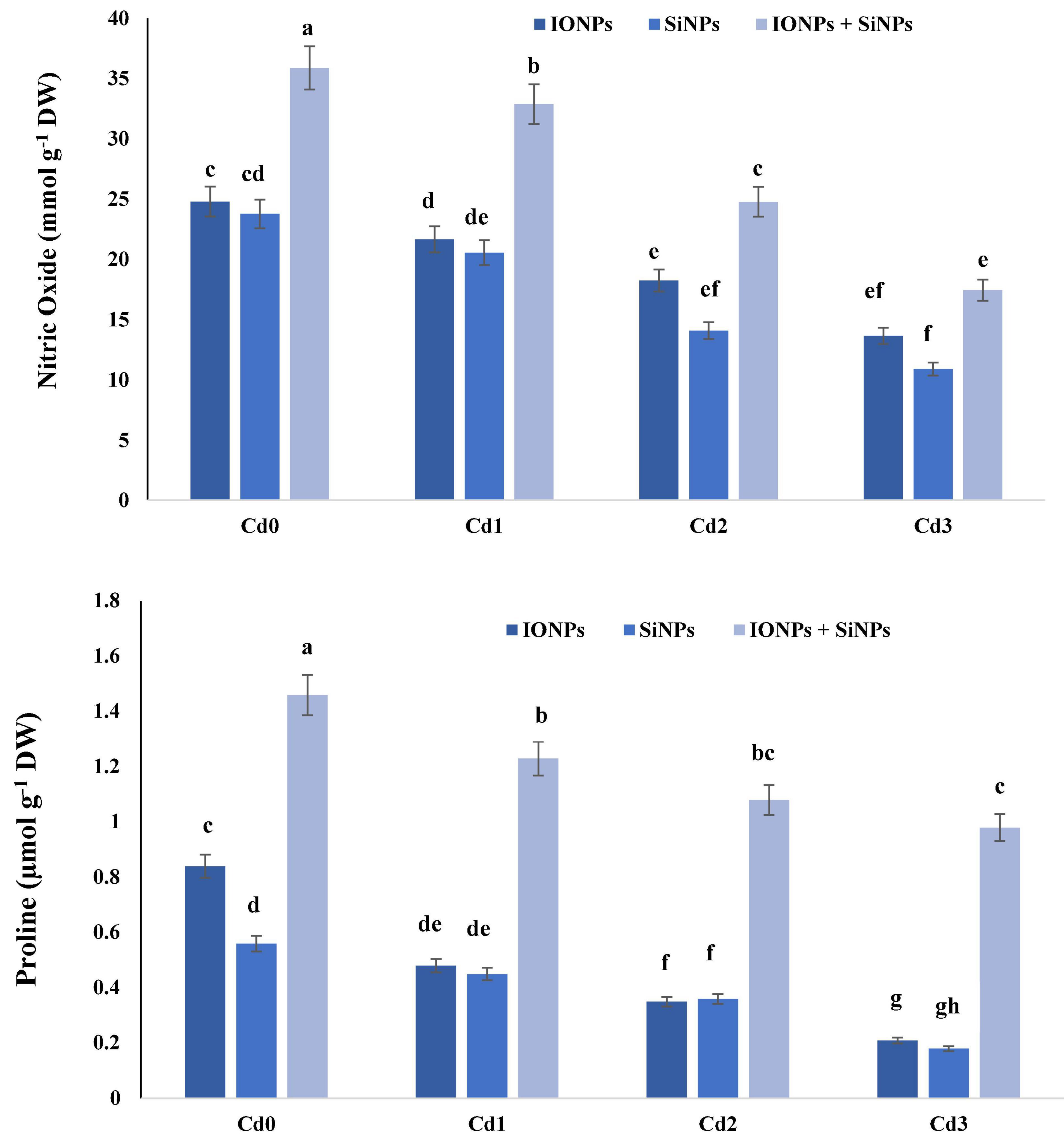
Figure 4. Effect of IONPs and SiNPs on nitric oxide and proline content of Phaseolus vulgaris grown in different concentrations of Cd. Here IONPs, SiNPs, Cd0, Cd1, Cd2, and Cd3 indicate iron oxide nanoparticles, silicon nanoparticles, 0 mM CdCl2, 1 mM CdCl2, 1.5 mM CdCl2, and 2 mM CdCl2. Different letters indicate significant difference among the treatments (P ≤ 0.05).
Effect of Iron Oxide Nanoparticles and Silicon Nanoparticles on the Activity of Antioxidant Enzymes in Phaseolus vulgaris
Figure 5 explains the role of IONPs and SiNPs on the activity of SOD and CAT in P. vulgaris seedlings grown in control and Cd-contaminated conditions. In the case of P. vulgaris seedlings grown in Cd0 treatment, combined application of IONPs and SiNPs improved the activity of SOD by 41 and 71%, respectively, in comparison with IONPs-only and SiNPs-only treatment. Synergistic treatment of IONPs and SiNPs enhanced SOD content by 72 and 90% as compared to individual treatments of IONPs and SiNPs, respectively, in P. vulgaris seedlings grown in 2 mM CdCl2. Likewise, combined treatment of IONPs and SiNPs enhanced CAT activity in P. vulgaris seedlings grown in normal and CdCl2-contaminated conditions. The combined application of IONPs and SiNPs escalated CAT activity by 71 and 50%, as compared to IONPs-only and SiNPs-only treatment, respectively, in P. vulgaris seedling exposed to 2 mM CdCl2.
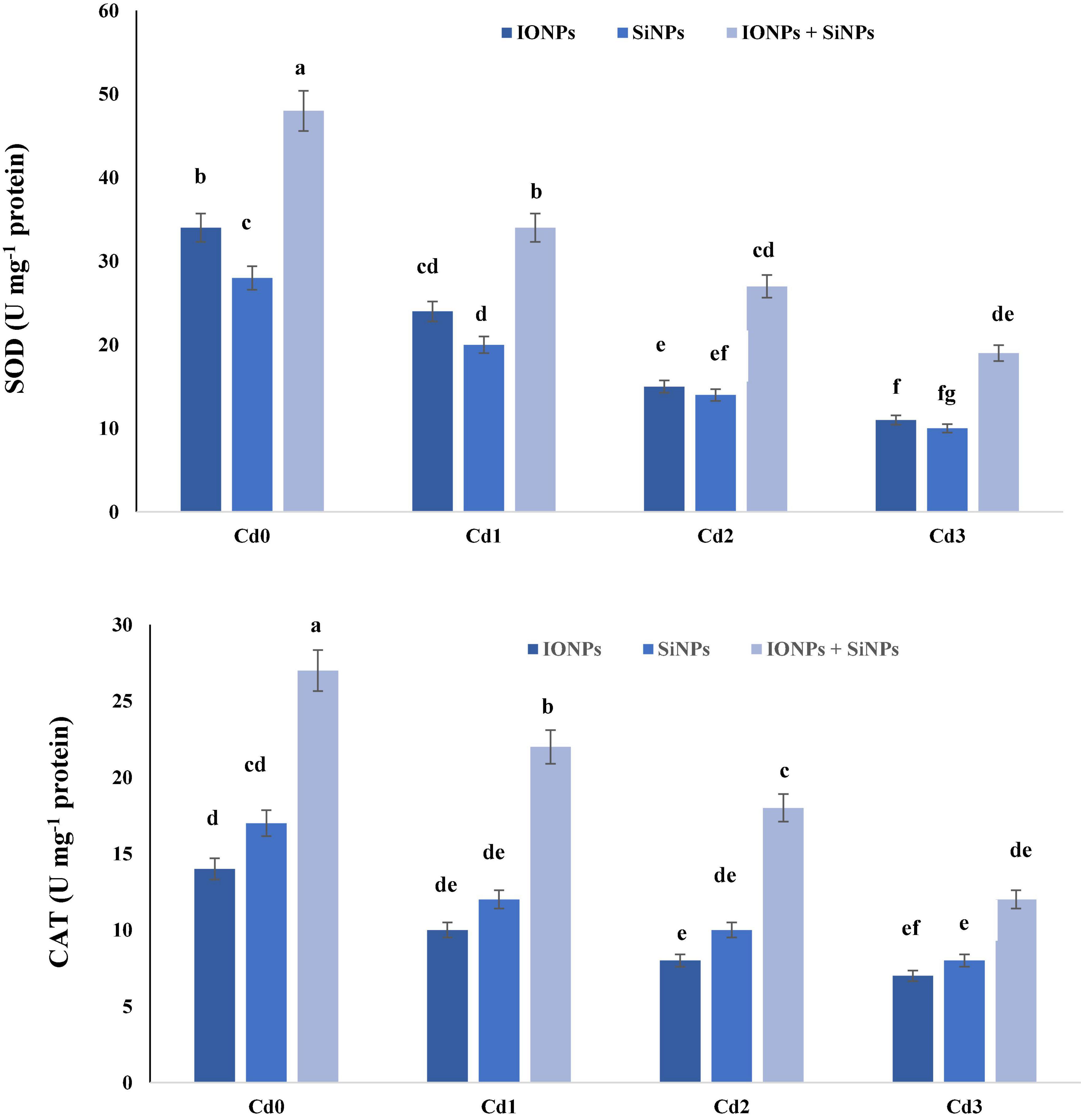
Figure 5. Effect of IONPs and SiNPs on SOD and CAT activity of P. vulgaris grown in different concentrations of Cd. Here IONPs, SiNPs, Cd0, Cd1, Cd2, and Cd3 indicate iron oxide nanoparticles, silicon nanoparticles, 0 mM CdCl2, 1 mM CdCl2, 1.5 mM CdCl2, and 2 mM CdCl2. Different letters indicate significant difference among the treatments (P ≤ 0.05).
Effect of Iron Oxide Nanoparticles and Silicon Nanoparticles on Cadmium Uptake of Phaseolus vulgaris
Table 2 shows that NP treatment reduced Cd uptake in P. vulgaris seedlings grown in CdCl2-contaminated soil. Synergistic application of IONPs and SiNPs reduced Cd uptake by 68% in P. vulgaris seedling grown in 2 mM CdCl2.
Effect of Iron Oxide Nanoparticles and Silicon Nanoparticles on the Nutritional Content of Phaseolus vulgaris
Table 4 describes the role of IONPs and SiNPs on the nutritional content of P. vulgaris seedlings grown in normal and different concentrations of CdCl2. A combined application of IONPs and SiNPs increased K+ content by 31 and 24%, respectively, as compared to IONPs-only and SiNPs-only treated P. vulgaris seedlings grown in non-contaminated potted soil.
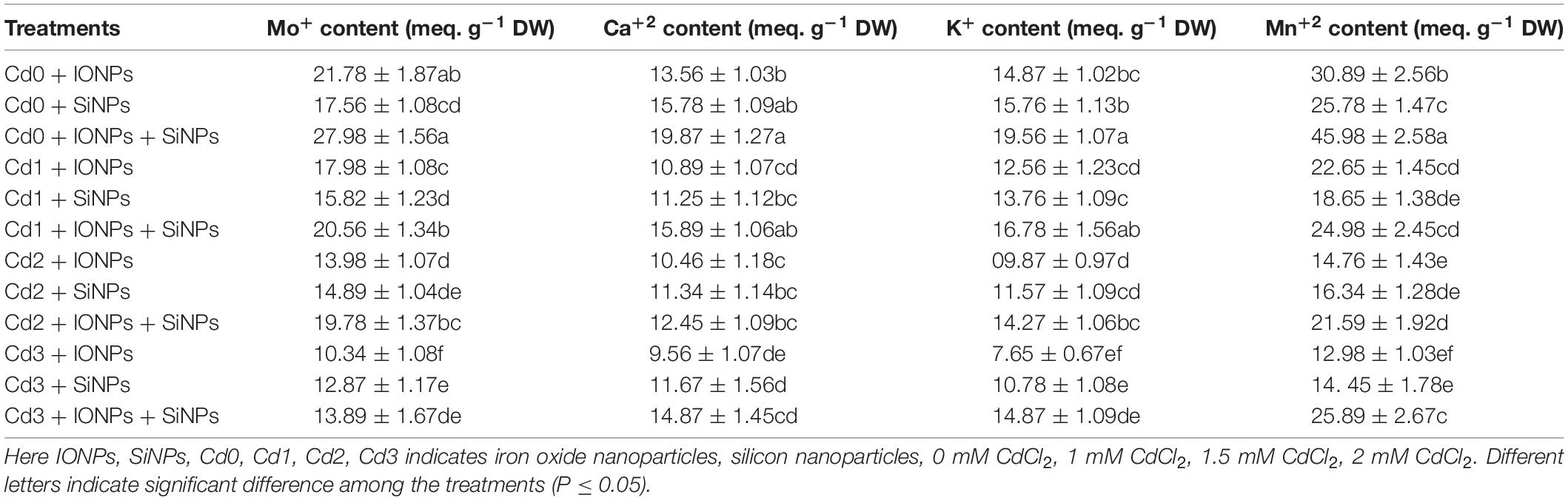
Table 4. Effect of IONPs and SiNPs on Mo+, Ca+, K+, and Mn+ of Phaseolus vulgaris grown in different concentrations of Cd.
Discussion
Cadmium toxicity reduces the ability of plants to absorb key nutrients (Mg, Ca, P, K) and water content from soil (Yang et al., 2020). Potassium is considered as crucial for plant growth, development, and metabolomics (Hasanuzzaman et al., 2018) because it alleviates abiotic stresses (Raza et al., 2021) by restricting transportation and accumulation of heavy metals in plants (Kerchev et al., 2020). Additionally, potassium not only immobilizes heavy metal content (Liu et al., 2018) but also behaves antagonistically to Cd and detoxifies Cd within plants (Shamsi et al., 2008). Cadmium is a non-essential metal and is involved in the hindrance of plant growth (Shah et al. 2020a) by causing a reduction in water content, biomass, fresh and dry weight of plants, as well as disruption in redox reaction (Raza et al., 2020). Potassium application stabilizes chlorophyll architecture, which leads to the regulation of photosynthate production (Hasanuzzaman et al., 2018). It was also documented that K+ application enhanced growth and yield in apple dwarf rootstock seedlings (Xu et al., 2020) via Cd assimilation by increased accumulation of K+ in plants (Norvell et al., 2000). In addition, the increase in K+ enhanced the activity of antioxidant enzymes and reduced MDA content in plants exposed to Cd toxicity. The results of the present study also indicated that synergistic application of IONPs and SiNPs enhanced K+ content in P. vulgaris seedlings grown in normal and Cd-contaminated soil. In the present study, IONPs and SiNPs treatment increased LRWC in P. vulgaris seedling (Table 1). Before, it was also reported that SiNP treatment enhanced gas exchange characteristics in plants exposed to Cd-contaminated media (Ur Rahman et al., 2021). Cadmium stress destabilized photosynthetic apparatus, yet IONPs and SiNPs application reduced injury to photosynthetic machinery. Iron oxide nanoparticles increased root and shoot length in Arachis hypogaea plants (Rizwan et al., 2019). In the present research, it was noted also that that synergistic application of IONPs and SiNPs increased photosynthesis and gas exchange characteristics in P. vulgaris seedlings.
Proline is crucial for the sustenance of metabolomics in plants (Ahmad et al., 2019). It was noted in the present investigation that P. vulgaris seedlings treated with IONPs and SiNPs showed an increased level of proline under Cd stress. The increased level of proline in NP-treated plants might lead to activation of proline synthesizing genes involved in the regulation of water content in plants.
Membrane stability is an essential characteristic required for the effective survival of plants under abiotic stress since damage to it will lead to cellular death. MDA is an indicator of cellular damage and membrane stability (Shah et al. 2020b). Under stress conditions, the generation of MDA and peroxidation of lipids increased causing damage to plants. In this study, Cd stress amplified MDA and EL in P. vulgaris plants grown in Cd-contaminated soil. Cadmium decreases the stability of membranous molecules due to the enhanced accumulation of ROS. Previous researchers revealed the role of IONPs and SiNPs in alleviating abiotic stresses in plants.
The total antioxidant activity of a plant is a good indicator of how well the plant’s antioxidant system is functioning in the presence of oxidative stress. Plants have a variety of antioxidative defense systems to deal with oxidative stress, particularly those produced by the generation of excess ROS as a result of abiotic stress. Superoxide peroxidase (SOD) is the first line of defense that is involved in the conversion of superoxide anion to peroxide. Catalase (CAT) is also a crucial antioxidant that plays an important role in plants’ defensive approach to abiotic stresses. This enzyme is involved in the conversion of H2O2 to O2 and H2O. Current research reported that IONPs and SiNPs enhanced the activity of CAT in P. vulgaris seedlings exposed to Cd stress.
Nanoparticles reduced MDA levels, while they increased peroxidase (POD) and SOD activity in metal-stressed wheat plants (Sardar et al., 2022). Additionally, osmoregulators control the structure of organelles and macromolecules in plants by adjusting osmosis and aid in the reduction of Cd-induced toxicity in plants (Thind et al., 2021). In the present study, we also noticed that Cd inhibited the production of total soluble proteins and proline concentration in P. vulgaris seedlings. The findings of the present study were in line with the findings of Azizi et al. (2021). Application of NPs in the present study enhanced proline accumulation in Cd treated P. vulgaris seedlings. Enhanced accumulation of proline and soluble sugar may be due to augmented proline playing some vital roles in scavenging ROS, adjusting osmotic equilibrium and determining the membrane attributes in plants exposed to stress (Aswani et al., 2019). Many authors investigated the positive effect of NPs on the enhancement of proline accumulation under stress conditions in broad bean plants in addition to adjusted antioxidant enzyme activities, soluble sugars, and amino acids (Sadak and Bakry, 2020). Furthermore, Azimi et al. (2021) claimed that NP supply can decline salt stress in tomatoes by improving photosynthetic machinery, phenolics, and antioxidant enzyme activities and yield as well.
Cadmium phytotoxicity increased the formation of ROS and produced oxidative burst by interfering with the antioxidant defense system (Kamran et al., 2021), which promotes the MDA content due to the high accumulation of lipid peroxidation of the membrane. During the normal metabolism, the plants have established well-organized antioxidant enzyme defense mechanisms to eliminate the ROS (Fan et al., 2020). This is because SOD acts as the first line of defense in plants against ROS. Superoxide dismutase converts the O2– to less toxic H2O2, and it forms the first line of defense in the antioxidant system of plants; to this end, CAT scavenges the H2O2 to water and oxygen molecules (Orabi and Abou-Hussein, 2019). The ameliorative influence of the NPs on Cd stress was also assessed by Hussain et al. (2018) in the wheat plant. This apparently will promote antioxidant impairments by decreasing H2O2 and MDA content and enhancing the activities of SOD, CAT, and increased tolerance against abiotic toxicity (Sharma et al., 2019).
Conclusion
Cadmium toxicity enhanced oxidative stress in P. vulgaris seedlings. Application of IONPs and SiNPs escalated the activity of antioxidant enzymes besides incrementation in K+ content. Additionally, synergistic application of IONPs and SiNPs increase NO content and Pro content in P. vulgaris seedlings grown in normal and CdCl2-polluted soil. Cd-stress alleviation is credited to increased activity of antioxidant enzymes and maintenance of PAs and K+ metabolism. It is further added that mechanisms and molecules involved in the interaction of different NPs can be exploited for the mitigation of numerous stresses in plants.
Data Availability Statement
The original contributions presented in the study are included in the article/supplementary material, further inquiries can be directed to the corresponding author/s.
Author Contributions
LK contributed toward designing the research and conceptualization. AU and AAS performed the experiments. AAS performed the statistical analysis. NAY and ZS contributed to the review and drafting. LR contributed to writing the manuscript. AR and TJ drafted the manuscript. MHS and SA were involved in funding acquisition and writing. All authors contributed to the article and approved the submitted version.
Conflict of Interest
The authors declare that the research was conducted in the absence of any commercial or financial relationships that could be construed as a potential conflict of interest.
Publisher’s Note
All claims expressed in this article are solely those of the authors and do not necessarily represent those of their affiliated organizations, or those of the publisher, the editors and the reviewers. Any product that may be evaluated in this article, or claim that may be made by its manufacturer, is not guaranteed or endorsed by the publisher.
Acknowledgments
The authors would like to extend their sincere appreciation to the Researchers Supporting Project Number (RSP-2021/194), King Saud University, Riyadh, Saudi Arabia.
References
Adeel, M., Ma, C., Ullah, S., Rizwan, M., Hao, Y., Chen, C., et al. (2019). Exposure to nickel oxide nanoparticles insinuates physiological, ultrastructural and oxidative damage: a life cycle study on Eisenia fetida. Environ. Pollut. 254:113032. doi: 10.1016/j.envpol.2019.113032
Aebi, H. (1974). “Catalalse,” in Methods of Enzymatic Analysis, ed. H. U. Bergmeyer (NewYork, NY: Academic press), 673–684.
Afzal, I., Shinwari, Z. K., Sikandar, S., and Shahzad, S. (2019). Plant beneficial endophytic bacteria: mechanisms, diversity, host range and genetic determinants. Microbiol. Res. 221, 36–49. doi: 10.1016/j.micres.2019.02.001
Ahmad, L., Siddiqui, Z. A., and Abd Allah, E. F. (2019). Effects of interaction of Meloidogyne incognita, Alternaria dauci and Rhizoctonia solani on the growth, chlorophyll, carotenoid and proline contents of carrot in three types of soil. Acta Agric. Scand. Sect. B Soil Plant Sci. 69, 324–331. doi: 10.1080/09064710.2019.1568541
Aswani, V., Rajsheel, P., Bapatla, R. B., Sunil, B., and Raghavendra, A. S. (2019). Oxidative stress induced in chloroplasts or mitochondria promotes proline accumulation in leaves of pea (Pisum sativum): another example of chloroplastmitochondria interactions. Protoplasma 256, 449–457. doi: 10.1007/s00709-018-1306-1
Azimi, F., Oraei, M., Gohari, G., Panahirad, S., and Farmarzi, A. (2021). Chitosan-selenium nanoparticles (Cs–Se NPs) modulate the photosynthesis parameters, antioxidant enzymes activities and essential oils in Dracocephalum moldavica L. under cadmium toxicity stress. Plant Physiol. Biochem. 167, 257–268.
Azizi, M., Fard, E. M., and Ghabooli, M. (2021). Piriformospora indica affect drought tolerance by regulation of genes expression and some morphophysiological parameters in tomato (Solanum lycopersicum L.). Sci. Hortic. 287:110260. doi: 10.1016/j.scienta.2021.110260
Bali, A. S., Sidhu, G. P. S., Kumar, V., and Bhardwaj, R. (2019). “Mitigating cadmium toxicity in plants by phytohormones,” in Cadmium Toxicity and Tolerance in Plants, eds M. Hasanuzzaman, M. N. V. Prasad, and M. Fujita (London: Academic Press), 375–396.
Bates, L. S., Waldren, R. P., and Teare, I. D. (1973). Rapid determination of free proline for water-stress studies. Plant Soil 39, 205–207. doi: 10.1007/BF00018060
Beyer, W. F. Jr., and Fridovich, I. (1987). Assaying for superoxide dismutase activity: some large consequences of minor changes in conditions. Anal. Biochem. 161, 559–566. doi: 10.1016/0003-2697(87)90489-1
Blum, A., and Ebercon, A. (1981). Cell membrane stability as a measure of drought and heat tolerance in wheat 1. Crop Sci. 21, 43–47. doi: 10.2135/cropsci1981.0011183X002100010013x
Bouyoucos, G. J. (1962). Hydrometer method improved for making particle size analyses of soils 1. Agron. J. 54, 464–465. doi: 10.2134/agronj1962.00021962005400050028x
Bradford, M. M. (1976). A rapid and sensitive method for the quantitation of microgram quantities of protein utilizing the principle of protein-dye binding. Anal. Biochem. 72, 248–254. doi: 10.1006/abio.1976.9999
Cavalcanti, F. R., Oliveira, J. T. A. Martins-Miranda, A. S. Viégas, R. A., and Silveira, J. A. G. (2004). Superoxide dismutase, catalase and peroxidase activities do not confer protection against oxidative damage in salt-stressed cowpea leaves. New Phytol. 163, 563–571. doi: 10.1111/j.1469-8137.2004.01139.x
Chen, D., Cao, B., Wang, S., Liu, P., Deng, X., Yin, L., et al. (2016). Silicon moderated the K deficiency by improving the plant-water status in sorghum. Sci. Rep. 6:22882. doi: 10.1038/srep22882
Dias, M. C., Mariz-Ponte, N., and Santos, C. (2019). Lead induces oxidative stress in Pisum sativum plants and changes the levels of phytohormones with antioxidant role. Plant Physiol. Biochem. 137, 121–129. doi: 10.1016/j.plaphy.2019.02.005
Fan, W. J., Feng, Y. X., Li, Y. H., Lin, Y. J., and Yu, X. Z. (2020). Unraveling genes promoting ROS metabolism in subcellular organelles of Oryza sativa in response to trivalent and hexavalent chromium. Sci. Total Environ. 744:140951. doi: 10.1016/j.scitotenv.2020.140951
Ghafariyan, M. H., Malakouti, M. J., Dadpour, M. R., Stroeve, P., and Mahmoudi, M. (2013). Effects of magnetite nanoparticles on soybean chlorophyll. Environ. Sci. Technol. 47, 10645–10652. doi: 10.1021/es402249b
Hasanuzzaman, M., Bhuyan, M. H. M., Nahar, K., Hossain, M., Mahmud, J. A., Hossen, M., et al. (2018). Potassium: a vital regulator of plant responses and tolerance to abiotic stresses. Agronomy 8:31.
Holá, D., Benešová, M., Honnerová, J., Hnilička, F. Rothová, O., Kočová, M., et al. (2010). The evaluation of photosynthetic parameters in maize inbred lines subjected to water deficiency: can these parameters be used for the prediction of performance of hybrid progeny? Photosynthetica 48, 545–558. doi: 10.1007/s11099-010-0072-x
Hussain, A., Ali, S., Rizwan, M. ur Rehman, M. Z., Javed, M. R., Imran, M., et al. (2018). Zinc oxide nanoparticles alter the wheat physiological response and reduce the cadmium uptake by plants. Environ. Pollut. 242, 1518–1526. doi: 10.1016/j.envpol.2018.08.036
Hussain, B., Lin, Q., Hamid, Y., Sanaullah, M., Di, L., Khan, M. B., et al. (2020). Foliage application of selenium and silicon nanoparticles alleviates Cd and Pb toxicity in rice (Oryza sativa L.). Sci. Total Environ. 712:136497. doi: 10.1016/j.scitotenv.2020.136497
Jaiswal, D. K., Verma, J. P., Prakash, S., Meena, V. S., and Meena, R. S. (eds). (2016). “Potassium as an important plant nutrient in sustainable agriculture: a state of the art,” in Potassium Solubilizing Microorganisms for Sustainable Agriculture, (Berlin: Springer), 21–29.
Kah, M., Tufenkji, N., and White, J. C. (2019). Nano-enabled strategies to enhance crop nutrition and protection. Nat. Nanotechnol. 14, 532–540. doi: 10.1038/s41565-019-0439-5
Karimi, R. (2017). Potassium-induced freezing tolerance is associated with endogenous abscisic acid, polyamines and soluble sugars changes in grapevine. Sci. Hortic. 215, 184–194.
Kamran, M., Wang, D., Alhaithloul, H. A. S., Alghanem, S. M., Aftab, T., Xie, K., et al. (2021). Jasmonic acid-mediated enhanced regulation of oxidative, glyoxalase defense system and reduced chromium uptake contributes to alleviation of chromium (VI) toxicity in choysum (Brassica parachinensis L.). Ecotoxicol. Environ. Saf. 208:111758. doi: 10.1016/j.ecoenv.2020.111758
Kaya, C., Akram, N. A., Ashraf, M., Alyemeni, M. N., and Ahmad, P. (2020a). Exogenously supplied silicon (Si) improves cadmium tolerance in pepper (Capsicum annuum L.) by up-regulating the synthesis of nitric oxide and hydrogen sulfide. J. Biotechnol. 316, 35–45. doi: 10.1016/j.jbiotec.2020.04.008
Kaya, C., Ashraf, M., Alyemeni, M. N., and Ahmad, P. (2020b). The role of nitrate reductase in brassinosteroid-induced endogenous nitric oxide generation to improve cadmium stress tolerance of pepper plants by upregulating the ascorbate-glutathione cycle. Ecotoxicol. Environ. Saf. 196:110483. doi: 10.1016/j.ecoenv.2020.110483
Kerchev, P., van der Meer, T., Sujeeth, N., Verlee, A., Stevens, C. V., Van Breusegem, F., et al. (2020). Molecular priming as an approach to induce tolerance against abiotic and oxidative stresses in crop plants. Biotechnol. Adv. 40:107503. doi: 10.1016/j.biotechadv.2019.107503
Kumar, A., and Prasad, M. N. V. (2018). Plant-lead interactions: transport, toxicity, tolerance, and detoxification mechanisms. Ecotoxicol. Environ. Saf. 166, 401–418. doi: 10.1016/j.ecoenv.2018.09.113
Li, M., Zhang, P., Adeel, M., Guo, Z., Chetwynd, A. J., Ma, C., et al. (2021). Physiological impacts of zero valent iron, Fe3O4 and Fe2O3 nanoparticles in rice plants and their potential as Fe fertilizers. Environ. Pollut. 269:116134. doi: 10.1016/j.envpol.2020.116134
Liu, G., Lin, S., Pile, L. S., Fang, Z., and Wang, G. G. (2018). Effect of potassium permanganate and pyrolysis temperature on the biochar produced from rice straw and suitability of biochars for heavy metal (Cd & Pb) immobilization in paper sludge. Fresenius Environ. Bull. 27, 9008–9018.
Liu, Y., Wu, T., White, J. C., and Lin, D. (2021). A new strategy using nanoscale zero-valent iron to simultaneously promote remediation and safe crop production in contaminated soil. Nat. Nanotechnol. 16, 197–205. doi: 10.1038/s41565-020-00803-1
Manzoor, A., Ahmad, T., Bashir, M. A., Hafiz, I. A., and Silvestri, C. (2019). Studies on colchicine induced chromosome doubling for enhancement of quality traits in ornamental plants. Plants 8:194. doi: 10.3390/plants8070194
Mei, X., Li, Q., Wang, H., Fang, H., Chen, H., Chen, X., et al. (2020). Effects of cultivars, water regimes, and growth stages on cadmium accumulation in rice with different radial oxygen loss. Plant Soil 453, 529–543. doi: 10.1007/s11104-020-04634-w
Moradi-Marjaneh, R., Hassanian, S. M., Mehramiz, M., Rezayi, M., Ferns, G. A., Khazaei, M., et al. (2019). Reactive oxygen species in colorectal cancer: the therapeutic impact and its potential roles in tumor progression via perturbation of cellular and physiological dysregulated pathways. J. Cell. Physiol. 234, 10072–10079. doi: 10.1002/jcp.27881
Norvell, W. A., Wu, J., Hopkins, D. G., and Welch, R. M. (2000). Association of cadmium in durum wheat grain with soil chloride and chelate-extractable soil cadmium. Soil Sci. Soc. Am. J. 64, 2162–2168. doi: 10.2136/sssaj2000.6462162x
Okturen Asri, F., and Sonmez, S. (2014). “Effects of potassium and iron applications on nutrient concentrations of tomato plants grown in soilless culture,” in Proceedings of the VI Balkan Symposium on Vegetables and Potatoes, Vol. 1142, Zagreb, 329–334.
Orabi, S. A., and Abou-Hussein, S. D. (2019). Antioxidant defense mechanisms enhance oxidative stress tolerance in plants. A review. Curr. Sci. Int. 8, 565–576.
Page, A. L., Miller, R. H., Keeney, D. R., and Baker, D. E. (1982). Methods of Soil Analysis. II. Chemical and Microbiogical Properties, 2nd Edn. Madison, WI: American Society of Agronomy.
Palansooriya, K. N., Shaheen, S. M., Chen, S. S., Tsang, D. C., Hashimoto, Y., Hou, D., et al. (2020). Soil amendments for immobilization of potentially toxic elements in contaminated soils: a critical review. Environ. Int. 134:105046. doi: 10.1016/j.envint.2019.105046
Palchoudhury, S., Jungjohann, K. L., Weerasena, L., Arabshahi, A., Gharge, U., Albattah, A., et al. (2018). Enhanced legume root growth with pre-soaking in α-Fe 2 O 3 nanoparticle fertilizer. RSC Adv. 8, 24075–24083.
Rady, M. M., Elrys, A. S. El-Maati, M. F. A., and Desoky, E. S. M. (2019). Interplaying roles of silicon and proline effectively improve salt and cadmium stress tolerance in Phaseolus vulgaris plant. Plant Physiol. Biochem. 139, 558–568. doi: 10.1016/j.plaphy.2019.04.025
Raghib, F., Naikoo, M. I., Khan, F. A., Alyemeni, M. N., and Ahmad, P. (2020). Interaction of ZnO nanoparticle and AM fungi mitigates Pb toxicity in wheat by upregulating antioxidants and restricted uptake of Pb. J. Biotechnol. 323, 254–263. doi: 10.1016/j.jbiotec.2020.09.003
Rahman, Z., and Singh, V. P. (2019). The relative impact of toxic heavy metals (THMs)(arsenic (As), cadmium (Cd), chromium (Cr)(VI), mercury (Hg), and lead (Pb)) on the total environment: an overview. Environ. Monit. Assess. 191:419. doi: 10.1007/s10661-019-7528-7
Raza, A., Charagh, S., Zahid, Z., Mubarik, M. S., Javed, R., Siddiqui, M. H., et al. (2021). Jasmonic acid: a key frontier in conferring abiotic stress tolerance in plants. Plant Cell Rep. 40, 1513–1541. doi: 10.1007/s00299-020-02614-z
Raza, A., Habib, M., Kakavand, S. N., Zahid, Z., Zahra, N., Sharif, R., et al. (2020). Phytoremediation of cadmium: physiological, biochemical, and molecular mechanisms. Biology 9:177. doi: 10.3390/biology9070177
Rizwan, M., Ali, S., Ali, B., Adrees, M., Arshad, M., Hussain, A., et al. (2019). Zinc and iron oxide nanoparticles improved the plant growth and reduced the oxidative stress and cadmium concentration in wheat. Chemosphere 214, 269–277. doi: 10.1016/j.chemosphere.2018.09.120
Sadak, M. S., and Bakry, B. A. (2020). Zinc-oxide and nano ZnO oxide effects on growth, some biochemical aspects, yield quantity, and quality of flax (Linum uitatissimum L.) in absence and presence of compost under sandy soil. Bull. Natl. Res. Centre 44, 1–12.
Sardar, R., Ahmed, S., and Yasin, N. A. (2022). Titanium dioxide nanoparticles mitigate cadmium toxicity in Coriandrum sativum L. through modulating antioxidant system, stress markers and reducing cadmium uptake. Environ. Pollut. 292:118373. doi: 10.1016/j.envpol.2021.118373
Seifikalhor, M., Hassani, S. B., and Aliniaeifard, S. (2020). Seed priming by cyanobacteria (Spirulina platensis) and salep gum enhances tolerance of maize plant against cadmium toxicity. J. Plant Growth Regul. 39, 1009–1021. doi: 10.1007/s00344-019-10038-7
Seshadri, B., Bolan, N. S., Wijesekara, H., Kunhikrishnan, A., Thangarajan, R., Qi, F., et al. (2016). Phosphorus-cadmium interactions in paddy soils. Geoderma 270, 43–59. doi: 10.1016/j.geoderma.2015.11.029
Shah, A. A., Ahmed, S., Ali, A., and Yasin, N. A. (2020a). 2-Hydroxymelatonin mitigates cadmium stress in Cucumis sativus seedlings: modulation of antioxidant enzymes and polyamines. Chemosphere 243:125308. doi: 10.1016/j.chemosphere.2019.125308
Shah, A. A., Khan, W. U., Yasin, N. A., Akram, W., Ahmad, A., Abbas, M., et al. (2020b). Butanolide alleviated cadmium stress by improving plant growth, photosynthetic parameters and antioxidant defense system of Brassica oleracea. Chemosphere 261:127728. doi: 10.1016/j.chemosphere.2020.127728
Shamsi, I. H., Jilani, G., Guo-Ping, Z., and Kang, W. (2008). Cadmium stress tolerance through potassium nutrition in soybean. Asian J. Chem. 20:1099.
Sharma, P., Jha, A. B., and Dubey, R. S. (2019). “Oxidative stress and antioxidative defense system in plants growing under abiotic stresses,” in Handbook of Plant and Crop Stress, 4th Edn, ed. M. Pessarakli (Boca Raton, FL: CRC Press), 93–136.
Smart, R. E., and Bingham, G. E. (1974). Rapid estimates of relative water content. Plant Physiol. 53, 258–260. doi: 10.1104/pp.53.2.258
Spormann, S., Soares, C., Teixeira, J., and Fidalgo, F. (2021). Polyamines as key regulatory players in plants under metal stress—A way for an enhanced tolerance. Ann. Appl. Biol. 178, 209–226.
Thind, S., Hussain, I., Ali, S., Rasheed, R., and Ashraf, M. A. (2021). Silicon application modulates growth, physio-chemicals, and antioxidants in wheat (Triticum aestivum L.) exposed to different cadmium regimes. Dose Response 19:15593258211014646. doi: 10.1177/15593258211014646
Toumey, C. (2020). Notes on Environmental Nanoscience. Doctoral dissertation. Berlin: Nature Portfolio.
Ur Rahman, S., Xuebin, Q., Zhao, Z., Du, Z., Imtiaz, M., Mehmood, F., et al. (2021). Alleviatory effects of Silicon on the morphology, physiology, and antioxidative mechanisms of wheat (Triticum aestivum L.) roots under cadmium stress in acidic nutrient solutions. Sci. Rep. 11:1958. doi: 10.1038/s41598-020-80808-x
Usman, M., Farooq, M., Wakeel, A., Nawaz, A., Cheema, S. A., Ur Rehman, H., et al. (2020). Nanotechnology in agriculture: current status, challenges and future opportunities. Sci. Total Environ. 721:137778. doi: 10.1016/j.scitotenv.2020.137778
Wang, B., He, J., Bianchi, V., and Shamsi, S. A. (2009). Combined use of chiral ionic liquid and CD for MEKC: part II. Determination of binding constants. Electrophoresis 30, 2820–2828. doi: 10.1002/elps.200800852
Wang, Y., Jiang, F., Ma, C., Rui, Y., Tsang, D. C., and Xing, B. (2019). Effect of metal oxide nanoparticles on amino acids in wheat grains (Triticum aestivum) in a life cycle study. J. Environ. Manag. 241, 319–327. doi: 10.1016/j.jenvman.2019.04.041
Wang, Z., Yue, L., Dhankher, O. P., and Xing, B. (2020). Nano-enabled improvements of growth and nutritional quality in food plants driven by rhizosphere processes. Environ. Int. 142:105831. doi: 10.1016/j.envint.2020.105831
Xu, X., Du, X., Wang, F., Sha, J., Chen, Q., Tian, G., et al. (2020). Effects of potassium levels on plant growth, accumulation and distribution of carbon, and nitrate metabolism in apple dwarf rootstock seedlings. Front. Plant Sci. 11:904. doi: 10.3389/fpls.2020.00904
Yang, J., Cao, W., and Rui, Y. (2017). Interactions between nanoparticles and plants: phytotoxicity and defense mechanisms. J. Plant Interact. 12, 158–169. doi: 10.1080/17429145.2017.1310944
Yang, J., Jiang, F., Ma, C., Rui, Y., Rui, M., Adeel, M., et al. (2018). Alteration of crop yield and quality of wheat upon exposure to silver nanoparticles in a life cycle study. J. Agric. Food Chem. 66, 2589–2597. doi: 10.1021/acs.jafc.7b04904
Yang, Y., Xiong, J., Tao, L., Cao, Z., Tang, W., Zhang, J., et al. (2020). Regulatory mechanisms of nitrogen (N) on cadmium (Cd) uptake and accumulation in plants: a review. Sci. Total Environ. 708:135186. doi: 10.1016/j.scitotenv.2019.135186
Keywords: growth, potassium, polyamines, antioxidant, stress, cadmium
Citation: Koleva L, Umar A, Yasin NA, Shah AA, Siddiqui MH, Alamri S, Riaz L, Raza A, Javed T and Shabbir Z (2022) Iron Oxide and Silicon Nanoparticles Modulate Mineral Nutrient Homeostasis and Metabolism in Cadmium-Stressed Phaseolus vulgaris. Front. Plant Sci. 13:806781. doi: 10.3389/fpls.2022.806781
Received: 01 November 2021; Accepted: 21 January 2022;
Published: 21 March 2022.
Edited by:
Maurizio Ruzzi, University of Tuscia, ItalyReviewed by:
Parvaiz Ahmad, Government Degree College, Pulwama, IndiaBasharat Ali, University of Agriculture, Faisalabad, Pakistan
Copyright © 2022 Koleva, Umar, Yasin, Shah, Siddiqui, Alamri, Riaz, Raza, Javed and Shabbir. This is an open-access article distributed under the terms of the Creative Commons Attribution License (CC BY). The use, distribution or reproduction in other forums is permitted, provided the original author(s) and the copyright owner(s) are credited and that the original publication in this journal is cited, in accordance with accepted academic practice. No use, distribution or reproduction is permitted which does not comply with these terms.
*Correspondence: Anis Ali Shah, YW5pc2FsaWJvdEBnbWFpbC5jb20=; Manzer H. Siddiqui, bWhzaWRkaXF1aUBrc3UuZWR1LnNh