- 1Unidad de Biotecnología, Instituto Nacional de Investigación Agropecuaria, Canelones, Uruguay
- 2Unidad Mixta Pasteur + INIA (UMPI), Institut Pasteur de Montevideo, Montevideo, Uruguay
- 3Unidad de Protección Vegetal, Instituto Nacional de Investigación Agropecuaria, Canelones, Uruguay
Red-banded stink bug Piezodorus guildinii (P. guildinii) has been described as the most damaging stink bug regarding soybean crops, leading to seed injury, low germination percentages, and foliar retention, at low population densities. In recent years, RNA interference (RNAi), a conserved eukaryote silencing mechanism has been explored to develop species-selective pesticides. In this work, we evaluated RNAi in P. guildinii to develop new pest-control strategies. For this, we assembled and annotated a P. guildinii transcriptome from a pool of all developmental stages. Analysis of this transcriptome led to the identification of 56 genes related to the silencing process encompassing siRNA, miRNA, and piRNA pathways. To evaluate the functionality of RNAi machinery, P. guildinii adults were injected with 28 ng/mg of body weight of double stranded RNA (dsRNA) targeting vATPase A. A mortality of 35 and 51.6% was observed after 7 and 14 days, respectively, and a downregulation of vATPase A gene of 84% 72 h post-injection. In addition, Dicer-2 and Argonaute-2 genes, core RNAi proteins, were upregulated 1.8-fold 48 h after injection. These findings showed for the first time that RNAi is functional in P. guildinii and the silencing of essential genes has a significant effect in adult viability. Taken together, the work reported here shows that RNAi could be an interesting approach for the development of red-banded stink bug control strategies.
Introduction
Soybean (Glycine max) is one of the most extensively grown legume used for protein meal and vegetable oil, with an estimated 6% usage of the worlds’ arable land (Hartman et al., 2011). Soybean cultivation in the United States covers large areas, being the largest producing countries Brazil, the United States, and Argentina, which have an adoption of biotech-enhanced soybean seedstock of more than 90%.1 Phytophagous stink bug complex (Pentatomidae family) is an important sanitary problem of the crop (Zerbino et al., 2016). In particular, red-banded stink bug or small-green stink bug Piezodorus guildinii (Westwood) (P. guildinii) has a Neotropical distribution, spanning from Argentina to the southern United States (Panizzi and Slansky, 1985). Economic damage, estimated at approximately 5% of the harvest, occurs when feeding, from pod formation to maturity (Bundy et al., 2019). The magnitude of the damage depends on the population reached, and the time of exposure to the infestation (Corrêa-Ferreira and De Azevedo, 2002). P. guildinii has been described as one of the most damaging stink bug regarding soybean, leading to reduced yield and quality, affecting seed weight and oil content, delaying crop maturity, and reducing the germination of the harvested seed (Depieri and Panizzi, 2011; Bundy et al., 2019). Current control strategies relay on the application of insecticides based on pyrethroids and neonicotinoids. Pyrethroids act on sucking and chewing insects with tumbling power, while neonicotinoids are highly residual systemic compounds used in sucking insects (Baur et al., 2010; Sosa-Gómez et al., 2020). It has been shown that these insecticides negatively impact natural enemies, are particularly harmful on bees, and have a detrimental effect on the environment (Giorio et al., 2021). Moreover, the lack of options for the control of these insects leads to the use of active principles in the same season and for several years with a similar mode of action, favoring the emergence of resistance (Temple et al., 2013).
RNA interference (RNAi) is a natural gene regulation mechanism present in eukaryotic cells (Svoboda, 2020). In this process, small RNA molecules (sRNA) associate with Argonaute proteins, forming the RNA-induced silencing complex (RISC), which uses complementary base pairing of the sRNA to identify target RNA molecules to be silenced (Ketting, 2011). RNAi pathways differ in the proteins involved, the origin and type of sRNA, the target RNA and, thus, the biological function. Biological roles of RNA silencing pathways include the regulation of endogenous gene expression (miRNA), antiviral immunity (siRNA), and genome protection against transposable elements (piRNA) (Ketting, 2011; Dowling et al., 2016). In siRNA, the basic mechanism consists of a long double stranded RNA (dsRNA) molecule (exogenous or endogenous) which is processed by ribonuclease III type Dicer-2 (Dcr-2) to a small duplex of 21–23 nucleotides. The resulting siRNAs are then loaded into RISC where the duplex is unbounded by the action of Ago-2, the sense strand is degraded, and the antisense strand guides the RISC to the target mRNA. Finally, Ago-2 provides the endonucleolytic activity, silencing gene expression (Mello and Conte, 2004; de Andrade and Hunter, 2016; Svoboda, 2020).
Given the growing need for alternatives to chemical pesticides, the use of RNAi emerges as a highly specific strategy, low environmental impact, and non-transgenic alternative (Christiaens et al., 2020). Reports of successful progress in pest control strategies in insects has been reviewed extensively (Christiaens et al., 2020; Zhu and Palli, 2020; Nitnavare et al., 2021). Nevertheless, the development of effective RNAi strategies in insects is a complex task and there is a great variability response in different insect orders. While coleopterans has shown to be highly susceptible (Baum et al., 2007; Baum and Roberts, 2014), insects from Lepidoptera (Terenius et al., 2011; Kolliopoulou and Swevers, 2014), Diptera (Maktura et al., 2021), and Hemiptera (Jain et al., 2021) respond with greater variability (Cooper et al., 2019). Several factors play a role in this observed differential RNAi efficiency: the presence of dsRNA degrading enzymes in the hemolymph and lumen (Christiaens and Smagghe, 2014; Song et al., 2017), inefficient intracellular transport of dsRNA including entrapment in the endosomes (Shukla et al., 2016; Yoon et al., 2017), or missing core components of the RNAi machinery (Arraes et al., 2021).
Several studies have shown that RNAi is efficient in stink bugs. In the brown marmorated stink bug Halyomorpha halys, administration of dsRNA by injection and feeding showed a reduction in the expression levels and mortality of up to 70% targeting essential genes (Mogilicherla et al., 2018). In the Neotropical brown stink bug Euschistus heros, RNAi core proteins were identified in the transcriptome, and adult injection of dsRNA targeting vATPase A caused a reduction in vATPase A gene expression and significant mortality (Cagliari et al., 2020). Moreover, the insecticidal effect with the same target in second instar nymphs reached 80% of mortality 14 days after injection. In feeding assays, the formulation with ethylenediaminetetraacetic acid (EDTA) or Liposome encapsulation enhanced the mortality up to 45 and 51%, respectively, compared with naked dsRNA against vATPae A, where no significant mortality was reported (Castellanos et al., 2019). In addition, a complex of a shRNA against troponin coupled to nanoparticles showed significant mortality by oral administration (Laisney et al., 2021). Studies in southern green stink bug Nezara viridula showed that injected dsRNA targeting essential genes could induce significant mortality (Riga et al., 2020; Gurusamy et al., 2021). Particularly, dsRNA targeting vATPase A achieved more than 80% mortality in injection assays, and 45% when feeding. This response was enhanced when specific N. viridula dsRNases were silenced by injection before oral administration in second instar nymphs targeting the αcop gene (Sharma et al., 2020, 2021). In the harlequin bug, Murgantia histrionica (Hahn), RNAi core proteins were identified and significant mortality was shown by injection and feeding targeting several genes (Howell et al., 2020). Finally, the brown-winged green stinkbug Plautia stali was very sensitive to vATPase E dsRNA injection, but a lower response was reported by oral feeding (Nishide et al., 2021).
In this work, we explored RNAi in P. guildinii towards the development of environmentally low impact pest control strategies. For this, we generated, to our knowledge, the first transcriptome of this stink bug species. Machinery RNAi genes were annotated, finding core RNAi genes, RISC associated genes, uptake, and intracellular transport related genes as well as RNAases. We demonstrated RNAi in vivo by dsRNA injection targeting vATPase A. Gene expression was significantly reduced in treated animals, and a 51.6% mortality rate was observed after 14 days. The gene expression of core proteins after injection in dsRNA treated animals was evaluated as well, with a significant overexpression of Dcr-2 and Ago-2 48 h after injection. These results showed that P. guildinii is susceptible to RNAi, and this approach could be exploited for the development of integrated pest control strategies.
Materials and Methods
Insect Rearing
Adults P. guildinii were collected in INIA La Estanzuela, Colonia, Uruguay (S34° 20′W 57°41′) and maintained at 26 ± 1°C, 80 ± 10% RH, and a 16:8 light:dark cycle. Insects were fed ad livitum with green bean pods (Phaseolus vulgaris), dry soybean seeds (G. max), and raw shelled peanuts (Arachis hypogaea), distilled water was supplied every day by moistened cotton. Eggs were removed to a different plastic container and nymphs were checked daily until adult emergence.
cDNA Libraries and Sequencing
Total RNA was purified from all nymphal stages (1st–5th) and adults (male and female) using an RNeasy Mini Kit Qiagen (Hilden, Germany), according to manufacturer instructions. RNA integrity was determined with a Bioanalyzer 2100 Agilent Technologies (Santa Clara, United States). Equal RNA quantity of every stage was pooled to a 1 μg sample used for cDNA library preparation and Illumina (San Diego, United States) sequencing was conducted by Macrogen, Inc. (Seoul, South Korea). TruSeq stranded mRNA libraries were generated with a TruSeq Stranded mRNA LT Sample Prep Kit Ilumina (San Diego, United States), and sequencing was performed with Illumina HiSeq 2500 platform with a coverage of 75 G (1 lane), 150 bp paired ends reads.
Transcriptome Assembly
The quality of raw reads from the Illumina sequencing was analyzed by FastQC software2 and filtered as follows: erroneous k-mers were eliminated with r-Corrector software (Song and Florea, 2015), adaptors and bases with a Phred score lower than 30 were trimmed with TrimmGalore.3 Additionally, reads were mapped against SILVA (LSU/SSU) Database4 using Bowtie25 to eliminate rRNA contaminant reads. The filtered reads were de novo assembled using Trinity software6 with default parameters, using a de Bruijn graph algorithm and a k-mer length of 25. The quality of transcriptome assembly was evaluated by mapping the reads over the assembled contigs with bowtie2. Completeness was analyzed with Benchmarking Universal Single-Copy Orthologs (BUSCO) using the Arthropoda odb9 database,7 and the number of fully reconstructed coding transcripts was evaluated by a BLASTx search against Swiss-Prot database with a cut off E-value ≤ 1e-20. All raw reads have been deposited in the sequence reads archive (SRA) at National Center for Biotechnology Information (NCBI), and may be accessed using the access code PRJNA772728.
Homology Search and Annotation
The generated contigs were analyzed by searching homology with a BLASTx tool against public databases, such as the non-redundant protein database (nr) filtered by Insecta Taxa (NCBI), Uni-Prot TrEMBL, and Swiss-Prot with a cut off E-value ≤ 1e-5. InterproScan available in OmicsBox software8 was used for conserved domains search (Zdobnov and Apweiler, 2001). Gene ontology (GO) categories were assigned from pooled BLAST and InterPro hits according to the pipeline available in the Blast2Go software (Götz et al., 2008).
RNAi Related Genes
Genes related to RNAi machinery classified as core RNAi (Table 1), auxiliary RISC factors (Table 2), uptake, nucleases, antiviral, and intracellular transport proteins (Table 3) previously described were used as reference for the search in the P. guildinii transcriptome (Prentice et al., 2015; Taning et al., 2016; Cagliari et al., 2020). Homologous sequences for these proteins were obtained from NCBI and used as queries in a tBLASTn search with a cut off E-value < 1e-5. Hits with the lowest E-value were further analyzed to confirm their identity. An ORF Finder tool from NCBI9 was used to predict Open Reading Frames and protein domains were predicted by the NCBI Conserved Domains Database.10 A BLASTp search against non-redundant protein database at NCBI was performed as well. To provide additional confirmation on the identity of core RNAi proteins, a phylogenetic analysis was performed. Proteins sequences from different insect orders were aligned using MUSCLE program from MEGA 7.0.26, and neighbor-joining algorithm with 1,000 bootstrap replicates was used to predict phylogeny.
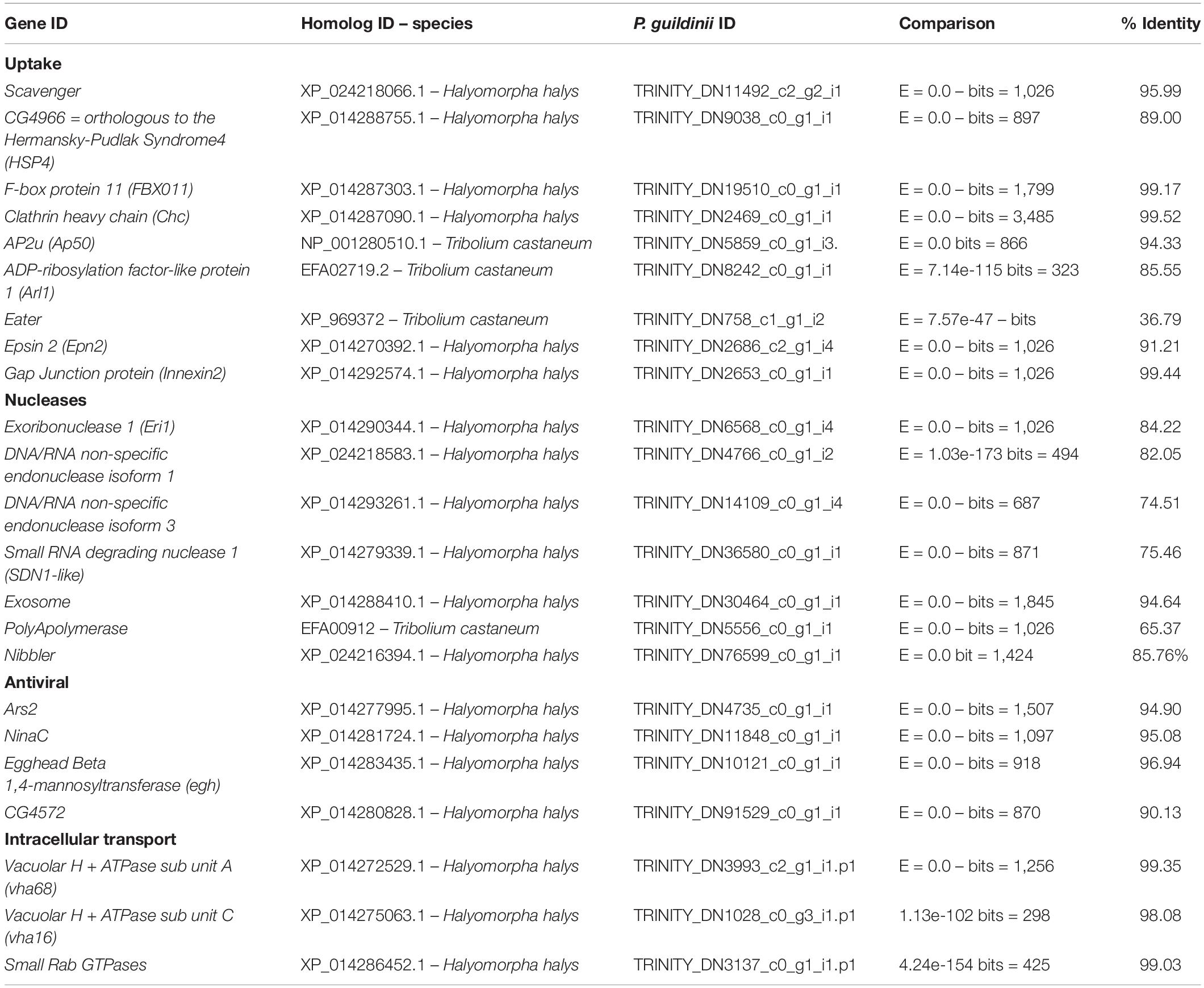
Table 3. Uptake, nucleases, antiviral, and intracellular transport genes identified in Piezodorus guildinii transcriptome.
dsRNA Synthesis and Purification
Piezodorus guildinii transcriptome was screened for vATPAase A nucleotide sequence using as a query H. halys protein sequence XM_014417043.2 (Table 3). A 300 bp sequence showing low cross-reactivity to other organisms evaluated by BLASTp against nr-NCBI database was selected. A 496 bp green fluorescent protein (GFP) dsRNA was designed from pRFHUE-eGFP plasmid Addgene (Watertown, United States). Plasmid DNA was purified from transformed Escherichia coli DH5α cells according to Green and Sambrook (2016) protocol. Specific primers were designed to amplify DNA templates that included T7 promoter sequence placed at the 5′-end of both forward and reverse primer to enable in vitro transcription (Table 4). Further, 1 μg of total RNA from adults P. guildinii was used as template to synthesize cDNA and oligodT primer using SuperScript™ IV Reverse Transcriptase Thermo Fischer Scientific (Waltham, United States), according to instructions from manufacturer. Templates were amplified from cDNA by PCR with 2.5 μl of cDNA template, 5 μl of 10× Buffer Thermo Scientific (Waltham, United States), 1.8 mM MgCl2, 0.25 mM DNTPs, 0.25 mM of each primer, and 2.5 U of Taq Polymerase Thermo Scientific (Waltham, United States) in a 50 μl reaction. PCR cycle consisted of 5 min at 94°C, 35 cycles of 30 s at 94°C, 30 s at 58°C, 45 s at 72°C, and a final extension of 10 min at 72°C. PCR products were purified by isopropanol precipitation, quantified by Nanodrop 8000 Thermo (Waltham, United States), and analyzed by agarose 1.5% gel electrophoresis. Sequences were confirmed by Sanger sequencing in Macrogen Inc. (Seoul, South Korea). DsRNAs were synthesized using a MEGAScript kit Thermo Fisher Scientific (Waltham, United States) from 1.2 μg of PCR product in a 16 h reaction and then purified by phenol:chloroform extraction following the manufacturer’s instructions. Integrity was evaluated by agarose 1.5% gel electrophoresis, and, finally, concentration was determined in a Nanodrop 8000 Thermo (Waltham, United States). Aliquots were stored at −80°C.
Adult dsRNA Injection
RNA interference in P. guildinii was evaluated by injection of 1.2 μg of dsRNA in adults (28 ng/mg of insect, average adult weight ∼45 mg) based on previous reports (Castellanos et al., 2019; Cagliari et al., 2020; Sharma et al., 2020). A 300 bp dsRNA targeting vATPase A was used to evaluate silencing and a 496 bp GFP dsRNA was used as control. Adults were anesthetized by incubation on ice for 5 min and then placed with the ventral side up under a binocular loupe (2×) (Supplementary Figure 3). A volume of 0.5 μl of dsRNA (2.4 μg/μl) was injected in the ventral septum between the thoracic and abdominal segments using a 25 μl Hamilton syringe with a 33G needle coupled to a PB600 repeater Hamilton (Reno, United States). Control group was injected with water. After injections, insects were placed in plastic plates containing green beans, peanut, and water ad libitum, and kept in the conditions previously described. For survival assays 30 adults were used per group, and mortality was evaluated every day for 14 days. Each assay was repeated two times. Survival curves were compared using the log-rank test (p < 0.01). For gene silence evaluation by real-time quantitative PCR (RT-qPCR), 27 adults were injected per group, and the pools of three individuals were processed at 24, 48, and 72 h post-injection by triplicate.
Real-Time Quantitative PCR
Total RNA was purified from insect samples corresponding to 24, 48, and 72 h post-injection using TRIzol Reagent Ambion (Austin, United States), following manufacturer’s instructions. RNA was quantified using a Nanodrop 8000 (Thermo) and quality was evaluated by 1.5% agarose gel electrophoresis. Then, 1 μg of total RNA was treated with DNAase I NZY (Lisbon, Portugal) and used as template to synthesize cDNA, as described. Primers were designed using Primer-Blast from NCBI based on Primer3 software,11 and were validated with a standard curve based on serial dilution of cDNA to determine amplification efficiency, calculated as E = [10^(−1/slope) − 1] × 100 (Table 4). A melting curve analysis with temperature range from 60 to 95°C and agarose gel electrophoresis confirmed primer specificity. RT-qPCRs were performed in a QuantStudioTM 3 Real-Time PCR System (Applied Biosystems) in 96 well plates. Each reaction included 2 μl of cDNA dilution, 5 μl iQ SYBR Green Supermix 2X Bio-Rad (Hercules, United States), and 0.25 mM of each primer in a 10 μl reaction. Amplification conditions were 10 min at 95°C, 40 cycles of 15 s at 95°C, and 1 min at 60°C, and a melting curve stage from 60 to 90°C. Data were analyzed using the Quant Studio design and analysis software v1.4.3. As endogenous control, 18S ribosomal protein (18S) and 60S ribosomal protein (60S) described previously for H. halys (Mogilicherla et al., 2018) were checked for stability with RefFinder software (Xie et al., 2012) and used for data normalization. Relative gene expression was calculated using the equation 2(–ΔΔCt) (Livak and Schmittgen, 2001). Normal distribution of the data was checked with Shapiro–Wilk test and Levene’s test to compare variances. The statistical significance was analyzed by an un-paired t-test (p < 0.05, n = 3) using R-studio.
Results
Piezodorus guildinii Transcriptome
Illumina sequencing led to 520,093,434 raw reads, corresponding to 260,046,717 unprocessed pairs with a GC content of 41.88%. Through the quality filtering process, 50,599,592 reads (19.4%) were eliminated (Supplementary Table 1). The de novo assembly of the P. guildinii transcriptome was performed with filtered reads resulting in 172,298 transcripts that correspond to 119,178 uni-genes. The assembled transcripts’ GC content was 35.57% and the average contig size was 750 bp. Assembly quality, as evaluated by mapping the filtered reads against the assembled transcripts, indicated that 97.49% of the paired reads were included in the assembly. Completeness of the transcriptome evaluated by BUSCO against an Arthropoda database showed that five out of 1,066 genes (0.5%) were not identified (Supplementary Table 1). Finally, the number of fully reconstructed transcripts was evaluated by means of a BLASTx search against the Swiss-Prot database, showing that 4,499 hits presented coverage greater than or equal to 90%.
The functional annotation of transcripts was performed by BLASTx, 55,706 hits (36.8%) were obtained against an Insecta subset of nr-NCBI protein database, 33,490 against Swiss-Prot (22%), while the BLASTx using insect TrEMBLE database yielded 52,721 matches (36.8%). Taking together, 58,922 hits were obtained, corresponding to 39% of the transcriptome (Figure 1A). Interestingly, when databases are compared, 53.60% of transcripts were identified by all three. Analysis of the sequences identified by nr-NCBI insect database showed that 76.8% of the hits corresponded to Hemiptera order as follows; Pentatomidae: 65% (H. halys, N. viridula), Aphidae: 4% (Aphis craccivora, Aphis glycines), Delphacidae: 2.16% (Nilaparvata lugens, Laodelphax striatellus), Miridae: 2.08% (Apolygus lucorum, Nesidiocoris tenterauis), Hemicidae: 1.79% (Cimex lectularius), and Psylliae: 0.8% (Diaphorina citri). The remaining hits were distributed among species of the orders Hymenoptera 3.9%, Coleoptera 2.4%, Lepidoptera 2.16%, and Diptera 1.19% (Figure 1B and Supplementary Table 2). It should be noted that BLAST analysis is based on sequence homology, which is dependent on the database used and does not imply phylogenetic relationships. Additionally, InterPro search identified 41,172 hits (27.2%). Sequences identified by both BLASTx and InterPro were used for the assignment of GO terms. A total of 42,316 terms were obtained, 54.5% from the Molecular Function category (MF), 30.5% from the Biological Process category (BP), and 15.02% from the Cellular Component category (CC). The 10 main components from each category are detailed in Figure 1C.
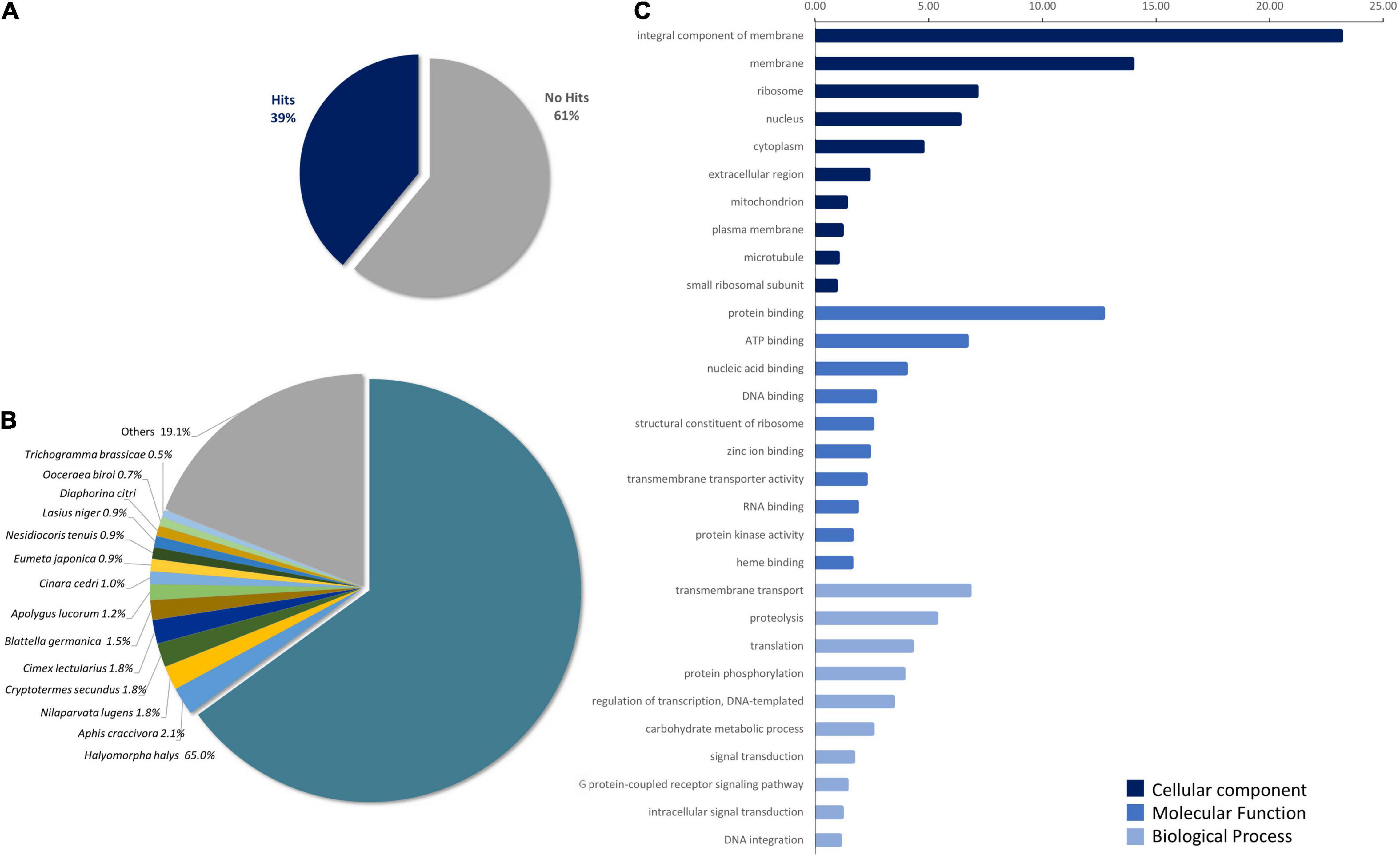
Figure 1. Piezodorus guildinii transcriptome homology analysis. (A) Percentage of hits identified by BLASTx in P. guildinii transcriptome against nr-NCBI, Swiss-Prot, and TrEMBLE databases (cutoff E-Value < 10e-5). (B) Species distribution of transcripts with known function, hits from nr-NCBI database. (C) Gene Ontology (GO) terms in P. guildini transcriptome. Percentage distribution of 10 principal GO terms classified in Molecular Function (MF), Biological Process (BP), and Cellular Component (CC) assigned to P. guildinii contigs. Analysis was performed using hits from BLASTx and InterProScan using OmicsBox software.
Identification of RNAi-Related Genes
To establish RNAi strategies as a tool to control the red banded stink bug, the assembled transcriptome was used to annotate contigs related to RNAi machinery by tBLASTn. A total of 56 contigs were identified and are detailed in Tables 1–3 and Supplementary Material.
Core RNAi machinery genes related to miRNA, siRNA, and piRNA pathways were predicted in the P. guildinii transcriptome (Table 1 and Supplementary Data 1). In relation to Dicer proteins, in the miRNA pathway, only a partial sequence of Dcr-1 was found, consisting in two RNAase III domains and a Double stranded RNA-binding domain (dsRBD). On the other hand, Dcr-2 from siRNA pathways contained all the conserved domains (an N-terminal helicase domain, a PAZ domain, two RNAase III domains, and a dsRBD), while Drosha protein sequence was found with two Ribonuclease III family domain and a dsRBD. To further analyze the identified sequences, a phylogenetic analysis was performed with Dicer sequences from the different orders of insects (Supplementary Figure 1). Three main clades were inferred, one containing Dcr-1, another with Dcr-2, and the third one with Drosha sequences. In all cases, P. guildinii proteins were grouped with Hemiptera Pentatomidae sequences from H. halys, N. viridula, and E. heros. Within Dcr-1 subclade, P. guildinii Dcr-1 was grouped with N. viridula, while for Dcr-2, P. guildinii was grouped with E. heros. Drosha protein from P. guildinii was found in a clade with E. heros and H. halys. Co-factors Pasha, Loquacious, and R2D2 were identified with two dsRBD conserved domains. Regarding Argonaute, Ago-1, Ago-2, Ago-3, Piwi, and Aubergine were identified, all presented conserved domains PAZ and PIWI (Supplementary Data 1). Argonaute protein phylogeny showed three main clades, separating AGO subfamily (Ago-1 and Ago-2), Ago-3, and another clade with Aubergine and Piwi. In Ago subfamily, P. guildinii Ago-1 was grouped with E. heros, H. halys, and N. viridula, but in a different final branch, showing minor differences. Regarding Ago-2, P. guildinii protein was clustered with N. viridula and H. halys. In the PIWI subfamily, P. guildinii Ago-3 was clustered with E. heros and H. halys, while Piwi and Aubergine were clustered with E. heros (Supplementary Figure 2). In addition, the endoribonuclease Zucchini, essential for the primary piRNA biogenesis and Exportin5, a protein related to the nuclear export of pre-miRNAs was also identified.
The presence of auxiliary factors of the RISC complex was examined. Overall, 20 sequences were identified related to intracellular factors that are associated with the activity of the RISC complex (Table 2). Holo-RISC related proteins Tudor-SN, Vasa-intronic gene, and fragile X related protein 1 were annotated with all conserved domains predicted. Translin and Translin-associated factor X (TRAX) components of C3P0 were also found. Additionally, nucleases involved in piRNA biogenesis, Armitage, Homeless (splindle-E), and Maesltrom were present as well. As detailed in Table 2, other contigs identified were GLD-1 homolog, Aco-1, Elp-1, RNA Belle helicase, Gawky, Staufen, helicase p68, and Clp-1. Partial sequences were identified from Hen-1 metyltransferase, without a dsRBD2 nor methyltransferase conserved domains, PRP16 helicase sequence lacked one DEAH Helicase- domain, and Arginine methyltransferase 7 did not present any SAM-dependent methyltransferase PRMT-type domain.
Nine proteins involved in dsRNA uptake were identified in P. guildinii transcriptome, as shown in Table 3. Clathrin heavy chain, Clathrin adaptor AP50, Epsin-2, and HSP4 related to receptor mediated endocytosis were found. In addition, three proteins related to intracellular vesicle transport were also annotated, Vacuolar H + ATPase subunit A (vha68), Vacuolar H + ATPase subunit C (vha16), and Small Rab GTPases. Particularly, Vacuolar H + ATPase subunit A (vATPase A) was used as a target to evaluate RNAi response in P. guildinii. Putative dsRNA receptors Scavenger and Eater were described as well. Interestingly, transcripts coding for sid-like proteins were not found.
Regarding RNAi related nucleases, Eri-1, SDN1-like, and Nibble were found and all conserved domains were predicted. Two DNA/RNA endonucleases isoforms were found, but only isoform 1 contained a nuclease conserved domain (Supplementary Data 1). In addition, Exosome complex exonuclease and Poly(A)polymerase were described.
Antiviral RNAi related genes Ars2 involved in RISC complex regulation, Egghead, a transmembrane-domain glycosyltransferase and ninaC, a protein related to vesicle transport, were identified as shown in Table 3.
dsRNA Injection in Piezodorus guildinii
The effect of dsRNA targeting vATPase A was studied by injection in P. guildinii adults. Significant differences were observed in the survival rates between insects treated with vATPase A, control GFP, or water assessed by log-rank test (χ2 = 42.76, df = 2 p < 0.0001). In vATPase A group, as shown in Figure 2, treated insects mortality was 35% after 7 days post-injection, reaching 51.6% after 14 days, while control groups behaved similarly. In GFP group, mortality ranged 10% on day 7 and 14% at the end of the assay; while in water injected insects, mortality was 11.5 and 15.5% after 7 and 14 days, respectively, as shown in Figure 2.
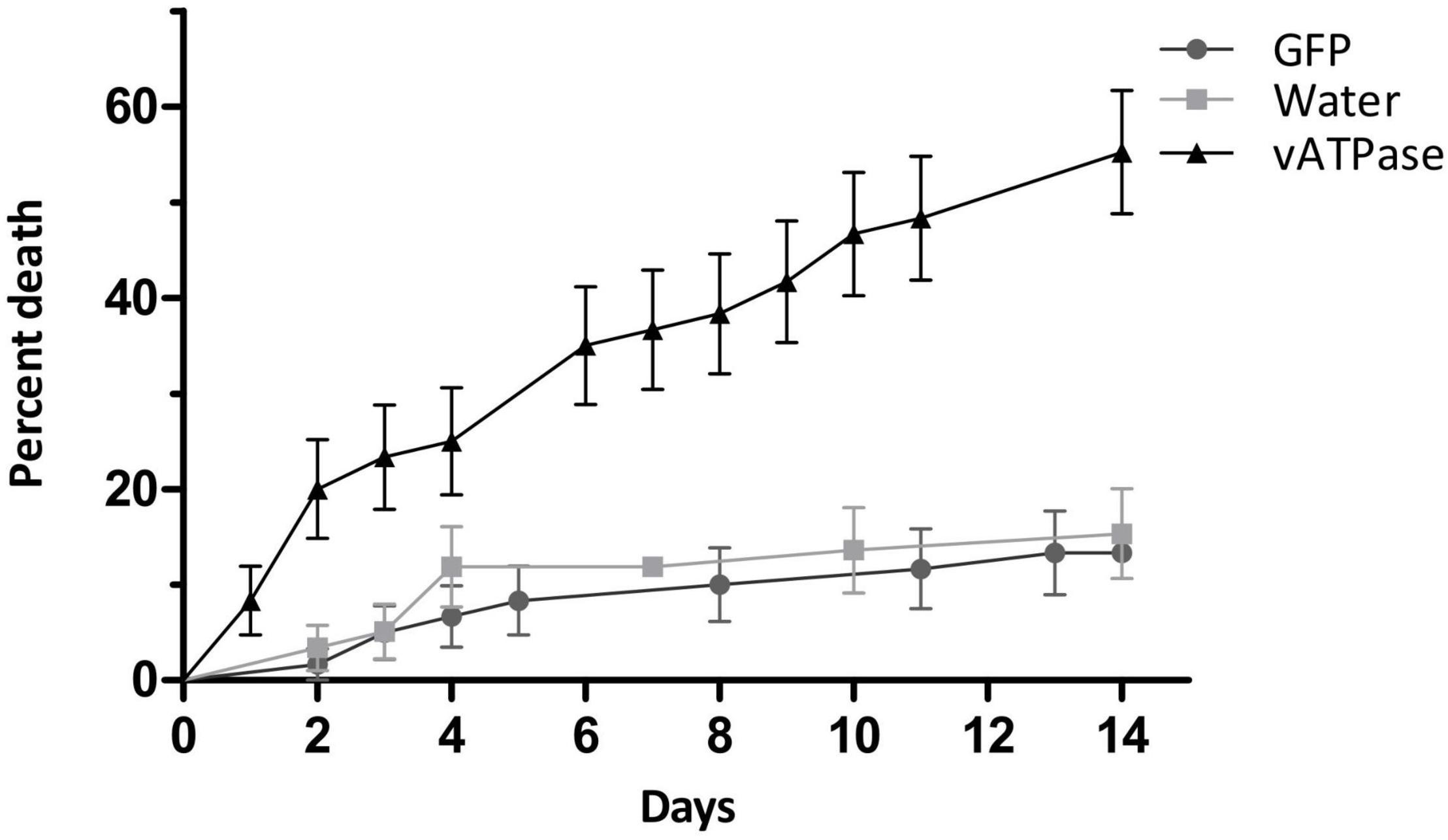
Figure 2. dsRNA injection in P. guildinii. Cumulative mortality expressed as percentage in P. guildinii adults after injection with dsRNA (28 ng/μl per mg of body weight) targeting vATPase A, green fluorescent protein (GFP), or water. Error bars represent SE from two independent assays.
RT-qPCR in Injection Assays
To confirm vATPase A gene silencing after dsRNA injection, RT-qPCR analysis was performed using cDNA from treated insects. The vATPase A transcript level showed a significant reduction of 64.4% at 24 h after injection (p = 0.025), at 48 h, gene expression decreased 74% (p = 0.019), and 84% of reduction (p = 0.002) at 72 h compared with control samples injected with dsGFP (Figure 3A).
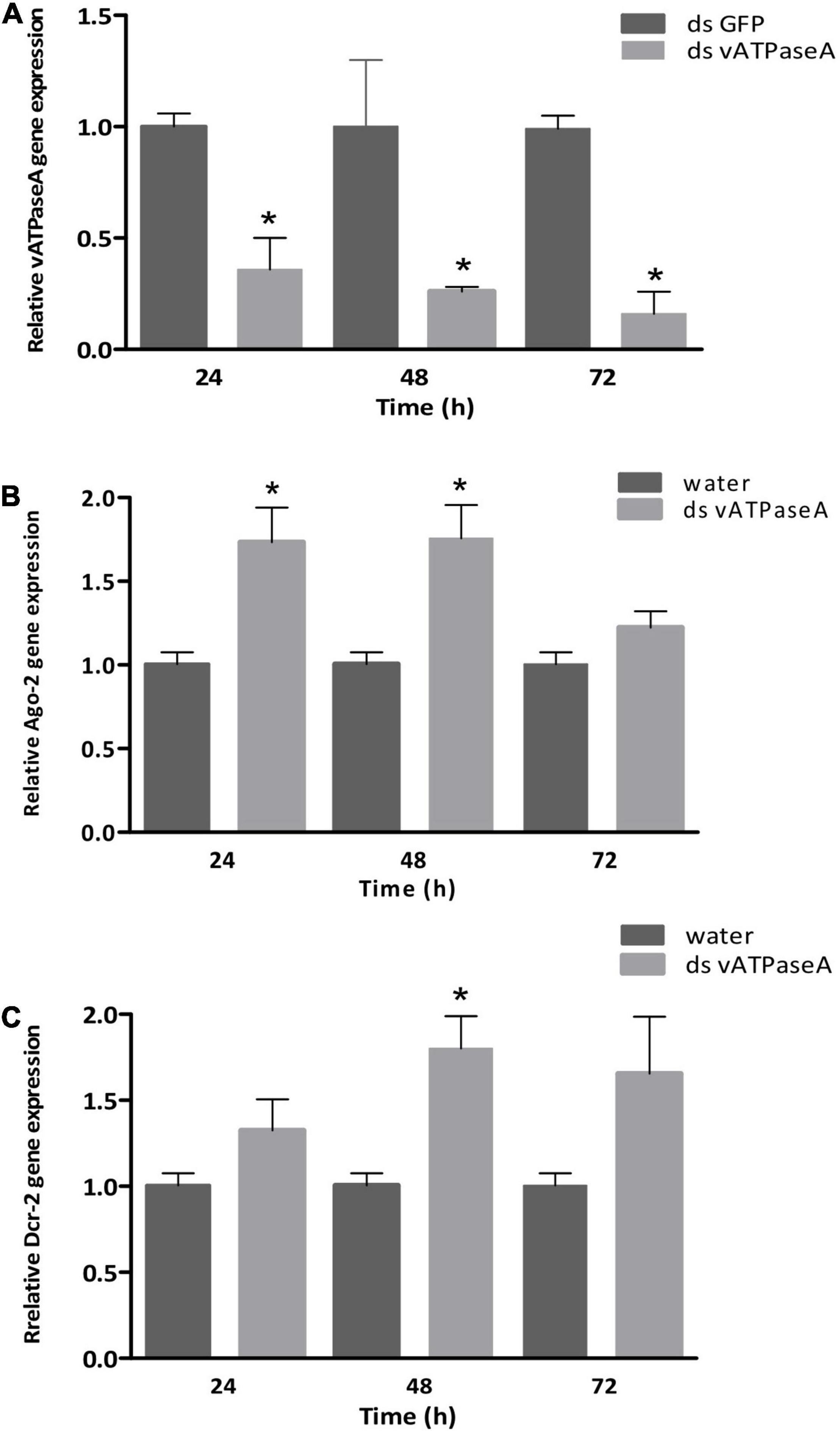
Figure 3. Effect of dsRNA injection on gene expression by RT-qPCR in P. guildinii. Adults were injected with 28 ng/μl per mg of body weight with dsRNA targeting vATPase A or GFP used as a negative control. Adults were sampled at 24, 48, and 72 h post-injection in both treatments. As internal control, 18S and 60S genes were used. (A) vATPase A relative expression, GFP treated insects were used for normalization. (B) Ago-2 relative gene expression in dsRNA treated insects in comparison with water-treated insects. (C) Dcr-2 relative expression of insects treated with ds-vATPase A; water-treated insects were used as control. Bars represent mean and SEM based on three biological repeats consisting of a pool of three insects each. Statistical significance was calculated by an unpaired t-test. *p ≤ 0.05.
To assess the involvement of the siRNA machinery following dsRNA injection, the differential expression of the Dcr-2 and Ago-2 genes was analyzed by RT-qPCR. Argonaute-2 showed a significant overexpression of 70 and 80% at 24 and 48 h, respectively, (p = 0.02); at 72 h, Ago-2 expression was 1.3-fold, which was not statistically significant (Figure 3B). For Dcr-2, after 24 h a 30% overexpression was observed, but showed no statistical significance, while at 48 h, a 1.8-fold expression of Dcr-2 was significantly different compared with control group (p = 0.04). A 1.6-fold expression was maintained at 72 h, even though this difference was not statistically significant (Figure 3C).
Discussion
To evaluate the potential of RNAi as a tool in the control of the red-banded stink bug, we first generated a P. guildinii transcriptome, since little sequence information was available for this species. This approach allowed to get insight into the RNAi gene machinery and to gather information about possible target genes and housekeeping genes sequences. Recently, a complete genome assembly of P. guildinii has been reported (Perera, 2021-preprint). Taken together, these data will generate complementary information that will broaden the knowledge in the biology of this insect species. Homology analysis showed a 39% of contigs of known functions, revealing that P. guildinii transcriptome contains a high percentage of genes with non-assigned functions, a similar proportion of what was reported in E. heros transcriptome (Cagliari et al., 2020). The stink bug H. halys showed the highest homology from the identified transcripts, covering 65% of the hits; this could be explained by database coverage rather than phylogenetical homology (Ioannidis et al., 2014).
Differences in RNAi efficacy among insects can be partially explained by the diversity in the RNAi pathway genes present in different lineages (Dowling et al., 2016). To increase our understanding about P. guildinii RNAi response, we first analyzed the transcriptome for the annotation of genes related to this process. As shown in Tables 1–3, we were able to identify 56 sequences. Core components of the RNAi machinery with small RNA fragments are the main effectors in gene silencing. They can be divided into three pathways based on Dicers, Argonautes, and small RNAs involved. The siRNA pathway is activated by long exogenous or endogenous dsRNAs and involves Dicer-2 (Dcr-2), co-factor R2D2, and Argonaute-2 (Ago-2). The miRNA pathway involves miRNA from endogenous transcripts, nuclear Dicer, Drosha and co-factor Pasha, cytoplasmic Dicer-1 (Dcr-1), co-factor Loquatios, and Argonaute-1 (Ago-1). Finally, the piRNA pathway is germline-specific, derived from single-stranded RNA (ssRNA) and independent of Dicer proteins. It is characterized by endonuclease Zucchini (Zuc) and Argonaute proteins of the PIWI class: Aubergine, Piwi, and Argonaute-3 (Ago-3) (Ambrus and Frolov, 2009; Zhu and Palli, 2020). All the above-mentioned core proteins were identified in P. guildinii transcriptome (Table 1 and Supplementary Data 1). Particularly, Dicer-1 lacked the N-terminal helicase and PAZ domain. Functional Dcr-1 lacking the N-terminal helicase domain has been reported previously in D. melanoganster (Lee et al., 2004; Kavi et al., 2008). In P. guildinii, Dicer-1 resembled nuclear RNAase III Drosha protein (two RNAse III domains and a carboxyterminal dsRBD). It would be interesting to further study if this Dicer-1 is functional in P. guildinii miRNA pathway. The phylogenetic analysis of these protein sequences (Supplementary Figures 1, 2) further validated the identity of the annotated transcripts. P. guildinii proteins were grouped with Hempitera:Pentatomidae species E. heros, N. viridula, and H. halys. Pasha, Loquacious, and R2D2 dsRNA binding proteins, co-factors of Drosha, Dicer-1, and Dicer-2, respectively, were also found with all their conserved domains. Although the structure of Ago proteins is highly conserved, the number of Ago proteins varies between different species from 1 in the fission yeast Schizosaccharomyces pombe to 27 in the nematode worm Caenorhabditis elegans (Höck and Meister, 2008; Meister, 2013). In insects, five Argonautes were reported for D. melanoganster, T. castaneum, Cylas puncticollis, and among others. Five members belonging to the Argonaute superfamily were identified in P. guildinii as well (Table 1). Argonaute subfamily: Ago-1 and Ago-2; and PIWI subfamily: Ago-3, Aubergine, and Piwi. In all cases, conserved PAZ and Piwi domains were predicted. Interestingly, the phylogenetic analysis showed that identified sequences were grouped within Hemiptera:Pentatomidae species clades with a common ancestor (Supplementary Figure 2). In addition, the Zucchini endonuclease involved in piRNA maturation was also annotated. RdRP protein that can amplify synthesizing small RNAs or dsRNAs on targeted RNA templates was not found. This is consistent with previous reports that indicate that no homologs of this protein have been described in vertebrate or insect genomes (Maida and Masutomi, 2011).
We then analyzed auxiliary RISC factors associated with the activity of the RISC complex. In siRNA described in D. melanoganster, Holo-RISC or the mature form of RISC is composed by Dcr-2 and R2D2 (components of the RISC Loading Complex), proteins Ago-2, Tudor-SN (Gutierrez-Beltran et al., 2016), Vasa intronic gene, Fragile X related protein (FXMR1) (Caudy et al., 2002), and C3P0, an heterodimer of Translin and Trax proteins (Kavi et al., 2008; Liu et al., 2009). Sequences for all the proteins mentioned were identified in P. guildinii transcriptome with their conserved domains predicted as shown in Table 2. In addition, Clp-1 kinase related to siRNAs phosphorylation before RISC loading was also annotated (Davies and Samuels, 2010). Helicases play different functions in the silencing processes, from unwinding RNA duplexes to proper RNA loading to RISC (Ambrus and Frolov, 2009). Helicases Belle, Armitage, p68 RNA helicase, Gemin 3, and a partial sequence of PRP16 were identified in P. guildinii. In addition, Piwi pathway related proteins Spindle E, Maelstrom, Armitage, methyltransferases PRMT, and a partial sequence of Hen-1 were also found. Other auxiliary factors, such as Elp-1, a component of pol II core elongation complex, Staufen, GLD-1, and ACO-1 were present as well.
Internalization of dsRNA into cells is an essential step for the generation of RNAi. Two different pathways have been described for dsRNA uptake in insects, Sid-1 channel protein-mediated pathway and the endocytic pathway (Joga et al., 2016). The first pathway, described in C. elegans, involves transmembrane proteins SID-1 and SID-2 (Hunter et al., 2006). Orthologous proteins to C. elegans SID-1, called SID-like proteins (SIL-A, SIL-B, and SIL-C), have been identified in several insect species, although their direct involvement in dsRNA uptake is still not clear (Vélez and Fishilevich, 2018). SID-2 has not been reported in any insect order (Hinas et al., 2012). In P. guildinii transcriptome, SilA, SilB, and SilC homologous sequences were absent (Table 3), similarly in Diptera, where no SID-like genes have been reported, and more recently in the Hemiptera E. heros transcriptome (Tomoyasu et al., 2008; Huvenne and Smagghe, 2010; Cagliari et al., 2020). The other uptake pathway described is Clathrin-mediated endocytosis. The sequences identified in the present transcriptome encompassed the endocytic pathway (Table 3 and Supplementary Material), from early vesicle formation, Clathrin heavy chain (Chc), Clathrin adapter protein AP50, to the late endosomal formation and release, HPS4, Rab7, endosome acidification proteins: Vacuolar H + ATPase sub unit A (vha68) and Vacuolar H + ATPase sub unit C (vha16), as reported previously (Vélez and Fishilevich, 2018). These findings suggest that besides the absence of SID-like proteins, dsRNA uptake could be mediated by endocytosis in P. guildinii. In Drosophila S2 cells, scavenger receptors, SR-CI and Eater, account for more than 90% of dsRNA uptake (Saleh et al., 2006; Ulvila et al., 2006). A scavenger receptor class B and Eater sequence was annotated in P. guildinii (Table 3 and Supplementary Material); further functional evaluation of these proteins would be needed to determine if they have an active role in dsRNA uptake.
A rapid degradation of dsRNA by dsRNases causes a reduction in RNAi response (Cooper et al., 2019). We described the presence of seven nucleases in the transcriptome of P. guildinii (Table 3 and Supplementary Material): Eri-1, an evolutionarily conserved 3′–5′ exoribonuclease related in siRNA and miRNA pathways (Kennedy et al., 2004; Thomas et al., 2014), Sdn1-like protein, a 3′–5′ exonuclease related to the degradation of mature miRNA (Ramachandran and Chen, 2008). In addition, Nibble, a 3′–5′ exoribonuclease described in Drosophila to process 3′-end trimming in miRNA pathway (Han et al., 2011) and a Poly(A)polymerase, involved in the mRNA degradation (Yamanaka et al., 2013). Most insects possess 2–4 dsRNase genes, as revealed by genome-wide analysis (Cooper et al., 2019). In P. guildinii transcriptome, we found two sequences, but only isoform 1 showed a nuclease conserved domain (Table 3 and Supplementary Material). Exosome complex exonuclease, a 3′−5′ exonuclease (Morlando et al., 2008) reported in N. viridula saliva, was also present (Lomate and Bonning, 2016). In addition, we report here the presence of anti-viral proteins (Table 3 and Supplementary Material), such as Ars2, related to the regulation of the RISC complex, (Sabin et al., 2009), Egghead (Egh), a seven transmembrane-domain glycosyltransferase, nina C, a protein involved in vesicle transport, and C4572 protein, a carboxypeptidase with unknown function (Saleh et al., 2009).
Once we identified transcripts homologous to essential RNAi genes in P. guilidinii transcriptome, we evaluated in vivo whether the machinery was functional after an injection of a dsRNA targeting vATPase A. Vacuolar-type proton pumping ATPase (V-ATPase) is a ubiquitous enzyme responsible for proton (H+) transport across membranes and the acidification of cellular compartments (Utai et al., 2019). Silencing and mortality after an administration of dsRNA vATPase A have been reported in different insect orders, such as Coleoptera (Baum et al., 2007; Whyard et al., 2009; Christiaens et al., 2016), Diptera (Whyard et al., 2009), Lepidoptera (Whyard et al., 2009; Camargo et al., 2016), Homoptera (Whyard et al., 2009), and Hemiptera (Singh et al., 2015; Basnet and Kamble, 2018). In P. guildinii, an injection of 28 ng/mg of body weight of dsRNA targeting vATPase A showed a mortality of 35% after 7 days, and of 51.6% after 14 days of injection. These results show that P. guildinii is susceptible to RNAi, with a significant mortality effect. In E. heros, an injection of dsRNA against vATPase A at the same concentration caused a mortality of 35% in adults 4 days after injection (Cagliari et al., 2020), while in second instar nymph a 50% mortality was shown after 7 days, reaching 80% after 14 days (Castellanos et al., 2019). A similar observation was reported in N. viridula, where second instar nymphs injected with 40 ng/mg of body weight of dsRNA against vATPase A showed 63 and over 80% mortality after 7 and 14 days, respectively (Sharma et al., 2020), while in adults, mortality rates were not significantly different to the control group (Gurusamy et al., 2021). These reports point out a differential response to RNAi regarding developmental stages in stink bugs. Further analysis in P. guildinii at different life stages could show higher mortality rates.
The effect of dsRNA injection was further assessed by RT-qPCR. The relative expression of vATPase A at 24, 48, and 72 h after injection was significantly reduced to 84% at 72 h, which is in accordance with a functional RNAi machinery. Moreover, Dicer-2 and Argonaute-2, two fundamental core components of the process, were significantly upregulated at 48 h as well. Even though at 72 h, the levels of these proteins were not significantly different from control non-treated samples, the effect in the reduction of the expression of vATPase A was maintained. The upregulation of core siRNA enzymes in response to exogenous dsRNA occurs in many insect species, and can be related not only to the exposure to dsRNA, but also to environmental factors, and pathogens have an influence as well (Cooper et al., 2019).
The establishment of RNAi pest control strategies is a complex task affected by several variables raging from the presence and copy number of core RNAi machinery proteins, the selection of target genes and dsRNA design to delivery strategies that must overcome nuclease degradation, dsRNA cellular uptake, and systemic spreading (Jain et al., 2021). In this context, the present work generated a dataset that will be useful for the selection and design of new target genes to be further evaluated. In addition, the direct effect of dsRNA on insect survival was evaluated bypassing the salivary system and the digestive tract, which may contain enzymes that degrade RNA and may reduce the effect observed, as reported previously in E. heros and N. viridula (Castellanos et al., 2019; Sharma et al., 2021). Delivery strategies to provide dsRNA protection to nuclease activity must be evaluated to achieve a robust P. guildinii control using RNAi.
Conclusion
In this work, we described the assemble and analysis of P. guildinii transcriptome focusing on RNAi machinery. In this sense, we described the annotation of RNAi machinery-related genes and showed that this process is functional in P. guildinii by injection of dsRNA into adults. The administration of dsRNA targeting vATPase A showed a reduction in the expression of this gene and it had significant effect on survival rates. Taken together, these results show that RNAi could be a potential tool for the development of new control strategies in P. guildinii in soybean crops.
Data Availability Statement
The datasets presented in this study can be found in online repositories. The names of the repository and accession number can be found below: https://www.ncbi.nlm.nih.gov/, PRJNA772728.
Author Contributions
MD-R, CS, and MM conceived and designed the experiments. CS and SM performed the experiments. CS and PF analyzed the data. CS and MD-R wrote the manuscript. All authors have discussed the findings, interpreted the results, read, and approved the final manuscript.
Funding
This work was funded by the National Agency of Investigation and Innovation (ANII) FSA_1_2018_1_151855 grant and CS received a Ph.D. scholarship by the National Institute of Agricultural Research (INIA).
Conflict of Interest
The authors declare that the research was conducted in the absence of any commercial or financial relationships that could be construed as a potential conflict of interest.
Publisher’s Note
All claims expressed in this article are solely those of the authors and do not necessarily represent those of their affiliated organizations, or those of the publisher, the editors and the reviewers. Any product that may be evaluated in this article, or claim that may be made by its manufacturer, is not guaranteed or endorsed by the publisher.
Acknowledgments
We would like to thank Mabel Pessio and Liliana del Pino for their help in insect rearing, and Laura Orrego for the bibliography support.
Supplementary Material
The Supplementary Material for this article can be found online at: https://www.frontiersin.org/articles/10.3389/fpls.2022.804839/full#supplementary-material
Footnotes
- ^ http://soystats.com/international-adoption-of-biotech-enhanced-seedstock/
- ^ http://www.bioinformatics.babraham.ac.uk/projects/fastqc
- ^ https://www.bioinformatics.babraham.ac.uk/projects/trim_galore/
- ^ https://www.arb-silva.de
- ^ http://bowtie-bio.sourceforge.net/bowtie2/
- ^ http://trinityrnaseq.sourceforge.net/
- ^ https://busco.ezlab.org/
- ^ https://www.biobam.com/omicsbox
- ^ https://www.ncbi.nlm.nih.gov/orffinder/
- ^ https://www.ncbi.nlm.nih.gov/Structure/cdd/wrpsb.cgi
- ^ https://www.ncbi.nlm.nih.gov/tools/primer-blast/
References
Ambrus, A. M., and Frolov, M. V. (2009). The diverse roles of RNA helicases in RNAi. Cell Cycle 8, 3500–3505. doi: 10.4161/cc.8.21.9887
Arraes, F. B. M., Martins-de-Sa, D., Noriega Vasquez, D. D., Melo, B. P., Faheem, M., de Macedo, L. L. P., et al. (2021). Dissecting protein domain variability in the core rna interference machinery of five insect orders. RNA Biol. 18, 1653–1681. doi: 10.1080/15476286.2020.1861816
Basnet, S., and Kamble, S. T. (2018). RNAi-Mediated Knockdown of vATPase Subunits Affects Survival and Reproduction of Bed Bugs (Hemiptera: Cimicidae). J. Med. Entomol. 55, 540–546. doi: 10.1093/jme/tjy001
Baum, J. A., and Roberts, J. K. (2014). Progress Towards RNAi-Mediated Insect Pest Management. 1st ed. London, United Kingdom: Elsevier Ltd, doi: 10.1016/B978-0-12-800197-4.00005-1
Baum, J. A., Bogaert, T., Clinton, W., Heck, G. R., Feldmann, P., Ilagan, O., et al. (2007). Control of coleopteran insect pests through RNA interference. Nat. Biotechnol. 25, 1322–1326. doi: 10.1038/nbt1359
Baur, M. E., Sosa-Gomez, D. R., Ottea, J., Leonard, B. R., Corso, I. C., Da Silva, J. J., et al. (2010). Susceptibility to insecticides used for control of piezodorus guildinii (Heteroptera: Pentatomidae) in the United States and Brazil. J. Econ. Entomol. 103, 869–876. doi: 10.1603/EC09364
Bundy, S., Esquivel, J., Panizzi, A., Eger, J., Davis, J., and Jones, W. (2019). “Piezodorus guildinii (Westwood),” in Invasive Stink Bugs and Related Species (Pentatomoidea): biology, Higher Systematics, Semiochemistry, and Management, ed. J. E. McPherson (Boca Raton: CRC Press), doi: 10.1201/9781315371221-16
Cagliari, D., Dias, N. P., dos Santos, E. Á, Rickes, L. N., Kremer, F. S., Farias, J. R., et al. (2020). First transcriptome of the Neotropical pest Euschistus heros (Hemiptera: Pentatomidae) with dissection of its siRNA machinery. Sci. Rep. 10, 1–16. doi: 10.1038/s41598-020-60078-3
Camargo, R. A., Barbosa, G. O., Possignolo, I. P., Peres, L. E. P., Lam, E., Lima, J. E., et al. (2016). RNA interference as a gene silencing tool to control Tuta absoluta in tomato (Solanum lycopersicum). PeerJ 4:e2673. doi: 10.7717/peerj.2673
Castellanos, N. L., Smagghe, G., Sharma, R., Oliveira, E. E., and Christiaens, O. (2019). Liposome encapsulation and EDTA formulation of dsRNA targeting essential genes increase oral RNAi-caused mortality in the Neotropical stink bug Euschistus heros. Pest Manag. Sci. 75, 537–548. doi: 10.1002/ps.5167
Caudy, A. A., Myers, M., Hannon, G. J., and Hammond, S. M. (2002). Fragile X-related protein and VIG associate with the RNA interference machinery. Genes Dev. 16, 2491–2496. doi: 10.1101/gad.1025202
Christiaens, O., and Smagghe, G. (2014). The challenge of RNAi-mediated control of hemipterans. Curr. Opin. Insect Sci. 6, 15–21. doi: 10.1016/j.cois.2014.09.012
Christiaens, O., Prentice, K., Pertry, I., Ghislain, M., Bailey, A., Niblett, C., et al. (2016). RNA interference: a promising biopesticide strategy against the African Sweetpotato Weevil Cylas brunneus. Sci. Rep. 6, 1–11. doi: 10.1038/srep38836
Christiaens, O., Whyard, S., Vélez, A. M., and Smagghe, G. (2020). Double-Stranded RNA Technology to Control Insect Pests?: current Status and Challenges. Front. Plant Sci. 11:451. doi: 10.3389/fpls.2020.00451
Cooper, A. M. W., Silver, K., Zhang, J., Park, Y., and Zhu, K. Y. (2019). Molecular mechanisms influencing efficiency of RNA interference in insects. Pest Manag. Sci. 75, 18–28. doi: 10.1002/ps.5126
Corrêa-Ferreira, B. S., and De Azevedo, J. (2002). Soybean seed damage by different species of stink bugs. Agric. For. Entomol. 4, 145–150. doi: 10.1046/j.1461-9563.2002.00136.x
Davies, M. A., and Samuels, Y. (2010). Analysis of the genome to personalize therapy for melanoma. Oncogene 29, 5545–5555. doi: 10.1038/onc.2010.323
de Andrade, E. C., and Hunter, W. B. (2016). “RNA Interference – Natural Gene-Based Technology for Highly Specific Pest Control (HiSPeC),” in RNA Interference, Chap. 19, ed. I. Y. Abdurakhmonov (Rijeka: IntechOpen), doi: 10.5772/61612
Depieri, R., and Panizzi, A. (2011). Duration of feeding and superficial and in-depth damage to soybean seed by selected species of stink bugs (Heteroptera: Pentatomidae). Neotrop. Entomol. 40, 197–203. doi: 10.1590/S1519-566X2011000200007
Dowling, D., Pauli, T., Donath, A., Meusemann, K., Podsiadlowski, L., Petersen, M., et al. (2016). Phylogenetic origin and diversification of RNAi pathway genes in insects. Genome Biol. Evol. 8, 3784–3793. doi: 10.1093/gbe/evw281
Giorio, C., Safer, A., Sánchez-Bayo, F., Tapparo, A., Lentola, A., Girolami, V., et al. (2021). An update of the Worldwide Integrated Assessment (WIA) on systemic insecticides. Environ. Sci. Pollut. Res. 28, 11709–11715. doi: 10.1007/s11356-021-12853-6
Götz, S., García-Gómez, J. M., Terol, J., Williams, T. D., Nagaraj, S. H., Nueda, M. J., et al. (2008). High-throughput functional annotation and data mining with the Blast2GO suite. Nucleic Acids Res. 36, 3420–3435. doi: 10.1093/nar/gkn176
Green, M. R., and Sambrook, J. (2016). Preparation of plasmid DNA by alkaline lysis with sodium dodecyl sulfate: minipreps. Cold Spring Harb. Protoc. 2016, 911–916. doi: 10.1101/pdb.prot093344
Gurusamy, D., Howell, J. L., Chereddy, S. C. R. R., Mogilicherla, K., and Palli, S. R. (2021). Improving RNA interference in the southern green stink bug, Nezara viridula. J. Pest Sci. 94, 1461–1472. doi: 10.1007/s10340-021-01358-3
Gutierrez-Beltran, E., Denisenko, T. V., Zhivotovsky, B., and Bozhkov, P. V. (2016). Tudor staphylococcal nuclease: biochemistry and functions. Cell Death Differ. 23, 1739–1748. doi: 10.1038/cdd.2016.93
Han, B. W., Hung, J. H., Weng, Z., Zamore, P. D., and Ameres, S. L. (2011). The 3’-to-5’ exoribonuclease nibbler shapes the 3’ ends of microRNAs bound to drosophila argonaute1. Curr. Biol. 21, 1878–1887. doi: 10.1016/j.cub.2011.09.034
Hartman, G. L., West, E. D., and Herman, T. K. (2011). Crops that feed the World 2. Soybean-worldwide production, use, and constraints caused by pathogens and pests. Food Secur. 3, 5–17. doi: 10.1007/s12571-010-0108-x
Hinas, A., Wright, A. J., and Hunter, C. P. (2012). SID-5 is an endosome-associated protein required for efficient systemic RNAi in C. elegans. Curr. Biol. 22, 1938–1943. doi: 10.1016/j.cub.2012.08.020
Höck, J., and Meister, G. (2008). The Argonaute protein family. Genome Biol. 9:210. doi: 10.1186/gb-2008-9-2-210
Howell, J. L., Mogilicherla, K., Gurusamy, D., and Palli, S. R. (2020). Development of RNAi methods to control the harlequin bug, Murgantia histrionica. Arch. Insect Biochem. Physiol. 104, 1–14. doi: 10.1002/arch.21690
Hunter, C. P., Winston, W. M., Molodowitch, C., Feinberg, E. H., Shih, J., Sutherlin, M., et al. (2006). Systemic RNAi in Caenorhabditis elegans. Cold Spring Harb. Symp. Quant. Biol. 71, 95–100. doi: 10.1101/sqb.2006.71.060
Huvenne, H., and Smagghe, G. (2010). Mechanisms of dsRNA uptake in insects and potential of RNAi for pest control: a review. J. Insect Physiol. 56, 227–235. doi: 10.1016/j.jinsphys.2009.10.004
Ioannidis, P., Lu, Y., Kumar, N., Creasy, T., Daugherty, S., Chibucos, M. C., et al. (2014). Rapid transcriptome sequencing of an invasive pest, the brown marmorated stink bug Halyomorpha halys. BMC Genomics 15:738. doi: 10.1186/1471-2164-15-738
Jain, R. G., Robinson, K. E., Asgari, S., and Mitter, N. (2021). Current scenario of RNAi-based hemipteran control. Pest Manag. Sci. 77, 2188–2196. doi: 10.1002/ps.6153
Joga, M. R., Zotti, M. J., Smagghe, G., and Christiaens, O. (2016). RNAi efficiency, systemic properties, and novel delivery methods for pest insect control: what we know so far. Front. Physiol. 7:553. doi: 10.3389/fphys.2016.00553
Kavi, H. H., Fernandez, H., Xie, W., and Birchler, J. A. (2008). Genetics and biochemistry of RNAi in Drosophila. Curr. Top. Microbiol. Immunol. 320, 37–75. doi: 10.1007/978-3-540-75157-1_3
Kennedy, S., Wang, D., and Ruvkun, G. (2004). A conserved siRNA-degrading RNase negatively regulates RNA interference in C. elegans. Nature 427, 640–645. doi: 10.1038/nature02320.1
Ketting, R. F. (2011). The Many Faces of RNAi. Dev. Cell 20, 148–161. doi: 10.1016/j.devcel.2011.01.012
Kolliopoulou, A., and Swevers, L. (2014). Recent progress in RNAi research in Lepidoptera: intracellular machinery, antiviral immune response and prospects for insect pest control. Curr. Opin. Insect Sci. 6, 28–34. doi: 10.1016/j.cois.2014.09.019
Laisney, J., Loczenski Rose, V., Watters, K., Donohue, K. V., and Unrine, J. M. (2021). Delivery of short hairpin RNA in the neotropical brown stink bug, Euschistus heros, using a composite nanomaterial. Pestic. Biochem. Physiol. 177:104906. doi: 10.1016/j.pestbp.2021.104906
Lee, Y. S., Nakahara, K., Pham, J. W., Kim, K., He, Z., Sontheimer, E. J., et al. (2004). Distinct roles for Drosophila Dicer-1 and Dicer-2 in the siRNA/miRNA silencing pathways. Cell 117, 69–81. doi: 10.1016/S0092-8674(04)00261-2
Liu, J., Yang, M., Wang, J., Xu, Y., Wang, Y., Shao, X., et al. (2009). Improvement of tumor targeting and antitumor activity by a disulphide bond stabilized diabody expressed in Escherichia coli. Cancer Immunol. Immunother. 58, 1761–1769. doi: 10.1007/s00262-009-0684-9
Livak, K. J., and Schmittgen, T. D. (2001). Analysis of Relative Gene Expression Data Using Real- Time Quantitative PCR and the 2(–ΔΔCt) Method. Methods 408, 402–408. doi: 10.1006/meth.2001.1262
Lomate, P. R., and Bonning, B. C. (2016). Distinct properties of proteases and nucleases in the gut, salivary gland and saliva of southern green stink bug, Nezara viridula. Sci. Rep. 6, 1–10. doi: 10.1038/srep27587
Maida, Y., and Masutomi, K. (2011). RNA-dependent RNA polymerases in RNA silencing. Biol. Chem. 392, 299–304. doi: 10.1515/BC.2011.035
Maktura, C. G., Paranhos, J. B., and Marques-Souza, H. (2021). RNAi in fruit flies (Diptera: Tephritidae): successes and challenges. J. Appl. Entomol. 145, 740–756. doi: 10.1111/jen.12905
Meister, G. (2013). Argonaute proteins: functional insights and emerging roles. Nat. Rev. Genet. 14, 447–459. doi: 10.1038/nrg3462
Mello, C. C., and Conte, D. (2004). Revealing the world of RNA interference. Nature 431, 338–342. doi: 10.1038/nature02872
Mogilicherla, K., Howell, J. L., and Palli, S. R. (2018). Improving RNAi in the Brown Marmorated Stink Bug: identification of target genes and reference genes for RT-qPCR. Sci. Rep. 8, 1–9. doi: 10.1038/s41598-018-22035-z
Morlando, M., Ballarino, M., Gromak, N., Pagano, F., Bozzoni, I., and Proudfoot, N. J. (2008). Primary microRNA transcripts are processed co-transcriptionally. Nat. Struct. Mol. Biol. 15, 902–909. doi: 10.1038/nsmb.1475
Nishide, Y., Kageyama, D., Tanaka, Y., Yokoi, K., Jouraku, A., Futahashi, R., et al. (2021). Effectiveness of orally-delivered double-stranded RNA on gene silencing in the stinkbug Plautia stali. PLoS One 16:e0245081. doi: 10.1371/journal.pone.0245081
Nitnavare, R. B., Bhattacharya, J., Singh, S., Kour, A., Hawkesford, M. J., and Arora, N. (2021). Next Generation dsRNA-Based Insect Control: success So Far and Challenges. Front. Plant Sci. 12:673576. doi: 10.3389/fpls.2021.673576
Panizzi, A. R., and Slansky, F. (1985). Review of Phytophagous Pentatomids (Hemiptera: Pentatomidae) Associated with Soybean in the Americas. Fla. Entomol. 68, 184–214. doi: 10.2307/3494344
Perera, O. P. (2021). Chromosome length genome assembly of the redbanded stink bug, Piezodorus guildinii. Preprint. doi: 10.21203/rs.3.rs-892524/v1
Prentice, K., Pertry, I., Christiaens, O., Bauters, L., Bailey, A., Niblett, C., et al. (2015). Transcriptome analysis and systemic RNAi response in the African sweetpotato weevil (Cylas puncticollis, Coleoptera, Brentidae). PLoS One 10:e0115336. doi: 10.1371/journal.pone.0115336
Ramachandran, V., and Chen, X. (2008). Degradation of microRNAs by a family of exoribonucleases in Arabidopsis. Science 321, 1490–1492. doi: 10.1126/science.1163728
Riga, M., Denecke, S., Livadaras, I., Geibel, S., Nauen, R., and Vontas, J. (2020). Development of efficient RNAi in Nezara viridula for use in insecticide target discovery. Arch. Insect Biochem. Physiol. 103, 1–9. doi: 10.1002/arch.21650
Sabin, L. R., Zhou, R., Gruber, J. J., Lukinova, N., Bambina, S., Lau, C., et al. (2009). Ars2 regulates both miRNA- and siRNA-dependent silencing and suppresses RNA virus infection in Drosophila. Cell 138, 340–351. doi: 10.1016/j.cell.2009.04.045.Ars2
Saleh, M. C., Tassetto, M., van Rij, R. P., Goic, B., Gausson, V., Berry, B., et al. (2009). Antiviral immunity in Drosophila requires systemic RNAi spread. Nature 458, 346–350. doi: 10.1038/nature07712.Antiviral
Saleh, M. C., van Rij, R. P., Hekele, A., Gillis, A., Foley, E., O’Farrell, P. H., et al. (2006). The endocytic pathway mediates cell entry of dsRNA to induce RNAi silencing. Nat. Cell Biol. 8, 793–802. doi: 10.1038/ncb1439
Sharma, R., Christiaens, O., Taning, C. N. T., and Smagghe, G. (2020). RNAi-mediated mortality in southern green stinkbug Nezara viridula by oral delivery of dsRNA. Pest Manag. Sci. 77, 77–84. doi: 10.1002/ps.6017
Sharma, R., Taning, C. N. T., Smagghe, G., and Christiaens, O. (2021). Silencing of double-stranded ribonuclease improves oral RNAi efficacy in southern green stinkbug nezara viridula. Insects 12, 1–16. doi: 10.3390/insects12020115
Shukla, J. N., Kalsi, M., Sethi, A., Narva, K. E., Fishilevich, E., Singh, S., et al. (2016). Reduced stability and intracellular transport of dsRNA contribute to poor RNAi response in lepidopteran insects. RNA Biol. 13, 656–669. doi: 10.1080/15476286.2016.1191728
Singh, A., Upadhyay, V., and Panda, A. (2015). “Solubilization and Refolding of Inclusion Body Proteins,” in Insoluble Proteins SE - 15 Methods in Molecular Biology, ed. E. García-Fruitós (New York: Springer), 283–291. doi: 10.1007/978-1-4939-2205-5_15
Song, H., Zhang, J., Li, D., Cooper, A. M. W., Silver, K., Li, T., et al. (2017). A double-stranded RNA degrading enzyme reduces the efficiency of oral RNA interference in migratory locust. Insect Biochem. Mol. Biol. 86, 68–80. doi: 10.1016/j.ibmb.2017.05.008
Song, L., and Florea, L. (2015). Rcorrector: efficient and accurate error correction for Illumina RNA-seq reads. Gigascience 4:48. doi: 10.1186/s13742-015-0089-y
Sosa-Gómez, D. R., Corrêa-Ferreira, B. S., Kraemer, B., Pasini, A., Husch, P. E., Delfino Vieira, C. E., et al. (2020). Prevalence, damage, management and insecticide resistance of stink bug populations (Hemiptera: Pentatomidae) in commodity crops. Agric. For. Entomol. 22, 99–118. doi: 10.1111/afe.12366
Svoboda, P. (2020). Key Mechanistic Principles and Considerations Concerning RNA Interference. Front. Plant Sci. 11:1237. doi: 10.3389/fpls.2020.01237
Taning, C. N. T., Andrade, E. C., Hunter, W. B., Christiaens, O., and Smagghe, G. (2016). Asian Citrus Psyllid RNAi Pathway-RNAi evidence. Sci. Rep. 6, 1–10. doi: 10.1038/srep38082
Temple, J. H., Davis, J. A., Hardke, J., Moore, J., and Leonard, B. R. (2013). Susceptibility of southern green stink bug and redbanded stink bug to insecticides in soybean field experiments and laboratory bioassays. Southwest. Entomol. 38, 393–406. doi: 10.3958/059.038.0304
Terenius, O., Papanicolaou, A., Garbutt, J. S., Eleftherianos, I., Huvenne, H., Kanginakudru, S., et al. (2011). RNA interference in Lepidoptera?: an overview of successful and unsuccessful studies and implications for experimental design. J. Insect Physiol. 57, 231–245. doi: 10.1016/j.jinsphys.2010.11.006
Thomas, M. F., L’Etoile, N. D., and Ansel, K. M. (2014). Eri1: a conserved enzyme at the crossroads of multiple RNA-processing pathways. Trends Genet. 30, 298–307. doi: 10.1016/j.tig.2014.05.003
Tomoyasu, Y., Miller, S. C., Tomita, S., Schoppmeier, M., Grossmann, D., and Bucher, G. (2008). Exploring systemic RNA interference in insects: a genome-wide survey for RNAi genes in Tribolium. Genome Biol. 9, 1–22. doi: 10.1186/gb-2008-9-1-r10
Ulvila, J., Parikka, M., Kleino, A., Sormunen, R., Ezekowitz, R. A., Kocks, C., et al. (2006). Double-stranded RNA is internalized by scavenger receptor-mediated endocytosis in Drosophila S2 cells. J. Biol. Chem. 281, 14370–14375. doi: 10.1074/jbc.M513868200
Utai, B. M. F., Ada, G. S. U. N., and Ada, Y. W. (2019). Review Vacuolar-type ATPase?: a proton pump to lysosomal trafficking. Proc. Jpn. Acad. Ser. B Phys. Biol. Sci. 95, 261–277. doi: 10.2183/pjab.95.018
Vélez, A. M., and Fishilevich, E. (2018). The mysteries of insect RNAi: a focus on dsRNA uptake and transport. Pestic. Biochem. Physiol. 151, 25–31. doi: 10.1016/j.pestbp.2018.08.005
Whyard, S., Singh, A. D., and Wong, S. (2009). Ingested double-stranded RNAs can act as species-specific insecticides. Insect Biochem. Mol. Biol. 39, 824–832. doi: 10.1016/j.ibmb.2009.09.007
Xie, F., Xiao, P., Chen, D., Xu, L., and Zhang, B. (2012). miRDeepFinder: a miRNA analysis tool for deep sequencing of plant small RNAs. Plant Mol. Biol. 80, 75–84. doi: 10.1007/s11103-012-9885-2
Yamanaka, S., Mehta, S., Reyes-Turcu, F. E., Zhuang, F., Fuchs, R. T., Rong, Y., et al. (2013). RNAi triggered by specialized machinery silences developmental genes and retrotransposons. Nature 493, 557–560. doi: 10.1038/nature11716
Yoon, J. S., Gurusamy, D., and Palli, S. R. (2017). Accumulation of dsRNA in endosomes contributes to inefficient RNA interference in the fall armyworm, Spodoptera frugiperda. Insect Biochem. Mol. Biol. 90, 53–60. doi: 10.1016/j.ibmb.2017.09.011
Zdobnov, E. M., and Apweiler, R. (2001). InterProScan – an integration platform for the signature-recognition methods in InterPro. Bioinformatics 17, 847–848. doi: 10.1093/bioinformatics/17.9.847
Zerbino, M. S., Altier, N. A., and Panizzi, A. R. (2016). Performance of Nymph and Adult of Piezodorus guildinii (Westwood) (Hemiptera: Pentatomidae) Feeding on Cultivated Legumes. Neotrop. Entomol. 45, 114–122. doi: 10.1007/s13744-015-0345-y
Keywords: stink bug, RNA-seq, dsRNA, RNAi, vATPase A, pest control
Citation: Schvartzman C, Fresia P, Murchio S, Mujica MV and Dalla-Rizza M (2022) RNAi in Piezodorus guildinii (Hemiptera: Pentatomidae): Transcriptome Assembly for the Development of Pest Control Strategies. Front. Plant Sci. 13:804839. doi: 10.3389/fpls.2022.804839
Received: 29 October 2021; Accepted: 28 February 2022;
Published: 01 April 2022.
Edited by:
Guy Smagghe, Ghent University, BelgiumReviewed by:
Ran Wang, Institute of Plant Protection and Environmental Protection, ChinaRohit Sharma, Ghent University, Belgium
Copyright © 2022 Schvartzman, Fresia, Murchio, Mujica and Dalla-Rizza. This is an open-access article distributed under the terms of the Creative Commons Attribution License (CC BY). The use, distribution or reproduction in other forums is permitted, provided the original author(s) and the copyright owner(s) are credited and that the original publication in this journal is cited, in accordance with accepted academic practice. No use, distribution or reproduction is permitted which does not comply with these terms.
*Correspondence: Marco Dalla-Rizza, bWRhbGxhcml6emFAaW5pYS5vcmcudXk=