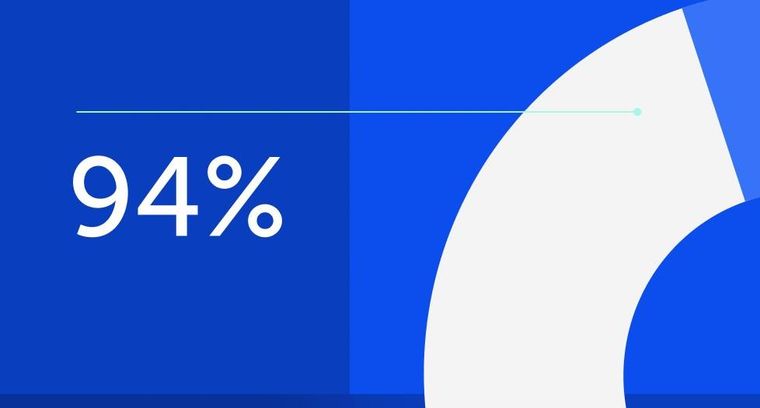
94% of researchers rate our articles as excellent or good
Learn more about the work of our research integrity team to safeguard the quality of each article we publish.
Find out more
ORIGINAL RESEARCH article
Front. Plant Sci., 18 January 2023
Sec. Crop and Product Physiology
Volume 13 - 2022 | https://doi.org/10.3389/fpls.2022.1109953
This article is part of the Research TopicPhytohormonal and Signaling Perspectives on the Response of Horticultural Products to Low Temperature StressView all 5 articles
Introduction: Potato tubers have the healing capacity to prevent surface water transpiration and pathogen invasion after mechanical damage. Previous research has shown the inability to form healing periderm in potatoes under low temperatures, but the potential mechanism is still unclear.
Methods: To explore the effects and mechanisms of low-temperature potato healing, wounded potatoes were stored at low temperature (4°C) and room temperature (22°C), respectively.
Results: In this study, compared with 22°C healing, low temperature reduced the content of hydrogen peroxide, and the down-regulation of StAMY23 inhibited the conversion of starch to sugar, alleviated the degradation of starch, and reduced the content of soluble sugars and sucrose. Meanwhile, inhibition of phenylalanine metabolism by suppression of StPAL1 and St4CL expression reduced lignin accumulation. Low temperature also down-regulated the expression of StKCS6, StFAOH, StGPAT5, and StPrx, causing the lower deposition amount of suberin in wounds of potato tubers.
Discussion: The above results suggested that low temperature led to less wound tissue deposition at the wound surfaces via suppressing phenylpropanoid metabolism and fatty acid biosynthesis in potato tubers.
Potato (Solanum tuberosum L.) is an important staple vegetable in the human diet (Liu et al., 2014). It is extremely susceptible to mechanical damage during the harvest and transportation process, the wound surfaces of tubers establish a channel for infectious pathogens, preventing dehydration, browning, softening, unpleasant aroma, and taste deterioration (Saltveitm, 2016). However, potato tubers possess the healing ability, which usually takes about two weeks to form the functional barrier periderm on the wounds against pathogen invasion and prevent evaporation, to maintain the quality of tubers and greatly reduce post-harvest losses (Patil et al., 2012). Previous research has shown the optimal healing temperature of potato tubers is 22°C (HYODO, 1976). In response to healing, the wounded site synthesizes and deposits the biopolymer lignin and suberin, which is an induced defense response to plant damage and microbial attack (Vance et al., 1980).
In addition, sugar serves as an energy and carbon source for lignin and suberin synthesis in plants (Su et al., 2021). The evidence from published studies reveals that sucrose formed in the leaves of potato tubers during the ripening process can be transported to potato tubers and then transformed into starch for storage. Transformation of starch and sugar occurs in potato tubers during storage. Starch is decomposed into soluble sugars under the action of α-amylase (AMY) and β-amylase (BAM) (Borchert and Mcchesney, 1973). For instance, AMY and BAMs are involved in the starch degradation of kiwifruit during postharvest ripening (Hu et al., 2016; Zhang et al., 2018). Interference with PtrBam1 in lemon inhibits starch degradation and soluble sugar content (Peng et al., 2014). In addition, ADP-glucose pyrophosphorylase (AGPase) is responsible for starch synthesis, and invertase (INV) is an irreversible enzyme in sucrose hydrolysis (Roitsch and Gonzalez, 2004). Up-regulated expression of BAM and INV has also been recently reported to be important for enhancing low-temperature resistance in potato tuber (Liu et al., 2021). Eventually, starch and sugar conversion generate glucose-6-phosphate (G-6-P) to enter the shikimic acid pathway (Li et al., 2018). Shikimic acid can be converted into erythrose for synthesizing phenylalanine (Tzin et al., 2013), an important precursor substance for potato wound formation (Li et al., 2018).
Phenylpropanoid metabolism is highly regulated by several stress factors and environmental stimulants (Zhang and Liu, 2015). Phenylalanine is an important substance involved in lignification (Ramamurthy et al., 2000). Phenylalanine ammonia-lyase (PAL) can catalyze L-phenylalanine to cinnamic acid and phenolics (Pendharkar and Nair, 1987). Members of StPAL families have different performances in potato tuber wounds, and the expression level of StPAL1 is the most significant (Han et al., 2017). 4-coumarate: coenzyme A ligase (4CL) catalyzes the conversion of phenolic acid compounds to corresponding phenolic acid CoA, which can be reduced to corresponding aldehydes under the action of cinnamoyl CoA reductase. Bernards et al. (2000) found that 4CL activity was induced in the same way as PAL activity and it showed a similar trend to PAL during the healing of potato tubers.
However, the biosynthesis of suberin requires the coordinated deposition of two different biopolymers. One is suberin polyphenolic (SPP), which is deposited in the primary cell wall (Negrel et al., 1996). Another is the suberin polyaliphatic (SPA), which is involved in the biosynthesis of aliphatic monomers by fatty acid metabolism (Graca and Pereira, 2000a; Graca and Pereira, 2000b). Peroxidase (Prx) can participate in the peroxidative crosslinking of phenolics as part of SPP synthesis (Lulai and Neubauer, 2014). 3-ketoacyl CoA synthase (KCS) participates in chain-length fatty acids (≥ C28) to obtain ultra-long chain fatty acids. Silencing of StKCS6 in potato periderm leads to reduced chain lengths of the suberin (Serra et al., 2009). Fatty acid ω-hydroxylase (FAOH) is a key enzyme for subcutaneous aliphatic biosynthesis in native periderm. Serra et al. (2009) applied RNAi technology and found that subcutaneous aliphatic in native periderm was reduced and ultra-structurally altered. Glycerol-3-phosphate acyltransferase (GPAT) encodes an acyl CoA-containing protein that catalyzes the key glycerol-based bridging between suberin SPP and SPA. It has been reported that in seeds, roots, and flowers, the reduction of very long-chain fatty acids due to the loss of GPAT5 activity affects the binding of monomers in different ways (Beisson et al., 2007).
Overall, sugar metabolism, fatty acid metabolism, and phenylpropanoid metabolism are essential for the defense of potatoes during healing. The mechanism and expression patterns of the above genes in low-temperature treated potatoes during healing remain unclear. Therefore, in this study, potato tubers were wounded and then stored at 22°C and 4°C for up to 14 days. Physiological and biochemical indexes and the variation of metabolism pathway-related genes were analyzed. This study aimed to investigate the metabolic pathway of wound periderm formation in low-temperature treated potato tubers, to provide a theoretical basis for low temperature healing and to solve the problem of massive labor consumption after potato harvesting.
The potato ‘V7’ were purchased from Xinfadi, Beijing in October 2021. About 50 kg tubers were transported to the laboratory of Institute of Food Science and Technology, Chinese Academy of Agricultural Sciences on the same day. Tubers of uniform size and shape, free from diseases and without any visual defect were selected for the research. The tubers were carefully rinsed twice with distilled water, then immersed in 1.5% (v/v) sodium hypochlorite for 3 min to disinfect surfaces, and dried naturally. Afterward, three artificial wounds (approximately length × 1 cm, width × 1 cm, and depth × 0.5 cm) per tuber were created around the equator with a peeling knife. The wounded tubers were packed in 10-size polyethylene bags with holes (1- mm - diameter holes distributed every 3 cm). The tubers were stored at 22°C, and 4°C with 80% - 90% relative humidity in dark for healing. Wound tissues (3 mm) were collected from the wounded site using a knife after 0, 3, 5, 7, and 14 d of storage. Three biological replicates per treatment were used in all experiments. The samples were stored at -80°C.
The weight of each potato was recorded at each sampling point. Eventually following calculation formula: Weight loss (%) = (m0 - m)/m0 ×100%, where ‘m0’ represents the wounded potato weight on the first day, ‘m’ represents the weight of the wounded potato at each sampling point of both control and treatments. Then take photos to record the wounded surface.
The vertical wound surface of the tuber was cut into slices (about thickness × 0.2 - 0.3 mm, width × 1 cm) with a blade. The slices were immediately rinsed with distilled water to remove starch granules and then immersed in 1% (w/v) phloroglucinol solution for 1.5 min on a glass slide with a few drops of concentrated hydrochloric acid. After 5 min, place the slide under a microscope (10×) to observe the lignin. A slice was cut in the vertical wound surface of the tuber, leaving the slices at 0.05% toluidine blue for 45 min. The residues were removed by washing with distilled water and 75% alcohol two times, respectively. Afterward, rinse twice with 95% alcohol. Finally, the tissue was stained with 1% neutral red III for 1 - 2 min and washed with distilled water and 75% alcohol. The SPA was observed by a (10×) microscope.
Lignin content was determined by Lignin Content Detection Kit (Solarbio, China). Take 3 mg of dry sample and add the reagents according to the instructions. After thorough mixing, the acetylation reaction was carried out in a water bath at 80°C for 40 min, centrifuged at 8000 g for 10 min, and the supernatant was added with glacial acetic acid. The test was conducted in a 96-well UV plate according to the manufacturer’s instructions strictly, and absorbance was read at 280 nm using a microplate reader. H2O2 content was determined by H2O2 Content Detection Kit (Solarbio, China). Weigh 0.05 g of fresh sample in 500 µL acetone ice bath homogenization, centrifuged 8000 g for 10 min at 4°C, and the reagents was added into 250 µL supernatant according to the instructions. The precipitate was dissolved after mixing and centrifuged at 4000 g for 10 min, and rested for 5 min. Absorbance values were measured at 415 nm using a 96-well plate according to the manufacturer’s instructions.
The starch, soluble sugar, and sucrose content were measured following the methods described by He (1985), and the absorbance value was measured at 620 nm. Take 0.01 g dry sample, and add 80% ethanol, 80°C water bath. After cooling, centrifuge to take the supernatant, and repeat the above operation. The supernatant was used to measure the sugar content and the precipitation was used to measure the starch. The supernatant was added 30% KOH and 300 µL anthrone reagent to measure the content of sucrose. The supernatant was added 300 µL anthrone reagent to measure the content of soluble sugar. The precipitation was diluted, add 9.2 mol L-1 perchloric acids, and centrifuged to obtain the supernatant. Add 300 µL anthrone reagent to the supernatant to measure starch content.
The total RNA of the potato tuber was extracted using RNA prep Pure Plant Kit (TIANGEN Biotech, China) according to the manufacturer’s instructions. The RNA integrity was determined using agarose gel electrophoresis (1% agarose gel, 0.5 × TAE, 100 V, 20 min), and purity was established at an absorbance of an OD260/OD280 ratio. Then the RNA was reverse transcribed to cDNA by iScript™ cDNA Synthesis Kit (TRANSGEN, China). All reactions for each cDNA sample were carried out in triplicate.
Primers used in the research were included in Supplementary Table 1. The EF1α gene was used as an internal control and was shown to be stable under the conditions used. The gene expression of Prx, PAL1 (Lulai and Neubauer, 2014), 4CL, KCS6, FAOH, GPAT5 (Lulai and Neubauer, 2014), AMY23, BAM1, AGPase and INV1 (Xie et al., 2018) were analyzed. cDNA was generated using Power SYBR Green PCR Master Mix kit (Applied Biosystems) and real-time quantitative PCR was conducted on ABI 7500 instrument (Applied Biosystems). The real-time PCR conditions were as follows: a pre-incubation at 94°C for the 30 s, then amplification of 40 cycles of 94°C for 5 s, subsequently 30 s at 60°C, 15 s at 95°C, 34 s at 60°C, and final extension step for 30 s at 95°C. The relative quantification of each gene was calculated using the 2− ΔCT method and compared with the gene at the initial time.
All data were analyzed with Microsoft Excel 2019 software, which was used to calculate the mean value and standard deviation. The pictures were prepared using origin 8.5 software (Microcal Software Inc., Northampton, MA, USA). Differences between the control and treated tuber were assessed with a significant level of p < 0.05.
During healing, the wound periderm formation in tubers of all treatments continued to increase. The low-temperature treated wound periderm was reduced to a brighter color than the control within 14 days (Figure 1A). The accumulation of lignin has been increasing during healing in all tubers. The lignin accumulation was slower in the tubers under low-temperature treatment than in the control (Figure 1B). Similarly, suberin accumulation of all tubers also increased continuously during healing, however, the accumulation in the low-temperature treated tubers was significantly slower than that in the control (Figure 1C). The cell layers thickness of lignin and suberin increased in all tubers, and low-temperature treated tubers were lower than the control within 14 days. It indicated that the low temperature inhibited wound periderm formation and the accumulation of lignin and suberin at the wound sites.
Figure 1 Effect of different temperatures on (A) observation of wound periderm surface, (B) lignin deposition, (C) suberin deposition, (D) weight loss, (E) lignin content, (F) H2O2 content, (G) starch content, (H) soluble sugar content and (I) sucrose content at the wound sites of potato tubers during healing. The symbol (*) indicates significant differences among different treatments at p < 0.05.
During healing, the weight loss of all wounded tubers continued to increase. The weight loss rate of the low-temperature treated tubers became significantly slower than the control within 14 d (Figure 1D). The H2O2 content in the low-temperature treatment was significantly lower than the control, the control tubers first increased, then maintained, and finally increased to a high level. At 3 d and 14 d of healing, the H2O2 contents in the control tubers were 2-fold and 3.7-fold higher than that of the low-temperature treated tubers, respectively (Figure 1E). The lignin content in the control tubers showed a rapid increase during healing. The lignin content in the low-temperature treated tubers was significantly lower than that of the control during healing (Figure 1F).
During healing, starch content in all tubers decreased continuously. The content of starch in low-temperature treated tuber was significantly higher than in the control (Figure 1G). The content of soluble sugar initially increased and then rapidly decreased in the control tuber while that in the low-temperature treated tubers increased at 7 d of healing (Figure 1H). The sucrose content variation trend was similar to the soluble sugar content during healing (Figure 1H).
Starch and sugars are important energy supply in tuber during healing (Figure 2A). The expression of StAMY23 in the control increased to the maximum level at 7 d of healing and was significantly higher than that in the low temperature-treated tubers (Figure 2B). Expression of StBAM1 in the treated tubers was 1.7-fold and 3.2-fold higher than that in the control at 7 d and 14 d of healing, respectively (Figure 2C). The expression of StAGPase reached the maximum expression level at 7 d of healing, and in treated tubers was 2.0-fold higher than that in the control (Figure 2D). StINV1 also decreased gradually during healing. The expression of StINV1 was significantly higher in the low-temperature treated tubers than that in the control (Figure 2E). The results showed that low-temperature could inhibit the conversion of starch to sugar in the wound periderm of tubers, thus suppressing energy and precursor substance supply for healing.
Figure 2 Figure 2. (A) The pathway of starch and sugar metabolism and effect of different temperatures on the expression of (B) alpha-amylase 23 (AMY23), (C) beta-amylase 1 (BAM1), (D) ADP-glucose pyrophosphorylase (AGPase) and (E) vacuolar1 (INV1) at the wound sites of potato tubers during healing. Starch is hydrolyzed into glucose under the alpha-amylase (AMY) and beta-amylase (BAM); G-6-P and glucose-1-phosphate (G-1-P) are interconverted to adenosine diphosphate glucose (ADPG) by ADP-glucose pyrophosphorylase (AGPase) for further starch synthesis. Sucrose is catalyzed into hexose (glucose and fructose) by sucrose invertase (INV). The symbol (*) indicates significant differences among different treatments at p < 0.05.
Fatty acid metabolism reflects the accumulation of suberin in the wound periderm of potato tubers during healing (Figure 3A). The expression of StKCS6 and StFAOH in the controls were significantly higher than the low-temperature treated tubers during healing, while those in low-temperature treated tubers maintained at a low level (Figures 3B, C). The expression of StGPAT5 rapidly increased in the control at 3 d and 7 d of healing compared to the low-temperature treated tubers (Figure 3D). The result indicates that low temperature could inhibit the expression of StKCS6, StFAOH, and StGPAT5 to suppress suberin formation during healing.
Figure 3 (A) The pathway of fatty acid metabolism and effects of different temperatures on the expression of (B) 3-Ketoacyl-CoA synthase (KCS6), (C) fatty acid-hydroxylase (FAOH), and (D) glycerol-3-phosphate acyltransferase (GPAT5) at the wound sites of potato tubers during healing. The symbol (*) indicates significant differences among different treatments at p < 0.05.
The phenylpropane metabolic pathway in the wound tissue reflects the ability of tuber healing (Figure 4A). During healing, the expression of StPAL1 and St4CL increased first and then decreased in control compared to the low-temperature treated tubers, the maximum expression reached 2.7-fold and 1.4-fold at 7 d, respectively (Figures 4B, C). The expression of StPAL1 and St4CL in low-temperature treatments were significantly lower than that of the controls, while the StPAL1 expression in the low-temperature treatment was stable during healing. The expression of StPrx in the control was rapidly up-regulated at 3 d, and the low-temperature treatment was significantly lower than the control. However, the control tubers declined to near basal levels of expression at 7 d during healing (Figure 4D). The results suggested that low temperature mainly inhibited StPAL1 and St4CL expressions to suppress lignin formation. Meanwhile, StPrx suppressed further conversion of phenolics to SPP.
Figure 4 (A) The pathway of phenylpropane metabolic and effects of different temperatures on the expression of (B) Phenylalanine ammonia-lyase (PAL1), (C) 4-coumarate-CoA (4CL) and (D) anionic peroxidase (Prx) at the wound sites of potato tubers during healing. The symbol (*) indicates significant differences among different treatments at p < 0.05.
Low temperature inhibits the conversion of starch to sugar in the wound periderm of tubers, thus suppressing energy and precursor substance supply for healing. Starch is converted to G-6-P via sugar metabolism. On the one hand, G-6-P enters the pathway of pentose phosphate and glycolysis, and its intermediate substances are converted to shikimic acid. Shikimic acid is converted into phenylalanine through phosphorylation and other reactions to enter the phenylpropane metabolic pathway. The phenylalanine is mainly catalyzed by phenolic acids, aldehydes, and alcohols, synthesizing less lignin and SPP. On the other hand, G-6-P is converted to glycerol-3-phosphate via the glycolytic pathway, which further forms super long chain fatty acids, ultimately, form small amounts of SPA that are deposited in the wound site (Figure 5).
It is widely studied that potatoes at room temperature can accelerate the formation of wound periderm during healing (Jiang et al., 2019). Therefore, temperature has a very important role in the healing of potato tubers. The wound-healing response of fresh-cut apples and pineapples was promoted at 4°C, increasing H2O2 production and enhancing the activities of PAL and peroxidase during storage (Wu et al., 2013). Storage of shredded carrots at low temperatures can heal, inducing a positive effect on the synthesis of phenolic substances and extending the shelf life of the product (Alegria et al., 2016). In our study, the reduced H2O2 content at low temperature suggested the inhibition of protective protein expression, and it was difficult to initiate the fruit system to acquire resistance in tubers (Xu and Tian, 2008). Thus, the healing effect on potato tubers is difficult to achieve under low temperature storage.
A variety of metabolism was involved in the formation of tuber wounds, among which, sugar metabolism, phenylpropanoid metabolism, and fatty acid biosynthesis played an important role in the formation of wounds (Schmutz et al, 1996; Graca and Pereira, 2000a; Su et al., 2021). We found that low-temperature treatment suppressed sugar metabolism in tubers, which may inhibit the supply of energy and precursor substance during healing (Su et al., 2021). StAMY23 and StBAM1 play distinct roles in starch degradation, particularly under cold conditions. From our results, the expression level of StAMY23 was lower at low temperatures during healing, and reducing the soluble sugar content (Figures 1G, 2). Silencing StAMY23 resulted in a lower accumulation of soluble sugar in potato tubers stored at 4°C (Hou et al., 2017). Different from StAMY23, the StBAM1 response was induced at low temperatures during healing (Figures 2C). Previous studies have demonstrated that both the expression of StBAM1 and StINV1 were constantly induced by low temperatures (Liu et al., 2021). The expression of StINV1 in the low-temperature treated potatoes was up-regulated, accelerating sucrose catabolism and decreasing sucrose content (Liu et al., 2021). In addition, the expression of StAGPase was up-regulated and promoted starch synthesis (Figure 1G, 2). The starch content of common wheat can be increased by overexpression of AGPase (Kang et al., 2013). Nakatani and Komeichi (1992) reported a positive correlation between AGPase activity and the starch content in the tubers of sweet potatoes. Thus, the low temperature inhibited the degradation of starch under the combined effect of StAMY23 and StAGPase, reducing the required energy substance. It is well known that long-term storage of potatoes at low temperatures results in cold-induced sweetening (CIS) (Xie et al., 2018). In this study, sucrose and soluble sugar contents were elevated in low-temperature treatment tubers at a later stage, which was presumably attributed to the sustained cold response of StBAM1 and StINV1. The expression of genes related to sugar metabolism is variable at low temperatures. There are still no studies demonstrating the differential expression of genes related to glucose metabolism in the wound periderm tissue and inside the tuber during the later stages of healing, and it is worth investigating.
Phenylpropanoid metabolism plays a vital role in plant defense reactions (HYODO, 1976; Ferrer et al., 2008). PAL and 4CL directly regulate the process of the synthesis of phenolics, particularly wound-induced metabolism (Li et al., 2022). Our study found the expression of StPAL1 was continuously inhibited by low temperatures, thus suppressing the conversion of phenylalanine to cinnamic acid and other phenolic acid monomers. The expression of St4CL was consistent with the trend of StPAL1 expression. Zhang et al. (2021) showed that up-regulation of PAL and 4CL activity and corresponding gene expression resulted in higher metabolite production in goji berry. Similar results have been reported in peach fruit (Zhou et al., 2020). Furthermore, StPrx involves in the crosslinking of phenolics and promotes the formation of a closed layer around the wound subcutaneously (Espelie and Kolattukudy, 1985). The expression of StPrx has been maintained at a high level during the formation of the tuber seal layer (Lulai and Neubauer, 2014). However, expression of StPrx in low temperature treated tubers was down-regulated and remained stable. It can be explained that this gene expression is delayed and therefore not yet involved in subsequent wounded periderm formation. Overall, these results demonstrated that phenylpropanoid metabolism was inhibited, reducing synthesis of lignin and phenolics, resulting in delayed SPP biosynthesis at low-temperature in potato tubers.
Fatty acids can synthesize and deposit the biopolymer suberin at the wound site. Suberin provides protection from dehydration and pathogens (Bajji et al., 2007). Fatty acids produce fatty acyl CoA through the action of long-chain acyl CoA synthase, which is converted into super long-chain fatty acid CoA under the action of KCS (Cassagne et al., 1994). According to our study, StKCS6 was lowly expressed in the periderm of potato tubers under low-temperature treatment (Figure 3B), which suppressed subsequent SPA assembling. It was shown that silencing the StKCS6 in potato tuber led to decreased periderms, suggesting that StKCS6 deficiency affects SPA deposition (Serra et al., 2009). Similarly, the expression of StFAOH and StGPAT5 was kept at a low base level in low-temperature treated tubers (Figures 3C, D), which was similar to previous reports (Lulai and Neubauer, 2014). These results illustrate that the fatty acid biosynthesis was suppressed at low temperatures, resulting in difficulty in the deposition of SPA at the wound site of the tubers.
This project was supported by the National Natural Science Foundation of China (32072281).
We thank Dr. Yuquan Duan and Dr. Yaoyao Zhao for their suggestions on experimental ideas.
The authors declare that the research was conducted in the absence of any commercial or financial relationships that could be construed as a potential conflict of interest.
All claims expressed in this article are solely those of the authors and do not necessarily represent those of their affiliated organizations, or those of the publisher, the editors and the reviewers. Any product that may be evaluated in this article, or claim that may be made by its manufacturer, is not guaranteed or endorsed by the publisher.
The Supplementary Material for this article can be found online at: https://www.frontiersin.org/articles/10.3389/fpls.2022.1109953/full#supplementary-material
Alegria, C., Gonçalves, E. M., Martins, M. M., Zevallosc, L. C., Abreu, M. (2016). Peel removal improves quality without antioxidant loss, through wound-induced phenolic biosynthesis in shredded carrot. Postharvest Biol. Biotechnol. 120, 232–239. doi: 10.1016/j.postharvbio.2016.07.004
Bajji, M., M’Hamdi, M., Gastiny, F., Delaplace, P., Fauconnier, M. L., Jardin, P. D. (2007). Catalase inhibition alters suberization and wound healing in potato (Solanum tuberosum) tubers. Physiol. Plant 129, 472–483. doi: 10.1111/j.1399-3054.2006.00844.x
Beisson, F., Li, Y. H., Bonaventure, G., Pollard, M., Ohlrogge, J. B. (2007). The acyltransferase GPAT5 is required for the synthesis of suberin in seed coat and root of arabidopsis. Plant Cell. 19, 351–368. doi: 10.1105/tpc.106.048033
Bernards, M. A., Lopez, M. L., Bedgar, D. L., Anterola, A. M., Lewis, N. G. (2000). Induced phenylpropanoid metabolism during suberization and lignification: a comparative analysis. J. Plant Physiol. 157, 601–607. doi: 10.1016/S0176-1617(00)80002-4
Borchert, R., Mcchesney, J. D. (1973). Time course and localization of DNA synthesis during wound healing of potato tuber tissue. Dev. Biol. 35, 293–301. doi: 10.1016/0012-1606(73)90025-0
Cassagne, C., Lessire, R., Bessoule, J. J., Moreau, P., Creach, A., Schneider, F., et al. (1994). Biosynthesis of very long chain fatty acids in higher plants. Prog. Lipid Res. 33, 55–69. doi: 10.1016/0163-7827(94)90009-4
Espelie, K. E., Kolattukudy, P. E. (1985). Purification and characterization of an abscisic acid-inducible anionic peroxidase associated with suberization in potato (Solanum tuberosum). Arch. Biochem. Biophys. 240, 539–545. doi: 10.1016/0003-9861(85)90060-8
Ferrer, J. L., Austin, M. B., Stewart, J. C., Noel, J. P. (2008). Structure and function of enzymes involved in the biosynthesis of phenylpropanoids. Plant Physiol. Biochem. 46, 356–370. doi: 10.1016/j.plaphy.2007.12.009
Graca, J., Pereira, H. (2000a). Methanolysis of bark suberins: analysis of glycerol and acid monomers. Phytochem. Anal. 11, 45–51. doi: 10.1002/(SICI)1099-1565(200001/02)11:1<45::AID-PCA481>3.0.CO;2-8
Graca, J., Pereira, H. (2000b). Diglycerol alkendioates in suberin: building units of a poly(acylglycerol) polyester. Biomacromol 1, 519–522. doi: 10.1021/bm005556t
Han, X. Y., Mao, L. C., Wei, X. P., Lu, W. J. (2017). Stimulatory involvement of abscisic acid in wound suberization of postharvest kiwifruit. Sci. Hortic. 224, 244–250. doi: 10.1016/j.scienta.2017.06.039
Hou, J., Zhang, H. L., Liu, J., Reid, S., Liu, T. F., Xu, S. J., et al. (2017). Amylases StAmy23, StBAM1 and StBAM9 regulate cold-induced sweetening of potato tubers in distinct ways. J. Exp. Bot. 68, 2317–2331. doi: 10.1093/jxb/erx076
Hu, X., Kuang, S., Zhang, A. D., Zhang, W. S., Chen, M. J., Yin, X. R., et al. (2016). Characterization of starch degradation related genes in postharvest kiwifruit. Int. J. Mol. Sci. 17, 2112. doi: 10.3390/ijms17122112
HYODO, H. (1976). Wound-induced phenylalanine ammonia-lyase in potato tuber. J. Biochem. 79, 277–282. doi: 10.1093/oxfordjournals.jbchem.a131068
Jiang, H., Wang, Y., Li, C. J., Wang, B., Ma, L., Ren, Y. Y. (2019). The effect of benzo-(1,2,3)-thiadiazole-7-carbothioic acid s-methyl ester (BTH) treatment on regulation of reactive oxygen species metabolism involved in wound healing of potato tubers during postharvest. Food Chem. 309, 125608. doi: 10.1016/j.foodchem.2019.125608
Kang, G. Z., Liu, G. Q., Peng, X. Q., Wei, L. T., Wang, C. Y., Zhu, Y. J., et al. (2013). Increasing the starch content and grain weight of common wheat by overexpression of the cytosolic AGPase large subunit gene plant physiol. Biochem 73, 93–98. doi: 10.1016/j.plaphy.2013.09.003
Li, B. R., Li, M. Q., Liu, J., Sun, W. W., Min, D. D., Li, F. J., et al. (2022). Methyl salicylate pretreatment maintains quality and antioxidant capacity of fresh-cut pitaya fruit by modulating phenylpropanoid metabolism and antioxidant system. Sci. Hortic. 309, 111705. doi: 10.1016/j.scienta.2022.111705
Li, X. A., Li, M. L., Wang, J., Wang, L., Han, C., Jin, P., et al. (2018). Methyl jasmonate enhances wound-induced phenolic accumulation in pitaya fruit by regulating sugar content and energy status. Postharvest Biol. Technol. 137, 106–112. doi: 10.1016/j.postharvbio.2017.11.016
Liu, X., Chen, L., Shi, W. L., Xu, X., Li, Z. J., Liu, T. F., et al. (2021). Comparative transcriptome reveals distinct starch-sugar interconversion patterns in potato genotypes contrasting for cold-induced sweetening capacity. Food Chem. 334, 127550. doi: 10.1016/j.foodchem.2020.127550
Liu, Y., Gao, M. J., He, W. M., Zhang, Q., Luo, Q. Y. (2014). Analysis on the basic trend and characteristics of world potato production. Chin. Agric. Sci. Bulletin. 30, 78–86. doi: 10.11924/j.issn.1000-6850.2013-2539
Lulai, E. C., Neubauer, J. D. (2014). Wound-induced suberization genes are differentially expressed, spatially and temporally, during closing layer and wound periderm formation. Postharvest Biol. Technol. 90, 24–33. doi: 10.1016/j.postharvbio.2013.11.010
Nakatani, M., Komeichi, M. (1992). Relationship between starch content and activity of starch synthase and ADP-glucose pyrophosphorylase in tuberous root of sweet potato. J. Crop Sci. 61, 463–468. doi: 10.1626/jcs.61.463
Negrel, J., Pollet, B., Lapierre, C. (1996). Ether-linked ferulic acid amides in natural and wound periderms of potato tuber. Phytochemistry 43, 1195–1199. doi: 10.1016/S0031-9422(96)00500-6
Patil, V. U., Gopal, J., Singh, B. P. (2012). Improvement for bacterial wilt resistance in potatoes by conventional and biotechnological approaches. Agric. Res. 1, 299–316. doi: 10.1007/s40003-012-0034-6
Pendharkar, M. B., Nair, P. M. (1987). Alteration in phenylpropanoid metabolism in gamma irradiated potatoes. Potato Res. 30, 589–602. doi: 10.1007/BF02367640
Peng, T., Zhu, X. F., Duan, N., Liu, J. H. (2014). PtrBAM1, a β-amylase-coding gene of poncirus trifoliata, is a CBF regulon member with function in cold tolerance by modulating soluble sugar levels. Plant Cell Environ. 37, 2754–2767. doi: 10.1111/pce.12384
Ramamurthy, M. S., Ussuf, K. K., Nair, P. M., Thomas, P. (2000). Lignin biosynthesis during wound healing of potato tubers in response to gamma irradiation. Postharvest Biol. Technol. 18, 267–272. doi: 10.1016/S0925-5214(99)00073-3
Roitsch, T., Gonzalez, M. C. (2004). Function and regulation of plant invertases: sweet sensations. Trends Plant Sci. 9, 606–613. doi: 10.1016/j.tplants.2004.10.009
Saltveitm, M. E. (2016). The three responses of plant tissue to wounding. Acta Hortic. 1141, 13–20. doi: 10.17660/ActaHortic.2016.1141.2
Schmutz, A., Buchala, A. J., Ryser, U. (1996). Changing the dimensions of suberin lamellae of green cotton fibers with a specific inhibitor of the endoplasmic reticulum-associated fatty acid elongases. Plant Physiol. 110, 403–411. doi: 10.1104/pp.110.2.403
Serra, O., Soler, M., Hohn, C., Sauveplane, V., Pinot, F., Franke, R. (2009). CYP86A33-targeted gene silencing in potato tuber alters suberin composition, distorts suberin lamellae, and impairs the periderm’s water barrier function. Plant Physiol. 149, 1050–1060. doi: 10.1104/pp.108.127183
Su, J., Zhang, C. X., Zhu, L. C., Yang, N. X., Yang, J. J., Ma, B. Q. (2021). MdFRK2−mediated sugar metabolism accelerates cellulose accumulation in apple and poplar. Biotechnol. Biofuels 14, 137. doi: 10.1186/s13068-021-01989-9
Tzin, V., Rogachev, I., Meir, S., Zvi, M. M. B., Masci, T., Vainstein, A., et al. (2013). Tomato fruits expressing a bacterial feedback-insensitive 3-deoxy-d-arabino-heptulosonate 7-phosphate synthase of the shikimate pathway possess enhanced levels of multiple specialized metabolites and upgraded aroma. J. Exp. Bot. 64, 4441–4452. doi: 10.1093/jxb/ert250
Vance, C. P., Kirk, T. K., Sherwood, R. T. (1980). Lignification as a mechanism of disease resistance. Ann. Rev. Phytopathol. 18, 259–288. doi: 10.1146/annurev.py.18.090180.001355
Wu, Z. S., Zhang, M., Adhikari, B. (2013). Effects of high pressure argon and xenon mixed treatment on wound healing and resistance against the growth of escherichia coli or saccharomyces cerevisiae in fresh-cut apples and pineapples. Food Control. 30, 265–271. doi: 10.1016/j.foodcont.2012.06.027
Xie, Y. J., Onik, J. C., Hu, X. J., Duan, Y. Q., Lin, Q. (2018). Effects of (S)-carvone and gibberellin on sugar accumulation in potatoes during low temperature storage. Molecules 23, 3118. doi: 10.3390/molecules23123118
Xu, X., Tian, S. (2008). Salicylic acid alleviated pathogen-induced oxidative stress in harvested sweet cherry fruit. Postharvest Biol. Technol. 49, 379–385. doi: 10.1016/j.postharvbio.2008.02.003
Zhang, X. B., Liu, C. J. (2015). Multifaceted regulations of gateway enzyme phenylalanine ammonia-lyase in the biosynthesis of phenylpropanoids. Mol. Plant 8, 17–27. doi: 10.1016/j.molp.2014.11.001
Zhang, H. Y., Liu, F. R., Wang, J. J., Yang, Q. R., Wang, P., Zhao, H. J., et al. (2021). Salicylic acid inhibits the postharvest decay of goji berry (Lycium barbarum l.) by modulating the antioxidant system and phenylpropanoid metabolites. Postharvest Biol. Technol. 178, 111558. doi: 10.1016/j.lwt.2020.110837
Zhang, A. D., Wang, W. Q., Tong, Y., Li, M. J., Grierson, D., Ferguson, I., et al. (2018). Transcriptome analysis identifies a zinc finger protein regulating starch degradation in kiwifruit. Plant Physiol. 178, 850–863. doi: 10.1104/pp.18.00427
Zhou, D. D., Liu, Q., Peng, J., Tu, S. C., Pan, L. Q., Tu, K. (2020). Metabolic analysis of phenolic profiles reveals the enhancements of anthocyanins and procyanidins in postharvest peach as affected by hot air and ultraviolet c. Postharvest Biol. Technol. 167, 111227. doi: 10.1016/j.postharvbio.2020.111227
Keywords: potato tuber, temperature, healing, phenylpropanoid metabolism, fatty acid biosynthesis
Citation: Zhang J, Yao J, Mao L, Li Q, Wang L and Lin Q (2023) Low temperature reduces potato wound formation by inhibiting phenylpropanoid metabolism and fatty acid biosynthesis. Front. Plant Sci. 13:1109953. doi: 10.3389/fpls.2022.1109953
Received: 28 November 2022; Accepted: 30 December 2022;
Published: 18 January 2023.
Edited by:
Ke Wang, Anhui Agricultural University, ChinaReviewed by:
Zhengke Zhang, Hainan University, ChinaCopyright © 2023 Zhang, Yao, Mao, Li, Wang and Lin. This is an open-access article distributed under the terms of the Creative Commons Attribution License (CC BY). The use, distribution or reproduction in other forums is permitted, provided the original author(s) and the copyright owner(s) are credited and that the original publication in this journal is cited, in accordance with accepted academic practice. No use, distribution or reproduction is permitted which does not comply with these terms.
*Correspondence: Lixia Wang, bGl4aWF3YW5nQDE2My5jb20=; Qing Lin, bGlucWlvbmcxMDI2QDEyNi5jb20=
†These authors have contributed equally to this work
Disclaimer: All claims expressed in this article are solely those of the authors and do not necessarily represent those of their affiliated organizations, or those of the publisher, the editors and the reviewers. Any product that may be evaluated in this article or claim that may be made by its manufacturer is not guaranteed or endorsed by the publisher.
Research integrity at Frontiers
Learn more about the work of our research integrity team to safeguard the quality of each article we publish.