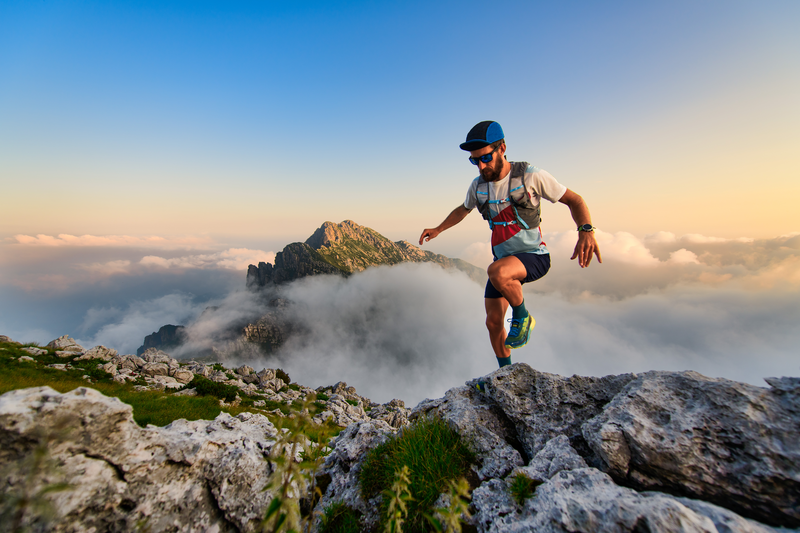
95% of researchers rate our articles as excellent or good
Learn more about the work of our research integrity team to safeguard the quality of each article we publish.
Find out more
ORIGINAL RESEARCH article
Front. Plant Sci. , 23 December 2022
Sec. Crop and Product Physiology
Volume 13 - 2022 | https://doi.org/10.3389/fpls.2022.1107687
This article is part of the Research Topic The Physiology, Molecular Biology and Biochemistry in Ripening and Stored Fruit View all 12 articles
Introduction: Chilling injury is a major hindrance to cucumber fruit quality during cold storage.
Methods and Results: In this study, we evaluated the effects of fucoidan on fruit quality, reactive oxygen species homeostasis, and energy metabolism in cucumbers during cold storage. The results showed that, compared with the control cucumber fruit, fucoidan-treated cucumber fruit exhibited a lower chilling injury index and less weight loss, as well as reduced electrolyte leakage and malondialdehyde content. The most pronounced effects were observed following treatment with fucoidan at 15 g/L, which resulted in increased 1,1-diphenyl-2-picrylhydrazyl and hydroxyl radical scavenging rates and reduced superoxide anion production rate and hydrogen peroxide content. The expression and activity levels of peroxidase, catalase, and superoxide dismutase were enhanced by fucoidan treatment. Further, fucoidan treatment maintained high levels of ascorbic acid and glutathione, and high ratios of ascorbic acid/dehydroascorbate and glutathione/oxidized glutathione. Moreover, fucoidan treatment increased the activities of ascorbate peroxidase, monodehydroascorbate reductase, dehydroascorbate reductase, and glutathione reductase and their gene expression. Fucoidan treatment significantly delayed the decrease in ATP and ADP, while preventing an increase in AMP content. Finally, fucoidan treatment delayed the decrease of energy charge and the activities and gene expression of H+-ATPase, Ca2+-ATPase, cytochrome c oxidase, and succinate dehydrogenase in cucumber fruits.
Conclusion: Altogether, our findings indicate that fucoidan can effectively enhance antioxidant capacity and maintain energy metabolism, thereby improving cucumber cold resistance during cold storage.
Cold storage is a common method of preserving horticultural products after harvest. However, for cold-sensitive horticultural products, such as cucumber (Wang et al., 2018; Wang et al., 2020a), peach (Song et al., 2022), and kiwifruit (Liu et al., 2023), low temperatures can cause chilling injury (CI). CI damages the permeability of the fruit cell membrane, leading to cytoplasmic leakage. When cucumber fruit is transferred from cold storage to room temperature, the symptoms of CI appear quickly, manifested as watery spots on the fruit epidermis and an unpleasant odor, which are generally deemed unacceptable by consumers (Tsuchida et al., 2010; Wang et al., 2018).
Cold stress induces the accumulation of reactive oxygen species (ROS) in plant tissues, leading to a series of oxidative damage events, such as membrane lipid peroxidation, and eventually inducing cell death (You and Chan, 2015; Mittler et al., 2022). The plant ROS-scavenging system consists of an enzymatic defense system and a non-enzymatic antioxidant defense system (Gill and Tuteja, 2010). The former includes peroxidase (POD), catalase (CAT) and superoxide dismutase (SOD). SOD can remove excess superoxide anions () in plants and reduce the damage caused by to the cell membrane. In turn, POD and CAT catalyze the decomposition of hydrogen peroxide (H2O2) to maintain H2O2 at a low level, minimize the production of hydroxyl radicals (-OH), and protect cell membrane structure (Yu et al., 2022). The ascorbic acid-glutathione (AsA-GSH) cycle is an important component of the non-enzymatic antioxidant system. The AsA-GSH cycle comprises ascorbate peroxidase (APX), monodehydroascorbate reductase (MDHAR), dehydroascorbate reductase (DHAR), and glutathione reductase (GR), which can directly remove H2O2 from plant tissues. The AsA-GSH cycle promotes the production of two non-enzymatic antioxidants (AsA and GSH) to maintain the balance of redox substances in plants (Peng et al., 2022). The ROS-scavenging system has been shown to be critical for the enhancement of cold resistance in many horticultural products, such as loquats (Cai et al., 2011), bell peppers (Endo et al., 2019), okra (Li et al., 2023), and bananas (Chen et al., 2021b). Therefore, improving antioxidant capacity is a promising approach for alleviating low temperature-induced oxidative damage in horticultural products.
Mitochondria are important sites for respiration and the production of ATP in living tissues, thus providing energy for all cellular activities (Chen et al., 2021a). H+-ATPase, Ca2+-ATPase, cytochrome c oxidase (CCO), and succinate dehydrogenase (SDH) are key enzymes involved in energy synthesis in the mitochondrial membrane (Li et al., 2021a). The decrease or loss of activity of these enzymes can lead to insufficient energy supply to cells, which in turn leads to cellular senescence and death. Numerous studies have shown that the occurrence of CI during the post-harvest cold storage of horticultural products, such as strawberries (Li et al., 2018), pears (Tao et al., 2019), and peaches (Jin et al., 2013), is related to energy metabolism. Energy loss leads to the accumulation of ROS, cell membrane damage, impaired metabolism, and a slow response to low temperature signals, thus accelerating the occurrence of CI.
Numerous preservation techniques, such as brief exposure to hot water (Nasef, 2018), pre-storage cold acclimation (Wang et al., 2018; Wang et al., 2021a), or treatment with hydrogen sulfide (Wang et al., 2022), melatonin (Madebo et al., 2021), or glycine betaine (Zhang et al., 2008), have been shown to extend the shelf life of cold-stored cucumber fruits. Polysaccharide coatings have also been used to improve cold resistance after harvest; for example, chitosan has been shown to improve cold resistance in cucumber fruit (Ru et al., 2020), and treatment with Astragalus polysaccharides inhibited the occurrence of CI in bananas (Tian et al., 2022). Fucoidan is a type of fucose-enriched and sulfated polysaccharide that extracted from different kinds of brown seaweed, which contains L-fucose and sulfate groups (Zhao et al., 2004). As a nontoxic natural heteropolysaccharide, fucoidan has multiple biological functions, such as anti-coagulation, anti-tumor, anti-thrombus, anti-virus, anti-oxidation and enhance the body’s immune function (Senthilkumar et al., 2013; Wu et al., 2016). In addition, fucoidan can be used as a preservative for storing fruit and vegetables after harvest. Some studies have shown that fucoidan improves antioxidant capacity and maintains post-harvest fruit quality; indeed, fucoidan can extend the shelf life of some fruits, such as mangos (Xu and Wu, 2021), and strawberries (Duan et al., 2019; Luo et al., 2020). However, to date, the effect of fucoidan on CI in cucumber fruits has rarely been reported. Therefore, here, we investigated whether fucoidan treatment can improve cold resistance in cucumbers stored at low temperature, with a focus on ROS homeostasis and energy metabolism. Our findings support the use of fucoidan as a new preservative for cucumber cold storage.
Cucumbers (Cucumis sativus cv. Sunstar) were collected during the commercial harvest period (approximately 10 d after flowering) from a farm in Nanchang, Jiangxi, China. Fruits were washed with distilled water and dried naturally. Fruits of uniform size and without any disease or defects were randomly separated into four groups and coated with 0 (control), 5, 10, or 15 g/L fucoidan (extract from brown algae, medium molecular weight, purity≥95%, Baichuan, Xi’an, China). Treatments were performed in triplicates comprising 300 fruits for each replicate. Treated fruits were packed in 0.01 mm thick polyethylene plastic bags and stored for 12 days at 4°C and 90% relative humidity. For each replicate, 20 fruits were collected every three days, 15 of which were placed at 20°C for two days to observe CI, and the pulp (without peel tissue and internal seeds) of the remaining 5 fruits was collected at random and stored at -80°C after quick freezing under liquid nitrogen.
For CI: The degree of cucumber CI was divided into five levels. 0 = no CI, 1 = 0< CI area ≤ 25%, 2 = 25%< CI area ≤ 50%, 3 = 50%< CI area ≤ 75%, 4 = 75%< CI area ≤ 100%. CI inedx was calculated as follows:
Ten tissue discs with uniform size (thickness: 1 mm, diameter: 8 mm) from five cucumber fruits were prepared for the measurement of electrolyte leakage. Electrolyte leakage, and malondialdehyde (MDA) content of the experimental cucumber fruits were measured as previously described (Wang et al., 2022).
For weight loss: five cucumber fruits were weighed using an electronic scale at a time, and the measurements were repeated three times. Weight loss was calculated as follows:
One gram of pulp from control and fucoidan-treated cucumber fruits was used for the measurement of 1,1-diphenyl-2-picrylhydrazyl (DPPH) scavenging rate, -OH scavenging rate, production rate, and H2O2 content using a DPPH scavenging rate detection kit (BC4750, Solarbio, Beijing, China), -OH scavenging rate detection kit (BC1320, Solarbio, Beijing, China), content detection kit (BC1290, Solarbio, Beijing, China), and H2O2 content detection kit (BC3595, Solarbio, Beijing, China), respectively. The unit percentage (%) represents the DPPH and -OH scavenging rates. The production rate and H2O2 content are expressed as μmol s-1 kg-1 and mmol kg-1, respectively.
To quantify POD, SOD, and CAT activities, 1.0 g of pulp from control and fucoidan-treated cucumber fruits was homogenized with 5 ml of 50 mM pre-cooled sodium phosphate buffer (pH 7.5) containing 5 mM dithiothreitol and 5% polyvinylpyrrolidone. The mixture was then centrifuged at 12,000 g for 15 min at 4°C and the supernatant was collected. POD, SOD, and CAT activities were determined as previously described (Chen et al., 2022), and expressed as U kg-1. One U of POD and CAT activities represents an absorbance change of 0.01 per gram of sample per minute at 470 nm and 240 nm, respectively. In turn, 1 U of SOD activity represents the amount of SOD per gram of sample in 1 mL of reaction solution when SOD inhibition reached 50%.
The activities of APX, MDHAR, DHAR, and GR were determined using the appropriate test kits (BC0220 for APX activity, BC0650 for MDHAR activity, BC0660 for DHAR activity, and BC1160 for GR activity; Solarbio, Beijing, China) with absorbance measured at 290, 340, 412, and 340 nm, respectively. Enzyme activities are expressed as U kg-1, where 1 U represents 1 μmol of enzyme oxidized per gram of sample per minute.
One gram of homogenized cucumber fruit was mixed with 15 mL of pre-cooled 5% (w/v) trichloroacetic acid and centrifuged at 12,000 rpm for 20 min at 4°C. The supernatant was collected and AsA and DHA content (mg kg-1) was determined as previously described (Zhang et al., 2021). DHA content was calculated as follows:
GSH and GSSG contents (mg kg-1) were detected using the appropriate test kits (BC1170 for GSH content; BC1180 for GSSG content; Solarbio, Beijing, China) with absorbance measured at 412 nm.
One gram of cucumber pulp tissue was ground under liquid nitrogen. Then, 6 mL pre-cooled 0.6 mol·L-1 perchloric acid solution was added and the sample was fully homogenized. The homogenate was placed on ice for 10 min and then centrifuged at 8000 rpm at 4°C for 15 min. After collecting the supernatant, the pH was adjusted to 6.8 with 1.0 mol·L-1 KOH. The neutralized sample was placed on ice for 30 min and then centrifuged at 8000 g at 4°C for 15 min. The supernatant was filtered through a 0.45 μm microporous membrane for use. ATP, ADP, and AMP contents (mg kg-1) were determined by high-performance liquid chromatography (LC-2030 Plus, Shimadzu, Kyoto, Japan) using a C18 column (250 mm × 4.6 mm, 5 μm, Waters) as previously described (Chen et al., 2021a). Energy charge was calculated as follows:
One gram of pulp from control and fucoidan-treated cucumber fruits was used for measurement of H+-ATPase and Ca2+-ATPase activities using an ATPase activity test kit (A016-1-2, Jiancheng Bioengineering Institute, Nanjing, China) with absorbance measured at 660 nm. One gram of pulp from control and fucoidan-treated cucumber fruits was used for measurement of CCO and SDH activities as previously described (Chen et al., 2022). All results were expressed as U kg-1.
0.5 g of pulp from control and fucoidan-treated cucumber fruits was used for RNA extraction and gene expression analysis as previously described (Gan et al., 2021). The 2X M5 HiPer Realtime PCR Super mix kit (Mei5bio, Beijing, China) was used to perform quantitative real-time polymerase chain reaction (qRT-PCR) using gene-specific primers (Supplementary Table S1). Relative gene expression levels were calculated using the 2-ΔΔCt method.
Each experiment was repeated in triplicate. Excel software was used to sort the data, and the results are expressed as the mean ± standard deviation (SD). Pairwise comparisons were made using the single factor method in SPSS26.0, and significant differences were analyzed using the least significant difference test and Duncan’s method (P< 0.05 or P< 0.01). Graphs were drawn using GraphPad Prism 8.0.
Water-soaked depressions of the epidermis are the most obvious symptom of CI in cucumbers. After 12 days of storage at 4°C, the CI index was 0.65. Compared with controls, coating with 5, 10, or 15 g/L fucoidan decreased the CI index of cold-stored cucumbers (Figures 1A, B). Furthermore, weight loss, electrolyte leakage, and MDA content were all reduced following fucoidan treatment (Figures 1C–E), reflecting the protective effect of fucoidan against CI. Although all fucoidan treatments reduced CI, treatment with 15 g/L fucoidan achieved the best results; thus, 15 g/L fucoidan was used for subsequent experiments.
Figure 1 (A) Photograph comparing cucumbers treated with 0 (control), 5, 10, or 15 g L-1 fucoidan after 12 d of storage at 4°C. (B-E) Chilling injury index (B), weight loss rate (C), electrolytic leakage (D), and MDA content (E) in cucumber fruit treated with 0, 5, 10, and 15 g L-1 fucoidan after 12 d of storage at 4°C. Values are presented as means ± SD of at least three replicates. Bars with different letters are significantly different at P< 0.05, as determined using Duncan’s multiple range tests.
The DPPH and -OH scavenging rates in cucumbers initially increased and then decreased during cold storage. The DPPH scavenging rate peaked on day 6 of storage, while the -OH scavenging rate peaked on day 9. The DPPH and -OH scavenging rates of fucoidan-treated cucumber fruits were higher than those of control cucumbers (Figures 2A, B).
Figure 2 (A-D) The DPPH scavenging rate (A), -OH scavenging rate (B), production rate (C), and H2O2 content (D) in fucoidan-treated (15 g L-1) and control cucumbers during storage at 4°C. Values are presented as means ± SD of at least three replicates. **P< 0.01.
The rate of production increased steadily throughout storage in both control and fucoidan-treated cucumbers. However, the production rate remained lower in the fucoidan-treated group than in the control group throughout the storage period. Control cucumbers exhibited a gradual increase in H2O2 content, which peaked on day 6 of storage and subsequently declined slightly. In contrast, fucoidan treatment significantly reduced the accumulation of H2O2 in cold-stored cucumber fruits (Figures 2C, D).
The activities of the POD and CAT enzymes initially increased in control cucumbers upon cold storage, reaching a maximum on day 6 of storage, and gradually declining thereafter. Conversely, POD and CAT activities increased by 65% and 39%, respectively, following fucoidan treatment, compared to the control group on day 6 of cold storage, and the subsequent decline in these activities was considerably less pronounced than that in the controls (Figures 3A, C). In turn, SOD activity in cucumber fruits steadily decreased throughout the storage period, and fucoidan-treated cucumbers maintained higher SOD activity than control cucumbers (Figures 3E). Consistent with these results, fucoidan treatment induced the expression of CsPOD, CsCAT2, and CsSOD (Figures 3B, D, F).
Figure 3 (A-F) The enzyme activities and gene expression of POD (A, B), CAT (C, D), and SOD (E, F) in fucoidan-treated (15 g L-1) and control cucumbers during storage at 4°C. Values are presented as means ± SD of at least three replicates. **P< 0.01.
The AsA content of cucumber fruits decreased gradually during cold storage in both treated and control fruit groups, but remained higher in the fucoidan-treated group than in the control group on day 6. On day 12, the AsA content of fucoidan-treated fruit was 12% higher than that in control group (Figure 4A). In contrast, DHA content in cucumber fruits was not affected by cold storage but was significantly lower in the fucoidan-treated group than in the control group on days 6 and 9 (Figure 4B). Although the AsA/DHA ratio decreased gradually during storage, the fucoidan-treated group maintained a higher AsA/DHA ratio than the control group (Figure 4C). The GSH content increased with increasing refrigeration time and was higher in fucoidan-treated cucumbers compared to controls throughout the entire storage period (Figure 4D). The GSSG content decreased gradually during cold storage, and was significantly higher in the fucoidan-treated group than in the control group from the 6th day of storage (Figure 4E). Finally, the GSH/GSSG ratio increased gradually during storage, and the fucoidan-treated group maintained a higher GSH/GSSG ratio than the control group did (Figure 4F).
Figure 4 (A-F) AsA content (A), DHA content (B), AsA/DHA ratio (C), GSH content (D), GSSG content (E), and GSH/GSSG (F) in fucoidan-treated (15 g L-1) and control cucumbers during storage at 4°C. Values are presented as means ± SD of at least three replicates. *P< 0.05; **P< 0.01.
APX activity initially increased and then decreased during cold storage, reaching a maximum on day 6. The APX activity of fruits in the fucoidan-treated group was significantly higher than that in the control group throughout the storage period (Figure 5A). Compared with the control group, MDHAR activity of fruits in the fucoidan-treated group was higher throughout the storage period, becoming 63% higher on day 6 of storage (Figure 5C). Similarly, DHAR and GR activities initially increased, reaching a maximum on day 3 before subsequently declining; the activities of both enzymes were significantly improved by fucoidan treatment (Figures 5E, G). The expression levels of CsAPX, CsMDHAR, and CsDHAR were consistent with the trends described for enzyme activity, with fucoidan treatment inducing the expression of these genes. Finally, the expression level of CsGR in the fucoidan-treated group was significantly higher than that in the control group on days 3 and 6 (Figures 5B, D, F, H).
Figure 5 (A-H) The enzyme activities and gene expression of APX (A, B), MDHAR (C, D), DHAR (E, F), and GR (G, H) in fucoidan-treated (15 g L-1) and control cucumbers during storage at 4°C. Values are presented as means ± SD of at least three replicates. *P< 0.05; **P< 0.01.
The ATP and ADP contents of cucumber fruits declined continuously during cold storage. However, ATP and ADP were significantly higher in fucoidan-treated cucumbers than in control ones throughout the storage period. On day 12, ATP and ADP contents in the treatment group were 14% and 35% higher than those in the control group, respectively (Figures 6A, B). Conversely, AMP content in refrigerated cucumber fruits increased gradually during the storage period. Thus, after 6 days of storage, AMP content in fucoidan-treated fruits was significantly lower than that in the control group, decreasing to a level that was 25% lower on day 12 (Figure 6C). Consistently, energy charge declined slowly from day 1 to 8 and then declined sharply from the 9th day, with extension of storage time. Compared to control, fucoidan-treated cucumbers maintained a higher energy charge during cold storage (Figure 6D).
Figure 6 (A-D) ATP content (A), ADP content (B), AMP content (C), and energy charge (D) in fucoidan-treated (15 g L-1) and control cucumbers during storage at 4°C. Values are presented as means ± SD of at least three replicates. **P< 0.01.
Activity of H+-ATPase initially increased and then decreased during cold storage. The activity of H+-ATPase in fucoidan-treated cucumbers peaked on day 6 and was 33% higher than that of the control group on day 12 (Figure 7A). In contrast, Ca2+-ATPase activity remained stable during cold storage, and fucoidan-treated fruit maintained a higher level of activity than control ones (Figure 7C). Similarly, CCO activity reached a maximum on day 6 of cold storage before declining. Fucoidan-treated cucumbers maintained a higher level of CCO activity after day 6, and it was 50% higher than that of the control group on day 12 (Figure 7E). Additionally, on day 3 of storage, SDH activity of both fucoidan-treated and control fruits peaked, with the former reaching a level 37% higher than that of the control group before beginning to decline (Figure 7G). The expression levels of CsH+-ATPase, CsCa2+-ATPase, CsCCO, and CsSDH corresponded with these trends in enzyme activity. Indeed, we found that fucoidan treatment significantly induced the expression of all four genes, thereby regulating the enhancement of their corresponding enzyme activities (Figures 7B, D, F, H) and maintaining metabolism in cucumber fruits to preserve normal physiological activities during cold storage.
Figure 7 (A-H) The enzyme activities and gene expression of H+-ATPase (A, B), Ca2+-ATPase (C, D), CCO (E, F), and SDH (G, H) in fucoidan-treated (15 g L-1) and control cucumbers during storage at 4°C. Values are presented as means ± SD of at least three replicates. *P< 0.05; **P< 0.01.
CI easily occurs in cold-stored cucumber fruits after harvest and leads to a decrease in fruit quality and commodity value (Wang et al., 2018; Madebo et al., 2021; Wang et al., 2022). Polysaccharide coatings are environment friendly materials for preservation and improving cold resistance of horticultural products. Carboxymethyl cellulose (CMC) coating help to reduce CI in pomegranate (Ehteshami et al., 2019) and mandarin fruit (Ali et al., 2021) during cold storage. Astragalus polysaccharides delayed the CI and attenuated postharvest peel browning of banana fruit (Tian et al., 2022). In vegetables, the coating of polysaccharide has a similar preservation effect to that of fruit. CMC-based water barrier coating is promising to control the water loss and further maintain the whole quality of pakchoi (Yu et al., 2022). Chitosan induced chilling resistance in cucumber fruit (Ru et al., 2020). Fucoidan has a wide range of biological activities (Zhao et al., 2004)., which has been used to maintain the quality of some fruits. Fucoidan treatment can effectively extend the shelf life of mangoes (Xu and Wu, 2021). Fucoidan treatment improved the cold resistance of strawberry fruit during cold storage (Duan et al., 2019; Luo et al., 2020). In this study, different concentrations of fucoidan (5, 10, and 15 g/L) effectively inhibited CI in cucumber fruits and delayed the appearance of CI symptoms. With the increase of fucoidan concentration, the CI index is lower, the treatment with 15 g/L fucoidan achieved the best results in this study. However, the best result of 15 g/L fucoidan is based on this work, may not the optimum concentration. Thus, the optimum concentration of fucoidan for reducing the CI in cucumber will be further explored.
Cold stress-induced plant damage typically involves an imbalance between ROS production and depletion (Duan et al., 2012); excessive accumulation of ROS, such as H2O2 and , can irreversibly damage organelles, including chloroplasts and mitochondria. Protecting cells from oxidative damage under stress is considered a major mechanism of plant resistance to low temperatures, and this resistance may depend on the antioxidant system (Mittler et al., 2022). The enzymatic antioxidant system is the main mode of ROS scavenging, and SOD, CAT, and POD are the key antioxidant enzymes involved in this process (Tan et al., 2020). These three antioxidant enzymes work together to reduce the oxidative damage to the cell membrane caused by H2O2 and O2-, thus maintaining its integrity and minimizing CI (Cao et al., 2018; Mirshekari et al., 2020). In this study, fucoidan treatment significantly enhanced the SOD activity of cucumber fruits, effectively reduced the O2- production rate, and maintained high CAT and POD activities, thereby inhibiting the accumulation of H2O2. Similarly, previous studies have reported that fucoidan treatment enhances antioxidant activity and improves the cold tolerance of strawberries (Duan et al., 2019; Luo et al., 2020). Thus, we conclude that fucoidan treatment is beneficial in regulating the redox state, and improves the antioxidant capacity of cold-stored cucumber fruits.
The AsA-GSH cycle is an important H2O2 scavenging system in plants. AsA and GSH are important non-enzymatic antioxidants in this cycle, which can scavenge excessive ROS produced by plant cells in response to low-temperature stress injury (Li et al., 2010). The AsA/DHA and GSH/GSSG ratios reflect the redox capacity of plants. Generally, the higher the content of reducing substances, the stronger the stress tolerance of plants (Li et al., 2023). In this study, fucoidan treatment consistently maintained higher AsA and GSH contents and AsA/DHA and GSH/GSSG ratios in cucumber fruits, consistent with results from tomatoes (Li et al., 2019), and bell peppers (Endo et al., 2019). GSH/GSSG ratios play an important role in cold tolerance of tomatoes (Li et al., 2019). Hot water treatment enhanced AsA-GSH cycle to alleviate CI in pepper fruit during postharvest cold storage (Endo et al., 2019). APX, MDHAR, DHAR, and GR are key enzymes involved in AsA-GSH metabolism that cooperate to scavenge excess H2O2 in cells and convert it into H2O and O2- (Li et al., 2010). Enhanced gene expression and activity of APX, MDHAR, DHAR, and GR has been shown to improve fruit cold tolerance and reduce the occurrence of CI in bell peppers (Wang et al., 2016; Endo et al., 2019), peaches (Song et al., 2016), okra (Li et al., 2023), and bananas (Chen et al., 2021b). These results support our findings that fucoidan treatment can regulate the activities of key enzymes and the expression of related genes in the AsA-GSH cycle of cucumber fruits during cold storage, thereby improving H2O2scavenging, reducing the degree of membrane lipid peroxidation, and delaying the occurrence of CI in cucumber fruits.
Energy molecules, such as ATP, play an important role in maintaining the integrity of cell membranes (Saquet et al., 2003). Energy deficits can impair the integrity of cell membranes and lead to a decline in the post-harvest quality of horticultural products (Wang et al., 2020c; Li et al., 2021a). ATP and ADP contents in loquats (Jin et al., 2015), kiwifruit (Wang et al., 2020b), and peaches (Hu et al., 2023), have been reported to drop rapidly and remain at low levels during cold storage. In this study, ATP, ADP, and energy charge declined rapidly in cucumber fruits during cold storage, and the symptoms of fruit CI were aggravated. Fucoidan treatment maintained higher levels of ATP, ADP, and energy charge in cucumber fruits, thereby reducing the ROS-induced membrane damage and alleviating CI. Mitochondria are responsible for energy synthesis; SDH, CCO, H+-ATPase, and Ca2+-ATPase are key respiratory enzymes located in the inner mitochondrial membrane, and their activities reflect the energy production status of mitochondria (Li et al., 2021b; Wang et al., 2021b). H+-ATPase catalyzes ATP synthesis by establishing an H+ electrochemical gradient on both sides of the cell membrane to generate transmembrane proton propulsion (Azevedo et al., 2008). Ca2+-ATPase is a Ca2+ pump responsible for transporting Ca2+ into the mitochondria, and a decrease in Ca2+-ATPase activity leads to the accumulation of Ca2+ in the cytoplasm, leading to the destruction of membrane structural integrity (Chung et al., 2000). SDH catalyzes the conversion of succinic acid to fumaric acid in the tricarboxylic acid cycle, leading to the production of H+ and ATP (Ackrell et al., 1978). CCO provides energy to cells mainly through oxidative phosphorylation and is a key enzyme in the oxidative phosphorylation process in the mitochondrial respiratory chain (Millar et al., 1995). Cold stress affects the production of ATP by affecting mitochondrial structure and the activity of these enzymes, and the loss of ATP impairs metabolism and destroys cell structure; this further aggravates CI, resulting in a decline in the quality of horticultural products (Jin et al., 2015; Wang et al., 2020b; Hu et al., 2023). Altogether together, the results of this study demonstrate that fucoidan treatment can maintain mitochondrial function by improving the activities of relevant enzymes in cucumbers during cold storage, thereby ensuring continual energy synthesis and ultimately reducing the occurrence of CI.
Fucoidan treatment effectively reduced CI in cucumber fruits by modulating ROS scavenging and energy metabolism. Fucoidan treatment can reduce the accumulation of H2O2 and and the degree of membrane lipid peroxidation by increasing the activity of antioxidant enzymes and the expression of key genes. Furthermore, fucoidan treatment helps maintain high energy levels by regulating the activities of enzymes related to mitochondrial metabolism, thus effectively improving the resistance of cold-stored cucumber fruits to low-temperature stress and reducing the symptoms of cold injury.
The original contributions presented in the study are included in the article/Supplementary Material. Further inquiries can be directed to the corresponding author.
DL: Conceptualization, methodology, software, writing—original draft preparation. RY: validation, formal analysis, data curation, writing—original draft preparation. MX: investigation, resources. SL: visualization, data curation. JC: writing—review and editing, supervision. ZG: writing—review and editing, project administration, funding acquisition. All authors contributed to the article and approved the submitted version.
This work was supported by the National Natural Science Foundation of China (32060704), Natural Science Foundation of Jiangxi Province of China (20202BAB215005), and Jiangxi 2011 Col-laborative Innovation Center of Postharvest Key Technology and Quality Safety of Fruit and Vegetables, China (JXGS-05).
The authors declare that the research was conducted in the absence of any commercial or financial relationships that could be construed as a potential conflict of interest.
All claims expressed in this article are solely those of the authors and do not necessarily represent those of their affiliated organizations, or those of the publisher, the editors and the reviewers. Any product that may be evaluated in this article, or claim that may be made by its manufacturer, is not guaranteed or endorsed by the publisher.
The Supplementary Material for this article can be found online at: https://www.frontiersin.org/articles/10.3389/fpls.2022.1107687/full#supplementary-material
Ackrell, B. A., Kearney, E. B., Singer, T. P. (1978). Mammalian succinate dehydrogenase. Methods enzymol. 53, 466–483. doi: 10.1016/s0076-6879(78)53050-4
Ali, S., Anjum, M. A., Ejaz, S., Hussain, S., Ercisli, S., Saleem, M. S., et al. (2021). Carboxymethyl cellulose coating delays chilling injury development and maintains eating quality of 'kinnow' mandarin fruits during low temperature storage. Int. J. Biol. Macromol. 168, 77–85. doi: 10.1016/j.ijbiomac.2020.12.028
Azevedo, I. G., Oliveira, J. G., da Silva, M. G., Pereira, T., Corrêa, S. F., Vargas, H., et al. (2008). P-type h+-ATPases activity, membrane integrity, and apoplastic pH during papaya fruit ripening. Postharvest Biol. Technol. 48, 242–247. doi: 10.1016/j.postharvbio.2007.11.001
Cai, Y. T., Cao, S. F., Yang, Z. F., Zheng, Y. H. (2011). MeJA regulates enzymes involved in ascorbic acid and glutathione metabolism and improves chilling tolerance in loquat fruit. Postharvest Biol. Technol. 59, 324–326. doi: 10.1016/j.postharvbio.2010.08.020
Cao, S. F., Shao, J. R., Shi, L. Y., Xu, L. W., Shen, Z. M., Chen, W., et al. (2018). Melatonin increases chilling tolerance in postharvest peach fruit by alleviating oxidative damage. Sci. Rep. 8, 806. doi: 10.1038/s41598-018-19363-5
Chen, C. Y., Cai, N., Wan, C. P., Kai, W. B., Chen, J. Y. (2022). Carvacrol delays phomopsis stem-end rot development in pummelo fruit in relation to maintaining energy status and antioxidant system. Food Chem. 372, 131239. doi: 10.1016/j.foodchem.2021.131239
Chen, C. Y., Peng, X., Chen, J. Y., Gan, Z. Y., Wan, C. P. (2021a). Mitigating effects of chitosan coating on postharvest senescence and energy depletion of harvested pummelo fruit response to granulation stress. Food Chem. 348, 129113. doi: 10.1016/j.foodchem.2021.129113
Chen, L. L., Shan, W., Cai, D. L., Chen, J. Y., Lu, W. J., Su, X. G., et al. (2021b). Postharvest application of glycine betaine ameliorates chilling injury in cold-stored banana fruit by enhancing antioxidant system. Sci. Hortic. 287, 110264. doi: 10.1016/j.scienta.2021.110264
Chung, W. S., Lee, S. H., Kim, J. C., Heo, W. D., Kim, M. C., Park, C. Y., et al. (2000). Identification of a calmodulin-regulated soybean Ca(2+)-ATPase (SCA1) that is located in the plasma membrane. Plant Cell 12, 1393–1407. doi: 10.1105/tpc.12.8.1393
Duan, Z. H., Duan, W. W., Li, F. P., Li, Y. Q., Luo, P., Liu, H. Z. (2019). Effect of carboxymethylation on properties of fucoidan from Laminaria japonica: Antioxidant activity and preservative effect on strawberry during cold storage. Postharvest Biol. Technol. 151, 127–133. doi: 10.1016/j.postharvbio.2019.02.008
Duan, M., Ma, N. N., Li, D., Deng, Y. S., Kong, F. Y., Lv, W., et al. (2012). Antisense-mediated suppression of tomato thylakoidal ascorbate peroxidase influences anti-oxidant network during chilling stress. Plant Physiol. Biochem. 58, 37–45. doi: 10.1016/j.plaphy.2012.06.007
Ehteshami, S., Abdollahi, F., Ramezanian, A., Dastjerdi, A. M., Rahimzadeh, M. (2019). Enhanced chilling tolerance of pomegranate fruit by edible coatings combined with malic and oxalic acid treatments. Sci. Hortic. 250, 388–398. doi: 10.1016/j.scienta.2019.02.075
Endo, H., Miyazaki, K., Ose, K., Imahori, Y. (2019). Hot water treatment to alleviate chilling injury and enhance ascorbate-glutathione cycle in sweet pepper fruit during postharvest cold storage. Sci. Hortic. 257, 108715. doi: 10.1016/j.scienta.2019.108715
Gan, Z. Y., Yuan, X., Shan, N., Wan, C. P., Chen, C. Y., Zhu, L. Q., et al. (2021). AcERF1b and AcERF073 positively regulate indole-3-acetic acid degradation by activating AcGH3.1 transcription during postharvest kiwifruit ripening. J. Agric. Food Chem. 69, 13859–13870. doi: 10.1021/acs.jafc.1c03954
Gill, S. S., Tuteja, N. (2010). Reactive oxygen species and antioxidant machinery in abiotic stress tolerance in crop plants. Plant Physiol. Biochem. 48, 909–930. doi: 10.1016/j.plaphy.2010.08.016
Hu, S. Q., Hou, Y. Y., Zhao, L. Y., Zheng, Y. H., Jin, P. (2023). Exogenous 24-epibrassinolide alleviates chilling injury in peach fruit through modulating PpGATA12-mediated sucrose and energy metabolisms. Food Chem. 400, 133996. doi: 10.1016/j.foodchem.2022.133996
Jin, P., Zhang, Y., Shan, T. M., Huang, Y. P., Xu, J., Zheng, Y. H. (2015). Low-temperature conditioning alleviates chilling injury in loquat fruit and regulates glycine betaine content and energy status. J. Agric. Food Chem. 63, 3654–3659. doi: 10.1021/acs.jafc.5b00605
Jin, P., Zhu, H., Wang, J., Chen, J. J., Wang, X. L., Zheng, Y. H. (2013). Effect of methyl jasmonate on energy metabolism in peach fruit during chilling stress. J. Sci. Food Agric. 93, 1827–1832. doi: 10.1002/jsfa.5973
Li, X., Bao, Z. Y., Chen, Y. N., Lan, Q. Q., Song, C. B., Shi, L. Y., et al. (2023). Exogenous glutathione modulates redox homeostasis in okra (Abelmoschus esculentus) during storage. Postharvest Biol. Technol. 195, 112145. doi: 10.1016/j.postharvbio.2022.112145
Li, C. Y., Cheng, Y., Hou, J. B., Zhu, J., Sun, L., Ge, Y. H. (2021a). Application of methyl jasmonate postharvest maintains the quality of nanguo pears by regulating mitochondrial energy metabolism. J. Integr. Agric. 20, 3075–3083. doi: 10.1016/S2095-3119(21)63611-0
Li, H. Z., Jiang, X. C., Lv, X. Z., Ahammed, G. J., Guo, Z. X., Qi, Z. Y., et al. (2019). Tomato GLR3.3 and GLR3.5 mediate cold acclimation-induced chilling tolerance by regulating apoplastic H2O2 production and redox homeostasis. Plant Cell Environ. 42, 3326–3339. doi: 10.1111/pce.13623
Li, Y. L., Liu, Y. F., Zhang, J. G. (2010). Advances in the research on the AsA-GSH cycle in horticultural crops. Front. Agric. China 4, 84–90. doi: 10.1007/s11703-009-0089-8
Li, D., Li, L., Xiao, G., Limwachiranon, J., Xu, Y., Lu, H., et al. (2018). Effects of elevated CO2 on energy metabolism and gamma-aminobutyric acid shunt pathway in postharvest strawberry fruit. Food Chem. 265, 281–289. doi: 10.1016/j.foodchem.2018.05.106
Liu, Q. L., Li, X., Jin, B. S., Dong, W. Q., Zhang, Y., Chen, W., et al. (2023). γ-aminobutyric acid treatment induced chilling tolerance in postharvest kiwifruit (Actinidia chinensis cv. hongyang) via regulating ascorbic acid metabolism. Food Chem. 404, 134661. doi: 10.1016/j.foodchem.2022.134661
Li, D., Wang, D., Fang, Y. D., Li, L., Lin, X. Y., Xu, Y. Q., et al. (2021b). A novel phase change coolant promoted quality attributes and glutamate accumulation in postharvest shiitake mushrooms involved in energy metabolism. Food Chem. 351, 129227. doi: 10.1016/j.foodchem.2021.129227
Luo, P., Li, F. P., Liu, H. Z., Yang, X. M., Duan, Z. H. (2020). Effect of fucoidan-based edible coating on antioxidant degradation kinetics in strawberry fruit during cold storage. J. Food Process. Preserv. 44, e14381. doi: 10.1111/jfpp.14381
Madebo, M. P., Luo, S. M., Wang, L., Zheng, Y. H., Jin, P. (2021). Melatonin treatment induces chilling tolerance by regulating the contents of polyamine, γ-aminobutyric acid, and proline in cucumber fruit. J. Integr. Agric. 20, 3060–3074. doi: 10.1016/S2095-3119(20)63485-2
Millar, A. H., Atkin, O. K., Lambers, H., Wiskich, J. T., Day, D. A. (1995). A critique of the use of inhibitors to estimate partitioning of electrons between mitochondrial respiratory pathways in plants. Physiol. Plant. 95, 523–532. doi: 10.1034/j.1399-3054.1995.950404.x
Mirshekari, A., Madani, B., Yahia, E. M., Golding, J. B., Vand, S. H. (2020). Postharvest melatonin treatment reduces chilling injury in sapota fruit. J. Sci. Food Agric. 100, 1897–1903. doi: 10.1002/jsfa.10198
Mittler, R., Zandalinas, S. I., Fichman, Y., Van Breusegem, F. (2022). Reactive oxygen species signalling in plant stress responses. Nat. Rev. Mol. Cell Biol. 23, 663–679. doi: 10.1038/s41580-022-00499-2
Nasef, I. N. (2018). Short hot water as safe treatment induces chilling tolerance and antioxidant enzymes, prevents decay and maintains quality of cold-stored cucumbers. Postharvest Biol. Technol. 138, 1–10. doi: 10.1016/j.postharvbio.2017.12.005
Peng, X., Zhang, Y. A., Wan, C. P., Gan, Z. Y., Chen, C. Y., Chen, J. Y. (2022). Antofine triggers the resistance against penicillium italicum in ponkan fruit by driving AsA-GSH cycle and ROS-scavenging system. Front. Microbiol. 13. doi: 10.3389/fmicb.2022.874430
Ru, L., Jiang, L. F., Wills, R. B. H., Golding, J. B., Huo, Y. R., Yang, H. Q., et al. (2020). Chitosan oligosaccharides induced chilling resistance in cucumber fruit and associated stimulation of antioxidant and HSP gene expression. Sci. Hortic. 264, 109187. doi: 10.1016/j.scienta.2020.109187
Saquet, A. A., Streif, J., Bangerth, F. (2003). Energy metabolism and membrane lipid alterations in relation to brown heart development in 'Conference' pears during delayed controlled atmosphere storage. Postharvest Biol. Technol. 30, 123–132. doi: 10.1016/s0925-5214(03)00099-1
Senthilkumar, K., Manivasagan, P., Venkatesan, J., Kim, S. K. (2013). Brown seaweed fucoidan: biological activity and apoptosis, growth signaling mechanism in cancer. Int. J. Biol. Macromol. 60, 366–374. doi: 10.1016/j.ijbiomac.2013.06.030
Song, L. L., Wang, J. H., Shafi, M., Liu, Y., Wang, J., Wu, J. S., et al. (2016). Hypobaric treatment effects on chilling injury, mitochondrial dysfunction, and the ascorbate-glutathione (AsA-GSH) cycle in postharvest peach fruit. J. Agric. Food Chem. 64, 4665–4674. doi: 10.1021/acs.jafc.6b00623
Song, C. B., Wang, K., Xiao, X., Liu, Q. L., Yang, M. J., Li, X., et al. (2022). Membrane lipid metabolism influences chilling injury during cold storage of peach fruit. Food Res. Int. 157, 111249. doi: 10.1016/j.foodres.2022.111249
Tan, X. L., Zhao, Y. T., Shan, W., Kuang, J. F., Lu, W. J., Su, X. G., et al. (2020). Melatonin delays leaf senescence of postharvest Chinese flowering cabbage through ROS homeostasis. Food Res. Int. 138, 109790. doi: 10.1016/j.foodres.2020.109790
Tao, D., Wang, J., Zhang, L., Jiang, Y., Lv, M. (2019). 1-methylcyclopropene alleviates peel browning of 'Nanguo' pears by regulating energy, antioxidant and lipid metabolisms after long term refrigeration. Sci. Hortic. 247, 254–263. doi: 10.1016/j.scienta.2018.12.025
Tian, J. X., Xie, S. Y., Zhang, P., Wang, Q., Li, J. K., Xu, X. B. (2022). Attenuation of postharvest peel browning and chilling injury of banana fruit by Astragalus polysaccharides. Postharvest Biol. Technol. 184, 111783. doi: 10.1016/j.postharvbio.2021.111783
Tsuchida, H., Kozukue, N., Han, G. P., Choi, S. H., Levin, C. E., Friedman, M. (2010). Low-temperature storage of cucumbers induces changes in the organic acid content and in citrate synthase activity. Postharvest Biol. Technol. 58, 129–134. doi: 10.1016/j.postharvbio.2010.06.006
Wang, H. B., Cheng, X., Wu, C. E., Fan, G. J., Li, T. T., Dong, C. (2021b). Retardation of postharvest softening of blueberry fruit by methyl jasmonate is correlated with altered cell wall modification and energy metabolism. Sci. Hortic. 276, 109752. doi: 10.1016/j.scienta.2020.109752
Wang, Q., Ding, T., Zuo, J. H., Gao, L. P., Fan, L. L. (2016). Amelioration of postharvest chilling injury in sweet pepper by glycine betaine. Postharvest Biol. Technol. 112, 114–120. doi: 10.1016/j.postharvbio.2015.07.008
Wang, T., Hu, M., Yuan, D., Yun, Z., Gao, Z., Su, Z., et al. (2020c). Melatonin alleviates pericarp browning in litchi fruit by regulating membrane lipid and energy metabolisms. Postharvest Biol. Technol. 160, 111066. doi: 10.1016/j.postharvbio.2019.111066
Wang, B., Shen, F., Zhu, S. J. (2018). Proteomic analysis of differentially accumulated proteins in cucumber (Cucumis sativus) fruit peel in response to pre-storage cold acclimation. Front. Plant Sci. 8. doi: 10.3389/fpls.2017.02167
Wang, B., Wang, G., Zhu, S. J. (2020a). DNA Damage inducible protein 1 is involved in cold adaption of harvested cucumber fruit. Front. Plant Sci. 10. doi: 10.3389/fpls.2019.01723
Wang, B., Wu, C. S., Wang, G., He, J. M., Zhu, S. J. (2021a). Transcriptomic analysis reveals a role of phenylpropanoid pathway in the enhancement of chilling tolerance by pre-storage cold acclimation in cucumber fruit. Sci. Hortic. 288, 110282. doi: 10.1016/j.scienta.2021.110282
Wang, F., Yang, Q. Z., Zhao, Q. F., Zhang, X. P. (2020b). Roles of antioxidant capacity and energy metabolism in the maturity-dependent chilling tolerance of postharvest kiwifruit. Postharvest Biol. Technol. 168, 111281. doi: 10.1016/j.postharvbio.2020.111281
Wang, J. D., Zhao, Y. Q., Ma, Z. Q., Zheng, Y. H., Jin, P. (2022). Hydrogen sulfide treatment alleviates chilling injury in cucumber fruit by regulating antioxidant capacity, energy metabolism and proline metabolism. Foods 11, 2749. doi: 10.3390/foods11182749
Wu, L., Sun, J., Su, X., Yu, Q. L., Yu, Q. Y., Zhang, P. (2016). A review about the development of fucoidan in antitumor activity: progress and challenges. Carbohydr. Polymers 154, 96–111. doi: 10.1016/j.carbpol.2016.08.005
Xu, B., Wu, S. (2021). Preservation of mango fruit quality using fucoidan coatings. LWT-Food Sci. Technol. 143, 111150. doi: 10.1016/j.lwt.2021.111150
You, J., Chan, Z. L. (2015). ROS regulation during abiotic stress responses in crop plants. Front. Plant Sci. 6. doi: 10.3389/fpls.2015.01092
Yu, K. B., Zhou, L., Xu, J., Jiang, F. H., Zhong, Z. W., Zou, L. Q., et al. (2022). Carboxymethyl cellulose-based water barrier coating regulated postharvest quality and ROS metabolism of pakchoi (Brassica chinensis l.). Postharvest Biol. Technol. 185, 111804. doi: 10.1016/j.postharvbio.2021.111804
Zhang, H. Y., Wang, Y. N., Han, T., Li, L. P., Xu, L. (2008). Effect of exogenous glycine betaine on chilling injury and chilling-resistance parameters in cucumber fruits stored at low temperature. Sci. Agricult. Sin. 41, 2407–2412. doi: 10.3724/SP.J.1011.2008.00145
Zhang, Y., Wang, K., Xiao, X., Cao, S. F., Chen, W., Yang, Z. F., et al. (2021). Effect of 1-MCP on the regulation processes involved in ascorbate metabolism in kiwifruit. Postharvest Biol. Technol. 179, 111563. doi: 10.1016/j.postharvbio.2021.111563
Keywords: cucumber fruit, chilling injury, fucoidan, antioxidant capacity, energy metabolism
Citation: Lin D, Yan R, Xing M, Liao S, Chen J and Gan Z (2022) Fucoidan treatment alleviates chilling injury in cucumber by regulating ROS homeostasis and energy metabolism. Front. Plant Sci. 13:1107687. doi: 10.3389/fpls.2022.1107687
Received: 25 November 2022; Accepted: 12 December 2022;
Published: 23 December 2022.
Edited by:
Zhenfeng Yang, Zhejiang Wanli University, ChinaReviewed by:
Zhengke Zhang, Hainan University, ChinaCopyright © 2022 Lin, Yan, Xing, Liao, Chen and Gan. This is an open-access article distributed under the terms of the Creative Commons Attribution License (CC BY). The use, distribution or reproduction in other forums is permitted, provided the original author(s) and the copyright owner(s) are credited and that the original publication in this journal is cited, in accordance with accepted academic practice. No use, distribution or reproduction is permitted which does not comply with these terms.
*Correspondence: Zengyu Gan, Z2FuenlAanhhdS5lZHUuY24=
†These authors have contributed equally to this work
Disclaimer: All claims expressed in this article are solely those of the authors and do not necessarily represent those of their affiliated organizations, or those of the publisher, the editors and the reviewers. Any product that may be evaluated in this article or claim that may be made by its manufacturer is not guaranteed or endorsed by the publisher.
Research integrity at Frontiers
Learn more about the work of our research integrity team to safeguard the quality of each article we publish.