- 1School of Forestry, Northeast Forestry University, Harbin, Heilongjiang, China
- 2Key Laboratory of Sustainable Forest Ecosystem Management-Ministry of Education, Northeast Forestry University, Harbin, China
Canopy leaves are sinks of dry and wet nitrogen (N) deposition, most studies have not considered the response of canopy leaves to the alternating processes of dry and wet N deposition. We manipulated a close top chamber experiment to observe the effects of simulated N deposition with the same total deposition flux but different dry to wet ratios on leaf structure and physiology by spraying NH4Cl solution or supplying gaseous NH3 over the canopy of seedlings of three species (Betula platyphylla, Fraxinus mandshurica, Pinus koraiensis) placed in the chamber. After 32 days of N deposition and relative to the control, the leaf morphology and mesophyll tissue structure of the three species had no significant changes under all N deposition treatments. With the increase in the ratio of dry to wet N deposition, the N concentration, N metabolizing enzyme activity and soluble protein concentration in leaves of all three species increased continuously, but for the leaf light-saturated net photosynthesis rate, B. platyphylla showed a continuous increase, F. mandshurica showed a continuous decrease, and P. koraiensis showed no significant change. We found that F. mandshurica was the only species whose foliar chlorophyll and potassium concentration decreased with the increase in the ratio of dry to wet N deposition and its leaf light-saturated net photosynthesis rate was positively correlated with foliar chlorophyll and potassium concentration, respectively. Our results indicate that dry deposition is relatively more important on leaf physiological functions in alternating deposition. B. platyphylla and P. koraiensis may better acclimate to canopy NH3/NH4+ deposition than F. mandshurica. Most importantly, the results indicate that a single simulated dry and wet deposition would overestimate and underestimate the response of leaf function to atmospheric N deposition, respectively. Alternating processes of dry and wet deposition should be considered for more realistic assessments of the effects of atmospheric N deposition in forests.
1. Introduction
Human activities have led to changes in the reactive nitrogen (Nr) component (NHx, reduced form; NOy, oxidized form) of nitrogen (N) deposition over much of the globe, NHx is gradually becoming the dominant form of deposited Nr (Gu et al., 2015; Li et al., 2016; Warner et al., 2017; Liu et al., 2020a). Previous studies on the response of plant morphology and function to N deposition have been based mainly on two independent types of experiments of spraying dissolved state Nr to the soil or canopy leaves (Liu et al., 2018; Liu et al., 2020c; Li et al., 2021) and exposing plants to gaseous state Nr (Sheppard et al., 2011). However, the alternating dry and wet N deposition caused by rainfall together constitute a significant component of N deposition in natural ecosystems (Pan et al., 2012; Xu et al., 2015; Liu et al., 2020a). The contribution of dry and wet N deposition to total N deposition per unit time varies greatly between geographic regions. For example, in China, the contribution of dry N deposition to annual total N deposition ranged from 21% to 71.9% (Pan et al., 2012; Shen et al., 2013). The morphology and function of plants will be altered by both dry and wet N deposition (Adrizal et al., 2006; Sparks, 2009; Khan et al., 2020; Zhu et al., 2020), so studies that neglect the alternating processes of dry and wet N deposition may incorrectly estimate the impacts of N deposition on plant growth.
The canopy is the first tissue of plants to come into contact with dry and wet N deposition. These deposited gaseous and dissolved Nr are mainly intercepted by the canopy leaves and absorbed by ion exchange in the cuticle and/or simple diffusion in the stomata and enter the apoplast (Rennenberg and Gessler, 1999; Sparks, 2009), which are then transported to the cells and assimilated and utilized by various enzyme systems such as nitrate reductase (NR), glutamine synthetase/glutamate synthase (GS/GOGAT) cycle in chloroplasts or plastids (Krupa, 2003; Sparks, 2009). This process eventually leads to an increase in leaf N content and N metabolites, which in turn induces physiological responses and affects photosynthetic carbon assimilation capacity (Castro et al., 2008; Mao et al., 2018; Hu et al., 2019; Wu et al., 2022), and also causes changes in leaf morphological and anatomical traits (Zhu et al., 2020; Khan et al., 2020). Therefore, canopy leaves are also the first vegetative organ of plants to respond to dry and wet N deposition.
However, in the results of previous gaseous and dissolved Nr addition studies, plants exposed to certain concentrations of gaseous Nr generally had higher N concentrations or recovery in leaves and shoots than when dissolved Nr was applied to the root medium or canopy. (Pérez-Soba and van der Eerden, 1993; Castro et al., 2008; Wang et al., 2021). For example, Jones et al. (2008) supplied 15NH3 to Calluna vulgaris in the flux chamber and found that 22% of applied NH3 was retained by leaf stomata and 53% by green shoots and leaf cuticles. Adriaenssens et al. (2012) sprayed four temperate tree species with 15NH4+ and 15NO3- solutions and found that only 1-3% of the applied N was retained by foliage and twigs. This difference indicated that plants may respond more rapidly and to a greater extent to dry N deposition than to wet N deposition, for example, Sheppard et al. (2011) found that dry deposition of gaseous NH3 drives cover of ombrotrophic bog species change faster than equivalent doses of wet deposition of NH4Cl solution.
Although previous studies conducted by applying gaseous or dissolved Nr to the canopies have improved our understanding of the retention, assimilation of Nr in plant canopy leaves, however, applying a single state of Nr is not representative of the N deposition in natural ecosystems, and the response of plants to it may also not accurately assess the effects of atmospheric N deposition on plants or ecosystems. Our understanding of the response of photosynthesis and photosynthetic system components in canopy leaves to the alternating processes of dry and wet N deposition and leaf sensitivity/tolerance to different states of Nr remains incomplete. Therefore, under the change of composition and state of atmospheric N deposition (Li et al., 2016; Warner et al., 2017; Liu et al., 2020a), revealing the changes in leaves morphological, anatomical and physiological traits under the alternating processes of dry and wet NHx deposition is critical for a more comprehensive understanding and accurate assessment of the effects of N deposition on plants and even forest ecosystem.
For many tree species, NH4+ toxicity will occur in the condition of high NH4+-level root medium, and trees usually show different NH4+ sensitivity/tolerance in response to increased NH4+ levels in the root medium due to different levels of preference for NH4+ (Cui and Song, 2007; Esteban et al., 2016). This sensitivity depends on tree species and their life forms and growth character (Cui and Song, 2007; Esteban et al., 2016). In this study, we conducted a short-term close top chamber experiment to simulate NHx deposition with different dry to wet ratios over the canopy of seedlings of three species: Betula platyphylla (lower NH4+ sensitivity) (Crabtree and Bazzaz, 1993; Zhang and Cui, 2011), Fraxinus mandshurica (higher NH4+ sensitivity) (Zhang et al., 2000; Wu et al., 2003), Pinus koraiensis (lower NH4+ sensitivity) (Cui and Song, 2007). Our aim was to more comprehensively understand the effects of atmospheric N deposition on plant canopy leaves by comparing the leaf morphology, anatomical structure and physiology responses of different NH4+-sensitive seedlings under simulated NHx deposition. We hypothesized that: (1) at the same total N deposition flux, dry deposition would have a greater effect on the morphology, anatomy and physiology of plant leaves than wet deposition; (2) the sensitivity of trees to canopy deposited NHx and to NH4+ in the root medium would be similar; this hypothesis was developed based on the findings of some previous studies that plants also show different sensitivity/tolerance in response to increased environmental NH3 concentration (Pitcairn et al., 1998; Adrizal et al., 2006).
2. Materials and methods
2.1. Plant material
In May 2021, B. platyphylla clones obtained from tissue culture were prepared in nutrient pots in a greenhouse, and then transferred to new nutrient pots (10×10×10 cm) together with one-year-old F. mandshurica seedlings and two-year-old P. koraiensis seedlings in late June. The source of culture substrate remained the same, which were 1:1 (v: v) black soil-vermiculite, black soil, 1:1 black soil-vermiculite respectively. In early July, 72 relatively uniform seedlings of each species were selected according to their height and basal diameter and transferred to the chamber for one week before starting the experiment. Each chamber contains three species, with 12 seedlings per species. The characteristics of the plant material and soil are shown in Table 1.
2.2. Experimental design
The close top chamber experiment was performed in the greenhouse of Northeast Forestry University in Harbin, China. The temperature in the chamber during the experiment was 25-32°C, the relative humidity was 55-60%, and the maximum illuminance was 23,600lux. We used a split-plot design, the main plots are six N application treatments conducted within six chambers respectively, three replicates of each treatment. N application treatments included a control that applied activated carbon filtered ambient air and deionized water, and five types of N deposition with the same total deposition flux but different dry to wet ratios simulated on the basis of control (0D+100W, the ratio of dry to wet N deposition (RDry/Wet) was 0:100; 25D+75W, RDry/Wet was 25:75; 50D+50W, RDry/Wet was 50:50; 75D+25W, RDry/Wet was 75:25; 100D+0W, RDry/Wet was 100:0). Three species were assigned to each split plots by complete block design.
The simulated alternating processes of dry and wet N deposition are composed of multiple 4-day dry and wet deposition cycles. One cycle of the 0D+100W treatment is composed of four consecutive days of wet deposition, one cycle of the 25D+75W treatment is composed of one day of dry deposition and three consecutive days of wet deposition, one cycle of the 50D+50W treatment is composed of two consecutive days of dry deposition and two consecutive days of wet deposition, one cycle of the 75D+25W treatment is composed of three day of dry deposition and one day of wet deposition and one cycle of the 100D+0W treatment is composed of four consecutive days of dry deposition.
The dry deposition was simulated by injecting gaseous NH3 at a concentration of 330 ± 10 μg m-3 of N into the chamber for 14 hours (5:00 h-19:00 h) per day. The ambient air compressed by the air compressor is pumped into the chamber (0.6×0.6×0.7 m) from the top of the chamber after passing through the reduction valve, activated carbons (to remove NH3 from the ambient air), rotor flowmeter and gas mixer in turn, the flow rate is 30 L min-1. NH3 is injected into the gas mixer from a cylinder containing with a calibrated gas mixture of 0.1% NH3 in N2 and mixed with the pumped air, adjusted to the desired concentration by rotor flowmeter. All tubes in contact with NH3 are made of Teflon, fans are installed in the chamber to reduce the difference in NH3 concentration over horizontal space. NH3 concentration in the inlet and outlet of the chamber is measured by pump suction NH3 detector (JA908-NH3; Yongqi Co. Ltd, GuangDong, China) and NH3 detection tube (3CG Ammonia; GASTEC, Tokyo, Japan). The gas exchange rate in the chamber was 30 L min-1 and the concentration of NH3 emitted from each chamber vent during the simulated dry deposition is less than 28 μg m-3 of N. After correcting the amount of NH3 adsorption by the chamber inner wall, the dry N deposition flux was calculated from the difference of NH3 concentration between the inlet and outlet of the chamber. The daily dry deposition flux was 7.06 ± 0.25 mg of N (see Figure 1).The wet deposition was simulated by uniformly spraying 305 ml of NH4Cl solution at a concentration of 23.1 mg L-1 of N over the canopy at 05:00 h per day. The daily wet deposition flux was 7.05 mg of N (see Figure 1). The total deposition flux for each N application treatment was equivalent to 72 kg ha-1 yr-1 of N, which was close to the high N level in the range of N deposition rates (0-70 kg ha-1 yr-1 of N) in different geographical regions of China (Wen et al., 2020).
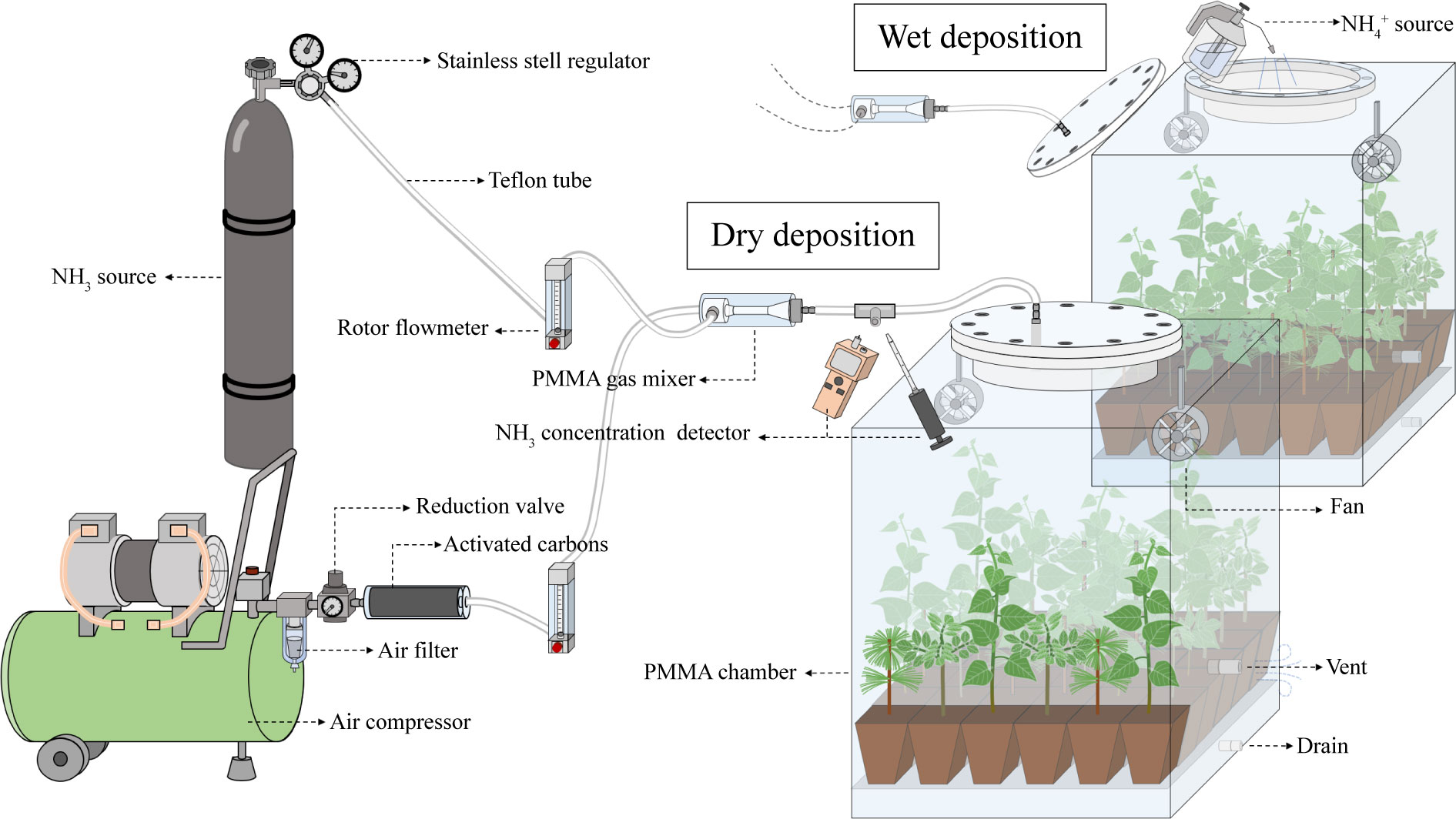
Figure 1 A schematic diagram showing the materials used to simulating dry and wet N deposition The ambient air compressed by the air compressor is pumped into the chamber from the top of the chamber after passing through the reduction valve, activated carbons, rotor flowmeter and gas mixer in turn. NH3 is injected into the gas mixer from a cylinder and mixed with the pumped air, and adjusted to the desired concentration by rotor flowmeter to simulate dry deposition. The top of the chamber consists of a flange interface that can be opened and used to simulate wet deposition. PMMA, polymethyl methacrylate.
Each N deposition treatment had the same gas exchange rate and the amount of deionized water applied. The same amount of water (deionized water, 305 ml) as in the simulated wet deposition was applied daily in the control and simulated dry deposition. To avoid additional adsorption of NH3 by the water film formed on the leaves after canopy spraying water (which is not representative of true dry N deposition process), the deionized water in the dry deposition treatment was applied to the soil surface through a syringe.
2.3. Leaf photosynthesis and sample collection
Between 09:00 h and 11:30 h on days 17 and 33 after the start of the N application, leaf light-saturated net photosynthesis rate (Pmax) was measured in situ using a LI-6400 with an integrated LED light source (Li-Cor, Lincoln, NE, USA). The chamber CO2 concentration was maintained at ambient level. To obtain light-saturated rates of photosynthesis, the leaf in the chamber was illuminated at 1000 μmol m-2 s-1 photosynthetic photon flux density. According to preliminary trials, we found that the CO2 uptake of all three species light saturated at 1000 μmol photons m-2 s-1. For each measurement, we randomly selected five seedlings from each species in per treatment. We selected mature, healthy, and integrated leaves located at the 3rd and 4th position from the top of B. platyphylla, the 1st and 2nd position from the top of compound leaves of F. mandshurica, and two clusters of current-year needles of P. koraiensis to measure Pmax. Recalibrate the Pmax of the needle after measuring the projected area of the needles. Averaged values of these two leaves (two clusters of needles) were used to represent the response of this seedling.
After the second photosynthesis measurements, leaf sampling was performed. The leaves that had been used for photosynthesis measurements were collected, part of the sample was wrapped in tinfoil and stored in liquid nitrogen, while the other part of the sample was put into a sealed plastic bag and placed in an ice box. we then randomly selected five seedlings from each species in per treatment, leaves located at the 3rd to 7th position from the top of B. platyphylla, the 2nd-6th position from the top of compound leaves of F. mandshurica and the current-year needles of P. koraiensis were collected. All samples collected were mature, healthy and integrated. Two leaves (two clusters of needles) from each seedling were gently washed in deionized water and immediately chemically fixed in Formalin-Aceto-Alcohol (FAA) solution (70% ethanol: glycerine: glacial acetic acid = 18:1:1) separately, the rest of the leaves were put into separate sealed plastic bag and placed in an ice box. All fresh samples were transported to the laboratory for immediate processing or analysis, samples stored in liquid nitrogen were transported to the laboratory and stored at -80°C and fixed samples were stored at 4°C, until further analysis.
2.4. Leaf morphology and foliar chemistry
The fresh leaf samples that were not used for photosynthesis measurements were washed with deionized water and fresh weight was measured. The leaf samples are scanned by a color scanner (12000XL; Expression, Nagano, Japan), and the scanned images were analyzed with root system analyzer software (WinRHIZO Pro 2016; Regent instruments Co., Ltd, Québec, Canada) to determine leaf area and projected area of needles (LA). The leaf samples were then oven-dried at 65°C until constant weight then weighed. Leaf mass per area (LMA) and specific leaf area (SLA) were calculated. Dried leaf samples were ground into fine powder using a ball mill (MM400; Retsch, Haan, Germany). The N concentration was determined using a macro elemental analyzer (vario MACRO CN; Elementar, Langenselbold, Germany). To measure the concentration of phosphorus (P) and potassium (K) in leaves, the fine powder was digested with sulphuric and perchloric acid. The P concentration was determined using a continuous flow analyzer (Auto Analyzer 3; SEAL Analytical Co., Ltd, Norderstedt, Germany), based on the ascorbic acid molybdate analysis, and the K concentration was determined using a flame photometer.
2.5. Foliar soluble proteins and photosynthetic pigments
For photosynthetic pigments analysis, fresh leaves that had been used for photosynthesis measurements were shredded after removing the major vein, 0.1g of shredded leaf tissue was placed in 10 ml of 80% acetone in the dark for 24 h, and centrifuged at 10,000g for 10 min. The supernatant was scanned at λ663, λ645 and λ470 (TU-1950; Presee Co., Ltd, Beijing, China) and the concentrations of chlorophylls (chlorophyll/Chla, Chlb) and carotenoids were quantified as per Zhang et al. (2020). For soluble protein analysis, leaves stored at -80°C were shredded after removing the major veins, 0.2 g of shredded leaf tissue were homogenized in 5ml deionized water, and then centrifuged at 10,000g for 30 min, and the supernatant was used for protein analysis according to the Kormas Brilliant Blue method.
2.6. Foliar nitrogen-metabolizing enzymes
The activities of nitrate reductase (NR, EC 1.6.6.1), glutamine synthetase (GS, EC 6.3.1.2) and glutamate synthase (Fd-GOGAT EC 1.4.7.1) in leaves stored at -80°C were measured using enzyme-linked immunosorbent assay (ELISA). Solid-phase antibody was made using purified plant NR (or GS, GOGAT) antibody. Then, combined with antibody labelled with horseradish peroxidase (HRP), NR (or GS, Fd-GOGAT) was added to microtiter plate wells to become an antibody-antigen-enzyme-antibody complex. This complex became blue with 3,3′,5,5′-tetramethyl benzidine (TMB) substrate solution after complete washing and converted to final yellow under the action of acid. The optical density (OD) values were measured spectrophotometrically at a wavelength of 450 nm to compare with the standard curves to determine the activity of NR (or GS, Fd-GOGAT) in the samples.
2.7. Leaf tissue anatomy
Leaf anatomical traits were determined following the protocols described by Guo et al. (2008). Leaves of broadleaved species fixed in the FAA were cut into small pieces of 1×2 cm along the main veins in the middle part of the leaves, and fixed needles were cut into small segments of 0.5 cm in length in their middle part. This excised leaf tissue was embedded in paraffin individually after dehydration by immersion in a sequence of alcohol solutions and cut into sections of 8μm thickness and then stained with safranin-fast green. The slides were photographed under a compound microscope (BX51; Olympus, Tokyo, Japan). The abaxial (ABE) and adaxial (ADE) epidermis thickness, spongy mesophyll thickness (SMT), palisade mesophyll thickness (PMT) and leaf thickness of broadleaved species, the combined epi-hypodermis thickness (EHT) and mesophyll area of P. koraiensis were extracted from the images using Motic Images Advanced 3.2.
2.8. Statistical analyses
The effect of N deposition on various traits of leaf morphology, anatomical structure, and physiology were assessed using one-way ANOVA, and least significant difference tests were conducted to compare means of these trait variables among treatments. Linear regression analyses were used to determine the relationships between Pmax and foliar K concentration, foliar chlorophyll concentration. All variables were tested for the normality of their distributions. Nonnormally distributed variables were log-transformed to meet normality assumptions before performing ANOVA. All statistical analysis mentioned above was performed using the SPSS software v19.0 (SPSS Inc., Chicago, IL, USA).
3. Results
3.1. Foliar chemistry
Under N application treatments, the foliar N concentrations of the three species increased continually with the increase in RDry/Wet (Figure 2A). Relative to the control, the foliar N concentrations of the three species under the 0D+100W treatment did not show significant differences, while the foliar N concentrations of B. platyphylla, F. mandshurica and P. koraiensis under the 100D+0W treatment increased by 16.6% (P = 0.051), 21.5% (P = 0.101) and 19.2% (P < 0.01), respectively. The foliar P concentrations of B. platyphylla and P. koraiensis did not show significant differences among all treatments, only the foliar P concentration of F. mandshurica decreased continually with the increase in RDry/Wet (Figure 2B). Relative to the control, the foliar K concentrations of B. platyphylla and P. koraiensis under the 0D+100W treatment decreased by 14.6% (P = 0.017) and 10.3% (P = 0.254), respectively, but increased continually with the increase in RDry/Wet. The foliar K concentration of both B. platyphylla and P. koraiensis under the 100D+0W treatment was not significantly different compared with that under the control (Figure 2C). It is noteworthy that the foliar K concentration of F. mandshurica did not show significant differences among all treatments but showed a continuous decrease with the increase in RDry/Wet. Relative to the control, the foliar K concentration of F. mandshurica under the 100D+0W treatment decreased by 19.8% (P = 0.041) (Figure 2C).
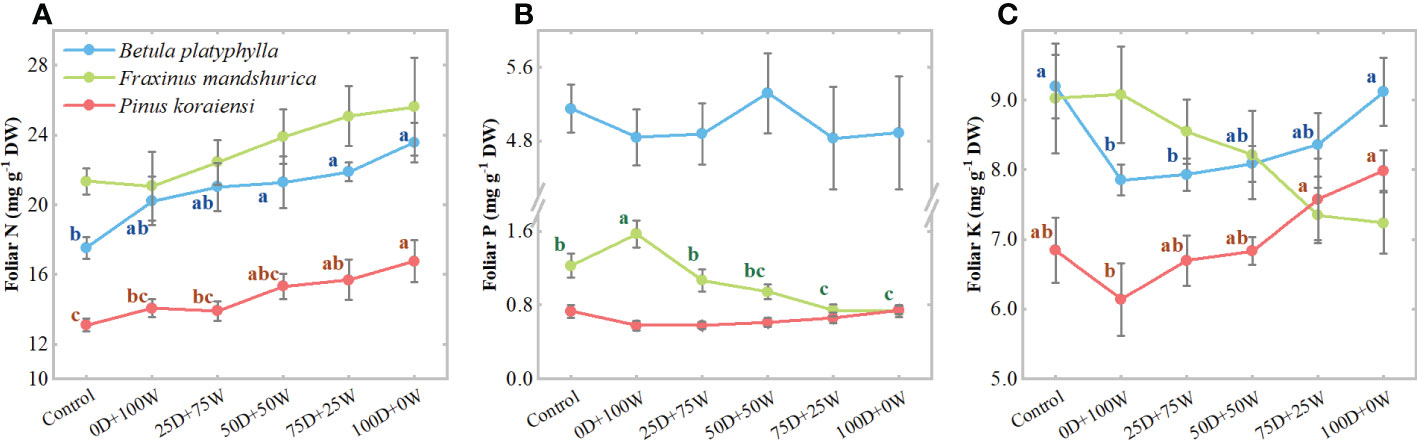
Figure 2 Concentrations (mg g-1 DW, A–C) of foliar: nitrogen (N), phosphorus (P) and potassium (K) of three species affected by six canopy N application treatments for 32 days. N application treatments included a control (no application of N) and five types of N deposition with dry to wet ratios of 0:100 (0D+100W), 25:75 (25D+75W), 50:50 (50D+50W), 75:25 (75D+25W) and 100:0 (100D+0W), respectively. Error bars indicate the standard error of the mean (n = 5). Within the same species in each panel, different letters on the left side of the error bar demonstrate significant differences among six treatments (P < 0.05) according to the least significant difference test. The scales of the y-axis are different in each panel.
3.2. Nitrogen -metabolizing enzymes activities
Relative to the control, except for the reduced NR activity in B. platyphylla leaves under the 0D+100W and 25D+75W treatments, the activities of NR, GS, and GOGAT in leaves of all three species increased under each N application treatment and increased continually with the increase in RDry/Wet (Figures 3A–C). Compared with the 0D+100W treatment, the NR activities in B. platyphylla leaves, F. mandshurica leaves and P. koraiensis needles under the 100D+0W treatment increased by 9.0% (P < 0.01), 14.5% (P < 0.01) and 15.1% (P < 0.01), respectively; the GS activities in B. platyphylla leaves, F. mandshurica leaves and P. koraiensis needles under the 100D+0W treatment increased by 6.7% (P = 0.026), 16.9% (P < 0.01), and 18.7% (P < 0.01), respectively; the Fd-GOGAT activities in B. platyphylla leaves, F. mandshurica leaves and P. koraiensis needles under the 100D+0W treatment increased by 4.7% (P = 0.093), 18.9% (P < 0.01), and 3.8% (P = 0.249), respectively.
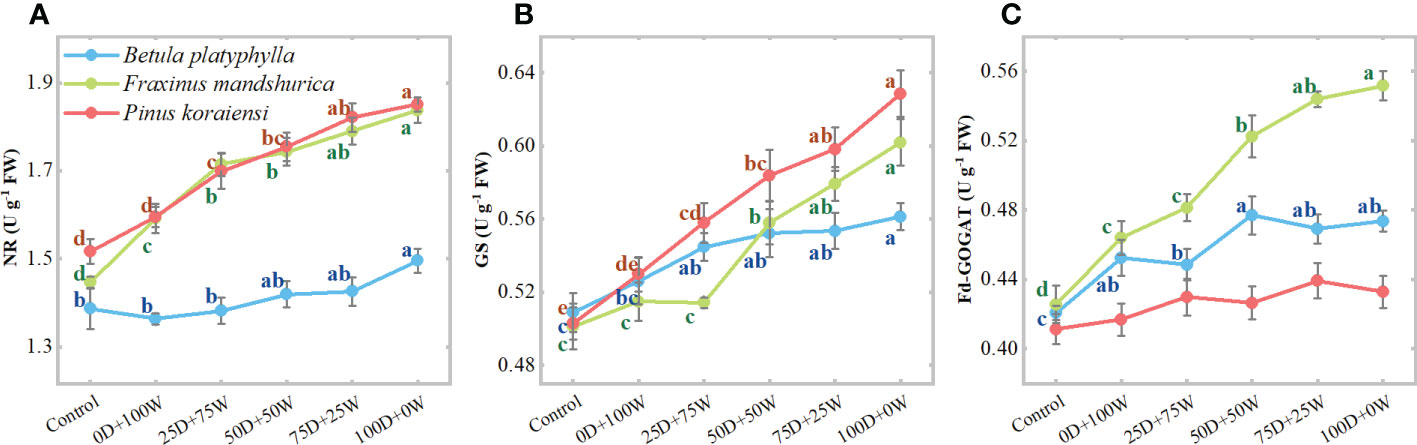
Figure 3 Activities (U g-1 FW, A-C) of nitrate reductase (NR), glutamine synthetase (GS) and glutamate synthase (Fd-GOGAT) in leaves of three species affected by six canopy nitrogen (N) application treatments for 32 days. N application treatments included a control (no application of N) and five types of N deposition with dry to wet ratios of 0:100 (0D+100W), 25:75 (25D+75W), 50:50 (50D+50W), 75:25 (75D+25W) and 100:0 (100D+0W), respectively. Error bars indicate the standard error of the mean (n = 5). Within the same species in each panel, different letters on the left side of the error bar demonstrate significant differences among six treatments (P < 0.05) according to the least significant difference test. The scales of the y-axis are different in each panel.
3.3. Soluble proteins and photosynthetic pigments concentrations
Relative to the control, the chlorophyll concentration in B. platyphylla leaves increased significantly under each N application treatment and increased continually with the increase in RDry/Wet (Figure 4A), which increased by 54.9% (P < 0.01) under the 0D+100W treatment and increased by 108.1% (P < 0.01) under the 100D+0W treatment. The chlorophyll concentrations in F. mandshurica leaves and P. koraiensis needles did not show significant differences among all treatments, it is noteworthy that the chlorophyll concentration in F. mandshurica leaves showed a continuous decrease with the increase in RDry/Wet (Figures 4B, C). Relative to the control, the chlorophyll concentration in F. mandshurica leaves increased by 5.9% (P = 0.64) under the 0D+100W treatment and decreased by 19.4% (P = 0.134) under the 100D+0W treatment.
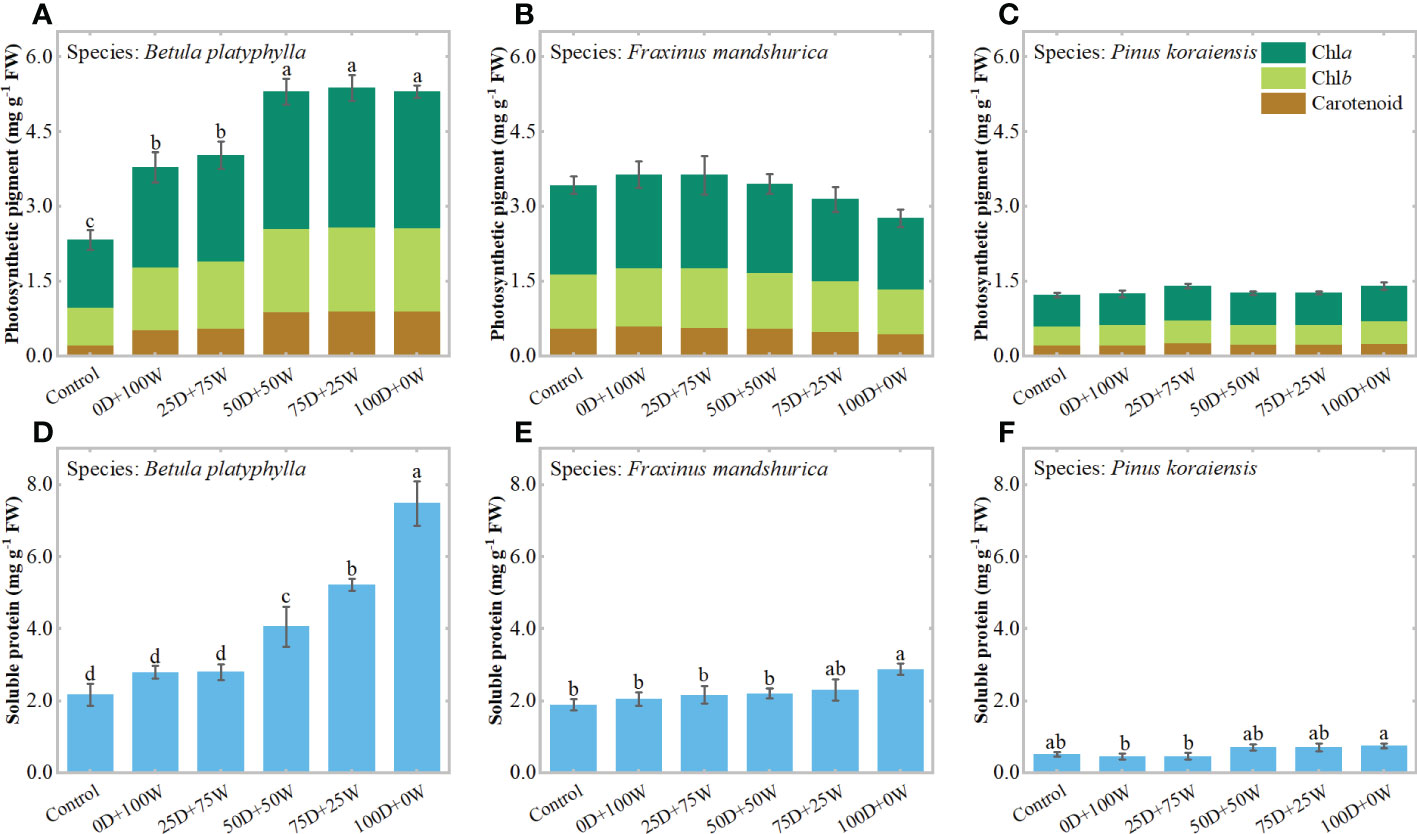
Figure 4 Concentrations of photosynthetic pigment (mg g-1 FW, A–C) and soluble protein (mg g-1 FW, D-F) in leaves of three species affected by six canopy nitrogen (N) application treatments for 32 days. N application treatments included a control (no application of N) and five types of N deposition with dry to wet ratios of 0:100 (0D+100W), 25:75 (25D+75W), 50:50 (50D+50W), 75:25 (75D+25W) and 100:0 (100D+0W), respectively. Error bars indicate the standard error of the mean of chlorophyll (a + b) concentration and soluble protein concentration (n = 5). Different letters above the error bar represent significant differences among six treatments (P < 0.05) according to the least significant difference test.
Relative to the control, the soluble protein concentrations in B. platyphylla leaves, F. mandshurica leaves and P. koraiensis needles under the 0D+100W treatment did not show significant differences but increased continually with the increase in RDry/Wet, the soluble protein concentrations in their leaves increased by 245.0% (P < 0.01), 52.3% (P < 0.01) and 45.3% (P = 0.063) under the100D+0W treatment, respectively (Figures 4D–F).
3.4. Leaf photosynthesis rates
After 16 and 32 days of N application and relative to the control, the Pmax of B. platyphylla under the 0D+100W treatment increased by 7.0% (P = 0.382) and 15.2% (P = 0.385), respectively. For F. mandshurica, the Pmax under the 0D+100W treatment increased by 4.3% (P = 0.675) and 10.2% (P = 0.197), respectively. However, with the increase in RDry/Wet, the Pmax of B. platyphylla on both measurement days increased continually, while that of F. mandshurica on both measurement days decreased continually. Relative to the control, the Pmax of B. platyphylla under the 100D+0W treatment increased by 28.3% (P < 0.01) and 51.3% (P < 0.01) after 16 and 32 days of N application, respectively. For F. mandshurica, the Pmax under the 100D+0W treatment decreased by 44.6% (P < 0.01) and 32.9% (P < 0.01) on two measurement days, respectively. Moreover, after 32 days of N application, there were significantly positive relationships between Pmax and foliar chlorophyll concentrations in B. platyphylla and F. mandshurica, for F. mandshurica, Pmax also had a positive relationship with foliar K concentration (Figures 5A, B). There was no significant difference in Pmax of P. koraiensis among all treatments on two measurement days (Figures 5A, B).
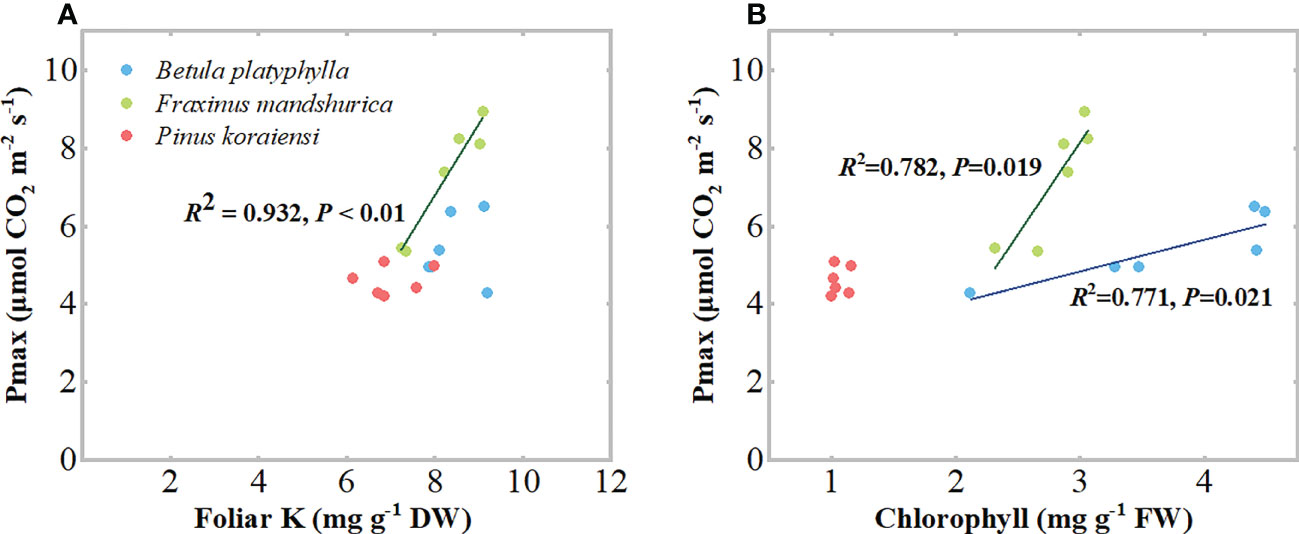
Figure 5 Leaf light-saturated net photosynthesis rate (Pmax, μmol CO2 m-2 s-1) of three species affected by six canopy nitrogen (N) application treatments for 16 (A) and 32 (B) days. N application treatments included a control (no application of N) and five types of N deposition with dry to wet ratios of 0:100 (0D+100W), 25:75 (25D+75W), 50:50 (50D+50W), 75:25 (75D+25W) and 100:0 (100D+0W), respectively. Error bars indicate the standard error of the mean (n = 5). Within the same species in each panel, different letters on the left side of the error bar demonstrate significant differences among six treatments (P < 0.05) according to the least significant difference test.
3.5. Leaf morphology and anatomy
The leaf area, SLA and LMA of the three species did not show significant differences among all treatments (Table S1), but some of the leaf anatomical traits were significantly affected by N application. Relative to the control, the ADE of B. platyphylla and F. mandshurica under the 0D+100W treatment increased by 66.0% (P < 0.01) and 23.2% (P < 0.01), respectively, but decreased continually with the increase in RDry/Wet (Table 2; Figure S2). The ADE of B. platyphylla and F. mandshurica under the 100D+0W treatment was not significantly different compared with that under the control. Relative to the control, the EHT of P. koraiensis increased under each N application treatment and showed significance only under the 50D+50W treatment. Besides, the ABE and leaf thickness of B. platyphylla showed the same trend as ADE under the N application treatments but were less affected by the N application.
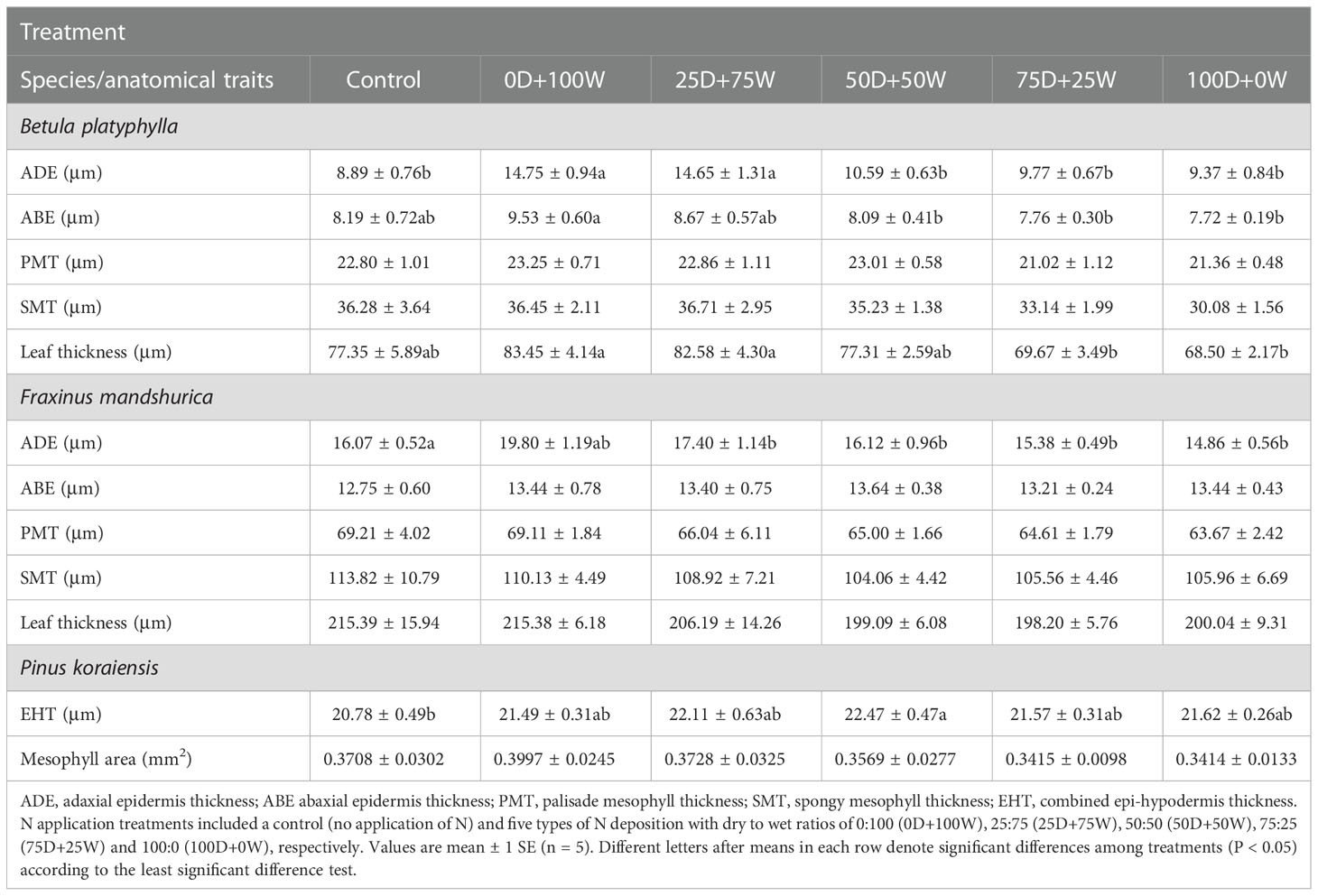
Table 2 Leaf anatomical traits of three species affected by six canopy nitrogen (N) application treatments for 32 days.
4. Discussion
4.1. The importance of considering the alternation of dry and wet nitrogen deposition
In the present study, N concentrations, GS and Fd-GOGAT activities in leaves of all three species slightly increased under wet deposition, but increased continually with the increase in RDry/Wet (Figures 2A, 3B, C). These results confirm that atmospherically deposited NH3 and NH4+ can be used as a foliar supply of N (Jones et al., 2008; Wang et al., 2021) and suggest that canopy leaves may retain more NH3 from dry deposition compared to NH4+ from wet deposition. Leaf NH3/NH4+ uptake kinetics were not investigated in the present study, as the focus of the work was to understand how alternating dry and wet N deposition with the same deposition flux affects the structure and physiology of canopy leaves. In fact, the retention and assimilation of gaseous NH3 or dissolved NH4+ by leaves has been widely studied (Krupa, 2003; Sparks, 2009). Therefore, the fact that N concentration and N metabolic enzyme activities increased with the increase in RDry/Wet indicates that dry and wet deposition have different effects on foliar N status. Relative to wet deposition, dry deposition of N will lead to higher foliar N concentration.
In previous studies, leaf morphology and anatomy showed high plasticity in response to changes of leaf N levels (Khan et al., 2020; Zhu et al., 2020). However, in the present study, except for the gradient changes of epidermis (epi-hypodermis) thickness under N application, we did not find significant effects of continuously increasing N concentration on leaf morphological (i.e., the leaf area, SLA and LMA of the three species) and anatomical traits (i.e., the PMT and SMT of B. platyphylla and F. mandshurica and mesophyll area of P. koraiensis) of the three species (Table 2; Table S1). In our results (Table 2; Figure S2), with the decrease in RDry/Wet, the continuous thickening of ADE in broadleaved species (B. platyphylla and F. mandshurica) may be a defense strategy for the leaves in response to the weak acidity of NH4Cl solution (Kateivas et al., 2022). For coniferous species (P. koraiensis), the EHT increased at first and then decreased (Table 2; Figure S2), suggesting that the needles may have the same defense strategy, but due to greater retention of wet deposition by needles, the EHT may be injured by higher doses of NH4Cl and then thinned. Although the above results were inconsistent with our first hypothesis, they were not unexpected, because the leaf morphological and structural traits may be invariable under short-term N application (Makoto and Koike, 2007).
We found that the continuous increase in foliar N concentrations of the three species was accompanied by a continuous increase in soluble protein concentrations in their leaves (Figures 4D–F; Figure S1). These results were consistent with previous studies that leaves can store the applied N in the form of some organic compounds in the organic N pool, so an increase in N concentration in leaves is usually accompanied by an increase in one and more amino acids, total free amino acids and proteins (Bubier et al., 2011; Hu et al., 2019). N is an important component of chlorophylls, photosynthesis-related enzymes and proteins, the addition of N inevitably changes the N levels and N metabolism in plants while also affecting the photosynthetic system composition and photosynthetic carbon assimilation capacity of plants (Tomaszewski and Sievering, 2007; Liu et al., 2020b). In the present study, the foliar chlorophyll concentrations and Pmax of B. platyphylla and F. mandshurica also showed a gradient change with the continuous increase in their foliar N concentrations. Relative to the control, the variation of these traits under dry deposition was much larger than those under wet deposition (Figures 4A, B, 6A, B). The above results suggest that dry deposition has a greater effect on plant leaf physiology than wet deposition.
Although we should be careful to deduct long-term leaf functional modifications by simulated N deposition from short-term acclimation studies, our leaf physiology results in the short term already suggest that a single simulated dry and wet N deposition would overestimate and underestimate effects of atmospheric N deposition on leaf function, respectively. Therefore, alternating processes of dry and wet N deposition should not be neglected.
4.2. Response of leaf photosynthetic capacity of different tree species to canopy NHx deposition
With the continuous increase in foliar N and soluble protein concentrations of the three species, their Pmax showed different trends. For broadleaved species, the Pmax of fast-growing B. platyphylla increased continuously, while the Pmax of slow-growing F. mandshurica decreased continuously (Figures 6A, B), and there was a significant positive correlation between Pmax and foliar chlorophyll concentrations for both species (Figure 5B). These results indicate that some of the excess N can be invested in light harvesting and photosynthesis in B. platyphylla leaves, while N was diverted away from the photosynthetic system of F. mandshurica leaves and photosynthetic capacity was suppressed. The divergent response of photosynthesis between B. platyphylla and F. mandshurica seems to be expected, because slow-growing species may lack a better strategy to use excess N input into their leaves than fast-growing species (Aerts and Chapin, 1999; Li et al., 2012; Mikola et al., 2021). For coniferous species, there was no significant change in Pmax and foliar chlorophyll concentration of P. koraiensis (Figures 4C, 6A, B). These results were comparable to the response of needles under high levels of N addition in previous studies (Warren et al., 2003; Bauer et al., 2004; Li et al., 2022), which suggested that the excess N in needles is stored as amino acids and soluble protein, rather than being invested in photosynthesis. This storage mechanism in needles is supposed to be a peculiar adaptation of evergreen species when N uptake from the environment exceeds immediate growth requirements (Warren et al., 2003; Makoto and Koike, 2007). In general, the life forms and growth character of tree species probably contribute to different results of photosynthesis among tree species under NHx deposition.
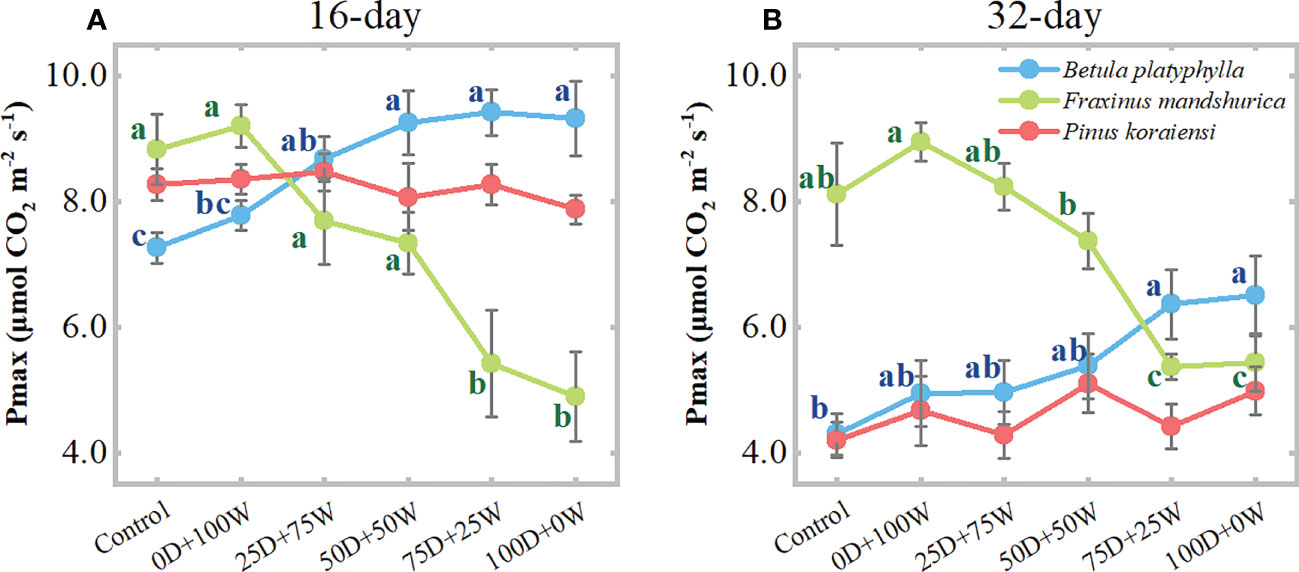
Figure 6 Relationships between light-saturated net photosynthesis rate (Pmax) and foliar Potassium (K) concentrations (A), chlorophyll concentrations (B) in the three species. Within the same species, each symbol represents data from one N application treatment. N application treatments included a control (no application of N) and canopy N deposition with dry to wet ratios of 0:100, 25:75, 50:50, 75:25 and 100:0, respectively. Data were collected after 32 days of N application treatments.
In fact, there is ample evidence from previous studies that appropriate NH4+ addition can increase the investment of N in photosynthetic system components, induce an increase in photosynthetic capacity of leaves and promote plant growth (Pérez-Soba and van der Eerden, 1993; Elvir et al., 2006; Hong et al., 2021). But above a certain threshold, NH4+ will be a stress and lead to a decrease in cation (K+, Ca2+ and Mg2+) concentrations in plant tissues (i.e. nutrient limitation of the plant), and have a negative impact on the leaf physiological function, induce a decrease in photosynthetic capacity (Esteban et al., 2016; Mao et al., 2018; Hong et al., 2021). In the root medium, this decrease is caused by the cation leaching due to NH4+-induced soil acidification and the competition of excess NH4+ with cations at the root cell membrane level (Britto and Kronzucker, 2002; Lu et al., 2014; Esteban et al., 2016). Therefore, in the traditional view, the NH4+ tolerance threshold (i.e., sensitivity to NH4+) of tree species and the nutrient limitation mechanism when NH4+ exceeds the threshold seem to better explain the results of photosynthesis the three species in this study. However, the process of our simulated alternating NHx deposition mainly occurred in the canopy and considering that the change in photosynthesis may also be related to the effect of NH3/NH4+ retention in leaves, the sensitivity of species to deposited NHx should be revisited.
Our Pmax results showed a higher sensitivity of F. mandshurica to canopy NHx deposition than B. platyphylla and P. koraiensis, which was consistent with our second hypothesis that the sensitivity of trees to canopy deposited NHx and to NH4+ in the root medium would be similar. This hypothesis was further supported by our foliar K concentrations results, where the decrease in Pmax of F. mandshurica was also accompanied by a decrease in its foliar K concentration (Figures 2C, 5A), and both foliar K concentration and Pmax showed a significant negative relationship with foliar N concentration (Figure S1), while the relatively positive response of Pmax of B. platyphylla and P. koraiensis was accompanied by an increase in their foliar K concentrations (Figure 2C), suggesting that the application of N only resulted in K limitation in F. mandshurica leaves. We also found that the decrease in foliar K concentration of F. mandshurica was accompanied by a decrease in foliar P concentration (Figure 2B), which may be caused by the decrease of cation concentrations in plant tissues and the consequent imbalance of other ions (Esteban et al., 2016). Our evidence of K concentrations is based on the fact that the retention of NH3 or NH4+ by leaves can also lead to the leaching of cations from plant tissues and a decrease in cation concentrations. This is due to the exchange of NH4+ with cations in leaves and the possible competition between NH4+ (protonated NH3 in the apoplast) and cations in leaves, particularly K+, in their function to maintain electroneutrality (Van der Eerden et al., 1992; Stachurski and Zimka, 2002; Mori et al., 2019; Turpault et al., 2021). Therefore, according to our findings, our Pmax results can also be explained by the sensitivity of tree species to NH4+ in the traditional view. Due to the higher NH4+ sensitivity of F. mandshurica, the deposited NH4+ may exceed the tolerance threshold of F. mandshurica and led to P and K limitation in leaves, so the photosynthetic capacity was reduced. For B. platyphylla and P. koraiensis, because of their lower NH4+ sensitivity, they may have avoided or alleviated the nutrient limitation in the leaves under NHx deposition, so the deposited NH4+ was invested into the photosynthetic system or stored in the organic N pool.
In addition, there is also evidence that NO3- signaling and reduction in plant tissues are important factors in the alleviation of NH4+ stress (Hachiya et al., 2012; Esteban et al., 2016). Therefore, in our results (Figure 3A), the continuous increase in NR activities in leaves of the three species may be a mechanism by which they alleviate the possible stress caused by deposited NH3/NH4+. The increase in NR activity was much lower of B. platyphylla than that of F. mandshurica and P. koraiensis, suggesting that the stimulation of B. platyphylla leaves by deposited NH3/NH4+ was probably the lowest and may be one of the reasons for the relatively most positive response of Pmax of B. platyphylla. Combined with the above discussion, we propose that B. platyphylla and P. koraiensis may better acclimate to canopy NHx deposition than F. mandshurica.
It is noteworthy that the foliar K concentration of B. platyphylla and P. koraiensis decreased significantly under the 0D+100W treatment compared with the control (Figure 2C), which may be attributed to the above canopy exchange of cations. However, we did not find the decrease in their physiological function, this may be due to the low NH4+stress caused by the lower NH4+ retention in leaves, and the lagged response of the leaves to additional NH4+ entering the soil through the canopy (Wang et al., 2021). This result suggests that the foliar K concentration was reduced before NH4+ exceeded the threshold. This was inconsistent with the possible positive relationship between photosynthesis and leaf K status under the increase in RDry/Wet in this study. Therefore, we expect that the acclimation of plants to dry deposition and wet deposition may be completely different. However, it merits a further study to track the effects of long-term alternating dry and wet N deposition on function and cation status of leaves in the future, before a clear conclusion can be reached. Given that studies on the response mechanism of plants to retained N by leaves and plant sensitivity/tolerance to retained N are still limited, the acclimation mechanism of plants under dry and wet N deposition is still an open question.
5. Conclusion
Our results show that, relative to wet deposition, dry deposition of N would lead to higher foliar N concentrations and induce a greater response of photosynthesis in plant canopy leaves. Dry deposition is relatively more important on leaf physiological functions in alternating deposition, ignoring alternating processes of dry and wet N deposition would result in a biased assessment of the response of forest canopy to atmospheric N deposition. B. platyphylla and P. koraiensis may better acclimate to canopy NHx deposition than F. mandshurica. Nevertheless, we found that dry and wet deposition had different effects on leaf K status, so we expect that the acclimation of plants to dry deposition and wet deposition may be completely different. Further long-term studies are needed to improve our understanding of how the structure and physiology of canopy leaves respond to alternating dry and wet N deposition under the realistic condition, and acclimation mechanism of plants to dry-wet alternating deposition of N. They will help us to better understand and project the response of forest ecosystems to atmospheric N deposition in the future.
Data availability statement
The raw data supporting the conclusions of this article will be made available by the authors, without undue reservation.
Author contributions
XW and CW designed the experiment. CW and XC built the field facility and simulated nitrogen deposition in the chamber. CW and SZ conducted laboratory experiments. CW analyzed the data and wrote the manuscript with the help of XW. All authors contributed to the article and approved the submitted version.
Funding
This research was funded by the Fundamental Research Funds for the Central Universities (no. 2572020DR05)
Acknowledgments
We are grateful for the greenhouse provided by the Seed Orchard of Betula platyphylla at Northeast Forestry University and for the help of the staff there.
Conflict of interest
The authors declare that the research was conducted in the absence of any commercial or financial relationships that could be construed as a potential conflict of interest.
Publisher’s note
All claims expressed in this article are solely those of the authors and do not necessarily represent those of their affiliated organizations, or those of the publisher, the editors and the reviewers. Any product that may be evaluated in this article, or claim that may be made by its manufacturer, is not guaranteed or endorsed by the publisher.
Supplementary material
The Supplementary Material for this article can be found online at: https://www.frontiersin.org/articles/10.3389/fpls.2022.1105075/full#supplementary-material
References
Adriaenssens, S., Staelens, J., Wuyts, K., Samson, R., Verheyen, K., Boeckx, P. (2012). Retention of dissolved inorganic nitrogen by foliage and twigs of four temperate tree species. Ecosystems 15, 1093–1107. doi: 10.1007/s10021-012-9568-5
Adrizal, Patterson, P. H., Hulet, R. M., Bates, R. M. (2006). Growth and foliar nitrogen status of four plant species exposed to atmospheric ammonia. J. Environ. Sci. Health Part B-Pesticides Food Contaminants Agric. Wastes 41, 1001–1018. doi: 10.1080/03601230600808703
Aerts, R., Chapin, F. S. (1999). The mineral nutrition of wild plants revisited: A re-evaluation of processes and patterns. Adv. Ecol. Res. 30, 1–67. doi: 10.1016/s0065-2504(08)60016-1
Bauer, G. A., Bazzaz, F. A., Minocha, R., Long, S., Magill, A., Aber, J., et al. (2004). Effects of chronic n additions on tissue chemistry, photosynthetic capacity, and carbon sequestration potential of a red pine (Pinus resinosa ait.) stand in the NE united states. For. Ecol. Manage. 196, 173–186. doi: 10.1016/j.foreco.2004.03.032
Britto, D. T., Kronzucker, H. J. (2002). NH4+ toxicity in higher plants: a critical review. J. Plant Physiol. 159, 567–584. doi: 10.1078/0176-1617-0774
Bubier, J. L., Smith, R., Juutinen, S., Moore, T. R., Minocha, R., Long, S., et al. (2011). Effects of nutrient addition on leaf chemistry, morphology, and photosynthetic capacity of three bog shrubs. Oecologia 167, 355–368. doi: 10.1007/s00442-011-1998-9
Castro, A., Stulen, I., De Kok, L. J. (2008). Atmospheric NH3 as plant nutrient: A case study with brassica oleracea. Environ. pollut. 154, 467–472. doi: 10.1016/j.envpol.2007.09.021
Crabtree, R. C., Bazzaz, F. A. (1993). Seedling response of four birch species to simulated nitrogen deposition: ammonium vs. nitrate. Ecol. applications: A Publ. Ecol. Soc. America 3, 315–321. doi: 10.2307/1941834
Cui, X. Y., Song, J. F. (2007). Soil NH4+/NO3- nitrogen characteristics in primary forests and the adaptability of some coniferous species. Front. Forestry China 2, 1–10. doi: 10.1007/s11461-007-0001-8
Elvir, J. A., Wiersma, G. B., Day, M. E., Greenwood, M. S., Fernandez, I. J. (2006). Effects of enhanced nitrogen deposition on foliar chemistry and physiological processes of forest trees at the bear brook watershed in Maine. For. Ecol. Manage. 221, 207–214. doi: 10.1016/j.foreco.2005.09.022
Esteban, R., Ariz, I., Cruz, C., Moran, J. F. (2016). Review: Mechanisms of ammonium toxicity and the quest for tolerance. Plant Sci. 248, 92–101. doi: 10.1016/j.plantsci.2016.04.008
Gu, B. J., Ju, X. T., Chang, J., Ge, Y., Vitousek, P. M. (2015). Integrated reactive nitrogen budgets and future trends in China. Proc. Natl. Acad. Sci. U S A 112, 8792–8797. doi: 10.1073/pnas.1510211112
Guo, D. L., Xia, M. X., Wei, X., Chang, W. J., Liu, Y., Wang, Z. Q. (2008). Anatomical traits associated with absorption and mycorrhizal colonization are linked to root branch order in twenty-three Chinese temperate tree species. New Phytol. 180, 673–683. doi: 10.1111/j.1469-8137.2008.02573.x
Hachiya, T., Watanabe, C. K., Fujimoto, M., Ishikawa, T., Takahara, K., Kawai-Yamada, M., et al. (2012). Nitrate addition alleviates ammonium toxicity without lessening ammonium accumulation, organic acid depletion and inorganic cation depletion in arabidopsis thaliana shoots. Plant Cell Physiol. 53, 577–591. doi: 10.1093/pcp/pcs012
Hong, M. H., Ma, Z. L., Wang, X. Y., Shen, Y. W., Mo, Z. Y., Wu, M. J., et al. (2021). Effects of light intensity and ammonium stress on photosynthesis in sargassum fusiforme seedlings. Chemosphere 273, 128605. doi: 10.1016/j.chemosphere.2020.128605
Hu, Y. B., Peuke, A. D., Zhao, X. Y., Yan, J. X., Li, C. M. (2019). Effects of simulated atmospheric nitrogen deposition on foliar chemistry and physiology of hybrid poplar seedlings. Plant Physiol. Biochem. 143, 94–108. doi: 10.1016/j.plaphy.2019.08.023
Jones, M. R., Raven, J. A., Leith, I. D., Cape, J. N., Smith, R. I., Fowler, D. (2008). Short-term flux chamber experiment to quantify the deposition of gaseous 15N-NH3 to calluna vulgaris. Agric. For. Meteorol. 148, 893–901. doi: 10.1016/j.agrformet.2007.12.003
Kateivas, K. S. B., Cairo, P. A. R., Neves, P. H. S., Ribeiro, R. S. S., Machado, L. M., Leitao, C. A. E. (2022). The impact of NOx and SO2 emissions from a magnesite processing industry on morphophysiological and anatomical features of plant bioindicators. Acta Physiologiae Plantarum 44, 77. doi: 10.1007/s11738-022-03411-9
Khan, A., Sun, J. J., Zarif, N., Khan, K., Jamil, M. A., Yang, L. X., et al. (2020). Effects of increased n deposition on leaf functional traits of four contrasting tree species in northeast China. Plants-Basel 9, 1231. doi: 10.3390/plants9091231
Krupa, S. V. (2003). Effects of atmospheric ammonia (NH3) on terrestrial vegetation: a review. Environ. pollut. 124, 179–221. doi: 10.1016/s0269-7491(02)00434-7
Li, H., Li, M. C., Luo, J., Cao, X., Qu, L., Gai, Y., et al. (2012). N-fertilization has different effects on the growth, carbon and nitrogen physiology, and wood properties of slow- and fast-growing populus species. J. Exp. Bot. 63, 6173–6185. doi: 10.1093/jxb/ers271
Li, Y., Schichtel, B. A., Walker, J. T., Schwede, D. B., Chen, X., Lehmann, C. M. B., et al. (2016). Increasing importance of deposition of reduced nitrogen in the united states. Proc. Natl. Acad. Sci. U S A 113, 5874–5879. doi: 10.1073/pnas.1525736113
Liu, T., Mao, P., Shi., L. L., Eisenhauer, N., Liu, S. J., Wang, X. L., et al. (2020c). Forest canopy maintains the soil community composition under elevated nitrogen deposition. Soil Biol. Biochem. 143, 107733. doi: 10.1016/j.soilbio.2020.107733
Liu, N., Wu, S. H., Guo, Q. F., Wang, J. X., Cao, C., Wang, J. (2018). Leaf nitrogen assimilation and partitioning differ among subtropical forest plants in response to canopy addition of nitrogen treatments. Sci. Total Environ. 637, 1026–1034. doi: 10.1016/j.scitotenv.2018.05.060
Liu, N., Zhang, S. K., Huang, Y., Cai, H. Y., Zhu, X. Y. (2020b). Understory and canopy additions of nitrogen differentially affect carbon and nitrogen metabolism of psychotria rubra in an evergreen broad-leaved forest. Sci. Total Environ. 724, 138183. doi: 10.1016/j.scitotenv.2020.138183
Liu, L., Zhang, X. Y., Xu, W., Liu, X. J., Zhang, Y., Li, Y., et al. (2020a). Fall of oxidized while rise of reduced reactive nitrogen deposition in China. J. Cleaner Production 272, 122875. doi: 10.1016/j.jclepro.2020.122875
Li, R. S., Yu, D., Zhang, Y. K., Han, J. M., Zhang, W. D., Yang, Q. P., et al. (2022). Investment of needle nitrogen to photosynthesis controls the nonlinear productivity response of young Chinese fir trees to nitrogen deposition. Sci. Total Environ. 840, 156537. doi: 10.1016/j.scitotenv.2022.156537
Li, X., Zhang, C., Zhang, B., Wu, D., Shi, Y., Zhang, W., et al. (2021). Canopy and understory nitrogen addition have different effects on fine root dynamics in a temperate forest: implications for soil carbon storage. New Phytol. 231, 1377–1386. doi: 10.1111/nph.17460
Lu, X. K., Mao, Q. G., Gilliam, F. S., Luo, Y. Q., Mo, J. M. (2014). Nitrogen deposition contributes to soil acidification in tropical ecosystems. Global Change Biol. 20, 3790–3801. doi: 10.1111/gcb.12665
Makoto, K., Koike, T. (2007). Effects of nitrogen supply on photosynthetic and anatomical changes in current-year needles of pinus koraiensis seedlings grown under two irradiances. Photosynthetica 45, 99–104. doi: 10.1007/s11099-007-0015-3
Mao, Q. G., Lu, X. K., Mo, H., Gundersen, P., Mo, J. M. (2018). Effects of simulated n deposition on foliar nutrient status, n metabolism and photosynthetic capacity of three dominant understory plant species in a mature tropical forest. Sci. Total Environ. 610, 555–562. doi: 10.1016/j.scitotenv.2017.08.087
Mikola, J., Koikkalainen, K., Rasehorn, M., Silfver, T., Paaso, U., Rousi, M. (2021). Genotypic traits and tradeoffs of fast growth in silver birch, a pioneer tree. Oecologia 196, 1049–1060. doi: 10.1007/s00442-021-04986-9
Mori, T., Zhou, K. J., Wang, S. H., Zhang, W., Mo, J. M. (2019). Effect of nitrogen addition on DOC leaching and chemical exchanges on canopy leaves in guangdong province, China. J. Forestry Res. 30, 1707–1713. doi: 10.1007/s11676-018-0800-9
Pan, Y. P., Wang, Y. S., Tang, G. Q., Wu, D. (2012). Wet and dry deposition of atmospheric nitrogen at ten sites in northern China. Atmospheric Chem. Phys. 12, 6515–6535. doi: 10.5194/acp-12-6515-2012
Pérez-Soba, M., van der Eerden, L. J. M. (1993). Nitrogen uptake in needles of scots pine (Pinus sylvestris l.) when exposed to gaseous ammonia and ammonium fertilizer in the soil. Plant Soil 153, 231–242. doi: 10.1007/bf00012996
Pitcairn, C. E. R., Leith, I. D., Sheppard, L. J., Suttona, M. A., Fowler, D., Munroa, R. C., et al. (1998). The relationship between nitrogen deposition, species composition and foliar nitrogen concentrations in woodland flora in the vicinity of livestock farms. Environ. pollut. 102, 41–48. doi: 10.1016/S0269-7491(98)80013-4
Rennenberg, H., Gessler, A. (1999). Consequences of n deposition to forest ecosystems - recent results and future research needs. Water Air Soil pollut. 116, 47–64. doi: 10.1023/A:1005257500023
Shen, J. L., Li, Y., Liu, X. J., Luo, X. S., Tang, H., Zhang, Y. Z., et al. (2013). Atmospheric dry and wet nitrogen deposition on three contrasting land use types of an agricultural catchment in subtropical central China. Atmospheric Environ. 67, 415–424. doi: 10.1016/j.atmosenv.2012.10.068
Sheppard, L. J., Leith, I. D., Mizunuma, T., Cape, J. N., Crossley, A., Leeson, S., et al. (2011). Dry deposition of ammonia gas drives species change faster than wet deposition of ammonium ions: Evidence from a long-term field manipulation. Global Change Biol. 17, 3589–3607. doi: 10.1111/j.1365-2486.2011.02478.x
Sparks, J. P. (2009). Ecological ramifications of the direct foliar uptake of nitrogen. Oecologia 159, 1–13. doi: 10.1007/s00442-008-1188-6
Stachurski, A., Zimka, J. R. (2002). Atmospheric deposition and ionic interactions within a beech canopy in the karkonosze mountains. Environ. pollut. 118, 75–87. doi: 10.1016/S0269-7491(01)00238-X
Tomaszewski, T., Sievering, H. (2007). Canopy uptake of atmospheric n deposition at a conifer forest: Part II-response of chlorophyll fluorescence and gas exchange parameters. Tellus Ser. B-Chemical Phys. Meteorol. 59, 493–501. doi: 10.1111/j.1600-0889.2007.00265.x
Turpault, M. P., Kirchen, G., Calvaruso, C., Redon, P. O., Dincher, M. (2021). Exchanges of major elements in a deciduous forest canopy. Biogeochemistry 152, 51–71. doi: 10.1007/s10533-020-00732-0
Van der Eerden, L. J., Lekkerkerk, L. J., Smeulders, S. M., Jansen, A. E. (1992). Effects of atmospheric ammonia and ammonium sulphate on Douglas fir (Pseudotsuga menziesii). Environ. pollut. 76, 1–9. doi: 10.1016/0269-7491(92)90109-N
Wang, X., Wang, B., Wang, C. Z., Wang, Z. H., Li, J., Jia, Z., et al. (2021). Canopy processing of n deposition increases short-term leaf n uptake and photosynthesis, but not long-term n retention for aspen seedlings. New Phytol. 229, 2601–2610. doi: 10.1111/nph.17041
Warner, J. X., Dickerson, R. R., Wei, Z., Strow, L. L., Wang, Y., Liang, Q. (2017). Increased atmospheric ammonia over the world's major agricultural areas detected from space. Geophysical Res. Lett. 44, 2875–2884. doi: 10.1002/2016GL072305
Warren, C. R., Dreyer, E., Adams, M. A. (2003). Photosynthesis-rubisco relationships in foliage of pinus sylvestris in response to nitrogen supply and the proposed role of rubisco and amino acids as nitrogen stores. Trees-Structure Funct. 17, 359–366. doi: 10.1007/s00468-003-0246-2
Wen, Z., Xu, W., Li, Q., Han, M. J., Tang, A. H., Zhang, Y., et al. (2020). Changes of nitrogen deposition in China from 1980 to 2018. Environ. Int. 144, 106022. doi: 10.1016/j.envint.2020.106022
Wu, G. L., Chen, D. X., Zhou, Z., Ye, Q., Wu, J. H. (2022). Canopy nitrogen addition enhance the photosynthetic rate of canopy species by improving leaf hydraulic conductivity in a subtropical forest. Front. Plant Sci. 13. doi: 10.3389/fpls.2022.942851
Wu, C., Wang, Z. Q., Fan, Z. Q., Sun, H. L. (2003). Effects of different concentrations and form ratios of nitrogen on chlorophyll biosynthesis, photosynthesis, and biomass partitioning in fraxinus mandshurica seedlings. Acta Phytoecologica Sin. 27, 771–779.
Xu, W., Luo, X. S., Pan, Y. P., Zhang, L., Tang, A. H., Shen, J. L., et al. (2015). Quantifying atmospheric nitrogen deposition through a nationwide monitoring network across China. Atmospheric Chem. Phys. 15, 12345–12360. doi: 10.5194/acp-15-12345-2015
Zhang, Y., Cui, X. Y. (2011). NH4+/NO3- absorption characteristics of betula platyphylla seedlings. J. Beijing Forestry Univ. 33, 26–30.
Zhang, Y. D., Fan, Z. Q., Wang, Q. C., Wang, Z. Q. (2000). Effect of different nitrogen forms on growth of fraxinus mandshurica seedlings. Chin. J. Appl. Ecol. 11, 665–667.
Zhang, Y. Y., Yu, T., Ma, W. B., Wang, F., Tian, C., Li, J. Q. (2020). Physiological and morphological effects of different canopy densities on reintroduced acer catalpifolium. Biodiver. Sci. 28, 323–332.
Keywords: canopy leaves, dry nitrogen deposition, wet nitrogen deposition, leaf morphology, leaf anatomy, leaf photosynthesis
Citation: Wu C, Zhou S, Cheng X and Wei X (2023) Alternating processes of dry and wet nitrogen deposition have different effects on the function of canopy leaves: Implications for leaf photosynthesis. Front. Plant Sci. 13:1105075. doi: 10.3389/fpls.2022.1105075
Received: 22 November 2022; Accepted: 21 December 2022;
Published: 09 January 2023.
Edited by:
Kaixiong Xing, Hainan Normal University, ChinaReviewed by:
Jun Wang, Chinese Academy of Sciences (CAS), ChinaDexiang Chen, Chinese Academy of Forestry, China
Copyright © 2023 Wu, Zhou, Cheng and Wei. This is an open-access article distributed under the terms of the Creative Commons Attribution License (CC BY). The use, distribution or reproduction in other forums is permitted, provided the original author(s) and the copyright owner(s) are credited and that the original publication in this journal is cited, in accordance with accepted academic practice. No use, distribution or reproduction is permitted which does not comply with these terms.
*Correspondence: Xing Wei, weixing94@nefu.edu.cn