- 1National Sugar Crops Improvement Center & Sugar Beet Engineering Research Center Heilongjiang Province & College of Advanced Agriculture and Ecological Environment, Heilongjiang University, Harbin, China
- 2Research Institute of Economic Crops, Xinjiang Academy of Agricultural Sciences, Urumqi, Xinjiang, China
- 3Zhejiang Key Laboratory of Crop Germplasm Resources, Department of Agronomy, College of Agriculture and Biotechnology, Zhejiang University, Hangzhou, China
- 4Safety and Quality Institution of Agricultural Products, Heilongjiang Academy of Agricultural Sciences, Harbin, China
Boron (B) deficiency severely affects the quality of sugar beet production, and the employment of nutrient-efficient varieties for cultivation is a crucial way to solve environmental and resource-based problems. However, the aspect of leaf photosynthetic performance among B-efficient sugar beet cultivars remains uncertain. The B deficient and B-sufficient treatments were conducted in the experiment using KWS1197 (B-efficient) and KWS0143 (B-inefficient) sugar beet cultivars as study materials. The objective of the present study was to determine the impacts of B deficiency on leaf phenotype, photosynthetic capacity, chloroplast structure, and photochemical efficiency of the contrasting B-efficiency sugar beet cultivars. The results indicated that the growth of sugar beet leaves were dramatically restricted, the net photosynthetic rate was significantly decreased, and the energy flux, quantum yield, and flux ratio of PSII reaction centers were adversely affected under B deficiency. Compared to the KWS0143 cultivar, the average leaf area ratio of the KWS1197 cultivar experienced less impact, and its leaf mass ratio (LMR) increased by 26.82% under B deficiency, whereas for the KWS0143 cultivar, the increase was only 2.50%. Meanwhile, the light energy capture and utilization capacity of PSII reaction centers and the proportion of absorbed light energy used for electron transfer were higher by 3.42% under B deficiency; KWS1197 cultivar managed to alleviate the photo-oxidative damage, which results from excessive absorbed energy (ABS/RC), by increasing the dissipated energy (DIo/RC). Therefore, in response to B deprivation, the KWS1197 cultivar demonstrated greater adaptability in terms of morphological indices and photosynthetic functions, which not only explains the improved performance but also renders the measured parameters as the key features for varietal selection, providing a theoretical basis for the utilization of efficient sugar beet cultivars in future.
Introduction
Boron (B) is a metalloid and a beneficial element for maintaining the structural integrity of cell walls, which can reduce the uptake and accumulation of harmful substances in crops, thereby alleviating physiological damage caused by abiotic stresses (Qin et al., 2021; Qin et al., 2022; Yan et al., 2022). Negative alterations in these aforementioned physiological processes lead to a significant reduction in leaf area as well as biomass, retarded plant growth, and declined yield quality (Wang et al., 2015; Wei et al., 2022). Boron deficiency in soils is a widespread problem in agricultural production. Currently, B deficiency has led to reductions in yields of 132 crops in over 80 countries (Latifi and Jalali, 2018; García-Sánchez et al., 2020). Moreover, the exogenous application of B was reported to enhance dry matter synthesis and accumulation, which leads to an increase in crop yield (Zhou et al., 2013; Ahmed et al., 2020). Additionally, B plays a vital role in photosynthesis and transportation of the photoassimilates (Sharma and Ramchandra, 1990; Mishra et al., 2018). Boron deficiency is known to cause the impairment of leaf structure and function of the plant, resulting in reduced photosynthetic pigment content, reduced CO2 assimilation rate, and modulated blade expansion (Mukhopadhyay et al., 2013; Cong et al., 2015).
The cultivated land in China has a deficiency of B spreading across 33 million hectares. Sugar beet is an economic crop in northern China with a high B requirement. Low B-containing soils inevitably affect yield and sugar content, leading to lower economic returns from sugar beet cultivation and seriously limiting the development of the sugar industry in China. Different crop genotypes vary in their B requirements and utilization capacity (Pommerrenig et al., 2018). Employing B-efficient crops can effectively ameliorate the environmental risks associated with B administration and may help to promote sustainable agricultural development. Earlier studies on screening B-efficient grape cultivars (Vitis vinifera L.) have been reported as early as 1941 (Scott, 1941). Currently, the identification of B-efficient genotypes, with in-depth knowledge of physiological and molecular mechanisms, has been widely carried out in plants such as cotton (Gossypium spp) (Wu et al., 2018), oilseed rape (Brassica napus L.) (Yang et al., 2013), citrus (Citrus sinensis Obs) (Liu et al., 2017) and other horticultural crops.
In previous studies, 57 sugar beet cultivars grown worldwide for B utilization efficiency were identified (Song et al., 2021a; Wu et al., 2021; Song et al., 2022), and have obtained sugar beet B-efficient cultivars. Nevertheless, it is still unclear whether the physiological traits of B-efficient sugar beet cultivars correlate with leaf photosynthetic parameters under B-deficient conditions. The study investigated the changes and associations of leaf growth parameters, chloroplast ultrastructure, photosynthetic rate, and chlorophyll fluorescence (ChlF) parameters in sugar beet cultivars differing in B-efficiency under B deficit. Moreover, the study demonstrated how B-efficient cultivars responded to B stress, leading to changes in the leaf morphology, structure, and photochemical efficiency in different cultivars. This study will provide a theoretical basis for the utilization of B-efficient sugar beet cultivars in future breeding programmes.
Materials and methods
Plant material and growth indices
The current study was carried out at the experimental field of Heilongjiang University, Harbin (longitude: 126.61°, latitude: 45.71°), Heilongjiang, China. The B-efficient (H) genotyped sugar beet cultivar KWS1197 and B-inefficient (L) genotyped cultivar KWS0143 were selected from a previous study conducted by (Song et al., 2021a). The plants were cultivated in an artificial climate chamber (24°C/20°C). The sugar beets were cultured in 2 L containers and the roots were kept in the dark. The relative humidity was 65% and the photoperiod of seedlings was maintained 12:12 L to D; detailed information on the nutrient solution is presented in Table 1 (Hoagland and Arnon, 1950). The plants were cultivated in the quarter-strength nutrient solution (25 μM H3BO3) in the first 7 days, then transplanted with half-strength nutrient solution at 7-day intervals. The plants were cultured in B-sufficient (50 μM H3BO3, B50) and B-deficient (0.1 μM H3BO3, B0.1) nutrient solution from the 7th day (Song et al., 2021b); each treatment had three replications. The sugar beets were harvested at 28 d after treatment. The fresh weight (FW) was measured and oven dried to a constant weight at 60°C and dry weight (DW) to obtain the following parameters.
Relative growth rate (RGR), net assimilation rate (NAR) and mean leaf area ratio (LARm) were determined according to the methods of Poorter (1999).
Measurement of gas exchange parameters
The net photosynthetic rate (Pn), stomatal conductance (Gs), transpiration rate (Tr), and intercellular CO2 concentration (Ci) of fully expanded leaves were measured by the LI-6400 (LI-COR., Lincoln, NE, USA) photosynthesis measurement system. Pn/Tr was used as the water use efficiency (WUE). portable photosynthesis measurement system. All measurements were performed between 9am and 12am, with relative humidity of 50–70%, CO2 concentration of 400 μmol mol−1, air temperature of 25°C to 28°C and photosynthetic photon flux density of 144 μmol m−2 s−1.
Measurement of chlorophyll pigments
The chlorophyll pigment contents were determined according to the method described by Li et al. (2022). The fresh leaves (0.2 g) were cut and immersed in 10 mL of 95% ethanol (48 h) and the absorbance values were measured at 665 nm, 649 nm, and 470 nm.
The content of chlorophyll pigments in the leaf was calculated by the following formula:
Transmission electron microscopy
The cell structure of leaves was analyzed according to the protocol standardized by Song et al. (2019). The blade sections (1mm×2mm) after immersing in 2.5% (v/v) glutaraldehyde solution (2 h), and washing with 0.1 M phosphate buffer (pH 6.8) for 15 min (3 times) at 4°C, were fixed in 1% (w/v) OsO4 for two hours, followed by immersion in ethanol. Post-embedding in Spurr resin, the samples were cut with an ultra-microtome and examined photographed under a transmission electron microscope (H-7500, Japanese).
Measurement of ChlF traits
The OJIP fluorescence induction curves of seedlings with different B efficiency under B deficient treatment were determined using Handy PEA (Hansatech Instruments Limited, Norfolk, UK). The OJIP curves were normalized to O-P and O-J: between Fo and FJ: Vt =(Ft−Fo)/(Fp−Fo), and the differences of samples to the CK (KWS0143 B0.1treatment); between Fo and FI: Wk=(Ft−Fo)/(Fk −Fo) and the differences of the CK (KWS0143 B0.1treatment), and IP phase: (Ft−Fo)/(FI−Fo)−1=(Ft−FI)/(FI−Fo). All ChlF parameters were measured following the methods described by Ye et al. (2019) and Wang et al. (2022). For this particular parameter, there were 9 replicates per treatment which were taken. Detailed information on the ChlF parameters evaluated in this study is presented in Table 2.
Statistics analysis
Significant differences in physiological parameters across the treatments were analyzed by one-way ANOVA using SPSS 25.0 (SPSS Inc, Chicago, IL) software. The illustration figure was drawn using Figdraw (https://www.figdraw.com/static/index.html). The graphs and PCA were drawn using Origin 2022 (Origin Lab Corporation, Wellesley Hills, Wellesley, MA, United States), whereas significant and extremely significant differences were expressed by “*” (P<0.05) and “**” (P<0.01).
Results
Effect of B deficiency stress on growth parameters of sugar beet seedlings with contrasting B efficiency
The development of different cultivars of sugar beet was inhibited by B-deficiency stress. The leaf mass ratio (LMR), support biomass of root (SBR), leaf mass fraction (LMF), and mean leaf area ratio (LARm), all showed an increasing trend under B deprivation. The LMR, LMF, and LARm of the KWS1197 sugar beet cultivar increased by 26.82%, 25.81%, and 56.15% respectively, while those of the KWS0143 cultivar increased by 2.50%, 11.32%, and 13.54% respectively. The increase was greater in the KWS1197 sugar beet cultivar than in the KWS0143 (Figures 1A–C, F). The RGR and NAR of the KWS1197 cultivar decreased by 9.17% and 41.91%, whereas for the KWS0143, it decreased by 13.60% and 24.13%, respectively (Figures 1D, E).
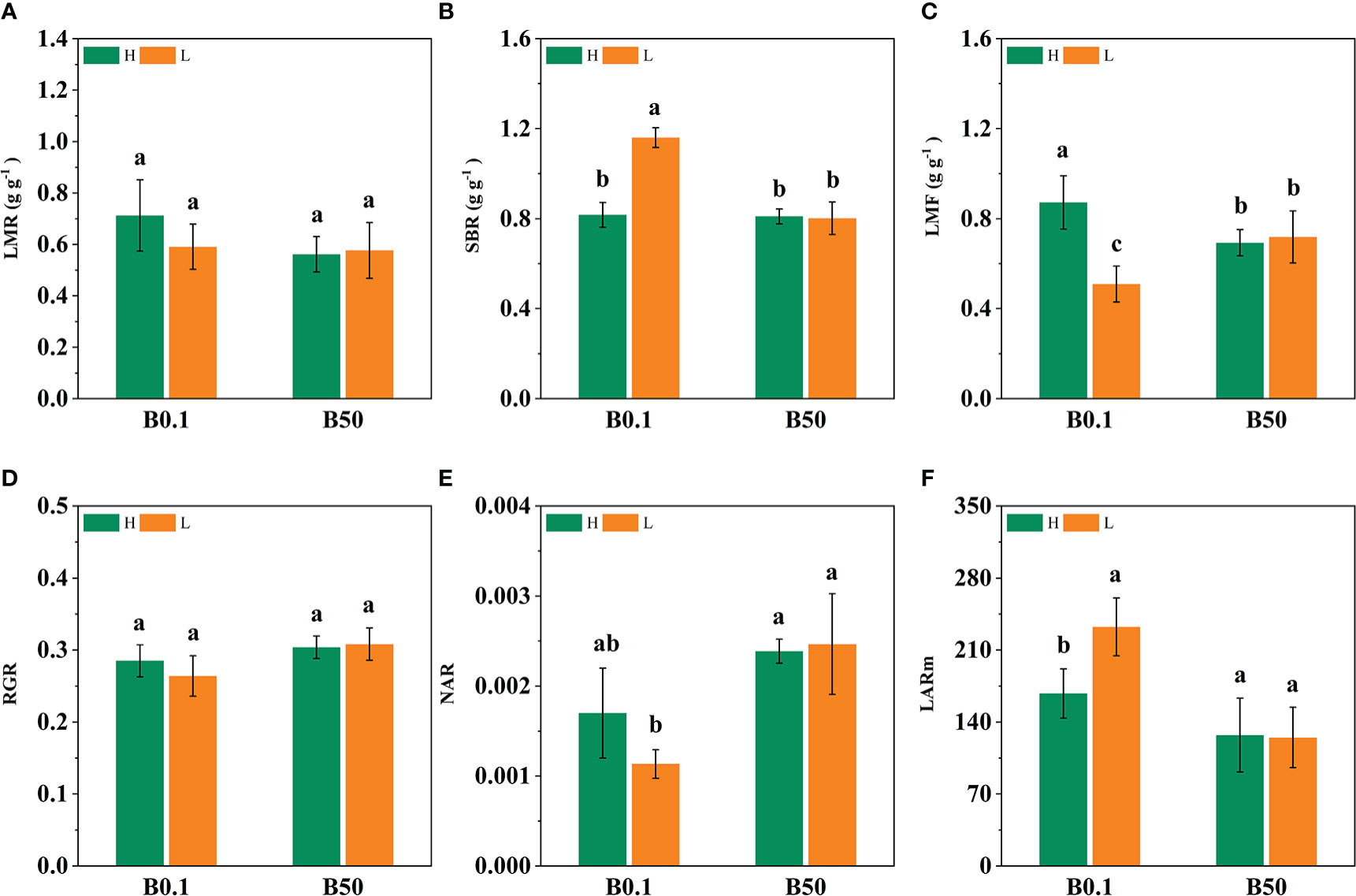
Figure 1 Effect of different B levels on growth paraments of two contrasting sugar beet cultivars (B-efficient: KWS1197(H) and B-inefficient: KWS0143 (L) cultivar). LMR is leaf mass ratio (A). SBR is supporting organ biomass ratio (B). LMF is leaf mass fraction (C). RGR is relative growth ratio (D). NAR is net assimilation ratio (E). LARm is mean leaf area ratio (F). Different letters (a, b, c) within a column represent significant differences at P<0.05. The seedlings were treated with B deficient (0.1 μM H3BO3, B0.1) and B sufficient (50 μM H3BO3, B50).
Effect of B deprivation on photosynthetic and chlorophyll indices of different cultivars of sugar beet
The Pn, Tr, Gs, and Ci of the two sugar beet cultivars’ foliage decreased (P<0.05) under B deficient growth conditions. Compared to the B50 treatment, Pn, Tr, Gs, and Ci of KWS1197 cultivar decreased by 15.29%, 22.84%, 26.84% and 12.08%, respectively (Figure 2). Moreover, the WUE of different sugar beet cultivars increased under B0.1 treatment; compared to the KWS0143 cultivar, the WUE was greater in the KWS1197 cultivar. The chlorophyll pigment content of sugar beet leaves showed no significant changes in response to B deprivation. Under the B0.1 treatment, the KWS0143 cultivars showed a reduction of 13.26%, 7.87%, and 5.75% in Chl a, Chl b, and Chl a/b, respectively. However, in the KWS1197 cultivar, the reduction in Chl a, Chl b, and Chl a/b was only 3.98%, 5.22% and 1.38%, respectively (Figures 3A, B, D). These results showed how the KWS1197 cultivars maintained the chlorophyll pigment system under low B stress conditions. Furthermore, in B-deficient conditions, the car content of sugar beet leaves increased by 2.03% in the KWS1197 cultivar, while a 10.61% decrease was recorded for KWS0143 cultivar (Figure 3C).
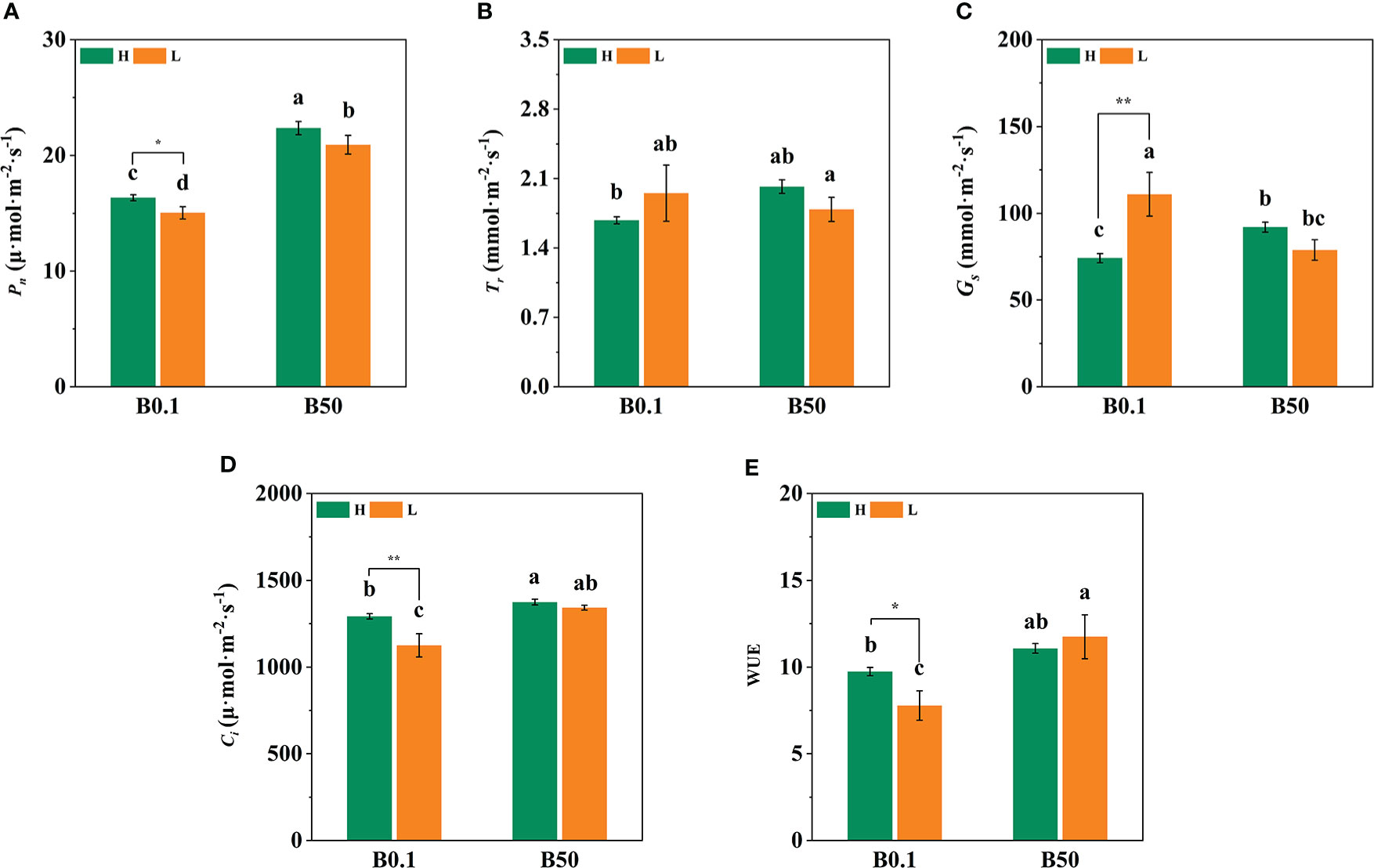
Figure 2 Effect of photosynthesis parameters in KWS1197 and KWS0143 cultivars. The seedlings were treated with B deficient (0.1 μM H3BO3, B0.1) and B sufficient (50 μM H3BO3, B50). Pn is the net photosynthetic rate (A), Tr is the transpiration rate (B), Gs is the stomatal conductance (C), Ci is the intercellular CO2 concentration (D) and WUE is the water use efficiency (E). Different lowercase letters (a, b, c) indicated significant differences between different treatment. Bars denote the mean (n=3) and error bars the standard error.
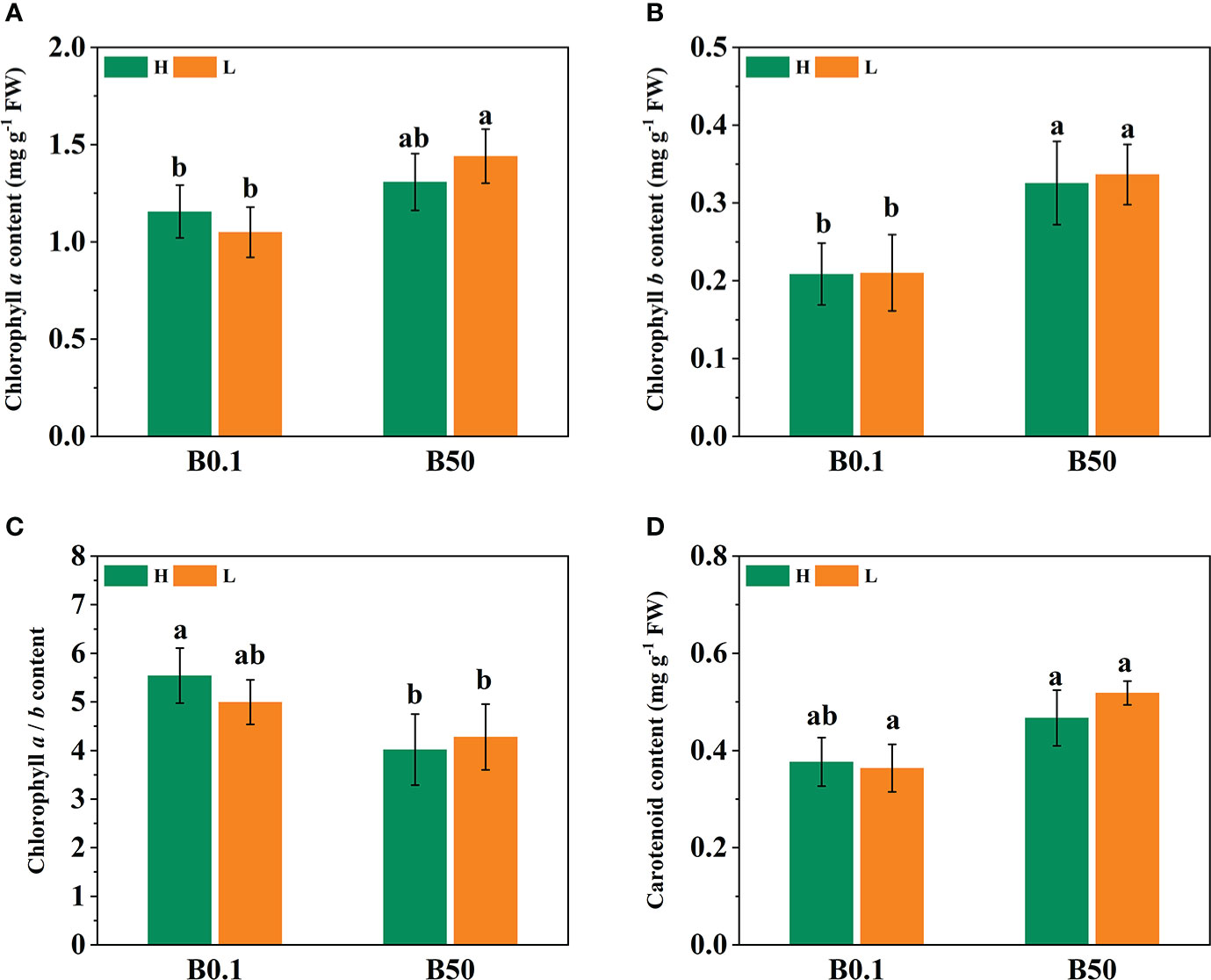
Figure 3 Effect of chlorophyll pigments in KWS1197 and KWS0143 cultivars. The seedlings were treated with B deficient (0.1 μM H3BO3, B0.1) and B sufficient (50 μM H3BO3, B50) treatments. The chlorophyll pigments include the content of chlorophyll a (A), chlorophyll b (B) carotenoid (D) and the ratio of chlorophyll a to chlorophyll b (C). Different lowercase letters (a, b, c) indicated significant differences between different treatments. Bars denote the mean (n=3) and standard error bars.
Effect of B deprivation at the ultrastructural level
The microscopic studies revealed that the internal structure of leaf chloroplast was retained under the B0.1 treatment in KWS1197. In terms of internal cellular structure, under B50 treatment, the cell structure of different cultivars of sugar beet leaves was intact, chloroplasts were well developed (Figures 4B, D), the lamellar structure was neatly arranged, and starch granules were visible. However, under B-deficient conditions, the intergranular thylakoid structure was loose, and the lamella was disordered (Figures 4A, C). Compared to the KWS0143 cultivar, the chloroplast lamellae of the KWS1197 sugar beet cultivar were more stable under B deficiency. There was no apparent disorder of the cystoid matrix compared to the KWS0143 cultivar sugar beet.
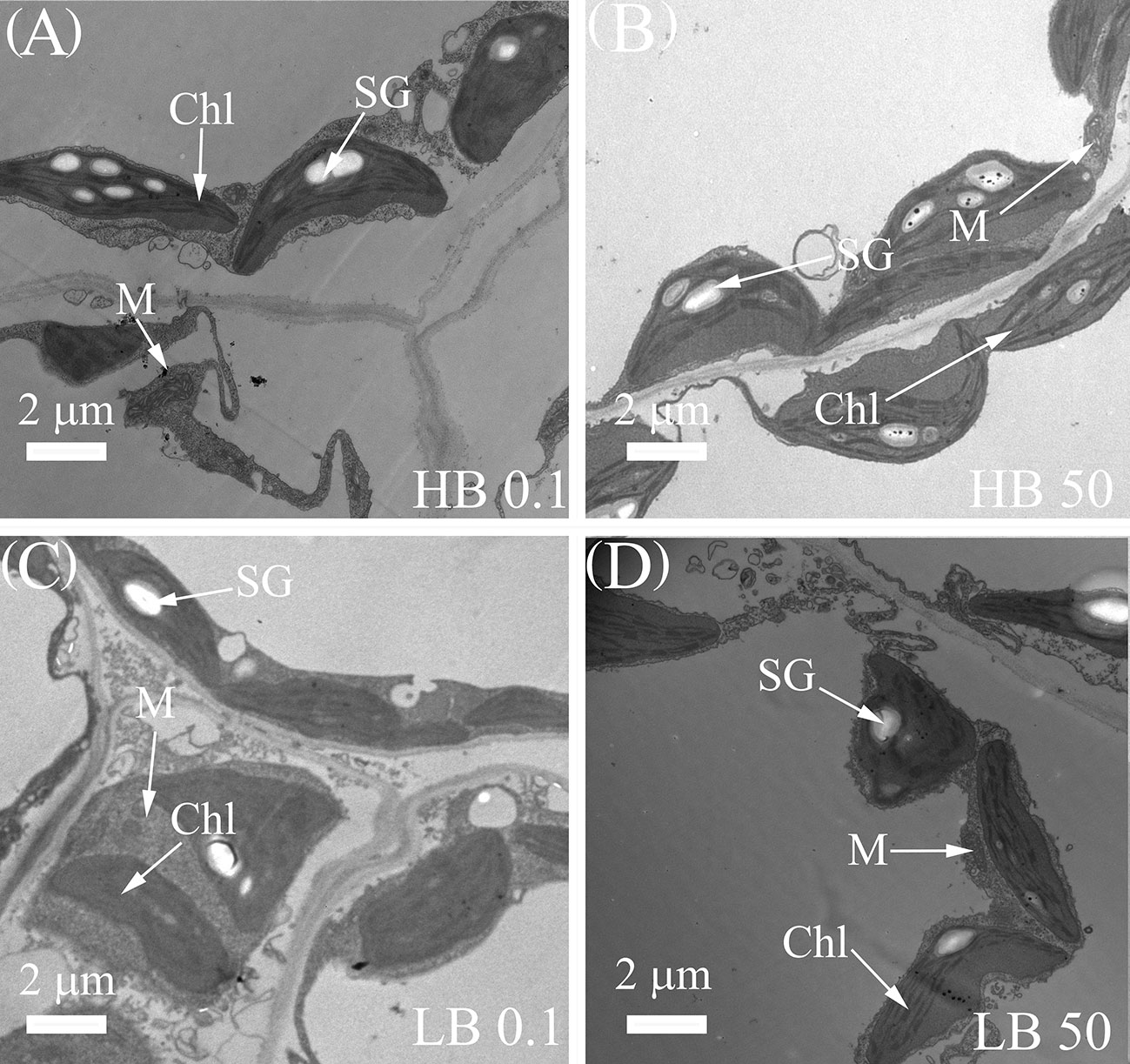
Figure 4 Changes in the subcellular structure of leaves of contrasting B efficiency sugar beet cultivars. The seedlings were treated with B deficient (0.1 μM H3BO3, B0.1) treatment in HB0.1 (A) and LB0.1 (C) and B sufficient (50 μM H3BO3, B50) treatments in HB50 (B) and LB50 (D). Chl, Chloroplast; M, Mitochondrion; SG, Starch Granule.
Effect of B-deprivation on the leaf OJIP transients and energy allocation parameters of PSII of sugar beet with contrasting B efficiency
Boron-deficient conditions caused significant changes in the ΔVt and ΔWk curves of different KWS1197 sugar beet leaves (Figure 5). In the study of ΔVt and ΔWk curves, it was found that in ΔVt curve, there were positive ΔK, ΔJ, and ΔI bands. The magnitude of change in the H sugar beet cultivar was less compared to the KWS0143 cultivar; there were ΔL-band in curve ΔWk. The OJIP curve, the Vt and Wk curves, and the (Ft-FI)/(FI-F0) curve did not show significant changes under B deficit. During B deficiency stress, the ABS/RC, TRo/Rc, and DIo/RC were suggested to increase. The ETo/RC and REo/RC decreased in the two sugar beet cultivars, reflecting increased energy uptake and dissipation in the PSII reaction center of individual reducible QA. However, in B deficiency, the electron energy transferred and the electron flux transported to the terminal electron acceptor of PSI for reduction was decreased, moreover the KWS1197 cultivar was less affected than the KWS0143 cultivar (Figure 6A). The quantum yield to flux ratio of the leaves of both sugar beet cultivars was affected by B deficiency, and its φPo, φEo, φEo/(1-φEo), φRo, ψEo, δRo, and δRo/(1-δRo) were reduced, indicating that the maximum photochemical efficiency, quantum yield for electron transfer, and quantum yield of the terminal electron acceptor on the reduced PSI receptor side, were all reduced by B-deficient stress; the KWS1197 cultivar was reduced to a lesser extent than KWS0143 cultivar (Figures 6B, D). In B deficiency, the flux per leaf cross section of different sugar beet cultivars were adversely affected, in which ABS/CSm and DIo/CSm increased. At the same time, REo/CSm and ETo/CSm decreased, while TRo/CSm indicated that the absorbed energy per unit leaf section increased under B deficiency. The energy flux captured by the PSII active reaction center was less affected when the transferred energy flux was reduced and heat dissipation increased (Figure 6C). In B-deficient conditions, PIABS, PItotal, DFABS, and DFtotal were reduced in the leaves of both cultivars, and the overall functional activity of PSII, PSI, and inter-systemic electron transport chains, as well as the collective driving force, were reduced but to a lesser degree in KWS1197 cultivar (Figure 6D).
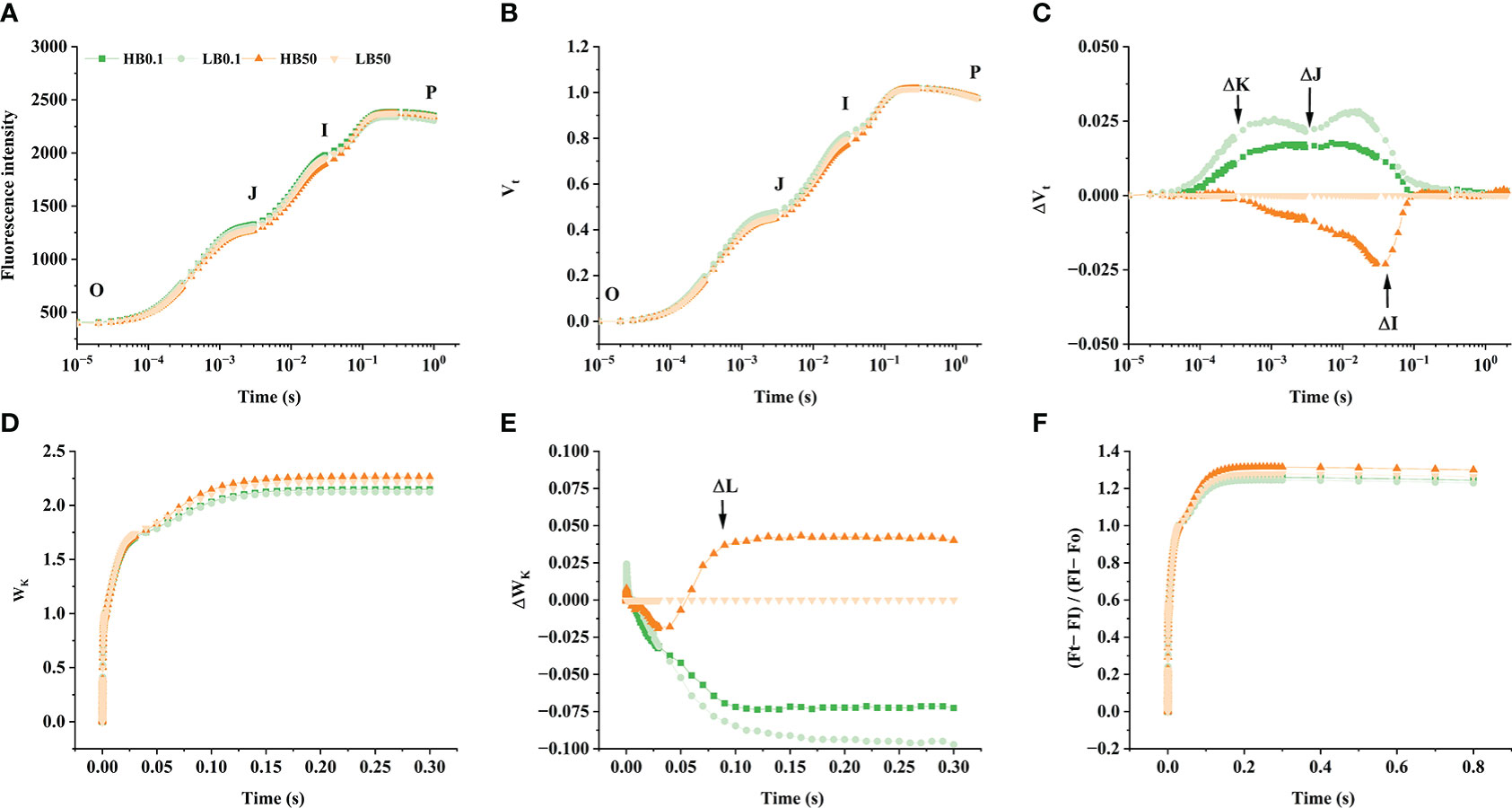
Figure 5 The effect of OJIP curve (A), Vt (B), ΔVt: the difference of Vt (C), Wk (D), ΔWk: the difference of Wk (E) and (Ft-FI)/(FI-F0) (F) of KWS1197(H) and KWS0143 (L) cultivars. The seedlings were treated with B deficient (0.1 μM H3BO3, B0.1) and B sufficient (50 μM H3BO3, B50) treatments.
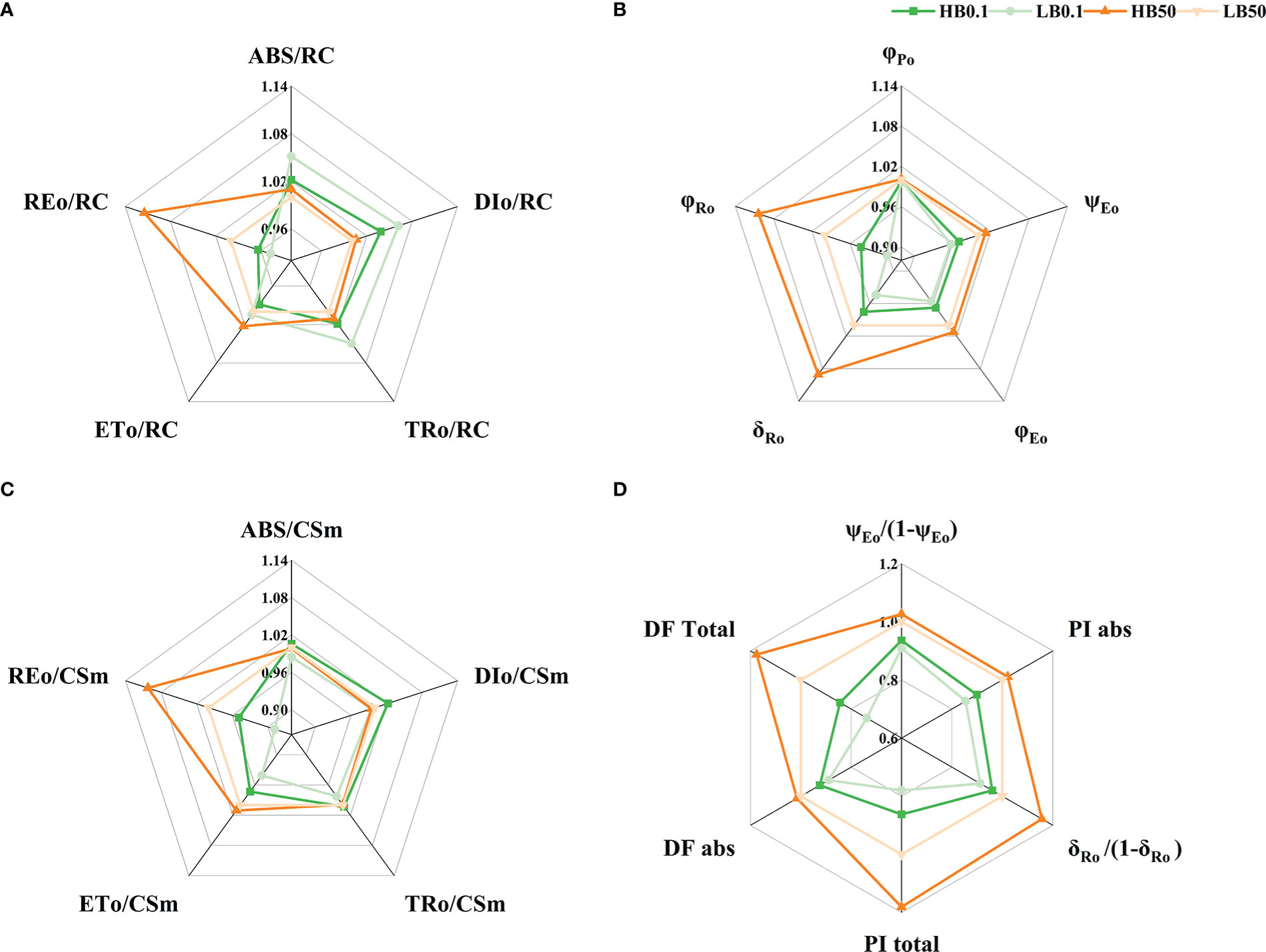
Figure 6 Relationships between parameters describing fluorescence and yield of photosystem II in KW1197(H) and KWS0143(L)cultivars. The seedlings were treated with B deficient (0.1 μM H3BO3, B0.1) and B sufficient (50 μM H3BO3, B50) treatments. The parameters of the energy flux of the PSII reaction center of reducible QA (A), quantum yield and flux ratio (B), phenomenological flux per unit leaf section (t= tFM) (C) and performance index (D). Values are means (n=9) after standardization.
Principal component analysis and correlation among the photosynthetic parameters
The main factors of chlorophyll fluorescence (ChlF) parameters in different sugar beet cultivars were investigated using PCA. PC1 distinguished the energy flux of the PSII reaction center of reducible QA from the quantum yield or flux ratio and the image-only flux per unit leaf section. The highest contribution of image-only flux per unit lobe cross-section indicates that these factors largely measure energy absorption, transfer, and dissipation in the PSII reaction center of reduced QA. PC2 distinguished the quantum yield from the energy flux in the PSII reaction center of reduced QA (Figure 7A). Correlation studies showed that the quantum yield was positively correlated with the ET0/RC and RE0/RC (Figure 7B).
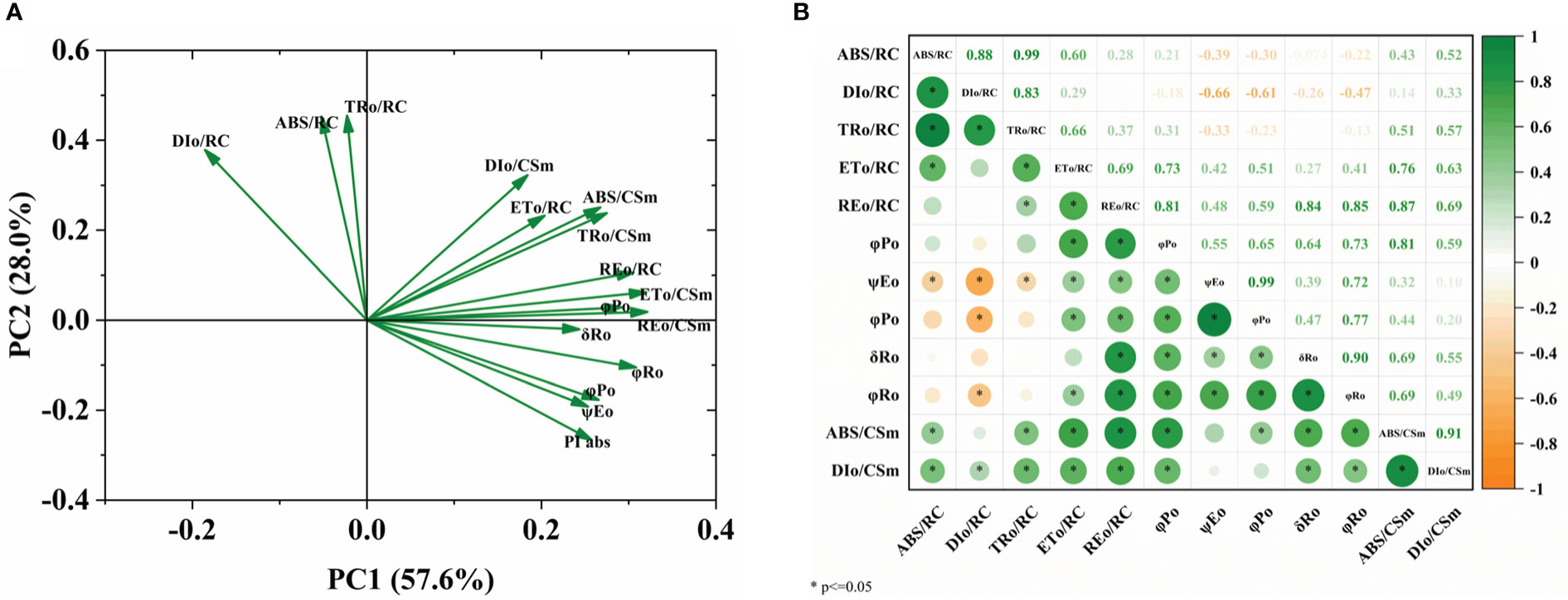
Figure 7 PCA of plant measured factors. (A) Loading plot, (B) correlation matrix. The plants were exposed to B deficient (0.1 μM H3BO3, B0.1) and B sufficient (50 μM H3BO3, B50) treatments.
Discussion
In current study, the KWS1197 cultivar was more advantageous in terms of leaf phenotypic traits, photosynthetic performance, and energy utilization of the PSII (Figure 8). Boron deficiency caused increased LMR and insignificant SBR changes in the two tested sugar beet cultivars, ultimately leading to an improved ratio of assimilated to non-assimilated tissues and a reduced plant growth rate. Compared to the KWS0143 cultivar, the higher growth rate of the KWS1197 seedlings suggest that this sugar beet cultivar’s foliage had a greater adaptive response to the B-deficient environment. It has also been shown that B-deficient growth conditions significantly reduced biomass in Sacha inchi and Neolamarckia cadamba (Yamuangmorn et al., 2021; Yin et al., 2022). Furthermore, a study conducted on citrus plants demonstrated that B-efficient genotypes experienced less reduction in biomass under low B conditions (Liu et al., 2015). Contrastingly, in a study based on B-inefficient genotype of Arabidopsis, the growth rate was significantly reduced, and growth/development was significantly delayed under B deprivation (Pommerrenig et al., 2018), which remains consistent with the present findings. Furthermore, it was revealed that B-deficiency stress significantly impacted the Pn of different sugar beet cultivar seedlings. However, the KWS1197 cultivar underwent lesser reduction and exhibited a rather greater net photosynthetic rate than the B-inefficient variety. Similarly, B deprivation was found to cause a decrease in Pn in cabbage and cotton plants by means of Hill reaction’s inhibition and a decrease in Ci (Choi et al., 2016). Previous studies have shown that B deficiency decreased gas exchange parameters in grapes, but the decrease was also smaller in B-efficient genotyped grape cultivars (Wei et al., 2022).
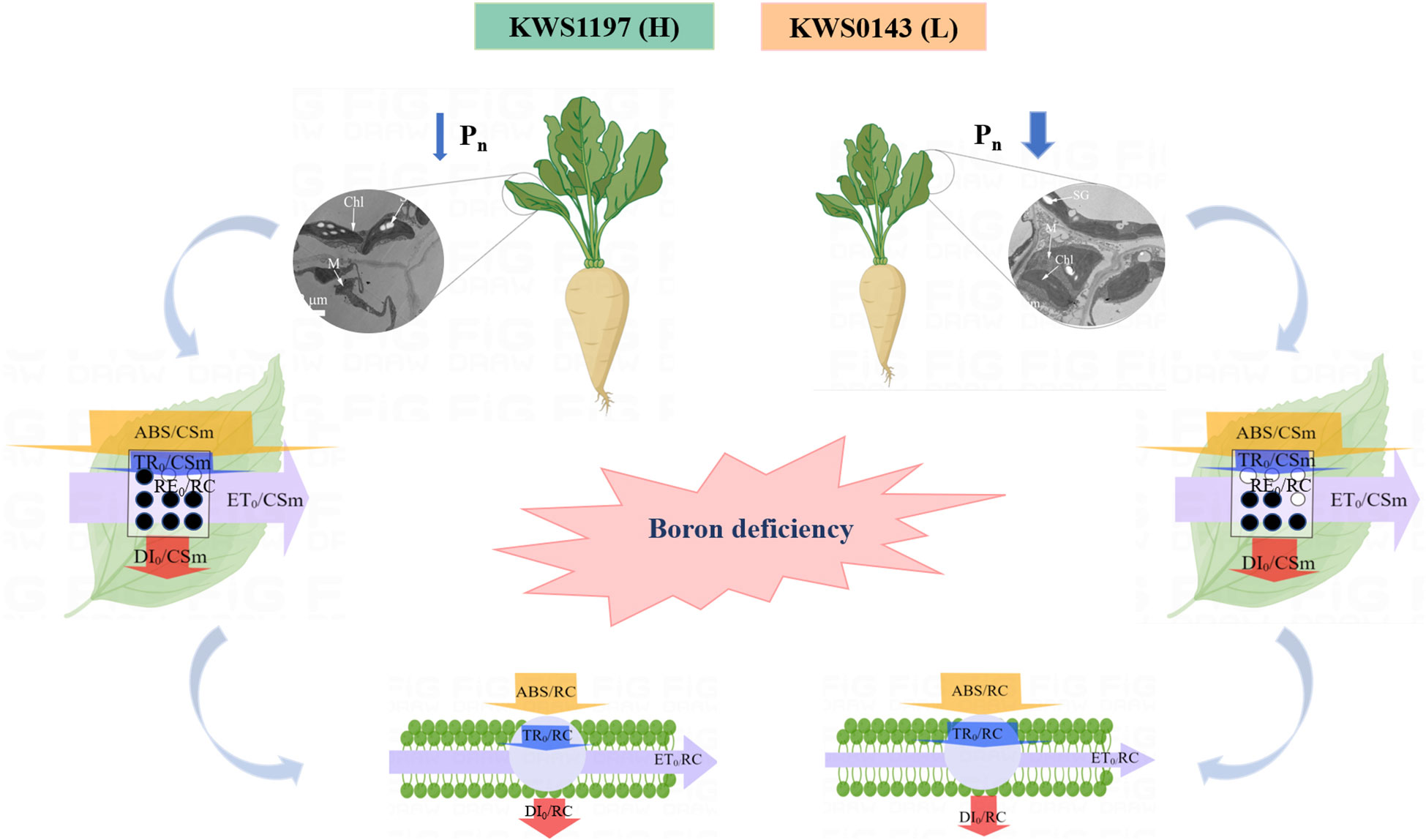
Figure 8 Photosynthetic physiological mechanism of efficient B utilization in seedling leaves of sugar beet cultivars.
Some studies have found that B-deficient environments also affect photosynthetic pigments’ content. For example, B deficiency was found to reduce chlorophyll content in studies on citrus (Hua et al., 2017; Yan et al., 2022). Albeit, other studies have found an increase in chlorophyll content after short-term low B treatment (Chen et al., 2014). Conversely, a study on cotton found that chlorophyll content changed unremarkably when B-deficient stress reduced the Pn of leaves (Liu et al., 2017). The current study found that under B-deficient conditions, the amount of photosynthetic pigment did not change significantly. It might be because the duration of B-deficient treatment and the degree of B-deficient stress were associated, indicating that the change in photosynthetic pigment content in this study was not the dominant factor causing the decline in leaf photosynthetic rate. On the other hand, the effect of B deprivation on the photosynthetic performance of plants was also reflected in the damage to photosynthetic organs. In citrus studies, it was revealed that chloroplast structure was also damaged in B-deficient stress, leading to reduced CO2 assimilation (Yan et al., 2022), and thus affecting photosynthetic rate (Yang et al., 2022). The present investigation established that grana were disorganized and structurally abnormal under B deficiency. Notably, compared to the KWS0143 cultivar, KWS1197 showed a complete chloroplast structure and neatly arranged lamellar structure of leaves.
It has been demonstrated earlier that B deficiency does not directly damage the PSII and impact photosynthesis, but rather the photoinhibition caused by B deficiency is associated with abnormal carbon metabolism (Han et al., 2008). The adverse effects of B deprivation on the photosynthetic system can be reflected by PSII, which eventually causes alterations in fluorescence. The current study showed that the positive ΔK-band indicated OEC deactivation, fewer antenna complex connections, and a distorted thylakoid membrane. Furthermore, it ultimately led to reduced energy transfer and uptake efficiency, which was also observed in studies of nitrogen-deficient as well as calcium-deficient crops (Aleksandrov et al., 2014; Cetner et al., 2017). Additionally, with B deficiency stress, the ABS/RC increased while ETo/RC and ETo/CSm decreased; the DIo/RC and DIo/CSm tended to increase, indicating that B deficiency increased the light energy absorbed while decreasing the energy used for electron transfer in sugar beet seedling leaves, which is consistent with the appearance of a positive ΔK-band. While the dissipated energy increased (DIo/RC and DIo/CSm), which in turn could reduce the excess excitation energy (ABS/RC) in PSII and positively protect the integrity of the electron transport chain. The KWS1197 cultivar’s photochemical efficiency was higher than the KWS0143. In citrus, light energy absorbed also increased because of B deficiency, which implies a partial inactivation of the reaction center (Han et al., 2009) and an increase in the energy dissipated to avoid photo-oxidative damage (Aleksandrov et al., 2014), a mechanism to protect damaged leaves. The same conclusion has been drawn in studies on Lolium Perenne L. (Zhang et al., 2018).
The leading site of impaired electron transport is the inactivation of the PSII receptor side, which indicates the occurrence of photo-inhibition damage (Ye et al., 2019). During B-deficient stress, ΔJ-band and ΔI-band appeared in different cultivars of sugar beet leaves, indicating that B deprivation affected the reduction of the PSII receptor side and oxidation of the PSI receptor side, resulting in the photo-inhibition. It became apparent that φEo, φRo decreased in B deficiency (Figure 6B), demonstrating photochemical reactions on PSII for electron capture as well as transfer. The reduced efficiency of the photochemical reaction on PSII for electron capture and transfer, together with the reduced PIabs, reflects the impaired reduction of the terminal electron acceptors of PSI in sugar beet. The magnitude of change was less in the KWS1197 cultivar than the KWS0143. It was found in studies involving tomato seedlings that the light energy absorbed by the leaf PSII reaction center increased in the presence of reduced REo/CSm (Kalaji et al., 2014). The same finding was established in the present study, which could be a protective mechanism for sugar beet seedlings to enable energy availability under B deficiency.
Conclusion
The current study alluded that B deficiency retarded leaf growth and development of contrasting B efficiency sugar beet cultivars, reducing the photosynthetic rate and photochemical efficiency of the PSII. Meanwhile, inactivation of the PSII receptor side induced the onset of photoinhibition, limiting light energy transfer, and thus reducing the CO2 assimilation rate of sugar beet. The KWS1197 cultivar of sugar beet leaves in comparison with the KWS0143 cultivar under B deficient growth conditions had less reduction in Pn. Meanwhile, the KWS1197 cultivar was suggested to have an accumulation of biomass, a better intact structure of thylakoid, higher energy transfer and absorption efficiency in PSII, a stronger activity of OEC and reaction center, higher light utilization efficiency, and was exposed to a less degree of photoinhibition. The advantage of the KWS1197 cultivar in photochemical utilization under B deficiency is one of the critical reasons for the difference under B efficient conditions, which enriches the theoretical basis for the effective use of B nutrition in sugar beet. The present study will help the breeders understand and select the B-efficient cultivars of sugar beet by screening photosynthetic parameters, which were shown to correlate with biomass and yield parameters.
Data availability statement
The original contributions presented in the study are included in the article/supplementary material. Further inquiries can be directed to the corresponding authors.
Author contributions
XS: Writing-review and editing. Investigation, Software. BS: Conceived and designed the experiments, Supervision and Funding acquisition. JH: Software and Writing review & editing. MFA and QJ and WW and WH: Writing-review & editing. HL and BS and AK and WH: Writing-review & editing and Funding acquisition All authors contributed to the article and approved the submitted version.
Funding
This work is supported by Xinjiang Academy of Agricultural Sciences Youth Science and Technology Backbone Innovation Ability Training Project (xjnkq-2020014) and China Agriculture Research System of MOF and MARA (CARS-170204 and CARS-170705).
Conflict of interest
The authors declare that the research was conducted in the absence of any commercial or financial relationships that could be construed as a potential conflict of interest.
Publisher’s note
All claims expressed in this article are solely those of the authors and do not necessarily represent those of their affiliated organizations, or those of the publisher, the editors and the reviewers. Any product that may be evaluated in this article, or claim that may be made by its manufacturer, is not guaranteed or endorsed by the publisher.
References
Ahmed, N., Azeem, M., Shoujun, Y., Qasim, M., Abbasi, M. W., Hanif, T., et al. (2020). Foliar enrichment of potassium and boron overcomes salinity barriers to improve growth and yield potential of cotton (Gossypium hirsutum l.). J. Plant Nutr. 44, 438–454. doi: 10.1080/01904167.2020.1845365
Aleksandrov, V., Krasteva, V., Paunov, M., Chepisheva, M., Kousmanova, M., Kalaji, H., et al. (2014). Deficiency of some nutrient elements in bean and maize plants analyzed by luminescent method. Bulg. J. Agric. Sci. 20, 24–29.
Cetner, M., Kalaji, H., Goltsev, V., Aleksandrov, V., Kowalczyk, K., Borucki, W., et al. (2017). Effects of nitrogen-deficiency on efficiency of light-harvesting apparatus in radish. Plant Physiol. Biochem. 119, 81–92. doi: 10.1016/j.plaphy.2017.08.016
Chen, M., Mishra, S., Heckathorn, S. A., Frantz, J. M., Krause, C. (2014). Proteomic analysis of arabidopsis thaliana leaves in response to acute boron deficiency and toxicity reveals effects on photosynthesis, carbohydrate metabolism, and protein synthesis. J. Plant Physiol. 171, 235–242. doi: 10.1016/j.jplph.2013.07.008
Choi, E. Y., Jeon, Y. A., Choi, K. Y., Stangoulis, J. (2016). Physiological and morphological responses to boron deficient chinese cabbage. Hortic Environ. Biotechnol. 57, 355–363. doi: 10.1007/s13580-016-0023-y
Cong, X. L., Jing, H. Y., Lin, N., Xia, Z. H., Huang, M. J., Jiang, X. Y. (2015). Boron deficiency affects cell morphology and structure of young leaves of radish. Acta Physiologiae Plantarum 37, 247–257. doi: 10.1007/s11738-015-2004-7
García-Sánchez, F., Simón-Grao, S., Martínez-Nicolás, J. J., Alfosea-Simón, M., Liu, C., Chatzissavvidis, C., et al. (2020). Multiple stresses occurring with boron toxicity and deficiency in plants. J. Hazard. Mater. 397, 122713. doi: 10.1016/j.jhazmat.2020.122713
Han, S., Chen, L. S., Jiang, H. X., Smith, B. R., Yangand, L. T., Xie, C. Y. (2008). Boron deficiency decreases growth and photosynthesis, and increases starch and hexoses in leaves of citrus seedlings. J. Plant Physiol. 165, 1331–1341. doi: 10.1016/j.jplph.2007.11.002
Han, S., Tang, N., Jiang, H. X., Yang, L. T., Li, Y., Chen, L. S. (2009). CO2 assimilation, photosystem II photochemistry, carbohydrate metabolism and antioxidant system of citrus leaves in response to boron stress. Plant Sci. 176, 143–153. doi: 10.1016/j.plantsci.2008.10.004
Hoagland, D. R., Arnon, D. I. (1950). The water-culture method for growing plants without soil (Berkely: The College of Agriculture).
Hua, Y., Feng, Y., Zhou, T., Xu, F. (2017). Genome-scale mrna transcriptomic insights into the responses of oilseed rape (Brassica napus l.) to varying boron availabilities. Plant Soil 416, 205–225. doi: 10.1007/s11104-017-3204-2
Kalaji, H. M., Oukarroum, A., Alexandrov, V., Kouzmanova, M., Brestic, M., Zivcak, M., et al. (2014). Identification of nutrient deficiency in maize and tomato plants by in vivo chlorophyll a fluorescence measurements. Plant Physiol. Biochem. 81, 16–25. doi: 10.1016/j.plaphy.2014.03.029
Latifi, Z., Jalali, M. (2018). Trace element contaminants in mineral fertilizers used in iran. Environ. Sci. pollut. Res. 25, 31917–31928. doi: 10.1007/s11356-018-1810-z
Li, X. F., Riaz, M., Song, B. Q., Liu, H. J. (2022). Phytotoxicity response of sugar beet (Beta vulgaris l.) seedlings to herbicide fomesafen in soil. Ecotoxicol. Environ. Saf. 239, 113628. doi: 10.1016/j.ecoenv.2022.113628
Liu, G. D., Wu, L. S., Peng, S. A., Wang, Y. H., Jiang, C. C. (2015). Differential changes in cell-wall content and boron and calcium concentration in newhall navel orange grafted on two rootstocks differing in boron-deficiency responses. Commun. Soil Sci. Plant Anal. 46, 439–453. doi: 10.1080/00103624.2014.997384
Liu, X., Zhang, J. W., Guo, L. X., Liu, Y. Z., Jin, L. F., Hussain, S. B., et al. (2017). Transcriptome changes associated with boron deficiency in leaves of two citrus scion-rootstock combinations. Front. Plant Sci. 8. doi: 10.3389/fpls.2017.00317
Mishra, S., Heckathorn, S. A., Frantz, J. M., Krause, C. (2018). The effect of boron availability, CO2, and irradiance on relative accumulation of the major boron transport proteins, bor1 and nip5;1. Biol. Plantarum 62, 121–128. doi: 10.1007/s10535-017-0744-5
Mukhopadhyay, M., Ghosh, P. D., Mondal, T. K. (2013). Effect of boron deficiency on photosynthesis and antioxidant responses of young tea plantlets. Russian J. Plant Physiol. 60, 633–639. doi: 10.1134/S1021443713030096
Pommerrenig, B., Junker, A., Abreu, I., Bieber, A., Fuge, J., Willner, E., et al. (2018). Identification of rapeseed (Brassica napus) cultivars with a high tolerance to boron-deficient conditions. Front. Plant Sci. 9. doi: 10.3389/fpls.2018.01142
Poorter, L. (1999). Growth responses of 15 rain-forest tree species to a light gradient: the relative importance of morphological and physiological traits. Funct. Ecol. 13, 396–410. doi: 10.1046/j.1365-2435.1999.00332.x
Qin, S. Y., Xu, Y. F., Liu, H. E., Li, C., Yang, Y., Zhao, P. (2021). Effect of different boron levels on yield and nutrient content of wheat based on grey relational degree analysis. Acta Physiologiae Plantarum 43, 127. doi: 10.1007/s11738-021-03290-6
Qin, S., Xu, Y., Nie, Z., Liu, H., Gao, W., Li, C., et al. (2022). Metabolomic and antioxidant enzyme activity changes in response to cadmium stress under boron application of wheat (Triticum aestivum). Environ. Sci. Pollut. Res. 29, 34701–34713. doi: 10.1007/s11356-021-17123-z
Scott, L. (1941). An instance of boron deficiency in the grape under field conditions. Proc. Amer. Soc Hortic. Sci. 536, 375–378.
Sharma, P. N., Ramchandra, T. (1990). Water relations and photosynthesis in mustard plants subjected to boron deficiency. Indian J. Plant Physiol. 85, 150–154.
Song, X., Hao, X. M., Song, B. Q., Zhao, X. Y., Wu, Z. Z., Wang, X., et al. (2021a). The oxidative damage and morphological changes of sugar beet (Beta vulgaris l.) leaves at seedlings stage exposed to boron deficiency in hydroponics. Sugar Tech. 24, 532–541. doi: 10.1007/s12355-021-01064-5
Song, B. Q., Hao, X. M., Wang, X. L., Yang, S. Y., Dong, Y. F., Ding, Y., et al. (2019). Boron stress inhibits beet (Beta vulgaris l.) growth through influencing endogenous hormones and oxidative stress response. Soil Sci. Plant Nutr. 65, 346–352. doi: 10.1080/00380768.2019.1617641
Song, X., Song, B. Q., Huo, J. L., Riaz, M., Wang, X. L., Huang, W. G., et al. (2022). Boron-efficient sugar beet (Beta vulgaris l.) cultivar improves tolerance to boron deficiency by improving leaf traits. J. Soil Sci. Plant Nutr. 22, 4217–4227. doi: 10.1007/s42729-022-01020-6
Song, X., Wang, X. L., Song, B. Q., Wu, Z. Z., Zhao, X. Y., Huang, W. G., et al. (2021b). Transcriptome analysis reveals the molecular mechanism of boron deficiency tolerance in leaves of boron-efficient beta vulgaris seedlings. Plant Physiol. Biochem. 168, 294–304. doi: 10.1016/j.plaphy.2021.10.017
Wang, L., Riaz, M., Song, B., Song, X., Huang, W., Bai, X., et al. (2022). Study on phytotoxicity evaluation and physiological properties of nicosulfuron on sugar beet (Beta vulgaris l.). Front. Plant Sci. 13. doi: 10.3389/fpls.2022.998867
Wang, N. N., Yang, C. Q., Pan, Z. Y., Liu, Y. Z., Peng, S. A. (2015). Boron deficiency in woody plants: various responses and tolerance mechanisms. Front. Plant Sci. 6. doi: 10.3389/fpls.2015.00916
Wei, R., Huang, M., Huang, D., Zhou, J. Z., Panand, X. J., Zhang, W. E. (2022). Growth, gas exchange, and boron distribution characteristics in two grape species plants under boron deficiency condition. Hortic 8, 374–388. doi: 10.3390/horticulturae8050374
Wu, X. W., Dong, X. C., Li, X. P., Riaz, M., Jiang, C. C. (2018). Leaf structure and chemical compositions are correlated with cotton boron efficiency. J. Plant Nutr. 41, 552–562. doi: 10.1080/01904167.2017.1392571
Wu, Z., Wang, X., Song, B., Zhao, X., Du, J., Huang, W. (2021). Responses of photosynthetic performance of sugar beet varieties to foliar boron spraying. Sugar Tech. 23, 1332–1339. doi: 10.1007/s12355-021-01008-z
Yamuangmorn, S., Laororng, K., Saenchai, C., Lordkaew, S., Dell, B., Prom-U-Thai, C. (2021). Boron requirement for vegetative growth of sacha inchi (Plukentia volubilis l.). J. Plant Nutr. 45, 845–853. doi: 10.1080/01904167.2021.1994594
Yang, L. T., Pan, J. F., Hu, N. J., Chen, H. H., Jiang, H. X., Lu, Y. B., et al. (2022). Citrus physiological and molecular response to boron stresses. Plants 11, 40. doi: 10.3390/plants11010040
Yang, L., Zhang, Q., Dou, J. N., Li, L., Guo, L. F., Shi, L., et al. (2013). Characteristics of root boron nutrition confer high boron efficiency in brassica napus cultivars. Plant Soil 371, 95–104. doi: 10.1007/s11104-013-1669-1
Yan, L., Riaz, M., Cheng, J., Jiang, C. C. (2022). Boron-deficiency and aluminum toxicity activate antioxidant defense and disorganize the cell wall composition and architecture in trifoliate orange leaf. Sci. Hortic. 297, 110961. doi: 10.1016/j.scienta.2022.110961
Ye, X., Chen, X. F., Deng, C. L., Yang, L. T., Lai, N. W., Guo, J. X., et al. (2019). Magnesium-deficiency effects on pigments, photosynthesis and photosynthetic electron transport of leaves, and nutrients of leaf blades and veins in citrus sinensis seedlings. Plants 8, 389–409. doi: 10.3390/plants8100389
Yin, Q., Kang, L., Liu, Y., Qaseem, M. F., Qin, W., Liu, T., et al. (2022). Boron deficiency disorders the cell wall in neolamarckia cadamba. Ind. Crops Prod. 176, 114332. doi: 10.1016/j.indcrop.2021.114332
Zhang, H., Xu, N., Li, X., Long, J., Sui, X., Wu, Y., et al. (2018). Arbuscular mycorrhizal fungi (Glomus mosseae) improves growth, photosynthesis and protects photosystem ii in leaves of lolium perenne L. in cadmium contaminated soil. Front. Plant Sci. 9. doi: 10.3389/fpls.2018.01156
Keywords: Beta vulgaris L., boron deprivation, chlorophyll fluorescence, gas exchange indices, ultrastructure
Citation: Song X, Song B, Huo J, Liu H, Adil MF, Jia Q, Wu W, Kuerban A, Wang Y and Huang W (2023) Effect of boron deficiency on the photosynthetic performance of sugar beet cultivars with contrasting boron efficiencies. Front. Plant Sci. 13:1101171. doi: 10.3389/fpls.2022.1101171
Received: 17 November 2022; Accepted: 19 December 2022;
Published: 16 January 2023.
Edited by:
Muhammad Ahsan Altaf, Hainan University, ChinaReviewed by:
Aqeel Ahmad, University of Florida, United StatesHamza Sohail, Huazhong Agricultural University, China
Sami Abou Fayssal, University of Forestry, Sofia, Bulgaria
Copyright © 2023 Song, Song, Huo, Liu, Adil, Jia, Wu, Kuerban, Wang and Huang. This is an open-access article distributed under the terms of the Creative Commons Attribution License (CC BY). The use, distribution or reproduction in other forums is permitted, provided the original author(s) and the copyright owner(s) are credited and that the original publication in this journal is cited, in accordance with accepted academic practice. No use, distribution or reproduction is permitted which does not comply with these terms.
*Correspondence: Baiquan Song, MTMyMTI5MjkyMjlAMTYzLmNvbQ==; Huajun Liu, Mjg1NDcxNjA5QHFxLmNvbQ==