- 1Department of Soil Science, The Islamia University of Bahawalpur, Bahawalpur, Pakistan
- 2Department of environmental science, Shaheed Benazir Bhutto University, Sheringal, Pakistan
- 3School of Biological Sciences, The University of Western Australia, Perth, WA, Australia
- 4National Research Center of Intercropping, The Islamia University of Bahawalpur, Bahawalpur, Pakistan
- 5Provincial Reference Fertilizer Testing Laboratory, Raiwind Lahore, Lahore, Pakistan
- 6Institute of Agroindustry and Environment, The Islamia University of Bahawalpur, Bahawalpur, Pakistan
- 7Plant Production Department, College of Food and Agriculture Sciences, King Saud University, Riyadh, Saudi Arabia
- 8Department of Agronomy, Faculty of Agriculture, University of Kafrelsheikh, Kafr el-Sheikh, Egypt
Introduction: The burgeoning population of the world is causing food insecurity not only by less food availability but also by the malnutrition of essential nutrients and vitamins. Malnutrition is mostly linked with food having micronutrients lower than the optimal concentration of that specific food commodity and becoming an emerging challenge over the globe. Microbial biofortification in agriculture ensures nutritional security through microbial nitrogen fixation, and improved phosphate and zinc solubilization, which increase the uptake of these nutrients. The present study evaluates the novel plant growth-promoting rhizobacteria (PGPR) to biofortify maize gain.
Methods: For this purpose, a pot and two field experiments for maize were conducted. PGPRs were applied alone and in combination for a better understanding of the biofortification potential of these strains. At physiological maturity, the growth parameters, and at harvest, the yield, microbial population, and nutritional status of maize were determined.
Results and discussion: Results revealed that the consortium (ZM27+ZM63+S10) has caused the maximum increase in growth under pot studies like plant height (31%), shoot fresh weight (28%), shoot dry weight (27%), root fresh (33%) and dry weights (29%), and microbial count (21%) in the maize rhizosphere. The mineral analysis of the pot trial also revealed that consortium of ZM27+ZM63+S10 has caused 28, 16, 20, 11 and 11% increases in P, N, K, Fe, and Zn contents in maize, respectively, as compared to un-inoculated treatment in pot studies. A similar trend of results was also observed in both field trials as the consortium of ZM27+ZM63+S10 caused the maximum increase in not only growth and biological properties but also caused maximum biofortification of mineral nutrients in maize grains. The grain yield and 1000-grain weight were also found significantly higher 17 and 12%, respectively, under consortium application as compared to control. So, it can be concluded from these significant results obtained from the PGPR consortium application that microbial inoculants play a significant role in enhancing the growth, yield, and quality of the maize. However, the extensive evaluation of the consortium may help in the formulation of a biofertilizer for sustainable production and biofortification of maize to cope with nutritional security.
1. Introduction
Cereals are the most planted crops and common person’s food all over the world. Maize provides vitamins, starch, fiber, protein, and sugar; 100 g of maize grains contains 361 calories. Maize at its vegetative stage is also used as animal feed/fodder and provides the chief source of energy for livestock and poultry feeding (Arain, 2013). Due to the rapid increase in the world’s population, the food demand has increased and hence cereals production needs to be increased with every passing day. However, the annual yield and productivity of cereals are declining in the developing world mainly in Asia. The use of balanced inputs (fertilizers, pesticides, herbicides) in the recent era is the key to gaining maximum production of cereals (Pingali and Heisey, 2001). The reason behind the lower production of maize in Pakistan includes water scarcity, non-availability at critical stages and the rising cost of chemical (fertilizers and pesticides), and the availability of certified hybrid seed in the market. Moreover, climate change is causing a 15-20% decline in the production of hybrid varieties to their actual potential (Ayub et al., 2021). Furthermore, climate change is posing serious threats to crop production in developing countries like Pakistan and shifting them from food surplus to food dearth countries (Abbas, 2022).
Imbalanced utilization of macronutrients, diminished use of organic fertilizers and natural manure, decreased incorporation of plant residues, and exhaustive maize yield in the previous decade have caused micronutrient inadequacies in the soils of Pakistan (Bashir et al., 2021). The greater parts of the soil in maize growing territories of Pakistan have turned out to be inadequate in Zn and Fe. Microorganisms in combination with chemical and organic fertilizers are increasing the uptake of micronutrients, which results in a higher concentration of micronutrients particularly Fe and Zn in plants (Steven, 1991; Mishra et al., 2004; Shivay et al., 2010). The nitrogen requirements of maize are increasing to get higher yields of maize. Balanced nitrogen application along with other macro/micronutrients is important for acquiring efficient grain yield and quality (Zebrath et al., 2009). Nitrogen deficiency is the most significant yield-limiting factor for grain crops (Shah et al., 2003). The use of phosphorus alongside Rhizobium inoculation improves root and plant development and ultimately yield of crops (Gentili and Huss-Danell, 2003; Fatima et al., 2007). Qualities of oat, for example, grain yield, chlorophyll, and protein contents were improved when PGPR were associated with roots (Tawfiq and Ahmad, 2014). Bio-fertilizers are helpful in the incorporation of microbes in the soil that colonize plant roots and expedite plant development by various mechanisms (Glick, 1995). The utilization of PGPR is consistently expanding in agribusiness because it enhances fertilizer use efficiency, decreases fertilizer requirements for crops, and in addition utilization of synthetic pesticides and different agrochemicals (Rana et al., 2012). The PGPR can enhance the uptake and micronutrient biofortification (N, P, Fe, Zn, and Cu) in cereal grains, through nutrient solubilization, siderophores, and exopolysaccharides production (Jalal et al., 2021; Shahane and Shivay, 2022). Biofortification through rhizobacteria has gained popularity to improve Zn and other micronutrient contents in grain crops. For instance, Gopalakrishnan et al. (2016) revealed the species from the genera Pseudomonas, Brevibacterium, Bacillus, Enterobacter, and Acinetobacter as potential candidates for biofortification and biocontrol in plants. These microorganisms also can incorporate micronutrients inside eatable plant tissues through solubilization of their indigenous insoluble sources present in the soil (Khalid et al., 2015; Gopalakrishnan et al., 2016). The Consultative Group on International Agricultural Research (CGIAR) has reported the hereditarian potential for increment in bioavailable Fe and Zn contents in grain crops for example; rice, wheat, and maize after harvest (Cakmak et al., 2010; de Santiago et al., 2011; Galindo et al., 2021).
The utilization of PGPR for biofortification not just encourages us to manage the issue of malnutrition among the population, in addition, improves cereals yield, soil fertility, and biodiversity (Bouis et al., 2003; White and Broadley, 2005; Ditta et al., 2022; Jalal et al., 2022). The soil microbial community is a solid pole to assess soil richness and directs the accessibility of supplements to harvest plants. Any change in the microbial community in this manner can influence the supplement take-up by harvest plants (Nuttall et al., 2017). The presence of Zn in the soil is not an issue in soils but its availability to plants remains an issue throughout the growing season of crops and hence lowers their fortification and fertility status of the soil (Sharma et al., 2013; Majeed et al., 2022). However, the Zn deficiency in Pakistani soils is mainly due to the calcareous and basic nature of our soils. The applied Zn solubilizing PGPR populations help in this regard to solubilize Zn in plant-available form and its uptake by plants (Rana et al., 2012). These microbes help plants to take up more Zn from the soil through Zn solubilization through organic acids secretions (Hussain et al., 2015) and expanding the surface area of roots through the production of auxins in the rhizosphere. Maize is the staple diet of most of the population of the world including Pakistan. Keeping in view the discussion above, it can be hypothesized that the sole and combined application of PGPR may have the potential to improve maize yield and quality. The objective of the present investigation is to biofortify maize by pre-isolated and characterized Zn solubilizing PGPR strains without any harmful effects on the environment from chemical fertilizer application and to cope with nutritional insecurity.
2. Materials and methods
2.1. Collection of bacterial strains
Pre-isolated Zn solubilizing and siderophore-producing bacterial strains Bacillus subtilis. ZM63, Bacillus aryabhattai. ZM31, Bacillus aryabhattai. S10 and Paenibacillus polymyxa. ZM27, having accession numbers KX788861, KX788860, KX788862, and KX788859, respectively, (Najm-ul-Sehar et al., 2015; Mumtaz et al., 2017) were taken from the Soil Microbiology and Biotechnology Laboratory, Department of Soil Science, The Islamia University of Bahawalpur. The strains were tested in the present study to investigate their potential for Fe and Zn biofortification in maize.
2.2. Preparation of consortium
Fresh cultures of each bacterial strain were inoculated in Luria-Bertani broth medium prepared in a 250 mL conical flask and kept in a shaking incubator at 30 ± 1°C for 48 h. After incubation, equal volumes from each strain (having optical density OD600 = 0.65) were mixed and vortexed for 1 min for homogenization of the inoculum to make a consortium as described by Dar et al. (2020).
2.3. Seed disinfection and inoculation
Hybrid seeds of the maize were soaked in sodium hypochlorite solution (5%) for 2 min followed by 30 s dipping in the 70% ethanol and rinsing six times with distilled autoclaved water for removal of chemicals from the seed surface. The surface sterilized seeds of the maize were moistened with 10% sugar solution and coated by slurring in a 4:5 carrier-to-inoculum ratio for single as well as co-inoculation, however, the control treatment was prepared by using sterilized Luria-Bertani broth (Zahir et al., 2018).
2.4. Pot trial
A pot experiment was carried out in the wirehouse of the Soil Science Department, at the Islamia University of Bahawalpur. The atmosphere of Bahawalpur is dry with normal precipitation is under 250 mm and the soil is dominated by Aridisols order as per the taxonomical classification of USDA (Soil Survey Staff, 2006). Soil from the field was collected, air-dried, sieved, and filled in the pots at 12 kg soil per pot. Before planting, the soil sample was taken, dried, blended, sieved, and examined for the physicochemical attributes of the soil (Table S1). The treatments (T0=Control, T1=ZM27, T2= ZM31, T3=ZM63, T4=S10, T5=ZM27+ZM31, T6=ZM27+ZM63, T7=ZM27+S10, T8=ZM31+ZM63, T9=ZM31+S10, T10=ZM63+S10, T11=ZM27+ZM31+ZM63, T12=ZM27+ZM31+S10, T13=ZM27+ZM63+S10, T14=ZM31+ZM63+S10, T15=ZM27+ZM31+ZM63+S10) in pots were arranged in a completely randomized design (CRD) replicated thrice. Full-recommended doses of P and K (90 kg ha-1 and 60 kg ha-1, respectively) and one-third of the N (120 kg ha-1) were applied before sowing as di-ammonium phosphate (46% P2O5 and 18% N), sulfate of potash (50% K2O), and urea (46% N), respectively. Whereas the remaining nitrogen was applied in two splits at the tillering and physiological maturity/flowering. After 70 days, the crop was reaped for growth parameters. Shoot samples were gathered, air-dried, ground, and stored for mineral determination.
2.5. Field trials
The validity of the pot experiment results was tested through field experimentation. Two field experiments were carried out in both seasons at the research farm, Department of Soil Science, the Islamia University of Bahawalpur. The treatments were arranged in a randomized complete block design (RCBD), with three blocks assumed as replications with an experimental unit size of 5x5 ft2. These field trials were conducted with similar treatments as those used in the pot experiment. The fertilizer requirements and application were also done as in pot experimentation. The maize crop was irrigated by available canal water. After maturity crop was harvested for growth and yield parameters. Growth parameters were noted at the time of harvesting, after that plant and grain samples were prepared to analyze for N, P, and K.
2.6. Nutrient analysis in shoots and grains
The plant samples were digested as per Wolf’s method (Wolf, 1982). Oven-dried and ground plant shoot and grain samples (0.1 g) were taken in a conical flask and placed overnight after adding 5 mL of concentrated H2SO4 at room temperature in a fume cabinet. Overnight incubated samples were spiked with 1 mL H2O2 (35%) before heating on a hot plate at 350 °C. The process of addition of H2O2 and heating was repeated until colorless/milky appearance. The material was filtered and diluted up to 50 mL with distilled water. The filtrate was used for the determination of Nitrogen through the Kjeldahl apparatus, phosphorus on the spectrophotometer, potassium on the flame photometer, and iron and zinc on the atomic absorption spectrophotometer following Ryan et al. (2001).
2.7. Bacterial population (CFU × 104) in the rhizosphere
The rhizospheric microbial population was determined from the rhizosphere soil samples taken at harvesting. These samples were immediately shifted to the laboratory and placed at 4°C until analyzed. These samples were analyzed for bacterial population (cfu/g soil) through serial dilution and spread plate technique using general purpose medium (GPM). The inoculated plates were placed in an incubator at 30 ± 2°C for 48h. Afterward, the colonies from the dilution ranging between 30-300 were counted and expressed in scientific notation as per the method described by Alexander (1982).
2.8. Statistical analysis
Data obtained from pot and field trials was computed statistically for significance through one-way ANOVA, respectively, on Statistix 8.1® computer-based software (Steel et al., 1997). However, the difference among treatment means was computed by applying Least Significance Difference (LSD) test at 5% probability (Montogomery, 2013).
3. Results
To evaluate the effectiveness of a novel bioinoculant for the biofortification of maize, a pot experiment and two field experiments were conducted at a wirehouse and research area of the Department of Soil Science, the Islamia University of Bahawalpur, respectively. The pre-identified and compatible PGPR strains ZM27 (KX788859), ZM31 (KX788860), ZM63 (KX788861), and S10 (KX788862) and their possible combination were tested in pot and field trials to determine the role of these bacterial strains in zinc and iron uptake and biofortification in maize grin to fulfill the nutritional requirement of zinc of the burgeoning population through natural sources.
3.1. Pot trial
3.1.1. Growth promotion of maize by PGPR application
Plant height and shoot fresh biomass were significantly enhanced because of the inoculation/co-inoculation of PGPR strains as compared to the un-inoculated control (Table 1). Single as well as consortium application of the bacterial strains had an impact on plant height and shoot fresh biomass of maize in a pot experiment. Plant height was significantly enhanced under sole inoculation of PGPR strains except for ZM27, which has caused a non-significant increase in plant height, to the un-inoculated control. Maximum plant height was caused in treatment receiving PGPR consortium (ZM27+ZM63+S10) followed by co-inoculation (ZM27+S10) causing 31 and 28%, increases as compared to control, respectively. Similar findings were also observed under shoot fresh biomass where ZM27+ZM63+S10 has caused a 28% increase in shoot fresh biomass as compared to the un-inoculated control. Whereas the impact of bio inoculants on shoot dry and root fresh weight of maize was also depicted in (Table 1). Among sole inoculation, S10 showed a maximum increase in shoot dry biomass followed by ZE27+ZM63+S10 has caused a 27 and 33% increase in shoot dry biomass and root fresh biomass, respectively. Results with respect to the capability of various PGPR strains for improving the root dry biomass of maize are presented in Table 1. Maximum root dry weight 7.28 g plant-1 was observed under consortia application of PGPR strains. Sole treatments of bacterial strains also exhibited significant improvements in root dry weight. Whereas, among co-inoculation, a significant increase (29%) in root dry biomass was noted under ZM27+S10 co-inoculation, as compared to control.
3.1.2. Mineral contents of maize
Consortium application of the PGPR strains gave more promising results compared to sole and co-inoculation. An increase in nitrogen content of 4% was found with the sole application of PGPR strains S10. Among co-inoculated treatments, a significant increment in nitrogen contents of 14 and 10% was observed under ZM27+S10 and ZM63+S10, respectively as compared to the un-inoculated control. The maximum increase in nitrogen contents was caused by the application of consortium (ZM27+ZM63+S10) which was 16%, as compared to the un-inoculated treatment. Effects of novel bio inoculant on phosphorous and potassium content in the shoot of maize are also demonstrated in Table 2. Treatments with sole application caused a non-significant increment in phosphorous content whereas the treatments with co-inoculation and consortium application have caused significant improvements in shoot phosphorus contents. However, the consortium application (ZM27+ZM63+S10) caused a maximum increment in phosphorous content showing a 23% increase in grain phosphorous. Potassium contents were significantly improved under all application methods, however, the co-inoculation and consortia application indicated more promising outcomes as a maximum increase in potassium contents (18%) was observed under the ZM27+ZM63+S10 consortium application.
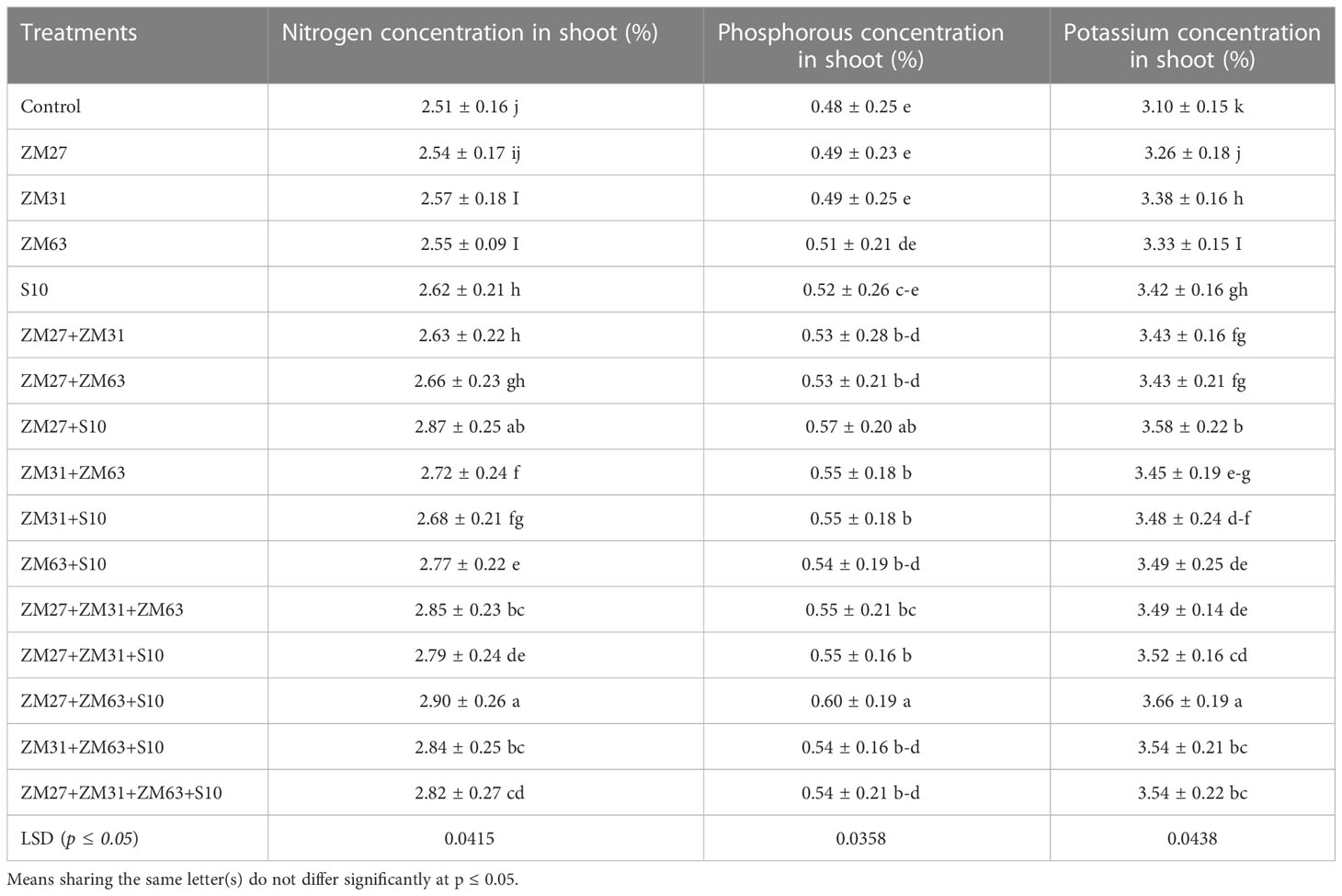
Table 2 Effect of novel bio inoculants on root dry biomass and macronutrients contents of maize in the pot trial.
3.1.3. Zn and Fe biofortification
The efficacy of novel bioinoculants in micronutrient (iron and zinc) uptake in maize shoots is presented in Figures 1, 2, respectively. The application of microbial inoculants significantly improves shoot Zn and Fe in maize as compared to the control treatment. The maximum increase in maize shoot Fe contents (18%) was caused by the consortium inoculation (ZM27+ZM63+S10) as compared to the non-inoculated treatment. Similar results regarding Zn shoot contents were also found under consortium application where ZM27+ZM63+S10 consortium has caused a 15% increase in maize shoot Zn contents followed by co-inoculation of ZM27+S10 where 13% more Zn contents were recorded as compared with the un-inoculated control.
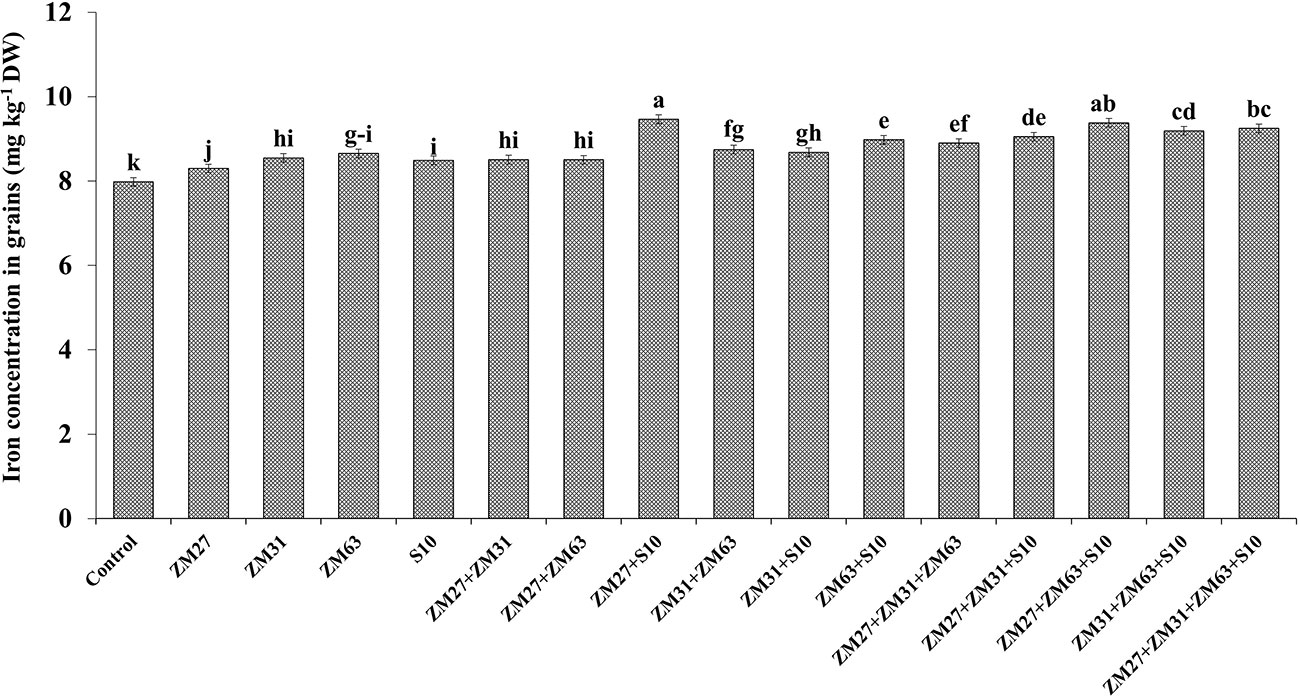
Figure 1 Effect of PGPR inoculation on shoot iron contents in maize in the pot trial. The bars with different letters are significantly different at p ≤ 0.05.
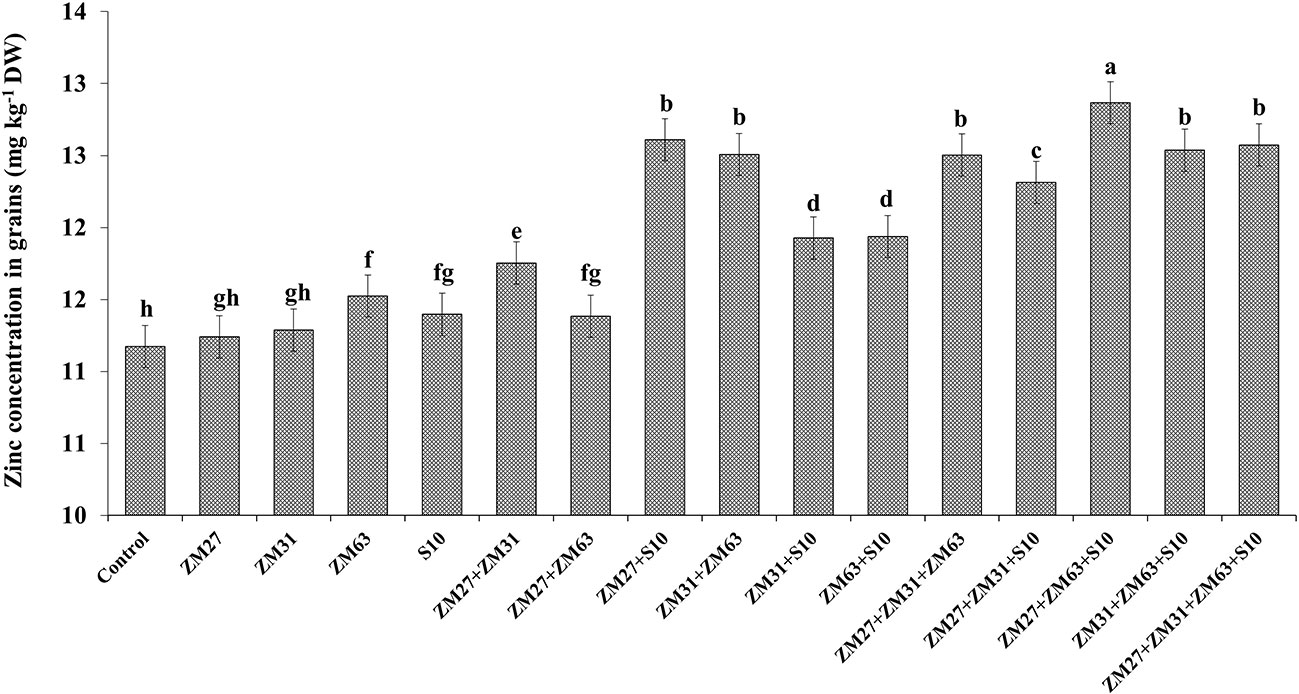
Figure 2 Effect of PGPR inoculation on shoot zinc contents in maize in the pot trial. The bars with different letters are significantly different at p ≤ 0.05.
3.1.4. Microbial population
The application methods of novel PGPR strains significantly improved the soil quality in terms of the bacterial population (CFU x 104) in the maize rhizosphere the results are presented in Figure 3. Under co-inoculated treatments, ZM27+S10 and ZM63+S10 caused maximum increment (15%) in the bacterial population as compared to the un-inoculated control. The correlation of sole, co, and consortium application revealed that consortia application as ZM27+ZM63+S10 showed the highest increment in microbial population by 23% followed by ZM31+ZM63+S10 that demonstrated a 21% increase in bacterial population as compared to the control treatment.
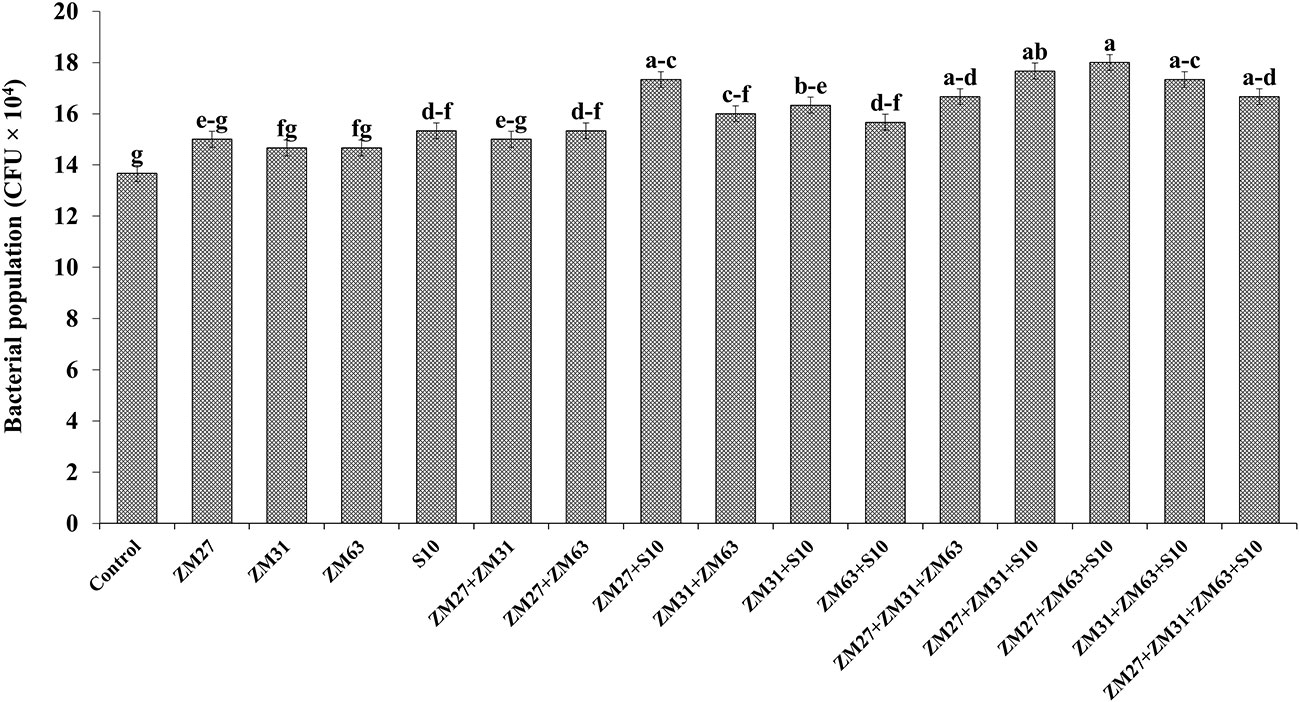
Figure 3 Effect of PGPR inoculation on microbial population in maize rhizosphere in the pot trial. The bars with different letters are significantly different at p ≤ 0.05.
3.2. Field trials
3.2.1. Growth promotion of maize by PGPR application
Different application methods of these promising PGPR strains (sole, co, and consortium application) significantly improved maize growth under both field trials (Table 3). Maximum increments in maize shoot lengths of 13 and 16% were caused by the consortium application (ZM27+ZM63+S10) in both field trials, respectively. However, co-inoculation (ZM27+S10) significantly improved the shoot length of maize by 11% in experiment I. Similarly, the PGPR application also significantly enhanced the shoot fresh weight of the maize; the maximum increase in shoot fresh weight was found under the PGPR consortium (ZM27+ZM63+S10) application which was 19 and 23% in field trial I and II, respectively, as compared to the un-inoculated control.
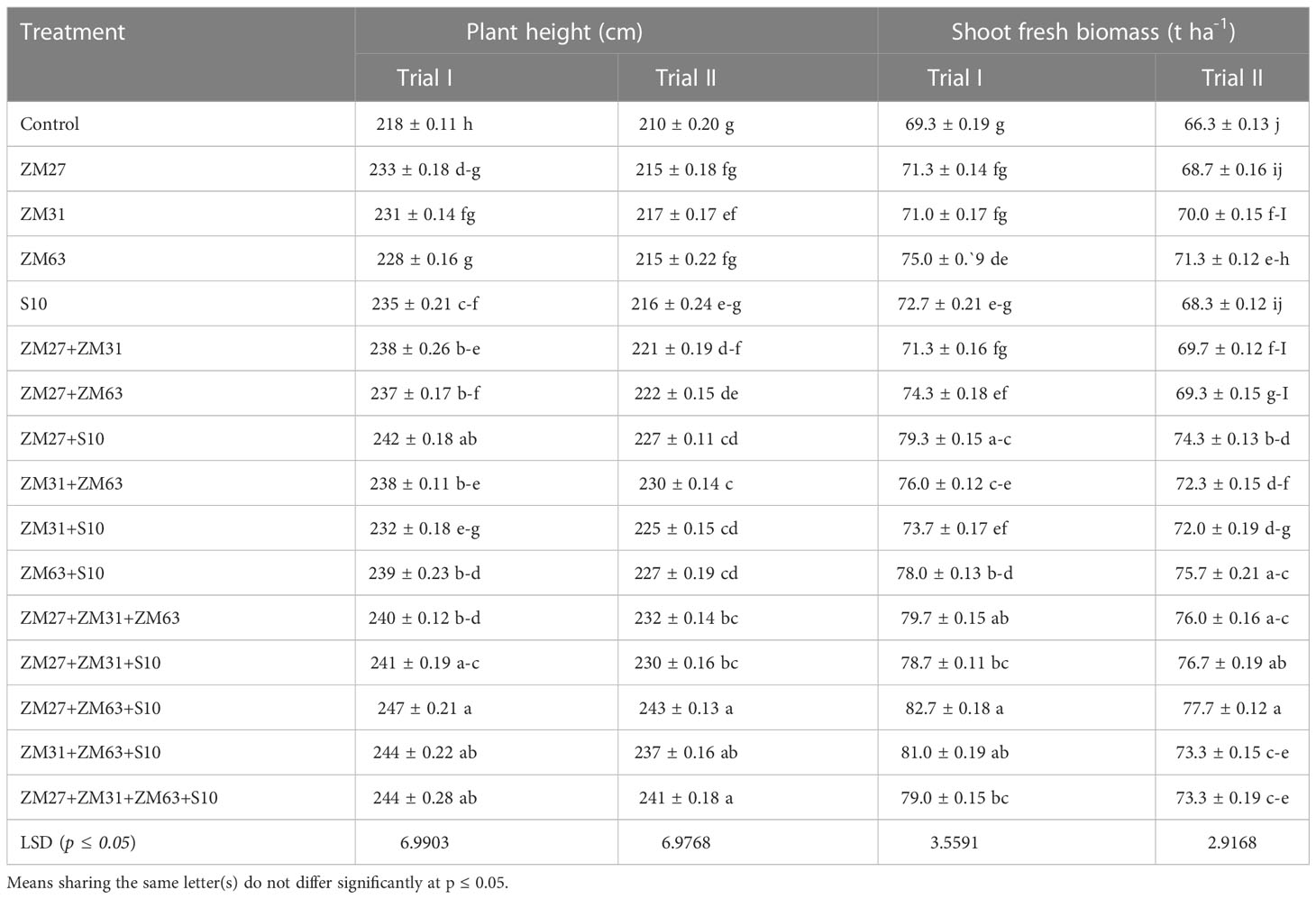
Table 3 Effect of novel bio inoculants on plant height and shoot fresh biomass of maize in field trials.
The PGPR inoculants improved the shoot dry biomass of maize under field conditions (Table 4). The data depicted that the sole inoculation of PGPR has improved by 13% shoot dry weight, while co-inoculation improved by 21% as compared to control. However, the maximum increase in shoot dry weight was observed under the consortium application of PGPR which was found 26% more than the un-inoculated control.
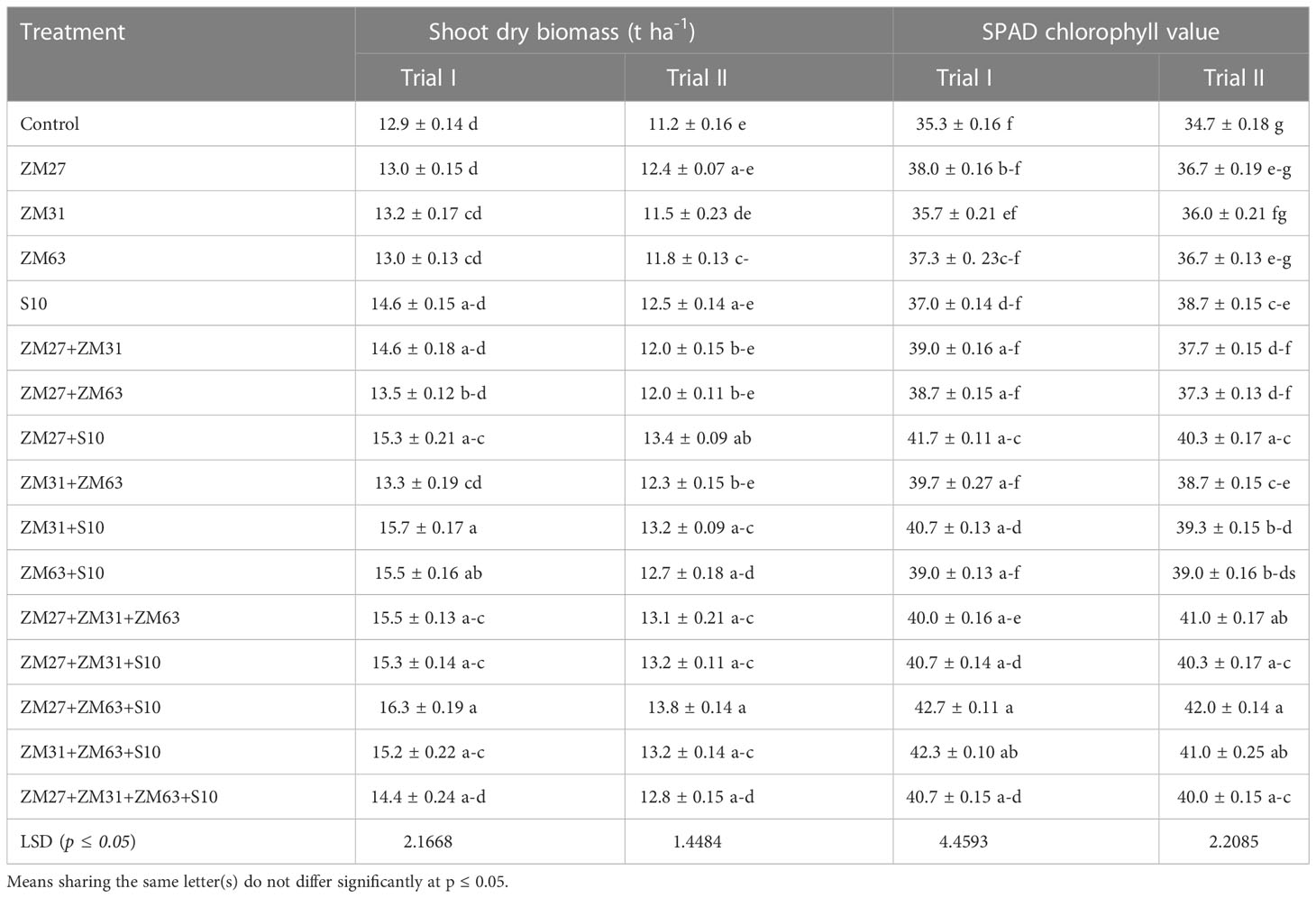
Table 4 Effect of novel bio inoculants on shoot dry biomass and SPAD value of maize in field trials.
3.2.2. Effect of PGPR on physiology and yield of maize
Results regarding the SPAD value of maize showed a significant increment under all application methods of novel PGPR strains (Table 4). The maximum increase in SPAD value under sole application was caused by S10 which was 12% higher than the control treatment in field trial II. However, the co-inoculation of ZM27+S10 showed a maximum increase in SPAD contents by 18% in field trial I which was at par with the consortium application of ZM27+ZM31+ZM63 in trial II. The consortium application caused the maximum increase in SPAD value under the M27+ZM63+S10 application which was 21% more than the un-inoculated control in both field trials.
Sole, co, and consortium PGPR strains caused significant improvement in the maize grain quality in terms of the number of grains cob-1 and 1000 grain weight (Table 5). The results indicated that sole inoculation of bacterial strains ZM27 in field trial I and S10 in field trial II caused a 2% increase in the number of grains cob-1 as compared to the control. Whereas S10 showed a 4% increase in 1000 grain weight followed by ZM63 with a 3% increase as compared to the control. The co-inoculation and consortia application indicated significantly higher results than sole inoculation. Co-inoculation of ZM27+S10 in both field trials and consortia treatment of ZM27+ZM63+S10 in trial II increased the number of grains cob-1 by 4 and 6%, respectively. Besides the individual use of PGPR, co-inoculation of ZM27+S10 showed 8% and consortia application of compatible bacterial strains (ZM27+ZM63+S10) caused a 12% increase in 1000 grain weight of maize. The results regarding grain yield depicted that the use of PGPR strains improved the grain yield of maize crop under field conditions (Table 6). A maximum increment in grain yield was observed in the consortium (ZM27+ZM63+S10) applied treatment, which was 17% more as compared to the un-inoculated control. However, co-inoculation of ZM27+S10 showed a 13% increase in grain yield, as compared to control in trial II.
3.2.3. Effect of PGPR inoculation on mineral contents of maize
Results about the impact of PGPR strains by different application methods on nitrogen content in grains are presented in Table 7 which showed a significant increase as compared to the un-inoculated control. The consortium of ZM27+ZM63+S10 strains has caused the highest increase of 13% in nitrogen content in grains of maize under both field conditions. However, the consortium application also has caused the highest increase in the case of phosphorus and potassium contents (Table 7). The highest increase in phosphorus and nitrogen content of maize grain was found at 12 and 10%, respectively, under consortium (ZM27+ZM63+S10) treatment as compared to the un-inoculated control treatment.
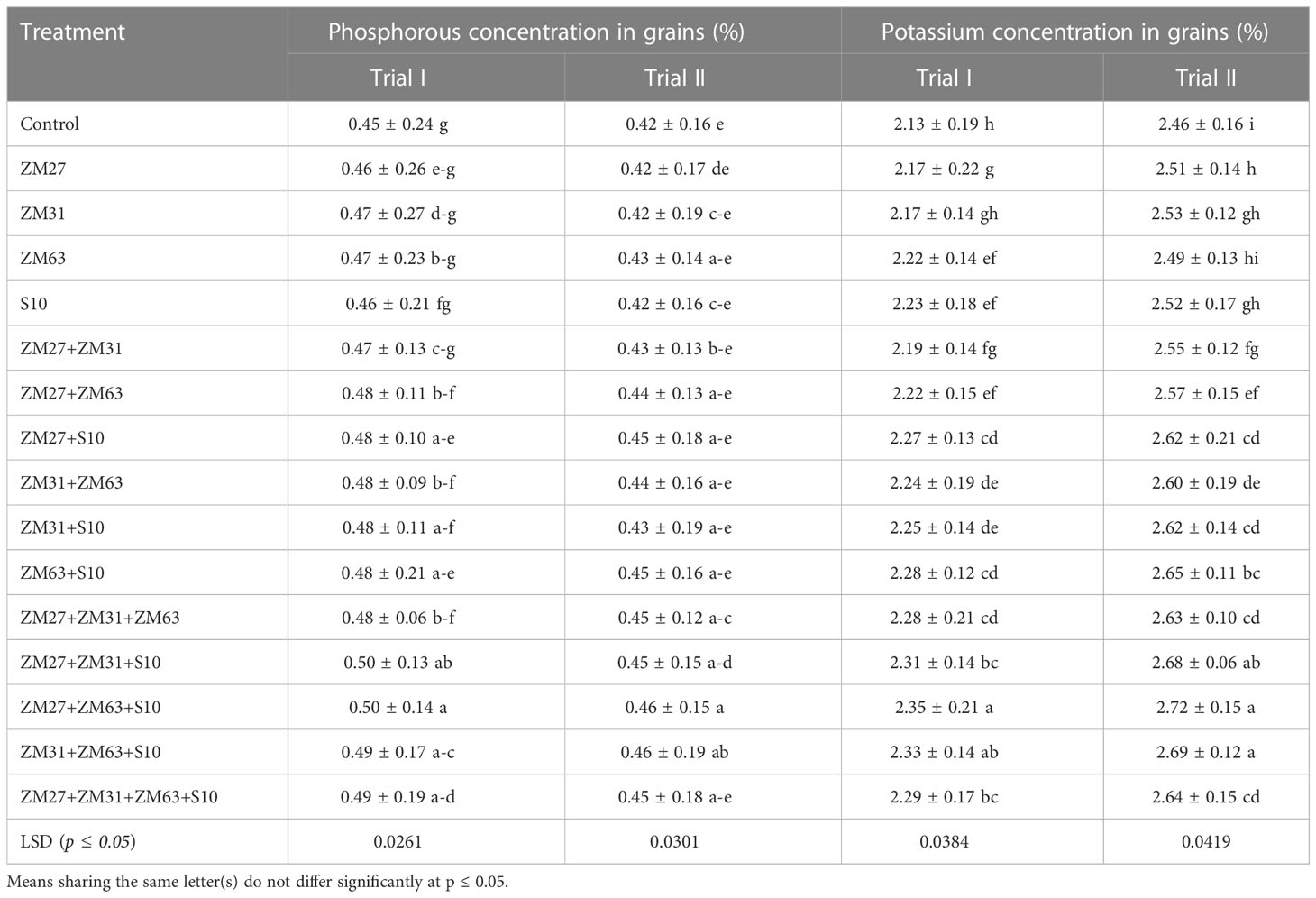
Table 7 Effect of novel bio inoculants on phosphorous and potassium concentration in the shoot of maize in field trials.
Results regarding the effect of different PGPR inoculation methods on micronutrient biofortification in maize grain depicted a significant increase in the uptake and biofortification of Fe and Zn in maize grain presented in Figures 4, 5, respectively. The co-inoculation of ZM27+S10 and ZM63+S10 showed a significant increase of 7% in Fe contents as compared to the control treatment. Moreover, the consortium application (ZM27+ZM63+S10) has caused 11. 4% more Fe concentration in maize grain under field trial. Similarly, the consortium of bacterial strains (ZM27+ZM63+S10) has caused significant improvement in maize grain zinc content by 11.6 and 10.0% in field trials II and I, respectively, as compared to the un-inoculated control treatment.
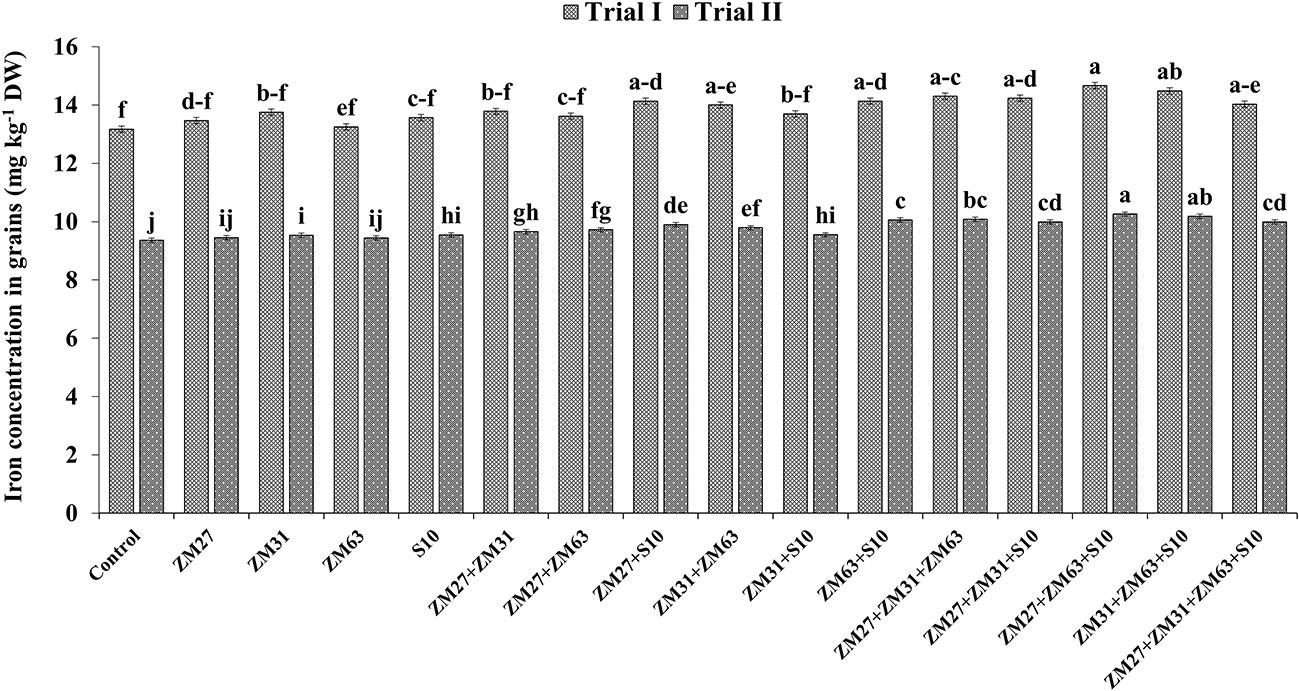
Figure 4 Effect of PGPR inoculation on grain iron contents in maize in the pot trial. The bars with different letters are significantly different at p ≤ 0.05.
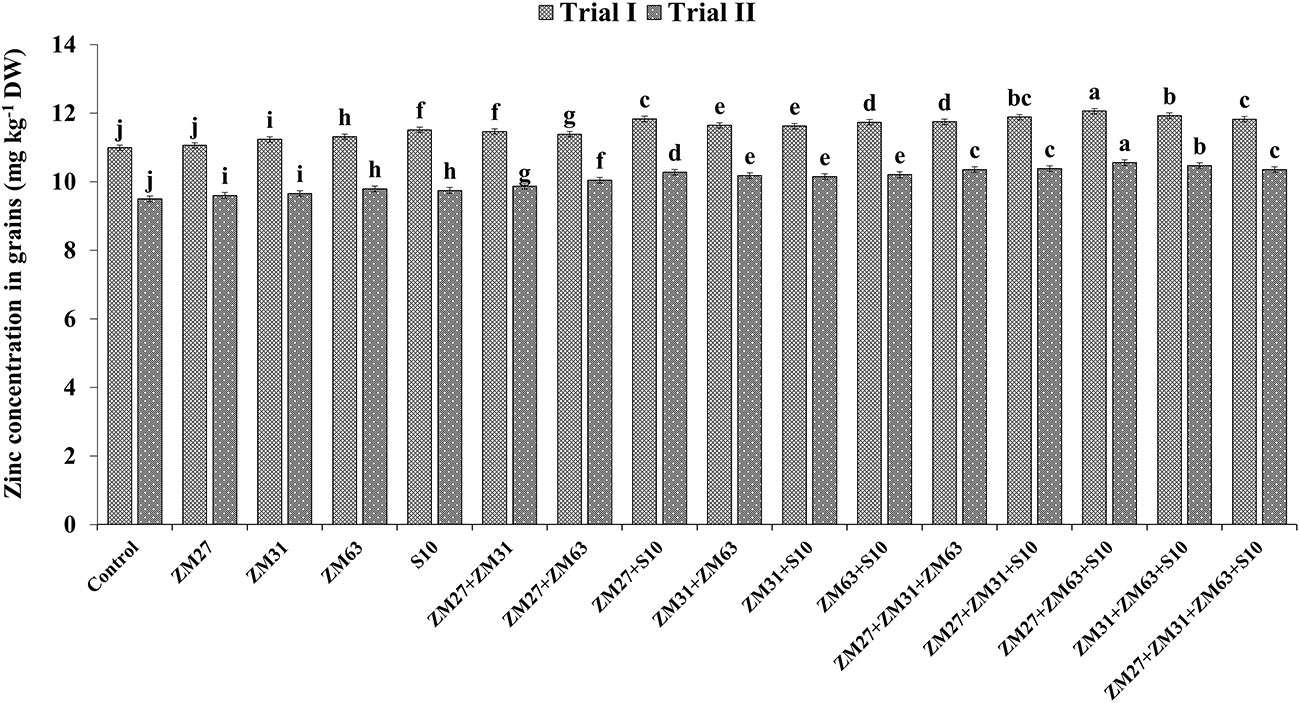
Figure 5 Effect of PGPR inoculation on grain zinc contents in maize in the pot trial. The bars with different letters are significantly different at p ≤ 0.05.
3.2.4. Effect of PGPR inoculation on rhizosphere microbial population of maize
The application of Zn solubilizing PGPR by different methods (sole, co, and consortium application) significantly increased the microbial population in the maize rhizosphere as compared to the un-inoculated control (Figure 6). The sole inoculation has caused the lowest increase (6.2 and 8.1%) in the microbial population in maize rhizosphere by ZM63 and S10, respectively, as compared to the uninoculated control in filed trial I. The co-inoculation of ZM27+S10 has caused the maximum increase in microbial population (15%) with respect to the control treatment. The maximum increment (23%) in the bacterial population under consortium application was caused by ZM27+ZM63+S10 in field trial I followed by ZM31+ZM63+S10 which showed a 21% increase in bacterial population over the control.
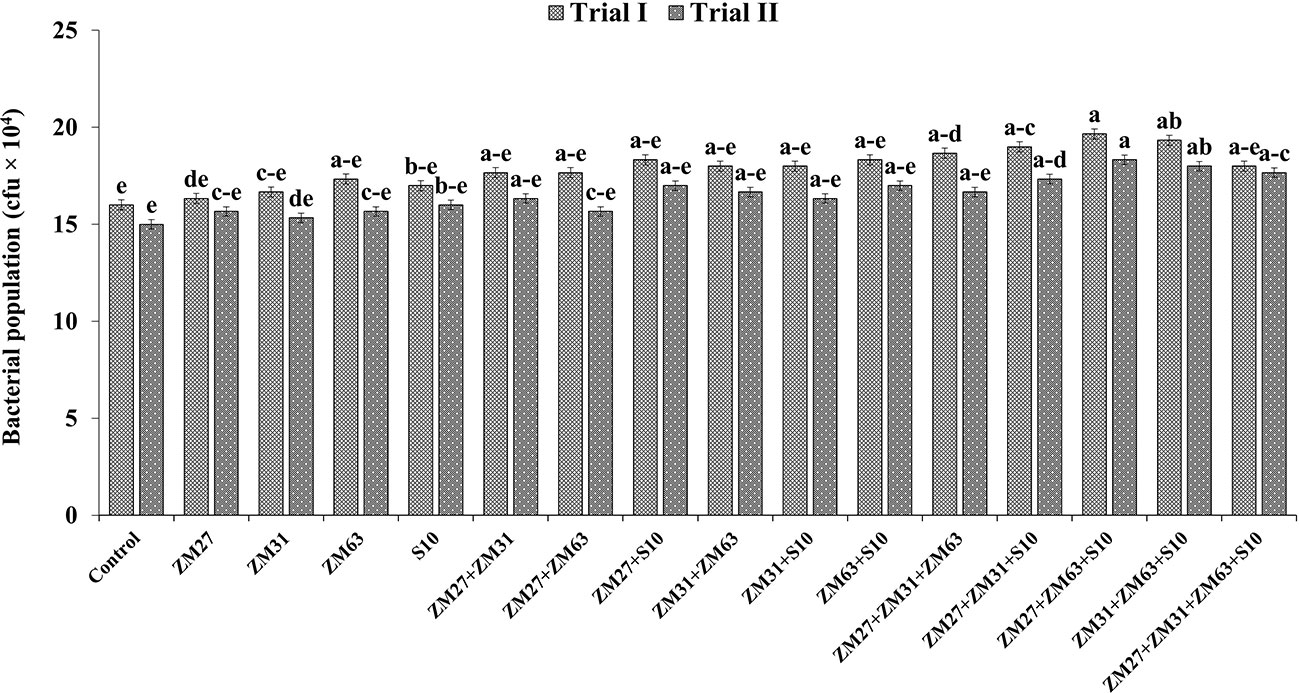
Figure 6 Effect of PGPR inoculation on the microbial count in maize rhizosphere in the field trials. The bars with different letters are significantly different at p ≤ 0.05.
4. Discussion
The current investigation revealed the role of Zn solubilizing PGPR and their sole, co, and consortium application on maize growth, yield, and micronutrients (Fe and Zn) biofortification. The results of the present study showed that all methods of biofertilizer application (sole, co, and consortium) increase the growth, yield, and nutrient status of the maize but the most promising results were obtained from the consortium (ZM31+ZM63+S10) application. The microbial inoculation of maize with PGPR strains i.e. Bacillus subtilis. ZM63, Bacillus aryabhattai. ZM31, Bacillus aryabhattai. S10 and Paenibacillus polymyxa. ZM27 has increased plant development, just as improving mineral nutrients in shoots and grains of maize. A positive relationship between plant biomass and nutrient content (N, P, Fe) was also recorded.
The role of microbial inoculants in enhancing maize growth and biofortification has already been described the microbial community does so through the degradation of organic matter and releasing entrapped nutrients which add up to the soil fertility status, and in turn, is responsible for improved plant growth (McDonagh et al., 1993; Phoomthaisong et al., 2003; Zou et al., 2019). The microorganisms solubilize the mineral nutrients by releasing organic acids into the rhizosphere and reducing the microsite pH suitable for micronutrients availability (Fe and Zn) and bio-fortify maize (Hussain et al., 2019; Merinero et al., 2022). Moreover, the increase in plant height, and shoot fresh and dry biomass of maize with inoculation of PGPR in the present study were at par with Khalid et al. (2004) and Pereira et al. (2020) and they conclude that the improvement was due to the growth hormones related substance produced by PGPR and better nutrient status of maize. The improvement in plant height, and shoot and root dry biomasses might be due to the enhanced nutrient uptake and partitioning especially higher nitrogen and phosphorus are responsible for growth and development at the early stage of the maize (Aquino et al., 2021). Pearson’s correlation of the growth parameters and nutrient contents of maize in pot trial and field is presented in supplementary data (Tables S2-S4) and also revealed that there was a positive correlation between the mineral nutrients uptake and maize growth parameters, which justifies the increased growth of maize attributed to the more mineral uptake.
Our previous investigations Ahmad et al. (2014); Mumtaz et al. (2017); Hussain et al. (2020), and Zahir et al. (2011) also advocated the role of the PGPR in enhancing the growth of different crops by enhancing the uptake of the entrapped nutrients through the release of organic acids. Moreover, various other studies also described the role of biofertilizers in improving the vegetative growth of different crops by enhancing nutrient uptake of crops and their partitioning among the different plant parts (Samavat et al., 2012), and biofortify micronutrients to cope with food insecurity and malnutrition (Ahmad et al., 2008; Farooq et al., 2009; Zafar-ul-Hye et al., 2013). Other growth-promoting traits such as auxin (IAA) production by these microbes is responsible for better root infrastructure development and proliferation in the soil, which is a possible reason for root biomass production in the present experiment (Zeb et al., 2018; Ahmad et al., 2019).
The increase in micronutrients (Fe and Zn) was significant under consortium application as compared to the sole and co-inoculation of the PGPR. The result of the present study is in line with Khan et al. (2019) for Zn biofortification while at par with Mumtaz et al. (2022) for Fe biofortification in maize grains. The higher uptake of the described micronutrients was due to the solubilization of these fixed micronutrients by the application of microorganisms, which are responsible for excreting acidic substances (organic acids) in the maize rhizosphere and lowering the microsite pH of soil. The lowering of pH is responsible for the solubilization of these micronutrients and their uptake in the maize plants and their fortification in maize grain (Sheikh et al., 2022). On the other hand, the microorganisms produce specified compounds like siderophores (Fe-loving compounds) which quench the Fe and the other micronutrients and made them available for plant use and reduce their uptake by pathogens (Singh and Prasanna, 2020). Therefore, the biofortification of Fe and Zn in the present investigation might be due to the production of siderophores by these bacterial strains.
The improvement in vegetative growth is responsible for enhancing crop production and higher outcomes from the same piece of land. The result regarding the grains per cob is slightly higher than Chen et al. (2022) which was due to the better growth of the maize due to optimal nutrition and biofertilizer solubilization of the fixed nutrients in the soil and their translocation to the reproductive organs of maize which is responsible for the increase in size and number of grains of the cobs (Amanullah et al., 2021; Inyang et al., 2021). The 1000-grain weight of the maize in the present experiment was at par with the Magar et al. (2021) which depicted that the grain weight improvement is linked with the optimal nutrition of the maize crop and its partitioning among the grains which results in an increased number of grains per cob and increased grain weight. A similar reason for the higher number of grains and thousand-grain weight has also been reported by many researchers (Naveed et al., 2008; Drury et al., 2017; Anwar et al., 2021; Drulis et al., 2022). The results regarding the yield of the present investigation are in line with the Mumtaz et al. (2022) which were due to the higher nutrient uptake, partitioning, and reproductive growth of maize (Al-Suhaibani et al., 2021; Singh et al., 2021; Ebrahimi Chamani et al., 2022). Pearson’s correlation analysis data between growth, nutrient contents, and yield parameters of maize under Field trials are presented in the supplementary data section as Tables S3, S4, which depicted a strong positive correlation between nutrients concentration, growth, and yield improvements in maize by application of these novel strains application under different methods.
5. Conclusions
It can be concluded that the sole, co, and consortium application of the PGPR significantly increases the growth, development, nutritional status, and yield of maize. The PGPR strains are responsible for solubilizing the essential nutrients in maize nitrogen, phosphorous, iron, zinc, and potassium while we compared them to the non-inoculated set of treatments. The results depicted that the application of the PGPR consortium (ZM27+ZM63+S10) the results was synergistic and caused a significant increase in shoot and root biomasses, nutrient status, and yield of maize when compared to un-inoculated control treatment. So, it is not wrong to say that the best consortium in the present study has the potential to be commercialized as a biofertilizer for biofortification (Fe and Zn) in wheat and sustainable production of maize.
Data availability statement
The original contributions presented in the study are included in the article/Supplementary Material. Further inquiries can be directed to the corresponding authors.
Author contributions
Conceptualization, MA and AH. Methodology, MA, ADa, and ML. Software, AH. Validation, AH, ADi, ADa and ML. Formal analysis, MA, ADi, ADa, ML, and ZI. Funding, AE-S. Investigation, MA, AH, ADa, ML, ZI, HA and FN. Resources, AH, ADa, ADi, AE-S, and ML. Data curation, ML and ZI. Writing—original draft preparation, MA. Writing—review and editing, ADa, ADi, AE-S, and ML. Visualization, AH, ZI, ADi, AE-S, and FN. Supervision, MA and AZ. All authors contributed to the article and approved the submitted version.
Funding
This research was funded by the Researchers Supporting Project No. (RSP2023R390), King Saud University, Riyadh, Saudi Arabia. The research is funded by the Endowment Fund Secretariat (EFS), Project Number (RSP-2020/180), University of Agriculture Faisalabad.
Acknowledgments
The authors extend their appreciation to Researchers Supporting Project number (RSP2023R390), King Saud University, Riyadh, Saudi Arabia.
Conflict of interest
The authors declare that the research was conducted in the absence of any commercial or financial relationships that could be construed as a potential conflict of interest.
Publisher’s note
All claims expressed in this article are solely those of the authors and do not necessarily represent those of their affiliated organizations, or those of the publisher, the editors and the reviewers. Any product that may be evaluated in this article, or claim that may be made by its manufacturer, is not guaranteed or endorsed by the publisher.
Supplementary material
The Supplementary Material for this article can be found online at: https://www.frontiersin.org/articles/10.3389/fpls.2022.1094551/full#supplementary-material
References
Abbas, S. (2022). Climate change and major crop production: Evidence from Pakistan. Environ. Sci. pollut. Res. 29 (4), 5406–5414. doi: 10.1007/s11356-021-16041-4
Ahmad, I., Kamran, M., Meng, X., Ali, S., Bilegjargal, B., Cai, T., et al. (2019). Effects of plant growth regulators on seed filling, endogenous hormone contents and maize production in semiarid regions. J. Plant Growth Regul. 38 (4), 1467–1480. doi: 10.1007/s00344-019-09949-2
Ahmad, M., Zahir, Z. A., Jamil, M., Nazli, F., Latif, M., Akhtar, M. F. (2014). Integrated use of plant growth-promoting rhizobacteria, biogas slurry, and chemical nitrogen for sustainable production of maize under salt-affected conditions. Pakistan J. Bot. 46, 375–382.
Ahmad, R., Arshad, M., Khalid, A., Zahir, Z. A. (2008). Effectiveness of organic-/bio-fertilizer supplemented with chemical fertilizers for improving soil water retention, aggregate stability, growth, and nutrient uptake of maize (Zea mays l.). J. Sustain. Agric. 31, 57–77. doi: 10.1300/J064v31n04_05
Alexander, M. (1982). “Most probable number method for the microbial population,” in 0-ccc method of soil analysis. part 2. agronomy no. 9. Ed. Page, A. L. (USA: American Society of Agronomy, Madison, WI), 815–820.
Al-Suhaibani, N., Selim, M., Alderfasi, A., El-Hendawy, S. (2021). Integrated application of composted agricultural wastes, chemical fertilizers and biofertilizers as an avenue to promote growth, yield and quality of maize in an arid agro-ecosystem. Sustainability 13 (13), 7439. doi: 10.3390/su13137439
Amanullah, Khalid, S., Muhammad, A., Yar, M., Ahmad, M., Akram, H. M., et al. (2021). Integrated use of biofertlizers with organic and inorganic phosphorus sources improve dry matter partitioning and yield of hybrid maize. Commun. Soil Sci. Plant Anal. 52 (21), 2732–2747. doi: 10.1080/00103624.2021.1956520
Anwar, Z., Basharat, Z., Hafeez, M. B., Khan, S., Zahra, N., Rafique, Z., et al. (2021). Biofortification of maize with zinc and iron not only enhances crop growth but also improves grain quality. Asian J. Agric. Biol. 64 (202102079), 10–35495. doi: 10.35495/ajab.2021.02.079
Aquino, J. P. A., Antunes, J. E. L., Bonifácio, A., Rocha, S. M. B., Amorim, M. R., Alcântara Neto, F., et al. (2021). Plant growth-promoting bacteria improve growth and nitrogen metabolism in maize and sorghum. Theor. Exp. Plant Physiol. 33 (3), 249–260. doi: 10.1007/s40626-021-00209-x
Arain, G. N. (2013). Maiz (corn) cultivation in pakistan. Agronomy.Center pivot irrigation system (Pakistan: Valley Irrigation Pakistan (private), limited).
Ayub, M., Ashraf, M. Y., Kausar, A., Saleem, S., Anwar, S., Altay, V., et al. (2021). Growth and physio-biochemical responses of maize (Zea mays l.) to drought and heat stresses. Plant Biosystems-An Int. J. Dealing all Aspects Plant Biol. 155 (3), 535–542. doi: 10.1080/11263504.2020.1762785
Bashir, S., Basit, A., Abbas, R. N., Naeem, S., Bashir, S., Ahmed, N., et al. (2021). Combined application of zinc-lysine chelate and zinc-solubilizing bacteria improves yield and grain biofortification of maize (Zea mays l.). PloS One 16 (7), e0254647. doi: 10.1371/journal.pone.0254647
Bouis, H. E., Chassy, B. M., Ochanda, O. (2003). Genetically modified food crops and their contribution to human nutrition and food quality. Trends Food Sci. Tech. 14, 191–209. doi: 10.1016/S0924-2244(03)00073-6
Cakmak, I., Pfeiffer, W. H., Clafferty, B. M. (2010). Biofortification of durum wheat with zinc and iron. Cereal Chem. 87, 10–20. doi: 10.1094/CCHEM-87-1-0010
Chen, X., Liu, P., Zhao, B., Zhang, J., Ren, B., Li, Z., et al. (2022). Root physiological adaptations that enhance the grain yield and nutrient use efficiency of maize (Zea mays l) and their dependency on phosphorus placement depth. Field Crops Res. 276, 108378. doi: 10.1016/j.fcr.2021.108378
Dar, A., Zahir, Z. A., Asghar, H. N., Ahmad, R. (2020). Preliminary screening of rhizobacteria for biocontrol of little seed canary grass (Phalaris minor retz.) and wild oat (Avena fatua l.) in wheat. Can. J. Microbiol. 66 (5), 368–376. doi: 10.1139/cjm-2019-0427
de Santiago, A., Quintero, J. M., Aviles, M., Delgado, A. (2011). Effect of Trichoderma asperellum strain T34 on iron, copper, manganese, and zinc uptake by wheat grown on a calcareous medium. Plant Soil 342, 97–104. doi: 10.1007/s11104-010-0670-1
Ditta, A., Ullah, N., Imtiaz, M., Li, X., Jan, A. U., Mehmood, S., et al. (2022). “Zn biofortification in crops through zn-solubilizing plant growth promoting rhizobacteria,” in Sustainable plant nutrition under contaminated environments. Ed. Mahmood, Q. (Switzerland: Springer Nature Switzerland AG).
Drulis, P., Kriaučiūnienė, Z., Liakas, V. (2022). The influence of different nitrogen fertilizer rates, urease inhibitors and biological preparations on maize grain yield and yield structure elements. Agronomy 12 (3), 741. doi: 10.3390/agronomy12030741
Drury, C. F., Yang, X., Reynolds, W. D., Calder, W., Oloya, T. O., Woodley, A. L. (2017). Combining urease and nitrification inhibitors with incorporation reduces ammonia and nitrous oxide emissions and increases corn yields. J. Environ. Qual. 46 (5), 939–949. doi: 10.2134/jeq2017.03.0106
Ebrahimi Chamani, H., Fallah Amoli, H., Niknejad, Y., Barari Tari, D. (2022). Effects of zeolite and biofertilizers on yield components, yield and nutrients uptake in grains of two corn cultivars (cv. 6010 and ns71). J. Plant Nutr. 45 (11), 1670–1681. doi: 10.1080/01904167.2021.2014876
Farooq, M., Wahid, A., Kobayashi, N., Fujita, D., Basra, S. M. A. (2009). Plant drought stress: effects, mechanisms and management. Agron. Sustain. Dev. 29 (1), 185–212. doi: 10.1051/agro:2008021
Fatima, Z., Zia, M., Chaudhary, M. F. (2007). Interactive effect of rhizobium strains and p on soybean yield, nitrogen fixation and soil fertility. Pak. J. Bot. 39 (1), 255–264.
Galindo, F. S., Bellotte, J. L. M., Santini, J. M. K., Buzetti, S., Rosa, P. A. L., Jalal, A., et al. (2021). Zinc use efficiency of maize-wheat cropping after inoculation with azospirillum brasilense. Nutrient Cycling Agroecosystems 120 (2), 205–221. doi: 10.1007/s10705-021-10149-2
Gentili, F., Huss-Danell, K. (2003). Local and systemic effects of phosphorus and nitrogen on nodulation and nodule function in alnus incana. J. Exp. Bot. 54, 2757–2767. doi: 10.1093/jxb/erg311
Glick, B. R. (1995). The enhancement of plant growth by free-living bacteria. Can. J. Microbiol. 41, 109–117. doi: 10.1139/m95-015
Gopalakrishnan, S., Vadlamudi, S., Samineni, S., Kumar, C. V. S. (2016). Plant growth-promotion and biofortification of chickpea and pigeonpea through inoculation of biocontrol potential bacteria, isolated from organic soils. Springerplus 5, 1882. doi: 10.1186/s40064-016-3590-6
Hussain, A., Ahmad, M., Nafees, M., Iqbal, Z., Luqman, M., Jamil, M., et al. (2020). Plant-growth-promoting bacillus and paenibacillus species improve the nutritional status of triticum aestivum l. PloS One 15 (12), e0241130. doi: 10.1371/journal.pone.0241130
Hussain, A., Arshad, M., Zahir, Z. A., Asghar, M. (2015). Prospects of zinc solubilizing bacteria for enhancing growth of maize. Pak. J. Agric. Sci. 52 (4), 915–922.
Hussain, A., Zahir, Z. A., Ditta, A., Tahir, M. U., Ahmad, M., Mumtaz, M. Z., et al. (2019). Production and implication of bio-activated organic fertilizer enriched with zinc-solubilizing bacteria to boost up maize (Zea mays l.) production and biofortification under two cropping seasons. Agronomy 10 (1), 39. doi: 10.3390/agronomy10010039
Inyang, P., Emmanuel, A., Chukwudi, U. P., Ikeogu, U. N. (2021). Environmental impact and genetic expressions of new drought tolerant maize genotypes in derived savannah agro-ecology. Notulae Scientia Biologicae 13 (1), 10691–10691. doi: 10.15835/nsb13110691
Jalal, A., da Silva Oliveira, C. E., Freitas, L. A., Galindo, F. S., Lima, B. H., Boleta, E. H. M., et al. (2022). Agronomic biofortification and productivity of wheat with soil zinc and diazotrophic bacteria in tropical savannah. Crop Pasture Sci. 11, 959. doi: 10.1071/CP21457
Jalal, A., Galindo, F. S., Boleta, E. H. M., Oliveira, C. E. D. S., Reis, A. R. D., Nogueira, T. A. R., et al. (2021). Common bean yield and zinc use efficiency in association with diazotrophic bacteria co-inoculations. Agronomy 11 (5), 959. doi: 10.3390/agronomy11050959
Khalid, A., Arshad, M., Zahir, Z. A. (2004). Screening plant growth-promoting rhizobacteria for improving growth and yield of wheat. J. Appl. Microbiol. 96, 473–480. doi: 10.1046/j.1365-2672.2003.02161.x
Khalid, S., Asghar, H. N., Akhtar, M. J., Aslam, A., Zahir, Z. A. (2015). Biofortification of iron in chickpea by plant growth promoting rhizobacteria. Pak. J. Bot. 47 (3), 1191–1194.
Khan, A., Singh, J., Upadhayay, V. K., Singh, A. V., Shah, S. (2019). “Microbial biofortification: a green technology through plant growth promoting microorganisms,” in Sustainable green technologies for environmental management (Singapore: Springer), 255–269.
Magar, B. T., Acharya, S., Gyawali, B., Timilsena, K., Upadhayaya, J., Shrestha, J. (2021). Genetic variability and trait association in maize (Zea mays l.) varieties for growth and yield traits. Heliyon 7 (9), e07939. doi: 10.1016/j.heliyon.2021.e07939
Majeed, A., Rashid, I., Niaz, A., Ditta, A., Sameen, A., Al-Huqail, A. A., et al. (2022). Balanced use of zn, Cu, fe, and b improves the yield and sucrose contents of sugarcane juice cultivated in sandy clay loam soil. Agronomy 12 (3), 696. doi: 10.3390/agronomy12030696
McDonagh, J. F., Toomsan, B., Limpinuntana, V., Giller, K. E. (1993). Estimates of the residual nitrogen benefit of groundnut to maize in northeast Thailand. Plant Soil 154 (2), 267–277. doi: 10.1007/BF00012532
Merinero, M., Alcudia, A., Begines, B., Martínez, G., Martín-Valero, M. J., Pérez-Romero, J. A., et al. (2022). Assessing the biofortification of wheat plants by combining a plant growth-promoting rhizobacterium (PGPR) and polymeric fe-nanoparticles: Allies or enemies? Agronomy 12 (1), 228. doi: 10.3390/agronomy12010228
Mishra, B. N., Prasad, R., Gangaiah, B. (2004). Organic manures for increased rice productivity and sustained supply of fe to rice. Acta Agron. Hungarica 52, 371–397. doi: 10.1556/AAgr.52.2004.4.8
Montogomery, D. C. (2013). Design and analysis of experiments. 8th Ed (Newyork USA: John Wiley and Sons Inc), 98–100.
Mumtaz, M. Z., Ahmad, M., Jamil, M., Hussain, T. (2017). Zinc solubilizing bacillus spp. potential candidates for biofortification in maize. Microbiol. Res. 202, 51–60. doi: 10.1016/j.micres.2017.06.001
Mumtaz, M. Z., Ahmad, M., Zafar-ul-Hye, M., Saqib, M., Akhtar, M. F. U. Z., Zaheer, M. S. (2022). Seed-applied zinc-solubilising bacillus biofertilisers improve antioxidant enzyme activities, crop productivity, and biofortification of maize. Crop Pasture Sci. 73 (5), 503–514. doi: 10.1071/CP21415
Najm-ul-Sehar, Ahmad, M., Akhtar, M. F., Jamil, M., Latif, M., Ahmad, I. (2015). Pesticide tolerant plant growth promoting rhizobacteria isolated from rhizosphere of okra. Soil Environ. 34, 111–118.
Naveed, M., Khalid, M., Jones, D. L., Ahmad, R., Zahir, Z. A. (2008). Relative efficacy of pseudomonas spp., containing ACC-deaminase for improving growth and yield of maize (Zea mays l.) in the presence of organic fertilizer. Pakistan J. Bot. 40 (3), 1243–1251.
Nuttall, J. G., O’leary, G. J., Panozzo, J. F., Walker, C. K., Barlow, K. M., Fitzgerald, G. J. (2017). Models of grain quality in wheat–a review. Field Crops Res. 202, 136–145. doi: 10.1016/j.fcr.2015.12.011
Pereira, S. I. A., Abreu, D., Moreira, H., Vega, A., Castro, P. M. L. (2020). Plant growth-promoting rhizobacteria (PGPR) improve the growth and nutrient use efficiency in maize (Zea mays l.) under water deficit conditions. Heliyon 6 (10), e05106. doi: 10.1016/j.heliyon.2020.e05106
Phoomthaisong, J., Toomsan, B., Limpinuntana, V., Cadisch, G., Patanothai, A. (2003). Attributes affecting residual benefits of N2-fixing mungbean and groundnut cultivars. Biol. fertility soils 39 (1), 16–24. doi: 10.1007/s00374-003-0676-4
Pingali, P. L., Heisey, P. W. (2001). Cereal-crop productivity in developing countries: past trends and future prospects. Agricultural science policy: Changing global agendas, pp.99–03.
Rana, A., Joshi, M., Prasann, R., Shivay, Y. S., Nain, L. (2012). Biofortification of wheat through inoculation of plant growth promoting rhizobacteria and cyanobacteria. Eur. J. Soil Biol. 50, 118–126. doi: 10.1016/j.ejsobi.2012.01.005
Ryan, J., Estefan, G., Rashid, A. (2001). Soil and plant analysis laboratory manual. 2nd Ed (Aleppo, Syria: International Center for Agriculture in Dry Areas (ICARDA), 172.
Samavat, S., Samavat, S., Mafakheri, S., Shakouri, M. J. (2012). Promoting common bean growth and nitrogen fixation by the co-inoculation of rhizobium and pseudomonas fluorescens isolates. Bulgarian J. Agric. Sci. 18 (3), 387–395.
Shah, Z., Shah, S. H., Peoples, M. B., Schwenke, G. D., Hrridge, D. F. (2003). Crop residue and fertilizer n effects on nitrogen fixation and yields of legume-cereal rotations and soil organic fertility. Field Crops Res. 83, 1–11. doi: 10.1016/S0378-4290(03)00005-4
Shahane, A. A., Shivay, Y. S. (2022). Agronomic biofortification of crops: Current research status and future needs. Indian J. Fertilisers 18 (2), 164–179.
Sharma, A., Patni, B., Shankhdhar, D., Shankhdhar, S. C. (2013). Zinc – an indispensable micronutrient. Physiol. Mol. Biol. Plants 19, 11–20. doi: 10.1007/s12298-012-0139-1
Sheikh, A. A., Javed, H., Rafique, A. (2022). Effect of biofertilizers (Fe and zn) on the growth of agronomic crops. Tobacco Regul. Sci. (TRS) 8, 538–549. doi: 10.18001/TRS.8.1.47
Shivay, Y. S., Prasad, R., Rahal, A. (2010). Studies on some nutritional quality parameters of organically or conventionally grown wheat. Cereal Res. Commun. 38, 345–352. doi: 10.1556/CRC.38.2010.3.5
Singh, D., Prasanna, R. (2020). Potential of microbes in the biofortification of zn and fe in dietary food grains. a review. Agron. Sustain. Dev. 40 (2), 1–21. doi: 10.1007/s13593-020-00619-2
Singh, S., Singh, H., Kumar, P., Singh, V., Kumar, S., Singh, R. (2021). Effect of NPK levels with bio-fertilizers on productivity of maize (Zea mays l.). IJCS 9 (1), 1476–1479. doi: 10.22271/chemi.2021.v9.i1u.11431
Soil Survey Staff (2006). “Soil taxonomy: A basic system of soil classification for making and interpreting soil surveys. soil conservation service,” in Department of agriculture handbook, 3rd edition (U.S: Natural Resources Conservation Service), 436.
Steel, R. G. D., Torrie, J. H., Dicky, D. A. (1997). Principles and procedures of statistics: A biometrical approach. 3rd ed (Singapore: McGraw-Hill Book International Co).
Steven, F. J. (1991). “Organic matter-micronutrient reactions in soil,” in Micronutrient in agriculture, soil science society of America. Eds. Mortvedt, J. J., Cox, F. R., Shuman, L. M., Welch, R. M. (Madison, WI, USA: Soil Science Society of America, Inc.), 145–186.
Tawfiq, S. I., Ahmad, K. R. (2014). The role of intercropping wheat with legumes (Chickpea or pea) in improving the yield and land equivalent ratio in rain fed regions. J. Zonkoy Sulaimani- Part A 16, 33–45. doi: 10.17656/jzs.10308
White, P. J., Broadley, M. R. (2005). Biofortifying crops with essential mineral elements. Trends Plant Sci. 10, 583–586. doi: 10.1016/j.tplants.2005.10.001
Wolf, B. (1982). A comprehensive system of leaf analyses and its use for diagnosing crop nutrient status. Commun. Soil Sci. Plant Anal. 13 (12), 1035–1059. doi: 10.1080/00103628209367332
Zafar-ul-Hye, M., Ahmad, M., Shahzad, S. M. (2013). Short communication synergistic effect of rhizobia and plant growth promoting rhizobacteria on the growth and nodulation of lentil seedlings under axenic conditions. Soil Environ. 32 (1), 79–86.
Zahir, Z. A., Ahmad, M., Hilger, T. H., Dar, A., Malik, S. R., Abbas, G., et al. (2018). Field evaluation of multi-strain biofertilizer for improving the productivity of different mungbean genotypes. Soil Environ. 37 (1), 45–52. doi: 10.25252/SE/18/61488
Zahir, Z. A., Zafar-ul-Hye, M., Sajjad, S., Naveed, M. (2011). Comparative effectiveness of pseudomonas and serratia sp. containing ACC-deaminase for coinoculation with rhizobium leguminosarum to improve growth, nodulation, and yield of lentil. Biol. Fertility Soils 47 (4), 457–465. doi: 10.1007/s00374-011-0551-7
Zeb, H., Hussain, A., Naveed, M., Ditta, A., Ahmad, S., Jamshaid, M. U., et al. (2018). Compost enriched with ZnO and zn-solubilizing bacteria improves yield and zn-fortification in flooded rice. Ital. J. Agron. 13 (4), 310–316. doi: 10.4081/ija.2018.1295
Zebrath, B., Karemangingo, C., Scott, P., Savoie, D., Brown, W. (2009). Nitrogen management for cereal crops: General fertilizer recommendations (Canada: New Brunswick Soil and CropImprovement Association).
Zou, C., Du, Y., Rashid, A., Ram, H., Savasli, E., Pieterse, P. J., et al. (2019). Simultaneous biofortification of wheat with zinc, iodine, selenium, and iron through foliar treatment of a micronutrient cocktail in six countries. J. Agric. Food Chem. 67 (29), 8096–8106. doi: 10.1021/acs.jafc.9b01829
Keywords: food security, undernourished, vitamins, biofortification, PGPR
Citation: Ahmad M, Hussain A, Dar A, Luqman M, Ditta A, Iqbal Z, Ahmad HT, Nazli F, Soufan W, Almutairi K and Sabagh AE (2023) Combating iron and zinc malnutrition through mineral biofortification in maize through plant growth promoting Bacillus and Paenibacillus species. Front. Plant Sci. 13:1094551. doi: 10.3389/fpls.2022.1094551
Received: 10 November 2022; Accepted: 19 December 2022;
Published: 01 February 2023.
Edited by:
Raul Antonio Sperotto, Universidade do Vale do Taquari - Univates, BrazilReviewed by:
Arshad Jalal, São Paulo State University, BrazilSukamal Sarkar, Ramakrishna Mission Vivekananda Educational and Research Institute, India
Sancar Bulut, Kayseri University, Turkey
Copyright © 2023 Ahmad, Hussain, Dar, Luqman, Ditta, Iqbal, Ahmad, Nazli, Soufan, Almutairi and Sabagh. This is an open-access article distributed under the terms of the Creative Commons Attribution License (CC BY). The use, distribution or reproduction in other forums is permitted, provided the original author(s) and the copyright owner(s) are credited and that the original publication in this journal is cited, in accordance with accepted academic practice. No use, distribution or reproduction is permitted which does not comply with these terms.
*Correspondence: Azhar Hussain, YXpoYXJoYXNlZW5AZ21haWwuY29t; Allah Ditta, YWxsYWguZGl0dGFAc2JidS5lZHUucGs7; YWxsYWguZGl0dGFAdXdhLmVkdS5hdQ==; Walid Soufan, d3NvdWZhbkBrc3UuZWR1LnNh