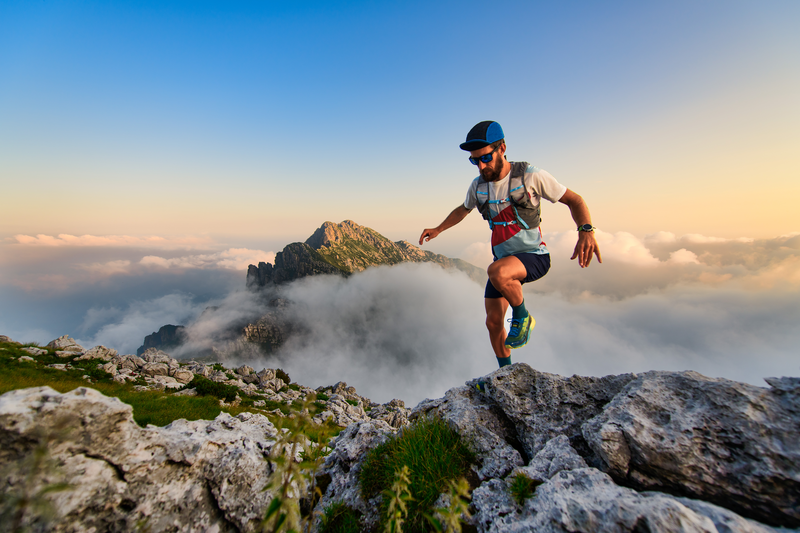
94% of researchers rate our articles as excellent or good
Learn more about the work of our research integrity team to safeguard the quality of each article we publish.
Find out more
ORIGINAL RESEARCH article
Front. Plant Sci. , 22 December 2022
Sec. Technical Advances in Plant Science
Volume 13 - 2022 | https://doi.org/10.3389/fpls.2022.1092857
This article is part of the Research Topic Advancements in plant omics for tackling biotic and abiotic stresses View all 6 articles
Lonicera japonica is not only an important resource of traditional Chinese medicine, but also has very high horticultural value. Studies have been performed on the physiological responses of L. japonica leaves to chilling, however, the molecular mechanism underlying the low temperature-induced leaves morphological changes remains unclear. In this study, it has been demonstrated that the ratio of pigments content including anthocyanins, chlorophylls, and carotenoids was significantly altered in response to chilling condition, resulting in the color transformation of leaves from green to purple. Transcriptomic analysis showed there were 10,329 differentially expressed genes (DEGs) co-expressed during chilling stress. DEGs were mainly mapped to secondary metabolism, cell wall, and minor carbohydrate. The upregulated genes (UGs) were mainly enriched in protein metabolism, transport, and signaling, while UGs in secondary metabolism were mainly involved in phenylpropaoids-flavonoids pathway (PFP) and carotenoids pathway (CP). Protein-protein interaction analysis illustrated that 21 interacted genes including CAX3, NHX2, ACA8, and ACA9 were enriched in calcium transport/potassium ion transport. BR biosynthesis pathway related genes and BR insensitive (BRI) were collectively induced by chilling stress. Furthermore, the expression of genes involved in anthocyanins and CPs as well as the content of chlorogenic acid (CGA) and luteoloside were increased in leaves of L. japonica under stress. Taken together, these results indicate that the activation of PFP and CP in leaves of L. japonica under chilling stress, largely attributed to the elevation of calcium homeostasis and stimulation of BR signaling, which then regulated the PFP/CP related transcription factors.
Lonicera japonica, which is native to East Asia, is prized in China, Korea, and Japan for its pharmacological actions and horticultural value (Miller and Gorchov, 2004). The flowers of L. japonica are used in traditional Chinese medicine, while the stems and leaves are used in Japanese medicine (Shang et al., 2011). As an ornamental plant resource, L. japonica has more and more popular properties such as its sprawling habit, numerous sweetly white and golden flowers, and attractive evergreen foliage. Now L. japonica has been naturalized in Argentina, Brazil, Mexico, Australia, New Zealand and United States (Guo et al., 2023). A pharmacological study has reported that L. japonica is used as an herbal medicine with anti-bacterial, anti-endotoxin, anti-inflammatory, and antipyretic effects (Li et al., 2015). In the recent years, L. japonica has become one of the key materials in traditional Chinese medicine with antiepidemic effect (Zhou et al., 2020; Wang et al., 2021). There are many chemical components in L. japonica such as flavonoids, organic acids, volatile oils, iridoids, triterpenoids, and saponins. The content of CGA and luteoloside are the main indicators for the quality assessment of L. japonica (Wang et al., 2020). Previous studies have addressed at the point of revealing the biosynthesis and regulation mechanism of secondary metabolites during the floral development of L. japonica (Fang et al., 2020; Xia et al., 2021). A systemic study by the integration of transcriptome, proteome, and metabolome revealed the transduction mechanism of phenylpropanoids and terpenoids biosynthesis in the stages of flower development (Wang et al., 2019b). However, although the endogenous hormones-regulated color transition of petals has been indicated by a transcriptomic analysis (Xia et al., 2021), studies on improving its horticultural and ornamental value is very limited.
As a plant resource with dual properties of medicinal and ornamental effects, it would be a meaningful exploration to borrow environmental conditions to promote the accumulation of active components of L. japonica and affect the external phenotype of its leaves or flowers. Recently, a study showed that light intensity had a significant effect on the flavonoids accumulation in the flower buds of L. japonica (Fang et al., 2020). Cold stress is one of the major abiotic stresses which limit the growth and yield of crops worldwide (Ke et al., 2020). It includes chilling (0°C–15°C) as well as freezing (< 0°C) stress (Ding et al., 2020). Growing evidences suggest that cold stress not only causes alteration in physiological and biochemical parameters (phenotype, photosynthesis, and/or antioxidant enzyme activities) of plants (Zareei et al., 2021) but also affects their metabolic pathways (Li et al., 2018). Peng et al. (2019) found that the expression of genes related to flavonol biosynthesis as well as flavonol content were increased in Tetrastigma hemsleyanum under chilling stress. Artemisinin biosynthetic pathway was also activated by chilling stress in Artemisia annua (Vashisth et al., 2018). Interestingly, the flavonoids and saponins content were more accumulated in the root of Tetrastigma hemsleyanum (Xiang et al., 2021) and Panax notoginseng (Xia et al., 2017), respectively, when the transformation occurred from Summer to Autumn. While Carpenter et al. (2014) not only demonstrated the reddening of L. japonica leaves, but also revealed the relationship between the photoprotective function of anthocyanin and leaves reddening. Cold stress induces plant response through activating signal pathways including mitogen-activated protein kinases (MAPKs), phytohormone, and oxidative pathway (Yuan et al., 2018). Ca2+ has been known to play critical role in cold stress response of plants (Cui et al., 2020). The triggered Ca2+ signals were relayed by Ca2+ and decoded into downstream signaling pathways like activation of MAPKs and the production of ROS (Reddy et al., 2011). Nevertheless, it is still not clear how Ca2+-mediated signaling interacts with other signaling pathways to regulate the accumulation of relevant secondary metabolites under cold stress in plants.
In our previous experiments, an interesting phenomenon was observed that the leaves color of L. japonica was changed into purple when plants were transferred to a chilling environment. Preliminary experiment demonstrated that content proportion of three pigment components (anthocyanins, chlorophylls, and carotenoids) was significantly changed under the chilling stress (Figure 1). The accumulation of anthocyanins was suggested to be key factor for the formation of fiber color in Gossypium hirsutum (Gao et al., 2019; Ke et al., 2022). Here, in order to understand the inherent regulation mechanism of the phenotypic response of L. japonica leaves to chilling stress, transcriptome sequencing combined with bioinformatic analysis were performed. Phytochemical and qRT-PCR analyses were carried out for confirmation of transcriptomic results.
Figure 1 Morphological observation and pigment content of the leaves of L. japonica. (A) Phenotypic changes of leaves under chilling stress; (B) Pigments content analysis; (C) 3D coordinate map. The location of spheres in the maps represents the ratio of contents of chlorophylls, carotenoids, and anthocyanins in leaves of L. japonica. Means with the same letter were not significantly different among samples while different letters indicate that the change is significant according to one-way ANOVA test (p< 0.05).
The seedings of L. japonica (Beihua No.1) were transplanted from the nursery garden in Linyi (35°18′28″N, 117°34′45″E) into potted containers and placed in a greenhouse in Zhejiang Sci-Tech University in Hangzhou (30°18′54″N, 120°21′27″E). The stem segments were cut to 5 cm in length, planted in pots with nutrient soil, and grown in an incubator (Ningbo Southeast Instrument, Zhejiang, China) with the condition of humidity of 70%, light intensity of 8,000 Lux, and temperature of 24°C in a 12 h-light/12 h-dark cycle per day. The seedlings with 8 leaves were used for chilling treatment in this study. In the treatment group, 15 seedlings were stressed with a temperature of 10°C for 30 days; in the control group, another 15 seedlings were grown at the normal temperature of 24°C for 30 days. Leaves of L. japonica at starting point (SP), treatment for 15 days (T15), and treatment for 30 days (T30) were collected for physiological and transcriptomic analyses. Three independent experiments were performed as biological replicates and the collected plant materials were frozen in liquid nitrogen and stored at −80°C.
Chlorophylls and carotenoids determination was carried out according to the method described by Luo et al. (2019) with minor modifications. Chlorophylls and carotenoids were extracted from 50 mg of leaves in 5 mL of dimethyl sulfoxide, after incubation at 65°C for 20 min (until the leaves turned white). The extraction solution was measured at 470 nm, 649 nm, and 665 nm using the UV-1800PC spectrophotometer (MAPADA, Shanghai, China). Content of chlorophylls (CT) and carotenoids (Cc) were calculated using the following equations (“V” represents the final volume of the reaction and “m” represents the mass of leaves used for metabolites extraction):
Anthocyanins were extracted and determined using the method of Wang et al. (2019a) with little modification. Briefly, 50 mg of frozen leaves was grounded into powder in liquid nitrogen, sonicated with 3 ml of 0.1% methanol hydrochloride for 1 h, and then shaken overnight. After centrifugation at 2,500 g for 10 min, 1 ml of the supernatant was mixed with 1 ml of water and the mixture was further mixed with 1ml of chloroform to remove chlorophyll. The resulted solution was measured at 530 nm for anthocyanin determination.
Total RNA was extracted from L. japonica leaves using an RNA extraction kit (Accurate Biotechnology, Hunan, China). The integrity of RNA was evaluated by gel-electrophoresis and the concentration and purity were determined by a NanoDrop spectrophotometer 1000 (Thermo Fisher, MA, USA). The mRNA was isolated and fragmented using the U-mRNAseq Library Prep Kit (Illumina, CA, USA). The mRNA fragments were reverse transcribed into double-stranded cDNA using Smart-RT Enzyme (Takara, Japan) and then purified with magnetic beads to repair the end of short fragments by adding a poly (A) tail and the sequencing connector. The cDNA from each group of three individuals (one per biological replicate) was pooled to build a sequencing library, which was purified using gel electrophoresis and quantitatively assayed by real-time PCR, respectively. The libraries were then sequenced by Illumina Novaseq 6000 (Illumina).
Raw Data was filtered using fastp software (https://github.com/OpenGene/fastp). The adaptor, sequences with fragment length< 50 bp, reads with a certain percentage of N bases (set to 5bp by default), and low-quality bases with quality values< 20 were removed to obtain clean data. Clean data were compared to the reference genomes of L. japonica (Pu et al., 2020) and Arabidopsis for similarity using hisat2 (https://daehwankimlab.github.io/hisat2/). The value of fragments per kilobase of exon million fragments mapped (FPKM) was used to represent the expression level of genes. The differential expression of genes was cognized under the criterion that a significant change of gene expression between samples is identified as the fold change of FPKM value above 1 with p< 0.05.
The function predication of genes derived from L. japonica was performed by transferring annotations to the Arabidopsis genome and consideration of orthologous genes. Gene functions were categorized using Mercator 4 (https://plabipd.de/portal/mercator4) (Lohse et al., 2014). Pathway mapping of identified genes was performed using MapMan software (http://gabi.rzpd.de/projects/MapMan/) (Thimm et al., 2004) and the Kyoto Encyclopedia of Genes and Genomes (KEGG) database (http://www.genome.jp/kegg/) (Kanehisa and Goto, 2000). A hierarchical clustering analysis was generated to show the fold change ratios of genes. The cluster analysis was performed using the K-Means in MeV (Multiple Experiment Viewer) (https://sourceforge.net/projects/mev-tm4/files/mev-tm4/).
Protein-protein interactions (PPI) were generated by exporting the orthologous gene IDs of Arabidopsis to STRING (Search Tool for the Retrieval of Interacting Genes, v9.1) (https://string-db.org/). The network was displayed using cytoscape 3.9.1 (https://cytoscape.org/).
The transcriptomic sequences of L. japonica and those which were obtained from NCBI were used for phylogenetic analysis. Mafft v7.464 was employed for multiple sequence alignment (Katoh and Standley, 2013) and the alignment results were used to reconstruct the phylogenetic tree by MEGA-X software (Kumar et al., 2018) with the method of Neighbor-Joining. The neighbor-joining tree was tested with 1000 bootstrap replicates.
CGA and luteoloside were extracted from frozen-dried leaves of L. japonica through ultra-sonication with 2 mL methanol for 60 min. After centrifugation at 12,000 g for 10 min, the supernatant was aspirated with a syringe and passed through 0.22 μm membrane filters (Jinteng, Tianjin, China). The supernatant was analyzed by high performance liquid chromatography (HPLC) analysis which were carried out on a Waters Alliance 2695 separation module with a 2998 photodiode array detector (Waters, MA, USA) and a Reversed-Phase 18 column (4.6mm × 250mm, 5 μm) (Agilent, CA, USA). The mobile phases were water with 0.1% phosphoric acid (A) and acetonitrile (B) with the flow rate of 1 mL/min. The injection volume is 10 μl, the column temperature was 30°C, and the detection wavelengths for CGA and luteoloside were 327 nm and 350 nm respectively. The gradient elution method was as follows: 0–2 min (12% B); 2–12 min (12%–20% B); 12–22 min (20% B); 22–47 min (20%–30% B).
To validate the accuracy of the gene expression obtained from the RNA-Seq analysis, 22 genes associated with metal ion mediated signal transduction were selected for qRT-PCR. Primer 5.0 software was used to design primers (Supplemental Table 1) and qRT-PCR were conducted using ABI7500 fluorescence quantitative PCR instrument (Applied Biosystems, CA, USA). The SYBR® Green Pro Taq HS qPCR Kit (Accurate Biotechnology Co., Ltd, Hunan, China) was used and three biological replicates were performed of each group sample. Relative expression levels were calculated based on the 2− ΔΔCt method (Livak and Schmittgen, 2001) using actin as the house-keeping gene (Cai et al., 2022).
The SPSS statistical software (version 22.0; IBM, Armonk, NY, USA) was used for statistical evaluation. Statistical significance was evaluated by the Student’s t-test when only two groups were compared or one-way ANOVA followed by Tukey’s test when multiple groups were compared. A p-value< 0.05 was considered as the statistical significance. Three independent biological replicates per sample were tested in this study.
In this study, the L. japonica plants were grown in a 10°C environment for 30 days. The leaves morphology had been monitored during the chilling phase. As it was identified that the color of leaves was changed into purple from green when the plants were treated with chilling for 15 days, and the purple color spread to the whole leaves when it reached 30 days (Figure 1A). The total chlorophylls, carotenoids, and anthocyanins were measured by using a spectrophotometric method (Figure 1B). The content of total chlorophylls was not significantly changed when the seedlings grown in both control and chilling treatment conditions. However, the content of carotenoids was dramatically increased in response to the chilling treatment that it was 84%-increase in leaves treated for 30 days compared with SP. Interestingly, the anthocyanins oppositely changed in the control and treatment groups. During the growth process, the content of anthocyanins slightly decreased under the control condition, however, it gradually increased under the chilling condition. The ratio of total chloropylls, carotenoids, and anthocyanins were calculated and located in the 3-dimensional diagram. Figure 1C shows that the locations of ratios at treatment for 15 days and 30 days were significantly changed.
To study the response mechanism of L. japonica to chilling treatment, leaves of L. japonica in the treatment group were collected for transcriptomic analysis. A total of 21,628, 21,651, and 21,442 genes were identified in leaves of L. japonica at SP, T15, and T30, respectively (Figure 2). During the identified genes, 20,417 genes were co-expressed among the three groups and 10,329 genes were differentially expressed under chilling stress. In Figure 2, it was shown that there were 8,293 and 8,211 genes that differentially expressed in response to T15 and T30. There were 3,185 genes differentially expressed when L. japonica were under chilling stress from 15 days to 30 days. Furthermore, the expression of 1,430 genes were significantly changed in response to both T15 and T30.
Figure 2 Venn diagram of transcriptomic data. (A) Venn diagram of totally identified genes; (B) Venn diagram of DEGs under chilling stress. The number on the arrow represents the DEGs among the co-identified genes in three samples.
To illustrate the effect of chilling stress on L. japonica, DEGs in L. japonica under chilling treatment for 30 days were functionally categorized using MapMan software (Figure 3). The mapped DEGs were mainly enriched in secondary metabolism, lipids, cell wall, and minor carbohydrate. The secondary metabolism was further dissected in Supplemental Figures 1 and 2. Most of the DEGs were involved in phenlypropanoids, flavonoids, and lignin/lignans. Very interestingly, genes related to non MVA pathway and sulfur containing metabolism were decreased in response to chilling stress, however, genes related to shikimate, chalcones, and isoflavonoids pathways were almost upregulated. It was further realized that genes in betains, simple phenols, and carotenoids were slightly upregulated in response to the stress. Anthocyanins, dihydroflavonols, flavonols related genes were differentially induced that they were upregulated and downregulated under the chilling stress. In the tetrapyrrole metabolism, although two genes involved in biosynthesis of chlorophyll a was upregulated, however, obviously, genes involved in biosynthesis of chlorophyll b and chlorphyllide a were downregulated in response to the chilling stress (Supplemental Figure 3).
Figure 3 MapMan analysis of DEGs in L. japonica under chilling treatment for 30 days. The genes expression was compared between SP and T30. The Log2FC values of the gene expression were used for MapMan visualization using MapMan software to obtain an overview of the affected metabolic processes. Each colored square indicates the Log2FC value of a differentially changed gene. Red, blue, and white colors indicate an increase, decrease, and no change in the Log2FC value of gene expression.
In order to explore the response mechanism of L. japonica to chilling stress, DEGs were clustered based on their temporal expression profiles (Figure 4). Twelve clusters (C1-C12) which represented the different expression trends during the treatment process were generated by the K-means algorithm. Genes in clusters C1-C3 and C12 were downregulated at T15 while upregulated at T30. Conversely, genes in C5-C6 and C8-C9 were upregulated at T15 and downregulated at T30. Genes in C4 and C7 were gradually downregulated at T15 and T30. In C10-C11, genes were gradually upregulated at T15 and T30. Notablely, the expression of genes in C8-C11 were significantly higher at T15 and T30 than that at SP. Genes in clusters C8-C11 were further functionally analyzed. A total of 2,230 and 1,905 genes in C8-C9 and C10-C11, respectively, were homologously annotated by Arabidopsis genome and the function was categorized using MapMan bin codes (Figure 5). Both groups of genes were mainly enriched in protein metabolism, RNA, transport, signaling, cell metabolism, lipid metabolism, secondary metabolism, and amino acid metabolism. The number of genes enriched in nucleotide metabolism, cell wall, glycolysis, mitochondrial electron transport and TCA pathways was bigger in C8-C9 than in C10-C11.
Figure 4 Cluster analysis of DEGs. DEGs were clustered and displayed in line chart using Mev. Twelve clusters were grouped. X-axis represents the time points during chilling stress including SP, T15, and T30. Y-axis represents normalized value of the DEGs expression level. The middle black line in each cluster is the zero line and the red line indicates the average expression level. The number in the upper left corner represents the number of DEGs in each cluster.
Figure 5 Functional category of DEGs in cluster 8 (C8), C9, C10, and C11. Gene function was predicted and categorized using MapMan bin codes. CFVM, Co-factor and vitamine metabolism; MET, mitochondrial electron transport; Others: Biodegradation of Xenobiotics, C1-metabolism, fermentation, major carbohydrate metabolism, metal handling, minor carbohydrate metabolism, oxidative pentose phosphate, polyamine metabolism, photosynthesis, S-assimilation, tetrapyrrole synthesis, and transporter.
Figure 5 shows that most DEGs in L. japonica under chilling stress were involved in transport and signaling processes. In order to further reveal the response mechanism of L. japonica to chilling treatment, DEGs related to transport and signaling processes in group C10-C11 were selected for protein-protein interaction analysis. In Table 1, 15 and 13 DEGs function as the metabolite transporters at the mitochondrial membrance and ABC transporters/multidrug resistance system, respectively, belonging to transport process, while there are 22 and 20 DEGs function as receptor kinases and G-proteins, respectively, belonging to signaling process. PPI analysis told that 21 genes were more connected with other genes based on their interaction in the database (Figure 6A). By using the local network cluster method, 17 clusters were produced with a false discovery rate below 0.05. The top three enrichment functions are transition metal ion transport (CL:27896 and CL:27898) and calcium transport/potassium ion transport (CL:28292) (Figure 6B). There are 6 genes including NCL, tonoplast Na+/H+ antiporter 2 (NHX2), NCRK, ACA9, CAX3, autoinhibited Ca (2+) ATPases 8 (ACA8) gathered in cluster of CL:28292. Interestingly, four of the six genes (NCL, ACA9, CAX3, and ACA8) concurrently work as sodium or calcium exchanger protein/cation transporting ATPase/C-terminus.
Figure 6 PPI analysis of DEGs related to signaling and transport in C10-C11. (A) Network of protein-protein interaction (PPI). The circles represent the proteins and the lines represent the interactions of two proteins. The size and color of circles indicate the degree value represented by the number of the interacting proteins. The circles with a high degree value (above 4) which was represented by the number of interacting proteins were pained by red color. (B) Bubble map of functional enrichment of genes. CL:27896, Mixed, incl. transition metal ion transport, and heavy metal-associated domain superfamily; CL:27898, Mixed, incl. transition metal ion transport, and heavy metal-associated domain superfamily; CL:28292, Calcium transport, and potassium ion transport; CL:14557, Mixed, incl. citrate transporter, and tlc atp/adp transporter; CL:14560, Citrate transporter, and plasma membrane pyruvate transport; CL:28494, Mixed, incl. anion transmembrane transporter activity, and lateral organ boundaries, lob; CL:14397, Mixed, incl. chloroplast membrane, and mitochondrial carrier protein; CL:28295, Sodium/calcium exchanger protein, and Cation transporting ATPase, C-terminus; CL:28140, Mixed, incl. abc transporter, cbio/ecfa subunit, and ferric-chelate reductase activity; CL:27899, Mixed, incl. transition metal ion transmembrane transporter activity, and heavy metal-associated domain superfamily; CL:27939, Mixed, incl. iron transport, and nickel transport; CL:3064, Mixed, incl. gtp-binding protein enga, and endoplasmic reticulum vesicle transporter; CL:27901, Mixed, incl. zinc ion transmembrane transport, and iron ion homeostasis; CL:28496, Mixed, incl. nitrate assimilation, and malate transport; CL:27902, Mixed, incl. iron ion homeostasis, and nicotianamine synthase; CL:28498, Mixed, incl. response to nitrate, and molybdenum cofactor biosynthesis; CL:28297, Sodium/calcium exchanger protein, and Cation transporting ATPase, C-terminus.
To illustrate the response mechanism of secondary metabolism to chilling stress, secondary metabolism related genes in C8-C9 and C10-C11 were mapped to KEGG database. As it was shown in Figure 7, genes of PAL and 4CL which are related to CGA biosynthesis involved in phenylalanine metabolism were upregulated under chilling stress. In the α-tocopherol biosynthetic pathway, VTE1 and VTE3 were together upregulated in reponse to the stress. Furthermore, DXR, ISPF, GGPS, and GGPPS in the geranylgeranyl-PP biosynthetic pathway, with PDS, ZDS, LCYE, LCYB in the β-carotene biosynthetic pathway were significantly activated by the chilling stress. Another obvious cue is the activation of luteolin biosynthetic pathway based on the upregulation of CHS and CYP75B1. Moreover, genes of F3’H, DFR, LAR, ANS, ANR, and F3oGT which function in the synthetic process of anthocyanins were also remarkably induced by the chilling stress. Additionally, CCoAR and CAD involved in lignins biosynthetic pathway were found to be upregulated in response to chilling stress.
Figure 7 Pathway mapping of secondary metabolism related DEGs in Figure 5. Genes identification and expression were represented by boxes with different color. Metabolites were represented by hollow circles. 4CL, 4-coumarate: CoA ligase; ANR, anthocyanidin reductase; ANS, anthocyanidin synthase; CAD, cinnamol dehydrogenase; CCR, cinnamoyl CoA reductase; CHS, chalcone synthase; LCYB, lycopene beta cyclase; LCYE, lycopene epsilon cyclase; CHYB, β-carotene hydroxylase, F3’H, flavonoid 3’-hydroxylase; DFR, dihydroflavonol 4-reductase, DXR, 1-deoxy-D-xylulose 5-phosphate reductase; F3oGT, flavonol-3-O-glucosyl transferase; GGPPS, geranylgeranyl pyrophosphate synthase; GPPS, geranyl pyrophosphate synthase; ISPF, 2C-methyl-d-erythritol 2,4-cyclodiphosphate synthase; LAR, leucoanthocyantin reducase, PAL, phenylalanine ammonia lyase; PDS, phytoene desaturase; VTE1, tocopherol cyclase; VTE3, 2-methyl-6-phytyl-1,4-benzoquinol methyltransferase; ZDS, ζ-carotene dehydrogenase; DXP, 1-deoxy-D-xylulose 5-phosphate; MEcPP, 2-C-methyl-d-erythritol-2,4-cyclopyrophosphate; MEP, 2-C-methyl-D-erythrifol 4-phosphat; DMGGBQ, 6-geranylgeranyl-2,3-dimethylbenzene-1,4-diol, MGGBQ, 6-geranylgeranyl-2-methylbenzene-1,4-diol.
PPI analysis proved that DEGs with more connections in both categories of transport and signaling participate in calcium transport process. Pathway mapping of secondary metabolism related DEGs indicated the activation of biosynthetic pathways of CGA and luteoloside. CGA and luteoloside content were measured by HPLC to reveal the effect of chilling stress on secondary metabolism of L. japonica leaves. Figure 8 shows that CGA and luteoloside were both gradually accumulated from SP to T30. At T30, the content of two metabolites had a dramatic increase that they were 23 and 17 folds compared with those at SP.
Figure 8 The content of chlorogenic acid and luteoloside in samples. Leaves of L. japonica were collected at SP, T15, and T30. CGA and luteoloside were extracted by methanol and analyzed by HPLC. Data are shown as mean ± S.D. from three independent biological replicates. Different lowercase letters indicate statistically significant differences as measured by Tukey’s test (p< 0.05).
Two Neighbor-Joining phylogenetic trees were constructed using 8 CAX3 genes and 14 NHX2 genes, respectively, to explore the evolutionary relationship between L. japonica and other selected species. Based on the nucleotide sequences of CAX3 in the species, it is learned that L. japonica is relatively closed to Helianthus annuus, while they are evolutionarily distant to Emiliania huxleyi CAX3, Nicotiana attenuate CAX3, Cucumis sativus CAX3, and Arabidopsis thaliana CAX3, which are highly homologous (Figure 9A). However, a lower evolutionary relationship is found between L. japonica NHX2 and Helianthus annuus NHX2 than CAX3 (Figure 9B). Interestingly, it seems that the evolution of NHX2 genes is not conservative among these species.
Figure 9 Phylogenetic analyses of CAX3 and NHX2 in L. japonica and other species. Phylogenetic trees were generated using CAX3 of L. japonica with other 7 species (A), and NHX2 of L. japonica with other 13 species (B), respectively. The numbers at nodes in the phylogenetic tree indicate bootstrap values per 1000 replicates. The coding sequences were obtained from NCBI and the source of data is noted after species names.
Based on the protein interaction results in Figure 6, 22 genes potentially associated with metal ion-mediated signaling were screened for qRT-PCR (Supplemental Figure 4). The results showed that compared with SP, the expression of the 22 genes under chilling stress was significantly upregulated, which is consistent with the results of transcriptome analysis. In the brassinosteroids (BR) pathway, DWF4, FK, SQE1, SQE3, SMT1, DETS, and CAS1 were collectively induced to a higher transcription level by chilling stress (Figure 10). Particularly, the dramatic regulation of chilling stress was identified on the expression of DWF4 and DET2. Furthermore, as the receptor protein of BR, the transcription expression of BRI was also elevated under the chilling stress.
Figure 10 Expression analysis of BR related genes. The expression level of genes was represented by FPKM value. Data are represented as the mean ± S.D. from 3 independent biological replicates. Means with the " * " were significantly different among samples according to one-way ANOVA test ("*", p< 0.05; "**", p< 0.01; "***", p< 0.001). BIN, brassinosteroid-insensitive; CAS1, cycloartenol synthase 1; DLSR, delta14-sterol reductase; SMT1, sterol methyltransferase 1; SMT2, sterol methyltransferase 2; SQE1, squalene epoxidase1; SQE3, squalene epoxidase 3.
Low temperature is one of the common abiotic stresses affecting plant growth. Plants adapt to low temperatures by changing their morphology and adjusting the expression of a series of genes involved in complex networks (Lantzouni et al., 2020; Li et al., 2021a). As an interesting phenotype response to chilling stress, leaves color changes have been concerned in many previous studies (Ahmed et al., 2015). It is suggested that, in most plants, the accumulation and composition of three pigments including chlorophylls, carotenoids, and anthocyanins determine the external expression of leaves color (Markwell and Namuth, 2003). When Brassica campestris L. was under low temperature, the leaves color was changed from green to yellow might contribute to the regulation of the pigments by HY5 and its downstream genes (Yuan et al., 2021). It was proved that the leaves of Chinese cabbage turned purple under the low temperature stress with the increased accumulation of anthocyanin (Dai et al., 2022). In this study, the leaves of L. japonica were predictably changed into purple when under chilling condition. Here, our experiments not only suggested the acute accumulation of anthocyanins and carotenoids, but also proved that the ratio of total chloropylls, carotenoids, and anthocyanins was significantly changed in response to chilling stress. The upregulated genes (UGs) in response to chilling stress were functionally enriched and secondary metabolism related genes were well mapped to anthocyanins and carotenoids biosynthetic processes (Figure 7). Additionally, the pathway mapping of UGs and HPLC analysis proved that the chilling stress also increased the content of CGA and luteoloside (Figure 8). As the two compounds are suggested to be the index components for evaluating the quality of L. japonica, our study indicates that chilling stress has potential application in improving the medicinal quality and enhancing the ornamental value of L. japonica.
The comparative transcriptomic strategy was conducted to demonstrate the molecular mechanism underlying the morphological response of L. japonica to chilling stress. The UGs were functionally categorized by MapMan bin codes and interacted by PPI software. Transport and signaling related genes were very positively induced to respond to chilling stress (Figure 5). Further analysis demonstrated that the interacted genes function in transition metal ion transport (Figure 6). It has been proved that Ca2+ level could be rapidly induced and increased in cytoplasm by cold stress (Liu et al., 2021). In melatonin treated Arabidopsis, Ca2+ efflux was induced accompanied by an increase of CAX3, and the CAX3 deletion resulted in decreased Ca2+ efflux (Li et al., 2021b). In L. japonica, the transcript level of CAX3 was detected to significantly increased under chilling stress, might indicating the rapid accumulation of calcium content. However, it is unknown whether calcium channels are involved in temperature sensing and how the Ca2+ signal is induced and decoded in response to chilling stress in L. japonica. Plants employee a combination of ion pumps, antiporters, and uniporters to control cytoplasmic calcium dynamics (Sanders et al., 2002) including a family of calmodulin-activated Ca2+-ATPase ion pumps like autoinhibited Ca2+ ATPases (ACAs) for Ca2+ transporter (Schiøtt et al., 2004). ACA8 is suggested to be as a prominent regulator of Ca2+ dynamics (Bonza et al., 2000). NCX family members play important roles in mediating the Ca2+ homeostasis of plant under stress environment. In response to temperature decrease, NCX elevates intracellular Ca2+, which activates Ca2+/calmodulin-dependent protein kinase II and accelerates transcriptional oscillations of clock genes (Kon et al., 2021). Moreover, NHXs was reported to have a role in Na+ uptake mechanisms and transport pathway (Shavrukov, 2014), by which the plants could mediate Na+ uptake and compartmentation from/into the vacuole to mediate the homoeostasis under salt tolerance. It was documented that the stimulation of dynamic Ca2+ level on MHX for its proton signaling is a conserved regulation mechanism (Allman et al., 2013). In our study, whatever, the increased transcriptional level of identified CAX3, NCX, NHX2, and ACA8 indicated chilling stress led to a high level homoeostasis of Ca2+ through improvement of ion transporting in leaves of L. japonica.
Although the complex regulation network of plants responding to chilling stress has not been fully elucidated (Ding et al., 2020), the accumulating evidences were located at the iron transporter as a cold sensor to initiate multiple responses (Zhang et al., 2019). The spraying of calcium induced the activation of flavonoid metabolism in grape (Zhang et al., 2021). The homeostasis of Ca2+ across the plasma membrane is critical for coordination of the downstream responses, suggesting a mechanistic link between the receptor complex and signaling kinases via Ca2+ as the secondary messenger. In Arabidopsis, ACA8 interacts with brassinosteroid insensitive 1 (BRI1) to regulate plant physiology (Schwessinger et al., 2011). It is known that BRI1 is one of the key positive regulators of BR signaling (Clouse, 2011). Publications have shown that BR enhances stress tolerance and prevents cellular damage by abiotic environmental conditions (Bajguz and Hayat, 2009). Studies illustrated that BR signaling differentially affected plant flavonoid biosynthesis depending on the downstream regulators. BR accelerated the induced flavonoid accumulation by JA in Arabidopsis (Peng et al., 2011) and nitric oxide in Camellia sinensis L. (Li et al., 2017) through regulating the relative gene expression. Alternatively, BR signaling was found to inhibit the flavonoid biosynthesis through repressing the expression of MYB11, MYB12, and MYB111 by BR1-EMS-Suppressor 1 (Liang et al., 2020). In fact, it has been realized that studies are very limited on explore the complex and exact regulation mechanism of BR signaling on flavonoids biosynthesis, especially under an extreme temperature like chilling stress. As it was detected that one of the genes annotated as MYB111 was upregulated in leaves of L. japonica in response to chilling stress (Supplemental Table 2). Moreover, evidence supplied for an attached effect of BR signaling that the overexpression of BRI1 in tomato enhanced the endogenous BR signaling intensity and increased the carotenoids production (Nie et al., 2017). In this study, the KEGG enrichment of DEGs showed that the steroid hormone biosynthesis pathway exhibited very positive response to the cold stress. BRI, DWF4, DLSR, and SMT2 were all upregulated, which were responsible for the biosynthesis of BR in leaves of L. japonica when under the chilling condition. Additionally, most of the PFP and CP related genes being identified to be upregulated, and the accumulated content of CGA, luteoloside, anthocyanins, and carotenoids indicated the PFP and CP were significantly activated by the chilling stress. The results above indicate that Ca2+ signaling might promote the biosynthesis of flavonoids and carotenoids in the chilling treated leaves of L. japonica through inducing the accumulation of BR and activity the BR signaling regulators.
In this study, comparative transcriptomics was carried out to reveal the molecular mechanism underlying the physiological phenotype of L. japonica under chilling stress. The results are as follows: (1) the leaves color was changed from green to purple and the content of carotenoids and anthocyanins were increased under chilling stress; (2) DEGs were mainly enriched in secondary metabolism, lipids, cell wall, and minor carbohydrate; (3) the UGs were functionally categorized in protein metabolism, RNA, transport, signaling, and cell metabolism; (4) the interacted DEGs in transport and signaling processes were functioned in transition ion transport and calcium transport/potassium ion transport; (5) NCL, NHX2, NCRK, ACA9, CAX3, ACA8 involved in the regulation of calcium homoeostasis were upregulated and (6) BRI, DWF4, DLSR, and SMT2 involved in BR biosynthesis and signaling were all upregulated in response to chilling stress; (7) the accumulation of CGA and luteoloside was increased in leaves of L. japonica under chilling stress. The results guide to form the overview of response mechanism of L. japonica to chilling stress (Figure 11): the calcium concentration was increased and the calcium homeostasis was regulated to a high level under the effect of signaling and transporter related genes, thereby triggering BR signal activity, by which the biosynthesis of flavonoids and carotenoids were promoted through the induction of related transcription factors. The changed ratios of pigments and accumulation of CGA and luteoloside indicate that the experimental chilling condition could be developed to artificial strategy to promote both the medicinal quality and horticultural value of L. japonica.
The data presented in the study are deposited in the National Center for Biotechnology Information Sequence Read Archive repository, accession number PRJNA903538.
BY and LZ conceived and designed the study. MZ wrote the manuscript. ML performed the bioinfomatic analysis. MZ, KW and XT performed the material treatment and collection, library construction, and qRT-PCR. RQ performed the pigment content measurement. JL, SG, and HF performed the HPLC analysis of metabolites. ZZ did the data integration and function annotation. BY and LZ reviewed and edited the manuscript. All authors contributed to the article and approved the submitted version.
This work was supported by the Natural Science Foundation of Zhejiang Province (Grant No. LY21H280011), the Research Initiation Funding of Zhejiang Sci-Tech University (Grant No. 19042112-Y), the Natural Science Foundation of Jiangsu Province (No. BK20190234), and the National Support Funding for Reform and Development of Local Universities (Grant No. 303013-2021-0007). We also thank Shanghai Biotree Biotech CO., LTD for the important academic suggestions.
Author JL and SG is employed by Shandong Anran Nanometer Industry Development Company Limited.
The remaining authors declare that the research was conducted in the absence of any commercial or financial relationships that could be construed as a potential conflict of interest.
All claims expressed in this article are solely those of the authors and do not necessarily represent those of their affiliated organizations, or those of the publisher, the editors and the reviewers. Any product that may be evaluated in this article, or claim that may be made by its manufacturer, is not guaranteed or endorsed by the publisher.
The Supplementary Material for this article can be found online at: https://www.frontiersin.org/articles/10.3389/fpls.2022.1092857/full#supplementary-material
ACA9, autoinhibited Ca (2+) ATPases 9; BR, brassinosteroids; BRI, BR insensitive; BRI1, brassinosteroid insensitive 1; CGA, chlorogenic acid; CP, carotenoids pathway; DEGs, differentially expressed genes; NHX2, tonoplast Na+/H+ antiporter 2; PFP, phenylpropaoids-flavonoids pathway; PPI, protein-protein interactions; SP, starting point; T15, treatment for 15 days; T30, treatment for 30 days; UGs, upregulated genes.
Ahmed, N. U., Park, J. I., Jung, H. J., Hur, Y., Nou, I. S. (2015). Anthocyanin biosynthesis for cold and freezing stress tolerance and desirable color in Brassica rapa. Funct. Integr. Genomics 15, 383–394. doi: 10.1007/s10142-014-0427-7
Allman, E., Waters, K., Ackroyd, S., Nehrke, K. (2013). Analysis of Ca2+ signaling motifs that regulate proton signaling through the Na+/H+ exchanger NHX-7 during a rhythmic behavior in Caenorhabditis elegans. J. Biol. Chem. 288, 5886–5895. doi: 10.1074/jbc.M112.434852
Bajguz, A., Hayat, S. (2009). Effects of brassinosteroids on the plant responses to environmental stresses. Plant Physiol. Biochem. 47, 1–8. doi: 10.1016/j.plaphy.2008.10.002
Bonza, M. C., Morandini, P., Luoni, L., Geisler, M., Palmgren, M. G., De Michelis, M. I. (2000). At-ACA8 encodes a plasma membrane-localized calcium-ATPase of arabidopsis with a calmodulin-binding domain at the n terminus. Plant Physiol. 123, 1495–1506. doi: 10.1104/pp.123.4.1495
Cai, Z., Wang, C., Chen, C., Zou, L., Yin, S., Liu, S., et al. (2022). Comparative transcriptome analysis reveals variations of bioactive constituents in Lonicera japonica flowers under salt stress. Plant Physiol. Biochem. 173, 87–96. doi: 10.1016/j.plaphy.2022.01.022
Carpenter, K. L., Keidel, T. S., Pihl, M. C., Hughes, N. M. (2014). Support for a photoprotective function of winter leaf reddening in nitrogen-deficient individuals of Lonicera japonica. Molecules 19, 17810–17828. doi: 10.3390/molecules191117810
Clouse, S. D. (2011). Brassinosteroid signal transduction: from receptor kinase activation to transcriptional networks regulating plant development. Plant Cell. 23, 1219–1230. doi: 10.1105/tpc.111.084475
Cui, Y., Lu, S., Li, Z., Cheng, J., Hu, P., Zhu, T., et al. (2020). Cyclic nucleotide-gated ion channels 14 and 16 promote tolerance to geat and chilling in rice. Plant Physiol. 183, 1794–1808. doi: 10.1104/pp.20.00591
Dai, Y., Zhang, L., Sun, X., Li, F., Zhang, S., Zhang, H., et al. (2022). Transcriptome analysis reveals anthocyanin regulation in Chinese cabbage (Brassica rapa l.) at low temperatures. Sci. Rep. 12, 6308. doi: 10.1038/s41598-022-10106-1
Ding, Y., Shi, Y., Yang, S. (2020). Molecular regulation of plant responses to environmental temperatures. Mol. Plant 13, 544–564. doi: 10.1016/j.molp.2020.02.004
Fang, H., Qi, X., Li, Y., Yu, X., Xu, D., Liang, C., et al. (2020). De novo transcriptomic analysis of light-induced flavonoid pathway, transcription factors in the flower buds of Lonicera japonica. Trees (Berl West). 34, 267–283. doi: 10.1007/s00468-019-01916-4
Gao, J., Shen, L., Yuan, J., Zheng, H., Su, Q., Yang, W., et al. (2019). Functional analysis of GhCHS, GhANR and GhLAR in colored fiber formation of Gossypium hirsutum l. BMC Plant Biol. 19, 455. doi: 10.1186/s12870-019-2065-7
Guo, X. M., Ma, M. H., Ma, X. L., Zhao, J. J., Zhang, Y., Wang, X. C., et al. (2023). Quality assessment for the flower of Lonicera japonica thunb. during flowering period by integrating GC-MS, UHPLC-HRMS, and chemometrics. Ind. Crop Prod. 191, 115938. doi: 10.1016/j.indcrop.2022.115938
Kanehisa, M., Goto, S. (2000). KEGG: kyoto encyclopedia of genes and genomes. Nucleic Acids Res. 28, 27–30. doi: 10.1093/nar/28.1.27
Katoh, K., Standley, D. M. (2013). MAFFT multiple sequence alignment software version 7: improvements in performance and usability. Mol. Biol. Evol. 30, 772–780. doi: 10.1093/molbev/mst010
Ke, L., Lei, W., Yang, W., Wang, J., Gao, J., Cheng, J., et al. (2020). Genome-wide identification of cold responsive transcription factors in Brassica napus l. BMC Plant Biol. 20, 62. doi: 10.1186/s12870-020-2253-5
Ke, L., Yu, D., Zheng, H., Xu, Y., Wu, Y., Jiao, J., et al. (2022). Function deficiency of GhOMT1 causes anthocyanidins over-accumulation and diversifies fibre colours in cotton (Gossypium hirsutum). Plant Biotechnol. J. 20, 1546–1560. doi: 10.1111/pbi.13832
Kon, N., Wang, H. T., Kato, Y. S., Uemoto, K., Kawamoto, N., Kawasaki, K., et al. (2021). Na+/Ca2+ exchanger mediates cold Ca2+ signaling conserved for temperature-compensated circadian rhythms. Sci. Adv. 7, 8132. doi: 10.1126/sciadv.abe8132
Kumar, S., Stecher, G., Li, M., Knyaz, C., Tamura, K. (2018). MEGA X: Molecular evolutionary genetics analysis across computing platforms. Mol. Biol. Evol. 35, 1547–1549. doi: 10.1093/molbev/msy096
Lantzouni, O., Alkofer, A., Falter-Braun, P., Schwechheimer, C. (2020). Growth-regulating factors interact with dellas and regulate growth in cold stress. Plant Cell. 32, 1018–1034. doi: 10.1105/tpc.19.00784
Liang, T., Shi, C., Peng, Y., Tan, H., Xin, P., Yang, Y., et al. (2020). Brassinosteroid-activated BRI1-EMS-SUPPRESSOR 1 inhibits flavonoid biosynthesis and coordinates growth and UV-b stress responses in plants. Plant Cell. 32, 3224–3239. doi: 10.1105/tpc.20.00048
Li, Y., Cai, W., Weng, X., Li, Q., Wang, Y., Chen, Y., et al. (2015). Lonicerae japonicae flos and Lonicerae flos: a systematic pharmacology review. Evid Based Complement Alternat Med. 2015, 905063. doi: 10.1155/2015/905063
Li, H., Guo, Y., Lan, Z., Zhang, Z., Ahammed, G. J., Chang, J., et al. (2021b). Melatonin antagonizes ABA action to promote seed germination by regulating Ca (2+) efflux and H(2)O(2) accumulation. Plant Sci. 303, 110761. doi: 10.1016/j.plantsci.2020.110761
Li, F., Lu, X., Duan, P., Liang, Y., Cui, J. (2021a). Integrating transcriptome and metabolome analyses of the response to cold stress in pumpkin (Cucurbita maxima). PloS One 16, e0249108. doi: 10.1371/journal.pone.0249108
Liu, Q., Ding, Y., Shi, Y., Ma, L., Wang, Y., Song, C., et al. (2021). The calcium transporter ANNEXIN1 mediates cold-induced calcium signaling and freezing tolerance in plants. EMBO J. 40, e104559. doi: 10.15252/embj.2020104559
Livak, K. J., Schmittgen, T. D. (2001). Analysis of relative gene expression data using real-time quantitative PCR and the 2– ΔΔCT method. Methods 25, 402–408. doi: 10.1006/meth.2001.1262
Li, S., Yang, Y., Zhang, Q., Liu, N., Xu, Q., Hu, L. (2018). Differential physiological and metabolic response to low temperature in two zoysiagrass genotypes native to high and low latitude. PloS One 13, e0198885. doi: 10.1371/journal.pone.0198885
Li, X., Zhang, L., Ahammed, G. J., Li, Z. X., Wei, J. P., Shen, C., et al. (2017). Nitric oxide mediates brassinosteroid-induced flavonoid biosynthesis in Camellia sinensis l. J. Plant Physiol. 214, 145–151. doi: 10.1016/j.jplph.2017.04.005
Lohse, M., Nagel, A., Herter, T., May, P., Schroda, M., Zrenner, R., et al. (2014). Mercator: a fast and simple web server for genome scale functional annotation of plant sequence data. Plant Cell Environ. 37, 1250–1258. doi: 10.1111/pce.12231
Luo, F., Cheng, S. C., Cai, J. H., Wei, B. D., Zhou, X., Zhou, Q., et al. (2019). Chlorophyll degradation and carotenoid biosynthetic pathways: Gene expression and pigment content in broccoli during yellowing. Food Chem. 297, 124964. doi: 10.1016/j.foodchem.2019.124964
Markwell, J., Namuth, D. (2003). Plant pigments and photosynthesis. J. Nat. Res. Life Sci. Edu. 32, 137–137. doi: 10.2134/jnrlse.2003.0137a
Miller, K. E., Gorchov, D. L. (2004). The invasive shrub, Lonicera maackii, reduces growth and fecundity of perennial forest herbs. Oecologia 139, 359–375. doi: 10.1007/s00442-004-1518-2
Nie, S., Huang, S., Wang, S., Cheng, D., Liu, J., Lv, S., et al. (2017). Enhancing brassinosteroid signaling via overexpression of tomato (Solanum lycopersicum) SlBRI1 improves major agronomic traits. Front. Plant Sci. 8, 1386. doi: 10.3389/fpls.2017.01386
Peng, Z., Han, C., Yuan, L., Zhang, K., Huang, H., Ren, C. (2011). Brassinosteroid enhances jasmonate-induced anthocyanin accumulation in arabidopsis seedlings. J. Integr. Plant Biol. 53, 632–640. doi: 10.1111/j.1744-7909.2011.01042.x
Peng, X., Wu, H., Chen, H., Zhang, Y., Qiu, D., Zhang, Z. (2019). Transcriptome profiling reveals candidate flavonol-related genes of Tetrastigma hemsleyanum under cold stress. BMC Genomics 20, 687. doi: 10.1186/s12864-019-6045-y
Pu, X., Li, Z., Tian, Y., Gao, R., Hao, L., Hu, Y., et al. (2020). The honeysuckle genome provides insight into the molecular mechanism of carotenoid metabolism underlying dynamic flower coloration. New Phytol. 227, 930–943. doi: 10.1111/nph.16552
Reddy, A. S., Ali, G. S., Celesnik, H., Day, I. S. (2011). Coping with stresses: roles of calcium- and calcium/calmodulin-regulated gene expression. Plant Cell. 23, 2010–2032. doi: 10.1105/tpc.111.084988
Sanders, D., Pelloux, J., Brownlee, C., Harper, J. F. (2002). Calcium at the crossroads of signaling. Plant Cell. 14, 401–417. doi: 10.1105/tpc.002899
Schiøtt, M., Romanowsky, S. M., Baekgaard, L., Jakobsen, M. K., Palmgren, M. G., Harper, J. F. (2004). A plant plasma membrane Ca2+ pump is required for normal pollen tube growth and fertilization. Proc. Natl. Acad. Sci. U.S.A. 101, 9502–9507. doi: 10.1073/pnas.0401542101
Schwessinger, B., Roux, M., Kadota, Y., Ntoukakis, V., Sklenar, J., Jones, A., et al. (2011). Phosphorylation-dependent differential regulation of plant growth, cell death, and innate immunity by the regulatory receptor-like kinase BAK1. PloS Genet. 7, e1002046. doi: 10.1371/journal.pgen.1002046
Shang, X., Pan, H., Li, M., Miao, X., Ding, H. (2011). Lonicera japonica thunb.: ethnopharmacology, phytochemistry and pharmacology of an important traditional Chinese medicine. J. Ethnopharmacol. 138, 1–21. doi: 10.1016/j.jep.2011.08.016
Shavrukov, Y. (2014). “Vacuolar h+-PPase (HVP) genes in barley: Chromosome location, sequence and gene expression relating to na+ exclusion and salinity tolerance,” in Barley: physical properties, genetic factors and environmental impacts on growth. Ed. Hasunuma, K. (Nova Science Publishers, Inc), 125–141. https://novapublishers.com/wp-content/uploads/2019/05/978-1-62948-904-9_ch6.pdf
Thimm, O., Bläsing, O., Gibon, Y., Nagel, A., Meyer, S., Krüger, P., et al. (2004). MAPMAN: a user-driven tool to display genomics data sets onto diagrams of metabolic pathways and other biological processes. Plant J. 37, 914–939. doi: 10.1111/j.1365-313X.2004.02016.x
Vashisth, D., Kumar, R., Rastogi, S., Patel, V. K., Kalra, A., Gupta, M. M., et al. (2018). Transcriptome changes induced by abiotic stresses in Artemisia annua. Sci. Rep. 8, 3423. doi: 10.1038/s41598-018-21598-1
Wang, H., Li, Y., Wang, S., Kong, D., Sahu, S. K., Bai, M., et al. (2020). Comparative transcriptomic analyses of chlorogenic acid and luteolosides biosynthesis pathways at different flowering stages of diploid and tetraploid Lonicera japonica. PeerJ 8, e8690. doi: 10.7717/peerj.8690
Wang, L., Lu, W., Ran, L., Dou, L., Yao, S., Hu, J., et al. (2019a). R2R3-MYB transcription factor MYB6 promotes anthocyanin and proanthocyanidin biosynthesis but inhibits secondary cell wall formation in Populus tomentosa. Plant J. 99, 733–751. doi: 10.1111/tpj.14364
Wang, J., Ren, Y., Liu, X., Luo, S., Zhang, X., Liu, X., et al. (2021). Transcriptional activation and phosphorylation of OsCNGC9 confer enhanced chilling tolerance in rice. Mol. Plant 14, 315–329. doi: 10.1016/j.molp.2020.11.022
Wang, T., Yang, B., Guan, Q., Chen, X., Zhong, Z., Huang, W., et al. (2019b). Transcriptional regulation of Lonicera japonica thunb. during flower development as revealed by comprehensive analysis of transcription factors. BMC Plant Biol. 19, 198. doi: 10.1186/s12870-019-1803-1
Xia, Y., Chen, W., Xiang, W., Wang, D., Xue, B., Liu, X., et al. (2021). Integrated metabolic profiling and transcriptome analysis of pigment accumulation in Lonicera japonica flower petals during colour-transition. BMC Plant Biol. 21, 98. doi: 10.1186/s12870-021-02877-y
Xia, P. G., Guo, H. B., Ru, M., Yang, D. F., Liang, Z. S., Yan, X. J., et al. (2017). Accumulation of saponins in panax notoginseng during its growing seasons. Ind. Crop Prod. 104, 287–292. doi: 10.1016/j.indcrop.2017.04.045
Xiang, Q., Hu, S., Ligaba-Osena, A., Yang, J., Tong, F., Guo, W. (2021). Seasonal variation in transcriptomic profiling of Tetrastigma hemsleyanum fully developed tuberous roots enriches candidate genes in essential metabolic pathways and phytohormone signaling. Front. Plant Sci. 12, 659645. doi: 10.3389/fpls.2021.659645
Yuan, P., Yang, T., Poovaiah, B. W. (2018). Calcium signaling-mediated plant response to cold stress. Int. J. Mol. Sci. 19, 3896. doi: 10.3390/ijms19123896
Yuan, L., Zhang, L., Wu, Y., Zheng, Y., Nie, L., Zhang, S., et al. (2021). Comparative transcriptome analysis reveals that chlorophyll metabolism contributes to leaf color changes in wucai (Brassica campestris l.) in response to cold. BMC Plant Biol. 21, 438. doi: 10.1186/s12870-021-03218-9
Zareei, E., Karami, F., Gholami, M., Ershadi, A., Avestan, S., Aryal, R., et al. (2021). Physiological and biochemical responses of strawberry crown and leaf tissues to freezing stress. BMC Plant Biol. 21, 532. doi: 10.1186/s12870-021-03300-2
Zhang, C., Cui, L., Zhang, P., Dong, T., Fang, J. (2021). Transcriptome and metabolite profiling reveal that spraying calcium fertilizer reduces grape berry cracking by modulating the flavonoid biosynthetic metabolic pathway. Food Chem. 2, 100025. doi: 10.1016/j.fochms.2021.100025
Zhang, J., Li, X. M., Lin, H. X., Chong, K. (2019). Crop improvement through temperature resilience. Annu. Rev. Plant Biol. 70, 753–780. doi: 10.1146/annurev-arplant-050718-100016
Keywords: Lonicera japonica, chilling, leaves color, calcium, brassinosteroids
Citation: Zhang M, Li M, Fu H, Wang K, Tian X, Qiu R, Liu J, Gao S, Zhong Z, Yang B and Zhang L (2022) Transcriptomic analysis unravels the molecular response of Lonicera japonica leaves to chilling stress. Front. Plant Sci. 13:1092857. doi: 10.3389/fpls.2022.1092857
Received: 08 November 2022; Accepted: 05 December 2022;
Published: 22 December 2022.
Edited by:
Mudassar Nawaz Khan, Hazara University, PakistanReviewed by:
Sue Lin, Wenzhou University, ChinaCopyright © 2022 Zhang, Li, Fu, Wang, Tian, Qiu, Liu, Gao, Zhong, Yang and Zhang. This is an open-access article distributed under the terms of the Creative Commons Attribution License (CC BY). The use, distribution or reproduction in other forums is permitted, provided the original author(s) and the copyright owner(s) are credited and that the original publication in this journal is cited, in accordance with accepted academic practice. No use, distribution or reproduction is permitted which does not comply with these terms.
*Correspondence: Bingxian Yang, eGlhbnliQHpqdS5lZHUuY24=; Lin Zhang, emhhbmdsaW5Aemp1LmVkdS5jbg==
†These authors have contributed equally to this work and share first authorship
Disclaimer: All claims expressed in this article are solely those of the authors and do not necessarily represent those of their affiliated organizations, or those of the publisher, the editors and the reviewers. Any product that may be evaluated in this article or claim that may be made by its manufacturer is not guaranteed or endorsed by the publisher.
Research integrity at Frontiers
Learn more about the work of our research integrity team to safeguard the quality of each article we publish.