- 1College of Agronomy, Hunan Agricultural University, Changsha, China
- 2Hunan Provincial Key Laboratory of Rice and Rapeseed Breeding for Disease Resistance, Changsha, China
- 3Hunan Hybrid Rice Research Center, Hunan Academy of Agricultural Sciences, Changsha, China
- 4State Key Laboratory of Hybrid Rice, Changsha, China
High temperature is one of the main constraints affecting plant growth and development. It has been reported that abscisic acid (ABA) synthesis gene 9-cis-epoxycarotenoid dioxygenase (NCED) positively regulates plant resistance to salt, cold, and drought stresses. However, little is known about the function of the NCED gene in heat tolerance of rice. Here, we found that OsNCED1 was a heat stress inducible gene. Rice seedlings overexpressing OsNCED1 showed enhanced heat tolerance with more abundant ABA content, whereas the knockout mutant osnced1 accumulated less ABA and showed more sensitive to heat stress. Under heat stress, increased expression of OsNCED1 could reduce membrane damage and reactive oxygen species (ROS) level of plants, and elevate the activity of antioxidant enzymes. Moreover, real time-quantitative PCR (RT-qPCR) analysis showed that overexpression of OsNCED1 significantly activated the expression of genes involved in antioxidant enzymes, ABA signaling pathway, heat response, and defense. Together, our results indicate that OsNCED1 positively regulates heat tolerance of rice seedling by raising endogenous ABA contents, which leads to the improved antioxidant capacity and activated expression of heat and ABA related genes.
Introduction
Rice (Oryza sativa L.) is a major food crop cultivated in countries around the world, especially in Asia, and feeds more than 50% of the global population. Rising temperature due to global warming has a serious impact on rice production, and the damage may continue to rise in the future, particularly for Asian rice (Qin-Di et al., 2021). Growing rice above its optimal growth temperature of 5°C results in a corresponding thermal response profile at the cellular and metabolic levels to maintain its own survival and growth (Barnabás et al., 2008). Heat stress is an essential environmental factor limiting rice growth and reproduction, causing different damage to different development stages of rice, and it will suffer from different degrees of heat stress from germination to seedling, anthesis, grain filling, and grain maturation stages (Qin-Di et al., 2021). It has been reported that rice yield decreases by 3.2% for every 1°C increasing in global temperature (Zhao et al., 2017). The difficulty of rice to maintain its normal ontogeny under high temperature conditions, including photosynthesis, respiration, enzyme activity, formation of organs of both sexes, nutrient uptake, and so on, which is the main reason why it affects rice yield (Barnabás et al., 2008).
Abscisic acid (ABA) is a sesquiterpenoid with 15 carbon atoms, which is synthesized indirectly by the carotenoid pathway in plants. The synthetic path of ABA is that zeaxanthin epoxidase (ZEP) catalyzes zeaxanthin (C40) to all-trans-violaxanthin, then the neoxanthin synthase (NSY) converts all-trans-violaxanthin to 9’-cis-neoxanthin, and finally the cis isomer is cracked by 9-cis-epoxycarotenoid dioxygenase (NCED) and product C15 xanthotoxin, which is an important step of ABA biosynthesis (Chen et al., 2020). ABA plays a crucial role in regulating plant stress responses. Its biosynthesis is induced by several environmental stresses, such as drought, salt, and cold stress (Nambara and Marion-Poll, 2005). Studies have shown that the bloating germination of Arabidopsis thaliana seeds at elevated temperatures is associated with the induction of elevated ABA levels by the zeaxanthin epoxidase gene ABA1 and the three 9-cis-epoxycarotenoid dioxygenase genes NCED2, NCED5 and NCED9 (Toh et al., 2008). Heat stress decreases auxin and gibberellin content, and increases endogenous ABA content in rice anthers (Tang et al., 2007). Exogenous ABA maintains carbon and energy balance of rice by increasing sucrose transport and accelerating sucrose metabolism, to prevent pollen abortion under heat stress, and improve seed setting rate (Rezaul et al., 2019). The bZIP transcription factor gene of the ABA signaling pathway, ZmbZIP4, can regulate ABA synthesis related genes to promote the synthesis of ABA to improve high temperature tolerance in maize (Zea mays) seedlings (Ma et al., 2018). The above findings indicate that ABA is involved in plant response to high-temperature stress, but the underlying mechanism of the effect of heat stress on endogenous ABA content in rice is still unclear.
9-cis-epoxycarotenoid dioxygenase (NCED) is a key rate limiting enzyme in ABA biosynthesis, and its activity affects endogenous ABA accumulation in plants (Kalladan et al., 2019). Overexpression of NCED promotes ABA synthesis in response to various abiotic stresses (Zhang et al., 2014; Huang et al., 2018), whereas knockdown of NCED reduces ABA accumulation, and shows abiotic stress sensitive (Frey et al., 2012). The first NCED gene to be discovered was viviparous 14 (VP14) in a maize mutant (Nambara and Marion-Poll, 2005), and NCED genes were subsequently isolated from other plant species, such as tomato (Solanum lycopersicum), Arabidopsis, and apple (Huang et al., 2018).
There are five members of the NCED family in rice, OsNCED1, OsNCED2, OsNCED3, OsNCED4 and OsNCED5 (Zhu et al., 2009). OsNCED1 expression is elevated by salt and drought stress but repressed by water stress (Huang et al., 2018; Jiang et al., 2019). It has also been shown that the cis-acting element ABRE region exists in the AhNCED1 promoter, and ABA treatment under water stress can increase promoter activity, promote AhNCED1 gene and protein expression, and promote ABA synthesis to enhance water stress (Liang et al., 2009), and that the ectopic expression of AhNCED1 will improve ABA accumulation to improve water stress tolerance in Arabidopsis plants (Wan and Li, 2006). In addition, OsNCED1 could indirectly regulate the seed setting rate of rice under low temperature stress at flowering stage by regulating ABA metabolic pathway (Huang et al., 2018). Both OsNCED2 and OsNCED3 expression levels correlate with delayed seed germination (Zhu et al., 2009; Song et al., 2012). OsNCED3 regulates seed dormancy, stomatal opening, plant growth and leaf senescence by altering ABA accumulation in rice (Huang et al., 2018). In addition, OsNCED3, OsNCED4 and OsNCED5 induce the expression and promote ABA production under water stress, which would affect plant growth and water stress (Teng et al., 2014; Zhang et al., 2015). We have reported that overexpression of OsNCED1 improved high temperature stress tolerance at heading and anthesis stages of rice (Zhou et al., 2022). However, the roles of OsNCED1 under heat stress in rice seedling stage remains unclear.
At present, little is known about the function of genes involved in the ABA synthesis pathway and signal regulation pathway in heat stress responses in rice. In this study, we constructed OsNCED1 overexpressing and gene edited transgenic rice plants, and aimed to explore the biological function of OsNCED1 under heat stress at the seedling stage of rice.
Materials and methods
Plant materials
The rice japonica variety Nipponbare (Nip), OsNCED1 overexpression lines (Nip), 252 and osnced1 mutants (252) were used in this study. 252 was an extreme heat tolerant individual of the recombinant inbred lines derived from a cross between the heat-tolerant rice line 996 and the sensitive line 4628. The overexpression vectors (pCAMBIA1300-OsNCED1) and gene editing vectors (pBWA(V)HU-ylcas9-osnced1) were constructed, and respectively transformed into Agrobacterium tumefaciens EHA105, and then introduced into Nip and 252 plants. Positive transgenic lines were selected and further identified by PCR and real time-quantitative PCR. Overexpression lines (OE-1 and OE-2) with higher transcript levels were used for further analysis, and osnced1 mutants (ko-1 and ko-2) were also obtained for further analysis by sequencing.
High temperature treatment
Seeds of wild-type (Nip and 252), OsNCED1 overexpression lines (OE-1 and OE-2), and osnced1 mutants (ko-1 and ko-2) were soaked in water at 37°C for 2 d, and sown in a rice seedling box in light incubator, with the temperature was 25°C, the light intensity was 30000 lux, the light cycle was 12/12 h (light/dark), and the relative humidity was 70%, and germinated for 8 d. Subsequently, 8-day-old transgenic lines and wild-type (WT) were transferred to a light incubator at 45°C for 48 h, and moved to a light incubator with 25°C for recovery. Corresponding WT and transgenic lines were grown in a 25°C light incubator and were set as controls. After 7 days of recovery, the phenotypes of the treated and control plants were photographed and the survival rate of seedling was counted. Four biological replicates were performed for each treatment, and 30 seedlings were replicated for each treatment.
Measurement of abscisic acid content
Eight-day-old transgenic lines and WT rice plants were transferred to a light incubator at 45°C for 48 h, while the corresponding WT and transgenic seedlings were placed in a light incubator with 25°C as controls. The corresponding WT and transgenic plants were sampled for the determination of ABA content before and 48 h after treatment, and the determination method was referred to the method as described previously (Huang et al., 2018). Briefly, 0.1 g of fresh plants was extracted with 1.5 ml of phytohormone extraction buffer and 2 ng of ABA-d6 internal standard was added, then sample was freeze-dried in nitrogen for 2 times. The ABA levels were quantified by liquid chromatography-tandem mass spectrometry (LC-MS/MS).
Measurement of physiological indexes
Eight-day-old transgenic lines and WT rice seedlings were treated in a light incubator and exposed to heat stress (45°C) for 48 h, and those in a light incubator at 25°C served as controls. After 48 h of treatment, plants under high temperature stress and control conditions were used for the determination of relative electrolyte leakage rate, malondialdehyde (MDA) content, hydrogen peroxide (H2O2) content and superoxide anion (O2-) content, superoxide dismutase (SOD) activity, peroxidase (POD) activity, and three biological replicates were performed. The electrolyte leakage rate conductivity of heat-treated and control plants was measured by the conductivity meter (DS-11A), the MDA content was measured by the thiobarbituric acid (TBA) colorimetric method, the SOD activity was measured by the riboflavin nitro blue tetrazolium (NBT) method, and the POD activity was measured by the guaiacol method. The electrolyte leakage rate, MDA content and antioxidant enzyme activities were determined by the method of Zhou et al. (2022), with a slight modification. Referring to the method of Sun et al. (2019), 3,3-diaminobenzidine (DAB) and nitro blue tetrazolium were used to detect the accumulation of H2O2 and O2- in the leaves of plants. The content of H2O2 and O2- of high temperature treated and control plants were determined using kits (BC3595, Solarbio).
Quantitative real-time PCR analysis
The WT plants treated with high-temperature for 0 h, 2 h, 4 h, and 8 h, the transgenic and WT plants of high-temperature treated for 48 h and the control were collected, and the corresponding RNA was extracted after snap freezing with liquid nitrogen. RT-qPCR was used to determine the expression levels of OsNCED1 along with the transcript levels of genes involved in antioxidant enzymes, ABA signaling pathway, heat response, and defense. Total RNA extraction was performed using RNA easy isolation reagent (R701-01, Vazyme) and was reverse transcribed for qPCR analysis using the HiScript® II Q RT SuperMIX for RT-qPCR (+gDNA wiper) kit (R223-01, Vazyme). Rice OsActin1 was used as an internal reference gene, and primers for amplification were designed by Primer Premier 6.0. The relative changes in gene expression levels were quantitated based on three biological replicates via the 2-ΔΔCt method.
Statistical analysis
All experiments were conducted in three biological replicates. Data are presented as mean ± SE, and statistical analysis was performed using DPS (version 7.05). Data were analyzed by one-way ANOVA and it was considered statistically significant at p < 0.05, p < 0.01. Plotted using GraphPad Prism (version 8.01).
Results
Response of OsNCED1 to heat stress at rice seedling stage
In our previous study, the quantitative proteomics technology of iTRAQ quantitative marker combined with LC-MS/MS analysis was used to compare and analyze the difference of anther protein expression between heat tolerant rice line 996 and heat sensitive rice line 4628 under heat stress, and it was found that OsNCED1 was significantly upregulated (Zhou et al., 2022). In order to further explore the response of OsNCED1 to heat stress at rice seedling stage, RT-qPCR was used to detect the expression level of OsNCED1 in seedling of Nip and 252, an extreme heat tolerant individual, at 45°C for 0 h, 2 h, 4 h, 8 h and 48 h. Upon heat stress, the expression of OsNCED1 increased significantly in Nip and 252, peaked at 4 h and then decreased at 8 h of the heat stress (Figures 1A, B). These results indicated that OsNCED1 responded to heat stress and its expression was strongly induced.
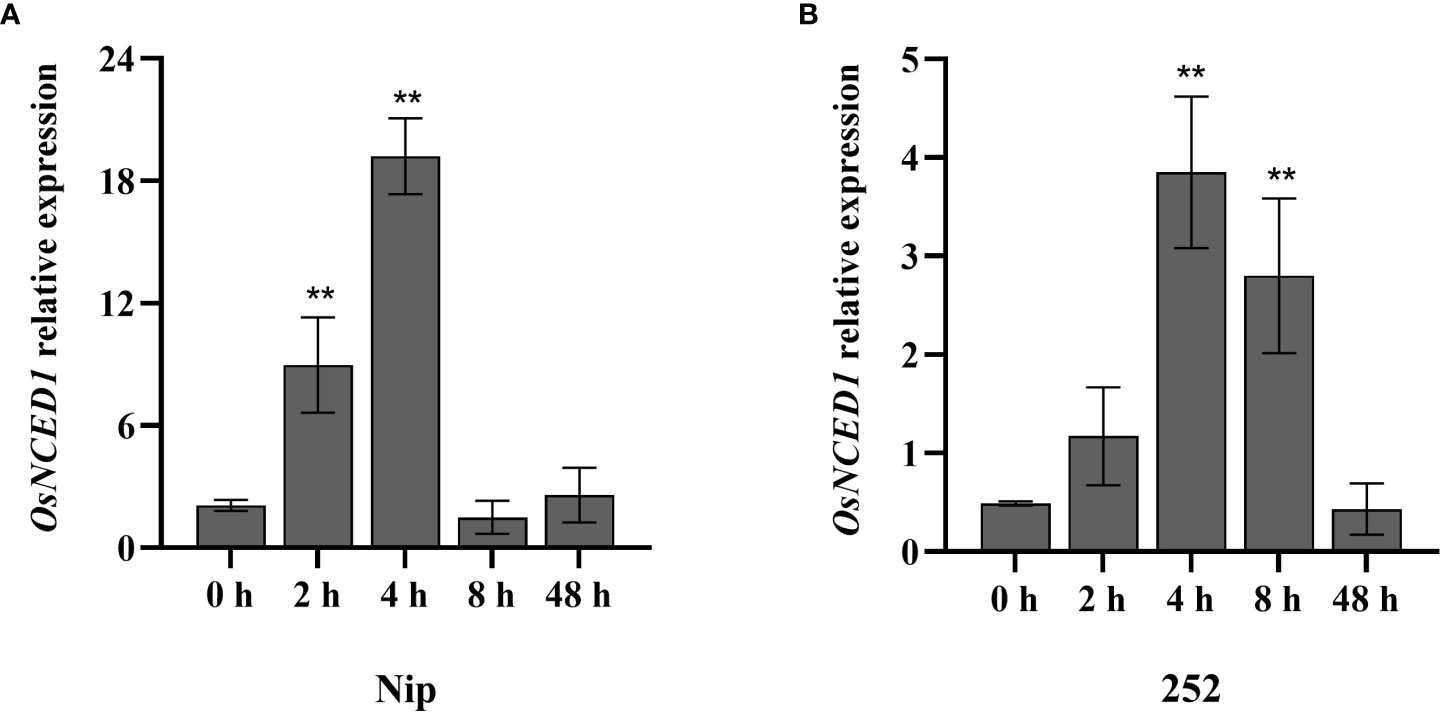
Figure 1 Transcript level of OsNCED1 in Nip and 252 under heat stress. (A) Transcript level of OsNCED1 in Nip under heat stress. (B) Transcript level of OsNCED1 in 252 under heat stress. Data are means ± SD (n = 3; **P< 0.01, Student’s t-test).
Effects of heat stress on survival rate and endogenous ABA content of OsNCED1 transgenic and WT seedlings
In order to study the role of OsNCED1 in heat stress, two mutants, ko-1 and ko-2, were constructed in the background of 252 (Figure 2A; Supplementary Figure 1). The ko-1 and ko-2 had two different mutation sites in the OsNCED1 exons, which caused premature termination of OsNCED1 protein. The overexpression lines OE-1 and OE-2 of OsNCED1 were constructed in the background of Nip. And the expression levels of the overexpression lines OE-1 and OE-2 were 50.9 times and 30.9 times higher than that of Nip, respectively (Figure 2B). As shown in Figures 2C, D, upon heat stress, the survival rate of Nip was significantly lower than that of the two overexpression lines (OE-1 and OE-2), while the survival rate of 252 was significantly higher than that of the two mutants (ko-1 and ko-2). Under the control conditions, there is no significant difference between transgenic plants and corresponding WT (Figure 2D). The results showed that OsNCED1 positively regulated the heat stress of rice seedlings.
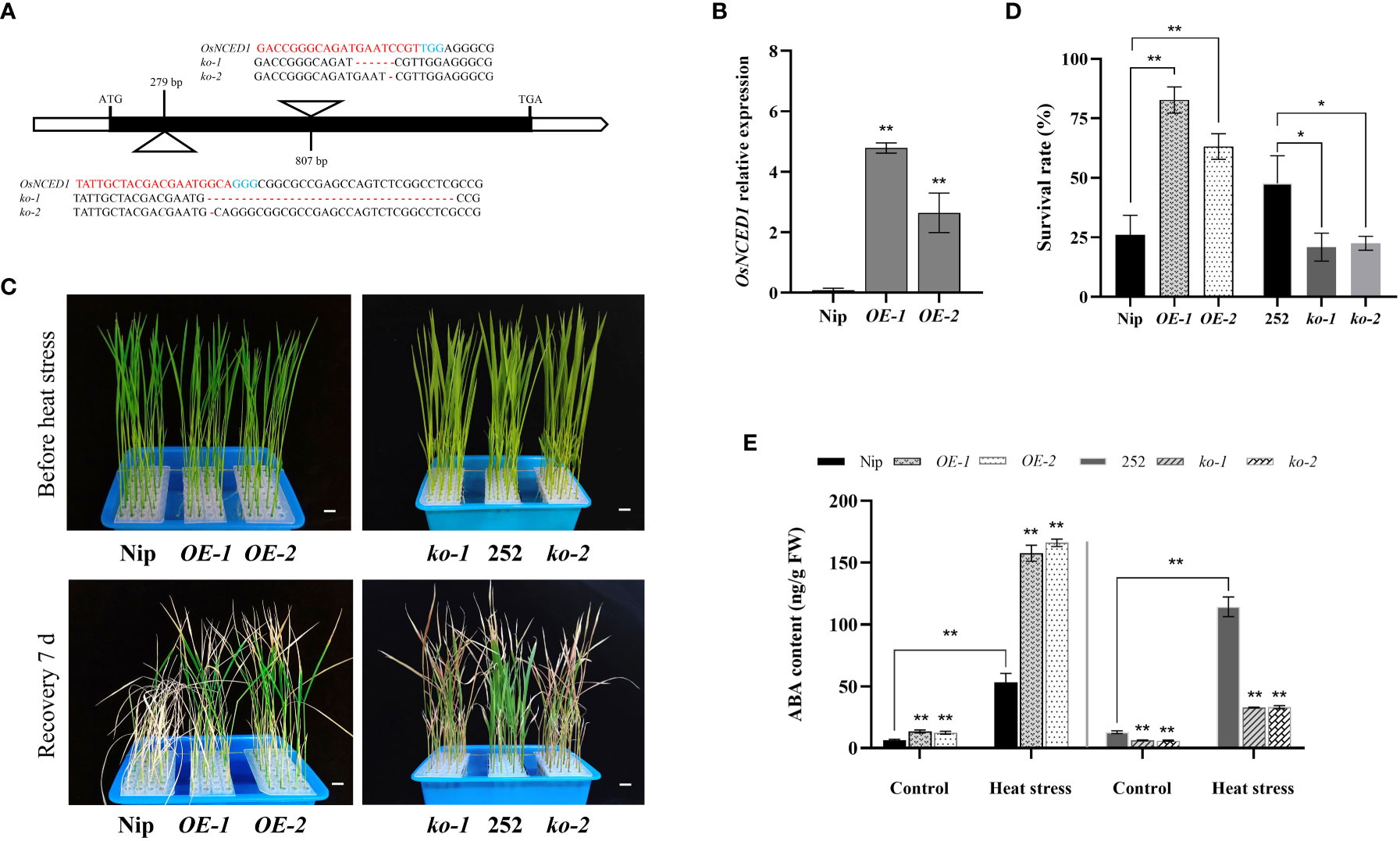
Figure 2 Phenotypic analyses of OsNCED1 transgenic lines under heat stress. (A) The diagram of the two CRISPR/Cas9 target sites, and nucleotide mutation sequences of ko-1 and ko-2 lines. The base pairs in blue indicated protospacer adjacent motif (PAM), and the red represented small guide RNA (sgRNA) sequence. (B) Real-time quantitative PCR analysis of OsNCED1 in OsNCED1 overexpression lines OE-1 and OE-2. Data are means ± SD (n = 3; **P< 0.01, Student’s t-test). (C) Phenotypes of two WT and OsNCED1 transgenic rice seedlings before heat stress and 7 days after moderate temperature recovery. Bars = 1 cm. (D) Survival rates (%) of two WT and OsNCED1 transgenic lines after heat stress for 48 h. Data are means ± SD (n = 4; *P< 0.05, **P< 0.01, Student’s t-test). (E) Endogenous ABA content in WT and OsNCED1 transgenic lines under control and heat stress. Data are means ± SD (n = 3; **P< 0.01, Student’s t-test).
ABA is in a dynamic equilibrium in response to physiological changes and stimuli from the external environment in plants. NCED is a key rate limiting enzyme for ABA biosynthesis, and affects the accumulation of endogenous ABA (Kalladan et al., 2019). The ABA content under control and heat stress revealed that the ABA levels of the overexpression lines OE-1 and OE-2 under control conditions were 13.5 ng/g and 12.4 ng/g, respectively, and significantly higher than that of Nip (6.4 ng/g), whereas the ABA levels of the mutants ko-1 and ko-2 were 6.2 ng/g and 5.9 ng/g, respectively, and significantly lower than that of 252 (12.9 ng/g) (Figure 2E). The ABA contents of the transgenic and WT plants were significantly increased under heat stress, indicating that heat tolerance of rice might be improved by increasing the ABA content. Thus, OsNCED1 might improve the tolerance to heat stress by affecting the accumulation of endogenous ABA in rice.
Changes in membrane lipid peroxidation and antioxidant enzyme activities in OsNCED1 transgenic and WT seedlings under heat stress
To reveal the underlying physiological mechanism which OsNCED1 improves heat tolerance in rice, MDA content, relative electrolyte leakage rate, SOD activity and POD activity of OsNCED1 transgenic and WT seedling were measured under heat stress. Malondialdehyde can reflect the rate and intensity of peroxidation of plant membrane lipids, and can also indirectly reflect the potential antioxidant capacity of plant cells (Rezaul et al., 2019). Relative electrolyte leakage rate conductivity is an important parameter to examine the membrane permeability of plant cells under abiotic stress (Huang et al., 2018). Under control conditions, there was no significant difference in MDA content and relative electrolyte leakage rate between transgenic and WT seedlings (Figures 3A, B). After 48 h of heat stress, the MDA content in both transgenic and WT seedlings increased significantly, but the increase of MDA content and relative electrolyte leakage rate of overexpression lines were significantly lower than that of Nip (Figures 3A, B), whereas the increase in the MDA content and relative electrolyte leakage rate of the mutants was significantly higher than that of 252, indicating that the increase in OsNCED1 can reduce membrane damage in plants under heat stress.
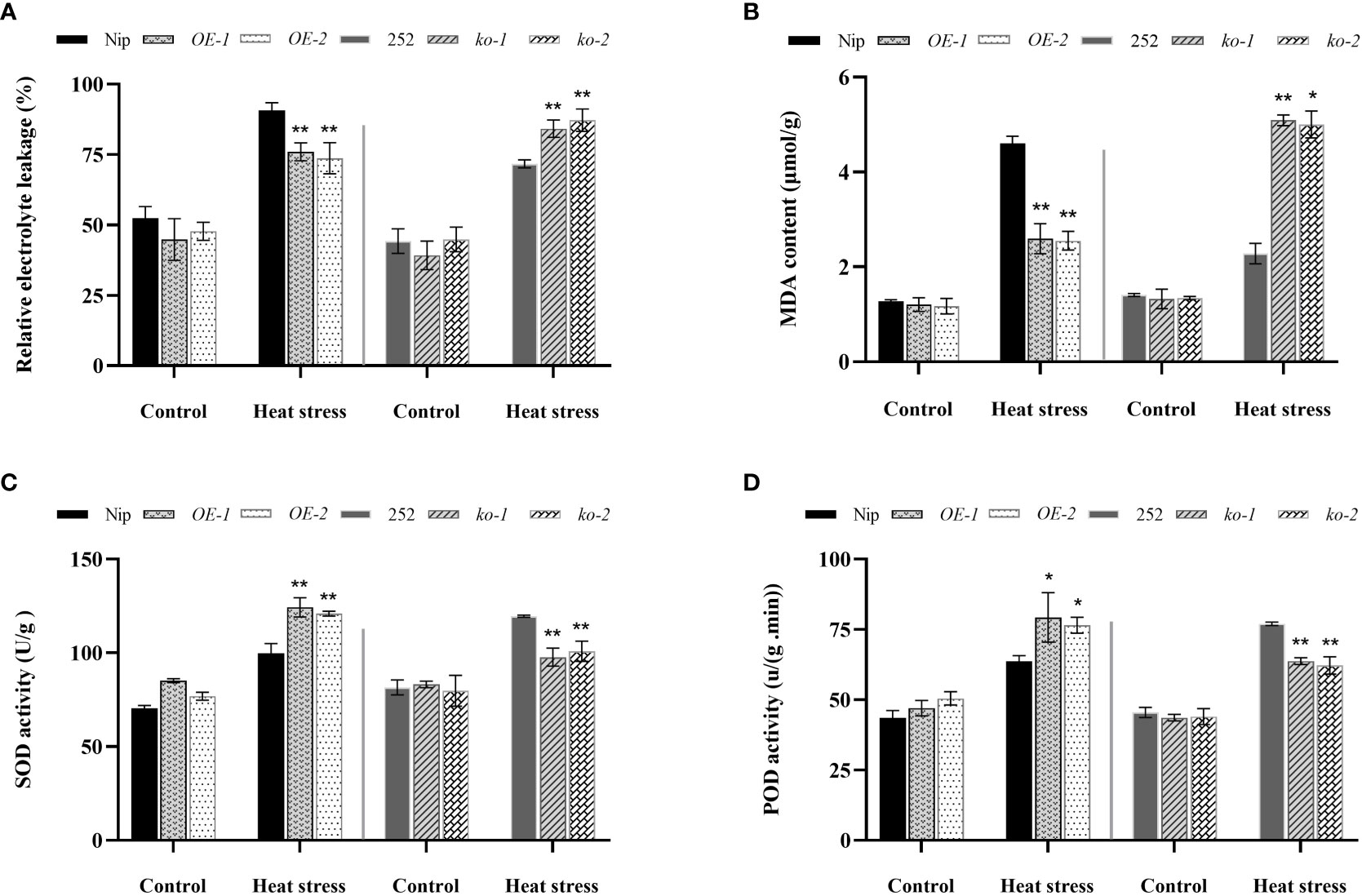
Figure 3 Changes in membrane lipid peroxidation and antioxidant enzyme activities in the transgenic lines and WT plants under heat stress. The electrolyte leakage rate (A) and MDA content (B) and SOD activity (C) and POD activity (D) in transgenic lines and WT after 48 h heat stress. Data are means ± SD (n = 3; *P< 0.05, **P< 0.01, Student’s t-test).
Next, the activities of SOD and POD of transgenic and WT seedlings under heat stress were measured. As shown in Figures 3C, D, after 48 h of heat stress, the WT and transgenic lines showed higher activities of both antioxidative enzymes than that of the control, and the increases in antioxidative enzyme activities were significantly greater in the OE-1 and OE-2 lines than that of Nip, and the activities of antioxidant enzymes in ko-1 and ko-2 lines were significantly lower than that of 252. These results suggested that OsNCED1 could enhance seedling antioxidant enzyme activities under heat stress and attenuated membrane damage upon heat stress in rice seedling.
Changes in reactive oxygen species levels in OsNCED1 transgenic and WT seedlings under heat stress
To examine whether OsNCED1 confers stress resistance to ROS, the accumulation of H2O2 and O2- was examined under heat stress. The DAB and NBT staining result showed no obvious difference between the transgenic and WT seedling leaves under control conditions, whereas the leaves of the overexpression lines OE-1 and OE-2 seedlings under high-temperature stress stained lighter than that of Nip, whereas the leaves of the mutants ko-1 and ko-2 seedlings stained darker than that of 252 (Figures 4A, B).
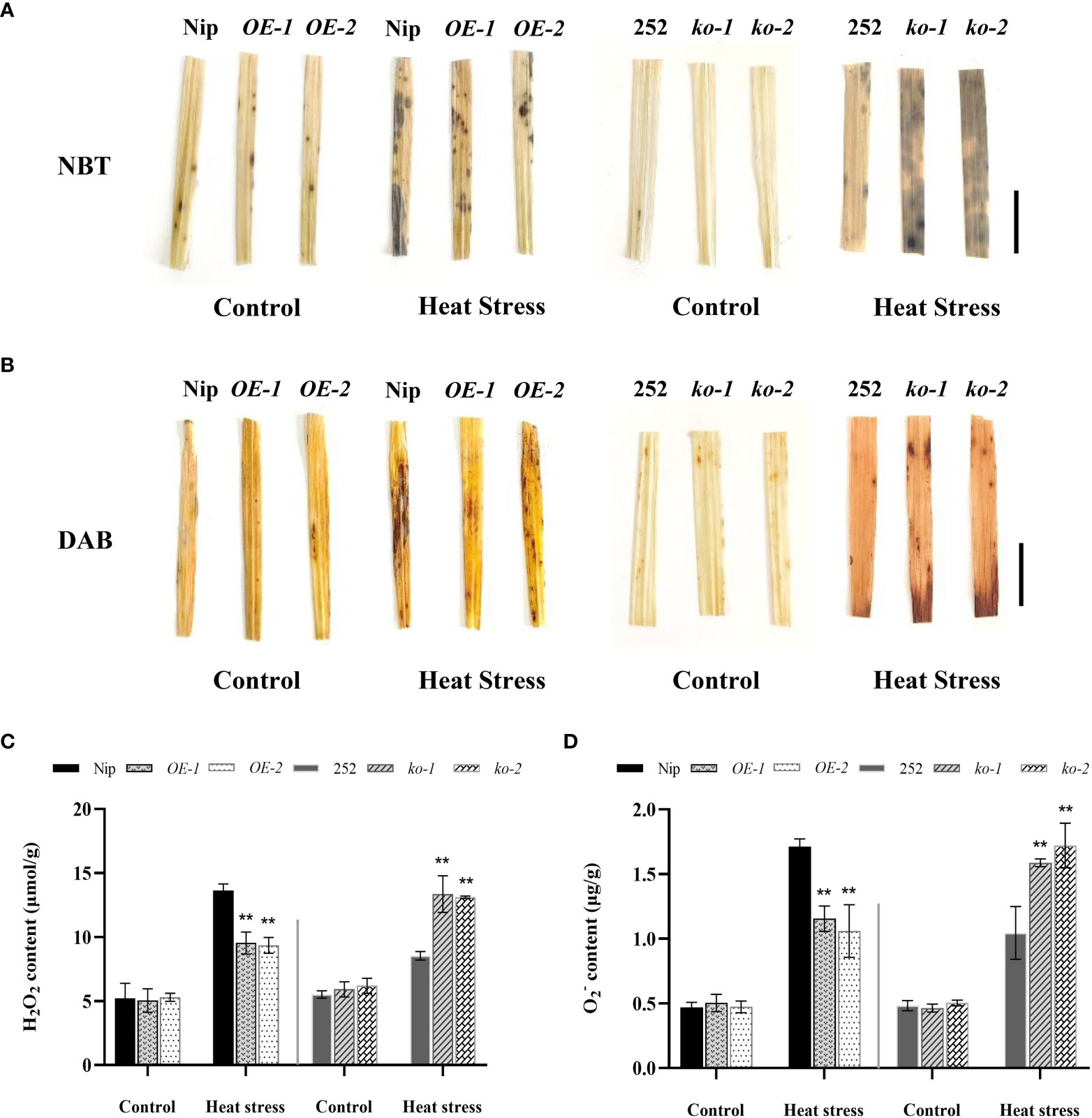
Figure 4 Accumulation of H2O2 and O2- in seedlings under heat stress. (A) O2- production in leaf discs of WT and transgenic lines upon heat exposure. Bar = 1 cm. (B) H2O2 accumulation in leaf discs of WT and transgenic lines upon heat exposure. Bar = 1 cm. (C) Quantitative measurement of total H2O2 content in WT and transgenic lines upon heat exposure. (D) Quantitative measurement of total O2- content in WT and transgenic lines upon heat exposure. Data are means ± SD (n = 3; **P< 0.01, Student’s t-test).
Further the determination result of H2O2 content and O2- content of OsNCED1 transgenic and WT seedlings showed that Nip accumulated significantly more H2O2 and O2- than that of overexpression lines OE-1 and OE-2, and the H2O2 content in Nip was 43% and 46% higher than that of overexpression lines OE-1 and OE-2, respectively; the O2- content was 48% and 61% higher than that of overexpression lines OE-1 and OE-2, respectively (Figures 4C, D). In addition, the accumulation of H2O2 and O2- in 252 was significantly lower than that of the mutants, the H2O2 content was 57% and 54% lower than that of the mutants ko-1 and ko-2, respectively; the O2- content was 52% and 65% lower than that of the mutants ko-1 and ko-2, respectively (Figures 4C, D). The results indicated that OsNCED1 positively regulated plant antioxidant resistance under heat stress, and its overexpression could alleviate oxidative damage under heat stress.
Transcriptional changes of antioxidant and defense related genes in OsNCED1 transgenic and WT seedlings under heat stress
To elucidate the potential molecular mechanism of OsNCED1 for heat tolerance, the transcript levels of several antioxidant and defense related genes in the transgenic and WT plants under control and heat stress conditions were detected by RT-qPCR assays. These genes included three antioxidant genes (catalase encoding gene OsCATB, superoxide dismutase gene Fe+SOD, and ascorbate peroxidase OsAXP1) (Feng et al., 2006; Ye et al., 2011; Li et al., 2015) and three defense genes (stress-responsive NAC transcription factor gene osSNAC1, AP2/EREBP transcription factor OsDREB2A, and late embryogenesis abundant enriched protein gene OsLEA3) (Hu et al., 2006; Xiao et al., 2007; Zhang et al., 2013), which had been shown to play critical roles in protecting rice against abiotic stress.
As shown in Figures 5A–C, there was no obvious difference in antioxidant genes between WT and transgenic lines seedlings under control conditions, but under heat stress, both WT and transgenic seedlings showed upregulated expression of antioxidant genes, especially OsCATB. Compared with Nip, the transcript levels of the three antioxidant related genes were significantly upregulated in the overexpression lines; the transcript levels of antioxidant related genes were significantly downregulated in the mutants compared to 252. Subsequently, the expression levels of three defense genes, OsSNAC1, OsDREB2A and OsLEA3, in the WT and OsNCED1 transgenic lines under heat stress were examined. As shown in Figures 5D–F, the transcript levels of the three defense related genes were significantly higher in the overexpression lines than that of Nip under heat stress, with OsLEA3 upregulation being the most significant. The transcript levels of defense related genes in the two mutants were significantly lower than that of 252. These data suggested that OsNCED1 induced the expression of antioxidant and defense related genes under heat stress.
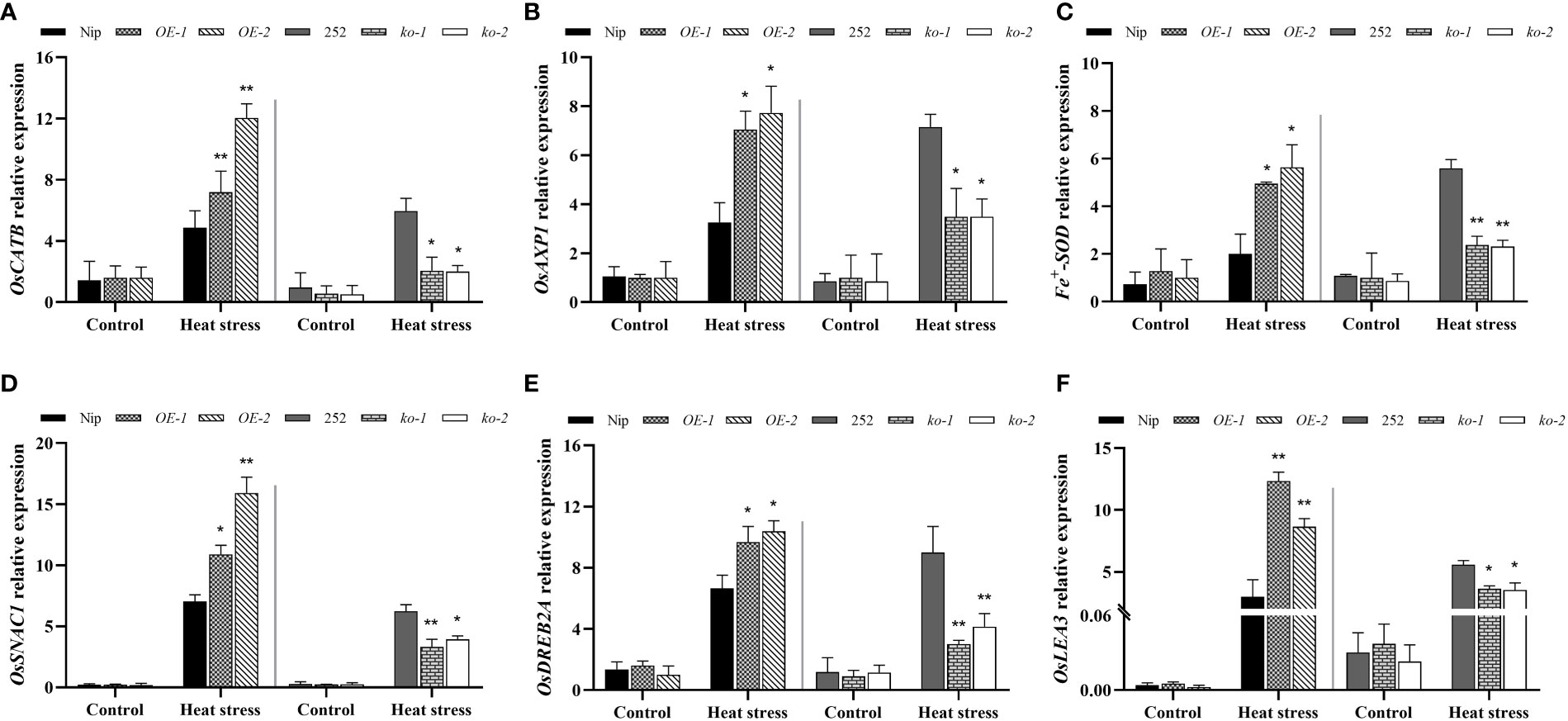
Figure 5 Transcriptional expression of antioxidant and defense related genes in seedlings of WT and transgenic lines under heat stress. Three antioxidant genes transcript levels of OsCATB (A), OsAXP1 (B), and Fe+SOD (C). Seedlings of WT and transgenic lines were subjected to heat stress at 45°C for 48 h, and leaves were harvested for RNA extraction, cDNA synthesis and RT-qPCR analysis. For each RT- qPCR, rice reference gene OsActin1 was used as a control to detect its transcript levels in different samples. Data are means ± SD (n = 3; *P< 0.05, **P< 0.01, Student’s t-test). Three defense genes transcript levels of OsSNAC1 (D), OsDREB2A (E), and OsLEA3 (F). Data are means ± SD (n = 3; *P< 0.05, **P< 0.01, Student’s t-test).
Transcriptional changes of genes related to heat and ABA responses in OsNCED1 transgenic and WT seedlings under heat stress
The main function of HSPs was to regulate the folding and unfolding of proteins, as well as their subcellular localization and degradation of unfolded and denatured proteins (Singh et al., 2016). SLG1 is able to interact with cytoplasmic tRNA 2-thiolated protein 1 (RCTU1) in rice to regulate tRNA thiolation levels and thus positively regulate rice heat tolerance (Xu et al., 2020). Therefore, the expression of HSPs genes and SLG1 in OsNCED1 transgenic and WT seedlings under heat stress was examined using RT-qPCR. As shown in Figures 6A–C, the transcript levels of OsHSP70, OsHSP90 and SLG1 of the overexpression lines were significantly higher than that of WT under 48 h heat stress, and the transcript levels of genes related to heat tolerance in the two mutants were significantly lower than that of 252. In contrast, there were no significant difference in the expression levels of these heat tolerance related genes between WT and transgenic lines under control conditions. OsNCED1 is involved in ABA biosynthesis, so the transcriptional change of ABA responsive genes between the transgenic lines and WT plants under heat stress was investigated. These ABA responsive genes included OsbZIP46 (ABRE binding protein), OsABI5 (ABRE binding factor), and OsSAPK10 (stress-activated protein kinase), which have been shown to be involved in ABA responsive responses (Zou et al., 2008; Yang et al., 2011; Wang et al., 2020). As shown in Figures 6D–F, the transcript levels of the three ABA responsive genes in the overexpression lines showed greater upregulation under heat stress than that of Nip, and the mutants were upregulated less than 252. These results indicated that OsNCED1 positively activated the expression of heat responsive genes and ABA responsive genes under heat stress.
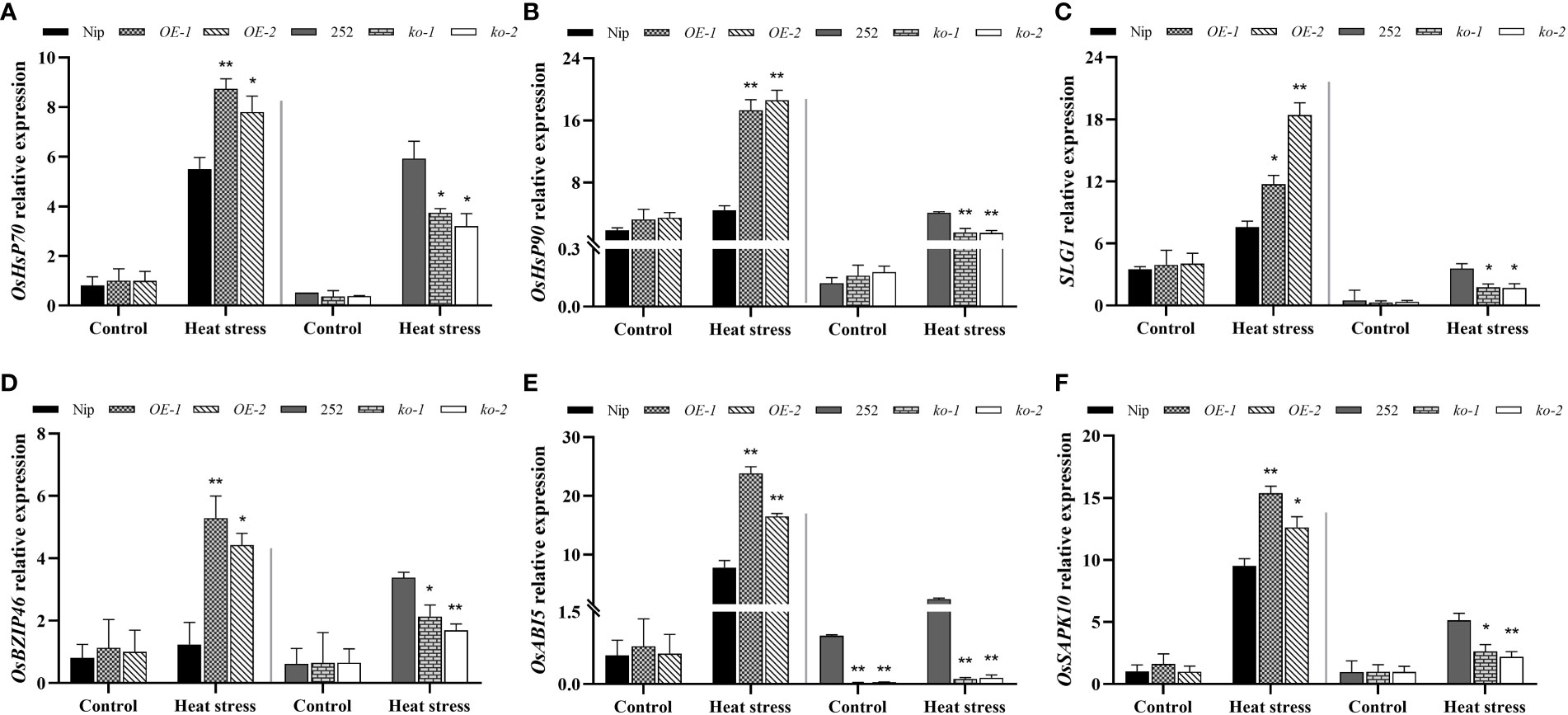
Figure 6 Transcriptional expression of heat tolerance and ABA related genes in WT and transgenic lines under heat stress. Three heat tolerance genes transcript levels of OsHSP70 (A), OsHSP90 (B), and SLG1 (C). Seedlings of WT and transgenic lines were subjected to heat stress at 45°C for 48 h, and leaves were harvested for RNA extraction, cDNA synthesis and RT-qPCR analysis. For each RT-qPCR, rice reference gene OsActin1 was used as a control to detect its transcript levels in different samples. Data are means ± SD (n = 3; *p < 0.05, **p < 0.05, Student’s t-test). Three heat ABA related genes transcript levels of OsbZIP46 (D), OsABI5 (E), and OsSAPK10 (F). Data are means ± SD (n = 3; *p < 0.05, **p < 0.05, Student’s t-test).
Discussion
Temperature is an important factor affecting rice growth and yield quality. With global warming, it is extremely important to understand how plants respond to high temperatures and breed high temperature tolerant rice varieties. Temperature of 10-15°C above ambient was generally considered as heat shock or heat stress in plants (Liu et al., 2018), whereas heat tolerance was the ability of plants to cope with heat stress, that was, the ability of plants to survive in an environment growing above the most suitable temperature (Liu et al., 2016). The optimal growth temperature at the seedling stage of rice was 25-28°C, and heat stress at seedling stage (42-45°C) lead to increased water loss, leaf wilting and yellowing, impaired root growth, and severe or even seedling death (Liu et al., 2016; Liu et al., 2018). In this study, we showed that overexpression of OsNCED1 increased the heat tolerance of rice seedlings, while osnced1 seedlings exhibited reduced heat tolerance (Figure 2), indicating that OsNCED1 positively regulates heat stress tolerance in rice seedling plants.
ABA is a hormone that is often involved in the stress response of plants. When subjected to abiotic stresses such as cold, drought and high temperature et al., plants will rapidly accumulate ABA to activate various stress responses. For example, ABA in grapes alleviated hyperthermic damage by increasing the accumulation of osmoregulation substances and endogenous hormone content (Lv et al., 2022). And SlSnRK2.3 regulated ABA signaling pathway regulates stomatal movement under heat stress to improve the heat tolerance in tomato (Li et al., 2022). Moreover, brassinosteroids enhanced tolerance of canola seedlings to heat stress might be due to the induction of elevated endogenous ABA concentrations (Kurepin et al., 2008). It had also been shown in rice that the application of exogenous ABA alleviated pollen sterility under high-temperature stress and was responsible for improving heat tolerance in rice by promoting sucrose transport and metabolism in spikelets, antioxidant enzyme activity, maintaining carbon balance and energy balance (Rezaul et al., 2019). And high temperature stress promoted ABA accumulation to regulate seed germination (Liu et al., 2019). NCED genes contributed to higher ABA levels, and increased abiotic stress tolerance in plants (Bang et al., 2013). Our results suggested that OsNCED1 enhanced rice seedling heat tolerance, possibly by regulating the endogenous ABA content. The reasons were as follows: firstly, the transcript levels of OsNCED1 in both WT plants were rapidly induced under heat stress (Figure 1). Secondly, ABA content of transgenic plants and WT increased after heat stress; while ABA content of the overexpression lines were significantly higher than that of Nip, and ABA content in osnced1 were lower than that in 252 (Figure 2). Thirdly, the transcript levels of ABA related genes were significantly upregulated in the overexpression lines under heat stress (Figures 5, 6). In support of this idea, previous reports had shown that ABA improved heat tolerance by inducing the accumulation of several HSPs, including HSP70 and HSP90 in plants (Li et al., 2014). There were also reports indicating that the expression of OsLEA3 in rice seedlings was induced by ABA (Xiao et al., 2007). And OsbZIP46 was involved in ABA signaling and abiotic stress response (Yang et al., 2011).
ROS were harmful substances reflecting oxidative metabolism. Both ROS production and scavenging affected protein, fat and nucleic acid damage and cell death (Raja et al., 2017). So, the dynamic balance of ROS was especially important for plant growth and development. Efficient enzymatic and nonenzymatic antioxidant defense systems play an important role in scavenging ROS, reducing membrane lipid peroxidation, maintaining ROS dynamic balance and redox signals (Hasanuzzaman et al., 2018). SOD dismutates superoxide to hydrogen peroxide, while peroxidases (PODs) further decompose hydrogen peroxide to water and molecular oxygen (Wang et al., 2016). MDA accumulation and the electrolyte leakage rate well reflected the degree of cell damage. In this study, the activities of SOD and POD were increased in both the transgenic and WT seedling under heat stress, and among them, the OsNCED1 overexpression lines showed higher SOD activity and POD activity, lower MDA content, electrolyte leakage rate and O2− content than Nip, thereby decreased cell membrane damage and oxidative stress, whereas the knockout lines happened to do the opposite, resulting in its poor survival (Figures 3, 4). Consistent with the antioxidant enzyme activities, we detected that the OsNCED1 overexpression lines showed a significant upregulation in the transcript levels of the antioxidant related genes OsCATB、Fe+SOD and OsAXP1 under heat stress, and the osnced1 mutants, although somewhat upregulated, it was not upregulated as much as 252 (Figure 5). Therefore, OsNCED1 overexpression lines might be to maintain the structure and function of cells under heat stress by regulating their own enzyme system activity and content of osmotic protective substances to scavenge toxic substances such as free radicals. Consistent with this paper, it was reported that copper/zinc superoxide dismutase 1 (CSD1) and CSD2 in Arabidopsis altered the redox status and scavenged ROS of cells to regulate heat tolerance (Guan et al., 2013). Similarly, there was also evidence that transgenic potatoes overexpressing CuZnSOD and APX had higher tolerance to high temperature and oxidative stress (Kim et al., 2010). On the contrary, the loss of AXP1 and CAT2 reduced the tolerance of Arabidopsis to high temperature stress (Vanderauwera et al., 2011). Reports had suggested that ABA in plants actively participated in antioxidant defense mechanisms through various MAPK cascades, such as ABA transiently activates MPK6 to regulate the expression of catalase 1 (CAT1), and maintaining ROS homeostasis (Raja et al., 2017).
Acting as a second messenger of ROS generating signals, H2O2 has a dual role in regulating plant physiological processes, since low concentrations of H2O2 initiate various signals in cells, whereas high concentrations of H2O2 cause oxidative damage (Quan et al., 2008; Gill and Tuteja, 2010). ABA as a stress signal plays an important role in abiotic stresses, but there are different claims about the interaction between H2O2 and ABA under abiotic stress. Our study showed that high-temperature treatment elevated endogenous ABA in the overexpression plants (Figure 2E), accompanied by reduced H2O2 content (Figures 4B, C), reduced oxidative damage, to improve heat tolerance at the seedling stage of rice. Consistent with our results, OsASR6 interacted with OsNCED1 to enhance endogenous ABA content and reduce H2O2 accumulation to improve rice salt tolerance (Zhang et al., 2022). In addition, the ABA accumulation in the OsIAA18 overexpressing rice seedlings was significantly higher than that in WT under both salt and drought stress, and the genes of ABA synthesis and signaling pathways were also evidently upregulated, along with obviously lower level of H2O2 and improved salt and drought (Wang et al., 2021). However, it has been shown that, under drought conditions, ABA induces ROS production and increases H2O2 content in Arabidopsis guard cells, which activates calcium ion channels to promote stomatal closure and reduces water loss (Pei et al., 2000). It has also been shown that exogenous ABA induces H2O2 production via OsDMI3 in rice zhonghua11 (Shi et al., 2012). We speculated that OsNCED1 in this study might reduce membrane damage and ROS levels in plants under heat stress by regulating ABA content. However, the exact role of OsNCED1 in ROS homeostasis under heat stress requires further investigation. Our study provides a valuable resource for the potential exploitation of OsNCED1 in the genetic improvement of heat tolerance in rice. Future studies on OsNCED1 will include determining how other genes, together with OsNCED1, are involved in other physiological functions not observed in osnced1 mutants. In addition, other novel regulatory functions of OsNCED1 can be investigated by identifying its interacting proteins.
Data availability statement
The original contributions presented in the study are included in the article/Supplementary Material. Further inquiries can be directed to the corresponding authors.
Author contributions
YZ and XiL: investigation, writing-original draft. WT and GZ: conceptualization, supervision. RS and XuL: formal analysis. FW, HD, YX, GC and GZ: writing-review and editing. All author contributed to the article and approved the submitted version.
Funding
This research was funded by the National Natural Science Foundation of China (31871599 and 31901528), the Natural Science Foundation of Hunan province (2021JJ30350), the Double first-class construction project of Hunan Agricultural University (SYL2019007), and the Hunan postgraduate scientific research innovation project (CX20200672). The APC was funded by the National Natural Science Foundation of China.
Conflict of interest
The authors declare that the research was conducted in the absence of any commercial or financial relationships that could be construed as a potential conflict of interest.
Publisher’s note
All claims expressed in this article are solely those of the authors and do not necessarily represent those of their affiliated organizations, or those of the publisher, the editors and the reviewers. Any product that may be evaluated in this article, or claim that may be made by its manufacturer, is not guaranteed or endorsed by the publisher.
Supplementary material
The Supplementary Material for this article can be found online at: https://www.frontiersin.org/articles/10.3389/fpls.2022.1092630/full#supplementary-material
References
Bang, S. W., Park, S. H., Jeong, J. S., Kim, Y. S., Jung, H., Ha, S. H., et al. (2013). Characterization of the stress-inducible OsNCED3 promoter in different transgenic rice organs and over three homozygous generations. Planta 237, 211–224. doi: 10.1007/s00425-012-1764-1
Barnabás, B., Jäger, K., Fehér, A. (2008). The effect of drought and heat stress on reproductive processes in cereals. Plant Cell Environ. 31, 11–38. doi: 10.1111/j.1365-3040.2007.01727.x
Chen, K., Li, G. J., Bressan, R. A., Song, C. P., Zhu, J. K., Zhao, Y. (2020). Abscisic acid dynamics, signaling, and functions in plants. J. Integr. Plant Biol. 62, 25–54. doi: 10.1111/jipb.12899
Feng, W., Hongbin, W., Bing, L., Jinfa, W. (2006). Cloning and characterization of a novel splicing isoform of the iron-superoxide dismutase gene in rice (Oryza sativa l.). Plant Cell Rep. 24, 734–742. doi: 10.1007/s00299-005-0030-4
Frey, A., Effroy, D., Lefebvre, V., Seo, M., Perreau, F., Berger, A., et al. (2012). Epoxycarotenoid cleavage by NCED5 fine-tunes ABA accumulation and affects seed dormancy and drought tolerance with other NCED family members. Plant J. 70, 501–512. doi: 10.1111/j.1365-313X.2011.04887.x
Gill, S. S., Tuteja, N. (2010). Reactive oxygen species and antioxidant machinery in abiotic stress tolerance in crop plants. Plant Physiol. Biochem. 48, 909–930. doi: 10.1016/j.plaphy.2010.08.016
Guan, Q., Lu, X., Zeng, H., Zhang, Y., Zhu, J. (2013). Heat stress induction of miR398 triggers a regulatory loop that is critical for thermotolerance in Arabidopsis. Plant J. 74, 840–851. doi: 10.1111/tpj.12169
Hasanuzzaman, M., Oku, H., Nahar, K., Bhuyan, M. H. M. B., Mahmud, J. A., Baluska, F., et al. (2018). Nitric oxide-induced salt stress tolerance in plants: ROS metabolism, signaling, and molecular interactions. Plant Biotechnol. Rep. 12, 77–92. doi: 10.1007/s11816-018-0480-0
Huang, Y., Guo, Y., Liu, Y., Zhang, F., Wang, Z., Wang, H., et al. (2018). 9-cis-epoxycarotenoid dioxygenase 3 regulates plant growth and enhances multi-abiotic stress tolerance in rice. Front. Plant Sci. 9. doi: 10.3389/fpls.2018.00162
Hu, H., Dai, M., Yao, J., Xiao, B., Li, X., Zhang, Q., et al. (2006). Overexpressing a NAM, ATAF, and CUC (NAC) transcription factor enhances drought resistance and salt tolerance in rice. Proc. Natl. Acad. Sci. U. S. A. 103, 12987–12992. doi: 10.1073/pnas.0604882103
Jiang, D., Zhou, L., Chen, W., Ye, N., Xia, J., Zhuang, C. (2019). Overexpression of a microRNA-targeted NAC transcription factor improves drought and salt tolerance in rice via ABA-mediated pathways. Rice 12, 76. doi: 10.1186/s12284-019-0334-6
Kalladan, R., Lasky, J. R., Sharma, S., Kumar, M. N., Juenger, T. E., Des Marais, D. L., et al. (2019). Natural variation in 9-cis-epoxycartenoid dioxygenase 3 and ABA accumulation. Plant Physiol. 179, 1620–1631. doi: 10.1104/pp.18.01185
Kim, M. D., Kim, Y. H., Kwon, S. Y., Yun, D. J., Kwak, S. S., Lee, H. S. (2010). Enhanced tolerance to methyl viologen-induced oxidative stress and high temperature in transgenic potato plants overexpressing the CuZnSOD, APX and NDPK2 genes. Physiol. Plant 140, 153–162. doi: 10.1111/j.1399-3054.2010.01392.x
Kurepin, L. V., Qaderi, M. M., Back, T. G., Reid, D. M., Pharis, R. P. (2008). A rapid effect of applied brassinolide on abscisic acid concentrations in Brassica napus leaf tissue subjected to short-term heat stress. Plant Growth Regul. 55, 165–167. doi: 10.1007/s10725-008-9276-5
Liang, J., Yang, L., Chen, X., Li, L., Guo, D., Li, H., et al. (2009). Cloning and characterization of the promoter of the 9-cis-epoxycarotenoid dioxygenase gene in Arachis hypogaea l. Biosci. Biotechnol. Biochem. 73, 2103–2106. doi: 10.1271/bbb.90133
Li, Y., Gao, Z., Lu, J., Wei, X., Qi, M., Yin, Z., et al. (2022). SlSnRK2.3 interacts with SlSUI1 to modulate high temperature tolerance via abscisic acid (ABA) controlling stomatal movement in tomato. Plant Sci. 321, 111305. doi: 10.1016/j.plantsci.2022.111305
Li, H., Liu, S. S., Yi, C. Y., Wang, F., Zhou, J., Xia, X. J., et al. (2014). Hydrogen peroxide mediates abscisic acid-induced HSP70 accumulation and heat tolerance in grafted cucumber plants. Plant Cell Environ. 37, 2768–2780. doi: 10.1111/pce.12360
Li, Z., Su, D., Lei, B., Wang, F., Geng, W., Pan, G., et al. (2015). Transcriptional profile of genes involved in ascorbate glutathione cycle in senescing leaves for an early senescence leaf (esl) rice mutant. J. Plant Physiol. 176, 1–15. doi: 10.1016/j.jplph.2014.09.020
Liu, J., Hasanuzzaman, M., Wen, H., Zhang, J., Peng, T., Sun, H., et al. (2019). High temperature and drought stress cause abscisic acid and reactive oxygen species accumulation and suppress seed germination growth in rice. Protoplasma 256, 1217–1227. doi: 10.1007/s00709-019-01354-6
Liu, J., Sun, X., Xu, F., Zhang, Y., Zhang, Q., Miao, R., et al. (2018). Suppression of OsMDHAR4 enhances heat tolerance by mediating H2O2-induced stomatal closure in rice plants. Rice 11, 38. doi: 10.1186/s12284-018-0230-5
Liu, J., Zhang, C., Wei, C., Liu, X., Wang, M., Yu, F., et al. (2016). The RING finger ubiquitin E3 ligase OsHTAS enhances heat tolerance by promoting H2O2-induced stomatal closure in rice. Plant Physiol. 170, 429–443. doi: 10.1104/pp.15.00879
Lv, J., Dong, T., Zhang, Y., Ku, Y., Zheng, T., Jia, H., et al. (2022). Metabolomic profiling of brassinolide and abscisic acid in response to high-temperature stress. Plant Cell Rep. 41, 935–946. doi: 10.21203/rs.3.rs-1060645/v1
Ma, H., Liu, C., Li, Z., Ran, Q., Xie, G., Wang, B., et al. (2018). ZmbZIP4 contributes to stress resistance in maize by regulating ABA synthesis and root development. J. Plant Physiol. 178, 753–770. doi: 10.1104/pp.18.00436
Nambara, E., Marion-Poll, A. (2005). Abscisic acid biosynthesis and catabolism. Annu. Rev. Plant Biol. 56, 165–185. doi: 10.1146/annurev.arplant.56.032604.144046
Pei, Z. M., Murata, Y., Benning, G., Thomine, S., Klüsener, B., Allen, G. J., et al. (2000). Calcium channels activated by hydrogen peroxide mediate abscisic acid signalling in guard cells. Nature 406, 731–734. doi: 10.1038/35021067
Qin-Di, D., Gui-Hua, J., Xiu-Neng, W., Zun-Guang, M., Qing-Yong, P., Shiyun, C., et al. (2021). High temperature-mediated disturbance of carbohydrate metabolism and gene expressional regulation in rice: a review. Plant Signal. Behav. 16, 1862564. doi: 10.1080/15592324.2020.1862564
Quan, L. J., Zhang, B., Shi, W. W., Li, H. Y. (2008). Hydrogen peroxide in plants: a versatile molecule of the reactive oxygen species network. J. Integr. Plant Biol. 50, 2–18. doi: 10.1111/j.1744-7909.2007.00599.x
Raja, V., Majeed, U., Kang, H., Andrabi, K. I., John, R. (2017). Abiotic stress: Interplay between ROS, hormones and MAPKs. Environ. Exp. Bot. 137, 142–157. doi: 10.1016/j.envexpbot.2017.02.010
Rezaul, I. M., Baohua, F., Tingting, C., Weimeng, F., Caixia, Z., Longxing, T., et al. (2019). Abscisic acid prevents pollen abortion under high-temperature stress by mediating sugar metabolism in rice spikelets. Physiol. Plant 165, 644–663. doi: 10.1111/ppl.12759
Shi, B., Ni, L., Zhang, A., Cao, J., Zhang, H., Qin, T., et al. (2012). OsDMI3 is a novel component of abscisic acid signaling in the induction of antioxidant defense in leaves of rice. Mol. Plant 5, 1359–1374. doi: 10.1093/mp/sss068
Singh, R. K., Jaishankar, J., Muthamilarasan, M., Shweta, S., Dangi, A., Prasad, M. (2016). Genome-wide analysis of heat shock proteins in C4 model, foxtail millet identifies potential candidates for crop improvement under abiotic stress. Sci. Rep. 6, 32641. doi: 10.1038/srep32641
Song, S., Dai, X., Zhang, W. H. (2012). A rice f-box gene, OsFbx352, is involved in glucose-delayed seed germination in rice. J. Exp. Bot. 63, 5559–5568. doi: 10.1093/jxb/ers206
Sun, K., Wang, H., Xia, Z. (2019). The maize bHLH transcription factor bHLH105 confers manganese tolerance in transgenic tobacco. Plant Sci. 280, 97–109. doi: 10.1016/j.plantsci.2018.11.006
Tang, R.-S., Zheng, J.-C., Jin, Z.-Q., Zhang, D.-D., Huang, Y.-H., Chen, L.-G. (2007). Possible correlation between high temperature-induced floret sterility and endogenous levels of IAA, GAs and ABA in rice (Oryza sativa l.). Plant Growth Regul. 54, 37–43. doi: 10.1007/s10725-007-9225-8
Teng, K., Li, J., Liu, L., Han, Y., Du, Y., Zhang, J., et al. (2014). Exogenous ABA induces drought tolerance in upland rice: the role of chloroplast and ABA biosynthesis-related gene expression on photosystem II during PEG stress. Acta Physiol. Plant 36, 2219–2227. doi: 10.1007/s11738-014-1599-4
Toh, S., Imamura, A., Watanabe, A., Nakabayashi, K., Okamoto, M., Jikumaru, Y., et al. (2008). High temperature-induced abscisic acid biosynthesis and its role in the inhibition of gibberellin action in Arabidopsis seeds. Plant Physiol. 146, 1368–1385. doi: 10.1104/pp.107.113738
Vanderauwera, S., Suzuki, N., Miller, G., van de Cotte, B., Morsa, S., Ravanat, J. L., et al. (2011). Extranuclear protection of chromosomal DNA from oxidative stress. Proc. Natl. Acad. Sci. U. S. A. 108, 1711–1716. doi: 10.1073/pnas.1018359108
Wang, Y., Hou, Y., Qiu, J., Wang, H., Wang, S., Tang, L., et al. (2020). Abscisic acid promotes jasmonic acid biosynthesis via a 'SAPK10-bZIP72-AOC' pathway to synergistically inhibit seed germination in rice (Oryza sativa). New Phytol. 228, 1336–1353. doi: 10.1111/nph.16774
Wang, F., Liu, J., Zhou, L., Pan, G., Li, Z., Zaidi, S. H., et al. (2016). Senescence-specific change in ROS scavenging enzyme activities and regulation of various SOD isozymes to ROS levels in psf mutant rice leaves. Plant Physiol. Biochem. 109, 248–261. doi: 10.1016/j.plaphy.2016.10.005
Wang, F., Niu, H., Xin, D., Long, Y., Wang, G., Liu, Z., et al. (2021). OsIAA18, an Aux/IAA transcription factor gene, is involved in salt and drought tolerance in rice. Front. Plant Sci. 12. doi: 10.3389/fpls.2021.738660
Wan, X. R., Li, L. (2006). Regulation of ABA level and water-stress tolerance of Arabidopsis by ectopic expression of a peanut 9-cis-epoxycarotenoid dioxygenase gene. Biochem. Biophys. Res. Commun. 347, 1030–1038. doi: 10.1016/j.bbrc.2006.07.026
Xiao, B., Huang, Y., Tang, N., Xiong, L. (2007). Over-expression of a LEA gene in rice improves drought resistance under the field conditions. Theor. Appl. Genet. 115, 35–46. doi: 10.1007/s00122-007-0538-9
Xu, Y., Zhang, L., Ou, S., Wang, R., Wang, Y., Chu, C., et al. (2020). Natural variations of SLG1 confer high-temperature tolerance in indica rice. Nat. Commun. 11, 5441. doi: 10.1038/s41467-020-19320-9
Yang, X., Yang, Y. N., Xue, L. J., Zou, M. J., Liu, J. Y., Chen, F., et al. (2011). Rice ABI5-Like1 regulates abscisic acid and auxin responses by affecting the expression of ABRE-containing genes. Plant Physiol. 156, 1397–1409. doi: 10.1104/pp.111.173427
Ye, N., Zhu, G., Liu, Y., Li, Y., Zhang, J. (2011). ABA controls H2O2 accumulation through the induction of OsCATB in rice leaves under water stress. Plant Cell Physiol. 52, 689–698. doi: 10.1093/pcp/pcr028
Zhang, Q., Liu, Y., Jiang, Y., Li, A., Cheng, B., Wu, J. (2022). OsASR6 enhances salt stress tolerance in rice. Int. J. Mol. Sci. 23, 9340. doi: 10.3390/ijms23169340
Zhang, X. X., Tang, Y. J., Ma, Q. B., Yang, C. Y., Mu, Y. H., Suo, H. C., et al. (2013). OsDREB2A, a rice transcription factor, significantly affects salt tolerance in transgenic soybean. PLos One 8, e83011. doi: 10.1371/journal.pone.0083011
Zhang, W., Yang, H., You, S., Xu, Y., Ran, K., Fan, S. (2014). Cloning, characterization and functional analysis of the role MhNCED3, a gene encoding 9-cis-epoxycarotenoid dioxygenase in Malus hupehensis rehd., plays in plant tolerance to osmotic and Cd2+ stresses. Plant Soil 381, 143–160. doi: 10.1007/s11104-014-2120-y
Zhang, D. P., Zhou, Y., Yin, J. F., Yan, X. J., Lin, S., Xu, W. F., et al. (2015). Rice G-protein subunits qPE9-1 and RGB1 play distinct roles in abscisic acid responses and drought adaptation. J. Exp. Bot. 66, 6371–6384. doi: 10.1093/jxb/erv350
Zhao, C., Liu, B., Piao, S., Wang, X., Lobell, D. B., Huang, Y., et al. (2017). Temperature increase reduces global yields of major crops in four independent estimates. Proc. Natl. Acad. Sci. U. S. A. 114, 9326–9331. doi: 10.1073/pnas.1701762114
Zhou, H., Wang, Y., Zhang, Y., Xiao, Y., Liu, X., Deng, H., et al. (2022). Comparative analysis of heat-tolerant and heat-susceptible rice highlights the role of OsNCED1 gene in heat stress tolerance. Plants 11, 1062. doi: 10.3390/plants11081062
Zhu, G., Ye, N., Zhang, J. (2009). Glucose-induced delay of seed germination in rice is mediated by the suppression of ABA catabolism rather than an enhancement of ABA biosynthesis. Plant Cell Physiol. 50, 644–651. doi: 10.1093/pcp/pcp022
Keywords: rice, seedling, heat stress, ABA, OsNCED1
Citation: Zhang Y, Liu X, Su R, Xiao Y, Deng H, Lu X, Wang F, Chen G, Tang W and Zhang G (2022) 9-cis-epoxycarotenoid dioxygenase 1 confers heat stress tolerance in rice seedling plants. Front. Plant Sci. 13:1092630. doi: 10.3389/fpls.2022.1092630
Received: 08 November 2022; Accepted: 05 December 2022;
Published: 20 December 2022.
Edited by:
Milan Skalicky, Czech University of Life Sciences Prague, CzechiaReviewed by:
Guanfu Fu, China National Rice Research Institute (CAAS), ChinaXuwu Sun, Henan University, China
Copyright © 2022 Zhang, Liu, Su, Xiao, Deng, Lu, Wang, Chen, Tang and Zhang. This is an open-access article distributed under the terms of the Creative Commons Attribution License (CC BY). The use, distribution or reproduction in other forums is permitted, provided the original author(s) and the copyright owner(s) are credited and that the original publication in this journal is cited, in accordance with accepted academic practice. No use, distribution or reproduction is permitted which does not comply with these terms.
*Correspondence: Wenbang Tang, tangwenbang@163.com; Guilian Zhang, zgl604@163.com
†These authors have contributed equally to this work