- College of Life Sciences, Yantai University, Yantai, China
Double fertilization is a unique and particularly complicated process for the generation alternation of angiosperms. Sperm cells of angiosperms lose the motility compared with that of gymnosperms. The sperm cells are passively carried and transported by the pollen tube for a long journey before targeting the ovule. Two sperm cells are released at the cleft between the egg and the central cell and fused with two female gametes to produce a zygote and endosperm, respectively, to accomplish the so-called double fertilization process. In this process, extensive communication and interaction occur between the male (pollen or pollen tube) and the female (ovule). It is suggested that small peptides and receptor kinases play critical roles in orchestrating this cell-cell communication. Here, we illuminate the understanding of phases in the process, such as pollen-stigma recognition, the hydration and germination of pollen grains, the growth, guidance, and rupture of tubes, the release of sperm cells, and the fusion of gametes, by reviewing increasing data recently. The roles of peptides and receptor kinases in signaling mechanisms underlying cell-cell communication were focused on, and directions of future studies were perspected in this review.
Introduction
The life cycle alternates between the diploid sporophytic generation and the haploid gametophytic generation in flowering plants. Meiosis and double fertilization are indispensable and crucial programs for the alternation of generations (Hater et al., 2020; Hafidh and Honys, 2021). The mature gametophyte, the pollen grain, is a cell-in-cell structure, which means that two small sperm cells are embedded in the cytoplasm of a big vegetative cell. The polygonum-type embryo sac is found in the majority of flowering plants. It is a polarized structure with two synergids, one egg, one central cell that contains two nuclei, and three antipodals arranged from the micropyle to the chalazal end. In contrast to animal sperms, the immobile sperm cells of angiosperms are transmitted passively for long distances by pollen tubes that target the female gametes for double fertilization (Bleckmann et al., 2014). Germination and the subsequent elongation of the pollen tubes for transmission of sperm cells are initiated as the recognition of compatible grains and papillar cells. Pollen tubes penetrate the transmitting tract and are guided to the funiculus, then the micropyle of the ovule. The sperm cells will be released when the tube enters the embryo sac through the micropyle (Dresselhaus and Franklin-Tong, 2013). Complicated and extensive communication or interaction is involved during the process mentioned above. In the guiding signaling network, it is indicated that small peptide ligands and receptor kinases play essential roles (Chevalier et al., 2011; Kawashima and Berger, 2011; Dresselhaus et al., 2016; Zhou and Dresselhaus, 2019; Hafidh and Honys, 2021; Kim et al., 2021).
The plant receptor-like kinases (RLKs) are classified as the largest RLKs/Pelle family, with more than 610 and 1130 members in Arabidopsis thaliana and Oryza sativa L., respectively (Shiu et al., 2004). RLKs are characterized into various subfamilies according to the different extracellular domains (ECDs), including Leucine-rich repeat RLKs, lysine motifs (LysM) RLKs (Franck et al., 2018a; Yu et al., 2021a), wall-associated kinases (WAKs), lectin RLKs, proline-rich receptor kinases (PERK), and others. There is also a class of co-receptors that are featured with basic structures similar to that of receptor kinases and facilitate the recognition of ligands (Kirkbride et al., 2005; Hohmann et al., 2017). Small peptides act as signals to bind to the ECDs of receptor kinases/co-receptors to induce multimerization and trans-phosphorylation, and further activate downstream signaling cascades. Currently, most of the small peptide signals identified in plant reproduction are small cysteine-rich peptides, defensin-like peptides, or rapid alkalinization factors (RALFs) (Okuda et al., 2009; Amien et al., 2010; Takeuchi and Higashiyama, 2012; Kanaoka, 2018; Somoza et al., 2021). Due to the remarkable diversity of peptide/receptor/co-receptor pairs, this communicating mechanism of peptide-receptor interaction is fundamental to many signaling pathways.
The communication of pollen-pistil during fertilization is involved in the five stages mentioned above. The signaling pathways orchestrated by peptide/receptor kinases in angiosperm fertilization are reviewed and discussed here. Peptides, receptors, and their functions are summarized in Table 1.
Recognition of pollen and stigma
The first checkpoint for plant fertilization is at the beginning of pollen-papilla interaction. The mature pollen grains (male gametophytes) land on the stigmatic papillae at the top of the pistil. The male and female are adhered to and recognized by each other. In self-incompatible plants, only the compatible grains can proceed with the subsequent hydration and germination (Rozier et al., 2020). The alien pollens from unrelated species or the self-incompatible (SI) ones are ineffective. In about half of the species in flowering plants, self-incompatibility and cross-pollination are applied to maintain genetic diversity within species populations (Murase et al., 2004; Zhang et al., 2021). Accumulating data indicate that this self/non-self-recognition in Brassica is triggered by S-haplotype-specific interaction between the male and female determinants. The S-haplotype consists of two or more closely linked polymorphic S loci. The pistil and pollen will show self-incompatibility given that the same S allele is present in the male and female. Rejection of pollen for the same individual Brassica plant is due to sporophytic self-incompatibility (Jany et al., 2019). The male determinant factor is a small peptide ligand, S-locus cysteine-rich protein/S-locus protein 11 (SCR/SP11), which is secreted from the anther tapetum cells, and diffused to the pollen wall and stored there. The female determinant factor is the S-locus receptor kinase (SRK) localized in the papillar cell membrane (Scandola and Samuel, 2019). The SI response is initiated by the haplotype-specific interaction between SCR/SP11 and SRK, the interaction through which the reciprocal communication between the pollen and papillar cells is regulated (Scandola and Samuel, 2019). SCR/SP11-SRK is involved in the self-incompatibility signal pathway in Brassica, as shown in Figure 1. In the stigma, SRK exists as a preassembled homodimer at the plasma membrane of papillae and remains inactive until pollination. SRK is activated by the S-haplotype-specific SCR/SP11 binding to its ECD and the autophosphorylation of its intracellular kinase. The intracellular serine/threonine M-LOCUS PROTEIN KINASEs (MLPKs) is anchored at the membrane of papillae, and acts as a downstream target for SRK (Murase et al., 2004). THL1 and THL2 are members of the thioredoxin-H family and function as negative effectors (Bower et al., 1996). The stigma-expressed E3 ubiquitin ligase ARMADILLO-REPEAT-CONTAINING1(ARC1) is a positive regulator in the pistil for the rejection of self-incompatible pollen in Brassica napus, Arabidopsis lyrata, and Arabidopsis thaliana (Stone et al., 2003; Goring et al., 2014). All these players mentioned above are involved in the self-incompatibility response.
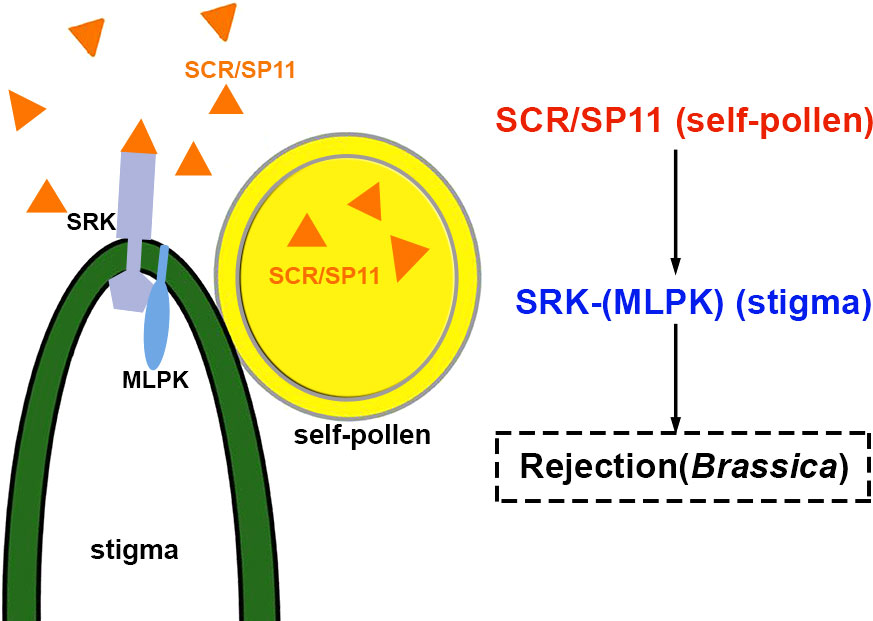
Figure 1 Schematic diagram of SCR/SP11 and SRK grouped for pollen-stigma interactions of Brassica self-incompatibility. The peptide triggering inhibition of signaling pathways is highlighted in red. The blue represents receptor complexes and the co-receptors are shown in parentheses. The source of peptides and receptors is shown in parentheses. Abbreviations for all small peptides and receptors are elucidated in the text.
In Brassica, in the absence of self-pollen, THL1/2 interacts with the intracellular domain of SRK to form a heterodimer that prevents SRK from homodimerization and activation (Bower et al., 1996; Mazzurco et al., 2001). In the presence of self-pollen, SCR/SP11 interacts with the ECD of SRK to promote SRK homodimerization and consequently results in the release of THL1 and THL2 from SRK. The active SRK recruits and phosphorylates MLPK (Murase et al., 2004; Liang and Zhou, 2018). The cytoplasmic kinase MLPK subsequently phosphorylates and activates ARC1 (Stone et al., 2003; Goring et al., 2014) to transduce the SI signaling. Phosphorylated ARC1 mediates ubiquitination and degradation of a series of downstream substrates involved in pollen hydration, exocytosis, and germination. For example, the factor for stigmatic compatibility: Exo70A1, is involved in pollen hydration (Kitashiba et al., 2011; Li et al., 2013; Zhang et al., 2016); the phospholipase D-1 protein (PLD1) plays role in germination (Scandola and Samuel, 2019). The glyoxalase 1 (GLO1) is required to detoxify plants’ methylglyoxal (MG) cytotoxicity. ARC1 was shown to ubiquitinate GLO1 effectively (Sankaranarayanan et al., 2015). In self-incompatible pollination, less GLO1 was found in stigma. It was suggested that the factors involved in compatible reactions were modified and disrupted by the enriched methylglyoxal. Eventually, the ‘self-pollen’ is rejected, and the papillae are degenerated (Sankaranarayanan et al., 2015).
In model plant A. thaliana, the acceptance of self-pollen by the stigma is attributed to the malfunction of self-incompatible genes SCRs and SRKs (Boggs et al., 2009). The Brassica pollen coat protein class B (PCP-B) are small cysteine-rich proteins (CRPs). Both PCP-Bs and SCRs are CRP proteins with eight conserved cysteines. However, it is shown that they belong to different small branches according to the phylogenetic analysis, and they are specialized for compatible pollination and self-incompatibility, respectively (Wang et al., 2017). In A. thaliana, defective pollen hydration and delay of tube growth are observed due to the disruption of genes for PCP-Bs (Wang et al., 2017). PCP-Bs serve as male factors for molecular dialogues of stigma—pollen to orchestrate pollen hydration, adhesion, and pollen tube growth (Wang et al., 2017). The reduction of reactive oxygen species (ROS) in papillar cells is closely correlated with the hydration and adhesion of pollen, especially at the adhesion sites (McInnis et al., 2006). When landed on the wild-type stigma, pollen from pcp-bγ and pcp-bβ/γ mutants showed markedly slow hydration because of severe suppression of the ROS reduction, in contrast to the response of wild-type pollen (Liu et al., 2021a). Wild-type stigmas treated with mature peptide PCP-Bγ exhibited a dose dependence on ROS reduction (Liu et al., 2021a). Fed with ROS inhibitor and ROS scavengers, the pistil with reduced ROS showed a significant acceleration of pollen hydration 10 minutes after pollination (Liu et al., 2021a).
The well-known receptor kinase FERONIA (FER) is expressed in various tissues and plays diverse essential roles (Escobar-Restrepo et al., 2007; Deslauriers and Larsen, 2010; Duan et al., 2010; Stegmann et al., 2017). In Arabidopsis, both receptor kinases ANJEA (ANJ) (Galindo-Trigo et al., 2020) and FER belong to Catharanthus roseus receptor-like kinase 1–like (CrRLK1L) family and are strongly expressed in stigma. The ANJ-FER receptor complexes act as essential female factors. They are involved in pollen—papilla communication by interacting with PCP-Bs for pollen hydration and germination (Liu et al., 2021a). When pollinated with wild-type pollen, rapid hydration phenotype was observed on the mutants pistil of fer-4, anj-1, and fer-4 anj-1, which was opposite to that of pcp-bs mutants. The phenotype was rescued in the complementation test (McInnis et al., 2006; Liu et al., 2021a).
The autocrine RALF23/33 peptides interact with the ECDs of FER and ANJ in stigma. Mutant ralf33 showed a similar phenotype to fer-4 and anj-1 at reduced ROS accumulation in stigmatic papillae and accelerated pollen hydration (Liu et al., 2021a). LORELEI-like-GPI-anchored proteins (LLG)1, the co-receptor of FER-ANJ, is one of the members of the LORELEI (LRE) family (Feng et al., 2019). Loss of LLG1 function also resulted in reduced ROS accumulation in papillae. The guanine nucleotide exchange factors (GEFs) of Rho-like GTPases (RAC/ROP) and NADPH oxidases (RESPIRATORY BURST OXIDASE HOMOLOG D, RBOHD) act downstream of FER-ANJ-LLG1 to regulate ROS generation. Data from ROS-producing mutants suggested that autocrine RALF23/33 induces ROS generation through the ROP2-RBOHD pathway (Liu et al., 2021a).
The dissociation constants (Kd) of PCP-Bγ and RALF33 binding FER ectodomain (FERecd) were 0.34 μM and 0.1604 μM, respectively (Liu et al., 2021a). It means that RALF33 has a greater affinity for FERecd than PCP-Bγ does. However, the inhibition constant (Ki) that PCP-Bγ competed to bind FERecd in the mixture of RALF33-FERecd was 2.5099 μM (Liu et al., 2021a). It seems that the results are somewhat contradictory. Without compatible pollen, RALF23 and RALF33 are secreted from stigmatic papillary cells and facilitate ANJ-FER and its co-receptor LLG1 to form polymer complexes that stimulate ROP2 to trigger RBOHD activity, which leads to maintaining the high level of ROS in papillae before pollination. (Liu et al., 2021a; Kou et al., 2022). It was proposed that after the landing of compatible pollen grains on the stigma, the pollen coat protein PCP-Bs competed with and replaced RALF23/33 to interact with the ANJ-FER receptor complexes in papilla cells. Consequently, the RALF23/33-initiated ROS signaling pathway was shut down. The ROS decreased rapidly in papillae to promote the hydration and germination of pollen grains. The maintenance of ROS content in papillar cells might be regulated through distinct signaling pathways stimulated by autocrine and paracrine peptides. The model is shown in Figure 2. These speculations need to be supported and verified by further experimental data.
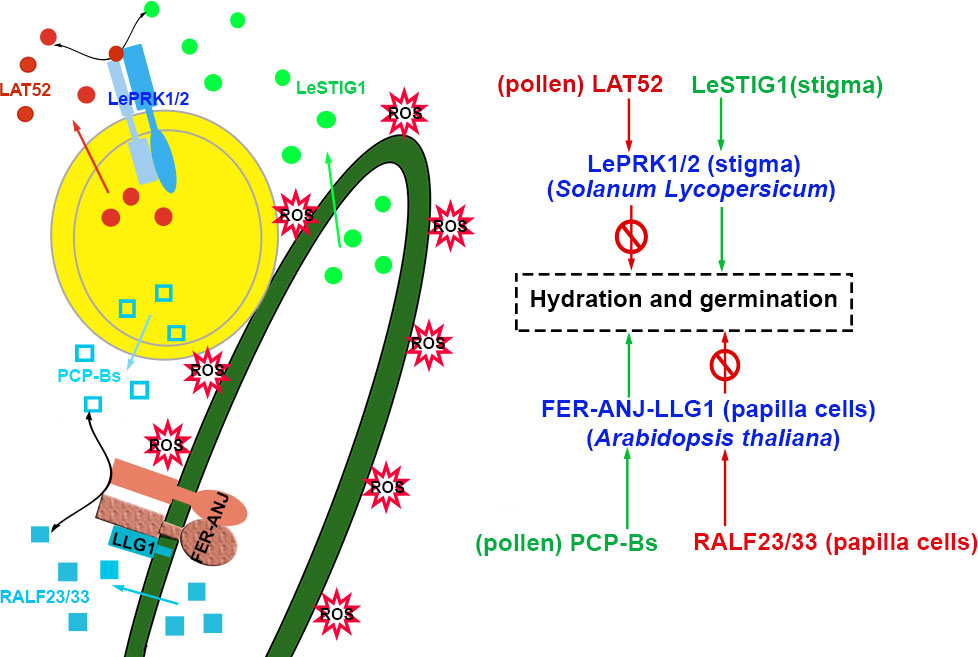
Figure 2 Pollen and stigma recognize each other to initiate the hydration and germination of pollen. In Solanum Lycopersicum, LAT52 interacts with LePRK1/2 to inhibit hydration and germination, and LeSTIG1 interacts with LePRK1/2 to facilitate hydration and germination. However, in Arabidopsis, pollen-specific PCP-Bs bind to FER/ANJ-LLG1 to promote hydration and germination, and stigmatic RALF23/33 interacts with FER/ANJ-LLG1 to maintain ROS accumulation and repress hydration of pollen. The peptides involved in the activation and inhibition of signaling pathways are highlighted in green and red, respectively. The receptor complexes are marked in blue and the co-receptors are shown in parentheses. The source of peptides and receptors is shown in parentheses. Abbreviations for all small peptides and receptors are elucidated in the text.
Coincidentally, ligands exchange also occurs in the process of pollen growth and germination in tomato. The signaling pathway is triggered by receptor kinases at the pollen surface but not from female tissue. In Solanum Lycopersicum, cysteine-rich late-anther tomato 52 (LAT52), the male player, interacts with pollen-specific receptor kinase 2 (LePRK2) and prevents pollen from germination before pollination (Tang et al., 2002; Johnson and Preuss, 2003). After germination, another cysteine-rich peptide, stigma-specific protein 1 (LeSTIG1), is secreted and matured to a 7-kD peptide as the female player. LeSTIG1 accumulates on the pollen tube surface and binds the LePRK1/2 receptor complexes to promote pollen tube growth (Tang et al., 2004; Zhang et al., 2008; Huang et al., 2014). The ligand of the LePRK1/2 complexes, LAT52, is substituted by LeSTIG1. The signals could be switched from repression to activation of germination and tube growth, as shown in Figure 2 (Tang et al., 2004). LeSTIG1-LePRK2 orchestrates ROS levels through phosphatidylinositol 3-phosphate PI(3)P signaling to promote pollen tube growth (Huang et al., 2014). The Kinase partner protein (KPP) of S. lycopersicum is a Rop-GEF that interacts with the cytoplasmic kinase domains of LePRK1 and LePRK2 through its C-terminus. It recruits Actin-Related Protein 2/3 (ARP2/3, actin nucleators) complexes to the membrane of the pollen tube tip and coordinates actin and cytoskeleton to enhance tube growth (Huang et al., 2014; Liu et al., 2020). It may also be in an essential manner through which tomato LeSTIG1 regulates the growth of pollen tubes in the transmitting tract. It is required to investigate whether LeSTIG1 is strongly expressed and localized in the stylar transmitting tract.
Pollen tubes grow in the transmitting tract
In Rosaceae’s Gametophytic Self-Incompatibility (GSI) pollination, the pollen germinates and grows on the stigma. The growth of pollen tube arrests at one-third distance through the stylar transmitting tract (Franklin-Tong and Franklin, 2003). The GSI of Rosaceae is jointly regulated by the products of S locus, S-RNase of pistil determinants and S-haplotype specific F-box proteins/S locus F-box brothers (SFB/SFBB) of pollen determinant (Sassa, 2016; Sun et al., 2018; Matsumoto and Tao, 2019). In the transmitting tract, pistil S-RNases penetrate the pollen tube to interact with the male determinants. Pollen SFB/SFBB recognizes each a subset of non-self S-RNases, ubiquitinates them, and mediates their degradation by 26S proteasome (Entani et al., 2014). Self-S-RNase disrupted tip-localized ROS and decreased the Ca2+ current, inducing nuclear DNA degradation and pollen tube disintegration (Wang and Kao, 2012; Del Duca et al., 2019).
The compatible pollen germinates, penetrates the papillae (Iwano et al., 2014), and continues to grow through the transmitting tract. The pollen tubes elongate considerably in style, and their integrity is maintained to prevent premature rupture. There are at least two signaling networks for the integrity of the pollen tube in the transmitting tract and the burst upon arrival at its destination.
RALF is a class of small peptide ligands widely known in various signaling pathways. The pollen tube-specific tomato (S. lycopersicum) RALF (SlPRALF) small peptides are responsible for pollen tube growth in different developmental windows (Covey et al., 2010). Eight pollen specifically expressed RALFs are clustered into three phylogenetic clades among the 37 RALFs in A. thaliana. RALF4 and RALF19 are grouped into one clade. The pollen tube of the homozygous and pollen-specific-promoter-amiRRALF4/19 transgenic plants fails to grow in the transmitting tract and cannot reach the ovule in vivo (Mecchia et al., 2017). Nearly 70% of pollen tubes from the amiRRALF4/19 plants burst in vitro germination of pollen tube assay (Mecchia et al., 2017). In the ralf4-1mutant, fertilization could occur, but 47% of pollen tubes burst in vitro germination (Mecchia et al., 2017). It is suggested that RALF4/19 plays a crucial and redundant role for pollen tube integrity and growth. Disruption of ANXUR1(ANX) and ANXUR2, the closest members to FERONIA in the CrRLK1L subfamily, phenocopies amiRRALF4/19 lines in pollen tube dehiscence and elongation repression (Boisson-Dernier et al., 2009; Miyazaki et al., 2009). The rupture of tubes in amiRRALF4/19 cannot be rescued with overexpression of ANX1/2 (Mecchia et al., 2017). It is indicated that ANX1/2 is the downstream receptor of RALFs, which might coordinate other components to regulate tube integrity.
Meanwhile, Mecchia et al., 2017 found that premature tube rupture resulted from the repression of pollen-specific ANX1 and ANX2 in vitro germination assay. A similar phenotype was also observed when BUDDHA’S PAPER SEAL (BUPS) 1/2 was knocked out (Ge et al., 2017). The T-DNA or knock-down mutant of bups1 showed apical rupture after exiting the style and cessation of growth at the apex of the transmission tract (Ge et al., 2017). It is demonstrated that the mutants failed to maintain the integrity of the pollen tube. BUPS1 is involved in the perception and response to mechanical stress when tubes pass through style. It is suggested that BUPS1 activates ROP1 GTPase signals directly to promote exocytosis that accelerates BUPS1-dependent secretion of RALF4 (Ge et al., 2019; Kou et al., 2022). RALF4, acting as a cognate ligand for BUPS1, activates BUPS1 to amplify signaling and strengthen the rigidification of the cell wall (Zhou et al., 2021). It is proposed that these four receptor kinases are involved in maintaining pollen tube integrity in Arabidopsis.
The pollen-tube-expressed glycosylphosphatidylinositol-anchored proteins (GPI-APs), LORELEI-like-GPI-anchored protein 2/3 (LLG2/3), are engaged in maintaining tube integrity. Early rupture of pollen tubes and severe fertility defects result from LLG2/3 disruption (Ge et al., 2019). LLG2/3 interacts with the extracellular domains of BUPS1/2 and ANX1/2 in vitro pull-down assay, and the interaction is enhanced by RALF4/19 (Ge et al., 2019). It is suggested that LLG2 and LLG3 act as co-receptors of the BUPSs/ANXs to regulate pollen tube integrity. RALF4 interacts with LLG3 and BUPSs/ANXs via different N-terminal domains. The N-terminal YISY motif of RALF4 is independent of the interaction but responsible for pollen tube integrity (Ge et al., 2019). It means that other proteins or receptors may interact with the N-terminal YISY domain of RALF4, which plays an essential role in pollen tube integrity in Arabidopsis.
LRX (LEUCINE-RICH REPEAT EXTENSIN) is the partner of RALFs in tomato. The pollen-specific LRX8/9/10/11 regulates the integrity of pollen tubes in Arabidopsis (Mecchia et al., 2017; Fabrice et al., 2018). LRXs play critical roles in maintaining cell wall rigidity during pollen tube growth and play essential roles in physical links between the intra- and extracellular components. The lrx multiple mutants showed premature rupture of pollen tubes and sterility to different extents (Mecchia et al., 2017). This phenotype was similar to that of mutants with the disruption of BUPSs and ANXs, and could not respond to the RALF4-triggered pollen tube growth. LRXs are linked through disulfide bonds to form dimers, and their N-terminal region physically interacts with RALF4 (Mecchia et al., 2017; Fabrice et al., 2018). RALF4/19 directly interacted with ANX1/2-BUPS1/2 to orchestrate the growth and integrity of pollen tubes (Ge et al., 2017). In addition, the N-terminal domains of LRX1/2/4/5 were discovered to interact with FER in vegetative tissues physically (Herger et al., 2020). It is very significant to explore whether LRX8/9/10/11 interacts with the ANX1/2-BUPS1/2 complexes in maintaining the integrity of pollen tubes. It is unknown whether LRXs interact with RALF4 through the N-terminal YISY motif of RALF4.
The coordination between RALF4-LRX8 and RALF4/19-ANX1/2-BUPS1/2 was identified via the downstream factors of the signaling cascade. The mri-3D was obtained in the anx1anx2 mutagenesis screening for repression of pollen tube rupture (Boisson-Dernier et al., 2015). Due to the R240C nonsynonymous replacement in the kinase activation loop of MARIS (MRI) resulting in excessive kinase activity, mri-3D showed the opposite phenotype. MRI is a member of the receptor-like cytoplasmic kinases (RLCKs) localized at the plasma membrane. MRI functions as the downstream player of ANX1/ANX2 to maintain the integrity of pollen tubes (Boisson-Dernier et al., 2015). ANX1/2 acts upstream of NADPH oxidase (RESPIRATORY BURST OXIDASE HOMOLOG H/J, RBOHH/RBOHJ) of the pollen tube membrane and affects the matrix exocytosis of cell wall for the tube integrity. NADPH oxidase generates tip-accumulated ROS to maintain the calcium gradient at the apex, ultimately regulating the growth and integrity of pollen tubes (Boisson-Dernier et al., 2013). MRIR240C displayed hyperactive and could partially rescue the phenotype of pollen tubes burst of anx1anx2 (Boisson-Dernier et al., 2015). It is proposed that MRI might function downstream of RBOHH/J. It is shown that both ANX1 and MRI cannot rescue the rupture of pollen tubes of amiRRALF4/19 when ANX1-YFP and MRI-YFP are exclusively expressed in pollen tubes (Mecchia et al., 2017). Therefore, it is proved that two parallel signaling pathways are activated by the cysteine-rich peptides RALF4/19 that are secreted by pollen tubes.
The protein phosphatase ATUNIS1/2 (AUN1/2) is discovered to act downstream of ANX1/2 via screening for suppression to untimely rupture of the anx1anx2 pollen tube (Franck et al., 2018b). However, it is the activity of AUN1D94N, but not the expression of MRIR240C, sufficient to attenuate male sterility and pollen burst phenotype in lrx8-11 quadruple mutants (Franck et al., 2018b). It is suggested that AUN1/2 phosphatases may equilibrate MRI kinase activity and regulate the phosphorylation status of target substrates for the growth and integrity of the tubes (Vogler et al., 2019).
Therefore, the integrity of pollen tubes is regulated jointly by parallel pathways, RALF4/19-LRX8/9/10/11-AUN1/2 and RALF4/19-ANXs/BUPSs-MRI signaling pathway, as shown in Figure 3. The phosphorylation status of downstream substrates is equilibrated and maintained by negative regulator AUN1/2 via the RALF4/19-LRX8/9/10/11-AUN1/2 signaling pathway. The positive effector MRI facilitates the phosphorylation of downstream targets by RALF4/19-ANXs/BUPSs-MRI pathway (Ge et al., 2017; Mecchia et al., 2017). However, RALF4/19 is allocated by an unknown mechanism. As mentioned previously, BUPS1-activated ROP1 promotes BUPS1-dependent secretion of RALF4. It was suggested that RALF4/19 triggered ANX1/2-BUPS1/2-MRI, and activated the kinase activity of MRI. Simultaneously, RALF4/19 activated LRX8/9/10/11-AUX1/2 signaling pathway, exerting the phosphatase activity of AUX1/2 to balance MRI kinase activity. These two pathways jointly coordinated and regulated tube growth and integrity.
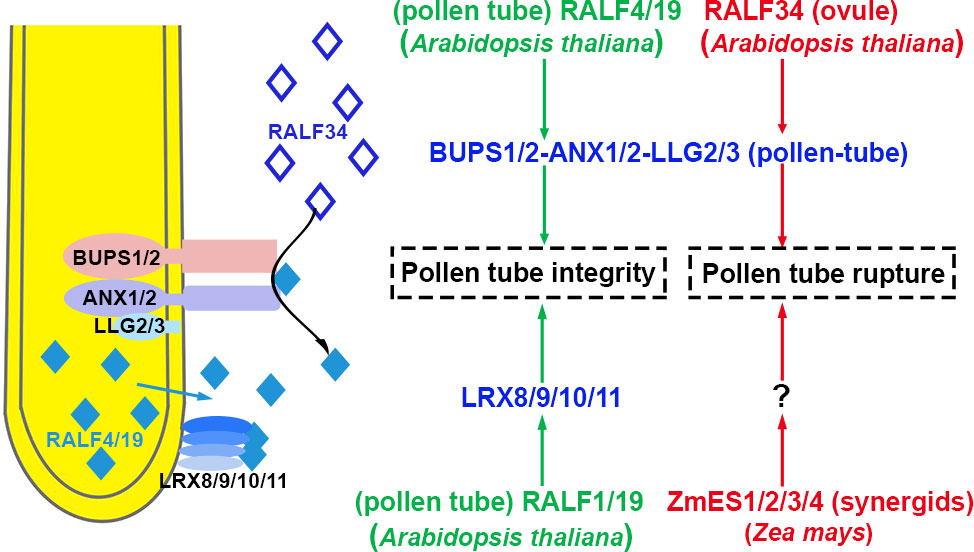
Figure 3 The integrity of pollen tube growth is maintained, and pollen tubes rupture In Arabidopsis thaliana, pollen-specific RALF4/19 orchestrates pollen tube receptor complexes BUPS1/2-ANX1/2-LLG2/3 and LRX8/9/10/11 to maintain pollen tube growth and integrity in the transmitting tract. After the pollen tube enters the ovule, the ovule-specific RALF34 interacts with BUPS1/2-ANX1/2-LLG2/3, resulting in pollen tube rupture. In Zea Mays, ZmES1/2/3/4 secreted from synergid cells causes pollen tube rupture. The peptides involved in the activation and inhibition of signaling pathways are highlighted in green and red, respectively. The blue represents receptor complexes and the co-receptors are shown in parentheses. Question marks indicate unidentified signal components. The source of peptides and receptors is shown in parentheses. Abbreviations for all small peptides and receptors are elucidated in the text.
It is suggested that the abundant extracellular matrix protein, arabinogalactan protein AGP is involved in tube elongation in the transmitting tract (Leszczuk et al., 2019). AGP is a hydroxyproline-rich glycoprotein that can adhere to the surface of the pollen tube. AGP can be integrated into the cell wall matrix of the pollen tubes and decrease after hydrolyzation by deglycosylase (Coimbra et al., 2008; Jia et al., 2015). Continuous adhesion and integration of AGP are necessary to promote tube elongation. The slow growth of pollen tubes resulted from decreased AGPs in the surface of pollen tubes from Arabidopsis agp6 agp11 mutants (Levitin et al., 2008; Costa et al., 2013). In addition, the growth and elongation of pollen tubes are also associated with the glutamate-derived signaling molecule GABA (Ramesh et al., 2015). Low-concentration GABA stimulates pollen tube growth in vitro; however, gradually increasing GABA is required for tube guidance in the transmission tract (Ma, 2003; Palanivelu et al., 2003). The role of AGP and GABA, especially AGP, as physiological signals still needs to be determined.
Pollen tube guidance and polytubey block
Chemoattractants emitted by the ovule (especially synergids) direct the growth of the pollen tube along the funiculus towards the micropyle. In Torenia fournieri (Linden. ex Fourn), four cells in a mature embryo sac, including a central cell, an egg cell, and two synergid cells, were ablated individually or in groups. The capability of pollen tube attraction is lost in the ovule with two ablated synergids (Higashiyama et al., 2001). It indicates that the synergid cell produces signals to attract the pollen tube growth toward the ovule. The synergid cells from T. fournieri were isolated and studied to identify the chemoattractant. A class of small cysteine-rich defensin-like peptides, abundantly and predominantly expressed in synergid cells, were determined. Mature peptides of LUREs (LURE1 and LURE2) produced in E. coli showed guiding activity for pollen tube targeting in vitro assay for pollen tube attraction (Okuda et al., 2009). AtLURE1 peptides were secreted merely from synergid cells and diffused along the funicular surface, guiding the growth of pollen tubes to micropyle (Takeuchi and Higashiyama, 2012). The AtLURE1s’ variants were inefficient in reorienting the pollen tube under the semi-in vivo pollen tube attraction assay (Zhong et al., 2019). Mutants of 23 pollen-specific receptor kinases were used to study the attraction capability of the AtLURE1s peptide. Pollen tubes from prk6 ignored attractants and could not be redirected toward AtLRUE1.2 (Takeuchi and Higashiyama, 2016). PRK6 served as a vital receptor for AtLURE1 signals in vitro attraction assay. PRK6-mRuby2 was asymmetrically localized at the surface of pollen tubes, with the attraction of AtLURE1.2. It was suggested that PRK6 acted as the male player to sense AtLURE1s signaling from synergid cells to guide pollen tube growth toward the micropyle (Takeuchi and Higashiyama, 2016).
Lost in Pollen tube guidance 1 and 2 (LIP1 and LIP2) are cytoplasmic receptor kinases localized at the membrane of tube tips through palmitoylation at the N-terminal cysteine site. In vivo, 46% of lip1 lip2 pollen tubes showed guidance defects to the micropyle. The transmitting effciency of male gametes was 43% in lip1 lip2. Thirty percent of lip1 lip2 pollen tubes were insensitive to the female attractant AtLURE1.2 in vitro attraction assay, although 95% of the wild-type pollen tubes were attracted (Liu et al., 2013). LIP1/2 interacted with tip-localized PRK6 and was involved in response to the AtLURE1s (Liu et al., 2013). It was demonstrated that LIP1 and LIP2 were not necessary components of the PRK6 receptor complexes in micropylar guidance signaling pathways (Liu et al., 2013; Takeuchi and Higashiyama, 2016).
Wang et al. reported that LUREs specifically interacted with the ECDs of three receptor kinases, MALE DISCOVERER1(MDIS1), MDIS1-INTERACTING RECEPTORS LIKE KINASE1 (MIK1), and MIK2, to attract pollen tubes in a self-favorite way (Wang et al., 2016). PRK6 contains six LRRs in its ectodomain to form a solenoid structure. AtLURE1s, which act as female participants of pollen tube guidance, bind directly to the juxta-membrane region of PRK6, despite the extracellular LRR domains that interact specifically with ligands (Takeuchi and Higashiyama, 2016). It was proposed that other ligands might interact with the LRR domain of PRK6 to initiate pollen tube guidance. In addition to PRK6, MDIS1/MIK1/MIK2 acted as other receptors for AtLURE1s.
LUREs’ attraction to pollen tubes was based on conspecific preferential principles so that the interspecific genetic isolation and maintaining of species specificity are promoted. Recombinant AtLURE1.3 peptides preferentially attracted A. thaliana but not A. lyrata pollen tubes in vitro (Takeuchi and Higashiyama, 2012; Zhong et al., 2019). Consistently, AtLURE1 expressed in T. fournieri synergids fascinated A.thaliana pollen tubes to T. fournieri ovule (Takeuchi and Higashiyama, 2012). It is suggested that LURE1s are species-specific pollen attractants for interspecies specificity and reproductive isolation. It implies that additional and common pollen-tube attractants might be released from ovules.
In addition, loss-of-function atlure1 septuple mutant and null prk6 showed natural fertility (Zhong et al., 2019). The A. lyrata pollen tubes were attracted to pass through the septum to the micropyles of ovules in null atlure1 pistil. It is proposed that general genus attractants are present in atlure1 ovules. The XIUQIUs, a close relative of AtLURE1 in Brassicaceae, are small cysteine-rich peptides secreted from synergid cells to accumulate near the filiform apparatus. The recombinant XIUQIUs indiscriminately attract the pollen tubes of A.thaliana and A. lyrata under semi-in vivo assay for pollen tube attraction (Zhong et al., 2019). XIUQIUs take roles as female players, and their pollen tube-localized receptors still need to be identified. Compared with non-species-specific XIUQIUs, AtLURE1s-PRK6 and AtLURE1s-MDIS1/MIK1/MIK2 control conspecific pollen tube-preferred signaling pathways at septum and micropyle, as shown in Figure 4 (Liu et al., 2021b).
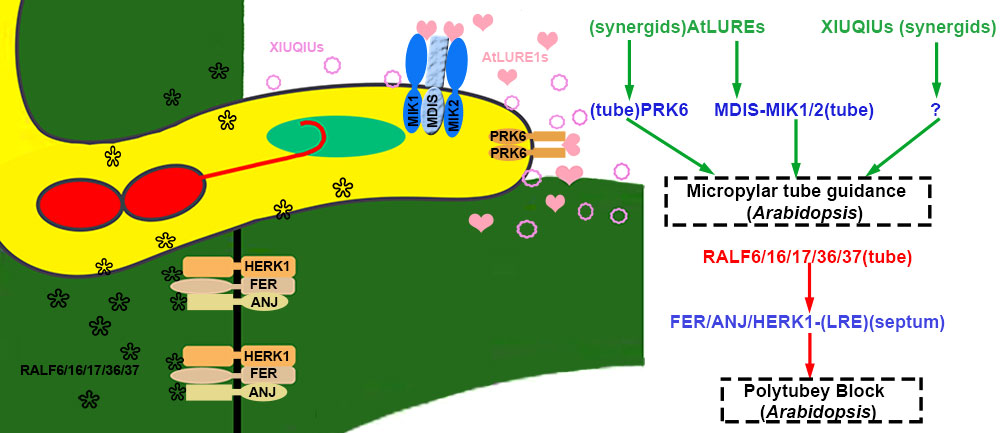
Figure 4 The micropyle guidance and polytubey block of pollen tubes. In Arabidopsis thaliana, pollen tubes penetrate the septum, and the RALF6/16/17/36/37 secreted by the pollen tube interacts with the septum-specific FER/ANJ/HERK1 to initiate the polytubey block. XIUQIUs and AtLURE1s secreted by synergid cells diffuse along the funiculus. AtLURE1s interact with PRK6 and/or MIK1/2-MDIS of the pollen tube to guide the pollen tube to grow towards the micropyle. The peptides are highlighted in green and red to represent activation or suppression, respectively. The blue represents receptor complexes and the co-receptors are shown in parentheses. Question marks indicate unidentified signal components. The source of peptides and receptors is shown in parentheses. Abbreviations for all small peptides and receptors are elucidated in the text.
Besides XIUQIUs and LUREs, there are 73 other CRP proteins expressed exclusively in synergid cells of Arabidopsis, and the roles of these CRPs are still unknown (Takeuchi and Higashiyama, 2012). The mutants showed an abortion rate of 20% in the seed sets after the knockout of all clade genes (CRP810), including XIUQIUs and LUREs (Liu et al., 2021b). It is suggested that attraction for the oriented growth of pollen tubes is lost incompletely. It indicates that there must be additional attractants of pollen tubes in synergids or other female gametophytic cells (Hater et al., 2020).
For instance, the capability of pollen tubes for chemotropic response to LURE is induced and enhanced by methyl-glucuronosyl arabinogalactan (AMOR) in T. fournieri ovules (Mizukami et al., 2016). As tube guiding attractants in maize, ZmEA1 is exclusively expressed in the egg apparatus, subsequently diffused toward the filiform apparatus, and finally localized at the nucellar cell wall below the micropylar end (Marton et al., 2005; Marton et al., 2012). The pollen tube-specific cognate receptor for the ZmEA1 has yet to be discovered. Coincidentally, the glycoproteins secreted into the obturator pore of the apple embryo sac were reported to direct the pollen tube toward the ovule (Malus × domestica) (Losada and Herrero, 2017).
Polytubey block occurs at the micropyle and the pistil’s septum. It is indispensable to ensure that only one conspecific pollen tube passes through the septum and grows along the funiculus to target the micropyle. GENERATIVE CELL SPECIFIC 1 (GCS1)/HAPLESS 2 (HAP2) is specifically expressed in sperm cells and plays a vital role in membrane fusion during double fertilization (Mori et al., 2006; von Besser et al., 2006). In null hap2, the double fertilization failure resulted from impaired membrane fusion. However, fertilization recovery is successful when the second pollen tube comes 7 hours after pollination. However, multiple pollen tubes crossed out of the septum for fertilization recovery since the polytubey block was disrupted in mutants. RNA-seq data from the transmission tract and septum of the pistil indicated that FER, ANJEA (ANJ), and HERCULES RECEPTOR KINASE 1 (HERK1), malectin-like domain-containing receptor-like kinase (MLD-RLK) (also known as C. roseus RLK1-LIKE or CrRLK1L), were highly expressed. Multiple pollen tubes grew along the funiculus 5 hrs after pollination in the pistils of fer-4, anj herk1, or fer anj herk1 (Zhong et al., 2022). These three CrRLK1L receptor kinases functioned as female players to communicate with pollen tube signals. In pollen-specific MYB mutants, myb97myb101myb120, the polytubey phenotype similar to the three null receptors was identified. The expression profiling data demonstrated that five RALFs (RALF6, RALF7, RALF16, RALF36, and RALF 37) were downregulated. Mutants of multiple combinations of RALFs knockout exhibited polytubey when pollinated to wild-type pistil. It is indicated that five RALFs (pollen tube-specific RALF6/7/16/36/37 peptides) function as ligands to be perceived and interact with the FER-ANJ-HERK1 receptor complexes (Zhong et al., 2022). It was speculated that a polyspermy block might be established through RALFs-FER/ANJ/HERK1 at the septum until tubes burst in the ovule after the removal of RALFs signaling as shown in Figures 4, 5. Polyspermy block initiated upon the exit of pollen tubes from the septum and ended up at the vanishing of RALFs signaling after tube discharge. The mechanisms underlying polytubey block might play roles in the recognition between pollen tubes and synergids. The particular block should be precisely restricted in an appropriate spatial and temporal window. If fertilization fails, the persisting synergid cell would be responsible for secreting signals to attract additional pollen tubes for fertilization recovery.
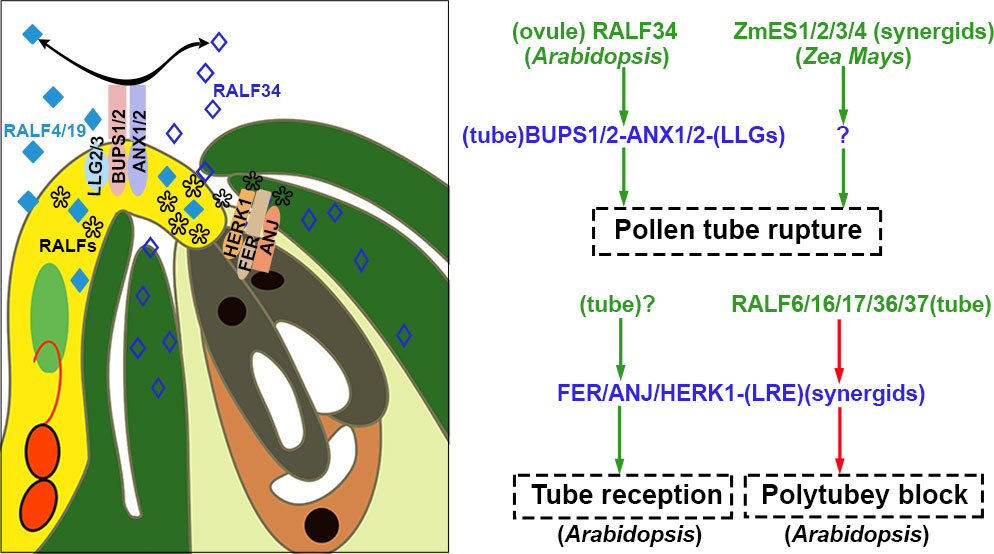
Figure 5 Pollen tube is received, polytubey block, and rupture. At the micropyle, FER/ANJ/HERK1-LLG1 derived from synergid cells is involved in the recognition and reception of pollen tube; they also interact with tube-specific RALF6/16/17/36/37 to cause polytubey block. After the pollen tube enters the ovule, the interaction between ovule-specific RALF34 and BUPS1/2-ANX1/2-LLGs of the pollen tube results in the rupture of the tube in Arabidopsis. ZmES1/2/3/4 secreted by synergids is related to the rupture of the pollen tube in Zea Mays. The peptides are highlighted in green and red to represent activation or suppression, respectively. The receptor complexes are marked in blue and the co-receptors are shown in parentheses. Question marks indicate unidentified signal components. The source of peptides and receptors is shown in parentheses. Abbreviations for all small peptides and receptors are elucidated in the text.
Discharge of sperm cells from pollen tubes
After arrival at the micropyle, the pollen tube is recognized by one of the two synergid cells, and the following communication occurs. Subsequently, the synergid cell undergoes programmed cell death, and the tube bursts to release two sperm cells. Two sperms fuse with two female gametes to form a zygote and primary endosperm, respectively. The persistent synergid cell undergoes degeneration and nourishes primary endosperm, preventing the attraction of excessive pollen tubes. Pollen tube reception and growth termination are tightly controlled by cell-cell communication between pollen tubes and synergid cells. The rupture of pollen tubes is also elaborately regulated through molecular communication of signals from the inner integuments and pollen tubes.
In fer, two or more pollen tubes enter a single ovule. It is demonstrated that the fer female gametophyte keeps attracting more tubes to enter the same ovule after the reception of the first tube. The polyspermy block mechanism is ineffective in fer (Pereira et al., 2016b). FER is localized at the membrane of the filiform apparatus and functions as receptor kinase to sense peptide signals in cell-cell communication. FER participates in receiving the first pollen tube and hinders extra tubes from polytubey and polyspermy (Huck et al., 2003; Escobar-Restrepo et al., 2007). The overgrowth of pollen tubes occurs when the A.thaliana fer is pollinated with pollen from A. lyrata or C. flexuosa (Escobar-Restrepo et al., 2007). It is suggested that FER plays an essential role in receiving signals from conspecific pollen tubes to maintain reproductive barriers.
HERK1 and ANJ are homologs of FER and localized in the filiform apparatus of the synergids. In anj herk1, a similar phenotype of tube overgrowth and failure of fertilization was identified, indicating that HERK1 and ANJ were functionally redundant regulators for pollen tube reception. (Galindo-Trigo et al., 2020). In fer anj herk1, pollen tubes were not able to be recognized and overgrown at the surface of the ovule, resulting in various rates of seed set abortion (Zhong et al., 2022). It was concluded that HERK1 and ANJ, as well as FER, served as the female participants for pollen tube reception and took roles in polytubey block at the micropyle. As pollen from different ralfs (ralf36 ralf37, and ralf6 ralf7 ralf16 ralf36 ralf37) were pollinated to the stigma of wild type, the phenotype of pollen tube overgrowth and seed set abortion were more severe as the increase of the disrupted RALFs. The phenotype was similar to that of fer anj herk1 (Zhong et al., 2022). The data were consistent with the physical interaction of RALFs with FER-ANJ-HERK1 in an in vitro pull-down assay.
The co-receptor LORELEI (LRE) is involved in the interaction between RALF6/7/16/36/37 and FER-ANJ-HERK1. FER-ANJ-HERK1 received pollen tube signals at the micropyle, leading to polar accumulation of the downstream NORTIA (MLO protein) at filiform apparatus. Conversely, NORTIA promotes the perception of pollen tubes to ensure the polytubey block for double fertilization (Ju et al., 2021).
Now it is known that mechanisms underlying the recognition of pollen tubes at the septum and filiform apparatus are triggered by the above players coordinately. RALFs and FER-ANJ-HERK1 take critical roles in the reception of pollen tubes at the filiform apparatus of synergids and act as the second tier of the polytubey barrier as shown in Figures 4, 5 (Zhong et al., 2022). Once the pollen tube rupture in the ovule, the RALFs derived from tubes are diluted and diffuse into embryo sac. The interaction between RALFs and FER-ANJ-HERK1 receptor complexes is disrupted, so the polytubey barrier controlled by RALFs-FER/ANJ/HERK1 is removed at the septum and micropyle.
RNAi knock-down of ZmES4 or in vitro treatment with ZmES4 (Zea mays Embryo Sac), the polymorphic defensin-like cysteine-rich protein from maize, induced tube rupture to release sperm cells in a species-preferred manner, as presented in Figure 5 (Amien et al., 2010). ZmES4 is accumulated in vesicles of mature synergid cells and released upon the arrival of pollen tubes. The subsequent interaction between ZmES4 and KZM1(K+ channel Zea mays 1) leads to channel opening, K+ influxion, plasma membrane depolarization, water uptake, and eventual osmotic pollen tube burst (Cordts et al., 2001; Philippar et al., 2003; Amien et al., 2010).
It was speculated that the pollen tube entered the degenerated synergid cell and continued to grow and release sperm cells at the cleft between the egg and central cell. Another class of ligands should shut down the signaling pathways mentioned above for pollen tube integrity and growth. Pollen-tube localized receptors ANX1/2-BUPS1/2 would perceive RALF4/19 peptides secreted from pollen to regulate the tube growth integrity (Ge et al., 2017; Mecchia et al., 2017). However, an antagonistic signal would be required to disengage signaling pathways triggered by RALF4/19-ANX1/2-BUPS1/2 in ovules. This signal for the rupture of pollen tubes might be derived from the female tissue and received by receptors on the pollen tubes.
In contrast to RALF4, required for maintaining pollen tube integrity, a low concentration (2nM) of RALF34 caused the rupture of 23% of pollen tubes, and almost 70% of tubes are ruptured when treated with 20 mM RALF34 (Ge et al., 2017). Before fertilization, RALF34 accumulates in the ovule, especially in the inner integument. After the pollen tube’s arrival, it diffuses toward the micropyle/synergid cell region. The ralf34 mutant did not display fertility defects, suggesting that other RALFs in female reproductive tissue are involved in the burst of pollen tubes and release of sperm cells (Ge et al., 2017). RALF34 binds the ECD of ANX1/2 and BUPS1/2 by competing with RALF4/19. The interaction between RALF34 and ANX1/2-BUPS1/2 shut off RALF4/19 signaling pathways for the maintenance of tube integrity. The model is shown in Figure 5. The pollen tube releases sperm cells subsequently (Ge et al., 2017). The same receptor complexes realize different biological processes by simply substituting the autocrine signal with the paracrine signal in the different spatiotemporal windows.
Gamete fusion and double fertilization
Two sperm cells released from the pollen tube are activated. Plasma membrane adhesion and nuclear fusion of male and female gametes occur consequently. In the process mentioned above, the cysteine-rich peptide EC1 (Egg cell 1) secreted by the egg cell plays an important role (Sprunck et al., 2012). Sprunck et al., 2012 found that EC1 is accumulated and constrained in spherical vesicle-like structures within unfertilized egg cells by observation of EC1-GFP fusion. During double fertilization, once sperm cells arrive, EC1 is detected outside the egg cell, especially in the apical region of the degenerated synergid (Sprunck et al., 2012), where gametes fusion occurs (Hamamura et al., 2012). It was shown quantitatively that the intensity of GFP signal gradually increased toward the egg cell membrane during the interaction and fusion of gametes. There are five EC1-encoding genes in Arabidopsis that are specifically expressed in egg cells. The recognition receptors in sperm cells or tubes are unknown. However, under the action of EC1, the potential viral-like Fusogen (HAP2/GCS1 that is related in structure to certain viral fusogens to promote the fusion of gamete cell membranes), was redistributed to the surface of sperm cells to regulate their adhesion and separation. (Sprunck et al., 2012; Cyprys et al., 2019). Gamete membrane fusion is promoted by sperm cell-specific expression of DUF679 membrane proteins, DMP8 and DMP9 (Takahashi et al., 2018; Cyprys et al., 2019).
Multiple pollen tubes are directed into one ovule for fertilization compensation in hap2-/-, a mutant with gamete fusion disorder (Mori et al., 2006; von Besser et al., 2006; Zhong et al., 2022). However, polyspermy block initiates rapidly after the completion of double fertilization. It is correlated with the clearance or degradation of pollen tube attractants and programmed cell death of the synergids. To prevent the attraction to excessive pollen tubes, the persistent synergid fused with primary endosperm is selectively disorganized during endosperm proliferation. The attractants pre-secreted are rapidly diluted and modified (Maruyama et al., 2015; Sprunck and Dresselhaus, 2015; Motomura et al., 2018). Ethylene signaling is activated by fertilization of egg and leads to nucleus disintegration of the synergid (Maruyama et al., 2015; Sprunck and Dresselhaus, 2015). In Arabidopsis, the ethylene-free mutants, knocked out five ethylene biosynthetic 1-aminocyclopropane-1-carboxylate oxidase (ACO) genes by CRISPR/Cas9 technology, were investigated (Li et al., 2022). It was indicated that specific components of ethylene signaling pathways, such as the transcription factors ethylene-insensitive 3 (EIN3) (Abozeid et al., 2017) and EIN3-LIKE 1 (EIL1) (Xiao et al., 2021), but not ethylene itself, are required for directing the death of persistent synergid (Li et al., 2022). It is necessary that the persistent synergid undergoes programmed cell death. It seems that the egg and central cell control independent signaling pathways to eliminate the persistent synergid cell and prevent polytubey fertilization (Pereira et al., 2016a). The molecular mechanism is unclear.
The arrival of the first pollen tube at the ovule leads to nitric oxide accumulation in the filiform apparatus. The process depends on de-esterified pectin that is mediated and maintained by FER. Nitric oxide nitrosates the precursor and the mature forms of the attractant AtLURE1 to impede AtLURE1 secretion and interaction with its receptors, and inhibit additional pollen tubes from entering the ovule for polytubey block (Duan et al., 2020). In addition to nitrosation of chemoattractants, AtLURE1s are also digested by egg cell-specific peptidases. The aspartate endopeptidases, EGG CELL-SPECIFIC1/2 (ECS1/2), are expressed specifically in egg cells. The transcripts are degraded immediately after gamete fusion. Once fertilization is completed, ECS1 and ECS2 are secreted from the cortical network at the apical region of Arabidopsis egg cell into extracellular space or to the persistent synergid, where it cleaves exclusively and decomposes AtLURE1 (Yu et al., 2021b). The clearing mechanism of XIUQIUs has yet to be discovered. If double fertilization fails, pollen tube attractors will be secreted from the persistent synergid to attract the second pollen tube for fertilization.
Conclusion remarks
In flowering plants, the cell-cell communication and the signaling network dominated by ligands/receptors are most vividly manifested in the process of fertilization. However, many signal pathways governed by ligand-receptor are still unclear. (1) When the pollen tube grows in the transmitting tract, are there peptide signals secreted by female tissue for rapid tube elongation? How can the two distinct signaling pathways of ANX1/2-BUPS1/2 and LRXs be selected by RALF4/19 for regulating the growth and integrity of pollen tubes? (2) Which factors regulate RALF34 to ensure the timely replacement of RALF4/19 to induce pollen tube rupture at the appropriate spatiotemporal window? (3) The arrest of pollen tube growth and synergid burst resulted from recognition of RALF6/7/16/36/37 by the FER-ANJ-HERK1 receptor. Whether FER-ANJ-HERK1 receives ligand signals secreted by synergid to replace RALF6/7/16/36/37 derived from pollen tubes, triggers the rupture of synergid cells that received pollen tubes? (4) What are the cognate receptors of XIUQIUs during micropylar guidance for pollen tubes? Is there a class of highly conserved receptors that recognize XIUQIUs during their evolution? (5) The EC1 secreted by the egg cell directs the sperm cells to be released at the cleft between the egg and central cell, and promotes gamete fusion and double fertilization. So, does EC1 have a cognate receptor of sperm cells?
With further advances in study, more signaling pathways directly provoked during the plant reproductive process will be discovered and investigated. However, due to the functional redundancy and homology in the genome, it is not easy to identify the phenotype of mutation in a homologous gene. It is suggested that unexpected difficulties in discovering ligands and receptors may be inevitable. Significant progress has been made since the application of CRISPR-Cas9 technology in knocking out multiple homologous genes simultaneously, and obtaining relevant functional mutants is greatly favored as a consequence. It is imperative to develop technologies for high-throughput protein expression and localization. It is expected to integrate high-throughput protein localization and efficient CRISPR-Cas9 knockout technology to leverage forward genetics and elucidate the molecular mechanism of functionally redundant receptor kinases and small peptide ligands in the future. Undoubtedly, the network for cell-cell communication will be dissected with the aid of the coming biotechnologies and ideas.
Author contributions
C-XX, W-JL, and BW performed the literature search and data analysis. C-XX and T-YY prepared the figure. T-YY drafted and revised the manuscript. All authors contributed to the article and approved the submitted version.
Funding
This work was supported by the National Natural Science Foundation of China (31871450), the Natural Science Foundation of Shandong (ZR2022MC217), and the Yantai University Top Talent Project (2220011).
Acknowledgments
We appreciate Dr. Dongqiao Shi from the Institute of Genetics and Developmental Biology, Chinese Academy of Sciences for her kind advice and revision on the manuscript.
Conflict of interest
The authors declare that the research was conducted in the absence of any commercial or financial relationships that could be construed as a potential conflict of interest.
Publisher’s note
All claims expressed in this article are solely those of the authors and do not necessarily represent those of their affiliated organizations, or those of the publisher, the editors and the reviewers. Any product that may be evaluated in this article, or claim that may be made by its manufacturer, is not guaranteed or endorsed by the publisher.
References
Abozeid, A., Ying, Z., Lin, Y., Liu, J., Zhang, Z., Tang, Z. (2017). Ethylene improves root system development under cadmium stress by modulating superoxide anion concentration in arabidopsis thaliana. Front. Plant Sci. 8. doi: 10.3389/fpls.2017.00253
Amien, S., Kliwer, I., Marton, M. L., Debener, T., Geiger, D., Becker, D., et al. (2010). Defensin-like ZmES4 mediates pollen tube burst in maize via opening of the potassium channel KZM1. PloS Biol. 8 (6), e1000388. doi: 10.1371/journal.pbio.1000388
Bleckmann, A., Alter, S., Dresselhaus, T. (2014). The beginning of a seed: regulatory mechanisms of double fertilization. Front. Plant Sci. 5. doi: 10.3389/fpls.2014.00452
Boggs, N. A., Nasrallah, J. B., Nasrallah, M. E. (2009). Independent s-locus mutations caused self-fertility in arabidopsis thaliana. PloS Genet. 5 (3), e1000426. doi: 10.1371/journal.pgen.1000426
Boisson-Dernier, A., Franck, C. M., Lituiev, D. S., Grossniklaus, U. (2015). Receptor-like cytoplasmic kinase MARIS functions downstream of CrRLK1L-dependent signaling during tip growth. Proc. Natl. Acad. Sci. U.S.A. 112 (39), 12211–12216. doi: 10.1073/pnas.1512375112
Boisson-Dernier, A., Lituiev, D. S., Nestorova, A., Franck, C. M., Thirugnanarajah, S., Grossniklaus, U. (2013). ANXUR receptor-like kinases coordinate cell wall integrity with growth at the pollen tube tip via NADPH oxidases. PloS Biol. 11 (11), e1001719–e1001719. doi: 10.1371/journal.pbio.1001719
Boisson-Dernier, A., Roy, S., Kritsas, K., Grobei, M., Jaciubek, M., Schroeder, J., et al. (2009). Disruption of the pollen-expressed FERONIA homologs ANXUR1 and ANXUR2 triggers pollen tube discharge. Development 136, 3279–3288. doi: 10.1242/dev.040071
Bower, M. S., Matias, D. D., Fernandes-Carvalho, E., Mazzurco, M., Gu, T., Rothstein, S. J., et al. (1996). Two members of the thioredoxin-h family interact with the kinase domain of a brassica s locus receptor kinase. Plant Cell 8 (9), 1641–1650. doi: 10.1105/tpc.8.9.1641
Chevalier, E., Loubert-Hudon, A., Zimmerman, E. L., Matton, D. P. (2011). Cell-cell communication and signalling pathways within the ovule: From its inception to fertilization. New Phytol. 192 (1), 13–28. doi: 10.1111/j.1469-8137.2011.03836.x
Coimbra, S., Jones, B., Pereira, L. G. (2008). Arabinogalactan proteins (AGPs) related to pollen tube guidance into the embryo sac in arabidopsis. Plant Signal Behav. 3 (7), 455–456. doi: 10.4161/psb.3.7.5601
Cordts, S., Bantin, J., Wittich, P. E., Kranz, E., Lorz, H., Dresselhaus, T. (2001). ZmES genes encode peptides with structural homology to defensins and are specifically expressed in the female gametophyte of maize. Plant J. 25 (1), 103–114. doi: 10.1046/j.0960-7412.2000.00944.x
Costa, M., Nobre, M. S., Becker, J. D., Masiero, S., Amorim, M. I., Pereira, L. G., et al. (2013). Expression-based and co-localization detection of arabinogalactan protein 6 and arabinogalactan protein 11 interactors in arabidopsis pollen and pollen tubes. BMC Plant Biol. 13, 7. doi: 10.1186/1471-2229-13-7
Covey, P. A., Subbaiah, C. C., Parsons, R. L., Pearce, G., Lay, F. T., Anderson, M. A., et al. (2010). A pollen-specific RALF from tomato that regulates pollen tube elongation. Plant Physiol. 153 (2), 703–715. doi: 10.1104/pp.110.155457
Cyprys, P., Lindemeier, M., Sprunck, S. (2019). Gamete fusion is facilitated by two sperm cell-expressed DUF679 membrane proteins. Nat. Plants 5 (3), 253–257. doi: 10.1038/s41477-019-0382-3
Del Duca, S., Aloisi, I., Parrotta, L., Cai, G. (2019). Cytoskeleton, transglutaminase and gametophytic self-incompatibility in the malinae (Rosaceae). Int. J. Mol. Sci. 20 (1), 209. doi: 10.3390/ijms20010209
Deslauriers, S. D., Larsen, P. B. (2010). FERONIA is a key modulator of brassinosteroid and ethylene responsiveness in arabidopsis hypocotyls. Mol. Plant 3 (3), 626–640. doi: 10.1093/mp/ssq015
Dresselhaus, T., Franklin-Tong, N. (2013). Male-Female crosstalk during pollen germination, tube growth and guidance, and double fertilization. Mol. Plant 6 (4), 1018–1036. doi: 10.1093/mp/sst061
Dresselhaus, T., Sprunck, S., Wessel, G. M. (2016). Fertilization mechanisms in flowering plants. Curr. Biol. 26(3) R125–R139. doi: 10.1016/j.cub.2015.12.032
Duan, Q., Kita, D., Li, C., Cheung, A. Y., Wu, H. M. (2010). FERONIA receptor-like kinase regulates RHO GTPase signaling of root hair development. Proc. Natl. Acad. Sci. U.S.A. 107 (41), 17821–17826. doi: 10.1073/pnas.1005366107
Duan, Q., Liu, M. J., Kita, D., Jordan, S. S., Yeh, F. J., Yvon, R., et al. (2020). FERONIA controls pectin- and nitric oxide-mediated male-female interaction. Nature 579 (7800), 561–566. doi: 10.1038/s41586-020-2106-2
Entani, T., Kubo, K., Isogai, S., Fukao, Y., Shirakawa, M., Isogai, A., et al. (2014). Ubiquitin-proteasome-mediated degradation of s-RNase in a solanaceous cross-compatibility reaction. Plant J. 78, 1014–1021. doi: 10.1111/tpj.12528
Escobar-Restrepo, J. M., Huck, N., Kessler, S., Gagliardini, V., Gheyselinck, J., Yang, W. C., et al. (2007). The FERONIA receptor-like kinase mediates male-female interactions during pollen tube reception. Science 317 (5838), 656–660. doi: 10.1126/science.1143562
Fabrice, T. N., Vogler, H., Draeger, C., Munglani, G., Gupta, S., Herger, A. G., et al. (2018). LRX proteins play a crucial role in pollen grain and pollen tube cell wall development. Plant Physiol. 176 (3), 1981–1992. doi: 10.1104/pp.17.01374
Feng, F., Sun, J., Radhakrishnan, G., Lee, T., Bozsoki, Z., Fort, S., et al. (2019). A combination of chitooligosaccharide and lipochitooligosaccharide recognition promotes arbuscular mycorrhizal associations in medicago truncatula. Nat. Commun. 10, 5047. doi: 10.1038/s41467-019-12999-5
Franck, C. M., Westermann, J., Boisson-Dernier, A. (2018a). Plant malectin-like receptor kinases: From cell wall integrity to immunity and beyond. Annu. Rev. Plant Biol. 69, 301–328. doi: 10.1146/annurev-arplant-042817-040557
Franck, C. M., Westermann, J., Burssner, S., Lentz, R., Lituiev, D. S., Boisson-Dernier, A. (2018b). The protein phosphatases ATUNIS1 and ATUNIS2 regulate cell wall integrity in tip-growing cells. Plant Cell 30 (8), 1906–1923. doi: 10.1105/tpc.18.00284
Franklin-Tong, N., Franklin, F. (2003). Gametophytic self-incompatibility inhibits pollen tube growth using different mechanisms. Trends Plant Sci. 8, 598–605. doi: 10.1016/j.tplants.2003.10.008
Galindo-Trigo, S., Blanco-Tourinan, N., DeFalco, T. A., Wells, E. S., Gray, J. E., Zipfel, C., et al. (2020). CrRLK1L receptor-like kinases HERK1 and ANJEA are female determinants of pollen tube reception. EMBO Rep. 21 (2), e48466. doi: 10.15252/embr.201948466
Ge, Z., Bergonci, T., Zhao, Y., Zou, Y., Du, S., Liu, M. C., et al. (2017). Arabidopsis pollen tube integrity and sperm release are regulated by RALF-mediated signaling. Science 358 (6370), 1596–1600. doi: 10.1126/science.aao3642
Ge, Z., Zhao, Y., Liu, M. C., Zhou, L. Z., Wang, L., Zhong, S., et al. (2019). LLG2/3 are Co-receptors in BUPS/ANX-RALF signaling to regulate arabidopsis pollen tube integrity. Curr. Biol. 29 (19), 3256–3265.e3255. doi: 10.1016/j.cub.2019.08.032
Goring, D. R., Indriolo, E., Samuel, M. A. (2014). The ARC1 E3 ligase promotes a strong and stable self-incompatibility response in arabidopsis species: Response to the nasrallah and nasrallah commentary. Plant Cell 26 (10), 3842–3846. doi: 10.1105/tpc.114.131243
Hafidh, S., Honys, D. (2021). Reproduction multitasking: The Male gametophyte. Annu. Rev. Plant Biol. 72, 581–614. doi: 10.1146/annurev-arplant-080620-021907
Hamamura, Y., Nagahara, S., Higashiyama, T. (2012). Double fertilization on the move. Curr. Opin. Plant Biol. 15 (1), 70–77. doi: 10.1016/j.pbi.2011.11.001
Hater, F., Nakel, T., Gross-Hardt, R. (2020). Reproductive multitasking: The female gametophyte. Annu. Rev. Plant Biol. 71, 517–546. doi: 10.1146/annurev-arplant-081519-035943
Herger, A., Gupta, S., Kadler, G., Franck, C. M., Boisson-Dernier, A., Ringli, C. (2020). Overlapping functions and protein-protein interactions of LRR-extensins in arabidopsis. PloS Genet. 16 (6), e1008847. doi: 10.1371/journal.pgen.1008847
Higashiyama, T., Yabe, S., Sasaki, N., Nishimura, Y., Miyagishima, S., Kuroiwa, H., et al. (2001). Pollen tube attraction by the synergid cell. Science 293 (5534), 1480–1483. doi: 10.1126/science.1062429
Hohmann, U., Lau, K., Hothorn, M. (2017). The structural basis of ligand perception and signal activation by receptor kinases. Annu. Rev. Plant Biol. 68, 109–137. doi: 10.1146/annurev-arplant-042916-040957
Huang, W. J., Liu, H. K., McCormick, S., Tang, W. H. (2014). Tomato pistil factor STIG1 promotes in vivo pollen tube growth by binding to phosphatidylinositol 3-phosphate and the extracellular domain of the pollen receptor kinase LePRK2. Plant Cell 26 (6), 2505–2523. doi: 10.1105/tpc.114.123281
Huck, N., Moore, J. M., Federer, M., Grossniklaus, U. (2003). The arabidopsis mutant feronia disrupts the female gametophytic control of pollen tube reception. Development 130 (10), 2149–2159. doi: 10.1242/dev.00458
Iwano, M., Igarashi, M., Tarutani, Y., Kaothien-Nakayama, P., Nakayama, H., Moriyama, H., et al. (2014). A pollen coat-inducible autoinhibited Ca2+-ATPase expressed in stigmatic papilla cells is required for compatible pollination in the brassicaceae. Plant Cell 26 (2), 636–649. doi: 10.1105/tpc.113.121350
Jany, E., Nelles, H., Goring, D. R. (2019). The molecular and cellular regulation of brassicaceae self-incompatibility and self-pollen rejection. Int. Rev. Cell Mol. Biol. 343, 1–35. doi: 10.1016/bs.ircmb.2018.05.011
Jia, Q. S., Zhu, J., Xu, X. F., Lou, Y., Zhang, Z. L., Zhang, Z. P., et al. (2015). Arabidopsis AT-hook protein TEK positively regulates the expression of arabinogalactan proteins for nexine formation. Mol. Plant 8 (2), 251–260. doi: 10.1016/j.molp.2014.10.001
Johnson, M. A., Preuss, D. (2003). On your mark, get set, GROW! LePRK2-LAT52 interactions regulate pollen tube growth. Trends Plant Sci. 8 (3), 97–99. doi: 10.1016/S1360-1385(03)00009-8
Ju, Y., Yuan, J., Jones, D. S., Zhang, W., Staiger, C. J., Kessler, S. A. (2021). Polarized NORTIA accumulation in response to pollen tube arrival at synergids promotes fertilization. Dev. Cell 56 (21), 2938–2951 e2936. doi: 10.1016/j.devcel.2021.09.026
Kanaoka, M. M. (2018). Cell-cell communications and molecular mechanisms in plant sexual reproduction. J. Plant Res. 131 (1), 37–47. doi: 10.1007/s10265-017-0997-2
Kawashima, T., Berger, F. (2011). Green love talks; cell-cell communication during double fertilization in flowering plants. AoB Plants 2011, plr015. doi: 10.1093/aobpla/plr015
Kim, M. J., Jeon, B. W., Oh, E., Seo, P. J., Kim, J. (2021). Peptide signaling during plant reproduction. Trends Plant Sci. 26 (8), 822–835. doi: 10.1016/j.tplants.2021.02.008
Kirkbride, K., Ray, B., Blobe, G. (2005). Cell-surface co-receptors: Emerging roles in signaling and human disease. Trends Biochem. Sci. 30, 611–621. doi: 10.1016/j.tibs.2005.09.003
Kitashiba, H., Liu, P., Nishio, T., Nasrallah, J. B., Nasrallah, M. E. (2011). Functional test of brassica self-incompatibility modifiers in arabidopsis thaliana. Proc. Natl. Acad. Sci. U.S.A. 108 (44), 18173–18178. doi: 10.1073/pnas.1115283108
Kou, X., Cao, P., He, Q., Wang, P., Zhang, S., Wu, J. (2022). PbrROP1/2-elicited imbalance of cellulose deposition is mediated by a CrRLK1L-ROPGEF module in the pollen tube of pyrus. Hortic. Res 6511232. doi: 10.1093/hr/uhab034
Leszczuk, A., Szczuka, E., Zdunek, A. (2019). Arabinogalactan proteins: Distribution during the development of male and female gametophytes. Plant Physiol. Biochem. 135, 9–18. doi: 10.1016/j.plaphy.2018.11.023
Levitin, B., Richter, D., Markovich, I., Zik, M. (2008). Arabinogalactan proteins 6 and 11 are required for stamen and pollen function in arabidopsis. Plant J. 56 (3), 351–363. doi: 10.1111/j.1365-313X.2008.03607.x
Liang, X., Zhou, J. M. (2018). Receptor-like cytoplasmic kinases: Central players in plant receptor kinase-mediated signaling. Annu. Rev. Plant Biol. 69, 267–299. doi: 10.1146/annurev-arplant-042817-040540
Li, S., Chen, M., Yu, D., Ren, S., Sun, S., Liu, L., et al. (2013). EXO70A1-mediated vesicle trafficking is critical for tracheary element development in arabidopsis. Plant Cell 25 (5), 1774–1786. doi: 10.1105/tpc.113.112144
Li, W., Li, Q., Lyu, M., Wang, Z., Song, Z., Zhong, S., et al. (2022). Lack of ethylene does not affect reproductive success and synergid cell death in arabidopsis. Mol. Plant 15 (2), 354–362. doi: 10.1016/j.molp.2021.11.001
Liu, H. K., Li, Y. J., Wang, S. J., Yuan, T. L., Huang, W. J., Dong, X., et al. (2020). Kinase partner protein plays a key role in controlling the speed and shape of pollen tube growth in tomato. Plant Physiol. 184 (4), 1853–1869. doi: 10.1104/pp.20.01081
Liu, C., Shen, L., Xiao, Y., Vyshedsky, D., Peng, C., Sun, X., et al. (2021a). Pollen PCP-b peptides unlock a stigma peptide-receptor kinase gating mechanism for pollination. Science 372 (6538), 171–175. doi: 10.1126/science.abc6107
Liu, M., Wang, Z., Hou, S., Wang, L., Huang, Q., Gu, H., et al. (2021b). AtLURE1/PRK6-mediated signaling promotes conspecific micropylar pollen tube guidance. Plant Physiol. 186 (2), 865–873. doi: 10.1093/plphys/kiab105
Liu, J., Zhong, S., Guo, X., Hao, L., Wei, X., Huang, Q., et al. (2013). Membrane-bound RLCKs LIP1 and LIP2 are essential Male factors controlling Male-female attraction in arabidopsis. Curr. Biol. 23 (11), 993–998. doi: 10.1016/j.cub.2013.04.043
Losada, J. M., Herrero, M. (2017). Pollen tube access to the ovule is mediated by glycoprotein secretion on the obturator of apple (Malus × domestica, borkh). Ann. Bot. 119 (6), 989–1000. doi: 10.1093/aob/mcw276
Ma, H. (2003). Plant reproduction: GABA gradient, guidance and growth. Curr. Biol. 13 (21), R834–R836. doi: 10.1016/j.cub.2003.10.015
Marton, M. L., Cordts, S., Broadhvest, J., Dresselhaus, T. (2005). Micropylar pollen tube guidance by egg apparatus 1 of maize. Science 307 (5709), 573–576. doi: 10.1126/science.1104954
Marton, M. L., Fastner, A., Uebler, S., Dresselhaus, T. (2012). Overcoming hybridization barriers by the secretion of the maize pollen tube attractant ZmEA1 from arabidopsis ovules. Curr. Biol. 22 (13), 1194–1198. doi: 10.1016/j.cub.2012.04.061
Maruyama, D., Volz, R., Takeuchi, H., Mori, T., Igawa, T., Kurihara, D., et al. (2015). Rapid elimination of the persistent synergid through a cell fusion mechanism. Cell 161 (4), 907–918. doi: 10.1016/j.cell.2015.03.018
Matsumoto, D., Tao, R. (2019). Recognition of s-RNases by an s locus f-box like protein and an s haplotype-specific f-box like protein in the prunus-specific self-incompatibility system. Plant Mol. Biol. 100, 367–378. doi: 10.1007/s11103-019-00860-8
Mazzurco, M., Sulaman, W., Elina, H., Cock, J., Goring, D. (2001). Further analysis of the interactions between the brassica s receptor kinase and three interacting proteins (ARC1, THL1 and THL2) in the yeast two-hybrid system. Plant Mol. Biol. 45, 365–376. doi: 10.1023/a:1006412329934
McInnis, S. M., Desikan, R., Hancock, J. T., Hiscock, S. J. (2006). Production of reactive oxygen species and reactive nitrogen species by angiosperm stigmas and pollen: potential signalling crosstalk? New Phytol. 172 (2), 221–228. doi: 10.1111/j.1469-8137.2006.01875.x
Mecchia, M. A., Santos-Fernandez, G., Duss, N. N., Somoza, S. C., Boisson-Dernier, A., Gagliardini, V., et al. (2017). RALF4/19 peptides interact with LRX proteins to control pollen tube growth in arabidopsis. Science 358 (6370), 1600–1603. doi: 10.1126/science.aao5467
Miyazaki, S., Murata, T., Sakurai-Ozato, N., Kubo, M., Demura, T., Fukuda, H., et al. (2009). ANXUR1 and 2, sister genes to FERONIA/SIRENE, are male factors for coordinated fertilization. Curr. Biol. 19, 1327–1331. doi: 10.1016/j.cub.2009.06.064
Mizukami, A. G., Inatsugi, R., Jiao, J., Kotake, T., Kuwata, K., Ootani, K., et al. (2016). The AMOR arabinogalactan sugar chain induces pollen-tube competency to respond to ovular guidance. Curr. Biol. 26 (8), 1091–1097. doi: 10.1016/j.cub.2016.02.040
Mori, T., Kuroiwa, H., Higashiyama, T., Kuroiwa, T. (2006). GENERATIVE CELL SPECIFIC 1 is essential for angiosperm fertilization. Nat. Cell Biol. 8 (1), 64–71. doi: 10.1038/ncb1345
Motomura, K., Kawashima, T., Berger, F., Kinoshita, T., Higashiyama, T., Maruyama, D. (2018). A pharmacological study of arabidopsis cell fusion between the persistent synergid and endosperm. J. Cell Sci. 131 (2), jcs204123. doi: 10.1242/jcs.204123
Murase, K., Shiba, H., Iwano, M., Che, F. S., Watanabe, M., Isogai, A., et al. (2004). A membrane-anchored protein kinase involved in brassica self-incompatibility signaling. Science 303 (5663), 1516–1519. doi: 10.1126/science.1093586
Okuda, S., Tsutsui, H., Shiina, K., Sprunck, S., Takeuchi, H., Yui, R., et al. (2009). Defensin-like polypeptide LUREs are pollen tube attractants secreted from synergid cells. Nature 458 (7236), 357–361. doi: 10.1038/nature07882
Palanivelu, R., Brass, L., Edlund, A. F., Preuss, D. (2003). Pollen tube growth and guidance is regulated by POP2, an arabidopsis gene that controls GABA levels. Cell 114 (1), 47–59. doi: 10.1016/s0092-8674(03)00479-3
Pereira, A. M., Lopes, A. L., Coimbra, S. (2016a). JAGGER, an AGP essential for persistent synergid degeneration and polytubey block in arabidopsis. Plant Signal Behav. 11 (8), e1209616. doi: 10.1080/15592324.2016.1209616
Pereira, A. M., Nobre, M. S., Pinto, S. C., Lopes, A. L., Costa, M. L., Masiero, S., et al. (2016b). "Love is strong, and you're so sweet": JAGGER is essential for persistent synergid degeneration and polytubey block in arabidopsis thaliana. Mol. Plant 9 (4), 601–614. doi: 10.1016/j.molp.2016.01.002
Philippar, K., Büchsenschutz, K., Abshagen, M., Fuchs, I., Geiger, D., Lacombe, B., et al. (2003). The k+ channel KZM1 mediates potassium uptake into the phloem and guard cells of the C4 grass zea mays. J. Biol. Chem. 278 (19), 16973–16981. doi: 10.1074/jbc.M212720200
Ramesh, S. A., Tyerman, S. D., Xu, B., Bose, J., Kaur, S., Conn, V., et al. (2015). GABA signalling modulates plant growth by directly regulating the activity of plant-specific anion transporters. Nat. Commun. 6, 7879. doi: 10.1038/ncomms8879
Rozier, F., Riglet, L., Kodera, C., Bayle, V., Durand, E., Schnabel, J., et al. (2020). Live-cell imaging of early events following pollen perception in self-incompatible arabidopsis thaliana. J. Exp. Bot. 71, 2513–2526. doi: 10.1093/jxb/eraa008
Sankaranarayanan, S., Jamshed, M., Samuel, M. A. (2015). Degradation of glyoxalase I in brassica napus stigma leads to self-incompatibility response. Nat. Plants 1, 15185. doi: 10.1038/nplants.2015.185
Sassa, H. (2016). Molecular mechanism of the s-RNase-based gametophytic self-incompatibility in fruit trees of rosaceae. Breed Sci. 66, 116–121. doi: 10.1270/jsbbs.66.116
Scandola, S., Samuel, M. A. (2019). A flower-specific phospholipase d is a stigmatic compatibility factor targeted by the self-incompatibility response in brassica napus. Curr. Biol. 29 (3), 506–512.e504. doi: 10.1016/j.cub.2018.12.037
Shiu, S. H., Karlowski, W. M., Pan, R., Tzeng, Y. H., Mayer, K. F., Li, W. H. (2004). Comparative analysis of the receptor-like kinase family in arabidopsis and rice. Plant Cell 16 (5), 1220–1234. doi: 10.1105/tpc.020834
Somoza, S. C., Sede, A. R., Boccardo, N. A., Muschietti, J. P. (2021). Keeping up with the RALFs: How these small peptides control pollen-pistil interactions in arabidopsis. New Phytol. 229 (1), 14–18. doi: 10.1111/nph.16817
Sprunck, S., Dresselhaus, T. (2015). Three cell fusions during double fertilization. Cell 161 (4), 708–709. doi: 10.1016/j.cell.2015.04.032
Sprunck, S., Rademacher, S., Vogler, F., Gheyselinck, J., Grossniklaus, U., Dresselhaus, T. (2012). Egg cell-secreted EC1 triggers sperm cell activation during double fertilization. Science 338 (6110), 1093–1097. doi: 10.1126/science.1223944
Stegmann, M., Monaghan, J., Smakowska-Luzan, E., Rovenich, H., Lehner, A., Holton, N., et al. (2017). The receptor kinase FER is a RALF-regulated scaffold controlling plant immune signaling. Science 355 (6322), 287–289. doi: 10.1126/science.aal2541
Stone, S. L., Anderson, E. M., Mullen, R. T., Goring, D. R. (2003). ARC1 is an E3 ubiquitin ligase and promotes the ubiquitination of proteins during the rejection of self-incompatible brassica pollen. Plant Cell 15 (4), 885–898. doi: 10.1105/tpc.009845
Sun, L., Williams, J., Li, S., Wu, L., Khatri, W., Stone, P., et al. (2018). S-locus f-box proteins are solely responsible for s-RNase-Based self-incompatibility of petunia pollen. Plant Cell 30, 2959–2972. doi: 10.1105/tpc.18.00615
Takahashi, T., Mori, T., Ueda, K., Yamada, L., Nagahara, S., Higashiyama, T., et al. (2018). The male gamete membrane protein DMP9/DAU2 is required for double fertilization in flowering plants. Dev. (Cambridge England) 145 (23), dev170076. doi: 10.1242/dev.170076
Takeuchi, H., Higashiyama, T. (2012). A species-specific cluster of defensin-like genes encodes diffusible pollen tube attractants in arabidopsis. PloS Biol. 10 (12), e1001449. doi: 10.1371/journal.pbio.1001449
Takeuchi, H., Higashiyama, T. (2016). Tip-localized receptors control pollen tube growth and LURE sensing in arabidopsis. Nature 531 (7593), 245–248. doi: 10.1038/nature17413
Tang, W., Ezcurra, I., Muschietti, J., McCormick, S. (2002). A cysteine-rich extracellular protein, LAT52, interacts with the extracellular domain of the pollen receptor kinase LePRK2. Plant Cell 14 (9), 2277–2287. doi: 10.1105/tpc.003103
Tang, W., Kelley, D., Ezcurra, I., Cotter, R., McCormick, S. (2004). LeSTIG1, an extracellular binding partner for the pollen receptor kinases LePRK1 and LePRK2, promotes pollen tube growth in vitro. Plant J. 39 (3), 343–353. doi: 10.1111/j.1365-313X.2004.02139.x
Vogler, H., Santos-Fernandez, G., Mecchia, M. A., Grossniklaus, U. (2019). To preserve or to destroy, that is the question: the role of the cell wall integrity pathway in pollen tube growth. Curr. Opin. Plant Biol. 52, 131–139. doi: 10.1016/j.pbi.2019.09.002
von Besser, K., Frank, A. C., Johnson, M. A., Preuss, D. (2006). Arabidopsis HAP2 (GCS1) is a sperm-specific gene required for pollen tube guidance and fertilization. Development 133 (23), 4761–4769. doi: 10.1242/dev.02683
Wang, L., Clarke, L. A., Eason, R. J., Parker, C. C., Qi, B., Scott, R. J., et al. (2017). PCP-b class pollen coat proteins are key regulators of the hydration checkpoint in arabidopsis thaliana pollen-stigma interactions. New Phytol. 213 (2), 764–777. doi: 10.1111/nph.14162
Wang, N., Kao, T. (2012). Self-incompatibility in petunia: a self/nonself-recognition mechanism employing s-locus f-box proteins and s-RNase to prevent inbreeding. Wiley Interdiscip. Rev. Dev. Biol. 1, 267–275. doi: 10.1002/wdev.10
Wang, T., Liang, L., Xue, Y., Jia, P. F., Chen, W., Zhang, M. X., et al. (2016). A receptor heteromer mediates the male perception of female attractants in plants. Nature 531 (7593), 241–244. doi: 10.1038/nature16975
Xiao, D., Li, X., Zhou, Y. Y., Wei, L., Keovongkod, C., He, H., et al. (2021). Transcriptome analysis reveals significant difference in gene expression and pathways between two peanut cultivars under Al stress. Gene 781, 145535. doi: 10.1016/j.gene.2021.145535
Yu, T. Y., Sun, M. K., Liang, L. K. (2021a). Receptors in the induction of the plant innate immunity. Mol. Plant Microbe Interact. 34 (6), 587–601. doi: 10.1094/MPMI-07-20-0173-CR
Yu, X., Zhang, X., Zhao, P., Peng, X., Chen, H., Bleckmann, A., et al. (2021b). Fertilized egg cells secrete endopeptidases to avoid polytubey. Nature 592 (7854), 433–437. doi: 10.1038/s41586-021-03387-5
Zhang, C., Brown, M. Q., van de Ven, W., Zhang, Z. M., Wu, B., Young, M. C., et al. (2016). Endosidin2 targets conserved exocyst complex subunit EXO70 to inhibit exocytosis. Proc. Natl. Acad. Sci. U.S.A. 113 (1), E41–E50. doi: 10.1073/pnas.1521248112
Zhang, L., Huang, J., Su, S., Wei, X., Yang, L., Zhao, H., et al. (2021). FERONIA receptor kinase-regulated reactive oxygen species mediate self-incompatibility in brassica rapa. Curr. Biol. 31 (14), 3004–3016.e3004. doi: 10.1016/j.cub.2021.04.060
Zhang, D., Wengier, D., Shuai, B., Gui, C. P., Muschietti, J., McCormick, S., et al. (2008). The pollen receptor kinase LePRK2 mediates growth-promoting signals and positively regulates pollen germination and tube growth. Plant Physiol. 148 (3), 1368–1379. doi: 10.1104/pp.108.124420
Zhong, S., Liu, M., Wang, Z., Huang, Q., Hou, S., Xu, Y. C., et al. (2019). Cysteine-rich peptides promote interspecific genetic isolation in arabidopsis. Science, 364(6443), eaau9564. doi: 10.1126/science.aau9564
Zhong, S., Li, L., Wang, Z., Ge, Z., Li, Q., Bleckmann, A., et al. (2022). RALF peptide signaling controls the polytubey block in arabidopsis. Science 375 (6578), 290–296. doi: 10.1126/science.abl4683
Zhou, L. Z., Dresselhaus, T. (2019). Friend or foe: Signaling mechanisms during double fertilization in flowering seed plants. Curr. Top. Dev. Biol. 131, 453–496. doi: 10.1016/bs.ctdb.2018.11.013
Keywords: double-fertilization, peptide, kinase, pollen tube guidance, polytubey block, cell-cell communication
Citation: Yu T-Y, Xu C-X, Li W-J and Wang B (2022) Peptides/receptors signaling during plant fertilization. Front. Plant Sci. 13:1090836. doi: 10.3389/fpls.2022.1090836
Received: 15 November 2022; Accepted: 02 December 2022;
Published: 15 December 2022.
Edited by:
Giampiero Cai, University of Siena, ItalyReviewed by:
Hidenori Takeuchi, Nagoya University, JapanJorge Lora, Spanish National Research Council (CSIC), Spain
Copyright © 2022 Yu, Xu, Li and Wang. This is an open-access article distributed under the terms of the Creative Commons Attribution License (CC BY). The use, distribution or reproduction in other forums is permitted, provided the original author(s) and the copyright owner(s) are credited and that the original publication in this journal is cited, in accordance with accepted academic practice. No use, distribution or reproduction is permitted which does not comply with these terms.
*Correspondence: Tian-Ying Yu, dHl5dUB5dHUuZWR1LmNu