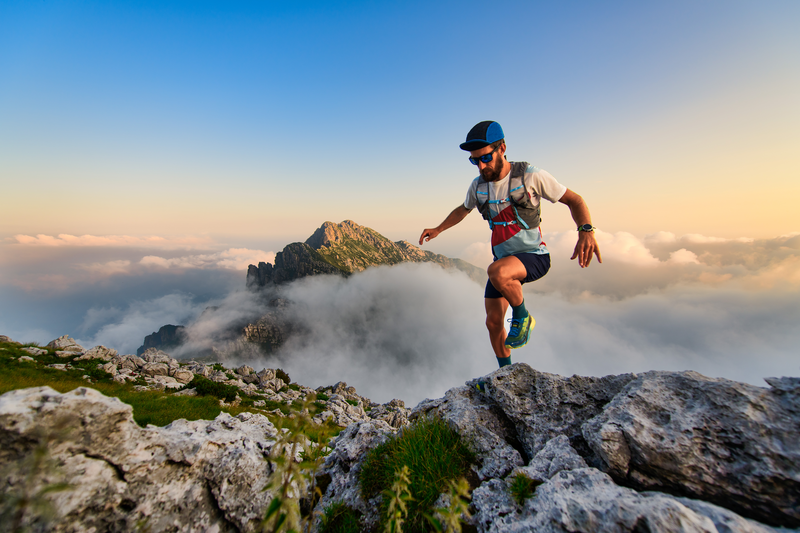
94% of researchers rate our articles as excellent or good
Learn more about the work of our research integrity team to safeguard the quality of each article we publish.
Find out more
ORIGINAL RESEARCH article
Front. Plant Sci. , 21 December 2022
Sec. Plant Metabolism and Chemodiversity
Volume 13 - 2022 | https://doi.org/10.3389/fpls.2022.1087899
This article is part of the Research Topic Chemistry and Biology of Plant Natural Products View all 9 articles
The medicinal plants were wildly library of natural products in drug discovery. The most active molecules with seven-membered rings skeleton represent a challenge for construction. A stereoselectivity (4 + 3) cycloadditions between allenyl ethers and substituted furans induced by chiral auxiliaries has been investigated. And the results showed significant stereoselectivities and regioselectivities. The optical cycloadducts with an oxygen-substituted seven-membered ring framework were generated by removing chiral auxiliaries under acidic conditions. The antiproliferative activity of the novel compounds displayed moderate antiproliferative effects toward T47D cells.
Natural products are important sources of drug discovery. The bioactive chemical constituents in medicinal plants are the effective material bases for the disease prevention and treatment (Harvey, 2008; Newman and Cragg, 2020; Atanasov et al., 2021). However, due to the low natural content of many natural products, separation and extraction difficulties and other factors that limit their subsequent activity research. How to achieve efficient synthesis of trace active natural products has always been an important scientific problem to be solved (Kim et al., 2021; Li et al., 2022). In recent years, as scientists pay more and more attention to the field of total synthesis of natural products, the total synthesis of many natural products has been realized (Nicolaou et al., 2019). The seven-membered rings construction represents a more challenging task than the preparation of the smaller five- or six-membered analogues due to their higher ring strain and to entropy issues (Foley and Maguire, 2010; Nguyen et al., 2013). In particular, this motif is ubiquitous in natural products with significant biological activities. As shown in Figure 1, (+)-ingenol was isolated from Euphorbia ingens for the first time, then from Euphorbia peplus (Evans and Kinghorn, 1977; Foley and Maguire, 2010). Its derivatives showed significant anti-cancer and anti-HIV activities, such as , picato (ingenol mebutate), which was recently approved by the FDA as a first-in-class drug for the topical treatment of actinic keratosis, and a precancerous skin lesions (Lebwohl et al., 2012). Highly oxygenated (+)-ineleganolide, which isolated from the soft coral Sinularia inelegans in 1999, showed anti-leukemic (Duh et al., 1999). Phobosol, which was first isolated from seeds of Croton tiglium in 1934, was one of the most famous natural products of Tigrian family (Flaschenträger and v. Wolffersdorff, 1934). Esters of phorbol showed tumorigenic when protein kinase C was activated. Phorbol-12-myristate-13-acetate (PMA) has been most used as a biomedical research tool in carcinogenic models (Wender et al., 1989; Wender and McDonald, 1990; Wender et al., 1997; Kawamura et al., 2016). Prostratin was also one of the natural products of the Tigliane family that had potent anti-HIV activity, even could fight against latent viral hosts. It was found in the bark of mamala tree Homalanthus Nutans of the Euphorbiaceae family, and traditionally used by the Samoan natives to treat hepatitis. Biological evaluation of sesquiterpenoid natural product (−)-englerins A, isolated from the Tanzanian plant Phyllanthus Engleri demonstrated strong and selective nanomolar cytotoxicity against various renal cancer cell lines. Nevertheless, some natural products cannot systematically carry out pharmacological activity research because of the lack of natural resources. Guaiacolide (+)-hedyotin A-C isolated from Hedyotis Orientalis had limited efforts to reveal its complete biological characteristics due to its deficient resource availability (Wang et al., 2015).
As one of the most direct methods for the construction of seven-membered carbocycles, the (4+3) cycloaddition between oxyallyl cations and dienes had attracted extensive attentions in the scientific community (Montana and Grima, 2001; Lohse and Hsung, 2011; Okamoto et al., 2018; Yin et al., 2018). Specifically, significant advances have been made by employing heteroatoms such as halogens, (Harmata et al., 1991; Lee and Cha, 1999) oxygen, (Masuya et al., 1998; Lee and Cha, 1999; Hayakawa and Shimizu, 2000; Saez et al., 2003; Sáez et al., 2005) sulfur, (Harmata et al., 1991; Hardinger et al., 1995; Fuchigami et al., 2012) and nitrogen (Myers and Barbay, 2001; Wei et al., 2003; He et al., 2014) to modify the stability and reactivity of oxyallyl species as well as to introduce an electronic bias in these intermediates that can lead to high regioselectivity and stereoselectivity.
As shown in Scheme 1, in 2019, our group developed a series of (4 + 3) cycloadditions based on oxygen-stabilized oxyallyl intermediates derived from epoxidations/ring-opening of allenyl ethers, opening the curtain on the involvement of oxyallyl cations in (4 + 3) cycloadditions (Huang et al., 2020). Although the (4 + 3) cycloadditions based on allenyl ethers had been successfully established, we believed that the stereoselective control of allenyl ethers (4 + 3) cycloadditions was necessary and meaningful, which could get a new practical method for the synthesis of optical seven-member carbon rings with oxygen atom substitution. As a continuation of allenyl ethers (4 + 3) cycloadditions, we reported the present progress of stereoselective (4 + 3) cycloadditions by assembling chiral auxiliaries on allene, and the cytotoxicities of the cycloadducts in vitro against a sort of human cancer cell lines were evaluated by MTT assay.
Stereoselective synthesis induced by chiral auxiliaries had been well developed (Whitesell et al., 1985; Potin et al., 1990; Harrington and Tius, 2000; Harrington et al., 2002). As shown in Table 1, six chiral auxiliaries were introduced on allene to explore the optimal stereoselectivity of (4 + 3) cycloadditions with furan as dienes. Using the classic allenyl ethers (4 + 3) reaction condition (KH2PO4, DMDO, CH2Cl2, −30°C) (Huang et al., 2020), the reaction did not give the desired products, but led to a mixture of unidentifiable side-products (entries 1 ~ 2) when induced with Aux-1 and Aux-2. When induced with Aux-3 and Aux-4, (4 + 3) products were obtained with d.r. (50: 50) and 54% yield respectively. In order to improve the stereoselectivity, the situation of Aux-5 induction was further explored. Encouragingly, the cycloadduct was obtained with 60% yield and the d.r. value increased to 84:16 when Aux-5 was induced (entry 5).
Considering the removal of the chiral auxiliary in the later stage and further improving the stereoselectivity, the camphor-derived chiral auxiliary Aux-5 with glycosidic bonds was selected, which was developed to asymmetric Nazarov cyclization by Tius in 2007 (Harrington et al., 2002). Under the induction of the chiral auxiliary, the cycloadduct 3a could be successfully obtained with a yield of 30% and a diastereoselectivity ratio of 100: 0 (entry 6), and an uncyclized allene reduction products was also produced, which led to low yields of cycloaddition products. The possible reason was the high activation energy caused by temperature.
With a highly stereoselective auxiliary Aux-6 in hand, allenyl ethers 1f was used as a common substrate for the (4 + 3) cycloadditions of furans with different substituents to explore the adaptability of its substrate. As shown in Scheme 2, firstly, to improve the reaction efficiency of the (4 + 3) cycloadditions, the temperature was reduced to −78°C with other conditions unchanged, the reaction yield was almost doubled, for instance, 3a were obtained with d.r. (100: 0) and 64% yield respectively. With the optimized conditions in hand, the scopes of regioselectivity and stereoselectivity of allenyl ethers (4 + 3) cycloaddition induced by Aux-6 was explored. Different substituted furans were employed for reaction with 1f. When 2-substituted furans were explored for the (4 + 3) cycloaddition, the carbon chain increased to five carbons, the yield remained between 43% to 53% (entries 2~4). Cycloaddition of 2-methylfuran and 2-ethylfuran with 1f provided cycloadducts 3b and 3c with moderate diastereoselectivity, specifically decreased to 91: 19 and 75: 25, while other alkyl substitutions, remained unchanged. Using methyl 2-furoate and 2-furonitrile as the substrate led to a mixture of unidentifiable isomers and side-product. The cycloadducts can be obtained with moderate yield and the diastereoselectivity was not affected when the second position of furan was substituted by alkyl ether (entries 7~8).
To further study the substrate scope and stereoselectivity of the (4 + 3) cycloadditions, we also employed 3-substituted for the (4 + 3) cycloadditions. The cycloadduct was not obtained by using methyl 3-furoate as the diene, while 3-bromofuran was explored as the substrate, the cycloadduct 3h was obtained at 40% yield with the diastereoselectivity of 100: 0. The absolute configuration of the cycloadducts obtained was established by X-ray analysis of enantiopure samples of compound 3h. The configuration of all other adducts 3a~3g, was established by assuming the same stereochemical outcome for all reactions based on mechanistic analogy.
To demonstrate the synthetic potential of this stereoselective (4 + 3) reaction, the possibility of removing the chiral auxiliary on the cycloadducts was explored. The chiral auxiliary was easily removed from the cycloadducts under acidic conditions to provide α-hydroxyl cycloheptanone with high stereoselectivity (Scheme 3).
Since 2020, breast cancer has become the highest morbidity malignancy in the world, (Sung et al., 2021), an estrogen-dependent (estrogen receptor positive, ER+) human breast cancer cell line T47D was selected to evaluate the antiproliferative activities of these novel cycloadducts with Ribociclib (an approval CDK4/6 inhibitor for advanced ER+ breast cancer treatment) as a positive control. As shown in Table 2, 3a, 3b, 3g, 3h and 4 displayed moderate antiproliferative effects towards T47D cells with inhibitory rates higher than or equal to 50% at 100 μmol/L, while 3c, 3d, 3e and 3f featuring a C2-electron-donor substituent were less potent than their counterparts. This indicated that a long chain electron-donating substituent at C2-position was detrimental to the antiproliferative effects of the novel compounds. In contrary, an electron-withdrawing substituent at C2 (8) or C3 (6) position exhibited more potent inhibitory activities in T47D cells. Intriguingly, compound 4 had the simplest scaffold and its maintenance of antiproliferative activity was observed, showing great potential for further modification.
All reactions were performed in flame-dried glassware under nitrogen atmosphere. Solvents were distilled prior to use. Reagents were used as purchased from Aladdin, Macklin, Innochem, or TLC unless otherwise noted. Chromatographic separations were performed using Silica Gel, AR, 200-300 mesh. 1H and 13C NMR spectra were obtained on a Bruker (Avance) 400 MHz NMR instrument using CDCl3 as the solvent, which was provided by School of Pharmaceutical Sciences, Guizhou University. Infrared spectra were obtained on a Shimadzu FT-IR-8400S spectrometer as KBr pellets. Optical rotations were obtained on a Insmark digital polarimeter using the sodium (589 nm, D line) lamp and are reported as follows: (c = g/100 mL, solvent). TLC analysis was visualized using UV, p-anisoladehyde and phosphomolybdic acid stains. High-resolution mass spectra were obtained using AB SCIEX X500R QTOF. All spectral data obtained for new compounds are reported here.
Biological reagents such as 3-(4,5-Dimethylthiazol-2-yl)-2,5- diphenyltetrazolium bromide (MTT), penicillin and streptomycin were obtained from Sigma. In addition, fetal bovine serum (FBS), phosphate buffered saline (PBS), 1640 medium, DMEM and trypsin were purchased from Biosera and Adriamycin was obtained from EBEWE Pharma. The antiproliferative activity results were recorded on a Bio-Rad microplate reader (Model 680).
Compound AUX-6 was prepared to correspond to literature, (Harrington et al., 2002) and the NMR data was corresponding to ones described in the literature.
As shown in Scheme 4, a pH 7 saturated phosphate buffer was made from H2O (70 mL), NaH2PO4 (3.8 g) and Na2HPO4 (5.6 g). Na3PO4 was added to a portion of the phosphate buffer together with some H2O until pH 8. m-CPBA was dissolved in diethyl ether and this organic phase was stirred for 1 h with a generous quantity of the modified phosphate buffer. The organic phase was separated, washed with brine (1×), dried over MgSO4 and evaporated in small portions at room temperature under reduced pressure. Purified and dried m-CPBA (4.3 g, 24.8 mmol) was suspended / partially dissolved in dichloromethane (19.8 mL) and trifluoromethanesulfonic acid (0.9 mL, 9.9 mmol) was added slowly, finally making a clear and slightly yellow solution. L-Camphor 5 (1.5 g, 9.9 mmol) was added in one portion and the solution left stirring for 3 h at room temperature. The resultant thick reaction mixture was quenched by the slow addition of aqueous NaOH (3 M) until pH 12. The aqueous phase was separated, extracted with EtOAc (3×) and the combined organic extracts were washed with saturated aqueous NaHCO3 (2×), brine (2×), dried over MgSO4. After filtration and concentration, the crude product was purified using silica gel flash column chromatography [eluent: 3.3% EtOAc/Petroleum ether] to give white crystalline lactone 6 (1.2 g, 71% yield). 1H NMR (400 MHz, CDCl3) δ 4.57 – 4.39 (m, 1H), 4.11 (dd, J = 10.8, 1.2 Hz, 1H), 2.20 – 2.04 (m, 2H), 1.97 – 1.66 (m, 3H), 1.18 (s, 3H), 1.10 (s, 3H), 0.98 (s, 3H).
To a solution of lactone 6 (1.2 g, 7.1mmol) in toluene at –78 °C was added DIBAL-H (9.5 mL, 14.2 mmol) via syringe pump for 2 h. After the addition, the reaction mixture was stirred for 1h, quenched with acetone (3.6 mL), warmed to room temperature, diluted with aqueous potassium sodium tartrate (4.5 g, 21.3 mmol in 22.5 mL H2O), stirred for 1 h and then diluted with EtOAc, sat. aq. NaCl and water. The aqueous phase was extracted with EtOAc (3×) and the combined organic extracts were washed with brine (2×), dried over MgSO4. After filtration and concentration, the crude product was purified using silica gel flash column chromatography [eluent: 4% EtOAc/Petroleum ether] to give white and crystalline lactol Aux-6 (1.0 g, 83% yield). 1H NMR (400 MHz, CDCl3) δ 4.97 – 4.85 (m, 1H), 4.07 (dt, J = 10.9, 1.3 Hz, 1H), 3.52 (dd, J = 10.9, 2.5 Hz, 1H), 3.12 – 2.97 (m, 1H), 2.03 – 1.77 (m, 2H), 1.70 – 1.48 (m, 3H), 1.08 (s, 3H), 0.91 (s, 3H), 0.87 (s, 3H).
Crystalline lactol Aux-6 (1.0 g, 5.9 mmol) and tetra-n-butylammonium hydrogen sulfate (0.30 g, 5.2 mmol) were dissolved in CH2Cl2 (23 mL) and cooled to 0°C. Aqueous sodium hydroxide (50%, 23 mL) was cooled to 0°C and added to the solution of the lactol and phase transfer catalyst. The mixture was stirred for 5 min and propargyl bromide (5.0 mL, 5.9 mmol) was added. The thick reaction mixture was stirred vigorously for 6 h at rt and diluted with EtOAc and water. The aqueous phase was extracted with EtOAc (3×), the combined organic extracts were washed with brine (2×), dried over MgSO4 and concentrated under vacuum to give a dark brown oil, which after purification by silica gel flash column chromatography [eluent: 0.5% EtOAc/Petroleum ether] gave a yellow oil of alkyne 7 (1.0 g, 81% yield).1H NMR (400 MHz, CDCl3) δ 4.74 (d, J = 0.9 Hz, 1H), 4.33 (dd, J = 12.2, 2.4 Hz, 2H), 4.04 (d, J = 10.9 Hz, 1H), 3.53 (dd, J = 10.9, 2.5 Hz, 1H), 2.40 (d, J = 2.4 Hz, 1H), 2.07 (ddd, J = 13.2, 9.9, 4.6 Hz, 1H), 1.94 – 1.73 (m, 1H), 1.69 – 1.45 (m, 2H), 1.41 – 1.20 (m, 1H), 1.09 (s, 3H), 0.90 (s, 3H), 0.86 (s, 3H).
Compound 1f was prepared to correspond to literature, (Huang et al., 2020) and the NMR data was corresponding to ones described in the literature.
To a solution of alkyne 7 (1.00 g, 4.8 mmol) in THF (48 mL) was added t-BuOK (1.0 M solution in THF, 7.2 mL, 7.2 mmol) at 0°C. The reaction was stirred at rt for 1 h before being concentrated under reduced pressure. Subsequently, the residue was first suspended in CH2Cl2 and then filtered through CeliteTM. The filtrate was concentrated under reduced pressure and the crude residue was purified using silica gel flash column chromatography [eluent: 0.5% EtOAc/Petroleum ether] to give a yellow oil allenyl ether 1f (0.9 g, 90% yield).1H NMR (400 MHz, CDCl3) δ 6.70 (t, J = 6.0 Hz, 1H), 5.42 (dd, J = 12.9, 6.1 Hz, 2H), 4.82 (s, 1H), 4.06 (d, J = 10.9 Hz, 1H), 3.59 (dd, J = 10.9, 2.4 Hz, 1H), 2.17 (s, 1H), 1.96 – 1.78 (m, 1H), 1.69 – 1.52 (m, 3H), 1.19 – 0.76 (m, 9H).
To a solution of allenyl ether 1f (1.0 equiv) in CH2Cl2 (0.05 M) containing anhydrous 4Å MS were added KH2PO4 (5.0 equiv) and furan 2a~h (10.0 equiv) at –78°C. After which, a dry-ice chilled solution of DMDO (5.0 equiv, 0.08 M solution in CH2Cl2,) was added via syringe pump over 1.5 h. The reaction mixture was stirred at this temperature for another 1 h before being filtered through CeliteTM. The filtrate was concentrated under reduced pressure and the crude residue was purified using silica gel flash column chromatography [eluent: EtOAc/Petroleum ether] to give a desired yellow oil cycloadduct 3a~h.
3a: 26 mg (64%); yellow oil; 1H NMR (400 MHz, CDCl3) δ 6.31 (ddd, J = 26.9, 6.1, 1.7 Hz, 2H), 5.08 (dd, J = 5.2, 1.8 Hz, 1H), 5.00 (d, J = 5.0 Hz, 1H), 4.81 (s, 1H), 4.39 (d, J = 5.2 Hz, 1H), 3.99 (d, J = 10.9 Hz, 1H), 3.50 (dd, J = 10.9, 2.5 Hz, 1H), 2.75 (dd, J = 15.5, 4.9 Hz, 1H), 2.34 (d, J = 15.5 Hz, 1H), 2.06 (td, J = 9.8, 5.0 Hz, 1H), 1.91 – 1.76 (m,1H), 1.67 – 1.45 (m, 3H), 1.06 (s, 3H), 0.94 (s, 3H), 0.89 (s, 3H); 13C NMR (100 MHz, CDCl3) δ 204.5, 134.2, 132.4, 103.2, 82.5, 80.7, 78.2, 68.4, 47.4, 45.8, 45.4, 42.5, 28.5, 26.0, 24.7, 17.7, 13.6; IR (KBr) cm-1 2962m, 2924m, 2869m, 1725m, 1463w, 1361w, 1322w, 1258w, 1175w, 1095s, 1012s, 964s, 804m, 723s; HRMS (ESI+): C17H24O4 for [M+Na]+, calculated 315.1572, found 315.1566; [α]D25 = + 9.921 (c 1.00, CHCl3).
3b: 15 mg (52% yield); yellow oil; 1H NMR (400 MHz, CDCl3) δ 6.26 (dd, J = 5.9, 1.8 Hz, 1H), 6.07 (d, J = 5.9 Hz, 1H), 5.10 – 5.00 (m, 1H), 4.81 (s, 1H), 4.35 (d, J = 5.2 Hz, 1H), 3.98 (d, J = 10.9 Hz, 1H), 3.49 (dd, J = 10.9, 2.5 Hz, 1H), 2.55 (s, 1H), 2.39 (d, J = 15.3 Hz, 1H), 2.10 – 1.99 (m, 1H), 1.91 – 1.77 (m, 1H), 1.63 – 1.52 (m, 3H), 1.49 (s, 3H), 1.05 (s, 3H), 0.93 (s, 3H), 0.88 (s, 3H); 13C NMR (100 MHz, CDCl3) δ 204.97, 137.38, 132.21, 103.07, 84.80, 81.21, 80.96, 68.40, 51.73, 47.42, 45.44, 42.54, 28.46, 25.95, 24.75, 22.77, 17.68, 13.62; IR (KBr) cm-1 2962m, 2927m, 2866m,1725m, 1460w, 1383w, 1325w, 1178w, 1115s, 1076s, 1006s, 830w, 736m, 727m; HRMS (ESI+): C18H26O4 for [ M+Na]+, calculated 329.3918, found 329.17248; = + 17.105, (c 0. 67, CHCl3)
3c: 21 mg (48% yield); yellow oil; 1H NMR (400 MHz, CDCl3) δ 6.27 (d, J = 6.0 Hz, 1H), 6.07 (d, J = 5.8 Hz, 1H), 5.07 (d, J = 5.2 Hz, 1H), 4.82 (s, 1H), 4.37 (d, J = 5.2 Hz, 1H), 3.98 (d, J = 11.0 Hz, 1H), 3.49 (d, J = 11.0 Hz, 1H), 2.55 (d, J = 15.2 Hz, 1H), 2.36 (d, J = 15.3 Hz, 1H), 2.07 (ddt, J = 14.8, 9.4, 5.3 Hz, 2H), 1.87 – 1.76 (m, 2H), 1.65 – 1.46 (m, 3H), 1.06 (s, 3H), 0.98 (t, J = 7.5 Hz, 3H), 0.94 (s, 3H), 0.89 (s, 3H); 13C NMR (100 MHz, CDCl3) δ 205.2, 136.0, 132.5, 103.1, 88.1, 81.6, 80.9, 68.4, 50.0, 47.4, 45.4, 42.5, 28.9, 28.5, 26.0, 24.8, 17.7, 13.6, 8.8; IR (KBr) cm-1 2959m, 2924m, 2850m, 1726m, 1470m, 1169w, 1120s, 1069s, 1008s, 967w, 826w, 804w, 733s; HRMS (ESI+): C19H28O4 for [ M+Na]+, calculated 343.4188, found 343.18769; [α]D25= + 16.221, (c 1.00, CHCl3).
3d: 22 mg (47% yield); yellow oil; 1H NMR (400 MHz, CDCl3) δ 6.26 (dd, J = 5.9, 1.8 Hz, 1H), 6.08 (d, J = 6.0 Hz, 1H), 5.06 (dd, J = 5.2, 1.9 Hz, 1H), 4.82 (s, 1H), 4.37 (d, J = 5.2 Hz, 1H), 3.99 (d, J = 10.9 Hz, 1H), 3.50 (dd, J = 10.9, 2.5 Hz, 1H), 2.54 (s, 1H), 2.38 (s, 1H), 2.11 – 2.03 (m, 2H), 1.87 – 1.80 (m, 1H), 1.77 – 1.70 (m, 2H), 1.62 – 1.55 (m, 2H), 1.51 (m, 2H), 1.06 (s, 3H), 0.97 (s, 3H), 0.94 (m, 3H), 0.90 (d, J = 2.4 Hz, 3H). 13C NMR (100 MHz, CDCl3) δ 205.3, 136.3, 132.2, 103.1, 87.7, 81.6, 80.8, 68.4, 50.3, 47.4, 45.4, 42.5, 38.3, 28.5, 26.0, 24.8, 17.7, 17.4, 14.4, 13.6; IR (KBr) cm-1 2961w, 2930s, 2852w, 1730m, 1604w, 1513w, 1467m, 1393m, 1263m, 1183w, 1085s, 1008s, 962m, 825m, 795m, 764m, 706m, 661m, 559m; HRMS (ESI+): C20H30O4 for [ M+Na]+, calculated 357.4458, found 357.20370; [α]D20= + 140.898, (c 0.20, CHCl3).
3e: 22 mg (43% yield); yellow oil; 1H NMR (400 MHz, CDCl3) δ 6.27 (dd, J = 6.0, 1.8 Hz, 1H), 6.08 (d, J = 6.0 Hz, 1H), 5.12 – 5.03 (m, 1H), 4.82 (s, 1H), 4.37 (d, J = 5.2 Hz, 1H), 3.99 (dd, J = 11.0, 1.3 Hz, 1H), 3.50 (dd, J = 10.9, 2.5 Hz, 1H), 2.56 (d, J = 15.2 Hz, 1H), 2.44 – 2.34 (m, 1H), 2.08 (ddd, J = 12.9, 9.8, 4.7 Hz, 2H), 1.90 – 1.78 (m, 1H), 1.74 – 1.48 (m, 3H), 1.06 (s, 3H), 0.95 (s, 4H), 0.93 – 0.85 (m, 8H); 13C NMR (100 MHz, CDCl3) δ 205.2, 136.4, 132.3, 103.1, 87.7, 81.6, 80.8, 68.4, 50.4, 47.4, 45.5, 42.5, 36.1, 32.1, 28.5, 26.0, 24.8, 23.7, 22.6, 17.7, 14.1, 13.6; IR (KBr) cm-1 2957m, 2924m, 2877m, 1731m, 1492m, 1457m, 1396w, 1362w, 1309w,1274m, 1206w, 1184m, 1122m, 1082s, 972s, 893w, 817w, 738w, 708w, 601w; HRMS (ESI+):C22H34O4 for [ M+Na]+, calculated 385.4998 found 385.47654; [α]D25 = + 121.312, (c 0.10, CHCl3).
3f: 17 mg (35% yield); yellow oil; 1H NMR (400 MHz, CDCl3) δ 6.34 (dd, J = 6.0, 1.8 Hz, 1H), 6.14 (d, J = 6.0 Hz, 1H), 5.12 (d, J = 5.3 Hz, 1H), 4.82 (s, 1H), 4.39 (d, J = 5.2 Hz, 1H), 3.99 (d, J = 10.9 Hz, 1H), 3.66 (s, 2H), 3.61 (dd, J = 7.0, 4.4 Hz, 1H), 3.51 (d, J = 2.5 Hz, 1H), 2.77 (d, J = 15.5 Hz, 1H), 2.38 (d, J = 15.4 Hz, 1H), 2.07 (ddd, J = 13.9, 9.7, 4.7 Hz, 1H), 1.84 (d, J = 6.0 Hz, 1H), 1.65 – 1.49 (m, 3H), 1.06 (s, 3H), 0.94 (s, 3H), 0.92 – 0.85 (m, 9H); 13C NMR (100 MHz, CDCl3) δ 204.9, 134.7, 133.1, 103.2, 87.3, 81.4, 81.2, 72.3, 68.4, 67.4, 47.4, 47.3, 45.4, 42.5, 28.5, 25.9, 24.8, 17.7, 15.0, 13.6; IR (KBr) cm-1 2963m, 2959m, 2857m, 1724m, 1467m, 1387w, 1263m, 1078s, 1011m, 965w,829m, 801m, 794m; HRMS (ESI+): C20H30O5 for [M+Na]+, calculated 373.4448, found 373.19847; [α]D25 = + 3.831, (c 0.10, CHCl3).
3g: 23 mg (45% yield); yellow oil; 1H NMR (400 MHz, CDCl3) δ 6.38 (d, J = 1.8 Hz, 1H), 6.20 (d, J = 6.0 Hz, 2H), 5.16 (dd, J = 5.2, 1.8 Hz, 2H), 5.05 (s, 2H), 4.82 (s, 2H), 4.39 (d, J = 5.2 Hz, 2H), 4.10 – 3.94 (m, 6H), 3.49 (dd, J = 11.0, 2.5 Hz, 2H), 2.76 (d, J = 15.5 Hz, 2H), 2.42 (d, J = 15.5 Hz, 2H), 2.12 – 2.00 (m, 3H), 1.83 (dt, J = 11.8, 5.8 Hz, 2H), 1.63 – 1.50 (m, 5H), 1.06 (s, 6H), 0.94 (s, 7H), 0.90 (d, J = 2.5 Hz, 11H); 13C NMR (100 MHz, CDCl3) δ 204.4, 133.7, 133.4, 103.2, 103.1, 88.2, 81.5, 81.3, 68.4, 65.9, 65.63, 5.40, 45.4, 44.8, 42.5, 28.5, 25.9, 24.7, 17.69, 13.6; IR (KBr) cm-1 2953m, 2918m, 2871m, 2853m, 1726m, 1467w, 1373w, 1315w, 1257w, 1176w, 1109s, 1078s, 1014m, 964m, 938m, 838w, 798w, 740m, 613w, 491w; HRMS (ESI+): C20H28O6 for [ M+Na]+, calculated 387.4278, found 387.17796; = + 73.604, (c 0.12, CHCl3).
3h: 21 mg (40% yield); white solid; mp 80.7~81.2°C; 1H NMR (400 MHz, CDCl3) δ 6.38 (d, J = 2.0 Hz, 1H), 4.97 (d, J = 5.1 Hz, 1H), 4.95 – 4.93 (m, 1H), 4.84 (s, 1H), 4.52 (d, J = 5.1 Hz, 1H), 4.00 (d, J = 10.9 Hz, 1H), 3.52 (dd, J = 10.9, 2.5 Hz, 1H), 2.77 (d, J = 4.8 Hz, 1H), 2.43 (d, J = 15.7 Hz, 1H), 2.24 (ddd, J = 13.8, 9.9, 4.6 Hz, 1H), 1.97 – 1.78 (m, 1H), 1.69 – 1.42 (m, 3H), 1.06 (s, 3H), 0.97 (s, 3H), 0.90 (s, 3H); 13C NMR (100 MHz, CDCl3) δ 203.4, 133.2, 123.1, 103.2, 83.5, 81.9, 79.4, 68.6, 47.5, 45.8, 45.4, 42.4, 28.2, 25.9, 24.7, 17.7, 13.6; IR (KBr) cm-1 2953m, 2927m, 2869m, 2847m, 1736m, 1463w, 1178w, 1111s, 1076s, 1008m, 967s, 829m, 736m, 701m; HRMS (ESI+): C17H23BrO4 for [ M+Na]+, calculated 394.2608, found 393.06668; = + 2.450, (c 1.00, CHCl3).
p-TsOH (17 mg, 0.089 mmol) was added to cycloadduct 3a (26 mg, 0.089 mmol) in MeOH (1.8 mL) and the mixture was stirred at room temperature for 2h, Pyridine (11 µL, 0.13 mmol) was added and the mixture was concentrated under reduced pressure. The crude residue was purified using silica gel flash column chromatography [eluent: 20% EtOAc/Petroleum ether] to give compound 4 (11 mg, 88% yield) as a white solid. 1H NMR (400 MHz, CDCl3) δ 6.32 (t, J = 1.5 Hz, 2H), 5.10 (td, J = 3.6, 1.7 Hz, 2H), 4.39 (s, 1H), 2.91 (dd, J = 15.0, 4.9 Hz, 1H), 2.55 – 2.45 (m, 1H). 13C NMR (100 MHz, CDCl3) δ 206.5, 134.6, 131.8, 80.7, 78.9, 78.5, 45.1; HRMS (ESI+): C7H8O3 for [M+Na]+, calculated 163.0366, found 163.0367; = + 103.195, (c 0.10, CHCl3).
T47D cells were maintained in RPMI 1640 supplemented with 10% FBS, and 100 units/ml penicillin-G and 100mg/ml streptomycin and 0.37% NaHCO3 and were incubated at 37°C in humidified air containing 5% CO2.
T47D cells were planted into 96-well micro-plates at a density of 7×104 cells/mL (100mL per well). After overnight incubation at 37°C, 100 µmol/L concentrations of test cycloadducts were added to the wells for preliminary screening and 5 different concentrations of test cycloadducts were added for GI50 testing. Cycloadducts were dissolved in DMSO before being diluted in the growth medium. The concentration of DMSO in the wells did not exceed 0.5%. Cells were further incubated for 96 h, at the end of the incubation time, fresh medium containing 0.5 mg/mL of MTT were added. Plates were incubated for another 4 h at 37 °C, the media was removed and formazan crystals formed in the cells were dissolved in 200mL of DMSO. Optical density was measured at 490 nm with DMSO as blank using an enzyme labeling instrument (Tecan, Switzerland). The inhibition ratio of viability for each concentration of the compounds was calculated with respect to the control and GI50 values were estimated with the software Graph pad Prism 7.04 Each experiment was repeated 3 times and the results were summarized in Table 3 as mean ± SD (inmM).
A novel stereoselectivity allenyl ether (4 + 3) cycloaddition was explored for the construction of substituted cycloheptanones. The valuable approach was investigated by the camphor-derived chiral auxiliary inducing to substrates. Furthermore, the chiral auxiliary was removed via p-TsOH to generate corresponding enantiomer from the α-hydroxyl cycloheptanone in high yields. The bioactivities of these cycloadducts were evaluated, which had moderate inhibitory effect on breast cancer cell line T-47D.
The original contributions presented in the study are included in the article/Supplementary Material. Further inquiries can be directed to the corresponding authors.
SH and HZ designed the experiment. YC, WL, HZ and YQ wrote the manuscript. WL, YS and AL performed experiments and analyzed the data. SH, YC and HZ supervised the entire project. All authors contributed to the article and approved the submitted version.
This work was supported by the National Natural Science Foundation of China (Grant No. 21861010, 22061008), the State Key Laboratory of Functions and Applications of Medicinal Plants, Guizhou Medical University (No. FAMP202102K), the Science and Technology Foundation of Guizhou Province (QianKeHe JiChu ZK [2021] YiBan 039), the Science and Technology Foundation of Guizhou Province [2020]1Y108.
The authors declare that the research was conducted in the absence of any commercial or financial relationships that could be construed as a potential conflict of interest.
All claims expressed in this article are solely those of the authors and do not necessarily represent those of their affiliated organizations, or those of the publisher, the editors and the reviewers. Any product that may be evaluated in this article, or claim that may be made by its manufacturer, is not guaranteed or endorsed by the publisher.
The Supplementary Material for this article can be found online at: https://www.frontiersin.org/articles/10.3389/fpls.2022.1087899/full#supplementary-material
Atanasov, A. G., Zotchev, S. B., Dirsch, V. M., International Natural Product Sciences, T, Supuran, C. T. (2021). Natural products in drug discovery: advances and opportunities. Nat. Rev. Drug Discov 20 (3), 200–216. doi: 10.1038/s41573-020-00114-z
Duh, C. Y., Wang, S. K., Chia, M. C., Chiang, M. Y. (1999). A novel cytotoxic norditerpenoid from the Formosan soft coral sinularia inelegans. Tetrahedron Lett. 40 (33), 6033–6035. doi: 10.1016/s0040-4039(99)01194-6
Evans, F., Kinghorn, A. D. (1977). A comparative phytochemical study of the diterpenes of some species of the genera Euphorbia and Elaeophorbia (Euphorbiaceae). Bot. J. Linn. Soc 74 (1), 23–35. doi: 10.1111/j.1095-8339.1977.tb01163.x
Flaschenträger, B., v. Wolffersdorff, R. (1934). Über den giftstoff des crotonöles. 1. die säuren des crotonöles. Helv Chim. Acta 17 (1), 1444–1452. doi: 10.1002/hlca.193401701179
Foley, D. A., Maguire, A. R. (2010). Synthetic approaches to Bicyclo[5.3.0]decane sesquiterpenes. Tetrahedron 66 (6), 1131–1175. doi: 10.1016/j.tet.2009.11.045
Fuchigami, R., Namba, K., Tanino, K. (2012). Concise [4 + 3] cycloaddition reaction of pyrroles leading to tropinone derivatives. Tetrahedron Lett. 53 (43), 5725–5728. doi: 10.1016/j.tetlet.2012.07.130
Hardinger, S. A., Bayne, C., Kantorowski, E., McClellan, R., Larres, L., Nuesse, M.-A. (1995). Bis(sulfonyl) ketones: A new oxyallyl cation source. J. Org. Chem. 60 (5), 1104–1105. doi: 10.1021/jo00110a005
Harmata, M., Fletcher, V. R., Claassen, II, R.J. (1991). Alkoxyvinyl thionium ions in intramolecular [4 + 3]-cycloaddition reactions. J. Am. Chem. Soc 113 (26), 9861–9862. doi: 10.1021/ja00026a028
Harrington, P. E., Murai, T., Chu, C., Tius, M. A. (2002). Asymmetric cyclopentannelation: Camphor-derived auxiliary. J. Am. Chem. Soc 124 (34), 10091–10100. doi: 10.1021/ja020591o
Harrington, P. E., Tius, M. A. (2000). Asymmetric cyclopentannelation. chiral auxiliary on the allene. Org. Lett. 2 (16), 2447–2450. doi: 10.1021/ol0001362
Harvey, A. L. (2008). Natural products in drug discovery. Drug Discov Today 13 (19-20), 894–901. doi: 10.1016/j.drudis.2008.07.004
Hayakawa, R., Shimizu, M. (2000). Novel carbon–carbon bond formation reaction of methoxyallene oxide promoted by TiI4. Org. Lett. 2 (25), 4079–4081. doi: 10.1021/ol006729u
He, S., Hsung, R. P., Presser, W. R., Ma, Z.-X., Haugen, B. J. (2014). An approach to cyclohepta[b]indoles through an allenamide (4 + 3) cycloaddition–grignard cyclization–chugaev elimination sequence. Org. Lett. 16 (8), 2180–2183. doi: 10.1021/ol5006455
Huang, X., Thor, W., Feng, X., Kang, L., Yang, M., Lee, C.-S., et al. (2020). (4 + 3) cycloadditions of allenyl ether-derived oxygen-stabilized oxyallyls with furans. Org. Chem. Front. 7 (2), 255–260. doi: 10.1039/C9QO01349K
Kawamura, S., Chu, H., Felding, J., Baran, P. S. (2016). Nineteen-step total synthesis of (+)-phorbol. Nature 532 (7597), 90–93. doi: 10.1038/nature17153
Kim, K. E., Kim, A. N., McCormick, C. J., Stoltz, B. M. (2021). Late-stage diversification: A motivating force in organic synthesis. J. Am. Chem. Soc 143 (41), 16890–16901. doi: 10.1021/jacs.1c08920
Lebwohl, M., Swanson, N., Anderson, L. L., Melgaard, A., Xu, Z., Berman, B. (2012). Ingenol mebutate gel for actinic keratosis. N. Engl. J. Med. 366 (11), 1010–1019. doi: 10.1056/NEJMoa1111170
Lee, K. H., Cha, J. K. (1999). A new approach to phorbol by [4 + 3] oxyallyl cycloaddition and intramolecular heck reaction. Org. Lett. 1 (3), 523–525. doi: 10.1021/ol990709e
Li, G., Lou, M., Qi, X. (2022). A brief overview of classical natural product drug synthesis and bioactivity. Org. Chem. Front. 9 (2), 517–571. doi: 10.1039/d1qo01341f
Lohse, A. G., Hsung, R. P. (2011). (4 + 3) cycloaddition reactions of nitrogen-stabilized oxyallyl cations. Chem. Eur. J. 17 (14), 3812–3822. doi: 10.1002/chem.201100260
Masuya, K., Domon, K., Tanino, K., Kuwajima, I. (1998). Highly regio- and stereoselective [3 + 2] cyclopentanone annulation using a 3-(Alkylthio)-2-siloxyallyl cationic species. J. Am. Chem. Soc 120 (8), 1724–1731. doi: 10.1021/ja972879x
Montana, A. M., Grima, P. M. (2001). New methodology for the [4 + 3] cycloaddition reactions: Generation of oxyallyl cations from α, α'-diiodoketones under sonochemical or thermal conditions. Tetrahedron Lett. 42 (44), 7809–7813. doi: 10.1016/s0040-4039(01)01671-9
Myers, A. G., Barbay, J. K. (2001). On the inherent instability of α-amino α’-fluoro ketones. evidence for their transformation to reactive oxyvinyliminium ion intermediates. Org. Lett. 3 (3), 425–428. doi: 10.1021/ol006931x
Newman, D. J., Cragg, G. M. (2020). Natural products as sources of new drugs over the nearly four decades from 01/1981 to 09/2019. J. Nat. Prod. 83 (3), 770–803. doi: 10.1021/acs.jnatprod.9b01285
Nguyen, T. V., Hartmann, J. M., Enders, D. (2013). Recent synthetic strategies to access seven-membered carbocycles in natural product synthesis. Synthesis 45 (07), 845–873.
Nicolaou, K. C., Rigol, S., Yu, R. (2019). Total synthesis endeavors and their contributions to science and society: A personal account. CCS Chem. 1 (1), 3–37. doi: 10.31635/ccschem.019.20190006
Okamoto, T., Shibata, M., Karanjit, S., Nakayama, A., Yoshida, M., Namba, K. (2018). Direct synthesis of polycyclic tropinones by a condensation- [4 + 3] -cycloaddition cascade reaction. Chem. Eur. J. 24 (38), 9508–9513. doi: 10.1002/chem.201802011
Potin, D., Dumas, F., D'Angelo, J. (1990). New chiral auxiliaries: Their use in the asymmetric hydrogenation of β-acetamidocrotonates. J. Am. Chem. Soc 112 (9), 3483–3486. doi: 10.1021/ja00165a036
Saez, J. A., Arno, M., Domingo, L. R. (2003). Lewis Acid-catalyzed [4 + 3] cycloaddition of 2-(Trimethyl silyloxy)acrolein with furan. insight on the nature of the mechanism from a DFT analysis. Org. Lett. 5 (22), 4117–4120. doi: 10.1021/ol035652h
Sáez, J. A., Arnó, M., Domingo, L. R. (2005). Lewis Acid induced [4 + 3] cycloadditions of 2-silyloxyacroleins. insights on the mechanism from a DFT analysis. Tetrahedron 61 (31), 7538–7545. doi: 10.1016/j.tet.2005.05.067
Sung, H., Ferlay, J., Siegel, R. L., Laversanne, M., Soerjomataram, I., Jemal, A., et al. (2021). Global cancer statistics 2020: GLOBOCAN estimates of incidence and mortality worldwide for 36 cancers in 185 countries. CA Cancer J. Clin. 71 (3), 209–249. doi: 10.3322/caac.21660
Wang, H., Wang, X., Liu, L., Qin, G., Kang, T. (2015). Tigliane diterpenoids from the Euphorbiaceae and Thymelaeaceae families. Chem. Rev. 115 (9), 2975–3011. doi: 10.1021/cr200397n
Wei, L., Xiong, H., Hsung, R. P. (2003). The emergence of allenamides in organic synthesis. Acc Chem. Res. 36 (10), 773–782. doi: 10.1021/ar030029i
Wender, P. A., Lee, H. Y., Wilhelm, R. S., Williams, P. D. (1989). Studies on tumor promoters. 7. the synthesis of a potentially general precursor of the tiglianes, daphnanes, and ingenanes. J. Am. Chem. Soc 111 (24), 8954–8957. doi: 10.1021/ja00206a049
Wender, P. A., McDonald, F. E. (1990). Studies on tumor promoters. 9. a second-generation synthesis of phorbol. J. Am. Chem. Soc 112 (12), 4956–4958. doi: 10.1021/ja00168a050
Wender, P. A., Rice, K. D., Schnute, M. E. (1997). The first formal asymmetric synthesis of phorbol. J. Am. Chem. Soc 119 (33), 7897–7898. doi: 10.1021/ja9706256
Whitesell, J. K., Chen, H. H., Lawrence, R. M. (1985). trans-2-Phenylcyclohexanol. a powerful and readily available chiral auxiliary. J. Org. Chem. 50 (23), 4663–4664. doi: 10.1021/jo00223a055
Keywords: (4+3) cycloadditions, stereoselectivity, chiral auxiliaries, allenyl ethers, anti-breast cancer activity
Citation: Lei W, Song Y, Long A, Que Y, He S, Zhong H and Chen Y (2022) Stereoselective (4 + 3) cycloadditions of allenyl ethers-furans by chiral auxiliaries inducing and evaluation of anti-breast cancer activity. Front. Plant Sci. 13:1087899. doi: 10.3389/fpls.2022.1087899
Received: 02 November 2022; Accepted: 14 November 2022;
Published: 21 December 2022.
Edited by:
Zhiping Che, Henan University of Science and Technology, ChinaReviewed by:
Shaojun Zheng, Jiangsu University, ChinaCopyright © 2022 Lei, Song, Long, Que, He, Zhong and Chen. This is an open-access article distributed under the terms of the Creative Commons Attribution License (CC BY). The use, distribution or reproduction in other forums is permitted, provided the original author(s) and the copyright owner(s) are credited and that the original publication in this journal is cited, in accordance with accepted academic practice. No use, distribution or reproduction is permitted which does not comply with these terms.
*Correspondence: Shuzhong He, c3poZUBnenUuZWR1LmNu; Hang Zhong, aHpob25nX2d6dV9lZHVAMTI2LmNvbQ==; Yang Chen, eWNoZW4xQGd6dS5lZHUuY24=
Disclaimer: All claims expressed in this article are solely those of the authors and do not necessarily represent those of their affiliated organizations, or those of the publisher, the editors and the reviewers. Any product that may be evaluated in this article or claim that may be made by its manufacturer is not guaranteed or endorsed by the publisher.
Research integrity at Frontiers
Learn more about the work of our research integrity team to safeguard the quality of each article we publish.