- College of Agriculture, Shihezi University, Shihezi, China
Scirpus planiculmis, an important weed in rice and cotton fields, stresses crop growth and development, leading to yield loss. However, it is unclear how stressed plants respond to this weed. In this study, we analysed the stress effect of S. planiculmis on cotton under different weed densities, competition periods, and distribution conditions from the perspective of morphogenesis, physiological metabolism and crop yield. The effect of a low dose of herbicide on the relationship between cotton and S. planiculmis was also explored. The results showed that plant height, stem diameter, fresh weight, root length, boll number, single boll weight and yield of cotton all decreased with increasing S. planiculmis density and damage. The spatial distribution of S. planiculmis had no significant effect on plant height, stem diameter, fresh weight or root length of cotton, but crop yield loss decreased with increasing distance. S. planiculmis stress altered cotton chlorophyll, soluble protein and malondialdehyde (MDA) content, and protective enzyme activities. Compared with superoxide dismutase (SOD) and peroxidase (POD) activities, catalase (CAT) activity was increased under different S. planiculmis stress conditions. Therefore, we concluded that CAT plays a key role in protecting enzymes involved in defence responses. Under low-dose herbicide action, the activities of protective enzymes were increased, which helped cotton plants to resist S. planiculmis stress. The results revealed that regulating protective enzyme activities is important in cotton responses to S. planiculmis stress.
Introduction
Cotton (Gossypium hirsutum L.) is an economically important crop widely planted in many parts of the world, and China is the world’s largest cotton producer (Li et al., 2021). In 2021, the cotton planting area in China reached 3.03 million hectares (National Bureau of Statistics, 2021). Weeds are one of the most important factors restricting development of the cotton industry (Wang et al., 2015). The incidence of weed damage in cotton fields can be up to 56%, causing annual yield losses of 14−16% (Li et al., 2019). Weeds are an important source of biological stress for cotton, competing for light, nutrients, water and space, and this stress occurs throughout the growth period (Vulchi et al., 2022). Weeds compete with cotton, and they cast shade, severely inhibiting growth, resulting in short cotton plants, yellow leaves, and even buds falling off, thus reducing cotton yield (Wang et al., 2015). In order avoid damage caused by weeds, it is necessary to clarify the competitive effects weeds have on cotton plants in the field.
Weed stress factors affecting cotton growth include species, density, competition period, and spatial distribution (Wang et al., 2015). Numerous studies have reported competition between weeds and cotton, examples of which include giant ragweed (Ambrosia trifida), goosegrass (Eleusine indica), redroot pigweed (Amaranthus retroflexus), velvetleaf (Abutilon theophrasti), and black nightshade (Solanum nigrum). (Barnett and Steckel, 2013; Ma et al., 2015a; Ma et al., 2015b; Ma et al., 2016; Zhang et al., 2022a). These studies provided insight into competition between weeds and cotton flowers at the population level. Thus, if weeds emerge earlier or at the same time as cotton seedlings, and the growth rate of the aboveground canopy of weeds exceeds that of cotton, shading will occur, and cotton will be at a competitive disadvantage, which will eventually lead to a decline in cotton yield (Ma et al., 2015b; Ma et al., 2016). Conversely, if weeds emerge later, or if they are small and adopt a creeping form, they will be shaded by cotton, and the competitiveness of the weeds is relatively weak (Ma et al., 2015a). In addition, weeds that emerge simultaneously with or earlier than cotton are more competitive than those that emerge later in the cotton growth cycle, causing greater losses in cotton yield (Barnett and Steckel, 2013). By studying the competitive effects between weeds and cotton, the critical period and density of weed control were determined, as was the control threshold (Charles et al., 2019a; Charles et al., 2019b; Charles et al., 2020; Zhang et al., 2022a). However, there are few reports on how cotton responds to weed stress from the perspective of weed and cotton physiology (Zhang et al., 2022a). Therefore, analysing the stress effects exerted by weeds on cotton from the perspective of physiological metabolism could help to further clarify the competition mechanism between weeds and cotton.
Defence responses of plants are triggered by various biotic stresses (Steinbrenner et al., 2022). In these complex stress response systems, basic metabolism, specialized metabolism and protective enzymes are known to play important roles (Ling et al., 2022; Swinnen et al., 2022). For example, in the face of attack by most herbivorous insects, plant protective enzyme activities and basal metabolic capacity are enhanced, effectively improving tolerance and reducing damage by herbivorous insects (Castano-Duque et al., 2018; Gao et al., 2022). Although weeds cannot induce defence responses of plants through direct damage, they can stimulate defence systems through allelopathy (Li et al., 2022a). Allelopathy affects plant cell structure, photosynthesis, respiration, hormone content, receptor enzyme activity and gene expression by releasing chemicals (Kato-Noguchi et al., 2017; Qin et al., 2018; Siyar et al., 2019; Mardani et al., 2019). Allelopathic plants reduce interference of allelopathy by regulating related tissue structure, basal metabolism, secondary metabolism, protective enzyme activity and other defensive measures (Šoln et al., 2022). Especially in plant communities with population competition, plants reduce the adverse effects of allelopathy on competition through defensive responses (Uesugi et al., 2019; Chia and Bittencourt-Oliveira, 2021). Competition between weeds and plants is obviously related to this process. However, when studying competition between weeds and plants, the competition mechanism from the perspective of plant defence metabolism is not usually investigated, even though it could be useful.
Scirpus planiculmis Fr. Schmidt is a perennial herb of the family Cyperaceae. In China, Korea, Japan, and other countries and regions, S. planiculmis is a common malignant weed in rice fields (Lee et al., 1991; Choi et al., 2000a). In Europe, it is considered to be a potential plant for widespread expansion (Hroudová et al., 2014). Previous studies have determined the economic threshold of S. planiculmis in rice fields (Kwon et al., 2008; Kwon et al., 2011) and screened chemical control agents (Choi et al., 2000b; Hwang et al., 2006; Park et al., 2011). However, with changes in cropping systems and cultivation patterns, S. planiculmis has become a major weed in cotton fields in Xinjiang and the Yellow River basin in China (Fang et al., 2016; Huang et al., 2020), leading to cotton yield reductions of 1.86−61.50% (Cai et al., 2020a). Due to the diversification of the breeding methods for S. planiculmis, plants can be sexually propagated through seeds and asexually propagated through bulbs (Yang et al., 2021; Zhang et al., 2022b). Strong saline-alkaline tolerance (Hroudová et al., 2014; An et al., 2021) and plastic film mulching in cotton fields provides a suitable growth environment (Hroudová et al., 2007; Liu et al., 2016), resulting in a density of up to 1.5×106 S. planiculmis plants per hm2 in cotton fields in Xinjiang, which severely inhibits nutrient uptake and growth of cotton (Cai et al., 2020a; Cai et al., 2021).
Compared with C3 plants (cotton), C4 plants (S. planiculmis) are better at absorbing CO2, water, nutrients, and light (Li and Yang, 2020). Therefore, during competition between the two, cotton is weaker than S. planiculmis. Our previous results showed that plant height, stem diameter, number of nodes on the main stem, boll number, and fiber quality of cotton were reduced with continuous extension of damage time and increase in the density of S. planiculmis, which eventually led to a decrease in cotton yield (Cai et al., 2020b; Cai et al., 2021). During the cotton flowering and boll-forming stage, S. planiculmis reduced potassium and soluble sugar content in cotton leaves under different population densities, and inhibited the accumulation of nutrients in cotton (Cai et al., 2020a). Further studies showed that the rhizosphere exudates of S. planiculmis reduced the content of cytokinin and auxin in cotton leaves, and significantly inhibited the growth of underground parts of cotton, including main root length, lateral root length and fresh weight (Cai, 2020c). However, how cotton responds to S. planiculmis stress through physiological metabolism has not been reported.
In this study, we conducted a 2-year experiment in 2019−2020, and these were field experiment. (1) Different densities of S. planiculmis were established, and the effects on the growth and physiological metabolism of cotton were measured at different stages (seedling, budding, flowering and boll); (2) the competition period of S. planiculmis and cotton was controlled to determine the effects of S. planiculmis on the growth and physiological metabolism of cotton; (3) by changing the distribution distance between S. planiculmis and cotton, effects on the growth and physiological metabolism of S. planiculmis on cotton were determined; (4) the physiological metabolic responses of cotton to S. planiculmis were further explored under herbicide mediation. The results illuminate the physiological response mechanism of cotton to S. planiculmis from the perspective of plant defences.
Materials and methods
Experimental field and plants
The experiment was carried out at the Shihezi University Educational Test Site, Xinjiang, China (44°C22′ N, 86°C05′ E) from 2019 to 2020. The soil was loam, pH 7.8, containing 7.22 mg kg-1 organic carbon, 1.43 g kg-1 total nitrogen, 52.58 mg kg-1 alkaline hydrolysis nitrogen, 166.33 mg kg-1 available potassium and 7.27 mg kg-1 available phosphorus. The soil porosity was 45.17%, and the field capacity was 35.02%. The land had been growing cotton for more than 5 years, and the base number of S. planiculmis was large, with a density range of 50−80 plants/m2.
The cotton variety used was Xinluzao 60 (upland cotton, provided by the Institute of Cotton Research, Chinese Academy of Agricultural Sciences, Zhengzhou, China), and seeds were treated with imidacloprid seed coating agent (Gaucho Bayer Crop Science; 600 mL/100 kg seeds). Seeds were sown on 21 April, 2019 and 25 April, 2020. Seeding was carried out under a mulch film at a width of 1.5 m. Rows were spaced at (20 + 60 + 20) cm, cotton plants were spaced ≥9 cm, and the planting density was 150,000 plants/hm2.
Experimental field management
Prior to sowing, the experimental field was covered with a plastic film, and two drip irrigation lines (Beijing Luckrain Inc., Beijing, China) were installed under each plastic film. The drip irrigation line had an inner diameter of 2.5 cm, an emitter distance of 50 cm, a flow rate of 2.7 L h-1, and 4500 kg hm-2 of oil residue (13% N, 2% P2O5 and 16% K2O) was applied as base fertiliser. In addition, 75 kg hm-2 of urea (46% N) and 216 kg hm-2 of triple superphosphate (45% P2O5) were applied throughout the growth period. Drip irrigation under the film was applied eight times during the whole cotton growth period. The irrigation time in 2020 was 53, 65, 76, 87, 97, 108, 119 and 127 days after cotton emergence, respectively. In 2021, it was 58, 67, 78, 89, 99, 110, 117 and 125 days after cotton emergence, respectively. Additionally, 98% Mepiquat chloride (MC; Sichuan Guoguang Agrochemical Co., Ltd.,Chengdu, China) was applied to control vegetative growth. MC solution at 208 g hm-2 concentration was sprayed five times throughout the whole cotton growth period. A 6 g hm-2 MC solution was sprayed from the cotyledon stage to the two-leaf stage, and 11 g hm-2 was sprayed at the 5- to 7-leaf stage. Moreover, 26, 45 and 120 g hm-2 MC was sprayed 2 days before the first irrigation, 2 days before the second irrigation, and 5−7 days after topping. To hasten crop maturation and defoliation, 600 g hm-2 50% thidiazuron defoliant (Sichuan Guoguang Agrochemical Co., Ltd.) combined with 3000 mL hm-2 40% ethephon (Sichuan Guoguang Agrochemical Co., Ltd.) was used in both years. Pest and disease control were carried out according to standard management practices, mainly to control cotton aphids, thrips and spider mites. Weeds were manually removed every 30 days retaining only experimental S. planiculmis.
Chemicals
Trifloxysulfuron (75%) (Swiss Syngenta Crop Protection Co., Ltd., Switzerland) and prometryn (40%) (Zhejiang Changxing First Chemical Co., Ltd., Changxing, China) were applied as wettable powders.
Effects of S. planiculmis density on growth of S. planiculmis and cotton
Based on the occurrence of S. planiculmis in cotton fields and preliminary test results (Cai et al., 2020a; Cai et al., 2021), we set S. planiculmis densities of 0, 20, 40, 60, 80 and 100 plants/m2, totalling six treatments. The experiment used a completely randomized block design with an area of 2.3 m2 (2.3 m × 1 m) per plot. The weed densities established for a whole plot (Swanton et al., 2015). Each treatment was repeated five times, totalling 30 plots. After cotton emergence, the emergence rate of S. planiculmis in each plot was investigated. If the density of S. planiculmis was higher than the set density, excess S. planiculmis plants were removed. If the density of S. planiculmis was lower than the set density, S. planiculmis plants were transplanted to the set density. During the whole growth period of cotton, other weeds in the plot were artificially removed leaving only S. planiculmis.
Plant height, stem diameter, fresh weight and root length of S. planiculmis in each plot were measured at the squaring stage of cotton, and leaves of S. planiculmis were collected. Levels of chlorophyll, soluble protein, malondialdehyde (MDA) and the activities of SOD, POD and CAT enzymes were determined in the laboratory. Six S. planiculmis plants were measured from each plot, and 30 S. planiculmis plants were measured for each treatment. Plant height, stem diameter, fresh weight and root length of cotton in each plot were measured at the seedling, budding, flowering and boll stages, and cotton leaves were collected. Levels of chlorophyll, soluble protein, MDA, and the activities of SOD, POD and CAT enzymes were measured in the laboratory. Six cotton plants were measured from each plot, and 30 cotton plants were measured for each treatment. At harvest, the number of cotton bolls, single boll weight, and yield were measured for each treatment. All remaining cotton plats in each plot were measured.
Effects of planting time of S. planiculmis on the growth of S. planiculmis and cotton
In the experiment, S. planiculmis seedlings were planted in April (April 21, 2019 and April 22, 2020), May (May 21, 2019 and May 2020), and June (June 21, 2019 and June 22, 2020). The planting density was set at 60 plants/m2 (Cai et al., 2020b). Based on the frequency of weeding in cotton fields and the results of preliminary experiments (Cai et al., 2020a), we set three competition periods for S. planiculmis and cotton: 0 (control, no S. planiculmis), 30 and 60 days (Swanton et al., 2015). Therefore, there were nine treatments in the experiment, and each treatment was repeated five times, totalling 45 plots, and each plot area was 2.3 m2 (2.3 m × 1 m). During the experiment, seedlings of S. planiculmis that died within 1 week were replanted, the density of S. planiculmis was kept at the set value, and weeds remaining in the plot were removed. Plant height, stem diameter, fresh weight and root length under different S. planiculmis planting times were measured on June 30, and leaves were collected to determine the contents of chlorophyll, soluble protein, MDA and the activities of SOD, POD and CAT enzymes. Six S. planiculmis plants were measured per plot, and 30 S. planiculmis plants were collected for each treatment. Plant height, stem diameter, fresh weight and root length of cotton were measured at 0, 30 and 60 days after planting. The contents of chlorophyll, soluble protein, MDA and the activities of SOD, POD and CAT enzymes were determined. Six cotton plants were measured from each plot, and 30 cotton plants were measured for each treatment. At harvest, the number of cotton bolls, single boll weight, and the cotton yield under different S. planiculmis planting times were measured.
Effects of S. planiculmis spatial distribution on the growth of S. planiculmis and cotton
After cotton emergence, the distance between S. planiculmis and cotton was set to 0, 5, 10, 15 and 20 cm, totalling five treatments. Each treatment was repeated five times, with 25 plots covering 2.3 m2 (2.3 m × 1 m) per plot. The number of S. planiculmis planted at all distances was eight (with cotton at the centre, a S. planiculmis plant was planted at each of the eight compass points (east, west, south, north, northeast, southeast, northwest and southwest) at different distances (Swanton et al., 2015). During the experiment, seedlings of S. planiculmis without emergence and death within 1 week were replanted in a timely manner. At the 5-leaf stage of S. planiculmis, plant height, stem diameter, fresh weight and root length of S. planiculmis and cotton were measured, and leaves were collected to determine chlorophyll, soluble protein, MDA content and SOD, POD and CAT enzyme activities. Six S. planiculmis and six cotton plants were measured from each plot, and 30 S. planiculmis and 30 cotton plants were included for each treatment. At harvest, the number of cotton bolls, single boll weight, and cotton yield were measured.
Effects of spraying herbicides on the physiological metabolism of cotton and S. planiculmis
According to the results of previous pesticide screening (Peng et al., 2012), at the 5-leaf stage of S. planiculmis, herbicide (0.015 g L-1 75% trifloxysulfuron + 4 g L-1 40% prometryn) was evenly sprayed using a 3WBD-20 L knapsack electric sprayer at a water amount of 500 kg hm-2. After 5 days, the contents of chlorophyll, soluble protein, MDA and the activities of SOD, CAT and POD enzymes in leaves of cotton and S. planiculmis were determined. Six S. planiculmis and six cotton plants were measured for each plot, and 30 S. planiculmis and 20 cotton plants were included for each treatment.
Analysis of plant height, stem diameter, root length and fresh weight of cotton and S. planiculmis
Twenty cotton plants were randomly selected from each treatment for analysis of plant height, stem diameter, root length and fresh weight. Plant height was determined as the length from the stem base to the growing point. Stem diameter was measured with a Vernier calliper. Root length was the length of the main root. Fresh weight was measured with an electronic balance.
Analysis of chlorophyll, soluble protein and MDA levels and protective enzyme activities in cotton and S. planiculmis
Chlorophyll content was measured using the acetone ethanol method (Arnon, 1949). Soluble protein was determined using the G-250 dye colorimetric method (Bradford, 1976). The MDA content was determined based on the thiobarbituric acid method (Dhindsa et al., 1981). CAT, POD and SOD activities were determined using the guaiacol method (Chance and Maehly, 1955), the UV absorption method (Amalo et al., 1994) and the riboflavin-NBT method (Giannopolities and Ries, 1977), respectively.
Analysis of boll number, single boll weight and yield of cotton
Seed cotton from each plot was hand-picked on 15 October, sun-dried, and weighed. One hundred fully opened bolls were sampled to calculate individual boll weight and lint percentage. Boll number was determined by counting bolls (> 2 cm in diameter) for each plant on 19 September and 21 September in 2019 and 2020, respectively.
Statistical analysis
Microsoft Excel 2012 was used for data processing and plotting. SPSS 20.0 was used for all statistical analyses, yielded mean values and standard errors. The LSD method in one-way ANOVA and t-test were used to test for significance between differences in means.
Results
Effects of population density of S. planiculmis on growth and physiological indices of S. planiculmis
With increasing S. planiculmis density, plant height tended to increase, and stem diameter tended to decrease; root length, fresh weight, MDA content and CAT activity first increased then decreased; chlorophyll content showed the opposite trend to soluble protein content and POD activity, while SOD activity first decreased then increased in S. planiculmis (Figure 1). When the density was 100 plants m-2, plant height was the highest (26.24 cm) and stem diameter was the lowest (2.16 mm; Figure 1A, B). When the density was 60 plants m-2, the fresh weight and root length of S. planiculmis reached maximum values of 2.97 g and 5.94 cm, respectively (Figure 1C, D). At this time, the chlorophyll content was also highest (1.40 mg g-1), 21.91% higher than that of 80 plants m-2 (p <0.05; Figure 1E). The highest soluble protein content was 274.53 mg g-1 when the density was 80 plants m-2 (Figure 1F). The MDA content was lowest at a density of 20 plants m-2, and highest at 40 plants m-2, at 0.043 and 0.06 μmol g-1, respectively (Figure 1G). When the density was 60 plants m-2, SOD and POD activities of S. planiculmis were lowest, at 8.67, and 616.73 U min−1 g−1, respectively (Figure 1H, I). When the density of S. planiculmis was 80 plants m-2, CAT activity was highest (20.18 U min−1 g−1), 18.11% higher than that of 60 plants m-2 (p <0.05; Figure 1J).
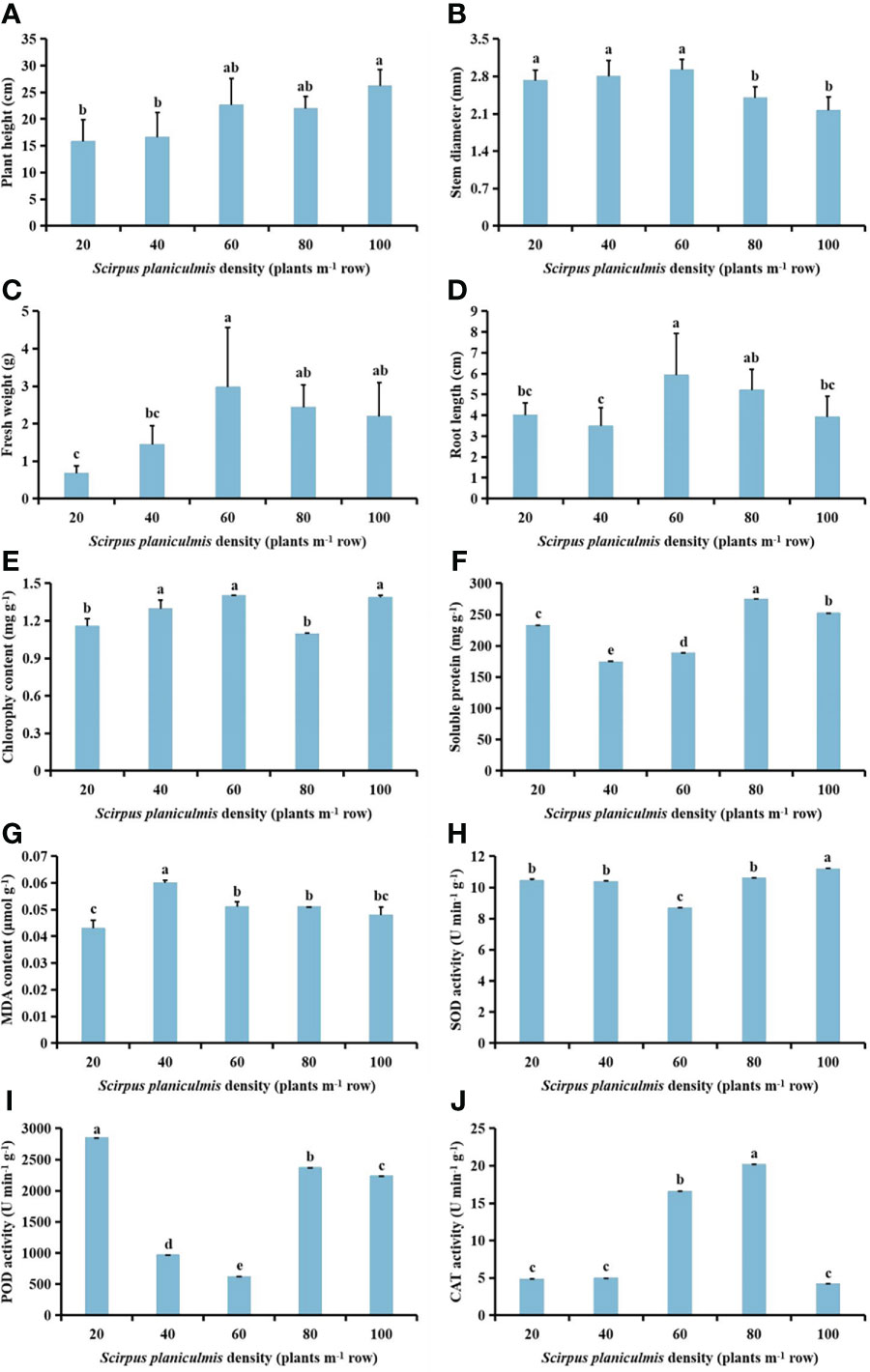
Figure 1 Effects of different Scirpus planiculmis densities on (A) plant height, (B) stem diameter, (C) fresh weight, (D) root length, (E) chlorophyll, (F) soluble protein (G) and malondialdehyde (MDA) content, and (H) superoxide dismutase (SOD), (I) peroxidase (POD) and (J) catalase (CAT) activities in S. planiculmis. The mean value ± standard data were presented in the figure. Different alphabetical letters indicate the significant differences between different S. planiculmis density (p <0.05).
Effects of S. planiculmis population density on agronomic traits and physiological indices in cotton
With increasing S. planiculmis density, plant height, stem diameter, fresh weight and root length of cotton at the seedling stage (SS) were barely affected, while plant height, stem diameter, fresh weight and root length at the budding stage (BS), and flowering and boll stage (FS) showed a decreasing trend (Figure 2A-D). When the densities of S. planiculmis were 20, 40 and 60 plants m-2, the chlorophyll content of cotton at the seedling, budding, flowering and boll stages was the lowest, 17.24%, 36.51% and 75.35% lower than that of controls (0 plants m-2), respectively (p <0.05; Figure 2E). The soluble protein content of cotton at each stage reached the lowest value at a S. planiculmis density of 100 plants m-2, 25.13%, 40.50% and 90.94% lower than that of controls (0 plants m-2), respectively (p <0.05; Figure 2F). At the flowering and boll stage, the MDA content under all densities was significantly lower than that of controls (0 plants m-2), and when the density of S. planiculmis was 80 plants m-2, the MDA content was lowest, 92.31% lower than that of controls (p <0.05, Figure 2G). At each growth stage, the SOD activity of cotton under all densities of S. planiculmis was lower than that of controls (0 plants m-2), while CAT activity was higher than that of controls (Figure 2H, J). POD activity at the seedling stage was significantly lower than that of controls (0 plants m-2), but at the budding, flowering and boll stages POD activity was increased significantly, reaching a maximum at 40 and 20 plants m-2, respectively, increased by 78.96% and 84.97% compared with controls (Figure 2I).
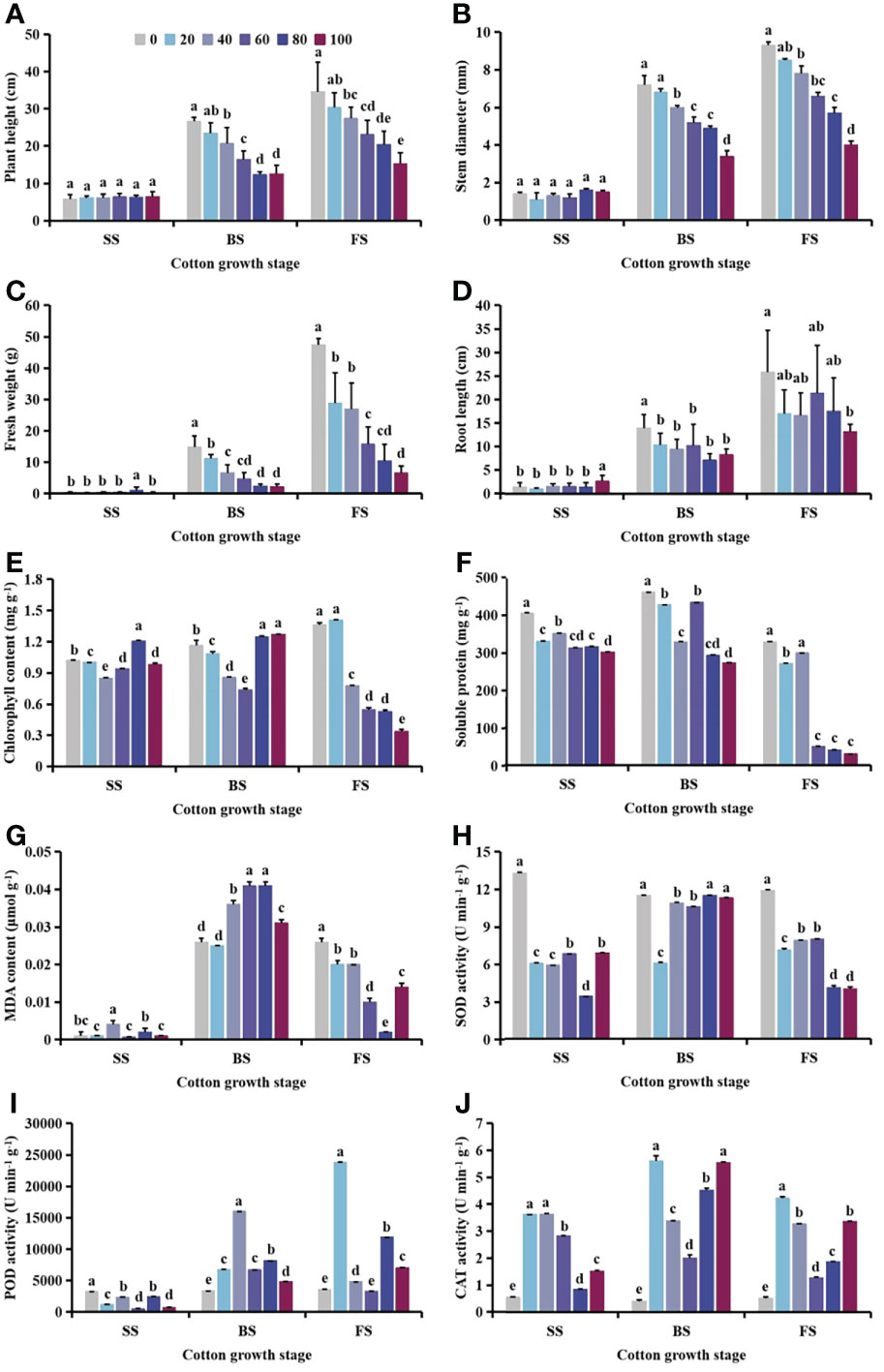
Figure 2 Under seedling, budding, and flowering and boll stages of cotton, the (A) plant height, (B) stem diameter, (C) fresh weight, (D) root length, (E) chlorophyll, (F) soluble protein (G) and malondialdehyde (MDA) content, and (H) superoxide dismutase (SOD). (I) peroxidase (POD) and (J) catalase (CAT) activities of cotton at different Scirpus planiculmis densities. SS, seedling stage, BS, budding stage, FS, flowering and boll stage. Results are mean ± standard error (p <0.05). The mean value ± standard data were presented in the figure. Different alphabetical letters indicate the significant differences between different S. planiculmis density (p <0.05).
Effects of growth stage on agronomic traits and physiological indices in cotton under different S. planiculmis population density
Plant height, stem diameter, fresh weight and root length of cotton increased with the growth of cotton under different densities of S. planiculmis (Figures 3A-D). When the density of S. planiculmis was ≤20 plants m-2, the chlorophyll content of cotton increased with the growth of cotton; when the density of S. planiculmis was 40−60 plants m-2, the chlorophyll content of cotton decreased with the growth of cotton; when the density of S. planiculmis was ≥80 plants m-2, the chlorophyll content of the budding stage was higher than that of the other stages, especially when the density of S. planiculmis was 100 plants m-2; the chlorophyll content of the budding stage was 23.05% and 73.56% higher than that of the seedling stage and the flowering and boll stage (p <0.05; Figure 3E). When the densities of S. planiculmis were 0, 20 and 60 plants m-2, the soluble protein content in the budding stage was significantly higher than in other stages, while the soluble protein content in cotton decreased with the growth of cotton at densities of 40, 80 and 100 plants m-2 (Figure 3F). At different densities of S. planiculmis, the MDA content in cotton at the seedling stage was lowest, and that in the budding stage was highest (Figure 3G). When the density of S. planiculmis was ≥40 plants m-2, SOD activity in cotton at the budding stage was significantly higher than that in other stages (Figure 3H). When the density of S. planiculmis was 0 plants m-2, there was no significant change in POD and CAT activities of cotton at different stages. When the density was 20, 80 and 100 plants m-2, POD activity was highest at the flowering and boll stage, and CAT activity was highest at the budding stage (Figures 3I, J).
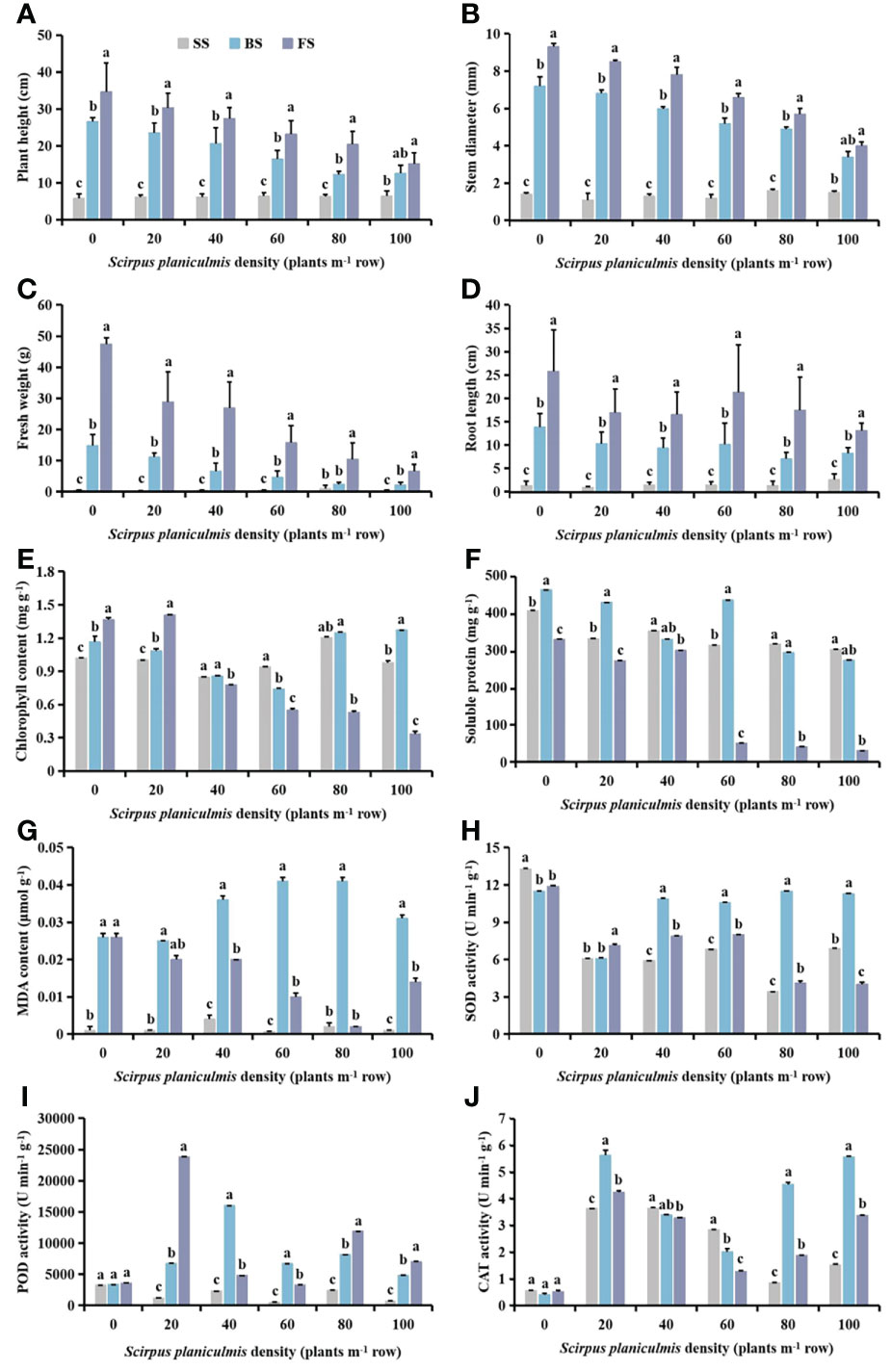
Figure 3 Under different Scirpus planiculmis densities, the (A) plant height, (B) stem diameter, (C) fresh weight, (D) root length, (E) chlorophyll, (F) soluble protein and (G) malondialdehyde (MDA) content, and (H) superoxide dismutase (SOD). (I) peroxidase (POD) and (J) catalase (CAT) activities of cotton at seedling, budding, and flowering and boll stages, SS, seedling stage; BS, budding stage, FS, flowering and boll stage. The mean value ± standard data were presented in the figure. Different alphabetical letters indicate the significant differences between different cotton growth stage (p <0.05).
Effects of S. planiculmis population density on yield in cotton
With increasing density of S. planiculmis, the boll number, boll weight and yield of cotton showed a downward trend (Figure 4). When the density of S. planiculmis was 100 plants m-2, the number of cotton bolls was lowest, 36.56−41.76% lower than that of controls (0 plants/m2; Figure 4A). Compared with controls, single boll weight decreased by 37.85−38.07% and cotton yield decreased by 61.50−71.22% (Figures 4B, C).
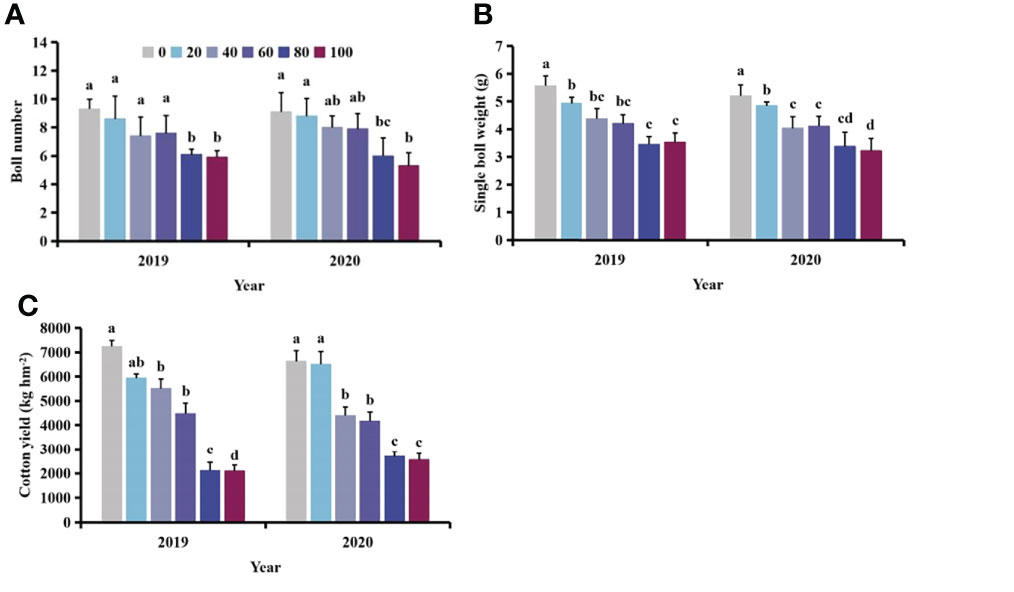
Figure 4 Effects of different Scirpus planiculmis densities on cotton (A) boll number, (B) single boll weight and (C) cotton yield. The mean value ± standard data were presented in the figure. Different alphabetical letters indicate the significant differences between different S. planiculmis density (p <0.05).
Effects of planting time of S. planiculmis on growth and physiological indices of S. planiculmis
With delayed planting time, plant height, stem diameter, fresh weight, root length, and chlorophyll, soluble protein and MDA contents of S. planiculmis in June were significantly lower than in April (p <0.05), while the activities of SOD, POD and CAT in May were higher than in April and June (Figure 5). Compared with S. planiculmis planted in April, plant height, stem diameter, fresh weight and root length of S. planiculmis planted in June were decreased by 32.11%, 19.46%, 69.09% and 48.33%, respectively (p <0.05; Figures 5A-D), while chlorophyll, soluble protein and MDA contents were decreased by 36.84%, 59.87% and 56.06%, respectively (p <0.05, Figures 5E-G). The activities of SOD, POD and CAT of S. planiculmis planted in June were decreased by 13.58%, 49.21% and 74.54%, respectively, compared with those planted in May (Figures 5H-J).
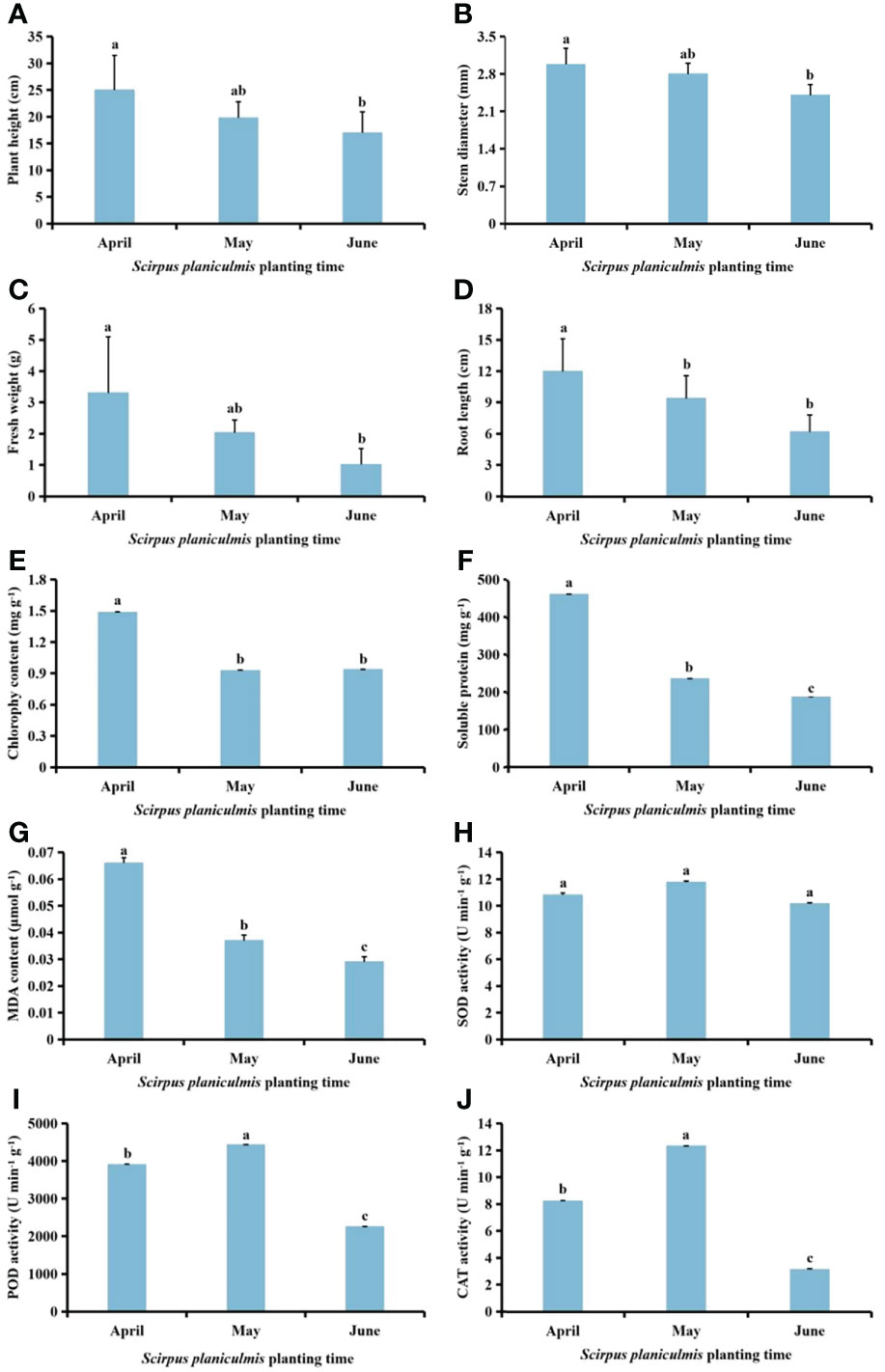
Figure 5 Effects of Scirpus planiculmis planting time on (A) plant height, (B) stem diameter, (C) fresh weight, (D) root length, (E) chlorophyll, (F) soluble protein and (G) malondialdehyde (MDA) content, and (H) superoxide dismutase (SOD), (I) peroxidase (POD) and (J) catalase (CAT) activities in S. planiculmis. The mean value ± standard data were presented in the figure. Different alphabetical letters indicate the significant differences between different S. planiculmis planting time (p <0.05).
Effects of competition periods of S. planiculmis on agronomic traits and physiological indices in cotton
Among different planting times of S. planiculmis, planting in April had no significant effect on plant height, stem diameter, fresh weight, root length or chlorophyll content (p >0.05; Figures 6A-E). Compared with controls (0 days), soluble protein and MDA contents and POD activity of cotton were significantly decreased after competition with S. planiculmis for 60 and 30 days (p <0.05), while soluble protein and MDA contents and POD activity of cotton were lowest at 30 days, at 312.20 mg g-1, 0.003 μmol g−1 and 350.25 U min−1 g−1, respectively (Figures 6F, G, I). SOD and CAT activities were significantly increased, and they were highest at 60 days, at 7.67 and 5.29 U min−1 g−1, respectively (Figure 6H, J). In May, plant height, stem diameter, fresh weight, root length and chlorophyll content of cotton decreased with increasing competition duration, compared with controls (0 days), and plant height, stem diameter, fresh weight, root length and chlorophyll content of cotton were decreased by 52.05%, 27.50%, 84.34%, 22.62% and 9.6%, respectively (p <0.05; Figures 6A-E). The soluble protein and MDA contents and POD activity of cotton were lowest at 30 days, at 366.20 mg g-1, 0.031 μmol g−1 and 3366.68 U min−1 g−1, respectively (Figures 6F, G, I). There were no significant differences in SOD activity among different treatments (p >0.05), but CAT activity increased with extended competition duration, by 88.08% and 78.62%, respectively, at 30 and 60 days (p <0.05; Figures 6H, J). In June, compared with controls (0 days), plant height, stem diameter, fresh weight, root length and chlorophyll content of cotton seedlings following competition with S. planiculmis for 30 and 60 days were decreased (Figures 6A-E). Soluble protein and MDA contents and CAT activity were lowest at 30 days, 13.29%, 31.03%, and 41.57% lower than those of controls (0 days), respectively (Figures 6F, G, J). SOD activity was decreased by 14.37% and 20.71% (p <0.05), and POD activity was increased by 30.96% and 29.10% at 30 and 60 days, respectively (p <0.05; Figures 6H, I).
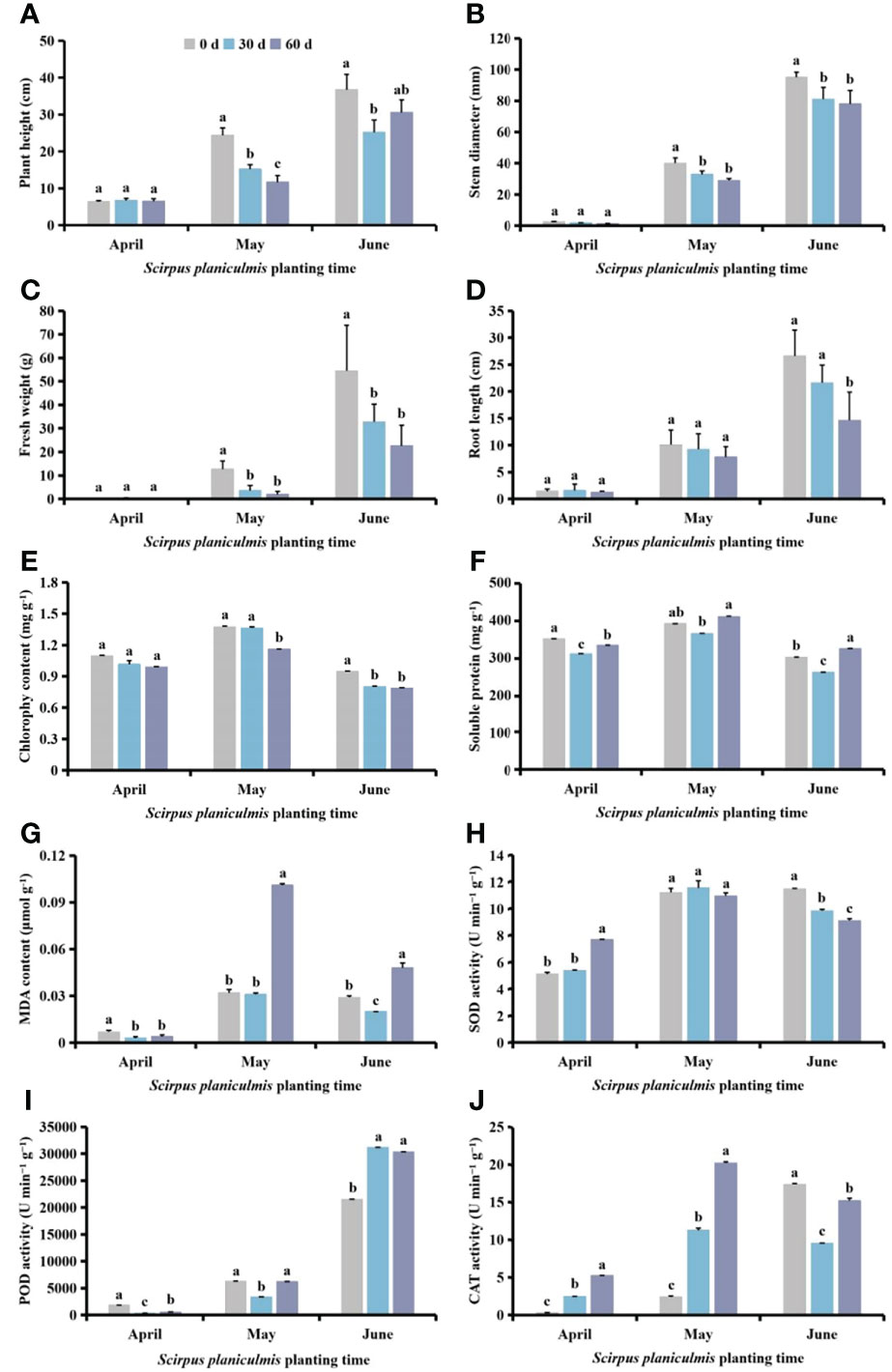
Figure 6 Under different Scirpus planiculmis planting time, the (A) plant height, (B) stem diameter, (C) fresh weight, (D) root length, (E) chlorophyll, (F) soluble protein and (G) malondialdehyde (MDA) content, and (H) superoxide dismutase (SOD), (I) peroxidase (POD) and (J) catalase (CAT) activities in cotton at different competition periods The mean value ± standard data were presented in the figure. Different alphabetical letters indicate the significant differences between different competition periods (p <0.05).
Effects of planting time of S. planiculmis on agronomic traits and physiological indices in cotton
Following different competition periods, plant height, stem diameter, fresh weight and root length of cotton increased with delayed S. planiculmis planting time. Plant height, stem diameter, fresh weight, root length and chlorophyll content of cotton planted in April and May were significantly lower than for those planted in June (p <0.05, Figures 7A-D). The chlorophyll, soluble protein and MDA contents of cotton in May were significantly higher than those in April and June (p <0.05; Figures 7E-G). The activities of SOD, POD and CAT in cotton were lowest in April, significantly lower than those in May and June (p <0.05; Figures 7H-J).
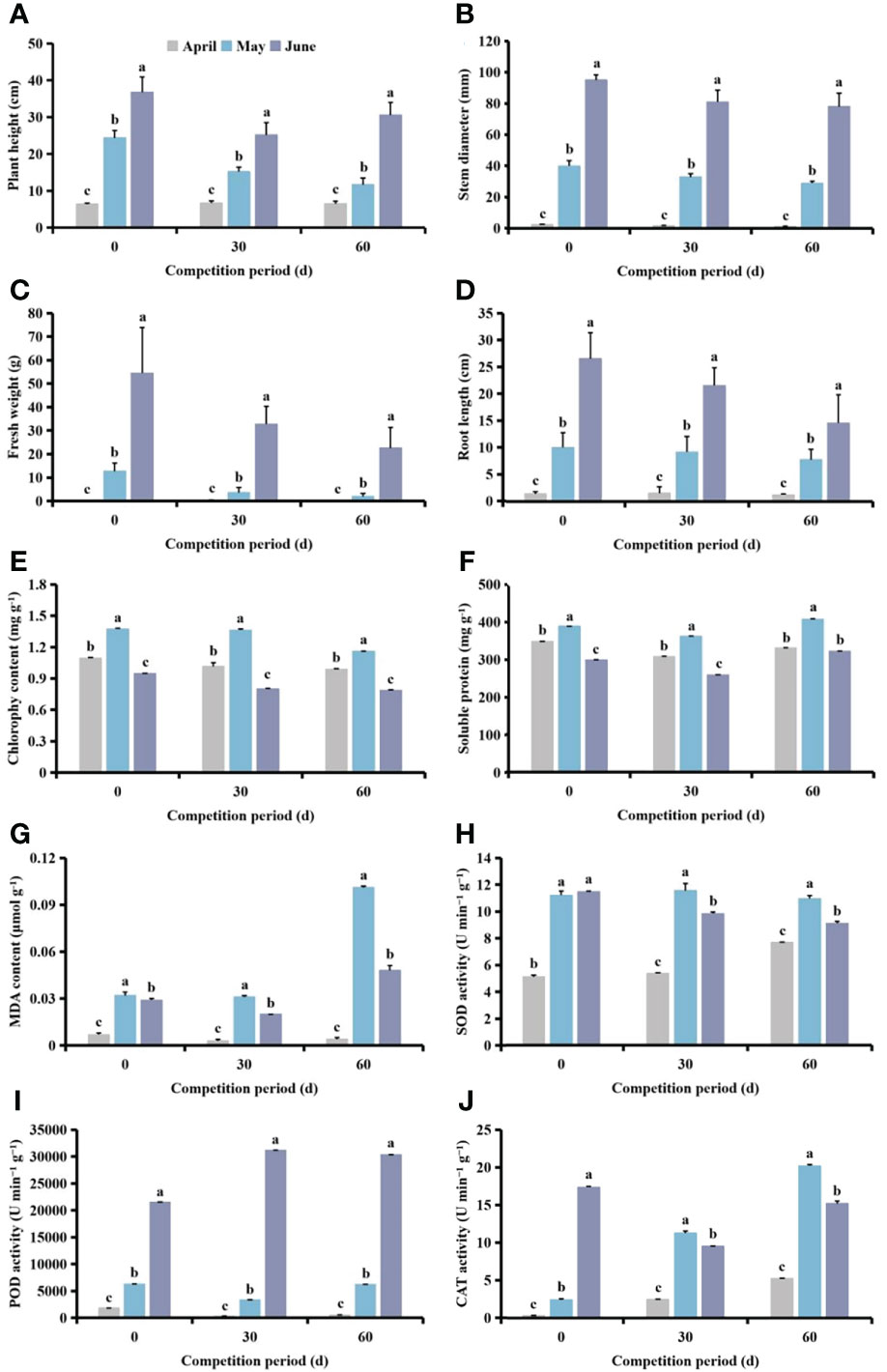
Figure 7 Under different competition periods, the (A) plant height, (B) stem diameter, (C) fresh weight, (D) root length, (E) chlorophyll, (F) soluble protein and (G) malondialdehyde (MDA) content, and (H) superoxide dismutase (SOD) (I) peroxidase (POD) and (J) catalase (CAT) activities in cotton at different Scirpus planiculmis planting time. The mean value ± standard data were presented in the figure. Different alphabetical letters indicate the significant differences between different different Scirpus planiculmis planting time (p <0.05).
Effects of competition periods of S. planiculmis on yield in cotton
Following different competition periods with S. planiculmis, the number of cotton bolls, single boll weight and yield of cotton following competition for 60 days were lowest, 13.06−17.73%, 17.89−19.12% and 17.02−19.50% lower than those of controls (0 days), respectively (p <0.05; Figure 8).
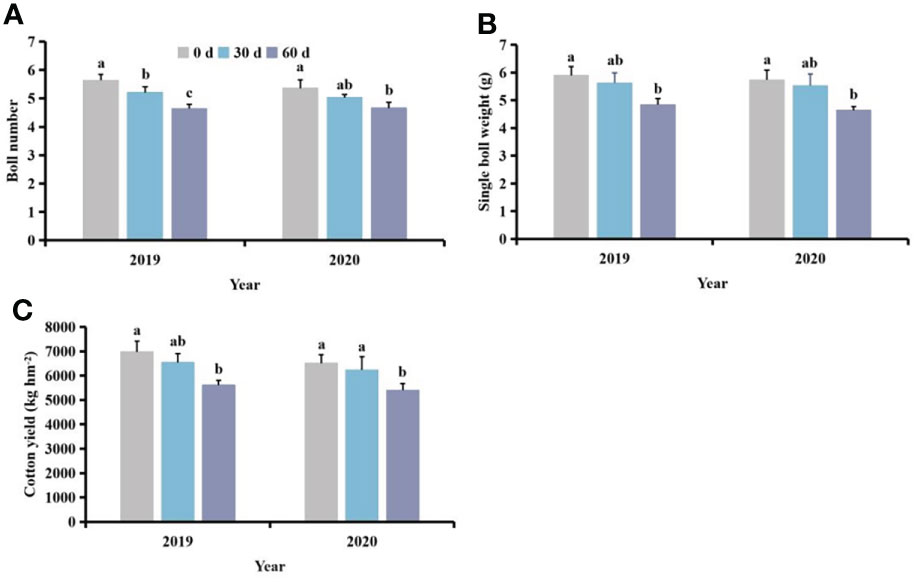
Figure 8 Effects of different competition periods on cotton (A) boll number, (B) single boll weight, and (C) cotton yield. The mean value ± standard data were presented in the figure. Different alphabetical letters indicate the significant differences between different competition periods (p <0.05).
Effects of spatial distribution of S. planiculmis on agronomic traits and physiological indices in cotton and S. planiculmis
The distance between cotton and S. planiculmis plants had no significant effect on plant height, stem diameter, fresh weight and root length of cotton, but with increasing distance, plant height and root length of S. planiculmis decreased, and stem diameter and fresh weight increased (Figures 9A-D). There was no significant difference in chlorophyll content of S. planiculmis at different distances (p >0.05), but when the distance was 5 cm, the chlorophyll content of cotton was lowest, 28.50% lower than that at 0 cm (p <0.05, Figure 9E). Compared with a distance of 0 cm, the soluble protein content of cotton at 5−20 cm distance was significantly increased, and that at 15 cm was 30.28% higher than that at 0 cm (p <0.05). When the distance was 20 cm, the soluble protein content of S. planiculmis was lowest, 76.85% lower than that at 0 cm (p <0.05; Figure 9F). When the distance between S. planiculmis and cotton was 15 cm, the MDA content of S. planiculmis and cotton was highest, 0.019 and 0.021 μmol g−1, respectively (Figure 9G). With differences in distance, cotton and S. planiculmis showed opposite trends (Figure 9H). When the distance was 15−20 cm, POD activity of cotton and S. planiculmis was significantly higher than that at 0 cm (p <0.05), and POD activity of cotton and S. planiculmis was lowest at 10 cm, at 7484.42, and 466.23 μmol/g/min, respectively (Figure 9I). Compared with 0 cm, CAT activity of cotton and S. planiculmis at a distance of 5−20 cm was increased significantly (p <0.05). When the distance was 15 cm, CAT activity of cotton was highest, 92.03% higher than that at 0 cm (p <0.05). When the distance was 5 cm, CAT activity of S. planiculmis was highest, 87.80% higher than that at 0 cm (p <0.05; Figure 9J).
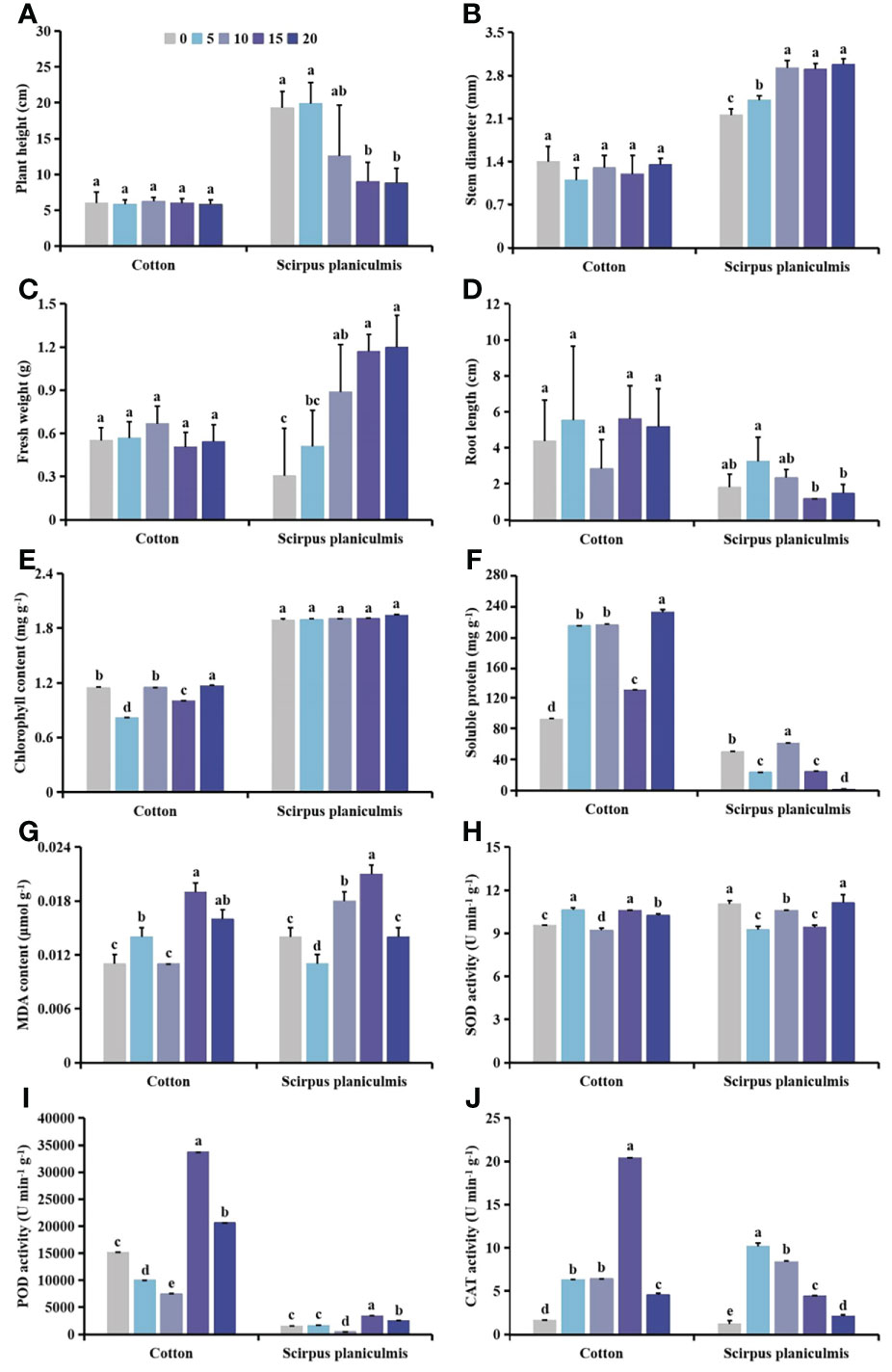
Figure 9 The (A) plant height, (B) stem diameter, (C) fresh weight, (D) root length, (E) chlorophyll, (F) soluble protein and (G) malondialdehyde (MDA) content, and (H) superoxide dismutase (SOD), (I) peroxidase (POD) and (J) catalase (CAT) activities in cotton and S. planiculmis with different spatial distribution of Scirpus planiculmis. The mean value ± standard data were presented in the figure. Different alphabetical letters indicate the significant differences between different distances (p <0.05).
At different distances, plant height and stem diameter of S. planiculmis were significantly smaller than those of cotton (Figures 10A, B). When the distance was ≥15 cm, fresh weight of cotton was significantly lower than that of S. planiculmis (p <0.05), while root length of cotton was significantly higher than that of S. planiculmis (p <0.05; Figures 10C, D). With increasing distance between S. planiculmis and cotton, the chlorophyll content of S. planiculmis was significantly higher than that of cotton, while the soluble protein content of cotton was significantly higher than that of S. planiculmis (p <0.05; Figures 10E, F). When the distance was >15 cm, there was no significant difference in MDA content between S. planiculmis and cotton (p >0.05; Figure 10G). When the distance was 0, 10 and 20 cm, SOD activity of S. planiculmis was higher than that of cotton. When the distance was 5 and 15 cm, SOD activity of S. planiculmis was lower than that of cotton (p <0.05; Figure 10H). POD activity of cotton was higher than that of S. planiculmis at each distance (p <0.05; Figure 10I). When the distance was 0 cm, there was no significant difference in CAT activity between S. planiculmis and cotton (p >0.05), while at 5−10 cm CAT activity of S. planiculmis was higher than that of cotton (p <0.05), and at 15−20 cm CAT activity of S. planiculmis was lower than that of cotton (p <0.05; Figure 10J).
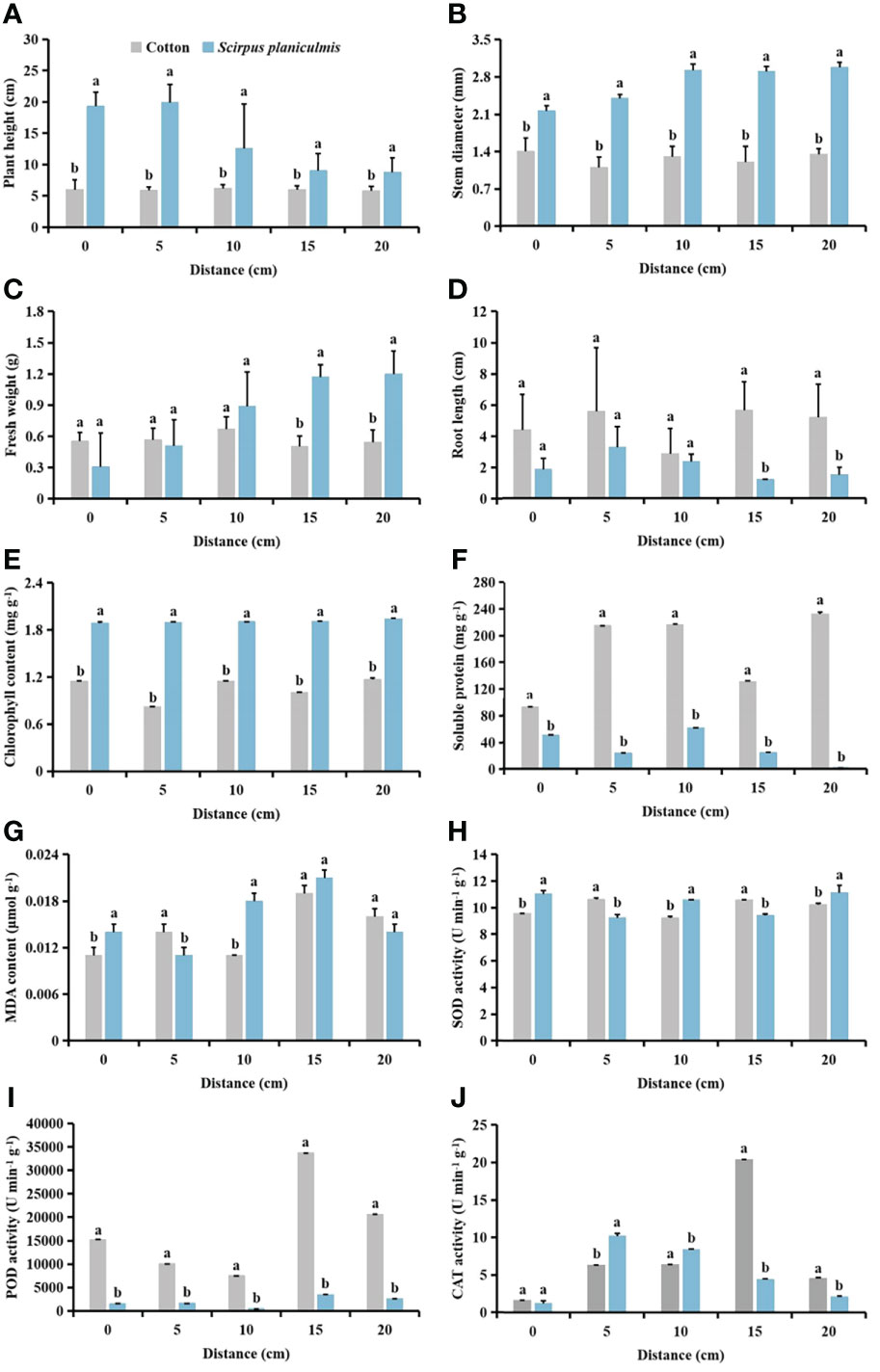
Figure 10 Under different spatial distribution of Scirpus planiculmis, the (A) plant height, (B) stem diameter, (C) fresh weight, (D) root length, (E) chlorophyll, (F) soluble protein and (G) malondialdehyde (MDA) content, and (H) superoxide dismutase (SOD). (I) peroxidase (POD) and (J) catalase (CAT) activities in cotton and S. planiculmis. The mean value ± standard data were presented in the figure. Different alphabetical letters indicate the significant differences between cotton and S. planiculmis (p <0.05).
Effects of spatial distribution of S. planiculmis on yield in cotton
With increasing distance between S. planiculmis and cotton, the effect of S. planiculmis on boll number, single boll weight and yield of cotton decreased (Figure 11). Compared with the distance of 20 cm, when the distance was 0 cm, the boll number and yield of cotton decreased by 35.90%-36.00% and 41.33%-47.08%, respectively (p <0.05; Figures 11A, C). The distance between S. planiculmis and cotton had no significant effect on the single boll weight of cotton (p >0.05; Figure 11B).
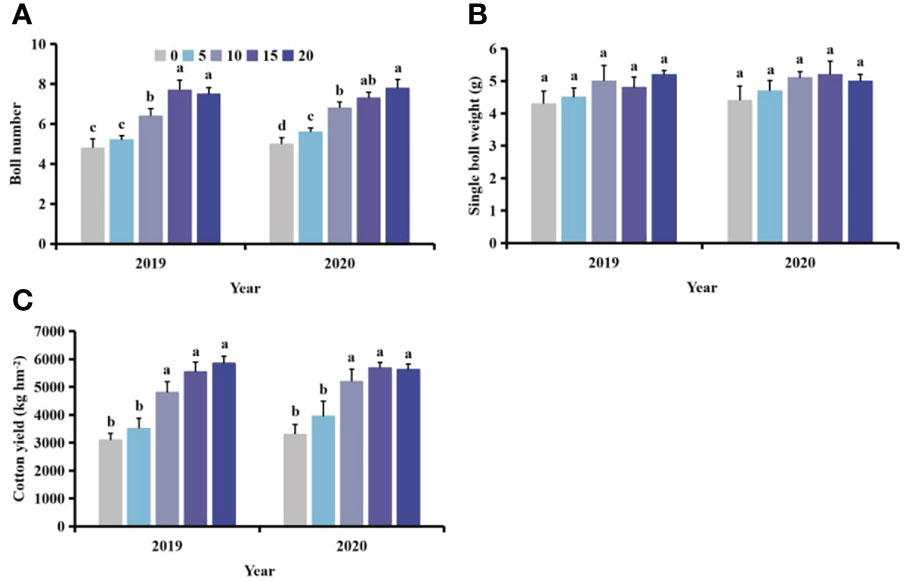
Figure 11 Effects of different spatial distributions of Scirpus planiculmis on cotton (A) boll number, (B) single boll weight and (C) cotton yield. The mean value ± standard data were presented in the figure. Different alphabetical letters indicate the significant differences between different distances (p <0.05).
Effects of spraying 0.015 g L-1 75% trifloxysulfuron + 4 g L-1 40% prometryn on physiological indices of cotton and S. planiculmis
After spraying herbicides, when the distance between S. planiculmis and cotton was 10 cm, the chlorophyll content of cotton was higher than that of S. planiculmis, increased by 13.77% (p <0.05; Figure 12A). Under different distance conditions, the soluble protein of cotton was higher than that of S. planiculmis (p <0.05; Figure 12B). When the distance was 15 cm, the MDA content of S. planiculmis was 72.38% higher than that of cotton (p <0.05; Figure 12C). SOD activity of S. planiculmis was 31.87% higher than that of cotton only at a distance of 10 cm (p <0.05; Figure 12D). At all distances, POD activity of S. planiculmis was higher than that of cotton (p <0.05; Figure 12E). When the distance was 0 cm, there was no significant difference in CAT activity between cotton and S. planiculmis (p >0.05), while at 5−10 cm CAT activity of S. planiculmis was higher than that of cotton, and at 15−20 cm the CAT activity of S. planiculmis was lower than that of cotton (p <0.05; Figure 12F). With increasing distance between S. planiculmis and cotton, the chlorophyll content of S. planiculmis increased (Figure 13A). Compared with 0 cm, the soluble protein content of cotton was decreased significantly at 5−20 cm (p <0.05; Figure 13B). As the distance between S. planiculmis and cotton increased, the MDA content of S. planiculmis at 5−20 cm was higher than that at 0 cm (p <0.05; Figure 13C). When the distance was 20 cm, SOD activity of cotton and S. planiculmis was highest, at 16.19, and 15.01 U min−1 g−1, respectively (Figure 13D). Compared with 0 cm, the POD activity of cotton was significantly increased at 5−20 cm, while the POD activity of S. planiculmis was significantly decreased (p <0.05; Figure 13E). Compared with 0 cm, the CAT activity of cotton was decreased significantly at 5−20 cm (p <0.05), and when the distance was 10 cm the CAT activity of S. planiculmis was highest, at 64.99 U min−1 g−1 (p <0.05; Figure 13F).
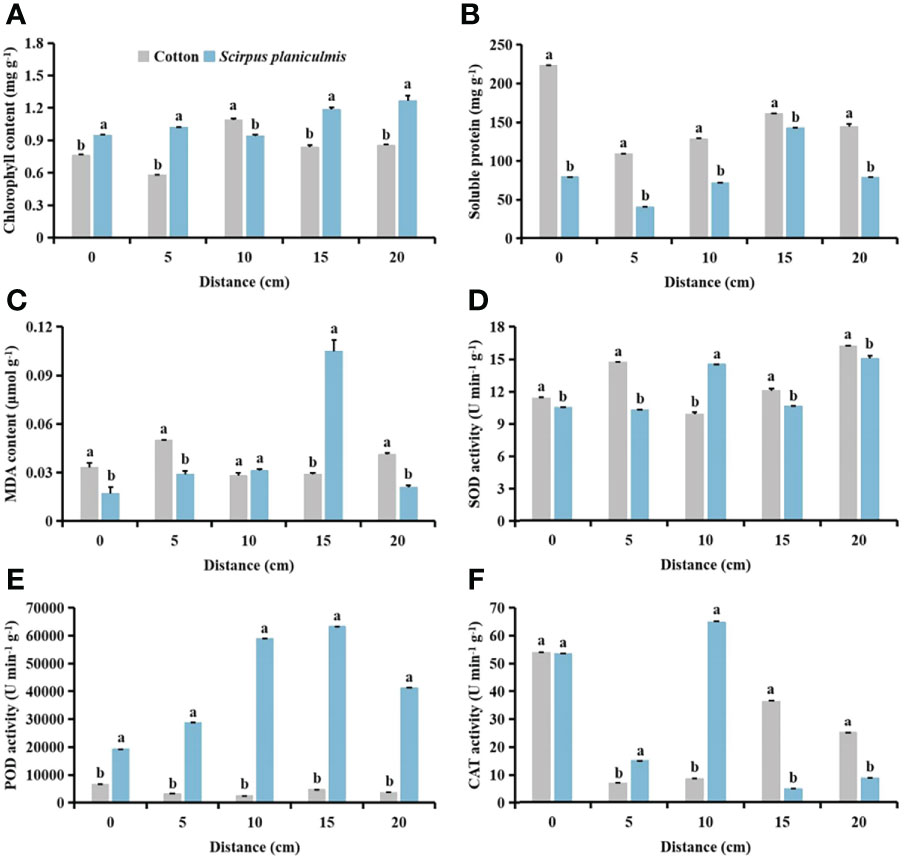
Figure 12 Effects of cotton and Scirpus planiculmis on (A) chlorophyll, (B) soluble protein and (C) malondialdehyde (MDA) content, and (D) superoxide dismutase (SOD), (E) peroxidase (POD) and (F) catalase (CAT) activities at different spatial distribution distances of S. planiculmis after spraying 0.015 g L-1 75% trifloxysulfuron + 4 g L-1 40% prometryn. The mean value ± standard data were presented in the figure. Different alphabetical letters indicate the significant differences between cotton and S. planiculmis (p <0.05).
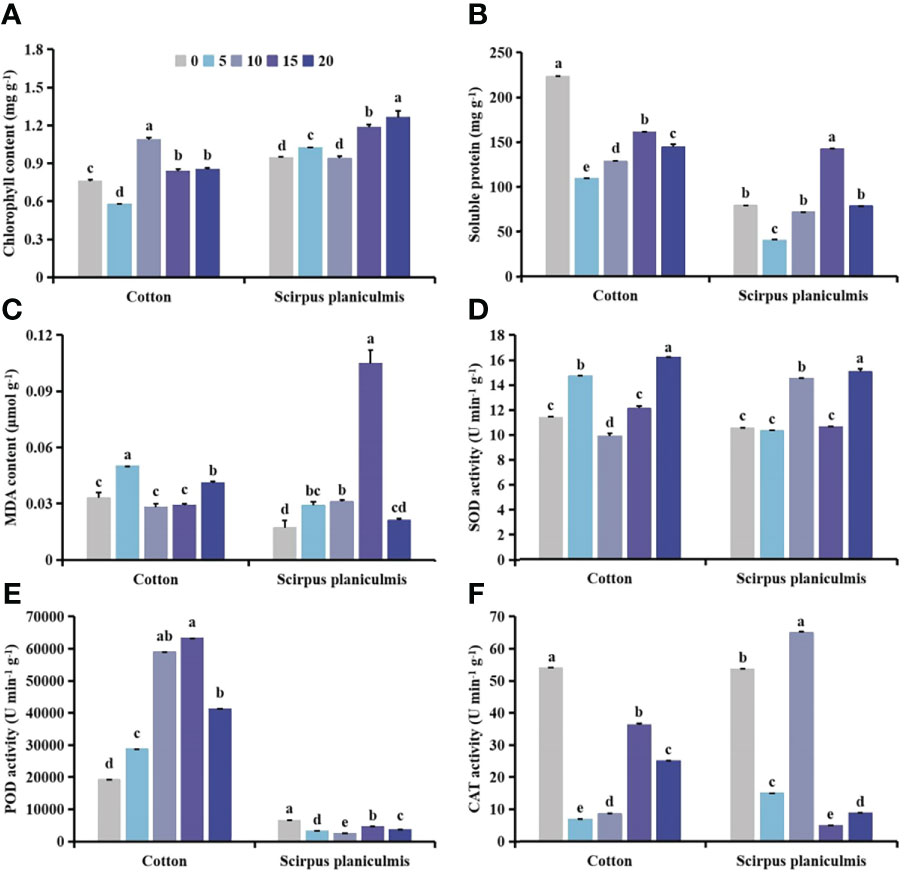
Figure 13 Effects of spraying 0.015 g L-1 75% trifloxysulfuron + 4 g L-1 40% prometryn on (A) chlorophyll, (B) soluble protein and (C) malondialdehyde (MDA) content, and (D) superoxide dismutase (SOD), (E) peroxidase (POD) and (F) catalase (CAT) activities in cotton and Scirpus planiculmis. The mean value ± standard data were presented in the figure. Different alphabetical letters indicate the significant differences between different distances (p <0.05).
Compared with no herbicide application, herbicide application decreased the chlorophyll content of cotton and S. planiculmis (Figures 14A and 15A), and increased MDA content, and SOD, POD and CAT activities of cotton and S. planiculmis (Figures 14C-F and 15C-F). At 0 and 15 cm, application of herbicides increased the soluble protein content of cotton by 58.74% and 17.87%, respectively (p <0.05; Figure 14B). However, application of herbicides increased the soluble protein content of S. planiculmis at all distances (p <0.05; Figure 15B).
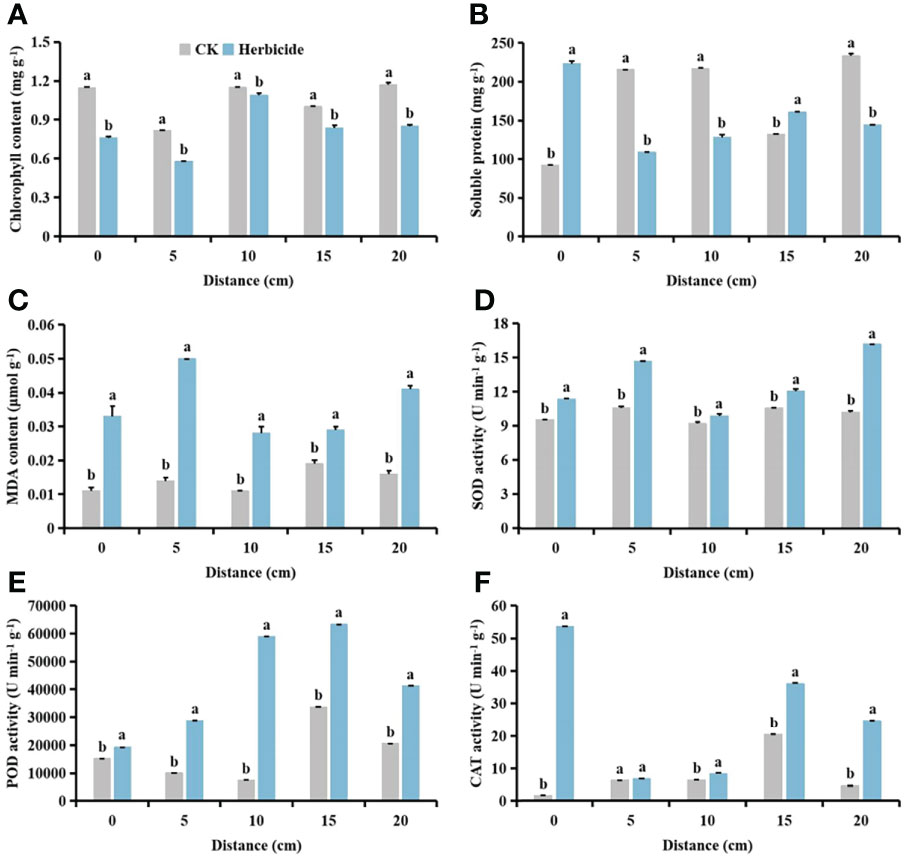
Figure 14 Effects of no herbicide application and spraying 0.015 g L-1 75% trifloxysulfuron + 4 g L-1, 40% prometrynon (A) chlorophyll, (B) soluble protein and (C) malondialdehyde (MDA) content, and (D) superoxide dismutase (SOD), (E) peroxidase (POD) and (F) catalase (CAT) activities in cotton at different spatial distribution distances. CK, no herbicide application: Herbicide, spraying 0.015 g L-1 75% trifloxysulfuron + 4 g L-1 40% prometryn. The mean value ± standard data were presented in the figure. Different alphabetical letters indicate the significant differences between CK and herbicide (p <0.05).
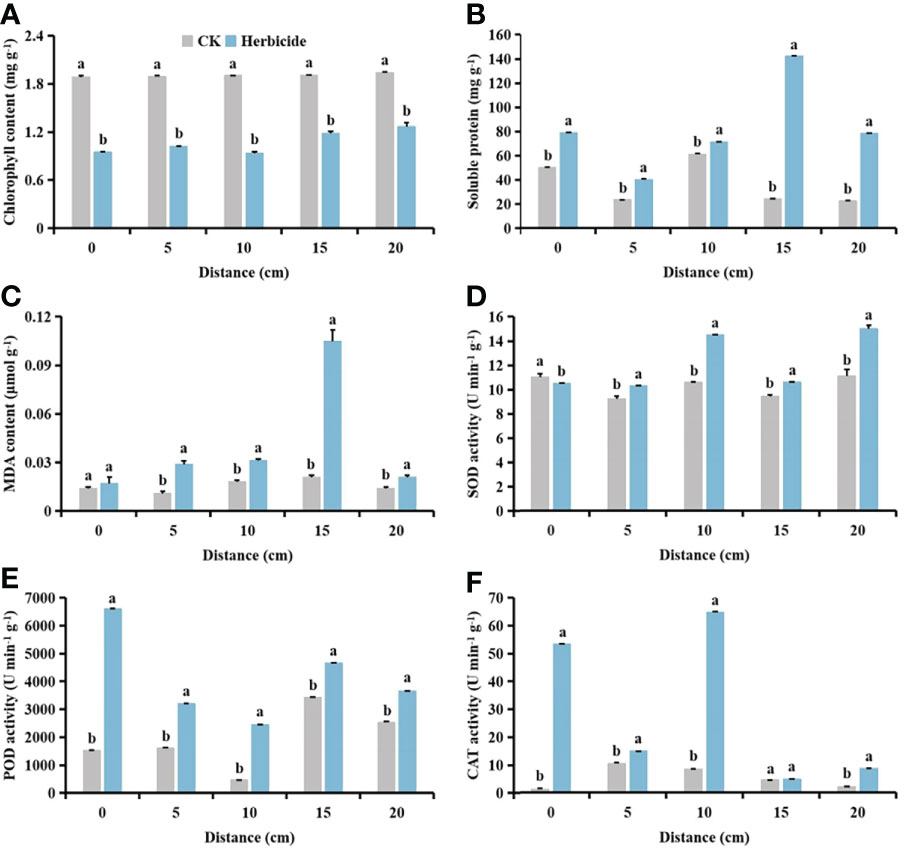
Figure 15 Effects of no herbicide application and spraying 0.015 g L-1 75% trifloxysulfuron + 4 g L-1 40% prometryn on (A) chlorophyll, (B) soluble protein and (C) malondialdehyde (MDA) content, and (D) superoxide dismutase (SOD), (E) peroxidase (POD) and (F) catalase (CAT) activities in Scirpus planiculmis at different spatial distribution distances. CK, no herbicide application; Herbicide, spraying 0.015 g L-1 75% trifloxysulfuron + 4 g L-1 40% prometryn. The mean value ± standard data were presented in the figure. Different alphabetical letters indicate the significant differences between CK and herbicide (p <0.05).
Discussion
The results of our study showed that with different stress patterns (density, competition period, and spatial distribution) of S. planiculmis, protective enzymes were involved in the response of cotton to S. planiculmis (Figures 2, 6 and 9). Compared with SOD and POD, cotton increase CAT activity under different stress patterns of S. planiculmis (Figure 2, 6 and 9). The protective enzyme activities of cotton and S. planiculmis were increased under low dose of herbicides (Figure 14, 15). Due to the lethal effect of herbicides on S. planiculmis, this will help cotton to further improve the protective enzyme activity to reduce the stress of S. planiculmis.
Cotton is an economically important crop that is very sensitive to weed competition (Wang et al., 2015). In the field, cotton growth and development are easily disrupted by weeds, resulting in dwarf plants, chlorosis, and even a decrease in the number of flowers and bolls, ultimately resulting in reduced cotton yield (Ma et al., 2016). Different weed species exert different interference effects on cotton. Herein, based on the literature, we classified weeds that compete with cotton into three categories according to plant type: low creeping weeds, comprising short plants have a poor shading effect on cotton, including goosegrass (Ma et al., 2015a); weeds with stout stems and lush branches and leaves that have a great influence on the photosynthetic utilization and morphological characterization of cotton, including Solanum nigrum (Zhang et al., 2022a), giant ragweed (Barnett and Steckel, 2013), redroot pigweed (Ma et al., 2015b) and velvetleaf (Ma et al., 2016); and intermediate weeds with a form between stout and tall plants and low creeping plants. Although members of this third class are taller, their stems are slender, and their shading effect on cotton is stronger than that of low creeping and weaker than that of stout and tall classes. S. planiculmis belongs to this third class (Cai et al., 2020a). Studies have shown that goosegrass in cotton fields does not significantly reduce the plant height of cotton, and at the maximum goosegrass density, the stem diameter and number of sympodial branches of cotton were only reduced by 6% compared with controls (Ma et al., 2015a), indicating that goosegrass had minimal effect on the morphological characteristics of cotton. However, in cotton fields containing S. nigrum (Zhang et al., 2022a), giant ragweed (Barnett and Steckel, 2013), redroot pigweed (Ma et al., 2015b) and velvetleaf (Ma et al., 2016), plant height and stem diameter of cotton were significantly affected, and plant height and stem diameter decreased regularly with increasing weed density. For example, for rows containing an extra velvetleaf plant, cotton plant height and stem diameter decreased by 5 cm and 2 mm, respectively (Ma et al., 2016). Similarly, plant height and stem diameter decreased by 2−13 cm and 2 mm, respectively, for each additional redroot pigweed per row (Ma et al., 2015b). This is due to the strong light interception effect of tall and stout weeds on cotton (Scott et al., 2000), which affects morphological characterization. Our results showed that S. planiculmis significantly reduced plant height and stem diameter of cotton only under certain density conditions (≥20 plants m-2) at budding, and flowering and boll stages (Figure 2). This indicates that under low density conditions (<20 plants m-2), S. planiculmis does not affect the upper morphological characteristics of cotton. In addition, we found that the chlorophyll content of cotton decreased with increasing density of S. planiculmis at the flowering and boll stage of cotton, but the chlorophyll content of cotton increased under high density of S. planiculmis (80−100 plants m-2) at the seedling stage and bud stage of cotton (Figure 2). This indicates that the effect of light interception of S. planiculmis in the cotton flowering and boll stage was stronger than that in the seedling stage and budding stage, while in the seedling stage and bud stage, a high density of S. planiculmis stimulated cotton photosynthesis and increased the chlorophyll content of the crop.
In general, weed damage can affect the entire growth and development cycle of cotton, and long-term persistent stress has an adverse effect on cotton morphogenesis (Charles and Taylor, 2021). Long-term competition by weeds for light and nutrient resources has reduces the ability of cotton to absorb and utilise nutrients, manifesting as slender, short plants (Wang et al., 2015). For example, following continuous damage to cotton by S. nigrum, levels of phosphorus, potassium, soluble protein and soluble sugar were reduced, and plant height and stem diameter of cotton were decreased with increasing stress duration (Han, 2021). Similarly, during competition between S. planiculmis and cotton, the content of potassium and soluble sugar in cotton decreased with prolongation of the competition period, which was not conducive to the absorption and accumulation of nutrients by cotton, resulting in decreased plant height and stem diameter of cotton (Cai, 2020c). In addition, after continuous competition of sunflower, Japanese millet and mungbean as mimic weeds with cotton, plant height and biomass of cotton were reduced to varying degrees, which had a negative effect on the vegetative growth of cotton (Charles et al., 2019a; Charles et al., 2019b; Charles et al., 2020). In the present study, we found that when S. planiculmis was planted in April, there was no significant change in plant height, stem diameter, fresh weight, root length or chlorophyll content of cotton with prolongation of the competition period between S. planiculmis and cotton (Figure 6). However, when S. planiculmis was planted in May and June, plant height, stem diameter, fresh weight, root length and chlorophyll content of cotton decreased with continuation of the competition period (Figure 6). This shows that S. planiculmis had little effect on the growth and photosynthesis of cotton in the early stages, but it had a great effect on the morphogenesis and photosynthesis of cotton in the middle and later stages. Additionally, we also found that when S. planiculmis and cotton competition period for 30 days, the soluble protein content in cotton was lowest, and after 60 days the soluble protein content in cotton gradually returned to normal levels (Figure 6). This may be due to long-term competition between cotton and S. planiculmis. Cotton enhances the absorption and accumulation of nitrate nitrogen, and converts nitrate nitrogen into soluble protein after assimilation in the body (Marschner, 2012; Cai, 2020c).
Most previous studies ignored the mediating effect of allelopathy on competition between cotton and weeds, but allelopathy of weeds is an objective inducing factor that affects the growth and development of cotton (Wang et al., 2022). For example, extracts of Medicago sativa, Flaveria bidentis, and Eclipta prostrata can inhibit the germination rate and radicle growth of cotton (Yuan et al., 2008; Peng et al., 2011; Yang et al., 2015). Our previous studies have shown that root exudates of S. planiculmis have no significant effect on the growth of above-ground parts of cotton, but can significantly inhibit the growth of underground parts, and high concentrations can reduce the content of cytokinin and auxin in cotton (Cai, 2020c). This indicates that allelopathy of S. planiculmis affected the growth and development of cotton by inhibiting the synthesis of cytokinin and auxin. It is worth noting that interference of root exudates of S. planiculmis on cotton is correlated with concentration, and therefore the strength of allelopathy (Li et al., 2020).
Interference of allelopathy on plants is related to the distance between plants (Li et al., 2022b). In this study, we analysed this effect by changing the distance between S. planiculmis and cotton. We found that plant height and stem diameter of cotton did not change significantly under different distance conditions, indicating that allelopathy of S. planiculmis did not affect the growth of aboveground parts of cotton, consistent with our indoor research results (Cai, 2020c). However, the difference is that fresh weight and root length of cotton did not change significantly with a difference in distribution distance (Figure 9), which may be due to the low density of flat stalks (eight plants) in this study. The allelopathic intensity of S. planiculmis was weak at this density, which could not significantly inhibit fresh weight and root length of cotton, while S. planiculmis at higher density (≥20 plants/m2) caused significant inhibition. This also indicates that allelochemicals of S. planiculmis can inhibit growth of the underground parts of cotton at high concentrations (Cai, 2020c).
Under allelochemical stress, receptor plants can alleviate damage caused by reactive oxygen species (ROS) by altering enzyme activity via a self-protective mechanism (Li et al., 2022a). Recent studies have shown that the allelopathic effect of Artemisia argyi water extract (AAWE) can lead to membrane system rupture and ROS release in rice, and under low AAWE concentration, an increase in SOD activity can compensate for a decrease in CAT and POD activities, which ensures that ROS levels remain relatively stable. However, with increasing AAWE concentration, POD and CAT activities continued to decrease, and MDA content increased, eventually leading to ROS imbalance (Li et al., 2022a). Our study showed that under different densities of S. planiculmis, SOD activity of cotton was decreased at each period, while CAT activity was increased, and POD activity was increased in the budding stage and the flowering and boll stage, whereas MDA content was decreased significantly in the flowering and boll stage (Figure 2). This shows that POD and CAT are protective enzymes that play a major role in cotton in response to the density effect of S. planiculmis. Only CAT activity showed an increasing trend with prolongation of competition period (Figure 6). This indicates that CAT in cotton plays a continuous role in resisting S. planiculmis under long-term competition. Regarding the distribution of S. planiculmis, only CAT activity was increased at different distance ranges (Figure 9). Therefore, we believe that an increase in CAT activity plays a key role in the responses of cotton to S. planiculmis stress.
We further explored the effects of herbicide application on physiological metabolism of S. planiculmis and cotton, and found that protective enzyme activities of both species were improved after herbicide application (Figure 14 and 15). The herbicide used in this study was selective and had a good control effect on S. planiculmis (Peng et al., 2012; Tian and Yu, 2020). Although the protective enzyme activity of S. planiculmis increased after application of low doses of herbicide, this is a normal response of weeds to herbicides (Peng et al., 2013a; Peng et al., 2013b). However, herbicides do not kill cotton; rather, they only stimulate protective enzyme activities (Peng et al., 2013a; Peng et al., 2013b). This indicates that during interaction between cotton and S. planiculmis, herbicides may gradually weaken the stress advantage of S. planiculmis, enhance the self-protection ability of cotton, and improve the resistance of cotton to S. planiculmis. Therefore, we believe that when controlling S. planiculmis, application of low-dose selective herbicides may help cotton to resist stress caused by weeds. Our previous studies showed that application of low-dose insecticides, fungicides and plant growth regulators can increase protective enzyme activities of cotton, and help resist pest-associated stress (Zhang et al., 2022c; Zhang et al., 2022d; Zhang et al., 2022e). Clearly, a low-dose pesticide can stimulate the defence response of cotton. On the one hand, pesticides can directly act on pests, and on the other hand, they can enhance the defence ability of cotton against pests, and resistance to pests is promoted through this dual role. This knowledge helps to explain pest resistance, and supports the sustainable development of agricultural production management.
It cannot be ignored that intraspecific competition of weeds is always accompanied by interactions between weeds and cotton (Wang et al., 2015). In previous reports, it was confirmed that during competition between cotton and giant ragweed (Barnett and Steckel, 2013), goosegrass (Ma et al., 2015a), redroot pigweed (Ma et al., 2015b) or velvetleaf (Ma et al., 2016), intraspecific competition of weeds led to a decrease in the biomass of individual plants. Specifically, the stem diameter of redroot pigweed and velvetleaf decreased with increasing density, while plant height increased (Ma et al., 2015b; Ma et al., 2016). We found that with increasing population density, plant height of S. planiculmis tended to increase, while stem diameter showed a downward trend, and fresh weight and root length first increased then decreased (Figure 1). Moreover, S. planiculmis responds to intraspecific competition by regulating photosynthesis, soluble protein synthesis, and protective enzyme activities, via a self-regulatory strategy characteristic of weed populations (Li et al., 2022b).
Weeds ultimately impair cotton yield by altering cotton plant morphology and nutrient metabolism (Wang et al., 2015). In this study, with increasing S. planiculmis density, cotton boll number, single boll weight and yield were diminished (Figure 4). In addition, continuous damage by S. planiculmis also led to a decrease in boll number, single boll weight and yield (Figure 8). However, with increasing distance between S. planiculmis and cotton, boll number, single boll weight and yield were less affected (Figure 11). Using equation fitting, we previously determined that the control threshold of S. planiculmis in cotton fields was 20 plants m-2, and the duration of damage should be less than 30 days (Cai et al., 2020a, 2021). Therefore, we suggest that S. planiculmis should be controlled in the field to avoid significant loss of cotton yield.
Moreover, temperature and rainfall are considered to be important climatic factors affecting plant growth (Khan et al., 2022; Kharivha et al., 2022). In order to adapt to abiotic stresses such as drought, high temperature and low temperature, plants also reduce the impact of climatic conditions on themselves by regulating defense responses (Akpinar and Cansev, 2022; Vital et al., 2022). Obviously, when studying the stress of weeds on cotton in the field, extreme climate may have an impact on cotton response to weed stress. Therefore, climate factors need to be included in this consideration. We documented changes in temperature and precipitation during the cotton growing stage (seedling to boll opening) from 2019 to 2020 (Figure S1). These climatic data reflect the characteristics of large temperature difference between day and night and low rainfall in the test area, which belongs to the typical temperate continental arid climate. Temperature difference led to the acceleration of plant growth and the occurrence of phenological events (Talukder et al., 2014; Lymperopoulos et al., 2018). To adapt to the environment with large temperature difference, cotton improves the adaptability to temperature difference by regulating photosynthesis, respiration and physiological metabolism (Wang et al., 2016, 2018). In order to reduce the effect of temperature difference on cotton, we chose plastic film mulching to maintain soil temperature. Especially in low temperature conditions, the film has played a role in raising soil temperature, which can reduce the temperature difference on cotton. Studies have shown that with the strengthening of drought stress, cotton plant height, stem diameter and other morphological indicators decreased, and by regulating photosynthesis and protective enzyme activity in a sustained manner to respond strongly to drought (Tian et al., 2017). In this study, we made up for the lack of rainfall and avoided the occurrence of drought stress by drip irrigation. In addition, the film played a good role in moisture conservation. Therefore, we think that the effects of climatic factors on cotton growth and physiology can be reduced by applying reasonable cultivation and management measures.
In summary, using S. planiculmis and cotton as an example, we analysed the stress effect of S. planiculmis on cotton under different densities, competition periods and distribution conditions, from the perspective of morphogenesis, physiological metabolism and yield, and revealed the defence pathway of cotton in response to S. planiculmis that involves regulation of protective enzyme activities. We concluded that CAT plays a key role in protecting enzymes involved in defence responses. On this basis, we further explored the effect of low-dose herbicide on the interaction between cotton and S. planiculmis. The results showed that low-dose herbicide could help cotton resist stress caused by S. planiculmis by elevating the activities of protective enzymes in cotton. Therefore, we believed that when attempting to control S. planiculmis, low doses of herbicide may protect cotton and control weeds. This strategy is also applicable to the control of other pests. In the future, we will further explore the response strategy of cotton to S. planiculmis from the perspective of gene regulation, and thereby obtain a deeper explanation for the stress mechanism of cotton in response to S. planiculmis.
Data availability statement
The raw data of the presented results of this study are available on request to the corresponding author.
Author contributions
QZ wrote the manuscript; JW designed the study; JP conducted experiments; QZ and JP analyzed data. All authors contributed to the article and approved the submitted version.
Funding
This research was supported by the National Natural Science Foundation of China (Grant No. 31260435, 31660519), and the Xinjiang Corps Major Science and Technology Project (Grant No. 2018AA06-02).
Acknowledgments
We thank reviewers and editors for valuable comments and suggestions on the manuscript.
Conflict of interest
The authors declare that the research was conducted in the absence of any commercial or financial relationships that could be construed as a potential conflict of interest.
Publisher’s note
All claims expressed in this article are solely those of the authors and do not necessarily represent those of their affiliated organizations, or those of the publisher, the editors and the reviewers. Any product that may be evaluated in this article, or claim that may be made by its manufacturer, is not guaranteed or endorsed by the publisher.
Supplementary material
The Supplementary Material for this article can be found online at: https://www.frontiersin.org/articles/10.3389/fpls.2022.1068419/full#supplementary-material
References
Akpinar, A., Cansev, A. (2022). Physiological and molecular responses of roots differ from those of leaves in spinach plants subjected to short-term drought stress. S. Afr. J. Bot. 151, 9–17. doi: 10.1016/j.sajb.2022.09.032
Amalo, K., Chen, G. X., Asade, K. (1994). Separate assays specific for ascorbate peroxidase and guaiacol peroxidase and for the chloro-plastic and cytosolic isozymes of ascorbate peroxidase implants. Plant Cell Physiol. 35, 497–504. doi: 10.1093/oxfordjournals.pcp.a078621
An, Y., Gao, Y., Tong, S. Z., Liu, B. (2021). Morphological and physiological traits related to the response and adaption of Bolboschoenus planiculmis seedlings grown under salt-alkaline stress conditions. Front. Plant Sci. 12, 567782. doi: 10.3389/fpls.2021.567782
Arnon, D. I. (1949). Copper enzymes in isolated chloroplasts. polyphenoloxidase in Beta vulgaris. Plant Physiol. 24, 1–15. doi: 10.1104/pp.24.1.1
Barnett, K. A., Steckel, L. E. (2013). Giant ragweed (Ambrosia trifida) competition in cotton. Weed Sci. 61, 543–548. doi: 10.1614/WS-D-12-00169.1
Bradford, M. M. (1976). A rapid and sensitive method for the quantitation of microgram quantities of protein utilizing the principle of protein–dye binding. Anal. Biochem. 72, 248–254. doi: 10.1016/0003-2697(76)90527-3
Cai, X. H. (2020c). Study on stress of bolboschoenus planiculmis on the growth and development of mechanical harvest cotton (China: Shihezi Unversity). doi: 10.27332/d.cnki.gshzu.2020.000677
Cai, X. H., Lin, P., Shi, Y. H., Han, R., Zhang, Y. D., Wang, J. G. (2021). Effects of population density of Bolboschoenus planiculmis on the morphological index, yield and quality of cotton. Plant Prot. 47, 90–95. doi: 10.16688/j.zwbh.2020179
Cai, X. H., Lin, P., Shi, Y. H., Han, R., Zhang, Y. D., Wu, N., et al. (2020a). Effects of population density of Bolboschoenus planiculmis on the absorption and accumulation of cotton nutrients. Cotton Sci. 32, 143–150. doi: 10.11963/1002-7807.cxhwjg.20200310
Cai, X. H., Lin, P., Shi, Y. H., Han, R., Zhang, Y. D., Wu, N., et al. (2020b). Effects of continuous damage of Bolboschoenus planiculmis (F. Schmidt) t. v. egorova on agronomic and economic traits of cotton. Xinjiang Agric. Sci. 57, 1151–1158. doi: 10.6048/j.issn.1001-4330.2020.06.020
Castano-Duque, L., Helms, A., Ali, J. G., Luthe, D. S. (2018). Plant bio-wars: maize protein networks reveal tissue-specific defense strategies in response to a root herbivore. J. Chem. Ecol. 44, 727–745. doi: 10.1007/s10886-018-0972-y
Chance, B., Maehly, A. C. (1955). Assay of catalase and peroxidase. Method. Enzymol. 2, 764–775. doi: 10.1016/S0076-6879(55)02300-8
Charles, G. W., Sindel, B. M., Cowie, A. L., Knox, O. G. G. (2019a). Determining the critical period for weed control in high yielding cotton using common sunflower as a mimic weed. Weed Technol. 33, 800–807. doi: 10.1017/wet.2019.68
Charles, G. W., Sindel, B. M., Cowie, A. L., Knox, O. G. G. (2019b). Determining the critical period for grass control in high yielding cotton using Japanese millet as a mimic weed. Weed Technol. 34, 292–300. doi: 10.1017/wet.2019.113
Charles, G. W., Sindel, B. M., Cowie, A. L., Knox, O. G. G. (2020). Determining the critical period for broadleaf weed control in high-yielding cotton using mungbean as a mimic weed. Weed Technol. 34, 689–698. doi: 10.1017/wet.2020.38
Charles, G. W., Taylor, I. N. (2021). Extending the critical period for weed control model to better include weed succession using common sunflower as a mimic weed in high-yielding cotton. Weed Technol. 35, 1029–1037. doi: 10.1017/wet.2021.91
Chia, M. A., Bittencourt-Oliveira, M. do. C. (2021). Allelopathic interactions between phytoplankton species alter toxin production, oxidative response, and nitrogen fixation. Hydrobiologia 848, 4623–4635. doi: 10.1007/s10750-021-04665-z
Choi, S. H., Shon, Y. G., Ju, G. S., Choi, J. H., Kim, M. H., Yu, Y. M., et al. (2000a). Sprouting and growth characteristics of sea club rush (Scirpus planiculmis). Weed Turfgrass Sci. 20, 276–283.
Choi, S. H., Shon, Y. G., Lee, J. J. (2000b). Effects of annual weed herbicides and sulfonylurea herbicides on sea club rush (Scirpus planiculmis). Weed Turfgrass Sci. 20, 297–302.
Dhindsa, R. S., Dhindsa, P. P., Thorpe, T. A. (1981). Leaf senescence: correlated with increased leaves of membrane permeability and lipidperoxidation, and decreased levels of superoxide dismutase and catalase. J. Exp. Bot. 32, 93–101. doi: 10.1093/jxb/32.1.93
Fang, F., Li, M., Gao, X. X., Li, J., Zhang, C. X. (2016). Species composition and characterization of weed communities in cotton fields in yellow river delta. Plant Prot. 42, 148–153. doi: 10.3969/j.issn.0529-1542.2016.05.026
Gao, H. L., Zou, J. Z., Lin, X. M., Zhang, H. H., Yu, N., Liu, Z. W. (2022). Nilaparvata lugens salivary protein NlG14 triggered defense response in plants. J. Exp. Bot. doi: 10.1093/jxb/erac354
Giannopolities, C. N., Ries, S. K. (1977). Superoxide dismutase: I. occurrence in higher plants. Plant Physiol. 595, 309–314. doi: 10.1104/pp.59.2.309
Han, R. (2021). The study on competition and control threshold for solanum nigrum l. and mechanical harvest cotton (China: Shihezi Unversity).
Hroudová, Z., Zákravský, P., Flegrová, M. (2007). The effect of burial depth on the tuber viability of Bolboshoenus laticarpus and B. planiculmis under terrestrial conditions. Belg. J. Bot. 140, 121–129. doi: 10.2307/20794629
Hroudová, Z., Zákravský, P., Flegrová, M. (2014). The tolerance to salinity and nutrient supply in four European Bolboschoenus species (B. maritimus, B. laticarpus, B. planiculmis and B. yagara) affects their vulnerability or expansiveness. Aquat. Bot. 112, 66–75. doi: 10.1016/j.aquabot.2013.07.012
Huang, H. J., Zhang, C. X., Jiang, C. L., Zhang, X. K., Huang, Z. F., Wu, W. L., et al. (2020). Diversity and community composition of weeds in cotton fields of northern xinjiang. J. Weed Sci. 38, 7–13. doi: 10.19588/j.issn.1003-935X.2020.01.002
Hwang, K. H., Kim, D. S., Lee, J. N., Koo, S. J. (2006). Herbicidal efficacy of flucetosulfuron + pyrazosulfuron - ethyl in controlling perennial sedges and sulfonylurea resistant weeds. Korean J. Pestic. Sci. 10, 320–328. doi: 10.19588/j.issn.1003-935X.2020.01.002
Kato-Noguchi, H., Kimura, F., Ohno, O., Suenaga, K. (2017). Lnvolvement of allelopathy in inhibition of understory growth in red pine forests. J. Plant Physiol. 218, 66–73. doi: 10.1016/j.jplph.2017.07.006
Khan, A. H., Min, L., Ma, Y. Z., Zeeshan, M., Jin, S. X., Zhang, X. L. (2022). High-temperature stress in crops: Male sterility, yield loss, and potential remedy approaches. Plant Biotechnol. J 1–18. doi: 10.1111/PBI.13946
Kharivha, T., Ruwanza, S., Thondhlana, G. (2022). Effects of elevated temperature and high and low rainfall on the germination and growth of the invasive alien plant Acacia mearnsii. Plants 11, 2633. doi: 10.3390/plants11192633
Kwon, O. D., An, K. N., Lee, Y., Shin, S. H., Park, H. G., Shin, H. R., et al. (2011). Rice yield loss and economic threshold levels by densities of Scirpus planiculmis and Lindernia dubia in wet-seeded rice paddy fields. Korean J. Weed Sci. 31, 348–354. doi: 10.5660/KJWS.2011.31.4.348
Kwon, O. D., Kuk, Y. I., Moon, B. C. (2008). Economic threshold levels based on rice yield and rice quality as affected by densities of Scirpus planiculmis in transplanting rice cultivation. Weed Turfgrass Sci. 28, 255–263.
Lee, K. S., Han, K. H., Park, S. H., Choi, S. Y. (1991). Growth habits of Scirpus planiculmis f. Schmidt in poldered paddy field. Weed Turfgrass Sci. 11, 111–116.
Li, Y., Li, C., Li, B. R., Ma, Z. H. (2021). Trifluralin residues in soils from main cotton fields of China and associated ecological risk. Chemosphere 284, 131300. doi: 10.1016/j.chemosphere.2021.131300
Li, J. Y., Lin, S. X., Ma, H. Y., Wang, Y. P., He, H. B., Fang, C. X. (2022b). Spatial-temporal distribution of allelopathic rice roots in paddy soil and its impact on weed-suppressive activity at the seedling stages. Front. Plant Sci. 13, 940218. doi: 10.3389/fpls.2022.940218
Li, H. H., Ma, X. Y., Jiang, W. L., Ma, Y. J., Song, X. P., Ma, Y. (2019). Current status and countermeasure of chemical weeding in cotton fields. China Cott. 46, 1–7. doi: 10.11963/1000-632X.lhhmy.20190430
Ling, S. Q., Qiu, H. L., Xu, J. Z., Gu, Y. P., Yu, J. X., Wang, W., et al. (2022). Volatile dimethyl disulfide from guava plants regulate developmental performance of Asian citrus psyllid through activation of defense responses in neighboring orange plants. Int. J. Mol. Sci. 23, 10271. doi: 10.3390/ijms231810271
Liu, B., Jiang, M., Tong, S. Z., Zhang, W. G., Zou, C. L., Wang, B., et al. (2016). Effects of burial depth and water depth on seedling emergence and early growth of Scirpus planiculmis fr. Schmidt. Ecol. Eng. 87, 30–33. doi: 10.1016/j.ecoleng.2015.11.026
Li, T., Yang, D. S. (2020). Advances of the damage and integrated control of Bolboschoenus planiculmis in cotton field in China. China Cott. 47, 1–4. doi: 10.11963/1000-632X.ltyds.20201010
Li, J. X., Zhao, T. T., Chen, L., Chen, H., Luo, D. D., Chen, C. J., et al. (2022a). Artemisia argyi allelopathy: a generalist compromises hormone balance, element absorption, and photosynthesis of receptor plants. BMC Plant Biol. 22, 368. doi: 10.1186/s12870-022-03757-9
Li, A. X., Zheng, R. L., Tian, L., Wei, Y., Wu, , J. Y., Hou, , X. C. (2020). Allelopathic effects of switchgrass on redroot pigweed and crabgrass growth. Plant Ecol. 222, 1–12. doi: 10.1007/s11258-020-01083-4
Lymperopoulos, P., Msanne, J., Rabara, R. (2018). Phytochrome and phytohormones: working in tandem for plant growth and development review. Front. Plant Sci. 9, 1037. doi: 10.3389/fpls.2018.01037
Mardani, H., Maninang, J., Appiah, K. S., Oikawa, Y., Azizi, M., Fujii, Y. (2019). Evaluation of biological response of lettuce (Lactuca sativa l.) and weeds to safranal allelochemical of saffron (Crocus sativus) by using static exposure method. Molecules 24, 1788.
Marschner, P (2012). Marschner's mineral nutrition of higher plants 3rd ed. (San Diego, CA, USA: Elsevier, Academic press) 1–651. doi: 10.3390/molecules24091788
Ma, X. Y., Wu, H. W., Jiang, W. L., Ma, Y. J., Ma, Y. (2015a). Goosegrass (Eleusine indica) density effects on cotton (Gossypium hirsutum). J. Integr. Agr. 14, 1778–1785. doi: 10.1016/S2095-3119(15)61058-9
Ma, X. Y., Wu, H. W., Jiang, W. L., Ma, Y. J., Ma, Y. (2015b). Interference between redroot pigweed (Amaranthus retroflexus l.) and cotton (Gossypium hirsutum l.): growth analysis. PloS One 10, e0130475. doi: 10.1371/journal.pone.0130475
Ma, X. Y., Yang, J. Y., Wu, H. W., Jiang, W. L., Ma, Y. J., Ma, Y. (2016). Growth analysis of cotton in competition with velvetleaf (Abutilon theophrasti). Weed Technol. 30, 123–136. doi: 10.1614/WT-D-15-00050.1
National Bureau of Statistics (2021) Announcement of national bureau of statistics on cotton yield in 2021. Available at: http://wap.stats.gov.cn/fb/202112/t20211214_1825241.html (Accessed 7 June 2022).
Park, M. S., Park, Y. S., Kim, S. M., Lee, J. D. (2011). Herbicidal activity of new rice herbicide pyrimisulfan + mefenacet SC against sulfonlurea-resistant sea club rush (Scirpus planiculmis). Weed Turfgrass Sci. 31, 192–198. doi: 10.5660/KJWS.2011.31.2.192
Peng, J., Ma, Y., Li, X. J., Ma, X. Y., Xi, J. P., Ma, Y. J., et al. (2011). Research progress in allelopathy of Flaveria bidentis (L.) kuntze. Weed Sci. 29, 17–22. doi: 10.19588/j.issn.1003-935x.2011.01.004
Peng, J., Song, Z. L., Deng, X. X., Jiang, H. L., He, Z. M., Ma, T. W., et al. (2012). Chemical control of cotton field Scirpus planiculmis fr. Schmidt based on orthogonal design. J. Shihezi Univ. 30, 147–151. doi: 10.13880/j.cnki.65-1174/n.2012.02.004
Peng, J., Song, Z. L., Meng, X. G., Wang, J., Deng, X. X., Jiang, H. L., et al. (2013a). The effect study of herbicide mixed with one of plant growth regulators on physiology and biochemistry of the Scirpus planiculmis fr. Schmidt. Agrochemicals 52, 519–524. doi: 10.16820/j.cnki.1006-0413.2013.07.015
Peng, J., Song, Z. L., Meng, X. G., Wang, X. D., Lv, D. B., Wang, J., et al. (2013b). Effects of herbicides on physiology and biochemistry of the Scirpus planiculmis fr. Schmidt. Acta Agric. Bor.-occid. Sin. 22, 192–197. doi: 10.7606/j.issn.1004-1389.2013.09.033
Qin, F. C., Liu, S., Yu, S. X. (2018). Effects of allelopathy end competition for water end nutrients on survival end growth of tree species in Eucalyptus urophylla plantations. For. Ecol. Manage. 424, 387–395. doi: 10.1016/j.foreco.2018.05.017
Scott, G. H., Askew, S. D., Wilcut, J. W., Brownie, C. (2000). Datura stramonium interference and seed rain in Gossypium hirsutum. Weed Sci. 48, 613–617. doi: 10.1614/0043-1745(2000)048[0613:DSIASR]2.0.CO;2
Siyar, S., Majeed, A., Muhammad, Z., Alic, H., Inayatc, N. (2019). Allelopathic effect of aqueous extracts of three weed species on the growth end leaf chlorophyll content of bred wheat. Acta Ecol. Sin. 39, 63–68. doi: 10.1016/j.chnaes.2018.05.007
Šoln, K., Klemenčič, M., Koce, J. D. (2022). Plant cell responses to allelopathy: from oxidative stress to programmed cell death. Protoplasma 259, 1111–1124. doi: 10.1007/s00709-021-01729-8
Steinbrenner, A. D., Saldivar, E., Hodges, N., Guayazán-Palacios, N., Chaparro, A. F., Schmelz, E. A. (2022). Signatures of plant defense response specificity mediated by herbivore-associated molecular patterns in legumes. Plant J. 110, 1255–1270. doi: 10.1111/tpj.15732
Swanton, C. J., Nkoa, R., Blackshaw, R. E. (2015). Experimental methods for crop-weed competition studies. Weed Sci. 63, 2–11. doi: 10.1614/WS-D-13-00062.1
Swinnen, G., De Meyer, M., Pollier, J., MolinaHidalgo, F. J., Ceulemans, E., VenegasMolina, J., et al. (2022). The basic helix-loop-helix transcription factors MYC1 and MYC2 have a dual role in the regulation of constitutive and stress-inducible specialized metabolism in tomato. New Phytol. 236, 911–928. doi: 10.1111/nph.18379
Talukder, A. S. M. H. M., McDonald, G. K., Gill, G. S. (2014). Effect of short-term heat stress prior to flowering and early grain set on the grain yield of wheat. Field Crops Res. 160, 54–63. doi: 10.1016/j.fcr.2014.01.013
Tian, Y. S., Fan, S. L., Pang, C. Y., Sun, G. Q., Zhang, G. L., Ye, C. X., et al. (2017). Effects of drought stress in the whole period on cotton morphology, physiology, photosynthesis and yield. Acta Agric. Bor.-Sin. 32, 224–231. doi: 10.7668/hbnxb.2017.05.033
Tian, Y., Yu, L. (2020). The control effect of three herbicides against Bolboschoenus planiculmis in xinjiang cotton field. China Cott. 47, 25–27. doi: 10.11963/1000-632X.tyty.20200807
Uesugi, A., Johnson, R., Kessler, A. (2019). Context-dependent induction of allelopathy in plants under competition. Oikos 128, 1492–1502. doi: 10.1111/oik.06389
Vital, R. G., Müller, C., Freire, F. B. S., Silva, F. B., Batista, P. F., Fuentes, D., et al. (2022). Metabolic, physiological and anatomical responses of soybean plants under water deficit and high temperature condition. Sci. Rep. 12, 16467. doi: 10.1038/s41598-022-21035-4
Vulchi, R., Bagavathiannan, M., Nolte, S. A. (2022). History of herbicide-resistant traits in cotton in the U.S. and the importance of integrated weed management for technology stewardship. Plants 11, 1189. doi: 10.3390/plants11091189
Wang, Q. S., Liu, N., Yang, X. Y., Tu, L. L., Zhang, X. L. (2016). Small RNA-mediated responses to low- and high-temperature stresses in cotton. Sci. Rep. 6, 35558. doi: 10.1038/srep35558
Wang, L. Q., Chen, J. H., He, N. Y., Guo, F. Q. (2018). Metabolic reprogramming in chloroplasts under heat stress in plants. Int. J. Mol. Sci. 19, 849. doi: 10.3390/ijms19030849
Wang, C., Liu, Z., Wang, Z. C., Pang, W. H., Long, Z., Wen, Z. Z., et al. (2022). Effects of autotoxicity and allelopathy on seed germination and seedling growth in Medicago truncatula. Front. Plant Sci. 13, 908426. doi: 10.3389/fpls.2022.908426
Wang, X. L., Ma, X. Y., Jiang, W. L., Ren, X. L., Ma, Y. J., Ma, Y. (2015). Review on competition between weeds and cotton. Cotton Sci. 27, 474–480. doi: 10.11963/issn.1002-7807.201505012
Yang, H., Kim, J. H., Lee, E. J. (2021). Seasonal flooding regime effects on the survival, growth, and reproduction of Bolboschoenus planiculmis under East Asian monsoon. Flora 285, 151960. doi: 10.1016/j.flora.2021.151960
Yang, J. Y., Ma, X. Y., Jiang, W. L., Ma, Y. J., Ma, Y. (2015). Allelopathic effect of aqueous extracts of Eclipta prostrata l.on cotton and common weeds in cotton field. Cotton Sci. 27, 53–61. doi: 1002-7807(2015)01-0053-09
Yuan, L., Yu, L., Wang, X. J., Lu, W. H. (2008). Study on the allelopathy effect and allelochemicals content of extracts from different ages of alfalfa on cotton. Pratacultural Sci. 25, 71–74. doi: 1001-0629(2008)12-0071-04
Zhang, Q. C., Deng, X. X., Wang, J. G. (2022c). The effects of mepiquat chloride (DPC) on the soluble protein content and the activities of protective enzymes in cotton in response to aphid feeding and on the activities of detoxifying enzymes in aphids. BMC Plant Biol. 22, 213. doi: 10.1186/s12870-022-03597-7
Zhang, Q. C., Shao, J. R., Han, R., Wang, J. G. (2022a). Effects of Solanum nigrum l. density on agronomic and yield traits, physiological indexes, and the economic threshold of mechanically-harvested cotton. Gesunde Pflanz 74, 879–888. doi: 10.1007/s10343-022-00665-8
Zhang, Q. C., Wang, J., Wang, J. G. (2022d). Use of plant growth regulators to reduce 2-methyl-4-chlorophenoxy acetic acid-Na (MPCA-Na) damage in cotton (Gossypium hirsutum). BMC Plant Biol 22, 533. doi: 10.21203/rs.3.rs-1777587/v1
Zhang, M. Y., Zhang, D. J., Qi, Q., Tong, S. Z., Wang, X. H., An, Y., et al. (2022b). Flooding effects on population and growth characteristics of bolboschoenus planiculmis in momoge wetland, northeast China. Ecol. Indic. 137, 108730. doi: 10.1016/j.ecolind.2022.108730
Zhang, Q. C., Zhou, M. H., Wang, J. G. (2022e). Increasing the activities of protective enzymes is an important strategy to improve resistance in cucumber to powdery mildew disease and melon aphid under different infection/infestation patterns. Front. Plant Sci. 13, 950538. doi: 10.3389/fpls.2022.950538
Keywords: chlorophyll, soluble protein, MDA, SOD, POD, CAT, defense response
Citation: Zhang Q, Peng J and Wang J (2022) Protective enzyme activity regulation in cotton (Gossypium hirsutum L.) in response to Scirpus planiculmis stress. Front. Plant Sci. 13:1068419. doi: 10.3389/fpls.2022.1068419
Received: 12 October 2022; Accepted: 08 November 2022;
Published: 28 November 2022.
Edited by:
Mariam Gaid, Independent researcher, Braunschweig, GermanyReviewed by:
Shashank Sagar Saini, Volcani Center, IsraelAgnieszka Synowiec, University of Agriculture in Krakow, Poland
Copyright © 2022 Zhang, Peng and Wang. This is an open-access article distributed under the terms of the Creative Commons Attribution License (CC BY). The use, distribution or reproduction in other forums is permitted, provided the original author(s) and the copyright owner(s) are credited and that the original publication in this journal is cited, in accordance with accepted academic practice. No use, distribution or reproduction is permitted which does not comply with these terms.
*Correspondence: Jungang Wang, d2FuZ2p1bmdhbmc5OEAxNjMuY29t
†These authors share first authorship