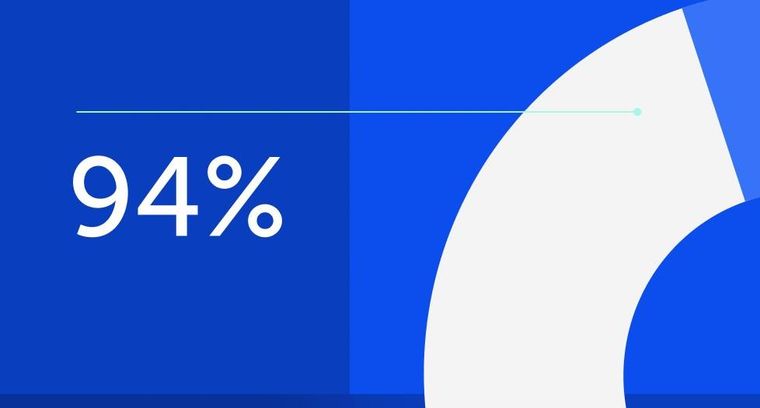
94% of researchers rate our articles as excellent or good
Learn more about the work of our research integrity team to safeguard the quality of each article we publish.
Find out more
MINI REVIEW article
Front. Plant Sci., 21 December 2022
Sec. Plant Systems and Synthetic Biology
Volume 13 - 2022 | https://doi.org/10.3389/fpls.2022.1064058
This article is part of the Research TopicSystem Biology to Regulatory Grids: New Tools and Clues Aimed at Improving Plant Evolutionary-Developmental (Evo-Devo) BiologyView all 6 articles
Root exudates play a key role in mediating plant–plant and plant–rhizomicrobiome interactions, including regulating biochemical/physiological aspects of plant-associated microorganisms, to enhance host plant growth and resilience. Root exudates can act as signals to reduce the competition from neighboring plants and recruiting/choreographing a wide range of diverse rhizomicrobiome members to make the host plant a good fit with its immediate environment. Root exudate production is a dynamic and key process, but there is a limited understanding of the metabolites or metabolic pathways involved in the inter-organismal communications facilitated by them. Given the well-known symbiotic relationships between plants and associated rhizomicrobiome members, adding root exudates to microbial isolation media may allow some of the large segments of rhizomicrobiome members that are not currently culturable to be grown in vitro. This will provide new insights into how root signals orchestrate associated microbes, will benefit agricultural production in the face of challenges posed by climate change, and will help to sustainably provide food for a growing global human population.
● Root exudate is part of the plant holobiont; its production varies with the surrounding circumstances.
● As a key aspect of root exudates, signal exchange is responsible for inter-organismal interactions such as plant–microbe, plant–kin, and neighbors in the rhizosphere and within the ecosystem.
● The combination of the advanced omics techniques is a promising approach to deeply explore and exploit the dynamics and abundance of root exudates.
● Plants recruit beneficial microbes through exudates to facilitate positive relationships and enhance plant development and resilience; specific elements of root exudates may also be required to allow growth of many phytomicrobiome members.
Root exudates contain a wide range of organic compounds including organic acids, sugars, and many other metabolites, which are secreted by plants into the rhizosphere (Baetz and Martinoia, 2014; Preece and Peñuelas, 2020; Vives-Peris et al., 2020) via diffusion, ion channel pumping, and vesicle transport (Vickers, 2017; Demidchik, 2018; He et al., 2021). Its production is generally considered to result in one of the most complex ecosystems, which is affected by both aboveground processes and conditions, and the belowground surrounding environment (Korenblum et al., 2020). Light (intensity, wavelength, and photoperiod) is among the most important aboveground factors affecting root exudation, as it directly affects CO2 fixation through photosynthesis, which determines the amount of material available for root secretion into the rhizosphere (Vives-Peris et al., 2020). Ten percent to 50% of photosynthetically fixed carbon is translocated into the root and released into the spaces between cells and the root-associated soil (Korenblum et al., 2020). Root exudation patterns are also correlated with functional traits of specific plants (Herz et al., 2018). More leaf area or taller canopies are closely linked to plant relative growth rate including the amount of root produced, which thus could contribute to the secretion of larger amounts of root exudates (Herz et al., 2018).
The belowground response to the root exudate composition mainly depends on soil conditions including nutrient availability and the rhizomicrobiome in rhizosphere (Korenblum et al., 2020; Gao et al., 2021; Jiang et al., 2022; Wen et al., 2022). Plants can shift exudation levels of specific metabolites to address phosphate deficiency stress for both domesticated maize (Zea mays subsp. mays) and wild species (Z. mays subsp. parviglumis) (Brisson et al., 2022). A similar response also happened for other plant species, such as maize (Zhengdan 958) under the nitrogen supply (Hao et al., 2022), pearl millet genotypes (sensitive (843-22B) under drought stress (Ghatak et al., 2022), and species from Curcurbitaceae family under salinity (Li et al., 2021). In one important aspect, all the above studies also noticed the alteration of rhizosphere microbial communities. As previously reported, maize cultivar Zman2 released less dolabralexins, which led to more diverse root microbiome, compared with its wild-type (WT) sibling, a cultivar with highly abundant dolabralexins in roots (Murphy et al., 2021). The number of bacterial genera significantly correlated with the level of the root-released organic carbon released by wheat (Triticum aestivum L.) (Chen et al., 2019). A subset of bacterial and fungal taxa were enriched under low phosphate, while another set of taxa were abundant under high-level phosphate conditions (Brisson et al., 2022). The quantity and quality of plant root exudates produced are also a function of plant genetics, which have profound effects on modulation of the rhizomicrobiome (Vives-Peris et al., 2020). Wippel et al. (2021) found that host preference in commensal bacteria from different taxa was related to their invasion of standing root-associated communities after comparing the root microbiota under different growth patterns among Lotus and Arabidopsis.
Plant roots coordinate the aboveground (leaf and shoot) and belowground (microbiota in the rhizosphere) elements of the holobiont, thus regulating and balancing the critical physiological functions in the microbiota–root–shoot environment (Hou et al., 2021). Organic compounds in root exudates can enhance nutrient supply to the soil/root microbiota (Sasse et al., 2018), which contributes to plant growth and soil health. In addition to being a carbon source, root exudates can also mediate biological activity such as nutrient cycling and the dynamics of soil-borne pathogen development (Coskun et al., 2017). Pathogenic microbes in soil can trigger plant immunity by modulating the root metabolism (resulting in specific exudate profiles) to recruit the root microbiota most effective in promoting plant defense and resistance to pest organisms (Berendsen et al., 2018; Yuan et al., 2018; Hou et al., 2020). Root exudates also conduct elements of communication between plants and associated microorganisms, and with neighboring plants (Preece and Peñuelas, 2020), including shaping the composition of the rhizomicrobiome and reducing inter-plant competition via allelopathy exudates (Calvo et al., 2017; Vives-Peris et al., 2017; Canarini et al., 2019; Wang et al., 2019; Jacoby et al., 2020; Tian et al., 2020). These communications/interactions among holobiont members are mediated through root exudates, which can act as signal compounds, establishing mutually beneficial relationships and making the host plant well adapted to its immediate environment.
While we know that root exudates have multiple functions, the inaccessibility of the root system has made it difficult to collect and characterize root exudates, resulting in limited understanding of the detailed mechanism(s) involved. In this paper, the role of root exudates in rhizospheric inter-organismal communications is introduced, including collection approaches and analysis techniques applied to root exudates, followed by a potential combination between root exudates and currently unculturable rhizomicrobiome members in vitro, finishing with considerations regarding future research required to understand the role of root exudates and their application to enhancement of agricultural productivity.
The rhizosphere is a hot spot of information transfer in microbe–plant, microbe–microbe, and plant–plant interactions, via signals including phytohormones and other secondary metabolites (Wang et al., 2020) from root exudates. Such communications/interactions are always accompanied by the signals released by plants, and in some cases by both plants and microbes. A signal produced by the plant can induce a return signal released by a microbe, or inhibit microbial signal production, so that it can be both positive and negative in nature (Buhian and Bensmihen, 2018; Lyu et al., 2020). Positive controls are most studied in symbiotic relationships, including legume–rhizobia, arbuscular mycorrhizal fungi (AMF)–SLs, and actinorhizal plant–Frankia interactions; such communication establishment relies on both root signals and microbe signals. Root exudates containing antagonistic “signals” directly inhibit or kill (static or cidal) pathogenic microbes [through root exudate signals: phenolic compounds, non-volatile terpenoids, volatile terpenes, and sulfurous compounds (Rizaludin et al., 2021)] or possibly by suppressing the production of microbe signals back to plants. The defense mechanism of plants is set off by regulating root exudation after receiving the signal released from beneficial or pathogenic bacteria (Hernández-Calderón et al., 2018). Therefore, the behavior of plants, with regard to releasing negative signals, is generally regarded as self-protective in nature.
Root exudate chemical signals detect disturbances within the rhizosphere or surrounding environments and assist plants in adapting to and resisting the potential negative effects of unfavorable conditions (Zhalnina et al., 2018; Tian et al., 2020). Allelochemical signals are produced to improve plant robustness by reducing competition from neighboring plants (Wang et al., 2020). For instance, root exudates produced by barnyardgrass can induce the production of allelochemicals by rice, such as phenolic acids, that trigger induced systematic resistance and reduce weed presence, or acclimate the plant to weed competition stress (Zhang et al., 2018); the presence of barnyardgrass root exudate can be one of the most important factors contributing to phenolic synthesis in rice.
Root signals produced in crop rotation cultivation systems also play pivotal roles in the fitness and performance of next-generation plants. Hu et al. (2018) illustrated that benzoxazinoids (secondary metabolites produced by cereals, including maize) assist in the suppression of herbivore prevalence in the next generation for maize (Zea mays L., genotypes B73). In addition, high levels of organic acid anions released into soil by soybean and cowpea (genotypes TGm 1511 and IT89KD-391, respectively) improve P acquisition efficiency under P deficiency, and subsequently, organic P that accumulated in the previous legume crop residues contributes to enhance the following maize crop (Jemo et al., 2006). Legumes (e.g., faba bean) can release root exudates containing fixed N (e.g., NH4+ and amino acids) as a source of N for subsequent maize growth (Coskun et al., 2017). In return, an isoflavonoid signal produced by maize contributes to N availability and biomass yield improvement of faba bean. The above examples illustrate how, through signals, root exudates can facilitate belowground interactions and communications.
Plant growth is affected by the rhizomicrobiome, the composition of which drives important elements of root activity, including root exudation of metabolites, and modulates signal transmission (Korenblum et al., 2020). Plants also contribute to the establishment of symbiotic relationships with associated microbes. In this process, root exudates as signals modulate the diversity and abundance of microbes in the rhizosphere (de Vries et al., 2020), which often happens under unfavorable environmental conditions (Morris and Moury, 2019; Rolfe et al., 2019). When under attack by the fungal pathogen Fusarium oxysporum, tryptophan is released from cucumber roots, acting as a signal recruiting Bacillus amyloliquefaciens to act against infection by the pathogen (Liu et al., 2017). In another example, tomato root exudates of lactic acid and hexanoic acid promoted the growth of the biocontrol strain of Bacillus cereus, reducing the infection rate of Ralstonia solanacearum (Wang et al., 2019). Exudates containing carbohydrate and organic acids can also increase the abundance of organic compound-degrading microorganisms (Lu et al., 2017; Zhalnina et al., 2018) so that their presence enhances the degradation of organic pollutants in the soil (Jia et al., 2016; Jia et al., 2018a). This is referred to as plant–soil feedback including both negative and positive feedback (Hu et al., 2018). Plants assemble the appropriate suite of beneficial microbes through changing the chemical composition of root exudates, thus improving plant resilience and fitness (Coskun et al., 2017). The type of root signals also varied significantly among plant species and ultimately determined the composition of the rhizomicrobiome (Wu et al., 2017; Luo et al., 2021). Rhizobia require the presence of isoflavonoid signals to activate nod genes, thus stimulating nodule formation by legumes (Coskun et al., 2017). Mycorrhizal fungi facilitate symbiotic interactions through strigolactone, a component of root exudates produced by plants (Akiyama and Hayashi, 2006; Ruyter-Spira and Bouwmeester, 2012). Therefore, rhizosphere communication is conducted and modulated by both host plants (root exudates) and microorganisms (Hortal et al., 2017; Mavrodi et al., 2021), contributing to the diversity and balance of the soil ecological web.
The recognition of the critical role of root exudates in the rhizosphere and in the soil ecosystem that leads to the actions of secreted exudates has been studied more intensely over the last two decades. The methodologies for root exudate collection differ substantially among studies, due to the inaccessibility of the root system; the set of methods mainly include the following: (1) hydroponic systems, (2) soil-hydroponic mixing systems, and (3) soil growth and sampling. Detailed description used to collect root exudates and the comparisons among improved methods are provided in Table 1. It is no doubt that the sampling techniques improve with the understanding of the dynamics of related rhizosphere processes. The critical aspect of all three sets of approaches uses artificial systems to collect components of the exudate under axenic conditions to avoid overwhelming by chemicals in the soil. Despite the numerous studies modifying the techniques, the accuracy of the results obtained from these artificial conditions, compared with the ecologically relevant exudates released under complex rhizosphere conditions (e.g., field soil), in the context of the rhizosphere processes contributed to by exudates and the presence of other organisms, remains unclear. Therefore, it is doubtful that using root exudates produced under near-sterile conditions, instead of the complex natural rhizosphere, to explore the mode of action in the interaction between root exudates and its surrounding organisms accurately reflects normal composition and will produce “real world” results. Although a standard approach for root exudate sampling is yet to be exploited, studies that explore the mode of actions of root exudates could focus on plant responses under a set of parallel experiments with or without certain treatments.
Considering the interaction within all organisms, root exudate identification should not be separated from the rhizospheric organisms. In such situation, multi-omics approaches help to visualize the temporal–spatial distributions of root exudates and the associated microbiome. With regard to the beneficial aspects of each technique, genome sequences can firstly identify the specific traits of functional bacterial isolates involved in growth strategies, substrate uptake, and extracellular enzyme production related to fitness of host in the rhizosphere (Mönchgesang et al., 2016; Zhalnina et al., 2018). Then, an exometabolomics approach based on the principle of mass spectrometry further determines how the isolated microbes interact with root exudate metabolites (Silva and Northen, 2015). To study in detail metabolically active microbes that assimilate or respond to root exudates, an effective tool to at least partially understand which metabolites are plant- or microbe-derived is isotopic labeling, which can track the passage of an isotope through a reaction, metabolic pathway, or cell (Basu et al., 2011). Knowing the relationship between organisms, transcriptomics is next used to detect the relevant gene response to root exudate production (Yi et al., 2017; Zhang et al., 2020). Finally, proteomic analyses will provide important information regarding how gene-expression manipulations relate to specific proteins involved in modifying plant development and behavior, and how this impacts interactions with microbes, making them compatible or incompatible with the surroundings that the host is facing (Afroz et al., 2013; Zhang et al., 2020).
For structural elucidation of root exudate metabolites, collected exudate can also be fractionated by flash chromatography, purified by high-performance liquid chromatography–solid phase extraction (HPLC-SPE), and then subjected to nuclear magnetic resonance (NMR) analysis (Kalala et al., 2020). To examine the symbiotic relationship models between microbes and a single compound or signal, purified compounds can be used to test its bioactivities associated with microorganismal isolates in the rhizosphere. Improving the understanding of root exudate composition has the potential to lead to development of new techniques, in order to obtain plant metabolites on a large scale and could also extend our collective understanding regarding interactions of plants and associated microbes. These multi-omics can help us explore interactions among organisms within the holobiont, instead of focusing on single aspects (plants or microbial communities). Additionally, multiple technique applications could provide insight into the role(s) of these signal exchanges in inter-organismal interactions and the overall functioning and viability of a given holobiont.
In the aspect of microbes involving root exudation production, pre-collection of exudates could be a part of screening to identify microbes that interact regularly with signals from plant roots and other associated microbes. Traditionally, microbial screening has been conducted in artificial growth media under sterile laboratory conditions to select microbes with potential key holobiont bioactivities. Microbes that are easily grown and that readily colonize plant roots are generally chosen for further analysis. There have been promising laboratory results from work in artificial media provided with approximately 100-fold higher concentrations of nutrients than generally occurs in the rhizosphere under field conditions (Lugtenberg et al., 2017). However, microbes isolated in vitro make up only about 1%–5% of total microbes (Pham and Kim, 2012). As the new update, Zhalnina et al. (2018) obtained 39 isolates representing approximately 10%–12% of the total bacterial community in the rhizosphere. It indicates that the true diversity of unculturable microorganisms in the rhizosphere is associated with in situ surroundings. Using rice as model, Edwards et al. (2015) found that methanogenic archaea were more enriched in the field (rice paddies) than in the greenhouse condition. The authors later verified that low levels of methane (CH4) production were detected under greenhouse growth (less rich methanogenic archaea) because methanogenic archaea cooperate with syntrophic partners to obtain H2 and formate for CH4 synthesis. In such cases, a novel genome-centric metatranscriptomics approach recently reported by Jia et al. (2018b); Hao et al. (2020), and Treu et al. (2016) can be used to effectively detect the low-abundant microbial members, specifically syntrophic bacteria, which cannot be analyzed based on isolates or enrichment cultures. This approach is useful for culture-independent identification of microbial involved in interaction with plant roots.
On the other hand, screening or growing in pure culture is still an irreplaceable technique to study the physiological properties of an organism. One of the possible reasons for uncultured microbes is the absence of density-dependent cell signaling under laboratory conditions (Camilli and Bassler, 2006; Pham and Kim, 2012), while these microbes have probably co-evolved with plants for a protracted period. The exogenous root exudate malic acid secreted from banana and added into LB medium induced the chemotactic response and biofilm formation of the B. amyloliquefaciens NJN-6 (Yuan et al., 2015). Malic acid released from tomato and citric acid detected in cucumber exudates also induced the motility of Paenibacillus polymyxa SQR-21 (Ling et al., 2011) and Bacillus amyloliquefaciens SQR9 (Zhang et al., 2014), respectively, thereby contributing to preferential colonization into roots. These findings suggest that plant roots have profound influence on the capacity of different rhizosphere colonization of soil bacteria (da Rocha et al., 2014). Therefore, providing microbes with pre-collected plant root exudates may allow them to receive right signals or improve the growth rate and colonization efficiency of microbes on non-host plant species (Figure 1).
Figure 1 The presence of root exudates affects the diversity and abundance of beneficial microbes in the rhizosphere compared to bulk soil (left) and could also affect culturable microbes under laboratory condition (right).
Plant evolution has always involved microorganisms, including in the form of important organelles (mitochondria and chloroplasts), developed through endosymbiosis of prokaryotes leading to a rigid and completely obligatory mutualism interaction present in all terrestrial plants (Lyu et al., 2021a; Lyu et al., 2021b). Plant evolution is meaningfully driven by interactions with symbiotic microbes (Delaux and Schornack, 2021), where the presence of the microbe substantially enhances the fitness of the plant. Root exudates play a critical role in the establishment of symbiosis relationships between plants and microorganisms. In the case of the legume nitrogen-fixing symbiosis, isoflavonoids produced by legume plants induce rhizobia to release lipo-chitooligosaccharides (LCOs) as signals back to the legume, initiating nodule formation, leading to nitrogen fixation; in addition, LCOs enhance stress resistance in legumes and also a wide range of non-legume plants, contributing to critical modifications of the physicochemical properties of target plants, thus emphasizing the importance of symbiosis establishment with legumes and the regulation of abiotic stress tolerance in many other plant groups (Lyu et al., 2020). In contrast, AMF can establish symbiotic relationships with 80% of higher plants, which rely on the fungal hyphae to assist in acquiring nutrients; AMF require contact with the host plant root to finish their life cycle (Wipf et al., 2019). In this symbiosis, specific root exudates (strigolactones and flavonoids) produced by the host can communicate with AMF to facilitate the AMF symbiosis via stimulation of the Myc Factor production to set the stage for the establishment of the symbiosis that will enhance the availability of nutrients for host plants in, for instance, maize–legume intercropping systems (Coskun et al., 2017).
The establishment of microbial communities on plants is influenced by the plant compartment (Bai et al., 2015) and host species (Ofek-Lalzar et al., 2014; Tkacz et al., 2015) and soils (del Pilar Martínez-Diz et al., 2019; Edwards et al., 2019). Plants are predominantly photosynthetic eukaryotes of the kingdom Plantae, which are commonly defined as C3, C4, and CAM (Crassulacean Acid Metabolism) according to the CO2 fixation mechanism (Sivaram et al., 2020). The photosynthetic carbon fixation pathways are critical for plants to convert CO2 into what they need for their growth and development (Heyneke and Fernie, 2018). Moreover, different types of plants ultimately release different quantities and qualities of compounds into the rhizosphere as root exudates. Some studies reported that a greater variety of dominant organic acids and sugars are released by C3 plants (Zhu et al., 2008; Meyer et al., 2010; Vranova et al., 2013), but a higher quantity of root exudates is secreted by C4 plants, as they can fix carbon more rapidly through greater photosynthetic rates (Bledsoe et al., 2020). Thus, the different compositions (i.e., carbohydrates, organic acids, and amino acid) of root exudates could affect the assemblage of associated microorganisms. For instance, meta-analysis has shown that there are more AMFs in the rhizosphere when C4 plants are grown in a soil than when C3 plants are in the same soil (Pirttilä et al., 2021). Prior studies also demonstrated that C4 plants recruit a higher abundance of the polycyclic aromatic hydrocarbon degrading bacterial community than C3 plants because photosystems of C3 and C4 led to differences in root secretions (Sivaram et al., 2020). However, C4 plants may be less efficient at photosynthesis under the higher CO2 levels of the near future. The composition of root exudates released by C3 and C4 plants may change, such as increases in organic acid production under higher atmospheric CO2 levels (Xiong et al., 2019). For instance, the abundance of organic acids, oxalate, citrate, and malate is increased in root exudates under elevated CO2 (Sánchez-Carrillo et al., 2018). Interestingly, the root exudation of organic C from C3 and C4 plants can be changed by the soil nutrient status, further affecting rhizosphere bacterial community structure (Carvalhais et al., 2011; Wu et al., 2012; Bledsoe et al., 2020). Host species with CAM metabolism can also assemble the unique bacterial, archaeal, and fungal communities in the rhizosphere (Citlali et al., 2018). Therefore, the plant–microbe interactions in a given environment is mainly determined by the C fixation or plant photosynthesis modes and the allocation patterns of C released by the plants (Cregger et al., 2021). The rate of C fixation affects the component of root exudates, which contributes to the network of plant–microbe–plant and plant–plant communications and assembly of the unique holobiont; thus, they can play a role in the establishment of symbiotic relationships and thereby contribute to plant physiology, development, and biomass production. However, the role of root exudates has been elucidated in only a few of these symbioses, encompassing only a few specific plant species. Future investigations of these interactions, involving a range of plant types, may uncover new modes of molecular symbiotic interaction.
Root exudate is a key element of plant homeostasis, in part through playing a key role in communication between aboveground and belowground elements of plants. Root signals affect interactions with the associated phytomicrobiome, leading to a functional and effective holobiont that benefits plant growth. However, there are certainly still unknown signals in inter-organismal communications, produced by both plants and microbes. Discovering and exploring more signals will extend our understanding of plant–plant and plant–microbe interactions. Moreover, exploiting new techniques for collecting plant metabolites will provide access to various inter-organismal signals on a large scale. In the long term, agricultural production will be able to take full advantage of the phytomicrobiome by manipulating plant root signal production. Importantly, plant C-fixation strategy is a major component driving rhizospheric development and maintenance. Evaluating the underground impact of biodesign programs leveraging C-fixation engineering in plants will thus be of great importance. All bioprospecting under laboratory conditions aims to identify the potential beneficial microbes that then could be efficiently employed as a sustainable strategy to improve crop plant resilience and overall crop productivity. One application of root exudates could be as bio-inoculants to trigger the signaling between plants and microbes and also recruit beneficial microbes, as well as remove contaminants from the soil, thus enhancing the crop yield and biomass. Thus, the identification of components of exudates and understanding the mechanisms by which they regulate rhizomicrobiome networks should be considered an important line of future research.
● Are there one or more specific root exudate compounds/materials that cause/allow specific activities in plant-associated bacteria, including the ability to grow in vitro? If so, are these specific root exudates spontaneous or are they induced?
● What are the effects of stressful growth conditions on root exudate production? To what extent is secondary metabolite production in other parts of plants changed with the root exudate?
● Were root exudates involved in the evolution of phytomicrobiome? If so, what does this tell us about the holobiont?
DL gathered literature and prepared the manuscript. DS provided feedback and oversaw progression of the manuscript. All authors gave final approval for publication and agreed to be held accountable for the work contained therein.
The authors acknowledge Natural Science and Engineering Research Council (NSERC) of Canada Discovery Grant RGPIN-2020-07047 and NSERC collaborative research development (CRD) grant CRDP-J 517552-17 for funding this work.
The authors declare that the research was conducted in the absence of any commercial or financial relationships that could be construed as a potential conflict of interest.
All claims expressed in this article are solely those of the authors and do not necessarily represent those of their affiliated organizations, or those of the publisher, the editors and the reviewers. Any product that may be evaluated in this article, or claim that may be made by its manufacturer, is not guaranteed or endorsed by the publisher.
Afroz, A., Zahur, M., Zeeshan, N., Komatsu, S. (2013). Plant-bacterium interactions analyzed by proteomics. Front. Plant Sci. 4, 21. doi: 10.3389/fpls.2013.00021
Akiyama, K., Hayashi, H. (2006). Strigolactones: Chemical signals for fungal symbionts and parasitic weeds in plant roots. Ann. Bot. 97, 925–931. doi: 10.1093/aob/mcl063
Baetz, U., Martinoia, E. (2014). Root exudates: the hidden part of plant defense. Trends Plant Sci. 19, 90–98. doi: 10.1016/j.tplants.2013.11.006
Bai, Y., Müller, D. B., Srinivas, G., Garrido-Oter, R., Potthoff, E., Rott, M., et al. (2015). Functional overlap of the arabidopsis leaf and root microbiota. Nature 528, 364–369. doi: 10.1038/nature16192
Basu, S. S., Mesaros, C., Gelhaus, S. L., Blair, I. A. (2011). Stable isotope labeling by essential nutrients in cell culture for preparation of labeled coenzyme a and its thioesters. Analytical Chem. 83, 1363–1369. doi: 10.1021/ac1027353
Berendsen, R. L., Vismans, G., Yu, K., Song, Y., de Jonge, R., Burgman, W. P., et al. (2018). Disease-induced assemblage of a plant-beneficial bacterial consortium. ISME J. 12, 1496–1507. doi: 10.1038/s41396-018-0093-1
Bledsoe, R. B., Goodwillie, C., Peralta, A. L. (2020). Long-term nutrient enrichment of an oligotroph-dominated wetland increases bacterial diversity in bulk soils and plant rhizospheres. mSphere 5, e00035–e00020. doi: 10.1128/mSphere.00035-20
Brisson, V. L., Richardy, J., Kosina, S. M., Northen, T. R., Vogel, J. P., Gaudin, A. C. (2022). Phosphate availability modulates root exudate composition and rhizosphere microbial community in a teosinte and a modern maize cultivar. Phytobiomes J. 6, 83–94. doi: 10.1094/PBIOMES-06-21-0041-R
Buhian, W. P., Bensmihen, S. (2018). Mini-review: nod factor regulation of phytohormone signaling and homeostasis during rhizobia-legume symbiosis. Front. Plant Sci. 9, 1247. doi: 10.3389/fpls.2018.01247
Calvo, O. C., Franzaring, J., Schmid, I., Müller, M., Brohon, N., Fangmeier, A. (2017). Atmospheric CO 2 enrichment and drought stress modify root exudation of barley. Global Change Biol. 23, 1292–1304. doi: 10.1111/gcb.13503
Camilli, A., Bassler, B. L. (2006). Bacterial small-molecule signaling pathways. Science 311, 1113–1116. doi: 10.1126/science.1121357
Canarini, A., Kaiser, C., Merchant, A., Richter, A., Wanek, W. (2019). Root exudation of primary metabolites: Mechanisms and their roles in plant responses to environmental stimuli. Frontiers in Plant Science 10. doi: 10.3389/fpls.2019.00157
Carvalhais, L. C., Dennis, P. G., Fedoseyenko, D., Hajirezaei, M. R., Borriss, R., von Wirén, N. (2011). Root exudation of sugars, amino acids, and organic acids by maize as affected by nitrogen, phosphorus, potassium, and iron deficiency. J. Plant Nutr. Soil Sci. 174, 3–11. doi: 10.1002/jpln.201000085
Chen, S., Waghmode, T. R., Sun, R., Kuramae, E. E., Hu, C., Liu, B. (2019). Root-associated microbiomes of wheat under the combined effect of plant development and nitrogen fertilization. Microbiome 7, 136. doi: 10.1186/s40168-019-0750-2
Citlali, F.-G., Desgarennes, D., Flores-Núñez, V. M., Partida-Martínez, L. P. (2018). “Chapter 12 - the microbiome of desert CAM plants: Lessons from amplicon sequencing and metagenomics,” in "Metagenomics". Ed. Nagarajan, M. (Academic Press), 231–254.
Coskun, D., Britto, D. T., Shi, W., Kronzucker, H. J. (2017). How plant root exudates shape the nitrogen cycle. Trends Plant Sci. 22, 661. doi: 10.1016/j.tplants.2017.05.004
Cregger, M. A., Carper, D. L., Christel, S., Doktycz, M. J., Labbé, J., Michener, J. K., et al. (2021). Plant–microbe interactions: From genes to ecosystems using populus as a model system. Phytobiomes J. 5, 29–38. doi: 10.1094/PBIOMES-01-20-0009-FI
da Rocha, U. N., Plugge, C., George, I., van Elsas, J., van Overbeek, L. (2014). Correction: The rhizosphere selects for particular groups of acidobacteria and verrucomicrobia. PloS One 8, 12–e82443. PLoS ONE 9, e94514. doi: 10.1371/journal.pone.0094514
Delaux, P.-M., Schornack, S. (2021). Plant evolution driven by interactions with symbiotic and pathogenic microbes. Science 371, eaba6605. doi: 10.1126/science.aba6605
del Pilar Martínez-Diz, M., Andrés-Sodupe, M., Bujanda, R., Díaz-Losada, E., Eichmeier, A., Gramaje, D. (2019). Soil-plant compartments affect fungal microbiome diversity and composition in grapevine. Fungal Ecol. 41, 234–244. doi: 10.1016/j.funeco.2019.07.003
Demidchik, V. (2018). ROS-activated ion channels in plants: biophysical characteristics, physiological functions and molecular nature. Int. J. Mol. Sci. 19, 1263. doi: 10.3390/ijms19041263
de Vries, F. T., Griffiths, R. I., Knight, C. G., Nicolitch, O., Williams, A. (2020). Harnessing rhizosphere microbiomes for drought-resilient crop production. Science 368, 270–274. doi: 10.1126/science.aaz5192
Edwards, J., Johnson, C., Santos-Medellín, C., Lurie, E., Podishetty, N. K., Bhatnagar, S., et al. (2015). Structure, variation, and assembly of the root-associated microbiomes of rice. Proc. Natl. Acad. Sci. 112, E911–E920. doi: 10.1073/pnas.1414592112
Edwards, J., Santos-Medellín, C., Nguyen, B., Kilmer, J., Liechty, Z., Veliz, E., et al. (2019). Soil domestication by rice cultivation results in plant-soil feedback through shifts in soil microbiota. Genome Biol. 20, 1–14. doi: 10.1186/s13059-019-1825-x
Egle, K., Römer, W., Keller, H. (2003). Exudation of low molecular weight organic acids by lupinus albus l., lupinus angustifolius l. and lupinus luteus l. as affected by phosphorus supply. Agronomie 23, 511–518. doi: 10.1051/agro:2003025
Gao, W., Reed, S. C., Munson, S. M., Rui, Y., Fan, W., Zheng, Z., et al. (2021). Responses of soil extracellular enzyme activities and bacterial community composition to seasonal stages of drought in a semiarid grassland. Geoderma 401, 115327. doi: 10.1016/j.geoderma.2021.115327
Ghatak, A., Schindler, F., Bachmann, G., Engelmeier, D., Bajaj, P., Brenner, M., et al. (2022). Root exudation of contrasting drought-stressed pearl millet genotypes conveys varying biological nitrification inhibition (BNI) activity. Biol. fertility soils 58, 291–306. doi: 10.1007/s00374-021-01578-w
Hao, C., Dungait, J. A., Wei, X., Ge, T., Kuzyakov, Y., Cui, Z., et al. (2022). Maize root exudate composition alters rhizosphere bacteria to control hotspots of hydrolase activity in response to nitrogen supply. Soil Biol. Biochem. 108717. doi: 10.1016/j.soilbio.2022.108717
Hao, L., Michaelsen, T. Y., Singleton, C. M., Dottorini, G., Kirkegaard, R. H., Albertsen, M., et al. (2020). Novel syntrophic bacteria in full-scale anaerobic digesters revealed by genome-centric metatranscriptomics. ISME J. 14, 906–918. doi: 10.1038/s41396-019-0571-0
Hernández-Calderón, E., Aviles-Garcia, M. E., Castulo-Rubio, D. Y., Macías-Rodríguez, L., Ramírez, V. M., Santoyo, G., et al. (2018). Volatile compounds from beneficial or pathogenic bacteria differentially regulate root exudation, transcription of iron transporters, and defense signaling pathways in sorghum bicolor. Plant Mol. Biol. 96, 291–304. doi: 10.1007/s11103-017-0694-5
Herz, K., Dietz, S., Gorzolka, K., Haider, S., Jandt, U., Scheel, D., et al. (2018). Linking root exudates to functional plant traits. PloS One 13, e0204128. doi: 10.1371/journal.pone.0204128
Heyneke, E., Fernie, A. R. (2018). Metabolic regulation of photosynthesis. Biochem. Soc. Trans. 46, 321–328. doi: 10.1042/BST20170296
He, W., Yuan, Y., Zhang, Z., Xiao, J., Liu, Q., Laiho, R., et al. (2021). Effect of n addition on root exudation and associated microbial n transformation under sibiraea angustata in an alpine shrubland. Plant Soil 460, 469–481. doi: 10.1007/s11104-020-04753-4
Hortal, S., Lozano, Y. M., Bastida, F., Armas, C., Moreno, J., Garcia, C., et al. (2017). Plant-plant competition outcomes are modulated by plant effects on the soil bacterial community. Sci. Rep. 7, 1–9. doi: 10.1038/s41598-017-18103-5
Hou, S., Thiergart, T., Vannier, N., Mesny, F., Ziegler, J., Pickel, B., et al. (2020). Microbiota-root-shoot axis modulation by MYC2 favours arabidopsis growth over defence under suboptimal light. bioRxiv. doi: 10.1101/2020.11.06.371146
Hou, S., Wolinska, K. W., Hacquard, S. (2021). Microbiota-root-shoot-environment axis and stress tolerance in plants. Curr. Opin. Plant Biol. 62, 102028. doi: 10.1016/j.pbi.2021.102028
Hu, L., Robert, C. A., Cadot, S., Zhang, X., Ye, M., Li, B., et al. (2018). Root exudate metabolites drive plant-soil feedbacks on growth and defense by shaping the rhizosphere microbiota. Nat. Commun. 9, 1–13. doi: 10.1038/s41467-018-05122-7
Jacoby, R. P., Chen, L., Schwier, M., Koprivova, A., Kopriva, S. (2020). Recent advances in the role of plant metabolites in shaping the root microbiome. F1000Research 9. doi: 10.12688/f1000research.21796.1
Jemo, M., Abaidoo, R. C., Nolte, C., Tchienkoua, M., Sanginga, N., Horst, W. J. (2006). Phosphorus benefits from grain-legume crops to subsequent maize grown on acid soils of southern Cameroon. Plant Soil 284, 385–397. doi: 10.1007/s11104-006-0052-x
Jia, H., Hou, D., Dai, M., Lu, H., Yan, C. (2018a). Effects of root exudates on the mobility of pyrene in mangrove sediment-water system. Catena 162, 396–401. doi: 10.1016/j.catena.2017.10.022
Jia, H., Lu, H., Dai, M., Hong, H., Liu, J., Yan, C. (2016). Effect of root exudates on sorption, desorption, and transport of phenanthrene in mangrove sediments. Mar. pollut. Bull. 109, 171–177. doi: 10.1016/j.marpolbul.2016.06.004
Jia, Y., Ng, S.-K., Lu, H., Cai, M., Lee, P. K. (2018b). Genome-centric metatranscriptomes and ecological roles of the active microbial populations during cellulosic biomass anaerobic digestion. Biotechnol. Biofuels 11, 1–15. doi: 10.1186/s13068-018-1121-0
Jiang, Z., Thakur, M. P., Liu, R., Zhou, G., Zhou, L., Fu, Y., et al. (2022). Soil p availability and mycorrhizal type determine root exudation in sub-tropical forests. Soil Biol. Biochem., 108722. doi: 10.1016/j.soilbio.2022.108722
Kalala, W. M., Foubert, K., Pieters, L., Maregesi, S. (2020). HPLC-SPE-NMR analysis of furanosesquiterpenoids from bark exudates of commiphora swynnertonii burrt. Phytochem. Lett. 38, 128–132. doi: 10.1016/j.phytol.2020.06.006
Kawasaki, A., Okada, S., Zhang, C., Delhaize, E., Mathesius, U., Richardson, A. E., et al. (2018). A sterile hydroponic system for characterising root exudates from specific root types and whole-root systems of large crop plants. Plant Methods 14, 114. doi: 10.1186/s13007-018-0380-x
Korenblum, E., Dong, Y., Szymanski, J., Panda, S., Jozwiak, A., Massalha, H., et al. (2020). Rhizosphere microbiome mediates systemic root metabolite exudation by root-to-root signaling. Proc. Natl. Acad. Sci. 117, 3874–3883. doi: 10.1073/pnas.1912130117
Li, H., La, S., Zhang, X., Gao, L., Tian, Y. (2021). Salt-induced recruitment of specific root-associated bacterial consortium capable of enhancing plant adaptability to salt stress. ISME J. 15, 2865–2882. doi: 10.1038/s41396-021-00974-2
Ling, N., Raza, W., Ma, J., Huang, Q., Shen, Q. (2011). Identification and role of organic acids in watermelon root exudates for recruiting paenibacillus polymyxa SQR-21 in the rhizosphere. Eur. J. Soil Biol. 47, 374–379. doi: 10.1016/j.ejsobi.2011.08.009
Liu, Y., Chen, L., Wu, G., Feng, H., Zhang, G., Shen, Q., et al. (2017). Identification of root-secreted compounds involved in the communication between cucumber, the beneficial bacillus amyloliquefaciens, and the soil-borne pathogen fusarium oxysporum. Mol. Plant-Microbe Interact. 30, 53–62. doi: 10.1094/MPMI-07-16-0131-R
Lugtenberg, B., Rozen, D. E., Kamilova, F. (2017). Wars between microbes on roots and fruits. F1000Research 6. doi: 10.12688/f1000research.10696.1
Luo, X., Liu, K., Shen, Y., Yao, G., Yang, W., Mortimer, P. E., et al. (2021). Fungal community composition and diversity vary with soil horizons in a subtropical forest. Front. Microbiol. 12. doi: 10.3389/fmicb.2021.650440
Luo, L.-F., Yang, L., Yan, Z.-X., Jiang, B.-B., Li, S., Huang, H.-C., et al. (2020). Ginsenosides in root exudates of panax notoginseng drive the change of soil microbiota through carbon source different utilization. Plant Soil 455, 139–153. doi: 10.1007/s11104-020-04663-5
Lu, H., Sun, J., Zhu, L. (2017). The role of artificial root exudate components in facilitating the degradation of pyrene in soil. Sci. Rep. 7, 1–10. doi: 10.1038/s41598-017-07413-3
Lyu, D., Backer, R., Subramanian, S., Smith, D. L. (2020). Phytomicrobiome coordination signals hold potential for climate change-resilient agriculture. Front. Plant Sci. 11, 634. doi: 10.3389/fpls.2020.00634
Lyu, D., Msimbira, L. A., Nazari, M., Antar, M., Pagé, A., Shah, A., et al. (2021a). The coevolution of plants and microbes underpins sustainable agriculture. Microorganisms 9, 1036. doi: 10.3390/microorganisms9051036
Lyu, D., Zajonc, J., Pagé, A., Tanney, C. A., Shah, A., Monjezi, N., et al. (2021b). Plant holobiont theory: The phytomicrobiome plays a central role in evolution and success. Microorganisms 9, 675. doi: 10.3390/microorganisms9040675
Mavrodi, O. V., McWilliams, J. R., Peter, J. O., Berim, A., Hassan, K. A., Elbourne, L. D., et al. (2021). Root exudates alter the expression of diverse metabolic, transport, regulatory, and stress response genes in rhizosphere pseudomonas. Front. Microbiol. 12. doi: 10.3389/fmicb.2021.651282
Meyer, S., De Angeli, A., Fernie, A. R., Martinoia, E. (2010). Intra-and extra-cellular excretion of carboxylates. Trends Plant Sci. 15, 40–47. doi: 10.1016/j.tplants.2009.10.002
Mönchgesang, S., Strehmel, N., Schmidt, S., Westphal, L., Taruttis, F., Müller, E., et al. (2016). Natural variation of root exudates in arabidopsis thaliana-linking metabolomic and genomic data. Sci. Rep. 6, 1–11. doi: 10.1038/srep29033
Morris, C. E., Moury, B. (2019). Revisiting the concept of host range of plant pathogens. Annu. Rev. Phytopathol. 57, 63–90. doi: 10.1146/annurev-phyto-082718-100034
Murphy, K. M., Edwards, J., Louie, K. B., Bowen, B. P., Sundaresan, V., Northen, T. R., et al. (2021). Bioactive diterpenoids impact the composition of the root-associated microbiome in maize (Zea mays). Sci. Rep. 11, 333. doi: 10.1038/s41598-020-79320-z
Oburger, E., Dell‘mour, M., Hann, S., Wieshammer, G., Puschenreiter, M., Wenzel, W. W. (2013). Evaluation of a novel tool for sampling root exudates from soil-grown plants compared to conventional techniques. Environ. Exp. Bot. 87, 235–247. doi: 10.1016/j.envexpbot.2012.11.007
Ofek-Lalzar, M., Sela, N., Goldman-Voronov, M., Green, S. J., Hadar, Y., Minz, D. (2014). Niche and host-associated functional signatures of the root surface microbiome. Nat. Commun. 5, 1–9. doi: 10.1038/ncomms5950
Pham, V. H., Kim, J. (2012). Cultivation of unculturable soil bacteria. Trends Biotechnol. 30, 475–484. doi: 10.1016/j.tibtech.2012.05.007
Phillips, R. P., Erlitz, Y., Bier, R., Bernhardt, E. S. (2008). New approach for capturing soluble root exudates in forest soils. Funct. Ecol. 22, 990–999. doi: 10.1111/j.1365-2435.2008.01495.x
Pirttilä, A. M., Mohammad Parast Tabas, H., Baruah, N., Koskimäki, J. J. (2021). Biofertilizers and biocontrol agents for agriculture: How to identify and develop new potent microbial strains and traits. Microorganisms 9, 817. doi: 10.3390/microorganisms9040817
Preece, C., Peñuelas, J. (2020). A return to the wild: root exudates and food security. Trends Plant Sci. 25, 14–21. doi: 10.1016/j.tplants.2019.09.010
Rizaludin, M. S., Stopnisek, N., Raaijmakers, J. M., Garbeva, P. (2021). The chemistry of stress: Understanding the ‘Cry for help’of plant roots. Metabolites 11, 357. doi: 10.3390/metabo11060357
Rolfe, S. A., Griffiths, J., Ton, J. (2019). Crying out for help with root exudates: adaptive mechanisms by which stressed plants assemble health-promoting soil microbiomes. Curr. Opin. Microbiol. 49, 73–82. doi: 10.1016/j.mib.2019.10.003
Ruyter-Spira, C., Bouwmeester, H. (2012). Strigolactones affect development in primitive plants. the missing link between plants and arbuscular mycorrhizal fungi? New Phytol. 195, 730–733. Available at: http://www.jstor.org/stable/newphytologist.195.4.730
Sánchez-Carrillo, S., Álvarez-Cobelas, M., Angeler, D. G., Serrano-Grijalva, L., Sánchez-Andrés, R., Cirujano, S., et al. (2018). Elevated atmospheric CO2 increases root exudation of carbon in wetlands: Results from the first free-air CO2 enrichment facility (FACE) in a marshland. Ecosystems 21, 852–867. doi: 10.1007/s10021-017-0189-x
Sasse, J., Martinoia, E., Northen, T. (2018). Feed your friends: do plant exudates shape the root microbiome? Trends Plant Sci. 23, 25–41. doi: 10.1016/j.tplants.2017.09.003
Silva, L. P., Northen, T. R. (2015). Exometabolomics and MSI: deconstructing how cells interact to transform their small molecule environment. Curr. Opin. Biotechnol. 34, 209–216. doi: 10.1016/j.copbio.2015.03.015
Sivaram, A. K., Subashchandrabose, S. R., Logeshwaran, P., Lockington, R., Naidu, R., Megharaj, M. (2020). Rhizodegradation of PAHs differentially altered by C3 and C4 plants. Sci. Rep. 10, 16109. doi: 10.1038/s41598-020-72844-4
Tian, T., Reverdy, A., She, Q., Sun, B., Chai, Y. (2020). The role of rhizodeposits in shaping rhizomicrobiome. Environ. Microbiol. Rep. 12, 160–172. doi: 10.1111/1758-2229.12816
Tkacz, A., Cheema, J., Chandra, G., Grant, A., Poole, P. S. (2015). Stability and succession of the rhizosphere microbiota depends upon plant type and soil composition. ISME J. 9, 2349–2359. doi: 10.1038/ismej.2015.41
Torabi, M., Mokhtarzadeh, A., Mahlooji, M. (2012). The role of hydroponics technique as a standard methodology in various aspects of plant biology researches. Hydroponics–a standard methodology for plant biological researches. InTech 113-134. doi: 10.5772/36612
Treu, L., Campanaro, S., Kougias, P. G., Zhu, X., Angelidaki, I. (2016). Untangling the effect of fatty acid addition at species level revealed different transcriptional responses of the biogas microbial community members. Environ. Sci. Technol. 50, 6079–6090. doi: 10.1021/acs.est.6b00296
Vickers, N. J. (2017). Animal communication: when i’m calling you, will you answer too? Curr. Biol. 27, R713–R715. doi: 10.1016/j.cub.2017.05.064
Vives-Peris, V., de Ollas, C., Gómez-Cadenas, A., Pérez-Clemente, R. M. (2020). Root exudates: From plant to rhizosphere and beyond. Plant Cell Rep. 39, 3–17. doi: 10.1007/s00299-019-02447-5
Vives-Peris, V., Gómez-Cadenas, A., Pérez-Clemente, R. M. (2017). Citrus plants exude proline and phytohormones under abiotic stress conditions. Plant Cell Rep. 36, 1971–1984. doi: 10.1007/s00299-017-2214-0
Vranova, V., Rejsek, K., Skene, K. R., Janous, D., Formanek, P. (2013). Methods of collection of plant root exudates in relation to plant metabolism and purpose: a review. J. Plant Nutr. Soil Sci. 176, 175–199. doi: 10.1002/jpln.201000360
Wang, N. Q., Kong, C. H., Wang, P., Meiners, S. J. (2020). Root exudate signals in plant–plant interactions. Plant Cell Environment. 44(4), 1044–1058. doi: 10.1111/pce.13892
Wang, N., Wang, L., Zhu, K., Hou, S., Chen, L., Mi, D., et al. (2019). Plant root exudates are involved in bacillus cereus AR156 mediated biocontrol against ralstonia solanacearum. Front. Microbiol. 10, 98. doi: 10.3389/fmicb.2019.00098
Wen, Z., White, P. J., Shen, J., Lambers, H. (2022). Linking root exudation to belowground economic traits for resource acquisition. New Phytol. 233, 1620–1635. doi: 10.1111/nph.17854
Wipf, D., Krajinski, F., van Tuinen, D., Recorbet, G., Courty, P. E. (2019). Trading on the arbuscular mycorrhiza market: from arbuscules to common mycorrhizal networks. New Phytol. 223, 1127–1142. doi: 10.1111/nph.15775
Wippel, K., Tao, K., Niu, Y., Zgadzaj, R., Kiel, N., Guan, R., et al. (2021). Host preference and invasiveness of commensal bacteria in the lotus and arabidopsis root microbiota. Nat. Microbiol. 6, 1150–1162. doi: 10.1038/s41564-021-00941-9
Wu, F. Y., Chung, A. K. C., Tam, N. F. Y., Wong, M. H. (2012). Root exudates of wetland plants influenced by nutrient status and types of plant cultivation. Int. J. Phytoremediation 14, 543–553. doi: 10.1080/15226514.2011.604691
Wu, H., Wang, X., He, X., Zhang, S., Liang, R., Shen, J. (2017). Effects of root exudates on denitrifier gene abundance, community structure and activity in a micro-polluted constructed wetland. Sci. Total Environ. 598, 697–703. doi: 10.1016/j.scitotenv.2017.04.150
Xiong, L., Liu, X., Vinci, G., Spaccini, R., Drosos, M., Li, L., et al. (2019). Molecular changes of soil organic matter induced by root exudates in a rice paddy under CO2 enrichment and warming of canopy air. Soil Biol. Biochem. 137, 107544. doi: 10.1016/j.soilbio.2019.107544
Yi, Y., de Jong, A., Frenzel, E., Kuipers, O. P. (2017). Comparative transcriptomics of bacillus mycoides strains in response to potato-root exudates reveals different genetic adaptation of endophytic and soil isolates. Front. Microbiol. 8, 1487. doi: 10.3389/fmicb.2017.01487
Yuan, J., Zhang, N., Huang, Q., Raza, W., Li, R., Vivanco, J. M., et al. (2015). Organic acids from root exudates of banana help root colonization of PGPR strain bacillus amyloliquefaciens NJN-6. Sci. Rep. 5, 13438. doi: 10.1038/srep13438
Yuan, J., Zhao, J., Wen, T., Zhao, M., Li, R., Goossens, P., et al. (2018). Root exudates drive the soil-borne legacy of aboveground pathogen infection. Microbiome 6, 1–12. doi: 10.1186/s40168-018-0537-x
Zhalnina, K., Louie, K. B., Hao, Z., Mansoori, N., da Rocha, U. N., Shi, S., et al. (2018). Dynamic root exudate chemistry and microbial substrate preferences drive patterns in rhizosphere microbial community assembly. Nat. Microbiol. 3, 470–480. doi: 10.1038/s41564-018-0129-3
Zhang, X., Cheng, W., Feng, Z., Zhu, Q., Sun, Y., Li, Y., et al. (2020). Transcriptomic analysis of gene expression of verticillium dahliae upon treatment of the cotton root exudates. BMC Genomics 21, 155. doi: 10.1186/s12864-020-6448-9
Zhang, Q., Li, L., Li, J., Wang, H., Fang, C., Yang, X., et al. (2018). Increasing rice allelopathy by induction of barnyard grass (Echinochloa crus-galli) root exudates. J. Plant Growth Regul. 37, 745–754. doi: 10.1007/s00344-017-9770-y
Zhang, N., Wang, D., Liu, Y., Li, S., Shen, Q., Zhang, R. (2014). Effects of different plant root exudates and their organic acid components on chemotaxis, biofilm formation and colonization by beneficial rhizosphere-associated bacterial strains. Plant Soil 374, 689–700. doi: 10.1007/s11104-013-1915-6
Zhao, M., Zhao, J., Yuan, J., Hale, L., Wen, T., Huang, Q., et al. (2021). Root exudates drive soil-microbe-nutrient feedbacks in response to plant growth. Plant Cell Environ. 44, 613–628. doi: 10.1111/pce.13928
Keywords: root exudate, signaling, rhizomicrobiome, holobiont, plant-microbes interaction, C-fixation
Citation: Lyu D and Smith DL (2022) The root signals in rhizospheric inter-organismal communications. Front. Plant Sci. 13:1064058. doi: 10.3389/fpls.2022.1064058
Received: 07 October 2022; Accepted: 06 December 2022;
Published: 21 December 2022.
Edited by:
Henrique De Paoli, Berkeley Lab (DOE), United StatesReviewed by:
Rupali Gupta, Volcani Center, IsraelCopyright © 2022 Lyu and Smith. This is an open-access article distributed under the terms of the Creative Commons Attribution License (CC BY). The use, distribution or reproduction in other forums is permitted, provided the original author(s) and the copyright owner(s) are credited and that the original publication in this journal is cited, in accordance with accepted academic practice. No use, distribution or reproduction is permitted which does not comply with these terms.
*Correspondence: Donald L. Smith, ZG9uYWxkLnNtaXRoQG1jZ2lsbC5jYQ==
Disclaimer: All claims expressed in this article are solely those of the authors and do not necessarily represent those of their affiliated organizations, or those of the publisher, the editors and the reviewers. Any product that may be evaluated in this article or claim that may be made by its manufacturer is not guaranteed or endorsed by the publisher.
Research integrity at Frontiers
Learn more about the work of our research integrity team to safeguard the quality of each article we publish.