- 1State Key Laboratory for Conservation and Utilization of Subtropical Agro-bioresources, Guangdong Province Key Laboratory of Microbial Signals and Disease Control, Integrative Microbiology Research Centre, South China Agricultural University, Guangzhou, China
- 2Guangdong Laboratory for Lingnan Modern Agriculture, College of Plant Protection, South China Agricultural University, Guangzhou, China
- 3Department of Food Science, Purdue University, West Lafayette, IN, United States
With the increasing resistance exhibited by undesirable bacteria to traditional antibiotics, the need to discover alternative (or, at least, supplementary) treatments to combat chemically resistant bacteria is becoming urgent. Quorum sensing (QS) refers to a novel bacterial communication system for monitoring cell density and regulation of a network of gene expression that is mediated by a group of signaling molecules called autoinducers (AIs). QS-regulated multicellular behaviors include biofilm formation, horizontal gene transfer, and antibiotic synthesis, which are demonstrating increasing pathogenicity to plants and aquacultural animals as well as contamination of wastewater treatment devices. To inhibit QS-regulated microbial behaviors, the strategy of quorum quenching (QQ) has been developed. Different quorum quenchers interfere with QS through different mechanisms, such as competitively inhibiting AI perception (e.g., by QS inhibitors) and AI degradation (e.g., by QQ enzymes). In this review, we first introduce different signaling molecules, including diffusible signal factor (DSF) and acyl homoserine lactones (AHLs) for Gram-negative bacteria, AIPs for Gram-positive bacteria, and AI-2 for interspecies communication, thus demonstrating the mode of action of the QS system. We next exemplify the QQ mechanisms of various quorum quenchers, such as chemical QS inhibitors, and the physical/enzymatic degradation of QS signals. We devote special attention to AHL-degrading enzymes, which are categorized in detail according to their diverse catalytic mechanisms and enzymatic properties. In the final part, the applications and advantages of quorum quenchers (especially QQ enzymes and bacteria) are summarized in the context of agricultural/aquacultural pathogen biocontrol, membrane bioreactors for wastewater treatment, and the attenuation of human pathogenic bacteria. Taken together, we present the state-of-the-art in research considering QS and QQ, providing theoretical evidence and support for wider application of this promising environmentally friendly biocontrol strategy.
1 Introduction
Quorum sensing (QS) is an effective mechanism, by which various bacteria can regulate their gene expression accordingly and synchronize their biological behaviors based on their population density (Fuqua et al., 1994; Wang et al., 2020). In a QS system, bacteria synthesize, secrete, and sense QS signaling molecules, which are also called auto-inducers (AIs). QS was first reported in the 1970s (Nealson et al., 1970), through which the marine bacterium Vibrio fischeri can regulate its biological luminescence. Since then, studies on QS have promoted the discovery of diverse QS signals and QS-regulated traits in a broad range of bacteria and archaea, and even in eukaryotes (Grandclément et al., 2016). QS is closely related to many microbial biological behaviors, including bioluminescence, biofilm formation, antibiotic synthesis, mobility, sporulation, and gene exchange (Nealson et al., 1970; Davies et al., 1998; Bhatt et al., 2022; Mishra et al., 2022).
In terms of their molecular structure, QS signaling molecules can be briefly categorized into three groups: (1) The QS signaling molecules in Gram-negative bacteria are mostly derivatives of fatty acids, such as N-acylhomoserine lactones (AHLs) and cis-11-methyl-2-dodecenoic acid (DSF); (2) the signaling molecules in Gram-positive bacteria are mostly oligopeptides, such as autoinducing peptides (AIPs); and (3) other signaling molecules, including autoinducer-2 (AI-2), Pseudomonas quinolone signal (PQS), integrated quorum-sensing signal (IQS), 3-hydroxy-methyl palmitate (3-OH-PAME), dialkyl resorcinols (DARs), α-pyrone, p-coumaroyl-HSL (aryl-HSL) (Shi, 2021; Brameyer et al., 2015a, b; Xu and Liu, 2011), and so on.
In the past few decades, antibiotics have been widely applied in various fields to kill the plant and human pathogens, resulting in increased pathogen resistance and decreased effectiveness of such chemicals. However, contrary to the trend of rising chemical resistance, the invention of new drugs has dramatically declined over the last several decades (Bhatt et al., 2020; Zhao, 2020), highlighting the necessity of discovering and/or inventing new anti-pathogen drugs.
Quorum quenching (QQ) refers to all processes involved in the disturbance of QS (Grandclément et al., 2016; Zhang et al., 2020), which has shown great advantages over traditional anti-bacterial strategies, due to its low probability of generating bacterial resistance (Wang H et al., 2022). Quorum quenchers can be divided into two categories: QQ enzymes, which inactivate QS signals, and QS inhibitors (QSIs), which chemically disrupt QS pathways (e.g., competitive inhibition of signal receptors). For example, an AHL-degradation enzyme purified from Rhodosporidium toruloides could inactivate a broad range of AHLs, from short-chain (C4-HSL) to long-chain ones (C14-HSL) (Luo, 2010). In another study, in silico, and in vitro tests demonstrated that a natural plant compound, phytol, bound to AHL receptors of Chromobacterium violaceum with high affinity and effectively reduced QS-regulated behaviors (e.g., biofilm formation, cell aggregation, and alkaline protease activity) Vargas et al., 2021. Aside from the QSIs and QQ enzymes, quorum quenching can also occur under purely physical conditions; for example, through lactonolysis of AHL compounds—the opening of the lactone ring with the addition of H2O occurs spontaneously in aqueous solutions (Grandclément et al., 2016).
In this review, we summarize decades of studies in quorum sensing and quorum quenching, highlighting the multi-faceted applications of quorum quenchers. In the first section, the significant role of quorum sensing in regulating biological behaviors is introduced. Next, we describe the various signaling molecules employed by various micro-organisms, as well as the action mode of the QS system. Moreover, QSIs and QQ enzymes are categorized in detail, according to different derivations or QQ mechanisms. Finally, applications of quorum quenching are presented in the context of different fields, including agriculture, aquaculture, and waste treatment, emphasizing the profound advantages of QQ enzymes and QQ bacteria, when compared to traditional strategies.
2 Quorum sensing
Quorum sensing (QS) is a sophisticated molecular mechanism, by which microorganisms sense their overall population density, allowing them to trigger the expression of target genes and synchronize their behavior (Fan et al., 2017). In the study of the bioluminescence of Photobacterium fischeri in 1970, scientists observed that luciferase is synthesized massively in a relatively short burst during the period of exponential growth, while it is inactive after transferring to a freshly inoculated culture (Nealson et al., 1970). The autoinducer excreted from Photobacterium fischeri was isolated and identified as N-(3-oxohexanoyl)-3-aminodihydro-2(3H)-furanone (Eberhard et al., 1981). In 1983, Engebrechte et al. (1983) cloned and identified LuxI (autoinducer synthetase gene), LuxR (autoinducer receptor gene) and LuxCDABEG (luciferase-generated genes clusters). In the 1990s, a diffusible conjugation factor (CF) was considered as an enhancer of Ti plasmid conjugal transfer in A. tumefaciens (Zhang and Kerr, 1991), and it was later identified as a member of AHL family with a similar structure to bioluminescence autoinducer of Vibrio fischeri (Zhang et al., 1993), suggesting AHLs are part of a variety of conserved signals involved in bacterial gene regulation. Subsequently, members of AHL family were identified in the regulation of virulence gene expression in Erwinia carotovora and Pseudomonas aeruginosa (Jones et al., 1993; Pearson et al., 1994). In a review written by Fuqua et al. (1994) “quorum sensing” was first proposed as a universal mechanism regulating population behaviors in various bacterial species. The brief history of QS discovery is depicted in Figure 1.
Until now, scientists have demonstrated that various group behaviors are correlated with QS, such as swarming motility, biofilm formation, expression of secretion systems, and the production of antibiotics, exopolysaccharides, elastase, protease, hemolysin, and rhamnolipids (Salmond et al., 1995; Uroz and Oger, 2017; Bzdrenga et al., 2017; Bhatt et al., 2022). In the pathogenic cycle, biofilm formation not only serves as important means for bacteria to cope with stress but also serves as an important pathogenic factor (Song et al., 2017). In many bacterial pathogens, QS is involved in the switching between commensal or saprophytic lifestyles to pathogenic cycles (Fan et al., 2017). This is exactly the case for Pseudomonas aeruginosa, an opportunistic human pathogen that can proliferate and accumulate QS signals in wounds; as a result, the expression of virulence factors is triggered (Fan et al., 2017). In another case, PhcB in Ralstonia solanacearum (a phytopathogenic bacterium) might be a small-molecule S-adenosylmethionine-dependent methyltransferase, which catalyzes the synthesis of 3-OH-PAME from a natural fatty acid. 3-OH-PAME stimulates the expression of eps (the biosynthetic locus for extracellular polysaccharide) and was considered as part of a new family of compounds in QS systems (Flavier et al., 1997).
3 QS signaling molecules and QS system
In this section, we categorize the different QS signaling molecules employed by different micro-organisms and demonstrate the action mode of QS systems.
3.1 AHLs and LuxI/LuxR system
Acyl homoserine lactones (AHLs), also known as autoinducer-1 (AI-1) (Bzdrenga et al., 2017), are the most widely existing and intensively studied QS signaling molecules (Deng et al., 2013). The chemical structure of AHL is composed of a lactone ring linked with an acyl chain by an amide bond. AHLs differ in their acyl side-chain, which usually contains 4 to 18 carbons with oxygen or hydroxyl substitution at the third carbon (Uroz and Oger, 2017; Du, 2021). This principle also provides nomenclature; for example, N-(hexanoyl)-L-homoserine lactone will appear as C6-HSL, while N-(3-hydroxyoctanoyl)-L-homoserine lactone can be abbreviated as OHC8-HSL (Grandclément et al., 2016).
In 1994, the mechanism of the QS-regulated LuxI/LuxR system responsible for bioluminescence was demonstrated (Fuqua et al., 1994). It was found that LuxI encodes the synthase of a signaling molecule, 3-oxo-C6-HSL (OC6-HSL) which, in turn, activates the expression of the LuxI gene cluster by binding to the LuxR protein (Fuqua et al., 1994). When the cell density is low, LuxI and LuxR are expressed constitutively but at low concentrations, and AHLs passively diffuse out of cells down the concentration gradient (Fuqua et al., 1994). As the concentration of AHL increases, in proportion to cell density, its intracellular concentration reaches a threshold that allows for specifically binding to LuxR, thus triggering the mass expression of target genes (Fuqua et al., 1994; Du, 2021).
After the landmark works of Nealson et al. (1970) and Fuqua et al. (1994), further studies have demonstrated the functional mechanisms of LuxI and LuxR. Evidence has shown that LuxI catalyzes the synthesis of AHL through S-adenosylmethionine (SAM) and ACP (Parsek et al., 1999). LuxR, which contains a ligand-binding domain on the N-terminal and a DNA-binding domain on the C-terminal, functions as both an AHL receptor and transcription regulation factor. Notably, some bacteria possess LuxR receptors, yet lack any LuxI-type synthase; these are, thus referred to as LuxR orphans or solos (Brameyer et al., 2015a). This is exactly the case for Escherichia coli, Salmonella enterica, and Photorhabdus asymbiotica.
More than two hundred bacteria was reported to produce signal factor of AHL family (Zhang, 2019), among which the majority utilize LuxI for its synthesis, while the rest use LuxM, AinS, VanM, or HtdS (Luo, 2010), among others. For example, Acinetobacter baumannii uses AbaI/AbaR as a homologue to the LuxI/LuxR system, to synthesize and sense AHLs (Dong et al., 2021). Methanosaeta harundinacea 6Ac is a methanogenic archaeon encoding a FilI protein responsible for the synthesis of carboxylated AHLs. FilI, a LuxI ortholog, can also be identified in other methanogenic genomes (Zhang et al., 2012).
The AHL-mediated system mediates diverse biological behaviors in microbes, including bioluminescence (Fuqua et al., 1994; Salmond et al., 1995), Ti plasmid conjugal transfer (Zhang et al., 2002), production of cell-wall degrading enzymes (Loh et al., 2002), type VI secretion system (Khajanchi et al., 2009), biofilm formation (Khajanchi et al., 2009), and protease production (Khajanchi et al., 2009).
3.2 Autoinducer-2
Autoinducer-2 (AI-2) refers to a group of molecules that are in equilibrium with each other and their precursor, 4,5-dihydroxy-2,3-pentanedione (DPD).
Unlike many quorum-sensing signaling molecules (e.g., AHLs) produced only by a particular species or a narrow range of closely related species (Defoirdt, 2018), AI-2 is conserved in several Gram-positive and Gram-negative bacteria (Zhao, 2020) and considered to be an inter-species communication signal among bacteria (Rao, 2021). AI-2 has been detected in pathogens such as Salmonella typhimurium and V. cholerae. Interestingly, AI-2 has also been detected in bacterial cultures lacking either a QS-like response to the signal or the LuxS gene (i.e., AI-2 synthase). Some authors have, therefore, proposed that it could be a universal metabolic by-product, rather than a QS signal (Grandclément et al., 2016).
3.3 DSF and RpfC/RpfG system
Diffusible signal factor (DSF) is a type of cis unsaturated fatty acid, with the chemical structure of cis-11-methyl-2-dodecylene acid (Ye et al., 2020a; Deng et al., 2015, 2016). Barber et al. (1997) have reported a model for the DSF system in Xanthomonas campestris pv. campestris (Xcc), a novel mechanism for regulating virulence factor synthesis (Barber et al., 1997). In fact, DSF and its homologs, such as cis-2-dodecenoic acid (BDSF), cis,cis-11-methyldodeca-2,5-dienoic acid (CDSF), and cis-10-methyl-2-dodecenoic acid (IDSF), are widely conserved in a variety of Gram-negative pathogens, including Xcc and Xanthomonas oryzae pv. oryzae (Xoo) (Deng et al., 2014; Ye et al., 2020b; Rao, 2021; Song et al., 2021).
The DSF-mediated QS system is composed of DSF synthase RpfF and regulation factor RpfC/RpfG (Rao, 2021). DSF activates RpfC for autophosphorylation and transports the phosphate group to RpfG, which can activate the catalytic activity of phosphodiesterase, thus down-regulating the concentration of c-di-GMP. Eventually, the concentration of Clp (CAP-like protein) increases, directly or indirectly regulating the expression of nearly 300 genes (He et al., 2007; He and Zhang, 2008; Tao et al., 2010; Rao, 2021).
3.4 Autoinducing peptides
Autoinducing peptides (AIPs), also referred to as peptide-pheromones, are mainly used by Gram-positive bacteria, and are typically species- or strain-specific (Fan et al., 2017). There are two forms of AIPs: there are cyclic AIPs, which have been detected in Staphylococcus aureus, while Streptococcus pneumoniae and Bacillus subtilis use linear AIPs (Rao, 2021). Unlike other signaling molecules, which diffuse through the cell membrane, AIPs are transported to extracellular space by the ABC transport system (Rao, 2021). AIPs are encoded by the agrD as propeptides, and are exported by the trans-membrane protein AgrB. At high population density, AIPs are sensed by a two-component system, which consists of a transmembrane receptor AgrC and a response regulator AgrA.
3.5 Other signaling molecules
In addition to the above-mentioned QS signaling molecules, some bacteria utilize other signaling molecules to mediate their QS system (Rao, 2021). In this section, we describe several less-reported signaling molecules.
AI-3 is a rarely reported signal, which is considered to be derived from tyrosine. It can be produced by the intestinal flora and is received by the QseC receptor (Zhao, 2020). Diketopiperazines (DKPs) are found in Pseudomonas fluorescens, Pseudomonas alcaligenes, Enterobacter agglomerans, and Citrobacter freundii (Luo, 2010). 3-Hydroxypalmitate methyl ester (3-OH-PAME) is utilized by Ralstonia solanacearum as a QS signal, with PhcS-PhcR as the regulation system (Rao, 2021). Dialkylresorcinols (DARs) and cyclohexanediones (CHDs) have been identified as QS signals in Photorhabdus asymbiotica, an insect and human pathogen (Brameyer et al., 2015a). These two molecules are synthesized by Pcf in the DarABC synthesis pathway, and are sensed by the LuxR homolog PauR (Brameyer et al., 2015a).
3.6 Co-existing multiple QS systems regulated by different QS signals
Numerous Gram-negative bacteria utilize more than one QS system, and may combine these systems either in an additive model (Luo, 2010; Bar-Rogovsky et al., 2013) with distinct or partially overlapping systems (Zhang et al., 2012), or as a hierarchical model, in which one system induces a second one (Eberhard et al., 1981). On one hand, some bacteria utilize different systems to synthesize and sense one signaling molecule; for example, Erwinia carotovora use CarI/CarR and ExpI/ExpR simultaneously to synthesize and sense OHHL (OC6-HSL) (Luo, 2010). On the other hand, some bacteria integrate multiple signal inputs for communication; for example, the QS system of the P. aeruginosa complex regulatory network consists of four sub-units, LasI/LasR, RhlI/RhlR, pqs, and iqs, which each use one signal: N-oxododecanoyl-L-homoserine lactone (OdDHL), N-butanoyl-L-homoserine lactone (BHL), Pseudomonas quinolone signal (PQS), and the Integrated quorum sensing signal (IQS), respectively (Uroz and Oger, 2017). The four sub-units are organized hierarchically, with the LasI/LasR system at the top of the hierarchy (Uroz and Oger, 2017). In another case, Burkholderia plantarii possesses two QS systems: One is the AHL-mediated system, that synthesizes AHL by PlaI which is received by PlaR; the other is the DSF-mediated system, which uses RpfF as a synthase and RpfC/RpfG or RpfR as receptors (Haruna et al., 2016).
4 Quorum quenching strategy
Many pathogens regulate the expression of virulence factors through the QS system (Yu et al., 2018); therefore, the antibacterial strategy of blocking QS has attracted more and more attention as a novel strategy against bacterial diseases (Yu et al., 2018). Unlike drug resistance generated by traditional antibiotics, quorum quenchers generate resistance in mutants called QS-non-responsive phenotype (Defoirdt, 2018); however, as quorum quenchers impose no direct selective pressure on bacteria, it is considered that resistance to quorum quenchers is far less common, compared to antibiotic resistance.
Quorum quenching (QQ) refers to mechanisms that interfere with quorum sensing systems, inhibiting the expression of QS-mediated genes and the appearance of QS-regulated traits through the utilization of quorum quenchers (e.g., QQ enzymes or QSIs) (Song et al., 2017). This strategy was first described in 2000, with the identification of an AHL-inactivating enzyme, AiiA, in Bacillus sp. 240B1 (Dong, 2000). The expression of aiiA in the transformed Erwinia carotovora strain SCG1 significantly decreased extracellular pectolytic enzyme activities, and attenuated its pathogenicity by reducing the release of AI (Dong, 2000).
4.1 Mechanisms of quorum quenching
QQ mechanisms can be categorized into five main groups, as detailed below:
(i) Those which competitively inhibit the synthesis and perception of signaling molecules (Haruna et al., 2016; Rao, 2021). Chung et al. (2011) have characterized two previously unknown QS inhibitors against Burkholderia glumae in a library of acyl-HSL analogs. The first inhibitor, J8-C8, competitively binds to C8-HSL synthase TofI, occupying the binding site for the acyl chain of acyl-carrier protein; the second inhibitor, E9C3oxoC6, competitively binds TofR, the C8-HSL receptor (Chung et al., 2011); (ii) those which promote the degradation of signaling molecules (Haruna et al., 2016; Tom Defoirdt, 2011), including QQ enzymes and biocontrol QQ bacteria; (iii) those which interfere with the binding between transcription regulation factors and gene promoter sequences and down-regulate the expression of synthases and receptor genes (Haruna et al., 2016). It has been reported that mono-unsaturated fatty acids, palmitoleic acids (PoAs), and myristoleic acids (MoAs) down-regulate the expression of abaR in the QS system, thereby reducing the binding between AHLs and AbaR (Nicol et al., 2018). Above mentioned mechanisms of quorum quenching are vividly depicted in Figure 2.
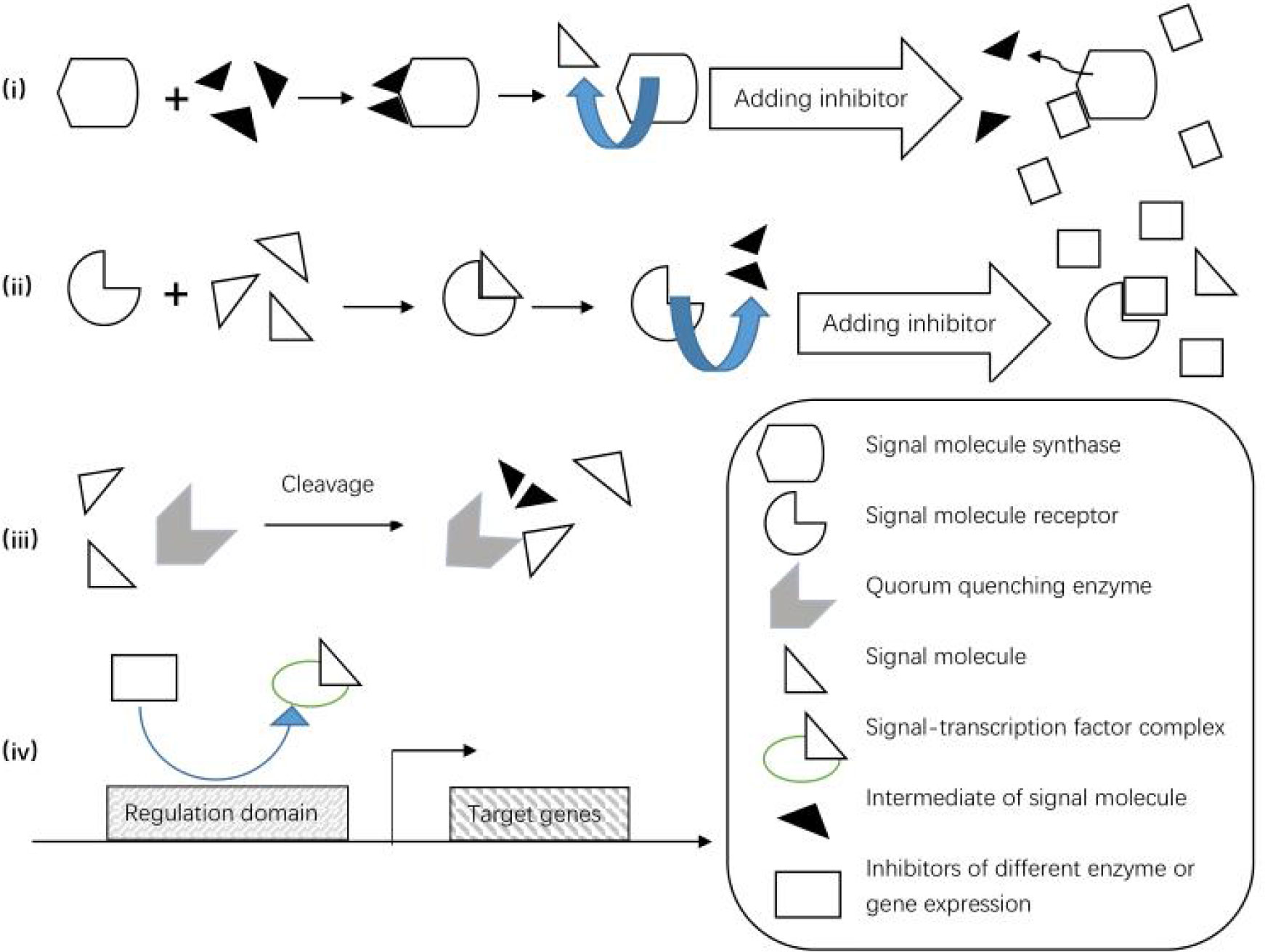
Figure 2 Mechanisms of quorum quenching, including signal production, perception and transmission through competitive inhibition or signal degradation, and inhibition of QS-related gene expression.
Taken together, different QQ strategies share the same goal: impeding signaling molecules from binding with a specific receptor to control the gene expression in microbial metabolism, such that group behaviors regulated by the signaling mechanism can be hampered (Nazzaro et al., 2019).
4.2 The discovery and design of various QSIs
4.2.1 QSIs from bacterial sources
Similarly, to the first report of QS in V. fischeri responsible for the bioluminescence, the QQ phenomenon was also first observed in marine organisms. An AHL homolog, a halogenated furanone produced by Dellsea pulchra, was found to be capable of inhibiting the swarming motility of Serratia liquefaciens, as well as the bioluminescence of V. harveyi and V. fischeri (Yu et al., 2018). Further studies have shown that halogenated furanones—one of the most common QSI families—target both AHL- and AI-2-mediated QS with distinct modes of action: the former reduces the stability or binding affinity of the LuxR regulator, while the latter inhibits the synthase, LuxS, through a covalent interaction (Bzdrenga et al., 2017). However, halogenated furanones are not the first choice for QQ strategies, due to their relatively poor stability.
4.2.2 QSIs from plant extracts
Some natural chemical components, such as ethanolic extracts of Mango Seed Kernel Extract, Guava Leaf Extract, and ϵ-Polylysine (ϵ-PL), have been found to affect Methicillin-resistant Staphylococcus aureus (MRSA), in terms of motility and expression of δ-hemolysin activity, by interfering with its QS activity (Divyakolu et al., 2021). These results indicate that QSIs could be used as an adjunct to antibiotics, to reduce the development of drug resistance (Divyakolu et al., 2021). Tannins in pomegranates and berries are capable of reducing QS-regulated physiological activity by 40% and reducing the level of signaling molecules produced by the intestinal pathogen Yersinia enterocolitica (Zhao, 2020).
4.2.3 Modern technology for QSI design
Computer-aided drug design has greatly improved the efficacy of exploring new QSIs. Among them, molecular docking is a widely-used method that can quickly and accurately simulate the docking of ligands with receptors, allowing for the determination of the molecular mechanism of substrate–enzyme interactions or efficiently obtaining target-specific compounds (e.g., those with the lowest binding affinity, binding energy, and docking score), including new types of QS inhibitors (Ewing et al., 2001; Bhatt et al., 2023). It has also been reported that the modification of natural AHLs—including introducing unsaturated bonds and altering the length of the carbon chain—is an effective and practical strategy for exploring chemical inhibitors of the LuxR family. For example, prolonging the carbon chain of C6-HSL to C10-HSL presented strong inhibition of CviR, the HSL receptor in Chromobacterium violaceum (Yan, 2018).
4.3 The family of AHL-quenching enzymes
Since the first discovery of the AHL lactonase in Bacillus cereus (Dong, 2000) and the isolation of the AHL amidase from Variovorax paradoxus (Leadbetter and Greenberg, 2000) in 2000, the number of reports on AHL-degrading enzymes has steadily increased. According to their mode of action, AHL-inactivating enzymes can be divided into three categories (Fan et al., 2017), including lactonases, amidases, and oxidoreductases, which degrade or modify AHLs in three different ways (shown in Figure 3). In the following, bacterial AHL-quenching enzymes are described in detail, along with a brief introduction to enzymes from non-bacterial sources.
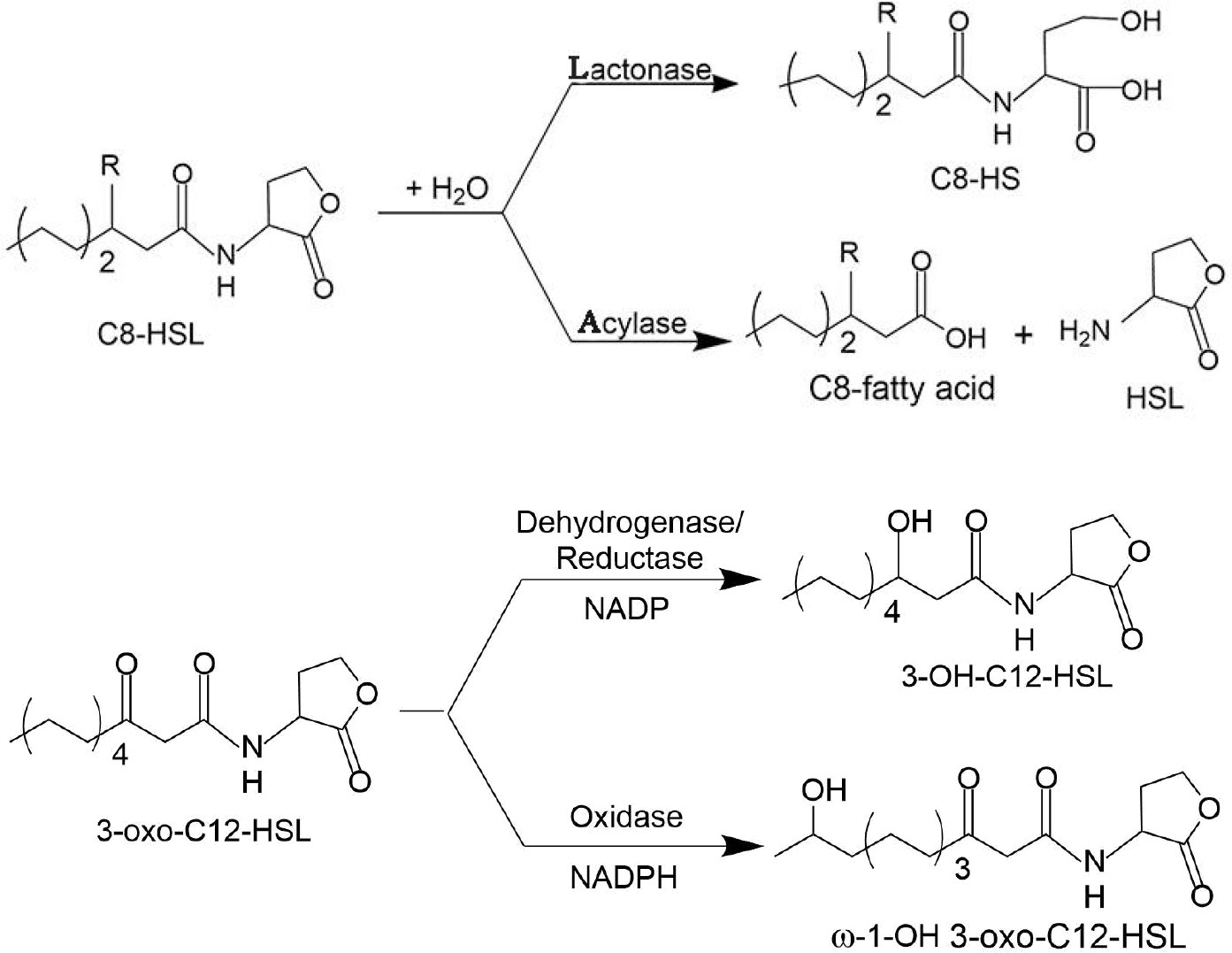
Figure 3 Mechanisms of AHL inactivation by lactonases, acylases, reductases, and oxidases: (1) AHL lactonases cleave homoserine lactone ring, generating acyl homoserine in reversible reaction; (2) AHL acylases catalyze the hydrolysis of AHL on amide bonds and generate fatty acid chains and homoserine lactone in an irreversible reaction; (3) NADP-dependent AHL reductases act on C3, reducing carbonyl groups to hydroxyl groups (Bijtenhoorn et al., 2011); (4) 3-Oxo-C12-HSL is oxidized at the ω-1, ω-2, ω-3, or even ω-4 and ω-5 carbons by NADPH-dependent oxidase (Chowdhary et al., 2007).
4.3.1 AHL lactonases
To the best of our knowledge, most of the AHL-degrading enzymes are lactonases. These can be categorized into four groups (Bzdrenga et al., 2017; Fan et al., 2017; Chang et al., 2019): Metallo-β-lactamases, paraoxonases, α/β hydrolase lactonases, and phosphotriesterase-like lactonases. Some categorizations further include amidohydrolases and glycosyl hydrolases. The phylogenetic relationships between AHL lactonases are detailed in Figure 4.
1. Metallo-β-lactamases have a highly-conserved Zn2+ binding domain HXHXDH, where Zn2+ is necessary for their catalytic activity (Rao, 2021). Among AHL lactonases, metallo-β-lactamases are the most thoroughly studied ones, including AiiA from Bacillus sp. 240B1 (Dong, 2000), and AttM and AiiB from Agrobacterium tumefaciens C58 (Rao, 2021).
2. Paraoxonases are a group of enzymes (PON1, PON2, and PON3), which are highly conserved in vertebrates and, in particular, in mammals. PON2 efficiently inactivates AHLs and exhibits arylesterase activity (Draganov Di, 2005). Purified PON1 and PON3 show lower catalytic activity toward AHLs, but act on a wide range of substrates, including organophosphates, arylesters, gamma-lactones, and delta-lactones (Draganov Di, 2005). The heterogeneous expression of human PON1 in Drosophila melanogaster reduced OC12-HSL-mediated P. aeruginosa virulence, down-regulated the superoxide anion level, and modified the gut microbiota composition (Stoltz et al., 2008).
3. α/β hydrolase lactonases share a conserved nucleophile–histidine–acid catalytic trimer domain, which consists of a nucleophile chemical structure (G-X-Nuc-X-G), one histidine, and one acidic amino acid (Asp or Glu). AidH isolated from Ochrobactrum sp. T63 (Mei et al., 2010), AiiM from Microbacterium testaceum StLB037 (Wang et al., 2010), and JydB from Rhodococcus sp. BH4 belongs to this family (Rao, 2021).
4. Phosphotriesterase-like lactonases (PLLs) are members of the amidohydrolase family (Rao, 2021), with a relatively wide range of substrates. Some PLLs are called paraoxonases, due to their catalytic activity for organophosphorus paraoxon (Rao, 2021); while some PLLs exhibit lower phosphotriesterase activity, but can proficiently hydrolyze different lactones (Afriat et al., 2006). The reported PLLs include QsdA isolated from Rhodococcus erythropolis (Uroz et al., 2008), GKL from Geobacillus kaustophilus (Chow et al., 2010), and GsP from G. stearothermophilus (Hawwa et al., 2009).
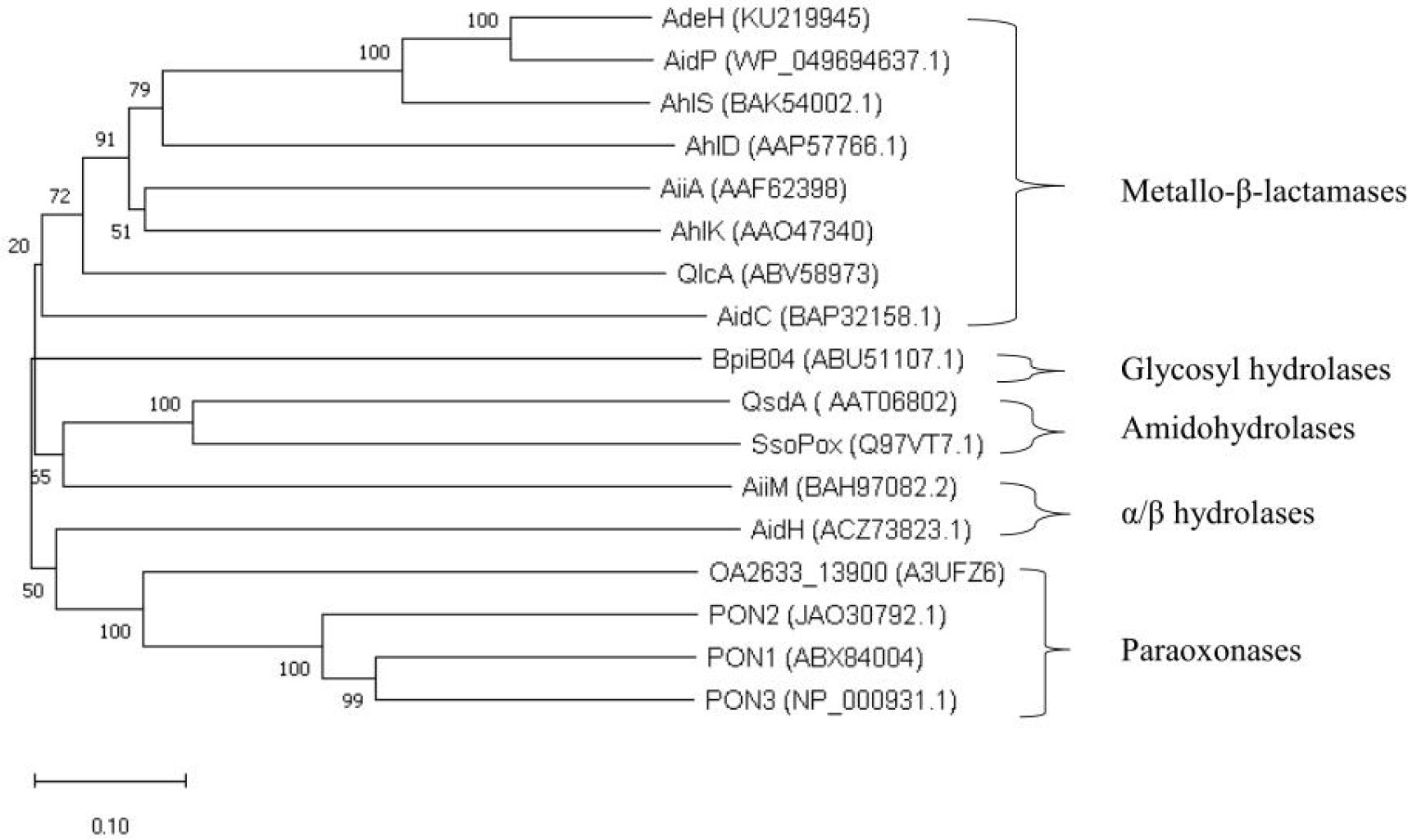
Figure 4 Phylogenetic tree of AHL lactonases. In this tree, the AHL-quenching enzymes are categorized into five families, and these quenching enzymes as follows: AidP (See-Too et al., 2017); AdeH (Garge and Nerurkar, 2016); AhlS (Morohoshi et al., 2012); AhlD (Park et al., 2003); AiiA (Dong, 2000); AhlK (Park et al., 2003); QlcA (Riaz et al., 2010); AidC (Wang et al., 2012); BpiB04 (Schipper et al., 2009); AiiM (Wang et al., 2010); AidH (Fan et al., 2020); QsdA (Hong et al., 2012); SsoPox (Park et al., 2006); OA2633_13900 (Oh et al., 2011) ; PON1, PON2, PON3 (Bar-Rogovsky et al., 2013). Using MEGA10.2.3, the 17 protein sequences were aligned by ClustalW, and the tree was constructed according to the neighbor-joining method.
4.3.2 AHL acylases
Shortly after the discovery of the first QQ lactonase AiiA, a strain of Variovorax paradoxus was isolated, which hydrolyses the AHLs into homoserine lactone and corresponding fatty acids, utilizing them as nitrogen source and energy source (Fan et al., 2017). Except that a minority of acylases belong to the α/β-hydrolase family (Fan et al., 2017), most QQ acylases can be categorized into the N-terminal nucleophile (Ntn) hydrolase superfamily (Fan et al., 2017; Liu et al., 2017; Morohoshi et al., 2017), which can be divided into three groups: The aculeacin A family, penicillin G acylase family, and amidase family (Yu et al., 2018). Some characterized AHL acylases categorized by protein families are listed in Table 1.
To date, AHL acylases have been less-reported than AHL lactonases, likely because their larger molecular weight presents more difficulties for purification. AHL acylase specializes in degrading AHLs with a long side chain, and is poor in terms of catalyzing the degradation of AHLs with a short side chain (Yu et al., 2018).
4.3.3 AHL oxidoreductases
AHL oxidoreductases, which can inactivate AHLs by oxidation or reduction, have been rarely reported. Unlike lactonases and acylases, the functional mechanism of AHL oxidoreductases varies from one to another, and may function on other molecules rather than QS signals (Yu et al., 2018). For example, CYP102A1 from Bacillus megaterium is a cytochrome P450 that can oxidize both saturated fatty acids and fatty acyl amino acids on ω-1, ω-2, and ω-3 carbons, with a preference for long fatty acid chain (Chowdhary et al., 2007). The activity of oxidized AHL dramatically declines, but remains at a low level (Fan et al., 2017). In the second case, bpiB09 isolated from a soil metagenome has been characterized as an NADP-dependent reductase, capable of inactivating 3-oxo-C12-HSL by reducing the C3 carboxyl group to a hydroxyl group. The expression of bpiB09 in P. aeruginosa down-regulates the expression of QS-regulated virulence genes and reduces the paralysis of Caenorhabditis elegans (Bijtenhoorn et al., 2011).
4.3.4 AHL-degrading enzymes in non-bacterial organisms
Other than bacteria, QQ enzymes have also been detected in other micro-organisms, and even in mammals. An AHL-degrading enzyme has been purified from Rhodosporidium toruloides, which was found to be tolerant to 2 mol/L NaCl or above, broadening the original scope of QQ enzymes in fungi (Luo, 2010). In a study of mammalian serum paraoxonases (PONs) related to bacterial homoserine lactonases (Bar-Rogovsky et al., 2013), it has been found that, similar to characterized bacterial PONX_OCCAL, mammalian ancestor PONs are capable of efficiently hydrolyzing N-acyl homoserine lactones, revealing the homology of PONs from mammals and bacteria.
4.4 Physical degradation of QS signals
In addition to digestion by QQ enzymes or inhibition by QSIs, QS signals can also be quenched under certain physical conditions. A featured case in point is the lactonolysis of AHL compounds—a phenomenon that should also affect all lactone derivatives—which can occur spontaneously in aqueous solutions. It is strongly favored at high temperatures and under alkaline pH, and can be reversed under acidic pH solutions. Under laboratory conditions, short-chain AHLs are more prone to degradation than long-chain AHLs, and the half-life of N-hexanoyl-homoserine lactone (C6-HSL) varies from over 21 days (pH 5.5, 4 °C) to less than 30 min (pH 8.5, 37 °C) (Grandclément et al., 2016).
Moreover, it has been shown that photocatalytic technology can effectively interfere with QS systems. Biofilm development was substantially delayed by TiO2 under UV irradiation, although no obvious cytotoxicity to cell growth was observed. The generated reactive oxygen species (ROS) were found to be capable of quenching the AI-2 secreted by E. coli K12 and suppressing the expression of two biofilm formation-related genes in E. coli K12 (motA and rcsB). Photogenerated ·OH and O2•− radicals are believed to non-selectively target various kinds of organic pollutants, such as AHLs, as well as membrane surface and bacterial cells (Xiao et al., 2016).
5 The application of QQ strategies
Considering the obvious disadvantages of traditional strategies against pathogens, including antibiotics, QQ enzymes have presented an attractive and profound potential for inhibiting community adherence and virulent attack of pathogens using QS systems. Considering the universality of QS-mediated pathogenic activity, QQ strategies are applicable in various fields to combat pathogens, including agriculture, aquaculture, and waste treatment. Some traditional fields in which QQ strategies have shown promise for solving troublesome issues are detailed below. Experimental and practical applications of different QS inhibitors in these fields are also discussed, as summarized in Table 2.
5.1 Virulence attenuation of agricultural pathogens
5.1.1 Necessities of applying quorum quenchers
Bacterial plant disease is one of the most important natural disasters in crops, causing serious economic losses. It not only leads to decreased crop yield but also seriously threatens the quality and safety of agricultural products (Alexander, 2010). Pesticides, traditionally used and widely applied agents to fight against plant pathogens (Morillo and Villaverde, 2017), cause serious soil and water pollution when used excessively (Carvalho, 2017; Li et al., 2022; Zhang et al., 2022). Therefore, the QS system provides a new target for the inhibition of pathogen virulence and avoiding traditional resistance, and has consequently drawn significant attention. It has been found that many bacterial pathogenic behaviors are under strict regulation of the QS system, where some typical examples are listed below.
On one hand, plant pathogens can form biofilms at the leaf, rhizosphere, and vascular bundle levels, to keep the cells aggregated for plant infection. For example, Xanthomonas campestris pv. campestris (Xcc), Pectobacterium carotovorum subsp. brasiliense, and Clavibacter michiganensis subsp. sepedonicus, form biofilms in plant vascular tissues, blocking and destroying infected tissues (Song et al., 2017).
On the other hand, plant pathogens can secret hydrolases for infection. Pectobacterium carotovorum subsp. carotovora, a pathogen that synthesizes extracellular hydrolases such as gumase, galacturonase, and pectinase, destroys plant cell walls and causes soft rot disease (Lee et al., 2013). The production of these extracellular hydrolases is strictly regulated by the ExpI/ExpR system (Andersson et al., 2000). Meanwhile, the exopolysaccharide production of Pantoea stewartii ssp. Stewartii is regulated by the EsaI/EsaR system (Koutsoudis et al., 2006).
5.1.2 The identification and characterization of biocontrol strains
To attenuate the pathogenicity of plant pathogens, many AHL or DSF-degrading strains have been isolated and characterized.
In 2004, B. thuringiensis, a traditional biological pesticide producing endotoxins lethal to moths, butterflies, and mosquitoes (Palma et al., 2014), was shown to produce a lactonase (called AiiA) which inactivates the AHL produced by Pectobacterium carotovorum, thereby reducing its pathogenicity on potato slices (Dong et al., 2004). To improve the catalytic efficacy of AiiA, Zhang et al. (2007) fused AiiA with a secretive protein to enhance its dispersion in the environment, resulting in increased tolerance to P. carotovorum in potato. Enormous efforts have been made to screen and identify QQ strains. For example, Pseudomonas nitroreducens strain W-7, a highly efficient and wide-range AHL-degrading strain, has been isolated from activated sludge samples. It was capable of degrading 0.2 mmol/L of OdDHL within 48 h, and could substantially attenuate the soft rot caused by Dickeya zeae EC1 (Zhang et al., 2021). In another case, Acinetobacter. sp 3-59, showed strong intracellular AHL-degrading activity, and the AHL-degrading gene aciJ exhibited above 90% similarity to the mono-oxygenase of Acinetobacter. sp (Zhang et al., 2018). In the research of Zheng et al. (2021), to screen out aiiA-housing bacteria against Ralstonia pseudosolanacearum from 253 biocontrol strains, degenerate primers were designed and aiiA was amplified by PCR. After 12 strains were obtained, B. cereus strain B15 was selected. Its biocontrol effect on bacterial wilt of tobacco was 68.33% after 14 days of inoculation, higher than other strains (0–60.00%) and chemical treatment (Zheng et al., 2021).
In recent years, some biocontrol strains capable of degrading DSF have been isolated, including Burkholderia anthina strain HN-8 (Ye et al., 2020a), Acinetobacter lactucae strain QL-1 (Ye et al., 2019), Cupriavidus pinatubonensis strain HN-2 (Xu et al., 2021), and Burkholderia sp. F25 (Yu et al., 2022). Burkholderia anthina HN-8 exhibited superb DSF degradation activity and completely degraded 2 mM DSF within 48 h. Further research on this strain presented the first evidence of a bacterium having a metabolic pathway for the complete degradation and metabolism of DSF, revealing that DSF could be inactivated by oxidation–reduction (Ye et al., 2020b). For strains QL-1 and HN-2, fadY encoding fatty acyl-CoA synthase and fadT encoding acyl-CoA dehydrogenase have been characterized as key DSF-degrading genes through whole-genome sequencing and comparative genomics studies (Ye et al., 2020c; Xu et al., 2021). Moreover, the expression of fadT and fadY in Xcc resulted in significantly decreased pathogenicity in host plants, such as Chinese cabbage and radish (Ye et al., 2020c; Xu et al., 2021).
5.1.3 The development of QQ enzyme-producing transgenic plants
Some plants have been genetically modified with QQ genes, such as those derived from Bacillus spp. or A. tumefaciens, which allow them to produce lactonases. The first transgenic lines, Bacillus aiiA-transformed tobacco, and potato lines, were reported in 2001 (Zhang et al., 2001). They presented an increased tolerance to P. carotovorum, with symptoms only appearing after inoculation with very high bacterial concentrations (Zhang et al., 2001). In transgenic tobacco expressing attM, a QQ gene cloned from Agrobacterium tumefaciens, wilt symptoms and mortality were dramatically decreased (Niu et al., 2012).
5.1.4 Limitations of studies considering agricultural applications
First, QQ enzyme agents protecting plants from bacterial infections are an attractive alternative to genetically modified plants but are impaired by the poor stability of the enzymes. To deal with this issue, the development of environmentally stable and chemically resistant enzymes is crucial.
Second, the impacts of QQ enzymes on beneficial or symbiotic bacteria must be further considered. The situation in the field is different from that in the laboratory, and further research is needed to balance its drawbacks against its beneficial impact.
Third, we should extend the sources of QQ agents. In rhizosphere soils, some biocontrol bacteria can release interspecies signaling molecules and interfere with the QS signaling pathway in pathogenic bacteria, causing the reduction of their pathogenic characteristics, thus shedding light on the development of new control strategies (Haruna et al., 2016).
5.2 Application of QQ strategies in aquaculture
Bacterial infections severely threaten aquaculture. Traditional methods, including probiotics, bacteriophage therapies, immunostimulants, and vaccines, fail to effectively control bacterial diseases (Bzdrenga et al., 2017). Some QQ strains have been identified and characterized for the attenuation of virulence in fish pathogens (Bzdrenga et al., 2017).
5.2.1 Fish intestinal flora and fish health
Bacterial enteritis is probably the most common intestinal disease in freshwater fish (Macpherson et al., 2012). Aeromonas hydrophila, a Gram-negative bacterium, is generally considered to be a significant pathogen causing fish enteritis and bacterial sepsis (Cascon et al., 2000). It is prevalent in many areas, and leads to high fish mortality rates, seriously restricting the development of aquaculture in China (Li, 2016). When infected by A. hydrophila, the proportion of the dominant bacteria (Pachychychia, Proteobacteria, and Bacillus) in the intestinal flora of grass carp decreases, while the proportion of Clostridium increases (Li, 2016), providing strong evidence that the pathogenicity is correlated with intestinal flora change in fish.
5.2.2 Virulence attenuation of aquacultural pathogens
QQ enzymes and QQ bacteria have been reported to attenuate the pathogenicity of A. hydrophila. It has also been acknowledged that QQ enzymes have a more rapid effect in regulating microbial flora than QQ bacteria (Zhao, 2020).
It has been confirmed that oral administration of QQ bacteria agents or purified QQ enzymes provides an effective means to attenuate A. hydrophila infection. Purified lactonase AiiAAI96 from Bacillus sp. strain AI96 has been shown to decrease infection in zebrafish, which was the first study to report that the oral administration of an AHL lactonase can effectively control A. hydrophila (Cao et al., 2012). AiiAAI96, categorized as a member of the metallo-β-lactamase superfamily, is resistant to protease and carp intestinal juice digestion, and maintains stability at 70 °C and pH 8.0 for at least 1 h (Cao et al., 2012). Zhou et al. (2016) fed Carassius auratus (goldfish) with a QQ bacteria, Bacillus sp. QSI-1, and observed an increase in Proteobacteria and decrease in Clostridium, demonstrating that QQ bacteria modify the fish gut microbiota. At the same time, the percentage of A. hydrophila decreased significantly, which strongly suggests the potential of QQ probiotics to control aquacultural bacterial diseases. According to a recent study, after AiiO-AIO6 was added to zebrafish feed, the PCR analysis showed that the transcription of many nutrient transporters and growth-related genes (e.g., the peptide transporter Pept1a, glucose transporter GLUT2, and growth hormone receptor GHra) were all significantly up-regulated, when compared with the control (Das et al., 2022).
Moreover, a report has shown that the injection of AiiA from B. licheniformis DAHB1 into the abdominal cavity successfully reduced the colonization of V. parahaemolyticus in Indian white shrimp, as well as the infection and mortality rates in shrimp (Vinoj et al., 2014). AiiA produced by B. licheniformis inactivated a wide range of AHL substrates and could tolerate the acidic environment in the shrimp intestine (Romero, 2012). Another report has shown that co-injection of purified YtnP and A. hydrophila altered the richness of intestinal flora and decreased mortality by more than 50% at 96 h, through attenuating the pathogenicity of A. hydrophila (Peng et al., 2021).
Taken together, these results provide valuable insights into the QQ enzymes and bacteria that modulate the microbiota structure, thus attenuating the virulence of pathogens in fish and shrimp, as well as even boosting their growth by regulating gene expression.
5.3 Biofouling mitigation of membrane bioreactors
Membrane bioreactors (MBRs) and anaerobic membrane bioreactors (AnMBRs), which combine a classic bioreactor system with a membrane filtration step, have been widely used in wastewater treatment for the bacterial cleaning of soluble pollutants through the retention of micro-organisms and solid particles (Drews, 2010; Anjum et al., 2021). As a major concern encountered in such systems (Ni and Wang, 2019), membrane fouling (particularly, biofouling) is mainly due to the attachment and proliferation of microbes on the membrane surface, resulting in biofilm formation (Ni et al., 2021). Membrane fouling causes lower membrane filterability, increases energy consumption, and calls for the frequent cleaning or replacement of membrane modules (Syafiuddin et al., 2021). In biofouling control of MBRs or AnMBRs systems, transmembrane pressure (TMP) is one of the indicators that can be used to evaluate membrane permeability (Anjum et al., 2021; Syafiuddin et al., 2021). The increase in TMP at different profiles could be due to pore blockage and EPS accumulation on the surface of the membrane (Syafiuddin et al., 2021). When the TMP increases, conventional cleaning strategies fail to perform well: physical cleaning presents an incapability to remove all fouling layers, while chemical cleaning potentially induces chemical resistance of the micro-organisms, thus reducing the biofouling control efficiency (Syafiuddin et al., 2021).
Therefore, current strategies focus on using biological approaches which aim to avoid the drawbacks associated with physical and chemical approaches (Shah and Choo, 2020). Since Yeon et al. demonstrated the relationship between QS signaling and membrane fouling (Yeon et al., 2009a), many scholars have attempted to apply QQ strategies to membrane filtration, and have investigated the potential of various QQ agents, such as QQ compounds, QQ enzymes, QQ bacteria, and so on (Ni and Wang, 2019). It is believed that QQ strategies have the potential to not only handle MBR biofilm formation but also exert no adverse effects on the capability of MBRs, in terms of organic and nutrient removal (Xiao et al., 2018), thus benefiting the long-term stability of MBRs and their application at wider scales.
5.3.1 QQ enzymes and QQ bacteria for biofouling control
It has been reported that immobilizing QQ enzymes provides an effective strategy for improving the stability and prolonging the lifespan of the enzymes. Kim et al. (2011) have found that an acylase-immobilized QQ membrane prohibited the formation of a mature biofilm, due to the reduced secretion of extracellular polymeric substances. This membrane maintained more than 90% of its initial enzyme activity for more than 20 iterative cycles of the reaction and washing procedure, as well as more than 90% of its initial flux after 38 h of operation. Yeon et al. (2009b) have reported a magnetic enzyme carrier (MEC) prepared by immobilizing acylase on magnetic particles. This MEC not only efficiently alleviates membrane biofouling but also presents great advantages over free enzymes in terms of stability, with no activity decrease under both continuous shaking for 14 days and 29 iterative cycles of reuse.
The isolation and application of QQ strains have also been frequently reported in the field of biofouling control. Noori et al. (2022) have isolated two QQ strains of Bacillus spp. from the activated sludge used to treat industrial wastewater containing two toxic pollutants: tetramethylammonium hydroxide (TMAH) and 1-methyl-2-pyrrolidinone. Co-culturing Bacillus spp. with P. aeruginosa PAO1 or activated sludge significantly reduced the biofilm formation of PAO1 and mixed communities in activated sludge.
Various carriers have been applied to load the QQ bacteria and improve the degradation rate of signaling molecules. Lee et al. (2020) have developed a mesoporous silica medium entrapping Rhodococcus sp. BH4, a dominant QQ bacteria strain employed to control MBR biofouling. The degradation rate of C8-HSL using the live BH4-entrapped medium was 80% higher than that of the control group (silica medium containing dead BH4). It is believed that the live BH4 medium can remove AHLs through both adsorption (mesoporous hydrophobic structure) and enzymatic decomposition (QQ) (Syafiuddin et al., 2021). Another carrier, QQ bacterial entrapping beads (QQ-beads) have also been commonly used as carriers of QQ strains for (An)MBRs biofouling control. QQ-beads are often prepared as mixtures of re-suspended QQ bacteria and polymer solutions (e.g., sodium alginate and polyvinyl alcohol). The beads can be further used directly or coated with a polymer before application in biofouling control. It has been suggested that a polymeric coating can improve the effective lifetime of beads, but likely slows the release of QQ enzymes into the MBR system (Syafiuddin et al., 2021). The addition of QQ-beads into a conventional MBR substantially affected the EPS concentrations, as well as microbial flora size in the mixed liquor, thus reducing TMP build-up (Lee et al., 2016). Other than mesoporous silica and beads, other forms of QQ agents have also been applied in MBR biofouling control. Shu et al. (2022) investigated the direct injection of different doses of unentrapped QQ strains (Rhodococcus sp. BH4) into a probiotic QQ MBR, which demonstrated effective biofouling mitigation in diverse MBR phases.
Other improvements can be made to maintain the good performance of MBRs: Khan et al. (2019) have reported the synergetic effects of QQ bacteria (Rhodococcus sp. BH4) and the electric field in a submerged membrane electro-bioreactor (SMEBR), and showed significant improvements, compared to conventional submerged MBR, in terms of delaying TMP increase, while maintaining higher removal of COD, ammonium-N (NH4+-N), and phosphorus-P (PO43-).
In long-term continuous MBR operations, TMP is seldom used as an indicator, except in the study of Dong et al. (2022). In this study, a QQ system exploiting two QQ bacteria (Serratia sp. Z4 and Klebsiella sp. Q2) and γ-caprolactone (GCL) was shown to delay the TMP increase by half during 40 days of operation in a continuous MBR with low effluent chemical oxygen demand and ammonium nitrogen (Dong et al., 2022). This highlighted the effectiveness of QQ bacteria in mitigating biofouling through the stimulation of GCL in the long-term operation of MBR.
5.3.2 Other QQ strategies in biofouling control
Multiple natural compounds can play the role of quorum quenchers in mitigating biofouling. Kappachery et al. (2010) have applied vanillin in bioreactors, and found that it can inhibit biofilm growth on the reverse osmosis membrane, with an inhibition rate of 97%. Xu and Liu (2011) observed that D-tyrosine dramatically down-regulated the concentration of AI-2 and extracellular polysaccharides, thus reducing microbial attachment to glass and polypropylene surfaces. A polyphenolic extract from Rosa rugose (Zhang et al., 2014), piper beetle extract (Siddiqui et al., 2012), and carvacrol (Gutierrez-Pacheco et al., 2018) have also been found to interfere with the bacterial QS system and attenuate biofilm formation on the membrane surface.
Physical degradation is also viewed as an effective strategy for quenching signaling molecules. In a photocatalytic membrane reactor system, the reactive oxygen species (ROS) generated by UV-excited TiO2 could oxidize the membrane surface foulants and quench AHLs. Additionally, this in-situ membrane cleaning strategy enhanced chemical oxygen demand (COD) removal, and almost complete disinfection of the effluent was realized in the photocatalytic QQ system. Treatment consisting of 45 min of UV irradiation (15 W) on QQ beads led to 95% degradation of AHL. This photocatalytic strategy can effectively mitigate the TMP from 30 kDa to 5 kDa in 4 h (Mehmood et al., 2021), showing its great potential for in-situ membrane cleaning.
5.3.3 Limitations of QQ strategies in antifouling applications
First of all, the cost of practical applications is the basic factor limiting the application of QQ strategies. For example, extracting plant-sourced QQ compounds and the following purification requires sophisticated procedures, which are considered to be economically unfriendly, restricting its application (Ni and Wang, 2019).
Second, considering the sophistication of micro-organism communities and the diversity of QS signals, we can hardly rely on a single quencher to effectively mitigate biofilm formation and accumulation (Ni and Wang, 2019); for example, the QQ consortium developed by Xiao et al. (2018) provided a selective pressure on the biocake not only by preventing Gram-negative bacteria but also enriching Gram-positive bacteria, due to its capability to degrade AHLs but not AIPs. The sophisticated mechanisms of QS still need to be uncovered, and the combined effect of multiple quenchers deserves further investigation.
Third, how to precisely limit the QQ effect on the membrane surface should be paid more attention to. As far as MBR is concerned, most QQ strategies have a durative effect, and the long-term inhibition of biofilm formation may harm normal bacterial growth, thus hampering the water purification efficacy.
5.4 Attenuating virulence of human pathogenic bacteria
Many studies have been conducted to verify the effect of diverse QQ agents on P. aeruginosa, a clinically noted human pathogen that regulates virulence via a complex QS system involving natural or synthetic compounds, QQ strains or enzymes, and even antibody targeting signal molecules (Defoirdt, 2018). Bernabè et al. (2022) identified GM-50 as the most active compound in a library of small phenolic derivatives. It prevents adhesion of PAO1 and inflammatory damage in the human A549 cell line and significantly reduces virulence factors in twenty P. aeruginosa clinical isolates from patients with respiratory tract infections.
Inhibition of the QS system is reported to attenuate bacterial antibiotic resistance and, as a consequence, its significantly reduced usage dose. Ivanova et al. (2022) clinically applied the antibiotic gentamicin with an acylase from Aspergillus melleus using an one-step ultrasound emulsification process and found that the generated hybrid gentamicin/acylase nanospheres showed 16-fold improved bactericidal activity toward P. aeruginosa compared with pure gentamicin. The nanohybrids also attenuated 97 ± 1.8% of the production of violacien (a virulence factor) in Chromobacterium violaceum.
In terms of the application of QQ agents in clinical treatment, the stability of QQ agents and their capacity to reach their targets are currently the major obstacles encountered in their use. New approaches of nanocarrier delivery are proven to be an effective tool, and this is supported by the effective delivery of niclosamide nanoparticles to inhibit P. aeruginosa QS at concentrations of 2.5−10 μM in aerosol form, providing a tool for the local treatment of P. aeruginosa lung infections as in the case of CF patients (Costabile et al., 2015). Another case in point is reported by Lu et al. (2015), in which QS signal-contained CAI-1 nanoparticles can diffuse across delivery barriers, such as mice intestinal mucus, and regulate V. cholerae QS responses much more effectively than free CAI-1.
When considering the clinical application of QQ agents, their influence on the host microbiota must be taken into account. Although QQ agents have long been considered to have fewer side effects toward nontarget organisms than conventional antibiotics, they still require further investigation on this aspect, especially for the broad spectrum QQ agents. QQ agents can interfere with the colonization of beneficial bacteria, diminishing their positive effects on the host.
6 Conclusions and future perspectives
Based on the quorum sensing mechanism, quorum quenching has been promoted as an efficient biological control strategy, with a promising future in agricultural, aquacultural, and waste management applications. Compared to traditional antibiotics, quorum quenchers generate much less selective pressure—and, thus, less resistance—serving as a potential substitute (or, at least, supplements) to traditional antibiotics. Among the various quorum quenchers, QQ enzymes exhibit the best efficacy and lowest cytotoxicity.
Regardless of their great advantages, several problems remain unsolved for their practical applications. First of all, it has been recognized that QQ enzymes exhibit better QQ efficacy; however, they present lower stability in catalytic activity, when compared to QQ bacteria. It is, therefore, of great significance that the stability of QQ enzymes is improved, to accommodate for application circumstances. Efforts have been dedicated to the isolation of robust enzymes from extreme environments or the immobilization of QQ bacteria and QQ enzymes. Moreover, the targeting and delivery of QQ enzymes or QQ molecules, as well as evaluation of their cytotoxicity or other side-effects at the population, organism, cellular, and sub-cellular levels, are worth consideration. Furthermore, other than disrupting AHL- or DSF-based QS mechanisms, the quest for enzymes targeting AI-2, AI-3, or even AIPs, is essential for uncovering the potential of inhibiting a wider panel of Gram-negative and Gram-positive pathogenic bacteria.
Author contributions
SC, L-HZ, KB and JW conceived of the presented idea. XZ contributed to the writing and prepared the figures and tables. W-JC, KB, ZZ, YH, SC and JW participated in revising the manuscript. All authors contributed to the article and approved the submitted version.
Funding
This work was funded by the Key-Area Research and Development Program of Guangdong Province, China (2020B0202090001), the Natural Science Foundation of Guangdong Province, China (2022A1515010869) and the Science and Technology Planning Project of Guangdong Province, China (2017A020208053).
Conflict of interest
The authors declare that the research was conducted in the absence of any commercial or financial relationships that could be construed as a potential conflict of interest.
Publisher’s note
All claims expressed in this article are solely those of the authors and do not necessarily represent those of their affiliated organizations, or those of the publisher, the editors and the reviewers. Any product that may be evaluated in this article, or claim that may be made by its manufacturer, is not guaranteed or endorsed by the publisher.
References
Afriat, L., Roodveldt, C., Manco, G., Tawfik, D. S. (2006). The latent promiscuity of newly identified microbial lactonases is linked to a recently diverged phosphotriesterase. Biochemistry 45, 13677–13686. doi: 10.1021/bi061268r
Alexander, H. M. (2010). Disease in natural plant populations, communities, and ecosystems: Insights into ecological and evolutionary processes. Plant Dis. 94, 492–503. doi: 10.1094/PDIS-94-5-0492
Andersson, R. A., Eriksson, A. R., Heikinheimo, R., Mae, A., Pirhonen, M., Koiv, V., et al. (2000). Quorum sensing in the plant pathogen erwinia carotovora subsp. carotovora: The role of expREcc. Mol. Plant Microbe Interaction 13, 384–393. doi: 10.1094/MPMI.2000.13.4.384
Anjum, F., Khan, I. M., Kim, J., Aslam, M., Blandin, G., Heran, M., et al. (2021). Trends and progress in AnMBR for domestic wastewater treatment and their impacts on process efficiency and membrane fouling. Environ. Technol. Innovation 21, 101204. doi: 10.1016/j.eti.2020.101204
Barber, C. E., Tang, J. L., Feng, J. X., Pan, M. Q., Wilson, T. J., Slater, H., et al. (1997). A novel regulatory system required for pathogenicity of Xanthomonas campestris is mediated by a small diffusible signal molecule. Mol. Microbiol. 24, 555–566. doi: 10.1046/j.1365-2958.1997.3721736.x
Bar-Rogovsky, H., Hugenmatter, A., Tawfik, D. S. (2013). The evolutionary origins of detoxifying enzymes. J. Biol. Chem. 288, 23914–23927. doi: 10.1074/jbc.M112.427922
Bernabè, G., Marzaro, G., Di Pietra, G. (2022). A novel phenolic derivative inhibits AHL-dependent quorum sensing signaling in Pseudomonas aeruginosa. Front. Pharmacol. 13. doi: 10.3389/fphar.2022.996871
Bhatt, P., Bhatt, K., Chen, W. J., Huang, Y., Xiao, Y., Wu, S., et al. (2023). Bioremediation potential of laccase for catalysis of glyphosate, isoproturon, lignin, and parathion: Molecular docking, dynamics, and simulation. J. Hazard. Mater. 443, 130319. doi: 10.1016/j.jhazmat.2022.130319
Bhatt, P., Bhatt, K., Huang, Y., Li, J., Wu, S., Chen, S. (2022). Biofilm formation in xenobiotic-degrading microorganisms. Crit. Rev. Biotechnol. 28, 1–21. doi: 10.1080/07388551.2022.2106417
Bhatt, P., Bhatt, K., Sharma, A., Zhang, W., Mishra, S., Chen, S. (2021). Biotechnological basis of microbial consortia for the removal of pesticides from the environment. Crit. Rev. Biotechnol. 41, 317–338. doi: 10.1080/07388551
Bhatt, P., Verma, A., Verma, S., Anwar, M. S., Prasher, P., Mudila, H., et al. (2020). Understanding phytomicrobiome: a potential reservoir for better crop management. Sustainability 12, 5446. doi: 10.3390/su12135446
Bijtenhoorn, P., Mayerhofer, H., Müller-Dieckmann, J., Utpatel, C., Schipper, C., Hornung, C., et al. (2011). A novel metagenomic short-chain dehydrogenase/reductase attenuates Pseudomonas aeruginosa biofilm formation and virulence on caenorhabditis elegans. PloS One 6, e26278. doi: 10.1371/journal.pone.0026278
Brameyer, S., Bode, H. B., Heermann, R. (2015a). Languages and dialects: Bacterial communication beyond homoserine lactones. Trends Microbiol. 23, 521–523. doi: 10.1016/j.tim.2015.07.002
Brameyer, S., Kresovic, D., Bode, H. B., Heermann, R. (2015b). Dialkylresorcinols as bacterial signaling molecules. Proc. Natl. Acad. Sci. 112, 572–577. doi: 10.1073/pnas.1417685112
Bzdrenga, J., Daudé, D., Rémy, B., Jacquet, P., Plener, L., Elias, M., et al. (2017). Biotechnological applications of quorum quenching enzymes. Chemico-Biological Interact. 267, 104–115. doi: 10.1016/j.cbi.2016.05.028
Cao, Y., He, S., Zhou, Z. G., Zhang, M. C., Mao, W., Zhang, H. T., et al. (2012). Orally administered thermostable n-acyl homoserine lactonase from Bacillus sp. strain AI96 attenuates Aeromonas hydrophila infection in zebrafish. Appl. Environ. Microbiol. 78, 1899–1908. doi: 10.1128/aem.06139-11
Carvalho, F. P. (2017). Pesticides, environment, and food safety. Food Energy Secur. 6, 48–60. doi: 10.1002/fes3.108
Cascon, A., Yugueros, J., Temprano, A., Sanchez, M., Hernanz, C., Luengo, J. M., et al. (2000). A major secreted elastase is essential for pathogenicity of Aeromonas hydrophila. Infection Immun. 68, 3233–3241. doi: 10.1128/IAI.68.6.3233-3241.2000
Chang, J., Shi, G., Zeng, M., Liu, Z. (2019). Quorum quenching enzymes-a review. Chin. J. Bioprocess Eng. 17, 244–250.
Chen, C., Chen, C., Liao, C., Lee, C. (2009). A probable aculeacin a acylase from the Ralstonia solanacearum GMI1000 is n-acyl-homoserine lactone acylase with quorum-quenching activity. BMC Microbiol. 9, 89. doi: 10.1186/1471-2180-9-89
Chowdhary, P. K., Keshavan, N., Nguyen, H. Q., Peterson, J. A., González, J. E., Haines, D. C. (2007). Bacillus megaterium CYP102A1 oxidation of acyl homoserine lactones and acyl homoserines. Biochemistry 46, 14429–14437. doi: 10.1021/bi701945j
Chow, J. Y., Xue, B., Lee, K. H., Tung, A., Wu, L., Robinson, R. C., et al. (2010). Directed evolution of a thermostable quorum-quenching lactonase from the amidohydrolase superfamily. J. Biol. Chem. 285, 40911–40920. doi: 10.1074/jbc.M110.177139
Chung, J., Goo, E., Yu, S., Choi, O., Lee, J., Kim, J., et al. (2011). Small-molecule inhibitor binding to an N-acyl-homoserine lactone synthase. Proc. Natl. Acad. Sci. United States America 108, 12089–12094. doi: 10.1073/pnas.1103165108
Costabile, G., d'Angelo, I., Rampioni, G., Bondì, R., Pompili, B., Ascenzioni, F., et al. (2015). Toward repositioning niclosamide for antivirulence therapy of Pseudomonas aeruginosa lung infections: development of inhalable formulations through nanosuspension technology. Mol. Pharmaceutics 12, 2604–2617. doi: 10.1021/acs.molpharmaceut.5b00098
Czajkowski, R., Krzyżanowska, D., Karczewska, J., Atkinson, S., Przysowa, J., Lojkowska, E., et al. (2011). Inactivation of AHLs by Ochrobactrum sp. A44 depends on the activity of a novel class of AHL acylase. Environ. Microbiol. Rep. 3, 59–68. doi: 10.1111/j.1758-2229.2010.00188.x
Das, S., Das, S., Ghangrekar, M. M. (2022). Bacterial signaling mechanism: An innovative microbial intervention with multifaceted applications in microbial electrochemical technologies: A review. Bioresource Technol. 344, 126218. doi: 10.1016/j.biortech.2021.126218
Davies, D. G., Parsek, M. R., Pearson, J. P., Iglewski, B. H., Costerton, J. W., Greenberg, E. P. (1998). The involvement of cell-to-cell signals in the development of a bacterial biofilm. Science 280, 295–298. doi: 10.1126/science.280.5361.295
Defoirdt, T. (2018). Quorum-sensing systems as targets for antivirulence therapy. Trends Microbiol. 26, 313–328. doi: 10.1016/j.tim.2017.10.005
Deng, Y., Boon, C., Chen, S., Lim, A., Zhang, L. H. (2013). Cis-2-dodecenoic acid signal modulates virulence of Pseudomonas aeruginosa through interference with quorum sensing systems and T3SS. BMC Microbiol. 13, 231. doi: 10.1186/1471-2180-13-231
Deng, Y., Lim, A., Wang, J., Zhou, T., Chen, S., Lee, J., et al. (2013). Cis-2-dodecenoic acid quorum sensing system modulates N-acyl homoserine lactone production through RpfR and cyclic di-GMP turnover in Burkholderia cenocepacia. BMC Microbiol. 13, 148. doi: 10.1186/1471-2180-13-148
Deng, Y., Lim, A., Lee, J., Chen, S., An, S., Dong, Y. H., et al. (2014). Diffusible signal factor (DSF) quorum sensing signal and structurally related molecules enhance the antimicrobial efficacy of antibiotics against some bacterial pathogens. BMC Microbiol. 14, 51. doi: 10.1186/1471-2180-14-51
Deng, Y., Liu, X., Wu, J. E, Lee, J., Chen, S., Cheng, Y., et al. (2015). The host-plant metabolite glucose is the precursor of diffusible signal factor (DSF) family signals in Xanthomonas campestris. Appl. Environ. Microbiol. 81, 2861–2868. doi: 10.1128/AEM.03813-14
Deng, Y., Wu, J. E., Yin, W. F., Li, P., Zhou, J., Chen, S., et al. (2016). Diffusible signal factor family signals provide a fitness advantage to Xanthomonas campestris pv. campestris in interspecies competition. Environ. Microbiol. 18, 1534–1545. doi: 10.1111/1462-2920.13244
Divyakolu, S., Chikkala, R., Kamaraju, S., Sritharan, V. (2021). Quorum quenching as a strategy for treating methicillin resistant s. aureus (MRSA) - effect of ϵ-polylysine, ethanolic extracts of guava leaves and mango seed kernel. Indian J. Biochem. Biophysics 58, 171–177.
Dong, Y. H. (2000). AiiA, an enzyme that inactivates the acylhomoserine lactone quorum-sensing signal and attenuates the virulence of erwinia carotovora. Proc. Natl. Acad. Sci. 97, 3526–3531. doi: 10.1073/pnas.060023897
Dong, H., Jin, Y., Cheng, G., Feng, X., Li, G. (2021). Research advances of quorum quenching in inhibiting biofilm formation and drug resistance of acinetobacter baumannii. Chin. J. New Drugs Clin. Remedies 40, 801–805. doi: 10.14109/j.cnki.xyylc.2021.12.01
Dong, X., Qin, J., Zhang, Y., Chen, X., Ge, S. (2022). Mitigation of membrane biofouling using quorum-quenching bacteria in a continuously operated membrane bioreactor. Int. Biodeterioration Biodegradation 166, 105339. doi: 10.1016/j.ibiod.2021.105339
Dong, Y., Zhang, X., Xu, J., Zhang, L. (2004). Insecticidal Bacillus thuringiensis silences erwinia carotovora virulence by a new form of microbial antagonism, signal interference. Appl. Environ. Microbiol. 70, 954–960. doi: 10.1128/AEM.70.2.954-960.2004
Draganov Di, T. J. S. A. (2005). Human paraoxonases (PON1, PON2, and PON3) are lactonases with overlapping and distinct substrate specificities. J. Lipid Res. 46, 1239–1247. doi: 10.1194/jlr.M400511-JLR200
Drews, A. (2010). Membrane fouling in membrane bioreactors–characterisation, contradictions, cause and cures. J. Membrane Sci. 363, 1–28. doi: 10.1016/j.memsci.2010.06.046
Du, H. (2021). Study on quorum quenching effect of pediococcus pentosaceus YF-8 on aeromonas sobria (Jinzhou, China: Bohai University). doi: 10.27190/d.cnki.gjzsc.2021.000149
Eberhard, A., Burlingame, A. L., Eberhard, C., Kenyon, G. L., Nealson, K. H., Oppenheimer, N. J. (1981). Structural identification of autoinducer of photobacterium fischeri luciferase. Biochemistry 20, 2444–2449. doi: 10.1021/bi00512a013
Engebrechte, J., Nealson, K., Silerman, M. (1983). Bacterial bioluminescence: isolation and genetic analysis of functions from vibrio fisheri. Cell 32, 773–781. doi: 10.1016/0092-8674(83)90063-6
Ewing, T. J. A., Makino, S., Skillman, A. G., Kuntz, I. D. (2001). DOCK 4.0: Search strategies for automated molecular docking of flexible molecule databases. J. Computer-Aided Mol. Design 15, 411–428. doi: 10.1023/A:1011115820450
Fan, X., Wang, H., He, J., Ye, T., Yang, F., Chen, S. (2017). Research progress on microbial quorum quenching enzymes and their control of plant diseases. Biotechnol. Bull. 33, 80–87. doi: 10.13560/j.cnki.biotech.bull.1985.2017-0223
Fan, X., Ye, T., Li, Q., Bhatt, P., Zhang, L., Chen, S. (2020). Potential of a quorum quenching bacteria isolate Ochrobactrum intermedium D-2 against soft rot pathogen Pectobacterium carotovorum subsp. carotovorum. Front. Microbiol. 11. doi: 10.3389/fmicb.2020.00898
Flavier, A. B., Clough, S. J., Schell, M. A., Denny, T. P. (1997). Identification of 3-hydroxypalmitic acid methyl ester as a novel autoregulator controlling virulence in Ralstonia solanacearum. Mol. Microbiol. 26, 251–259. doi: 10.1046/j.1365-2958.1997.5661945.x
Fuqua, W. C., Winans, S. C., Greenberg, E. P. (1994). Quorum sensing in bacteria: The LuxR-LuxI family of cell density-responsive transcriptional regulators. J. Bacteriology 176, 269–275. doi: 10.1128/jb.176.2.269-275.1994
Garge, S. S., Nerurkar, A. S. (2016). Attenuation of quorum sensing regulated virulence of Pectobacterium carotovorum subsp. carotovorum through an AHL lactonase produced by Lysinibacillus sp. Gs50. . PloS One 11, 1–23. doi: 10.1371/journal.pone.0167344
Grandclément, C., Tannières, M., Moréra, S., Dessaux, Y., Faure, D. (2016). Quorum quenching: Role in nature and applied developments. FEMS Microbiol. Rev. 40, 86–116. doi: 10.1093/femsre/fuv038
Gutierrez-Pacheco, M. M., Gonzalez-Aguilar, G. A., Martinez-Tellez, M. A., Lizardi-Mendoza, J., Madera-Santana, T. J., Bernal-Mercado, A. T., et al. (2018). Carvacrol inhibits biofilm formation and production of extracellular polymeric substances of pectobacterium carotovorum subsp. carotovorum. Food Control 89, 210–218. doi: 10.1016/j.foodcont.2018.02.007
Haruna, M., Wang, M., Gui, W., Guo, Y., Zhu, G. (2016). Research progress in quorum sensing and quorum quenching of the rice pathogen burkholderia plantarii. Chin. J. Pesticide Sci. 18, 273–281. doi: 10.16801/j.issn.1008-7303.2016.0037
Hawwa, R., Aikens, J., Turner, R. J., Santarsiero, B. D., Mesecar, A. D. (2009). Structural basis for thermostability revealed through the identification and characterization of a highly thermostable phosphotriesterase-like lactonase from geobacillus stearothermophilus. Arch. Biochem. Biophysics 488, 109–120. doi: 10.1016/j.abb.2009.06.005
He, Y., Ng, A. Y., Xu, M., Lin, K., Wang, L., Dong, Y., et al. (2007). Xanthomonas campestris cell-cell communication involves a putative nucleotide receptor protein clp and a hierarchical signalling network. Mol. Microbiol. 64, 281–292. doi: 10.1111/j.1365-2958.2007.05670.x
He, Y. W., Zhang, L. H. (2008). Quorum sensing and virulence regulation in Xanthomonas campestris. FEMS Microbiol. Rev. 32, 842–857. doi: 10.1111/j.1574-6976.2008.00120.x
Hong, K., Koh, C., Sam, C., Yin, W., Chan, K. (2012). Quorum quenching revisited–from signal decays to signalling confusion. Sensors 12, 4661–4696. doi: 10.3390/s120404661
Huang, J. J., Han, J. I., Zhang, L. H., Leadbetter, J. R. (2003). Utilization of acyl-homoserine lactone quorum signals for growth by a soil pseudomonad and Pseudomonas aeruginosa PAO1. Appl. Environ. Microbiol. 69, 5941–5949. doi: 10.1128/AEM.69.10.5941-5949.2003
Huang, J. J., Petersen, A., Whiteley, M., Leadbetter, J. R. (2006). Identification of QuiP, the product of gene PA1032, as the second acyl-homoserine lactone acylase of Pseudomonas aeruginosa PAO1. Appl. Environ. Microbiol. 72, 1190–1197. doi: 10.1128/AEM.72.2.1190-1197.2006
Ivanova, K., Ivanova, A., Hoyo, J., Pérez-Rafael S Tzanov, T. (2022). Nano-formulation endows quorum quenching enzyme-antibiotic hybrids with improved antibacterial and antibiofilm activities against Pseudomonas aeruginosa. Int. J. Mol. Sci. 23, 7632. doi: 10.3390/ijms23147632
Jones, S., Yu, B., Bainton, N. J., Birdsall, M., Bycroft, B. W., Chhabra, S. R., et al. (1993). The lux autoinducer regulates the production of exoenzyme virulence determinants in erwinia carotovora and Pseudomonas aeruginosa. EMBO J. 12, 2477–2482. doi: 10.1002/j.1460-2075.1993.tb05902.x
Kappachery, S., Paul, D., Yoon, J., Kweon, J. H. (2010). Vanillin, a potential agent to prevent biofouling of reverse osmosis membrane. Biofouling 26, 667–672. doi: 10.1080/08927014.2010.506573
Khajanchi, B. K., Sha, J., Kozlova, E. V., Erova, T. E., Suarez, G., Sierra, J. C., et al. (2009). N-acyl homoserine lactones involved in quorum sensing control the type VI secretion system, biofilm formation, protease production, and in vivo virulence in a clinical isolate of Aeromonas hydrophila. Microbiol. (Society Gen. Microbiology) 155, 3518–3531. doi: 10.1099/mic.0.031575-0
Khalid, S. J., Ain, Q., Khan, S. J., Jalil, A., Siddiqui, M. F., Ahmad, T., et al. (2022). Targeting acyl homoserine lactones (AHLs) by the quorum quenching bacterial strains to control biofilm formation in Pseudomonas aeruginosa. Saudi J. Biol. Sci. 29, 1673–1682. doi: 10.1016/j.sjbs.2021.10.064
Khan, M., Khan, S. J., Hasan, S. W. (2019). Quorum sensing control and wastewater treatment in quorum quenching/ submerged membrane electro-bioreactor (SMEBR(QQ)) hybrid system. Biomass Bioenergy 128, 105329. doi: 10.1016/j.biombioe.2019.105329
Kim, J., Choi, D., Yeon, K., Kim, S., Lee, C. (2011). Enzyme-immobilized nanofiltration membrane to mitigate biofouling based on quorum quenching. Environ. Sci. Technol. 45, 1601–1607. doi: 10.1021/es103483j
Koutsoudis, M. D., Tsaltas, D., Minogue, T. D., von Bodman, S. B. (2006). Quorum-sensing regulation governs bacterial adhesion, biofilm development, and host colonization in pantoea stewartii subspecies stewartii. Proc. Of Natl. Acad. Of Sci. Of United States Of America 103, 5983–5988. doi: 10.1073/pnas.0509860103
Leadbetter, J. R., Greenberg, E. P. (2000). Metabolism of acyl-homoserine lactone quorum-sensing signals by variovorax paradoxus. J. Bacteriology 182, 6921–6926. doi: 10.1128/JB.182.24.6921-6926.2000
Lee, K., Choo, K., Ng, H. Y., Lee, C. (2020). Preparation of a mesoporous silica quorum quenching medium for wastewater treatment using a membrane bioreactor. Biofouling (Chur Switzerland) 36, 369–377. doi: 10.1080/08927014.2020.1749601
Lee, D. H., Lim, J., Lee, J., Roh, E., Jung, K., Choi, M., et al. (2013). Characterization of genes required for the pathogenicity of pectobacterium carotovorum subsp. carotovorum Pcc21 in Chinese cabbage. Microbiology 159, 1487–1496. doi: 10.1099/mic.0.067280-0
Lee, S., Park, S., Kwon, H., Lee, S. H., Lee, K., Nahm, C. H., et al. (2016). Crossing the border between laboratory and field: Bacterial quorum quenching for anti-biofouling strategy in an MBR. Environ. Sci. Technol. 50, 1788–1795. doi: 10.1021/acs.est.5b04795
Li, D. L. (2016). Study of the intestinal flora structure of grass carp infection with aAeromonas hydrophila (Yanglin, China: Northwest A&F University).
Li, J., Chen, W. J., Zhang, W., Zhang, Y., Lei, Q., Wu, S., et al. (2022). Effects of free or immobilized bacterium Stenotrophomonas acidaminiphila Y4B on glyphosate degradation performance and indigenous microbial community structure. J. Agric. Food Chem. 70, 13945–13958. doi: 10.1021/acs.jafc.2c05612
Lin, Y. H., Xu, J. L., Hu, J., Wang, L. H., Ong, S. L., Leadbetter, J. R., et al. (2003). Acyl-homoserine lactone acylase from ralstonia strain XJ12B represents a novel and potent class of quorum-quenching enzymes. Mol. Microbiol. 47, 849–860. doi: 10.1046/j.1365-2958.2003.03351.x
Liu, C., Guo, S., Turak, A., Zhang, J., Zhang, L. (2017). AidE encodes an n-acyl homoserine lactonase in Acinetobacter. Chin. J. Biotechnol. 33, 1625–1639. doi: 10.13345/j.cjb.170156
Loh, J., Pierson, E. A., Pierson, L. S., Stacey, G., Chatterjee, A. (2002). Quorum sensing in plant-associated bacteria. England: Elsevier Ltd 5, 285–290. doi: 10.1016/S1369-5266(02)00274-1
Luo, L. (2010). The separation and purification of AHL-degrading enzyme in rhodosporidium toruloides (Tianjin, China: Hebei University of Technology).
Lu, H. D., Spiegel, A. C., Hurley, A., Perez, L. J., Maisel, K., Ensign, L. M., et al. (2015). Modulating vibrio cholerae quorum-sensing-controlled communication using autoinducer-loaded nanoparticles. Nano Lett. 15, 2235–2241. doi: 10.1021/acs.nanolett.5b00151
Macpherson, H. L., Bergh, Ø, Birkbeck, T. H. (2012). An aerolysin-like enterotoxin from vibrio splendidus may be involved in intestinal tract damage and mortalities in turbot, scophthalmus maximus (L.), and cod, gadus morhua l., larvae. J. Fish Dis. 35, 153–167. doi: 10.1111/j.1365-2761.2011.01331.x
Maisuria, V. B., Nerurkar, A. S. (2015). Interference of quorum sensing by delftia sp. VM4 depends on the activity of a novel n-acylhomoserine lactone-acylase. PloS One 10, 1–15. doi: 10.1371/journal.pone.0138034
Mehmood, C. T., Waheed, H., Tan, W., Xiao, Y. (2021). Photocatalytic quorum quenching: A new antifouling and in-situ membrane cleaning strategy for an external membrane bioreactor coupled to UASB. J. Environ. Chem. Eng. 9, 1–10. doi: 10.1016/j.jece.2021.105470
Mei, G. Y., Yan, X. X., Turak, A., Luo, Z. Q., Zhang, L. Q. (2010). AidH, an alpha/beta-hydrolase fold family member from an ochrobactrum sp. strain, is a novel n-acylhomoserine lactonase. Appl. Environ. Microbiol. 76, 4933–4942. doi: 10.1128/AEM.00477-10
Mishra, S., Huang, Y., Li, J., Wu, X., Zhou, Z., Lei, Q., et al. (2022). Biofilm-mediated bioremediation is a powerful tool for the removal of environmental pollutants. Chemosphere 294, 133609. doi: 10.1016/j.chemosphere.2022.133609
Morillo, E., Villaverde, J. (2017). Advanced technologies for the remediation of pesticide-contaminated soils. Sci. Total Environ. 586, 576–597. doi: 10.1016/j.scitotenv.2017.02.020
Morohoshi, T. U. U. J., Nakazawa, S., Ebata, A., Kato, N., Ikeda, T. (2008). Identification and characterization of n-acylhomoserine lactone-acylase from the fish intestinal shewanella sp. Strain MIB015. Bioscience Biotechnology Biochem. 72, 1887–1893. doi: 10.1271/bbb.80139
Morohoshi, T., Sato, N., Iizumi, T., Tanaka, A., Ikeda, T. (2017). Identification and characterization of a novel n -acyl-homoserine lactonase gene in Sphingomonas ursincola isolated from industrial cooling water systems. J. Bioscience Bioengineering 123, 10. doi: 10.1016/j.jbiosc.2016.12.010
Morohoshi, T., Tominaga, Y., Someya, N., Ikeda, T. (2012). Complete genome sequence and characterization of the n-acylhomoserine lactone-degrading gene of the potato leaf-associated solibacillus silvestris. J. Bioscience Bioengineering 113, 20–25. doi: 10.1016/j.jbiosc.2011.09.006
Nasuno, E., Suzuki, T., Suzuki, R., Okano, C., Kurokura, T., Iimura, K., et al. (2017). Novel quorum quenching enzymes identified from draft genome of roseomonas sp. TAS13. Genomics Data 12, 22–23. doi: 10.1016/j.gdata.2017.02.001
Nazzaro, F., Fratianni, F., Acierno, A. D., De Feo, V., Ayala-Zavala, F. J., Gomes-Cruz, A., et al. (2019). Quorum sensing:molecular mechanism and biotechnological application. Lightning Source Inc., La Vergne, TN, USA. 195–223. doi: 10.1016/B978-0-12-814905-8.00008-3
Nealson, K. H., Platt, T., Hastings, J. W. (1970). Cellular control of the synthesis and activity of the bacterial luminescent system. J. Bacteriology 104, 313–322. doi: 10.1128/jb.104.1.313-322.1970
Nicol, M., Alexandre, S., Luizet, J., Skogman, M., Jouenne, T., Salcedo, S., et al. (2018). Unsaturated fatty acids affect quorum sensing communication system and inhibit motility and biofilm formation of acinetobacter baumannii. Int. J. Mol. Sci. 19, 214. doi: 10.3390/ijms19010214
Niu, Y., Li, T., Gu, C., Yang, X., Qian, G., Hu, B., et al. (2012). Increasing resistance to Ralstonia solanacearum in transgenic tobacco by expressing a quorum-quenching gene attM. J. Nanjing Agric. Univ. 35, 64–70.
Ni, L., Wang, Y. (2019). Advances in MBR biofouling control based on quorum sensing and quenching. J. Harbin Institute Technol. 51, 191–200. doi: 10.11918/j.issn.0367-6234.201811067
Ni, L., Zhu, Y., Ma, J., Wang, Y. (2021). Novel strategy for membrane biofouling control in MBR with CdS/MIL-101 modified PVDF membrane by in situ visible light irradiation. Water Res. 188, 116554. doi: 10.1016/j.watres.2020.116554
Noori, A., Kim, H., Kim, M. H., Kim, K., Lee, K., Oh, H. (2022). Quorum quenching bacteria isolated from industrial wastewater sludge to control membrane biofouling. Bioresource Technol. 352, 127077. doi: 10.1016/j.biortech.2022.127077
Palma, L., Muñoz, D., Berry, C., Murillo, J., Caballero, P. (2014). Bacillus thuringiensis toxins: An overview of their biocidal activity. Toxins 6, 3296–3325. doi: 10.3390/toxins6123296
Park, S. Y., Hwang, B. J., Shin, M. H., Kim, J. A., Kim, H. K., Lee, J. K. (2006). N-acylhomoserine lactonase producing rhodococcus spp. with different AHL-degrading activities. FEMS Microbiol. Lett. 261, 102–108. doi: 10.1111/j.1574-6968.2006.00336.x
Park, S., Lee, S. J., Oh, T., Oh, J., Koo, B., Yum, D., et al. (2003). AhlD, an n-acylhomoserine lactonase in arthrobacter sp., and predicted homologues in other bacteria. Microbiology 149, 1541–1550. doi: 10.1099/mic.0.26269-0
Parsek, M. R., Val, D. L., Hanzelka, B. L., Cronan, J. J. E., Greenberg, E. P. (1999). Acyl homoserine-lactone quorum-sensing signal generation. Proc. Natl. Acad. Sci. 96, 4360–4365. doi: 10.1073/pnas.96.8.4360
Pearson, J. P., KM, G. A. R. Y., Passador, L., Tucker, K. D., Eberhard, A., Iglewski, B. H., et al. (1994). Structure of the autoinducer required for expression of Pseudomonas aeruginosa virulence genes. Proc. Natl. Acad. Sci. United States America 91, 197–201. doi: 10.1073/pnas.91.1.197
Peng, M., Tong, W., Zhao, Z., Xiao, L., Wang, Z., Liu, X., et al. (2021). Attenuation of Aeromonas hydrophila infection in carassius auratus by YtnP, a n-acyl homoserine lactonase from Bacillus licheniformis T-1. Antibiotics 10, 631. doi: 10.3390/antibiotics10060631
Philem, P. D., Yadav, Y., Vellore Sunder, A., Ghosh, D., Prabhune, A., Ramasamy, S. (2020). Structural and enzymatic analysis of a dimeric cholylglycine hydrolase like acylase active on n-acyl homoserine lactones. Biochimie 177, 108–116. doi: 10.1016/j.biochi.2020.07.017
Rao, R. (2021). Screening and characterization of quorum quenching enzyme gene from shinella sp (Nanning, China: Guangxi University), 37–31.
Romero, J. (2012). Antibiotics in aquaculture-use, abuse and alternatives. IntechOpen 2012, 159–197.
Salmond, G. P. C., Bycroft, B. W., Stewart, G. S. A. B., Williams, P. (1995). The bacterial ‘enigma’: Cracking the code of cell-cell communication. Mol. Microbiol. 16, 615–624. doi: 10.1111/j.1365-2958.1995.tb02424.x
Schipper, C., Hornung, C., Bijtenhoorn, P., Quitschau, M., Grond, S., Streit, W. R. (2009). Metagenome-derived clones encoding two novel lactonase family proteins involved in biofilm inhibition in Pseudomonas aeruginosa. Appl. Environ. Microbiol. 75, 224–233. doi: 10.1128/AEM.01389-08
See-Too, W. S., Ee, R., Lim, Y., Convey, P., Pearce, D. A., Yin, W., et al. (2017). AidP, a novel n-acyl homoserine lactonase gene from Antarctic planococcus sp. Sci. Rep. 7, 1–11. doi: 10.1038/srep42968
Shah, S. S. A., Choo, K. (2020). Isolation and characterization of novel indigenous facultative quorum quenching bacterial strains for ambidextrous biofouling control. Bioresource Technol. 308, 123269. doi: 10.1016/j.biortech.2020.123269
Shepherd, R. W., Lindow, S. E. (2009). Two dissimilar N-acyl-homoserine lactone acylases of pseudomonas syringae influence colony and biofilm morphology. Appl. Environ. Microbiol. 75, 45–53. doi: 10.1128/AEM.01723-08
Shi, W. (2021). Directed screening of endophytic bacteria with new QS quenching enzyme gene from pogostemon cablin and their effects on Ralstonia solanacearum wilt (Guangzhou, China: Guangdong Pharmaceutical University).
Shu, N. N., Park, H., Shah, S. S. A., Mameda, N., Yoo, H. J., Min, J., et al. (2022). Probiotic strategy for biofouling control through direct injection of quorum-quenching bacteria into membrane bioreactors. Chem. Eng. J. 438, 135572. doi: 10.1016/j.cej.2022.135572
Siddiqui, M. F., Sakinah, M., Singh, L., Zularisam, A. W. (2012). Targeting n-acyl-homoserine-lactones to mitigate membrane biofouling based on quorum sensing using a biofouling reducer. J. Biotechnol. 161, 190–197. doi: 10.1016/j.jbiotec.2012.06.029
Song, L., Fang, R., Jia, Y. (2017). Function of biofilms in phytopathogenic bacterial-host interactions. Chin. J. Biotechnol. 33, 1640–1653. doi: 10.13345/j.cjb.170079
Song, K., Guo, X., Chen, B., He, Y. (2021). Molecular mechanisms and biological significance of the naturally occurring turnover of DSF-family quorum sensing signal in the phytopathogen xanthomonas. Acta Microbiologica Sin. 61, 68–76. doi: 10.13343/j.cnki.wsxb.20200598
Stoltz, D. A., Ozer, E. A., Taft, P. J., Barry, M., Liu, L., Kiss, P. J., et al. (2008). Drosophila are protected from Pseudomonas aeruginosa lethality by transgenic expression of paraoxonase-1. J. Clin. Invest. 118, 3123–3131. doi: 10.1172/JCI35147
Syafiuddin, A., Boopathy, R., Mehmood, M. A. (2021). Recent advances on bacterial quorum quenching as an effective strategy to control biofouling in membrane bioreactors. Bioresource Technol. Rep. 15, 100745. doi: 10.1016/j.biteb.2021.100745
Tao, F., He, Y., Wu, D., Swarup, S., Zhang, L. (2010). The cyclic nucleotide monophosphate domain of Xanthomonas campestris global regulator clp defines a new class of cyclic di-GMP effectors. J. Bacteriology 192, 1020–1029. doi: 10.1128/JB.01253-09
Terwagne, M., Mirabella, A., Lemaire, J., Deschamps, C., De Bolle, X., Letesson, J., et al. (2013). Quorum sensing and self-quorum quenching in the intracellular pathogen brucellamelitensis. PloS One 8, 1–14. doi: 10.1371/journal.pone.0082514
Tom Defoirdt, P. S. A. P. (2011). Alternatives to antibiotics for the control of bacterial disease in aquaculture. Curr. Opin. Microbiol. 14, 251–258. doi: 10.1016/j.mib.2011.03.004
Uroz, S., Oger, P. M. (2017). Screening for n-AHSL-Based-Signaling interfering enzymes. Methods Mol. Biol. (Clifton N.J.) 668, 1539. doi: 10.1007/978-1-60761-823-2_14
Uroz, S., Oger, P. M., Chapelle, E., Adeline, M., Faure, D., Dessaux, Y. (2008). A rhodococcus qsdA-encoded enzyme defines a novel class of large-spectrum quorum-quenching lactonases. Appl. Environ. Microbiol. 74, 1357–1366. doi: 10.1128/AEM.02014-07
Vargas, E. L. G., de Almeida, F. A., de Freitas, L. L., Pinto, U. M., Vanetti, M. C. D. (2021). Plant compounds and nonsteroidal anti-inflammatory drugs interfere with quorum sensing in chromobacterium violaceum. Arch. Microbiol. 203, 5491–5507. doi: 10.1007/s00203-021-02518-w
Vinoj, G., Vaseeharan, B., Thomas, S., Spiers, A. J., Shanthi, S. (2014). Quorum-quenching activity of the AHL-lactonase from Bacillus licheniformis DAHB1 inhibits vibrio biofilm formation in vitro and reduces shrimp intestinal colonisation and mortality. Mar. Biotechnol. 16, 707–715. doi: 10.1007/s10126-014-9585-9
Wang, D., Chen, H., Li, J., Li, T., Ren, L., Liu, J., et al. (2022). Screening and validation of quorum quenching enzyme PF2571 from pseudomonas fluorescens strain PF08 to inhibit the spoilage of red sea bream filets. Int. J. Food Microbiol. 362, 109476. doi: 10.1016/j.ijfoodmicro.2021.109476
Wang, H., Liao, L., Chen, S., Zhang, L. (2020). A quorum quenching bacterial isolate contains multiple substrate-inducible genes conferring degradation of diffusible signal factor. Appl. Environ. Microbiol. 86, e02930-19. doi: 10.1128/AEM.02930-19
Wang, H., Lin, Q., Dong, L., Wu, W., Liang, Z., Dong, Z., et al. (2022). A bacterial isolate capable of quenching both diffusible signal factor- and n-acylhomoserine lactone-family quorum sensing signals shows much enhanced biocontrol potencies. J. Agric. Food Chem. 70, 7716–7726. doi: 10.1021/acs.jafc.2c01299
Wang, W. Z., Morohoshi, T., Ikenoya, M., Someya, N., Ikeda, T. (2010). AiiM, a novel class of n-acylhomoserine lactonase from the leaf-associated bacterium microbacterium testaceum. Appl. Environ. Microbiol. 76, 2524–2530. doi: 10.1128/AEM.02738-09
Wang, W., Morohoshi, T., Someya, N., Ikeda, T. (2012). AidC, a novel n-acylhomoserine lactonase from the potato root-associated cytophaga-flavobacteria-bacteroides (CFB) group bacterium chryseobacterium sp. strain StRB126. Appl. Environ. Microbiol. 78, 7985–7992. doi: 10.1128/AEM.02188-12
Werner, N., Petersen, K., Vollstedt, C., Garcia, P. P., Chow, J., Ferrer, M., et al. (2021). The komagataeibacter europaeus GqqA is the prototype of a novel bifunctional n-acyl-homoserine lactone acylase with prephenate dehydratase activity. Sci. Rep. 11, 12255. doi: 10.1038/s41598-021-91536-1
Xiao, Y., Waheed, H., Xiao, K., Hashmi, I., Zhou, Y. (2018). In tandem effects of activated carbon and quorum quenching on fouling control and simultaneous removal of pharmaceutical compounds in membrane bioreactors. Chem. Eng. J. 341, 610–617. doi: 10.1016/j.cej.2018.02.073
Xiao, X., Zhu, W., Liu, Q., Yuan, H., Li, W., Wu, L., et al. (2016). Impairment of biofilm formation by TiO2 photocatalysis through quorum quenching. Environ. Sci. Technol. 50, 11895–11902. doi: 10.1021/acs.est.6b03134
Xue, B., Chow, J. Y., Baldansuren, A., Yap, L. L., Gan, Y. H., Dikanov, S. A., et al. (2013). Structural evidence of a productive active site architecture for an evolved quorum-quenching GKL lactonase. Biochemistry 52, 2359–2370. doi: 10.1021/bi4000904
Xu, H., Liu, Y. (2011). Reduced microbial attachment by d-amino acid-inhibited AI-2 and EPS production. Water Res. 45, 5796–5804. doi: 10.1016/j.watres.2011.08.061
Xu, X., Ye, T., Zhang, W., Zhou, T., Zhou, X., Dai, W., et al. (2021). Identification of FadT as a novel quorum quenching enzyme for the degradation of diffusible signal factor in Cupriavidus pinatubonensis strain HN-2. Int. J. Mol. Sci. 22, 9862. doi: 10.3390/ijms22189862
Yan, X. (2018). Design, synthesis and biological evaluation of antagonists of bacterial quorum sensing (Changchun, China: Jilin University).
Yeon, K., Cheong, W., Oh, H., Lee, W., Hwang, B., Lee, C., et al. (2009a). Quorum sensing: a new biofouling control paradigm in a membrane bioreactor for advanced wastewater treatment. Environ. Sci. Technol. 43, 380–385. doi: 10.1021/es8019275
Yeon, K., Lee, C., Kim, J. (2009b). Magnetic enzyme carrier for effective biofouling control in the membrane bioreactor based on enzymatic quorum quenching. Environ. Sci. Technol. 43, 7403–7409.
Ye, T., Zhang, W., Feng, Z., Fan, X., Xu, X., Mishra, S., et al. (2020a). Characterization of a novel quorum-quenching bacterial strain, Burkholderia anthina HN-8, and its biocontrol potential against black rot disease caused by Xanthomonas campestris pv. campestris. Microorganisms 8, 1485. doi: 10.3390/microorganisms8101485
Ye, T., Zhou, T., Fan, X., Bhatt, P., Zhang, L., Chen, S. (2019). Acinetobacter lactucae strain QL-1, a novel quorum quenching candidate against bacterial pathogen Xanthomonas campestris pv. campestris. Front. Microbiol. 10. doi: 10.3389/fmicb.2019.02867
Ye, T., Zhou, T., Li, Q., Xu, X., Fan, X., Zhang, L., et al. (2020b). Cupriavidus sp. HN-2, a novel quorum quenching bacterial isolate, is a potent biocontrol agent against Xanthomonas campestris pv. campestris. Microorganisms 8, 45. doi: 10.3390/ijms22189862
Ye, T., Zhou, T., Xu, X., Zhang, W., Fan, X., Mishra, S., et al. (2020c). Whole-genome sequencing analysis of quorum quenching bacterial strain Acinetobacter lactucae QL-1 identifies the FadY enzyme for degradation of the diffusible signal factor. Int. J. Mol. Sci. 21, 6729. doi: 10.3390/ijms21186729
Yu, M., Liu, N., Zhao, Y., Zhang, X. (2018). Quorum quenching enzymes of marine bacteria and implication in aquaculture. J. Ocean Univ. China 48, 34–43. doi: 10.16441/j.cnki.hdxb.20170202.
Yu, H., Chen, W. J., Bhatt, K., Zhou, Z., Zhu, X., Liu, S., et al. (2022). A novel bacterial strain Burkholderia sp. F25 capable of degrading diffusible signal factor signal shows strong biocontrol potential. Front. Plant Sci. 13. doi: 10.3389/fpls.2022.1071693
Zhang, L. H. (2019). Research progress of microbial quorum sensing systems. J. South China Agric. Univ. 40, 50–58. doi: 10.7671/j.issn.1001-411X.201905061
Zhang, W., Fan, X., Li, J., Ye, T., Mishra, S., Zhang, L., et al. (2021). Exploration of the quorum-quenching mechanism in Pseudomonas nitroreducens W-7 and its potential to attenuate the virulence of Dickeya zeae EC1. Front. Microbiol. 12. doi: 10.3389/fmicb.2021.694161
Zhang, L. H., Kerr, A. (1991). A diffusible compound can enhance conjugal transfer of the Ti plasmid in Agrobacterium tumefaciens. J. Bacteriology 173, 1867–1872. doi: 10.1128/JB.173.6.1867-1872.1991
Zhang, W., Luo, Q., Zhang, Y., Fan, X., Ye, T., Mishra, S., et al. (2020). Quorum quenching in a novel Acinetobacter sp. XN-10 bacterial strain against Pectobacterium carotovorum subsp. carotovorum. Microorganisms 8, 1100. doi: 10.3390/microorganisms8081100
Zhang, L. H., Murphy, P. J., Kerr, A., Tatet, M. E. (1993). Agrobacterium conjugation and gene regulation by n-acyl-L-homoserine lactones. Nature 362, 446–447. doi: 10.1038/362446a0
Zhang, L., Ruan, L., Hu, C., Wu, H., Chen, S., Yu, Z., et al. (2007). Fusion of the genes for AHL-lactonase and s-layer protein in Bacillus thuringiensis increases its ability to inhibit soft rot caused by erwinia carotovora. Appl. Microbiol. Biotechnol. 74, 667–675. doi: 10.1007/s00253-006-0696-8
Zhang, J., Rui, X., Wang, L., Guan, Y., Sun, X., Dong, M. (2014). Polyphenolic extract from Rosa rugosa tea inhibits bacterial quorum sensing and biofilm formation. Food Control 42, 125–131. doi: 10.1016/j.foodcont.2014.02.001
Zhang, H., Wang, L., Zhang, L. (2002). Genetic control of quorum-sensing signal turnover in Agrobacterium tumefaciens. Proc. Natl. Acad. Sci. 99, 4638–4643. doi: 10.1073/pnas.022056699
Zhang, G., Zhang, F., Ding, G., Li, J., Guo, X., Zhu, J., et al. (2012). Acyl homoserine lactone-based quorum sensing in a methanogenic archaeon. ISME J. 6, 1336–1344. doi: 10.1038/ismej.2011.203
Zhang, Q., Zhang, Y., He, L., Jiang, T., Tong, Y. (2018). Identification and bioinfomatics analysis of quorum-quenching genes from Acinetobacter sp. 3-59. J. Yangzhou Univ. (Agricultural Life Sci. Edition) 39, 105–110. doi: 10.16872/j.cnki.1671-4652.2018.01.019
Zhang, X., Zhang, L., Wang, L., Zhang, H., Xu, J., Dong, Y. (2001). Quenching quorum-sensing-dependent bacterial infection by an n-acyl homoserine lactonase. Nature 411, 813–817. doi: 10.1038/35081101
Zhang, W., Li, J., Zhang, Y., Wu, X., Zhou, Z., Huang, Y., et al. (2022). Characterization of a novel glyphosate-degrading bacterial species, Chryseobacterium sp. Y16C, and evaluation of its effects on microbial communities in glyphosate-contaminated soil. J. Hazard. Mater. 432, 128689. doi: 10.1016/j.jhazmat.2022.128689
Zhao, Z. (2020). Study of quorum quenching enzyme YtnP on grass carp intestinal microbiota diversity and immune response regulation (Shanghai, China: Shanghai Ocean University).
Zheng, Y., Hu, Y., Hu, F., Cai, X. (2021). Screening of biocontrol bacteria against ralstonia pseudosolanacearum with gene aiiA and identification of the bacteria. J. Nucl. Agric. Sci. 35, 1322–1328. doi: 10.11869/j.issn.100-8551.2021.06.1322
Zhou, Z., Wu, X., Li, J., Zhang, Y., Huang, Y., Zhang, W., et al. (2022). A novel quorum quencher, Rhodococcus Pyridinivorans XN-36, is a powerful agent for the biocontrol of soft rot disease in various host plants. Biol. Control 169, 104889. doi: 10.1016/j.biocontrol.2022.104889
Keywords: quorum quenching, quorum sensing, biocontrol, acyl homoserine lactones, diffusible signal factor
Citation: Zhu X, Chen W-J, Bhatt K, Zhou Z, Huang Y, Zhang L-H, Chen S and Wang J (2023) Innovative microbial disease biocontrol strategies mediated by quorum quenching and their multifaceted applications: A review. Front. Plant Sci. 13:1063393. doi: 10.3389/fpls.2022.1063393
Received: 17 October 2022; Accepted: 15 December 2022;
Published: 12 January 2023.
Edited by:
Jesús Murillo, Public University of Navarre, SpainReviewed by:
Bilal Ahmed, Yeungnam University, South KoreaGeeta Bhandari, Swami Rama Himalayan University, India
Copyright © 2023 Zhu, Chen, Bhatt, Zhou, Huang, Zhang, Chen and Wang. This is an open-access article distributed under the terms of the Creative Commons Attribution License (CC BY). The use, distribution or reproduction in other forums is permitted, provided the original author(s) and the copyright owner(s) are credited and that the original publication in this journal is cited, in accordance with accepted academic practice. No use, distribution or reproduction is permitted which does not comply with these terms.
*Correspondence: Kalpana Bhatt, a2FscGFuYS5rYW51YmhhdHRAZ21haWwuY29t; Shaohua Chen, c2hjaGVuQHNjYXUuZWR1LmNu; Junxia Wang, anVueGlhd2FuZ0BzY2F1LmVkdS5jbg==
†These authors have contributed equally to this work