- 1Amity Institute of Biotechnology, Amity University Uttar Pradesh, Lucknow, India
- 2Indian Council of Agricultural Research (ICAR)-National Bureau of Agriculturally Important Microorganisms, Kushmaur, Maunath Bhanjan, India
- 3Indian Council of Agricultural Research (ICAR)-Indian Institute of Seed Sciences, Kushmaur, Maunath Bhanjan, India
In this study, rhizospheric and endophytic bacteria were tested for the alleviation of salinity stress in rice. Endophytic isolates were taken from previous studies based on their salt stress-alleviating traits. The rhizospheric bacteria were isolated from rice and screened based on salt tolerance and plant growth-promoting traits. Molecular identification indicated the presence of class Gammaproteobacteria, Bacillota, and Actinomycetia. Two-two most potential isolates each from rhizospheric and endophytic bacteria were selected for in planta trials. Results showed that microbial inoculation significantly improved germination and seedling vigor under elevated salinity. The confocal scanning laser microscopy showed higher bacterial colonization in inoculated rice roots than in control. Based on this experiment, rhizospheric bacteria Brevibacterium frigoritolerans W19 and endophytic Bacillus safensis BTL5 were selected for pot trial along with a growth-inducing compound melatonin 20 ppm. Inoculation of these two bacteria improved the levels of chlorophyll, proline, phenylalanine ammonia-lyase, catalase, superoxide dismutase, polyphenol oxidase, root-shoot length, and dry weight under elevated salt concentration. The gene expression studies showed modulation of SOD1, CATa, NHX1, and PAL1 genes by the bacterial strains and melatonin application. The inoculation was found to have additive effects with 20 ppm melatonin. This enhancement in dry matter accumulation, compatible solute production, and oxidative stress regulation could help plants in mitigating the ill effects of high salinity. Exploring such a combination of microbes and inducer molecules could be potentially useful in developing stress-alleviating bioformulations.
1. Introduction
Rice (Oryza sativa L.) is a staple crop offering food to >2 billion human population (Ziska et al., 2015) and the significance further intensifies with the projected population growth, expected to reach 9.7 billion by 2050 (World Population Prospects, 2019). On the other hand, the acreage of arable soil is diminishing, and increasing soil salinity is one of the major causes behind it, affecting >20% of irrigated land globally (Shrivastava and Kumar, 2015). Soil salinity negatively alters plant growth, production, and grain quality. One of the deleterious effects is salt-induced germination failure and growth retardation in seedlings (Hakim et al., 2010; Bai et al., 2017). Despite the huge commercial importance of different Basmati rice varieties, soil salinity affects germination, early seedling growth, ion content, and other physiological parameters (Jamil et al., 2012).
Various plant growth-promoting bacteria (PGPB) have the potential to alleviate the deleterious effects of salinity (Shahzad et al., 2017; Kashyap et al., 2019; Bakhshandeh et al., 2020). Khan et al. (2020) showed that halophytic endophytes from genera Curtobacterium, Enterobacter, Bacillus, and Micrococcus resulted in enhanced growth of Waito‐C rice under salinity stress. It is necessary to explore ways to improve the growth and performance of the seedlings at the nursery level, as the cost of seeds contributes to a major portion of the cost of cultivation. Early growth improvement has greater significance in the good plant stand. Thus, microbes that could enhance seedling establishment could directly contribute to better yield. Different phytohormones produced by the microbes are reported to improve seed germination and growth (Shahzad et al., 2017; Khan et al., 2020).
Microbes having the potential to enhance the availability of nutrients in the rhizosphere could compensate for the nutrient deprivation caused by high salinity (Jiménez-Mejía et al., 2022). The exopolysaccharides (EPS) production and the formation of biofilms that accumulate EPS around the rhizosphere reduce the available Na+ content around the plant roots, thus reducing the harmful effects of soil salinity (Singh and Jha, 2016; Sagar et al., 2022). Also, enhancement of nutrient uptake by phosphate solubilizing microorganisms, zinc solubilizing microorganisms, iron quenchers, phytohormone producers/inducers, etc. could help plants grow under saline conditions (Sahu et al., 2021a; Jiménez-Mejía et al., 2022). Therefore, microbes having the potential for production of exopolysaccharides, siderophores, IAA, and solubilization of plant nutrients like phosphorus, and Zn, could guard the plants against salinity stress to some extent and supplement nutrition to keep the plants healthy. Since microbial diversity is huge with varied functional behavior, exploring a wide diversity of stress-tolerant microorganisms could be a viable option to help overcome the germination problem in saline areas. Thus, in the present study, both endophytic and rhizospheric microbes were screened to harness benefits from both niches.
Apart from the plant growth promoting potential of the microorganisms, colonization efficiency is a major factor that affects their function. Effective colonization in the plant system indicates inoculum efficacy for producing plant health and growth promotion activities, referred to as rhizosphere competence (Raaijmakers and Weller, 2001) in the case of rhizospheric microbes, and as internal colonization competence for an endophyte realizing its functional potential (Compant et al., 2005). Colonization and its further survival in the rhizosphere and endosphere are also important to evaluate the effectiveness of delivery techniques (Compant et al., 2005). Although several reports suggest the evaluation of colonization competence of applied inoculants by different techniques such as molecular tracking (Ownley et al., 2008), electron microscopy (Ownley et al., 2008), gfp and gusA (Compant et al., 2005), qPCR (Barelli et al., 2018), confocal scanning laser microscopy (Álvarez et al., 2020; Sahu et al., 2020), etc., the colonization with respect to the presence of elevated stress is yet to be explored in greater detail. So, this study was done to find out how well endophytic and rhizospheric microbes colonize plants when they are under salt stress.
In addition to the microbial potential, several plant growth-inducing compounds have been screened for their effects in alleviating abiotic stresses. Melatonin (N-acety-5-methoxytryptamine) is one of them, which is being explored for growth induction and stress alleviation (Liang et al., 2017). It was found to induce root growth, photosynthetic pigments, reactive oxygen species scavengers, etc. and to reduce electrolyte leakage under elevated salinity (Altaf et al., 2021). Despite the potential roles of melatonin, the combined effects of melatonin with microbes are the least worked out. Therefore, in this study, the effects of potential microbes on salinity-induced germination failure, root colonization, and their complementation with melatonin were assessed. The present investigation was focused on exploring microbes from different niches for salinity alleviation, colonization competence under salt stress, and compatibility with melatonin to improve plant performance under salinity.
2. Material and methods
2.1. Microbial cultures and characterization for salinity alleviation traits
2.1.1. Microbial cultures
Rhizosphere soil samples were isolated from six paddy fields from the saline-sodic patches of the Indo-Gangetic plains of Eastern Uttar Pradesh. Two bacterial endophytes, potential alleviators for biotic and abiotic stress tolerance, Bacillus haynesii 2P2 from Solanum lycopersicum (Sahu et al., 2019) and Bacillus safensis BTL5 from Ocimum tenuiflorum (Sahu et al., 2021b), were taken from PMI and RB Lab, ICAR-NBAIM and stored in glycerol stock till further use.
2.1.2. Growth under hypersaline conditions and production of exopolysaccharides
The rhizospheric isolates were screened for salt tolerance. An actively growing bacterial suspension (24 h old) was spotted onto the nutrient agar medium enriched with five different NaCl concentrations (400, 800, 1200, 1600, and 2000 mM) and incubated for 24 h at 28 ± 0.5°C. Production of EPS was qualitatively assayed as per the method described in Siddikee et al. (2011). For 5 days, the rhizospheric isolates were grown in 50 mL minimal media in a shaker (150 rpm) at 28 ± 0.5°C. The cultures were centrifuged (10000g for 10 min) and the supernatants were collected. The formation of a precipitate after the addition of ice-cold ethanol indicated a positive reaction for EPS production.
2.2. Characterization for growth-promoting traits in-vitro
The phosphate (P) solubilization capacity of rhizospheric isolates was determined by spotting actively grown cultures in modified Sperbers’ agar medium as described in Sahu (2012). After incubation for 48 h, the development of a clear halo around the spots was considered a positive response for P-solubilization. Siderophore producing capacity was determined as per the methodology of Schwyn and Neilands (1987), by the chrome azurol S assay. The formation of the orange halo zone around the colonies after 48 hours of incubation at 28 ± 0.5°C indicated a positive response for siderophore production. Zinc (Zn) solubilization by bacterial strains was assessed as per the method described in Fasim et al. (2002), following incubation for 48 h at 28 ± 0.5°C in mineral salts agar medium supplemented with 0.1% of insoluble ZnO. Solubilization of insoluble zinc compound resulted in a clear zone formation around the spots. Production of ammonia by the bacterial isolates was assessed using the method described in Cappuccino and Sherman (1992) with slight modifications. In brief, 24 hour old (diluted to 0.2 OD) cultures were inoculated into 5 mL of peptone broth and incubated for 72 hours at 28 ± 0.5°C. After incubation, each culture was centrifuged and the supernatant was mixed with 0.5 mL of Nessler’s reagent. The development of yellow-brown to dark brown was considered a positive response for ammonia production. Indole-3-acetic acid (IAA) production by bacterial isolates was studied as per the method described elsewhere (Patten and Glick, 1996). The intensity of the developed pink color was read at 530 nm for quantitative measurement.
2.3. Molecular identification of bacterial isolates
Freshly grown cultures (24 h old) were used for isolating DNA from rhizospheric isolates using the Genetix Nucleopore kit following the manufacturer’s instructions. PCR amplification of the 16S rRNA gene was done using primers PA forward (5’-AGAGTTTGATCCTGGCTCAG-3’) and PH reverse (5’-AAGGAGGTGATCCAGCCGCA-3’) with a target product length of 1500 bp (approx). The amplified 16S rDNA was purified and sequencing was done by the Sanger method. The sequences obtained were curated and the sequence similarity was checked in the EZbiocloud database (https://www.ezbiocloud.net) as well as with the NCBI Blastn program. Sequences with the nearest match identities were submitted to the NCBI-GeneBank database. The phylogenetic tree was constructed using the neighbor-joining method in MEGA7 (version=7.0.25) (Figure 1).
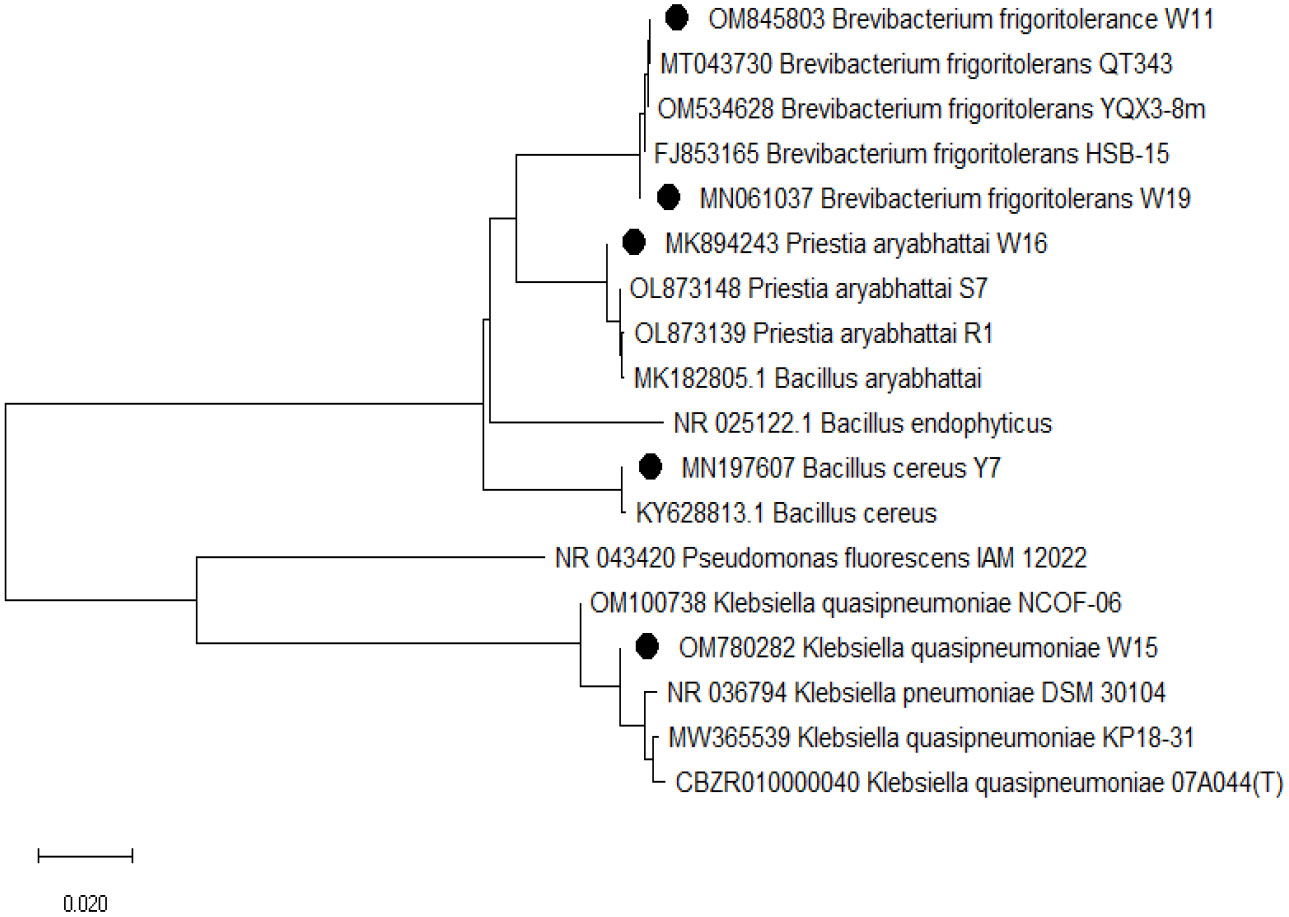
Figure 1 Phylogenetic tree indicating the evolutionary relationship of the bacterial isolates under study with other close relatives as inferred by the Neighbor-Joining (NJ) method in the program MEGA 7 (version=7.0.25). Bootstrap analysis was undertaken on 1000 random samples assessed from the multiple alignments.
2.4. In-vivo experiments
Based on salinity tolerance and plant growth promoting traits, two rhizospheric isolates (W15 and W19) along with two endophytic isolates (2P2 and BTL5) were selected for in vivo studies. In the first experiment, the effects on germination, seedling emergence, and colonization potential were tested on the rice cultivar Pusa Basmati-1 (PB-1) in a roll towel assay. In the second trial, one rhizospheric (W19) and one endophytic strain (BTL5) were used in a pot trial with a growth inducer compound, melatonin.
2.4.1. Effect on salinity-induced germination failure and seedling vigor
Surface sterilized rice seeds were inoculated with 24-hour cultures and the mixtures incubated for 30 min. Three sets of treatments were made, one without additional salt, one with 100 mM NaCl, and one with 200 mM NaCl. The treatments in each set were (T1) Control, (T2) W15, (T3) W19, (T4) 2P2, and (T5) BTL5. Twenty-five seeds were placed on sterile germination paper and rolled. The assembly is placed in sterile conditions and incubated for 2 weeks.
2.4.2. Colonization under different salt concentrations
To understand the colonization behavior under elevated salinity, root samples from two salt concentrations (i.e. control and 200 mM NaCl) from roll towel assay were taken for the colonization experiment. The fine roots were stained with the LIVE/DEAD™ BacLight™ bacterial viability kit (Invitrogen, USA) as described elsewhere (Sahu et al., 2019) and visualized using a Confocal Scanning Laser Microscope (CSLM; Nikon Eclipse 90i) under a 488 nm channel. The artifacts arising from autofluorescence were avoided by comparing the samples with un-inoculated control samples so that the fluorescence from bacterial colonization could be differentiated.
2.4.3. Effect on salinity alleviation in pot trials
Two bacterial isolates from the first in vivo trial were used in pot trials with seven treatments and 3 replications each, in a randomized complete block design. The treatments were -T1= Negative control, T2= Positive control (200 mM NaCl), T3 = 200 mM NaCl + Melatonin 20 ppm, T4 = 200 mM NaCl + Bacillus safensis BTL5, T5 = 200 mM NaCl + Brevibacterium frigoritolerans W19, T6 = 200 mM NaCl + Bacillus safensis BTL5 + Melatonin 20 ppm, T7 = 200 mM NaCl + Brevibacterium frigoritolerans W19 + Melatonin 20 ppm. Pots were prepared and seedling inoculation was done as per the method described elsewhere (Sahu et al., 2019). Two paddy seedlings per hill having equal height, and two hills per pot were transplanted in pots. The salinity was maintained by 200 mM NaCl in respective pots. In the case of melatonin treatment, 20 ppm solution was sprayed on the foliar parts on 30 days old plants.
2.4.3.1. Chlorophyll content
Leaves were harvested 50 days after transplanting for assessing chlorophyll content. One gram of leaf tissue was crushed in 80% pre-chilled acetone and the volume was made up to 100 mL. The absorbance of the supernatant was recorded at 645, 663, and 645 nm using a UV-vis 1700 spectrophotometer, Shimadzu, Japan. The amount of chlorophyll (mg/g) in leaf tissue was calculated by the formula given in Sadashivam and Manickam (1996).
2.4.3.2. Proline content
Proline content in different treatments was measured 50 days after transplanting (DAT) by crushing 0.5 g sample in 10 mL 3% aqueous sulphosalicylic acid followed by filtering with Whatman no. 2 filter paper. Two mL of glacial acetic and 2 mL acid ninhydrin were added to 2 mL of filtrate. The mixture was kept in a boiling water bath for a period of 1 h. The reaction was terminated by keeping the mixture in an ice bath. Further, toluene (4 mL) was mixed by stirring for 20-30 sec, and the mixture was kept at room temperature for toluene layer separation. The upper layer was taken, and the absorbance of the red color was measured at 520 nm as described in Sadashivam and Manickam (1996).
2.4.3.3. PAL content
Phenylalanine ammonia-lyase (PAL) content in shoot tissues was measured by preparing an enzyme extract as per the protocol of Havir (1987). Further, the enzyme extract (0.2 mL) was mixed with borate buffer (0.5 mL) and water (1.3 mL). In this mixture, one mL of L-phenylalanine solution was added to this mixture to initiate the reaction, which was incubated at 32 ± 0.5°C for 60 min. The reaction was stopped by adding 0.5 mL of 1 M Tri-chloroacetic acid. The absorbance at 290 nm was measured using a UV-vis 1700 spectrophotometer.
2.4.3.4. Antioxidant enzymes
The activities of catalase (CAT), superoxide dismutase (SOD), and polyphenol oxidase (PPO) in shoot tissues were assessed at 50 DAT following the protocol of Sadashivam and Manickam (1996). Briefly, 1 g of fresh plant tissue was ground in 3 mL of 0.1 M phosphate buffer (pH 7.0) and centrifuged at 12000 rpm for 15 min to prepare the enzyme extract. This enzyme extract was used for the estimation of CAT, SOD, and PPO. CAT activity was measured after mixing the enzyme extract (100 µl) with 3 mL phosphate buffer (50 mM). The reaction was started by adding 30 mL hydrogen peroxide (12.3 mM). The absorbance was recorded at 240 nm. In the case of SOD activity, the reaction mixture was prepared in 50 mM phosphate buffer (pH 7), and contained 1 mM EDTA, 13 mM methionine, 5 mM nitroblue tetrazolium and 300 µL of enzyme extract. In this reaction mixture, 30 µL of 2 mM riboflavin was added at the end and mixed well. The tubes were kept under light for 10 min to start the reaction, which was stopped by incubation in the dark. The absorbance was read at 560 nm. Polyphenol oxidase activity in rice leaves was determined by adding 100 µL of enzyme extract to a mixture containing 1.4 mL citrate phosphate buffer (0.1 M), 0.5 mL 1,3,5-Trinitrobenzene, and 1 mL catechol solution (2 mM). Absorbance was read after adding the enzyme extract at 412 nm in 30 sec intervals for 3 min.
2.4.3.5. Gene expression study
The total plant RNA was isolated using PureLink™ RNA mini Kit (Invitrogen, USA) following manufacturer’s instruction. Total RNA was converted to cDNA using High Capacity RNA-to-cDNA kit (Thermo Fisher Scientific, USA) following manufacturer’s instructions. Yield of cDNA among different treatments of two cultivars was assessed using nanodrop and a final concentration of 50 ng/µl was used uniformly for assessing the differences in the transcript levels among the treatments. The cDNA was be used for RT-PCR studies with key genes for salinity stress tolerance.
The gene expression study using RT-qPCR (Bio-Rad, USA) was taken up for four salt stress responsive genes (Supplementary Table 1). For this study, Eva Green SYBR Green Supermix Kit (Bio-Rad, USA) was used. Final concentration of gene-specific primers was maintained at 10pmol/µl and internal controls were utilized to determine and normalize the transcript level of mRNA. In the reaction mixture of 10 µl, 2 µl cDNA (50 ng/µl), 1.5 µl each of forward and reverse primers (10pmol/µl), and 5 µl real-time master mix. The RT-qPCR cycles were- denaturation at 95°C for 2 min; 40 repeats at 95°C for 30 s; 60°C for 30 s; and 72°C for 30 s. The fold changes in gene expression was assessed using 2-ΔΔCT method (Livak and Schmittgen, 2001) using endogenous gene Actin.
2.4.3.6. Plant growth parameters
Root-shoot length and dry weight were recorded from different replicas of each treatment at 75 DAT. The pot soil was washed carefully to harvest the entire root mass. Dry weight was taken by drying the plant samples in a hot air oven at 70 °C until samples gained a stable weight.
2.5. Statistical analysis
In these studies with rhizospheric and endophytic bacteria, the experiments were performed in a randomized complete block design. Paper towel assay was performed with 15 treatments and three replicas each. The pot experiment was performed with 7 treatments and three replications each. Data were analyzed by one-way ANOVA and the means were compared by DMRT at p ≤ 0.05. The principal component analysis (PCA) biplot was done for growth attributes and ROS scavenging systems of rice plants using Displayr software.
3. Results
3.1. Isolation of rhizospheric microbes and characterization for salinity alleviation traits
A total of 27 bacterial cultures were isolated from the rhizosphere soil of rice. These isolates were screened for growth under hypersaline conditions and shown to have differential tolerance to NaCl concentrations up to 2000 mM (Figure 2). More than 33% of the isolates could survive a salt concentration of 1200 mM. Amongst them, four isolates could survive even at a 2000 mM NaCl concentration. Exopolysaccharide production was also recorded from these plates. Among 27 isolates, 51.84% of isolates could produce EPS. In these, 14.81% of the isolates (Y1, P1, W15, and W16) were producing a good amount of EPS. Low EPS production was recorded from 37.03% of the isolates. In general, higher EPS production was recorded in the plates with relatively higher salt concentrations. Relatively higher EPS production was found in the plates amended with 1200 mM NaCl.
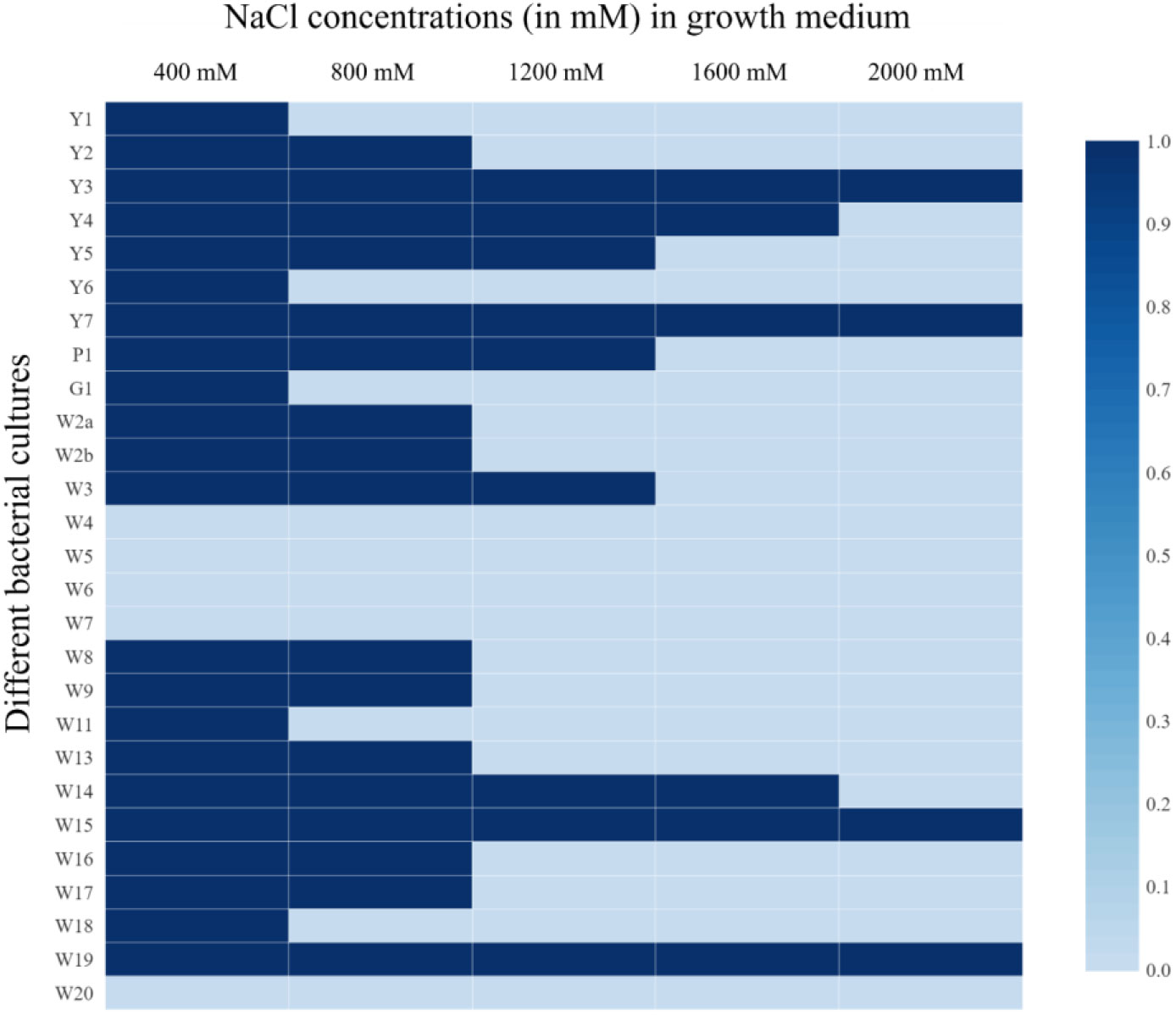
Figure 2 Salt tolerance of bacterial isolates from rice rhizosphere under 400, 800, 1200, 1600, and 2000 mM NaCl concentration; 1= Growth, and 0= No growth.
3.2. Characterization for growth-promoting traits in-vitro
Among the rice rhizospheric isolates, ten isolates (37.03%) were found to be positive for P-solubilization (Figure 3). The largest zone size was reported from isolates W3, W15, and W18, followed by W17. In the case of Zn solubilization, results indicated that seven isolates were able to solubilize ZnO (Figure 3), and the largest zone was reported from W18 (12 mm). Siderophore production was found in 25.92% of the isolates. Results indicated that seven isolates were able to produce siderophores (Figure 3). The largest orange halo zone was reported for W19 (16 mm). Ammonia production was seen in 85% of the isolates. Among them, 26% of the isolates had higher ammonia production, whereas 11% and 48.10% of the isolates had medium and low ammonia production, respectively. In this qualitative measurement, the development of yellow to the pale yellow color indicated low ammonia production, the brown color indicated medium, and the development of dark brown color indicated higher ammonia production. In IAA production, both tryptophan-dependent and independent pathways were reported. Among tryptophan-dependent IAA producers, the highest production was reported for W4 (15.34 µg/mL) followed by G1 (12.22 µg/mL) and W14 (10.01 µg/mL). Among the tryptophan-independent IAA producers, Y6 was the highest IAA producer (15.78 µg/mL), followed by W16 (9.88 µg/mL), and W4 (9.71 µg/mL). However, tryptophan-dependent IAA production was more common (66.67%) than tryptophan-independent IAA production (22.22%).
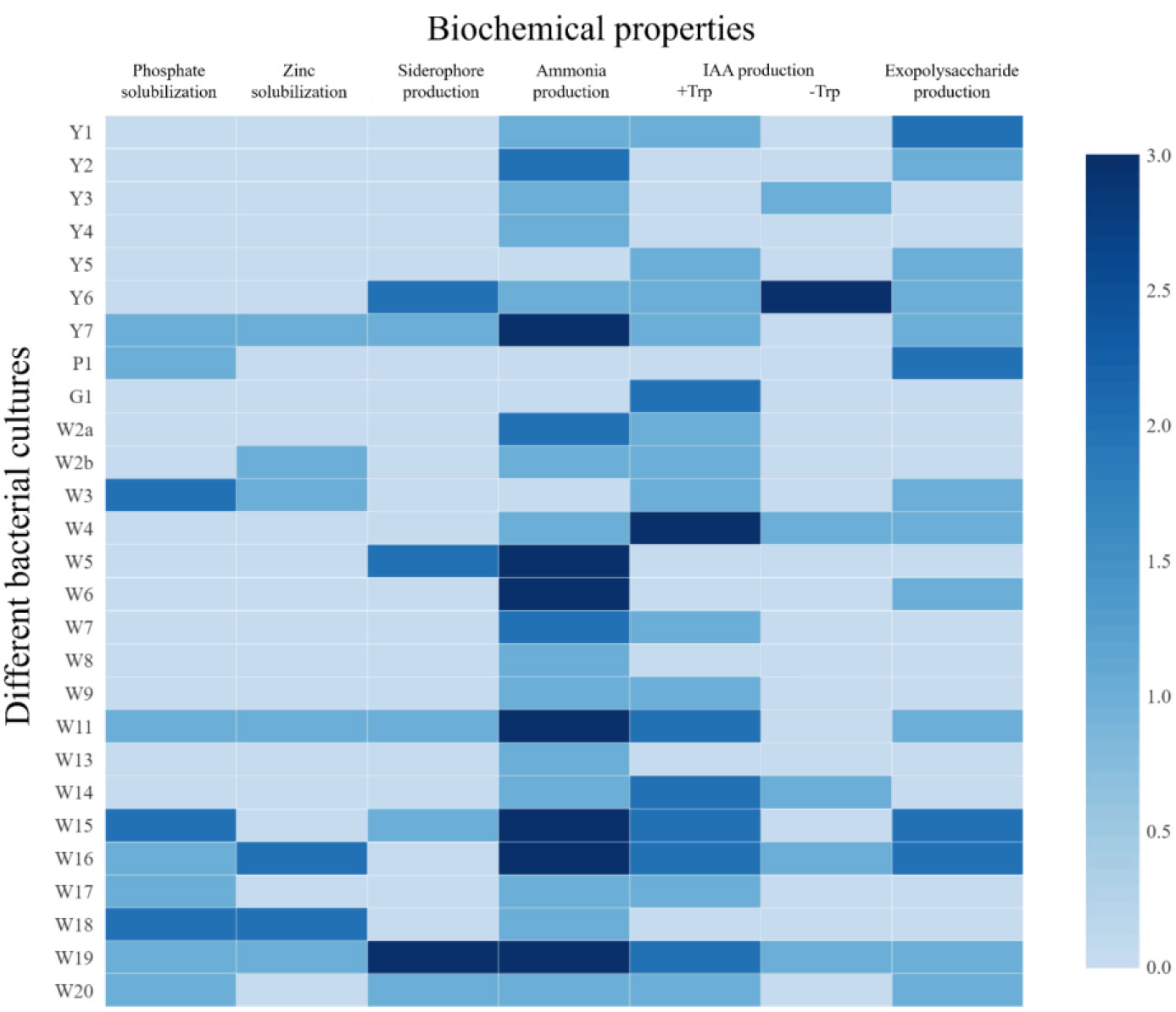
Figure 3 Different plant growth promoting attributes of the bacterial isolates from rice rhizosphere. For PSB, ZSB, and siderophore production: 0 = <10 mm, 1 = 10-12 mm, 2 = 12-14 mm, and 3 = > 14 mm (size of clear zone). For NH3 and EPS production: 0= no production, 1 = low, 2 = medium, and 3 = high. For IAA production (with and without Tryptophan): 0 = <5 µg/mL, 1 = 5-10 µg/mL, 2 = 10-15 µg/mL, and 3 = >15 µg/mL.
3.3. Molecular identification
Based on 16S rRNA sequencing, the five isolates belong to classes Gammaproteobacteria, Bacillota (Firmicutes), and Actinomycetia (Figure 1). Isolates were from three genera (Brevibacterium, Klebsiella, and Bacillus) and four species (Brevibacterium frigoritolerance W11, Klebsiella quasipneumoniae W15, Bacillus aryabhattai W16, Brevibacterium frigoritolerans W19, and Bacillus cereus Y7). The 16S rRNA sequences of these isolates were deposited at NCBI (BankIt) with the accession numbers OM845803, OM780282, MK894243, MN197607, and MN061037.
3.4. In-vivo trials
3.4.1. Effect on salinity-induced germination failure and seedling vigor
3.4.1.1. Effect on germination
Among the non-saline treatments, there was no statistically significant difference (p ≤ 0.05) in germination percent. Under 100 mM NaCl, W19, 2P2, and BTL5 treatments resulted in significantly higher germination percentages as compared to the 100 mM Positive control and to W15. In the case of 200 mM NaCl treatments, all the bacterial inoculated treatments had significantly higher germination than the 200 mM NaCl control (Table 1).
3.4.1.2. Effect on plant height
Under the non-saline conditions, a significantly higher mean root length was recorded with the inoculation of BTL5 (28.70 cm), followed by 2P2 and W19, whereas the lowest was recorded in the control (23.17 cm), followed by W15 (Table 1). Under 100 mM NaCl, BTL5 and 2P2 treatments resulted in significantly higher root lengths, whereas under 200 mM NaCl, BTL5, 2P2, and W19 caused significantly higher mean root lengths. The mean shoot length was significantly higher with BTL5 (12.80 cm) followed by 2P2 and W15 inoculations, whereas the lowest was recorded in the control plants (10.13 cm). Under 100 mM NaCl, BTL5, 2P2, and W15 treatments led to significantly higher shoot length than the control, whereas, under 200mM mM NaCl, BTL5, 2P2, and W19 caused significantly higher mean shoot lengths.
3.4.1.3. Effect on biomass accumulation
Under the non-saline conditions, a significantly higher mean root fresh weight was recorded with the inoculation of W19 (0.18 g) followed by BTL5 as compared to the control (Table 1). Under 100 mM NaCl, W15 and W19 treatments caused significantly higher (0.15g) root fresh weight, followed by BTL5, whereas under 200 mM NaCl, W19, W15, and BTL5 addition resulted in significantly higher mean root fresh weight. A significantly higher mean shoot fresh weight was recorded from the inoculation of BTL5 (0.14 g) as compared to all other non-saline treatments. Under 100 mM NaCl, all inoculated treatments yielded significantly higher shoot fresh weights than the control, whereas, under 200mM mM NaCl, BTL5 inoculation caused significantly higher mean shoot fresh weight (0.06 g) followed by 2P2, W19, and W15.
3.4.1.4. Effect on seedling vigor
Among non-saline treatments, the seedling vigor index was highest in BTL5 (4022.33), and 2P2 (3893.00), followed by other treatments. Among the 100 mM NaCl treatments, 2P2 (2453.43) and BTL5 (2394.00) resulted in significantly higher vigor index. Under 200 mM NaCl, treatments with BTL5, 2P2, and W19 led to significantly higher vigor index (1017.97, 295 986.67, and 844.00, respectively) than the control and W15 treatments (Table 1).
3.4.2. Colonization under different salt concentrations
The study indicated that the rhizospheric and endophytic isolates could colonize the rice root surface, at least to a concentration of 200 mM NaCl (Figure 4). There is a difference in colonization with an increase in salt concentration for the endophytes B. safensis BTL5 and B. haynesii 2P2. BTL5 treated plants were found to have good colonization, uniform in all the cells under salinity stress, whereas in 2P2 more signals were found in root hairs. In the case of both rhizospheric isolates (W15 and W19), colonization could be seen in both saline and non-saline treatments. The bacterial signals were relatively much less in the uninoculated control plants.
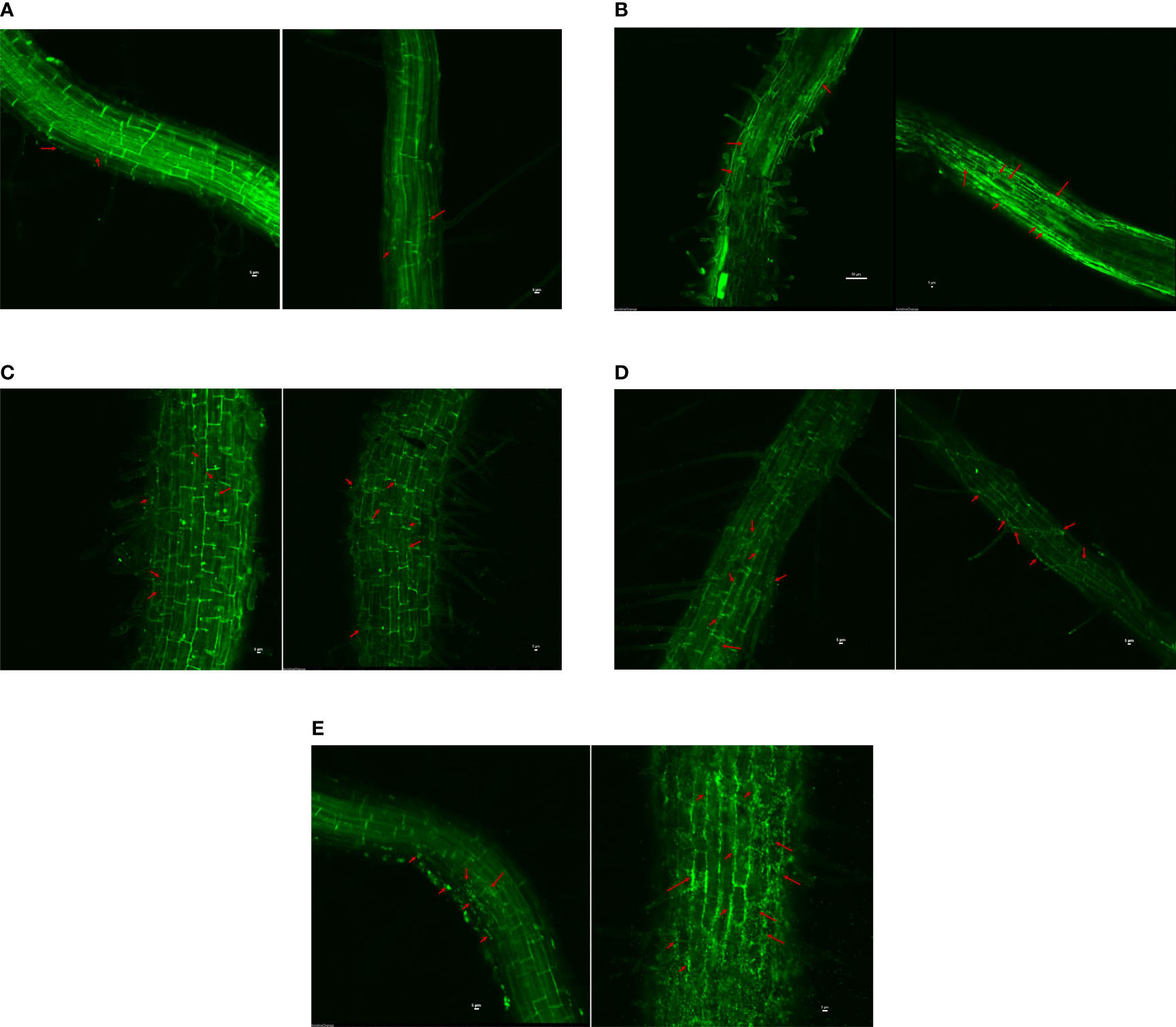
Figure 4 Confocal scanning laser microscopy to assess colonization efficiency of endophytes and rhizospheric bacteria at two salinity levels (with and without 200 mM NaCl), (A) Uninoculated Control, (B) Klebsiella quasipneumoniae W15, (C) Brevibacterium frigoritolerans W19, (D) Bacillus haynesii 2P2, and (E) Bacillus safensis BTL5. Red arrows indicate the colonization of bacteria in the plant roots of respective treatments.
3.4.3. Effect on salinity alleviation in pot trials
In general, elevated salinity retarded the growth parameters (chlorophyll, plant height, and biomass accumulation) and enhanced the reactive oxygen species levels in the plant, as seen from enzymatic and non-enzymatic antioxidant activity (Figures 5–8). Inoculation of both bacterial strains could improve the root-shoot dry weight and reduce oxidative stress. Additionally, the spray of melatonin with inoculants has further enhanced the growth and development in a synergistic manner (Figure 5). The highest root dry weight was recorded for W19+melatonin treatment (8.27 g/pot) and BTL5+melatonin treatment (8.09 g/pot). In the case of shoot dry weight, BTL5+melatonin (10.41 g/pot) and W19 (10.03 g/pot) treatments led to significantly higher values than the other treatments. Application of melatonin increased biomass accumulation, however, inoculation of both BTL5 and W19 resulted in higher biomass accumulation than the melatonin alone. The reduction in growth was clearly visible for the Positive control treatment. Inoculation with microbes and melatonin improved chlorophyll content (Figure 7). Considering total chlorophyll, T5, T6, and T7 led to significantly higher content than the other treatments. Among the microbes applied, W19 could perform better than BTL5. However, plants with BTL5 + melatonin gave better synergistic effects on chlorophyll content than other treatments. The effect could also be seen for shoot length, which was the highest in plants treated with BTL5 + melatonin (64.78 cm) as compared to all other treatments. The plant height was affected by elevated salt, and the microbial and melatonin application helped plants to recover from salinity stress.
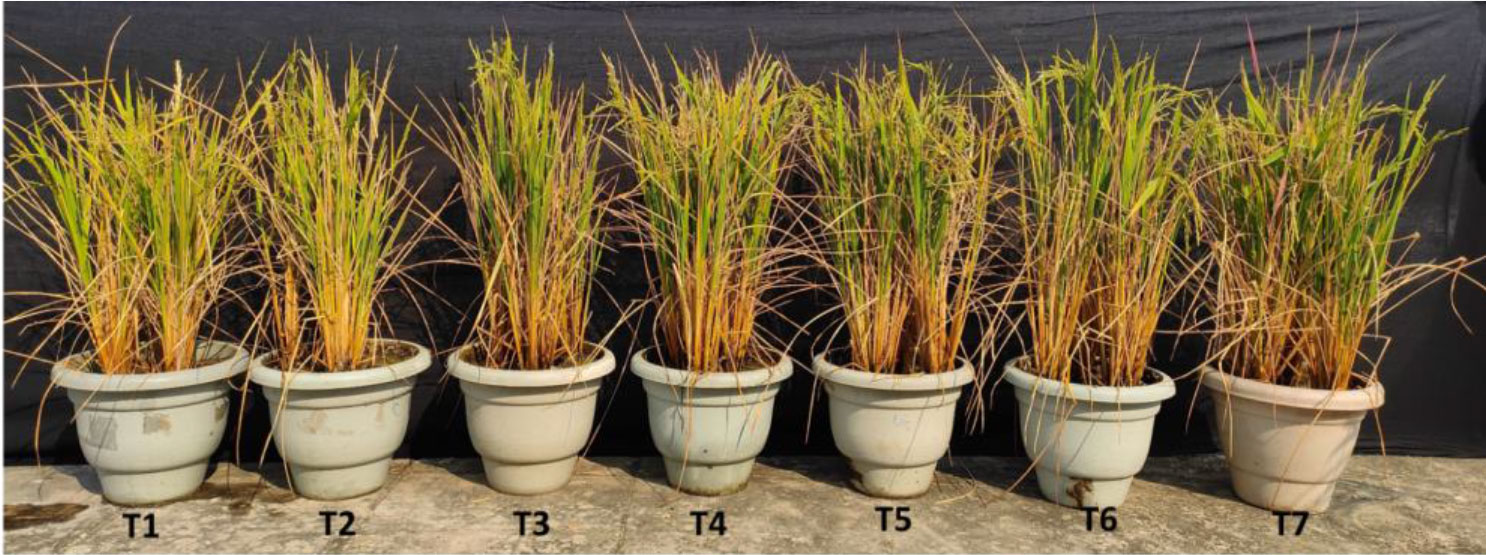
Figure 5 Effect of bacterial inoculation and melatonin in rice cultivar Pusa Basmati 1; T1=Negative control, T2=Positive control, T3 = 200 mM NaCl + Melatonin 20 ppm, T4 = 200 mM NaCl + Bacillus safensis BTL5, T5 = 200 mM NaCl + Brevibacterium frigoritolerans W19, T6 = 200 mM NaCl + Bacillus safensis BTL5 + Melatonin 20 ppm, and T7 = 200 mM NaCl + Brevibacterium frigoritolerans W19 + Melatonin 20 ppm. Error bars represent the standard deviation. The treatments with different letters are significantly different as determined by DMRT (p ≤ 0.05).
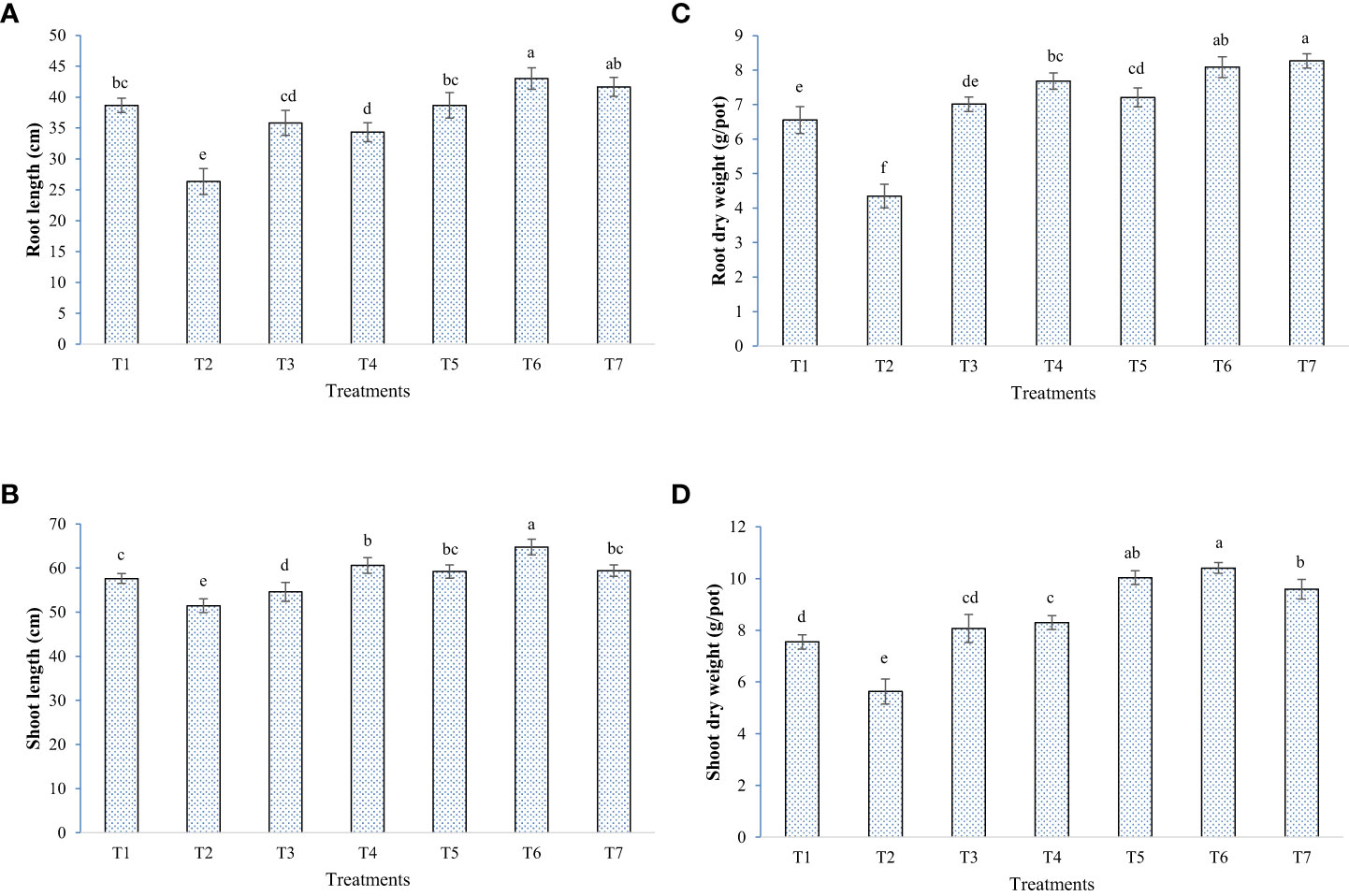
Figure 6 Effect of bacterial inoculation and melatonin in rice cultivar Pusa Basmati 1 (PB1), (A) Root length, (B) Shoot length, (C) Root dry weight, and (D) Shoot dry weight; T1=Negative control, T2=Positive control, T3 = 200 mM NaCl + Melatonin 20 ppm, T4 = 200 mM NaCl + Bacillus safensis BTL5, T5 = 200 mM NaCl + Brevibacterium frigoritolerans W19, T6 = 200 mM NaCl + Bacillus safensis BTL5 + Melatonin 20 ppm, and T7 = 200 mM NaCl + Brevibacterium frigoritolerans W19 + Melatonin 20 ppm. Error bars represent the standard deviation. The treatments with different letters are significantly different as determined by DMRT (p ≤ 0.05).
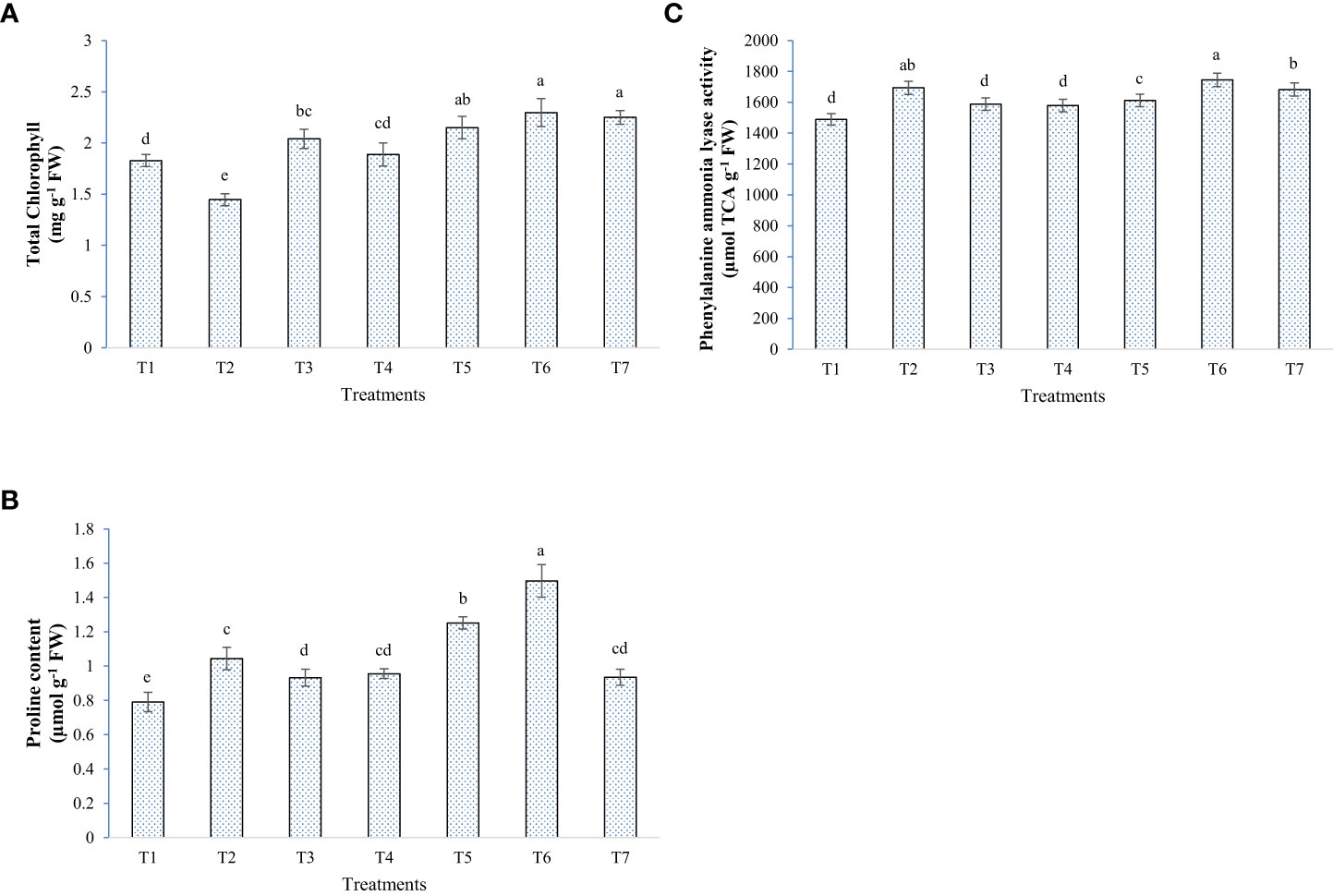
Figure 7 Effect of bacterial inoculation and melatonin in rice cultivar Pusa Basmati 1 (PB1), (A) Total chlorophyll content, (B) Proline content, and (C) Phenylalanine ammonia-lyase activity; T1=Negative control, T2=Positive control, T3 = 200 mM NaCl + Melatonin 20 ppm, T4 = 200 mM NaCl + Bacillus safensis BTL5, T5 = 200 mM NaCl + Brevibacterium frigoritolerans W19, T6 = 200 mM NaCl + Bacillus safensis BTL5 + Melatonin 20 ppm, and T7 = 200 mM NaCl + Brevibacterium frigoritolerans W19 + Melatonin 20 ppm. Error bars represent the standard deviation. The treatments with different letters are significantly different as determined by DMRT (p ≤ 0.05).
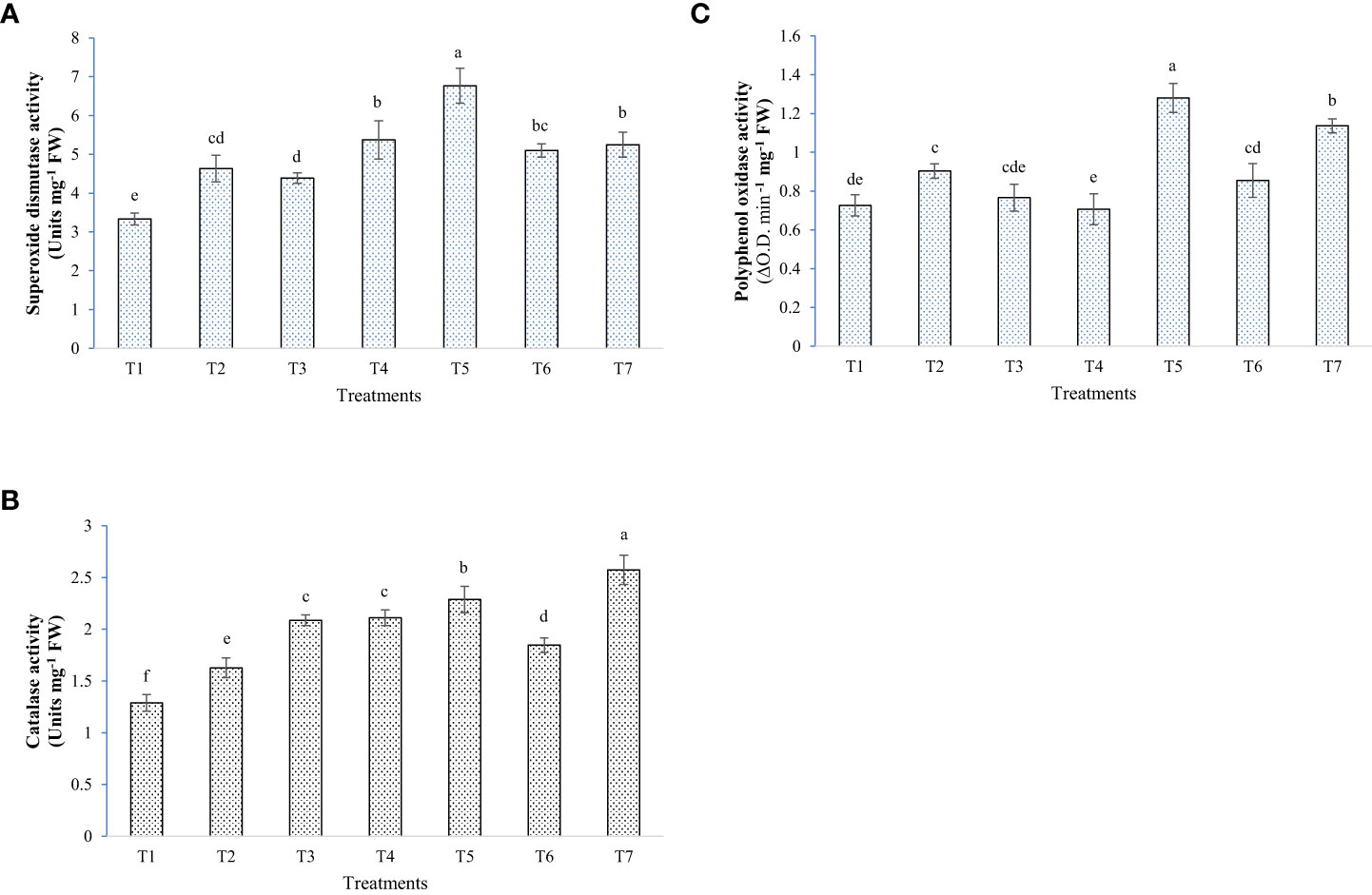
Figure 8 Effect of bacterial inoculation and melatonin in enzymatic antioxidants activities of rice, (A) Superoxide dismutase activity, (B) Catalase activity, and (C) Polyphenol oxidase activity; T1=Negative control, T2=Positive control, T3 = 200 mM NaCl + Melatonin 20 ppm, T4 = 200 mM NaCl + Bacillus safensis BTL5, T5 = 200 mM NaCl + Brevibacterium frigoritolerans W19, T6 = 200 mM NaCl + Bacillus safensis BTL5 + Melatonin 20 ppm, and T7 = 200 mM NaCl + Brevibacterium frigoritolerans W19 + Melatonin 20 ppm. Error bars represent the standard deviation. The treatments with different letters are significantly different as determined by DMRT (p ≤ 0.05).
The application of microbes and melatonin had a mixed effect on the levels of the antioxidant systems of the rice plants under elevated salinity. Some of the treatments resulted in higher ROS scavenging activity than the Positive control, in opposition to some other treatments. PAL, CAT, and proline content were higher in the plants treated with microbes + melatonin, whereas in the case of SOD and PPO, plants with W19 alone had significantly higher enzyme activity (Figures 7, 8). In the general comparative performance of microbes with melatonin, BTL5 and melatonin application was a more synergistic treatment than W19 + melatonin. However, both inoculation of microbes and application of melatonin improved plant growth and performance under high salt concentrations.
The results of gene expression studies showed that inoculation of bacterial strains and melatonin up-regulated salinity stress tolerance gene taken under study (Figures 9A–D). The expression of SOD1 gene was enhanced by seven folds in T5 and T6, as compared to the negative control. In CATa gene, expression was significantly enhanced by almost five times in T4, T5 and T7 against negative control. In T6, the expression was lower than the positive control. The PAL1 expression was highest in T7 followed by T5 against negative control. The expression was lower than positive control in T3, T4, and T6. In NHX1, eight times up-regulation was found in T7 plants followed by T4 and T6, as compared to the negative control.
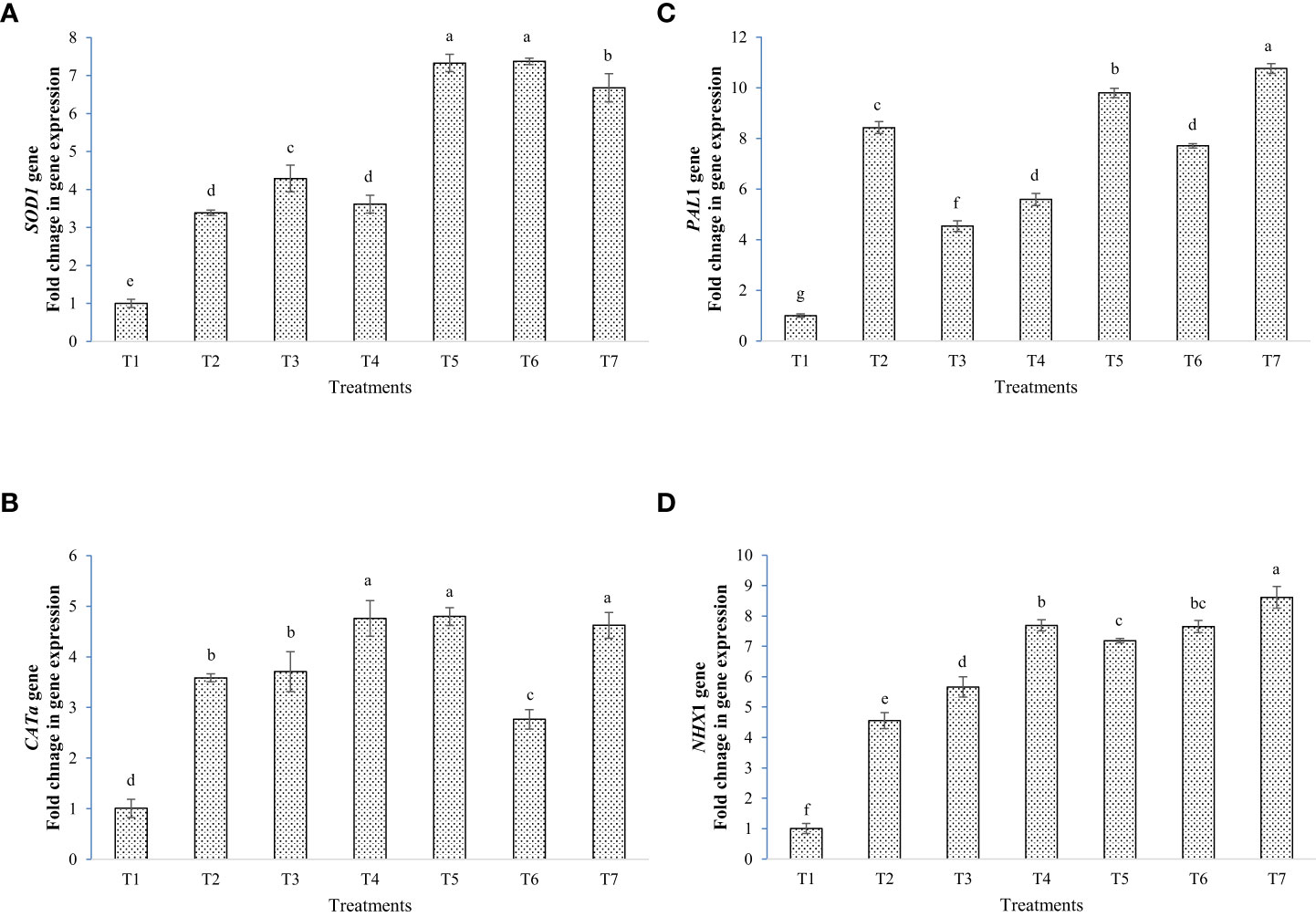
Figure 9 Fold changes in gene expression by the application of bacterial inoculants and melatonin, (A) SOD1, (B) CATa, (C) PAL1, and (D) NHX1; T1=Negative control, T2=Positive control, T3 = 200 mM NaCl + Melatonin 20 ppm, T4 = 200 mM NaCl + Bacillus safensis BTL5, T5 = 200 mM NaCl + Brevibacterium frigoritolerans W19, T6 = 200 mM NaCl + Bacillus safensis BTL5 + Melatonin 20 ppm, and T7 = 200 mM NaCl + Brevibacterium frigoritolerans W19 + Melatonin 20 ppm. Error bars represent the standard deviation. The treatments with different letters are significantly different as determined by DMRT (p ≤ 0.05).
In the principal component analysis biplot of growth attributes, the first two principal components accounted for 98.6% of the variation (90.5 and 8.1%, respectively) in 7-dimensional space (Figure 10). In the PCA biplot for ROS scavenging systems, the first two principal components accounted for 100% of the variation (95.6 and 4.4%, respectively) in 7-dimensional space (Figure 11). In both PCA biplots, treatments with melatonin and bacterial inoculation were found to have a greater impact on growth and ROS scavenging under elevated salinity. In PCA of growth attributes, T7 (W19+Mel), T6 (2P2+Mel), and T5 (W19) were found to have higher impacts, similar to T1 (Negative control). This also indicates that albeit melatonin alone has effects on plant growth attributes, adding to the microbial inoculants resulting in a synergistic effect. In the case of PCA for ROS scavengers, the induced tolerance was higher for the bacterial inoculation alone, followed by an ensemble of bacteria plus melatonin.
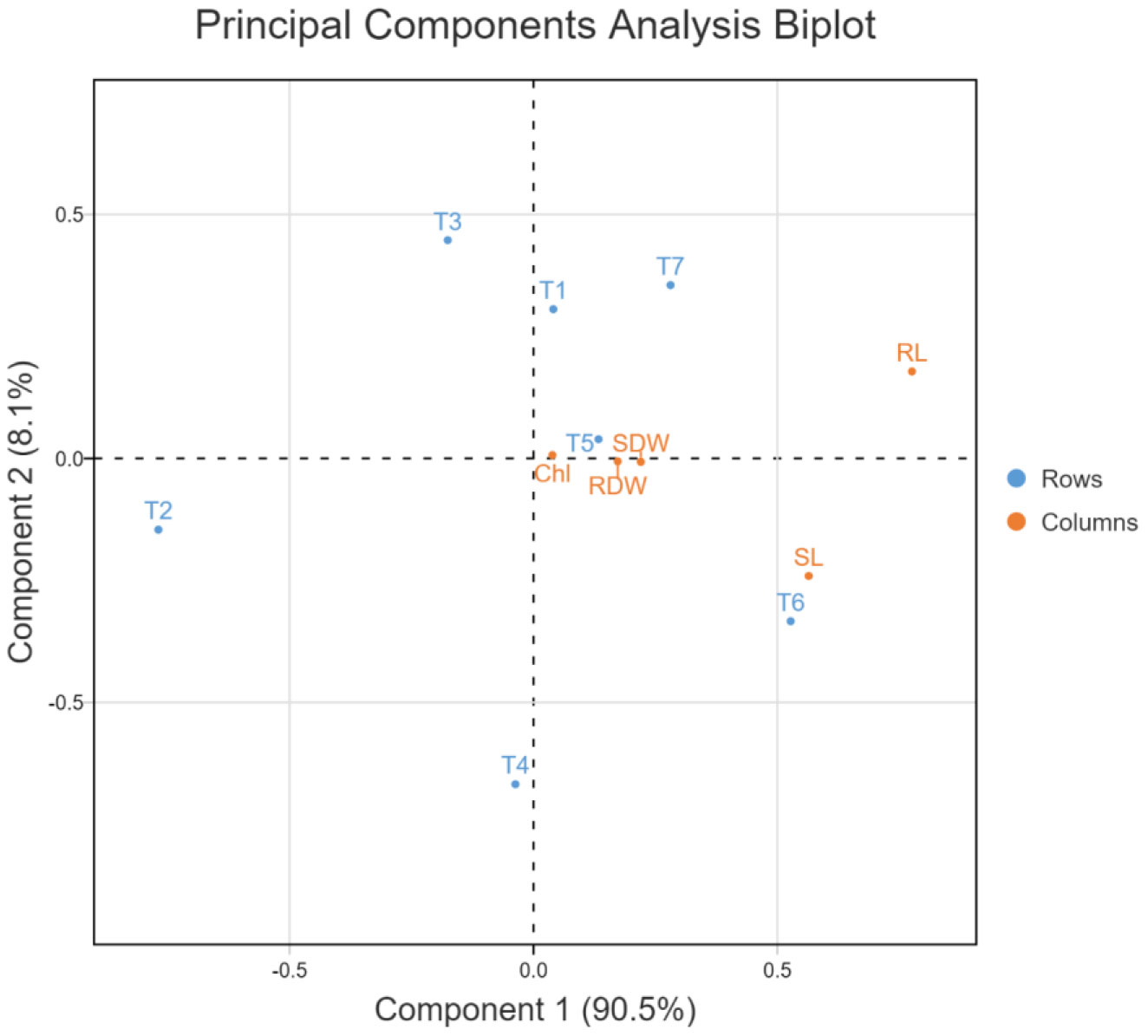
Figure 10 Principal component analysis biplot for growth attributes of rice plant as affected by bacterial inoculation and melatonin application under elevated salinity, Chl, Total chlorophyll; RL, Root length; SL, Shoot length; SDW, Shoot dry weight; RDW, Root dry weight.
4. Discussion
In the present study, bacterial strains were isolated, screened, and tested for their capability to support plant growth and development under salinity. Early seedling establishment, colonization efficacy, and synergism with the growth inducer compound melatonin were studied by two in planta trials.
The rhizosphere bacteria isolated in this study were found to have high salt tolerance (Figure 2), a fact that could be connected to their habitat. Saline soils harbor microbes that could survive under high salinity and perform plant growth-promoting activities under such conditions (Yadav and Saxena, 2018), and the crop rhizosphere is a rich habitat to search for trait-specific, salt-tolerant bacterial strains (Ling et al., 2022). High EPS production by these microbes could also contribute to salt tolerance (Figure 3) (Balsanelli et al., 2014; Singh et al., 2022), as EPS keeps a physical barrier between plant roots and the hypersaline environment (Singh and Jha, 2016; Sagar et al., 2022). These rhizospheric isolates were found to harbor multiple plant growth-promoting traits that can contribute to crop nutrition and productivity (Figure 3). The results are in line with the finding of Ling et al. (2022), which indicates that the rhizosphere is rich in microbes with specific functions. Microbes supplementing zinc and iron nutrition could help with nutrient fortification in grains (Singh et al., 2017) and help fight nutrient deficiency. The rhizospheric isolates were also found to have tryptophan-dependent as well as independent pathways for indole-3-acetic acid production (Figure 3). The presence of phytohormone-producing bacteria in the rice rhizosphere has been reported by earlier workers (Liu et al., 2021a). However, in the present study, bacteria with the tryptophan-dependent pathway were predominant.
The five isolates from this study belong to three genera: Brevibacterium, Klebsiella, and Bacillus (Figure 1). Bacillus is one of the very promising growth-promoting and salt stress alleviating bacterial agents reported in different host plants (Liu et al., 2021a; Sahu et al., 2021b). Brevibacterium frigoritolerance is reported as a salt stress alleviating bacteria from the wheat rhizosphere (Huang et al., 2022). Ibrahim and Ikhajiagbe (2021) reported Klebsiella and Bacillus as plant growth-promoting bacteria (PGPB) in rice. This kind of bacterial diversity is seen in the rhizospheres of different crops and has been linked to stress-relieving traits. It could also hold potential inoculants for saline soils.
Salinity reduced seedling emergence and growth, and inoculation of bacterial strains alleviated the effects of salinity (Table 1). This could be due to the reduction of Na+ toxicity by maintaining osmotic and ionic balance along with the reduction of oxidative stress. Endophytic and rhizospheric microbes are reported to improve germination and early seedling growth under salinity (Bai et al., 2017). These applied bacterial strains could be useful in a good plant stand at the nursery level.
Plant-microbe interactions are mediated by a complex cross-talk for successful colonization (Lahrmann et al., 2013). The present study showed good colonization of rhizospheric and endophytic bacteria in normal as well as under elevated salinity (Figure 4). The microbial association is decided by plant requirements (Gross, 2022). Our results evidence such principle, since the endophytic community was enriched with elevated salinity. A good amount of EPS production could also be one of the determinants contributing to the colonization competence of the rhizospheric and endophytic bacteria in the present study (Singh et al., 2022). EPS help bacteria adhesion to soil particles and plant roots while protecting microbes from the unfavorable environment. Good colonization could contribute to the stable in planta effects and is very much required for the popularization of microbial inoculants among the farming communities. Microbes with a wider host range could add to the utility of an inoculant for field application (Sahu et al., 2021b). Thus, microbes with high colonization competence to a wide range of crops at higher salinity can have high commercial value in the inoculant market. Colonization under elevated salinity could be an additional useful trait for the success of inoculation as it would further benefit the plants with added growth-promoting effects and increase nutrient uptake and tolerance under abiotic and biotic adversities. Jacquiod et al. (2022) recently hypothesized that continuous artificial shaping of microbiota in a plant can bring stabilization of microbial community structure that may be heritable to further generations. This gives experimental proof to the fact that inoculation of microbes with great plant growth-promoting and stress alleviating potential could substantially strengthen the plant function even for further generations.
In the pot trial, growth and biomass accumulation were improved by Brevibacterium frigoritolerans W19, Bacillus safensis BTL5, and melatonin (Figure 5). Since the overall plant function is a result of interaction between plants’ own genome and the plant-associated microbiome (Vandenkoornhuyse et al., 2015), the effects of the growth inducer molecule (melatonin) on the associated microbiome could be one of the crucial determinants in plant productivity under stress. Though some leads have been worked out in the case of AM fungi, where improvement in AM fungi colonization has been found due to exogenous melatonin application (Zhang et al., 2020), the area is still under-explored. Improvement in plant height and biomass accumulation was recorded from rice plants inoculated with bacteria and melatonin (Figure 6). These bacterial strains also produced IAA, which is reported by Kumar et al. (2021) to be a key inducer of seedling growth. The bacteria trigger salinity stress tolerance mechanisms of the plant (Sahu et al., 2021a). Bacillus safensis BTL5 had nutrient solubilizing and phytohormone-producing capability, which might have contributed to the salinity stress alleviation of rice plants and helped in higher nutrient uptake followed by improved utilization. The modulation of phytohormones and reduced osmotic/oxidative stress also contributed to the improved functioning of photosynthetic machinery (as evident from Figure 7A). Higher photosynthesis results in higher biomass accumulation, as seen in Figure 6C, D. Improvement in growth under salinity is crucially dependent on effective alleviation of oxidative and osmotic stress (Altaf et al., 2021). Figure 8 shows the higher accumulation of enzymatic antioxidants from the inoculation of bacteria and melatonin. This could be due to induced systemic tolerance. Bacterial agents induce the over-expression of Na+/K+ antiporters to lock excess Na+ ions in the vacuoles along with the induction of aquaporins (Sahu et al., 2021b). This also maintains water homeostasis (Molina-Montenegro et al., 2020), which could be a reason for growth and development under high salinity.
In our previous study, Bacillus safensis BTL5 was reported to reduce salinity stress by increasing nutrient uptake, maintaining Na+/K+ balance, alleviating oxidative stress, and activating genes for osmotic adjustment (Sahu et al., 2021b). Together, all these effects would be responsible for the improved growth and development of rice. Induced systemic tolerance by inoculated bacteria and melatonin could be a key reason behind the induction of the ROS scavenging systems in rice (Vaishnav et al., 2020; Sahu et al., 2021b). In the present study, both proline-induced osmoregulation and maintenance of water homeostasis might play a role in maintaining the water status of salt-affected plants inoculated with Bacillus safensis BTL5+melatonin and Brevibacterium frigoritolerans W19 (Hayat et al., 2012; Shafi et al., 2019) (Figures 7B, C). Reduction in proline and other ROS scavenger’s content as observed in some bacterial and melatonin treatments is also a beneficial trait, which may possibly indicate that plants are experiencing lowered salt stress (Bhise et al., 2017).
The results of oxidative stress scavengers’ activity showed that melatonin improved certain parameters at a higher rate with one bacterial inoculant over the other, indicating preferential induction/synergism of some microbes with melatonin. Melatonin has been reported to regulate rhizogenesis and fortify reactive oxygen species (ROS) scavenging mechanisms, root architecture, photosynthetic capacity, and ionic balance of plants under salinity stress (Yan et al., 2020; Altaf et al., 2021).
The gene expression studies showed up-regulation of antioxidant genes by the application of melatonin with bacterial strains. Here, synergism was seen in the expression of SOD1, CATa, and PAL1 expression. In addition, it indicated differential activation of salt tolerance genes by the inoculation of BTL5 and W19. Previous literature validated microbe-mediated induction of these genes for improving salinity mitigation in plants (Molina-Montenegro et al., 2020). The expression of NHX1 (Na+/H+ antiporter) gene was highest in all inoculated plants, and in the case of W19 + melatonin, the expression was further enhanced. This indicates the synergistic interaction of melatonin with W19. This upregulation in antiporter gene expression would be helping plants in the selective uptake of potassium and exclusion of excess sodium ions from plants’ system (Barragán et al., 2012). Up-regulation of the NHX1 gene by bacterial inoculation is reported earlier in our previous studies (Sahu et al., 2021b). The melatonin has also been earlier reported to up-regulate membrane transporters genes for salinity mitigation (Liu et al., 2020). In the case of CATa gene, the BTL5 + melatonin treatment was having lesser expression than in BTL5 alone. This could be due to the activation of other catalase genes than CATa, or the activation of other antioxidant machinery than CATa. In the expression of PAL1, W19 and W19+melatonin was having higher expression than the positive control. Both BTL5 and W19 showed synergism with melatonin. Reduction in expression of some of the inoculated treatments than positive control is similar to the enzyme activity results, indicating reduced stress level in inoculated plants. SOD1 gene expression was high in W19 and BTL5 + melatonin inoculated plants as seen from enzyme activity studies where W19 got a higher accumulation of SOD. In gene expression, BTL5 showed synergism with melatonin. Such up-regulation of salt stress-responsive genes in rice by melatonin was earlier reported by Li et al. (2017).
Treatment with melatonin (T3) in rice was found to lead to higher plant growth and biomass compared with control plants under salinity. This increment in the growth and biomass accumulation could be related to the enhancement of the quantum efficiency of photosystem-II and thus the net photosynthesis rate by the addition of melatonin, as reported by Han et al. (2017) in rice. There have been a few reports on the improvement in plant performance after the combined application of microbes and melatonin (Liu et al., 2021b), which support our results that show synergistic effects with bacterial inoculants and melatonin. Yan et al. (2020) reported that pre-treatment with melatonin reduced membrane damage and oxidative stress in rice under salinity. Thus, the stage of application, as well as the concentration are crucial for the melatonin role. The present study reinforces the importance of melatonin in plants’ tolerance to salinity and establishes the compatibility of two bacterial inoculants with 20 ppm melatonin. Further studies are required to decipher the main determinants of synergism for the different bacterial inoculants.
The PCA biplots for growth attributes and ROS scavenging systems indicated the synergism between the bacterial inoculation and melatonin treatment (Figures 9, 11). ROS scavenging is more impactful with the bacterial inoculation, which could be explained by the rhizospheric and endophytic bacterial roles in the reduction of the impact of abiotic stress by induction of systemic tolerance (Vaishnav et al., 2020; Sahu et al., 2021b). However, in the PCA of growth attributes, both melatonin and bacterial inoculation had higher impacts, which could be due to improvement in root system architecture and a reduction in sodium toxicity (Altaf et al., 2021; Verma et al., 2021).
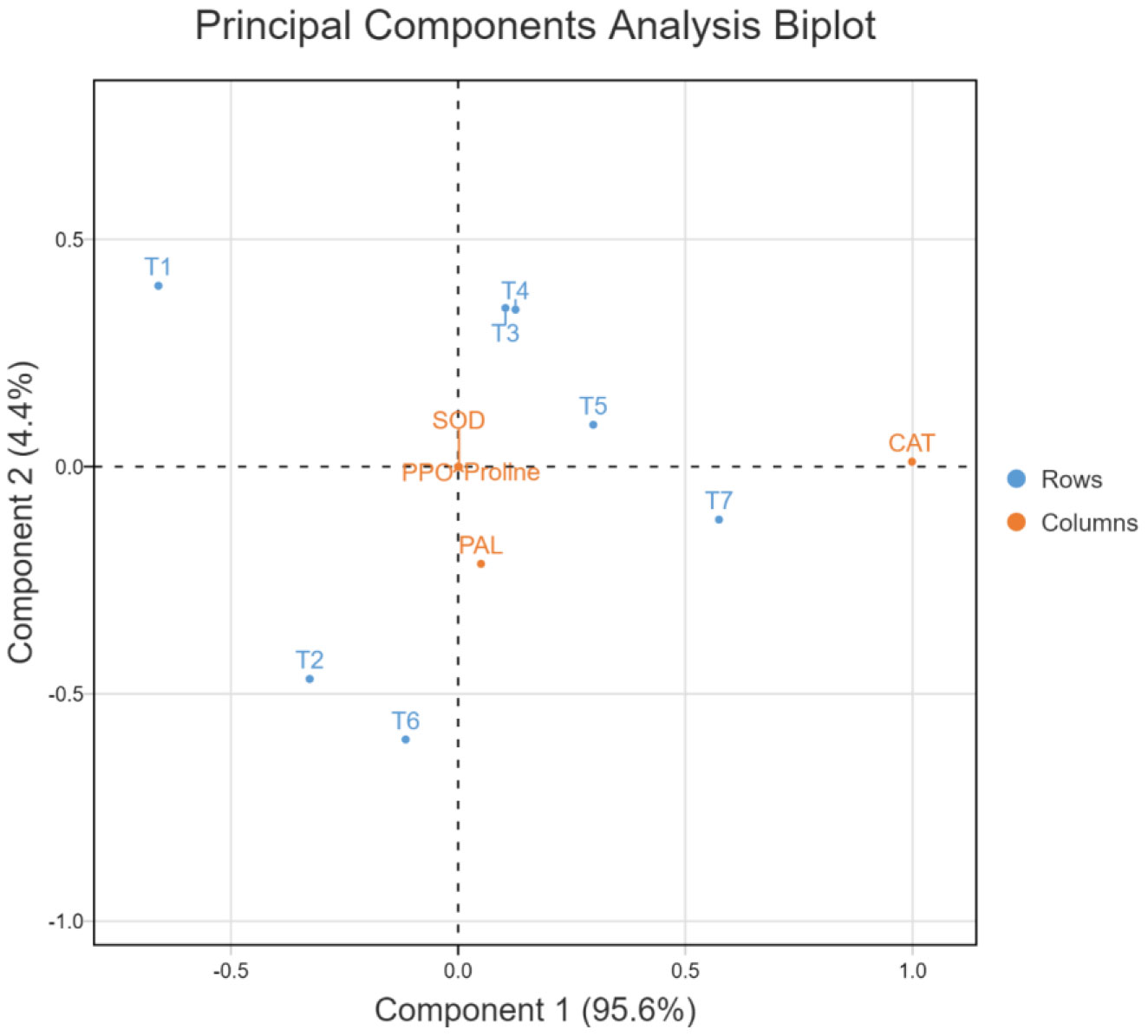
Figure 11 Principal component analysis biplot for ROS scavenging systems of rice plant as affected by bacterial inoculation and melatonin application under elevated salinity, SOD, Superoxide dismutase; CAT, Catalase; PPO, Polyphenol oxidase; PAL, Phenylalanine ammonia-lyase; PRL, Proline.
5. Conclusion and future prospects
Exploring microbes with PGP traits and the ability of root colonization under high salinity may help in initiating symbiosis with the plant and protecting it against salinity stress. Also, strains having the ability of increased colonization under salinity stress could be a useful tool for successful field inoculation. Inoculation of Brevibacterium frigoritolerans W19 and Bacillus safensis BTL5 resulted in significant positive effects on rice, but there was a difference in colonization with the increase in salt concentration, Bacillus safensis BTL5 was found to have good colonization under elevated salinity. Though melatonin addition showed synergism with rhizospheric and endophytic bacterial inoculants, there was a difference in the induction of tolerance. Overall, exploring microbes with good colonization potential, stress alleviating attributes, and synergism with other growth-inducing compounds could be fundamental in making successful inoculants. The improved induction of plant tolerance with microbial inoculants and growth inducers should be further investigated.
Data availability statement
The datasets presented in this study can be found in online repositories. The names of the repository/repositories and accession number(s) can be found in the article/Supplementary Material.
Author contributions
RT, RS, PS conceived the idea and designed the experiments. AG performed the experiments. AG, PS and AS analyzed the data. RT, PS, AS and RS wrote and edited the manuscript. All authors contributed to the article and approved the submitted version.
Acknowledgments
The authors gratefully acknowledge the ICAR-NBAIM Mau, ICAR-IISS Mau, and Amity Institute of Biotechnology, Amity University Uttar Pradesh, Lucknow Campus, Lucknow for providing the facilities to conduct the research and other support received for carrying out the experimentations. This manuscript is a part of Ph.D. research of Amrita Gupta.
Conflict of interest
The authors declare that the research was conducted in the absence of any commercial or financial relationships that could be construed as a potential conflict of interest.
Publisher’s note
All claims expressed in this article are solely those of the authors and do not necessarily represent those of their affiliated organizations, or those of the publisher, the editors and the reviewers. Any product that may be evaluated in this article, or claim that may be made by its manufacturer, is not guaranteed or endorsed by the publisher.
Supplementary material
The Supplementary Material for this article can be found online at: https://www.frontiersin.org/articles/10.3389/fpls.2022.1060287/full#supplementary-material
References
Altaf, M. A., Shahid, R., Ren, M. X., Altaf, M. M., Khan, L. U., Shahid, S., et al. (2021). Melatonin alleviates salt damage in tomato seedling: A root architecture system, photosynthetic capacity, ion homeostasis, and antioxidant enzymes analysis. Sci. Hortic. 285, 110145. doi: 10.1016/j.scienta.2021.110145
Álvarez, C., Navarro, J. A., Molina-Heredia, F. P., Mariscal, V. (2020). Endophytic colonization of rice (Oryza sativa l.) by the symbiotic strain Nostoc punctiforme PCC 73102. Mol. Plant-Microbe Interact. 33 (8), 1040–1045. doi: 10.1094/MPMI-01-20-0015-SC
Bai, R., Chen, L., Zhang, X., Wei, G., Wei, C. (2017). Effect of salinity and soil temperature on the growth and physiology of drip-irrigated rice seedlings. Arch. Agron. Soil Sci. 63 (4), 513–524. doi: 10.1080/03650340.2016.1223842
Bakhshandeh, E., Gholamhosseini, M., Yaghoubian, Y., Pirdashti, H. (2020). Plant growth promoting microorganisms can improve germination, seedling growth and potassium uptake of soybean under drought and salt stress. Plant Growth Regul. 90 (1), 123–136. doi: 10.1007/s10725-019-00556-5
Balsanelli, E., Baura, V. A., Pedrosa, F. D., Souza, E. M., Monteiro, R. A. (2014). Exopolysaccharide biosynthesis enables mature biofilm formation on abiotic surfaces by Herbaspirillum seropedicae. PloS One 9 (10), e110392. doi: 10.1371/journal.pone.0110392
Barelli, L., Moreira, C. C., Bidochka, M. J. (2018). Initial stages of endophytic colonization by Metarhizium involves rhizoplane colonization. Microbiology 164 (12), 1531–1540. doi: 10.1099/mic.0.000729
Barragán, V., Leidi, E. O., Andrés, Z., Rubio, L., De Luca, A., Fernández, J. A., et al. (2012). Ion exchangers NHX1 and NHX2 mediate active potassium uptake into vacuoles to regulate cell turgor and stomatal function in arabidopsis. Plant Cell 24 (3), 1127–1142. doi: 10.1105/tpc.111.095273
Bhise, K. K., Bhagwat, P. K., Dandge, P. B. (2017). Synergistic effect of Chryseobacterium gleum sp. SUK with ACC deaminase activity in alleviation of salt stress and plant growth promotion in Triticum aestivum l. 3. Biotech 7 (2), 1–3. doi: 10.1007/s13205-017-0739-0
Cappuccino, J. G., Sherman, N. (1992). Biochemical activities of microorganisms. microbiology, a laboratory manual Vol. 76 (California, USA: The Benjamin/Cummings Publishing Co).
Compant, S., Reiter, B., Sessitsch, A., Nowak, J., Clément, C., Ait Barka, E. (2005). Endophytic colonization of Vitis vinifera l. by plant growth-promoting bacterium Burkholderia sp. strain PsJN. Appl. Environ. Microbiol. 71 (4), 1685–1693. doi: 10.1128/AEM.71.4.1685-1693.2005
Fasim, F., Ahmed, N., Parsons, R., Gadd, G. M. (2002). Solubilization of zinc salts by a bacterium isolated from the air environment of a tannery. FEMS Microbiol. Lett. 213 (1), 1–6. doi: 10.1111/j.1574-6968.2002.tb11277.x
Gross, M. (2022). How plants grow their microbiome. Curr. Biol. 32 (3), 97–100. doi: 10.1016/j.cub.2022.01.044
Hakim, M. A., Juraimi, A. S., Begum, M., Hanafi, M. M., Ismail, M. R., Selamat, A. (2010). Effect of salt stress on germination and early seedling growth of rice (Oryza sativa l.). Afr. J. Biotechnol. 9 (13), 1911–1918. doi: 10.5897/AJB09.1526
Han, Q. H., Huang, B., Ding, C. B., Zhang, Z. W., Chen, Y. E., Hu, C., et al. (2017). Effects of melatonin on anti-oxidative systems and photosystem II in cold-stressed rice seedlings. Front. Plant Sci. 8, 785. doi: 10.3389/fpls.2017.00785
Havir, E. A. (1987). L-phenylalanine ammonia-lyase from soybean cell suspension cultures. Meth. Enzymol. 142, 248–253. doi: 10.1016/S0076-6879(87)42034-X
Hayat, S., Hayat, Q., Alyemeni, M. N., Wani, A. S., Pichtel, J., Ahmad, A. (2012). Role of proline under changing environments: a review. Plant Signal Behav. 7, 1456–1466. doi: 10.4161/psb.21949
Huang, Z., Wang, C., Feng, Q., Liou, R. M., Lin, Y. F., Qiao, J., et al. (2022). The mechanisms of sodium chloride stress mitigation by salt-tolerant plant growth promoting rhizobacteria in wheat. Agronomy 12 (3), 543. doi: 10.3390/agronomy12030543
Ibrahim, M. S., Ikhajiagbe, B. (2021). The growth response of rice (Oryza sativa l. var. FARO 44) in vitro after inoculation with bacterial isolates from a typical ferruginous ultisol. Bull. Natl. Res. Cent. 45 (1), 1–20. doi: 10.1186/s42269-021-00528-8
Jacquiod, S., Spor, A., Wei, S., Munkager, V., Bru, D., Sørensen, S. J., et al. (2022). Artificial selection of stable rhizosphere microbiota leads to heritable plant phenotype changes. Ecol. Lett. 25 (1), 189–201. doi: 10.1111/ele.13916
Jamil, M., Bashir, S., Anwar, S., Bibi, S., Bangash, A., Ullah, F., et al. (2012). Effect of salinity on physiological and biochemical characteristics of different varieties of rice. Pak. J. Bot. 44 (1), 7–13.
Jiménez-Mejía, R., Medina-Estrada, R. I., Carballar-Hernández, S., Orozco-Mosqueda, M., Santoyo, G., Loeza-Lara, P. D. (2022). Teamwork to survive in hostile soils: Use of plant growth-promoting bacteria to ameliorate soil salinity stress in crops. Microorganisms 10 (1), 150. doi: 10.3390/microorganisms10010150
Kashyap, B. K., Ara, R., Singh, A., Kastwar, M., Aaysha, S., Mathew, J., et al. (2019). “Halotolerant PGPR bacteria: Amelioration for salinity stress,” in Microbial interventions in agriculture and environment. Eds. Singh, D., Gupta, V., Prabha, R. (Singapore: Springer), 509–530.
Khan, M. A., Asaf, S., Khan, A. L., Adhikari, A., Jan, R., Ali, S., et al. (2020). Plant growth-promoting endophytic bacteria augment growth and salinity tolerance in rice plants. Plant Biol. 22 (5), 850–862. doi: 10.1111/plb.13124
Kumar, A., Singh, S., Mukherjee, A., Rastogi, R. P., Verma, J. P. (2021). Salt-tolerant plant growth-promoting Bacillus pumilus strain JPVS11 to enhance plant growth attributes of rice and improve soil health under salinity stress. Microbiol. Res. 242, 126616. doi: 10.1016/j.micres.2020.126616
Lahrmann, U., Ding, Y., Banhara, A., Rath, M., Hajirezaei, M. R., Döhlemann, S., et al. (2013). Host-related metabolic cues affect colonization strategies of a root endophyte. Proc. Natl. Acad. Sci. U.S.A. 110, 13965–13970. doi: 10.1073/pnas.1301653110
Liang, C., Li, A., Yu, H., Li, W., Liang, C., Guo, S., et al. (2017). Melatonin regulates root architecture by modulating auxin response in rice. Front. Plant Sci. 8, 134. doi: 10.3389/fpls.2017.00134
Ling, N., Wang, T., Kuzyakov, Y. (2022). Rhizosphere bacteriome structure and functions. Nat. Comm. 13 (1), 1–3. doi: 10.1038/s41467-022-28448-9
Liu, L., Li, D., Ma, Y., Shen, H., Zhao, S., Wang, Y. (2021b). Combined application of arbuscular mycorrhizal fungi and exogenous melatonin alleviates drought stress and improves plant growth in tobacco seedlings. J. Plant Growth Regul. 40 (3), 1074–1087. doi: 10.1007/s00344-020-10165-6
Liu, J., Shabala, S., Zhang, J., Ma, G., Chen, D., Shabala, L., et al. (2020). Melatonin improves rice salinity stress tolerance by NADPH oxidase-dependent control of the plasma membrane k+ transporters and k+ homeostasis. Plant. Cell Environ. 43 (11), 2591–2605. doi: 10.1111/pce.13759
Liu, Z., Zhang, X., Li, L., Xu, N., Hu, Y., Wang, C., et al. (2021a). Isolation and characterization of three plant growth-promoting rhizobacteria for growth enhancement of rice seedling. J. Plant Growth Regul. 41, 1382–1393. doi: 10.1007/s00344-021-10393-4
Livak, K. J., Schmittgen, T. D. (2001). Analysis of relative gene expression data using real-time quantitative PCR and the 2(-delta C(T)) method. Methods 25, 402–408. doi: 10.1006/meth.2001.1262
Li, X., Yu, B., Cui, Y., Yin, Y. (2017). Melatonin application confers enhanced salt tolerance by regulating na+ and cl– accumulation in rice. Plant Growth Regul. 83 (3), 441–454. doi: 10.1007/s10725-017-0310-3
Molina-Montenegro, M. A., Acuña-Rodríguez, I. S., Torres-Díaz, C., Gundel, P. E., Dreyer, I. (2020). Antarctic Root endophytes improve physiological performance and yield in crops under salt stress by enhanced energy production and na+ sequestration. Sci. Rep. 10 (1), 5819. doi: 10.1038/s41598-020-62544-4
Ownley, B. H., Griffin, M. R., Klingeman, W. E., Gwinn, K. D., Moulton, J. K., Pereira, R. M. (2008). Beauveria bassiana: endophytic colonization and plant disease control. J. Invertebr. Pathol. 98 (3), 267–270. doi: 10.1016/j.jip.2008.01.010
Patten, C. L., Glick, B. R. (1996). Bacterial biosynthesis of indole-3-acetic acid. Can. J. Microbiol. 42 (3), 207–220. doi: 10.1139/m96-032
Raaijmakers, J. M., Weller, D. M. (2001). Exploiting genotypic diversity of 2, 4-diacetylphloroglucinol-producing Pseudomonas spp.: characterization of superior root-colonizing p. fluorescens strain Q8r1-96. Appl. Environ. Microbiol. 67 (6), 2545–2554. doi: 10.1128/AEM.67.6.2545-2554.2001
Sadasivam, S., Manickam, A. (1996). Biochemical methods for agricultural sciences (New Delhi: New Age International (P) Ltd), 1–97.
Sagar, A., Rai, S., Ilyas, N., Sayyed, R. Z., Al-Turki, A. I., El Enshasy, H. A., et al. (2022). Halotolerant rhizobacteria for salinity-stress mitigation: Diversity, mechanisms and molecular approaches. Sustainability 14 (1), 490. doi: 10.3390/su14010490
Sahu, P. K. (2012). Development of fluid bed dried (FBD) inoculant formulation of consortium of agriculturally important microorganisms (AIM) (Bengaluru, India: Masters Dissertation, University of Agricultural Science).
Sahu, P. K., Kumari, N., Gupta, A., Manzar, N. (2021a). “Rhizospheric and endophytic microorganisms and their role in alleviation of salinity stress in plants,” in Plant, soil and microbes in tropical ecosystems. Eds. Dubey, S. K., Verma, S. K. (Singapore: Springer), 19–37).
Sahu, P. K., Singh, S., Gupta, A. R., Gupta, A., Singh, U. B., Manzar, N., et al. (2020). Endophytic bacilli from medicinal-aromatic perennial holy basil (Ocimum tenuiflorum l.) modulate plant growth promotion and induced systemic resistance against Rhizoctonia solani in rice (Oryza sativa l.). Biol. Control 150, 104353. doi: 10.1016/j.biocontrol.2020.104353
Sahu, P. K., Singh, S., Gupta, A., Singh, U. B., Brahmaprakash, G. P., Saxena, A. K. (2019). Antagonistic potential of bacterial endophytes and induction of systemic resistance against collar rot pathogen Sclerotium rolfsii in tomato. Biol. Control 137, 104014. doi: 10.1016/j.biocontrol.2019.104014
Sahu, P. K., Singh, S., Singh, U. B., Chakdar, H., Sharma, P. K., Sarma, B. K., et al. (2021b). Inter-genera colonization of Ocimum tenuiflorum endophytes in tomato and their complementary effects on Na+/K+ balance, oxidative stress regulation, and root architecture under elevated soil salinity. Front. Microbiol. 12. doi: 10.3389/fmicb.2021.744733
Schwyn, B., Neilands, J. B. (1987). Universal chemical assay for the detection and determination of siderophores. Anal. Biochem. 160 (1), 47–56. doi: 10.1016/0003-2697(87)90612-9
Shafi, A., Zahoor, I., Mushtaq, U. (2019). “Proline accumulation and oxidative stress: Diverse roles and mechanism of tolerance and adaptation under salinity stress,” in Salt stress, microbes, and plant interactions: Mechanisms and molecular approaches. Ed. Akhtar, M. S. (Singapore: Springer), 269–300.
Shahzad, R., Khan, A. L., Bilal, S., Waqas, M., Kang, S. M., Lee, I. J. (2017). Inoculation of abscisic acid-producing endophytic bacteria enhances salinity stress tolerance in Oryza sativa. Environ. Exp. Bot. 136, 68–77. doi: 10.1016/j.envexpbot.2017.01.010
Shrivastava, P., Kumar, R. (2015). Soil salinity: A serious environmental issue and plant growth promoting bacteria as one of the tools for its alleviation. Saudi J. Biol. Sci. 22 (2), 123–131. doi: 10.1016/j.sjbs.2014.12.001
Siddikee, M. A., Glick, B. R., Chauhan, P. S., jong Yim, W., Sa, T. (2011). Enhancement of growth and salt tolerance of red pepper seedlings (Capsicum annuum l.) by regulating stress ethylene synthesis with halotolerant bacteria containing 1-aminocyclopropane-1-carboxylic acid deaminase activity. Plant Physiol. Biochem. 49 (4), 427–434. doi: 10.1016/j.plaphy.2011.01.015
Singh, R. P., Jha, P. N. (2016). A halotolerant bacterium Bacillus licheniformis HSW-16 augments induced systemic tolerance to salt stress in wheat plant (Triticum aestivum). Front. Plant Sci. 7, 1890. doi: 10.3389/fpls.2016.01890
Singh, D., Rajawat, M. V., Kaushik, R., Prasanna, R., Saxena, A. K. (2017). Beneficial role of endophytes in biofortification of zn in wheat genotypes varying in nutrient use efficiency grown in soils sufficient and deficient in zn. Plant Soil 416 (1), 107–116. doi: 10.1007/s11104-017-3189-x
Singh, P., Singh, R. K., Zhou, Y., Wang, J., Jiang, Y., Shen, N., et al. (2022). Unlocking the strength of plant growth promoting Pseudomonas in improving crop productivity in normal and challenging environments: a review. J. Plant Interact. 17 (1), 220–238. doi: 10.1080/17429145.2022.2029963
Vaishnav, A., Singh, J., Singh, P., Rajput, R. S., Singh, H. B., Sarma, B. K. (2020). Sphingobacterium sp. BHU-AV3 induces salt tolerance in tomato by enhancing antioxidant activities and energy metabolism. Front. Microbiol. 11, 443. doi: 10.3389/fmicb.2020.00443
Vandenkoornhuyse, P., Quaiser, A., Duhamel, M., Le Van, A., Dufresne, A. (2015). The importance of the microbiome of the plant holobiont. New Phytol. 206 (4), 1196–1206. doi: 10.1111/nph.13312
Verma, S. K., Sahu, P. K., Kumar, K., Pal, G., Gond, S. K., Kharwar, R. N., et al. (2021). Endophyte roles in nutrient acquisition, root system architecture development and oxidative stress tolerance. J. Appl. Microbiol. 131 (5), 2161–2177. doi: 10.1111/jam.15111
World Population Prospects (2019). Data Booklet, United Nations, ST/ESA/SER.A/424 (United Nations, New York, USA: Department of Economic and Social Affairs, Population Division).
Yadav, A. N., Saxena, A. K. (2018). Biodiversity and biotechnological applications of halophilic microbes for sustainable agriculture. J. Appl. Biol. Biotechnol. 6 (1), 48–55. doi: 10.7324/JABB.2018.60109
Yan, Y., Shi, Q., Gong, B. (2020). “Review of melatonin in horticultural crops,” in Melatonin-the hormone of darkness and its therapeutic potential and perspectives, vol. 26 . Ed. Vlachou, M. (London: IntechOpen).
Zhang, X., Zhang, H., Zhang, H., Tang, M. (2020). Exogenous melatonin application enhances Rhizophagus irregularis symbiosis and induces the antioxidant response of Medicago truncatula under lead stress. Front. Microbiol. 11, 516. doi: 10.3389/fmicb.2020.00516
Keywords: rhizospheric bacteria, endophytes, salt tolerance, PGP traits, colonization under stress
Citation: Gupta A, Tiwari RK, Shukla R, Singh AN and Sahu PK (2023) Salinity alleviator bacteria in rice (Oryza sativa L.), their colonization efficacy, and synergism with melatonin. Front. Plant Sci. 13:1060287. doi: 10.3389/fpls.2022.1060287
Received: 03 October 2022; Accepted: 12 December 2022;
Published: 12 January 2023.
Edited by:
Prafull Salvi, National Agri-Food Biotechnology Institute, IndiaReviewed by:
Mamoona Rauf, Abdul Wali Khan University Mardan, PakistanDeepak Kumar, Uttar Banga Krishi Viswavidyalaya, India
Copyright © 2023 Gupta, Tiwari, Shukla, Singh and Sahu. This is an open-access article distributed under the terms of the Creative Commons Attribution License (CC BY). The use, distribution or reproduction in other forums is permitted, provided the original author(s) and the copyright owner(s) are credited and that the original publication in this journal is cited, in accordance with accepted academic practice. No use, distribution or reproduction is permitted which does not comply with these terms.
*Correspondence: Amrita Gupta, YW1yaXRhc29uaTkwQGdtYWlsLmNvbQ==; Rajesh Kumar Tiwari, cmt0aXdhcmlAbGtvLmFtaXR5LmVkdQ==
†Present addresses: Amrita Gupta, ICAR-Indian Institute of Seed Sciences, Kushmaur, Maunath Bhanjan, India
Renu Shukla, Indian Council of Agricultural Research, New Delhi, India