- 1Biotechnology Research Institute, Chinese Academy of Agricultural Sciences, Beijing, China
- 2State Key Laboratory of North China Crop Improvement and Regulation, Hebei Agricultural University, Baoding, China
- 3National Nanfan Research Institute (Sanya), Chinese Academy of Agricultural Sciences, Sanya, China
CRIPSR/Cas9 gene editing system is an effective tool for genome modification in plants. Multiple target sites are usually designed and the effective target sites are selected for editing. Upland cotton (Gossypium hirsutum L., hereafter cotton) is allotetraploid and is commonly considered as difficult and inefficient to transform, it is important to select the effective target sites that could result in the ideal transgenic plants with the CRISPR-induced mutations. In this study, Agrobacterium rhizogenes-mediated hairy root method was optimized to detect the feasibility of the target sites designed in cotton phytoene desaturase (GhPDS) gene. A. rhizogenes showed the highest hairy root induction (30%) when the bacteria were cultured until OD600 reached to 0.8. This procedure was successfully applied to induce hairy roots in the other three cultivars (TM–1, Lumian–21, Zhongmian–49) and the mutations were detected in GhPDS induced by CRISPR/Cas9 system. Different degrees of base deletions at two sgRNAs (sgRNA5 and sgRNA10) designed in GhPDS were detected in R15 hairy roots. Furthermore, we obtained an albino transgenic cotton seeding containing CRISPR/Cas9-induced gene editing mutations in sgRNA10. The hairy root transformation system established in this study is sufficient for selecting sgRNAs in cotton, providing a technical basis for functional genomics research of cotton.
1 Introduction
Cotton is an important economic crop and natural fiber source (Huang and Zhu, 2021). In 2007, the Cotton Genome Consortium set a strategic plan to sequence cotton genomes (Chen et al., 2007). Gossypium raimondii was the first cotton species to be performed whole genome sequencing (WGS) (Paterson et al., 2012; Wang et al., 2012). The draft genome of Gossypium arboreum was released in 2014 (Li et al., 2014). In 2015, the draft genome of Gossypium hirsutum cultivar TM–1 was released (Li et al., 2015; Zhang et al., 2015). Over the next three years, new sequencing platforms and assembly strategies were used to further refine the upland cotton genome. In the same year, Gossypium barbadense genome was also sequenced (Liu et al., 2015; Yuan et al., 2015). In the next few years, a large amount of genomic information has been obtained by sequencing and resequencing, which provides a good foundation for functional genomics research.
The identification of gene function is usually achieved by overexpression and knockout. CRISPR/Cas9 has been developed into a recognized gene editing tool because of its wide applicability and diverse editing types. Compared with other genome-editing systems, CRISPR/Cas9 system has obvious advantages such as simple construction, low cost and easy implementation in the laboratory, so it has been widely used in genome editing of many plants (Jiang et al., 2013; Brooks et al., 2014; Raffan et al., 2021) and has shown great application prospects in cotton functional genomic research (Wang et al., 2017; Janga et al., 2019; Gao et al., 2020). Obtaining transgenic plants is particularly important for gene function research. For cotton transformation, it is particularly difficult to obtain transgenic plants from regeneration cultures using traditional plant transformation methods (Trolinder and Goodin, 1987; Vijaya et al., 2015). It typically takes about one year to obtain a transgenic cotton plant. Therefore, the reliability of the vector used for the transformation is particularly important.
sgRNA is one of the important elements of CRISPR/Cas9 system. Previous studies have shown that many sgRNAs do not work effectively (Farboud and Meyer, 2015). Therefore, the selection and editing efficiency of target sites are important issues to be considered before stable transformation. Transient transformation of protoplast and cotyledon with complete editing vectors are the two reported methods for selecting sgRNAs (Chen et al., 2017; Gao et al., 2017). However, due to the low transient transformation efficiency and the large size of the Cas9 gene (more than 4000 bp), the gene editing event is not easy to be detected.
Agrobacterium rhizogenes (Rhizobiaceae), a gram-negative soil bacterium, contains the Ri plasmid and could induce hairy roots production from plants (Smith and Townsend, 1907). Since hairy roots have the advantages of rapid growth and multiple branches, it is easier to perform the induction of hairy roots using A. rhizogenes than to generate transgenic plants using Agrobacterium tumefaciens. A. rhizogenes-mediated transformation system has been established to test the efficiency of zinc-finger nucleases (ZFNs) and CRISPR/Cas9 construct in soybean (Curtin et al., 2011; Cheng et al., 2019) and other plants (Gai et al., 2015; Zhang et al., 2020). However, the validation of sgRNAs for CRISPR/Cas9 in cotton by A. rhizogenes-mediated hairy root system has not been reported yet. In this study, we developed a cotton hairy root system to validate sgRNAs for CRISPR/Cas9 gene editing in cotton within one month, which provides a reliable reference for stable transformation. This method was successfully used for the validation of sgRNAs targeting GhPDS gene. Moreover, CRISPR/Cas9-induced mutations were detected at GhPDS-sgRNA10 target site in a transgenic albino cotton seeding.
2 Results
2.1 Hairy root induction in cotton using A. rhizogenes
To determine the optimal A. rhizogenes concentration for inducing hairy roots, a gradient optical density (OD600) experiment was conducted. R15 cotton seedings with the fully unfolded first true leaf were injected at the apical bud region with four OD600 (0.4, 0.6, 0.8, 1.0) suspensions of A. rhizogenes. Hairy roots emerged within one month, the ratio of R15 that produced hairy roots was calculated. The suspension of A. rhizogenes at OD600 0.8 showed the highest induction frequency of 30%, followed by that at OD600 0.6 (27.5%) (Figure 1A). This result indicated that OD600 0.6–0.8 is the most suitable concentration range for inducing hairy roots. Later, we evaluated the procedure in the other three cultivars (Zhongmian–49, Lumian–21, TM–1). A. rhizogenes could induce hairy roots from these three cultivars and no significant difference in induction frequency of Zhongmian–49, Lumian–21, TM–1 and R15 when the bacteria were cultured to OD600 0.8 (Figure 1B, C). These results indicated that this method is suitable for cotton materials with different genetic backgrounds.
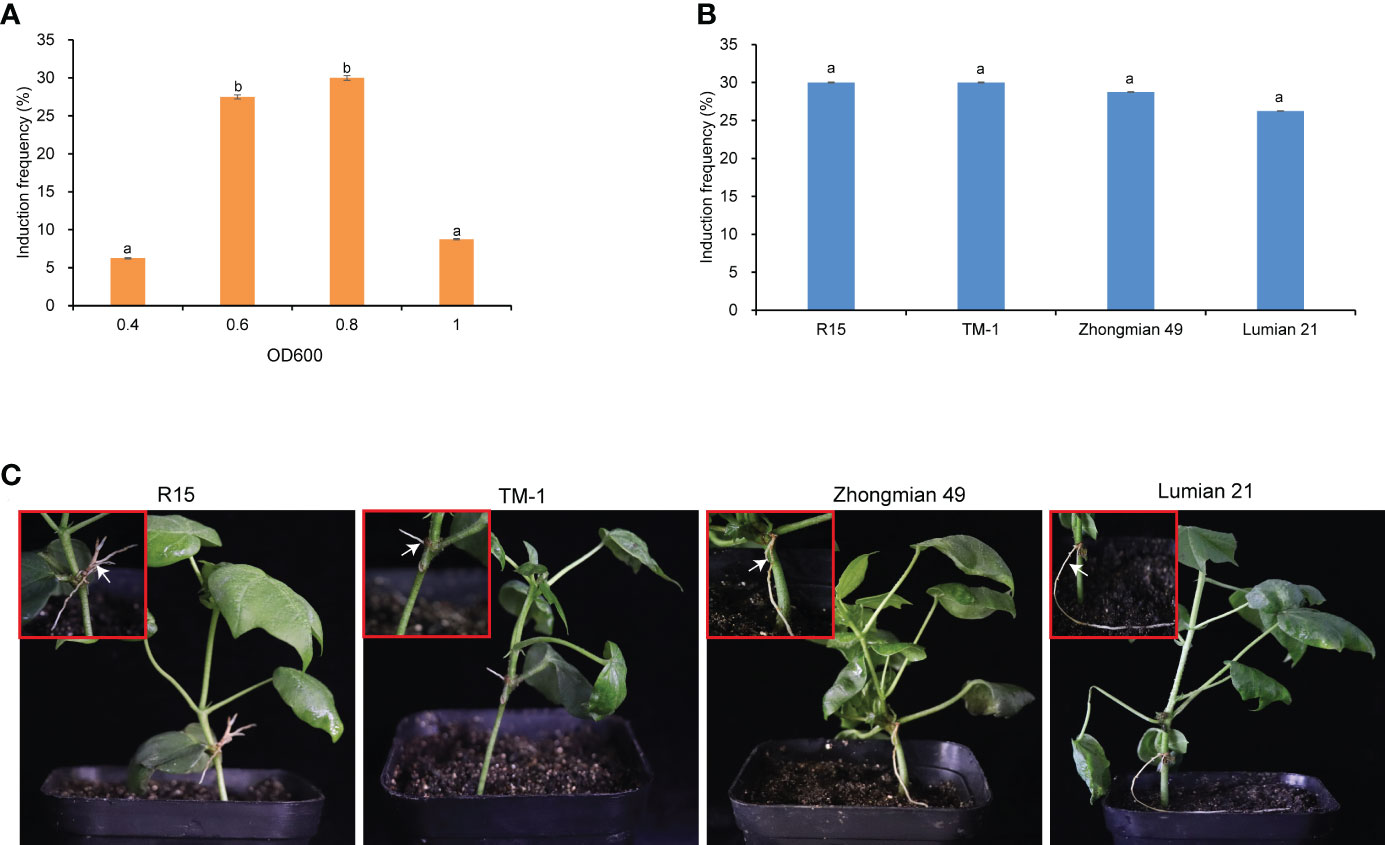
Figure 1 Induction of cotton hairy roots. (A) Comparison of hairy root induction frequency of A rhizogenes with different OD600 (0.4, 0.6, 0.8 and 1.0) in R15. (B) Hairy root induction frequency of A rhi-zogenes (OD600 0.8) in four cotton cultivars (R15, TM-1, Zhongmian-49 and Lumian-21). Values are mean ± SD of four biological replicates, means with different letters denote a significance dif-ference while similar letters denote no significance (Tukey’s HSD test, p < 0.05). (C) Hairy root growth status in four cotton cultivars. Scale bars in c, 1.0cm.
2.2 CRISPR/Cas9 vector construction and target selection
The CRISPR vector used here encodes Cas9 driven by the CaMV35S promoter and the sgRNA driven by the Arabidopsis U6 promoter (Figure 2A). Most genes in cotton are duplicated, so we designed the target sites that could target GhPDS in both A and D genomes simultaneously. sgRNA5 and sgRNA10 were designed to guide Cas9 to cleave the target site within the fifth and tenth exons of GhPDS, respectively, and the primer sites for amplification of the region surrounding GhPDS target sites were identical in the two genomes (Figure 2B). Therefore, there were two binary vectors generated to be tested in hairy-root tissues.
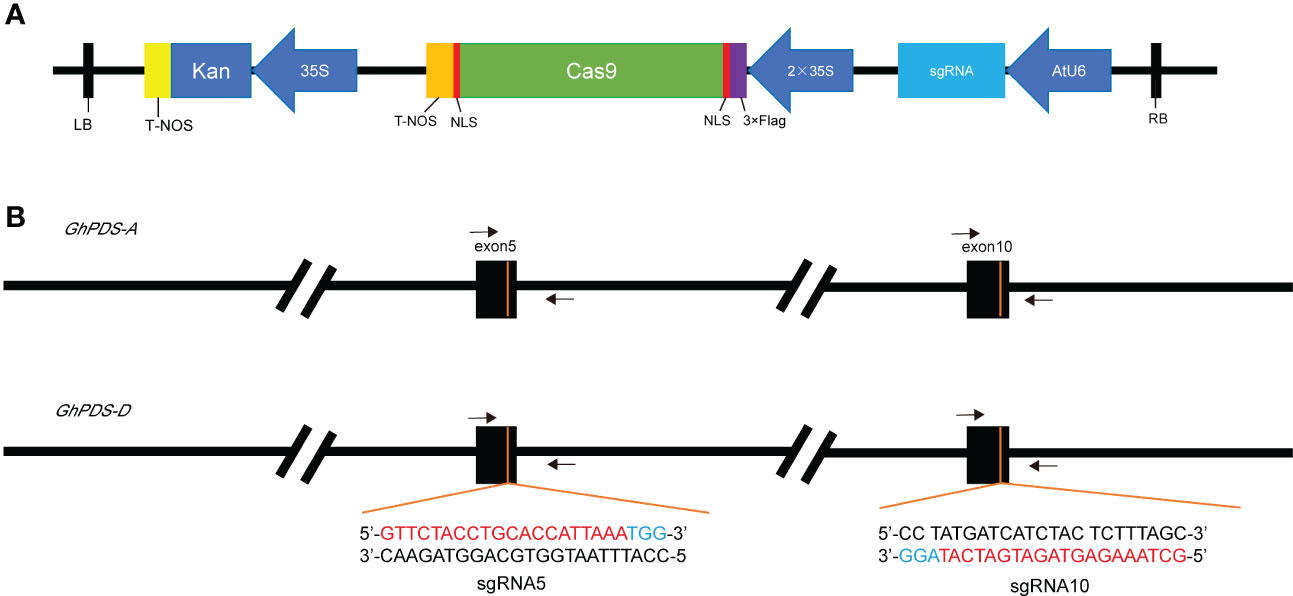
Figure 2 Diagram of single gRNA CRISPR/Cas9 vector, target sequence and target location. (A) CRISPR/Cas9 vector for cotton transformation with pCAMBIA2301 backbone, kan gene as selection marker for stable transformation, Cas9 and sgRNA were driven by CaMV35S promoter and Arabidopsis U6 promoter respectively. NOS, Nopaline Synthase terminator. (B) Location of target sites in GhPDS in A genome and D genome and gene-specific primers used for sequencing were indicated by arrows.
2.3 CRISPR/Cas9-induced mutagenesis in cotton hairy-root tissues
The validation of target sites was conveniently assessed using the developed hairy root system. A. rhizogenes strain K599 harboring the CRISPR plasmid was transferred into R15 apical bud. Ten hairy root lines were randomly selected for each sgRNA. The presence of Cas9 gene in hairy root was detected by PCR using specific primers (Figure 3A, 4A), and virB was not amplified in any lines for excluding A. rhizogenes contamination (Figure 3B, 4B). These results demonstrated ten hairy root lines were positive. The two target regions in GhPDS were then amplified by PCR in ten positive hairy roots and the amplicons were sequenced by Hi-TOM. The results revealed that mutations were detected at sgRNA5 and sgRNA10 in hairy roots. Samples with target mutation ratio (target mutation reads/total reads containing target site) >1% were considered as edition occurred at the target site. Deletions ranging from -1 bp up to -7 bp in sgRNA5 were identified in 4 (L1, L3, L4, L7) out of 10 hairy root lines (Figure 3C) and deletions ranging from -2 to -6 bp in sgRNA10 were identified in 5 (L2, L5, L6, L8, L9) out of 10 hairy root lines (Figure 4C). These results demonstrated Cas9 and sgRNAs induced mutations could be detected in cotton hairy roots.
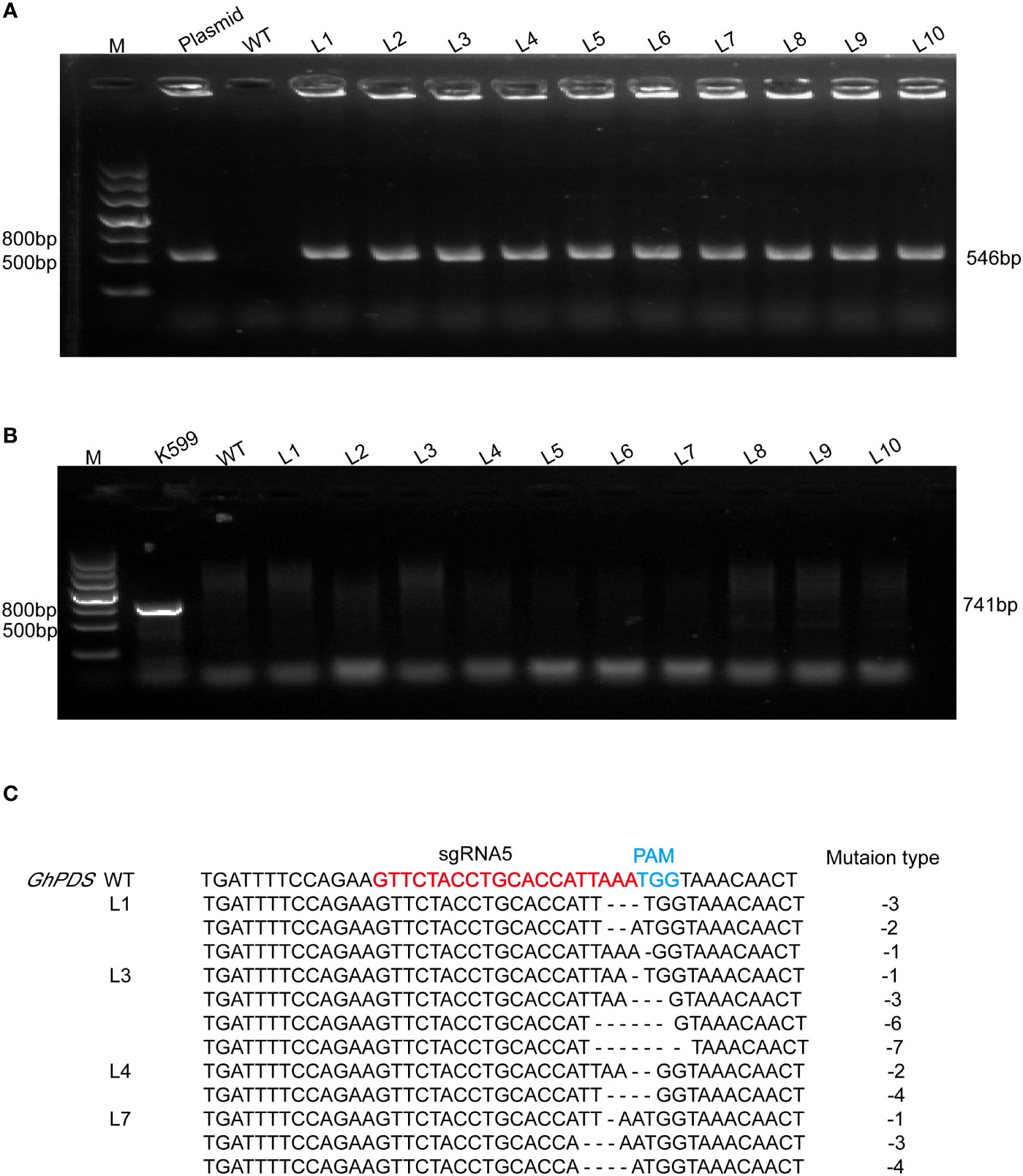
Figure 3 Analysis of mutations at sgRNA5 in R15 hairy roots. (A) Amplification products of Cas9 from genomic DNA of R15 hairy roots using specific primer (Supplementary Table S1). M, 2-kb DNA marker; Plasmid, p2301-GhPDS-sgRNA5-CRISPR plasmid DNA (positive control); WT, wild-type hairy root DNA (negative control); L1-L10, ten independent cotton hairy roots. (B) Amplification products of virB from R15 hairy roots. K599, A rhizogenes strain K599 (positive control). (C) Hi-TOM sequencing analysis of sgRNA5 target site in positive hairy roots.
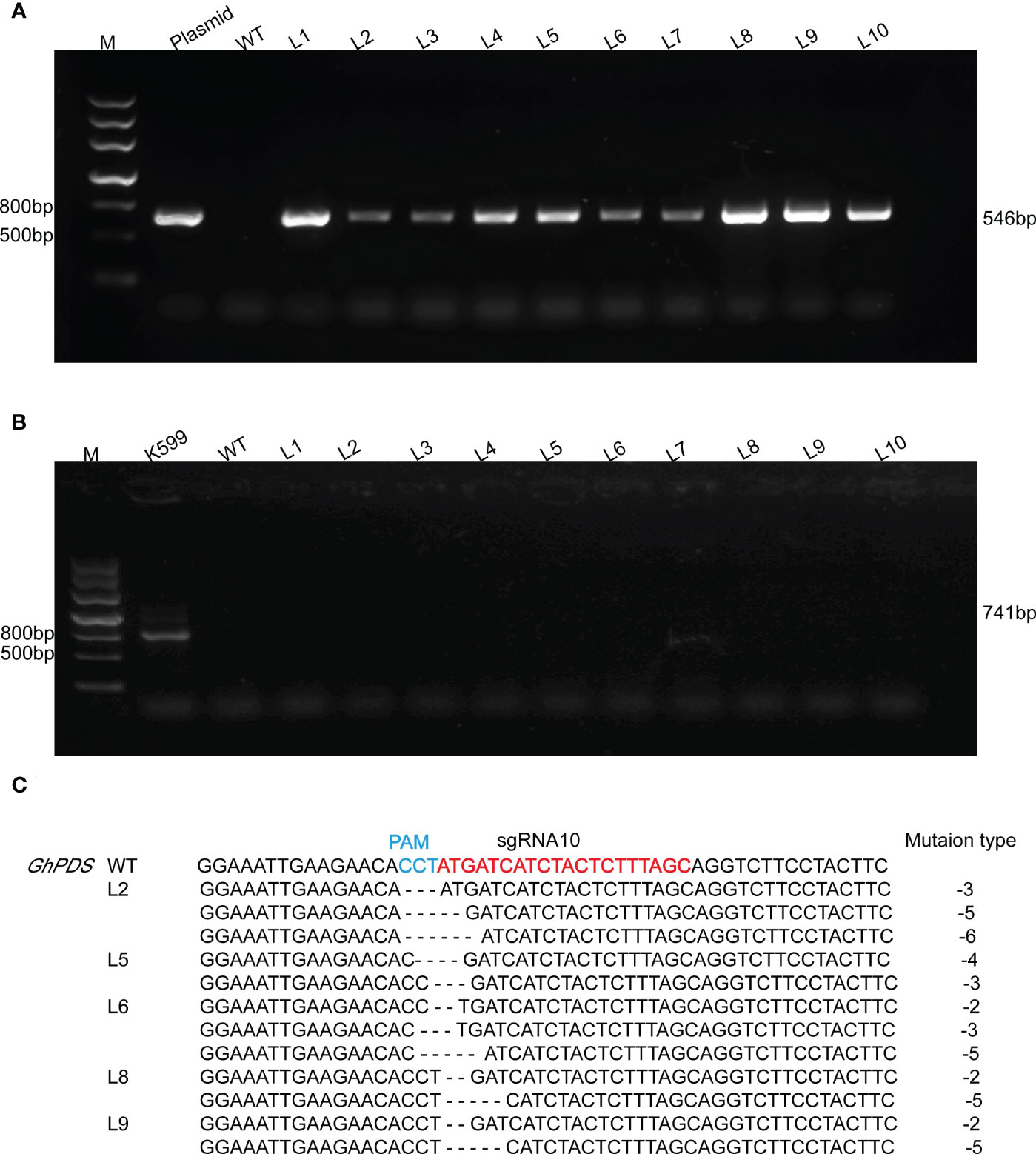
Figure 4 Analysis of mutations at sgRNA10 in R15 hairy roots. (A) Amplification products of Cas9 from genomic DNA of R15 hairy roots using specific primer (Supplementary Table S1). M, 2-kb DNA marker; Plasmid, p2301-GhPDS-sgRNA10-CRISPR plasmid DNA (positive control); WT, wild-type hairy root DNA (negative control); L1-L10, ten independent cotton hairy roots. (B) Amplification products of virB from R15 hairy roots. K599, A rhizogenes strain K599 (positive control). (C) Hi-TOM sequencing analysis of sgRNA5 target site in positive hairy roots.
2.4 Targeted mutagenesis in transgenic cotton plants
Our method was efficient in selecting CRISPR/Cas9 target sites, but could not produce stable mutant plants. To test whether gene mutations detected in hairy roots could be detected in cotton, we performed stable transgenic transformation using A. tumefaciens-mediated hypocotyl transformation with the two CRISPR/Cas9 constructs. Some callus regenerated on selection medium about 4 months after transformation. One independent regenerated plant for the GhPDS-sgRNA10 target was obtained and showed an albino phenotype in the leaves (Figure 5A). However, no transgenic plants for the GhPDS-sgRNA5 were obtained. CRISPR/Cas9-induced mutations at sgRNA10 in the albino plantlet were analyzed by Sanger sequencing, the sequencing results showed successful editing events at sgRNA10 site in two homologous sequences of GhPDS were detected. A total of 66 clones were analyzed with 12.12% of them harboring deletions (-1, -2, -3, -6 bp), 1.52% containing insertions (+1 bp) and 10.60% containing substitutions. The frequency of deletion mutations was higher than that of insertions and substitutions (Figure 5B). Whereas, only deletion mutations were detected in the hairy roots. These results further demonstrate the feasibility of detection of sgRNAs using hairy root system in cotton.
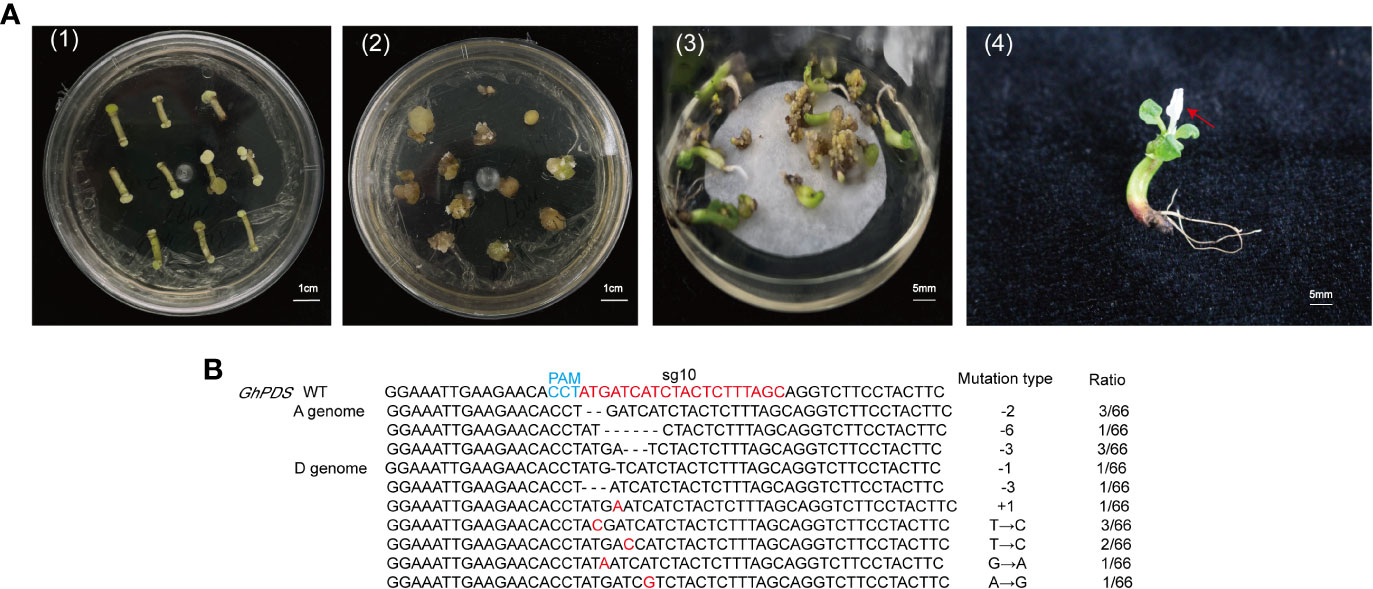
Figure 5 Mutations analysis of GhPDS in transgenic plants. (A) Generation of transgenic cotton plant via A tumefaciens-mediated transformation. (1) Induction of resistant calluses on selection medium; (2) embryonic calluses with different morphologies. (3) embryonic calluses were developed on regeneration medium. (4) regenerated transgenic cotton growing on regeneration medium. Red arrows indicated albino phenotype. (B) Sanger sequencing analysis of mutations at sgRNA10 target site in albino plantlet. The mutation types are present in the penultimate column. The number of amplicons giving mutant sequences out of the total clones are presented in the column to the right. -, deleted nucleotides; +, inserted nucleotides; →, substituted nucleotides.
3 Materials and methods
A workflow diagram for the experiments is given in Figure 6.
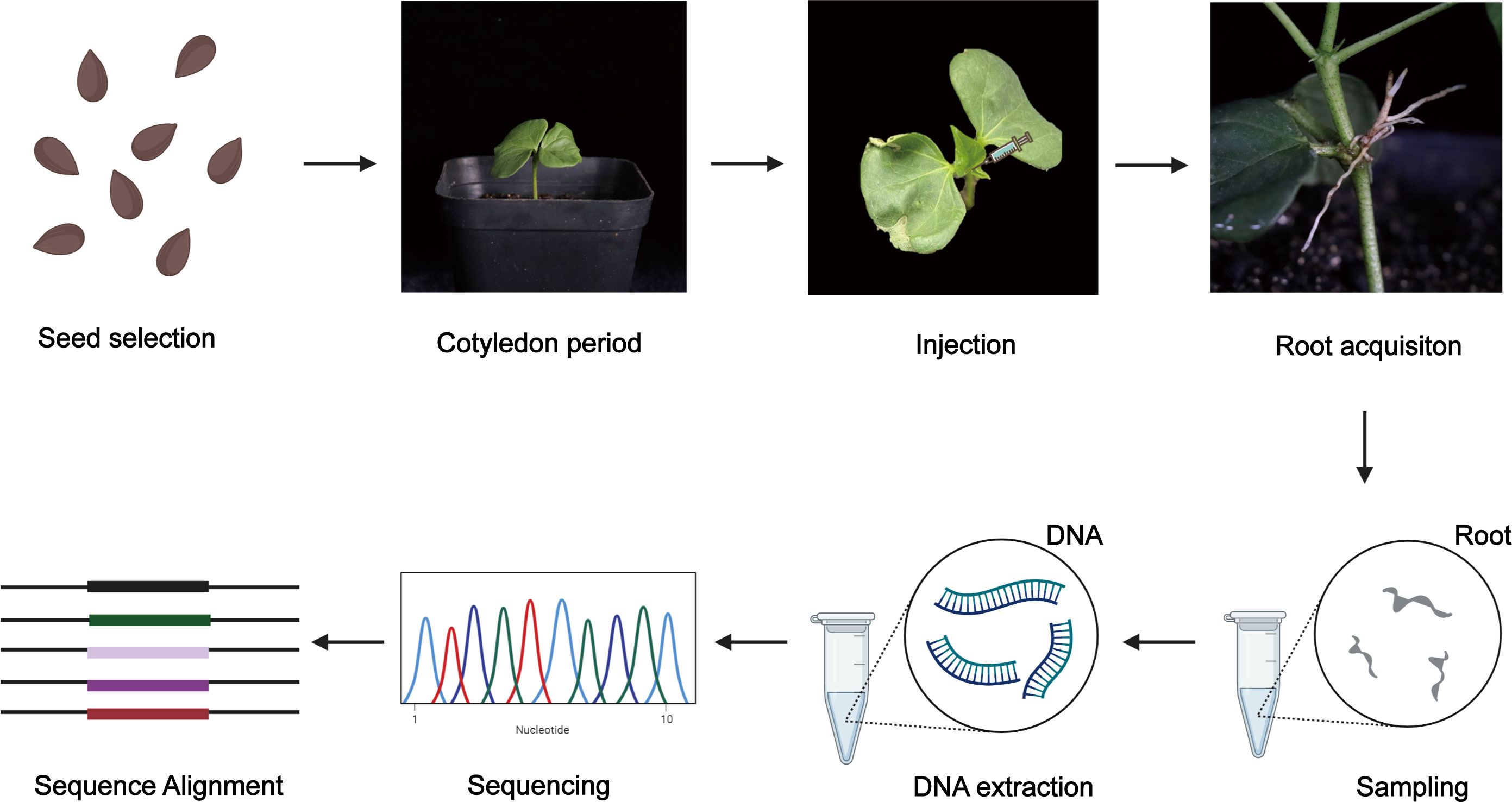
Figure 6 A workflow diagram of experiment. First, the plump cotton seeds were selected and planted in nutrient soil. Injection could be conducted when the first true leaf of cotton seedings unfurled. Then A. rhizogenes with OD600 0.6–0.8 were prepared and used for injecting at the cotton apical bud. Hairy roots emerged within one month. Next, DNA was extracted from hairy roots and the positive hairy roots were detected using the specific primers. Fragments containing the target site were amplified with the specific primer and sequenced. Finally, alignment between sequencing results and original sequences was conducted.
3.1 Plants material and growth conditions
Four cotton (Gossypium hirsutum L.) cultivars (Coker 312 (R15), TM–1, Lumian–21 and Zhongmian–49) preserved in our laboratory were used in this study. These four cotton cultivars were planted in a growth chamber (16-h light/8-h dark; 100 µmol m-2 s-1 light intensity; 28°C; 70% humidity). Plants could be used for inducing hairy root when the first true leaf fully unfold (about 14 days after sowing). Coker 312 was used for stable transformation.
3.2 Construction of CRISPR/Cas9 vector
The CRISPR/Cas9 vectors were gifts from Jian-Kang Zhu (Feng et al., 2013). sgRNAs design according to standards mentioned previously (Li et al., 2017). Two sgRNAs (sgRNA5 and sgRNA10) DNA oligonucleotides were synthesized and annealed to generate two dimers. Two dimers were then cloned into the Bbs I site of pAtU6-26-SK to generate pSK-AtU6-sgRNA5 and pSK-AtU6-sgRNA10. These two pSK-AtU6-sgRNAs were cut with restriction enzyme Kpn I/Sal I (New England Biolabs, USA), and p35S-Cas9-SK was digested with restriction enzyme Sal I/EcoR I. Two fragments were assembled into pCAMBIA2301 using KpnⅠ/EcoRⅠ restriction digestion followed by ligation to generate the p2301-GhPDS-sgRNA5-CRISPR and p2301-GhPDS-sgRNA10-CRISPR constructs. The kan gene, driven by the CaMV 35S promoter of pCAMBIA2301, was used as selection marker for cotton stable transformation.
3.3 Agrobacterium rhizogenes-mediated transient transformation of cotton
A. rhizogenes strain K599 was used to deliver gene editing vector has been described (Fan et al., 2020). The binary vector was introduced into strain K599 purchased from WeiDi (Shanghai, China) using ‘heat shock’ procedure. A. rhizogenes strain K599 wild-type or carrying binary vectors were used for infection. Wild-type bacteria were cultured at 28°C with shaking at 180 rpm to different OD600 (0.4, 0.6, 0.8, 1.0). Afterwards, the bacterial suspension was used to inject the apical bud of 20 R15 plants with a concentration without centrifugation and resuspension. Plants were then grown in the growth chamber (16-h light/8-h dark; 28°C; 70% humidity) for 1 month, then hairy roots emerged and the number of plants with hairy roots was counted. The other three cotton cultivars were then injected in the same way. A. rhizogenes strain K599 harboring p2301-GhPDS-sgRNA5/10-CRISPR vector were cultured until the OD600 reached to 0.8 for injection as described above.
3.4 Genetic transformation of cotton
The cotton transformation was conducted according to the following method (Wang et al., 2017). Hypocotyl segments from R15 sterile seedings were immersed in the Agrobacterium strain LBA4404 (OD600 0.4–0.5) for 5 min, then the infected hypocotyl fragments were co-cultured on MSB1 medium (Sigma, St. Louis, USA) for 48 h at 28°C in the dark. Next, the hypocotyls were transferred to MSB1 medium with 50 mg L-1 kanamycin and 500 mg L-1 cephalosporin for resistant calluses formation. The resistant calluses were grown on MSB2 medium for 4 months until embryogenic calluses (ECs) were obtained. ECs were then grown on MSB3 medium for 3–4 months. Finally, regenerated plants were transferred to soil.
3.5 Genomic DNA extraction and mutations analyses
DNA was extracted from hairy roots and leaves using the DNAsecure Plant Kit (TIANGEN, Beijing, China). Primers Cas9-F/R were designed based on cas9 sequence to detect exogenous T-DNA, and primers VirB-F/R were designed based on VirB gene to detect A. rhizogenes contamination (Supplementary Table S1). The region spanning the target site was amplified using 2x Taq Plus Master Mix II (Vazyme, Nanjing, China) with the specific primer pair (Supplementary Table S1). The amplicons were sequenced by high-throughput (HiTOM) sequencing platform (Liu et al., 2019) and Sanger sequencing.
3.6 Statistical analyses
Samples were collected from more than three technical replicates. The number of plants with hairy roots data were analyzed using SPSS software (IBM, Armonk, NY, USA). Analysis of variance was used to compare the statistic difference based on Tukey’s HSD test at significance level of p < 0.05.
4 Discussion
A. rhizogenes K599 strain was able to induce hairy roots has been reported earlier in cotton (Che et al., 2019) and other plants (Wei et al., 2016; Aggarwal et al., 2018; Bazaldúa et al., 2019). Previous studies have indicated that some experimental steps need to be optimized for effective utilization of transformation ability of A. rhizogenes. In this study A. rhizogenes K599 strain could infect cotton apical bud and induced the infected cells to form hairy roots at 30% efficiency when bacteria were cultured until the OD600 reached to 0.8 (Figure 1A) and the procedure could be completed within one month. Previously, the procedure of hairy root transformation needs to be done in a sterile environment (Verma et al., 2009; Cui et al., 2020), whereas our method needs no sterile conditions. Compared with A. tumefaciens-mediated transformation, A. rhizogenes-mediated hairy root transformation is genotype-independent (Hosseini et al., 2017; Aggarwal et al., 2018; Gomes et al., 2019). Our study showed that A. rhizogenes K599 strain could induce hairy roots from four cotton cultivars and hairy root induction frequency showed no significant difference between them (Figures 1B, C).
Plant mutants are particularly important for studying gene functions and molecular mechanisms, and the CRISPR/Cas9 system provides an efficient method to induce mutations in target genes for functional research and genetic improvement of crops. CRISPR/Cas9 technology has been successfully applied in many crops for genome engineering, including cotton (Farboud and Meyer, 2015; Gao et al., 2017; Gao et al., 2020). Multiple sgRNAs are designed to ensure that the target gene is edited. A. tumefaciens-mediated genetic transformation procedure of cotton is time-consuming and inefficient. Therefore, it is essential to select efficient sgRNAs to reduce the workload. Hairy root system has been used for genome editing of various plant species, such as Medicago truncatula (Zhang et al., 2020), cucumber (Nguyen et al., 2021), oilseed rape (Jedličková et al., 2022). For cotton, the hairy root system has been applied for studying promoter activity (Kim et al., 2011), secondary metabolites production (Verma et al., 2009; Kim et al., 2011), plant generation (Cui et al., 2020), cotton-nematode interaction (Wubben et al., 2009), however, it has not been used for genome editing. Cotton is an allotetraploid and A and D genomes have a high content of repetitive DNA (Li et al., 2015). It is necessary to mutate multiple homoeoalleles for gene functional studies. Hairy root system is useful to assess the activity of sgRNA. In our study, two sgRNAs (sgRNA5 and sgRNA10) targeting GhPDS in A and D genomes were designed and the deletions were detected at the both sites in hairy roots (Figures 3C, 4C), the most common mutations induced by CRISPR/Cas9 were deletions, which is consistent with the results reported in rice, Arabidopsis, tobacco and other species (Nekrasov et al., 2013; Li et al., 2014; Xu et al., 2014; Zhou et al., 2014; Fan et al., 2015; Sun et al., 2015). Most practical applications of CRISPR/Cas9 system need obtained transgenic plants when studying important agronomic traits (Gao et al., 2017). The mutations in sgRNA10 in transgenic hairy roots were also observed in transgenic cotton which showed an albino phenotype (Figure 5B). This rapid and simple method could rapidly validate CRISPR/Cas9 sgRNA targets and reduce the workload of cotton stable transformation.
The hairy roots induced by A. rhizogenes are easy to cultivate and grow rapidly and have been widely used in the production and extraction of active ingredients of medicinal plants, such as ginseng (Fukuyama et al., 2012; Kochan et al., 2013), Artemisia annua (Patra and Srivastra, 2014), Salvia miltiorrhiza (Kai et al., 2011; Wei et al., 2019), Camptotheca acuminata (Lorence et al., 2004). Therefore, hairy root system provides an efficient method for secondary metabolites research in cotton (Verma et al., 2009). Compared with hairy root from cotyledon and hypocotyl (Verma et al., 2009; Cui et al., 2020), transgenic hairy root and composite plant could be used to study the transport of small signaling molecules like peptides and metabolites between roots and other parts of the plant (Che et al., 2019). Altogether, this method provides an opportunity to study root biology and gene function studies.
5 Conclusion
In this study, a simple and effective method under non-sterile conditions for cotton hairy root induction and transformation was developed for four cotton cultivars. The targeting capability of sgRNA could be evaluated successfully using this method. The results provided a potential tool for gene expression and gene editing studies in cotton.
Data availability statement
The original contributions presented in the study are included in the article/Supplementary Material. Further inquiries can be directed to the corresponding authors.
Author contributions
Conceptualization, LZ and YW. Methodology, PW. Software, CW. Validation, LZ, YW and JW. Formal analysis, LZ. Investigation, JW. Resources, CW. Data curation, PW. Writing original draft preparation, LZ. Writing review and editing, LZ and YW. Visualization, JW. Supervision, HC. Project administration, XW. Funding acquisition, XW and HC. All authors contributed to the article and approved the submitted version.
Funding
This research was funded by State Key Laboratory of North China Crop Improvement and Regulation, Hebei Key Technology R&D program (21326314D) and the Agricultural Science and Technology Innovation Program of CAAS (No. CAAS-ZDRW202009).
Acknowledgments
We thank Professor Jiankang Zhu for providing the CRISPR/Cas9 vector.
Conflict of interest
The authors declare that the research was conducted in the absence of any commercial or financial relationships that could be construed as a potential conflict of interest.
Publisher’s note
All claims expressed in this article are solely those of the authors and do not necessarily represent those of their affiliated organizations, or those of the publisher, the editors and the reviewers. Any product that may be evaluated in this article, or claim that may be made by its manufacturer, is not guaranteed or endorsed by the publisher.
Supplementary material
The Supplementary Material for this article can be found online at: https://www.frontiersin.org/articles/10.3389/fpls.2022.1059404/full#supplementary-material
References
Aggarwal, P. R., Nag, P., Choudhary, P., Chakraborty, N., Chakraborty, S. (2018). Genotype-independent Agrobacterium rhizogenes-mediated root transformation of chickpea: a rapid and efficient method for reverse genetics studies. Plant Methods 14, 55. doi: 10.1186/s13007-018-0315-6
Bazaldúa, C., Cardoso-Taketa, A., Trejo-Tapia, G., Camacho-Diaz, B., Arellano, J., Ventura-Zapata, E., et al. (2019). Improving the production of podophyllotoxin in hairy roots of Hyptis suaveolens induced from regenerated plantlets. PloS One 14, e0222464. doi: 10.1371/journal.pone.0222464
Brooks, C., Nekrasov, V., Lippman, Z. B., Eck, V. J. (2014). Efficient gene editing in tomato in the first generation using the clustered regularly interspaced short palindromic Repeats/CRISPR-Associated9 system. Plant Physiol. 166, 1292–1297. doi: 10.1104/pp.114.247577
Che, B., Cheng, C., Fang, J., Liu, Y., Jiang, L., Yu, B. (2019). The recretohalophyte Tamarix TrSOS1 gene confers enhanced salt tolerance to transgenic hairy root composite cotton seedlings exhibiting virus-induced gene silencing of GhSOS1. Int. J. Mol. Sci. 20, 2930. doi: 10.3390/ijms20122930
Cheng, Q., Dong, L., Su, T., Li, T., Kong, F. (2019). CRISPR/Cas9-mediated targeted mutagenesis of GmLHY genes alters plant height and internode length in soybean. BMC Plant Biol. 19, 562. doi: 10.1186/S12870-019-2145-8
Chen, X. G., Lu, X. K., Shu, N., Wang, S., Wang, J. J., Wang, D. L., et al. (2017). Targeted mutagenesis in cotton (Gossypium hirsutum l.) using the CRISPR/Cas9 system. Sci. Rep. 7, 44304. doi: 10.1038/srep44304
Chen, Z. J., Scheffler, B. E., Dennis, E., Triplett, B. A., Zhang, T., Guo, W., et al. (2007). Toward sequencing cotton (Gossypium) genomes. Plant Physiol. 145, 1303–1310. doi: 10.1104/pp.107.107672
Cui, M. L., Liu, C., Piao, C. L., Liu, C. L. (2020). A stable agrobacterium rhizogenes-mediated transformation of cotton (Gossypium hirsutum l.) and plant regeneration from transformed hairy root via embryogenesis. Front. Plant Sci. 11:604255. doi: 10.3389/fpls.2020.604255
Curtin, S. J., Zhang, F., Sander, J. D., Haun, W. J., Starker, C., Baltes, N. J., et al. (2011). Targeted mutagenesis of duplicated genes in soybean with zinc-finger nucleases. Plant Physiol. 156, 466–473. doi: 10.1104/pp.111.172981
Fan, D., Liu, T., Li, C., Jiao, B., Li, S., Hou, Y., et al. (2015). Efficient CRISPR/Cas9-mediated targeted mutagenesis in populus in the first generation. Sci. Rep. 5, 12217. doi: 10.1038/srep12217
Fan, Y. L., Zhang, X. H., Zhong, L. J., Wang, X. Y., Jin, L. S., Lyu, S. H. (2020). One-step generation of composite soybean plants with transgenic roots by Agrobacterium rhizogenes-mediated transformation. BMC Plant Biol. 20, 208. doi: 10.1186/s12870-020-02421-4
Farboud, B., Meyer, B. J. (2015). Dramatic enhancement of genome editing by CRISPR/Cas9 through improved guide RNA design. Genetics 199, 959–971. doi: 10.1534/genetics.115.175166
Feng, Z., Zhang, B., Ding, W., Liu, X., Yang, D. L., Wei, P., et al. (2013). Efficient genome editing in plants using a CRISPR/Cas system. Cell Res. 23, 1229–1232. doi: 10.1038/cr.2013.114
Fukuyama, N., Shibuya, M., Orihara, Y. (2012). Antimicrobial polyacetylenes from Panax ginseng hairy root culture. Chem. Pharm. Bull. (Tokyo) 60, 377–380. doi: 10.1248/cpb.60.377
Gai, Q. Y., Jiao, J., Luo, M., Wei, Z. F., Zu, Y. G., Ma, W., et al. (2015). Establishment of hairy root cultures by Agrobacterium rhizogenes mediated transformation of Isatis tinctoria l. for the efficient production of flavonoids and evaluation of antioxidant activities. PloS One 10, e0119022. doi: 10.1371/journal
Gao, W., Lu, L., Tian, X., Xu, F., Ji, L., Singh, P. K., et al. (2017). Genome editing in cotton with the CRISPR/Cas9 system. Front. Plant Sci. 8. doi: 10.3389/fpls.2017.01364
Gao, W., Xu, F. C., Long, L., Li, Y., Song, C. P. (2020). The gland localized CGP1 controls gland pigmentation and gossypol accumulation in cotton. Plant Biotechnol. J. 18, 1573–1584. doi: 10.1111/pbi.13323
Gomes, C., Dupas, A., Pagano, A., Grima-Pettenati, J., Paiva, J. A. P. (2019). Hairy root transformation: a useful tool to explore gene function and expression in Salix spp. recalcitrant to transformation. Front. Plant Sci. 10. doi: 10.3389/fpls.2019.01427
Hosseini, S. M., Bahramnejad, B., Douleti Baneh, H., Emamifar, A., Goodwin, P. H. (2017). Hairy root culture optimization and resveratrol production from Vitis vinifera subsp. Sylvesteris. World J. Microbiol. Biotechnol. 33, 67. doi: 10.1007/s11274-017-2235-4
Huang, G., Zhu, Y. X. (2021). Breeding cotton with superior fiber quality: identification and utilization of multiple elite loci and exotic genetic resources. Sci. Chian Life Sci. 64, 1197–1198. doi: 10.1007/s11427-021-1942-3
Janga, M. R., Pandeya, D., Campbell, L. M., Konganti, K., Villafuerte, S. T., Puckhaber, L., et al. (2019). Genes regulating gland development in the cotton plant. Plant Biotechnol. J. 17, 1142–1153. doi: 10.1111/pbi.13044
Jedličková, V., Mácová, K., Štefková, M., Butula, J., Staveníková, J., Sedláček, M., et al. (2022). Hairy root transformation system as a tool for CRISPR/Cas9-directed genome editing in oilseed rape (Brassica napus). Front. Plant Sci. 13. doi: 10.3389/fpls.2022.919290
Jiang, W., Zhou, H., Bi, H., Michael, F., Bing, Y., Weeks, D. P. (2013). Demonstration of CRISPR/Cas9/sgRNA-mediated targeted gene modification in Arabidopsis, tobacco, sorghum and rice. Nucleic Acids Res. 41, e188. doi: 10.1093/nar/gkt780
Kai, G., Xu, H., Zhou, C., Liao, P., Xiao, J., Luo, ,. X., et al. (2011). Metabolic engineering tanshinone biosynthetic pathway in Salvia miltiorrhiza hairy root cultures. Metab. Eng. 13, 319–327. doi: 10.1016/j.ymben.2011.02.003
Kim, H. J., Murai, N., Fang, D. D., Triplett, B. A. (2011). Functional analysis of gossypium hirsutum cellulose synthase catalytic subunit 4 promoter in transgenic arabidopsis and cotton tissues. Plant Sci. 180, 323–332. doi: 10.1016/j.plantsci.2010.10.003
Kochan, E., Wasiela, M., Sienkiewicz, M. (2013). The production of ginsenosides in hairy root cultures of American ginseng, Panax quinquefolium l. and their antimicrobial activity. In Vitro Cell Dev. Biol. Plant 49, 24–29. doi: 10.1007/s11627-012-9469-5
Li, F., Fan, G., Lu, C., Xiao, G., Zou, C., Kohel, R. J., et al. (2015). Genome sequence of cultivated upland cotton (Gossypium hirsutum TM-1) provides insights into genome evolution. Nat. Biotechnol. 33, 524–530. doi: 10.1038/nbt.3208
Li, F., Fan, G., Wang, K., Sun, F., Yuan, Y., Song, G., et al. (2014). Genome sequence of the cultivated cotton Gossypium arboreum. Nat. Genet. 46, 567–572. doi: 10.1038/ng.2987
Li, C., Unver, T., Zhang, B. (2017). A high-efficiency CRISPR/Cas9 system for targeted mutagenesis in cotton (Gossypium hirsutum l.). Sci. Rep. 7, 43902. doi: 10.1038/srep43902
Liu, X., Zhao, B., Zheng, H. J., Hu, Y., Lu, G., Yang, C. Q., et al. (2015). Gossypium barbadense genome sequence provides insight into the evolution of extra-long staple fiber and specialized metabolites. Sci. Rep. 5, 14139. doi: 10.1038/srep14139
Liu, Q., Wang, C., Jiao, X., Zhang, H., Song, L., Li, Y., et al. (2019). Hi-TOM: a platform for high-throughput tracking of mutations induced by CRISPR/Cas systems. Sci. China Life Sci. 62, 1–7. doi: 10.1007/s11427-018-9402-9
Lorence, A., Medina-Bolivar, F., Nessler, C. L. (2004). Camptothecin and 10-hydroxycamptothecin from Camptotheca acuminata hairy roots. Plant Cell Rep. 22, 437–441. doi: 10.1007/s00299-003-0708-4
Nekrasov, V., Staskawicz, B., Weigel, D., Jones, J. D., Kamoun, S. (2013). Targeted mutagenesis in the model plant nicotiana benthamiana using Cas9 RNA-guided endonuclease. Nat. Biotechnol. 31, 691–693. doi: 10.1038/nbt.2655
Nguyen, D. V., Hoang, T. T., Le, N. T., Tran, H. T., Nguyen, C. X., Moon, Y. H., et al. (2021). An efficient hairy root system for validation of plant transformation vector and CRISPR/Cas construct activities in cucumber (Cucumis sativus l.). Front. Plant Sci. 12. doi: 10.3389/fpls.2021.770062
Paterson, A. H., Wendel, J. F., Gundlach, H., Guo, H., Jenkins, J., Jin, D., et al. (2012). Repeated polyploidization of Gossypium genomes and the evolution of spinnable cotton fibres. Nature 492, 423–427. doi: 10.1038/nature11798
Patra, N., Srivastava, A. K. (2014). Enhanced production of artemisinin by hairy root cultivation of Artemisia annua in a modified stirred tank reactor. Appl. Biochem. Biotechnol. 174, 2209–2222. doi: 10.1007/s12010-014-1176-8
Raffan, S., Sparks, C., Huttly, A., Hyde, L., Halford, N. G. (2021). Wheat with greatly reduced accumulation of free asparagine in the grain, produced by CRISPR/Cas9 editing of asparagine synthetase gene TaASN2. Plant Biotechnol. J. 19, 1602–1613. doi: 10.1111/pbi.13573
Smith, E. F., Townsend, C. O. (1907). A plant-tumor of bacterial origin. Science 25, 671. doi: 10.1126/science.25.643.671
Sun, X., Hu, Z., Chen, R., Jiang, Q., Song, G., Zhang, H., et al. (2015). Targeted mutagenesis in soybean using the CRISPR-Cas9 system. Sci. Rep. 5, 10342. doi: 10.1038/srep10342
Trolinder, N. L., Goodin, J. R. (1987). Somatic embryogenesis and plant regeneration in cotton (Gossypium hirsutum l.). Plant Cell Rep. 6, 231–234. doi: 10.1007/bf00268487
Verma, P. C., Trivedi, I., Singh, H., Shukla, A. K., Kumar, M., Upadhyay, S. K., et al. (2009). Efficient production of gossypol from hairy root cultures of cotton (Gossypium hirsutum l.). Curr. Pharm. Biotechnol. 10, 691–700. doi: 10.2174/138920109789542048
Vijaya, N. J., Gopala, K. M., Giridara., K. S., Kirti, P. B. (2015). Current status of tissue culture and genetic transformation research in cotton (Gossypium spp.). Plant Cell Tiss Org. 120, 841. doi: 10.1007/s11240-014-0640-z
Wang, C., Lu, G., Hao, Y., Guo, H., Cheng, H. (2017). ABP9, a maize bZIP transcription factor, enhances tolerance to salt and drought in transgenic cotton. Planta 246, 453–469. doi: 10.1007/s00425-017-2704-x
Wang, Y., Meng, Z., Liang, C., Meng, Z., Wang, Y., Sun, G., et al. (2017). Increased lateral root formation by CRISPR/Cas9-mediated editing of arginase genes in cotton. Sci. China Life Sci. 60, 524–527. doi: 10.1007/s11427-017-9031-y
Wang, K., Wang, Z., Li, F., Ye, W., Wang, J., Song, G., et al. (2012). The draft genome of a diploid cotton Gossypium raimondii. Nat. Genet. 44, 1098–1103. doi: 10.1038/ng.2371
Wei, T., Gao, Y., Deng, K., Zhang, L., Yang, M., Liu, X., et al. (2019). Enhancement of tanshinone production in Salvia miltiorrhiza hairy root cultures by metabolic engineering. Plant Methods 15, 53. doi: 10.1186/s13007-019-0439-3
Wei, P., Wang, L., Liu, A., Yu, B., Lam, H. M. (2016). GmCLC1 confers enhanced salt tolerance through regulating chloride accumulation in soybean. Front. Plant Sci. 7. doi: 10.3389/fpls.2016.01082
Wubben, M. J., Callahan, F. E., Triplett, B. A., Jenkins, J. N. (2009). Phenotypic and molecular evaluation of cotton hairy roots as a model system for studying nematode resistance. Plant Cell Rep. 28, 1399–1409. doi: 10.1007/s00299-009-0739-6
Xu, R., Li, H., Qin, R., Wang, L., Li, L., Wei, P., et al. (2014). Gene targeting using the agrobacterium tumefaciens-mediated CRISPR-cas system in rice. Rice. 7, 1–4. doi: 10.1186/s12284-014-0005-6
Yuan, D., Tang, Z., Wang, M., Gao, W., Tu, L., Jin, X., et al. (2015). The genome sequence of Sea-island cotton (Gossypium barbadense) provides insights into the allopolyploidization and development of superior spinnable fibres. Sci. Rep. 5, 17662. doi: 10.1038/srep17662
Zhang, H., Cao, Y., Zhang, H., Xu, Y., Zhou, C., Liu, W., et al. (2020). Efficient generation of CRISPR/Cas9-mediated homozygous/biallelic Medicago truncatula mutants using a hairy root system. Front. Plant Sci. 11. doi: 10.3389/fpls.2020.00294
Zhang, T., Hu, Y., Jiang, W., Fang, L., Guan, X., Chen, J., et al. (2015). Sequencing of allotetraploid cotton (Gossypium hirsutum l. acc. TM-1) provides a resource for fiber improvement. Nat. Biotechnol. 33 (5), 531–537. doi: 10.1038/nbt.3207
Keywords: cotton, agrobacterium rhizogenes, hairy root, GhPDS, CRISPR/Cas9
Citation: Zhou L, Wang Y, Wang P, Wang C, Wang J, Wang X and Cheng H (2022) Highly efficient Agrobacterium rhizogenes-mediated hairy root transformation for gene editing analysis in cotton. Front. Plant Sci. 13:1059404. doi: 10.3389/fpls.2022.1059404
Received: 01 October 2022; Accepted: 06 December 2022;
Published: 23 December 2022.
Edited by:
Muhammad Ali Abid, Agricultural Genomics Institute at Shenzhen (CAAS), ChinaReviewed by:
Muhammad Aamir Manzoor, Anhui Agricultural University, ChinaManzar Abbas, Yibin University, China
Copyright © 2022 Zhou, Wang, Wang, Wang, Wang, Wang and Cheng. This is an open-access article distributed under the terms of the Creative Commons Attribution License (CC BY). The use, distribution or reproduction in other forums is permitted, provided the original author(s) and the copyright owner(s) are credited and that the original publication in this journal is cited, in accordance with accepted academic practice. No use, distribution or reproduction is permitted which does not comply with these terms.
*Correspondence: Xingfen Wang, Y290dG9uQGhlYmF1LmVkdS5jbg==; Hongmei Cheng, Y2hlbmdob25nbWVpQGNhYXMuY24=
†These authors share first authorship